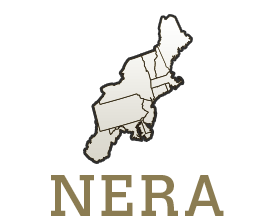
NE2338: Weed Emergence in a Changing Climate
(Multistate Research Project)
Status: Active
NE2338: Weed Emergence in a Changing Climate
Duration: 10/01/2023 to 09/30/2028
Administrative Advisor(s):
NIFA Reps:
Non-Technical Summary
Statement of Issues and Justification
Background
Unlike crops, which have been selected for uniform emergence, weed species have evolved variability in their emergence timing. Even seeds maturing on the same plant may germinate at different times. This “bet-hedging” strategy, with which a weed avoids putting all its “seed in one basket” of emergence timing, enables weeds to escape control measures. Post-emergence management carried out too early will yield low returns for the effort, investment, and ecological cost of the management (herbicide off-target effects, soil compaction, etc.), as weed seeds that have yet to germinate are often unaffected. On the other hand, most weed management tactics such as post-emergent herbicides and cultivation are most effective when weeds are small, at or near the seedling stage (Norsworthy et al. 2012). Therefore, delayed management operations may result in reduced weed control efficacy and greater yield loss (Davis 2006). Thus, weed management should be timed to occur soon after the emergence of most problem weeds.
The timing of weed emergence is not easy to predict because it reflects a multitude of speciesspecific parameters (e.g., base temperature, base water potential) and environmental factors (e.g., soil temperature and moisture). While many weedy species can germinate under a broad range of environmental conditions (Baker 1974), weeds in most agricultural systems have evolved to germinate when the appropriate microenvironmental cues are detected (Long et al 2016) to maximize fitness during favorable environmental conditions (Grime 1977). Weed growth, competitiveness, and fecundity are strongly influenced by emergence timing relative to the crop (Hartzler et al 2004, Wu et al 2014). Temperature is a particularly dominant influence on emergence timing in many annual weed species (Werle et al 214a; Werle et al 2014b). Understanding how changes in winter temperatures affect weed germination and emergence is crucial to designing weed management systems that are resilient to climate change.
Changing winters in northern climates.
In northern latitudes, winters are warming faster than any other season (Hayhoe et al 2007, Karmalkar et al 2017, Brown et al 2010, USGCRP 2017). Winters are also shortening as spring advances earlier in the year and autumn senescence is delayed, resulting in a longer growing season (Piao et al 2015, Monahan et al 2016, Contosta et al 2020). Against this backdrop of milder winters and longer, warmer growing seasons, climate change is driving greater winter weather variability (Chen et al 2018) related to changes in the Arctic jet stream (Francis et al 2015, Cohen 2016, Overland et al 2016). This variability may result in extreme cold temperatures (Cohen et al 2013, Kug et al 2015, Overland 25 al 2011, Cohen et al 2018). One extreme occurred in 2018–2019, when the incursion of the polar vortex plunged temperatures below –20°C across the Northeast. While swings between extremely cold and warm temperatures are somewhat rare, freeze-thaw cycles in which temperatures fluctuate around 0°C may become increasingly common as the climate warms (Henry 2008) with significant implications for agricultural productivity (Rotz et al 2016) and concomitant weed management.
Weed seed bank dynamics and their responses to temperature.
Weeds are a persistent challenge for crop production. They reduce crop yield and quality, sometimes even causing stand failure (Grekul and Bork 2004, Baker and Mohler 2014, Hatzler 2004, Rosenbaum et al 2011). Weed seed banks are the primary source of weed recruitment in most agroecosystems. Weed issues are likely to be exacerbated by a warming climate (Hatfield et al 2011, 2014), which will impact weed seedbanks as well as emerged weeds. Weed seed persistence within the soil seedbank is strongly regulated by soil temperature (Smith et al 2018, Kreyling et al 2010, Walck et al 2011). For this reason and others, changes in temperature are likely to drive shifts in weed community composition and abundance that could pose new challenges for cropping systems in the region.
Warmer temperatures affect weed seed dormancy.
Most weed species exhibit seed dormancy (Cavers et al 1989). Seed dormancy, which prevents germination at times that would result in low survival, is controlled by species-specific physiological, physical, and/or chemical mechanisms that may confer both dormancy and defense (Baskin and Baskin 2014, Davis et al 2016). Dormancy mechanisms are strongly influenced by temperature (Benech-Arnold 2000). Maternal plants exposed to warmer air temperatures during seed set can produce seeds with lower dormancy levels (Gutterman 2000). Warmer temperatures following seed dispersal can increase the rate of afterripening and thus the fraction of germinable weed seeds within the soil (Dwyer 2016).
Increased soil freeze-thaw cycles and warmer temperatures affect seed longevity.
Soil freeze-thaw cycles directly affect weed seed persistence by breaking down hard seed coats (Baskin and Baskin 2014). In species with physical dormancy, fractures to the weed seed coat release dormancy and thereby increase germination, emergence, and recruitment (e.g., velvetleaf (Abutilon theophrasti)). Fracturing of the seed coat also increases vulnerability to soil pathogens and decay (Connolly and Orrock 2015). Indirect effects of increased soil temperatures and freeze-thaw cycles on seed longevity may be mediated by increased activity of pathogenic fungi and other microorganisms (Classen et al 2015). Another indirect effect is that soil heaving associated with freeze-thaw cycles moves weed seeds in the soil profile (Chambers and Macmahon 1994). Some seeds are moved into deeper layers where they are more protected from seed predators (Omani et al 1999, Korres et al 2018).
Importance of the Work
Weed management is a priority issue for Northeastern farmers, particularly given the increasing prevalence of organic production, the rise of herbicide-resistant weeds, and the recent increase in small farms and urban farming. Interest in local food is also increasing, so specific, regionally focused data and tools for the Northeast could provide great benefits to growers and consumers while reducing negative impacts on the environment. Weeds are the major cause of yield losses in organic production (Baker and Mohler 2014; Jerkins and Ory 2016). Yield losses to weed competition are an increasing problem for conventional farmers as well, as the incidence of herbicide-resistant weeds continues to increase (Heap 2023). Preventing yield losses requires weed management operations such as cultivation or herbicide applications conducted at the proper time. The failure to account for the temporal variability of emergence can result in mistimed application of these control measures, leading to poor efficacy. Poor efficacy may necessitate repeated operations that are not only costly to the farmers, but also detrimental to our environment. Thus, better timed, and more effective use of herbicides and/or cultivation will protect yield and minimize unintended consequences like the spread of herbicide resistance in weed populations.
As the climate warms, changes to weed emergence patterns or weed community composition are likely to impact crop yield and farm profitability. Accurate predictions about near-term effects of increased temperatures on weed communities will allow farmers throughout the Northeast region to proactively respond to these changes.
Technical Feasibility
We need effective and affordable methods to simulate increasing temperatures and increasing weather variability in the field to better understand the impacts climate change may have on weed emergence. Our current research has shown that hexagonal open top chambers (“OTCs”, shown in Figure 1 (Marion et al 1997)) meet this need and passively increase air and soil temperature, while having a minimal effect on soil moisture. OTCs have been used to simulate warming throughout a wide range of climates and environments (Bjorkman et al 2017; Seipel et al 2019). We found that our OTC design was relatively easy to implement and effective at modifying temperatures. The OTCs had substantial effects on air and soil temperature (Figures 2 and 3) and are built of plastic that allows 95% light transmission (Carolyn Lowry, pers. comm.). On average we obtained an increase in air temperature within the OTC of approximately 0.5°C, and soil temperatures by approximately 0.4°C. If funded, we will test light transmission and the frequency of transmitted light, to test whether collected data are impacted by a shift in light ratio.
For example, fall maximum air temperature within the OTC was up to 5°C warmer compared with the control plots, while the OTC increased maximum air temperature by as much as 10°C in spring. The change in autumn air temperature within the OTC decreased cold hardiness accumulation by 20 chilling degree day units (base 5°C). Interestingly, the magnitude by which our OTCs decreased chilling degree days is consistent with the predicted decline in chilling degree days for future climate change scenarios in northeastern North America (Bélanger 2002). In the spring, the OTC increased the number of days that maximum air temperature was above 15°C (the temperature at which alfalfa breaks dormancy) by 40% compared with the control. While the OTC did not impact winter maximum air temperature as much as in autumn and spring, we did observe up to an 8°C increase in the minimum daily temperature in winter. Throughout the spring, the OTC doubled the number of days that air temperature was greater than 30°C compared with the control plots without an OTC.
This OTC design is already being implemented by two multistate collaborators in an AFRI-funded grant focused on alfalfa management in Pennsylvania and New Hampshire. Importantly, the proposed work will provide complementary information to the existing project focused on weed responses in a wider range of Northeastern US states and climate conditions.
Figure 1, found in Attachments section. Picture of OTCs at Rock Springs, PA (photo credit A. Isaacson).
Figures 2 and 3, found in Attachments section. Figure 2. Average monthly difference in temperature (ºC) for year one (A. 2020-2021) and year two (B. 2021-2022) in State College, PA. Data are monthly averages of the daily average temperatures of temperature sensors at a 10 cm height in an alfalfa-orchardgrass mixture. Figure 3. Daily maximum (top) and minimum (bottom) soil temperature at 5 cm depth in Pennsylvania (PA, left) and New Hampshire NH, right) buried in a plot with an OTC (“constant warming ’’) and without (“control”).
Multistate Advantages
Weather patterns, soil types, and weed communities are highly variable across the Northeast, making the collection of data from across the region critical for understanding the response of agricultural weed emergence to climate change. Additionally, Cordeau et al. (2017) found that populations of weed species had different emergence patterns in different Northeastern states. It is not yet clear whether that difference is due to genetic variability within the species or plasticity in emergence patterns depending on climatic conditions. A multistate project will allow us to replicate the same weed emergence experiment at multiple sites across the region. Participating researchers will include Richard Smith (New Hampshire), Carolyn Lowry (Pennsylvania), Mark VanGessel (Delaware), Antonio DiTommaso (New York), and Thierry Besancon (New Jersey). Our thorough coverage of the region will ensure that results capture regional variability in weed emergence and climate.
Likely Impact
Current research on Northeastern weed emergence does not account for warming temperatures which are occurring at a much more rapid rate than predicted (Karmalkar and Horton 2021). The findings from this research will allow weed scientists to model changing patterns of weed emergence under warming conditions and will provide more accurate information for our growers and other stakeholders in the region.
The findings of this research will empower farmers to better predict the emergence patterns of common weeds, thereby improving weed management efficacy and efficiency. While weed emergence timing is one of many factors that determine treatment windows for farmers, application outside of the optimal window of emergence and early growth is drastically less effective. Better information on emergence timing will be especially helpful for newer farmers; with many of our experienced farmers aging out of farm management, new farmers are likely to become more common in the next decade. Improving farmers’ ability to manage weeds effectively in a rapidly changing climate will be an important aspect of cropping system adaptation and resiliency to deteriorating stressors of climate change. Optimized weed management programs will simultaneously enhance farm profitability and reduce negative environmental impacts.
Related, Current and Previous Work
This proposal builds on past work conducted through the multistate Federal Capacity Funds, extending the research we have conducted on ecological management of weeds and on weed emergence to application in a changing climate. Weed emergence is variable by species; while it is likely that warming temperatures will trigger earlier emergence, it is also possible that the responses of individual species will alter weed abundance and community composition in the Northeast (Westbrook et al 2022). Climate change may also increase variability in weed emergence, rendering existing models less accurate.
Cornell University weed ecologists are at the forefront of weed emergence research (Brown et al. 2022, Cordeau et al. 2017) and on the effects of climate change on weed performance and weedcrop interactions (e.g., Averill et all 2022 A & B, DiTommaso et al 2021, Westbrook et al. 2021, Young et al. 2017). Dr. DiTommaso and coauthors have included 200 additional species into the recently released second edition of Weeds of the Northeast relative to the first edition released in 1997, largely due to the expansion of more southern species into the Northeastern region (Neal et al 2022). He has also co-authored a chapter on modeling invasive species and climate change (Westbrook et al 2022). The DiTommaso lab has led multistate weed ecology studies since 2011 through Multistate projects NE-1047 “Ecological Bases for Weed Management in Sustainable Cropping Systems” and NE-1838 “Development of a Weed Emergence Model for the Northeastern US” which is in its final months. In NE-1838, we collected three years of weekly weed emergence data across five to eight Northeastern states, correlated emergence with temperature and soil moisture, and are using resulting emergence models to build a decision support tool for farmers to help predict emergence for several problematic summer annual weed species. These projects brought together networks of weed scientists from the region to identify effective non-chemical weed management strategies, and to develop a weed emergence decision support tool for farmers. While the decision support tool under development applies to the current climate conditions of the Northeast, it may not apply under the warmer conditions expected in the near future. The proposed research will assist in extending the value of previous work by incorporating future climate conditions.
Penn State collaborator Carolyn Lowry’s recent work has examined how extreme precipitation events affect weed management efficacy (Lowry, in progress), how abiotic factors influence the composition of weed communities (Lowry et al., in review), and how management factors influence crop-weed competition (Lowry et al. 2019). Dr. Lowry is a co-PI on an AFRI project (PENW-202109936) focused on the implications of warmer climate conditions on perennial forage and weed communities; this proposed work will directly support that work by adding more locations and more weed-focused research to the existing project.
University of New Hampshire collaborator Richard Smith’s recent work has investigated how soil weed seedbank communities are structured by climate and edaphic factors (Smith et al. 2018) and agricultural management practices (Smith et al. 2016). Dr. Smith Dr. is a co-PI on an AFRI project (PENW-2021-09936) focused on the implications of warmer climate conditions on perennial forage and weed communities; this proposed work will directly support that work by adding more locations and more weed-focused research to the existing project.
University of Delaware collaborator Mark van Gessel has participated in a wide range of weed emergence research projects, including the previous two multistate programs mentioned above. These projects have modelled weed emergence in a variety of Northeastern weed species (Myers et al. 2004; Myers et al. 2005) including winter annuals (VanGessel et al. 2015) and horseweed (Conyza canadensis; Dauer et al. 2007).
Rutgers University collaborator Thierry Besancon is researching environmental parameters that govern germination and emergence of volunteer cranberry (Vaccinium macrocarpon) and Carolina redroot (Lachnanthes caroliana). These weeds are challenging for New Jersey cranberry growers because of genetic pollution of the cranberry beds or direct competition with the cranberry vines.
Objectives
-
Evaluate how weed emergence timing and weed communities vary under ambient (control) and increased temperature conditions across multiple sites with different environmental conditions (NH, PA, NY, DE, NJ).
Comments: To achieve this objective, we will deploy open-top chambers (OTCs) for in situ manipulation of air and soil temperatures at each site to determine how increasing temperature affects emergence of winter and summer annual weeds.
Methods
Experimental Design:
The study will consist of two treatments: 1) “Warmed”: a warmer-climate treatment with an OTC, recording OTC-modified temperature, soil moisture, and weed emergence, 2) “Control”: a control treatment with no OTC, recording ambient temperature, soil moisture and weed emergence. These will be arranged in a randomized complete block design with four replications. Plots will have no crops planted and will be 1 m2, which is the maximum area that can be consistently warmed within the OTC structures. Data will be collected from the center 0.25m2, but the data collection area will be adjusted to 0.5 m2 if weed emergence is low or subsampled if emergence is very high. Plots will be at least 6 m apart to limit any effect of OTCs on snow fall in neighboring plots, which might affect the conditions overwintering seeds experience. The experiment will be moved each year so that plots are on novel locations, to avoid exhaustion of the seed bank, but researchers will attempt to keep them in the same general area to reduce variability introduced by changing soil types.
Warmed Plots: To simulate increasing temperatures associated with climate change we will use hexagonal open-top chambers (OTCs). OTCs will be constructed from 1 mm thick Sun-Lite HP (Solar Components Corporation) attached to a metal frame, with a 2.65 m basal diameter, a top opening diameter of 1.75 m, and a height of 0.8 m. OTCs will be anchored into randomly fixed points in the ground. The OTCs will remain on the plots across all seasons for the entire duration of the experiment, and only be removed temporarily for emergence counts and plot maintenance (seeding out new species). The OTCs described above have been deployed in central PA for over two years. We will test light transmission through the OTCs in year 1 of the experiment.
For this experiment, researchers will record weed emergence for the most common 10 summer annual weeds at their site, and will also include if present these species of interest:
- Smooth pigweed or redroot pigweed (Amaranthus hybridus or retroflexus)
- Velvetleaf (Abutilon theophrasti)
- Lambsquarters (Chenopodium album)
- Large crabgrass (Digitaria sanguinalis)
- Foxtail (Setaria )
- Palmer amaranth (Amaranthus palmeri)
- bur-cucumber (Sicyos angulatus)
- ivyleaf morningglory (Ipomoea hederacea)
Control Plots: No OTC present, ambient temperatures.
- Initial fall tillage will occur prior to establishing the experiment and after that soil will remain for the most part untilled, but in sites where soil cursting occurs we will use a scuffle hoe to break up soil crusting as needed.
- Researchers will locate plots in weedier fields.
- Plots will be monitored weekly. Emerging seedlings of the 10 most common species, and any of the priority species listed above, will be counted and removed; at the end of the sampling, all seeds will be either clipped and removed or sprayed with herbicide. Sampling will begin before weed emergence in the spring, and will continue until none of the target species have emerged for three sampling periods or until the end of October.
- We will use soil temperature and soil moisture sensors attached to data loggers, to quantify the relationship between weed seedling emergence and both soil GDD accumulation and soil moisture.
Data analysis: To examine the effect that warming has on relative timing of weed emergence, we will first convert emergence to cumulative emergence (%) based on the total seedling emergence per experimental unit per year (each species will be analyzed separately). Cumulative emergence of each weed species will be modeled using a Weibull function (Weibull 1951): Y=M ∗{1–exp[–exp(lrc) ∗ (GDD–z)c]} where Y is cumulative percent emergence, M is the upper horizontal asymptote, lrc is the natural log of the rate of increase, GDD is growing degree days and is the predictor variable, z is the time of first emergence, and c is the curve shape parameter [Werle et al 2014b, Goplen et al 2018). We will use the model to extract time to 25%, 50%, and 75% emergence, and then examine whether the fixed effects of climate manipulation treatments affect the relative timing of emergence with block nested in year and site as random factors.
Measurement of Progress and Results
Outputs
- field data Comments: Our field research will provide three years of emergence data from five Northeastern states on annual weed species under ambient and elevated temperature conditions.
- Peer-reviewed research articles Comments: The synthesized results of our research will be published in peer-reviewed literature, probably in Weed Science or Weed Research.
- Extension materials Comments: The synthesized results of our research will be extended to Northeastern farmers through extension articles, website information, field days, and presentations.
Outcomes or Projected Impacts
- Improve weed management efficacy in the face of climage change This project will help maintain weed control efficacy in the face of climate change. We anticipate that researchers will be able to build on our findings to determine how different crops influence emergence timing. If this method is successful, future research can apply this technique to other problem weed species, and as we accrue a larger data set, we may be able to predict responses in similar weed species. Extension educators will use information derived from our research to communicate scientifically sound weed management practices to farmers across the Northeast. Farmers will use the weed emergence information to optimize their weed management programs and proactively address changes in weed emergence patterns. We hope that the results of this work will be used to launch a common garden experiment to determine how phenotypic variations affect differences in emergence timing across the Northeast.
Milestones
(1):Finalize field research plans(1):Season 1 data collection
(2):Season 1 data management
(2):Season 2 data collection
(3):Season 2 data management
(2):initial extension talks
(3):Season 3 data collection
(3):Outreach to extend research outcomes
(3):Write and publish related papers
(3):Final report
Projected Participation
View Appendix E: ParticipationOutreach Plan
The project will produce useful weed management information for farmers, extension personnel, crop consultants, and the general public. The results will be shared in research papers, on the Cornell University Weed Science website, and in talks by extension professionals in New York, Delaware, and New Jersey where our collaborators have extension positions. Some likely venues include:
Weed Science Society of America annual meeting
Northeastern Plant, Pest and Soils annual conference
Tri-Society of America annual meeting
New York Cooperative Extension Agricultural In-Service
Delaware Cooperative Extension In-Service
Cornell University Musgrave Research Farm Field Day
Delaware University’s Weed Science Field Day
What’s Cropping Up
Extension Insider (a weekly internal New York extension publication)
Field day / twilight tours / field walks
County extension meetings
The results of our research will also be incorporated into our respective undergraduate and graduate weed science and integrated pest management teaching programs.
Organization/Governance
The multistate research group will have an elected Chair, Chair-elect, and Secretary; we will elect them for the duration of our funded cycle. Administrative guidance will be provided by an assigned Administrative Advisor and a NIFA Representative.
Literature Cited
Averill, K.M., A.S. Westbrook, S.H. Morris, E. Kubinksi, and A. DiTommaso. 2022. Silage corn yield is reduced by burcucumber competition and drought in New York State. Weed Technology 36(1):86-92. DOI: https://doi.org/10.1017/wet.2021.80
Averill, K.M., S.H. Morris, A.S. Westbrook, M.C. Hunter, and A. DiTommaso. 2022. Ivyleaf morningglory competition is not intensified by drought in silage corn in central New York State. Canadian Journal of Plant Science. In Press. https://cdnsciencepub.com/doi/pdf/10.1139/CJPS-2022-0002
Baker, B. P., & Mohler, C. L. (2015). Weed management by upstate New York organic farmers: Strategies, techniques and research priorities. Renewable Agriculture and Food Systems, 30(5), 418-427. https://doi.org/10.1017/S1742170514000192
Baker, H. G. (1974). The evolution of weeds. Annual Review of Ecology and Systematics, 5(1), 1-24. https://doi.org/10.1146/annurev.es.05.110174.000245
Baskin, C. C., & Baskin, J. M. (2014). Seeds: Ecology, Biogeography, and Evolution of Dormancy and Germination (2nd ed.). Academic Press.
Bélanger, G., Rochette, P., Castonguay, Y., Bootsma, A., Mongrain, D., & Ryan, D. A. J. (2002). Climate change and winter survival of perennial forage crops in eastern Canada. Agronomy Journal, 94(5), 1120-1130. https://doi.org/10.2134/agronj2002.1120
Benech-Arnold, R. L., Sánchez, R. A., Forcella, F., Kruk, B. C., & Ghersa, C. M. (2000). Environmental control of dormancy in weed seed banks in soil. Field Crops Research, 67(2), 105-122. https://doi.org/10.1016/S0378-4290(00)00087-3
Bjorkman, A. D., Vellend, M., Frei, E. R., & Henry, G. H. R. (2017). Climate adaptation is not enough: warming does not facilitate success of southern tundra plant populations in the high Arctic. Global Change Biology, 23(4), 1540-1551. https://doi.org/10.1111/gcb.13417
Brown, B., E.R. Gallandt, A. DiTommaso, P. Salon, R.G. Smith, M.R. Ryan, and S. Cordeau. 2022. Improving weed management based on the timing of emergence peaks: A case study of problematic weeds in the Northeast USA. Frontiers in Agronomy. https://www.frontiersin.org/articles/10.3389/fagro.2022.888664/full
Brown, P. J., Bradley, R. S., & Keimig, F. T. (2010). Changes in extreme climate indices for the northeastern United States, 1870–2005. Journal of Climate, 23(24), 6555-6572. https://doi.org/10.1175/2010JCLI3363.1
Cavers, P. B., & Benoit, D. L. (1989). Seed banks in arable land. In M. A. Leck, V. T. Parker, & R. L. Simpson (Eds.), Ecology of Soil Seed Banks (pp. 309–328). Academic Press.
Chambers, J. C., & MacMahon, J. A. (1994). A day in the life of a seed: Movements and fates of seeds and their implications for natural and managed systems. Annual Review of Ecology and Systematics, 25(1), 263-292. https://doi.org/10.1146/annurev.es.25.110194.001403
Chen, S., Wu, R., Chen, W., & Yao, S. (2018). Enhanced linkage between Eurasian winter and spring dominant modes of atmospheric interannual variability since the early 1990s. Journal of Climate, 31(9), 3575-3595. https://doi.org/10.1175/JCLI-D-17-0525.1
Classen, A. T., Sundqvist, M. K., Henning, J. A., Newman, G. S., Moore, J. A. M., Cregger, M. A., Moorhead, L. C., & Patterson, C. M. (2015). Direct and indirect effects of climate change on soil microbial and soil microbial-plant interactions: What lies ahead? Ecosphere, 6(8), 130. https://doi.org/10.1890/ES15-00217.1
Cohen, J. (2016). An observational analysis: Tropical relative to Arctic influence on midlatitude weather in the era of Arctic amplification. Geophysical Research Letters, 43(10), 5287-5294. https://doi.org/10.1002/2016GL069102
Cohen, J., Jones, J., Furtado, J. C., & Tziperman, E. (2013). Warm Arctic, cold continents: A common pattern related to Arctic sea ice melt, snow advance, and extreme winter weather. Oceanography, 26(4), 150-160.
Cohen, J., Pfeiffer, K., & Francis, J. A. (2018). Warm Arctic episodes linked with increased frequency of extreme winter weather in the United States. Nature Communications, 9(1), 869. https://doi.org/10.1038/s41467-018-02992-9
Connolly, B. M., & Orrock, J. L. (2015). Climatic variation and seed persistence: Freeze–thaw cycles lower survival via the joint action of abiotic stress and fungal pathogens. Oecologia, 179(2), 609-616. https://doi.org/10.1007/s00442-015-3369-4
Contosta, A. R., Casson, N. J., Nelson, S. J., & Garlick, S. (2020). Defining frigid winter illuminates its loss across seasonally snow-covered areas of eastern North America. Environmental Research Letters, 15(3), 034020. https://doi.org/10.1088/1748-9326/ab54f3
Cordeau, S., Smith, R. G., Gallandt, E. R., Brown, B., Salon, P., DiTommaso, A., & Ryan, M. R. (2017). How do weeds differ in their response to the timing of tillage? A study of 61 species across the northeastern United States. Annals of Applied Biology, 171(3), 340-352. https://doi.org/10.1111/aab.12377
Dauer, J. T., Scott, B. A., VanGessel, M. J., & Mortensen, D. A. (2007). Effects of emergence periodicity on growth and fecundity of horseweed [Poster]. Proceedings of the Sixty-first Annual Meeting of the Northeastern Weed Science Society, Baltimore, MD. https://www.newss.org/proceedings/proceedings_2007.pdf
Davis, A. (2006). When does it make sense to target the weed seed bank? Weed Science, 54(3), 558-565. https://doi.org/10.1614/WS-05-058R.1
Davis, A. S., Fu, X., Schutte, B. J., Berhow, M. A., & Dalling, J. W. (2016). Interspecific variation in persistence of buried weed seeds follows trade-offs among physiological, chemical, and physical seed defenses. Ecology and Evolution, 6(19), 6836-6845. https://doi.org/10.1002/ece3.2415
DiTommaso, A., K.M. Averill, Z. Qin, M. Ho, A.S. Westbrook, and C.L. Mohler. 2021. Biomass allocation of Vincetoxicum rossicum and V. nigrum (Apocynaceae) in contrasting competitive environments. American Journal of Botany 108(9):1646-1661. https://doi.org/10.1002/ajb2.1734
Dwyer, J. M., & Erickson, T. E. (2016). Warmer seed environments increase germination fractions in Australian winter annual plant species. Ecosphere, 7(10), e01497. https://doi.org/10.1002/ecs2.1497
Francis, J., & Skific, N. (2015). Evidence linking rapid Arctic warming to mid-latitude weather patterns. Philosophical Transactions of the Royal Society A: Mathematical, Physical and Engineering Sciences, 373(2045), 20140170. https://doi.org/10.1098/rsta.2014.0170
Goplen, J. J., Sheaffer, C. C., Becker, R. L., Moon, R. D., Coulter, J. A., Breitenbach, F. R., Behnken, L. M., & Gunsolus, J. L. (2018). Giant ragweed (Ambrosia trifida) emergence model performance evaluated in diverse cropping systems. Weed Science, 66(1), 36-46. https://doi.org/10.1017/wsc.2017.38
Green, J., Witt, W., & Martin, J. (2006). Weed management in grass pastures, hayfields, and other farmstead sites. University of Kentucky Extension Service AGR-172.
Grekul, C. W., & Bork, E. W. (2004). Herbage yield losses in perennial pasture due to Canada thistle (Cirsium arvense). Weed Technology, 18(3), 784-794. https://doi.org/10.1614/WT-03-196R
Grime, J. P. (1977). Evidence for the existence of three primary strategies in plants and its relevance to ecological and evolutionary theory. The American Naturalist, 111(982), 1169-1194. https://doi.org/10.1086/283244
Gutterman, Y. (2000). Maternal effects on seeds during development. In M. Fenner (Ed.), Seeds: The Ecology of Regeneration in Plant Communities (2nd ed., pp. 59-84). CAB International. https://doi.org/10.1079/9780851994321.0059
Hartzler, R. G., Battles, B. A., & Nordby, D. (2004). Effect of common waterhemp (Amaranthus rudis) emergence date on growth and fecundity in soybean. Weed Science, 52(2), 242-245. https://doi.org/10.1614/WS-03-004R
Hatfield, J., Takle, G., Grotjahn, R., Holden, P., Izaurralde, R. C., Mader, T., Marshall, E., & Liverman, D. (2014). Chapter 6. Agriculture. In J. M. Melillo, T. C. Richmond, & G. W. Yohe (Eds.), Climate Change Impacts in the United States: The Third National Climate Assessment (pp. 150-174). U.S. Global Change Research Program. https://doi.org/10.7930/J02Z13FR
Hatfield, J. L., Boote, K. J., Kimball, B. A., Ziska, L. H., Izaurralde, R. C., Ort, D., Thomson, A. M., & Wolfe, D. (2011). Climate impacts on agriculture: Implications for crop production. Agronomy Journal, 103(2), 351-370. https://doi.org/10.2134/agronj2010.0303
Hayhoe, K., Wake, C. P., Huntington, T. G., Luo, L., Schwartz, M. D., Sheffield, J., Wood, E., Anderson, B., Bradbury, J., DeGaetano, A., Troy, T. J., & Wolfe, D. (2007). Past and future changes in climate and hydrological indicators in the US Northeast. Climate Dynamics, 28(4), 381-407. https://doi.org/10.1007/s00382-006-0187-8
Heap, I. (2023). The International Herbicide-Resistant Weed Database. http://weedscience.org
Henry, H. A. L. (2008). Climate change and soil freezing dynamics: Historical trends and projected changes. Climatic Change, 87(3), 421-434. https://doi.org/10.1007/s10584-007-9322-8
Jerkins, D., & Ory, J. (2016). National Organic Research Agenda. Organic Farming Research Foundation.
Karmalkar, A. V., & Bradley, R. S. (2017). Consequences of global warming of 1.5 °C and 2 °C for regional temperature and precipitation changes in the contiguous United States. PLoS ONE, 12(1), e0168697. https://doi.org/10.1371/journal.pone.0168697
Karmalkar , A.V., & Horton, R.S. (2021). Drivers of exceptional coastal warming in the Northeastern United States. Nature Climate Change 11, 854-860 . https://doi.org/10.1038/s41558-021-01159-7
Korres, N. E., Norsworthy, J. K., Young, B. G., Reynolds, D. B., Johnson, W. G., Conley, S. P., Smeda, R. J., Mueller, T. C., Spaunhorst, D. J., Gage, K. L., Loux, M., Kruger, G. R., & Bagavathiannan, M. V. (2018). Seedbank persistence of Palmer amaranth (Amaranthus palmeri) and waterhemp (Amaranthus tuberculatus) across diverse geographical regions in the United States. Weed Science, 66(4), 446-456. https://doi.org/10.1017/wsc.2018.27
Kreyling, J., Beierkuhnlein, C., & Jentsch, A. (2010). Effects of soil freeze-thaw cycles differ between experimental plant communities. Basic and Applied Ecology, 11(1), 65-75. https://doi.org/10.1016/j.baae.2009.07.008
Kug, J.-S., Jeong, J.-H., Jang, Y.-S., Kim, B.-M., Folland, C. K., Min, S.-K., & Son, S.-W. (2015). Two distinct influences of Arctic warming on cold winters over North America and East Asia. Nature Geoscience, 8(10), 759-762. https://doi.org/10.1038/ngeo2517
Long, R. L., Gorecki, M. J., Renton, M., Scott, J. K., Colville, L., Goggin, D. E., Commander, L. E., Westcott, D. A., Cherry, H., & Finch-Savage, W. E. (2015). The ecophysiology of seed persistence: A mechanistic view of the journey to germination or demise. Biological Reviews, 90(1), 31-59. https://doi.org/10.1111/brv.12095
Lowry, C. J., & Brainard, D. C. (2019). Strip intercropping of rye–vetch mixtures: Effects on weed growth and competition in strip-tilled sweet corn. Weed Science, 67(1), 114-125. https://doi.org/10.1017/wsc.2018.83
Marion, G. M., Henry, G. H. R., Freckman, D. W., Johnstone, J., Jones, G., Jones, M. H., LÉVesque, E., Molau, U., MØLgaard, P., Parsons, A. N., J, S., & Virginia, R. A. (1997). Open-top designs for manipulating field temperature in high-latitude ecosystems. Global Change Biology, 3(S1), 20-32. https://doi.org/10.1111/j.1365-2486.1997.gcb136.x
Monahan, W. B., Rosemartin, A., Gerst, K. L., Fisichelli, N. A., Ault, T., Schwartz, M. D., Gross, J. E., & Weltzin, J. F. (2016). Climate change is advancing spring onset across the U.S. national park system. Ecosphere, 7(10), e01465. https://doi.org/10.1002/ecs2.1465
Myers, M. W., Curran, W. S., VanGessel, M. J., Calvin, D. D., Mortensen, D. A., Majek, B. A., Karsten, H. D., & Roth, G. W. (2004). Predicting weed emergence for eight annual species in the northeastern United States. Weed Science, 52(6), 913-919, 917. https://doi.org/10.1614/WS-04-025R
Myers, M. W., Curran, W. S., Vangessel, M. J., Majek, B. A., Mortensen, D. A., Calvin, D. D., Karsten, H. D., & Roth, G. W. (2005). Effect of soil disturbance on annual weed emergence in the northeastern United States. Weed Technology, 19(2), 274-282, 279. https://doi.org/10.1614/WT-03-242R1
Neal, J. C., Uva, R. M., DiTomaso, J. M., and DiTommaso, A. (2023). Weeds of the Northeast. Comstock Publishing Associates. ISBN: 9781501755729
Norsworthy, J. K., Ward, S. M., Shaw, D. R., Llewellyn, R. S., Nichols, R. L., Webster, T. M., Bradley, K. W., Frisvold, G., Powles, S. B., Burgos, N. R., Witt, W. W., & Barrett, M. (2012). Reducing the risks of herbicide resistance: Best management practices and recommendations. Weed Science, 60(sp1), 31-62. https://doi.org/10.1614/WS-D-11-00155.1
Omami, E. N., Haigh, A. M., Medd, R. W., & Nicol, H. I. (1999). Changes in germinability, dormancy and viability of Amaranthus retroflexus as affected by depth and duration of burial. Weed Research 39(5), 345-354. https://doi.org/10.1046/j.1365-3180.1999.00149.x
Overland, J. E., Dethloff, K., Francis, J. A., Hall, R. J., Hanna, E., Kim, S.-J., Screen, J. A., Shepherd, T. G., & Vihma, T. (2016). Nonlinear response of mid-latitude weather to the changing Arctic. Nature Climate Change, 6(11), 992-999. https://doi.org/10.1038/nclimate3121
Overland, J. E., Wood, K. R., & Wang, M. (2011). Warm Arctic—cold continents: climate impacts of the newly open Arctic Sea. Polar Research, 30(1), 15787. https://doi.org/10.3402/polar.v30i0.15787
Piao, S., Tan, J., Chen, A., Fu, Y. H., Ciais, P., Liu, Q., Janssens, I. A., Vicca, S., Zeng, Z., Jeong, S.-J., Li, Y., Myneni, R. B., Peng, S., Shen, M., & Peñuelas, J. (2015). Leaf onset in the northern hemisphere triggered by daytime temperature. Nature Communications, 6(1), 6911. https://doi.org/10.1038/ncomms7911
Rosenbaum, K. K., Bradley, K. W., & Roberts, C. A. (2011). Influence of increasing common ragweed (Ambrosia artemisiifolia) or common cocklebur (Xanthium strumarium) densities on forage nutritive value and yield in tall fescue pastures and hay fields. Weed Technology, 25(2), 222-229. https://doi.org/10.1614/WT-D-10-00114.1
Rotz, C. A., Skinner, R. H., Stoner, A. M., & Hayhoe, K. (2016). Evaluating greenhouse gas mitigation and climate change adaptation in dairy production using farm simulation. Transactions of the American Society of Agricultural and Biological Engineers, 59(6), 1771-1781. https://doi.org/10.13031/trans.59.11594
Seipel, T., Ishaq, S. L., & Menalled, F. D. (2019). Agroecosystem resilience is modified by management system via plant-soil feedbacks. Basic and Applied Ecology, 39, 1-9. https://doi.org/10.1016/j.baae.2019.06.006
Smith, R. G., Atwood, L. W., Morris, M. B., Mortensen, D. A., & Koide, R. T. (2016). Evidence for indirect effects of pesticide seed treatments on weed seed banks in maize and soybean. Agriculture, Ecosystems & Environment, 216, 269-273. https://doi.org/10.1016/j.agee.2015.10.008
Smith, R. G., Birthisel, S. K., Bosworth, S. C., Brown, B., Davis, T. M., Gallandt, E. R., Hazelrigg, A., Venturini, E., & Warren, N. D. (2018). Environmental correlates with germinable weed seedbanks on organic farms across northern New England. Weed Science, 66(1), 78-93. https://doi.org/10.1017/wsc.2017.40
Sousa-Ortega, C., A. Royo-Esnal, A. DiTommaso, J. Izquierdo, I. Loureiro, A.I. Marí, F. Cordero, M. Vargas, M. Saavedra, J.A. Paramio, J.L. Fernández, J. Torra, J.M. Urbano. 2020. Modeling the emergence of Centaurea diluta, an increasingly troublesome weed in Spain. Weed Science 68(3):268-277. doi.org/10.1017/wsc.2020.22
USGCRP. (2017). Climate Science Special Report: Fourth National Climate Assessment (D. J. Wuebbles, D. W. Fahey, K. A. Hibbard, D. J. Dokken, B. C. Stewart, & T. K. Maycock, Eds.). U.S. Global Change Research Program. https://doi.org/10.7930/J0J964J6.
VanGessel, M. J., Zhang, Z., Ilvento, T. W., Scott, B., & Johnson, Q. R. (2015). Winter annual weed communities as a result of fall or spring weed control. Abstracts Annual Meeting of the Weed Science Society of America, Lexington, KY. http://wssaabstracts.com/public/30/proceedings.html
Walck, J. L., Hidayati, S. N., Dixon, K. W., Thompson, K. E. N., & Poschlod, P. (2011). Climate change and plant regeneration from seed. Global Change Biology, 17(6), 2145-2161. https://doi.org/10.1111/j.1365-2486.2010.02368.x
Weibull, W. (1951). A statistical distribution function of wide applicability. Journal of Applied Mechanics, 18(3), 293-297. https://doi.org/10.1115/1.4010337
Werle, R., Bernards, M. L., Arkebauer, T. J., & Lindquist, J. L. (2014). Environmental triggers of winter annual weed emergence in the midwestern United States. Weed Science, 62(1), 83-96. https://doi.org/10.1614/WS-D-13-00091.1
Werle, R., Sandell, L. D., Buhler, D. D., Hartzler, R. G., & Lindquist, J. L. (2014). Predicting emergence of 23 summer annual weed species. Weed Science, 62(2), 267-279. https://doi.org/10.1614/WS-D-13-00116.1
Westbrook, A.S., R. Han, J. Zhu, S. Cordeau, and A. DiTommaso. 2021. Drought and competition with ivyleaf morningglory (Ipomoea hederacea) inhibits corn and soybean growth. Frontiers in Agronomy 3:720287. https://doi.org/10.3389/fagro.2021.720287
Westbrook, A.S., Nikkel, E., Clements, D.R., and A. DiTommaso. 2022. Modeling and Managing Invasive
Weeds in a Changing Climate. In Invasive Species and Global Climate Change, 2nd Edition, ed. Lewis H. Ziska 2023. CAB International. ISBN 978-1-80062-143-5
Wu, C., & Owen, M. D. K. (2014). When is the best time to emerge: Reproductive phenology and success of natural common waterhemp (Amaranthus rudis) cohorts in the midwest United States? Weed Science, 62(1), 107-117. https://doi.org/10.1614/WS-D-13-00079.1
Young, S. L., Clements, D. R., & DiTommaso, A. (2017). Climate Dynamics, Invader Fitness, and Ecosystem Resistance in an Invasion-Factor Framework. Invasive Plant Science and Management. 10:215-231.