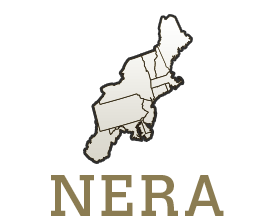
NE2336: Improving Quality and Reducing Losses in Specialty Fruit and Vegetable Crops through Storage Technologies
(Multistate Research Project)
Status: Active
NE2336: Improving Quality and Reducing Losses in Specialty Fruit and Vegetable Crops through Storage Technologies
Duration: 10/01/2023 to 09/30/2028
Administrative Advisor(s):
NIFA Reps:
Non-Technical Summary
Statement of Issues and Justification
The need as indicated by stakeholders
US growers produce an abundance of fresh fruits and vegetables, but deterioration of quality, storage disorders, decay and contamination with mycotoxins continue to cause considerable losses. As a result, the fresh fruit and vegetable industries rely on numerous pre- and postharvest practices to ensure minimization of losses. Several key developments in production and storage technologies make this project highly relevant for the fruit and vegetable industries in North America.
Postharvest losses for fresh produce are severe and vary widely, depending upon the crop and handling conditions employed. Gustavsson et al. (2011) noted that global food losses are about 1.3 billion tons annually. The FAO Sustainable Development Goal 12.3 aims, “by 2030, to halve per capita global food waste at the retail and consumer levels and reduce food losses along production and supply chains, including postharvest losses” (Fabi and English, 2019). Considering factors of climate change, a growing population reaching Malthusian limits, and less agricultural land being used to produce more food, new technologies, crops and decay management strategies are needed to increase food security and help slow food loss and waste.
Apples are a prime example of a commodity that is subject to significant losses during storage and transport. However, pre and postharvest rots caused by Colletotrichum (bitter rot) are significant problems for apple, citrus, blueberry and vegetables like tomato (Barad et al., 2017; Jurick II and Cox 2016; Liu et al., 2020). Postharvest rots of fresh fruits and vegetables not only reduce quality, but also limit their availability for consumption, and sometimes processed products can contain mycotoxins that harm human health. While postharvest fungicides are approved for use to control these pathogens on a limited number of these crops, concerns over maximum residue limits, antimicrobial resistance, and environmental impacts necessitate development of new tools and strategies to abate decay. New disorders in apple (skin wrinkling and leather blotch) appear to be more prevalent, as well as continued occurrence of others such as bitter pit and soft scald. The ability to identify conditions that lead to disorder development is dependent on a collaborative effort.
More frequent and deleterious environmental stresses, particularly high heat, have impacted produce quality and caused losses in the storage and supply chain. It is anticipated that this will increase losses due to poor quality and higher incidence of storage disorders. Preharvest growing conditions such as high temperature have increasingly become more stressful for plants, and this can have a deleterious effect on the postharvest outcome. High temperatures during maturation can delay apple coloration and force growers to delay harvest, but with limitations for storage life. Grower use of reflective ground covers to address poor color can impact fruit maturity and postharvest conditions. Unknown growing conditions are involved in the development of storage disorders, such as apple bitter pit. Therefore, a coordinated effort is needed to identify the conditions that lead to greater incidence and our predictive capacity.
Fresh produce items are sold in a wide range of locations from local farmers’ markets and roadside stands to local and regional markets to school lunch programs and to export destinations. These locations place varying demands on the techniques and technologies necessary to extend postharvest quality while reducing losses during this value chain. The fresh produce industry also provides reliable and constant employment to significant numbers of U.S. and foreign workers.
Increased consumer expectations that fruits and vegetables are available year-round and often out of season have created changes in which cultivars are grown and have expanded the regions where fruits are produced. This has been coupled with the development of new cultivars with specific adaptations to regional climates outside traditional production regions. Blueberries, strawberries, cherries and peaches are examples of this phenomenon. New types of these traditional fruits such as crisp-fleshed blueberries and firm-fleshed peaches have expanded the potential for marketing highly perishable fruits. Where once only round and cherry tomatoes were available in supermarkets, nowadays typical produce sections sell a variety of tomato types including on-the-vine (aka cluster), grape, varietal, heirloom, Roma, and hydroponic-grown, by both conventional and by organic methods. A similar phenomenon has occurred with other types of vegetables such as Ripe bell peppers and cucumbers. Consumer interest in new types of fruits such as muscadine grapes and elderberries has increased but with relatively unknown postharvest needs. New cultivars of virtually every type of produce are continually being adopted that may have unknown issues.
New cultivars typically have unknown postharvest needs that make their handling challenging. Small-scale operations oftentimes are at the forefront of testing and marketing new cultivars. However, the postharvest practices differ between large-scale operations with extended storage durations and small-scale operations with rapid marketing and brief periods of storage. Consequently, new cultivars initially tested for small-scale production may be unsuitable for large-scale production with concomitant stringent postharvest expectations of quality. Honeycrisp is a good example of an apple cultivar grown to satisfy demand, but with many storage issues.
Techniques for season extension, most notably high tunnels, have also contributed to expanded production of fruits and vegetables for small-scale local markets, but with unknown impact on quality. In addition, production in protected culture such as high tunnels and greenhouses is increasing in the U.S. with simultaneous changes in cultivars and postharvest needs. Therefore, it is essential that our growers, packers and shippers be provided with the latest postharvest information to thrive in this highly competitive arena. Limited research on the postharvest needs has necessitated the need for multistate research to gain an understanding of how these new cultivars and growing environments contribute to preservation of quality and prevention of decay at the physiological, molecular, genomic and genetic levels.
Severe limitations in the availability of labor have forced the fruit and vegetable industry to investigate greater use of mechanical harvesting and to adopt cultivars that are better adapted to mechanization. Many fruits and vegetables ripen at uneven rates. Thus, to maintain quality during marketing, fruit and vegetables are spot-picked to ensure harvest at the optimum stage of maturity. However, mechanical harvesting often necessitates a once-over harvest that requires the crop to be at a uniform stage of maturity to maximize yield and overall quality. Crops that can be mechanically harvested for the fresh market include blueberries, cranberries, leafy crops and root crops, and for processing markets include grapes, prunes, oranges, peaches, cherries, jalapeno peppers, tomatoes and olives. Research is needed to determine the impact of mechanical harvesting on consumer acceptability and shelf-life.
Continued demand for reduced reliance on chemicals and for organic fruit is accompanied by a requirement for compatible storage technologies. Improvements in the use of nonchemical approaches for preventing storage scald of apples called dynamic controlled atmosphere (DCA) storage comes with risks and additional need for how to apply this method to new cultivars. Highly sophisticated and sometimes risky postharvest storage technologies can be used in place of synthetic chemical controls, but uncertainties of their efficacies for the many different cultivars remain. Consumer demand for reduced Minimum Residue Levels is a significant driving factor to develop novel tools to maintain quality, reduce losses and mitigate toxins. Postharvest chemical applications remain an important method of preventing decay, but new nonchemical approaches have been developed to meet the needs of organic markets, and to minimize losses of fruit during storage and transport. New approaches to preventing losses come with potential risks whereas, generally recognized as safe (GRAS) chemical alternatives that prevent decay are largely untested. Testing of reduced-risk materials such as essential oils has shown effectiveness for controlling decay in some fruits, but large-scale application and impact on eating quality are untested. A better understanding of relationships between postharvest physiology of fruits and their susceptibility to physiological disorders and decay pathogens is essential for developing improved control measures and reducing chemical use.
New postharvest technologies that extend postharvest storage and shelf life need additional testing to confirm efficacy and identify risks for the different cultivars and types of produce. Elevated CO2 storage for raspberry can extend shelf-life, but research is needed to pinpoint optimum levels for the many cultivars and regions where raspberries are grown. Physiological disorders can be affected positively or negatively by these new storage technologies. For example, superficial scald of apples is inhibited by 1-MCP, DPA, and DCA storage, while other disorders, especially carbon dioxide-related ones tend to be increased by 1-MCP. The adoption of the plant growth regulator 1- MCP has altered the risks of long-term storage. These practices have increased greatly, largely in response to the need to maintain quality during storage and marketing. New application methods for in-carton application of 1-MCP such as sachets and incorporation into films have the potential to improve storage of other types of produce, but with many unanswered questions regarding efficacy.
Growers increasingly rely on predictive tools and rapid tests to determine storage potential and relative susceptibility to postharvest losses. Low-cost, rapid tests that measure postharvest characteristics and predict storage life or potential losses will allow growers to segregate high-risk produce and make informed decisions regarding storage duration. Peel analysis and the “passive” tests for predicting bitter pit in apples are examples of industry use of tests that were developed through collaborative research. Additional research is needed to understand how regional variation will impact application of these predictive tools. Recent advances in understanding how loss of xylem function leads to bitter pit can be combined with these predictive tests to improve grower ability to prevent severe cases of this disorder. Water loss, an important cause for quality loss in several fruits and vegetables can be measured using a low cost, nondestructive sealed chamber. Wide scale testing is needed for its commercial application. Other predictive or rapid tests currently in development include rancidity in walnuts, surface moisture measurement on leafy vegetables for preventing decay and measuring aroma volatiles to predict chilling injury in peaches. Knowing the status of produce going into storage is critical and can extend shelf life by a few days for highly perishable fruits and vegetables such as asparagus. Storage and marketing practices such as “first in first out” logistics lead to losses because they do not consider the physiological maturity or any adverse preharvest and harvest conditions (high temperatures, poor cooling to remove field heat) that compromise shelf-life in different batches of harvested fruit and vegetables.
Members of the NE1836 have collaborated to address the multiple disorders of apple cultivars that create complex storage requirements with the added need for predictive tools. In addition, recent advances in understanding the causes of disorders, mycotoxin abatement, and prevention will require multi-year testing and refinement in the many regions where they are now grown, and a coordinated effort to speed up real-world application of these new tools.
Expanding our knowledge on the harvest and storage of these specialty crops is critical to ongoing success in maintaining product quality of the fresh fruit and vegetable industries. Sharing of knowledge, especially about application of new technologies, increases the probability of successful outcomes. The project involves postharvest scientists in the different geographical regions of the US and Canada, nearly all with extension/outreach responsibilities, thereby providing a powerful platform for development and extension of this knowledge.
Research in this project will focus on these and other needs under the following objectives:
- Enhance and/or adapt current handling, storage and postharvest practices/technologies to ensure high-quality products to increase their acceptability by consumers.
- Expand and translate fundamental plant biology to develop new storage technologies and plant materials that will enhance human nutrition and energy-efficient postharvest systems.
- Advance our fundamental knowledge of host-pathogen-microbe interactions to maintain high-quality fruits and vegetables while reducing food loss and waste.
Importance of the Work
Previous and current versions of this project (NE103, NE1018, NE1036, NE1336, NE1836) have made major contributions to the fresh fruit industry. These include industry adoption of innovative applied methods developed by the group, and basic research on postharvest problems such as bitter pit in apple, and chilling injury (CI) in pome and stone fruits. The efforts of this project have led to more effective control measures and new knowledge of the genetic and biochemical causes of the disorders and diseases. Other environmental impacts during fruit growth, especially elevated temperature and solar irradiation, are major contributors to annual postharvest losses in major production regions. By necessity, our collective work continues to assess the fundamental basis of these losses and to identify novel solutions to mitigate them in a changing regulatory environment. Nonchemical and reduced risk chemical methods of preventing losses have been studied/developed to extend storage life of highly perishable fruits such as berries. Studies on apples have continued to be a major focus with an emphasis on newly emerging problems such as increased CO2 injury in storage and the ability to predict disorders that cause major losses for producers. 1-Methylcyclopropene (1-MCP), an ethylene action and ripening inhibitor has been used to maintain quality in storage of apple and is now being used for several other fruits and vegetables. New methods of application can potentially expand the use of the compound for the benefit of small-scale producers.
This multistate project was traditionally focused on fruits, but with research on vegetables being conducted concurrently. Research on vegetable storage practices is just as important and will benefit from the collective abilities of the group.
Technical Feasibility of the Research and Advantages for Doing the Work as a Multistate Effort
A goal of this group is to actively collaborate and exchange results from different systems to find solutions and to develop methods for rapid implementation to maintain industry profitability. We can accomplish this by conducting applied research in conjunction with a strong basic program while seeking to understand fruit/vegetable and pathogen physiology and biochemistry, particularly concerning responses to genetic differences among cultivars, and responses to technologies such as 1-MCP and modified/controlled atmosphere storage regimes. Many years have gone into selection of apple cultivars that maintain quality during long storage durations, but this type of coordinated effort is also needed to identify cultivars of other fruits and vegetables that may be better suited to the rigors of storage and marketing. The researchers in this project have diverse skill sets that span several scientific fields and have an established track record for collaboration on projects across North America.
Members of the group have the ability and expertise to study physiological, biochemical, and genetic/genomic basis of quality and development of storage disorders as well as fungal and bacterial pathology. Combined efforts have led to finding the causes of browning disorders in apple, quality loss and decay in blueberry and to predict or prevent such problems. Recent research has combined applied and basic approaches to identify underlying causes and potential solutions for such issues as apple bitter pit disorder, senescence associated genes (SAGS) in broccoli and lettuce, and changes in texture, peel elasticity and weight loss that predict shelf life in 60 blueberry cultivars. Furthermore, research that is based in many regions of the US and Canada contributes to the thorough study of many storage disorders that are impacted by local growing conditions so that cultural practices and storage conditions can be designed for regional needs. The broad geographical distribution of the team in this project provides a unique opportunity by which responses of fruits and vegetables to a wide range of growing conditions can be studied and the difficulties posed by the intrinsic variability of a fruit and vegetable crop can be overcome.
Members of the NE1836 have access to state-of-the-art facilities to conduct sensory and consumer testing. Integration of sensory testing into postharvest research is needed to determine how production and storage practices impact consumer preferences since technical measurements do not encompass the full human experience of quality. The combination of new cultivars and changes in pre- and postharvest practices can influence consumer acceptance.
As in the past, the current NE1836 members work as a research and extension team to solve industry problems and to provide rapid dissemination of research results that is greatly enhanced by the organization of a multistate project. The strong connections with producers that many members possess is a strength that ensures research is relevant and addresses the most pressing issues they face. The multistate project combines the respective strengths of each member for coordinated effort to investigate postharvest problems and to find much needed solutions to the specialty crop producers, both regionally and nationally.
Impact
The accomplishments of the NE1836 project include thorough postharvest evaluation of commercially important and emerging fruit cultivars, development of methodologies that enhance storage life, quality, and flavor and elucidation of mechanisms involved in flavor and storage disorder development in fruits. An example of impact resulting from our continued collaboration is the testing and development of a predictive test for bitter pit disorder for the Honeycrisp apple in various regions of the U.S. and Canada. This and other tests will allow producers to segregate at-risk produce to prevent their loss in storage. The collaborative efforts of the NE1836 program have also generated key information that has provided solutions to storage of apples including the development of storage regimes that address CO2 sensitivity in CA storage. Continued and wide-scale industry use of previous recommendations has been important for the economic success of Honeycrisp producers in the northeastern US. Successful transfer of information to researchers and industries is well integrated within the project and has been done via peer-reviewed publications, grower meetings, trade publications and websites.
Most members of this project have strong extension/outreach programs associated with their research, and they have been successful at guiding practices of fruit growers. The North American apple industry, for instance, has directly and demonstrably benefitted from our research and extension efforts and now employs a protocol for dynamic CA storage for apples with reduced reliance on postharvest chemicals. NE1836 members have worked with the National Mango Board (NMB) to develop the handling practices such as for harvest maturity, temperature management, ripening and transport that are recommended to that industry. The same is true for many other crops such as stone fruits, strawberries, and tomatoes. The primary goals of our new project are to increase competitiveness for domestic fruit and vegetable production and preserve 'fresh-picked' sensory and nutritional quality, which in turn will increase the availability of locally grown and highly perishable fruit. The large increase in new cultivars has the potential to increase availability of highly perishable produce in the market, but this will depend on research that leads to cultivar selection that is appropriate to commercial harvesting practices and storage. The overall impact of this project will be to improve the long-range health of the American populace via greater consumption of fresh fruits and vegetables, and to increase farm profitability at all levels of scale.
Annual reports are available on the NIMSS project website: NE1836: Improving Quality and Reducing Losses in Specialty Fruit Crops through Storage Technologies – NIMSS
Related, Current and Previous Work
A search of the NIMSS database revealed that, apart from efforts by the NE1836, there is an absence of multistate projects and coordinating committees that focus on the biology and technologies used to preserve the quality and condition of fresh specialty crops. This project is needed to provide coordination and collaboration among scientists to service diverse and valuable fruit and vegetable industries and avoid duplication of effort and inefficient use of resources.
Related Work.
The following projects were found to address some aspects of postharvest preservation of food crops.
S294 - Quality and Safety of Fresh-cut Vegetables and Fruits. This project emphasizes food safety and standardization of microbiological procedures, instrumental and subjective methods for sensory quality analysis, flavor-based shelf-life measurement and emerging treatments and techniques for assuring fresh-cut quality. The focus is on packaged products, not whole perishables.
NE2231 - Collaborative Potato Breeding and Variety Development Activities to Enhance Farm Sustainability in the Eastern US. This project aims to improve yields, fresh market appeal, processing or value-added traits, and pest resistance, but does not focus on postharvest problems per se.
WERA27: Potato Variety Development. This project focuses on potato germplasm development, seed potato evaluation, decay and disease resistance, and storability and processability of germplasm lines. The project acknowledges the need for “postharvest management research” but does not target specific postharvest problems.
NC1023: Engineering for Food Safety and Quality. This project tries to develop a systems-level understanding of food quality and safety development in complex processes, but does not deal with living produce.
NCCC212: Small Fruit and Viticulture Research. The top priority is “breeding and releasing superior, adapted cultivars”. One objective is to evaluate pre- and postharvest fruit quality components, but does not focus on the development or application of technologies to improve storability.
Current and Previous Work.
Over the last several decades, extensive research has been conducted nationally and internationally on maintaining fruit quality after harvest. Despite this long-term effort, the preservation of quality in the postharvest period continues to be a global issue of great importance at all levels of the value chain (Gustavsson et al., 2011). Historically, members of this multistate project have contributed significantly to this effort, albeit primarily on fruits. Previous work also includes fundamental research on processes related to fruit quality, the development of postharvest interventions to suppress quality decline, and evaluation of fruit responses to storage conditions (e.g., disorder incidence, and decay). While the focus of this multistate project has traditionally served the fruit industries, the lack of a counterpart program to focus on vegetable crops makes the inclusion of vegetables in the research portfolio a natural extension of this multistate project. The following sections detail current and previous work as it relates to proposed objectives and the inclusion of vegetables in future efforts.
Storage disorders. The control of storage disorders has been an important focus of postharvest research for fruits and vegetables. Important among these are those related to storage temperature (chilling injury) and atmosphere (high CO2 and low O2 injury). Many other storage-related disorders are physiological in nature, but still are influenced by the storage regimen.
Chilling injury (CI) is a significant storage disorder for numerous perishables. The multi-state project for fruit has led to methods of managing chilling injury in apple and stone fruit that have been adopted industry wide. The apple cultivar ‘Honeycrisp’ is particularly sensitive to low temperature storage, and work in this project has led to the development of a preventative treatment (conditioning at 10 to 20 °C for up to seven days followed by storage at 3.5 °C; (Watkins et al., 2004; Watkins et al., 2005). Conditioning treatments developed as part of the NE1836 activities are now standard practices (Beaudry, 2014). Conditioning treatments and the application of the antioxidant diphenylamine also help to suppress CO2 injury in Honeycrisp (Contreras et al., 2014). Chilling injuries are not limited to apple fruit; many peach cultivars are highly sensitive to low temperature storage (Lurie and Crisosto, 2005). Protocols for the diminution and/or avoidance of chilling in peaches have emerged from efforts of NE1836 team members (Manganaris et al., 2019; Moore and Farcuh, 2020). Early indicators of CI symptoms in fruits (e.g. loss of key volatiles, sugar metabolic shifts) can be used as potential predictors/diagnostic tools of susceptibility and may be helpful in developing management practices.
Bitter pit in apple is another serious disorder occurring in all growing regions in North America and is especially challenging for Honeycrisp and Granny Smith. Current efforts have focused on methods that predict disorders at or before harvest or that reduce risk of bitter pit (NE1836 publication in preparation). This work will allow growers to market high risk fruit before disorders develop and to put low risk orchards in long-term storage with minimal losses. Additional tests such as peel analysis (Baugher et al., 2017) can improve prediction accuracy (Moran, unpublished; Robinson et al. in Milkovich, 2023) and be performed by growers at relatively low cost. Conditioning treatments to suppress chilling injury in Honeycrisp enhance bitter pit (Watkins et al., 2004). However, a reduction in the O2 concentration during the warm temperature conditioning period (“rapid CA”) can suppress bitter pit formation (Serban et al., 2019), but the elevated CO2 during rapid CA sometimes leads to internal browning of this CO2-sensitive cultivar. As this method is an effective bitter pit/soft scald/soggy breakdown control strategy, determining “safe” combinations of temperature and CO2 is needed to reduce losses due to CO2 sensitivity during conditioning.
Sun stress has been evaluated by NE1836 team members as it relates to metabolic and spectral fingerprinting and quality of Granny Smith, Honeycrisp, and other apple cultivars (McTavish et al., 2020). Recently, hyperspectral analysis has identified indices that may be a predictive tool to minimize physiological disorders development, in particular sunscald, and reduce fruit quality variability in the supply chain (Torres, unpublished).
Storage disorders also plague the potato industry. In the U.S., potatoes are usually stored for up to 12 months in bulk storages with piles reaching 16 to 18 feet high (Muthukumarappan et al., 1994). Under such conditions potatoes can develop a defect called flattening or pressure bruise (Castleberry and Jayanty, 2012). The development of this defect is incompletely understood and might be related to multiple factors including cultivar susceptibility, hydration status of tubers, temperature, and ventilation, in addition to static pressure (Jayanty, 2009; Thornton and Bohl, 1998). While the physiological mechanism of this injury is only partially understood (Storey and Davies, 1992), several factors may play a role including membrane permeability (Herremans et al., 2014), mechanical resistance of a rigid cell wall (Castleberry and Jayanty, 2017; Ralet et al., 2016), cell hydration (Singh et al., 2014), and tissue organization and cellular properties (Gancaerz, 2016; Hudson, 1975; Zafeiri et al., 2021).
Tools to modify perishable physiology to maintain sensory and nutritional quality. Common postharvest treatments include placing fruits or vegetables in a modified atmosphere, the application of hormones or chemicals through sprays or dips, irradiation with ultraviolet light, or exposure to reduced or elevated temperatures (Elmnasser, et al., 2007; Garcia, et al., 1996; Lurie and Pedreschi, 2014; Perez, et al., 1999; Yaun, et al., 2004). The suitability of a particular strategy depends on the product, its condition at harvest and the intended market (Gross et al., 2016). Despite a long history of successes with notable contributions from the NE1836 membership, innovation continues. Technologies for applying modified atmospheres for the storage of some fruits and vegetables continue to evolve. For apple, dynamic controlled atmosphere (DCA) storage, used to apply the lowest possible O2 levels (Mditshwa et al., 2018), is of particular interest for organic apple production. However, DCA can negatively impact fruit aroma (Aubert et al., 2015), so there is some concern regarding the application of this technology. On the other hand, research in the current multistate project has revealed that DCA storage can markedly reduce internal browning disorders in expanding and emerging apple cultivars (DeEll and Lum, 2017). Modified atmosphere packaging (MAP) with or without an ethylene scrubber has potential to improve shipping mangoes at the tree-ripe stage.
1-Methylcyclopropene is a highly effective inhibitor of ethylene action, originally developed by members of the NE1836 team (Sisler and Blankenship, 1996). Despite the expiration of patents for use and formulation, new commercial delivery systems continue to be developed (Beaudry, 2021), some of which need evaluation for the development of commercial recommendations (da Silva et al., 2023). Testing the efficacy of these technologies and, importantly, recovery from 1-MCP for completion of ripening, is essential for providing up-to-date information for the industry. Moreover, many of the newer apple cultivars have very good post-storage quality maintenance, making the use of 1-MCP less critical. Nevertheless, the determining responses of new apple cultivars and other fruits to 1-MCP is a continuing need.
Other growth affecting chemicals with potential for postharvest applications for strawberry include methyl jasmonate (MJ), abscisic acid (ABA), salicylic acid (SA), and CaCl2. MJ treatment (la Pena Moreno et al., 2010) leads to a rapid decline in antioxidant content and the concentration of individual anthocyanins, providing some promise for improved coloration. Interestingly, MJ treatment can also suppress chilling injury in peach (Jin et al., 2009). The treatment can also accelerate ripening (la Pena Moreno et al., 2010), as does CaCl2 or naphthalene acetic acid when applied independently or in combination (Figueroa et al., 2012). In contrast, Garcia et al. (1996) reported that CaCl2 treatment improved strawberry firmness and total soluble solids. Treatment with ABA (Li et al. 2014) did not help maintain firmness, but increased redness (color factor a*) and higher soluble solids and anthocyanin levels. Salicylic acid can enhance vitamin C concentrations and reduce decay (Salari et al., 2013). Interestingly, benzo-thiadiazole-7-carbothioic acid S-methyl ester, a functional analogue of salicylic acid, enhanced total anthocyanin concentrations up to three-fold (Cao et al., 2010).
UV light treatment has the potential to enhance the red coloration and nutritional quality of apple fruit (Onik, 2019). Red coloration in apple fruits is highly desirable; however, poor coloration occurs with hot and humid conditions combined with the poor light environment. Delayed harvest to obtain color can result in harvest at an overripe stage which harms fruit quality, nutrition, safety, and storability. A focus, therefore, is to increase red skin coloration in a way that allows harvesting fruit at the optimal maturity, thus reducing fruit losses, increasing environmental sustainability, grower profitability and consumer acceptability. UV technology is easy to adapt with low cost of implementation, so we foresee it could potentially become a viable technology for ensuring high quality products while increasing consumer acceptability. There is a need to translate this work to diverse apple cultivars and other crops.
Heat and UV treatment, water stress, controlled/modified air, and application of natural compounds, such as jasmonates, chitosan, salicylic acid can impact antioxidant content of perishables (Gonzalez-Aguilar et al., 2010; Papoutsis and Edelenbos, 2021; Romanazzi et al., 2016a; Romanazzi et al., 2016b; Ruiz-García and Gómez-Plaza, 2013). There is a need for further investigation into the impact of these treatments on enzymatic and/or non-enzymatic antioxidant systems of fresh produce as they contribute the maintenance of fruit quality, antioxidant potential and disease resistance.
Edible coatings present a sustainable supplemental or alternative to standard storage methods for fresh produce and have attracted increasing attention due to environmental considerations and consumption trends toward the use of convenience foods (Dhall, 2013). Edible coatings can modify the internal atmosphere of perishables, inhibit metabolic processes like ripening or softening, but can also reduce water loss. Furthermore, edible films can be bioactive molecules (Dharini et al., 2021). Edible films of protein/polysaccharide coatings are quite attractive because of their impressive gas barrier properties and the fact that they can supplement or otherwise enhance the nutritive value of produce. However, the different fruit species behave differently during postharvest storage and handling. In addition, composite edible coatings such as AKORN that combine beneficial properties of a few components provide desired protective effect to the fruits (Mendes-Oliveira et al., 2022).
Tools for pathogen and decay management. Current disease control heavily relies on synthetic chemical input, but the unprecedented rate of emergence of resistance to fungicides is a serious challenge (Fisher et al. 2018). Numerous tools for decay control other than fungicides have been evaluated, but the adoption of new cultivars and technological options can alter efficacy.
CA treatments have been evaluated by several groups with mixed or sometimes contradictory results. For example, one research team concluded that CO2 enrichment suppressed non-microbial fruit decay (Gil et al., 1997; Holcroft & Kader, 1999), whereas other groups found that CO2 treatment increased decay (Alsmairat et al., 2011). Enrichment with O2 to levels of 60 kPa or more resulted in a reduced rate of decay and increased concentration of phenolics (Zheng, et al., 2007). Further investigation into atmosphere modification for decay control is warranted. Alsmairat et al. (2011) demonstrated that blueberry cultivars differ markedly in their tolerance to fungistatic CA, with some cultivars experiencing debilitating internal browning while others were seemingly unaffected.
UV-C treatment inhibits microbial growth in a dose-dependent manner and is not detrimental to quality traits at low doses (Erkan, et al., 2011; Pombo et al., 2011; Shin, et al., 2012). UV-C can stimulate a surge of PAL activity (Pombo et al., 2011), but flavonoid and anthocyanin concentrations were lower in treated than non-treated strawberries (Li et al., 2014). Work by NE1836 team members has revealed that the safer and less damaging UV-B can be used in place of UV-C. Strawberries showed a slight, but significant increase in total soluble phenolic content at the low UV-B dose (Du et al., 2014).
Thermal treatment to suppress decay is common for mango, but there is opportunity to evaluate its use on other crops. Exposure to hot air at 45 ˚C (Dotto et al., 2011) or immersion in hot water at 45 to 60 ˚C (Garcia, et al., 1995; Jing et al., 2010) can reduce decay on strawberries, but no evaluation of their effect on antioxidant or anthocyanin contents has been reported. Since strawberries are sensitive to cooling delay and delays more than 12 hours are detrimental (Kitazawa et al., 2013), strawberries are cooled shortly after harvest and stored under refrigeration. Therefore, it is not unexpected that there are no reports of chilling treatments, such as those done with cherry tomatoes (Gharezi et al., 2013), being used on strawberries.
The use of biocontrol agents to replace and or augment synthetic fungicides has many challenges, and there are untapped opportunities for the discovery of new biocontrol agents. Several products have been developed for postharvest biocontrol (e.g. BioSave, a formulation of Pseudomonas syringae), however optimizing antifungal activity has challenges. Additionally, some essential oils significantly reduce fruit decay of strawberry (Leepipattanawit et al, 1997). For instance, in one trial (Taghavi et al., 2021), nonenal had the lowest fungal decay, while clove bud oil had the highest fungal decay after four weeks of storage. The off-flavor shown in a small taste panel prevents the commercial application of the essential oils as sprays or dips. Eugenol had the lowest effect on the flavor and thymol had the most off-flavor.
Fundamental research on the physiology of perishables. Research on practical tools to preserve the quality of perishables and control pathogens is useful, but is also important to understand the biology of the crop to better apply postharvest interventions and to guide the creation and selection of cultivars with improved quality characteristics.
Significant ongoing research among NE1836 project team members uses integrated approaches to characterize fruit quality-related pathways. The climacteric ‘Santa Rosa’ plum and its unique non-climacteric bud-sport ‘Sweet Miriam’ (Minas et al., 2015) have served as tools for understanding the biology of ripening. Key sugar metabolism-related genes that affect fruit sugar composition were identified through integration of transcriptomics and metabolomics data, and subsequent pathway validation through molecular, biochemical and metabolic analysis. Key genes identified in this work could potentially be used as tools for manipulating these aspects in fruits, with direct effects on fruit sugar composition, nutritional value and postharvest storage capacity. Evidence has emerged of distinct hormone accumulation pathways and interactions occurring in fruits with contrasting ripening behaviors.
Ongoing efforts have identified key fruit texture and flavor-related pathways. In melon, phenotyping platforms have been developed to unravel genetic and metabolic linkages to fruit quality, shelf-life capacity and consumer acceptance. In apple, a novel pathway leading to the formation of branched-chain esters has been discovered and described (Sugimoto et al., 2021). The identified gene exists in two forms. One is functional and one is not. Knowledge of the sequence of this gene will help guide the selection of more highly flavored apple varieties. This work compliments earlier findings demonstrating that one of the primary apple ester odorants, hexyl acetate, is largely derived from the action of a single lipoxygenase (Schiller et al., 2015).
Fruit red skin color development is an economically important trait that is influenced by biological and environmental factors. This pathway interacts or is regulated by sugar and hormones, but how this occurs is unknown. In addition, recent results by team members support the notion that ethylene plays a key role in shifting plum fruit flavonoid profiles, which are also associated with changes in fruit sugars (Minas et al, 2015). These changes can directly affect fruit postharvest capacity, its nutritional value, as well as consumer acceptability.
Current work with small fruits is designed to understand the genetic basis of fruit texture, sugars, and organic acids. In blueberry, identifying potential markers for important quality traits will help to deliver higher quality fruit to the consumer. Recently introduced seedless muscadine storage life and fruit composition have been found to be like that of seeded muscadines.
Fundamental research on the physiology of decay organisms. Fitness costs associated with fungicide resistance impact resistance management, but are yet poorly understood (Hawkins and Fraaije 2018). Traditional methods to study fitness costs mainly involve in vitro assays of isolates with different resistance phenotypes (Chen et al. 2016). However, the major drawback is that isolates typically have a different genetic background, making it impossible to exclude potential impact on fitness from many genetic variations other than specific mutations conferring fungicide resistance. One way to overcome this hurdle is to generate transformants carrying mutation(s) or variations of interest only, without leaving footprints via CRISPR/Cas system. Additionally, genetic mechanisms of fungal virulence and those mediating the quiescent stage and transition into pathogenic lifestyle in the bitter rot fungus are largely unexplored, and a system to evaluate candidate genes using CRISPR/Cas would increase our understanding of infection biology.
Species within the genus of Colletotrichum often cause yield losses of fruits and vegetables (Dowling et al. 2020). Work by project team members has identified the molecular basis of fungicide resistance in C. siamense, a pathogen of blueberry and strawberry fruit, as being linked to E198A mutation in ß-tubulin gene.
Bitter rot, caused mainly by Colletotrichum spp, is a major disease of apple fruit (Kou et al., 2013). Phenotypic, metabolomic and biological investigations of wild apple germplasm with resistance to bitter rot are helping to build genomic resources to study sequence variation, determine sources of resistance, and the movement of specific loci and genes for breeders to improve decay caused by Colletotrichum fioriniae (Jurick II et al., 2011; Sun et al., 2017; Janisiewicz et al., 2016). Genes, regulators and genetic networks conferring resistance to bitter rot can also be transferred to commercial apple cultivars via the fast-trac breeding system to make decay management less reliant on synthetic pesticides.
Specific compounds identified in decay resistant wild apple germplasm may either play a direct role in abating decay and or prime host defenses against postharvest pathogens in apple fruit, or both (Sun et al., 2016). Such compounds may also impact biosynthetic pathways of the bitter rot fungi. Complementary to the chemical and genetic work, microbiome and microbial ecology have yielded many new bacterial and yeast strains that can be evaluated to combat decay pathogens.
Objectives
-
Adapt or develop harvest, handling and storage technologies to improve fruit and vegetable quality, increase consumption and reduce food waste.
-
Expand and translate fundamental plant biology to develop new storage technologies and plant materials that will enhance human nutrition and energy-efficient postharvest systems.
-
Advance our fundamental knowledge of host-pathogen-microbe interactions to maintain high-quality fruits and vegetables while reducing food loss and waste.
Methods
Methods
Due to size limitations, some details on methodology have been omitted.
Obj. 1. Adapt or develop harvest, handling and storage technologies to improve fruit and vegetable quality, increase consumption and reduce food waste.
1.1 Prediction and prevention of storage disorders and quality loss in ’Honeycrisp’ and other apple varieties (MD, ME, MI, NY, ONT, WA).
Prediction tools for chilling injury will be developed for ‘Honeycrisp’ (ME) using apples placed in a chest freezer set to -0.5 °C. Chilling injury after 3 weeks will be compared with chilling injury after 4 months cold storage. This test can be used to identify potential failures despite conditioning in fruit from high-risk orchards.
To address CO2 sensitivity during rapid CA, ‘Honeycrisp’ apples will be harvested from different orchards and at three maturities to account for differing risk of disorders (USDA-WA). Apples will be left unconditioned or conditioned and stored at varying CO2 and O2 concentrations. A small subset to be conditioned under the riskiest conditions for CO2 sensitivity will be treated with diphenylamine or 1-MCP, which controls or exacerbates, respectively, CO2-related disorders. After 6 months storage, fruit quality and disorders will be evaluated. Peel and flesh metabolites associated with elevated risk CO2 sensitivity will be measured periodically during storage.
To accelerate red coloration, ‘Honeycrisp’ apples will be submitted to different postharvest UV irradiation treatments during a 7-day conditioning period, and transferred to cold storage at 3 ºC, along with dark and white light controls (MD). UV treatment is at a preliminary stage with future plans to collaborate with engineers to develop a method for commercial application. This research is to determine the wavelengths that promote color without affecting quality. Evaluations will include measurements of ethylene concentration, fruit physicochemical properties, gene expression as well as metabolite quantification of color-related pathways. We expect to contribute to the identification of optimal dosage application to enhance fruit coloration, and consequently fruit nutritional value.
We will define harvest maturity that limits greasiness of WA 38 apples by studying year to year variation in fruit maturity (WA). We will also investigate the role of ethylene mitigation in greasiness and off-flavor. Fruit maturity will be determined in apples from two commercial blocks in 3 consecutive years. Peel greasiness will be measured starting 3-4 weeks before commercial harvest. Fruit will be stored in air or CA (2.5% O2, 1.5% CO2) for 6 months. Fruit quality will be assessed monthly. Off-flavors will be studied using trained panelists and metabolic profiles.
1.2 Determine the storage potential of new fruit varieties (CO, FL, MD, NC, NY, ONT)
Muscadine grapes will be held in large plastic bins (Janny MT) at 2 to 3 °C and 90% RH (NC). One bin will be sealed to increase CO2 to 10 kPa and the other will be left at ambient CO2. Oxygen and CO2 will be monitored in each bin using a Tiempo sensor. Tubing of 2 lengths will be inserted for sampling at different depths. Two flats of clamshells of fruit will be held in the same cooler to follow weight loss, color change, and development of decay or other storage problems.
The University of Florida blueberry, peach and strawberry breeding program evaluates these for eventual production in Florida. Cooling efficacy will continue to be studied for these crops (FL) and also for mango, mandarin, avocado and passionfruit. Tests will include validation of nontraditional cooling methods that show promise to extend postharvest quality by reducing cooling time and increasing cooling uniformity within the load, whether within a carton or within a pallet. Hydrocooling is an excellent, rapid and cost-effective method for cooling crops; of particular interest is to compare efficacy of drench hydrocooling with immersion hydrocooling. Shower hydrocooling may be more efficient and require less water than immersion hydrocooling, a key factor in drought-impacted states. Pulp temperatures of these crops will be measured before, during and after cooling by several methods including hydrocooling, forced-air cooling and vacuum cooling. Resultant crop quality will be assessed at regular intervals.
Quality and incidence of disorders in new apple cultivars will be compared at harvest and after air or CA/DCA storage to identify optimum maturity, temperatures and atmospheres (ME, MD, NY, ONT). Storage operator interest in DCA has increased greatly because of its potential to reduce internal browning. Cultivars will include those from the Midwest Apple Improvement Association (‘Evercrisp’, ‘Rosalee’, ‘Summerset’, ‘Sweet Zinger’) and breeding programs at CU (‘SnapDragon’, ‘RubyFrost’) and WSU (‘Cosmic Crisp’), as well as ‘Ambrosia’ and ‘Triumph’. Apples will be harvested and stored prior to quality evaluation and assessment of disorder susceptibility. The DCA will include multiple stress indicators, including chlorophyl fluorescence, and increases in ethanol and CO2 production. Each partner contributing to this sub-objective has access to multiple storage rooms and CA equipment. Teams in respective states/provinces will work together on cultivars of common interest.
Fifteen new and standard peach cultivars will be assessed for maturity and quality destructively and using the non-destructive sensors, DA meter and F-750 (CO, ME). We will develop cultivar specific models that can predict peach fruit maturity (index of absorbance difference, IAD) and internal quality (dry matter content and soluble solids). We will validate our developed models with independent groups of fruit under laboratory and field conditions. We will perform storage potential studies on the effect of maturity stage as determined by IAD and internal quality with focus on fruit softening and chilling injury.
1.3 Development of dynamic controlled atmosphere (DCA) storage for apple (MI, NY, ONT, WA)
Pre-and postharvest practices to optimize organic apple quality during and after long-term storage (MI, ONT, NY, WA). We will focus on ‘Gala’ as well as the new cultivars listed in section 1.2. Our goals are, using the same cultivars in different locations wherever possible, to define the effects of fruit maturity, storage temperatures, the pulldown time of O2, levels of kPa O2, and the effects of kPa CO2. The lower O2 limit for each variety will be determined in each year to account for year-to-year variation. As above, multiple indicators of product stress will be included to address cultivar high CO2 stress limits.
We will compare postharvest systems and protocols to optimize quality of apples organically grown in warm and cool production sites in WA state to incorporate climatic variability as an influencing factor (WA). Honeycrisp and Gala apples will be stored in RA, CA and DCA using respiratory quotient based on initial O2 level at 1°C or 3 °C depending on the cultivar. Dynamic control atmosphere (DCA) system will be managed using respiratory quotient (LabPods, Storage Control System, MI, USA). Fruit quality and physiological disorders will be evaluated after 3, 6, and 9 months of storage. The impact of DCA in combination with 1-MCP on aroma recovery will be documented for Honeycrisp, Gala, Evercrisp, Jonagold, Red Delicious, Fuji, and Golden Delicious (MI). The lower oxygen limit for each variety will be determined.
1.4 Determine efficacy of edible, water soluble corn zein films for shelf-life extension of fruit (CO). AKORN coating technology and other commercially available coatings and waxes will be evaluated in the following fruit species and cultivars: Gala and Honeycrisp apples, Bartlett and D’Anjou pears, Santa Rosa and Angeleno plums, May Glo and Honey Blaze nectarines, and Rojo Brillande persimmons. Coatings and waxes will be sprayed on half of the fruit (300 fruit per fruit species per treatment) using a system that has been developed by AKORN Technologies and will be compared to an untreated control. Ripening behavior and postharvest performance will be assessed at receiving and every 2 days for up to 10 days at 20oC following treatment.
1.5. Determining the storage potential of vegetables (CA, FL, MI).
Leafy greens and herbs will be stored under different temperatures and atmospheres to determine the best conditions to extend postharvest life (CA). The impact of chilling temperatures on the sensory quality of leafy herbs will be studied to determine the conditions after harvest that best maintain condition and sensory quality. Cooling applications will be tested for minor vegetables with potential for increased production in Florida, such as Asian vegetables, specialty potatoes, bamboo shoots, broccoli, Brussels sprouts, artichoke, and sweet potatoes (FL). The new in-package delivery methods for 1-MCP also show promise for several vegetables such as summer squash, cucumbers and leafy crops. The ability of CA storage (10 kPa CO2 and 11 kPa O2) on asparagus to help manage the glut during the peak production period will be evaluated. The effect of harvest date and cutting or snapping of the spear base will be determined (MI).
1.6. Evaluate the release kinetics of 1-MCP from various 1-MCP delivery systems (MI).
Several new delivery systems for 1-MCP have been developed, but growers and storage operators lack experience and perspective on the best way to deploy them. We will evaluate the rate of 1-MCP emanations from available 1-MCP release technologies using gas chromatography. Release rate will be described as a function of temperature, humidity, solution agitation, and adsorption by non-target materials.
Obj. 2. Expand and translate fundamental plant biology to develop new storage technologies and plant materials that will enhance human nutrition and energy-efficient postharvest systems.
2.1. Mechanism and control of physiological disorders (NS, ONT, MI and NY)
Identify mechanisms involved in disorders by applying ‘omic’ studies (volatiles, metabolomics, quantitative proteomics including peptide dimethyl labeling and multiple reaction monitoring with LC/MS) for profiling features regulating disorders. ‘Honeycrisp’ apples will be harvested from multiple commercial orchards and stored under CA conditions (2.0 kPa O2, 1.0 kPa CO2) at 1°C and 3.5 °C. After 0, 3, and 7 months of storage plus 1 week at 20 °C, firmness, soluble solids, titratable acidity and soft scald will be evaluated. At each evaluation, peels and flesh from 12 apples will be sectioned, pooled, frozen in liquid N2 and stored at -86 °C for further analysis. Volatile compounds in whole or homogenized fruit tissue will be collected from headspace by extraction with polymer traps or by using solid phase micro extraction (SPME) and analyzed by 2D-GC-MS. Untargeted (global) metabolomics will be applied for the comprehensive comparative analysis of metabolites. Protein extraction and quantification will be performed using a modified phenol extraction followed by ammonium acetate-methanol precipitation with detailed procedure for protein digestion and peptide desalting. The resulting peptides of total tryptic digestion from different biological samples will be labeled with isotopomeric dimethyl labels. Peptides analysis will be conducted on a mass spectrometer (Waters, q-TOF, Synapt, Milford, MA) equipped with a nano-electrospray source and nano-lock spray interface. Chromatographic separations will be conducted using a nano-Acquity UPLC system (Waters, Milford, MA).
2.2 Establish genetic maps of apple aroma, flavor volatile expression and bioactive compounds for improvement of fruit attributes and human nutrition (NS)
Fruit samples from an association mapping population (i.e., ABC, the biodiversity collection) will be harvested over two years at commercial harvest. Fruit samples will be stored at 3-4 °C. Texture, aroma volatiles, sensory attributes and bioactive compounds will be evaluated after 1 month. Metabolites involved with flavor volatiles will be measured using 2D-GC-MS. Volatile compounds in whole or homogenized fruit tissue will be collected from headspace by extraction with polymer traps or by using solid phase micro extraction (SPME) and analyzed by 2D-GC-MS. Untargeted (global) metabolomics that uses a Synapt qTOF (Waters Corp) liquid chromatograph-high resolution LC-MS will be applied for the comprehensive comparative analysis of metabolites. Genome-wide genotype data are available for the association mapping populations as part of ongoing research with Dr. Myles’ lab. Data collected from storage experiments to phenotype fruit attributes at Kentville will be analyzed with genome-wide genotype data. All phenotyping data collected will be linked with genotyping data and possible genetic control mechanisms will be identified through linkage mapping or GWAS. Identifications and quantitation from proteomic, genomic and metabolic analysis will be combined to reveal further possible biochemical pathways and validate key regulatory mechanisms of flavor, texture and bioactive compound metabolism.
2.3. Control of potato bruise symptoms after unloading from bulk storage conditions (ID). Due to the inevitable contact with oxygen after potatoes are unloaded from bulk storage, treatment with 1-MCP and nitric oxide (NO) may minimize the blackening of pressure-flattened tissues. ‘Russet Burbank’ potatoes will be harvested and submitted to two conditions:1) without pile pressure – 0 lb ft2 (control) and 2) constant force of ~375 lb ft2. Tubers will be stored at 7°C and 95% RH for up to 8 months. Five days before unloading, potatoes will be treated with 1-MCP for 48 hours with 1 mMol/L NO for 5 hours. Unloaded potatoes will be air-dried and transferred to ambient conditions to allow pressure bruise browning to develop. Samples will be taken 0, 2, 4, and 6 days after unloading when bruises will be evaluated using a visual scale. Electrolyte leakage will be assessed, and samples will be taken for PPO and POD activity determination.
2.4. Tracking senescence/freshness by monitoring changes in gene expression in broccoli and lettuce (FL and KS). We will focus on (1) using RNA-seq to identify senescence-associated genes (SAGs) for broccoli and lettuce that are associated with physiological age and (2) determining which of those SAGs are most highly conserved while also being expressed only at discrete stages of senescence. Similarities and differences in gene expression between natural (on-plant) and postharvest senescence will be compared to develop a better understanding of both processes, particularly the possible role of abiotic stress in postharvest senescence. A goal is to develop a quick test device utilizing colorimetric quantitative loop-mediated isothermal amplification (qLAMP) to measure product physiological age based on the presence of diagnostic freshness indicator genes.
2.5. Predictors of chilling injury development during peach cold storage (MD). We will characterize differences in fruit ripening patterns, physicochemical properties, chilling injury incidence, and aroma volatile composition between sound fruit and fruit exposed to CI-inducing conditions. ‘Red Haven’ peaches stored at 0, 5 and 20°C, will be analyzed for the traits listed above after 1 to 30 days of storage and after 3 days at 20°C. Overall, our results contribute to the identification of potential key aroma volatile compounds that could be used as early predictors of chilling injury. Also, we plan to identify similar volatile markers in other susceptible fruit crops.
2.6. Changes climacteric and non-climacteric plums and other fruits during postharvest storage (MD). We will study the impact of changes in ethylene and sugars in flavonoid metabolism-related pathways of the climacteric and non-climacteric plums and other fruits. Fruits subjected to ethylene treatments will be examined for transcript profiles of structural and regulatory genes of flavonoid-related pathways and their associated metabolites in skin and flesh, integrated with multivariate analyses of ethylene and sugar metabolism.
2.7 Characterization of aroma forming pathways in fruit (MI). The contribution of the citramalate pathway in the formation of esters in apple will be explored using highly specific inhibitors of pathways related to branched-chain amino acid metabolism. Whole fruit will be treated with sulfonyl urea and the volatile profile determined using GC/MS. Tissue disks from treated and untreated fruit will be fed with 13C-acetate and the incorporation of label into pathway constituents (alpha keto acids, amino acids, alcohols, aldehydes, and esters) determined. The impact of the suppression of the branched-chain esters on sensory perception will be assessed using a duo-trio test. The same pathway will be evaluated in banana and melon using molecular and biochemical approaches to characterize pathway enzymes. Initial work suggests a transcriptional modification leads to the synthesis of proteins lacking feedback regulation in a manner reminiscent of the citramalate pathway. We will explore the possibility that the ripening-dependent creation of a ‘non-regulated’ pathway is a common mechanism to enable precursor synthesis in ripening fruits and vegetables.
Obj. 3. Advance our fundamental knowledge of host-pathogen-microbe interactions to maintain high-quality fruits and vegetables while reducing food loss and waste.
3.1. Systems-based discovery of genetic resistance mechanisms in fruit and vegetables and fungal virulence genes (USDA-MD)
Specific apple accessions with resistance to bitter rot will be sequenced, assembled, annotated, deposited and made publicly available in the NCBI database. Our sequence data can be placed online for public access, and the publications, pipelines, and gene annotation programs used to develop these resources will also be available with open access.
We propose to use high-throughput Illumina platform sequencing to obtain whole genome sequence data for each of the 452 accessions that comprise 4 unique accessions with resistance. This will help identify causal SNPs and INDELs that coincide with a given phenotype.
High-quality wild apple (PI 369855 and GMAL 3689.h) and 4 Colletotricum fioriniae genomes will be obtained, using a combination of short- (Illumina) and long-read (Nanopore or PacBio HiFi) sequencing technologies combined with high-throughput chromatin conformation capture sequencing (Hi-C), to achieve sufficient coverage and genomic organization to assemble near gapless host and pathogen genomes. After sequence data is obtained, raw reads will be assessed for quality and downstream applications (e.g. assembly and annotation) using a combination of online, command line, and bioinformatics tools (e.g. Geneious). We will use established pipelines to determine transposon number, type and gene location, AntiSMash to determine SM gene cluster identity and type CAZyme, TF, etc. and SNP calling between fungal isolates.
Once high-quality C. fiorinae and wild apple genomes are sequenced, assembled and annotated, the next step will be to obtain fruit from susceptible commercial cultivars and wild apples with immunity to bitter rot. Five individual fruits will be inoculated. This experiment will help elucidate the genetics modulating wound responses and will also help ascertain signaling networks in the fruit controlling induced antimicrobial compounds, resistance genes in the host and virulence factor genes in the fungus. Total RNA will be isolated using standard procedures to assess total RNA quality and quantity of the samples. Total RNA will be sent to a company for library construction, sequencing and QC analysis of output data. Transcriptome sequence data will be assembled using a genome-guided mapping approach coupled with open-source R-based differential gene expression analysis pipelines. We will derive the list of differentially expressed genes (DEGs) for groups 3 and 4 which represent the transcriptomic changes associated with defense against the target pathogens at 24 and 96 hpi, respectively.
3.2. Develop and optimize chemical and biotechnological tools to abate decay and maintain fruit and vegetable quality (MD, USDA-MD)
Based on previous work from Sun et al., (2016), we will test the following specific purified compounds (epi-catechin, chlorogenic acid, procyanidin B1, B2, C1, querticin-3-O-glucoside, quinic acid, phloridizin, and Phloretin-xylosyl-glucoside) at physiological concentrations found in PI 369855 wild apple fruit in vitro Petri plate assays. Additionally, genes encoding proteins involved in the production of specific biochemical compounds and intermediates, identified from our wild apple fruit inoculated with the bitter rot fungus from our dual RNAseq analysis, will also be examined. These purified compounds will be tested in vitro using conidial germination and mycelial growth assays to assess their impact on C. fiorinae growth. Concentrations to inhibit 50% (EC50) growth will be determined.
Purified compounds with demonstrated in vitro efficacy will be evaluated using assays with susceptible varieties (Honeycrisp, Gala, Fuji) to test their ability to control decay caused by bitter rot. Finally, any compound or combination that shows >90% inhibition of decay, will be added to Shellac based wax or other industry utilized coating (e.g. Shieldbright 40), and the above tests will be conducted to see if the compound can be applied with wax to reduce decay. Tests with each compound as dip treatments will also be conducted to mimic drench treatments. To ensure that the decay control findings are practical and potentially impactful, inoculated fruit will also be stored at 1 °C in regular atmosphere over a 6-month period and monitored monthly for decay with balanced number of untreated fruit as a control group. Since we do not have CA or DCA storage at Beltsville MD, we will replicate this study using DCA and or CA (as available) with other collaborators in the project to increase the rigor and variety of commercial environments.
In another experiment, we will screen for potential bacterial isolates and natural compounds with high antagonistic activity against a set of postharvest pathogens. A commercial biofungicide Cease, Bacillus subtilis, will be the positive control against diseases (such as Colletotrichum acutatum and Alternaria alternata). About 70 bacterial isolates and several natural compounds will be screened in a dual culture system in vitro (Lahlali et al 2020).
We will study physical and chemical treatments that trigger plant defense responses through signal molecules. We propose to generate a marker-free CRISPR/Cas9 system to create resistant Colletotrichum spp. mutants with the point mutation E198A in ß-tubulin gene and other targets that mediate fungal virulence as determined in dual RNAseq studies proposed in the previous sub-objective of this proposal.
Fitness tests and assessment of fungal virulence: At least five resistant transformants will be randomly selected and tested for their resistance stability in vitro as described previously (Hu et al. 2014). Fitness components including mycelial growth at different temperatures in vitro, osmotic sensitivity in vitro, oxidative sensitivity in vitro, and aggressiveness/spore production in vivo will also be determined.
3.3. Evaluate newly developed tools for their impacts on postharvest shelf life, sensory aspects, and customer acceptability. VA
Newly discovered compounds, coatings, essential oils, microbial consortia and/or treatments that can thwart postharvest rot will be tested for their impact on sensory and consumer acceptability using trained panels and consumer panels. Treatment effects on apple fruit color, starch, sugar level, titratable acidity, pH, firmness will be conducted with standard fruit varieties (Honeycrisp, Gala, Fuji).
We will test plant volatiles (essential oils) for their efficacy in ex vitro, and in vitro conditions for decay control in strawberry. Fruit quality parameters (weight loss, total soluble solids, titratable acidity, and fruit decay) will be assessed. We are also looking for ways to reduce unwanted side-effects (off-flavor) and increase the efficacy of antifungal properties of natural compounds.
For both experiments, commercially used antifungal materials will be used as a control.
Measurement of Progress and Results
Outputs
- Basic understanding of the action of 1-MCP and NO on the potato pressure bruise development after unloading from bulk storage.
- Storage recommendations for new fruit cultivars that maintain high quality through storage and marketing.
- Recommendations for optimizing environmental conditions that favor storage of fresh vegetables and herbs
- Nonchemical methods for long-term storage and quality preservation of organic and conventional apples.
- Predictive tools for identifying fruit and vegetable storage problems at harvest.
- Twenty published research papers
Outcomes or Projected Impacts
- Extended shelf life of fresh fruits and vegetables and reduced waste, improved postharvest practices and increased profitability along the supply chain.
- Greater availability of high quality fruits and vegetables for consumers.
Milestones
Projected Participation
View Appendix E: ParticipationOutreach Plan
Results of this research will be made available through publications in refereed journals, grower and trade magazines, conference papers and proceedings, project reports, on-line sources (web), and presentations to industry. Several participants have partial extension appointments and develop outreach materials through fact sheets, web-based resources (e.g. https://www.uidaho.edu/extension/publications) and other extension publications. Extension publications include the NY Fruit Quarterly, the Good Fruit Grower, Spudman and the Potato Grower. In addition, states such as FL, MI, NY and WA have extensive formal industry venues for presentation of results to growers. These include storage workshops in MI and NY, which are held every two years, the New England Vegetable and Fruit Growers Conference, The NY Fruit and Vegetable Expo, Carolinas Fruit and Vegetable Expos, the NC Winter vegetable meeting, the SE Fruit and Vegetable Expo, Florida AgExpo, Annual Hood River Winter Horticultural Meeting, Pear Packers Pre-harvest meeting, Great Lakes Horticulture meeting, the Idaho Potato Conference (bilingual) and other fruit schools. Several project participants attend and are invited to present their results at grower meetings in other states and Canadian provinces. Overall, the project members have established excellent performance in ensuring that research results to colleagues in North America and to fruit growers and storage operators are made available in a timely manner.
Organization/Governance
One person at each participating agency is designated, with approval of the agency director, as a voting member of the Technical Committee. Other persons at agencies are encouraged to participate as non-voting members. The Chair, Secretary, and Administrative Advisor will conduct the activities of the multistate project between annual meetings. Officers can be any member, including the official voting representatives. The officers are elected every second year by voting members and serve a two-year term.
Literature Cited
Alsmairat, N., C. Contreras, J. Hancock, P. Callow, and R. Beaudry. 2011. Use of combinations of commercially-relevant O2 and CO2 partial pressures to evaluate the sensitivity of nine highbush blueberry fruit cultivars to controlled atmospheres. HortScience 46: 74-79.
Aubert, C., Mathieu-Hurtiger, V., and Vaysse, P. 2015. Effects of dynamic atmosphere on volatile compounds, polyphenolic content, overall fruit quality, and sensory evaluation of 'Pink Lady (R)' apples. Acta Hort. 1071: 275-280.
Barad, S., Sela, N., Dubey, A.K., Kumar, D., Luria, N., Ment, D., Cohen, S., Schaffer, A.A., Prusky, D. 2017. Differential gene expression in tomato fruit and Colletotrichum gloeosporioides during colonization of the RNAi-SlPH tomato line with reduced fruit acidity and higher pH. BMC Genomics. 4;18(1):579. doi: 10.1186/s12864-017-3961-6.
Baugher, T., R. Marini, J. Schupp., and C. Watkins. 2017. Prediction of bitter pit in ‘Honeycrisp’ apples and best management implications. HortScience 52(10): 1368-1374. https://doi.org/10.21273/HORTSCI12266-17.
Beaudry, R.M. 2014. Toward optimizing CA storage of Honeycrisp apples: Minimizing prestorage conditioning time and temperature. New York Fruit Quarterly 22:9-12.
Beaudry, R. 2021. Options for sourcing 1-MCP for 2021. https://www.canr.msu.edu/news/options-for-sourcing-1-mcp.
Cao, S., Hu, Z., Zheng, Y., and Lu, B. 2010. Effect of BTH on anthocyanin content and activities of related enzymes in strawberry after harvest. J. Agric. Food Chem. 58: 5801-5805.
Castleberry, H., Jayanty, S. 2012. An experimental study of pressure flattening during long-term storage in four russet cultivars with differences in at-harvest tuber moisture loss. Am. J. Potato Res. 89: 269–276.
Castleberry, H., Jayanty, S. 2017. Susceptibility to pressure flattening correlates with texture analysis of potato tubers. Am. J. Potato Res. 94: 556–566.
Contreras, C., N. Alsmairat, and R. Beaudry. 2014. Prestorage conditioning, and diphenylamine improve resistance to controlled-atmosphere-related injury in ‘Honeycrisp’ apples. HortScience 49: 76-81.
Chen, S. N., Luo, C. X., Hu, M. J., and Schnabel, G. 2016. Fitness and competitive ability of Botrytis cinerea isolates with resistance to multiple chemical classes of fungicides. Phytopathology 106: 997–1005.
da Silva, A. P. G., P. Engelgau, N. Sugimoto, R. Beaudry. 2023. Factors affecting 1-MCP release from an a-cyclodextrin encapsulant dissolved in water. XXXI International Horticultural Congress, Angers France, 14-18 August, 2022. Acta Hort. (in press).
DeEll, J. R., and Lum, G. B. 2017. Effects of low oxygen and 1-methylcyclopropene on storage disorders of ‘Empire’ apples, HortScience, 52(9): 1265-1270.
Dhall, RK. 2013. Advances in edible coatings for fresh fruits and vegetables: a review. Crit Rev Food Sci Nutr. 53(5):435-50. doi: 10.1080/10408398.2010.541568. PMID: 23391012.
Dharini, S., T.G. Nurdan, and R. Gianfranco. 2021. A comprehensive review on the impact of edible coatings, essential oils, and their nano formulations on postharvest decay anthracnose of avocados, mangoes, and papayas. Frontiers in Microbiology, Vol. 12, Article 711092. DOI=10.3389/fmicb.2021.711092.
Dotto, M.C., Pombo, M.A., Martinez, G.A., and Civello, P.M. 2011. Heat treatments and expansin gene expression in strawberry fruit. Scientia Horticulturae 130, 775-780.
Dowling, M., Peres, N., Villani, S., and Schnabel, G. 2020. Managing Colletotrichum on fruit crops: A “complex” challenge. Plant Disease. 104: 2301–2316.
Du, W.-X., Avena-Bustillos, R.J., Breksa III, A.P., and McHugh, T.H. 2014. UV-B light as a factor affecting total soluble phenolic contents of various whole and fresh-cut specialty crops. Postharvest Biol. Technol. 93: 72-82.
Elmnasser, N., Guillou, S., Leroi, F., Orange, N., Bakhrouf, A., and Federighi, M. 2007. Pulsed-light system as a novel food decontamination technology: A review. Canadian J. Micro. 53: 813-821.
Erkan, M., Wang, S.Y., and Wang, C.Y. 2011. Effect of UV treatment on antioxidant capacity, antioxidant enzyme activity and decay in strawberry fruit. Postharvest Biol. Tech. 48: 163-171.
Fabi, C., and English, A. 2019. Methodological Proposal for Monitoring SDG Target 12.3. Sub-Indicator 12.3.1.a The Food Loss Index Design, Data Collection Methods and Challenges. FAO. 60 pp.
Figueroa, C.R., Opazo, M.C., Vera, P., Arriagada, O., Diaz, M., and Moya-Leon, M.A. 2012. Effect of postharvest treatment of calcium and auxin on cell wall composition and expression of cell wall-modifying genes in the Chilean strawberry (Fragaria chiloensis) fruit. Food Chem. 132: 2014-2022.
Fisher, M. C., Hawkins, N. J., Sanglard, D., and Gurr, S. J. 2018. Worldwide emergence of resistance to antifungal drugs challenges human health and food security. Science. 360:739–742.Florida Tomato Committee. 2022. Annual report. Maitland, Florida. 24 pp.
Gancarz, M. Correlation between cell size and blackspot of potato tuber parenchyma tissue after storage. Postharvest Biol. Technol., v.117, p.161–167, 2016.
Garcia, J.M., Aguilera, C., and Albi, M.A. 1995. Postharvest heat treatment on Spanish strawberry (Fragaria x ananassa cv. Tudla). J. Agric. Food Chem. 43: 1489-1492.
Garcia, J.M., Herrera, S., and Morilla, A. 1996. Effects of postharvest dips in calcium chloride on strawberry. J. Agric. Food Chem. 44: 30-33.
Gharezi, M., Joshi, N., and Sadeghian, E. 2013. Effect of postharvest treatment on stored cherry tomatoes. J. Nutr. Food Sci. 2:8. DOI: 10.4172/2155-9600.1000157.
Gil, M.I., Holcroft, D.M., and Kader, A.A. 1997. Changes in strawberry anthocyanins and other polyphenols in response to carbon dioxide treatments. J. Agric. Food Chem. 45: 1662-1667.
Gonzalez-Aguilar, G.A., Villa-Rodriguez, J.A., Ayala-Zavala, J.F., and Yahia, E.M. 2010. Improvement of the antioxidant status of tropical fruits as a secondary response to some postharvest treatments. Trends Food Sci. Technol. 21: 475-482.
Gross K.C., Wang C.Y, and M. Saltveit. 2016. The Commercial Storage of Fruits, Vegetables, and Florist and Nursery Stocks. Agriculture Handbook Number 66.
Gustavsson, J., C. Cederberg, U. Sonesson, R. van Otterdijk, and A. Meybeck. 2011. Global Food Losses and Food Waste: Extent, Causes and Prevention. FAO, United Nations, Rome. 29 pp.
Hawkins, N. J., and Fraaije, B. A. 2018. Fitness Penalties in the Evolution of Fungicide Resistance. Annu. Rev. Phytopathol. 56:339–360.
Herremans, E., Melado-Herreros, A., Defraeyea, T., Verlindenc, B., Hertoga, M., Verbovena, P., Val J., Fernández-Vallee, E.M., Bongaers, E., Estrade, P., Wevers, M., Barreiro, P., Nicolaï, B. 2014. Comparison of X-ray CT and MRI of watercore disorder of different apple cultivars. Postharvest Biol. Technol. 87: 42-50.
Holcroft, D.M., and Kader, A.A. 1999. Carbon dioxide-induced changes in color and anthocyanin synthesis of stored strawberry fruit. HortScience 34, 1244-1248.
Hudson, D.E. 1975. The relationship of cell size, intercellular space, and specific gravity to bruise depth in potatoes. Am. Potato J. 52: 9-14.
Hu, M. J., Ma, Q. Y., Li, K. B., Lin, Y., and Luo, C. X. 2014. Exploring mechanism of resistance to isoprothiolane in Magnaporthe oryzae, the causal agent of rice blast. J. Plant Pathol. 96:249–259.
Janisiewicz, W.J., Evans, B.E., Bauchan, G.R., Chao, C.T., and Jurick II, W.M. 2016. Wound responses of wild apples suggest multiple resistance mechanism against blue mold decay. Postharvest Biol. Technol. 177: 132-140.
Jayanty, S. 2009. Prediction of pressure bruises susceptibility based on early tuber weight loss in storage. Spud Items 61: 1-4.
Jin, P., Y. Zheng, S. Tang, H. Rui, C.Y. Wang. 2009. A combination of hot air and methyl jasmonate vapor treatment alleviates chilling injury of peach fruit. Postharvest Biol. Technol. 52: 24–29.
Jing, W., Tu, K., Shao, X.F., Su, Z.P., Zhao, Y., Wang, S., and Tang, J. 2010. Effect of postharvest short hot-water rinsing and brushing treatment on decay and quality of strawberry fruit. J. Food Quality 33: 262-272.
Jurick II,W.M., and Cox, K.D. 2016. Pre and postharvest fungal apple diseases. Chapter 17 In Achieving sustainable apple production. Edited by Kate Evans.
Kitazawa, H., Sato, T., Nakamura, N., and Motoki, S. 2013. Effects of post-harvest cooling delay on weight loss, soluble solid and ascorbic acid contents of strawberry fruit. J. Food Agric. Env. 11: 372-376.
Kou, L., Gaskins, V.L., Luo, Y., and Jurick II, W.M. 2013. First report of Colletotrichum fioriniae causing postharvest decay on ‘Nittany’ Apple fruit in the United States. Plant Disease. 98:7.
la Pena Moreno, F., Monagas, M., Blanch, G., Bartolome, B., and Ruiz del Castillo, M. 2010. Enhancement of anthocyanins and selected aroma compounds in strawberry fruits through methyl jasmonate vapor treatment. Eur. Food Res. Technol. 230: 989-999.
Leepipattanawit, R., R.M. Beaudry and R.J. Hernandez. 1997. Control of decay in modified-atmosphere packages of sliced apples using 2-nonanone. J. Food Sci. 62:1043-1047.
Li, D., Luo, Z., Mou, W., Wang, Y., Ying, T., and Mao, L. 2014. ABA and UV-C effects on quality, antioxidant capacity and anthocyanin contents of strawberry fruit (Fragaria ananassa Duch.). Postharvest Biol. Technol. 90: 56-62.
Liu, X., Zheng, X., Khaskheli, M.I., Sun, X., Chang, X., Gong, G. 2020. Identification of Colletotrichum Species Associated with Blueberry Anthracnose in Sichuan, China. Pathogens. 9: 718, doi: 10.3390/pathogens9090718.
Lurie, S. and C. Crisosto. 2005. Chilling injury in peach and nectarine. Postharvest Biol. Technol. 37: 195-208.
Lurie, S., and Pedreschi, R. 2014. Fundamental aspects of postharvest heat treatments. Hort. Res. 1, 2014,14030.
Manganaris, G.A., A.R. Vicente, P.J. Martínez-García, and C.H. Crisosto. 2019. Peach and Nectarine. Chapter 13, pp 253-259. In: Postharvest Physiological Disorders in Fruits and Vegetables. Boca Raton: CRC Press, https://doi.org/10.1201/b22001.
McTavish, CK., BC. Poirier, CA. Torres, JP. Mattheis, DR. Rudell. 2020. A convergence of sunlight and cold chain: The influence of sun exposure on postharvest apple peel metabolism. Postharvest Biol. Technol. 164, 2020, 111164, ISSN 0925-5214, https://doi.org/10.1016/j.postharvbio.2020.111164.
Mditshwa, A., Fawole, O.A., and Opara, U.L. 2018. Recent developments on dynamic controlled atmosphere storage of apples - A review. Food Pkg. Shelf Life 16: 59-68. 10.1016/j.fpsl.2018.01.011
Mendes-Oliveira, G., Gu, G., Luo, Y., Zografos, A., Minas, I. Nou, X. 2022. Edible and water-soluble corn zein coating impregnated with nisin for Listeria monocytogenes reduction on nectarines and apples. Postharvest Biol. Technol. 185, 111811.
Milkovich, 2023. Honeycrisp storage passport stamped. Cornell researchers refine bitter pit prediction method. Good Fruit Grower Feb. 21, 2023. Honeycrisp storage passport stamped | Good Fruit Grower
Minas, I.S., Font i Forcada, C., Dangl, G.S., Gradziel, T.M., Dandekar, A.M., Crisosto, C.H. 2015. Discovery of non-climacteric and suppressed climacteric bud sport mutations originating from a climacteric Japanese plum cultivar (Prunus salicina Lindl.) Front. Plant Sci., Vol. 6, https://doi.org/10.3389/fpls.2015.00316.
Moore, K and M. Farucuh. 2020. What is chilling injury in peaches, what causes it, and how can you manage it? (FS-1141), is a series of publications of the University of Maryland Extension and the Department of Plant Sciences. https://extension.umd.edu/sites/extension.umd.edu/files/publications/What%20is%20Chilling%20Injury%20in%20Peaches%20%28Draft%29.pdf
Muthukumarappan, K., Gunasekaran, S., Curwen, D., Buelow, F.H. 1994. Investigations on potato storage management for control of pressure bruising. ASAE Paper No. 946582. Presented at the ASAE 1994 Winter meeting, December 13-16, Atlanta.
Onik, JC, Y. Xie, Y. Duan, X. Hu, Z. Wang, Q. Lin. 2019. UV-C treatment promotes quality of early ripening apple fruit by regulating malate metabolizing genes during postharvest storage. PLOS One, https://doi.org/10.1371/journal.pone.0215472
Papoutsis, K., and Edelenbos, M. 2021. Postharvest environmentally and human-friendly pre-treatments to minimize carrot waste in the supply chain caused by physiological disorders and fungi. Trends Food Sci. Technol. 112: 88-98.
Perez, A.G., Sanz, C., Rios, J.J., Olias, R., and Olias, J.M. 1999. Effects of ozone treatment on postharvest strawberry quality. Journal of Agricultural and Food Chem. 47: 1652-1656.
Pombo, M.A., Rosli, H.G., Martinez, G.A., and Civello, P.M. 2011. UV-C treatment affects the expression and activity of defense genes in strawberry fruit (Fragaria x ananassa, Duch.). Postharvest Biol. Technol. 59: 94-102.
Ralet, M.C., Buffetto, F., Capron, I., Guillon, F. Cell wall polysaccharides of potato. In: Singh, J., Kaur, L. (Eds). Advances in Potato Chemistry Technology. Second edition, Elsevier Inc., London. 2016. p.33-56.
Romanazzi, G., Sanzani, S.M., Bi, Y., Tian, S.P., Martinez, P.G., and Alkan, N. 2016a. Induced resistance to control postharvest decay of fruit and vegetables. Postharvest Biol. Technol. 122: 82-94.
Romanazzi, G., Smilanick, J.L., Feliziani, E., and Droby, S. 2016b. Integrated management of postharvest gray mold on fruit crops. Postharvest Biol. Technol. 113: 69-76.
Ruiz-García, Y., Gómez-Plaza, E. 2013. Elicitors: A tool for improving fruit phenolic content. Agriculture 3: 33-52.
Salari, N., Bahraminejad, A., Afsharmanesh, G., and Khajehpour, G. 2013. Effect of salicylic acid on post-harvest quantitative and qualitative traits of strawberry cultivars. Advances Env. Biol. 7: 94-99.
Schiller, D., C. Contreras, J. Vogt, F. Dunemann, B. Defilippi, R. Beaudry, and W. Schwab. 2015. A dual positional specific lipoxygenase functions in the generation of flavor compounds during climacteric ripening of apple. Hort. Research (Nature) 2, 15003; doi:10.1038/hortres.2015.3
Serban, C., Kalcsits, L., DeEll, J., Mattheis, J.P. 2019. Responses of ‘Honeycrisp’ apples to short-term controlled atmosphere storage established during temperature conditioning. HortScience. 54(9):1532-1539. https://doi.org/10.21273/HORTSCI14182-19.
Shin, Y.J., Song, H.Y., and Song, K.B. 2012. Effect of a combined treatment of rice bran protein film packaging with aqueous chlorine dioxide washing and ultraviolet-C irradiation on the postharvest quality of 'Goha' strawberries. J. Food Eng. 113: 374-379.
Singh, F., Katiyar, V.K., Singh, B.P. 2014. Analytical study of turgor pressure in apple and potato tissues. Postharvest Biol. Technol. 89: 44–48.
Sisler E.C., Blankenship SM. 1996. Methods of counteracting an ethylene response in plants, U.S. Patent 5518988.
Storey, R.M.J. and Davies, H.V. 1992. Tuber quality. In: P. Harris (Ed.), The Potato Crop. Chapman and Hall, London, pp. 507-569.
Sugimoto, N., P. Engelgau, A.D. Jones, J. Song, and R.M. Beaudry. 2021. Citramalate synthase yields a biosynthetic pathway for isoleucine and straight- and branched-chain ester formation in ripening apple fruit. Proc. Nat. Acad. Sci. 118(3) e2009988118. 10 pp. https://doi.org/10.1073/pnas.2009988118.
Sun, J., Janisiewicz, W.J., Nichols, B., Jurick II, W.M., and Chen, P. 2016. Composition of phenolic compounds in wild apple with multiple resistance mechanisms against postharvest blue mold decay. Postharvest Biol. Technol. 127: 68-75.
Taghavi, T., Bahamdan, G., Bell, M., Patel, H., Taylor, E. and Rafie, R. 2021. Comparison of five essential oils on postharvest strawberry fruit quality. Acta Hortic. 1309, 941-946
DOI: 10.17660/ActaHortic.2021.1309.134
Thornton, M., Bohl, W. (1998). Preventing Potato Bruise Damage. University of Idaho Extension Publications BUL 725.
Watkins, C.B., J.F. Nock, S.A. Weis, S. Jayanty, and R.M. Beaudry. 2004. Storage temperature, diphenylamine, and pre-storage delay effects on soggy breakdown, soft scald and bitter pit of ‘Honeycrisp’ apples. Postharvest Biol. Technol. 32: 213-221.
Watkins, C.B., M. Erkan, J.F. Nock, K.A. Iungerman, R.M. Beaudry, and R.E. Moran. 2005. Harvest date effects on maturity, quality and storage disorders of ‘Honeycrisp’ apples. HortScience 40: 164-169.
Yaun, B.R., Sumner, S.S., Eifert, J.D., and Marcy, J.E. 2004. Inhibition of pathogens on fresh produce by ultraviolet energy. International J. Food Micro. 90: 1-8.
Zafeiri, I., Beri, A., Linter, B., Norton, I. Understanding the mechanical performance of raw and cooked potato cells. Food Res. Int. 2021 Sep;147:110427
Zheng, Y., Wang, S.Y., Wang, C.Y., and Zheng, W. 2007. Changes in strawberry phenolics, anthocyanins, and antioxidant capacity in response to high oxygen treatments. LWT - Food Sci. Technol. 40: 49-57.