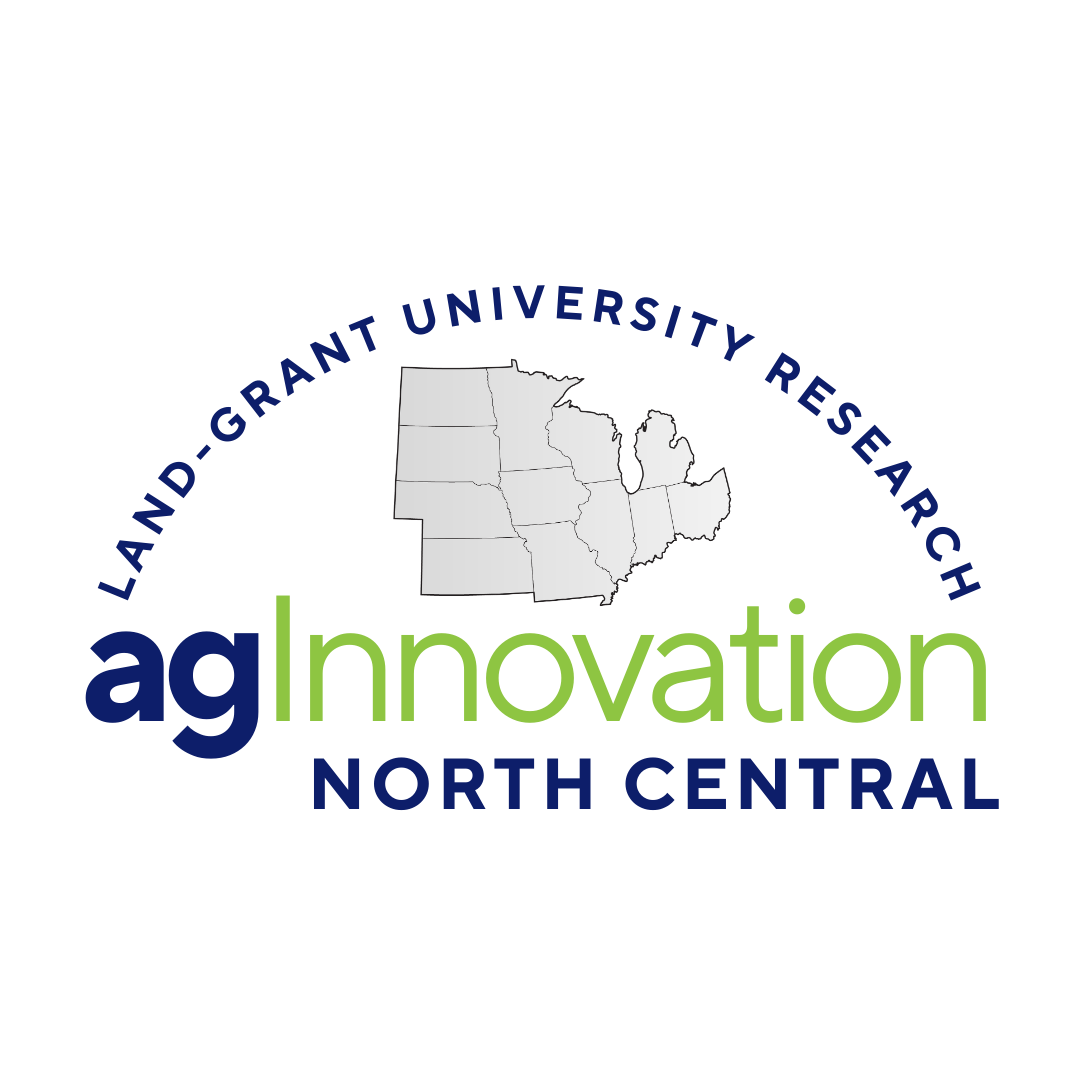
NC1170: Advanced Technologies for the Genetic Improvement of Poultry
(Multistate Research Project)
Status: Active
NC1170: Advanced Technologies for the Genetic Improvement of Poultry
Duration: 10/01/2023 to 09/30/2028
Administrative Advisor(s):
NIFA Reps:
Non-Technical Summary
Statement of Issues and Justification
How the proposed research addresses regional and/or national priorities and in context of stakeholder input:
The poultry industry in the United States underpins the global food system providing an efficient, economical and nutritious source of animal-based protein (meat and eggs). The industry foundation consists of breeding companies and growers/producers distributed throughout the nation (and the world) with a heavy concentration in states from which the members of Multistate Research Project NC1170 are drawn (e.g., Arkansas, California, Delaware, Georgia, Iowa, Minnesota, North Carolina, Virginia). The USDA Economic Research Service reports that the U.S. poultry industry is the world’s largest producer and second-largest exporter of poultry meat and a major egg producer. The USDA National Agricultural Statistics Service reported that the combined value of production from broilers, eggs, and turkeys in 2021 was approximately $47 billion. Many allied industries support and are impacted by the poultry industry, e.g., from grain producers and distributors to housing/cage manufacturers. Thus, the economic impact of the poultry industry to the financial health of the U.S. is enormous. The interactions of the poultry researchers involved in NC1170 with their stakeholders takes place via a variety of formats and opportunities so as to integrate our expertise with their needs and goals. These formats include, but are not limited to, organized informal meetings, workshops, and conferences (e.g., Poultry workshop at annual International Plant and Animal Genome meetings, annual National Breeders Roundtable, annual Poultry Science Association meeting), listservs, web-based tools/utilities useful to the industry, as well as collaborative ventures among research institutions and poultry industry businesses. Many NC1170 members have leveraged USDA-AFRI or other federal grants and have substantive collaborations with poultry breeders and producers. It is noteworthy that many NC1170 researchers not only utilize specialized poultry genetic lines for experimental analysis but also routinely employ commercially-selected foundational lines and crosses in their work.
The importance of the work, and what the consequences are if it is not done
Genetic improvements enabled the creation of a poultry industry that now produces the most widely consumed livestock commodity around the globe. The demand for poultry over the next decade is projected to increase even further as economic growth in certain regions drives the development of new markets. At the same time, the industry continues to face new and more complex challenges – rising feed costs, new disease outbreaks, changing climate – that threaten its ability to maintain production of an affordable and safe source of complete protein. The work of NC1170 focuses on using the power of poultry genomes to adapt to these challenges by making genetic improvements to chickens, turkeys, and other economically important avians. More specifically, NC1170 utilizes cutting-edge methods for genomics and bioinformatics to deeply mine poultry genomes and extract the pathways and molecular mechanisms that underlie phenotypes and determine how avians respond and adapt to their environment. This work produces invaluable fundamental knowledge about poultry genomes, and it also provides the functional information that is needed to guide improvements in the industry’s breeding programs and management practices. These methods, and the sophistication in approach enabled by the expertise of this group, allow a depth of knowledge that is not achievable through individual efforts or more conventional research methodologies. The work of NC1170 will contribute knowledge needed to further adapt poultry to their environment, enhance disease resistance, improve welfare, and maintain efficiency of growth and reproduction. Because NC1170 brings investigators together with stakeholders, this work is tightly aligned with the needs of the industry. It is also important to emphasize that the work of NC1170 is important for training the next generation of the poultry workforce, particularly in the ‘omics tools and approaches that are fundamental to maintaining the industry. Undergraduate and graduate students, as well as postdoctoral scientists, benefit from training in the labs of NC1170 investigators, and are exposed early on to the value of interdisciplinary solutions and the utility of new technologies. They also have opportunities to interact with other scientists and stakeholders through the activities of NC1170 members and through attending the annual poultry workshop. The importance of this role of our multistate group should not be overlooked.
The technical feasibility of the research.
The collection of investigators who participate in NC1170 are deeply entrenched in mining poultry genomes to benefit the poultry industry, and ultimately to feed the world. Some members of this multistate have been involved with sequencing and annotation of poultry genomes since the first draft sequence for chicken was published in 2004. Sequencing and analysis of both the chicken and turkey genomes was accomplished with the direction and collaboration of NC1170 researchers. NC1170 members are experts in their various fields, and collectively bring a breadth of technical expertise to the table. These investigators are at the leading edge of applying the latest ‘omics techniques to their work, often enhancing methods and analytical tools in the process. Because of the collaborative nature of this group and its productive history, these advances are readily shared with other members, keeping the group itself at the leading edge of poultry genomics. Each of the investigators has extensive expertise in poultry and avian biology, which enables them to recognize and utilize the wealth of opportunities that are presented by participation in in NC1170. The group includes domain experts in virtually every aspect of avian physiology, which enables the work of this group to be conducted from a systems perspective. The methods employed by the NC1170 investigators are state of the art techniques for genomics, transcriptomics, proteomics, and bioinformatics, for the purpose of exploring the fundamental biological mechanisms underpinning growth, development, physiology, nutrition, immunity and animal health. Efforts range among all scales, from fundamental to translational to applied. Due to the nature of ‘omics work, each investigator is well-versed in the skills needed to manage and mine very large datasets. Further, one can easily have confidence that the investigators involved in the NC1170 project will be at the forefront of development and application of new technologies during the next five-year project.
The advantages for doing the work as a multistate effort.
Using advanced technologies to genetically improve poultry is an inherently interdisciplinary effort that requires expertise from multiple domains. A multistate effort is an ideal vehicle to bring together principal investigators who can contribute the needed knowledge from various disciplines to tackle a challenge as complex as improving poultry production. NC1170 brings together geneticists, genome biologists, bioinformaticians, physiologists, immunologists, nutritionists, etc. in a way that would be very difficult to achieve in the absence of a multistate group. Moreover, it creates opportunities for the group at large to interact and exchange information with stakeholders, so that the research efforts can be aligned with needs of the industry. In effect, the NC1170 project is a sophisticated research tool that enables a systems level approach to improvement of poultry that would be very difficult to achieve in the absence of the multistate mechanism. New goals of the industry can be more effectively addressed by the combination of resources available by the NC1170 team approach. The NC1170 researchers possess an exciting combination of expertise to create and contribute new tools and knowledge to apply to poultry improvement. And the ability to meet, interact, and share information and resources fosters an environment for multidisciplinary interactions needed to address the complex biological questions facing researchers today.
What the likely impacts will be from successfully completing the work.
The poultry industry will likely face new and potentially more complex challenges to its ability to continue to supply the world with an affordable source of complete protein during the period covered by this proposal. It is expected that the work of the NC1170 will continue to help the industry to combat these challenges by contributing new knowledge that can be used to enhance breeding strategies and management systems. These new strategies can in turn be applied to improve growth, development, immunity, and physiology, and to maintain the economic and environmental sustainability of the industry. In addition to these translational impacts, completion of this work will make a major contribution of new, fundmental knowledge about avian genomes, including their similarities and differences, that will be valuable to the scientific community at large. Ultimately, completion of this work will support the production of an affordable, safe, and nutritious source of complete protein for the global population, while markedly advancing understanding of genome-to-phenome relationships in poultry.
Related, Current and Previous Work
A CRIS search was conducted to review active projects with the keywords "poultry", "genetics", and "genomics", resulting in 8 projects. Of these, 6 projects are led by members of NC1170; 5 of the 6 are HATCH projects, with the final one a USDA-AFRI grant award.
Previous Work and Accomplishments NC1170: Years 2017-2022
Membership and Institutional Abbreviations: Beckman Research Institute at the City of Hope (COH: M. Miller); California State University- Fresno (CS: K. Tarrant); Cornell University (CU, P. Johnson); Iowa State University (IA: S. Lamont, J. Dekkers); Michigan State University (MI: J. Dodgson, G. Strasburg); Mississippi State University (MS: M. Edelmann, B. Nanduri); North Carolina State University (NC: C. Ashwell, J. Petitte), Oregon State University (OR: D. Froman); Pennsylvania State University (PA: A. Johnson); Purdue University (PU: W. Muir); University of Arizona (AZ: F. McCarthy, S. Burgess); University of Arkansas (AR: B. Kong, D. Rhoads, W. Kuenzel); University of California, Davis (CA: M. Delany, H. Zhou), University of Delaware (DE: B. Abasht); University of Florida (FL: M. Edelmann), University of Georgia (GA: S. Aggrey); University of Maryland (MD: T. Porter, J. Song); University of Minnesota (MN: K. Reed); Virginia Tech (VA: Eric Wong, E. Smith); University of Tennessee (TN: B. Voy) University of Wisconsin (WI: G. Rosa); USDA-ARS-Avian Disease and Oncology Lab (ADOL: H. Cheng).
Below we describe by objective the major accomplishments achieved by the NC1170 Multistate Research Project covering 2018 to the summer of 2022. Achievements included the application of next generation sequencing technologies to improving poultry genomes and numerous contributions to advances in bioinformatics, annotation, and transcriptomics as applied to fundamental disciplines in poultry biology. Our work is conducted under the auspices of many collaborative arrangements and with stakeholders in the allied poultry industries for the purpose of improving poultry production. The scholarly publications resulting from this project (see appendix) includes over 300 articles in peer-reviewed journals, books and proceedings. Special note should be made of the large number of publications involving collaboration among members of this Project as well as international researchers.
Objective 1: Create and share data and technology to enhance the development and application of genomics, epigenomics, and systems biology in poultry.
With the development of high-throughput deep sequencing and the applications of start-of-art modern biotechnologies in the post-genomic era, NC1170 participants have achieved tremendous success in understanding poultry genetics, genomics, and biology, which further fosters collaborations and avoids duplication of effort.
ADOL and AZ invested significant effort into improving the quality and annotation of the chicken genome, which is critical for the efforts of members of NC1170. This work included release of a new chicken reference genome, GRCg6a, that improved the assembly based on the Red Jungle Fowl. More recently, new references assemblies were generated based on a commercial layer (bGalGal1.pat.whiteleghornlayer.GRCg7w) and a commercial broiler (bGalGal1.mat.broiler.GRCg7b). These reference assemblies have resulted in a marked increase in the number of transcripts identified in the chicken genome and new annotations of various regions of the genome. AZ provided standardized chicken gene nomenclature for GRCg7 broiler and leghorn annotations to support comparative studies, and annotations to support functional genomics analysis of poultry data sets. A workflow for predicting function of novel ncRNAs was also developed by AZ.
CA advanced the use of the chicken genome by functionally annotating regulatory elements across multiple tissues. These data, which are shared with the community, support efforts of multiple investigators to identify potential causative variants for economically important traits. These sets of eQTL are a valuable resource for deciphering the genetic and biological basis of complex traits in chickens.
Several groups created and shared data that are being used to understand that genetic basis for susceptibility to various infectious diseases in poultry. As part of efforts to use the genome to combat Marek’s disease (MD), a highly contagious and deadly viral disease of poultry, ADOL generated assemblies of the T cell receptor (TCR) locus for two chicken lines that differ in genetic resistance to the causative virus. ADOL also used single-cell RNA sequencing to define chicken immune cell types and their accompanying response to Marek’s disease virus (MDV) infection. WU focused on functional annotation of genes that are relevant to immune function in chickens. The transcriptomes of 20 immune cell and tissue types were defined using RNA seq, including characterizing the repertoire of lncRNA in each cell or tissue type. WU also utilized both ATAC-seq and CHiP-seq to analyze the epigenomes of each source. This effort included optimization of a new methodology (Cut and Tag). Data collection is nearly complete and data analysis is ongoing. CA also contributed to the study of the epigenome by developing and enhancing methods for ChIP-seq and ATAC-seq across tissues for use by the poultry genome community. MI used chemical proteomics approaches to annotate catalytically active kinases and deubiquitinases from chicken liver and spleen, both of which are relevant to pathogenesis of infectious disease. Machine learning and deep learning approaches were also used to identify farm practice variables that contribute to pathogen prevalence and multidrug resistance in pastured poultry.
Other valuable datasets were generated and shared by investigators working under Objective 1. TX created and shared (on pubic repositories) multiple whole genome, transcriptomic, and microbiome datasets that enhance the application of the systems approach in poultry. NC worked at the intersection of genetics and the environment by evaluating different genetic strains of layer hens, and how unique genetic features of each strain facilitated adaptation to various production housing systems.
Objective 2: Facilitate the creation and sharing of poultry research populations and the collection and analysis of relevant new phenotypes including those produced by gene transfer.
Given the high scientific value of characterized chicken lines in the genomic era and the significant costs required to maintain live flocks, NC1170 participants have been very proactive in sharing birds and derived materials and information, which further fosters collaborations and avoids duplication of effort. ADOL, IA, and CA each maintained and shared numerous lines of various types (e.g., inbred, congenic) for use in a range of genetic and disease model studies.
ADOL maintains a large number of highly inbred or closed chicken lines that are characterized for a number of traits, especially those associated with viral diseases, including maintenance under specific pathogen free (SPF) conditions. Besides providing unique genetic resources to our location, ~1,500 embryos or chicks are supplied yearly to academic institutions or companies in the United States, e.g., line 6x7 F1 birds were the primary individuals characterized by CA in their chicken annotation efforts. IA maintained chicken genetics research lines (including highly inbred, MHC-congenic, and closed populations; and advanced intercross lines) which are used and distributed as the biological discovery platform for research at IA, by collaborators in NC-1170 and others, e.g. ADOL, COH, DE, VT, and CA. Studies of these lines identified genomic regions, genes, and genetic networks of economic importance.
CA maintained and shared chicken genetic stocks (inbred, congenic inbred, and mutant) of specific genotypes expressing unique disease-related or developmental phenotypes. Highly inbred lines that differ in disease response to viral infection were sequenced to help understand the underlying genetic basis. Additionally, regulatory elements (e.g., enhancers, promoters, insulators) were functionally annotated in a number of lines.
COH evaluated MHC-Y haplotypes segregating in experimental inbred and highly-selected lines, e.g., Fayoumi and White Leghorn lines from IA, and high and low antibody selected lines from VA. Investigation has demonstrated a relationship between certain MHC-Y haplotypes and immune responses
TX maintained a Red Junglefowl population of the Richardson strain. This well documented research population has become a key resource to look at the developmental biology of morphological traits in chicken. Additionally, a RJF x Silver Sebright cross is being used to map feathering and pigmentation traits.
Objective 3: Elucidate genetic mechanisms that underlie economic traits and develop new methods to apply that knowledge to poultry breeding practices.
The ability to utilize poultry genomes and their encoded information to maintain healthy, profitable and sustainable production is critical for the poultry industry. Members of NC1170 are vested in using their expertise to identify new ways to prevent disease, enhance growth, and promote welfare and wellbeing through genetic improvements. Accordingly, a range of physiological conditions and phenotypic traits have been intensively studied as part of Objective 3.
Disease susceptibility and infection.
ADOL determined that somatic mutations are required in addition to Marek’s disease virus (MDV) infection for virulent MDV to induce tumors. Ikaros was identified and validated as a major cancer driver gene for MD. ADOL also investigated the roles of T cell receptor (TCR) genes and usage in response to MDV infection. UM characterized copy number variations and epigenome modifications associated with the resistance to MD using next-generation sequencing.
WU elucidated molecular mechanisms of differences in in disease resistance and susceptibility in chicken lines with different MHC-B haplotypes. Several potential causative differences were found, including that more effective macrophage responses were found to underlie enhanced disease resistance in B2/B2 vs. B19/B19 lines. WU also investigated the role of epigenetic responses to viral infections, and genetic variation in the immune response to avian coronavirus. CSU identified MHC haplotypes in breeder silkie populations.
AR successfully developed a new hybrid model to induce bacterial chondronecrosis with osteomyelitis (BCO) and lameness in broiler chickens. The new model induces lameness up to 70-80% in birds raised on litter flooring. This model was used to identify a prebiotic that reduces the incidence of BCO lameness. AR also advanced understanding of genetic factors that increase the incidence and severity of ascites, a deadly consequence of pulmonary hypertension in broiler chickens. This work demonstrated that the genetics of ascites are intimately dependent on specific genetic background, which lays the groundwork for follow-on studies.
MN investigated the response to aflatoxin exposure by characterizing the transcriptome level changes in the liver, intestine and spleen of birds exposed to AFB1. RNAseq datasets produced from this work were shared publicly.
Susceptibility to stress and the environment
IA characterized genetic variation in commercial, research, and global indigenous chicken populations in collaboration with CA. This work identified genomic regions and candidate genes associated with response to heat stress, and to bacterial and viral infections in chickens. It also identified gene expression responses associated with body composition, heat stress, and disease status. CA in collaboration with IA, developed a low-density panel associated with resistance to heat stress and Newcastle disease virus infection that can be used to breed more resilient chickens in adaption to hot climates in developing countries and improved knowledge of genes associated with an enhanced immune response may inform further information on vaccine efficacy in poultry production. IA determined gene expression responses associated with body composition, heat stress, and disease status.
UG identified highly deleterious alleles that are more frequent in recent inbreeding, revealed that heat stress limits the reproductive capacity of Eimeria maxima in broiler chickens, Eimeria acervulina infection alters lipid absorption and utilization, and genomic matrix should evolve from a measure of realized additive relationships to an optimum measure of genetic similarities between individuals.
AR identified genes associated with the stress response of birds and identified an additional component of the functional stress axis. AR also used whole genome resequencing to identify copy number variants that diverge between high stress and low stress lines of quail.
Growth, development, and muscle myopathies
MD used cell and molecular technologies to define the mechanisms underlying pituitary development and cell differentiation, and to identify genes and gene networks involved in neuroendocrine (hypothalamic and pituitary) control of growth, development, and body composition in broiler chickens. TN incorporated transcriptomics and metabolomics to understand the mechanisms through which the broiler breeder hen diet can be used to developmentally program body composition in offspring. MI identified Death-associated protein (DAP) that would likely negatively affect muscle development, discovered circular RNAs are differentially expressed with temperature changes and among genetic lines, and found growth selection resulting in large muscle fibers may lead to hypoxia which may lead to altered glucose metabolism, cellular detoxification, and muscle degeneration.
AR identified miRNAs associated with broiler breast muscle myopathies. Small molecule and lipid metabolite biomarkers of myopathies were also identified through a combination of metabolomic and lipidomic profiling of blood from affected and unaffected broilers. In collaboration with DE, AR identified specific signaling pathways associated with muscle growth and determined that phytase supplementation was significantly reduced woody breast. DE identified promising candidate genes and circulating biomarkers for Wooden Breast and White Striping in modern broilers, developed a new tool (VADT; https://github.com/mjtiv/VADT) for the detection of allele-specific expression (ASE) from RNA-seq data, and characterized molecular and morphological changes in the breast muscle of modern broiler chickens over the growth period and in respect to the onset and development of Wooden Breast and White Striping.
TX used WGS and optical mapping to identify genome structural variants associated with metabolic disorders in fast-growth broilers. MN, in collaboration with MI and OR, quantified climate change impacts on poultry breast muscle growth and development, morphological structure, intramuscular fat deposition, and protein functionality to develop appropriate strategies to mitigate the undesirable changes in meat quality. CSU assessed mitochondrial DNA concentration in broiler chickens with woody breast.
Gut health and the gut microbiome
MN advanced the understanding of interactions between the turkey gastrointestinal microbiome and host during maturation and microbiome modulation. TX developed new knowledge to enhance commercially important traits (breast meat quality, early life microbiome assembly, early life immune responses), which are helping improve breeding and management practices in poultry. They showed the role of lighting in influencing gut microbiome assembly.
Poultry production
CU used RNAseq to investigate the molecular basis for declining productivity with age in laying hens. Upstream regulator analysis revealed the estrogen receptor as a predicted upstream regulator of laying persistence. UM characterized hypothalamic, pituitary, and ovarian tissue transcriptomes in turkey hens with high and low egg production rates. PA studied the expression of genes related to steroidogenesis, such as FSHR, STAR, CYP11A1, HSD3B, and progesterone secretion was significantly decreased in response to metformin treatment in a dose-dependent manner in broiler breeders. WI in collaboration with UM, evaluated the effect of Se deficiency and high dietary Se on tissue Se and selenoenzyme activity in turkeys to better define Se requirements and safe upper limits for dietary Se supplementation
WI used deep learning to predict complex traits in broilers by utilizing genomic information, assessed current trends of local chicken breeding and factors that influence their production performance in Benin, Africa, and developed a QTL-directed dependency graph mapping approach to investigate potential causal phenotypic networks for egg traits in an F2 chicken population. IA Developed and evaluated strategies for the implementation of genomics in poultry breeding programs.
Objectives
-
Create and share data and technology to enhance the development and application of genomics and systems biology in poultry.
-
Facilitate the creation and sharing of poultry research populations and the collection and analysis of relevant new phenotypes including those produced by gene transfer.
-
Elucidate genetic mechanisms that underlie economically important traits, including genetic variants and functional regulatory elements within the genomes of poultry species, and develop new methods to apply that knowledge to poultry breeding practices.
Methods
Objective 1. Create and share data and technology to enhance the development and application of genomics, epigenomics, and systems biology in poultry.
Objective 1 will collect and disseminate both data and methods to improve our understanding of the interaction between the genome and the environment. Environment encompasses all levels including the nucleus, cell, tissue, organism and external environment. Ultimately, it is the combination of the genome and environment that gives rise to the phenotypes important to poultry production. Systems biology approaches will be applied to model and integrate data across these various levels to improve our ability to apply genomic understanding to improving poultry production. Advanced data mining methods (e.g., machine learning) will be applied to extract patterns from the ever-growing scope of data, made by possible by continual advances in ‘omics methods and platforms. The new reference assemblies of the chicken genome will be fully annotated, which will augment work by all members of NC1170. Advanced DNA sequencing platforms and tools to mine transcriptomes and epigenomes at the single cell level will be fully utilized to maintain the cutting-edge nature of the work done by NC1170. Other ‘omics tools – e.g., metabolomics, lipidomics, proteomics – will be enlisted to collect additional large and complementary data sets. Where possible, these tools will be applied to novel lines and genetic models created and or maintained by members of this group.
ADOL will continue to improve the chicken genome assembly, including aiding the development of a pan-genome and assemblies of various MHC and TCR haplotypes, and aid in annotation efforts, especially concerning identifying full-length transcripts, alternative transcription start sites (TSS) or splicing events, RNA methylation or editing, associated proteins, etc.; and will develop biomarkers for specific immune cell populations. Meanwhile, ADOL will aid in developing chicken cell lines for tissues of interest, e.g., muscle and fat.
CA will aid in improving the chicken genome assembly, including assemblies of various MHC and TCR haplotypes, and annotate and validate regulatory elements in vitro in developing high-throughput technology. CA will also develop iCRISPR and aCRISPR cell lines to evaluate the functions of regulatory elements in DF-1 and muscle cell lines.
AZ will create and share data and technology to enhance the development and application of genomics, epigenomics, and systems biology in poultry, provide predicted functional annotations for novel mRNAs and ncRNAs identified by FAANG and related projects, and continue to develop standardized chicken gene nomenclature, focusing on genes specific to chicken & on supporting gene nomenclature in other poultry species.
TX will continue the creation and dissemination of new assays and datasets, particularly focusing on broiler and broiler breeder performance.
NC will evaluate the array of gene expressions (stress, nutrient absorption, immune response) that can be altered by a different housing density in layers hens, and correlate gene expression in different thicknesses with layers hen’s production, health status, and welfare.
MI will apply machine learning and deep learning approaches to mine microbiome data beyond diversity metrics to identify microbiome signatures that predict pathogen prevalence in poultry meat.
WU will continue to share data on cis-regulatory elements in chicken tissues and immune cells and build enhancer maps using Hi-C and will also create single-cell data for chicken tissues of economic importance.
OR will elucidate the molecular and cellular mechanisms of intestinal immune responses to enteric diseases in chickens by performing RNA-seq and/or scRNA-seq on sorted immune cells that confer protection to enteric diseases (coccidiosis and necrotic enteritis). The metabolic role of intraepithelial lymphocytes in the progression of wooden breast syndrome in broilers will also be investigated.
Objective 2. Facilitate the creation and sharing of poultry research populations and the collection and analysis of relevant new phenotypes including those produced by gene editing
We will achieve this objective by maintenance and development of specialized poultry populations, cell lines and resources for researchers to effectively investigate key production traits. We support the creation, sharing and phenotyping of genetic material (e.g., chicks, fertile eggs, blood, tissue, DNA or RNA) for collaborative studies. This includes the maintenance or development of avian cell lines to support in vitro studies, as well as resources for in vivo research.
Our approach is to identify both in vivo and in vitro resources that serve as tools for identifying the causal agents for economically important traits. Poultry lines and populations include (but are not limited to) inbred lines, congenic lines and advanced intercross lines that are genotyped for relevant traits and phenotypes, as well as pathogen-free populations. We also support the development and maintenance of avian resources for furthering in vitro studies. These studies may include (but are not limited to) investigating molecular mechanisms underlying important production traits, understanding how gene expression is regulated to produce observed phenotypes and determining function of genetic elements that contribute to traits and phenotypes. Resources to support mechanistic and functional in vitro or in ovo studies include the development of cell lines, and technologies for creating transgenics and mutants (e.g., gene delivery, over-expression, knock downs and targeted mutagenesis).
ADOL will maintain and distribute to NC1170 collaborators and more, a large number of highly inbred or closed chicken lines that are characterized for a number of traits, especially those associated with viral diseases, including maintenance under specific pathogen free (SPF) conditions. Efforts are already planned to use one or more of these lines for genomic modification via CRISPR by collaborators at PGRU.
IA will maintain and share genetic material from unique chicken research lines (including diverse, highly inbred lines; MHC-congenic lines; and highly advanced intercross lines of more than 30 generations of intercrossing) as resources for identifying genes, genomic regions, and genetic networks of economic importance.
NC will create a Red Junglefowl flock as a source of genetic material to study the evolution of gut health in poultry and the genetic markers. TX will continue to maintain a Red Junglefowl population (Richardson Strain) and provide access to live birds as well as tissues to the community of poultry scientists and broader avian biologists.
CA will maintain 16 chicken lines with special genetic characteristics for muscle dystrophy, other mutations or viral susceptibility that will be shared with NC1170 groups and other outside collaborators.
Objective 3. Elucidate genetic mechanisms that underlie economically important traits (genotype to phenotype) including genetic variants and functional regulatory elements within the genomes of poultry species, and develop new methods to apply that knowledge to poultry breeding practices.
Objective 3 will address the mechanistic underpinnings of production-relevant traits by applying advanced ‘omics tools and data mining methods to further understand growth, development, performance, and disease-resistance in poultry. We will use both well-established genomic methods (e.g., whole genome RNAseq, proteomics) as well as newly established and emerging methods (e.g., single-cell RNAseq, spatial transcriptomics) in combination with key animal resources (stem cells, inbred research lines, commercial lines, and exotic ecotypes).
Disease susceptibility and infection.
ADOL will study the molecular basis for genetic resistance to MD, identify specific alleles in cancer driver genes associated with MD genetic resistance, determine if MD genetic resistance and/or MD vaccines contribute to MDV evolution to higher virulence, and determine if there is MHC or non-MHC genetic resistance to infectious bursal disease (IBD) or infectious laryngotracheitis (ILT). CA will collaborate with IA and ADOL to determine the molecular basis for genetic resistance to NDV and AIV, and determine the roles of functional regulatory elements in regulating immune response and disease resistance. UM will explore the mechanisms and epigenetic modifications in chicken immune CD4+ T during MDV infection, anticipating discovering key regulator factors and targets in MD resistance.
WU will evaluate the role of CHIR in disease resistance, investigate KIR in chicken reproduction, investigate epigenetics of viral infection in chickens with different genetic backgrounds, and determine the effect of trained immunity on disease resistance in different chicken lines. CS will evaluate MHC haplotypes association with Northern Fowl Mite infestation in laying hens. OR will elucidate the molecular and cellular mechanisms of intestinal immune responses to enteric diseases in chickens using RNA-seq and/or scRNA-seq on sorted immune cells that confer protection to enteric diseases (coccidiosis and necrotic enteritis). AR will continue to work on BCO and lameness.
Susceptibility to stress and the environment
IA will identify genetic determinants of host response of chickens to biotic and abiotic stressors. AR will continue to work on the neuroendocrine regulation of stress. GA will focus on adaptation to heat stress and water needs, including elucidating the molecular basis for variation in water needs. Genetic influences on protein deposition and feed efficiency under heat stress will also be investigated.
Growth, development, and muscle myopathies
OR will investigate the metabolic role of intraepithelial lymphocytes in the progression of wooden breast syndrome in broilers. AR will continue to work on breast muscle and myopathies, including woody breast. DE will study spatial transcriptomics of the breast muscle at the single-cell level, identify causal genes for Wooden Breast and White Striping in modern broilers, and study systemic lipid metabolism and anomalies in vasculature.
UMN will continue to work on poultry breast muscle growth and development, in collaboration with MI and OR, to develop strategies to mitigate undesirable changes in meat quality. CA will collaborate with IA and ADOL to identify potential causative variants associated with growth rate and feed efficiency, and determine the roles of functional regulatory elements in regulating immune response growth-related complex traits.
UM will investigate the critical regulatory factors in chicken embryonic development. UM will also perform RNAseq and single-cell RNAseq on tissues from turkeys and chickens, to define the mechanisms regulating reproduction in turkeys and growth and body composition in broiler chickens. WI will investigate the feeding behavior of broilers and its relationship with feed efficiency and production traits; develop predictive models for the detection of more efficient animals, and estimate genetic parameters (e.g., heritability and genetic correlations) for feeding behavior and feed efficiency in broilers. TN will focus on extracting the transcriptomic and epigenomic mechanisms thorough which the hen diet alters body composition in broilers.
Gut health and the gut microbiome
NC will investigate gene expression alterations correlated with nutrient absorption, inflammatory gut response, and immune response in broiler breeders under alternative feed program during the early development, and egg production phase.
Poultry production
IA will develop and evaluate strategies for implementing genomics in poultry breeding programs. NC will evaluate the transgenerational (epigenetic) effect of broiler breeders alternative feed program on the progeny. TX plans to continue working on broiler breeder issues, including reproductive performance, immune maturation, and microbiome modulation.
PA will determine the extent to which metformin and PPAR-gamma agonists affect hepatic lipogenesis and yolk precursor synthesis, estrogen receptor signaling in the liver and in hepatocytes, and determine the appropriate feed level and metformin treatment duration to maximize fertile egg production from broiler breeder hens.
Measurement of Progress and Results
Outputs
- Continued structural and functional annotation of poultry genomes, including the new reference assemblies for chicken
- Enhancement of bioinformatic methods and tools for use in genome analysis of poultry
- Establishment and maintenance of genetically and phenotypically characterized poultry populations, cell lines, and associated resources that support genetic and functional analysis of production traits in poultry.
- Identification of genes, pathways and mechanisms that underlie variation in production-relevant phenotypes, disease resistance, and adaptation to the environment.
- New knowledge to guide selection of specific breeds and genetic lines for sustainable poultry production in challenging environmental conditions
Outcomes or Projected Impacts
- Advances in genetic and functional information that will inform breeding programs aimed at improvements in poultry production
- Development of new tools for bioinformatics and genome analysis for research community use made available in easily accessible databases
- Richer and more granular functional annotation and fundamental knowledge of poultry genomes for broad use by the scientific community and stakeholders
- New, comprehensive, publicly-available ‘omics datasets
- Education of students and postdoctoral scholars and collaboration with many visiting international scholars drawing their attention to our project goals
Milestones
(1):Timeline 1. Continued structural and functional annotation of poultry genomes, including the new reference assemblies for chicken -- Years 1-3 2. Enhancement of bioinformatic methods and tools for use in genome analysis of poultry -- Years 1-5 3. Establishment and maintenance of genetically and phenotypically characterized poultry populations, cell lines, and associated resources that support genetic and functional analysis of production traits in poultry -- Years 2-4 4. Identification of genes, pathways and mechanisms that underlie variation in production-relevant phenotypes, disease resistance, and adaptation to the environment -- Years 1-5 5. New knowledge to guide selection of specific breeds and genetic lines for sustainable poultry production in challenging environmental conditions -- Years 3-5Projected Participation
View Appendix E: ParticipationOutreach Plan
Technical Committee (TC) members will publish NC1170-project-generated data in the peer reviewed literature, thus making content accessible to the scientific community. In addition, members participate in conferences and workshops (e.g., Poultry Science annual meeting, National Breeders Roundtable, International Plant and Animal Genome annual meeting, and many disciplinary or technology-related meetings) providing oral presentations of results and proceedings (written communications). Most conference and workshop environments welcome stakeholder participation and interaction. In addition, committee members frequently provide seminars on their work at other academic institutions and companies. Those conducting genome sequencing and analysis deposit their results in relevant federally-funded databases allowing access by the scientific community and stakeholders. Several NC1170 members also participate in other multistate research projects (e.g., NE1834) allowing for cross-fertilization of techniques and relevant content. Several members are organizers of databases as part of their project efforts and ensure dissemination of NC1170 data, tools and content.
Organization/Governance
The organization and planning of the NC1170 Project are the responsibility of the Technical Committee (TC) members consisting of scientists from land-grant or non-land grant research institutions, the USDA-ARS, and industry. Administrative guidance will be provided by an assigned Administrative Advisor and a USDA-NIFA Representative. Voting membership shall consist of the TC members; only one member representing each participating agency shall be eligible to vote. Our governance includes a two-year term for a Chair and a Secretary. An executive committee of Chair, Secretary and immediate past-Chair (if needed) coordinate business between meetings. Volunteer members of the TC organize objective content for necessary reports. Members are expected to write and distribute annual reports to the Technical Committee and administrators through NIMSS prior to each annual meeting. In addition, members affiliated with agriculture experiment stations submit results to NIFA through NRS, NIFA’s Reporting System. Members are expected to attend annual meetings at least three of five years. Attendees at meetings can include emeritus members, collaborating scientists, invited colleagues, scientists interested in the topical areas discussed.
Literature Cited
NC1170 publications (350) during the reporting period
- Abasht B*, Papah MB, Qiu J. 2021. Evidence of vascular endothelial dysfunction in Wooden Breast disorder in chickens: Insights through gene expression analysis, ultra-structural evaluation and supervised machine learning methods. PLoS One. 16(1):e0243983.
- Abasht B*, Zhou N, Lee WR, Zhuo Z, Peripolli E. 2019. The metabolic characteristics of susceptibility to wooden breast disease in chickens with high feed efficiency. Poultry Science. 98(8):3246-3256.
- Adentunji, M., Lamont, S.J., Abasht, B.A., and Schmidt, C. J. 2019. Variant analysis pipeline for accurate detection of genomic variants from transcriptome sequencing data. PLOS ONE 14(9): e0216838.doi.org/10.1371/journal.pone.0216838
- Adetunji MO, Lamont SJ, Abasht B, Schmidt CJ. 2019. Variant analysis pipeline for accurate detection of genomic variants from transcriptome sequencing data. PLoS One. 14(9):e0216838.
- Adetunji, M.O., Lamont, S.J., and Schmidt, C.J. 2018. TransAtlasDB: an integrated database connecting expression data, metadata and variants. Database 2018:1-15. doi: 10.1093/database/bay014
- Adoligbe, C., Fernandes, A., Osei-Amponsah, R., Adje, N. D., Gbedevi, R., Fonton, M.-C., Rosa, G. J. M. and Souaibou, F. S. Native chicken farming: A tool for wealth creation and food security in Benin. International Journal of Livestock Production 11(4): 146-162, 2020.
- Aggrey, S. E., F. González-Cerón, R. Rekaya and Y. Mercier, 2018. Gene expression differences in the methionine remethylation and transsulfuration pathways under methionine restriction and recovery with D, L-methionine or D,L-HMTBA in meat-type chickens. Journal of Animal Physiology and Nutrition 102: e468-e475.
- Aggrey, S.E., M. C. Milfort, A. L. Fuller, Y. Jiamin and R. Rekaya, 2019. Effect of host genotype and Eimeria acervulina infection on the metabolome of meat-type chickens. PLoS One 14(10):e0223417. DOI: 10.1371/journal.pone.0223417
- Aguanta, B.N., A. L. Fuller, M. C. Milfort, S. M. Williams, R. Rekaya and S. E. Aggrey, 2018. Histological effects of concurrent heat stress and coccidial infection on the lymphoid tissue of broiler chickens. Animal Diseases 62: 345-350 https://doi.org/10.1637/11907-052818-Reg.1
- Aguirre ME, Leyva-Jimenez H, Travis R, Lee J, Athrey G & Alvarado CZ (2020) Evaluation of growth production factors as predictors of the incidence and severity of white striping and woody breast in broiler chickens. Poult Sci.
- Al-Ajeeli MN, Hubert SM, Leyva-Jimenez H, Hashim MM, Abdaljaleel RA, Alsadwi AM, Athrey G & Bailey CA (2020) Impacts of Dietary Protein and Prebiotic Inclusion on Liver and Spleen Gene Expression in Hy-Line Brown Caged Layers. Animals (Basel) 10.
- Alber, A., Morris, K.M., Bryson, K.J., Sutton, K., Monson, M.S., Chintoan-Uta, C., Borowska, D., Lamont, S.J., Schouler, C., Kaiser, P., Stevens, M., Vervelde, L. 2020. Avian pathogenic Escherichia coli (APEC) strain-dependent immunomodulation of respiratory granulocytes and mononuclear phagocytes in CSF1R-reporter transgenic chickens. Frontiers Immunol. doi: 10.3389/fimmu.2019.03055
- Alfieri, J. M., G. Wang, M. M. Jonika, C. A. Gill, H. Blackmon, and G. N. Athrey. 2022. A Primer for Single-Cell Sequencing in Non-Model Organisms. Genes (Basel) 13.
- Aljarbou WA, England EM, Velleman SG, Reed KM, and Strasburg GM. 2021. Phosphorylation state of pyruvate dehydrogenase and metabolite levels in turkey skeletal muscle in normal and pale, soft, exudative meats. British J Poultry Science, 8:1-8. doi: 10.1080/00071668.2020.1855629. Epub ahead of print. PMID: 33225715.
- Alrubaye, A. A. K., N. S. Ekesi, A. Hasan, E. Elkins, S. Ojha, S. Zaki, S. Dridi, R. F. Wideman, M. A. Rebollo and D. D. Rhoads (2020). Chondronecrosis with Osteomyelitis in Broilers: Further Defining Lameness-Inducing Models with Wire or Litter Flooring, to Evaluate Protection with Organic Trace Minerals. Poultry Science 99(11): 5422-5429.
- Alrubaye, A., N. S. Ekesi, A. Hasan, D. A. Koltes, R. Wideman Jr and D. Rhoads (2020). Chondronecrosis with osteomyelitis in broilers: Further defining a bacterial challenge model using standard litter flooring and protection with probiotics. Poultry Science 99 (12): 6474-6480.
- Aston E.J., Wang, Y., Tracy, K.E., Gallardo, R.A., Lamont, S.J., Zhou, H. 2021. Comparison of cellular immune responses to avian influenza virus in two genetically distinct, highly inbred chicken lines. Vet. Immunol. Immunopathol. 235: 110233. doi.org/10.1016/j.vetimm.2021.110233
- Athrey G (2020) Poultry genetics and breeding. In Animal Agriculture pp. 317-330. Elsevier.
- Athrey G, Cosme LV, Popkin-Hall Z, Pathikonda S, Takken W & Slotman MA (2017) Chemosensory gene expression in olfactory organs of the anthropophilic Anopheles coluzzii and zoophilic Anopheles quadriannulatus. BMC Genomics 18, 751.
- Athrey G, Faust N, Hieke A-SC & Brisbin IL (2018) Effective population sizes and adaptive genetic variation in a captive bird population. PeerJ 6, e5803.
- Athrey G, Popkin-Hall Z, Cosme LV, Takken W & Slotman MA (2020) Species and sex-specific chemosensory gene expression in Anopheles coluzzii and An. quadriannulatus antennae. Parasit Vectors 13, 212.
- Athrey G, Popkin-Hall ZR, Takken W & Slotman MA (2021) The Expression of Chemosensory Genes in Male Maxillary Palps of Anopheles coluzzii (Diptera: Culicidae) and An. quadriannulatus. J Med Entomol.
- Athrey, G., Z. Popkin-Hall, L. V. Cosme, W. Takken, and M. A. Slotman. 2020. Species and sex-specific chemosensory gene expression in Anopheles coluzzii and An. quadriannulatus antennae. Parasit. Vectors 13:212.
- Ayoola MB, Pillai N, Nanduri B, Rothrock MJ, Ramkumar M. Preharvest environmental and management drivers of multidrug resistance in major bacterial zoonotic pathogens in pastured poultry flocks. Microorganisms 2022, 10(9), 1703; https://doi.org/10.3390/microorganisms10091703.
- Bai H, He Y, Ding Y, Chang S, Zhang H, Chen J, *Song J. Parent-of-origin has no detectable effect on survival days of Marek's disease virus infected White Leghorns. Poult Sci. 2019 May 10. pii: pez209. doi: 10.3382/ps/pez209.
- Bai H, He Y, Ding Y, Chu Q, Lian L, Heifetz EM, Yang N, Cheng HH, Zhang H, Chen J, *Song J. Genome-wide characterization of copy number variations in the host genome in genetic resistance to Marek's disease using next generation sequencing. BMC Genet. 2020 Jul 16;21(1):77. doi: 10.1186/s12863-020-00884-w.
- Bai Y, Yuan, P., Zhang, H. Ramachandran, Yang, N. *Song J. Adiponectin and its receptor genes expression in response to MDV infection of White Leghorns. Poultry Science. DOI: 10.1016/j.psj.2020.06.004
- Bai, H., He, Y., Ding, Y., Carrillo, J.A., Selvaraj, R.K., Zhang, H., Chen, J., Song, J. 2019. Allele-specific expression of CD4+ T cells in response to Marek's disease virus infection. Genes. 10(9):718. https://doi.org/10.3390/genes10090718.
- Bai, H., He, Y., Ding, Y., Chang, S., Zhang, H., Chen, J., Song, J. 2019. Grandparental lineage in a reciprocal cross showed no detectable effect on survival days of F2 White Leghorns in response to a very virulent plus Marek's disease virus challenge. Poultry Science. 98.10: 4498-4503. https://doi.org/10.3382/ps/pez209.
- Bai, H., He, Y., Ding, Y., Chu, Q., Lian, L., Heifetz, E.M., Yang, N., Cheng, H.H., Zhang, H., Chen, J., Song, J. 2020. Genome-wide characterization of copy number variations in the host genome in genetic resistance to Marek's disease using next generation sequencing. BMC Genetics. 21:77. https://doi.org/10.1186/s12863-020-00884-w
- Bai, H.; He, Y.; Ding, Y.; Carrillo, J.A.; Selvaraj, R.K.; Zhang, H.; Chen, J.; Song, J. Allele-Specific Expression of CD4+ T Cells in Response to Marek's Disease Virus Infection. Genes 2019, 10, 718.
- Barnes NE, Strasburg GM, Velleman SG, and Reed KM. 2019. Thermal challenge alters the transcriptional profile of the breast muscle in turkey poults. Poultry Science, 98:74-91. doi: 10.3382/ps/pey401.
- Barrett, N.W., Schmidt, C.J., Lamont, S.J., Ashwell, C.M., Persia, M.E. 2019. Effects of acute and chronic heat stress on the performance, egg quality, body temperature and blood gas parameters of laying hens. Poultry Science. http://dx.doi.org/10.3382/ps/pez541
- Barrington WT, Wulfridge P, Wells AE, Rojas CM, Howe SYF, Perry A, Hua K, Pellizzon MA, Hansen KD, Voy BH, Bennett BJ, Pomp D, Feinberg AP, Threadgill DW. Improving Metabolic Health Through Precision Dietetics in Mice. Genetics. 2018 Jan;208(1):399-417. doi: 10.1534/genetics.117.300536. Epub 2017 Nov 20. PMID: 29158425; PMCID: PMC5753872.
- Baxter M.F.A., Greene E.S., Kidd M.T., Tellez-Isaias G., Orlowski S., Dridi S (2020). Water amino acid-chelated trace mineral supplementation decreases circulating and intestinal HSP70 and proinflammatory cytokine gene expression in heat-stressed broiler chickens. J Anim Sci. Mar 1;98(3). pii: skaa049. doi: 10.1093/jas/skaa049.
- Botchway, P.K., Amuzu-Aweh, E.N., Naazie, A., Aning, G. K., Otsyina, H.R., Saelao, P., Wang, Y., Zhou, H., Walugembe, M., Dekkers, J., Lamont, S.J., Gallardo, R.A., Kelly, T.R., Bunn, D. and Kayang, B.B. (accepted 8/2022) Host response to successive challenges with lentogenic and velogenic Newcastle disease virus in local chickens of Ghana. Poultry Science
- Bottje W, Lassiter K, Kuttappan V, Hudson N, Owens C, Abasht B, Dridi S, Kong B. 2021. Upstream regulator analysis of wooden breast myopathy proteomics in commercial broilers and comparison to feed efficiency proteomics in pedigree male broilers. Foods. 10(1):104. doi: 10.3390/foods10010104.
- Bowman, A., C. Fitzgerald, J.F. Pummill, D. Rhoads, T. Yamashita (2021) Reduced toxicity of Centruroides vittatus (Say, 1821) scorpion may result from lowered sodium toxin gene expression and toxin protein production. Toxins (in press).
- Brady K, Liu HC, Hicks JA, Long JA, Porter TE (2020) Transcriptome analysis of the hypothalamus and pituitary of turkey hens with low and high egg production. BMC Genomics 21(1):647. doi: 10.1186/s12864-020-07075-y
- Brady K, Liu HC, Hicks JA, Long JA, Porter TE (2021) Transcriptome Analysis During Follicle Development in Turkey Hens With Low and High Egg Production. Front Genetics 12: 619196. doi: 10.3389/fgene.2021.619196.
- Brady K, Long JA, Liu HC, Porter TE (2020) Differences in in vitro responses of the hypothalamo-pituitary-gonadal hormonal axis between low and high egg producing turkey hens. Poult Sci. 99:6221-6232 doi: 10.1016/j.psj.2020.08.048.
- Brady K, Long JA, Liu HC, Porter TE (2021) Characterization of hypothalamo-pituitary-thyroid axis gene expression in the hypothalamus, pituitary gland, and ovarian follicles of turkey hens during the preovulatory surge and in hens with low and high egg production. Poult Sci 100:100928. doi: 10.1016/j.psj.2020.12.026.
- Brady K, Porter TE, Liu HC, Long JA (2019) Characterization of gene expression in the hypothalamo-pituitary-gonadal axis during the preovulatory surge in the turkey hen. Poult Sci. 98(12):7041-7049. doi: 10.3382/ps/pez437.
- Brady K, Porter TE, Liu HC, Long JA (2020) Characterization of the hypothalamo-pituitary-gonadal axis in low and high egg producing turkey hens. Poult Sci. 99(2):1163-1173. doi: 10.1016/j.psj.2019.12.028.
- Brothers B, Zhuo Z, Papah MB, Abasht B*. 2019. RNA-Seq Analysis Reveals Spatial and Sex Differences in Pectoralis Major Muscle of Broiler Chickens Contributing to Difference in Susceptibility to Wooden Breast Disease. Frontiers in Physiology. 10:764.
- Bucher, M. G., B. Zwirzitz, A. Oladeinde, K. Cook, C. Pymel, G. Zock, S. E. Aggrey, C. Ritz, T. Looft, E. Lipp, G. E. Agga, and K. Sistani, 2020. Reuse poultry litter microbiome with competitive exclusion potential against Samonella Heidelberg. Journal of Environmental Quality 2020; 1-13. https://doi.org/10.1002/jeq2.20081.
- K. Tudeka, G. K. Aning, A. Naazie, P. K. Botchway, E. N. Amuzu?Aweh. G. K. Agbenyegah, B. Enyetornye, D. Fiadzomor, P. Saelao, Y. Wang, T. R. Kelly, R. Gallardo, J. C. M. Dekkers, . J. Lamont, H. Zhou, B. B. Kayang. 2022. Response of three local chicken ecotypes of Ghana to lentogenic and velogenic Newcastle disease virus challenge. Tropical Animal Health and Production 54:134 https://doi.org/10.1007/s11250-022-03124-8
- Cauble R., Greene e., Orlowski S., Walk C., Bedford M., Apple J., Kidd M., Dridi S (2020). Research Note: Dietary phytase reduces broiler woody breast severity via potential modulation of breast muscle fatty acid profiles. Poult Sci 99:4009-4015.
- Chang L.Y., Toghiani S, S.E. Aggrey, Rekaya R, 2019. Increasing accuracy of genomic selection in presence of high density marker panels through the prioritization of relevant polymorphisms. BMC Genet. 2019 Feb 22;20(1):21. doi: 10.1186/s12863-019-0720-5.
- Chang, L.-Y. S. Toghiani, A. Ling, S. E. Aggrey, and R. Rekaya, 2018. High density marker parnels, SNPs prioritizing and accuracy of genomic selection. BMC Genetics 19 (1) DOI 10.1186/s12863-017-0595-2
- Chang, L.-Y., S. Toghiani, A. Ling, E. H. Hay, S. E. Aggrey and R. Rekaya, 2017. Analysis of multiple binary responses using a threshold model. J. Agric. Biol. And Environ. Stat. 22: 640-651 DOI: 10.1007/s13253-017-0305-6.
- Chang, L.-Y., S. Toghiani, E. H. Hay, S. E. Aggrey, and R. Rekaya, 2019. A weighted genomic relationship matrix based on Fst prioritized SNPs for genome selection. Genes. Genes 10(11) DOI: 10.3390/genes10110922
- Chanthavixay, G., Kern, C., Wang, Y., Saelao, P., Lamont, S.J., Gallardo, R.A., Rincon, G., Zhou, H. 2020. Integrated transcriptome and histone modification analysis reveals NDV infection under heat stress affects bursa development and proliferation in susceptible chicken line. Front. Genet. 11:567812. doi: 10.3389/fgene.2020.567812
- Chen H, Jin, K., Song J, Zuo Q, Yang H, Zhang Y, Li B. 2018 Functional characterization of the Sox2 , c-Myc , and Oct4 promoters. Journal of Cellular Biochemistry. DOI: 10.1002/jcb.27374
- Chen, W., Liu, Y., Li, A., Li, X., Li, H., Dai, Z., Yan, Y., Zhang, X., Shu, D., Zhang, H., Lin, W., Ma, J., Xie, Q. 2017. A premature stop codon within the tvb receptor gene results in decreased susceptibility to infection by avian leukosis virus subgroups B, D, and E. Oncotarget. 8(62):105942-105956.
- Cheng S, Wang M, Wang Y, Zhang C, Wang Y, Song J, Zuo Q, Zhang Y, Li B. RXRG associated in PPAR signal regulated the differentiation of primordial germ cell. J Cell Biochem. 2018 May 8. doi: 10.1002/jcb.26891.
- Cheng, H.H., Warren, W.C., Zhou, H. 2021. Avian genomics. Book Chapter. In: Scanes, Collin G., Dridi, Sami, editors. Sturkie's Avian Physiology. 7th edition. San Diego, CA: Academic Press. p. 7-16. https://doi.org/10.1016/B978-0-12-819770-7.00047-5.
- Chu, Q., Ding, Y., Cai, W., Liu, L., Zhang, H., Song, J. 2019. Marek's disease virus infection induced mitochondria changes in chickens. International Journal of Molecular Sciences. 20(13): 3150. https://doi.org/10.3390/ijms20133150.
- Cogburn LA, Trakooljul N, Wang X, Ellestad LE, Porter TE (2020) Transcriptome analyses of liver in newly-hatched chicks during the metabolic perturbation of fasting and re-feeding reveals THRSPA as the key lipogenic transcription factor. BMC Genomics. 21(1):109. doi: 10.1186/s12864-020-6525-0.
- Collisson E, Griggs L, Drechsler Y. Macrophages from disease resistant B2 haplotype chickens activate T lymphocytes more effectively than macrophages from disease susceptible B19 birds. Dev Comp Immunol. 2017 Feb;67:249-256.
- Da Silva A. P. Hauck R, Zhou H, Gallardo RA. 2017. Understanding immune resistance to infectious Bronchitis using major histocompatibility complex chicken lines. Avian Diseases 61(3):358-365. doi: 10.1637/11666-050117
- Deist, Herrmann, R. Gallardo, D. Bunn, J. Dekkers, H. Zhou, S. Lamont. 2018. Novel analysis of the Harderian gland transcriptome response to Newcastle disease virus in two inbred chicken lines. Scientific Reports. 8(1):6558. doi: 10.1038/s41598-018-24830-0.
- Deist, Herrmann, R. Gallardo, D. Bunn, J. Dekkers, H. Zhou, S. Lamont. 2017. Resistant and susceptible chicken lines show distinctive responses to Newcastle disease virus infection in the lung transcriptome. BMC Genomics201718:989.
- Deist, Herrmann, R. Gallardo, D. Bunn, T. Kelly, J. Dekkers, H. Zhou, S. Lamont. 2017. Novel mechanisms revealed in the trachea transcriptome of resistant and susceptible chicken lines following infection with Newcastle disease virus. Clinical and Vaccine Immunology 24(5). pii: e00027-17. doi: 10.1128/CVI.00027-17.
- Deist, Herrmann, R. Gallardo, J. Dekkers, H. Zhou, S. Lamont. 2020. Novel combined tissue transcriptome analysis after lentogenic Newcastle disease virus challenge in inbred chicken lines of differential resistance. Frontiers in Genetics doi.org/10.3389/fgene.2020.00011.
- Deist, M.S., and Lamont, S.J. 2018. What makes the Harderian gland transcriptome different from other chicken immune tissues? A gene expression comparative analysis. Frontiers Physiol. 9:492. doi: 10.3389/fphys.2018.00492
- Del Vesco A., M. Monson. K. M. Kaiser, H. Zhou, S. Lamont. 2020. Genetic responses of inbred chicken lines illustrate importance of eIF2 family and immune-related genes in resistance to Newcastle disease virus. Sci Rep 10, 6155 (2020). https://doi.org/10.1038/s41598-020-63074-9.
- Del Vesco, A.P., Jang, H.J., Monson, M.S., and Lamont, S.J. 2021. Role of chicken oligoadenylate synthase like gene during in vitro Newcastle disease virus infection. Poultry Sci. doi.org/10.1016/j.psj.2021.101067
- Deng, C., Tan, H., Zhou, H., Wang, M., Lu, Y., Xu, J., Zhang, H., Han, L., Ai, Y. 2019. Four cysteine residues contribute to homodimerization of chicken interleukin-2. International Journal of Molecular Sciences. 20(22):5744. https://www.doi.org/10.3390/ijms20225744.
- Dhamad A.E., Greene E., Sales M., Nguyen P., Beer L., Liyanage R., Dridi S (2020). 75-kDa glucose-regulated protein (GRP75) is a novel molecular signature for heat stress response in avian species. Am J Physiol Cell Physiol. 2020 Feb 1;318(2):C289-C303.
- Dong, K., Chang, S., Xie, Q., Black Pyrkosz, A.A., Zhang, H. 2017. Comparative transcriptomics of genetically divergent lines of chickens in response to Marek's disease virus challenge at cytolytic phase. PLoS One. 12(6):e0178923. https://doi.org/10.1371/journal.pone.0178923.
- Dong, K., Chang, S., Xie, Q., Zhao, P., Zhang, H. 2019. RNA Sequencing revealed differentially expressed genes functionally associated with immunity and tumor suppression during latent phase infection of a vv+MDV in chickens. Scientific Reports. 9:14182. https://doi.org/10.1038/s41598-019-50561-x.
- Dong, K., Heidari, M., Mays, J.K., Chang, S., Xie, Q., Zhang, L., Ai, Y., Zhang, H. 2022. LncRNA signature of avian lymphoid leukosis-like lymphomas differed from normal controls. PLoS ONE. https://doi.org/10.1016/j.micpath.2022.105688.
- Drobik-Czwarno, W., Wolc, A., Fulton, J.E. and Dekkers, J.C., 2018. Detection of copy number variations in brown and white layers based on genotyping panels with different densities. Genetics Selection Evolution, 50(1), p.54. doi.org/10.1186/s12711-018-0428-4
- Drobik-Czwarno, W., Wolc, A., Fulton, J.E., Arango, J., Jankowski, T., O'Sullivan, N.P. and Dekkers, J.C.M., 2018. Identifying the genetic basis for resistance to avian influenza in commercial egg layer chickens. Animal, 12(7), pp.1363-1371. DOI:10.1017/S1751731117002889
- Drobik-Czwarno, W., Wolc, A., Fulton, J.E., Jankowski, T., Arango, J., O'Sullivan, N.P. and Dekkers, J., 2018. Genetic basis of resistance to avian influenza in different commercial varieties of layer chickens. Poultry Science 97:3421-3428. doi: 10.3382/ps/pey233.
- Drobik-Czwarno, W., Wolc, A., Kucharska, K.,Martyniuk. E. 2019. Genetic basis of resistance to highly pathogenic avian influenza in chicken. Review article in Polish. Scientific Annals of Polish Society of Animal Production. doi.org/10.3382/ps/pey233
- Dunkley, C. and S. E. Aggrey, 2020. The amino acid composition of organic soybean meal for the formulation of organic poultry feed. http://eorganic.ord/node/25491
- EBERLE, K.N., MARTIN, M.P., SHAH, S., MALHEIROS, R.D., LIVINGSTON, K.A., ANDERSON, KE. A novel non-invasive method for evaluating electroencephalograms on laying hens. Poultry Science, Volume 97, Issue 3, 1 March 2018, Pages 860-864
- EBERLE-KRISH, K.N., MARTIN, M.P. MALHEIROS, R.D., SHAH, S.B., LIVINGSTON, .A., ANDERSON, K.E. Evaluation of Ventilation Shutdown in a Multi-level Caged System. J. Appl. Poult. Res. (2018) v.27: 555-563.
- Ekesi, N.S., A. Hasan, A. Parveen, A. Shwani, D. Rhoads (2021). Embryo lethality assay for evaluating virulence of isolates from bacterial chondronecrosis with osteomyelitis in broilers. Poultry Science 100:101455.
- Ekesi, N.S., B. Dolka, A. Alrubaye, D. Rhoads (2021). Analysis of genomes of bacterial isolates from lameness outbreaks in broilers. Poultry Science: 100(7): 101148
- El Sabry, M. I., M. H. Abdelfattah, H. A. Abdellatif, S. E. Aggrey, and S. Elnesr, 2020. Physiochemical properties of magnetic water and its effect on egg production traits in hens at late laying period. J. Animal and Plant Science https://doi.org/10.36899/JAPS.2021.1.0219
- Elbeltagy, A.R., Bertolini, F., Fleming, D.S., Van Goor, A., Ashwell, C.M., Schmidt, C.J., Kugonza, D., Lamont, S.J., Rothschild, M.F. 2019. Natural selection footprints among African chicken breeds and village ecotypes. Front. Genet. 10:376. doi: 10.3389/fgene.2019.00376
- Ellestad LE, Cogburn LA, Simon J, Le Bihan-Duval E, Aggrey SE, Byerly MS, Duclos MJ, Porter TE (2019) Transcriptional profiling and pathway analysis reveal differences in pituitary gland function, morphology, and vascularization in chickens genetically selected for high or low body weight. BMC Genomics 20(1):316. doi: 10.1186/s12864-019-5670-9.
- Emami NK, Jung U, Voy B, Dridi S. Radical Response: Effects of Heat Stress-Induced Oxidative Stress on Lipid Metabolism in the Avian Liver. Antioxidants (Basel). 2020 Dec 30;10(1):35. doi: 10.3390/antiox10010035. PMID: 33396952; PMCID: PMC7823512.
- Evenson JK, Sunde RA. Metabolism of tracer 75Se selenium from inorganic and organic selenocompounds into selenoproteins in rats, and the missing 75Se metabolites. Front Nutr 2021;8:699652. PMID: 34322513
- Fagundes, N. S., M. C. Milfort, S. M. Williams, M. J. Da Costa, A. L. Fuller, J. F. Menten, R. Rekaya and S. E. Aggrey, 2020. Dietary methionine level alters growth, digestibility, and gene expression of amino acid transporters in meat-type chickens. Poultry Sci. 99: 67-75.
- FERKET, P.R., MALHEIROS, R.D., MORAES, V.M.B., AYOOLA, A.A., BARASCH, I., TOOMER, OT., TORRENT. J. Effects of functional oils on the growth, carcass and meat characteristics, and intestinal morphology of commercial turkey toms. 2020 Poultry Science, 99:3752-3760, doi.org 10.1016
- Ferver A., Dridi S (2020). Regulation of avian uncoupling protein (av-UCP) expression by cytokines and hormonal signals in quail myoblast cells. Comp Biochem Physiol A Mol Integr Physiol. DOI: 10.1016/j.cbpa.2020.110747
- Flees J., Greene E., Ganguly B., Dridi S (2020). Phytogenic feed- and water-additives improve feed efficiency in broilers via modulation of (an)orexigenic hypothalamic neuropeptide expression. Neuropeptides. 2020 Jan 3:102005. doi: 10.1016/j.npep.2020.102005. [Epub ahead of print]
- Foutz, J.C., M. C. Milfort, A. L. Fuller, W. K. Kim, R. Rekaya, and S. E. Aggrey, 2020. Supplementation of diets with Brazil nut powder can meet dietary methionine requirement of organic broiler chickens. Organic Agriculture. DOI: 10.1007/s13165-019-00276-0.
- Ghareeb AFA, Schneiders GH, Foutz JC, Milfort MC, Fuller AL, Yuan J, Rekaya R, Aggrey SE. Heat Stress Alters the Effect of Eimeria maxima Infection on Ileal Amino Acids Digestibility and Transporters Expression in Meat-Type Chickens. Animals (Basel). 2022 Jun 16;12(12):1554. doi: 10.3390/ani12121554.
- Ghareeb AFA, Schneiders GH, Richter JN, Foutz JC, Milfort MC, Fuller AL, Yuan J, Rekaya R, Aggrey SE. Heat stress modulates the disruptive effects of Eimeria maxima infection on the ileum nutrient digestibility, molecular transporters, and tissue morphology in meat-type chickens. PLoS One. 2022 Jun 3;17(6):e0269131. doi: 10.1371/journal.pone.0269131.
- Giuffra E, Tuggle CK; Archibald AL, Acloque H, Chamberlain AJ, Cochrane G, Daetwyler HD, Djebali S, Eory L, Foissac S, Goddard ME, Groenen MA, Halstead M, Harrison PW, Hayes BJ, Kern C, Khatib H, Kuo RI, MacHugh DE, Macleod IM, Madsen O, Reecy JM, Ross PJ, Watson M, Wells JM, Zhou H. 2019. Functional Annotation of Animal Genomes (FAANG): Current Achievements and Roadmap. Annu Rev Anim Biosci. 7:65-88. doi: 10.1146/annurev-animal-020518-114913.
- GIVISIEZ, P.E.N., ALEXANDRE L. B. MOREIRA FILHO,Y MAYLANE R. B. SANTOS, HERALDO B. OLIVEIRA, PETER R. FERKET, CELSO J. B. OLIVEIRA, AND RAMON D. MALHEIROS. Chicken embryo development: metabolic and morphological basis for in ovo feeding technology. 2020 Poultry Science, 99:6774-6782 doi.org 10.1016.
- Glass, M.C., Smith, J.M., Cheng, H.H., Delany, M.E. 2021. Marek's disease virus telomeric integration profiles of neoplastic host tissues reveal unbiased chromosomal selection and loss of cellular diversity during tumorigenesis. Genes. 12(10):1630. https://doi.org/10.3390/genes12101630.
- Glenn, T.C, R.A Nilsen, T.J. Kieran, J.W. Finger, T.W. Pierson, K.E. Bentley, S.L. Hoffberg, S. Louha, F.J. Garcia-De Leoen, M.A.D.R. Portilla, K.D. Reed, J.L. Anderson, J.K. Meece, S.E. Aggrey, R. Rekaya, M. Alabady, M. Belanger, K. Winker and B.C. Faircloth, 2017. Adapterama I: Universal stubs and primers for thousands of dual-indexed Illumina libraries 1 (iTru & iNext). doi: https://doi.org/10.1101/049114
- Gopalan L, Sebastian, A, Praul C, Istvan A, Ramachandran R (2021) Metformin affects the transcriptomic profile of ovarian cancer cells. Genes, 13:30
- Goto, T., Fernandes, A. F. A., Tsudzuki, M. and Rosa, G. J. M. Causal phenotypic networks for egg traits in an F2 chicken population. Molecular Genetics and Genomics 294: 1455-1462, 2019.
- Greene E., Cauble R., Dhamad A., Kidd M., Kong B., Howard S., Castro H., Campagna S., Bedford M., Dridi S (2020). Muscle Metabolome Profiles in Woody Breast-(un)Affected Broilers: Effects of Quantum Blue Phytase-Enriched Diet. Front Vet Sci 2020 (https://doi.org/10.3389/fvets.2020.00458)
- Greene E., Cauble R., Kadhim H., Mallmann B., Gu I., Lee S.O., Orlowski S., Sami Dridi (2020). Protective effects of the phytogenic feed additive "comfort" on growth performance via modulation of hypothalamic feeding- and drinking-related neuropeptides in cyclic heat-stressed broilers. Domest Anim Endocrinol. doi: 10.1016/j.domaniend.2020.106487. Epub 2020 Apr 18.
- Greene E.S., Zampiga M., Sirri F., Ohkubo T., Dridi S (2020). Orexin system is expressed in avian liver and regulates hepatic lipogenesis via ERK1/2 activation. Sci Rep 2020 Nov 5;10(1):19191. doi: 10.1038/s41598-020-76329-2.
- Guo, Y., L. Chai, S. E. Aggrey, A. Oladeinde, J. Johnson and G. Zock, 2000. A machine vision-based method for monitoring broiler chicken floor distribution. Sensors 20: 3179. doi:10.3390/s20113179.
- Guo, Y., S. E. Aggrey, A. Oladeinde, J. Johnson, G. Zock and L. Chai, 2021. A machine vision-based method optimized for restoring broiler chicken images occluded by feeding and drinking equipment. Animals (Basel). 2021 Jan 8;11(1):123. doi: 10.3390/ani11010123.
- Habashy, W. S., M. C. Milfort, K. Adomako, Y. A. Attia, R. Rekaya and S. E. Aggrey, 2017. Effect of Heat Stress on Amino Acid Digestibility and Transporters in meat-type Chickens. Poultry Science. 96: 2312-2319.
- Habashy, W., M. C. Milfort, A. L. Fuller, Y. A. Attia, R. Rekaya and S. E. Aggrey, 2017. Effect of heat stress on protein utilization and nutrient transporters in meat-type chickens. International Journal of Biometerology 61: 2111-2118.
- Habashy, W., M. C. Milfort, R. Rekaya and S. E. Aggrey, 2018.Expression of genes that encodes cellular oxidant/antioxidant enzyme are affected by heat stress in meat-type chickens. Molecular Biology Reports 45(3):389-394. doi: 10.1007/s11033-018-4173-0
- Habashy, W., M. C. Milfort, R. Rekaya and S. E. Aggrey, 2021. Molecular and cellular responses of DNA methylation and thioredoxin systems to heat stress in meat-type chickens. Animal 11(7), 1957; https://doi.org/10.3390/ani11071957.
- Habashy, W.S., M. C. Milfort, R. Rekaya and S. E. Aggrey, 2019. Cellular antioxidant enzyme activity and biomarkers for oxidative stress are affected by heat stress. Int. Journal of Biometeorology. doi: 10.1007/s00484-019-01769-z.
- Hadley JA, Ocon-Grove OM, Ramachandran R (2020) Adiponectin is secreted by theca layer cells isolated from chicken ovarian follicles. Reproduction, 159:275-288
- Hanlon C, Ramachandran R, Zuidhof MJ, Bedecarrats GY (2020). Should I lay or should I grow? Photoperiodic versus metabolic cues in chickens. Front Physiol. 11:707
- HARDING, K.L.; VU, T.; WYSOCKY, R.; MALHEIROS, R.; ANDERSON, K.E.; TOOMER, O.T. The Effects of Feeding a Whole-in-Shell Peanut-Containing Diet on Layer Performance and the Quality and Chemistry of Eggs Produced. Agriculture 2021, 11, 1176. doi.org/10.3390/agriculture11111176
- Hauser, S. S., G. Athrey, and P. L. Leberg. 2021. Waste not, want not: Microsatellites remain an economical and informative technology for conservation genetics. Ecol. Evol. 11:15800-15814.
- He Y, Han B, Ding Y, Zhang H, Chang S, Zhang L, Zhao C, Yang N, *Song J. Linc-GALMD1 Regulates Viral Gene Expression in the Chicken. Front Genet. 2019 Nov 14;10:1122. doi: 10.3389/fgene.2019.01122. eCollection 2019.
- He Y, Zuo Q, Edwards J, Zhao K, Lei J, Cai W, Nie Q, Li B, Song J. DNA Methylation and Regulatory Elements during Chicken Germline Stem Cell Differentiation. Stem Cell Reports. 2018 Apr 17. pii: S2213-6711(18)30145-0. doi: 10.1016/j.stemcr.2018.03.018.
- HEFLIN, L.E., MALHEIROS, R.D., ANDERSON, K.E., JOHNSON, L, K., RAATZ, S. Mineral content of eggs differs with hen strain, age, and rearing environment. Poultry Science (2018) v.97:1605-1613.
- Heidari, M., Zhang, L., Zhang, H. 2020. MicroRNA profiling in the bursae of MDV-infected resistant and susceptible chicken lines. Genomics. 112:2564-2571. https://doi.org/10.1016/j.ygeno.2020.02.009.
- Hicks JA, Porter TE, Liu HC (2017) Identification of microRNAs controlling hepatic mRNA levels for metabolic genes during the metabolic transition from embryonic to posthatch development in the chicken. BMC Genomics 18:687.
- Hicks JA, Porter TE, Sunny N, Liu HC (2019) Delayed feeding alters transcriptional and post-transcriptional regulation of hepatic metabolic pathways in peri-hatch broiler chicks. Genes 10(4). pii: E272. doi: 10.3390/genes10040272.
- Hieke A-SC, Hubert SM & Athrey G (2019) Circadian disruption and divergent microbiota acquisition under extended photoperiod regimens in chicken. PeerJ 7, e6592.
- Hoencamp, C., Dudchenko, O., Elbatsh, A.M., Brahmachari, S., Raaijmakers, J.A., Van Schaik, T., Sedeño Cacciatore, A., Contessoto, V., Hildebrandt, E.C., Cheng, H.H., Lieberman Aiden, E., Rowland, B.D. 2021. 3D genomics across the tree of life reveals condensin II as a determinant of architecture type. Science. 372(6545):984-989. https://doi.org/10.1126/science.abe2218.
- Horton KA, Sporer KRB, Tempelman RJ, Malila Y, Reed KM, Velleman SG, and Strasburg GM. 2020. Knockdown of death-associated protein expression induces global transcriptome changes in proliferating and differentiating muscle satellite cells. Frontiers in Physiology 11:1036. doi: 10.3389/fphys.2020.01036.
- Hou Z, Wang Z, Yin ZT, Zhang F, Li XQ, Chen SR, Yang N, Porter TE (2019) Dynamics of transcriptome changes during subcutaneous preadipocytes differentiation in ducks. BMC Genomics 20:688. doi: 10.1186/s12864-019-6055-9.
- Hubbard, A. H., Zhang, X., Singh, A., Jastrebski, S., Lamont, S.J., and Schmidt, C. 2018. Identifying mechanisms of regulation to model carbon flux during heat stress and generate testable hypotheses. PLOS ONE 13(10): e0205824. https:// doi.org/10.1371/journal.pone.0205824
- Hubert SM & Athrey G (2020) Energy metabolism and sources of oxidative stress in wooden breast - a review. F1000Res 9, 319.
- Hubert SM, Al-Ajeeli M, Bailey CA & Athrey G (2019) The role of housing environment and dietary protein source on the gut microbiota of chicken. Animals (Basel) 9.
- Hubert SM, Williams TJ & Athrey G (2018) Insights into the molecular basis of wooden breast based on comparative analysis of fast- and slow-growth broilers. BioRxiv.
- Hubert, S., and G. Athrey. 2022. Transcriptomic signals of mitochondrial dysfunction and OXPHOS dynamics in fast-growth chicken. PeerJ 10:e13364.
- Ibrahim, M. M. A., J. R. Nelson, G. S. Archer, and G. Athrey. 2021. Effects of monochromatic lighting during incubation and vaccination on the splenic transcriptome profiles of chicken. Front. Genet. 12:628041.
- Irizarry KJL, Downs E, Bryden R, Clark J, Griggs L, Kopulos R, Boettger CM, Carr TJ Jr, Keeler CL, Collisson E, Drechsler Y. RNA sequencing demonstrates large-scale temporal dysregulation of gene expression in stimulated macrophages derived from MHC-defined chicken haplotypes. PLoS One. 2017 Aug 28;12(8)
- Jang, H.-J., Monson, M., Kaiser., M., Lamont, S.J. 2020. Induction of chicken host defense peptides within disease-resistant and -susceptible lines. GENES 11:1195; doi:10.3390/genes11101195
- Jehl F, Degalez F, Bernard M, [and 20 others, including Abasht B]. 2021. RNA-seq data for reliable SNP detection and genotype calling: interest for coding variant characterization and cis-regulation analysis by allele-specific expression in livestock species. Frontiers in Genetics. 12, p.1104.
- Ji, J., Shuang, H., Zhang, H., Li, H., Chang, S., Ma, J., Bi, Y., Xie, Q. 2017. Temporal changes of microRNA gga-let-7b and gga-let-7i expression in chickens challenged with subgroup J avian leukosis virus. Veterinary Research Communications. https://doi.org/10.1007/s11259-017-9681-1.
- Jiang J, Chen C, Cheng S, Yuan X, Jin J, Zhang C, Sun X, Song J, Zuo Q, Zhang Y, Chen G, Li B. Long Noncoding RNA LncPGCR Mediated by TCF7L2 Regulates Primordial Germ Cell Formation in Chickens. Animals (Basel). 2021 Jan 24;11(2):292. doi: 10.3390/ani11020292. PMID: 33498947; PMCID: PMC7912682.
- Jin K, Chen C., Sun, X., Zhu, C., Ahmed, M. Zuo, Q., Song J, Li B. Identification and Generation of Transgenic Mice and Goats with Capra hircus SCD1 Gene. Pakistan Journal of Zoology DOI: 10.17582/journal.pjz/20181013161028
- Jin K, Li D, Jin J, Song J, Zhang Y, Chang G, Chen G, Li B. C1EIP Functions as an Activator of ENO1 to Promote Chicken PGCs Formation via Inhibition of the Notch Signaling Pathway. Front Genet. 2020 Jul 24;11:751. doi: 10.3389/fgene.2020.00751. PMID: 32849782; PMCID: PMC7396672.
- Jin K, Zhou J, Zuo Q, Song J, Zhang Y, Chang G, Chen G, Li B. Transcriptome Sequencing and Comparative Analysis of Amphoteric ESCs and PGCs in Chicken (Gallus gallus). Animals (Basel). 2020 Nov 27;10(12):E2228. doi: 10.3390/ani10122228. PMID: 33261034.
- Jin K, Zuo Q, Song J, Zhang Y, Chen G, Li B. CYP19A1 (aromatase) dominates female gonadal differentiation in chicken (Gallus gallus) embryos sexual differentiation. Biosci Rep. 2020 Oct 30;40(10):BSR20201576. doi: 10.1042/BSR20201576.
- Jing, Y., Yuan, Y., Monson, M. Wang, P., Mu, F., Zhang, Q., Na, W., Zhang, K., Wang, Y., Leng, L., Li, Y., Luan, P., Wang, N., Guo, R., Lamont, S., Li, H., and Yuan, H. 2022. Multi-omics association reveals the effects of intestinal microbiome-host interactions on fat deposition in broiler lines divergently selected for abdominal fat content. Frontiers in Microbiology 12:815538. doi: 10.3389/fmicb.2021.815538
- Johnson, J, B. Zwirzitz, A. Oladeinde, M. Milfort, T. Looft, L. Chai, G. Zock, M. Sommers, S. Tunim and S. E. Aggrey, 2021. Succession patterns of the bacterial community in poultry little after bird removal and sodium bisulfate application. J. Environ. Quality 50(1): https://doi.org/10.1002/jeq2.20248
- JR Mushi, GH Chiwanga, EL Mollel, M Walugembe, RA Max, PM Msoffe, R. Gallardo, T. Kelly, S. Lamont, J. Dekkers, H. Zhou, A. Muhairwa. 2021. Antibody response, viral load, viral clearance and growth rate in Tanzanian free-range local chickens infected with lentogenic Newcastle disease virus. Journal of Veterinary Medicine and Animal Health 13 (2), 98-105
- Jung U, Kim M, Piacquadio K, Shepherd E, Voy BH. Technical note: An optimized method to isolate, purify, and differentiate satellite cells from broiler chicks. J Anim Sci. 2022 Oct 22:skac342. doi: 10.1093/jas/skac342.
- Rowland. Wolc. J. H. Zhou, R. Gallardo, T. Kelly, A. Dekkers, Lamont. S.J. Genome-Wide Association Study of a Commercial Egg Laying Line Challenged with Newcastle Disease Virus. Front. Genet., 20 August 2018 | https://doi.org/10.3389/fgene.2018.00326.
- Kadhim, H.J., S.W. Kang and W.J. Kuenzel. 2021. Possible roles of brain derived neurotrophic factor and corticotropin releasing hormone neurons in the nucleus of hippocampal commissure functioning within the avian neuroendocrine regulation of stress. Stress 24(5):590-601. org/10.1080/10253890.2021.1929163
- Kadhim, H.K., M. Kidd Jr., S.W. Kang and W.J. Kuenzel. (2020). Differential delayed responses of arginine vasotocin and its receptors in septo-hypothalamic brain structures and anterior pituitary that sustain hypothalamic-pituitary-adrenal (HPA) axis functions during acute stress. Gen. Comp. Endocrinol. 286:113302. doi.org/10.1016/j.ygcen.2019.113302.
- Kaiser, M., Hsieh, J., Kaiser, P. and Lamont, S.J. 2022. Differential Immunological Response Detected in mRNA Expression Profiles Among Diverse Chicken Lines in Response to Salmonella Challenge. Poultry Sci. 101: 101605 https://doi.org/10.1016/j.psj.2021.101605
- Kang, S.W., K.D. Christensen, D. Aldridge and W.J. Kuenzel. (2020). Effects of light intensity and dual light intensity choice on plasma corticosterone, central serotonergic and dopaminergic activities in birds, Gallus gallus. Gen. Comp. Endocrinol. 285: 113289. doi.org/10.1016/j.ygcen.2019.113289.
- Kang, S.W., M.T. Kidd Jr., H.J. Kadhim, S. Shouse, S.K. Orlowski, J. Hiltz, N.B. Anthony, W.J. Kuenzel and B.C. Kong. (2020). Characterization of stress response involved in chicken myopathy. Gen. Comp. Endocrinol. 295:113526. doi.org/10.1016/j.ygcen.2020.113526.
- Katarzyna B, Taylor RM, Szpunar J, Lobinski R, Sunde RA. Identification and determination of selenocysteine, selenosugar, and other selenometabolites in turkey liver. Metallomics 2020;12:758-66. PMID: 32211715
- Kern, C. P. P. Saelao, Y. Wang, M. Halstead, J. L. Chitwood, I. Korf, M. Delany, H. Cheng, J. F. Medrano, A. Van Eenennaam, C. W. Ernst. J. Ross, Zhou, H. 2018. Genome-wide identification of tissue-specific long non-coding RNA in three farm animal species. BMC Genomics doi.org/10.1186/s12864-018-5037-7.
- Kern, C., Wang, Y., Chitwood, J., Korf, I., DeLany, M., Cheng, H.H., Medrano, J.F., Van Eenennaam, A.L., Ernst, C., Ross, P., Zhou, H. 2018. Genome-wide identification of tissue-specific long non-coding RNA in three farm animal species. BMC Genomics. 19:684. https://doi.org/10.1186/s12864-018-5037-7.
- Kern, C., Ying, W., Xu, X., Pan, Z., Halstead, M., Chanthavixay, G., Saelao, P., Waters, S., Xiang, R., Chamberlain, A., Korf, I., Delany, M.E., Cheng, H.H., Medrano, J.F., Van Eenennaam, A.L., Tuggle, C.K., Ernst, C., Flicek, P., Quon, G., Ross, P., Zhou, H. 2021. Functional annotations of three domestic animal genomes provide vital resources for comparative and agricultural research. Nature Communications. 12:1821. https://doi.org/10.1038/s41467-021-22100-8.
- Kim M, Jung U, Shepherd E, Mihelic R, Voy BH. Isolation of Preadipocytes from Broiler Chick Embryos. J Vis Exp. 2022 Aug 4;(186). doi: 10.3791/63861. PMID: 35993709.
- Kim M, Voy BH. Fighting Fat With Fat: n-3 Polyunsaturated Fatty Acids and Adipose Deposition in Broiler Chickens. Front Physiol. 2021 Sep 29;12:755317. doi: 10.3389/fphys.2021.755317. PMID: 34658934; PMCID: PMC8511411.
- Kim, T.H. and H. Zhou. 2018. Overexpression of chicken IRF7 increased viral replication and programmed cell death to the avian influenza virus infection through TGF-beta/FoxO signaling axis in DF-1. Frontiers in Genetics. doi: 10.3389/fgene.2018.00415.
- Kim, T.H., C. Kern, and H. Zhou. 2020. Knockout of IRF7 Highlights its Modulator Function of Host Response Against Avian Influenza Virus and the Involvement of MAPK and TOR Signaling Pathways in Chicken. Genes 11, 385; doi:10.3390/genes11040385
- Klerks PL, Athrey GN & Leberg PL (2019) Response to selection for increased heat tolerance in a small fish species, with the response decreased by a population bottleneck. Front Ecol Evol 7.
- Kong B, Khatri B, Kang S, Shouse S, Kadhim H, Kidd M Jr, Lassiter K, Hiltz J, Mallmann B, Orlowski S, Anthony N, Bottje W, Kuenzel W and Owens C. 2021. Blood plasma biomarkers for woody breast disease in commercial broilers. Front. Physiol. 12:712694. doi: 10.3389/fphys.2021.712694
- Kuenzel, W.J., S.W. Kang and A. Jurkevich. (2020). The vasotocin system and its role in the regulation of stress in birds. In: Vasopressin (Vitamins and Hormones) (Ed. G. Litwack)Vol. 113:183-216, Academic Press/ Elsevier, Oxford, UK
- Lai B, Tang Q, Jin W, Hu G, Wangsa D, Cui K, Stanton BZ, Ren G, Ding Y, Zhao M, Liu S, Song J, Ried T, Zhao K. Trac-looping measures genome structure and chromatin accessibility. Nat Methods. 2018 Aug 27. doi: 10.1038/s41592-018-0107-y
- Lake JA, Abasht B*. 2020. Glucolipotoxicity: A Proposed Etiology for Wooden Breast and Related Myopathies in Commercial Broiler Chickens. Frontiers in Physiology.11:169.
- Lake JA, Brannick EM, Papah MB, Lousenberg C, Velleman SG, Abasht B*. 2020. Blood gas disturbances and disproportionate body weight distribution in broilers with Wooden Breast. Frontiers in Physiology. 11:304.
- Lake JA, Papah MB, Abasht B*. 2019. Increased Expression of Lipid Metabolism Genes in Early Stages of Wooden Breast Links Myopathy of Broilers to Metabolic Syndrome in Humans. Genes (Basel). 10(10)
- Lake, J.A., Dekkers, J.C. and Abasht, B., 2021. Genetic basis and identification of candidate genes for wooden breast and white striping in commercial broiler chickens. Scientific Reports, 11(1), pp.1-13. https://doi.org/10.1038/s41598-021-86176-4
- Lee, K., N. Anthony, S. Orlowski, D. Rhoads (2021). SNP-based breeding for broiler resistance to ascites and evaluation of correlated production traits. Hereditas (accepted pending revisions).
- Lei,Z., Y. Wu, W. Nie, D. Yin, X. Yin, Y. Guo, S.E. Aggrey, and J. Yuan, 2018.Transcriptomic analysis of xlan oligosaccharide utilization systems in Pediococcus acidilactici strain BCC-1. J. Agric Food Chem. 66: 4725-4733.
- Levy J, Tamborindeguy C, Athrey G, Scheuring DC, Koym JW & Miller JC (2018) Transcriptome of Russet Norkotah and its clonal selection, TXNS278. BMC Res Notes 11, 160.
- Li, X., Chen, W., Zhang, H., Li, A., Shu, D., Li, H., Dai, Z., Yan, Y., Zhang, X., Lin, W., Ma, J., Xie, Q. 2017. Naturally occurring frame-shift mutations in the tvb receptor gene are responsible for decreased susceptibility to subgroups B, D, and E. Journal of Virology. 92(8):e01770-17. https://doi.org/10.1128/JVI.01770-17.
- Liao, L., Chen, W., Zhang, X., Zhang, H., Li, A., Yan, Y., Zhang, X., Li, H., Lin, W., Ma, J., Xie, Q. 2022. Semen Exosomes Mediate Vertical Transmission of Subgroup J Avian Leukosis Virus. Journal of Virology. https://doi.org/10.1016/j.virs.2022.01.026.
- Liao, Z., Dai, Z., Cai, C., Zhang, X., Li, A., Zhang, H., Yan, Y., Lin, W., Wu, Y., Li, H., Li, H., Xie, Q. 2019. Knockout of Atg5 inhibits proliferation and promotes apoptosis of DF-1 cells. In Vitro Cellular and Developmental Biology - Animals. 55(5):341-348. https://doi.org/10.1007/s11626-019-00342-7.
- Liao, Z., Zhang, X., Song, C., Lin, W., Chen, Y., Xie, Z., Chen, S., Nie, Y., Li, A., Zhang, H., Li, H., Li, H., Xie, Q. 2020. ALV-J inhibits autophagy through the GADD45ß/MEKK4/P38MAPK signaling pathway and mediates apoptosis following autophagy. Cell Death & Disease. 11:684. https://doi.org/10.1038/s41419-020-02841-y.
- Ling, A., E.H. Hay, S.E. Aggrey and R. Rekaya, 2018. A Bayesian approach for analysis of ordered categorical responses subject to misclassification. PLoS ONE 13(12):e0208433. DOI: 10.1371/journal.pone.0208433
- Ling, A.S., E. H. Hay, S. E. Aggrey, and R. Rekaya, 2021. Dissection of the impact of prioritized QTL-linked and unlinked SNP markers on the accuracy of genomic selection. BMC Genetics 22(1) Article number 26. DOI: 10.1186/s12863-021-00979-y
- Liu L, Wang D, Mi S, Duan Z, Yang S, Song J, Xu G, Yang N, Yu Y. The different effects of viral and bacterial mimics maternal stimuli on ethology of hens and reproduction of their offspring. Poult Sci. 2019 Apr 15. pii: pez189. doi: 10.3382/ps/pez189.
- Liu L, Yang N, Xu G, Liu S, Wang D, Song J, Duan Z, Yang S, Yu Y. Transgenerational transmission of maternal stimulatory experience in domesticated birds. FASEB J. 2018 Sep 27:fj201800762RR. doi: 10.1096/fj.201800762RR.
- Ma L, Li H, Hu J, Zheng J, Zhou J, Botchlett R, Matthews D, Zeng T, Chen L, Xiao X, Athrey G, Threadgill D, Li Q, Glaser S, Francis H, Meng F, Li Q, Alpini G & Wu C (2020) Indole Alleviates Diet-induced Hepatic Steatosis and Inflammation in a Manner Involving Myeloid Cell PFKFB3. Hepatology.
- Maharjan P, Beitia A, Weil J, Suesuttajit N, Hilton K, Caldas J, Umberson C, Martinez D, Kong B, Owens C, Coon C. 2021. Woody breast myopathy broilers show age-dependent adaptive differential gene expression in Pectoralis major and altered in-vivo triglyceride kinetics in adipogenic tissues. Poultry Science. 100(7): 101092. doi.org/10.1016/j.psj.2021.101092.
- Malila Y, Uengwetwanit T, Arayamethakorn S, Srimarut Y, Thanatsang KV, Soglia F, Strasburg GM, Rungrassamee W, Visessanguan W. Transcriptional Profiles of Skeletal Muscle Associated With Increasing Severity of White Striping in Commercial Broilers. Frontiers in Physiology Jun 16;11:580. doi: 10.3389/fphys.2020.00580. eCollection 2020
- Malila, Y., Thanatsang, K,, Arayamethakorn, S., Uengwetwanit, T., Srimarut, Y., Petracci, M., Strasburg, G.M., Rungrassamee, W., Visessanguan, W. (2019). Absolute expressions of hypoxia-inducible factor-1 alpha (HIF1A) transcript and the associated genes in chicken skeletal muscle with white striping and wooden breast myopathies. PLoS One. 2019 Aug 8;14(8):e0220904. doi: 10.1371/journal.pone.0220904. eCollection 2019
- Mandal A, Mandal R, Yang Y, Khatri B, Kong B, and Kwon Y. 2021. In vitro characterization of chicken gut bacterial isolates for probiotic potentials. Poultry Science. 100(2):1083-1092. doi: 10.1016/j.psj.2020.11.025.
- Mays, J.K., Black-Pyrkosz, A., Mansour, T., Schutte, B.C., Chang, S., Dong, K., Hunt, H.D., Fadly, A.M., Zhang, L., Zhang, H. 2019. Endogenous avian leukosis virus in combination with serotype 2 Marek's disease virus boosted the incidence of LL-like bursal lymphomas in susceptible chickens. Journal of Virology. 93:e00861-19. https://doi.org/10.1128/JVI.00861-19.
- McCarthy, F.M., Jones, T.E., Kwitek, A.E., Smith, C.L., Vize, P.D., Westerfield, M., Bruford, E.A. The Case for Standardising Gene Nomenclature Across Vertebrates. Preprints 2021, 2021090485. doi: 10.20944/preprints202109.0485.v2
- McCarthy, F.M., Pendarvis, K., Cooksey, A.M., Gresham, C.R., Bomhoff, M., Davey, S., Lyons, E., Sonstegard, T.S., Bridges, S.M. and Burgess, S.C., 2019. Chickspress: a resource for chicken gene expression. Database, 2019. https://doi.org/10.1093/database/baz058
- McPherson, M.C., Cheng, H.H., Smith, J.M., Delany, M.E. 2018. Vaccination and host Marek's disease-resistance genotype significantly reduce oncogenic gallid alphaherpesvirus 2 telomere integration in host birds. Cytogenetics and Genome Research. 156(4):204-214. https://doi.org/10.1159/000495174.
- Meyer, M.M., Lamont, S.J., and Bobeck, E.A. 2022. Mitochondrial and glycolytic capacity of peripheral blood mononuclear cells isolated from diverse poultry genetic lines: optimization and assessment. Frontiers in Veterinary Sci. 8:815878. doi: 10.3389/fvets.2021.815878
- Mihelic R, Winter H, Powers JB, Das S, Lamour K, Campagna SR, Voy BH. Genes controlling polyunsaturated fatty acid synthesis are developmentally regulated in broiler chicks. Br Poult Sci. 2020 Oct;61(5):508-517. doi: 10.1080/00071668.2020.1759788. Epub 2020 Sep 16. PMID: 32316746.
- Mihelic, R.*, E. R. Urban, T. Gill, M. O. Smith, and B. H. Voy. Increasing Charcoal Efficiency for Brooding Broiler Chickens in Rural Rwanda. African Journal of Poultry Farming.
- Momen, M., Kranis, A., Rosa, G. J. M., Muir, P. and Gianola, D. Predictive assessment of single-step BLUP with linear and non-linear similarity RKHS kernels: A case study in chickens. Journal of Animal Breeding and Genetics 00:1-12, 2021 (DOI: 10.1111/jbg.12665)
- Monson, M.S. and Lamont, S.J. 2021. Genetic resistance to avian pathogenic Escherichia coli (APEC): current status and opportunities. Avian Pathology, DOI: 10.1080/03079457.2021.1879990
- Monson, M.S., Bearson, B.L., Sylte, M.J., Looft, T., Lamont, S.J., and Bearson, S.M.D. 2021.Transcriptional response of blood leukocytes from turkeys challenged with Salmonella enterica serovar Typhimurium UK1. Vet. Immunol. Immunopathol.232: doi.org/10.1016/j.vetimm.2020.110181
- Monson, M.S., Van Goor, A.G., Ashwell, C.M., Persia, M.P., Rothschild, M.F. Schmidt, C.J., Lamont, S.J. 2018. Immunomodulatory effects of heat stress and lipopolysaccharide on the bursal transcriptome in two distinct chicken lines. BMC Genomics. 2018 Aug 30;19(1):643. doi: 10.1186/s12864-018-5033-y
- Monson, M.S., Van Goor, A.G., Persia, M.E., Rothschild, M. F., Schmidt, C.J., Lamont, S.J. 2019. Genetic lines respond uniquely within the chicken thymic transcriptome to acute heat stress and low dose lipopolysaccharide. Scientific Reports 9:13649 doi.org/10.1038/s41598-019-50051-0
- MOREIRA FILHO, A.L.B., FERKET, P.R., MALHEIROS, R.D., OLIVEIRA, C.J.B., ARISTIMUNHA, P.C., WILSMANN, D.E, GIVISIEZ, P.E.N. Enrichment of amnion with threonine in chicken embryos affects the small intestine development, ileal gene expression and performance of broilers between 1 and 21 days of age. Poultry Science, (2019), v.98, P. 1363-1370
- Mushi JR, Chiwanga GH, Amuzu-Aweh EN, Walugembe M, Max RA, Lamont SJ, Kelly TR, Mollel EL, Msoffe PL, Dekkers J, Gallardo R, Zhou H, Muhairwa AP. Phenotypic variability and population structure analysis of Tanzanian free-range local chickens. BMC Vet Res. 2020 16(1):360. doi: 10.1186/s12917-020-02541-x.
- Mushi, J.R., Chiwanga, G.H., Mollel, E.L., Walugembe, M., Max, R.A., Msoffe, P.M., Gallardo, R.A., Kelly, T.R., Lamont, S., Dekkers, J., Zhou, H, and Muhairwa, A. 2021. Antibody response, viral load, viral clearance, and growth rate in Tanzanian free-range local chickens infected with lentogenic Newcastle disease virus. J Vet Med Animal Health. Vol. 13(2): 98-105, DOI: 10.5897/JVMAH2021.0912
- Nanduri B, Gresham CR, Hui WW, Ou M, Bailey RH, Edelmann MJ. An atlas of the catalytically active liver and spleen kinases in chicken identified by chemoproteomics. J Proteomics. 2020 Aug 15;225:103850. doi: 10.1016/j.jprot.2020.103850. Epub 2020 Jun 2.
- Nanduri B, Gresham CR, Jones G, Bailey RH, Edelmann MJ. Identification of active deubiquitinases in the chicken tissues. Proteomics. 2022 Jan;22(1-2):e2100122. doi: 10.1002/pmic.202100122. Epub 2021 Oct 23.
- Neerukonda, S. N., Tavlarides-Hontz, P., McCarthy, F., Pendarvis, K., & Parcells, M. S. (2019). Comparison of the transcriptomes and proteomes of serum exosomes from Marek's disease virus-vaccinated and protected and lymphoma-bearing chickens. Genes, 10(2), 116. https://doi.org/10.3390/genes10020116
- Nelson JR, Ibrahim M, Sobotik EB, Athrey G & Archer GS (2020) Effects of yeast fermentate supplementation on cecal microbiome, plasma biochemistry and ileal histomorphology in stressed broiler chickens. Livest Sci, 104149.
- Nelson JR, Sobotik EB, Athrey G & Archer GS (2020) Effects of supplementing yeast fermentate in the feed or drinking water on stress susceptibility, plasma chemistry, cytokine levels, antioxidant status, and stress- and immune-related gene expression of broiler chickens. Poult Sci.
- Nguyen XD, Zhao Y, Evans JD, Lin J, Voy B, Purswell JL. Effect of Ultraviolet Radiation on Reducing Airborne Escherichia coli Carried by Poultry Litter Particles. Animals (Basel). 2022 Nov 16;12(22):3170. doi: 10.3390/ani12223170. PMID: 36428397.
- Nguyen, X., Y. Zhao, J. D. Evans, J. Lin, L. Schneider, B. H. Voy, S. A. Hawkins, and J. L. Purswell. Evaluation of bioaerosol samplers for collecting airborne Escherichia coli carried by dust particles from poultry litter. Transactions of the ASABE, 65(4), 825-833
- Niu Q, Zhang T, Xu L, Wang T, Wang Z, Zhu B, Zhang L, Gao H, Song J, Li J, Xu L. Integration of selection signatures and multi-trait GWAS reveals polygenic genetic architecture of carcass traits in beef cattle. Genomics. 2021 Sep;113(5):3325-3336. DOI: 10.1016/j.ygeno.2021.07.025. Epub 2021 Jul 25. PMID: 34314829.
- Oladeinde A, Abdo Z, Zwirzitz B, Woyda R, Lakin SM, Press MO, Cox NA, Thomas JC 4th, Looft T, Rothrock MJ Jr, Zock G, Plumblee Lawrence J, Cudnik D, Ritz C, Aggrey SE, Liachko I, Grove JR, Wiersma C. Litter Commensal Bacteria Can Limit the Horizontal Gene Transfer of Antimicrobial Resistance to Salmonella in Chickens. Appl Environ Microbiol. 2022 May 10;88(9):e0251721. doi: 10.1128/aem.02517-21. Epub 2022 Apr 13.
- Oladeinde, A., Z. Abdo, M.O. Press, K. Cook, N.A. Cox, B. Zwirtiz, R. Woyda, S.M.Lakin, J.C. Thomas IV, T. Looft, D.E. Cosby, A. Hinton, Jr., J. Guard, E. Line, M. J. Rothrock, M. E. Berrang, K. Herrington, G. Zock, J. Plumbee, D. Cudnik, S. House, S. Ingram, L. Lariscy, M. Wagner, S. E. Aggrey, L. Chai and C. Ritz, 2021. Horizontal gene transfer is the main driver of antimicrobial resistance in broiler chicks infected with Salmonella enterica Serovar Heidelberg. mSystems 6(4) https://doi.org/10.1128/mSystems.00729-21
- Orlowski S.K., Tabler T., Hiltz J.Z., Greene E.S., Anthony N.B., Dridi S (2020). Processing evaluation of random bred broiler populations and their common ancestor at 55 days under chronic heat stress conditions. Poultry Science 99, 3491-3500.
- Overbey EG, Ng TT, Catini P, Griggs LM, Stewart P, Tkalcic S, Hawkins RD and Drechsler Y*: Transcriptomes of an Array of Chicken Ovary, Intestinal, and Immune Cells and Tissues. Front. Genet. 2021; 12:664424. doi: 10.3389/fgene.2021.664424
- Pacheco Santana, T., Gasparino, E., de Souza Khatlab, A., Oliveira Brito, C., Teixeira Barbosa, L., Lamont, S., and Del Vesco. A. 2021. Effect of prenatal ambient temperature on the performance and oxidative metabolism of Japanese quail (Coturnix coturnix japonica) layers exposed to heat stress during growth. Scientific Reports 11:9809, https://doi.org/10.1038/s41598-021-89306-0
- Papah MB, Abasht B*. 2019. Dysregulation of lipid metabolism and appearance of slow myofiber-specific isoforms accompany the development of Wooden Breast myopathy in modern broiler chickens. Scientific Reports. 9(1):17170.
- Papah MB, Brannick EM, Schmidt CJ, Abasht B*. 2017. Evidence and role of phlebitis and lipid infiltration in the onset and pathogenesis of Wooden Breast Disease in modern broiler chickens. Avian Pathology. 46(6):623-643.
- Papah MB, Brannick EM, Schmidt CJ, Abasht B*. 2018. Gene expression profiling of the early pathogenesis of wooden breast disease in commercial broiler chickens using RNA-sequencing. PLoS One. 13(12):e0207346.
- PARK, I, PASQUETTI, T., MALHEIROS, RD. FERKET, PR., KIM, SW. Effects of supplemental L-methionine on growth performance and redox status of turkey poults compared with the use of DL-methionine, Poultry Science, Volume 97, Issue 1, 1 January 2018, Pages 102-109.
- Parveen A, C Jackson, S Dey, K Tarrant, N Anthony, DD Rhoads (2020) Identification and validation of quantitative trait loci for ascites syndrome in broiler chickens using whole genome resequencing. BMC Genetics 21:54.
- Passafaro, T. L., Lopes, F. B., Dorea, J. R. R., Craven, M., Breen, V., Hawken, R. J. and Rosa, G. J. M. Would large dataset sample size unveil the potential of deep neural networks for improved genome-enabled prediction of complex traits? The case for body weight in broilers. BMC Genomics 21: 771, 2020.
- Peichel C, Nair DVT, Dewi G, Donoghue AM, Reed KM, and Johny AK. 2019. Effect of lemongrass (Cymbopogon citratus) essential oil on the survival of multidrug-resistant Salmonella enterica serovar Heidelberg in contaminated poultry drinking water. Journal of Applied Poultry Research, doi: 10.3382/japr/pfz076.
- Puckett D, Alquraishi M, Alani DS, Chahed S, Frankel VD, Donohoe D, Voy B, Whelan J, Bettaieb A. Zyflamend, a unique herbal blend, induces cell death and inhibits adipogenesis through the coordinated regulation of PKA and JNK. Adipocyte. 2020 Dec;9(1):454-471. doi: 10.1080/21623945.2020.1803642. PMID: 32779962; PMCID: PMC7469463.
- Ramírez GA, Keshri J, Vahrson I, Garber AI, Berrang ME, Cox NA, González-Cerón F, Aggrey SE, Oakley BB. Cecal Microbial Hydrogen Cycling Potential Is Linked to Feed Efficiency Phenotypes in Chickens. Front Vet Sci. 2022 Jun 21;9:904698. doi: 10.3389/fvets.2022.904698.
- Ramírez GA, Richardson E, Clark J, Keshri J, Drechsler Y, Berrang ME, Meinersmann RJ, Cox NA, Oakley BB. Broiler chickens and early life programming: Microbiome transplant-induced cecal community dynamics and phenotypic effects. PLoS One. 2020 Nov 13;15(11): e0242108. doi: 10.1371/journal.pone.0242108. PMID: 33186366; PMCID: PMC7665843
- Reed KM, Mendoza KM, Abrahante JE, and Coulombe RA. 2018. Comparative response of the hepatic transcriptomes of domesticated and wild turkey to aflatoxin B1. Toxins (Basel), 10(1):42. doi: 10.3390/toxins10010042.
- Reed KM, Mendoza KM, Abrahante JE, Velleman SG, and Strasburg GM. 2021. Data mining identifies circular RNAs in skeletal muscle of thermally challenged turkey poults. Frontiers in Physiology, 12:1406. doi:10.3389/fphys.2021.732208.
- Reed KM, Mendoza KM, and Coulombe RA Jr. 2019. Differential transcriptome responses to aflatoxin B1 in the cecal tonsil of susceptible and resistant turkeys. Toxins (Basel), 11(1): 55. doi: 10.3390/toxins11010055.
- Reed KM, Mendoza KM, and Coulombe RA. 2019. Altered gene response to aflatoxin B1 the spleens of susceptible and resistant turkeys. Toxins (Basel), 11(5):242; doi.org/10.3390/toxins11050242.
- Reed KM, Mendoza KM, Strasburg GM, and Velleman SG. 2022. Transcriptome response of proliferating muscle satellite cells to thermal challenge in commercial turkey. Frontiers in Physiology, 13:970243.doi: 10.3389/fphys.2022.970243
- Resnyk, C. W., W. Carré; X. Wang, T. E. Porter, J. Simon, E. Le Bihan- Duval, M. J. Duclos, S. E. Aggrey and L. A. Cogburn, 2017. Transcriptional analysis of abdominal fat in chickens divergently selected on bodyweight at two ages reveals novel mechanisms controlling adiposity: Validating visceral adipose tissue as a dynamic endocrine and metabolic organ, BMC Genomics 18(1)626 doi: 10.1186/s12864-017-4035-5.
- Rexroad III, C.E., Vallet, J.L., Matukumalli, L.K., Ernst, C., Van Tassell, C.P., Cheng, H.H., Reecy, J., Fulton, J., Taylor, J., Lunney, J.K., Liu, J., Cockett, N., Smith, T.P., Van Eenennaam, A., Clutter, A., Telugu, B., Purcell, C., Bickhart, D.M., Blackburn, H.D., Neibergs, H., Wells, K., Boggess, M.V., Sonstegard, T. 2019. Genome to phenome: improving animal health, production, and well-being: a new USDA blueprint for animal genome research 2018-2027. Frontiers in Genetics. 10:327. https://doi.org/10.3389/fgene.2019.00327.
- Rhoads DD, Robert F. Wideman J. (2020). Physiological challenges in poultry breeding, 18pp. In Aggrey SE, Zhou H, Tixier-Boichard M, Rhoads D (ed), Advances in poultry genetics and genomics. Burley Dodds Science Publishing, Cambridge, UK.
- Rowland K, Saelao P, Wang Y, Fulton JE, Liebe GN, McCarron AM, Wolc A, Gallardo RA, Kelly T, Zhou H, Dekkers JCM, Lamont SJ. 2018. Association of Candidate Genes with Response to Heat and Newcastle Disease Virus. Genes (Basel). 9(11). pii: E560. doi: 10.3390/genes9110560.
- Rowland, K., Ashwell, C.M. Persia, M.P., Rothschild, M.F., Schmidt, C., Lamont, S.J. 2019. Genetic analysis of production, physiologic, and egg quality traits in heat-challenged commercial white egg-laying hens using 600k SNP array data. Genetics Selection Evolution 51:31 doi.org/10.1186/s12711-019-0474-6
- Rowland, K., Persia, M., Rothschild, M., Schmidt, C., Lamont, S. 2019. Blood gas and chemistry components are moderately heritable in commercial white egg-laying hens under acute or chronic heat exposure. Poultry Science 0:1-5. http://dx.doi.org/10.3382/ps/pez204
- Rowland, K., Saelao, P., Wang, Y., Fulton, J.E., Liebe, G. N., McCarron., A.M., Wolc, A., Gallardo, R.A., Kelly, T., Zhou, H., Dekkers, J.C.M., and Lamont, S.J. 2018. Association of candidate genes with response to heat and Newcastle disease virus. GENES 9, 560; doi:10.3390/genes9110560
- Rowland, K., Wolc, A., Gallardo, R.A., Kelly, T., Zhou, H., Dekkers, J.C.M. and Lamont, S.J. 2018. Genetic analysis of a commercial egg laying line challenged with Newcastle Disease Virus. Frontiers Genet doi.org/10.3389/fgene.2018.00326
- Saelao, P. Wang, Y. Chanthavixay, G., Yu, V., Gallardo, R.A., Lamont, S.J., Dekkers, J.M., Kelly, T., Zhou, H. 2018. Integrated proteomic and transcriptomic analysis of differential expression of chicken lung tissue in response to NDV infection during heat stress. GENES 9, 579; doi:10.3390/genes9120579
- Saelao, P., Wang, Y., Chanthavixay, G., Gallardo, R.A., Wolc, A., Dekkers, J.C.M., Lamont, S.J., and Zhou, H. 2019. Genetics and genomic regions affecting response to Newcastle disease virus infection under heat stress in layer chickens. Genes 10(1), 61; https://doi.org/10.3390/genes10010061
- Saelao, P., Wang, Y., Chanthavixay, G., Yu, V., Gallardo, R.A., Dekkers, J.C.M., Lamont, S.J., Kelly, T., and Zhou, H. 2021. Distinct transcriptomic response to Newcastle disease virus infection during heat stress in chicken tracheal epithelial tissue. Scientific Reports.11:7450, doi.org/10.1038/s41598-021-86795-x
- Saelao, P., Y. Wang, K. C, J. Dekkers, R. Gallardo, T.R. Kelly, S.J. Lamont. Zhou, H. 2018. Novel insights into the host immune response of chicken Harderian gland tissue during Newcastle disease virus infection and heat treatment. BMC Veterinary Research https://doi.org/10.1186/s12917-018-1583-0
- Schilling, M., Memari, S., Cavanaugh, M., Katani, R., Deist, M.S., Radzio-Basu, J., Lamont. S.J., Buza, J.J., and Kapur, V. 2019. Conserved, breed-dependent, and subline-dependent innate immune responses of Fayoumi and Leghorn chicken embryos to NDV infection. Scientific Reports 9:7209 doi.org/10.1038/s41598-019-43483-1
- Schilling, M.A., Katani, R., Memari, S., Cavanaugh, M., Buza, J., Radzio-Basu, J., Mpenda, F.N., Deist, M.S., Lamont, Kapur, V. 2018. Transcriptional innate immune response of the developing chicken embryo to Newcastle disease virus infection. Frontiers Genet doi: 10.3389/fgene.2018.00061
- Schneiders, G. H., J. C. Foutz, M. C. Milfort, A. F. A. Ghareeb, U. G. Sorhue, J. N. Richter, A. L. Fuller, S. M. Williams, R. Rekaya, and S. E. Aggrey, 2020. Ontogeny of intestinal permeability in chickens infected with Eimeria maxima: Implications for intestinal health. J. Adv. in Parasitol. 6(2) dx.doi.org/1
Attachments
Land Grant Participating States/Institutions
AR, AZ, CA, DE, GA, IA, MD, MI, MN, MS, NC, OH, PA, TN, TX, VA, WINon Land Grant Participating States/Institutions
California State University, Fresno, Queen Mother Hospital for Animals, The Royal Veterinary College, USDA-ARS-Avian Disease & Oncology Laboratory, USDA-ARS/Missouri, Western University of Health Sciences