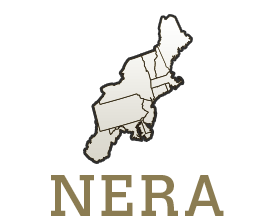
NE2101: Eastern White Pine Health and Responses to Environmental Changes
(Multistate Research Project)
Status: Active
NE2101: Eastern White Pine Health and Responses to Environmental Changes
Duration: 10/01/2021 to 09/30/2026
Administrative Advisor(s):
NIFA Reps:
Non-Technical Summary
Statement of Issues and Justification
Title: Managing Eastern White Pine Health and Forest Responses to a Changing Environment
Start Date: October 2021
End Year: 2026
Overall hypothesis or goal: Climate-adapted management (CAM), i.e., silvicultural strategies that increase tree vigor and wood value, will enhance health, services, productivity, resilience & utilization of forest ecosystems, while targeted decision tools and extension/education will increase social acceptance and use of management practices for forest systems across broad spatial scales.
Specific Objectives (Long-term underlined, short-term follow)
- Assess, evaluate, and forecast forest productivity and sustainability over 25 years by writing proposals and receiving funding to establishing the Eastern White Pine Permanent Plot Network to meet three uses: intensive tree measurements, ecosystem services measurements, and demonstration of CAM benefits. Management treatments will be established on permanent plots in EWP forests and tracked through time. The innovative network of forests dedicated to long-term observations on the benefits of forest management will be established across the EWP range, from NC to ME to WI.
- Use interdisciplinary research to assess CAM effects on ecosystem services and reduce losses associated with insects, fungal pathogens, and drought. The demonstration forest network will also include i) quantifying current status of insect pests, fungal pathogens; ii) assessing similarities and differences in health problems; and iii) writing additional proposals to conduct transdisciplinary research to assess forest health, climate, and management interactions.
- Improve local rural economies by assessing eastern white pine product and market opportunities across the range.
- Transfer knowledge on CAM to students, land managers, and surrounding communities. This will be achieved writing a proposal and receiving funding to: i) host hands-on field workshops; ii) conduct multi-media technology-transfer programs that will prepare managers to implement best practices; and iii) develop a strategic multi-dimensional outreach program that will help surrounding communities understand and support management practices enhancing forest health and productivity.
The need as indicated by stakeholders.
Eastern white pine (EWP) is a major component of the eastern forest with over 186 million mbf (15 billion ft3) in 25 states. The resource can be underutilized as 10 states now have over 5 million mbf, but only 4 of them harvest more than 1.5% of the available resource. In Maine, EWP saw logs can sell for $190 to $300 mbf-1, but in another states, logs sell for $20-$80 mbf-1 despite being abundant. The species responds extremely well to management as it can naturally regenerate after harvesting and continues rapid growth at even large sizes (>20 in. DBH) if densities are kept low. Managing stand densities can also ameliorate losses due to drought, fungal pathogens, and insect pests. Unmanaged stands can suffer mortality over 50%, but general social resistance to harvesting can hinder proper management, and investments are often not made.
This project provides an ideal forest system that supports and highlights goals that are found in all Forest Action Plans (https://www.stateforesters.org/forest-action-plans/) in the region. One common goal is to protect and manage forests from threats. The health of white pine is adversely affected by invasive organism (white pine blister rust) and native pests and disease (white pine weevil, pine bast scale, Caliciopsis canker, needle pathogens). Changing climate results in more weather extremes resulting in drought damage (growth loss, increased susceptibility to pathogen damage) or increased white pine needle damage due to high precipitation amounts in the spring. Changing land use, especially field abandonment, creates un-natural conditions under which developing white pine stands are suffering from weevil damage and drought stress.
The importance of the work, and what the consequences are if it is not done.
Lack of EWP management has adverse effects on eastern white pine forests such that some states have low number of regenerating stems to replace older trees while other states have dense stands that increase the risk of damage due to drought, pests, and disease. Fortunately, EWP responds well to management which reduces health risks and defects because it can regenerate naturally after harvesting and utilizing low-density management can mitigate risks. The managed stands will fare well under climate change due EWP adaptability and high dispersal ability This project will use demonstration forests to show how EWP can be managed to reduce risks and improve forest resilience and value.
The technical feasibility of the research, the advantages for doing the work as a multistate effort.
A Letter of Intent and three proposals have been developed by multi-state teams. A Letter of Intent to the 2019 Sustainable Agricultural Systems Program (NIFA) attracted involvement from 23 investigators and 10 collaborators from 12 states (ME, NH, VT, MA, NY, PA, VA, NC, OH, MI, WI, MN) but was not selected for proposal development. The University of Maine and SUNY – ESF submitted a $500,000 proposal in April 2020 to the USDA-AFRI program on Sustainable Agroecosystems: Health, Functions, Processes and Management. If funded, the proposed work would study fundamental tree processes affecting tree health and value, how the processes are affected by EWP management, and how management affects ecosystem services as represented by birds, xylophytic beetles, and carbon dynamics. A second proposal was submitted by the University of New Hampshire, University of Maine, and University of Massachusetts to the USDA Renewable Resource Extension Act – National Focus Fund Projects (RREA-NFF), and the two year project will be funded for $100,000 to support a symposium, establishing the EWP Management Institute, and to provide published materials and videos in support of EWP management. The symposium on EWP Health and Management is scheduled for June 2021 in Durham, NH, and will be coordinated with the IUFRO Working Party Meetings on tree diseases and rust diseases. A third proposal was submitted in September 2020 to the USDA Forest Service Landscape Scale Restoration Fund for $550,000 that would help establish a network of demonstration forests in 6 states (ME, NH, VT, NY, PA, VA).
What the likely impacts will be from successfully completing the work.
We expect at least 500 forest resource professionals will participate in the symposium and EWP Management Institute activities. These professionals will then share the information with another 10,000 clients affecting at least 400,000 acres. These positive changes will be achieved by, first, sharing knowledge about EWP health threats and management options for improving EWP resilience, regeneration, and sustainability across its range. Current plans will be implemented across 9 states (ME, NH, VT, NY, PA, VA, NC, MI, WI) at 12 locations and utilize over 30 treatments. There will be multiple examples of shelterwood treatment and low density management that replicate the process across the region, with site to site customizations that make the treatments better suited for local needs, but also can provide new ideas and strategies for other locations. Second, allowing people to directly view recommended management practices at the demonstration forests with supporting inventory data. Being able to compare management and non-treated stands will directly illustrate the importance of management. Third, generating an effective outreach program involving EWP Management Institute, field manual, fact sheets, workshops, and webinars will prepare forest resource professionals to work with clients in the public and private sector to improve EWP management. Fourth, sharing of information among states and demonstration forests will continually improve the knowledge about effective EWP management and improve strategies for getting management practices implemented by forest resource professionals and forest owners. Each participating state is establishing at least one demonstration forest and will sponsor workshops best suited for the state’s situation. By establishing the EWP Management Institute, there will be infrastructure for forest resource professionals and scientists to share information and experiences that can help those in other states.
Related, Current and Previous Work
Related, Current and Previous Work
EWP Health Risks. Eastern white pine is a major component of the eastern forest with over 186 million mbf (18 billion ft3) in 25 states (Figure 1, See Attachments) (U.S. Department of Agriculture, 2020). Unmanaged stands can suffer mortality over 50% due to a range of factors including insect pests, fungal diseases, and drought (Livingston & Kenefic, 2018). Risk of health problems will increase as increasing spring precipitation intensifies needle damage due to fungal pathogens, and increased severity of droughts intensifies damage due to Caliciopsis canker, bark beetles, and root disease (Costanza, et al., 2018).
The most immediate threat to EWP is “white pine needle damage” (WPND) (Bergdahl, et al., 2020). The disease involves pathogenic fungi infecting expanding needles of EWP in late spring during periods of high precipitation after which the fungi colonize and kill the needles by the following spring. One year after infection, the 1 yr old and older needles turn yellow, the fungi sporulate, and the pathogen cycle continues if the precipitation is high. Since 2010, there have been 7 years of high June precipitation (Livingston, et al., 2019) after which EWP needles are shed one year later. The repeated loss of needles results in reduced growth, and continued defoliation will likely start killing trees. Because WPND is a new, climate related health problem on EWP (Wyka, et al., 2018; Wyka, et al., 2017), observations on growth impacts and potential control strategies are limited to just a few locations and studies (McIntire, et al., 2018). There is an urgent need to quantify impacts of WPND on trees and to document how well control strategies can mitigate potential losses from the disease.
Another climate related health problem on EWP is drought (Livingston & Kenefic, 2018) and subsequent infection and damage by Caliciopsis pinea (Costanza, et al., 2018). Drought stress can result from a combination of factors involving stand density, rooting depth, and time of low water content in soils (Livingston & Kenefic, 2018). Under severe conditions, up to half of the EWP in pole-size stands (5-10 in.[1] dia. at breast height or DBH) can die. The stressed trees suffer from infection by Caliciopsis which infects stems resulting in small cankers and resin (Costanza, et al., 2018). Original establishment of Caliciopsis on EWP likely involves the pine bast scale, Matsucoccus macrocicatrices (Schulz, et al., 2018b; Schulz, et al., 2018; Mech, et al., 2013). The presence of Caliciopsis symptoms on EWP is widespread in New England and New York, in up to 70% of the EWP stands (Munck, et al., 2015; Munck, et al., 2016). If predisposing risks are not reduced, EWP stands are likely to suffer from increased canker infections and mortality.
Additional health risks to EWP include the well-known white pine blister rust stem canker caused by Cronartium ribicola and the white pine weevil (Pissodes strobi) (Livingston, et al., 2019). Blister rust primarily infects and kills young EWP, with most infections occurring within 6 ft of the ground. White pine weevil kills terminal shoots resulting in stem deformities and value loss but does not kill trees. Although cultural practices for reducing risks are well known for blister rust and the weevil (Livingston, et al., 2019), climate extremes may increase these health risks.
Half of the prediction models of EWP show increasing importance values as the climate changes, but extreme drought events and pests may limit its sustainable management (Prasad, et al., 2014). Therefore, additional research is needed to identify the relationship between EWP management and how it can improve tree growth and health.
Low density management of EWP improves tree health and value. Fortunately, EWP responds extremely well to silvicultural management (Figure 2, see Attachments) as it can naturally regenerate after harvesting and continues rapid growth at even large sizes (>20 in. DBH) if densities are kept low (Seymour, et al., 2009). Low density stands have been shown in limited studies to reduce the risk of decline (Livingston & Kenefic, 2018) and WPND (McIntire, et al., 2018). The reduction in stand density appears to increase individual tree resistance and resilience to drought, as has been found in other systems (Gleason, et al., 2017). In addition to promoting vigorous trees, low densities may also facilitate the influx of dry air that can limit WPND severity and spread (McIntire, et al., 2018). However, leaf physiology and water relations of EWP are sensitive to drought and dry air (Maier & Teskey, 1992). Therefore, low density management may temporary increase transpirational stress (“thinning shock”) as a previously shaded canopy is exposed (Simonin, et al., 2006). There is a strong need for a clear understanding of the mechanistic links between the change in microclimate driven by thinning and how that impacts the physiology of EWP including water relations and the ability to resist disease.
The potential stress due to thinning shock is likely temporary, because management recommendations recognize the importance of maintaining low densities in EWP stands, i.e., below the C line on the stocking guide (Figure 3, see Attachments) (Leak & Lamson, 1999). Low density management reduces competition and increases live-crown ratios which:
- Favors rapid production of high value sawtimber trees
- Reduced health risks and increases resilience
A major difficulty in achieving low density management in EWP is the need to regenerate EWP at high densities.
The regeneration of managed stands of EWP typically involves a partial cut to open up the canopy to at most 50% cover to allow more sunlight to reach developing seedlings. Mature EWP trees in the residual canopy provide a seed source for the regenerating seedlings. Harvesting operations for opening the stand will scarify the soil, also aiding the establishment of EWP seedlings (Livingston, et al., 2019). A high density of regeneration dominated by EWP, with densities over 10,000 stems/ac, typically results.
High densities of EWP are desired to reduce infections by WPBR and to reduce damage by white pine weevil (Livingston, et al., 2019). However, as seedlings grow larger into saplings, increasing density-related competition begins to affect the health of the EWP. A recent study (Costanza, et al., 2020) found Caliciopsis cankers developing on EWP beginning at 10 ft height on the stem with a maximum number of cankers at 30-60 ft in height, indicating that EWP becomes vulnerable to Caliciopsis infection as saplings (>1 in DBH) develop into pole-size stands (5-12 in DBH). To improve the health of EWP, precommercial thinning (PCT) is needed to reduce stress and likely infections by Caliciopsis.
Lower stem densities resulting from PCT redistributes resources to fewer trees, thus increasing their growth rates, vigor, and survival. Greater tree growth is achieved at some loss to overall stand volume growth per acre. However, PCT remains advantageous because it shortens the time until the retained trees are of a harvestable size, and any tending costs are recouped earlier. PCT improves growing stock by removing unhealthy or damaged trees, and trees with low vigor or value. Failure to remove malformed trees at PCT preserves trees that will continue to increase in size but perhaps little in value if logs are defective or become downgraded by large knots. Growing stock may also be improved by removing species that do not meet management goals (e.g., enhancing wood production, biodiversity, forest health, or critical wildlife habitat). Seymour (2007) recommends repeated light thinning that maintains rapid growth (large logs) and long live crowns to maximize value recovery from EWP trees.
Benefits of traditional PCT may be promoted more or less by crop-tree release (CTR) where cutting is restricted to the immediate vicinity of evenly spaced crop trees (Figure 4, see Attachments) (Miller, et al., 2007). Using CTR releases crop trees from neighbor tree competition but leaves them isolated within a dense unthinned matrix. CTR is usually less expensive than PCT because fewer trees are cut.
Effects of PCT on wood quality differ among species, their end-uses, and sawmill infrastructure. Trees growing at wider spacing can keep low branches alive, resulting in low crown base height, larger branch size and inter-grown ‘red knots’, lower height-to-diameter ratio and more conical form than trees grown under higher densities. Conversely, postponing PCT keeps lower branches in the shade longer where they grow slowly or die (Maguire, et al., 1991; Weiskittel, et al., 2009). This leads to lose ‘black knots’ that downgrade EWP logs more than red knots. Branch retention is a concern in EWP management.
Branch pruning in concert with PCT, including CTR, can be used to improve wood quality and value when branches are removed over an entire log length (e.g., up to 18 feet above ground for a 16-foot log), and this is done early enough to allow clearwood to form over many years after pruning (O'Hara, et al., 1995). Large, long, knot-free EWP logs make the ‘select’ grade which earns roughly twice the price paid for knotty logs. The economics of pruning are more favorable after timely PCT on better sites where rapid diameter growth allows for earlier harvest to recoup pruning costs incurred years earlier. To optimize pruning investment economics, only a subset of crop trees designated for retention to attain large sizes should be pruned for clearwood timber production. During PCT, additional ‘unpruned follower’ trees should also be retained to allow for subsequent thinning of knotty sawlogs while pruned trees are allowed to continue growing larger to produce enough clearwood for adequate recovery of knot-free lumber during milling (Ashton & Kelty, 2018, p. 432). A study on EWP crop trees (Seymour, et al., 2009) found low-density, crop tree management had the highest overall net present value at the end of the 100-yr projection (Figure 5, see Attachments). Pruning can also be done to reduce risk of white pine blister rust entering lower branches of young pines (O'Hara, et al., 2010). PCT alone, or combined with pruning, is expected to create space for better air circulation throughout the stand, reducing humidity and risk of fungal problems (McIntire, et al., 2018).
Increased management of EWP is needed throughout its range. The resource is underutilized as 10 states now have over 5 million mbf, but only 4 of them harvest more than 1.5% of the available resource (U.S. Department of Agriculture, 2020). When EWP annual growth is highly utilized (>60%) (ME, NH) saw-logs can sell for $190/mbf (avg) to $350/mbf (max), but if utilization is below 50% (e.g., NY, WI), logs sell for $100/mbf to $200/mbf. Demonstrating the benefits of management for improving EWP health, growth, and value would be helpful to convince more landowners to manage the resource. Another consideration landowners have in making management decisions is the impact management has on ecosystem services.
Low density management systems promote biodiversity. Intensive forest management can be consistent with some ecosystem services, such as carbon storage (Nunery & Keeton, 2010) but can be at odds with other ecosystem services, such as biodiversity (Burton, et al., 2013). At the landscape scale, these values are often distributed across: 1) reserves which are expected to provide for biodiversity, 2) intensive management areas, which provide for timber production and 3) the matrix, where ecologically-based forest management represents a compromise between timber production and biodiversity (Seymour & Hunter, 1999; U.S. Department of Agriculture and U. S. Department of the Interior, 1994). In contrast, low-density management of EWP may provide opportunities for integrated management of timber production and other ecosystem services, namely biodiversity and carbon sequestration. Plant diversity. Intensive forest management typically promotes the development of dense, even-aged conifer forests that prevent the development and/or persistence of an early-seral community (Davis & Puettmann, 2009; Swanson, et al., 2011). In contrast, by increasing light transmittance, as well as soil resources (Trentini, et al., 2017), low density management of EWP forests may promote the development and persistence of diverse early-seral plant communities in EWP stands. As reductions in fire frequency may have reduced the abundance of early-seral pine forests (Lorimer & White, 2003), low-density white pine management could play a critical role in the conservation of early-seral plant communities and dependent organisms. Bird diversity. In northern forests, including this project’s study area, bird abundance has declined by 33% since 1970 with steeper declines for some insectivorous bird species (Rosenberg, et al., 2019). Forest management practices that support diverse forest bird assemblages are needed to maintain and increase forest bird populations. The decline in forest birds also has potential cascading effects on the diversity and abundance of forest insects and other taxa. Bird foraging guilds will respond differently to PCT. An increase in foliage-dwelling insect abundance and diversity should support a corresponding increase in the % time foliage-foraging birds feed in EWP. This would support the plant vigor hypothesis, which states that vigorous, healthy plants support more herbivorous insect biodiversity and abundance, particularly for folivores, sap-feeders, and gall-makers (Price, 1991; Cornelissen, et al., 2008). Conversely, an increase in % time bark-foraging birds feed in EWP would support the plant stress hypothesis, which states that stressed plants support more herbivorous insect biodiversity, particularly for wood-borers (Rhoades, 1983; Koricheva, et al., 1998). In order to maintain and/or increase the diversity and abundance of bark-foraging birds, it would be important to allow some trees to remain stressed or dying in the stand. Currently, the relationship between wood-boring insects and bark-foraging birds is not well understood for EWP. Promoting EWP health and vigor using PCT by CTR could support both foliage -foraging birds, due to larger crowns on the crop trees and more plants in the gaps around the crop trees, and the bark foraging birds, due to the insect community on the stressed trees in the uncut matrix around the crop trees.
The urban/rural interface and eastern white pine health. EWP is a common tree species in urban, suburban, and rural communities throughout its native range. Their natural abundance, rapid growth, adaptation to a variety of environments and range of cultivars available have resulted in widespread planting in managed landscapes (Dirr & Warren, 2020). Within the Frank A. Waugh Arboretum on the University of Massachusetts, Amherst campus, EWP makes up 7% of all inventoried trees (538/7797), including 16% of all trees ≥70′ in height. In the nearby city of Northampton, MA EWP comprises a similar percentage of public shade trees (739/9887; 7%). The composition and structure of trees on private land is hard to quantify, but a study of private landscapes in Indiana showed that EWP ranked as the seventh most abundant tree species with the fifth largest basal area (Schmitt-Harsh, et al., 2013).
EWP also comprises a significant percentage of trees requiring attention from tree care professionals. A study examining tree failure rates after a catastrophic windstorm in Massachusetts found that EWP was the second most common species suffering storm-related failures (Kane, et al., 2008). Increasingly, however, arborists and tree care professionals are taking note of the serious issues affecting EWP in the region. As a result, EWP is no longer considered a suitable tree for planting in urban landscapes (McElhinney & Harper, 2019).
Related work from CRIS projects
Studies have looked at reproduction of EWP in gaps (Seymour, 2018). Wick and Brazee (2019) are evaluating susceptibility of EWP to an non-native nematode that is found on the white pine weevil. MacLean and Clouston (2020) are investigating suitability of EWP cor three-layer cross-laminated-timber. Lee (2015) reported 3.5 fold increase in EWP seedling establishment and 50% in seedling height growth on plots where buckthorn density if reduced 80%. Germain (2015) reported that EWP quality is frequently reduced due to black knots (dead branches on stems) and that pruning the butt log is prudent and profitable. Stands should be kept at low densities to promote a faster growth rate of pruned trees and a decrease in black knots due to branch survival. Chhin (2018)is studying growth patterns and forest stand dynamics of mix forests in West Virginia, including some EWP, and relating the measures to climate data and stand history. Some forest stands will be thinned and receive prescribed fire to help improve growth. Orrock and Connolly (2021) are investigating what limits tree and plant recruitment in southern Wisconsin forests, including EWP. Langston (2007) reported on how humans contributed to the reduction of EWP in Wisconsin through historic harvests and slash fires followed by unsuccessful attempts at farming.
[1] Because the study depends on direct cooperation from forest managers, the English unit system is used.
Objectives
-
1. Assess, evaluate, and forecast forest productivity and sustainability over 25 years by writing proposals and receiving funding to establishing the Eastern White Pine Permanent Plot Network to meet three uses: intensive tree measurements, ecosystem services measurements, and demonstration of CAM benefits.
Comments: Management treatments will be established on permanent plots in EWP forests and tracked through time. The innovative network of forests dedicated to long-term observations on the benefits of forest management will be established across the EWP range, from NC to ME to WI. -
2. Use interdisciplinary research to assess CAM effects on ecosystem services to reduce losses associated with insects, fungal pathogens, and drought.
Comments: The Permanent Plot Network will also include i) quantifying current status of insect pests, fungal pathogens; ii) assessing similarities and differences in health problems; and iii) writing additional proposals to conduct transdisciplinary research to assess forest health, climate, and management interactions. -
3. Improve local rural economies
Comments: This will be achieved by assessing eastern white pine product and market opportunities across the range. -
4. Transfer knowledge on CAM to students, land managers, and surrounding communities.
Comments: This will be achieved writing a proposal and receiving funding to: i) host hands-on field workshops; ii) conduct multi-media technology-transfer programs that will prepare managers to implement best practices; and iii) develop a strategic multi-dimensional outreach program that will help surrounding communities understand and support management practices enhancing forest health and productivity.
Methods
Eastern White Pine Permanent Plot Network (PPN):
The Eastern White Pine Permanent Plot Network (PPN) will have 3 categories of forest treatments for which proposals will be developed: i) Demonstration Forests, ii) Intensive Measurement Forests, and iii) Ecosystem Services Forests.
Demonstration Forests:
To improve the resilience, value, and services from eastern white pine (EWP) forests, one group of collaborators will establish a network of demonstration forests that utilize best practices in EWP management. An Eastern White Pine Management Institute will coordinate information sharing among the forests and outreach efforts to engage foresters and forest owners to utilize management for improving EWP sustainability across the landscape. The effort includes Maine, New Hampshire, Vermont, New York, Pennsylvania, Virginia, North Carolina, Michigan, and Wisconsin. This project will establish a network of research and demonstration forests and outreach efforts for eastern white pine (EWP) across nine states. The effort will facilitate increased utilization of forest management for improving the resilience, value, and ecosystem services for EWP forests across the landscape in the face of non-native and native pests, stresses due to climate change, and lack of management leading to unhealthy forests and poor stand development. The management prescription for each forest will address key factors for regenerating and/or developing healthy, valuable EWP. Forests will have public access and signage explaining the management. Forest management prescriptions involve treating at least 5 ac with a non-treated 5 ac stand for comparison. Regeneration treatments involve variations of shelterwood cuts in which at least 50% of the overstory is removed, soil is scarified, and naturally regenerated EWP is favored. Treatments on developing EWP stands involve thinning and vegetation control to achieve stands of low-density, crop trees.
Intensive Measurement Forests
Collaborators will write proposals to establish 4-6 locations involving permanent plots for assessing how PCT and pruning affect EWP health and value (Figure 6, see Attachments). PCT will include “PCT by CTR” and “PCT by uniform spacing” (Figure 6). Pruning will involve sawing branches off the main stem of the tree up to 60% of the tree’s height so that 40% of the tree’s height remains as live crown (live crown ratio or LCR is 40%) to maintain vigor and health. Pruning significantly increase the value of crop trees (Germain, et al., 2016). Each set of six treatments will be replicated three times at each forest location. Each treatment will cover about 2 acres, or 12 acres per replicate for 36 acres total at each location (Figure 6). Treatments will occur during the winter of the second year of the project. Treatments for the 2-acre parcels include:
- PCT by CTR-Pruned: Precommercial thinning (PCT) by crop tree release (CTR), white pine at 17-ft spacing, 150 trees per acre (TPA). The release will be achieved by removing all trees that touch the crown of the crop tree, but trees and other vegetation in the lower strata are retained in a matrix to serve as “training” trees to promote straightness and branch shedding. Prune crop trees.
- PCT by CTR: one PCT by CTR block not pruned.
- PCT by Uniform Spacing-Pruned: Uniform PCT 10-ft spacing 450 TPA, for WP dominance/promote diverse species mix with 33-50% WP. Crop trees, 150 TPA, in block will be pruned.
- Uncut-pruned: No PCT but crop trees identified and pruned
- Uncut, delay PCT by CTR-Pruned: One block will be reserved for PCT by CTR-Pruned in 10 years
- Control: one block with no cutting and not pruned.
Ecosystem Services Forests
Ecosystem Services Treatment Group will have two treatments, about 10 acres each, at each of 3 to 4 locations. The treatments will be:
- PCT by CTR , white pine at 17-ft spacing, 150 TPA. Crop trees will be pruned.
- Uncut control
Measurements on trees and other plants at all PPN locations.
Measurements will use circular plots with a 10 m radius to inventory trees. Three to five permanent plots will be established in each treatment in a Demonstration Forest location (n=6-10 total at each location), five permanent plots will be established in each of the six silvicultural treatments across three replicates (n = 90 total at each location) at the Intensive Measurement Forest locations, and 10 permanent plots will be established in each treatment on the Ecosystem Services Forest locations (n = 20 total at each location). Measurements made during the first summer prior to treatments will provide a reference for evaluating near-term effects of treatments over three to five years. All trees larger than 10 cm DBH will be measured for species diameter, height, crown position, and live crown ratio. Saplings (tree species >1.4 m tall, 0, 1200, and 2400. The percent cover of tree seedlings (< 1.4 m tall), shrubs, vines, forbs and graminoids will be estimated in 1 m2 quadrats nested in the sapling subplots. Additionally, tree seedlings will be tallied by height class:
Symptoms of WPND will be assessed in all sample plots by rating crown symptoms at the end of June (Broders, et al., 2015). Trees will be rated on a numerical scale (0–3), where 0 corresponds to a healthy crown free of signs and symptoms of WPND, 1 infers that ≤1/3 of the crown is affected, 2 infers that 1/3≥2/3 of the crown is affected, and a score of 3 infers that >2/3 of the crown exhibits infection.
Symptomatic needles representative of what is found on a tree will be collected from 10 randomly selected trees in each plot. Collected needles will be incubated in moist chambers, and resulting fungal sporulation will be used to identify the fungal species present[1]. Up to 5 species of fungi are involved in WPND. Another measure of WPND is crown density in August after the infected needles are shed. Overall crown density is estimated in 10% increments (U.S. Department of Agriculture, 2011a).
Severity of Caliciopsis symptoms of resin and damaged bark will be assessed on all sample trees. Symptom severity is measured by counting the number of streaks, up to 10 for each section of the bole (Top=T, M=Middle, B=Bottom), for two opposite sides/faces of the tree (T1 or T2). The counts will be summed for the tree. For older symptoms of bark damage, estimate symptom severity using these categories on each section and side of the bole: “0”=no symptoms, “1”= 1-5% of stem with symptoms, “2”= 5-50% of the stem with symptoms, and “3”= >50% of the stem with symptoms. Scores will be summed for the tree. Presence of any Caliciopsis fruiting structures will be noted for each tree.
Trees will be sampled for the pine bast scale during winter. Five trees, representative of what’s growing, will be harvested in the treated and untreated Ecosystem Services stands. Two living branches will be cut from the lowest whorl on the tree, and the proximal 3 ft of the branch will be examined. Viable scales (small, black, shiny) will be counted on each branch; empty shells of emerged cysts will also be counted as an indicator of levels of previous generations. Careful observation will be made to locate the scale under lichen and inside crevices. Association of scales with whorls, cankers, and lichen will be noted. For a standardized density measure, the number of cysts per unit area of the branch sample will be calculated. Branch sample area is estimated as a truncated cone [A = sπ (r1+r2) + πr12 + πr22]. “s” refers to the length and “r” refers to each radius of the branch section or bolt.
Measurements on ecophysiology. These measurements will occur only on the Intensive Measurement forest plots. The goal of the proposed ecophysiology measurements is to quantify the impact that thinning has on EWP water relations, physiology, and microclimate to determine the effect on tree health and vigor. We hypothesize that although the thinning treatment will temporarily reduce water use and photosynthesis of remaining trees, tree vigor will rapidly increase in the second year following treatment as trees acclimate to the new microclimate conditions. The increased tree vigor and new microclimate conditions are expected to reduce disease severity. To test this hypothesis in each of the two treatments (control and PCT by CTR) located in the Ecosystem Services Treatment Group, 10 trees will be monitored intensively for sap flow using the heat ratio method (Burgess, et al., 2001), the year before treatment and in the three years following treatment. Sensors will be custom made in collaboration with Asbjornsen at the University of New Hampshire. Each sensor contains three probes: one heating probe and two sensor probes that contain thermocouples that can be used to calculate transpiration rate. One sap flow sensor will be installed per tree at breast height by removing bark, drilling pilot holes, and inserting the sensors. Sensors will be insulated and connected to a datalogger and multiplexer (Campbell Scientific, Utah, USA). Based on experience with measuring sap flow in EWP, the high resin content of EWP xylem necessitates that sensors be replaced each year. With these sensors, we will quantify pre, immediate, and longer-term responses of whole tree water flux to thinning at high temporal resolution.
To determine the physiological traits that relate to whole-tree water relations and to expand our results to include the remaining sites, we will conduct a series of leaf and branch physiological tests to quantify how well adapted EWP is to extreme drought and how that response is influenced by thinning. Measurements include maximum photosynthesis (LI-6400 Portable Photosynthesis System, LI-COR), leaf water potential (Scholander Pressure Chamber, PMS Instruments), leaf traits that correspond to drought tolerance (including minimum epidermal conductance, leaf mass, leaf area, and turgor loss point) as well as how those traits contribute to whole branch water dynamics via lab dry-downs (Blackman, et al., 2019). Maximum photosynthesis will be measured on 20 branches from each Ecosystem Services Treatment immediately following the thinning and in the subsequent year to track acclimation to the new light conditions. Water potential will be tracked on these same trees periodically throughout the growing season to quantify the thinning impact on response to summer drought. Leaf and branch physiology will be measured mid-summer on samples from those same trees in the year following treatment and additional leaf samples will be collected from other sites to assess how representative the measurements are of the broader region.
Finally, sap flow and physiological trait data will be linked to the local environmental change by monitoring both available moisture in the soil (HS2, Campbell Scientific, USA) and microclimate conditions (temperature, vapor pressure) within the canopies of trees. Four trees per PCT by CTR treatment will be selected for intensive microclimate measurements and will be tracked over three years. iButton temperature and humidity loggers will be suspended at three different canopy positions in each tree (upper canopy, lower canopy, and ground level) and log every hour (iButton data loggers, DS1923-F5#, Maxim Integrated Products, USA). iButtons will be in protective shields to protect from direct radiation (Wason, et al., 2017) and suspended within the canopy. When the proposed ecophysiological studies are completed, we will identify the mechanistic links between drought resilience, disease severity, and microclimate.
The ecophysiology measurements on EWP will be of interest to others doing research on drought impacts on 5 needle pines, such as studies by Bisbing (2020) on how drought and climate change will impact the health and distribution of high elevation 5 needle pines in Nevada.
Measurements on bird diversity (Objective 3c). Observations will occur only in the Ecosystem Services Treatment forests. To determine whether bird diversity increases in EWP following PCT by CTR, spot mapping will be used to estimate species richness and relative abundance by establishing a grid of transect lines in each site (Robbins, 1970). Distances to foliage- and bark-foraging insectivorous bird species will be estimated to establish densities which will allow for better assessment of changes in these foraging guilds in response to PCT by CTR. Following transect surveys each morning, an individual will be randomly selected from among our mapped foliage- or bark-foraging insectivorous birds. Individuals will be followed for 20-minutes, until they leave the site, or are lost. During this time, all bird behaviors will be recorded in foraging behavioral categories (e.g. searching, attacking) and type of attack (e.g. leaf-gleaning, bark-gleaning) in addition to recording bird species, sex, duration of each behavior, tree substrate (e.g. trunk, branch, foliage) of the behavior, and tree species (Remsen & null Robinson, 1990). Additional new individuals will be observed by selecting a different sex in the same territory, conspecfics in another territory (identified by counter-singing), or a new species until 1:00pm or no new individuals of interest are found (Wood, et al., 2012). From these observations, a time-activity budget will be developed to describe the proportion of time spent by target bird species in each tree species. The proportion of time foraging in EWP will be compared to the other tree species and between the different time periods (before PCT, 1 year after PCT, and 2 years after PCT). Following the PCT by CTR treatment, it is expected that there will be a decrease in the abundance and diversity of bark-foragers (a less rich guild in healthy EWP forest) and increases in abundance and diversity for foliage-gleaners (a more rich guild in healthy EWP forest) associated with the EWP trees remaining in the stand. However, cut EWP trees left in the stand and stressed trees left in the uncut matrix will serve as a resource for saproxylic insects could also be a resource for bark-foraging birds, resulting in a net increase in diversity. EWP preference by a bird species would be indicated by a high positive deviation in foraging frequency and proportion of time spent foraging relative to the importance value for EWP (Holmes & Robinson, 1981).
Measurements on carbon. Sampling will occur in the Ecosystem Services Forests. Biomass estimates will be based on procedures used by Puhlick et al. (2016a). Living, woody biomass will be estimated from DBH measurements with a C concentration estimate of 50%. Herbaceous vegetation will be identified, clipped, and collected from a 2.7 ft2 square plot located 5 ft north of each inventory plot, six total per location. Samples will be oven-dried at 65oC to a constant mass, weighed, and ground to 1/30th in. Subsamples will be analyzed for total C by combustion at 1350oC using a LECO CN-2000. For non-bryophyte sample, C content will be calculated by multiplying the sample’s oven dry mass by its TC estimate. Fine woody debris will be measured along three line transects per inventory plot (Brown, 1974). Downed woody debris and stumps will be measured in 1/50th acre plots, 26 ft radius, placed at the main plot center, and snags will be measured in 1/5th acre plots as described by Puhlick et al. (2016a). Bryophytes and soil organic horizon depth will be measured along six transects in each 1/5th acre plots (Puhlick, et al., 2016b) for a total of 30 observations per plot. Mineral soil samples will be collected beneath the O horizon using a 2 in corer, 1 core near the center of each plot. Stump and root biomass will be estimated using biomass equations from which C storage is estimated (Puhlick, et al., 2016a). A soil pit will be excavated outside of each plot, and a soil scientist will classify the soil profile.
Product and Market Opportunities: White pine market will be assessed by surveying mills to see what they are processing and what they would consider using EWP for if markets were favorable. The market assessment will also consider a broader suite of ecosystem service markets (e.g., carbon) and weigh those benefits relative to timber value. Regional stumpage price and harvesting will be analyzed for the northeast to examine the impact on EWP utilization. Loggers, foresters, and mill management will be surveyed to improve understanding of barriers and opportunities to EWP utilization. Case studies will be developed to deep dive into mills around the region that are milling EWP and/or mill EWP as part of a larger mix of species to better understand their operation, motivation, etc. The environment in areas with high EWP utilization will be compared to areas with low utilization to explore opportunities for EWP utilization in other mills. More specifically, we will examine:
- whether lower growth to harvest ratios are an indicator of strong markets, more intensive management, and more productive forests,
- whether higher growth to harvest ratios are an indicator of weak markets, less deliberate management, and less productive forests.
- the potential for developing a new market for EWP via the manufacturing of cross laminated timber (CLT) panels used in mass timber construction.
Using the USFS FIA database, we will compare growth to harvest ratios across all states within the range of EWP. For each state, we will assess growing stock quality by examining variables such as tree grades, age distribution and regeneration. These state-specific data will be cross-referenced with the number, capacity and procurement pressure of regional EWP primary manufacturing facilities. To examine the relationship between EWP growing stock and procurement pressure from EWP primary manufacturing facilities we will use a GIS-based geospatial analysis of sawmill (other facilities) woodsheds.
- Individual woodshed maps will be digitized in ArcGIS and joined to procurement data from the survey data and other sources. This will allow each woodshed to be weighted based on procurement pressure.
- Once the individual woodsheds are digitized and weighted, they will be converted to rasters and summed in groups to visualize EWP procurement pressure (Anderson, 2011).
Anticipating that viable EWP markets are inadequate across a high proportion of its range, we will conduct a case study in NY to examine the potential to improve EWP market conditions. Given the recent study (Kaboli, 2020) reporting that EWP meets the specifications for CLT panel manufacturing, we will assess the potential of EWP to serve as a feedstock for a burgeoning mass timber industry.
Additional Measurements and Studies.
Additional studies will be encouraged for the Permanent Plot Network, such as changes in insect communities, changes in white pine weevil populations, and measurements on white pine blister rust if present.
[1] Isabel Munck, USDA Forest Service Forest Pathologist, will provide training for identifying WPND fungi.
Measurement of Progress and Results
Outputs
- Research proposals to support establishment forest network of 6-9 demonstration forests of low density management and regeneration in EWP (Objective 1). Comments: If proposals are successful, the forests will be established and biodiversity monitored.
- Research proposals to support establishment of intensive research measurements on tree physiology, insect pests, fungal pathogens, and biodiversity in EWP forests (Objective 2). Comments: If proposals are successful, studies will generate peer-reviewed publications on how low density management of EWP affects tree physiology, insect pests, and fungal pathogens.
- Establishment of the Eastern White Pine Management Institute which will organize a symposium, update a field manual and fact sheets, organize field workshops and webinars, and produce video (Objective 3).
- Research proposals to support studies on eastern white pine product and market opportunities. Comments: If proposals are successful, studies will generate peer-reviewed publications describing differences in product and market opportunities across the range of EWP.
Outcomes or Projected Impacts
- We expect at least 100 foresters and 100 arborists will participate in the symposium and EWPMI activities.
- These professionals will then share the information with another 2,000 clients.
- We expect at least 2,000 copies of a revised manual and fact sheets will be utilized during the first year after they become available to a broader audience.
Milestones
(2022):Symposium on EWP management in spring 2022.(2022):EWPMI established.
(2023):Field manual and fact sheets updated.
(2022):Field workshops and webinars available
(2022):Proposals submitted for funding
(2024):Funding for objectives 1 through 3 are received, and work begins
(2025):Manuscripts submitted for publication
Projected Participation
View Appendix E: ParticipationOutreach Plan
- Peer-reviewed publications will be used to communicate research results. Scientists and students will also attend professional meetings and present results, e.g., Entomological Society of America, American Phytopathological Society, Ecological Society of America, Society of American Foresters.
Eastern White Pine Management Institute:
Improvement of EWP health and productivity will largely depend on actions by professional natural resource professionals, consulting foresters, arborists, and public officials. The professionals will need to understand the issues, problems, and solutions so that the EWP situation can be effectively communicated with landowners. An effective way to engage natural resource professionals is demonstrated by the Northeast Silviculture Institute for Foresters (NSIF): (http://www.northeastsilvicultureinstitute.org/about/)
Through a grant from the USDA Forest Service, State and Private Forestry, the North East State Foresters Association (NEFA), NSIF developed a series of training workshops in graduate level silviculture in 2017 and in 2018. Starting in early 2018, a full online training option with 92 separate training videos filmed at the 2017 training, has also been made available. To date, 596 courses have been completed by 325 natural resource professionals, mostly foresters. The training is delivered by an academic team coordinated by the Universities of New Hampshire and Vermont – drawing on the best experts from the other universities in the region as well as other experts.
An Eastern White Pine Management Institute (EWPMI) can be developed using the NSIF as a model. As a first step, an eastern white pine management symposium will be organized to assemble the current knowledge and to provide natural resource professionals the opportunity to set priorities for EWPMI content and outreach approaches. The EWPMI can also sponsor field workshops that will provide the basis for online content. Once fully implemented, the EWPMI will provide a source of knowledge, training, and recommendations the natural resource professionals can use to help landowners improve the health and productivity of EWP.
For arborists and public officials, all forms of extension outreach and education must be utilized to effectively disseminate EWP health and management information. Increasingly, online platforms are being utilized for extension outreach and education aimed at professional practitioners (Barton, et al., 2017; Robinson & Poling, 2017). The impetus behind this increased use of online formats is varied, but includes: (i) enhanced ability to reach a wider and younger group of stakeholders; (ii) increased use and familiarity with technology among professionals; (iii) growing costs and time associated with creating printed materials and implementing in-person seminars, and; (iv) flexibility in how and when education materials can be utilized. These online platforms include social media (e.g. FaceBook, Instagram and Twitter, etc.), online newsletters and listservs, live webinars, and recorded video (e.g. YouTube channels). These formats have allowed extension personnel to expand their exposure to stakeholders that prefer online learning modes (Guldin, 2018). Additionally, online formats can complement traditional forms of communication, like peer-reviewed journals, in-person seminars and hands-on training workshops. The COVID-19 pandemic has only accelerated this transition to online learning as in-person meetings have been drastically reduced. The lack of in-person seminars and training workshops has hindered the ability of practitioners to obtain both continuing education credits (CEUs) and pesticide credits necessary to maintain applicator licenses. Established extension webinar series have slowly cultivated a growing audience with a wide geographic viewership (Dettenmaier & Locklear, 2018) and have been poised to capture stakeholders seeking education and outreach.
USDA RREA-EFF funding will support the development of the EWPMI. There will be a symposium on “Synthesis of Knowledge and Developing Priorities for Eastern White Pine Health and Management” in March 2022. Topics will include health issues, management of EWP in natural systems, and management of EWP at the rural/urban interface. Speakers will be experts on EWP health and professionals who have extensive experience in managing EWP. Twelve speakers will be invited, and the lodging and food expenses will be paid. Speakers will be expected to submit a paper with their talk, and papers will be published in a proceedings by the University of Maine Center for Research on Sustainable Forests (CRSF). Speakers will be invited to talk on white pine weevil and scale, white pine blister rust, Caliciopsis canker, white pine needle damage, white pine decline, common shade tree issues, shelterwood management of EWP, group selection management of EWP, use of fire in management of EWP, low density management of EWP, and shade tree management of EWP. Stakeholders will have the opportunity to provide feedback on the priorities on what knowledge is most needed for EWP management and the most preferred modes for outreach. Symposium participants will be given a survey at the conclusion that will ask for feedback on evaluations. The stakeholders can indicate which information is most helpful in their jobs of working with landowners, and in what format the information should be presented for training and for landowner education.
The EWPMI will be organized based on a web site hosted by UNH. The web site will host downloadable print resources (field manuals, fact sheets, videos), calendar of events (workshops, EWP topics at other professional meetings, webinars), membership list, and online training. Stakeholders can register with the institute to keep track of training records and earning of continuing education credits needed for various licenses. The EWPMI will coordinate the development of a Field Manual Revision (Livingston, et al., 2019) and fact sheets. The online institute will also facility offerings of field workshops and webinars. Professional photographers will be used to record symposium and field workshop events to create about 20 minutes of video. Some additional field shots will be made to supplement what is recorded in the scheduled meetings. The professional recordings will be used to create interest in the EWPMI institute goals and resources. Recordings of symposium talks, workshops, and webinars will also be provided.
Organization/Governance
The project will use the standard form of governance. Participating institution will send at least one representative to an annual meeting, and meeting attendees will be considered the Technical Committee. The meeting will be held in conjunction with another professional meeting or workshop, such as the Northeast Forest Pest Council or the Southern Forest Insect Work Conference. The Technical Committee will elect officers of chair, chair-elect, and secretary at the annual meeting. The chair-elect normally succeeds to chair the following year. Each officer is eligible for re-election. Sub-committees focused on particular objectives will confer as needed by teleconference to plan and coordinate details of the multi-state project.
One sub-committee to be established is one for the EWPMI. Membership in the EWPMI sub-committee is made by request to the Chair of the Technical Committee. Members of the EWPMI sub-committee will select a chair, and committee members will provide an advisory group for operations of the service. There will be synergistic relationship between NE 2101 and the EWPMI will because:
Literature Cited
U.S. Department of Agriculture and U. S. Department of the Interior, 1994. Record of Decision on Management of Habitat for Late-successional and Old-growth Forest Related Species within the Range of the Northern Spotted Owl [Northwest Forest Plan]., Portland: s.n.
Ashton, M. S. & Kelty, M. J., 2018. The Practice of Silviculture: Applied Forest Ecology. 10th ed. Hoboken, NJ: Wiley.
Åström, M., Pettersson, L. B., Öckinger, E. & Hedin, J., 2013. Habitat preferences and conservation of the marbled jewel beetle Poecilonota variolosa (Buprestidae). Journal of Insect Conservation, Volume 17, pp. 1145-1154.
Barton, E. et al., 2017. Using technology to enhance extension education and outreach. HortTechnology, Volume 27, p. 177–186.
Bergdahl, A. et al., 2020. Monitoring Eastern White Pine Decline and its Causes in New England and New York: NE-IM-17-04, Raleigh, NC: USDA Forest Service, Forest Health Monitoring Workshop (poster).
Bisbing, S. A., 2020. Quantifying thresholds and predicting changes in great basin forest community stability under drought and ongoing climate change, s.l.: CRIS PROJ NO NEV05296.
Blackman, C. J. et al., 2019. Drought response strategies and hydraulic traits contribute to mechanistic understanding of plant dry-down to hydraulic failure. Tree Physiology, 6, Volume 39, p. 910–924.
Broders, K. et al., 2015. Characterization of Fungal Pathogens Associated with White Pine Needle Damage (WPND) in Northeastern North America. Forests, Volume 6, pp. 4088-4104.
Brown, J., 1974. Handbook for inventorying downed woody material.. s.l.:USDA Forest Service Gen. Tech. Rep. INT-16..
Burgess, S. S. et al., 2001. An improved heat pulse method to measure low and reverse rates of sap flow in woody plants. Tree physiology, Volume 21, pp. 589-598.
Burton, J., Ares, A., Olson, D. & Puettmann, K., 2013. Management trade-off between aboveground carbon storage and understory plant species richness in temperate forests. Ecological Applications, Volume 23, p. 1297–1310.
Burton, J. I., Mladenoff, D. J., Clayton, M. K. & Forrester, J. A., 2011. The roles of environmental filtering and colonization in the fine-scale spatial patterning of ground-layer plant communities in north temperate deciduous forests. Journal of Ecology, Volume 99, pp. 764-776.
Chhin, S. O., 2018. Quantitative forest management to build adaptive capacity, s.l.: CRIS PROJ NO WVA00808.
Cornelissen, T., Fernandes, G. W. & Vasconcellos-Neto, J., 2008. Size does matter: variation in herbivory between and within plants and the plant vigor hypothesis. Oikos, Volume 117, p. 1121–1130.
Costanza, K. K., Livingston, W. H., Fraver, S. & Munck, I. A., 2020. Dendrochronological analyses and whole tree dissections reveal Caliciopsis canker (Caliciopsis pinea) damage associated with declining growth and climatic stressors of eastern white pine (Pinus strobus). Forests, 11(347), p. doi:10.3390/f11030347.
Costanza, K. K. L. et al., 2018. A synthesis of emerging health issues of eastern white pine (Pinus strobus) in eastern North America. Forest Ecology and Management, Volume 423, pp. 3-17.
Davis, L. & Puettmann, K., 2009. Initial response of understory vegetation to three alternative thinning treatments. Journal of Sustainable Forestry, Volume 28, p. 904–934.
Dettenmaier, M. & Locklear, J., 2018. Start-to-finish techniques regarding the practicalities of producing purposeful and impactful webinars. Journal of Extension, Volume 56, p. 2.
Dirr, M. & Warren, K., 2020. The Tree Book. Portland(OR): Timber Press, Portland, OR.
Elton, C. S., 1966. The pattern of animal communities. s.l.:Wiley, New York.
Fraver, S., Ringvall, A. & Jonsson, B. G., 2007. Refining volume estimates of down woody debris. Canadian Journal of Forest Research, 3, Volume 37, pp. 627-633.
Germain, R., 2015. Sustaining white pine on high quality sites, s.l.: CRIS PROJ NO NYZ265520027.
Germain, R. H., Nowak, C. A. & Wagner, J. E., 2016. The Tree That Built America—Not Making the Grade. Journal of Forestry, Volume 114, pp. 552-561.
Gilliam, F., 2014. The herbaceous layer in forests of eastern North America. New York, NY USA: Oxford University Press.
Gleason, K. E. et al., 2017. Competition amplifies drought stress in forests across broad climatic and compositional gradients. Ecosphere, Volume 8, p. e01849.
Guldin, R., 2018. How today’s professionals prefer to find the science they need to do their jobs. Journal of Forestry, Volume 116, p. 451–459.
Halpern, C. & Spies, T., 1995. Plant species diversity in natural and managed forests of the Pacific Northwest. Ecological Applications, Volume 5, p. 913–934.
Harmon, M. E., 2011. Differences between standing and downed dead tree wood density reduction factors : a comparison across decay classes and tree species. s.l.:U.S. Dept. of Agriculture, Forest Service, Northern Research Station, NRS-15.
Holmes, R. & Robinson, S., 1981. Preferences of foraging insectivorous birds in a northern hardwoods forest. Oecologia, Volume 48, p. 31–35.
Huston, M. A., 1996. Modeling and management implications of coarse woody debris impacts on biodiversity.. In: McMinn, J., Crossley, D.A. (Eds.), Biodiversity and Coarse Woody Debris in Southern Forests. USDA Forest Service General Technical Report SE-94, Athens, GA.. Asheville, N.C: Southern Research Station.
Jonsell, M. & Hansson, J., 2007. Comparison of methods for sampling saproxylic beetles in fine wood. Entomologica Fennica, Volume 18, p. 232–241.
Kane, B., Mcelhinney, A. & Harper, R., 2008. Planting for Resilience: Selecting Urban Trees in Massachusetts. Urban Forestry & Urban Greening, Volume 7, p. 15–23.
Kerchner, C. D. & Keeton, W. S., 2015. California's regulatory forest carbon market: Viability for northeast landowners. Forest Policy and Economics, 1, Volume 50, pp. 70-81.
Kinn, D. N., 1986. Studies on the flight capabilities of dendroctonus frontalis and IPS calligraphus: preliminary findings using tethered beetles. s.l.:Research Note SO-324 USDA, Forest Service, Southern Forest Experiment Station. 3p..
Koricheva, J., Larsson, S. & Haukioja, E., 1998. Insect performance on experimentally stressed woody plants: a meta-analysis. Annual Review of Entomology, Volume 43, p. 195–216.
Langston, N., 2007. The history of adaptive management in wisconsin forestry, s.l.: CRIS PROJ NO WIS04781.
Leak, W. B. & Lamson, N. I., 1999. Revised white pine stocking guide for managed stands., s.l.: USDA Forest Service, Northeastern Area State and Private Forestry. NA-TP-01-99. 2 p..
Lee, T., 2015. Assessing and limiting the impact of invasive shrubs on tree growth and regeneration in eastern white pine - hardwood forest, s.l.: CRIS PROJ NO NH00069M.
Livingston, W. H. & Kenefic, L. S., 2018. Low densities in white pine stands reduce risk of drought-incited decline. Forest Ecology and Management, Volume 423, pp. 84-93.
Livingston, W. H. et al., 2019. Field Manual for Managing Eastern White Pine Health in New England., Orono, ME: University of Maine, Maine Agricultural and Forest Experiment Station, MP764.
Lorimer, C. & White, A., 2003. Scale and frequency of natural disturbances in the northeastern US: implications for early successional forest habitats and regional age distributions. Forest Ecology and Management, Volume 185, p. 41–64.
MacLean & Clouston, P. E., 2020. Accounting for carbon: the potential of new and innovative technology to store forest carbon and allow for new growth, s.l.: CRIS PROJ NO MAS00042.
Maguire, D. A., Kershaw, J. A. J. & Hann, D. W., 1991. Predicting the effects of silvicultural regime on branch size and crown wood core in Douglas-fir. Forest Science, 11, Volume 37, pp. 1409-1428.
Maier, C. A. & Teskey, R. O., 1992. Internal and external control of net photosynthesis and stomatal conductance of mature eastern white pine (Pinusstrobus). Canadian Journal of Forest Research, 9, Volume 22, p. 1387–1394.
McElhinney, A. M. & Harper, R. W., 2019. Planting for Resilience: Selecting Urban Trees in Massachusetts.. [Online]
Available at: http://www.urbanforestrytoday.org/publications.html
[Accessed 26 June 2020].
McIntire, C. D., Munck, I. A., Ducey, M. J. & Asbjornsen, H., 2018. Thinning treatments reduce severity of foliar pathogens in eastern white pine. Forest Ecology and Management, Volume 423, pp. 106-113.
Mech, A. M. et al., 2013. Matsucoccus macrocicatrices (Hemiptera: Matsucoccidae): First Report, Distribution, and Association With Symptomatic Eastern White Pine in the Southeastern United States. Journal of Economic Entomology, Volume 106, pp. 2391-2398.
Miller, G. W., Stringer, J. W. & Mercker, D. C., 2007. Technical Guide to Crop Tree Release in Hardwood Forests, Knoxville, TN: University of Tennessee Extension Publication PB1774.
Müller, J., Noss, R. F., Bussler, H. & Brandl, R., 2010. Learning from a “benign neglect strategy” in a national park: Response of saproxylic beetles to dead wood accumulation. Biological Conservation, Volume 143, pp. 2559-2569.
Munck, I. et al., 2015. Extent and Severity of Caliciopsis Canker in New England, USA: An Emerging Disease of Eastern White Pine (Pinus strobus L.). Forests, Volume 6, pp. 4360-4373.
Munck, I. et al., 2016. Soil and Stocking Effects on Caliciopsis Canker of Pinus strobus L. Forests, Volume 7, p. 269.
Nunery, J. S. & Keeton, W. S., 2010. Forest carbon storage in the northeastern United States: Net effects of harvesting frequency, post-harvest retention, and wood products. Forest Ecology and Management, 3, Volume 259, pp. 1363-1375.
O'Hara, K. L., Grand, L. A. & Whitcomb, A. A., 2010. Pruning reduces blister rust in sugar pine with minimal effects on tree growth. California Agriculture, Volume 64, pp. 31-36.
O'Hara, K. L., Larvik, D. A. & Valappil, N. I., 1995. Pruning costs for four northern Rocky Mountain species with three equipment combinations. Western Journal of Applied Forestry, 4, Volume 10, pp. 59-65.
Orrock, J. L. & Connolly, M., 2021. Optimizing sustainable management of midwestern forests by identifying context-specific limits to tree and plant recruitment, s.l.: CRIS PROJ NO WISW202005111.
Platt, W., 1975. The Colonization and Formation of Equilibrium Plant Species Associations on Badger Disturbances in a Tall-Grass Prairie. Ecological Monographs, Volume 45, p. 285–305.
Prasad, A., Iverson, L., Peters, M. & Matthews, S., 2014. Climate change tree atlas. Northern Research Station, U.S. Forest Service, Delaware, OH.. [Online]
Available at: http://www.nrs.fs.fed.us/atlas
[Accessed 31 march 2020].
Price, P., 1991. The plant vigor hypothesis and herbivore attack. Oikos, Volume 62, p. 244–251.
Puhlick, J. J. et al., 2016a. Long-term influence of alternative forest management treatments on total ecosystem and wood product carbon storage. Canadian Journal of Forest Research, 1, Volume 46, pp. 1404-1412.
Puhlick, J. J. et al., 2016b. Factors influencing organic-horizon carbon pools in mixed-species stands of central Maine, USA. Forest Ecology and Management, 3, Volume 364, pp. 90-100.
Remsen, J. & null Robinson, 1990. A classification scheme for foraging behavior of birds in terrestrial habitats. Studies in Avian Biology, Volume 12, p. 144–160.
Rhoades, D., 1983. Variable plants and herbivores in natural and managed systems. New York: Academic.
Robbins, C., 1970. An international standard for a mapping method in bird census work. International Bird Census Committee. Audubon Field Notes, Volume 24, p. 722–726.
Robinson, J. & Poling, M., 2017. Engaging participants without leaving the office: planning and conducting effective webinars. Journal of Extension, Volume 55, p. 9.
Rosenberg, K. et al., 2019. Decline of the North American avifauna. Science, Volume 366, p. 120–124.
Russell, M. B. et al., 2014. Residence Times and Decay Rates of Downed Woody Debris Biomass/Carbon in Eastern US Forests. Ecosystems, 8, Volume 17, pp. 765-777.
Schmitt-Harsh, M. et al., 2013. Private residential urban forest structure and carbon storage in a moderate-sized urban area in the Midwest. Urban Forestry & Urban Greening, Volume 12, p. 454–463.
Schulz, A. N. et al., 2018. Assessment of abiotic and biotic factors associated with eastern white pine (Pinus strobus L.) dieback in the Southern Appalachian Mountains. Forest Ecology and Management, Volume 423, pp. 59-69.
Schulz, A. N. et al., 2018b. Association of Caliciopsis pinea Peck and Matsucoccus macrocicatrices Richards with eastern white pine (Pinus strobus L.) seedling dieback. Forest Ecology and Management, Volume 423, pp. 70-83.
Seymour, R., 2018. Silviculture and production ecology of the Acadian forest, s.l.: CRIS PROJ NO ME0M80052913.
Seymour, R. & Hunter, M., 1999. Principles of Ecological Forestry. In: M. L. Hunter, ed. Maintaining Biodiversity in Forest Ecosystems. Cambridge: Cambridge University Press, p. 499–524.
Seymour, R. S., 2007. Low-Density Management of White Pine Crop Trees: A Primer and Early Research Results. Northern Journal of Applied Forestry, Volume 24, pp. 301-306.
Seymour, R. S., Guiterman, C. H., Weiskittel, A. R. & Kimball, A. J., 2009. Growth, yield, and financial returns of eastern white pine under contrasting silvicultural systems: low-density crop-tree management vs. conventional thinning., Durham, NH: Northeastern States Research Cooperative, USDA Forest Service..
Simonin, K., Kolb, T. E., Montes-Helu, M. & Koch, G. W., 2006. Restoration thinning and influence of tree size and leaf area to sapwood area ratio on water relations of Pinus ponderosa. Tree Physiology, 4, Volume 26, p. 493–503.
Swanson, M. et al., 2011. The forgotten stage of forest succession: early-successional ecosystems on forest sites. Frontiers in Ecology and the Environment, Volume 9, p. 117–125.
Trentini, C. et al., 2017. Thinning of loblolly pine plantations in subtropical Argentina: Impact on microclimate and understory vegetation. Forest Ecology and Management, Volume 384, p. 236–247.
U.S. Department of Agriculture, F. S., 2011a. Field Methods for Forest Health (Phase 3) Measurements, Crowns: Measurements and Sampling. [Online]
Available at: https://www.fia.fs.fed.us/library/field-guides-methods-proc/index.php
[Accessed 15 March 2020].
U.S. Department of Agriculture, F. S., 2011b. Field Methods for Forest Health (Phase 3) Measurements, Soil Measurements and Sampling. [Online]
Available at: https://www.fia.fs.fed.us/library/field-guides-methods-proc/index.php
[Accessed 15 March 2020].
U.S. Department of Agriculture, F. S., 2020. March 13, 2020. Forest Inventory and Analysis Database, St. Paul, MN: U.S. Department of Agriculture, Forest Service, Northern Research Station. [Available only on internet: https://apps.fs.usda.gov/fia/datamart/datamart.html]. [Online]
Available at: https://apps.fs.usda.gov/fia/datamart/datamart.html
[Accessed 13 March 2020].
Wason, J. W., Bevilacqua, E. & Dovciak, M., 2017. Climates on the move: Implications of climate warming for species distributions in mountains of the northeastern United States. Agricultural and Forest Meteorology, 11, Volume 246, p. 272–280.
Weiskittel, A., Kenefic, L., Seymour, R. & Phillips, L., 2009. Long-term effects of precommercial thinning on the stem dimensions, form and branch characteristics of red spruce and balsam fir crop trees in Maine, USA. Silva Fennica, Volume 43.
Wick, R. & Brazee, N. I., 2019. Bursaphelenchus antoniae; evaluation of pathogenicity to several native American Pinus spp. and investigation of its prevalence in southern New England, s.l.: CRIS PROJ NO MAS00039.
Wood, E., Pidgeon, A., Liu, F. & Mladenoff, D., 2012. Birds see the trees inside the forest: the potential impacts of changes in forest composition on songbirds during spring migration. Forest Ecology and Management, Volume 280, p. 176–186.
Wyka, S. A. et al., 2018. Effect of Climatic Variables on Abundance and Dispersal of Lecanosticta acicola Spores and Their Impact on Defoliation on Eastern White Pine. Phytopathology, Volume 108, pp. 374-383.
Wyka, S. A. et al., 2017. Emergence of white pine needle damage in the northeastern United States is associated with changes in pathogen pressure in response to climate change. Global Change Biology, Volume 23, pp. 394-405.
Zhang, Q., Byers, J. & Zhang, X., 1993. Influence of bark thickness, trunk diameter and height on reproduction of the longhorned beetle, Monochamus sutor (Col., Cerambycidae) in burned larch and pine. Journal of Applied Entomology, Volume 115, p. 145–154.