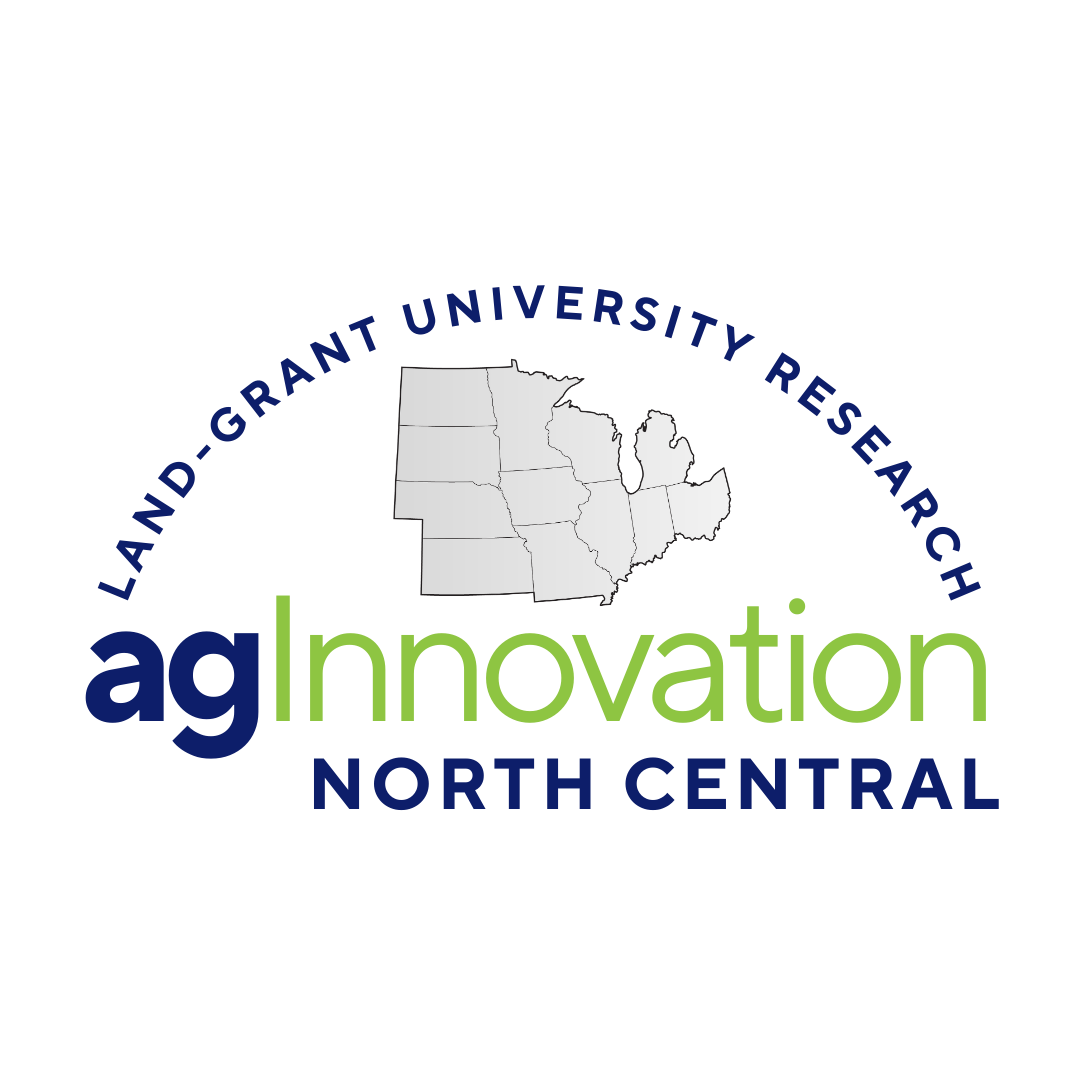
NC140: IMPROVING ECONOMIC AND ENVIRONMENTAL SUSTAINABILITY IN TREE-FRUIT PRODUCTION THROUGH CHANGES IN ROOTSTOCK USE
(Multistate Research Project)
Status: Active
NC140: IMPROVING ECONOMIC AND ENVIRONMENTAL SUSTAINABILITY IN TREE-FRUIT PRODUCTION THROUGH CHANGES IN ROOTSTOCK USE
Duration: 10/01/2022 to 09/30/2027
Administrative Advisor(s):
NIFA Reps:
Non-Technical Summary
Statement of Issues and Justification
STATEMENT OF ISSUES AND JUSTIFICATION:
The NC-140 Regional Research Project addresses economically and environmentally sustainable improvement in temperate fruit production by focusing on rootstocks and root systems. The project meets the guidelines presented by the North Central Regional Association (NCRA) in Guidelines for Multistate Research Activities (August 2017) by addressing high priorities within the crosscutting research areas of agricultural production, processing, distribution, genetic resource development and manipulation, integrated pest management, and economic development and policy. The project involves researchers and extension specialists from multiple disciplines in multiple states as well as international collaborators. Researchers involved in this project have leveraged federal and state dollars to add significant financial and in-kind resources to address this important research area. Outreach is integrated within the project and includes electronic information transfer through web sites, written material for growers and other stakeholder groups, on-farm demonstrations, and numerous diverse educational programs conducted at local, national, and international levels for stakeholders and peer scientists.
Needs Identified by Stakeholders
The needs of the stakeholders addressed in this project have been identified through periodic regional and national sources and surveys. Many of the members of this project have Cooperative Extension appointments and serve as educational liaisons with the tree fruit industries in their respective states working directly with their stakeholders to identify and address needs. Additionally, through the NIFA-USDA-SCRI and USDA-SCBG programs, multiple meetings have been held with tree fruit representatives, including growers, allied industries such as packing houses and nurseries, researchers, and extension specialists. Through this process, a list of stakeholder research and extension priorities has been compiled and updated periodically. Among the critical needs identified were the identification of rootstocks with specific traits that: optimize production of consumer-preferred varieties like 'Honeycrisp' apple; facilitate labor- and input-efficient orchard systems; and can alleviate problematic root and soil interactions (e.g., replant disease, high pH, etc.). As residential developmental pressure on desirable orchard land (and its associated upward pressure on land prices) continues to increase, sustaining fruit production in some regions often requires establishing new orchards in sites with less-than-ideal soil conditions. All these needs are encompassed in the updated NC-140 objectives.
Importance of the Work and Consequences If It Is Not Done
Tree-fruit growers must adopt economically and environmentally sustainable orchard management strategies to remain competitive in regional, national, and international markets; to meet consumer demand for high quality fruit; to address the pressure to reduce chemical use; and to enhance production efficiency. The root system is a key orchard component that can impact these issues, and tree fruit growers address these issues by utilizing the genetic traits of rootstocks, onto which the fruiting varieties are grafted. Rootstocks can provide a range of tree vigor control and final tree size when matched to optimize local soil and climatic conditions, allowing for higher density plantings with smaller trees that are more efficient per unit of land area. Higher density plantings facilitate earlier production and greater cumulative yield potentials, but also must provide appropriate vigor for the site so that canopy shade and pruning do not become excessive. To be profitable, growers must establish high-density orchards with appropriate rootstocks grafted to cultivars that are in demand by consumers and have high market value. However, establishment costs for high-density orchards are 10 to 20 times more per land area than lower-density plantings, thus greatly increasing economic risk. Grafting to a rootstock inappropriate for the regional climate or orchard soil type can be economically disastrous. Potential economic returns from high-density orchards can far exceed that of low-density orchards, particularly during the first 10 years. Past NC-140 research successfully identified reliable size-controlling, early-bearing rootstocks for apple and cherry, and has led to the integration of their use in high-density production systems to reduce tree size, labor costs and significant tree and/or production losses from disease and environmental stresses. Size-controlling rootstocks for peaches, pears, apricots, and plums are now in their relatively early stages of development, concomitant with potentially major changes in tree training as they are integrated into more efficient and productive orchard systems.
In addition to tree size, the rootstock profoundly affects canopy training strategies that impact fruit quality, pest and disease resistance, labor efficiency, and amenability to mechanization technologies, as well as adaptability to different soil types, stress tolerance, and ultimately sustainable productivity and profitability. Among these, potential advances in orchard mechanization technologies have changed dramatically in the past 5 years, with the introduction of imaging technologies for orchard mapping, crop load quantification, plant stress detection, selective "smart" spray applications, and even robotic harvesters. The precision of such technologies is tied closely to smaller, simpler tree canopies facilitated by vigor-controlling rootstocks. Yet, many commercially available rootstocks also have inherent weaknesses and have not been evaluated thoroughly for potential problems in different production environments. Tree losses due to low temperature injury, disease, scion incompatibility, and adverse soil conditions are an economic cost for the industry that can be ameliorated by improving rootstock genotype knowledge and options.
Furthermore, rootstock resiliency to changing climate and more frequent extreme climatic events is an increasing concern. Over the past 5 years, many production regions have experienced both periodic drought conditions as well as excessive rainfall events that cause temporary flooding. Where drought conditions have persisted even across years, irrigation water availability increasingly may be curtailed. Tree fruit growers and nurseries demand timely research that anticipates and solves production problems and provides information that can prevent costly mistakes, given the long-term (25+ year) investment for establishing orchards and new orchard technologies. A warming climate has been manifested in later leaf senescence in the fall and earlier budbreak in the spring, subjecting temperate zone tree fruits to increased risk of tissue damage from fall and spring freeze events. Similarly, the more variable climate can negatively affect cold acclimation and de-acclimation as well as the winter chilling hour accumulation required for the transition of endormancy to ecodormancy, subsequently affecting healthy bud break and flowering in spring. Rootstock genotype can significantly influence these canopy responses to the environment, and evaluation of rootstocks must place a greater emphasis on characterizing these responses across North America's diverse tree fruit production regions.
This next phase of the NC-140 project will continue to identify and evaluate rootstocks for critical genetic traits that include size-control and adaptation to different soils and climates. Project research will lead the evolution of orchard systems as new rootstocks are integrated into orchard strategies and technologies to increase production, reduce labor, improve fruit quality and yields, and facilitate environmental sustainability across the range of temperate zone tree fruit production.
Technical Feasibility
Success with new orchard systems depends upon reliable research-based recommendations for a wide range of production conditions that can only be accomplished with coordinated multistate research. Since the inception of the NC-140 project, North American tree fruit growers have received critical rootstock recommendations that have transformed their industry into one of the most productive in the world. New pome- and stone-fruit rootstock recommendations are based upon multi-site research investigations of soil and climatic adaptability, root anchorage, size-control, precocity, productivity, and pest resistance. Tree fruit are long-lived perennials, so a minimum of eight years is necessary to develop a thorough understanding of rootstock performance and to accurately assess the potential for improved profitability, reduction of grower inputs, and enhancement of production efficiency. With multi-state research, new rootstocks are quickly and systematically exposed to widely varying soil and climatic conditions which shortens the time necessary for a thorough evaluation and the development of site-specific recommendations across growing environments. Consequently, the vast majority of growers rely on research-based NC-140 recommendations as their primary resource for rootstock selection.
Major innovations in orchard systems have occurred over the past fifteen years to address the increasingly high cost and shortage of labor, and to improve orchard yields and returns-on-investment. Using size-controlling rootstocks, apple and cherry growers have adopted high-density orchard systems that are evolving to facilitate efficiencies in orchard management, including tree maintenance operations from the ground or mobile platforms, eliminating dangerous and expensive use of ladders. Expanded mechanization is being coupled experimentally with new imaging, sensing, data acquisition, and artificial intelligence (machine learning) technologies, for greater precision in orchard management decision-making as well as potential autonomous task implementation, including robotics for selective spraying, pruning and harvesting. The successful implementation of such technologies is strongly influenced by the simplification of high density orchard systems on appropriate rootstocks. NC-140 researchers are critical partners with faculty and industry colleagues in agricultural biosystems and computer engineering for these on-going technological developments.
If the U.S. temperate zone tree fruit industry is going to remain competitive in international markets as well as meet increasing domestic and local consumer demands, new improved genetic materials will need to be identified, tested, and adopted to address emerging problems. Through traditional plant breeding methods and novel genomic tools, NC-140 (and other) researchers have incrementally incorporated insect and disease resistance into existing rootstock germplasm, as well as developed rootstocks with enhanced horticultural performance and tolerance to environmental stresses. Obtaining putatively improved rootstock genotypes from research programs throughout the world for testing by NC-140 cooperators has been an integral part of the project. Promising new clonal rootstocks for apple, pear, peach, apricot, plum, and cherry have been obtained from domestic and international breeding programs. Development and use of genetic markers can speed up selection, but depend on coordinated evaluation of economically important traits in multiple environments. Meanwhile, rootstock breeding programs have generated many new elite selections that may show differential adaptation to specific regions of North America. These new rootstocks require coordinated testing under diverse North American climates and soil types, with training and pruning techniques modified to optimize rootstock traits with local growing conditions.
Advantages of a Multistate Effort
Collaborative research by the NC-140 project team has demonstrated that tree fruit rootstock growth characteristics can differ widely across North American production regions. Low or high temperatures, soil conditions, and susceptibility to endemic pests can limit the adaptability of some rootstocks in certain regions. A greater understanding of stress factors and the physiological mechanisms behind these responses, as well as rapid screening of genotypes for susceptibility or tolerance to biotic and abiotic stress factors, will improve tree survival and sustain productivity in diverse soils and climates.
Outreach is integral to the NC-140 project. Using regionally-focused extension web sites, NC-140 members continually expand the digital availability of rootstock information. Associated outreach efforts include written materials for growers and other stakeholder groups, as well as video and on-site educational programs in individual states and at national and international grower and scientific meetings. On-farm research and demonstration trials, including farm tours and field days, are extremely effective for disseminating NC-140 research results and recommended new techniques and technologies directly to growers.
Collaborative, coordinated evaluation of new and existing rootstocks by NC-140 researchers continues to generate financial support from fruit and nursery industry partnerships for propagation and establishment of test plantings. Individual researchers use industry support as seed money to seek and leverage state, federal and private foundation grants (competitive and non-competitive) for associated rootstock studies. It is estimated that during the current project period (2017-2022), approximately $2,000,000 has been generated to support NC-140 research as seed funding/matching and/or direct support from sources other than universities, Hatch funds, and RRF funds, and more than half of this total comes from grower organizations. Additionally, more than $7 million in USDA-SCRI funding has been procured during this period by teams that include NC-140 members for intensive apple and cherry studies that are essentially outgrowths of existing NC-140 trials that have served as unique research resources.
Likely Impacts
As evidenced in the previous work and performance of this project, the proposed and on-going research will enhance the economic viability of tree fruit growers through improved selection of rootstocks, input-efficient rootstock management practices, and development of rootstock-facilitated orchard technologies that result in greater production efficiencies and improved fruit quality. Orchard labor and land will be utilized more efficiently, with higher sustainable yields and fewer tree losses to pests and environmental stresses, leading to a faster and greater return on investment.
The need to initiate new coordinated research studies across diverse growing environments for temperate-zone tree fruit rootstocks is compelling, as new genetic materials are developed or made available, and societal (labor availability) and climatic (changing) pressures increase for producers. Successful utilization of new rootstocks can require, or facilitate, significant changes in orchard cultural practices. Continued thorough evaluation of promising rootstocks, multiple genetic systems, and associated new mechanical and computer-aided technologies is critical for successful implementation of planting and training system efficiencies. The NC-140 research project will continue to develop research-based recommendations for growers and nurseries that are based on extensive and collaborative multi-state work, providing an increased understanding of rootstock adaptability and performance for economically and environmentally sustainable production.
Related, Current and Previous Work
Rootstocks have been used to improve the performance of desirable fruit tree cultivars for thousands of years, with the earliest documentation in 412 B.C. (Mudge et al., 2009). With on-going advances in new orchard technologies (including autonomous equipment and robotics) and dynamic industry cost and market factors, the continued development of improved rootstocks will be critical to the future economic sustainability of North America’s tree fruit industries (Elkins et al., 2012; Reighard, 2013; Lang, 2019; Lang and Whiting, 2021). Stakeholder profitability will depend on establishment of new orchards that are uniform, yield efficient, stress tolerant and markedly less reliant on increasingly expensive and difficult-to-source labor; subsequently, new orchards must be developed and managed to capture sunlight efficiently and be compatible with automation and mechanization technologies (Robinson, 2008; Lang et al., 2019; Zhang et al., 2020; Jiang and He, 2021).
Indeed, orchard systems have become highly structured and increasingly intensive over a relatively short time span for apple (Dorigoni et al., 2011; Robinson et al., 2011), pear (Robinson and Dominguez, 2015), and sweet cherry (Du et al., 2012; Lang et al., 2014; Long et al., 2015; Musacchi et al., 2015; Lang et al., 2019). These innovative and profitable systems both capitalize, and depend, on the use of specific rootstock genotypes to confer precocity, improve flowering habits (Maguylo et al., 2004), alter ripening and fruit quality (Marini et al., 2008) and impart dwarfing (Robinson et al., 2003; Vercammen, 2004) to the scion. No single rootstock is widely adapted to the diverse soil and climatic conditions of North America; therefore, a suite of new rootstocks is required to provide alternatives for new cultivars and emerging production challenges (e.g., labor, environmental sustainability [replant fumigants], pest and disease threats, climate change, etc.). The role of the NC-140 project is to develop and/or acquire, and objectively and widely evaluate, the performance of novel rootstocks via coordinated, long-term field trials comprising diverse sites, modern training systems and commercially-important cultivars with emphases on production efficiency, disease resistance and stress tolerance/avoidance.
Such trials have become increasingly complex as orchard production systems evolve to higher densities (introducing root competition physiological factors) and more precise structures (introducing additional horticultural intervention factors). Intensive orchard systems are rapidly evolving towards two-dimensional (planar, continuous fruiting wall) canopy architectures comprised of minimized structural wood, simplified fruiting units, and optimized leaf area (for high light interception with minimal shade). Simplified planar canopy structures are more labor-efficient as well as more readily facilitate mechanization, orchard imaging, and potential robotic/autonomous orchard technologies (Lang and Whiting, 2021). The interaction between rootstocks and intensive orchards has led to the recognition that rootstock evaluation trials must also evolve, with more complex designs for interactive evaluations to provide meaningful information to growers in diverse growing regions. This is especially challenging for tree fruits such as peaches for which orchard systems have evolved in very minor increments due to a lack of vigor-controlling rootstocks. With the recent advent of new peach rootstocks that exhibit significant vigor control (e.g., DeJong et al., 2011; Beckman and Chaparro, 2015; Hilaire et al., 2021), evaluation of new rootstock traits suitable for modern orchards must occur concomitantly as the modern orchard systems are being developed and refined.
The value of the traditional rootstock trait/scion interactive trait known as "yield efficiency", as determined by yield/trunk cross-sectional area (TCSA), is essentially limited to the timeframe during which trees are filling their allotted space in the orchard training system (Westwood and Roberts, 1970); once the viable leaf area for that space has been reached, rootstock-influenced genetic yield potential has likely been reached as well (Lang and Shane, 202x). After this point in orchard tree development, horticultural interventions (i.e., pruning to contain the canopy within its space and to renew fruiting sites) can increasingly influence yield even as TCSA continues to increase for the life of the orchard (thus likely decreasing the "yield efficiency" value), with rootstock genetics influencing the degree of horticultural intervention required more than its inherent yield potential. Thus, NC-140 uniform evaluation trials designed to also incorporate contemporary (usually high density and, increasingly, narrow fruiting wall canopies) must utilize a new parameter or parameters to quantify and explain rootstock effects on yield potential that reflect both direct (partitioning to reproductive outputs) and indirect (vegetative growth suitability for the training system) traits. One such parameter to be implemented in the 2022-2027 project work is to modify the yield efficiency denominator to reflect the annual increment of TCSA increase (rather than absolute TCSA value) once trial trees have filled their allotted space (T.L. Robinson, personal communication).
Historically, NC-140 evaluation trials with rootstocks that might vary significantly in vigor were planted at wide distances so that tree-to-tree competition was minimized, and growth and fruiting results could be compared to identify inherent vegetative and reproductive rootstock traits (e.g., Perry et al., 1997). As noted above, this is no longer relevant for contemporary orchard production systems. To evaluate the inherent traits of rootstocks that impart different levels of vigor for use in high density orchards, tree spacing must be varied in proportion to rootstock and scion vigor. Additionally, the inherent vigor associated with each site (e.g., factors including soils and climate) must be considered for optimal tree spacing. This greatly complicates "uniform" trial design, but ultimately will yield data of greater value for recommendations to growers across the country as well as provide better, more objective data sets for scientific interpretation of site effects on rootstock performance. Such trials may need to be designed in two phases - a small preliminary trial with low replication to quantify rootstock x site vigor (with no tree-to-tree competition), followed by a larger orchard systems-based trial utilizing site-specific tree spacing according to the vigor (and mortality) results of the preliminary trial (G.A. Lang, personal communication).
Elucidating the mechanisms regulating fundamental traits and physiological behaviour of rootstocks and rootstock/scion interactions is central to advancing rootstock science. The study of these systems is challenging, given that fruit trees are long-lived, woody perennial, compound genetic plants (Koepke and Dhingra, 2013). Interactions often exist between rootstocks and scions (Tworkoski and Miller, 2007; Lordan et al., 2019; Valverdi and Kalcsits, 2021a, 2021b) that accumulate and are expressed over time (DeJong et al., 2011). Scion vigor, for example, is affected by the xylem structure and anatomy of the rootstock (Solari and DeJong, 2006; Olmstead et al., 2010; Tworkoski and Fazio, 2011; DeJong et al., 2013). Graft transmissible agents can regulate scion physiology of apple (Kanehira et al., 2010; Xu et al., 2010), cherry (Maguylo et al., 2004), and peach (Reighard et al., 2001). Rootstock-modulated changes in scion gene expression were correlated with dwarfing and hormone relations of sweet cherry (Prassinos et al., 2009) and apple (Jensen et al., 2010, 2011; Li et al., 2012). Apple scion hormone profiles and levels can be affected by rootstocks (Lordon et al., 2017). Rootstocks can directly and variably affect mineral nutrient uptake efficiencies (Lang et al., 2011; Fallahi et al., 2013a, 2013b; Reighard et al., 2013; Neilsen and Hampson, 2014; Fazio et al., 2015; Thompson et al., 2019; Saraf et al., 2021; Valverdi and Kalcsits, 2021a) as well as by soil water management decisions (Neilsen and Kappel, 1996; Neilsen et al., 2004, 2007, 2015; Neilsen and Hampson, 2014; Xu and Ediger, 2021).
Novel technologies such as gene or mRNA transfer to impart pathogen resistance (Aldwinckle et al., 2009; Malnoy et al., 2010; Song et al., 2013; Zhao et al., 2020) may indeed play an important role in the development of the next generation of rootstocks, and this underscores the value of identifying molecular mechanisms and markers (Rusholme et al., 2004, 2008; Fazio et al., 2011) that improve genetic material and earlier selection of valuable new genotypes. For example, identification of genomic loci associated with dwarfing in pear (Wang et al., 2011) and apple (Celton et al., 2009; Fazio et al., 2014), as well as fireblight (Shamshin et al., 2021) and root rot (Zhu, and Saltzgiver, 2020) resistance in apple, has the potential to markedly decrease the time to discovery. Whether rootstock improvements are realized via traditional breeding or transgenic approaches, field performance trials will be a prerequisite to industry adoption and acceptance by consumers.
Concomitant with breeding efforts, the acquisition of novel germplasm from international sources has led to improved tree fruit performance in North America. Promising new tree fruit rootstocks possessing superior attributes to standard genotypes are continually being introduced from worldwide sources (DeJong et al., 2011; Elkins et al., 2012; Hrotkó, 2016; Khanizadeh et al., 2011; Univer et al., 2011; Zhang et al., 2011; Zurawicz et al., 2011; Reighard et al., 2013). Evaluation of the diverse germplasm collected and housed at the USDA-National Clonal Germplasm Repositories, for example, has markedly decreased the time required to advance new cold-hardy quince rootstocks for pear production (Einhorn et al., 2011) and apple germplasm with resistance to fungal components of replant disease (Fazio et al., 2009). For the latter, selection of progeny from Malus sieversii crosses represent the future of apple rootstocks. Replant disease has been characterized as a high priority by many fruit growers as regulations on chemical fumigants become increasingly strict (Mazzola et al., 2009). New apple rootstocks adaptable to replant disease sites facilitate sustainable production practices for existing fruit production regions and improve the efficiency and profitability of new plantings (Robinson et al., 2003; Zhu et al., 2018). Subsequently, field evaluation of apple rootstocks with purported tolerance to replant disease is ongoing throughout various regions of the U.S. (St. Laurent et al., 2010; Auvil et al., 2011; Parker et al., 2011). In addition, major advances in apple rootstock resistance to fire blight (Norelli et al., 2003; LoGiudice et al., 2006), and woolly apple aphid and powdery mildew (Fazio et al., 2006; Russo et al., 2007) have all been documented. Virus tolerance of sweet cherry dwarfing rootstocks (Lang and Howell, 2001; Lankes, 2011) and peach rootstock resistance to Armillaria and peach tree short life (Beckman, 2011) are critical areas of research. Developing genotypes resistant or tolerant to biotic stresses is fundamental to the sustainable management and profitability of tree fruit production.
Research efforts also have focused on improved resistance to abiotic stresses. The apparent acceleration of diverse changes in climate, e.g., more frequent frost and hail events, greater temperature ("heat domes", "polar vortex" events) and precipitation extremes (droughts and floods), underscore the need for increased climatic impact modeling (Cai et al., 2014) and the advancement of rootstock resilience and efficiency in resource acquisition (e.g., nutrient and water; Fazio et al., 2013; Jimenez et al., 2013; Neilsen et al., 2015; Tworkowski et al., 2016; Fazio et al., 2018). Rootstock evaluations have documented genetic variations in the ability to impart tolerance or sensitivity to cold stress under episodic events (Warmund et al., 2002) and in controlled environments to simulate sub-freezing conditions for pear (Einhorn et al., 2011) and apple (Privé et al., 2001; Moran et al., 2011, 2018). Pear producers in North America cannot adopt the precocious, dwarfing quince rootstocks commonly used in Europe due to freeze sensitivity. Consequently, little progress has been made with Pyrus rootstock germplasm over the past few decades (Azarenko et al., 2000; Elkins et al., 2012; Einhorn et al., 2013). More than 20 quince genotypes have been observed to possess sufficient hardiness for northern regions, but they need to be tested in major pear-producing regions.
Identification of rootstock genetic traits for tolerating abiotic stresses may not only result in greater tree fruit profitability, but potentially retain traditional areas for production as significant climatic changes occur over time, as well as facilitate expansion into previously marginal climates or sites when necessary. The NC-140 project's broad disciplinary scientific collaborations and diverse rootstock evaluation sites provide foundational resources to model the horticultural adaptability and physiological responses of important rootstocks over a wide-ranging combination of environmental conditions.
Rootstocks impart characteristics to fruiting scions such as vigor control, precocity, productivity, disease resistance, drought tolerance, and nutrient uptake effectiveness, and thus knowledge of such characteristics are critical for growers to make informed orchard training system decisions. The NC-140 project has inarguably benefitted growers and nurseries by critically evaluating new rootstocks from global breeding and selection efforts, and disseminating information and knowledge to the North American tree fruit industries via eXtension, multiple state web sites of project cooperators, and regional, national and international grower educational meetings and publications (e.g., Reighard et al., 2015; Autio et al., 2017, 2020; Fazio et al., 2018; Roper et al., 2019; Cline et al., 2021).
Objectives
-
To evaluate the influence of rootstocks on vegetative and reproductive growth and development of temperate-zone fruit trees, orchard productivity and labor efficiency, and sustainable orchard management practices across diverse soils and climatic regions.
-
To develop and/or acquire genetically-improved temperate-zone fruit tree rootstocks from global breeding and selection efforts.
-
To investigate the influence of rootstocks on physiological processes and resilience to biotic and abiotic stresses.
-
To accelerate adoption of new rootstocks by improving propagation and nursery tree development techniques to provide growers with trees suitable for new orchard systems and precocious production.
-
To integrate and disseminate research-based information and decision support tools that facilitate successful stakeholder adoption of rootstock technologies.
Methods
Objective 1
Promising new and existing fruit tree rootstocks are selected, grafted to commercial cultivars, and planted in coordinated uniform trials across North America. Each trial is evaluated by the local project coordinator for survival, precocity, productivity, size control, anchorage, root suckering, pest resistance, adaptability, and production efficiency. Some trials may include adaptability to specific or varied orchard systems and/or technologies. Trials generally are conducted for 10 growing seasons. Specific trial establishment, management, and data collection protocols are developed by the technical committee for each fruit species. Vegetative growth is typically measured by changes in trunk cross-sectional area, tree height, and canopy spread. Reproductive performance is typically measured by bloom precocity, yield, yield efficiency, and fruit quality parameters (e.g., size, soluble solids, etc.). Season-specific factors such as climatic data and/or observations on unusual climatic events, as well as annual pest and disease pressure, are also recorded.
Each trial has a designated coordinator who collects, archives, and analyses data for all sites for the duration of the trial. Data are processed annually for progress reports and shared with trial cooperators and the full membership at the annual meeting. Standard statistical analyses are performed on all data, and for each trial, results are usually summarized for two publications that describe the 1) orchard establishment and 2) mature orchard management phases.
Current NC-140 coordinated rootstock trials under active evaluation include:
- a) 2013 Pear. Rootstock and training system trial established in 2013 with 'Bosc' (NY), 'Bartlett' (CA), and 'd’Anjou' (OR) as scion cultivars. Rootstocks: OH×F 87 (standard), Pyro 2-33 and OH×F 69, were combined with three training systems (Bi-axis, tall spindle, and perpendicular V), planted at 1, 1.5 or 2 m in-row spacing.
- b) 2014 Apple. Rootstock trial established in 2014 at sites in the U.S. (AL, GA, ID, MA, ME, MI, MN, NJ, NY, PA, SC, UT, VA, WA, WI), Mexico and Canada (Ontario, 2 sites) with 'Honeycrisp' and 'Fuji' as scion cultivars. Rootstocks included M.9 and M.26 (standards), and V.1, V.5, V.6, V.7, G.41, G.935, G.30, G.11, G.202, G.9809, and B.10.
- c) 2015 Organic Modi Apple. Rootstock trial established in 2015 with 'Modi' as the scion in certified organic blocks at 15 sites (CA, CO, ID, IA, MA, MI, NJ, NM, NY [2 sites], VT, WI, and Nova Scotia). Rootstocks included M.9T337 (standard), G.11, G.16, G.30, G.41, G.202, G.214, G.222, G.890, G.935, and G.969.
- d) 2017 Peach. Rootstock trial established in 2017 with 'Cresthaven' as the scion cultivar at 10 sites (AL, CO, GA, MI, NC, NY, PA, SC, UT, and Ontario). Rootstocks included Lovell and/or Guardian® (standards), Controller 6, 7 and 8; Rootpac 20 (Densipac) and Rootpac 40 (Nanopac) from Agromillora Iberica; and MP-29 (USDA-Georgia).
- e) 2017 Sweet Cherry. Rootstock trial established in 2017 with 'Benton' as the scion cultivar at 5 sites (CO, ID, MI [2 sites], and NY). Rootstocks included Gi5 and Gi12 (standard semi-dwarfing and semi-vigorous rootstocks, respectively), Gi3, Cass, Clare, Clinton, Lake and MXM 14, all trained to three canopy systems (KGB, TSA, UFO).
- f) 2017 Tart Cherry High Density. Rootstock trial established in 2017 with 'Montmorency' as the scion cultivar at 7 sites (MI [2 sites], NY, UT [2 sites], and WI [2 sites]). Rootstocks included Mahaleb seedling (standard), Gi5, Gi12, Cass, Clare, Clinton, Crawford, and Lake, all planted at a high density suitable for over-the-row mechanical harvest (instead of the current industry standard of trunk shaker mechanical harvest).
- g) 2019 Apple. Rootstock trial established in 2019 with 'Buckeye Gala' as the scion cultivar at 13 sites (CO[x2], IA, KY, MD, MI, NC, NY, PA, UT, WA, and British Columbia and Nova Scotia). Rootstocks included M.9T337 and M.26 (standards), B.10, G.11, G.41, G.214, G.935, G.969, G.4814, NZ.1, NZ.2, and NZ.5.
New NC-140 coordinated rootstock trials under development for planting during 2022-2027 include:
- a) 2022 Pear. Rootstock trial for evaluation at 5 sites (CA, MI, OR, NY, WA) with new cold-hardy Amelanchier and quince selections on 'Comice' interstem, and pear standards.
- b) 2023 Cider Apple. Rootstock trial for evaluation at 17 sites (CO, KY, MA, MI, MT, NC, NJ, NY[x3], OH, VT, WA, WI, Nova Scotia, Quebec, and Ontario) with 13 rootstock genotypes.
- c) 2023 Peach Orchard Systems. Rootstock trial for evaluation of rootstock-by-training interactions in high density fruiting wall orchard systems at 11 sites (AL, CO, GA, KY, MI, NC, NY, SC, UT, VA, WA) with 4-5 rootstocks ranging from dwarfing to semi-dwarfing to semi-vigorous.
- d) 2024 Apple Orchard Systems. Rootstock trial for evaluation of rootstock-by-training interactions in multi-leader high density fruiting wall orchard systems at 13 or more sites (CO, ID, MI, NC, NY, PA, SC, UT, VA, WA, WI, British Columbia, Nova Scotia) with at least 3 new USDA/Geneva semi-dwarfing rootstocks.
- e) 2024 Apricot. Rootstock trial for evaluation at 6 sites (MI, NJ, NY, VA, British Columbia, Ontario) with new U.S. and European genotypes.
- f) 2024 Sweet Cherry. Rootstock trial for evaluation at 6 sites (ID, MI, NM, OR, NY, British Columbia) with 10 semi-dwarfing and semi-vigorous, rootstock genotypes.
- g) 2024 Tart Cherry. Rootstock trial with 'Montmorency' for evaluation at 4 sites (MI, NY, UT, WI) with 10 semi-vigorous and vigorous rootstock genotypes.
Other multi-state/province rootstock trials are or may be conducted independently on a local or regional basis by project members, but will not necessarily involve coordination of uniform establishment and data protocols. However, these are reported at the NC-140 annual meeting for providing supplemental information on rootstocks of interest. Tree performance is evaluated using most of the parameters noted above. These current and planned projects include the following:
Apple
Trials include a range of scion cultivars on rootstocks developed at USDA/Geneva and from internationally sources, evaluated under high pH soil conditions (CO), in various training systems (ID, NY, PA, WA), and for suitability for cider apple production (NY, ON).
Peach
Trials include a range of scion cultivars on rootstocks developed at USDA/Byron, University of California-Davis, and from international sources, evaluated under high pH soil conditions (CO) and in various training systems (CO, GA, MI, Ontario, PA).
Cherry
Trials include 'Montmorency' tart cherry and a range of sweet cherry scion cultivars on rootstocks developed at Michigan State University and from international sources, evaluated in various training systems and soil/climatic conditions (MI, NY, UT).
Pear
Trials include a range of scion cultivars on Amelanchier-, Cydonia-, and Pyrus-based pear rootstocks from international sources, evaluated in various training systems and climatic conditions (CA, MI, NY, OR).
Objective 2
Traditional and marker-assisted U.S. public breeding programs will develop improved rootstocks for apples (NY), peaches (GA, SC), and pears (WA). Specific breeding program objectives include: (1) peach rootstock tolerance or resistance to root-knot nematodes (GA), and peach tree short life complex and Armillaria root rot (GA, SC); (2) mapping the Prunus genome (SC) and isolation of markers for nematode and Armillaria resistance (SC); (3) understanding the genetic mechanisms underlying important rootstock traits (dwarfing, precocity and disease resistance) using a segregating/mapping population from an interspecific apple rootstock cross (O.3 x Robusta 5), both on their own roots and with 'Gala' as a scion (data from these populations will be combined with a genetic map to identify potential molecular markers for specific traits) (NY); and (4) pear rootstock vigor control and resistance to various biotic and abiotic stresses (WA).
Each breeding program director will provide progress reports from their programs for the NC-140 annual meeting. As needed, NC-140 project cooperators may collaborate for second- or third-phase testing of biotic stress resistance traits (see Objective 3). Periodically, progeny identified as elite selections will enter the uniform evaluation trial process described in Objective 1. Additionally, manuscripts or their published citations resulting from these studies will be shared with the members at-large and stakeholders.
Designated coordinators of the trials in Objective 1 also will maintain communications with international rootstock intellectual property stakeholders (nurseries, propagation license holders, and breeding program directors) to collect information about new and improved rootstock genotypes, and facilitate their limited procurement for objective scientific evaluations.
Objective 3
To develop foundational knowledge about the influence of rootstocks on physiological processes and resilience to biotic and abiotic stresses, basic rootstock performance data collected under Objective 1 will be complemented with additional, more-detailed studies, led and conducted by individual project members and cooperators at various institutions. These include responses to low and high temperatures, resistance to pests and pathogens, adaptation to ranges in soil pH, and inherent nutrient uptake efficiencies. Trial coordinators or cooperators will canvas project members to identify collaborative interest in conducting in-depth studies of specific parameters of importance. Those leading each effort will provide procedural protocols for collaborators and compile/analyze data for progress reports to be shared with the full membership at annual meetings. Additionally, manuscripts of these studies or their published citations will be shared with the members at-large and stakeholders.
Stress-related studies will include the following:
Low-temperature stress: assessment of cold acclimation, midwinter hardiness, and deacclimation of 1) apple, peach, and pear/quince rootstock effects on cold hardiness of vegetative, reproductive, and rootstock tissues (CO, IA, MI, MO, ON, OR, UT); 2) rootstock influence on acclimation, de-acclimation and re-acclimation of peach and apple in response to simulated changes in climate (ME, NY). Cold hardiness assessments generally are conducted with programmable freezers, differential thermal analysis (Quamme, 1991; Quamme and Hampson, 2004; Yu and Lee, 2020), or assessment of oxidative browning and electrolyte leakage (Kovaleski and Grossman, 2021; Moran et al., 2021).
General climate, water, nutritional, and physiological stress: 1) elucidate the relationships between rootstock, soil, climate, moisture and nutrient uptake for apple and cherry (BC, NS); 2) elucidate the relationship between rootstock, nutrient uptake and apple fruit quality and disorders such as bitter pit (ID, MI, NY, WA); 3) evaluate peach, apple, and cherry rootstocks for adaptation to alkaline soils (NM, UT); 4) evaluate tart cherry rootstocks with and without irrigation (WI); 5) evaluate apple rootstock/scion combinations for susceptibility/tolerance to sunburn (WA); 6) evaluate almond/peach rootstocks for root traits related to effective nutrient uptake such as root architecture and fine root population dynamics (CA). Physiological measurements may include leaf water potential, leaf photosynthetic rate, leaf fluorescence, quantification of canopy interception of photosynthetically-active radiation, and various techniques for leaf and fruit mineral analysis (Casagrande-Biasuz and Kalcsits, 2021a,b; Reighard et al., 2013; Valverdi and Kalcsits, 2021; Valverdi and Kalcsits, 2021a,b).
Biotic stress: 1) develop improved inoculation techniques to accelerate field evaluation of Armillaria resistance of peach rootstocks (GA); 2) evaluate peach tree short life (GA, NC, SC), Armillaria (GA, SC), and root-knot nematode resistance (GA, SC); 3) evaluate Armillaria resistance of cherry rootstocks (MI); 4) evaluate rootstock (CO, NC, NJ, NY, ON) and biofumigant (CO) effects on apple replant disease; 5) evaluate peach and apple rootstocks for adaptation to organic cultivation (CO); 6) evaluate apple rootstocks for fire blight resistance/tolerance (MD, MI, NY); 7) evaluate apple rootstocks for tomato ringspot virus (TRSV) tolerance/resistance (NY); and 8) evaluate the relationship between rootstock, scion and fire blight in Asian pears (AL).
Objective 4
Critical nursery tree production research will address rapid and high output rootstock clonal production techniques (e.g., tissue culture), high budding success of scions on rootstocks, graft incompatibilities, production of nursery trees free of known harmful viruses, and development of nursery trees with specific characteristics that meet fruit grower needs (e.g., tree caliper, branch number and type, etc.) (GA, NY, WA). In collaboration with the Northwest Nursery Improvement Institute and apple rootstock breeding programs, the propagation of new rootstock accessions will be optimized for stool bed, layering, and tissue culture methodologies. The inheritance of rootstock tolerance or susceptibility to important grafting-transmitted viruses is being analyzed within breeding populations and mapped genetically (NY). Furthermore, studies for methodologies to eliminate latent viruses (cryotherapy, thermotherapy) are on-going (NY).
As orchard training system characteristics inform ideal nursery tree development for rapid canopy establishment and precocious fruiting, work with stakeholder nurseries will be conducted to develop, adapt, and evaluate orchard system- and rootstock-specific nursery trees (e.g., bareroot vs. potted, whip vs. feathered, single leader vs. dual leader, on-farm nursery production protocols, etc.) (CA, MI, NY, WA). Root system establishment of nursery trees and fine root production of orchard trees will be studied with minirhizotrons as well as LiDAR scanning, which can detect root crown cracking that may be related to root disease development (CA).
Objective 5
Project research results will be interpreted to generate improved management practices for new rootstocks, including irrigation and fertilizer recommendations that minimize inputs and maximize genetic potential. Internet-based fruit tree extension research updates, production guides, decision-support applications, industry advisories, and calendar-based newsletters for growers are utilized by most of the NC-140 project members for timely and regular communications with their state and/or regional industry stakeholders. Face-to-face conferences, field tours, and virtual (e.g., Zoom) meetings with growers also are used to introduce information developed in the project. A vast quantity of the apple-related information developed by NC-140 and other researchers has been archived on, and periodically added to, the NC140 website (www.nc140.org) and the Apple eXtension website.
Measurement of Progress and Results
Outputs
- 1. Comments: Reporting Results: NC-140 research results will be communicated in ~40 papers at scientific conferences and in ~200 presentations to grower audiences. At least 15 refereed articles will be published, including 4 interim and 7 final trial reports, all based on the NC-140 coordinated regional rootstock research trials. Approximately 125 additional articles will be published by NC-140 members, based on NC-140-related research. These will include refereed and non-refereed articles targeted for scientific and fruit grower audiences.
- 2. Comments: New Rootstock Introductions: Seven new tree fruit rootstocks will be introduced from North American breeding programs under the guidance of NC-140. Twenty new tree fruit rootstocks will be introduced from international breeding programs to North American fruit growers with detailed recommendations regarding their suitability for various production areas.
- 3. Comments: Information Dissemination: The NC-140 (www.nc140.org) and the eXtension Apple (articles.extension.org/apples) websites will continue to be updated periodically to provide NC-140 results to all stakeholders, as will individual NC140 member websites, blogs, and other social media outlets.
Outcomes or Projected Impacts
- Enhance Orchard Profitability: NC-140 rootstock and orchard production recommendations and educational programs will guide the planting of 200,000+ acres of fruit trees in the next 5 years across North America, resulting in a more economically and environmentally sustainable fruit industry, providing a broad range of fruit for consumers. As new peach, cherry, and pear production systems using improved rootstocks follow the impacts we have seen from improved apple rootstocks and orchard production systems with smaller, narrower tree canopies, pesticide usage will be reduced, yielding environmental benefits and a savings we estimate at ~$150,000,000 in pesticide materials and application costs. The use of disease-resistant and stress-tolerant rootstocks will reduce annual tree losses by 10%.
- Improve Yield and Fruit Quality: By utilizing NC-140 recommended rootstocks and orchard production systems, growers will receive significantly earlier returns on investments related to tree establishment, achieving an average break-even point on investment by 5 years after planting. We estimate that adoption of improved rootstocks and orchard production systems will increase yields at maturity by 15% per acre, fruit size by 10%, and fruit meeting the highest grade category by 20%, compared with orchard systems 10 years ago. Narrower tree canopies will result in more uniform fruit ripening and quality compared with wider canopies from 10 years ago.
- Improved Labor Efficiency: By utilizing NC-140 recommended rootstocks and orchard production systems, orchard labor efficiency is estimated to increase by 20% per acre, compared with 10 years ago. The annual financial benefit to U.S. fruit growers from earlier returns, greater yield, higher fruit quality, and more efficient use of labor is estimated at $250,000,000 as a direct result of the implementation of NC-140 recommendations.
- Reduce Negative Effects of Climate Change: The use of NC-140 recommended dwarfing rootstocks and orchard production systems with smaller, narrower tree canopies will facilitate the increased use of orchard covering systems to protect tree fruit crops from damaging climatic events such as hail, rain, wind-bruising, sunburn, and/or frost damage. Adoption of such systems is anticipated to increase by 4,000 acres during 2022-2027.
- Cumulative Benefits of Adopting New Orchard Systems: It is estimated that cumulative state and federal investment in NC-140-related research projects will be about $15,000,000 over the next 5 years. Cumulative, measurable benefits to the U.S. temperate tree-fruit industries are estimated to be more than $400,000,000. Less easily measured benefits, such as averted losses and enhanced environmental quality, will increase the financial value of NC-140 to well beyond $500,000,000 over the next 5 years.
Milestones
(2022):For the first year of Objective 1: Interim reports for three trials established in 2017 (Sweet Cherry, tart Cherry and Peach) and a final report for the 2014 Fuji Apple trial will be submitted for publication in a refereed journal. The 2022 Pear trial will be planted.(2023):For the second year of Objective 1: The Final report for the 2013 pear Systems trial will be submitted for publication and the 2023 Cider Apple and Peach Orchard Systems trials will be planted.
(2024):For the third year of Objective 1: The interim report for the 2019 apple trial and the final report for the 2014 Honeycrisp trial will be submitted for publication. the 2024 trials for the Apple Orchard Systems, Apricot Rootstocks, and Sweet and Tart Cherry trials will be planted.
(2025):For the fourth year of Objective 1: The final report for the Organic Modi apple trial will be submitted for publication.
(2027):For the fifth year of objective 1: Final reports for the 2017 Sweet Cherry, 2017 Tart Cherry and the 2017 peach trials will be submitted for publication.
(2027):For Objective 2: New rootstock genotypes will be released from breeding programs and acquired from international sources throughout the 5-year project duration for evaluation in coordinated multi-site trials.
(2027):For Objective 3: Site-specific supplemental physiological evaluations of rootstock effects on biotic and abiotic stresses, tree development, and fruiting will be conducted by individual NC-140 cooperators based on regional needs and research expertise. These study timelines will vary based on the stage of each trial and external funding opportunities.
(2027):For Objective 4: Individual NC-140 cooperators will work with stakeholder nurseries and commodity research boards to develop, adapt, and evaluate orchard system-specific nursery trees based on regional grower needs and cooperator research expertise.
(2027):For Objective 5: Research-based new rootstock information will be discussed and interpreted at the annual project meeting, and disseminated regionally and nationally every winter. Interim and final reports will be developed according to the Objective 1 timeline and published/presented to stakeholder and scientific audiences as appropriate.
Projected Participation
View Appendix E: ParticipationOutreach Plan
The NC-140 project is committed to disseminating research-based results and information to stakeholders, commercial and small-scale orchardists, fruit tree nursery operators, industry representatives, professional colleagues, county extension educators, and home gardeners, master gardeners, and consumers.
Results from each state and province will be communicated at the annual technical committee meeting and posted online at the project site, www.nc140.org. The site contains cooperator contact information, annual reports/minutes, rootstock research planting descriptions, report summaries, and research results. Results include abstracts of refereed publications, links to journals for full articles, links to trade magazines, extension newsletter articles, and Powerpoint or pdf files of posters and professional talks. Outreach efforts have been enhanced with formal participation in eXtension for Apples, www.extension.org/apples. This website broadens access to results and information generated from this project.
Results are made available in refereed journals, grower publications, major national trade publications (print and electronic formats), and extension publications (print and electronic) prepared by each state participant; oral presentations at professional meetings (i.e., American Society for Horticultural Science and the International Society for Horticultural Science) as well as national and international fruit grower meetings. Numerous presentations will be made annually at regional, state and local fruit grower meetings (clientele and stakeholders) and producer field days sponsored by state extension programs, often in conjunction with state and provincial horticulture associations. In addition, cooperators will publish articles including results and recommendations in appropriate extension newsletters, fact sheets, and experiment station production manuals and bulletins.
Organization/Governance
This regional Technical Committee will be organized for the North Central Region as outlined in the Guidelines for Multistate Research Activities. The executive committee shall consist of the chairperson, vice chairperson, secretary, immediate past chairperson, the coordinator of each NC-140 uniform coordinated trial, and the crop committee chairs. Each year, a secretary will be elected to serve for one year, and the past vice-chairperson and the secretary will advance to the next higher office commencing in January. Members of the executive committee will set the annual meeting agenda, write and distribute the minutes and annual report, and act on the Technical Committee’s behalf as necessary. An Administrative Advisor will act as an advisor to the Technical Committee on procedures and policies related to regional research and provide coordination and communication with other regional projects and the North Central Directors. Annual meetings will be held for the purpose of evaluating current work, planning future work, and coordinating publications which may result from the work undertaken in the regional project. Each year, the chairperson will be responsible for organizing and leading the annual meeting, the vice chairperson will submit the annual report, and the secretary will submit the meeting minutes.
For uniform coordinated projects established under Objective 1, coordinators will be appointed on a continuing basis to coordinate the trials: Einhorn (MI)-2013 Pear; Cline (ONT)-2014 Apple; Bradshaw (VT)-2015 Organic Apple; Lang (MI)-2017 Sweet Cherry; Lang (MI)-2017 Tart Cherry; Minas (CO)-2017 Peach; Einhorn (MI)-2019 Apple. Each coordinator will provide technical oversight for their respective trials, maintain contact with the participants through correspondence, transmit pertinent information to participants and the Technical Committee to insure uniformity of the studies, prepare data collection forms and details of coordinated procedures which will permit a consolidation of the research results, assemble and analyze combined data or summarize data previously analyzed, initiate all publications regarding the coordinated trial, and report annually to the Technical Committee on trial progress.
Standing committees for each major crop will be appointed to plan, acquire plant genotypes, and organize propagation of trees for future multi-location uniform trials: these are currently led by Musacchi (WA)-apple; Minas (CO)-peach; Einhorn (MI)-pear; and Lang (MI)-cherry.
The Hatch Multi-State Research Funds expended on NC-140 (among all the cooperating states) will leverage approximately $2,000,000 of additional funds from various granting organizations, including Federal and state agencies, local grower organizations, and the International Fruit Tree Association. The Executive Committee will oversee funding requests in support of NC-140.
Literature Cited
Aldwinckle, H., E. Borejsza-Wysocka, P. Abbott, and S. Kuehne. 2009. Transformation of apple for disease resistance: Evolving strategies. Phytopathology. 99: S191-S191.
Autio, W., T. Robinson, B. Black, S. Blatt, D. Cochran, W. Cowgill, C. Hampson, E. Hoover, G. Lang, D. Miller, I. Minas, R. Parra Quezada, and M. Stasiak. 2017. Budagovsky, Geneva, Pillnitz, and Malling apple rootstocks affect ‘Honeycrisp’ performance over the first five years of the 2010 NC-140 Honeycrisp Apple Trial. Journal of the American Pomological Society. 71(3): 149-166.
Autio, W., T. Robinson, S. Blatt, D. Cochran, P. Francescatto, E. Hoover, M. Kushad, G. Lang, J. Lordan, D. Miller, I. Minas, R. Parra Quezada, M. Stasiak, and H. Xu. 2020. Budagovsky, Geneva, Pillnitz, and Malling apple rootstocks affect ‘Honeycrisp’ performance over eight years in the 2010 NC-140 Honeycrisp apple rootstock trial. Journal of the American Pomological Society. 74(4): 182-195.
Auvil, T.D., T.R. Schmidt, I. Hanrahan, F. Castillo, J.R. McFerson, and G. Fazio. 2011. Evaluation of dwarfing rootstocks in Washington apple replant sites. Acta Horticulturae. 903: 265-271.
Azarenko, A., Anderson, R., Brown, G., Embree, C., Ferree, D., Gaus, A., Hunter, D., Kappel, F., Ketchie, D., Meilke, E., Renquist, R., and Sugar, D. 2000. Final evaluation of the NC-140 national rootstock trial. ISHS 8th International Pear Symposium: 90 (abstract).
Beckman, T.G. 2011. Progress in developing Armillaria resistant rootstocks for use with peach. Acta Horticulturae. 903: 215-220.
Beckman, T.G. and Chaparro, J.X. (2015). Peach rootstock development for the southeastern United States. Acta Horticulturae. 1084: 171-178.
Cai, S., Zidek, J.V., Newlands, N.K., and Neilsen, D. 2014. Statistical modeling and forecasting of fruit crop phenology under climate change. EnvironMetrics. 25: 621-629.
Casagrande-Biasuz, E. and L. Kalcsits. 2021a. Rootstocks induce functional differences that affect carbon isotope discrimination and water relations in the apple scion. Authorea. July 08, 2021. DOI: https://doi.org/10.22541/au.162578061.13443316/v1.
Casagrande-Biasuz, E. and L.A. Kalcsits. 2021b. Variation in scion water relations mediated by two newly released Geneva series rootstocks. bioRxiv. preprint. DOI: https://doi.org/10.1101/2021.07.20.453132.
Celton, J.M., D.S. Tustin, D. Chagne, and S.E. Gardiner. 2009. Construction of a dense genetic linkage map for apple rootstocks using SSRs developed from Malus ESTs and Pyrus genomic sequences. Tree Genetics & Genomes. 5: 93-107.
Cline, J.A., W. Autio, J. Clements, W. Cowgill, R. Crassweller, T. Einhorn, E. Fallahi, P. Francescatto, E. Hoover, G. Lang, J. Lordan, R. Moran, M. Muehlbauer, S. Musacchi, M. Stasiak, R. Parra Quezada, T. Robinson, S. Serra, S. Sherif, R. Wiepz, and J. Zandstra. 2021. Early performance of ‘Honeycrisp’ apple trees on several size-controlling rootstocks in the 2014 NC-140 rootstock trial. Journal of the American Pomological Society. 74(4): 189-202.
DeJong, T.M., R.S. Johnson, and K.R. Day. 2011. Controller 5, Controller 9 and Hiawatha peach rootstocks: their performance and physiology. Acta Horticulturae. 903: 221-228.
DeJong, T. M., S. Tombesi, B. Basile and D. Da Silva. 2013. Beakbane and Thompson (1939, East Malling) had it right: scion vigour is physiologically linked to the xylem anatomy of the rootstock. Aspects of Applied Biology 119: 51-58.
Dorigoni, A., P. Lezzer, N. Dallabetta, S. Serra, and S. Musacchi. 2011. Bi-axis: an alternative to slender spindle for apple orchards. Acta Horticulturae. 903: 581-588.
Du, X., Chen, D., Zhang, Q., Scharf, P.A., Whiting, M.D. 2012. Dynamic responses of sweet cherry trees under vibratory excitations. Biosystems Engineering. 111: 305-314.
Einhorn, T., Postman, J., and Turner, J. 2011. Characterization of cold hardiness in quince: Potential pear rootstock candidates for Northern pear production regions. Acta Horticulturae 909: 137-144.
Einhorn, T., Castagnoli, S., Smith, T., Turner, J., and Mielke, E. 2013. Summary of the 2002 Pacific Northwest Pear Rootstock Trials: Performance of ‘d’Anjou’ and ‘Golden Russet Bosc’ pear on eight Pyrus rootstocks. J. Amer. Pom. Soc. 67: 80-88.
Elkins, R.B.and T.M. DeJong. 2011. Performance of 'Golden Russet Bosc' pear on five training systems and nine rootstocks. Acta Horticulturae. 903: 689-694.
Elkins, R., R. Bell, and T. Einhorn. 2012. Needs assessment for future US pear rootstock research directives based on the current state of pear production and rootstock research. J. Amer. Pom. Soc. 66:153-163.
Elkins, R.B., S. Castagnoli, C. Embree, R. Parra-Quezada, T.L. Robinson, T.J. Smith and C.A. Ingels. 2011. Evaluation of potential rootstocks to improve pear tree precocity and productivity. Acta Horticulturae. 909: 183-194.
Fallahi, E., K. Arzani, and B. Fallahi. 2013a. Long-term leaf mineral nutrition in ‘Pacific Gala’ apple (Malus domestica Borkh.) as affected by rootstock type and irrigation system during six stages of tree development. Journal of Horticultural Science & Biotechnology 88 (6) 685–692. https://doi.org/10.1080/14620316.2013.11513025
Fallahi, E., B. Fallahi, and B. Shafii. 2013b. Irrigation and rootstock influence on water use, tree growth, yield, and fruit quality at harvest at different ages of trees in ‘Pacific Gala’ apple. HortScience. 48:588-593.
Fazio, G., H.S. Aldwinckle, R.P. McQuinn, and T.L. Robinson. 2006. Differential susceptibility to fire blight in commercial and experimental apple rootstock cultivars. Acta Horticulturae. 704: 527-530.
Fazio, G., H.S. Aldwinckle, T.L. Robinson, and Y. Wan. 2011. Implementation of molecular marker technologies in the Apple Rootstock Breeding program in Geneva - challenges and successes. Acta Horticulturae. 903: 61-68.
Fazio, G., H.S. Aldwinckle, G.M. Volk, C.M. Richards, W.J. Janisiewicz, and P.L. Forsline. 2009. Progress in evaluating Malus sieversii for disease resistance and horticultural traits. Acta Horticulturae. 814: 59-66.
Fazio, G., L. Chang, M. Grusak, and T. Robinson. 2015. Apple rootstocks influence mineral nutrient concentration of leaves and fruit. NY Fruit Quarterly. 23:11-15.
Fazio, G., D. Kviklys, M. Grusak, and T. Robinson. 2013. Phenotypic diversity and QTL mapping of absorption and translocation of nutrients by apple rootstocks. Aspects of Applied Biology 119:37-45.
Fazio, G., J. Lordan, P. Francescatto, and T.L. Robinson. 2018. Breeding apple rootstocks to match cultural and nutrient requirements of scion varieties. Fruit Quarterly. 26(2): 25-30.
Fazio, G., Y. Wan, D. Kviklys, L. Romero, R. Adams, D. Strickland and T. Robinson. 2014. Dw2 a new dwarfing locus in apple rootstocks and its relationship to induction of early bearing in apple scions. J. Amer. Soc. for Hort. Sci. 139:87-98.
Hilaire, C., Ruesch, J., Montrognon, Y., Courthieu, N. and Blanc, P. 2021. Peach-nectarine: comparison of the behavior of eleven rootstocks - first observations realized in France from an evaluation network. Acta Horticulturae. 1304: 49-56.
Hrotkó, K., 2016. Potentials in Prunus mahaleb L. for cherry rootstock breeding. Scientia Horticulturae, 20:70-78.
Jensen, P.J., I. Makalowska, N. Altman, G. Fazio, C. Praul, S.N. Maximova, R.M. Crassweller, J.W. Travis, and T.W. McNellis. 2010. Rootstock-regulated gene expression patterns in apple tree scions. Tree Genetics & Genomes. 6: 57-72.
Jensen, P.J., T.W. McNellis, N. Halbrendt, J.W. Travis, N. Altman, C.A. Praul, S.N. Maximova, R.M. Crassweller, and I. Makalowska. 2011. Rootstock-regulated gene expression profiling in apple trees reveals genes whose expression levels are associated with fire blight resistance. Acta Horticulturae. 903: 87-93.
Jiang, X. and L. He. 2021. Investigation of effective irrigation strategies for high-density apple orchards in Pennsylvania. Agronomy. 11: 732.
Jimenez, S., J. Dridi, D. Gutierrez, D. Moret, J.J. Irigoyen, M. Moreno, and Y. Gogorcena. 2013. Physiological biochemical and molecular responses in four Prunus rootstocks submitted to drought stress. Tree Physiology 33:1061-1087.
Kanehira, A., K. Yamada, T. Iwaya, R. Tsuwamoto, A. Kasai, M. Nakazono, and T. Harada. 2010. Apple phloem cells contain some mRNAs transported over long distances. Tree Genetics & Genomes. 6: 635-642.
Khanizadeh, S., Y. Groleau, R. Granger, G.L. Rousselle, J.P. Prive, and C.G. Embree. 2011. St Jean Morden (SJM) dwarf winter hardy rootstock series. Acta Horticulturae. 903: 191-192.
Koepke, T. and A. Dhingra. 2013. Rootstock scion somatogenetic interactions in perennial composite plants. Plant Cell Reports. 32: 1321-1337.
Lang, G.A. 2019. The cherry industries in the USA: current trends and future perspectives. Acta Horticulturae. 1235:119-132.
Lang, G.A., S. Blatt, C. Embree, J. Grant, S. Hoying, C. Ingels, D. Neilsen, G. Neilsen and T. Robinson. 2014. Developing and evaluating intensive sweet cherry orchard systems: The NC140 regional research trial. Acta Horticulturae. 1058:113-120.
Lang, G.A. and W. Howell. 2001. Lethal sensitivity of some new cherry rootstocks to pollen borne viruses. Acta Horticulturae. 557: 151-154.
Lang, G.A. and W.W. Shane. 202x. Experimental canopy training effects on peach/nectarine vigor advance planar training system development as well as evaluation and adoption of vigor-limiting rootstocks. Acta Horticulturae. (in review).
Lang, G.A. and M.D. Whiting. 2021. Canopy architecture - optimizing the interface between fruit physiology, canopy management, and mechanical/robotic efficiencies. Acta Horticulturae. 1314:287-296.
Lang, G.A., T. Wilkinson, and J.E. Larson. 2019. Insights for orchard design and management using intensive sweet cherry canopy architectures on dwarfing to semi-vigorous rootstocks. Acta Hort. 1235:161-167.
Lankes, C. 2011. GiSelA cherry rootstocks compared for virus tolerance and field performance. Acta Horticulturae. 903: 521-527.
Lankes, C.and G. Baab. 2011. Screening of apple rootstocks for response to apple proliferation disease. Acta Horticulturae. 903: 379-383.
Li, H.L., H. Zhang, C. Yu, L. Ma, Y. Wang, X.Z. Zhang, and Z.H. Han. 2012. Possible roles of auxin and zeatin for initiating the dwarfing effect of M9 used as apple rootstock or interstock. Acta Physiologiae Plantarum. 34: 235-244.
LoGiudice, N., Fazio, G., Robinson, T.L., Aldwinckle, H.S. 2006. The nature of resistance of the 'B.9' apple rootstock to fire blight. Acta Horticulturae. 704:515-519.
Long, L., G. Lang, S. Musacchi, and M. Whiting. 2015. PNW 667 cherry training systems. Pacific Northwest Extension Publication. 667.
Lordon, J., Fazio, G., Francescatto, P., and Robinson, T. L. 2017. Effects of apple (Malus x domestica) rootstocks on scion performance and hormone concentration. Scientia Horticulturae. 225: 96-105.
Lordan, J., Fazio, G., Francescatto, P., and Robinson, T. L. 2019. II. Horticultural performance of ‘Honeycrisp’ grown on a genetically diverse set of rootstocks under Western New York climatic conditions. Scientia Horticulturae. 257: 108686.
Maguylo, K., Lang, G.A., and Perry, R.L. 2004. Rootstock genotype affects flower distribution and density of `Hedelfinger sweet cherry and `Montmorency sour cherry. Acta Horticulturae. 636:259-266.
Malnoy, M., E.E. Boresjza-Wysocka, J.L. Norelli, M.A. Flaishman, D. Gidoni, and H.S. Aldwinckle. 2010. Genetic transformation of apple (Malus x domestica) without use of a selectable marker gene. Tree Genetics & Genomes. 6: 423-433.
Marini, R.P., R. Moran, C. Hampson, M. Kushad, R.L. Perry, and T.L. Robinson. 2008. Effect of dwarf apple rootstocks on average 'Gala' fruit weight at six locations over three seasons. J. Amer. Pom. Soc. 62: 129-136.
Mazzola, M., J. Brown, X. Zhao, A. Izzo, and G. Fazio. 2009. Interaction of Brassicaceous seed meal and apple rootstock on recovery of Pythium spp. and Pratylenchus penetrans from roots grown in replant soils. Plant Disease. 93: 51-57.
Moran, R.E., Y. Sun, F. Geng, and D. Zhang. 2011. Cold temperature tolerance of one- and two-year old apple rootstocks. HortScience 46: 1460-1464.
Moran, R., B. Peterson, G. Fazio, and J. Cline. 2018. Genotypic variation in apple rootstock low temperature tolerance during spring and fall. Journal of the American Society for Horticultural Science. 143: 319-332.
Mudge K., J. Janick, S. Scofield, and EE Goldschmidt. 2009. A history of grafting. In: Janick, J (ed) Horticultural Reviews. John Wiley & Sons Inc., NJ, pp 437-439.
Musacchi, S., F. Gagliardi, and S. Serra. 2015. New training systems for high-density planting of sweet cherry. HortScience. 50: 59-67.
Neilsen, G. and Hampson, C. 2014. ‘Honeycrisp’ apple leaf and fruit nutrient concentration is affected by rootstock during establishment. J. Amer. Pom. Soc. 68: 178-189.
Neilsen, G. and Kappel, F. 1996. ‘Bing’ sweet cherry leaf nutrition is affected by rootstock. HortScience. 31: 1169–1172.
Neilsen, G., Kappel, F., and Neilsen, D. 2004. Fertigation method affects performance of ‘Lapins’ sweet cherry on Gisela 5 rootstock. HortScience. 39: 1716–1721.
Neilsen, G., Kappel, F., and Neilsen, D. 2007. Fertigation and crop load affect yield, nutrition and fruit quality of ‘Lapins’ sweet cherry on Gisela 5 rootstock. HortScience. 42: 1456–1462.
Norelli, J.L., Holleran, H.T., Johnson, W.C., Robinson, T.L., and Aldwinckle, H.S. 2003. Resistance of Geneva and other apple rootstocks to Erwinia amylovora. Plant Disease. 8:26-32.
Olmstead, M.A., N.S. Lang, and G.A. Lang. 2010. Carbohydrate profiles in the graft union of young sweet cherry trees grown on dwarfing and vigorous rootstocks. Scientia Hortic. 124:78-82. https://doi.org/10.1016/j.scienta.2009.12.022
Parker, M.L., D. Ritchie, and G.L. Reighard. 2011. Guardian peach rootstock performance and preplant soil fumigation effects in a fallow site. Acta Horticulturae. 903: 469-473.
Perry, R., G. Lang, R. Andersen, L. Anderson, A. Azarenko, T. Facteau, D. Ferree, A. Gaus, F.
Kappel, F. Morrison, C. Rom, T. Roper, S. Southwick, G. Tehrani, and C. Walsh. 1997. Performance of the NC-140 cherry rootstock trials in North America. Acta Horticulturae. 451: 225-229.
Prassinos, C., J.H., Ko, G. Lang, A.F., Iezzoni, and K.H., Han. 2009. Rootstock-induced dwarfing in cherries is caused by differential cessation of terminal meristem growth and is triggered by rootstock-specific gene regulation. Tree Physiology. 29: 927-936.
Privé, J.P., Zhang, M.I.N., Embree, C., and Hebb, D. 2001. The influence of freeze-thaw cycling in cold hardiness studies on apple rootstocks. Acta Horticulturae. 557:123-130.
Quamme, H.A. 1991. Application of thermal analysis to breeding fruit crops for increased cold hardiness. Hortscience. 26: 513-517. https://doi.org/10.21273/HORTSCI.26.5.513
Quamme, H. and Hampson, C.R. 2004. Winter hardiness measurements on 15 new apple cultivars. Journal of the American Pomological Society. 58: 98-107.
Reighard, G.L. 2013. Peach, plum and apricot rootstocks for the 21st century. Aspects of Applied Biology 119:59-66.
Reighard, G., Bridges, Jr., W., Archbold , D., Wolfe, D., Atucha , A., Pokharel, R., Autio, W., Beckman, T., Black , B., Lindstrom, T., Coneva, E., Day , K., Johnson, R.S., Kushad, M., Parker, M., Robinson, T., Schupp, J. and Warmund, M. 2015. NC-140 peach rootstock testing in thirteen U.S. States. Acta Horticulturae. 1084: 225-232.
Reighard, G.L., W. Bridges, B. Rauh and N.A. Mayer. 2013. Prunus rootstocks influence peach leaf and fruit nutrient content. Acta Horticulturae 984:117-124.
Reighard, G.L., Ouellette, D.R., and Brock, K.H. 2001. Modifying phenotypic characters of peach with graft transmissible agents. Acta Horticulturae. 557:163-167.
Robinson, T.L. and L. Dominguez. 2015. Yield and profitability of high-density pear production with Pyrus rootstocks. Acta Horticulturae. 1094: 247-256.
Robinson, T., Aldwinckle, H., Holleran, T., and Fazio, G. 2003. The Geneva series of apple rootstocks from Cornell: performance, disease resistance, and commercialization. Acta Horticulturae. 622:513-522.
Robinson, T.L. 2008. The evolution towards more competitive apple orchard systems in the USA. Acta Horticulturae. 772: 491-500.
Robinson, T.L., S.A. Hoying, and G.H. Reginato. 2011. The Tall Spindle planting system: principles and performance. Acta Horticulturae. 903: 571-579.
Roper, T., B. Black, M. Stasiak, R. Marini, J. Cline, T. Robinson, G. Lang, L. Anderson, R. Andersen, J. Freer, G. Greene, and R. Perry. 2019. Performance of 'Montmorency' tart cherry (Prunus cerasus L.) on potential size-controlling rootstocks at six locations in North America: results of the 1998 NC-140 Trial. Journal of the American Pomological Society. 73(3):168-177.
Rusholme, R.L., S.E. Gardiner, H.C.M. Bassett, D.S. Tustin, S.M. Ward, and A. Didier. 2004. Identifying genetic markers for an apple rootstock dwarfing gene. Acta Horticulturae: 405-409.
Rusholme-Pilcher L, Celton JM, Gardiner SE. 2008. Genetic markers linked to the dwarfing trait of apple rootstock ‘Malling 9’. J. Amer. Soc. Hort. Sci. 133, 100–106.
Russo, N.L., T.L. Robinson, G. Fazio, and H.S. Aldwinckle. 2007. Field evaluation of 64 apple rootstocks for orchard performance and fire blight resistance. Hortscience. 42: 1517-1525.
Shamshin, I.N., Mslova, M.V., Drenova, N.V., Dubrovsky, M.L., and Parusova, O.V. 2021. Assessment of fire blight resistance in apple clonal rootstocks using molecular markers. Proceedings on Applied Botany Genetics and Breeding. 4:185-191.
Solari, L.I., and T.M., DeJong. 2006. The effect of root pressurization on water relations, shoot growth, and leaf gas exchange of peach (Prunus persica) trees on rootstocks with differing growth potential and hydraulic conductance. Journal of Experimental Botany. 57: 1981-1989.
Song, G.-Q., K.C. Sink, A.E. Walworth, M.A. Cook, R.F. Allison, and G.A. Lang. 2013. Engineering cherry rootstocks with resistance to Prunus necrotic ring spot virus through RNAi-mediated silencing. Plant Biotechnology Journal doi: 10.1111 / pbi.12060.
St. Laurent, A., I.A. Merwin, G. Fazio, J.E. Thies, and M.G. Brown. 2010. Rootstock genotype succession influences apple replant disease and root-zone microbial community composition in an orchard soil. Plant and Soil. 337: 259-272.
Tworkoski, T. and G. Fazio. 2011. Physiological and morphological effects of size-controlling rootstocks on 'Fuji' apple scions. Acta Horticulturae. 903: 865-872.
Tworkoski, T. and S. Miller. 2007. Rootstock effect on growth of apple scions with different growth habits. Scientia Horticulturae. 111: 335-343.
Tworkoski, T., G. Fazio, and D.M. Glenn, 2016. Apple rootstock resistance to drought. Scientia Horticulturae 204:70-78
Univer, T., N. Univer, and K. Tiirmaa. 2011. The results of the Estonian apple rootstock breeding program. Acta Horticulturae. 903: 151-157.
Valverdi, N.A., Kalcsits, L. 2021a. Rootstock affects scion nutrition and fruit quality during establishment and early production of ‘Honeycrisp’ apple. HortScience. 56:261-269.
Valverdi, N.A., Kalcsits, L. 2021b. Apple rootstock genotype affects scion responses to water limitations under field conditions. Acta Physiologia Plantarum. 43:97.
Vercammen, J. 2004. Dwarfing rootstocks for sweet cherries. Acta Horticulturae. 658:307-311.
Wang, C., Y., Tian, E.J., Buck, S.E., Gardiner, H. Dai, and Y. Jia. 2011. Genetic mapping of PcDw determining pear dwarf trait. J. Amer. Soc. Hort. Sci. 136: 48-53.
Westwood, M.N. and A.N. Roberts. 1970. The relationship between trunk cross-sectional area and weight of apple trees. Journal of the American Society for Horticultural Science. 95: 28-30.
Xu, H. and D. Ediger. 2021. Rootstocks with different vigor influence scion-water relations and stress responses in Ambrosia™ apple trees (Malus Domestica var. Ambrosia). Plants. 10, 614. https://doi.org.10.3390/plants10040614.
Zhang, X., He, L., Zhang, J., Whiting, M.D., Karkee, M., and Zhang, Q. 2020. Determination of key canopy parameters for mass mechanical apple harvesting using supervised machine learning and principal component analysis (PCA). Biosystems Engineering. 193: 247-263.
Zhao, D.Y., Zhong, G.Y., and Song, G.Q. 2020. Transfer of endogenous small RNAs between branches of scions and rootstocks in grafted sweet cherry trees. Plos One. 15:e0236376
Zhu, Y., and Saltzgiver, M. 2020. A systematic analysis of apple root resistance traits to Pythium ultimum infection and the underpinned molecular regulations of defensive activation. Horticulture Research. 7:62. https://doi.org/10.1038/s41438-020-0286-4
Zhu, Y., Zhao, J., and Zhou, Z. 2018. Identifying an elite panel of apple rootstock germplasm with contrasting root resistance to Pythium ultimum. Journal of Plant Pathology and Microbiology. 9:1000461.