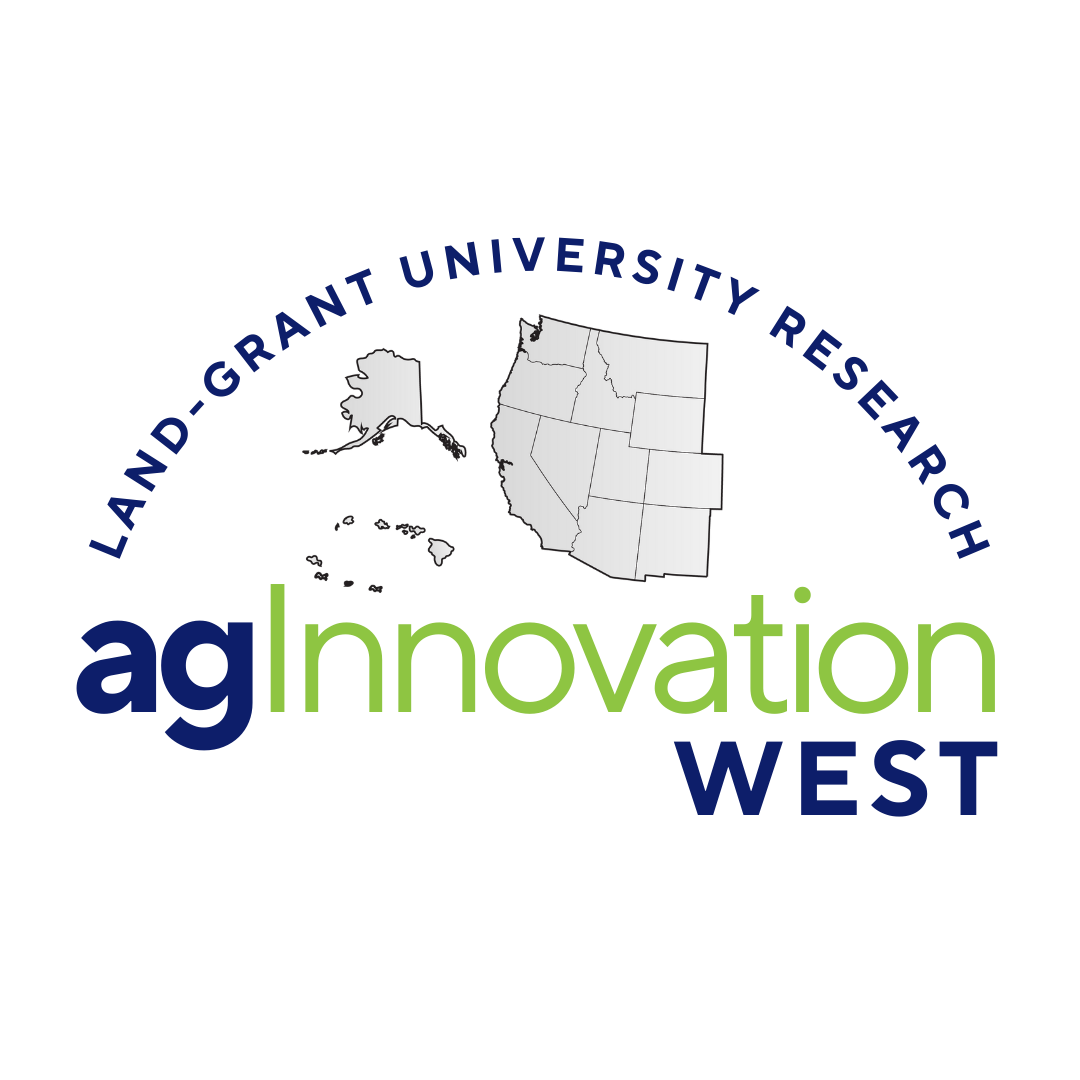
W4170: Beneficial Use of Residuals to Improve Soil Health and Protect Public, and Ecosystem Health
(Multistate Research Project)
Status: Inactive/Terminating
W4170: Beneficial Use of Residuals to Improve Soil Health and Protect Public, and Ecosystem Health
Duration: 10/01/2019 to 09/30/2024
Administrative Advisor(s):
NIFA Reps:
Non-Technical Summary
Statement of Issues and Justification
STATEMENT OF ISSUES AND JUSTIFICATION
STATEMENT OF ISSUES:
Over 260 million tons of residual by-products, such as urban wastes (biosolids, recycled water, food scraps and other municipal solid waste), agricultural waste (manure), industrial sludges and waste byproducts (e.g., bioenergy residue byproducts) are currently produced in the U.S. (US EPA, 2018). According to the US EPA (2018), approximately 8.9, 12.8, and 25.8% of these materials are either composted, combusted for energy recovery or recycled, respectively, while the remaining 52.5% are landfilled. Specifically in terms of material disposal, ~50% of biosolids, 98% of food scraps and 45% of yard trimmings are currently landfilled or incinerated at a substantial cost to the industry and public (King et al., 2011). Thus, increasing reuse of various residual materials such as soil amendments offers the potential to replace disposal costs with beneficial agronomic and environmental uses. It is predicted that 52% of the world’s population will live in regions under water stress by 2050 (Schlosser et al., 2014), resulting in high demand for wastewater recycling and reuse for agricultural production. In addition, treated liquid wastes, such as wastewater effluent, recycled water and other non-potable waters, present opportunities for beneficial reuse in lieu of surface water discharge or expensive treatment. However, there are consistently numerous obstacles that prevent the full beneficial utilization of the above materials.
Obstacles that limit the beneficial use of residual products include conflicting regulations or the absence of regulations on residuals use, lack of public outreach and communication, and limited research on optimizing residuals-based product development. Fortunately, current and increasing evidence exits exemplifying that land application of a variety of residuals do provide agronomic and environmental benefits, which were either not previously well understood or are critical to addressing emerging environmental issues (e.g., system resiliency in the face of changing environmental factors highlighted in Brown et al., 2011). The W3170 workgroup, who consists of some of the best minds and resources within and outside the State Agricultural Experiment Station System (e.g., US EPA, municipality personnel, private entities), proposes to continue to conduct research towards sustainable use of residual products. The W3170 proposes to continue investigating the biogeochemical cycling of plant nutrients, movement and potential adverse impacts of trace element and trace organic contaminants (TOrCs) within ecosystems and the food chain, their long-term bioavailability in residual-amended soils, and soil health effects from application of residuals. These investigations will generate knowledge needed to develop and promote residuals recycling practices that are protective of human health and the environment. Outcomes will provide data for continuing one-health based risk assessment required by the US EPA Part 503 Rule for biosolids land-application as well as for developing regulations for land-based recycling of residuals and reclaimed water. Research will also focus on reuse benefits including field and watershed scale effects on soil quality/health, plant drought response, soil carbon sequestration, water quality, greenhouse gas emissions and climate change impacts associated with soil-based residuals and reclaimed water reuse. We will also explore the potential for residual based product usage in urban and agriculture systems, and green stormwater infrastructures as well in restoration of degraded lands. W3170 members are currently conducting and synthesizing both short- and long-term research that will enable the development of guidelines for the sustainable use of a wide variety of residuals and residual by-products: maximizing benefits while minimizing the potential for unintended negative consequences.
JUSTIFICATION:
The beneficial use of residuals requires an understanding of both potential hazards and the value of constituents in the by-products. Investigation of the fate and behavior of contaminants and nutrients in biosolids-amended soils has been the historic research focus of the W3170 multistate workgroup. Research conducted previously by the workgroup formed the basis for the U.S. EPA Part 503 biosolids rule, which is one of the few rules to include bioavailability assessments in the development of limits for critical contaminants (National Research Council, 2009). Two subsequent NAS reviews, focused on the 503 rule, confirmed that scientific basis for this rule and risk assessment were valid (NRC, 2002). Members of the W3170 group have contributed research and technical expertise that has led to guidance that permit beneficial reuse of many industrial and municipal byproduct for land application or as feedstocks in manufactured soil blends, including spent foundry sands (U.S. EPA, 2014), water treatment waste residuals, and many other residuals (Ohio, EPA). Most recently, research conducted by W3170 members included evaluating the occurrence of per- and polyfluoroalkyl substances (PFAS), a large persistent class of TOrCs of high concern, in municipal solid waste composts (Choi et al., 2019). Their data contributed to the successful passage in 2018 of the State of Washington’s Healthy Food Packaging Act which bans the use of the entire class of PFAS in paper food packaging after January 1, 2022 assuming suitable alternatives are identified (HB2658-2017-18). This type of law will help prevent the use of products from other countries where PFAS-related bans may not exist and will prevent unwanted contaminants in our food, reduce their input into wastewater treatment plants, thus biosolids, and will minimize their entry into compostable materials where their presence has been pervasive.
This past year, the U.S. EPA Office of Inspector General released their report “EPA unable to assess the impact of hundreds of unregulated pollutants in land-applied biosolids on human health and the environment” (U.S. EPA, 2018). The U.S. EPA “identified 352 pollutants in biosolids but cannot yet consider these pollutants for further regulation due to either a lack of data or risk assessment tools. Pollutants found in biosolids can include pharmaceuticals, steroids, and flame retardants.” The W3170 committee is “the” USDA NIFA Multistate Committee that can continue to provide data and technical expertise to U.S. EPA for addressing the regulatory needs they identified in the U.S. EPA OIG report. U.S. EPA Office of Water and Office of Research and Development personnel continue to be active members of W3170. Our university members are recognized internationally as leaders in research on the environmental fate of TOrCs, e.g, pharmaceuticals, steroids, chemicals from personal care products, flame-retardants and PFAS, that can be land-applied with biosolids applications. Addressing U.S.EPA OIG data and research gaps will be a priority in the proposed W3170 renewed project.
Human and ecological risk assessment (HERA) science continues to mature with biogeochemical availability-based risk assessment frameworks being developed and/or considered for implementation in the U.S., Canada, the European Union, Australia and other countries. Continued research is needed to provide the scientific basis for risk-based methods and to evaluate residuals and residual-treated soils for adoption by HERA frameworks. Research needed to evaluate contaminants in residuals includes the following: (i) trace element speciation by advanced spectroscopic methods and wet chemical speciation methods; (ii) chemical lability and plant uptake potential of frequently detected trace elements and TOrCs in residuals-amended soils in the field; (iii) use of bioavailability to adjust human and ecological exposure in risk assessment frameworks by using inexpensive novel bioaccessibility methods and in vivo methods; and (iv) total combined effects of residuals on soil physical, chemical, nutrient and biological components in order to ascertain alterations in soil quality/health following residuals land application.
Several members of the W3170 were extensively involved in the development of the Part 503 regulation and continue to be involved in the development of risk assessment for other residuals (e.g., the EPA risk assessment for land application of cement kiln dust and foundry sand) and for other pollutants not initially regulated or considered in Part 503 (e.g., barium, TOrCs). The scientific approach used in the development of Part 503 has been applied by this group to an expanded variety of residuals, contaminants and receptors. As the understanding of bioavailability is expanding, the group is broadening its focus to develop linkages between quantitative understanding of the contaminant forms and their bioavailability to a range of receptors.
In the past, our group has conducted cooperative projects involving laboratory incubations, greenhouse studies and x-ray absorption spectroscopy to elucidate the role of organic and inorganic components of biosolids and other residuals in binding metals (Brown et al., 2003a; Hettiarachchi et al., 2003a, b, 2006; Ippolito et al., 2009, 2013; Obrycki et al., 2016; Ryan et al., 2003, 2004a; Scheckel et al., 2004). We have demonstrated that biosolids and other soil amendments can reduce the bioavailability of metals in contaminated systems (e.g., Attanayake et al., 2014; 2015; 2017; Barbarick et al., 2015, 2016, 2017; Basta et al., 2001; Brown et al 2003b, 2007; DeVolder et al., 2003; Hettiarachchi and Pierzynski, 2002; Ippolito et al., 2010, 2014; Meiman et al., 2012), while developing various extractants to assess bioavailability of mineral and bioavailable fractions of inorganic contaminants (e.g., Basta and Juhasz, 2014; Basta et al., 2003; Brown et al., 2004; Rodriguez et al., 2003; Ryan et al., 2004; Schroder et al., 2003; Whitacre et al., 2017).
Our group’s research has broadened to encompass a range of measurement endpoints as we realize and understand the potential for metals to affect a range of receptors. The goal of this research is to evaluate functions of restored ecosystems utilizing tools such as in vivo and in vitro assays, toxicity assays and measures of microbial community dynamics (Alexander et al., 2003; Basta and Juhasz, 2014; Basta et al., 2003; Brown et al., 2004; Schroder et al., 2003; Sullivan et al., 2005). As a result of cooperative research conducted by members of W3170, in situ remediation options, including biosolids and other residuals, have been utilized on a number of EPA Superfund NPL (National Priority List) sites, contaminated urban soils, mine lands, forest fire burned areas and overgrazed and degraded ecosystem sites. The tools developed for this research have broadened our understanding on the functioning of soils amended with biosolids or residuals.
The sustainability of biosolids application to agricultural lands has also been demonstrated by evaluating the effects of biosolids application on various aspects of soil functionality, recently termed “soil health.” While the implications of this research are being recognized in agroecosystems and remediation of contaminated sites, such research is also needed for rehabilitation of urban landscapes. Among the greatly expanding potential markets for exceptional quality (EQ) biosolids are uses for urban agriculture, turfgrass and other vegetated urban landscapes on anthropogenic soils.
Among the challenges in urban agriculture and other anthropogenic soil landscapes are their commonly degraded nature and potential presence of contaminants in such soils. In contrast to natural soils, human activities strongly influence urban soils through the removal of topsoil, physical disturbances, heavy vehicle traffic and addition of foreign materials (e.g., asphalt, bricks, glass, plastic, etc.), which ultimately result in a highly impaired soil (Craul, 1985; Gregory et al., 2006; Beniston and Lal, 2012). These disturbed soils are characterized by soil compaction, increased bulk density, reduced water infiltration rates and aeration, low water holding capacity, low organic matter content and low fertility, which ultimately limit crop growth and yields and create major constraints for productive urban agriculture (Gregory et al., 2006; Beniston and Lal, 2012).
EQ biosolids can provide organic matter and nutrients necessary to improve anthropogenic soils and promote vegetative establishment and growth. EQ biosolids are by-products of wastewater treatment generated by Processes to Further Reduce Pathogens (PFRPs) and possess low vector attraction and pollutant concentrations. Such products are suitable amendments for landscapes having high public access. Research needs for these newly developed products include quantifying N and P availability and C sequestration potential in anthropogenic soils. Initial work on these questions has been performed (Alvarez-Campos et al., 2018; Alvarez-Campos and Evanylo, 2019a; Brown et al., 2016c), but additional research is needed to ensure appropriate EQ biosolids management for urban landscapes. Biosolids blended with compost and/or other residuals have been shown useful in reducing exposure to Pb contaminated soil, improve soil health and protect public health (Obrycki et al., 2017). The multistate research group will continue research to improve our understanding of this in field studies, focusing on changes in soil attributes that may have implications for altering soil quality/health (e.g., soil physical, chemical, nutrient, and biological characteristics).
Another area of concern is the fate of residuals-borne trace organic chemicals (TOrCs) in soils. Classes of TOrCs include estrogenic compounds, personal care products, pharmaceuticals, and PFAS (Codling et al., 2018; Dalahmeh et al., 2018; Stahl et al., 2013; Sepulvado et al., 2011; Topp and Colucci, 2004; Xia and Pillar, 2004). A wide range of TOrCs in streams in the vicinity of wastewater treatment plants and concentrated animal feeding operations (CAFOs) has been frequently reported. The fate and persistence of these compounds during biosolids stabilization processes or following land application of residuals and non-potable water are not fully understood. Persistence in soils and potential for ecosystem effects as a result of residuals and non-potable water land application is a critical area requiring additional research.
In particular, some of the greatest challenges for expanding the beneficial use of treated wastewater effluent and residuals (such as biosolids) are concerns associated with PFAS. Based on this extensive use in a myriad of products, their existence in the environment is ubiquitous. Land application of biosolids has been shown to disseminate PFAS in soils and subsequently be assimilated by crops and leached to groundwater (Bizkarguenaga et al., 2016; Blaine et al., 2014a, b; Lee et al., 2014; Lindstrom et al., 2011; Sepulvado, et al., 2011; Navarro et al., 2017). Biological degradation processes that often time reduce TOrC loads in wastewater treatment and in land-applied biosolids do not alleviate PFAS load since any microbially degradation that occurs leads to persistent perfluoroalkyl acids (PFAAs) (Liu et al., 2013). Even small contributions of PFAAs to water sources can be problematic from a management standpoint given the low ppt levels being proposed or already implemented as screening levels or actual health advisory levels by some states (e.g., 14 ppt in NY). On March 22, 2019, Maine’s Department of Environmental Protection (DEP) released a memorandum requiring sludge/biosolids program licensees and sludge/biosolids composting facilities to test their materials for PFAS. Any material exceeding the screening standards set for three PFAAs (2.5 mg/kg PFOA, 5.2 mg/kg PFOS or 1,900 mg/kg PFBS) may not be land-applied until DEP approves otherwise.
A final area of concern for the W3170 workgroup has been on irrigation water sources. This particularly holds true due to the fact that fresh water sources for irrigation are limited, and impending water shortages are motivating the intentional reuse of degraded waters (O’Connor et al., 2008; Blaine et al., 2014b). While the application of treated or partially treated wastewater effluents to cropped and forested lands has long been practiced, other sources of degraded water (stormwater, irrigation return flow, gray water, and CAFO effluents) are available to meet anticipated shortfalls. In conjunction with degraded water use, soil application of residual products may, in fact, have positive effects on drought-stressed crops. Several researchers have noted improvements in crop drought tolerance and crop yield with the biosolids land application (e.g., Cogger et al., 2013; Heckman et al., 1987; Zhang et al., 2005), with speculation geared towards improvements in soil water relations (Brown et al., 2011). Since many of the major reuse opportunities involve water applications to soil systems (e.g., irrigation), with or without residuals application, addressing the benefits, risks, and sustainability of degraded water reuse logically fits within the scope of the proposed research project.
In summary, there is a continued need for research on contaminant lability and bioavailability in both residual-amended soils and in contaminated soils restored with residuals. New ecological endpoints must be investigated to improve risk assessment to ensure human health and environmental safety. Research is also needed to maximize yields via the use of residuals and reclaimed wastewater. Both offer potential alternatives to current, sometimes environmentally detrimental, agricultural and land restoration practices. The experience and expertise of W3170 in addressing these issues are widely recognized and W3170 is well positioned for continued success. The W3170 membership offers the advantage of including institutions and entities (universities, USDA-ARS, US EPA, municipal government utilities) from across the entire US. Such collaboration enables discoveries to account for widespread differences in climate, soils and residual types and sources, whose fate, transport and impact will vary due to across these factors.
Related, Current and Previous Work
W3170 Objective 1. Evaluate the short- and long-term chemistry and bioavailability of pharmaceuticals and personal care products, persistent organic contaminants, and pathogens in residuals, reclaimed water, and amended soils in order to assess the environmental and human health risk-based effects of their application at a watershed scale.
Early work on the effect of residuals application to agricultural or undisturbed soils focused on changes in total metal and plant-available metal concentrations. As our understanding of ecosystem function and nutrient cycling has become increasingly sophisticated, additional measures that include a wider range of endpoints are being included in research efforts. W3170, the premiere US residuals reuse workgroup, has continued to focus efforts on cutting-edge topics such as reductions in TOrCs associated with beneficial use of residuals (e.g., solids, semi-solids, reclaimed waters, wastewaters) in amended soils.
Fate of residuals-borne trace organic chemicals (TOrCs) in wastewaters and residuals-amended soils. Classes of TOrCs include estrogenic compounds, pharmaceuticals and personal care products (PPCPs) and per- and polyfluoroalkyl substances (PFAS) (Codling et al., 2018; Dalahmeh et al., 2018; Stahl et al., 2013; Sepulvado et al., 2011; Topp and Clucci, 2004; Xia and Pillar, 2004). Quantifying the persistence and fate of TOrCs in soils and evaluating their impacts to soil health and the ecosystem are critical areas requiring additional research. The W3170 workgroup has been consistently diligent in studying the fate and effects of TOrC for a wide array of scenarios.
Antibiotics are frequently found in soil and water environments, thus the potential to exert selective pressure on surrounding bacteria for development and enrichment of antibiotic resistant strains. Xu et al. (2014) and Zhang et al. (2014a,b) used an E. coli bioreporter to investigate the uptake of the antibiotic tetracycline (TET) and evoke antibiotic resistance genes from solution under varying solution conditions. Tetracycline is ionizable resulting in several species altering charge location and net charge. Antibiotic resistance gene expression in the E. coli bioreporter responded linearly to intracellular TET levels. Mg2+ and Ca2+ in solutions reduced TET uptake and organic acid ligands enhanced TET uptake by E. coli. Zwitterionic TET was identified as the predominant species that most readily passed through the cell membrane eliciting activation of the antibiotic resistance gene in the E. coli.
Xia and coworker (Garner et al., 2018) investigated the potential for wastewater reuse to disseminate antibiotic resistance genes (ARGs). Samples were collected seasonally in 2014−2015 from four U.S. utilities’ reclaimed and potable water distribution systems before treatment, after treatment and at five points of use (POU). Shotgun metagenomic sequencing was used to profile the resistome (i.e., full contingent of ARGs) of a subset (n = 38) of samples. Certain ARGs were elevated in all (sul1; p ≤ 0.0011) or some (blaTEM, qnrA; p ≤ 0.0145) reclaimed POU samples compared to corresponding potable samples. Bacterial community composition was weakly correlated with ARGs (Adonis, R2 = 0.1424−0.1734) and associations were noted between 193 ARGs and plasmid-associated genes. Results indicate that reclaimed water could convey greater abundances of certain ARGs than potable waters and provide insight into controlling factors of ARG occurrence in reclaimed water systems.
Qin et al. (2017) investigated deactivating antibacterial activity using Fe3+-saturated montmorillonite in Virginia municipal wastewater effluents. Microbial deactivation efficiency was similar UV-disinfected effluent. Furthermore, 99.6-99.9% of total coliforms, E. coli and enterococci in a secondary wastewater effluent were deactivated with a 1-h exposure to Fe3+-saturated montmorillonite. These results demonstrate the potential use of Fe3+-saturated montmorillonite in both small-scale point-of-use drinking water treatment devices all the way to large-scale drinking and wastewater treatment facilities.
Xia and coworkers (Oh et al., 2018) tested MetaCompare, a publicly available tool for ranking ‘resistome risk’, which is defined as the potential for antibiotic resistance genes (ARGs) to be associated with mobile genetic elements (MGEs) and mobilize to pathogens. A computational pipeline is being developed in which each ARG is evaluated based on relative abundance, mobility, and presence within a pathogen. Previously published metagenomic data derived from distinct aquatic environments were tested. Based on unsupervised machine learning, the test samples clustered in the hazard space in a manner consistent with their origin. The derived scores produced a well-resolved resistome risk ranking of wastewater hospital sewage > dairy lagoon effluent > wastewater treatment plant effluent.
Minimizing the presence of TOrCs coming in our wastewater or treating wastewater streams prior to their discharge or use as well as knowing when treatment is needed are all important ways to minimize environmental contamination. Chao et al. (2015) and Qin et al. (2017) demonstrated adding Fe3+-saturated montmorillonite to catalyze rapid 17b-estradiol transformation (98%) into less harmful oligomers., which were found to be >107 times less water soluble, thus, less bioavailable and mobile.
Pepper’s research group at the UA (Sherchan et al., 2013; Sherchan et al, 2014; Pepper and Gerba, 2018; Pepper et al., 2018) evaluated the efficacy of real-time on-line sensors for use as a process control for advanced treatment of reclaimed water prior to subsequent potable reuse. Absorbance at 254 nM and total fluorescence and excitation/emission fluorescence measurements (EEMS) provided the most effective process control for PPCPs. Light emission via the ATP luciferin/luciferase system showed the most potential for process control of microbial contaminants. Implementation of control measures can rapidly reduce loads coming in our wastewater treatment plants. Recently, Krogh et al. (2017) and Brose et al. (2019) (Brose et al., 2019; Krogh, Lyons, & Lowe, 2017) found statistically significant decreases in triclosan and/or triclocarban concentrations in wastewater influent in 2014 compared to previous years, which could attributed to a policy change by the U.S. FDA affecting the source of these compounds in consumer products.
In the wastewater treatment (WWT) process, many TOrCs become concentrated in WWT residuals and thus enter the environment when residuals are land-applied for their nutrient and carbon value. Dr. Linda Lee and her team (Purdue Univ.) analyze 33 TOrCs in commercially available biosolids-based and non-biosolids-based fertilizer products. TOrCs included synthetic musks and fragrances (SMFs), PFAS, and PPCPs. Triclosan and triclocarbon, typically present in many PPCPs, were found at the highest levels of 285-20,000 mg kg-1 and 190-17,800 mg kg-1, respectively. BPA alternatives were found in all products with BPA being highest (468 - 68,200 mg kg-1) followed by BPS, TBBPA and BPAF. In aerobic soil biodegradation studies, BPS degraded rapidly with t1/2 <1 d; however, BPAF persisted (t1/2 ~4.5-6 weeks). The pharmaceuticals found included diphenhydramine > miconazole, cimetidine > ciprofloxacin > ibuprofen. Only one estrogen was detected, estrone (the primary estradiol metabolite) along with two UV filters used in sunscreens and as a UV protectant in food-grade plastics. For the SMFs, tonalide and galaxolide were osbseved at high concentrations. Nonylphenol ranged from limited detection in composted biosolids to several thousand in some of the other products. Parabens, which are naturally occurring, were observed in all products.
Lee and coworkers (Purdue) did more detailed studies focused on the occurrence of perfluoroalkyl acids (PFAAs) and other PFAS in the waste-based fertilizers including biosolid-based materials and the composted (organic fraction) municipal solid wastes (OFMSW), e.g., yard trimmings, food wastes, food packaging, etc. Total PFAA loads in OFMSW composts were about half of what was observed in biosolids-based products (Choi et al.., 2019; Lazcano Kim et al., in review). Perfluorooctanoic acid (PFOA) and perfluorooctanesulfonate (PFOS) were detected in all biosolids-based fertilizers and all OFMSW composts; however, OFMSW composts were dominated by short-chain PFAAs (>64%) of which > 68% were perfluoroalkyl carboxylates, particularly the C6 PFCA. Of the total PFAA load in the OFMSW composts, 25−49% was released to porewater (∼1 g/2 mL 48-h static contact time) (Choi et al., 2019), which was generally similar to what was observed with the biosolids-based fertilizer. PFAA porewater concentrations versus PFAA loads as well as OC-normalized sorption coefficients versus the number of PFAA CF2 units are strongly correlated (R2 > 0.85) and similarly for the biosolids-based fertilizers (R2 > 0.8). Heat-treatment, composting, blending, and thermal hydrolysis of biosolids did not reduce PFAA loads except for blending due to dilution (Lazcano Kim et al., 2019). Total PFAA concentrations increased in heat-treated and composted products, which was likely due to the breakdown of PFAA precursors during post-treatment. Several PFAA precursors were identified in samples before post-treatment. PFAA levels change with different post treatment processes.
Routine application of biosolids, which are nutrient-and carbon-enriched materials, to soils improves fertility and other characteristics, but the presence of TrOCs in amendments raises questions about potential adverse effects on biota and in the case of antibiotics, the development of ARGs. Bioavailability and hazard risks are largely dictated by sorption-desorption and diffusion behavior in amendments. Tian et al. (2016), Silveira et al. (2017) and Sidhu et al (2019c) assessed the phytoavailability of two biosolids-borne antibiotics, ciprofloxacin (CIP) and azithromycin (AZ) in range-finding and greenhouse studies using lettuce, radishes and fescue grass using media represented differences in exchange capacities and organic matter content. Plant uptake and toxicity from biosolids-borne CIP and AZ were negligible even in a worst-case scenario (i.e., sand as a growth medium) at the high end of environmentally relevant concentrations. Plant data are consistent with the hypothesis that compound retention limit biosolids-borne CIP and AZ bioaccessibility and bioavailability to plants and other terrestrial organisms. Likewise, research at Purdue University also found little to no degradation in biosolid-soil mixes for four model biosolids-borne contaminants (carbamazepine, miconazole, triclocarban and triclosan in temperature and moisture-controlled aerobic microcosms over 180 days. Comparisons with spiked soils data further suggests that the biosolids matrix limits TOrC bioavailability relative to lab-spiked soil. O’Connor et al. also found this to be true when evaluating CIP and AZ in sorption/desorption studies by spiking antibiotics to soils amended with or without biosolids and a second approach where a biosolids amendment was pre-equilibrated with CIP and AZ prior to soil application (Sidhu, O’Connor et al., 2019a). Biosolids-borne CIP and AZ behavior was dominated by biosolids-sorption with limited desorption potential. In assessing bioavailability and toxicity potentials of biosolids-borne CIP and AZ to earthworms neither compound was toxic to earthworms even at much greater than environmentally relevant concentrations, but both accumulated in the earthworms (Sidhu, O’Connor et al., 2019b). Potential effects of biosolids-borne CIP and AZ on soil microorganism health was assessed based on microbial respiration and genes involved in N and P cycling in a 90-day aerobic incubation study (Sidhu, O’Connor et al., 2019b). Initially, environmentally relevant concentrations of biosolids-borne CIP and AZ did adversely affect at least a few microbes in biosolids, but to a much less extent in biosolids-receiving soils. The adverse effects were minimal on microbial respiration and on genes involved in N and P cycling. Inhibition of bacterial amoA expression indicated that both TOrCs can stress microbes, at least initially, and minor TOrC concentration-induced increases in ARG expressions occurred (qnrS, mefE, ermB) suggesting that stress-induced ARG development and spreading may be facilitated by biosolids.
Longer-term (especially field) studies using various Class A and Class B biosolids and quantitative frameworks are needed to fully assess potential biosolids-borne TOrC impacts on microbial resistance. A tiered integrated risk assessment for biosolids-borne CIP and AZ was conducted (Sidhu, O’Connor et al., 2019c) and biosolids-borne triclosan and triclocarban (Snyder and O’Connor, 2018) using the framework developed by WHO (2001). The initial screening identified three pathways of concern: 1) Biosolids ® soil ® plant; 2) Biosolids ® soil-soil organism; and 3) Biosolids ® soil ® soil organism ® predator. Reasonable refinements of the screening level assumptions resulted in negligible estimates of risks of CIP and AZ in all pathways to both human and ecological health under real-world biosolids application scenarios, and calculation of preliminary pollutant limits. The analysis does not include a pathway to address antibiotic resistance development, which will require long-term field investigations and more quantitative frameworks to assess associated risks. For triclocarbon, screening-level hazard quotients (HQs) were <1 for 14 pathways and no HQ values were >1 in human pathways. Screening-level HQs were >1 for soil organism predators and aquatic organisms.
D’Angelo and Starnes (2016) in complimentary research also showed that the hazard risks associated with application of antibiotic laden-biosolids to soils largely depends on release rates from biosolids particles, which is governed by the fraction of the total concentration that can be released to solution and diffusion rate in the biosolids aggregates. These processes were determined for CIP in a Class A municipal biosolids (D’Angelo and Starnes, 2016) and later for TET in municipal biosolids, poultry manure, wood chip litter, and rice hull litter (D’Angelo, 2017) using a combination of sorption-desorption isotherms, diffusion cell, diffusion gradient in thin films (DGT) and numerical modeling approaches.Depending on amendment, TET sorption increased 4-40 times when treated with alum. Differences in TET sorption by amendments was strongly explained by an exponential relationship to Mehlich-3 Al3+ in amendments (R2=0.94).
W3170 members at PSU (Elliot) and in collaboration with Purdue (Lee and Mashtare) have performed several types of studies on the TOrC fate and transport. The team studied three vernal pools impacted by spray-irrigated wastewater effluent to assess the impact of weekly irrigation on the occurrence, persistence and fate of estrogens five estrogens in an 8-week study. Nearly 100% of the daily samples (n>130) collected contained estrogens, and the concentrations were several times higher compared to the wastewater (Kibuye et al., 2019; Mina et al., 2018). Data suggest transformation of estrone back to the more estrogenic 17a- and 17b-estradiol likely due to anaerobic conditions similar to observed for land-applied manure and animal lagoon effluent irrigation (Gall et al., 2011; 2013). A field study was also conducted to compare surface broadcast and shallow disk injection for dairy manure application on estrogen transport in surface runoff (Mina et al., 2017). The estrogen loads leaving the fields that had received manure applications via shallow disk injection were an average of two orders of magnitude lower than the loads leaving the fields that had received manure via surface broadcast. The team also developed a Hormone Export and Recovery Dynamics (HERD) model, a hydrologic and biogeochemical model that predicts the fate and transport of estrogens in tile-drained fields receiving animal residual applications (Gall et al., 2016). HERD simulations suggested that manure application history matters to adequately predict field observations and that the long-term application of animal wastes may result in the build-up of legacy sources within the soil profile that could result in a water quality recovery lag time on the order of a few decades similar to nutrient recovery lag times.
Kibuyea et al. (2019) conducted a 14-mo study to evaluate the fate and potential risks of seven commonly used PPCPs in a local WWTP and from 13 groundwater monitoring wells at a spray-irrigation site where effluent has been spray-irrigated since the early 1980s. Acetaminophen and trimethoprim were the most frequently detected (93%) PPCPs in WWTP influent, while in the effluent, caffeine and trimethoprim were detected most frequently (70%). WWT generally reduced concentrations of acetaminophen and caffeine by >88%; however, some compounds had low removal or were present at higher concentrations in the effluent compared with influent (e.g. naproxen, sulfamethoxazole, trimethoprim and ofloxacin). Risk calculations suggest that the risk posed by PPCPs that persisted in the effluent are medium to high to aquatic organisms. Detection frequencies and concentration magnitudes of PPCPs were lower in groundwater samples compared to effluent, Human health risk assessments indicate that concentrations in groundwater appear to pose minimal risk.
Chao et al. (2015), Kulesza et al. (2016) and Chen and Xia (2017) conducted rainfall simulations on plots receiving three manure treatments (surface application, subsurface injection, and no manure control) to determine the fate and transport of five different antibiotics commonly used in dairy production. Surface application, compared with subsurface injection, resulted in 80-97% greater mass loss of all five antibiotics investigated during a rainfall event after the manure application. Horizontal and vertical diffusion of antibiotics were greatest for plots receiving rainfall immediately, compared to rainfall at day 3 and 7 after manure application. Le et al., (2018) also conducted rainfall simulations to test the surface runoff of four dairy production antibiotics of plots receiving liquid dairy manure via surface application and subsurface injection at different time gaps (day 0, 3, and 7) between manure application and a subsequent rain event. Liquid dairy manure spiked with pirlimycin, tylosin, chlortetracycline, and sulfamerazine was applied to 1.5x2 m test-plots at an agronomic N rate via surface application and subsurface injection. Runoff was a significant route for transporting antibiotics from manure-applied fields with subsurface injection substantially reducing antibiotic losses in runoff as did manure application three days or longer before a subsequent rain event.
In antibiotics-containing manure from treated animals and composted the manure using the FDA recommended static and turned techniques, all antibiotics, except pirlimycin, disappeared following a bi-phasic first-order kinetics. However, individual antibiotics displayed different fate patterns in response to the treatments. Reduction in concentration of chlortetracycline (71 to 84%) and TET (66 to 72%) was substantial, while near-complete removal of sulfamethazine (97 to 98%) and pirlimycin (100%) was achieved. Tylosin concentration was highly variable and its removal during composting was poor. After incubating for 120-days, regardless of the compost type (static vs. turned), antibiotic concentrations in compost-applied soils were significantly lower (p<0.0001) than those in the manure-applied soils. Soil type had no significant effect on antibiotic dissipation rates (p>0.05) (Chao et al., 2015; Kulesza et al., 2016; Chen and Xia, 2017).
Xia and coworker (Chen et al., 2018; Wind et al., 2018) compared the effects of crop (lettuce or radish), soil amendment type (inorganic fertilizer, raw dairy manure, composted dairy manure, or no amendment), and prior antibiotic use of manure-derived amendments on the incidence of culturable antibiotic-resistant fecal coliforms in agricultural soils through a controlled field-plot experiment. Antibiotic-resistant culturable fecal coliforms were recoverable from soils across all treatments immediately after application, although persistence throughout the experiment varied by antibiotic class and time. Compost-amended soils had the highest levels of cephalosporin resistant fecal coliforms, regardless of whether the cows from which the manure was derived were administered antibiotics. This study demonstrated that antibiotic-resistant fecal coliforms can become elevated in soils receiving manure-derived amendments. Composting can reduce initial antibiotic inputs, while degradation in the rhizosphere further enhances dissipation of some antibiotics. Gene markers for antibiotic resistance and mobility remained higher in the compost-amended soils than in chemically-fertilized soils at harvest time.
The W3170 workgroup has also focused some attention on residuals treatment technologies and removal of pathogens. Dr. Ian Pepper et al. UA developed a new method for converting Class B biosolids to Class A (Kitajima et al., 2014; Schechan et al., 2014). One new treatment involved the use of the soil fumigant sodium metam used for human root crops, which was shown to kill both human viruses (e.g., poliovirus) and helminths (e.g., Ascaris). Lab and field evaluation showed that sodium metam use resulted in a 4.7 log reduction of fecal coliforms, 2.6 log reduction of Ascaris and 4 log reduction of poliovirus, which resulted in its approval by the EPA Pathogen Equivalency Committee as a “Process to Further Reduce Pathogens” (PFRP) (Rachmadi et al., 2016; Schmitz et al., 2016b).
Pepper et al. (AZ) evaluated 4 E.coli hosts recommended by the U.S. EPA for male specific and somatic phage detection as an indicator for enteric viruses during wastewater treatment. They collected wastewater samples from three wastewater treatment trains in two plants and analyzed phage incidence through cultural assay. Key findings were: the double agar overlay assay is superior to the single agar overlay; the E.coli host CN-13 for somatic phage may be the optimal host for cultural coliphage detection; and the Bardenpho process results in greater removal of coliphage than conventional activated sludge or trickling filters. The group also monitored viruses in raw influent and final treated effluent using qPCR for a year at four wastewater treatment plants with secondary treatment consisting of trickling filter, activated sludge, or a 5-stage Bardenpho process. Monitoring data provided an indication of relative abundance (incidence), seasonal variation, and the extent of removal during wastewater treatment. Based on these criteria, the pepper mild mottle virus appeared to be best candidate for use as a model viral indicator of sewage pollution. In addition, the Bardenpho process was shown to be more efficient than the trickling filter or activated sludge process for virus removal (Schmitz et al., 2016). The Pepper research group (Sassi et al., 2018a,b) used viral surrogates to evaluate ebola fate during flushing toilets. The Corona virus was utilized based on the it having the same viral order as Ebola, nucleic acid type and lipid content. Murine norovirus was also utilized as a “worse case” for virus survival. Medical waste containing the virus was flushed down the toilet with and without disinfection. Data showed that flushing medical waste containing the virus can result in extensive contamination due to aerosols. However flushing with half a cup of bleach eliminated restroom and toilet contamination.
W3170 Objective 2. Evaluate the uses and associated environmental benefits for residuals and wastewaters in various ecosystems (e.g., agricultural, urban, recreational, forest, rangeland, mine-impacted, disturbed, degraded) with respect to changes in soil physical, chemical, biological, nutrient, and trace/heavy metals with respect to soil quality/soil health.
Some of the major soil properties that influence contaminant solubility, bioavailability, and exposure to ecological and human receptors include physical (soil bulk density, clay type and content, aggregate stability, and porosity; chemical (pH, salts, non-essential heavy metal(oid)s, and redox potential; microbial (microbial biomass C, mineralizable N content, macrofauna and microbial activity and function; plant-available essential nutrients with organic matter being the soil physical/chemical/biological/nutrient nexus. he premiere US residuals reuse workgroup, W3170, has continued to focus efforts on cutting edge topics related to beneficial use of residuals, reclaimed waters, wastewaters, and amended soils associated with agronomic and environmental benefits to aid in developing a soil health framework with respect to residuals land application.
The application timing, fate and effects on above ground plant growth of land-applied, residuals-based nutrients continues to be studied under a variety of environmental scenarios. Bamber et al. (2016) compared the N availability, use efficiency and leaching potential in a two-application timing strategies of lime stabilized and anaerobically digested biosolids with routine inorganic N fertilization practices for wheat in the Virginia coastal plain. Grain yields, N use efficiency, and N recovery for both biosolids products with both application timing strategies were greater than or equal to inorganic fertilizer on soils of varying textures. The advantages of biosolids over inorganic fertilizer occurred whether the residuals were applied all at planting or 50% at planting with the remaining 50% agronomic N rate topdressed at the end of winter. On coarse-textured soil, the split anaerobically digested biosolids-fertilizer treatment increased yield and N use efficiency above that of the 100% agronomic anaerobically digested biosolids N rate at planting, but there were no differences between timing strategies in finer-textured soils or for lime-stabilized biosolids.
Newly developed EQ biosolids products specially tailored for urban soil were compared with established products to assess their capability to support germination and plant growth, as well as to quantify their plant available nitrogen (N) and phosphorus (P) (Alvarez-Campos, Evanylo et al., 2019). Seven EQ biosolids products derived from treatment processes such as thermal drying, com-posting, and blending with complementary organic and mineral materials and an inorganic fertilizer control were compared in greenhouse bioassays employing soybean (Glycine max L.) and tall fescue (Festuca arundinacea Schreb.). The biosolids applied at estimated agronomic N rates enabled adequate germination and plant growth. N uptake by tall fescue grown with the biosolids amendments compared with known rates of inorganic N confirmed organic N availability to be approximately 40%, 20%, and 15% for thermally dried, blended, and composted EQ biosolids products, respectively. EQ biosolids were compared to a synthetic fertilizer on urban soil properties and vegetable yield in a field study (Alvarez-Campos and Evanylo, 2019b). Three EQ biosolids were applied bi-annually at agronomic nitrogen (N) rates (fall 2016, 2017, and summer 2017, 2018), and annually at land reclamation rates (5x agronomic N rate; fall 2016-2017). A heat-dried and pelletized EQ biosolids product and inorganic fertilizer were applied bi-annually at agronomic N rate only. Compared to the inorganic fertilizer, the biosolids increased soil C by 37% to 84% after two years; lowered soil N availability likely due to low N mineralization OC was low and clay concentrations high; and increased yields of cabbage and kale yields (fall 2017). Despite limiting soil physicochemical conditions, biosolids addition at reclamation rates showed greater potential to increase vegetable yield after two years of application.
Badzmierowski, Evanylo et al. (2019) conducted a 3-yr field study comparing the effects of irrigation rate and fertility treatments on tall fescue (Festuca arundinacea Schreb.) growth and quality and properties of a post-development soil. Greater turfgrass yield and quality, higher soil C and macro- and micronutrient concentrations and reduced soil bulk density were observed for biosolids-blended products compared with synthetic fertilizer. Applying biosolids at the agronomic P rate plus supplemental fertilizer N did not yield desirable turfgrass quality; however, applying at the agronomic N rate continuously may lead to potential P loss if rates are not reduced.
Ippolito (2015) completed a study on the beneficial reuse of Al-Water Treatment Residuals (WTR) in an engineered urban wetland in Boise, ID. Al-WTR application significantly increased P capture and lessened offsite P movement into adjacent waterbodies. Ippolito et al. are currently working on a similar project with the City of Fort Collins, CO as well as studying Al-WTR’s ability to sorb organic P from swine wastewater containing ~ 7000 mg organic P L-1. Almost all organic P (99.9%) was sorbed within 1 day resulting in the resulting Al-WTR composite having potential to release 380 to 485 mg total P kg-1. A greenhouse trial is underway to compare this Al-WTR composite material to a common P fertilizer. In New Mexico, Xu et al. also has continued investigating the adsorption capacities of metals and metalloids by WTR.
In two replicated experiments, Pietrzykowski et al. (2015) studied the long-term (10 to 15 year) effects of woody waste compost additions on soil and vegetation properties in created forested wetlands in eastern Virginia at different loading rates. Compost additions clearly aided initial establishment and long-term growth of herbaceous and woody vegetation (Betula nigra and Quercus palustris) at the loading rate experimental site, but had no net effect at the second experiment when compared with topsoil return. However, installation of microtopographic variability (e.g. pits and mounds) had very strong effects on tree growth (Taxodium distichum) at this sandy site. Overall, optimal compost addition rates are 75 to 224 Mg/ha, depending on long-term management goals.Lower rates are adequate for development of appropriate (low) soil redox conditions. However, meeting certain regulatory goals (e.g., 5% OM in surface A horizons) may require the higher loading rates.
Ippolito and his research team (Colorado) found that certain biochars can stabilize extracellular enzymes necessary for nutrient cycling and maintain or enhance microbial community activities when applied with or without manure (Elzobair et al., 2016a,b), improve nutrient aspects, hydraulic properties, and reduce greenhouse gas emissions when applied along or in conjunction with manure (Ippolito et al., 2016a,b; Kammann et al., 2017; Laird et al., 2017; Spokas et al., 2017), and sorb/sequester heavy metals, improve plant productivity, and overall soil health in biochar amended systems (Mehmood et al., 2017), including mine spoils (Novak et al., 2016; Ippolito et al., 2017).
The fate of land-applied residuals-borne trace and heavy metals, and metalloids, have also been extensively studied decades. For example, the long-term benefits of biosolids additions to dryland winter wheat agroecosystems has been studied for 37 years in CO. At agronomic rates based on N needs of wheat, Barbarick et al. (2015) found that uptake coefficients for nutrients and trace metals in biosolids-amended soils were much lower than those used for risk analysis by the US EPA. Furthermore, when biosolids land application occurs in dryland agroecosystems, biosolids tend to concentrate Cu and Zn in the soil surface preventing appreciable downward movement (Ippolito et al., 2015). More importantly, Zn addition may be beneficial in semi-arid cropping systems such as those found in eastern CO, as plant-available Zn soil concentrations may be close to or below sufficient concentrations for plant growth. Barbarick et al. (2016) furthered their biosolids research to determine direct and indirect influences on grain concentrations and cumulative uptake of Cu, Fe, Ni, P, and Zn and that Zn application across biosolids amended sites may offset potential Zn deficiency symptoms present in semi-arid soils consistent with Ippolito et al. (2015).
Hettiarachchi’s team at KSU, in conjunction with other W3170 members (Brown, UW; Chaney, USDA-ARS; Basta, OSU; Scheckel, USEPA), evaluated the long-term bioavailability of As, Cd, Pb, and Zn in mildly contaminated urban brownfields soils and mine waste materials amended with different organic amendments (manures, various compost types, composted and non-composted class A biosolids products). X-ray absorption spectroscopy and in vitro bioaccessibility extraction tesst (also known as Physiologically-Based Extraction Test) showed that the dominant Pb species in the urban soils were Pb adsorbed to Fe oxy(hydr)oxides (Pb-Fh) and Pb adsorbed to organic C (Pb-Org C), which increased as soil-compost mixture aged in the field. During the in vitro extraction test that Pb initially sorbed to Fe oxy(hydr)oxides dissolved, and Pb-org C and hydroxypyromorphite were formed. Aged soil-compost mixture reduced Pb-Fh dissolution during the in vitro extraction (Defoe et al; 2014; Attanayake et al., 2015; Brown et al., 2016a; Henry et al., 2015; Attanayake et al., 2017).
Brown et al. (2017) co-authored a book chapter summarizing carbon accounting associated with mine land reclamation. Examples included biosolids, coal bottom ash, and pulp sludge for restoring a range of disturbed sites. Carbon accounting typically considers increases in soil carbon as a result of mine site restoration as well as fugitive emissions avoidance for diverting organics from landfills to beneficial use. Benefits of inorganic materials such as coal ash for reclamation was included in this work.
Arsenic is one of the most common contaminants of concern exceeding risk criteria with with As-soil contaminated soil ingestion being the primary human health risk driver at many urban, military, U.S. Brownfields and CERCLA sites. Use of total contaminant content instead of bioavailability is often overly conservative and can result in costly and unnecessary soil remedial action. Basta et al. (2016), Obrycki et al. (2017) and Whitacre et al. (2017) completed several projects that determined the ability of in vitro bioaccessible (IVBA) methods to predict relative bioavailable (RBA) As, which resulted in four primary conclusions. First, total soil As concentration was not correlated with RBA as determined by adult mouse (r2=0.24) or the juvenile swine (r2 = 0.09) bioassays. Secondly, all of the IVBA methods generally were predictive of RBA for both bioassays. Thirdly, IVBA arsenic gastric extraction GE) is a better and more conservative predictor than IVBA arsenic from the intestinal extraction. Lastly, the California Bioaccessibility (CAB) method was developed to provide a conservative estimate of RBA As on CA mining site soils, which was was an accurate predictor of swine RBA As for soils with high oxide content and soil As concentrations <1,200 mg As/kg.
In Hawaii, crops such as taro and watercress are grown in flooded soils where As can become more available due to reducing conditions and microbial activity. Thus, Hue (2015) evaluated the phytoavailability of arsenic (As) in agricultural soils that have had a long history of sodium arsenite application, which has been used extensively as a herbicide between 1913 and 1945. As accumulation in watercress (Nasturtium officinale) was measured in greenhouse studies (Hue, 2013).The Mehlich-3 extraction was found to be reasonably and positively correlated to bioaccessible As based on As concentration in fern fronds. Hue (2015) identified three primary processes having potential to reduce As toxicity and availability: (1) oxidation of As(+3) to As(+5), (2) methylation by bacteria and fungi, and/or (3) extraction by plants (phytoremediation). This review presents detailed information about these remediation processes.
Developing management recommendations for contaminated urban systems is necessary to address public questions regarding best practices for using urban soils for food and recreation. Brown and co-workers (Brown et al., 2016c,d) have co-edited a 2-volume series on urban agriculture entitled Sowing Seeds in the City. The first focuses on ecosystem and municipal services. It includes chapters on use of alternative water (stormwater, grey water and reclaimed water), residuals use (biosolids, food and yard waste) and greenhouse gas implications of residuals use in urban agriculture. The second focuses on human health and includes contributed chapters from Basta (OSU) and Hettiarachchi (KSU).
Brown et al. (2016b) have been defining standards for green stormwater infrastructure in urban areas. Green stormwater infrastructure relies on enhancing soil/plant systems’ ability to both allow stormwater to infiltrate and to remove contaminants from stormwater. These systems are being used both alone and in combination with grey or engineered systems as a way to limit combined sewer overflows in urban areas. They are also likely to enhance off peak stream flow and reduce the flashiness of urban streams. Current standards for these systems vary widely by location and appear to be more qualitative than quantitative. This research tested the importance of compost feedstock for removal of nutrients and metals and found that feedstock (manure, biosolids, yard/food scraps) was a poor predictor of system performance. They also tested the potential for the phosphorus saturation index (PSI) to serve as a better predictor of P movement in these systems. Furthermore, the team also demonstrated that Fe-containing water treatment residuals were beneficial in these systems. This builds on the literature and research done within the W3170 group (e.g., Basta – OSU; Elliott – PSU; O’Connor – UF; Ippolito - CSU) on the PSI as a tool for predicting P movement in biosolids amended soils.
Brown (2016) and Jay et al. (2017) tested the importance of compost feedstock in predicting the performance of stormwater bioretention soil mixtures in a replicated greenhouse trial using biosolids/yard waste-, animal manure/sawdust-, and food/yard waste-based composts. They investigated the movement of P (compared to the PSI predictions), N, metals and PAHs. This work builds on the literature and research done within the group, e.g., Basta (OSU), Elliott (PSU), O’Connor (UF) on the PSI as a tool for predicting P movement in biosolids amended soils as well as work done by Evanylo (VT) and Ippolito (CSU) on bioretention soil mixtures. Morris et al. (2017) worked with Dr. Sally Brown, as part of a team tasked by the Oregon DEQ to evaluate the impact of different food waste management alternatives. Composting, in-sink disposal, direct anaerobic digestion and combustion were considered. In addition to traditional Life cycle assessment parameters, this study included a section on the soil impact of each end use/disposal method (Morris et al., 2017).
Brown authored a pamphlet on how compost production and use can impact Climate Change per request of the US Compost Council in 2017, which provides an easy to understand guide on how land application of composts and other organics such as municipal biosolids can be an effective tool to both reduce fugitive gas emissions and to sequester carbon. Brown (2017) authored a chapter on the potential to create soils from urban wastes for a book on urban soil. The benefits and obstacles for diverting residuals to beneficial use were discussed and materials that would be suitable as feedstocks for soils and soil amendments were described.
Hacheney and Brown (2017) started a cooperation with inmates at the Monroe Correctional Facility in WA State. The inmates compost a majority of the food waste that is generated in the prison using Vermi -composting, Bokashi fermentation and black soldier fly composting. Dr. Brown co-authored an article for Biocycle with a prison inmate leading the compost program based on the data he generated. The cooperation is continuing with field plots with select garden vegetables where the relationship between soil health, yield and nutrient concentrations will be explored.
Basta et al. (2015), Carlson et al. (2015), Henry et al. (2015), Kaiser et al. (2015), Li et al. (2015), Nelson et al. (2016) and Obrycki et al. (2016) have performed focused investigations on the addition of phosphates to lead-contaminated soils as a management technique for reducing risk of Pb exposure in children. Pb contaminated soils (790 to 1,300 mg Pb kg-1) from a garden and a city lot in Cleveland, OH were incubated in a bench scale experiment for 1 year. Six phosphate amendments including bone meal (BM), fish bone (FB), poultry litter (PL), monoammonium phosphate (MAP), diammonium phosphate (DAP), and triple super phosphate (TSP) were added to pots at two application rates. Six phosphate amendments showed mixed results on their ability to reduce soil Pb bioaccessibility (IVBA Pb) and exposure risk to children. Soil amendments were largely ineffective in reducing IVBA Pb in these two urban soils when using EPA Method 1340. However, P treatments were much more effective when evaluated using modifications of EPA Method 1340. The greatest reductions in IVBA Pb were found at pH 2.5. Reductions in bioaccessible Pb from soil treatment ranged from 5-26% for the pH 2.5 extractions. A modified EPA Method 1340 that does not contain glycine and uses pH 2.5 rather than 1.5 has potential to predict efficacy of P soil amendments to reduce bioaccessible and bioavailable Pb.
University of Minnesota researchers (Jelinski et al.) screened 58 houses and over 2,500 soil samples from households across the Twin Cities for soil Pb via pXRF and wet chemical methods. Over half of investigated cores now have their maximum Pb concentrations at depths deeper than 10 cm, and in some cases to 20 or 40 cm, suggesting ongoing physical or chemical processes which are redistributing Pb in urban soils likely through biotic and abiotic processes. Therefore, decades-old predictions of the surficial Pb concentrations may now overestimate the current loading and risk of Pb at the surface. Understanding the depth distribution of Pb is also important for making better recommendations for urban agricultural uses of soil, where contaminant screening in the top portion of the soil may not accurately reflect the total Pb loadings available once the soil is mixed more deeply, or if plant roots reach those soil depths. The researchers are planning to select a 220-samples epresenting a moderate to high range in soil Pb and a range of depth and locations from an existing 600+ sample household soils archive. This subset will be assayed for soil Pb bioaccessibility by PBET, RBALP 1.5 and RBALP 2.5 methods as well as for soil organic matter, P and pH resulting in for bounding the range in variability of soil Pb bioaccessibility at the household scale and soil characteristics, which can be utilized to predict bioaccessibility.
Brown et al. (2016a) and Laidlaw et al. (2017) cooperated with a large group to address the hazards associated with Pb contamination in urban soils. Research summarized the use of biosolids to reduce Pb availability in situ. Potential transfer of Pb from soils to food crops was evaluated using peer reviewed literature. Direct ingestion of soil with elevated Pb was also considered. The authors developed practical guidelines to minimize any potential risk of exposure. Beyer et al. (2017) reviewed use of soil amendments including municipal biosolids and other residual based materials to reduce metal toxicity and restore ecosystem function to metal contaminated waste materials at mining sites. Conclusions drawn suggest that wider spread use of biosolids in urban areas has the potential to offer protection against Pb and other heavy metal exposure.
Hettiarachchi and her team evaluated the uses and associated agronomic and environmental benefits for residuals in agricultural and urban systems using lab, greenhouse and field research. Their research continues to be designed to assess the ability of residuals rich in C, P and Fe (for example non-composted or composted Class A biosolids) to reduce contaminant bioavailability in Brownfields. Chemical methods are used to evaluate reduction in risk from contaminants to human and ecological receptors. Research to date indicates that the potential exposure pathway of concern is direct exposure of contaminated soils to humans with the pathway from contaminated soil to plant to human being insignificant (Attanayake et al., 2015, 2017; Brown et al., 2016; Henry et al., 2016; Karna et al., 2016; Wijesekara et al., 2016).
The W3170 workgroup has also played a role in wastewater treatment and reuse, which ultimately will affect soil health quantification with respect to residuals land application. Following wastewater treatment, dewatering of biosolids (at Tres Rios WWTP in Tucson, AZ) results in ≃ 0.5 mgd of effluent with concentrations 1000 mg L-1 NH4. This effluent is returned to the head works and the high NH4 concentration results in a need for extra oxygen to be pumped into the effluent to enhance nitrification. This results in significantly enhanced energy costs (= 30% total energy costs). Therefore, there is a need to remove the NH4 prior to re-entry into the head works. A current W3170 project (Pepper et al.) evaluates an alternative method of removing the NH4 from the effluent using anaerobic oxidation of ammonia or anammox. Anammox relies on a consortium of autotrophic bacteria to oxidize NH4 utilizing nitrite as a terminal electron acceptor. The team is evaluating the efficacy of anammox as an alternative side stream treatment of effluent, and the influence of anammox on human pathogenic virus inactivation. The primary goal of the project is to develop a real-world anammox technology for sidestream treatment of effluent with high ammonia concentrations. A secondary goal of the project is to evaluate the incidence of human pathogenic virus in the dewatered water and potential inactivation by the anammox process and high ammonium concentrations.
Tian et al. (2016) evaluated fate of P in soils irrigated with secondary effluent for about 40 years. They performed a total phosphorus (TP) mass balance for adjacent cropped and forest sites that had been receiving wastewater irrigation for 40 years which indicated that 63 and 70% of net (applied minus harvested) TP could not be accounted for in the top 75 cm of soil in the field and forest, respectively. It is likely that surface runoff and subsurface lateral flow of effluent P, documented by other researchers, is partially responsible for the deficit of P in the 0-75 cm soil layer. Moreover, changes in the P-retention ability of the soil and the high hydraulic loading rate (irrigation plus natural precipitation of ~300 cm water per year) has probably caused leaching of P below the 75 cm depth. However, the surface Mehlich-3 P level stabilized at about 110 mg kg-1, indicating the soils have tremendous capacity to accommodate P-containing effluent without increasing the concern for runoff loss of P.
Singer and Brown (2018) began researching the impact of soil filtration on water quality for two types of reclaimed water. This was done to investigate the potential to use soil filtration as an alternative to engineered treatment as well as to see if sites permitted for irrigation could also be used to augment subsurface flows. One water was treated by sand filtration and the other was treated using a membrane bioreactor system. Both waters met Washington State standards for unrestricted use for irrigation. Metals, nutrients and estrogenic activity of the leachate were measured. In general, filtration through soil did not significantly alter water quality with the exception of greatly reduced estrogenic activity in the sand filter water. Results suggest that over irrigation using reclaimed water is a means to augment subsurface flows.
Plant systems have a significant capacity to remediate marginal waters through several phytoremediation processes including uptake (e.g., nutrients, trace elements), accumulation (e.g. salts) and assist with biotransformation of inorganic compounds (e.g., nutrients, trace elements). Salicornia could be a suitable halophilic plant to capitalize on its salt-tolerance potential for treating marginal waters. A greenhouse study was initiated to determine ability of Salicornia europaea to grow in flue gas desulfurization (FGD) wastewater, which is high in salts and selenium (Se), or brackish waters. Results showed 100% retention of Se with Se retained as stable species of reduced Se(IV), organic Se, and Se(0) as determined by bulk- and micro-Y-ray absorption near-edge spectroscopy. Selenium sorption competition occurred when in the presence of brackish water salinity (Galkaduwa et al., 2017; Paedez et al., 2017). Similarly, preliminary greenhouse work by Elliott et al. (PA; not yet published) established thresholds for negative effects on vegetation and soils from Marcellus Shale production water. The salinity threshold for negative effects on grasses (fescue and ryegrass) was an electrical conductivity of about 40 mS cm-1 for a single spill with periodic small rain events. Concomitantly, drought and diminishing freshwater have created a need for alternate water sources in arid areas of the US (e.g., NM).
Soil quality/health can be defined as the ability of soil to function, within natural or managed ecosystems, to sustain plant and animal productivity, maintain or enhance water and air quality, and support human health and habitation (Karlen et al., 1997). The concept of quantifying soil quality, based on various soil indicators, has been explored in a variety of ecosystems worldwide, e.g., agroforestry (Rousseau et al., 2012), forested/native vegetation vs agricultural settings (Tripathi et al., 2016; Cherubin et al., 2016) and flooded conditions (Zhang et al., 2016). However, yet little attention has been paid to quantifying soil quality/health changes due to residuals land application. One of the only studies to begin to quantify residuals land application effects on soil quality was published Jin et al. 2015 from a biosolids surface application in Texas. Soil organic C, water retention and available water-holding capacity increased (all positive soil quality changes), bulk density decreased (a positive soil quality attribute), yet decreases were observed in water-stable aggregates (a negative soil quality characteristic). Consideration of site-specific management decisions was recommended when utilizing residuals materials in land application programs similar to previous and current work from the W3170 workgroup as outlined below.
Improvements in soil nutrient quality are being evaluated. In 2017, Rosen et al. began a three-year field study to assess the effects of P-based sewage sludge byproducts on soil, microbial and plant analyses. Year 1 data reflect high grain yields with no significant response due to source or rate. Similarly, end-of-season stover (biomass and husk) did not show a significant response to source or rate. Chemical analyses of plant material (grain, stover, and husk) are still being performed. Ssoil analysis overall showed minimal differences between fertilizer types as most differences were due to application rates. Olsen-P and Bray-P concentrations increased with increasing application rate for all sources but there were no significant differences between fertilizer types. DTPA-extractable Zn and Cu, and exchangeable Na also increased significantly with application rate and had highly significant effects in biosolids and ash applications. Otherwise, neither source nor rate had significant impacts on other available or total elements. Plant yields and changes in soil quality will continue to be monitor in the next 2 field seasons.
Dr. Ian Pepper and his team (AZ) are evaluating a novel and potentially cost-effective approach using Class B biosolids to solve the problem of pecan tree Zn-deficiency. Similar to the positive biosolids-borne Zn findings of Ippolito et al. (2015) and Barbarick et al. (2016), it is hypothesized that biosolids will act as a slow release fertilizer supplying Zn and nitrogen to pecan trees. The solution of a one-time annual application of biosolids could eliminate all of the issues associated with foliar Zn applications and traditional inorganic soil fertilizers. An added benefit of the biosolids application is that all essential micronutrients will be more available to pecan trees. Moreover, the one-time annual application via subsurface soil injection eliminates the issue of any microbial pathogens associated with Class B biosolids and any pathogen issue is further negated by the fact that pecan harvesting occurs 7-8 months after the springtime annual application, during which any pathogens introduced into the soil would no longer be viable. In this study, an existing pecan orchard will be utilized such that real-world field data would be collected that would be applicable to all pecan orchards in Arizona. The experiment will consist of three treatments (4 replicates): i) Control plots: no amendment; ii) Plots amended with chelated Zn fertilizer; and iii) Plots amended with Class B biosolids. Leaf tissue composition will be used to determine the impacts of biosolids application on nutrient uptake. Nut quality will be determined by analysis of a subsample of ≃5 kg of harvested nuts to determine meat yield, % of good nuts and stick-tight nuts (fruit with shuck remaining stuck to the shell after harvest). It is hypothesized that quality will increase because biosolids applications will alleviate any micronutrient deficiency that may be unseen and unknown in orchards receiving inorganic fertilizers.
Evaluating alterations in soil biological quality has been or is being performed. Washington State researchers (Bertagnolli et al., 2016; Meinhardt et al., 2015) evaluated changes in the soil microbial community with a focus on ammonia oxidation for switchgrass fertilized with synthetic fertilizer or biosolids. These communities were compared with changes in cultivated row crops and native soils. The largest observed changes were from native soils to agricultural soils. Reduced diversity in both archaea and bacteria were seen as lands were cultivated. As part of the same study energy costs/ benefits of alternative fertilizers for switchgrass production were anlayzed. Here co-planting alfalfa and switchgrass and use of biosolids were compared with traditional fertilizers. Yield and chemical characteristics of the harvested biomass were used to estimate total ethanol potential. Nitrous oxide measures in combination with calculated energy costs of fertilizer production were used to determine offsets/ costs of fertilizer alternatives. The alfalfa switchgrass mixtures had lower yield as well as lower ethanol potential than conventionally fertilized switchgrass. Reduced N2O emissions and fertilizer offsets were not sufficient to compensate for reduced TEP. Municipal biosolids did not impact quality or yield of the switchgrass. Measured N2O emissions, while higher than synthetic fertilizers, were significantly lower than default emissions and lower than fertilizer emissions when considered on a total N applied basis. Use of biosolids provided an approximately 10% reduction in the greenhouse gas intensity of the switchgrass ethanol.
An Alfalfa (cv. 6829R) trial irrigated with either canal water or treated municipal wastewater was initiated recently in New Mexico (Lauriault et al., 2018). There was no difference due to irrigation source in the number of plants/m2; however, dry weight/m2 and dry matter percentage were both higher for alfalfa irrigated for establishment using canal water compared to treated wastewater. Greater dry weight may have been due to more rapid germination allowing for more mature plants at the time of sampling. Preplant soil analysis revealed no apparent issues in regard to fertility (including toxicities) or potential salt problems. Total microbial biomass and diversity index in the soil were not different between water sources after establishment; however, there was a difference in the proportion of total microbial biomass being arbuscular mycorrhizae. The lack of arbuscular mycorrhizae in the wastewater area may indicate the presence of compounds of concern. Lack of precipitation or irrigation from early October until sampling time also may have been a factor.
Improvements in soil physical and chemical quality of reclaimed mine land using residuals has been a focus of the W3170 workgroup. Daniels et al. (2016), Clark et al. (2017), Orndorff et al. (2015) and Yarwood et al. (2015) studied the long-term effects (30+ years) of biosolids application (0, 56, 112 and 224 Mg ha-1) on reclaimed Appalachian hard rock coal mine spoils via observational changes in morphology and chemical/physical properties. Field morphological results indicate that application of the higher biosolids rates (112 and 224 Mg ha-1) 24 years later were visually noticeable with surface horizons having darker and thicker and improved aggregate characteristics. Greater total C, N and P levels in the 112 and 224 Mg biosolids ha-1 also existed compared to the inorganically fertilized and 56 Mg biosolids ha-1 treatments.
Brown and Chaney (2016) and Wijesekara et al. (2016) tested the resilience of a long-term mine waste impacted site amended with biosolids to maintain a plant cover and reduce hazards associated with the contaminants in situ. A range of measures, including animal trapping and kidney and liver pathology, were used to confirm the safety of this approach. In addition to confirming the efficacy of the use of amendments for restoration, the researchers compared the relative ecosystem costs of topsoil harvesting and replacement with biosolids use for restoring the large acreage site. They estimated the costs of topsoil harvesting by using the rate of soil formation in combination with the current payback rate for the USDA Conservation Reserve Program to estimate costs of topsoil replacement. The greenhouse gas benefits of remediation with biosolids were used for comparison. The results clearly showed the benefits of residuals use, both as a result of effectiveness, for ecosystem services, and for improvements in soil quality.
The use of pyrolysis residuals (e.g., biochars) for improving soil quality has been a recent focus of the W3170 workgroup, with significant efforts currently being made in Hawaii for improvements in highly weathered soils by Hue and co-workers (Berek and Hue, 2016; Berek et al., 2018). They have been assessing the effect of biochars in combination with composts on nutrient retention capacities, changes in soil properties and plant health using a set of model test plants in Hawaii soils. Interaction between biochar and compost additions significantly increased pH, EC, P and K of both soils; improved Ca, Mg and Fe uptake; and increased shoot and total cabbage fresh and dry matter weights. Exchangeable Al in the Ultisol decreased to virtually zero and Mehlich-3 extractable Mn in the Oxisol decreased by > 2 times. Chinese cabbage growth in the Ultisol amended with the lac tree (Schleichera oleosa) wood biochar at 2% plus 2% vermicompost was almost two times greater than with lime + vermicompost at the same rate. Other plants (forage legume, corn, okra and soybean and soybean) showed similar patterns in greenhouse and/or field studies. At an application rate of approximately 25 tons ha-1, a kiawe-wood biochar reduced Al toxicity and increase the legume growth as much as CaCO3 applied at 3 1.5 tons ha-1. At a same total N rate of 200 mg kg-1, cabbage yield was much higher in the presence of a wood-based biochar (at 2%) than when urea or organic N fertilizer and yield increased became even more pronounced in the second harvest. This increased N use efficiency could be attributed to biochar properties, such as large surface area and numerous tiny pores. Under field conditions, corn yield (first season) was nearly doubled in the presence of 2% biochar (derived from macadamia shells) when N was applied as urea or blood meal (10% total N) at 150 or 300 kg N ha-1 rate. Interestingly, the effect of biochar on plant growth seemed to extend beyond N nutrition because the treatments receiving biochar but no N input also out-yielded those having N input but no biochar.
Brewer et al. (2016) evaluated solid waste processing using pyrolysis to recover water and nutrients for water, food, and energy sustainability. Biochars were created from agricultural residues, halophyte biomass, invasive species biomass and solid wastes on spacecraft. Researchers are in the process of testing their impacts on soil quality, their potential for soil-less growth media and their ability to be used as adsorbents for trace organic contaminants in water.
Residuals land application and the use of soil quality assessment tools is currently in its infancy. Ippolito et al. is investigating the use of the Soil Management Assessment Framework (SMAF), which is an excel-based tool to determine soil quality changes. They are using the SMAF to ascertain differences in soil quality at a site that received 0, 2.2, 4.4, 6.7, 9 or 11.2 Mg biosolids ha-1 or inorganic N ha-1 every other year over a 22-year period. Following wheat harvest after the 22nd year, soils from the 0-20 cm depth (e.g., zone of biosolids and N fertilizer incorporation) were evaluated for % clay, % OC, wet aggregate stability, microbial biomass C, potentially mineralizable N, pH, EC, Bd, Olsen-extractable P and K and beta-glucosidase activity and entered in the SMAF. Increasing inorganic N fertilizer rates only improved the nutrient soil quality index whereas increasing biosolids rates improved soil chemical, biological, and overall soil quality indices.
Objectives
-
Objective #1
Comments: Evaluate the short- and long-term fate, bioavailability and persistence of trace organic contaminants (TOrCs) with an emphasis on per- and polyfluoroalkyl substances (PFAS) and pathogens in residuals, reclaimed water, and amended soils to aid in assessing and minimizing environmental and human health risks from their application at a watershed scale. Specific tasks: i) Quantify and evaluate the uptake, accumulation and transport of TOrCs in residuals, wastewaters and residuals- and wastewater-treated soils (e.g., agricultural, urban and brownfields); ii) Predict the long-term bioavailability, persistence and toxicity of TOrCs in residuals- and wastewater-amended soils; iii) Evaluate ecological effects of TOrCs from soils amended with residuals and reclaimed wastewaters; and iv) Evaluate long-term effects of residuals and wastewater application on the emergence/spread of antibiotic resistance. -
Objective #2
Comments: Evaluate and optimize the uses and associated environmental benefits of residuals and wastewaters applied to various ecosystems (e.g., agricultural, urban, recreational, forest, rangeland, mine-impacted, other anthropogenic) on soil physical, chemical, and biological properties and plant nutrition, health, and yield. Specific tasks: i) Quantify the effects of biosolids and other municipal, industrial, and agricultural residuals on indicators of soil health; ii) Quantify the effects of biosolids and other residuals on pollutant (TOrCs and metals/metalloids) availability, assimilation, phytotoxicity, and remediation; and iii) Develop and evaluate treatment strategies of residuals and wastewaters to reduce contaminant or pathogen loads.
Methods
Objective 1. Evaluate the short- and long-term fate, bioavailability and persistence of trace organic contaminants (TOrCs) with an emphasis on per- and polyfluoroalkyl substances (PFAS) and pathogens in residuals, reclaimed water, and amended soils to aid in assessing and minimizing environmental and human health risks from their application at a watershed scale.
Specific tasks: i) Quantify and evaluate the uptake, accumulation and transport of TOrCs in residuals, wastewaters and residuals- and wastewater-treated soils (e.g., agricultural, urban and brownfields); ii) Predict the long-term bioavailability, persistence and toxicity of TOrCs in residuals- and wastewater-amended soils; iii) Evaluate ecological effects of TOrCs from soils amended with residuals and reclaimed wastewaters; and iv) Evaluate long-term effects of residuals and wastewater application on the emergence/spread of antibiotic resistance.
We are interested in a science-based understanding of fate and environmental and human impact of TOrCs, persistent organic contaminants, and pathogens in land-applied residuals and reclaimed waters. Contaminant reaction products will be identified, occurrence of antibiotic resistance will be evaluated, and contaminant bioavailability to plants, animal (including humans), and other ecological receptors will be measured. This will be accomplished via US EPA’s fate, transport and transformation test standard guidelines (US EPA, 1998; US EPA, 2008a, 2008b) in conjunction with methods previously established by W3170 workgroup members (Bhalsod et al., 2018; Li et al., 2018). Studies will be conducted in laboratory, greenhouse and field settings.
Subgroups of researchers from the Arizona (Pepper, McLain; UAZ), Colorado (Borch, CSU), Florida (Judy, UFL), Illinois (Brose & Kumar, MWRD of Chicago), Kentucky (D’Angelo, UKY), Michigan (Li, MSU), Pennsylvania (Watson, PSU), Indiana (Lee, PU), Texas (Gentry, TA&M), Virginia (Xia, VT) and Northeast-NE (Beecher, NEBRA) will collaborate to achieve the research addressed in the tasks of Objective 1. Specific subgroups are identified in the descriptions of specific research covered under Objective 1.
Tasks i-iii: i) Quantify and evaluate the uptake, accumulation and transport of TOrCs in residuals, wastewaters and residuals- and wastewater-treated soils (e.g., agricultural, urban and brownfields); ii) Predict the long-term bioavailability, persistence and toxicity of TOrCs in residuals- and wastewater-amended soils; iii) Evaluate ecological effects of TOrCs from soils amended with residuals and reclaimed wastewaters. (FL, IL, IN, MI, NE, VA)
Most published studies on the environmental fate and transport of trace organic compounds (TOrCs), except for those of W3170 members, have been conducted using experimental design of laboratory tests in soil-only systems in accordance with standard guidelines that have been established for pesticides. It has become evident that those standard guidelines that are derived from direct application of pesticides are not appropriate due since many TOrCs enter a soil system in residuals-partitioned states; residuals-partitioned TOrwill behave differently than a direct chemical application, and residuals can alter the physical, chemical and biological properties of soil, thus influences environmental fate of TOrCs. Therefore, we will continue to employ comprehensive laboratory and field investigations to gain further systematic and mechanistic understandings of fate and transport of residuals-borne TOrCs. The information generated will be utilized to better understand the environmental behavior of residuals-borne TOrCs at the macro- and field-scale in a wide range of residuals-amended soil systems. Ultimately, knowledge gained will help site managers an environmental engineers design better residuals land management systems to minimize environmental impact of residuals-borne TOrCs.
With the increasing awareness and concerns of PFAS in wastewater and treatment residuals, we will focus considerable attention on developing a better understanding of the occurrence and fate of PFAS in agricultural and urban landscapes and watersheds from application of wastewater and treatment residuals. We will conduct research at the laboratory, greenhouse and field scale to better ascertain the influence of land-applied residuals and wastewaters on PFAS inputs to water sources and accumulation in plants including under more realistic agricultural production settings. We will evaluate PFAS load in biosolids and quantify and correlate PFAS leaching potential from biosolids as a function of biosolids characteristics including iron, aluminum and organic matter (OM) content, production practices and PFAS properties. We will evaluate the uptake and accumulation of PFAS in vegetables and grain crops from the soils amended with biosolids. PFAS can be released from biosolids, transport with water flow from soils to vegetables/crops and accumulate in plants. Accumulation will be related to plant type and vary in different plant tissues and as a function of growth condition as well as soil water distribution and flow patterns in soil-water-plant systems. Soils will be amended with biosolids samples, and investigate plant uptake of selected PFASs from the soils. Lettuce or kale and radish or turnips will be used as model vegetables and corn will represent grain crops. Approaches will include (1) characterizing of the major PFAS present in the biosolids received, (2) investigating the uptake of representative PFAS in selected vegetables and grain crop under relevant environmental settings, and (3) evaluating plant constituents and soil water influencing the uptake and distribution of PFAS. The results obtained from these studies will provide more reliable information to evaluate the potential accumulation of PFAS in grain crop and vegetables produced in soils amended with biosolids. PFAS transformation kinetics, metabolites, plant uptake, bioconcentration factors, their potential to leaching and environmental factors affecting the fate and impact of PFAS will be characterized. This research will be done collaboratively between Michigan (Li, MSU), Indiana (Lee, PU), Florida (Judy, UF), Illinois (Brose, Kumar – MWRD).
Task iv) Evaluate long-term effects of residuals and wastewater application on the emergence/spread of antibiotic resistance (AZ, KY, VA, TX).
Pepper and McLain (UAZ) will evaluate characteristics of antibiotic resistant pathogens associated with biosolids, such as survival in soil relative to similar non-pathogenic pathogens to generate data to be used to generate risk assessment for antibiotic resistant bacteria. D'Angelo and coworkers at University of Kentucky will conduct laboratory experiment to investigate antibiotic resistance development in biosolid-amended soils, the effect of biosolids application to soils on nutrient enrichment, cyanobacterial dynamics, and cyanotoxin production in aquatic ecosystems, the fate of cyanotoxins in drinking water treatment systems, and the sorption, desorption, and diffusion behavior of cyanobacterial toxins in drinking water residuals. Research at Virginia Tech (Xia et al.) will investigate the fate of animal manure-borne antibiotic resistant elements and the best manure land management methods for minimizing their adverse environmental and human impact. Gentry (Texas A&M) will develop and use molecular technologies to detect and identify microbial pathogens from animal, human, and natural sources and characterize microbial populations and communities contributing to applied remediation processes, such as the bioremediation of organic contaminants.
Objective 2. Evaluate and optimize the uses and associated environmental benefits of residuals and wastewaters applied to various ecosystems (e.g., agricultural, urban, recreational, forest, rangeland, mine-impacted, other anthropogenic) on soil physical, chemical, and biological properties and plant nutrition, health, and yield. Specific tasks: i) Quantify the effects of biosolids and other municipal, industrial, and agricultural residuals on indicators of soil health; ii) Quantify the effects of biosolids and other residuals on pollutant (metals/metalloids) availability, assimilation, phytotoxicity and remediation; and iii) Develop and evaluate treatment strategies of residuals and wastewaters to reduce contaminant or pathogen loads.
Task i) Quantify the effects of biosolids and other municipal, industrial, and agricultural residuals on indicators of soil health (AZ, CO, FL, KS, MN, PA, VA, WA)
We will collect data on the effects of residuals on traditional and developing indicators of soil health. Land application of residuals has been demonstrated by our W3170 members to improve agricultural, urban, forested, rangeland, mine-impacted, and other anthropogenically-disturbed soils by improving physical, chemical, and biological properties and providing nutrient sources for improving plant productivity (Avery et al., 2018; Badzmierowski et al., 2019; Bamber et al., 2016; Barbarick et al., 2016; Barbarick et al., 2017; Ducey et al., 2013; Elzobair et al., 2016; Ippolito et al., 2012; Li and Evanylo, 2013;). However, optimum rates of various residuals necessary to beneficially alter soil properties without incurring unnecessary expense or creating an associated detrimental effect(s) are not well-established. Depending on whether the purpose of the residual is to improve soil physical (tilth, water holding capacity, aggregate stability, porosity, bulk density) or chemical (cation exchange capacity, pH, essential plant nutrient supply, C sequestration) properties will determine the ideal application rate. Potential detrimental effects (pH, soluble salts/electrical conductivity, phytotoxic micronutrients) of the amended soil on the vegetation to be grown will pose limitations that must be understood.
As new products are developed from residuals employing alternative treatment processes (esp., those that generate Exceptional Quality biosolids and sludges via thermal hydrolysis, thermophilic anaerobic digestion, advanced alkaline stabilization, composting, pyrolysis) and co-utilization and blending with other municipal, industrial, and agricultural by-products, the characteristics and potential beneficial uses of these must be understood. Some such blended residuals were found to have positive attributes and beneficial effects for rehabilitating urban (Alvarez-Campos et al., 2018) and mine land soils (Daniels et al, 2017; Koropchak et al., 2015). Predicting nutrient release from these new products is essential for their beneficial use (Alvarez-Campos and Evanylo, 2019).
Recent scientific discoveries suggest biosolids may provide additional benefits under a changing climate. Specifically, they can increase crop drought tolerance and productivity beyond that explained by increases in essential plant nutrients and moisture availability alone. W3170 researchers (Zhang et al., 2012, 2014) previously demonstrated that biosolids-enhanced drought tolerance is associated with increased production of plant growth-promoting substances, particularly indole-3-acetic acid (IAA)1–3; however, the mechanisms that facilitate these benefits are not known. Discovering the biological mechanisms for how biosolids increase plant drought tolerance will further the use of such byproducts. Our central thesis is that biosolids induce drought tolerance through a complex set of interactions among soil chemistry, microorganisms and plant physiological responses. We propose a transdisciplinary approach to elucidate these interactions. Transcriptomics—the sequencing of RNA being actively produced by an organism—is a powerful new approach to analyze gene expression and identify physiological mechanisms a plant is using to mitigate stress. Meanwhile, metagenomics—the sequencing of the combined DNA pool of whole microbial communities—provides a similar approach for studying soil microbial diversity and metabolic capacity. By coupling transcriptomics and metagenomics with analyses of soil chemistry and plant growth stimulants, we aim to understand how biosolids induce drought tolerance across the entire plant-microbe-soil system. We will achieve these objectives by: 1) determining the genetic basis for changes in physiological drought stress responses in growing maize plants following biosolids application, 2) identifying key changes that occur in soil microbial communities near drought stressed plants following biosolids application, and 3) quantifying changes in plant metabolites and soil fertility to more completely link mechanisms through the entire plant-soil-microbial system. Badgley and Evanylo at Virginia Tech will assess the utility of biological indicators of soil health to land use or management changes, the impact of soil amendment with residuals on soil microbial abundance, activity, and biogeochemistry, particularly related to greenhouse gas emissions, and the application of residuals that may boost plant growth promoting bacteria. Pepper et al (University of Arizona) will develop and employ a new indicator of soil health, the AMP Stress Index plus transcriptonomics, to evaluate in field studies the effects of labile organic matter (biosolids) in mitigating soil moisture stress.
The W4170 workgroup proposes to: 1) continue collecting soil physical, chemical, biological, and nutrient characteristics under current and new residuals and wastewater scenarios; and 2) investigate the ability of residuals and wastewaters to alter soil quality. Laboratory, greenhouse, and field research designed to assess the ability of residuals and wastewaters to alter various soil characteristics and ultimately soil quality, will be conducted. Soil bulk density, water stable aggregates, plant available water, pH, EC, inorganic trace elements, OM, microbial biomass, potentially mineralizable N and beta-glucosidase activity will be compared among residuals- and fertilizer-amended soils. These soil attributes and others as they come on-line (e.g., microbial community structure profiling techniques) will be analyzed separately and collectively in order to create a framework for understanding how residuals applications to soils affect various aspects of soil quality, and ultimately how connectivity between soil quality alterations are linked to human health.
Collaborative research among W4170 members at Colorado State University (Ippolito), Washington State University (Bary), Virginia Tech (Daniels), Kansas State University (Hettiarachchi) and Ohio State University (Basta) will determine long-term benefits of biosolids land application to agricultural and disturbed soils, esp., with respect to soil health and function (including C sequestration and greenhouse gas emissions).
Task ii) Quantify the effects of biosolids and other residuals on pollutant (TrOCs and metals/metaloids) availability, assimilation, phytotoxicity, and remediation (FL, IN, KS, OH, WA)
At Fort Riley, Kansas, Hettiarachchi et al. will determine the feasibility of growing miscanthus in biosolids-amended contaminated military sites. These sites will be monitored to determine the effect of soil amendments on miscanthus growth, soil-plant transfer of Pb and other contaminants, bioaccessibility of soil Pb and other contaminants, and soil health. In Washington, Brown et al. will use develop soil blends from Class A biosolids with other materials including foundry sand, woody debris, biochar, water treatment residuals to create end products suitable for broad use in urban areas including urban agriculture and green stormwater infrastructure, ranging in scale from rain gardens to larger scale bioretention systems. The impact of biosolids blends on urban soil contaminants including Pb, As, and TOrCs and on urban soil physical characteristics, and growth response and nutritional value of crops grown in urban gardens will be investigated in this work. Lee from IN and Judy from FL will collaborate with Brown (WA) and Hettiarachchi et al. (KS) to specifically evaluate PFAS presence and impact associated with their field studies.
Further research is needed to develop and evaluate advanced spectroscopic methods to predict the long-term bioavailability and toxicity of trace elements in residuals and residual-amended soils. Our ability to measure the form of a contaminant in soil may provide a more accurate assessment of the long-term fate and bioavailability of contaminants in amended soils. The research gaps on trace element bioavailability are (i) development and application of methods to evaluate the soil ingestion (i.e. human and ecological) exposure pathway, (ii) development and evaluation of methods used to evaluate trace element bioavailability to ecological receptors and risk-based ecological endpoints, and (iii) development and application of advanced solid phase spectroscopic methods.
In-situ (on site) soil remediation methods, where inexpensive materials (e.g., municipal, industrial residual byproducts) are added to contaminated soil to reduce the mobility and bioavailability of heavy metal and toxic organic chemical contaminants, offer a cost-effective alternative to traditional dig and haul approaches. Incidental ingestion is an important exposure pathway for assessing human health exposure and risk associated with trace-element contaminated soils. The bioavailability of Pb, As and other soil contaminants can be determined using in vitro gastrointestinal (IVG) methods that have been developed that simulate human gastrointestinal conditions in liu of expensive animal models. However, there is currently no information on IVG methods for evaluatinge soil ingestion in a HERA framework for residuals (Juhasz and Basta, 2013). In order to define cleanup and remediation standards for contaminated sites, site-specific data. Relative bioavailability (RBA), a type of IVG, is a measure of the amount of the contaminant that is absorbed by a receptor as a result of contaminated soil exposure (i.e. incidental ingestion of soil). It is dependent on mineralogy, soil properties and the residence time of the contaminant in the soil (Ruby et al. 1999). RBA is often less than the assumed 100%. This may have a significant influence on human exposure and risk assessment. Adjustments to RBA may be achieved by conducting in vivo bioavailability or in vitro bioaccessibility studies. Over the past 2 decades, a limited number of studies have established the relationship between in vivo RBA and in vitro bioaccessibility for As, Cd, and Pb (Basta et al. 2007; Bradham et al., 2011; Denys et al., 2012; Hettiarachchi et al., 2003; Juhasz et al. 2007b; Juhasz et al. 2009; 2010, 2011 Juhasz et al. 2011; Rodriguez et al. 1999; Ryan et al., 2004; Smith et al., 2011). Currently, the Relative Bioaccessibility Leaching Procedure (RBALP) developed by Drexler and Brattin (2007) is accepted for use in human health risk assessment (USEPA, 2007b). However, the viability for this test for sites outside of the CERCLA or NPL programs has not been demonstrated. Basta (2013) reported that RBALP did not predict reductions in bioavailable Pb for soil treatments previously shown to reduce bioavailable Pb (Scheckel et al., 2009). This is a serious obstacle because in situ remediation methods cannot be evaluated without an accurate in vitro method to access their ability to reduce Pb bioavailability and human exposure. We will develop and evaluate in vitro (including chemical speciation) and novel in vivo methods to correlate human and ecological health responses with risk-based bioavailability of trace elements in residuals and residual-treated soils.
Task iii) Develop and evaluate treatment strategies of residuals and wastewaters to reduce contaminant or pathogen loads. (FL, IN, IL)
W3170 members have already been developing and testing treatment strategies associated with nutrients, metalloids and pathogens, which will continue. The aspect of treatment will be focused on PFAS. Opportunities exist to decrease PFAS concentrations in both WWTP effluent and sludge from which biosolid-based fertilizers originate, by treating influent inputs of smaller volume but higher PFAS loads such as the dewatering solution/filtrate (centrate) resulting from the dewatering of sludge that is recycled back into the WWTP and landfill leachate. To address removal of PFAS from WWTP centrate, we will determine the technical and economic feasibility using a treatment train approach consisting of nanofiltration followed by electrochemical oxidation of the PFAS present in the nanofiltration retentate solutions. We will evaluate PFAS load in biosolids (Objective 1) and potential treatment options her in Objective 2. We will determine the reduction in PFAS leaching from biosolids after amending biosolids with sorbents at different rates, including drinking water treatment residuals (WTRs) and biochars. We will also evaluate if low temperature O2-free pyrolysis may reduce PFAS lability in laboratory and greenhouse studies. We will use a treatment train consisting of ultrafiltration (UF) followed by nanofiltration (NF) and subsequent electrochemical oxidation using microporous and UF REMs to reduce PFAS concentrations in centrate and targeted landfill leachates. We will quantify PFAS release from amended and unamended biosolids as a function of specific PFAS loads and characteristics, aluminum, iron, OM, and water-soluble carbon concentrations. This research will be done collaboratively between Indiana (Lee, PU), Florida (Judy, UF), Illinois (Brose, Kumar – MWRD).
For pyrolysis treatment of biosolids, we propose the use of low temperature (350 °C – 600 °C) low to no O2 pyrolysis as a technology to reduce PFAS release associated with biosolids by entrapment and sorption but retain the value of the biosolids. We will evaluate if biochar produced from biosolids under low temperature pyrolysis of biosolids can lead to PFAS entrapment thereby reducing PFAS leachability and bioavailabilty and if biosolid-derived biochar can serve as a sorptive sink for PFAS released from untreated biosolids, thus allowing the mix to maintain key fertilizer properties while minimizing PFAS mobility and plant uptake. These hypotheses will be tested using both laboratory- and greenhouse-based studies. Our ultimate goal is to target the biosolids with high PFAS loads for pyrolysis andshow that a mix of this biochar with untreated biosolids could be safe for field-application. This would prevent biosolids from ending up in landfills and incineration and allow them to continue to be used as a rich nutrient source to improve soil and crop health. This research will be done collaboratively between Indiana (Lee, PU), Florida (Judy, UF), Illinois (Brose, Kumar – MWRD and Zheng, UI).
Overall, the subgroups of researchers from Arizona (Pepper), Colorado (Ippolito, CSU), Florida (Silveira & Judy, UF), Indiana (Lee, PU), Illinois (Brose, MWRD of Chicago; Chaplin, UI-Chicago, and Zheng-UI), Kansas (Hettiarachchi, KSU), Minnesota (Rosen, UMN), Ohio (Basta, OSU), Pennsylvania (Elliott, PSU), and Virginia (Badgley, Daniels, Evanylo; VT) will collaborative to achieve the various tasks under Objective 2.
Measurement of Progress and Results
Outputs
- We will improve our understanding of the fate and transport of TOrCs (with an emphasis on PFAS) and pathogens in residuals, reclaimed water, and amended soils to assess the environmental and human health risk-based effects of their application.n.
- We will evaluate the capability of residuals and wastewaters to improve soil physical, chemical, and biological, including the development of testing protocols and comparison of management practices that provide accurate nitrogen and phosphorus application rates, ameliorate heavy metal and arsenic contaminated soils, reduce greenhouse gas emissions, increase carbon sequestration, identify beneficial microbial communities, and promote plant drought tolerance.
- We will develop strategies to minimize the presence and/or mobility and bioavailability of PFAS in biosolids- and waste-based fertilizer products.
Outcomes or Projected Impacts
- Improved mechanistic understanding of transformation of contaminants in residuals and reclaimed waters and in amended soils; their plant and human bioavailability; and human health risk and exposure associated with contaminants.
- Sound decisions for use of residuals and wastewaters in a variety of ecosystems to improve soil health, air and water quality, and vegetative growth and quality. The research results will enable scientists to provide policy makers with information for developing residuals’ use practices across the US.
Milestones
(2023):Data generation from field and lab studies.(2024):Compilation of information on bioavailability, chemistry, transport, and toxicology of residuals- and wastewater-borne TOrCs and their effects on soil quality.
(2024):Compilation of information on the effects of biosolids and other residuals on pollutant availability, assimilation, phytotoxicity, and remediation.
(2024):Compilation of information on treatment and reduction of PFAS mobility and bioavailability in biosolid- and waste-based products.
Projected Participation
View Appendix E: ParticipationOutreach Plan
The Wx170 group traditionally includes members from industry such as Metropolitan Water Reclamation District of Greater Chicago (MWRDGC) and the CA Assoc. of Sanitation Agencies (CASA) as well as government (EPA). The group maintains close relationships with other water and wastewater utilities related associations, e.g., Water Environment Federation (WEF); Northwest Biosolids Management Association; North East Biosolids and Residuals Association (NEBRA); Mid Atlantic Biosolids Association; National Association of Clean Water Agencies. Through direct participation in conferences and workshops as well as webinar opportunities (e.g., those hosted by EPA, NEBRA, WEF), research findings will be reported to industry cooperators. The Wx170 group will continue to publish collaborative research reviews that are available to industry and academic partners, publish findings in scientific journals and present at national and international meetings. We will continue to participate in training sessions organized by local wastewater treatment agencies to provide their members with relevant information from our workgroup. Regional/national level outreach activities will be organized during our annual meetings as well as national/international conferences (e.g., ASA, SSSA, ACS, SETAC).
Our members who hold extension appointments (representing CO, FL, IN, VA) with help from the other members will develop and provide educational programming including extension publications beneficial to educators, regulators, local government decision makers, the concerned public, other scientists, and stakeholders from entities that generate potentially recyclable wastes. We will continue to convey the science on the risks and benefits of constituents in land-applied residuals and reclaimed waters through written publications, field demonstrations, workshops, webinars, and direct interaction with stakeholders and clientele.
Organization/Governance
The W3170 technical committee will consist of project leaders for the contributing states, the administrative advisor, and NIFA representatives. Voting membership includes all persons with contributing projects; however, only one vote is permitted for each research location.
Co-chairpersons, one from the western and one from the other regions, will be elected at the first authorized committee meeting after the renewal project has been accepted. The co-chairpersons will serve multiple years, if so desired by project participants, and will be responsible for meeting arrangements, the annual reports, coordination of research activities, compilation of regional research data, and preparation of the renewal proposal. One representative each from the western and the other regions and a secretary will be elected annually. Office terms for annually elected positions begin immediately after completion of the annual committee meeting. An Executive Committee, consisting of the co-chairs, the secretary, and the two regional representatives, will serve as a guidance body in matters such as new project participant additions and meeting agenda.
In addition to the official project representatives, other researchers with activities that contribute to the project have been and will continue to be invited to participate on a regular basis.
Literature Cited
Alvarez-Campos, O., M. Badzmierowski, G.K. Evanylo, K. Bamber, H-C Yu. 2018. Development and testing of exceptional quality biosolids-based by-products for urban landscapes. Compost Science & Utilization 26(4): 234-245.
Alvarez-Campos, O., and G.K. Evanylo. 2019a. Plant available nitrogen estimation tools for a biosolids-amended, clayey urban soil. Soil Sci. Soc. Am. J. (Accepted Feb 22, 2019)
Alvarez-Campos, O., and G.K. Evanylo. 2019b. Biosolids improves urban soil properties and vegetable production in urban agriculture. Urban Agriculture & Regional Food Systems. (Accepted with minor revisions, May 17, 2019)
Alvarez-Campos, O., M. Badzmierowski, and G.K. Evanylo. 2018. Development and assessment of exceptional quality biosolids products for urban gardens. Compost Science & Utilization 26(4): 234-245.
Badzmierowski, M., G.K. Evanylo, E.H. Ervin, A. Boyd, and C. Brewster. 2019. Biosolids-based amendments improve tall fescue establishment and urban soils. Crop Science 59:1273-1284.
Abiven, S., S. Menasseri, C. Chenu. 2009. The effects of organic inputs over time on soil aggregate stability—a literature analysis. Soil Biol. Biochem. 41:1–12.
Attanayake, C.P., G.M. Hettiarachchi, Q. Ma, G.M. Pierzynski, M.D. Ransom. 2017. Lead speciation and in vitro bioaccessibility of compost-amended urban garden soils. J. Environ. Qual. 46:1215-1224.
Attanayake, C.P., G.M. Hettiarachchi, S. Martin, G.M. Pierzynski. 2015. Potential bioavailability of lead, arsenic, and polycyclic aromatic hydrocarbons in compost-amended urban soils. J. Environ. Qual. 44:930-944.
Attanayake, C.P., G.M. Hettiarachchi, A. Harms, D. Presley, S. Martin, and G.M. Pierzynski. 2014. Field Evaluations on Soil Plant Transfer of Lead from an Urban Garden Soil. J. Environ. Qual. Vol. 43, 475-487.
Avery, E., M. Krzic, B. Wallace, R.F. Newman, S.M. Smukler, G.E Bradfield. 2018. One-time application of biosolids to ungrazed semiarid rangelands: 14 yr soil responses. Canadian J. Soil Sci. 98:696-708.
Badzmierowski, M., G.K. Evanylo, E.H. Ervin, A. Boyd, and C. Brewster. 2019. Biosolids-based amendments improve tall fescue establishment and urban soils. Crop Science 59:1273-1284.
Bamber, K. W., G. K. Evanylo, W.E. Thomason. 2016. Importance of Soil Properties on Recommended Biosolids Management for Winter Wheat. Soil Sci. Soc. of Am. J. 80:919-929.
Bamber, K.W., G.K. Evanylo, W.E. Thomason. 2018. Rapid estimation of potentially mineralizable N in early spring following fall biosolids applications to winter wheat. Commun. Soil Sci. Plant Anal. 49: 567-575.
Barbarick, K.A., J.A. Ippolito, J.P. McDaniel. 2015. Uptake Coefficients for Biosolids-Amended Dryland Winter Wheat. J. Environ. Qual. 44:286-292.
Barbarick, K.A., J.A. Ippolito, J. McDaniel. 2016. Path Analysis of grain P, Zn, Cu, Fe, and Ni in a biosolids-amended dryland wheat agroecosystem. J. Environ. Qual. 45:1400-1404.
Barbarick, K.A., J.A. Ippolito, J. McDaniel. 2017. Meta-analysis of biosolids effect in dryland wheat agroecosystems. J. Environ. Qual. 46:452-460.
Basta, N.T., A. Juhasz. 2014. Chapter 9: Using In Vivo Bioavailability and/or In Vitro Gastrointestinal Bioaccessibility Testing to Adjust Human Exposure from Soil Ingestion. In : R.J.Bowell, J. Majzlan and C.Alpers (eds.) Geochemistry, Mineralogy and Microbiology of Arsenic in Environment, Reviews in Mineralogy and Geochemistry, Mineralogical Society of America.
Basta, N.T., D.M. Busalacchi, L.S. Hundal, K. Kumar, R.P. Dick, R.P. Lanno, J. Carlson, A.E. Cox, T.C. Granato. 2015. Restoring ecosystem function in degraded urban soil using biosolids, biosolids blend and compost. J. Environ. Qual. Special Issue: Soil in the City. 45:74-83.
Basta, N.T., D.M. Busalacchi, L.S. Hundal, K. Kumar, R.P. Dick, R.P. Lanno, J. Carlson, A.E. Cox, T.C. Granato. 2016. Restoring ecosystem function in degraded urban soil using biosolids, biosolids blend and compost. Special Issue: Soil in the City. J. Environ. Qual. 45:74-83.
Basta, N.T., R. Gradwohl, K.L. Snethen, J.L. Schroder. 2001. Chemical immobilization of lead, zinc, and cadmium in smelter-contaminated soils using biosolids and rock phosphate. J. Environ. Qual. 30:1222-1230.
Beniston, J., R. Lal. 2012. Improving Soil Quality for Urban Agriculture in North Central U.S. In R. Lal, and B. Augustin (eds), Carbon Sequestration in Urban Ecosystems. Springer Dordrecht Heidelberg London New York, p. 173-196.
Bhalsod, G.D., Y.H. Chuang, S. Jeon, W.J. Gui, H. Li, E.T. Ryser, A.K. Guber, W. Zhang. 2018. Uptake and Accumulation of Pharmaceuticals in Overhead- and Surface-Irrigated Greenhouse Lettuce. J. Agric. Food Chem. 66:822-830.
Biegel, L.B., M.E. Hurtt, S.R. Frame, J.C. O'Connor, J.C. Cook. 2001. Mechanisms of extrahepatic tumor induction by peroxisome proliferators in male CD rats. Toxicol. Sci.60:44-55.
Berek, A., N. Hue. 2016. Characterization of biochars and their use as an amendment to acid soils. Soil Sci. 181:412-426.
Bertagnoli, A.D., K.A. Meinhardt, M. Pannu, S. Brown, S. Strand, S.C. Fransen, D.A. Stahl. 2016. Influence of edaphic and management factors on the diversity and abundance of ammonia-oxidizing thaumarchaeota and bacteria in soils of bioenergy crop cultivars. Environ. Microbiology Reports. 7:2:312-320.
Bizkarguenaga, E., I. Zabaleta, L. Mijangos, A. Iparraguirre, L.A. Fernandez, A. Prieto, O. Zuloaga. 2016. Uptake of perfluorooctanoic acid, perfluorooctane sulfonate and perfluorooctane sulfonamide by carrot and lettuce from compost amended soil. Sci. Total Environ. 571:444-451.
Blaine, A. C.; Rich, C. D.; Hundal, L. S.; Lau, C.; Mills, M. A.; Harris, K. M.; Higgins, C. P. 2013. Uptake of perfluoroalkyl acids into edible crops via land applied biosolids: Field and greenhouse studies. Environmental science & technology, 47(24):14062-14069.
Blaine, A.C., C.D. Rich, E.M. Sedlacko, L.S. Hundal, K. Kumar, C. Lau, M.A. Mills, K.M. Harris, C.P. Higgins. 2014a. Perfluoroalkyl Acid Distribution in Various Plant Compartments of Edible Crops Grown in Biosolids-Amended soils. Environ. Sci. Technol. 48:7858-7865.
Blaine, A.C., C.D. Rich, E.M. Sedlacko, K.C. Hyland, C. Stushnoff, E.R.V. Dickenso, C.P. Higgins 2014b. Perfluoroalkyl Acid Uptake in Lettuce (Lactuca sativa) and Strawberry (Fragaria ananassa) Irrigated with Reclaimed Water. Environ. Sci. Technol. 48:14361-14368.
Brewer, C.E., E.T. Hall, K. Schmidt-Rohr, D.A. Laird, R.C. Brown, K. Zygourakis. 2016. Temperature and reaction atmosphere effects on properties of corn stover biochar, Environmental Progress & Sustainable Energy, 36:696-707.
Bhalsod, G.D., Y.H. Chuang, S. Jeon, W.J. Gui, H. Li, E.T. Ryser, A.K. Guber, W. Zhang. 2018. Uptake and Accumulation of Pharmaceuticals in Overhead- and Surface-Irrigated Greenhouse Lettuce. J. Agric. Food Chem. 66:822-830.
Brown, S. 2016. Greenhouse gas accounting for landfill diversion of food scraps and yard waste. Compost Sci. 24:11-19.
Brown, S.L. 2017. Making Soils from Urban Wastes. In Advances in Soil Science: Urban Soils R. Lal and B.A. Stewart (Ed) Taylor and Francis Publishers.
Brown, S.L., R.L. Chaney. 2016. Use of amendments to restore ecosystem function to metal mining impacted sites: tools to evaluate efficacy. Current Pollution Reports. 2:91-102
Brown, S. L., R.L. Chaney, J. Hallfrisch, J.A. Ryan, W.R. Berti. 2004. In situ soil treatments to reduce the phyto- and bioavailability of lead, zinc, and cadmium. J. Environ. Qual. 33:522-531.
Brown, S. L., R.L. Chaney, G.M. Hettiarachchi. 2016a. Lead in urban soils: a real or perceived concern for urban agriculture? J. Environ. Qual. 45:26-36.
Brown, S.L., A. Corfman, K. Mendrey, K. Kurtz, D. Grothkopp. 2016b. Stormwater Bioretention systems- testing the phosphorus saturation index and compost feedstocks as predictive tools for system performance. J. Environ. Qual. 45:98-106.
Brown, S., K. Kurtz, A. Bary, C. Cogger. 2011. Quantifying benefits associated with land application of organic residuals in Washington State. Environ. Sci. Technol. 45:7451-7458.
Brown, S.L., K. McIvor, E. Snyder (Eds). 2016c. Sowing seeds in the city: Ecological and Municipal Considerations. Springer Publishers.
Brown, S.L., K. McIvor, E. Snyder (Eds). 2016d. Sowing seeds in the city: Human Dimensions. Springer publishers.
Brown, S.L., A. Trlica, J. Lavery, M. Teshima. 2017. Carbon sequestration potential on mined lands. In Mine Site Rehabilitation and Restoration. N. Bolan, M.B. Kirkham and Y. Ok (eds.) Taylor and Francis Publishers.
Calafat, A.M., L-Y. Wong, Z. Kuklenyik, J.A. Reidy, L.L. Needham. 2007. Polyfluoroalkyl chemicals in the US population: Data from the National Health and Nutrition Examination Survey (NHANES) 2003-2004 and comparisons with NHANES 1999-2000. Environ. Health Perspectives. 115:1596-1602.
Carlson, J., J. Saxena, N. Basta, L. HUndal, D. Busalacchi. 2015. Application of organic amendments to restore degraded soil: effects on microbial properties. Environ. Monit. Assess 187(3):1-15.
Chao Q., D. Troya, C. Shang, S. Hildreth, R. Helm, K. Xia. 2015. Surface Catalyzed Oxidative Oligomerization of 17β-estradiol by Fe3+-Saturated Montmorillonite. Environ. Sci. Technol. 49:956–964.
Chen, C. Q., K. Xia. 2017. Fate of Land Applied Emerging Organic Contaminants in Waste Materials. Curr. Pollution Rep. 3:38-54.
Chen, C. Q., G. K. Guron, A. Pruden, M. Ponder, Pang. Du, K. Xia. 2018. Antibiotics and antibiotic resistance genes in bulk and rhizosphere soils subject to manure amendment and vegetable cultivation. J. Environ. Qual. 47:1318-1326.
Cherubin, M.R., D.L. Karlen, C.E.P. Cerri, A.L.C. Franco, C.A. Tormena, C.A. Davies, C.C. Cerri. 2016. Soil quality indexing strategies for evaluating sugarcane expansion in Brazil. Plos One. DOI: 10.1371/journal.pone.0150860.
Choi, Y., R. Kim Lazcano, P. Yousefi, H. Trim and L.S. Lee. 2019. Perfluoroalkyl Acid Characterization in U.S. Municipal Organic Solid Waste Composts, Environ. Sci. Technol. Letters. Accepted and published online ez-2019-00280t.
Clark, E.V., C. Zipper, W.L. Daniels, Z.W. Orndorff, M.J. Keefe. 2017. Modeling Patterns of Total Dissolved Solids Release from Central Appalachia, USA Mine Spoils. J. Environ. Qual. 46:55-63.
Codling, G., S. Hosseini, M.B. Corcoran, S. Bonina, T. Lin, A. Li, N.C. Sturchio, K.J. Rockne, K. Ji, H. Peng, J.P. Giesy. 2018. Current and historical concentrations of poly and perfluorinated compounds in sediments of the northern Great Lakes - Superior, Huron, and Michigan. Environ. Pollut. 236:373-381.
Cogger, C.G., A.I. Bary, E. A. Myhre, A. Fortuna. 2013. Biosolids applications to tall fescue have long-term influence on soil nitrogen, carbon and phosphorus. J. Environ Qual. 42:516-522.
Craul, P.J. 1985. A description of urban soils and their desired characteristics. J. Arboriculture 11:330-339.
Dalahmeh, S., S. Tirgani, A.J. Komakech, C.B. Niwagaba, L. Ahrens. 2018. Per- and polyfluoroalkyl substances (PFASs) in water, soil and plants in wetlands and agricultural areas in Kampala, Uganda. Sci. Tot. Environ. 631-632:660-667.
D’Angelo, E.M. 2017. Sorption-desorption equilibrium and diffusion of tetracycline in poultry litters and municipal biosolid soil amendments. Chemosphere. 188:494-501.
D’Angelo, E.M., D. Starnes. 2016. Desorption Kinetics of Ciprofloxacin in Municipal Biosolids Determined by Diffusion Gradient in Thin Films. Chemosphere. 164:215-224.
Daniels, W.L., C.E. Zipper, Z.W. Orndorff, J. Skousen, C.D. Barton, L.M. McDonald, M.A. Beck. 2016. Predicting total dissolved solids release from central Appalachian coal mine spoils. Env. Pollut. 2016:371-379.
Daniels, W. L., Z.W. Orndorff, C. Stilson, C. Zimmerman, and A. Haywood. 2017. Development of Effective Rehabilitation Protocols for Mineral Sands Mining in Virginia USA. In Mined Land Reclamation: From Start to Finish. Carlton, VIC, Australia: Australia Institute for Mining and Minerals. Retrieved from http://www.ausimm.com/
Defoe, P.P, G.M. Hettiarachchi, C. Benedict, S. Martin. 2014. Safety of Gardening on Lead- and Arsenic-Contaminated Urban Brownfields. J. Environ. Qual. 43:2041- 2078.
Diacono, M., F. Montemurro. 2010. Long-term effects of organic amendments on soil fertility. A review. Agron. Sustain. Develop. 30:401–422.
Ducey, T.F., J.A. Ippolito, K.B. Cantrell, J.M. Novak, R.D. Lentz. 2013. Addition of activated switchgrass biochar to an aridic subsoil increases microbial nitrogen cycling gene abundances. Applied Soil Ecol. 65:65-72.
Elzobair, K.A., M.E. Stromberger, J.A. Ippolito. 2016a. Stabilizing effect of biochar on soil extracellular enzymes after a denaturing stress. Chemosphere. 142:114-119.
Elzobair, K.A., M.E. Stromberger, J.A. Ippolito, R.D. Lentz. 2016b. Contrasting effects of biochar versus manure on soil microbial communities and enzyme activities in an Aridisol. Chemosphere. 142:145-152.
Felizeter, S., M.S. McLachlan, P. de Voogt. 2012. Uptake of Perfluorinated Alkyl Acids by Hydroponically Grown Lettuce (Lactuca sativa). Environ. Sci. Technol. 46:11735-11743.
Flores, A.M., M.K. Shukla, D. Daniel, A.L Ulery, B.J. Schutte, G.A. Picchioni, S. Fernald. 2016. Evapotranspiration changes with irrigation using saline groundwater and RO concentrate. Journal of Arid Environ. 43:1-7.
Frisbee, S.J., A. Shankar, S.S. Knox, K. Steenland, D.A. Savitz, T. Fletcher, A.M. Ducatman. 2010. Perfluorooctanoic Acid, Perfluorooctanesulfonate, and Serum Lipids in Children and Adolescents. Arch. Pediatrics Adoles. Medic. 164:860-869.
Galkaduwa, M.B., G.M. Hettiarachchi, G.J. Kluitenberg, S.L. Hutchinson, L. Erickson, L. Davis. 2017. Transport and transformation of selenium and other constituents of flue-gas desulfurization wastewater in water-saturated soil materials. J Environ Qual. 46:384-392.
Gall, H.E., N.B. Basu, M.L. Mashtare, P.S.C. Rao, and L.S. Lee. 2016. Assessing the impacts of anthropogenic and hydro-climatic drivers on estrogen legacies and trajectories. Advances in Water Resources, 87:19-28.
Gall, H.E., S.A. Sassman, B. Jenkinson, L.S. Lee, and C.T. Jafvert. 2013. Hormone loads exported by a tile-drained agroecosystem receiving animal manure wastes. Hydrological Processes, doi:10.1002/hyp.9664.
Gall, H.E., S.A. Sassman, L.S. Lee, and C.T. Jafvert. 2011. Hormone discharges from a Midwest tile-drained agroecosystem receiving animal wastes. Environmental Science & Technology. 45:8755-8764.
Garner, E., C. Q. Chen, K. Xia, J. Bowers, D. M. Engalthaler, J. McLain, M. A. Edwards, A. Pruden. 2018. Metagenomic characterization of antibiotic resistance genes in four full-scale reclaimed water distribution systems and corresponding potable systems. Environ. Sci. Technol. 2018, 52, 6113−6125.
Gebbink, W.A., C.E. Hebert, R.J. Letcher. 2009 Perfluorinated Carboxylates and Sulfonates and Precursor Compounds in Herring Gull Eggs from Colonies Spanning the Laurentian Great Lakes of North America. Environ. Sci. Technol. 43:7443-7449.
Giesy, J.P., K. Kannan. 2001. Global distribution of perfluorooctane sulfonate in wildlife. Environ. Sci. Technol. 35:1339-1342.
Gottschall, N.; Topp, E.; Edwards, M.; Russell, P.; Payne, M.; Kleywegt, S.; Curnoe, W.; Lapen, D. 2010. Polybrominated diphenyl ethers, perfluorinated alkylated substances, and metals in tile drainage and groundwater following applications of municipal biosolids to agricultural fields. Science of the total environment, 408, (4), 873-883.
Gottschall, N.; Topp, E.; Edwards, M.; Payne, M.; Kleywegt, S.; Lapen, D. 2017. Brominated flame retardants and perfluoroalkyl acids in groundwater, tile drainage, soil, and crop grain following a high application of municipal biosolids to a field Science of the total environment, 408, (4), 873-883.
Gregory, J.H., M.D. Dukes, P.H. Jones, G.L. Miller. 2006. Effect of urban soil compaction on infiltration rate. J. Soil and Water Conservation 61:117-124.
Hacheney, N., S. Brown. 2017. Correctional facility adopts multiple food scrap practices. Biocycle. 58:9:34.
Harb, M. & P.Y. Hong. 2017. Anaerobic Membrane Bioreactor Effluent Reuse: A Review of Microbial Safety Concerns, Fermentation 3(3), https://doi.org/10.3390/fermentation3030039
Heckman, J.R., J.S. Angle, R.L. Chaney. 1987. Residual effects of sewage sludge on soybeans: II. Accumulation of soil and symbiotically fixed nitrogen. J. Environ. Qual. 16:118-124.
Henry, H., M.F. Naujokas, C. Attanayake, N.T. Basta, Z. Cheng, G.M. Hettiarachchi, M. Maddaloni, C. Schadt, K.G. Scheckel. 2015. Bioavailability-based in situ remediation to meet future lead (Pb) standards in urban soils and gardens. Environ. Sci. Technol. 49:8948–8958.
Hettiarachchi, G. M., G. M. Pierzynski. 2002. In situ stabilization of soil lead using phosphorus and manganese oxide: Influence of plant growth. J. Environ. Qual. 31: 564-572.
Hettiarachchi, G.M., G.M. Pierzynski, F.W. Oehme, O. Sonmez, J.A. Ryan. 2003a. Treatment of contaminated soil with phosphorus and manganese oxide reduces absorption of lead by Sprague-Dawley rats. J. Environ. Qual. 32: 1335-1345.
Hettiarachchi, G.M., J.A. Ryan, R.L. Chaney, C.M. La Fleur. 2003b. Adsorption of cadmium onto different fractions of biosolids-amended soils. J. Environ. Qual. 32: 1684-1693.
Hettiarachchi, G.M., J. A. Ryan, K. G. Scheckel, S. R. Sutton, M. Newville. 2006. µ-XANES and µ-XRF investigations of metal binding mechanisms in biosolids. J. Environ. Qual. 35: 342-351.
HB2658-2017-18. Concerning the Use of Perfluorinated Chemicals in Food Packaging.; 65th Legislature 2018 Regular Session: Washington State, 2018
Houtz, E., M. Wang, J-S. Park. 2018. Identification and Fate of Aqueous Film Forming Foam Derived Per- and Polyfluoroalkyl Substances in a Wastewater Treatment Plant. Environ. Sci. Technol. 52:13212-13221.
Hue, N.V. 2013. Arsenic chemistry and remediation in Hawaiian soils. International J. Phytoremediation, 15:105-116.
Hue, N.V. 2015. Bioremediation of arsenic toxicity. In Arsenic Toxicity and prevention. Narayan Chakrabarty (Ed.). CRC Press. pp.155-165.
Berek, A., N. Hue, T. Radovich & A. Ahmad. 2018. Biochars Improve Nutrient Phyto-Availability of Hawaii’s Highly Weathered Soils, Agronomy 8 (10), 203
Ippolito, J.A. 2015. Aluminum-based water treatment residuals use in a constructed wetland for capturing urban runoff phosphorus: Column study. Water, Air, and Soil Pollut. 226:334. DOI 10.1007/s11270-015-2604-2.
Ippolito, J.A., K.A. Barbarick, R.B. Brobst. 2015. Copper and zinc speciation in a biosolids-amended, semiarid grassland soil. J. Environ. Qual. 43:1576-1584.
Ippolito, J.A., K.A. Barbarick, M.W. Paschke, R.B. Brobst. 2010. Infrequent composted biosolids applications effect semi-arid grassland soils and vegetation. J. Environ. Management. 91:1123-1130.
Ippolito, J.A., C.M. Berry, D.G. Strawn, J.M. Novak, J. Levine, A. Harley. 2017. Biochars reduce mine land soil bioavailable metals. J. Environ. Qual. 46:411-419.
Ippolito, J.A., D.L. Bjorneberg, D.E. Stott, D.L. Karlen. 2017. Soil quality improvement through conversion to sprinkler irrigation. Soil Sci. Soc. Am. J. 81:1505-1516.
Ippolito, J.A., D.A. Laird, W.J. Busscher. 2012. Environmental benefits of biochar. J. Environ. Qual. 41:973-989.
Ippolito, J.A., K.G. Scheckel, K.A. Barbarick. 2009. Selenium adsorption to aluminum-based water treatment residuals. J. Colloid Interf. Sci. 338:48-55.
Ippolito, J.A., M.E. Stromberger, R.D. Lentz, R.S. Dungan. 2016a. Hardwood biochar and manure co-application to a calcareous soil. Chemosphere. 142:86-91.
Ippolito, J.A., T.F. Ducey, K.B. Cantrell, J.M. Novak, R.D. Lentz. 2016b. Designer, acidic biochar influences calcareous soil characteristics. Chemosphere. 142:184-191.
Jay, J.G., S.L. Brown, K. Kurtz, F. Grothkopp. 2017. Predictors of phosphorus leaching from bioretention soil media. J. Environ. Qual. 46:1098-1105
Jin, V.L., K.N. Potter, M-V. V. Johnson, R.D. Harmel, J.G. Arnold. 2015. Surface-Applied Biosolids Enhance Soil Organic Carbon and Nitrogen Stocks but Have Contrasting Effects on Soil Physical quality. Appl. Environ. Soil Sci. http://dx.doi.org/10.1155/2015/715916.
Kibuye, F. A., Gall, H. E., Elkin, K. R., Ayers, B., Veith, T. L., Miller, M., Jacob, S., Hayden, K. R., Watson, J. E. and Elliott, H. A., Mar 1 2019. Fate of pharmaceuticals in a spray-irrigation system: From wastewater to groundwater, Science of the Total Environment. 654:197-208.
Kaiser, M.L., M.L. Williams, N. Basta, M. Hand, S. Huber. 2015. When vacant lots become urban gardens: Characterizing the perceived and actual food safety concerns of urban agriculture in Ohio. J. Food Protect. 78(11):2070-2080.
Kammann, C., N. Borchard, M. Cayuela, N. Hagemann, J. Ippolito, S. Jeffery, J. Kern, D. Rasse, S. Sanna, H-P. Schmidt, K. Spokas, N. Wrage-Mönnig. 2017. Biochar as a tool to reduce the agricultural greenhouse burden – Knowns, unknowns and future research needs. J. Environ. Engineer. Landscape Management. 25:114-139.
Kannan, K. 2011. Perfluoroalkyl and polyfluoroalkyl substances: current and future perspectives. Environ. Chem. 8:333-338.
Kannan, K., S. Corsolini, J. Falandysz, G. Fillmann, K.S. Kumar, B.G. Loganathan, M.A. Mohd, J. Olivero, N. Van Wouwe, J.H. Yang, K.M. Aldous. 2004. Perfluorooctanesulfonate and related fluorochemicals in human blood from several countries. Environ. Sci. Technol. 38:4489-4495.
Kannan, K., J.C. Franson, W.W. Bowerman, K.J. Hansen, J.D. Jones, J.P. Giesy. 2001. Perfluorooctane sulfonate in fish-eating water birds including bald eagles and albatrosses. Environ. Sci. Technol. 35:3065-3070.
Kannan, K., S.H. Yun, J.T. Evans. 2005. Chlorinated, brominated, and perfluorinated contaminants in livers of polar bears from Alaska. Environ. Sci. Technol. 39:9057-9063.
Karlen, D.L., M.J. Mausbach, J.W. Doran, R.G. Cline, R.F. Harris, G.E. Shuman. 1997. Soil quality: A concept, definition, and framework for evaluation (A guest editorial). Soil Sci. Soc. Am. J. 61:4-10.
Karna, R., G.M. Hettiarachchi, M. Newville, C-J Sun, Q. Ma. 2016. Synchrotron-Based X-Ray Spectroscopy Studies for Redox-Based Remediation of Lead, Zinc, and Cadmium in Mine Waste Materials. J. Environ. Qual. 45:1883-1893.
Khaleel, R., K.R. Reddy, M.R. Overcash. 1981. Changes in soil physical properties due to organic waste applications: a review. J. Environ Qual. 10:133–141.
Kibuyea, F.A, G.E. Gall, K.R. Elkinm B. Ayers, T.L. Veith, M. Miller, S. Jacob, K.R. Hayden, J.E. Watson, and H.A. Elliot. 2019. Fat of pharmaceuticals in a spray-irrigation system: From wastewater to groundwater. Sci. Total Environ., 654:182-208.
Kirchmann, H., M.H. Gerzabek, (Eds.). 2002. Pore size changes in a long-term field experiment with organic amendments. Elsevier Science B.V., Amsterdam, Netherlands.
Koropchak, S., W. Daniels, A. Wick, G.R. Whittecar, N. Haus. 2015. Beneficial use of dredge materials for soil reconstruction and development of dredge screening protocols. J. Environ. Qual. 45:62-73.
Krippner, J., H. Brunn, S. Falk, S. Georgii, S. Schubert, T. Stahl. 2014 Effects of chain length and pH on the uptake and distribution of perfluoroalkyl substances in maize (Zea mays). Chemosphere 94:85-90.
Kulesza, S.B., R.O. Maguire, K. Xia, J. Cushman, K.F. Knowlton, P. Ray. 2016. Impact of manure injection on pirlimycin transport in surface runoff. J. Environ. Qual. 45:511–518.
Laidlaw, M.A.S., G.M. Filippelli, S. Brown, J. Paz-Ferreiro, S.M. Reichman, P. Netherway, A. Truskewzcz, A.S. Ball, H.W. Mielke. 2017. Case studies and evidence-based approaches to addressing urban soil lead contamination. Appl. Geochem. 83:14-30.
Laird, D.A., J.M. Novak, H.P. Collins, J.A. Ippolito, D.L. Karlen, R.D. Lentz, K.R. Sistani, K. Spokas, R.S. Van Pelt. 2017. Multi-year and multi-location soil quality and crop biomass yield responses to hardwood fast pyrolysis biochar. Geoderma. 289:46-53.
Lau, C., J.R. Thibodeaux, R.G. Hanson, M.G. Narotsky, J.M. Rogers, A.B. Lindstrom, M.J. Strynar. 2006. Effects of perfluorooctanoic acid exposure during pregnancy in the mouse. Toxicol. Sci. 90:510-518.
Kim Lazcano, R., C. de Perre, M.L. Mashtare and L.S. Lee. 2019. Per- and Polyfluoroalkyl Substances in Commercially Available Biosolid-Based Fertilizer Products: The Effect of Post-treatment Processes, Water Environ. Research, WER-2019-04-0209
Kim Lazcano, R., C. de Perre, M.L. Mashtare and L.S. Lee. 2019. Characterizing Per- and Polyfluoroalkyl Substances in Commercially Available Biosolid- versus Nonbiosolid-based Organic Fertilizers, In submission to Environmental Science & Technology.
Kitajima, M., B.C. Iker, I.L. Pepper, and C.P. Gerba. Relative abundance and treatment reduction of viruses during wastewater treatment processes — Identification of potential viral indicators. Sci. Total Environ. 488-489:290-296.
Lauriault, L.M., M.K. Darapuneni, G.K. Martinez, J. Box1, P.L. Cooksey, J. Jennings, S. Jenings & A. Williams. 2018. Impact of Water Source on Alfalfa Establishment and Production, https://tucumcarisc.nmsu.edu/documents/2018-annual-report.pdf
Le, H.T.V., R.O. Maguire, K. Xia. 2018. Method of dairy manure application and time before rainfall affects antibiotics in surface runoff. J. Environ. Qual. 47:1310-1317.
Lee, H., A.G. Tevlin, S.A. Mabury, S.A. Mabury. 2014. Fate of Polyfluoroalkyl Phosphate Diesters and Their Metabolites in Biosolids-Applied Soil: Biodegradation and Plant Uptake in Greenhouse and Field Experiments. Environ. Sci. Technol. 48:340-349.
Letcher, R.J., G.Y. Su, J.N. Moore, L.L. Williams, P.A. Martin, S.R. de Solla, W.W. Bowerman. 2015. Perfluorinated sulfonate and carboxylate compounds and precursors in herring gull eggs from across the Laurentian Great Lakes of North America: Temporal and recent spatial comparisons and exposure implications. Sci. Total Environ. 538:468-477.
Li, J., G.K. Evanylo. 2013. The effects of long-term application of organic amendments on soil organic carbon accumulation. Soil Sci. Soc. Am. J. 77:964–973.
Li, J., K. Li, X-Y. Cui, N.T. Basta, L-P. Li, H-B. Li, L.Q. Ma. 2015. In vitro bioaccessibility and in vivo relative bioavailability in 12 contaminated soils: Method comparison and method development. Sci. Total Environ. 532:812-820.
Li, Y., J.B. Sallach, W. Zhang, S.A. Boyd, H. Li. 2019. Insight into the Distribution of Pharmaceuticals in Soil-Water-Plant Systems. Water Res. 152:38-46.
Lindstrom, A.B., M.J. Strynar, A.D. Delinsky, S.F. Nakayama, L. McMillan, E.L. Libelo, M. Neill, L. Thomas. 2011. Application of WWTP Biosolids and Resulting Perfluorinated Compound Contamination of Surface and Well Water in Decatur, Alabama, USA. Environ. Sci. Technol. 45:8015-8021.
Liu, Z.Y., Y.L. Lu, Y.J. Shi, P. Wang, K. Jones, A.J. Sweetman, A.C. Johnson, M. Zhang, Y.Q. Zhou, X.T. Lu, C. Su, S. Sarvajayakesavaluc, K. Khan. 2017. Crop bioaccumulation and human exposure of perfluoroalkyl acids through multi-media transport from a mega fluorochemical industrial park, China. Environ. Int. 106:37-47.
Loganathan, B.G., K.S. Sajwan, E. Sinclair, K.S. Kumar, K. Kannan. 2007. Perfluoroalkyl sulfonates and perfluorocarboxylates in two wastewater treatment facilities in Kentucky and Georgia. Water Res. 41:4611-4620.
Mehmood, K., E. Chávez Garcia, M. Schirrmann, B. Ladd, C. Kammann, N. Wrage-Mönnig, C. Siebe, J.M. Estavillo, T. Fuertes, M. Cayuela, G. Sigua, K. Spokas, A.L. Cowie, J. Novak, J.A. Ippolito, N. Borchard. 2017. Biochar research activities and their relation to development and environmental quality. A meta-analysis. Agron. Sustain. Dev. 37:22.
Meinhardt, K.A., A. Bertagnolli, M. Pannu, S.E. Strand, S.L. Brown, D.A. Stahl. 2015. Evaluation of revised polymerase chain reaction primers for more inclusive quantification of ammonia-oxidizing archaea and bacteria. Environ. Microbiology Reports, 7:2:354-363.
Metzger, I., B. Yaron. 1987. Influence of sludge organic matter on soil physical properties. Adv. Soil Science. 7:141–163.
Mina, O., Gall, H. E., Saporito, L. S., Elliott, H. A. & Kleinman, P. J. A., 2017. Relative role of transport and source-limited controls for estrogen, TDP, and DOC export for two manure application methods, Agriculture, Ecosystems and Environment. 247:308-318.
Mina, O., Gall, H. E., Elliott, H. A., Watson, J. E., Mashtare, M. L., Langkilde, T. L., Harper, J. P. & Boyer, E. W., Apr 1 2018. Estrogen occurrence and persistence in vernal pools impacted by wastewater irrigation practices, Agriculture, Ecosystems and Environment. 257:103-112.
Morris, J., S. Brown, M. Cotton, H.S. Matthews. 2017. Life-cycle assessment harmonization and soil science ranking results on food-waste management methods. Environ. Sci. Technol. 51:5360-5367.
National Research Council. 2009. Urban stormwater management in the United States. Washington, DC: Press. Available at: https://www.nap.edu/catalog/12465/urban-stormwater-management-in-the-united-states.
Nelson, W.B., N.T. Basta, R. Chaney, P.F.P. Henry, T. May, D. Mosby, B.A. Rattner, K.G. Scheckel, D. Sprague. 2016. Bioaccessibility tests accurately estimate bioavailability of lead to quail. Environ. Toxicol. Chem. 35:2311-2319.
Novak, J.M., J.A. Ippolito, R.D. Lentz, K.A. Spokas, C.H. Bolster, K. Sistani, K.M. Trippe, M.G. Johnson. 2016. Soil health, crop productivity, microbial transport, and mine spoil response to biochars. Bioenerg. Res. 9:454-464.
Navarro, I., A. de la Torre, P. Sanz, M.A. Porcel, J. Pro, G. Carboneb, M.D. Martinez. 2017. Uptake of perfluoroalkyl substances and halogenated flame retardants by crop plants grown in biosolids-amended soils. Environ. Res. 152:199-206.
Obrycki, J.F., N.T. Basta, K. Scheckel, A. Juhasz, B.N. Stevens, K.K. Minca. 2016. Phosphorus amendment efficacy on soil Pb depends upon bioaccessible method conditions. J. Environ. Qual. Special Issue: Soil in the City. 45(1): 37-44.
Obrycki, John F., Nicholas T. Basta, Steven W. Culman. 2017. Management Options for Contaminated Urban Soils to Reduce Public Exposure and Maintain Soil Health. J. Environ. Qual. 46:420-430.
Obrycki, J.F., N.T. Basta, R.S. Wilson. 2017. Evaluating public and regulatory acceptance for urban soil management approaches. J. Environ. Qual. 46: 20-26.
Oh, M.; A. Pruden, C. Q. Chen, L. Heath, K. Xia, and L. Q. Zhang. 2018. MetaCompare: A computational pipeline for prioritizing environmental resistome risk. FEMS Microbiology Ecology, 94, 2018, fiy079. doi: 10.1093/femsec/fiy079.
Ohno, T., G.M. Hettieaachchi. 2018. Soil chemistry and the One Health Initiative: Introduction to the special section. J. Environ. Qual. 47:1305-1309.
Orndorff, Z., W. Daniels, C. Zipper, M. Eick, M. Beck. 2015. A column evaluation of Appalachian coal mine spoils’ temporal leaching behavior. Environ. Pollut. 204:39-47.
Ozores-Hampton, M., P.A. Stansly, T.P. Salame. 2011. Soil Chemical, Physical, and Biological Properties of a Sandy Soil Subjected to Long-Term Organic Amendments. J. Sustain. Ag. 35:243-259.
Pagliai, M., L.V. Antisari. 1993. Influence of waste organic matter on soil micro- and macrostructure. Bioresource Technol. 43:205–213.
Paredez, J.M, N. Mladenov, M.B. Galkaduwa, G.M. Hettiarachchi, G.J. Kluitenberg, S.L. Hutchinson. 2017. A soil column study to evaluate treatment of trace elements from saline industrial wastewater. Journal of Water Science and Technology. 76:2698-2709.
Pepper, I.L., J.P. Brooks, C.P. Gerba. 2018. Antibiotic resistant bacteria in municipal wastes: is there reason for concern? Environ. Sci. Technol. 52:3949-3959.
Pepper, I.L., C.P. Gerba. 2018. Risk of infection from Legionella associated with spray irrigation of reclaimed water. Wat. Res. 139:101-107.
Pietrzykowski, M., W.L. Daniels, S.C. Koropchak. 2015. Microtopographic effects on growth of young bald cypress (Taxodium distichum L.) in a created freshwater forested wetland in southeastern Virginia. Ecol. Eng. 83:135-143.
Prevedouros, K., I.T. Cousins, R.C Buck, S.H. Korzeniowski. 2006. Sources, fate and transport of perfluorocarboxylates. Environ. Sci. Technol. 40:32-44.
Punshon, T., D.C. Adriano, J.T. Weber. 2002. Restoration of drastically eroded land using coal fly ash and poultry biosolid. Sci. Tot. Environ. 296:209-225.
Qin, C., C. Chen, C. Shang, K. Xia. 2017. Fe3+-Saturated Montmorillonite Effectively Deactivates Microorganisms in Wastewater. Sci. Total Environ. 622-623:88-95.
Rachmadi, A.T., M. Kitajima, I.L. Pepper, C.P. Gerba. 2016. Enteric and indicator virus removal by surface flow wetlands. Sci. Tot. Environ. 542:976-982.
Ray, P, C.Q. Chen, K. F. Knowlton, A. Pruden, K. Xia. 2017. Fate and effect of antibiotics in beef and dairy manure during static and turned composting. J. Environ. Qual. 46:45-54.
Rodriguez, R.R., N.T. Basta, S.W. Casteel, F.P. Armstrong, D.C. Ward. 2003. Chemical extraction methods to assess bioavailable As in contaminated soil and solid media. J. Environ. Qual. 32:876-884.
Rousseau, G.X., O. Deheuvels, I. Rodriguez Arias, E. Somarriba. 2012. Indicating soil quality in cacao-base agroforestry systems and old-growth forests: The potential of soil microfauna assemblage. Ecol. Indic. 23:535-543.
Sassi, H.P., L.A. Ikner,S. Abd-Elmaksoud, C.P. Gerba, I.L. Pepper. 2018a. Comparative survival of viruses during thermophilic and mesophilic anaerobic digestion. Sci. Tot. Environ. 615:15-19.
Sassi, H.P., K.A. Reynolds, I.L. Pepper, I.L., C.P. Gerba. 2018b. Evaluation of hospital-grade disinfectants on viral deposition on surfaces after toilet flushing. Am. J. Infect. Cont. 46:507-511.
Schmitz, B., M. Kitajima, M. Campillo, C. Gerba, I. Pepper. 2016a. Virus reduction during advanced Bardenpho and conventional wastewater treatment processes. Environ. Sci. Technol. 50:9524-9532.
Schmitz, B., J. Pearce-Walker, C.P. Gerba, I.L. Pepper. 2016b. A method for determining Ascaris viability based on early-to-late stage in vitro ova development. J. Res. Sci. Technol. 13:275-286.
Schnell, R.W., D.M. Vietor, C.L. Munster, R.H. White, T.L. Provin. 2010. Effect of turfgrass establishment practices and composted biosolids on water quality. J. Environ. Qual. 39:697-705.
Schultz, M.M., C.P. Higgins, C.A. Huset, R.G. Luthy, D.F. Barofsky, J.A. Field. 2006. Fluorochemical mass flows in a municipal wastewater treatment facility. Environ. Sci. Technol. 40:7350-7357.
Sepulvado, J. G.; Blaine, A. C.; Hundal, L. S.; Higgins, C. P. 2011. Occurrence and Fate of Perfluorinated Compounds in Soil Following Land Application of Biosolids. Environ. Sci. Technol. 45:8106–8112.
Sinclair, E., K. Kannan. 2006. Mass loading and fate of perfluoroalkyl surfactants in wastewater treatment plants. Environ. Sci. Technol. 40:1408-1414.
Silveira, M.L., G.A. O’Connor, J. Vendramini. 2017. Utilization of biosolids in forage production systems in Florida. SL444 (EDIS # SS658).
Schlosser, C.A., K.M. Strzepek, X. Gao, A. Gueneau, C. Fant, S. Paltsev, B. Rasheed, T. Smith-Greico, E. Blanc, H.D. Jacoby, J.M. Reilly. Joint Program Report Series. Environmental Science & Technology, 2014, pp. 30.
Sepulvado, J. G., Blaine, A. C., Hundal, L. S., and Higgins, C. P. 2011. Occurrence and fate of perfluorochemicals in soil following the land application of municipal biosolids. Environmental science & technology, 45(19):8106-8112
Sherchan, S.P., S.A. Snyder, C.P. Gerba et al. Online monitoring of Escherichia coli and Bacillus thuringiensis spore inactivation after advanced oxidation treatment. J. Env. Sci. Hlth. Part A. 49:933-939.
Sherchan, S., C.P. Gerba, and I.L. Pepper. Evaluation of Real-Time Water Quality Sensors for the Detection of Intentional Spore Contamination of Potable Water. . J. Biosensors & Bioelectronics 4:141.
Sherchan, S., S.A. Snyder, C.P. Gerba, and I.L. Pepper. Inactivation of MS2 Coliphage by UV and Hydrogen Peroxide: Comparison by Cultural and Molecular Methodologies. J. Env. Sci. Hlth., Part A. 49:397-403.
Sidhu, H.S.,, E. D'Angelo, G.A. O'Connor. 2019a. Retention-release of ciprofloxacin and azithromycin in biosolids and biosolids-amended soils. Sci. Total Environ., 650:173-183.
Sidhu, H.S.,, G.A. O'Connor, A. Ogram, K. Kumar. 2019b. Bioavailability of biosolids-borne ciprofloxacin and azithromycin to terrestrial organisms: microbial toxicity and earthworm responses. Sci. Total Environ., 650:18-26.
Sidhu, H.S., O'Connor, G, & McAvoy, D. 2019c. Risk assessment of biosolids-borne ciprofloxacin and azithromycin. Sci Total Environ,, 651:3151-3160.
Sidhu, H.S., G.A. O'Connor, J. Kruse. 2019c. Plant toxicity and accumulation of biosolids-borne ciprofloxacin and azithromycin, Sci. Total Environ., 648:1219-1226.
Singer, R. & S Brown. 2018. Impact of Soil Filtration on Metals, Nutrients, and Estrogenic Activity of Reclaimed Water, J. Environ. Quality, 47(6):1504-1512.
Snyder, E.H., G.A. O'ConnorRisk assessment of land-applied biosolids-borne triclocarban (TCC)., Sci. Total Environ., 442 (2013), pp. 437-444
Spokas, K.A, R. Weis, G. Feyereisen, D.W. Watts, J.M. Novak, T.J. Lee, J.A. Ippolito. 2017. Biomass or biochar – Which is better at improving soil hydraulic properties? Acta Horticulturae. 1146.31:235-242.
Stahl, T., R.A. Riebe, S. Falk, K. Failing, H. Brunn. 2013. Long-Term Lysimeter Experiment To Investigate the Leaching of Perfluoroalkyl Substances (PFASs) and the Carry-over from Soil to Plants: Results of a Pilot Study. J. Agric. Food Chem. 61:1784-1793.
Stahl, L.L., B.D. Snyder, A.R. Olsen, T.M. Kincaid, J.B. Wathen, H.B. McCarty. 2014. Perfluorinated compounds in fish from US urban rivers and the Great Lakes. Sci. Total. Environ. 499:185-195.
Stevenson, B.A., S. McNeill, A.E. Hewitt. 2015. Characterising soil quality clusters in relation to land use and soil order in New Zealand: An application of the phenoform concept. Geoderma. 239-240:135-142.
Stoffella, P.J., M. Ozores-Hampton, N.E. Roe, Y.C. Li, T.A. Obreza. 2003. Compost utilization in vegetable crop production systems. Acta horticulturae. 125-128.
Sullivan, T.S., M.E. Stromberger, M.W. Paschke, J.A. Ippolito. 2005. Long-term impacts of infrequent biosolids application on chemical and microbial properties of a semi-arid rangeland soil. Biol. Fert. Soils. 42:258-266.
Sydnor, M.E.W., E.F. Redente. 2002. Reclamation of High-Elevation, Acidic Mine Waste with Organic Amendments and Topsoil. J. Environ. Qual. 31:1528-1537.
Taniyasu, S., K. Kannan, Y. Horii, N. Hanari, N. Yamashita. 2003. A survey of perfluorooctane sulfonate and related perfluorinated organic compounds in water, fish, birds, and humans from Japan. Environ. Sci. Technol. 37:2634-2639.
Tian, G., A. Cox, K. Kumar, T. Granato. G. O'Connor, H. Elliott. 2016. Assessment of plant availability and environmental risk of biosolids-phosphorus in a U.S. Midwest corn-belt soil. J. Environ. Mgt. 172: 171-176.
Tripathi, R., A.K. Shukla, Md. Shahid, D. Nayak, C. Puree, S. Mohanty, R. Raja, B. Lal, P. Gautam, P. Bhattacharyya, B.B. Panda, A. Kumar. 2016. Soil quality in mangrove ecosystem deteriorates due to rice cultivation. Ecol. Engineer. 90:163-169.
US EPA. 2018. National overview: Facts and figures on materials, wastes and recycling. Available at: https://www.epa.gov/facts-and-figures-about-materials-waste-and-recycling/national-overview-facts-and-figures-materials (verified December 14, 2018).
US EPA. 2008a. Fate, Transport and Transformation Test Guidelines: OPPTS 835.0001 Principles and Strategies Related to Biodegradation Testing of Organic Chemicals under the Toxic Substances Control Act (TSCA).
US EPA. 2008b. Fate, Transport and Transformation Test Guidelines: OPPTS 835.1230 Adsorption/Desorption (Batch Equilibrium).
US EPA. 2007a. Method 1694: Pharmaceuticals and Personal Care Products in Water, Soil, Sediment, and Biosolids by HPLC/MS/MS. U.S. Environmental Protection Agency, Office of Water, Office of Science and Technology Engineering and Analysis Division (4303T), Washington, DC. EPA-821-R-08-002.
US EPA. 2007b. Method 1614: Brominated Diphenyl Ethers in Water Soil, Sediment and Tissue by HRGC/HRMS. U.S. Environmental Protection Agency, Office of Water, Office of Science and Technology Engineering and Analysis Division (4303T), Washington, DC. EPA-821-R-07-005.
US EPA. 1998. Fate, Transport and Transformation Test Guidelines: Sediment and Soil Adsorption/Desorption Isotherm. EPA 712–C–98–048.
Venkatesan, A.K., R.U Halden. 2013 National inventory of perfluoroalkyl substances in archived US biosolids from the 2001 EPA National Sewage Sludge Survey. J. Hazard. Mat.252:413-418.
Washington, J. W.; Yoo, H.; Ellington, J. J.; Jenkins, T. M.; Libelo, E. L. 2010. Concentrations, distribution, and persistence of perfluoroalkylates in sludge-applied soils near Decatur, Alabama, USA. Environmental science & technology, 44(22):8390-8396.
Wen, B., L.F. Li, H.N. Zhang, Y.B. Ma, X.Q. Shan, S.Z. Zhang. 2014. Field study on the uptake and translocation of perfluoroalkyl acids (PFAAs) by wheat (Triticum aestivum L.) grown in biosolids-amended soils. Environvironmental Pollution 184:547-554.
Wen, B., L.F. Li, Y. Liu, H.N. Zhang, X.Y. Hu, X.Q. Shan, S.Z. Zhang. 2013. Mechanistic studies of perfluorooctane sulfonate, perfluorooctanoic acid uptake by maize (Zea mays L. cv. TY2). Plant Soil. 370:345-354.
Wen, B., Y.L. Wu, H.N. Zhang, Y. Liu, X.Y. Hu, H.L. Huang, S.Z. Zhang. 2016. The roles of protein and lipid in the accumulation and distribution of perfluorooctane sulfonate (PFOS) and perfluorooctanoate (PFOA) in plants grown in biosolids-amended soils. Environ. Pollut. 216:682-688.
Whitacre, S.D., N.T. Basta, B.N. Stevens, V. Hanley, R.H. Anderson, K.G. Scheckel. 2017. Modification of an Existing In vitro Method to Predict Relative Bioavailable Arsenic in Soils. Chemosphere 180:545-552.
White, R.E., S.I. Torri, R.S. Correa. 2011. Biosolids soil application: Agronomic and environmental implications. Appl. Environ. Soil Sci. http://dx.doi.org/10.1155/2011/928973.
Whitacre, S.D., N.T. Basta, B.N. Stevens, V. Hanley, R.H. Anderson, K.G. Scheckel. 2017. Modification of an Existing In vitro Method to Predict Relative Bioavailable Arsenic in Soils. Chemosphere 180:545-552.
Wijesekara, H., N.S. Bolan, M. Vithanage, Y.Xu, S. Mandal, S.L. Brown, G.M. Hettiarachchi, G.M. Pierzynski, L. Huang, Y. S. Ok, M.B. Kirkham, C. Saint. A. Surapaneni. 2016. Utilization of biowaste for mine spoil rehabilitation. Adv. Agron. 138:97-173.
Wind, L., L. H. Krometis, W. C. Hession, C. Q. Chen, P. Du, K. Jacobs, K. Xia, and Amy Pruden. 2018. Fate of Pirlimycin and Antibiotic-Resistant Fecal Coliforms in Field Plots Amended with Dairy Manure or Compost during Vegetable Cultivation. J. Environ. Qual. 47:436-444.
Xia, K., L.S. Hundal, K. Kumar, K. Armbrust, A.E. Cox, T.C. Granato. 2010. Triclocarban, triclosan, polybrominated diphenyl ethers, and 4-nonylphenol in biosolids and in soil receiving 33-year biosolids application. Environ. Toxic. Chem. 29:597-605.
Xu, H., X. Qu, H. Li, C. Gu, D. Zhu. 2014. Sorption of Tetracycline to Varying-Sized Montmorillonite Fractions. J. Environ. Qual. 43:2079-2085.
Yarwood, S., A. Wick, M. Williams, W. Daniels. 2015. Parent material and vegetation influence early soil microbial community establishment following 30-years of rock weathering. Microbial. Ecol. 69:383-94.
Yoo, H., J.W. Washington, T.M. Jenkins, J.J. Ellington. 2011. Quantitative Determination of Perfluorochemicals and Fluorotelomer Alcohols in Plants from Biosolid-Amended Fields using LC/MS/MS and GC/MS. Environ. Sci. Technol. 45:7985-7990.
Zhang, X., D. Zhou, E.H. Ervin, G.K. Evanylo, D. Cataldi, and J. Li. 2012. Biosolids impact antioxidant metabolism associated with drought tolerance in tall fescue. HortScience 47(10):1550-1555.
Zhang, X., Ervin, E. H., Evanylo, G. K., Li, J. and Harich, K. 2013. Corn and soybean hormone and antioxidant metabolism responses to biosolids under two cropping systems. Crop Sci. 53, 2079–2089.
Zhang, G. J. Bai, M. Xi, A. Zhao, Q. Lu, J. Jia. 2016. Soil quality assessment of coastal wetlands in the Yellow River Delta of China based on the minimum data set. Ecol. Indic. 66:458-466.
Zhang, Y., S.A. Boyd, B.J. Teppen, J.M. Tiedje, H. Li. 2014a. Role of Tetracycline Speciation in the Bioavailability to Escherichia coli for Uptake and Expression of Antibiotic Resistance. Environ. Sci. Technol. 48:4893-4900.
Zhang, Y., S.A. Boyd, B.J. Teppen, J.M. Tiedje, H. Li. 2014b. Organic Acids Enhance Bioavailability of Tetracycline in Water to Escherichia coli for Uptake and Expression of Antibiotic Resistance. Water. Res. 65:98-106.
Zhang, H.N., B. Wen, X.Y. Hu, Y.L. Wu, Y. Pan, H.L. Huang, L. Liu, S.Z. Zhang. 2016. Uptake, Translocation, and Metabolism of 8:2 Fluorotelomer Alcohol in Soybean (Glycine max L. Merrill). Environ. Sci. Technol. 50:13309-13317.
Zhao, S.Y., T. Zhou, L.Y. Zhu, B.H. Wang, Z. Li, L.P. Yang, L.F. Liu. 2018. Uptake, translocation and biotransformation of N-ethyl perfluorooctanesulfonamide (N-EtFOSA) by hydroponically grown plants. Environ. Pollut. 235:404-410.
Zhou, L.N., M.J. Xia, L. Wang, H. Mao. 2016 Toxic effect of perfluorooctanoic acid (PFOA) on germination and seedling growth of wheat (Triticum aestivum L.). Chemosphere. 159:420-425.