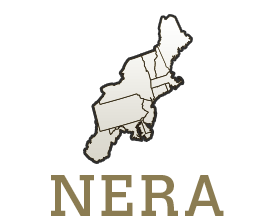
NE1335: Resource Management in Commercial Greenhouse Production
(Multistate Research Project)
Status: Inactive/Terminating
NE1335: Resource Management in Commercial Greenhouse Production
Duration: 10/01/2013 to 09/30/2018
Administrative Advisor(s):
NIFA Reps:
Non-Technical Summary
Statement of Issues and Justification
The team members represent multi-institutional and interdisciplinary collaborations. Our members include the following representatives and focus areas:
Greenhouse Engineering:
A.J. Both, Rutgers University
Richard Gates, University of Illinois
Gene Giacomelli, University of Arizona
Murat Kacira, University of Arizona
Peter Ling, Ohio State University
George Meyer, University of Nebraska
Plant Production and Physiology:
Stephanie Burnett, University of Maine
Martin Gent, Connecticut Agricultural Experiment, New Haven
Meriam Karlsson, University of Alaska
Chieri Kubota, University of Arizona
Joyce Latimer, Virginia Tech
Richard McAvoy, University of Connecticut, Storrs
Neil Mattson, Cornell University
George Wulster, Rutgers University
Economics:
Robin Brumfield, Rutgers University
Statement of Issues and Justification
Statement of Issues:
Horticulture, including greenhouse production, is a vibrant and economically important sector of agriculture. The wholesale value of horticulture specialty crops (defined as greenhouse, nursery, and related crops such as seeds and vegetable transplants) was $11.7 billion in 2009, the last year such comprehensive data was available (USDA NASS, 2010). The members of our group serve growers in several states (AK, AZ, CT, ME, NE, NJ, NY, OH, PA, and VA) that encompass a large range of climate and growing conditions. Greenhouse and nursery production is ranked as the top grossing agricultural commodity in two member states: CT and NJ (USDA NASS, 2007). Greenhouse production, a.k.a. controlled environment agriculture (CEA), is particularly important for growers faced with temperate and colder climates that make structures such as greenhouses and high tunnels vital for season extension and year-round production of flowers and edibles.
Over the last decade, growers have been faced with resource management issues, particularly related to fuels for heat and water for irrigation, that have significantly impacted their livelihoods. Energy prices (oil in particular) have risen dramatically and as a result of shortages in some parts of the country, water (used mainly for crop irrigation) has not always been available in sufficient quantities to grow horticultural crops using traditional production practices. While year-round crop production in greenhouse facilities often requires significant amounts of water and energy, the fact that all aspects of the growing environment can be accurately controlled typically results in much higher resource use efficiencies per unit produced compared to outdoor field production. Hence the optimized resource management is vital for the successful future of CEA operations.
Greenhouse and nursery facilities are high input systems using vast amounts of water, fertilizers, chemicals, plastics, and labor to produce crops. Consumers are exhibiting greater degrees of environmental awareness, mass marketers are adopting strict purchasing guidelines that encourage environmental sustainability, and government policies to reduce carbon emissions have resulted in a demand for crops that not only meet aesthetic expectations but are also produced, distributed, and marketed using sustainable methods. The use of renewable and biodegradable inputs while growing an aesthetically pleasing and healthy plant will meet these demands. Green industry stakeholders have identified production practices which reduce plastic and water use as a major focus to increase sustainability even though the environmental and economic costs associated with these specific practices are undetermined.
The members of our team represent a complementary knowledge base that is absolutely essential for solving the resource management challenges facing the greenhouse industry. Members include greenhouse engineers, plant scientists and an economist with diverse areas of expertise and all have experience solving problems in multidisciplinary environments. Team members have frequent interactions with a wide variety of industry stakeholders and communicate regularly through presentations at regional and national meetings, trade publications, onsite grower visits, and informal contacts resulting from grower questions. In addition, they work closely with a large number of Extension agents in their respective states.
Our team proposes to address resource management issues related to water and nutrient applications, as well as to energy use. We propose to use sensors to collect improved information about the plant status, the growing environment and outdoor conditions. Thus, the whole continuum in the greenhouse and crop system is considered, evaluated, and decisions are made for optimized resource use. We want to develop and implement environmental control strategies that optimize resource management and maximize crop quality and yield, while also maximizing the economic return to the grower.
Justification:
The increased severity and duration of water shortages throughout many parts of the United States have revealed the importance of water management for crop production, including crops grown in CEA facilities. Climate change has, and will continue to, create prolonged drought that will make water an even more important issue for CEA in the future (IPCC, 2008). In some parts of the United States, water is limited not only in quantity (Ackerman and Stanton, 2011), but also in quality (e.g., hardness, acidity level, high or low concentrations of specific nutrients, contamination with undesirable constituents). Water management is closely tied to nutrient management, particularly in greenhouse production where plants typically receive nutrients along with every irrigation event (termed fertigation). When crops are fertigated, careful attention to watering practices such as the use of sensor-based irrigation or water capture and reuse are thereby also important to reduce the quantity of fertilizer applied (Burnett and van Iersel, 2008; Rose and White, 1994). A reduction in fertilizer inputs can directly reduce the environmental impact related to fertilizer mining and production. In addition, nutrients readily leach from root substrates and this can cause environmental problems on site and in neighboring soils and/or bodies of water (Bilderback, 2002). CEA facilities can be outfitted with systems that maximize water use efficiency while at the same time minimize or eliminate leachate from contaminating the outdoor environment (e.g., recirculating ebb and flood irrigation). However, only a limited number of greenhouse operations use the latest water and nutrient management technologies. Our team believes that further reductions in water and nutrient use can result in improved economics for CEA operations and enhance their environmental sustainability.
At the same time, significant increases in oil prices over the last decade have had a major impact on the greenhouse industry (Brumfield et al., 2009). In order to stay in business, growers have been looking for ways to reduce their fuel consumption (Mears et al., 2009; Both and Mears, 2008). They have implemented strategies to reduce greenhouse heat loss (Both et al., 2007b), installed more energy efficient equipment (Reiss et al., 2007) and investigated alternative energy sources (Both et al., 2011, Mears et al., 2009). While natural gas prices have not seen such a dramatic increase compared to oil prices, growers using natural gas have also been impacted because many supplies and most transportation depends on oil. Reductions in energy consumption per unit growing area also reduce the carbon footprint of greenhouse operations and this is becoming an important issue as consumers are increasingly concerned about sustainable production practices. Our team believes that additional energy savings are feasible and that they can improve the bottom line for CEA operations.
One of the major benefits of growing plants in a greenhouse is the ability to use environmental sensors to monitor the production environment and plant responses so as to make informed decisions about production processes such as fertilization, irrigation, heating, cooling, and lighting (Kacira and Ling, 2001; Kacira et al., 2002a, b; Nemali and van Iersel, 2006; Meyer and Camargo, 2008; Meyer et al., 2009; Story et al., 2010; Streimer et al., 2011, Kim et al., 2011; Li et al., 2012). Advanced growers utilize computer control systems and a variety of sensors and senor networks to accurately manage the greenhouse environment. The benefit of these systems is that they reduce temporal and spatial variation in the greenhouse environment, thus improving the conditions for uniform and predictable plant production. In addition, accurate climate control and proper control strategies can help reduce waste of resources and improve resource use efficiency. A variety of sensors are available in the market, but the challenge is to convince more growers of their value and teach them their proper use. Our team believes that sensors and control strategies are indispensible tools for efficient resource management in greenhouse operations.
Related, Current and Previous Work
Water and Nutrient Management Our group has explored a variety of techniques to improve water management efficiency in CEA. These include the use of sensor-automated irrigation systems, climate control strategies and the use of closed, sub-irrigation systems where excess irrigation water is captured and reused. Our work has not only focused on saving water, but also on maintaining a desired greenhouse internal climate. Finally, our research has explored techniques for reducing nutrient use and runoff in greenhouse production. Because operations vary in the crops they produce, growing facilities, and their ability to meet new capital expenditures, a one-size fits all approach to conserving irrigation water is not feasible. Therefore our group members investigate several parallel approaches frequently sharing their results and incorporating feedback from other members into new research. For example, ME used a capacitance sensor based automated irrigation system to improve the sustainability of greenhouse irrigation (Nemali and van Iersel, 2006). This system automates irrigation based on the volumetric water content of the soil (VWC = volume of water ÷ volume of soil). We have the ability to irrigate at VWC set points and are thus able to maintain relatively constant VWC in substrates. Using this system, we have determined how morphology and physiology of greenhouse-grown plants respond to VWC. For example, Heuchera americana Dale's Strain, or American alumroot, has the greatest shoot biomass when maintained at a VWC of 0.35 L· L-1. Plants grown at lower VWC have lower leaf water and osmotic potential (petunia) and biomass (Heuchera, petunia, and Gaura lindhiemeri; Burnett and van Iersel, 2008; Garland et al, 2012; van Iersel et al., 2010). The work demonstrates that high quality marketable plants can be grown with very little water so long as irrigation is precisely controlled such as by capacitance sensors. Daily water use was 100 mL/plant (American alumroot) or 12 to 44 mL/plant (petunia). This irrigation system is a zero-leach system and eliminates nutrient run-off from greenhouses. Group members at the University of Nebraska have researched and successfully implemented capillary mats as a sub-irrigation system that reduces water and fertilizer consumption but has lower capital costs than flood floors or benches. In this system, capillary mat irrigation is automated via capacitance sensors similar to the drip irrigation system utilized in Maine. This irrigation system and other low-tech engineering solutions developed at the University of Nebraska, have allowed farmers in Nebraska to explore greenhouse-grown strawberries as an off-season crop so that they may stay profitable during long winters. Researchers in CT collaborated with Geremia Farms, Inc. to test the effects of partial- and full-saturation in closed sub-irrigation systems on crop growth and disease management. The objectives of this research were to characterize the effects of these irrigation regimes on plant growth and development, disease transmission, post-harvest quality, and nutrient and water use efficiency. A variety of crops and container configurations have been tested in an effort to optimize management practices. Geranium and poinsettia under partial-saturation took up 20-30% less water and fertilizer over the course of a crop cycle than plants under full saturation. Biomass until flower development and bloom, and stem height, were also reduced by 10-20% under partial saturation. Overall, plants were more compact under partial saturation (Gent and McAvoy, 2011), which could enhance their shipping and retail shelf-life characteristics. Excessive irrigation leading to a water-logged substrate can promote the development of root diseases. Partial-saturation sub-irrigation also shows promise with respect to disease control. In one experiment with Poinsettia, inoculation with Pythium resulted in wilting of 20 of 240 non-inoculated plants grown under full saturation. By comparison, no plants were affected under partial saturation (Gent et al, 2011). In a second study, poinsettia, geranium or chrysanthemum crops were grown under partial- and full- saturation sub-irrigation in five separate experiments. For each experiment, plants were artificially inoculated with Pythium aphanidermatum or Pythium ultimum. In three of the five experiments, disease was significantly reduced under partial saturation as compared to the full-saturation. In some instances, root infection as evidenced by re-isolation of the pathogen on agar, was less on plants receiving partial saturation than on standard saturation (Elmer et al, 2012). Another option our group has explored to reduce both disease and drought stress is the use of Si in greenhouse production. Recent research at both NY and CT found that when poinsettia plants were exposed to this beneficial nutrient in irrigation water, they showed increased tolerance to severe water stress and reduced disease. Our work has also focused on examining methods to reduce fertilizer run-off from greenhouse production. Overhead irrigation is the most commonly used irrigation method for commercial containerized plant production. However, sprinkler and hand-watering methods can be very inefficient because much water falls in-between containers and excess water intercepted by containers can cause nutrient leaching. When comparing sub-irrigation to traditional overhead irrigation for vegetable transplant production, the Mattson Lab at Cornell University found a substantial reduction in fertilizer application rates could be adopted with sub-irrigation (Liu et al., 2011). The Mattson Lab has also collaborated with researchers at the Long Island Horticulture Research and Extension Center to test controlled release fertilization (CRF) strategies that optimize plant growth while reducing nutrient leaching. This strategy could be particularly useful to conserve fertilizer when operations have inefficient irrigation systems and do not have capital available to implement sub-irrigation systems or sensor-based irrigation. It was found that five to ten times as much nitrate and phosphate leached from liquid fertilizer treated plants than from plants receiving CRF. However liquid fed plants were significantly larger than CRF plants. A combined approach that used liquid fertilizer for the first four weeks and CRF only for the remaining weeks reducing leaching three-fold and produced plants the same size as liquid only. Following these experiments CRF use has been evaluated in greenhouse production of bedding plants and poinsettias. Again, leaching was reduced by more than five-fold compared to liquid-fed only plants. Work that reduces leaching from greenhouses, such as that conducted in New York and Maine, is vital for protecting watersheds throughout the United Sates. In Virginia, this work along with other research has been used to develop new water management recommendations as the EPA approved Watershed Implementation Plan (WIP) for Virginia will require significant upgrades in greenhouse and nursery facilities to retain waste water by 2017. This is a vital project since most of Virginia falls in the Chesapeake Bay watershed. J. Latimer and Virginia Cooperative Extension along with the major greenhouse and nursery associations in the state started working on assessing how the green industry is currently using resources and facilities so that they can document progress toward improved environmental stewardship. Initially, they conducted an online survey augmented with phone interviews to document current fertilizer, irrigation and energy consumption practices. Water use efficiency in CEA can be higher than that of open field based agriculture. Our study with tomato plants grown in an open hydroponics system (no recirculation of used irrigation water) in a greenhouse located in semi-arid climate determined that the water use efficiency (WUE, kg yield per m3 water use) of the irrigation was 30 kg m-3. The study showed that with irrigation and pad and fan cooling system combined total greenhouse WUE was 11 kg m-3. Thus, the study indicated that WUE in greenhouse production can be further improved by using closed (recirculating) hydroponics systems, use of a well-controlled high-pressure fog cooling system by simultaneously reducing evaporative cooling water use and reducing plant water uptake by maintaining lower inside vapor pressure deficit (VPD) levels, and with improved climate control strategy applications (Sabeh et al., 2011). A recent culmination of our groups work was the publication of a nine article Water Wisely series printed in Greenhouse Grower magazine in 2012. The series was a collaborative effort sharing group expertise in selecting and implementing irrigation systems, practical strategies to reduce water and fertilizer resources, and including many research results from the last five years. Alternative Energy Sources and Energy Conservation Past and current work includes the development of energy audit materials (e.g., questionnaires, calculation spreadsheets) that were used to conduct audits at various greenhouse operations. Thomas Manning (PE, Rutgers University) completed training to become a Certified Energy Manager. Several fact sheets were developed to explain energy bills (Manning et al., 2010) and to provide an overview of recommended energy strategies for greenhouse operations (Runkle and Both, 2011). Presentations were made to a variety of audiences including many farm and greenhouse operators. In addition, work was performed on the development of a decision support system for a 250 kW landfill gas-fired microturbine at the 1-acre New Jersey EcoComplex Research and Demonstration Greenhouse (Both et al., 2011). Electricity generated from the landfill gas (previously considered a waste product and flared off in order to significantly reduce the emissions of methane gas) can be used on-site to operate a supplemental lighting system, exported to the local grid for additional income, or a combination of the two. Research in greenhouses was conducted to investigate alternative energy management strategies (Both et al., 2007b; Both et al., 2005) as well as the impact of energy conservation systems on crop growth and development (Blanchard et al., 2012). Work was performed on low-tech high tunnel production systems with the aim of extending the growing season for tomato production using manually deployed energy curtains at night (Both et al., 2007a; Reiss et al., 2004). Finally, an AZ study has been underway and focused on integrating solar photovoltaics into a lower cost greenhouse system for meeting the power and food production demands, and evaluated the system dynamics of an off-the-grid greenhouse (OGGH) in collaboration with and support by five industry partners. It was then compared to a grid-connected greenhouse (GCGH) system. The research has focused on documenting and analyzing all the resource inputs required for crop production (water, fertilizer, energy, labor) and resource outputs obtained from the integrated production system (energy produced, yield) to help in the evaluation of the system's capabilities and limitations. Sensors and Control Strategies For every new growing substrate, there is a need to accurately measure moisture content in the root zone and develop proper irrigation strategies. AZ worked on physical characterization of a new substrate (foam glass, a highly porous substrate) for automated irrigation management using microtensiometers (MT) to trigger irrigation cycles. Research involved the physical characterization of foam glass with particle sizes of less than 1.25 cm and the development of a MT. The developed sensor showed promise for its accuracy and use in automated irrigation applications with the new substrate (Lopez et al., 2008). NE worked on capillary mat system (CapMat, Phytotronics, Inc, Earth City, MO USA) to water and fertigate strawberry in a University of Nebraska campus Quonset greenhouse (Paparozzi et al., 2010, 2011). Plants were grown for 4 winter seasons (2010-2013) on separate 6 ft. by 60 ft. benches with improved CapMat designs. Six-inch pots were interfaced with the CapMat fabric, along with white top/black bottom polyethylene as a reflective mulch and vapor barrier on top of the mat and black polyethylene on the bottom. CapMats were wetted using twin wall tubing installed under the top white/black polyethylene cover. A special jig device was used to cut holes in the plastic for individual pot capillary access the mat. Flag pots of strawberries were monitored with capacitance moisture sensors (Decagon Devices, Inc. Pullman, WA), calibrated to the potting mix formula, and installed at three zone locations (north, middle, and south) over the sixty ft. length of each bench. A timer controlled the fertigation cycles of the CapMats. Daily watering was adjusted according to crop and environmental conditions to minimize night time humidity and plant disease and pest pressure. An electronic meter was used to measure greenhouse water use. The pots were adequately watered at the three zones (50-60% volumetric water content). An electronic gas meter was also used to monitor natural gas use. Furnace and ventilation events controlled by a Groton II system (ACME Engineering and Manufacturing, inc., Muskogee, OK) were also monitored using electronic split core, current sensors and LabVIEW programming. Stomatal resistance has been simulated (Meyer, et al., 2009), as a LabVIEW PID control variable, for the strawberries grown in NE Research Greenhouse under the CapMat fertigation system. An adaptive, real-time dynamic energy model (VI) based on the First and Second Laws of Thermodynamics and psychrometrics accounted for canopy entropy production, net radiation, sensible and latent heat exchanges for the strawberries. Measured canopy TLeaf and air temperatures Tair, relative humidity Æair, and radiation data were transmitted wirelessly from three bench zone locations to a host computer, using sensors and National Instruments LabVIEW® software. Stomatal resistance (rLeaf - s/m) was simulated as a LabVIEW Proportional Integral Differential (PID) controller (subVI) in order to balance net energy for the canopy. Latent results compared favorably from draw-down pot moisture contents, measured with the capacitance sensors. The CapMat® system provided an effective and efficient water and fertilization system for potted plant crops (Paparozzi and Meyer, 2012). Three hundred plants used less than 60 gallons of water during an eight-month period. Strawberry water use can be estimated by using a single pot energy balance calculation (simulating canopy resistance control) in real-time. Growers typically monitor crops manually to assess growth and development, disease and insect issues. However, machine vision is an excellent tool to monitor plant growth and development automatically. Individual leaves can be extracted from high definition web cam images (Camargo Neto, et al., 2006, Meyer et al., 2008, and Meyer, 2011) and analyzed for morphological development, leaf size, insect infestations, moisture condensation, and disease potential, as well as greenness. The possibility of complete control of both demand and supply conditions for crop production requires continuous and real-time sensing and understanding of plant and microclimate interactions. Ultimately, this can lead to resource-conserving climate control and management in greenhouse systems. Kacira and Ling (2001) have demonstrated early, pre-visual plant drought stress detection potential using machine vision technology. Today, advances in the field of micro-precision, sensors, and hardware technology allow lower cost and continuous monitoring of dynamic plant responses as an indicator of crop status and needs. Thus, using plants as sensors, integrating crop response based climate monitoring and control strategies, along with web based decision making and grower support platforms, we can significantly improve resource use efficiency, production quality, and environmentally friendliness of crop production in controlled environment agriculture systems. In AZ, the Kacira Lab designed and developed a machine vision guided system using morphological, textural and temporal plant features for the automated non-contact monitoring of plant health and growth. This was used to evaluate the capability for early detection of tip burn associated with calcium deficiency in greenhouse grown lettuce crop. The plant features extracted to determine overall plant growth and health status included Top Projected Canopy Area (TPCA) as a morphology feature; Red-Green-Blue (RGB), Hue-Saturation-Luminance (HSL) values as color features; entropy, energy, contrast, and homogeneity as textural features. The developed methodology was capable of identifying the calcium deficient lettuce plants one day prior to the visual stress detection by human vision (Story et al., 2010). In OH, Lings team has developed a novel gas exchange monitoring system for rapid assessment of plant responses to their environments. The team also designed plant welling monitoring systems using various sensors including soil moisture probe, infrared thermometer, temperature and relative humidity sensors, and load cells. Future efforts will focus on a multi-sensor based plant monitoring platform to improve sensing, data mining, and diagnostics capability of the existing system and its integration to a web based decision support platform for improved climate, production management, and optimized resource use efficiency. AZ developed a new control strategy for a naturally vented greenhouse equipped with variable high pressure fogging system. The strategy controlled the amount of fog introduced into the greenhouse, as well as the percentage of vent openings to maintain desired values of vapor pressure deficit (VPD) and enthalpy, respectively, which would consequently affect air temperature. The performance was compared to constant fogging rate strategy, which was based on VPD. On average, the new strategy saved 36% water and consumed 30% less electric energy. Smaller air temperature and relative humidity fluctuations, and more consistent control, were achieved by varying the fog system operating pressure to provide a more optimum amount of fog. It was demonstrated by simulations that dynamically varying the fog rate and properly selecting the number of nozzles, savings of water and electric energy were increased, while still maintaining acceptable VPD and temperature (Villarreal-Guerrero et al., 2012a, 2012b, 2013). AZ developed and validated a 3D computational fluid dynamics model comparing simulation results with experimental data obtained from fog cooling research. The comprehensive CFD model included a turbulence model, solar radiation model, crop evapotranspiration model (with a user defined function), and a discrete phase change model. By incorporating the crop existence in the CFD models, not only was the greenhouse environment characterized more realistically, but also, the physics-based physiological processes of the plant (photosynthesis, transpiration) were also modeled (Tamimi et al., 2012). By environmental manipulation, it may be possible to increase the production and/or quality of compounds important for the nutritional value of specific plants. An example is lycopene in tomato. It has been reported that light quality and intensity (Alba et al., 2000), air temperature (Krumbein et al., 2006), nutrient concentration (Fanasca et al., 2006), and salinity level of nutrient concentration (Krauss et al., 2006) affected lycopene concentration in fruit. This information can be used to select set points for greenhouse environment controls to optimize lycopene concentration. Leafy greens are a crop that is especially susceptible to manipulation of composition through environmental control. The concentration of nitrate and sugar in greenhouse-grown plants is affected by seasonal changes in light and temperature (Drews et al 1995, Burns et al 2011), and by nutrient solution composition (Gent, 2003). Production of leafy greens in greenhouses has been increasing rapidly in the northeast USA, due to a year-round demand for locally grown and organic produce. Locally grown and distant (supermarket) greens differ in composition (Gent, 2011). A better understanding of how greenhouse environment affects the nutritional value of leafy greens will improve the health of local consumers, and increase the desirability of leafy greens produced in greenhouses. CT studied use of shade to optimize production of greenhouse tomato. Total yield decreased linearly with increasing shade, but the market fraction increased, so there was no difference in yield of marketable fruit in any of the three years in this study (Gent, 2007). Cracked skin was the defect most affected by shade. Whereas the effect of shade on water was nearly instantaneous the effects on nutrient use and rate of fruit production were delayed by up to 6-weeks after application of shade (Gent, 2008). Shade had little or no effect on elemental composition of the fruit, but it increased elemental composition of leaves, and decreased sugars and starch (Gent, 2008). Furthermore, CT examined the effect of time of day, and nitrogen supply on composition of hydroponic lettuce. There were diurnal variations in sugars and nitrate, with a maximum of sugars and a minimum of nitrate in the afternoon (Gent, 2012a). However, the loss of water or increase in dry matter ratio resulted in higher yield of plants harvested early in the morning. Plant size and time of year were also important factors in determine tissue composition of lettuce shoots (Gent, 2012a). Withdrawal of nitrate from the nutrient solution resulted in rapid depletion of nitrate in the plant (Gent, 2012b). The effect on composition of various metabolites was reversible, if the nitrate was re-introduced within 6 days. However, the time course of changes in metabolites was different in the withdrawal and recovery periods (Gent, 2012b). AZ evaluated using UV-A, blue, green, red, and far-red light-emitting diodes (LEDs) and their effects of different supplemental light qualities on phytochemicals and growth of Red Cross baby leaf lettuce (Lactuca sativa L.) grown at a high planting density under white fluorescent lamps. Anthocyanins concentration increased with supplemental UV-A and blue respectively, carotenoids concentration increased with supplemental blue, phenolics concentration increased with supplemental red while supplemental far red decreased anthocyanins, carotenoids and chlorophyll concentration, compared to those under in the white light control. Fresh weight, dry weight, stem length, leaf length and leaf width significantly increased with supplemental far red light compare to white light, presumably due to enhanced light interception by enlarged leaf area under supplemental far red light. Although the mechanisms of changes in phytochemicals under different supplemental light quality are not well known, the results demonstrated that supplemental light quality could be used to enhance nutritional value and growth of baby leaf lettuce grown under white light (Li and Kubota, 2009).
Objectives
-
To develop up-to-date water and nutrient as well as energy management guidelines for greenhouse crop production and provide stakeholders with educational opportunities that teach proper implementation at their own facilities.
-
To develop these guidelines using research and development involving sensors and control strategies devised by current team members, and through and future collaborations among team members who may become part of this research project.
Methods
1. Develop and test irrigation and fertilization practices to improve water and fertilizer use efficiency in greenhouse production As noted above our group members study several approaches to reduce water and fertilizer consumption in both overhead- and sub-irrigation systems. We plan a three-step approach to integrate and compile the multi-state information gained from these different systems. We will develop comprehensive metrics to compare these systems to each other and to develop outreach materials with guidelines for commercial greenhouse operations to implement these water and fertilizer saving techniques. Our approach involves A) continued research to further refine the irrigation/fertilization systems; B) testing the irrigation systems in parallel utilizing the same set of greenhouse crops and cultural conditions to develop metrics on water and fertilizer efficiency, crop quality, and capital costs of implementation; C) develop outreach materials so commercial greenhouse operations can effectively implement these systems to save water and fertilizer resources. The approach is described in more detail below. 1A. Conduct research to further refine irrigation/fertilization systems Our group members have identified various areas as high priority needs that must be addressed relative the irrigation/fertilization systems, prior to testing these systems to determine their efficiency metrics. One advantage of our combined research group is that individual members have access to a variety of sustainable irrigation and fertilization systems at their station greenhouses. Through collaboration, our group is able to compare results from various irrigation systems and make recommendations to growers regarding the variety of systems they would be likely to purchase or have on-site. The over-arching goal is to be able to help greenhouse growers save water and reduce run-off during production. The irrigation systems we plan to refine and research include sensor-automated drip irrigation (ME), sensor automated fog systems for propagation (ME), sub-irrigation (CT, NY), and fog cooling (AZ). ME will develop species-specific water requirements using the sensor automated drip irrigation system. For this research, we will grow ornamental plants at volumetric water contents (volume of water ÷ volume of soil) ranging from 0.05 to 0.40 L·L-1. We will also work to improve our existing model for predicting whole plant water use based on crop growth and environmental conditions. ME has built a system for automating fog systems for propagating cuttings using sensors. In the past, all propagation systems were set to a timer, and mist or fog were not initiated based on the physiological needs of the cuttings. ME will evaluate this system and determine if it may be used to improve rooting percentage and/or reduce water use. As part of this approach, we plan to upgrade our prototype system, which utilizes EC-5 sensors, to a propagation system that uses LWS leaf wetness sensors (Decagon Devices, Pullman, WA) to estimate leaf wetness based on the dielectric properties of a simulated leaf. The potential advantages of this system are: 1) It enables propagators to reduce water usage on mist or fog benches, and 2) It increases the quality of cuttings due to the greater control over the degree of leaf wetness. CT-New Haven and CT-Storrs will continue to work with sub-irrigation. Their work will characterize the effects of salinity in raw source water on crop response and salt accumulation in the root medium under partial v. full-saturation sub-irrigation regimes. To allow for a greater amount of recirculation, water sources with low salinity and low concentrations of ions are favored for recirculating nutrient solutions. While NaCl builds up in nutrient solutions and may result in decreased yield, nutrients cations such as K, Ca, and Mg will be depleted by plant-uptake and need to be added to the solution. Hence, balancing NaCl and cation levels in a nutrient solution is important for plant nutrition and growth. Several economically important crops such as (Poinsettia, tomato, petunia, and geranium) will be grown under a variety of irrigation regimes, each with or without the addition of NaCl to simulate reduced quality of source water. Irrigation regimes will include sub-irrigation using partial-saturation on large scale flooded floors, or drip irrigation. The size of these plots will allow cultivar trials to be conducted under all irrigation regimes, to look for germplasm that is resistant to salt accumulation in the medium. Building off of this information, NY will collaborate with CT to test the effectiveness of silicon (Si) supplementation to the fertilizer to mitigate high salts in these species. Cultivar specific responses, disease incidence and nutrient accumulation in both plant tissue and potting medium will be determined. NY will work with CT to develop guidelines for managing and mitigating NaCl accumulation in sub-irrigation systems utilizing species and cultivar specific salt thresholds, management of fertilizer salts, and use of beneficial substances such as Si to mitigate damage to crops. Although not directly related to irrigation, active cooling systems in greenhouses utilize substantial water as well. This is particularly true in arid climates where water is extremely limiting for agricultural production and society. Thus, as a related topic, AZ will assess and develop management strategies for water use related to evaporative cooling of greenhouse structures. This technique is particularly useful in arid climates, but it is also used to a limited degree in more humid parts of the country. In CT, we have the ability to test real-world applications of irrigation technology, including the interaction of irrigation and disease. This is vital because it allows us to evaluate research under situations encountered by the commercial growers we work with. CT-Storrs will develop strategies for the continuous re-use of irrigation water in a completely closed system. Grower Direct Inc. the largest greenhouse business in CT uses flooded floor irrigation throughout most of the company's production range and is working to develop economically viable strategies for the continuous reuse of irrigation water. Disease load in irrigation water reused on a succeeding crop is a concern. Grower Direct is testing a strategy to pasteurize residual water at the end of each crop cycle by heating the wastewater using the biofuel boiler system. We will monitor water samples before and after pasteurization for nutrient content and disease load. Because most growers utilize fertigation practices in which dissolved fertilizers are delivered in the irrigation water, enhanced fertilizer use efficiency is expected when implementing sub-irrigation and sensor based overhead irrigation systems due to the reduction in water volume applied and water leached out of containers. When operations cannot implement such practices due to the investment costs, other non-traditional approaches may be used to improve efficiency such as controlled release fertilizers (CRF) as well as endo- and ecto- mycorrhizal fungi. NY will test several controlled release fertilizer (CRF) materials with different patterns of nutrient release for their suitability in the production of the most economically important bedding and potted plant species. It is important to match CRF release patterns (such as high release at the beginning, middle, or end of crop production) to specific crop growth patterns to ensure that nutrients are not limiting for optimal plant growth. Beyond plant growth, leachate analysis will be conducted to determine the leaching of nitrogen, phosphorus, and potassium from CRF as compared to conventional water soluble fertilizers (WSF) to demonstrate the feasibility of using CRFs to reduce nutrient leaching to the environment. The use of CRF and WSF in both sub- and overhead- irrigation systems will be considered. CT will examine the effects of a blend of endomycorrhizal and ectomycorrhizal fungi on water and nutrient use efficiency. One way to reduce waste from irrigation is to improve both water use efficiency and nutrient use efficiency. Fungal mycorrhizal associations with plant roots are known to enhance both water and nutrient uptake. The effect of inoculation with mycorrhizal fungi on containerized ornamental crops in conjunction with partial saturation and low fertility levels has not been reported. Nutrient accumulation in poinsettia and other species inoculation with endomycorrhizal and ectomycorrhizal fungi at various dosages will be assessed. During this stage of the project, VA will spearhead our groups efforts to survey current irrigation/fertilization practices utilized by the commercial greenhouse industry and potential grower acceptance of both low and high capital cost methods to improve water and fertilizer resource use. Water samples from greenhouse operations will also be collected to test for water quality factors. Such information can be used to determine the potential for high salt accumulation if sub-irrigation practices are adopted. Further VA will explore opportunities for pathogen transfer during production and handling of produce grown in hydroponic solutions. 1B. Test irrigation systems in parallel utilizing the same set of greenhouse crops and cultural conditions to develop metrics for their use. It is intended that 1A will take about two years to implement. A primary topic at the annual meeting prior to year 3 will be developing an experimental system with four specific crops (petunia, geranium, poinsettia, and tomato) so that project members can test their irrigation systems in parallel to determine metrics on water and fertilizer efficiency, crop quality, and capital costs of implementation. The strategies to be tested include: ME sensor based overhead irrigation, CT full and partial saturation sub-irrigation, NE capillary mat sub-irrigation, and NY overhead hand watering and sub-irrigation. Regarding fertilization both WSF and CRF will be tested at each location. In the planning stage for this step we will collaborate closely with our group members that work in the areas of sensors and controls to make ensure our facilities are adequately equipped to monitor the growth environment and water and fertilizer use so that we can appropriate compare our irrigation strategies using an apples-to-apples comparison. The four crops (petunia, geranium, poinsettia, and tomato) are among the most economically important bedding, potted, and vegetable plants. They will be grown by each of the locations using the systems described above. Water use and fertilizer use efficiency will be calculated on both a per plant basis as well as per g of crop biomass basis. Additional information will be collected such as crop quality indices (plant height and width, leaf chlorophyll content) and incidence of crop disorders (such as plant disease). Implementation costs of the above systems and ongoing water/fertilizer costs will be estimated. 1C. Develop outreach materials for commercial greenhouse operations for effectively implementing these systems to save water and fertilizer resources. A critical component of this section of the project is outreach to convey our findings to commercial greenhouse growers. The metrics obtained from 1B will be integrated into a comprehensive report, which can be used by growing operation to help guide operating and future investment decisions. In order to allow growers to access this easily and inexpensively, this will be published as an e-book incorporating our irrigation and fertilization work into a greenhouse best management practices guide. VA will assess and guide the development and implementation of these best management practices in small to medium sized operations that want to decrease irrigation water, fertilizer, and pesticide use. This will allow us to determine the true impact of our irrigation and fertilization research. As a more short-term goal, the entire group will periodically connect with growers through the joint development of trade-journal articles, similar to our Water Wisely series. These will also be presented as brief online presentations developed in collaboration with the major greenhouse trade magazines. All of these short presentations, trade publications, and our e-book will be developed into a central Water Wisely website for this project. This site will serve as a clearinghouse for growers who wish to quickly find information regarding sustainable irrigation and fertilization, as well as a best management practices guide. 2. Develop guidelines and approaches to improve greenhouse heating system efficiency NJ will collect information about available heating systems and their suitability for greenhouse applications. Where possible, measurements will be conducted at greenhouse operations to determine the performance of novel or improved heating systems and to develop optimized operational strategies. As much as feasible, these measurements will be conducted using the wireless sensor networks to be developed by AZ and NE. 3. Develop guidelines and approaches for the use of alternative energy sources NJ will continue work on a landfill gas fired microturbine system. A decision support system for such a distributed energy generation system is under development and it can be used for other small scale generators even if they use fossil fuels to produce electricity and waste heat (combined heat and power). Opportunities to use the waste heat for cooling purposes (especially during the summer months using absorption chilling) as well as the carbon dioxide (for enrichment of the crop production environment) will be investigated. AZ will continue to work on solar power generation systems, and their strategic integration to greenhouse production systems to meet the energy demands, improve greenhouse internal climate and crop requirements. Opportunities for evaluating various photovoltaic power generation systems, panels, system dynamics, and glazing materials will be studied. 4. Evaluate and develop novel sensors and environmental control strategies NJ will use historic weather data (for a variety of locations throughout the Northeastern US) and published energy calculation strategies, to evaluate and simulate alternate control strategies. Where appropriate and doable, novel strategies will be implemented and tested in research and commercial greenhouse facilities. In addition, simulation studies will be conducted using the computational fluid dynamics models developed by AZ. NE will evaluate leaf and other significant greenhouse boundary temperatures using low-cost infrared thermocouple sensors, along with potting media temperatures. Aerial temperatures will be compared to thermal image temperatures. Furthermore, miniature integrated-circuit moisture sensors will be applied to leaf surfaces for monitoring condensation. NE will determine the performance of a CapMat as a uniform watering/fertigation system, pot water content capacitance and new post multi-sensors (temperature, moisture content, and electrical conductivity) (e.g. Decagon GS3, Pullman, WA). Sensor placement and spatial distribution will be studied to determine the minimum number of sensors needed. NE will test and evaluate low cost CO2 sensors and compare the practicality, accuracy and cost to those more expensive units. In addition, the CO2 management will be investigated with both ventilation and new high efficiency lighting systems to provide adequate moles of light. NE will work on the development of virtual reality, light rendering and understanding the effectiveness of lamp reflectors and mulches for bathing plants as much as possible in light (Meyer, et al. 2011). Canopy architecture is an important aspect of plant growth and development and impacts plant transpiration and water uptake. Non-destructive, three dimensional scanning of greenhouse plants in pots is now possible using a 3D Digital Corp® ESCAN (Sandy Hook, CT) laser scanner and turntable. Scanned data will then pinned and assembled into a virtual reality model which can be further analyzed using heat transfer, plant mass, and optical processes and properties, using COMSOL® (Burlington, MA) multi-physics software. AZ and NE will work on evaluating low cost wireless sensors and data acquisition system to characterize greenhouse climate uniformity, accuracy of sensors in greenhouse settings, required numbers and distribution of wireless sensors deployment in a given greenhouse domain. They will also investigate low cost and practical climate monitoring systems for small farmers. AZ will continue to work on multi-sensor based crop diagnostics platform using canopy temperature, spectral and morphological features to determine crop health and growth status and use the crop responses in greenhouse climate control. The developed system will be integrated into a web based decision support platform for remote access and system control. AZ will continue to use computational fluid dynamics (CFD) approach to evaluate greenhouse climate uniformity under various greenhouse glazing materials such as diffuse, near infrared blocking for low and high tech greenhouses. Our modeling effort will also include greenhouse climate analysis for greenhouses located in hot and humid climates. Furthermore, the CFD and climate modeling studies will evaluate the greenhouse climate and designs for low cost high tunnel type of structures to come up with improved designs, climate control strategies for manufacturers and user of that type of greenhouse systems. OH will continue to work on the models they developed to assess greenhouse cooling and these models will be extended for specific locations by including greenhouse specific characteristics. OH will also revise the models and use them for estimating the amount of energy greenhouses can recycle by using exhaust heat from cooling operations for future heating needs. OH will continue their effort to develop a rapid whole canopy gas exchange measurement system. The system has demonstrated its capability for quantifying transient as well as steady state plant responses to their environmental stimuli. Further evaluation, validation, and refinement of the system is planned for whole plant phenotyping applications. NJ will evaluate two production inputs, plant containers and irrigation/fertigation, to assess the economic and environmental impacts of sustainable production practices in nursery and greenhouse operations. Use of Greenprocesses based upon quantitative data will result in improved farm incomes while sustaining environmental quality by reducing the carbon, water, and chemical foot-prints of production systems. 5. Outreach activities (presentation, publications, demonstrations) to stakeholders All participants: Existing resources will be collected and Extension publications and outreach programs on water and fertilizer management will be conducted for greenhouse operators and Extension personnel. Growers will be educated on local watershed implementation plan requirements and deadlines. Implementation will be encouraged through education and discussions that detail the economics of irrigation and fertilizer efficiency, as well as their potential as effective marketing tools towards consumers, neighbors and community relations. Results will be reported in trade journal articles, and peer reviewed publications, as well as through outreach presentations. Where appropriate, Extension Fact Sheets will be developed.Measurement of Progress and Results
Outputs
- We will develop enhanced irrigation protocols for geranium, petunia, poinsettia, and tomato that reduce water and nutrient use compared to traditional growing systems.
- We will develop a low-cost (possibly wireless) sensor system for crop monitoring and climate control that will lead to improved resource management for small to medium-sized greenhouse operations.
- We will develop and test a propagation system that utilizes leaf wetness sensors for environment control using Geranium as a model crop.
- We will characterize the response and resulting recommended management practices of petunia, geranium, and poinsettia to elevated salinity levels under various sub-irrigation and drip irrigation regimes.
- We will characterize changes in water and nutrient use efficiency of petunia, geranium, and poinsettia when inoculated with endomycorrhizal and ectomycorrhizal fungi.
- We will document the water and nutrient use efficiency and resulting recommended management practices for using sub-irrigation (full and partial saturation) and overhead-irrigation (hand water or sensor based zero leach systems) for the production of petunia, geranium, poinsettia, and tomato.
- We will develop novel operational and control strategies that aim to reduce the energy consumption of greenhouse heating systems, including co-generation installations.
- We will develop comprehensive CFD models which will analyze complex greenhouse aerodynamics problems and provide improved design considerations for greenhouse structures and climate control system manufacturers and providers.
- We will evaluate potentials and techno-economic feasibility of off-grid greenhouse system and document its resource inputs and system outputs, limitation and capabilities.
- We will develop enhanced climate control strategies, including real-time monitoring of both greenhouse climate and crop canopy leading to improved water and energy use.
- We will produce at least three Extension publications and a webpage devoted to improved irrigation, fertilizer, and energy management for small to medium sized operations. The webpage will include budget tools for calculating potential impacts of changes in production practices and energy sources.
- We will document the reduced expenses and benefits of plant containers and irrigation/fertigation that are more green and environmentally sustainable than current practices. Reducing the carbon footprint on the environment is a priority for the long-term sustainability of the industry.
Outcomes or Projected Impacts
- Sensing techniques and control algorithms integrated with zero- or low- leach irrigation systems and enhanced climate control strategies generated from this project will enable producers of greenhouse crops to reduce their water and fertilizer use by 10-20%.
- Information gathered through this project and results shared with the greenhouse community will enable commercial greenhouse growers to reduce their energy consumption by 10-30%, resulting in significant savings.
- The development of the proposed guidelines for water, nutrients and energy use in greenhouses can become part of an water and nutrient management e-book for controlled environment crop production that can help both the greenhouse industry as well as the regulatory agencies to improve understanding and oversight of enhanced crop production practices. Such overall guidelines will help growers stay competitive.
- Information gathered and shared through this project will empower growers to improve production practices through the use of (remote) monitoring and control of the greenhouse environment and plant status.
- The realtime and web based greenhouse and climate control platforms developed will help growers to reduce 5-10% of their labor use dedicated to greenhouse climate monitoring, recording, and management
Milestones
(2014): Develop irrigation protocols for geranium, petunia, poinsettia, and tomato using partial and full saturation sub-irrigation and sensor-automated irrigation systems.(2015): Develop sensor-based propagation system that will save water. Determine the effects of elevated salinity on our four crops grown in sub-irrigation. Develop nutrient management recommendations for four crops grown in partial and full saturation sub-irrigation, drip irrigation, hand watering, and sensor automated irrigation.
(2016): Develop wireless crop monitoring system for small to medium sized growers. Develop control strategies for energy reduction in greenhouse heating.
(2017): Evaluate the inputs and outputs and feasibility of off-grid greenhouses. Provide growers with real-time monitoring tools for greenhouses that will lead to improved water and energy use. Publish at least three Extension publications on reducing water and energy use in greenhouses. Document reduced expenses and benefits of 'green' and environmentally sustainable greenhouse practices.
(2018): Provide recommendations to greenhouse climate control manufacturers on improved design considerations based on CFD models. Publish a webpage devoted to improved irrigation, fertilizer, and energy management for small to medium sized growers.
Projected Participation
View Appendix E: ParticipationOutreach Plan
Our team works closely with, and has many ties with the greenhouse industry throughout the United States. Several team members have (partial) Extension appointments, providing for additional connections with the industry. We plan to share the results of our research through a variety of methods to reach both our peers at research institutions, as well as greenhouse growers and Extension personnel. Results will be published in refereed articles and Extension publications. Our team recently collaborated on a series of Extension publications that was published in Greenhouse Grower Magazine (a well-known trade journal) on sustainable irrigation practices. We will expand on this effort by developing a Water Wisely e-book and webpage to serve as a clearinghouse for the information we develop on conserving water and fertilizer. In addition, we plan to publish a series of Extension publications on energy savings, ventilation and cooling, and alternative energy use. Over the years, members of this project have provided valuable greenhouse engineering technology to the industry throughout our history through technology transfer. Some examples include the use of air-inflated double-layer polyethylene films as greenhouses cover material, advances in hydroponic production systems and supplemental lighting, floor heating for greenhouses, and the use of energy curtains. Many of the technologies originally developed by members of this project are now industry standards for improving sustainability and/or conserving energy. Several key industry members received part of their education at the institutions (and in some cases were instructed by members of our team) involved in this project. Our group is uniquely qualified to jointly develop strategies to address resource management in commercial greenhouse production as our membership contains a complement of both plant scientists and greenhouse engineers as well as an agricultural economist (NJ). This breadth of expertise allows our group to approach commercial greenhouse production from a truly multi-disciplinary perspective and it provides valuable information and practical solutions to greenhouse growers.
Organization/Governance
The technical committee (and its predecessors) has organized itself by annually appointing an incoming secretary, who then serves as the secretary for the following year (including the next annual meeting). After completing a term (one year) as secretary, the individual served as the committee chair the following year. We do not have the position of vice chair (chair elect). Therefore, officers served for two-year terms. This limited the time commitment requested from incoming officers and since the committee was small and informal, sufficient institutional memory could be tapped in case procedural questions came up. This organizational model has worked well for many years, and we plan to continue with it if our proposal is approved for continued funding. Annual meetings were organized on a rotating basis after the membership was polled for availability and interest. The model has work well also, and we plan to continue it as well. Many of the participating members meet each other at other scientific meetings throughout the year, ensuring sufficient opportunity for interaction in addition to the annual project meetings.
Literature Cited
Ackerman F. and E. A. Stanton. 2011. The Last Drop: Climate Change and the Southwest Water Crisis. Stockholm Environment Institute US 11 Curtis Avenue Somerville, MA. Alba R., M.M. Cordonnier-Pratt, and L.H. Pratt. 2000. Fruit-localized phytochromes regulate lycopene accumulation independently of ethylene production in tomato. Plant Physiol. 123: 363-370 Bilderback, T.E. 2002. Water management is key in reducing nutrient runoff from container nurseries. HortTechnology 12: 541-544. Blanchard, M.G., E.S. Runkle, A.J. Both, and H. Shimizu. 2012. Greenhouse energy curtains influence shoot-tip temperature of new guinea impatiens. HortScience 47:483-488. Both, A.J., T.O. Manning, A. Martin, D.R. Specca, and E. Reiss. 2011. Operating a 250 kW landfill gas fired microturbine at a 0.4 hectare research and demonstration greenhouse. Acta Horticulturae. 893:397-404. Both, A.J. and D.R. Mears. 2008. Building and maintaining greenhouses for energy savings. In Horticulture: Principles and Practices, 4th ed. by G. Acquaah; included in Chapter 12 Controlled-Environment Horticulture. Prentice Hall, Inc. Upper Saddle River, NJ. pp. 406-417. Both, A.J., E. Reiss, J.F. Sudal, K.E. Holmstrom, C.A. Wyenandt, W.L. Kline, and S.A. Garrison. 2007a. Evaluation of a manual energy curtain for tomato production in high tunnels. HortTechnology 17(4):467-472. Both, A.J., D.R. Mears, T.O. Manning, E. Reiss, P.P. Ling. 2007b. Evaluating energy savings strategies using heat pumps and energy storage for greenhouses. ASABE paper No. 07-4011. ASABE, 2950 Niles Road, St. Joseph, MI 49085-9659, USA. 16 pp. Both, A.J., E. Reiss, D.R. Mears, and W. Fang. 2005. Designing environmental control for greenhouses: Orchid production as example. Acta Horticulturae 691(2):807-813. Brumfield, R.G., A.J. Both, and G. Wulster. 2009. How are greenhouse growers coping with rising energy costs? Southern Nursery Association Research Conference Proceedings. Georgia World Congress Center, Atlanta, GA. February 12-13, 2009. pp. 304-307. Available at: http://www.sna.org/content/Economics and marketing 2009_1.pdf Burnett, S.E. and M.W. van Iersel. 2008. Morphology and irrigation efficiency of Gaura lindhiemeri grown with capacitance sensor-controlled irrigation. HortScience 43:1555-1560. Burns, I.G., K. Zhang, M.K. Turner, M. Meacham, K. Al-Redhiman, J. Lynn, M.R. Broadley, J. Camargo Neto, G.E. Meyer, and D.D. Jones. 2006. Individual leaf extractions from young canopy images using Gustafson-Kessel clustering and a genetic algorithm. Computers and Electronics in Agriculture 51:65-85. Drews M., I. Schonhof, and A. Krumbein. 1995. Influence of growth season on the content of nitrate, vitamin C, b-carotene and sugars in head lettuce under greenhouse conditions [German]. Gartenbauwissenschaft 60:180-187. Elmer, W.H., M.P.N. Gent, and R.J.McAvoy. 2012. Partial saturation under ebb and flow irrigation suppresses Pythium root rot of ornamentals. Crop Protection 33:29-33. Fanasca S., G. Colla, Y. Rouphael, F. Saccardo, G. Maiani, E. Venneria, and E. Azzini. 2006. Evolution of nutrient value of two tomato genotypes grown in soilless culture as affected by macrocation proportions. HortScience 41:1584-1588. Garland, K., S.E. Burnett, M. Day, and M.W. van Iersel. 2012. Influence of substrate water content and daily light integral on photosynthesis, water use efficiency, and morphology of Heuchera americana. J. Amer. Soc. Hort. Sci. 137:57-67. Gent M.P.N, W.H. Elmer, and R.J. McAvoy. 2011. Rapid watering to achieve partial saturation of root medium on flooded floors. Acta Horticulturae 893:1065-1072. Gent M.P.N., W.H. Elmer, and R.J. McAvoy. 2012. Water use efficiency with rapid watering of potted plants on flooded floors. Acta Horticulturae. 927: 101-108. Gent M.P.N, and R.J. McAvoy. 2011. Water and nutrient uptake and use efficiency with partial saturation ebb and flow watering. HortScience 46(5):791798. 2011. Gent, M.P.N. 2003. Solution electrical conductivity and ratio of nitrate to other nutrients affect accumulation of nitrate in hydroponic lettuce. HortScience 38:222-227. Gent, M.P.N. 2007. Effect of degree and duration of shade on quality of greenhouse tomato. HortScience 42:514-520. Gent, M.P.N. 2008. Density and duration of shade affect water and nutrient use in greenhouse tomato. J. Amer. Soc. Hort. Sci. 133:619-627. Gent, M.P.N. 2011. Composition of salad greens: A comparison of locally-grown and supermarket produce. Connecticut Agric. Experiment Station Bulletin 1032. 5 pp. http://www.ct.gov/caes/lib/caes/documents/publications/bulletins/b1032.pdf Gent, M.P.N. 2012a. Composition of hydroponic lettuce: Effect of time of day, plant size, and season. J. Sci. Food. Agric. 92:542-550. Gent, M.P.N. 2012b. Rate of change of composition of lettuce in response to nitrogen depletion or resupply. J. Sci. Food. Agric. 92:3007-3015. Hand P, Paul M, Pink D. 2011. Screening for genotype and environment effects on nitrate accumulation in 24 species of young lettuce. J. Sci. Food. Agric. 91:553-562. International Governmental Panel on Climate Change (IPCC). 2008. Climate change and water. 9 May 2013. http://www.ipcc.ch/pdf/technical-papers/climate-change-water-en.pdf Kacira, M., and P. Ling. 2001. Design and development of an automated and non-contact sensing system for continuous monitoring of plant health and growth. Trans. Am. Soc. Agr. Eng. (44):989-996. Kacira, M., P. P. Ling, T. H. Short. 2002a. Establishing crop water stress index (CWSI) threshold values for early and non-contact detection of plant water stress. Transactions of ASAE, 45(3): 775-780. Kacira, M., P. P. Ling and T. H. Short. 2002b. Machine vision extracted plant movement for early detection of plant water stress. Transactions of the ASABE, 45(4): 1147-1153. Kim, J., M.W. van Iersel, and S.E. Burnett. 2011. Estimating daily water use of two petunia cultivars based on plant and environmental factors. HortScience 46:1287-1293. Krauss S, W.H. Schnitzler, J. Grassmann, and M. Woitke. 2006. The influence of different electrical conductivity values in a simplified recirculating soilless system on inner and outer fruit quality characteristics of tomato. J. Agric. Food Chem. 54:441-448. Krumbein, A., D. Schwarz, and H.P. Kläring. 2006. Effects of environmental factors on carotenoid content in tomato (Lycopersicon esculentum Mill.) grown in a greenhouse. J. Appl. Bot. Food Quality 80:160-164. Li, Q. and C. Kubota. 2009. Effects of supplemental light quality on growth and phytochemicals of baby leaf lettuce. Environ. Experiment. Botany. 67:59-64. Li, H., L. Zhao, P. Ling, and J. Liu. 2012. A model for predicting wireless signal transmission performance of ZigBee-based sensor networks in residential houses. Transactions of ASHRAE. 118(1): 994-1007. Lopez, J.C., P. Waller, G. Giacomelli, M. Tuller. 2008. Physical characterization of greenhouse substrates for automated irrigation management. Acta Horticulturae 797:333-338. Manning, T., A.J. Both, and J. Rabin. 2010. Understanding on-farm utility costs and billing, Rutgers Cooperative Extension Fact Sheet FS1128. Liu, J., W.R. Leatherwood and N.S. Mattson. 2012. Irrigation method and fertilizer concentration differentially alter growth of vegetable transplants. HortTechnology 22:56-63. Mears, D.R., A.J. Both, L. Okushima, S. Sase, M. Ishii, and H. Moriyama. 2009. Some alternatives to burning fuels for greenhouse heating (in Japanese). Journal of Agricultural Meteorology 65(3):303-308. Meyer, G.E. and J. Camargo Neto, 2008. Verification of color vegetation indices for automated crop imaging applications. Computers and Electronics in Agriculture 63:282-293. Meyer, G.E., D. Mabie, F.J. Hay, S. Adams, T. Bartels, and J.B. Fitzgerald. 2009. Improved instrumentation and controls for biomass heating and impact on greenhouse profitability. ASABE Paper Number 09-096884. The American Society of Agricultural and Biological Engineering, St Joseph MI. Meyer, G.E., E.T. Paparozzi, E.A. Walter-Shea, E.E. Blankenship, and S.A. Adams, 2011. An investigation of reflective mulches for use over capillary mat systems for winter-time greenhouse strawberry production. Engineering in Agriculture 28(2):271-279. Meyer, G.E., 2011. Machine vision identification of plants. In D. Krezhova (Ed.), Recent Trends for Enhancing the Diversity and Quality of Soybean Products (ISBN: 978-953-307-533-4). InTech, Rijeka, Croatia, pp 401-420. Nemali, K.S. and M.W. van Iersel. 2006. An automated system for controlling drought stress and irrigation in potted plants. Scientia Hort. 110:292-297. Olaimat, A. N. and R. A. Holley. 2012. Factors influencing the microbial safety of fresh produce: A review. Food Microbiology 32(1):1-19. Paparozzi, E. T., Adams, S. A., Meyer, G., Conley, M. E., Schlegel, V., Blankenship, E. and P. E. Read. 2010. Selecting strawberry cultivars for winter greenhouse production. HortScience 45(8):S230 (Abstr.). Paparozzi, E.T., G.E. Meyer, M. E. Conley, S.A. Adams, E.E. Blankenship, P.E. Read and V.L. Schlegel. 2011. Cultivar choice affects quality and production of winter-grown strawberries. HortScience 46(9): S112 (Abstr.). Paparozzi, E.T., G.E.Meyer, 2012. The return of capillary mats. Greenhouse Grower, August: 78-84. Reiss, E., D.R. Mears, T.O. Manning, G.J. Wulster, and A.J. Both. 2007. Numerical modeling of greenhouse floor heating. Transactions of the ASABE 50(1):275-284. Reiss, E., A.J. Both, S. Garrison, W. Kline, and J. Sudal. 2004. Season extension for tomato production using high tunnels. Acta Horticulturae 659:153-160. Rose, M.A. and J.W. White. 1994. Nitrogen rate and timing of nitrogen application in poinsettia (Euphorbia pulcherrima Willd. Ex Klotz.). HortScience 29:1309-1313. Runkle, E. and A.J. Both. 2011. Greenhouse energy conservation strategies. MSU Extension Bulletin E-3160. Sabeh, N., G. A. Giacomelli, and C. Kubota. 2011. Water use in a greenhouse in a semi-arid climate. Transactions of ASABE, 54(3): 1069-1077. Santos, K.M., P.R. Fisher, and W.R. Argo. 2008. A survey of water and fertilizer management during cutting propagation. HortTechnology 18:597-604. Story, D., M. Kacira, C. Kubota, A. Akoglu, and L. An. 2010. Lettuce calcium deficiency detection with machine vision computed plant features in controlled environments. Computers and Electronics in Agriculture 74(2):238-243. Striemer, G.M., D. L. Story, A. Akoglu and M. Kacira. 2011. A Node and Network Level Self-Recovering Distributed Wireless Sensor Architecture for Real-Time Crop Monitoring in Greenhouses. Transactions of ASABE, 54(4): 1521-1527. Tamimi, E., M. Kacira, C. Choi, and L. An. 2012. Analysis of microclimate uniformity in a naturally vented greenhouse with high pressure fogging system. Transactions of ASABE (In Review). United States Department of Agriculture, National Agriculture Statistics Service. 2010. Census of Horticultural Specialties, Volume 3 AC-07-SS-3. 595 pp. United States Department of Agriculture, National Agriculture Statistics Service. 2007. Census of Agriculture, United States Summary and State Data, Volume 1 AC-07-A-51, 739 pp. van Iersel, M.W., S. Dove, J.G. Kang, and S.E. Burnett. 2010. Growth and water use of petunia as affected by substrate water content and daily light integral. HortScience 45:277-282. Villarreal-Guerrero, F., M. Kacira, E. Fitz-Rodríguez, C. Kubota, G.A. Giacomelli, R. Linker, and A. Arbel. 2012a. Comparison of three evapotranspiration models for a greenhouse cooling strategy with natural ventilation and variable high pressure fogging. Scientia Horticulturae 134:210-221. Villarreal-Guerrero, F., M. Kacira, E. Fitz-Rodríguez, R. Linker, C. Kubota, G.A. Giacomelli, and A. Arbel. 2012b. Simulated performance of a greenhouse cooling control strategy with natural ventilation and fog cooling. Biosystems Engineering 111:217-228. Villarreal-Guerrero, F., M. Kacira, E. Fitz-Rodríguez, R. Linker, G.A. Giacomelli, A. Arbel, and C. Kubota. 2013. Implementation of a greenhouse cooling strategy with natural ventilation and variable fogging rates. Transactions of ASABE 56(1):295-304. Virginia Department of Conservation and Recreation: Chesapeake Bay TMDL http://www.dcr.virginia.gov/vabaytmdl/index.shtml