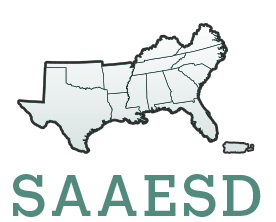
S1030: Flies Impacting Livestock, Poultry and Food Safety
(Multistate Research Project)
Status: Inactive/Terminating
S1030: Flies Impacting Livestock, Poultry and Food Safety
Duration: 10/01/2007 to 09/30/2012
Administrative Advisor(s):
NIFA Reps:
Non-Technical Summary
Statement of Issues and Justification
Muscoid flies are among the most important pests in livestock and poultry production systems. Two species in particular, house fly (Musca domestica) and stable fly (Stomoxys calcitrans), are responsible for damage and control costs in excess of a billion dollars per year in the United States. In addition to the direct damage these flies inflict upon livestock, their presence as a byproduct of confined livestock and poultry operations has been repeatedly cited as a common nuisance, especially when the flies enter the vicinity of human habitations and urban environments. Law suits, zoning limitations and animosity between farmers and homeowners have resulted (Thomas and Skoda 1993). Both of these flies use manure or manure mixed with vegetative materials for larval developmental sites. In spite of their ubiquitous presence, importance as pests, and association with diseases of humans and livestock, our knowledge of the biology of these species is seriously wanting and available control technologies remain inadequate.
House flies are considered to be the #1 nuisance pest associated with dairy and other confined animal operations (Geden and Hogsette 1994, Hinkle and Hickle 1999). These flies are capable of carrying more than 65 disease organisms that affect humans and animals (Greenberg 1971) such as the virulent Escherichia coli strain O157:H7 (Sasaki et al. 2000). In poultry production, house flies can transmit Salmonella among flocks; and the spotting of eggs with fly specks, reduces the eggs' market value. Stable flies recently surpassed horn flies as the most important arthropod pest of cattle production (Campbell et al. 2001a). With their painful bites, they can reduce weight gains of cattle on finishing rations up to 20% (Campbell et al. 1977). Kunz et al. (1991) estimated that stable flies caused $432 million dollars in losses, primarily to confined livestock, in the US. Campbell et al. (2001a) indicated that stable flies have emerged as primary pests of pasture cattle as well, doubling their economic impact.
In 2003, the Northeastern IPM Center Livestock and Field Crop working group created a list of prioritized needs (http://northeastipm.org/work_livepriority.cfm). The group indicated that the "development of new integrated management of key pests of livestock and poultry in confined and pasture settings" was a top priority with specific reference to "stable fly breeding and migration in pasture systems" and "fly control methods for pasture and feedlot situations." Ten of the working group's 17 assessed needs and seven of the top 10 directly referred to muscoid flies. The 3 objectives of this proposal address 10 of the 17 needs. Packaging the information derived from this proposal for delivery to the stakeholders will address 2 additional priorities of this working group. Similarly, a 1994 Pest Control Survey in Texas indicated that house flies and stable flies were significant pests (Hall et al. 1996).
The proposed research is technically feasible and the expertise to accomplish the objectives of this project exists within the university and USDA-ARS systems. However, expertise is widely dispersed with few states having more than one livestock entomologist and many having none. A Multistate project will serve to coordinate this research effort, maximizing synergy and minimizing duplicated effort. Given the dispersal potential for these flies as well as varying cultural and climatic conditions throughout North America, multistate collaborations are necessary to address many of the issues of biology and control of muscoid flies.
Successful completion of this project will provide a better understanding of the interactions between livestock production systems and the life cycles of pestiferous flies. Exploitation of these interactions will provide economically feasible and environmentally friendly technology for reducing the impact of flies on livestock production and human health. The project will provide quantitative data to analyze fly borne spread of pathogens from animal production systems into the urban environment and the ability to assess the risk of fly-borne illness associated with different production techniques and distances from production facilities. The project will develop new control technologies for muscoid flies. These technologies will be disseminated to producers to reduce the abundance of muscoid flies thereby increasing the health and quality of livestock and reducing their economic impact.
Related, Current and Previous Work
This project will replace two existing projects S-1005 "Sources, Dispersal and Management of Stable Flies on Grazing Beef and Dairy Cattle" and S-1006 "Insect and Manure Management in Poultry Systems: Elements Relative to Food Safety and Nuisance Issues" Most of the recent work cited below has been the result of these two projects or one of their predecessors S-274 and NC-154.
Dispersal and population biology of stable flies and house flies
Larval habitats of stable flies: The S-1005 project developed information on stable fly developmental sites and dispersal from those sites, especially with respect to stable flies in the pasture environment. Stable fly populations peaked under relatively wet conditions with moderate temperatures (daily maxima under 30°C). In the central plains states (Kansas & Nebraska) this translates to bimodal populations with peaks in early and late summer. Large round bale winter feeding sites have been identified as the primary producers of the early summer stable fly populations (Broce et al. 2005). Research on the phenology of these sites and the interactions of feeding devices and methods with stable fly populations are on going. Preliminary recommendations for reducing stable fly development in winter feeding sites have been developed and research to validate and refine these recommendations is in progress.
Climatic factors affecting stable fly populations: Contributors to S-1005 have amassed 57 sets of time-series measures of stable fly abundance in a total of 15 separate geographic locations over 2-16 consecutive years, depending on location. Patterns among the site-years vary substantially. Most are sharply to broadly unimodal, but some exhibit two peaks, one in late spring and a second in early fall. These data sets await formal analysis in conjunction with matching weather data. Methods for statistical analysis of time-series data have advanced substantially beyond correlation analysis. Mixed models can discern relations among population growth (change in numbers), time-lagged density dependence, and phenologically appropriate measures of relevant weather variables (e.g., Goulson et al. 2005). Preliminary models developed for individual sites have found temperature during the previous winter to be negatively correlated with populations (Broce et al. 2005, Taylor unpubl. data). Temperature 1 to 2 weeks prior and rainfall 3 to 5 weeks prior are also significant contributors to stable fly populations (Taylor unpubl.). These results agree in general with those of previous studies (Greene 1989, Mullens and Peterson 2005).
Stable fly dispersal: Three mark-recapture studies have been conducted as part of the S-1005 project. Although data analyses are incomplete, preliminary analysis indicates dispersal patterns from larval developmental sites differs among sites. Dispersal and recaptures were much lower from developmental sites near large numbers of animals (average distance to recapture 0.13 km) whereas more flies were recaptured further from developmental sites when fewer animals were in the vicinity (average distance to recapture 1 km). Stable flies were collected up to 7 km from their emergence site and males and females appear to disperse equally (Moon et al., in prep.). Earlier studies indicate stable flies are capable of long distance dispersal (Hogsette and Ruff 1985, Hansens 1951, Voegtline et al. 1965).
Overwintering of stable fly in North America: Sources of spring populations of stable flies in temperate zones and the relative contributions of overwintering and migration to those populations are unclear. Since no freeze-tolerant, diapausing, form of S. calcitrans has been reported, it has been assumed that stable flies breed continuously in northern climates (Berkebile and Thomas 1995; Somme 1961) possibly inside heated barns. Models based on laboratory investigations of larval development at cooler temperatures (Beerwinkle et al. 1978; Berry et al. 1976; Berry et al. 1977; Kunz et al. 1977; Lysyk 1998) demonstrated the ability of S. calcitrans larvae to delay pupation for extended periods of time at low temperatures. This may explain their ability to overwinter as active larvae deep within heat-producing media such as ground piled silage (Scholl et al. 1981; Berkebile et al. 1994) and manure mounds (Berry et al. 1978). This phenomenon requires that the substrate be heat producing and loose enough for larvae to migrate within the habitat as the temperature profile changes due to external, climatic factors and internal, heat production. The relative contribution of this form of overwintering to spring populations of stable flies in the northern temperate zone is not known.
House fly dispersal: The production and dispersal of house flies is one of the most challenging problems for animal agriculture (Hinkle and Hickle 1999). House flies are commonly associated with confined animal facilities where they breed in the manure, animal feed, and other organic materials (Axtell 1986, Meyer and Schultz 1990). Flies may disperse from agricultural operations into the surrounding area (Lysyk and Axtell 1996, Winpisinger et al. 2005) and enter human residences where they can be a nuisance and transmit pathogens (Lole 2005).
Previous research under S-1006 indicated that house flies do not disperse great distances in urban environments where homes and landscaping provide ample vertical structure for resting and food is plentiful (e.g. garbage cans and pet droppings) (Gerry, personal observation). In contrast, house flies in agricultural settings may disperse great distances away from developmental sites as the environment surrounding these developmental sites often lacks the vertical resting surfaces and food sources require.
Monitoring house fly abundance: Pest monitoring systems are key components of IPM programs allowing for a substantial reduction in pesticide usage by providing early indications of increasing populations. Early detection provides the opportunity to respond with non-chemical means such as sanitation before adult fly numbers reach nuisance or treatment thresholds. Sanitation provides better and more sustainable control of house flies than insecticides and is especially effective when directed by monitoring systems (Axtell 1986). Monitoring also provides feedback to evaluate the effectiveness of sanitation efforts for fly control. Qualitative observations of fly numbers can be misleading and unreliable.
Nuisance thresholds provide objective measures for abundance above which negative outcomes are expected. When abundance values rise above the nuisance threshold, immediate control efforts utilizing adult targeted insecticides to suppress house fly numbers are required (Geden and Hogsette 1994, Moon 2002). Abundance values below the nuisance threshold do not require immediate suppression, but may indicate that non-chemical control efforts such as sanitation are needed as the nuisance threshold is approached.
Standardized monitoring systems to determine the abundance of house flies in large scale animal operations are needed to encourage the confined animal agriculture industry to adopt a true IPM approach for house fly control. Without a monitoring system that can be recommended by extension personnel and county agents, a population based program of insecticide use will not gain widespread acceptance.
Fly-borne dispersal of human and animal pathogens
Identification of bacteria: Food borne pathogens including; E. coli, and Campylobacter spp., are persistent and widespread problems in food production and preparation. Although great strides have been made in the post-harvest interval to prevent contamination of meats, little progress has been made in the pre-harvest interval on the farm and in the community (Mead et al. 1999). Synanthropic flies are vectors of food associated pathogens such as Escherichia coli and Campylobacter coli (Elder et al. 2000, Greenberg 1973). House flies and stable flies frequent animal manure and move to cattle, feed, water, and the adjacent environments (Elder et al. 2000). These flies disperse within farm operations, among farms, and from farm to human habitation, including food preparation sites. House flies are the biotic factor most closely associated with the spread of pathogenic E. coli from livestock to children in Japan (Sasaki et al. 2000). While filth flies are vectors of enteric disease organisms to humans, the extent to which these flies contribute to the maintenance and spread of pathogens within and between livestock operations and the community is unknown. As part of S-1006, we developed and field tested methods for sampling synanthropic flies for enteric bacteria (Geden 2005, Likirdopulos et al. 2005). A rapid PCR method for the identification and detection of food borne pathogens was developed (Szalanski et al. 2004), as was a method for the preservation of the enteric agents (Owens and Szalanski 2005). Quantitative models for the dispersal of flies and pathogens were developed and verified in a recent study of the transmission of the Porcine Reproductive and Respiratory Syndrome Virus (PRRSV) conducted at the University of Minnesota (Schurrer et al 2004).
Management of stable flies and house flies
Stable fly control tactics: As part of S-1005, round bale feeders modified to reduce hay wastage did not appreciably reduce stable fly development. The effects of hay to manure ratios in larval media on survival are being evaluated (Talley & Broce, unpubl.). Non-insecticidal treatments such as lime, sulfur and citric acid for control of stable flies in hay feeding sites are being explored as well. Entomopathogenic nematodes were shown to reduce stable fly populations in round bale feeding sites by up to 50% (Foil and Szalanski, unpubl.).
Preliminary studies as part of S-1005 (Foil unpubl.) indicate treated cloth targets may be a viable option for adult stable fly control. Permethrin-treated Alsynite traps provided more than 30% reduction of stable fly populations at two sites in Florida when used at a rate of one trap per five head of cattle (Foil unpubl.). Comparative studies indicate cloth targets are more effective than Alsynite traps, and that stable fly control should be possible with a reasonable number of targets. One treated target can potentially eliminate more than 10,000 adult stable flies per day.
House fly control
Biological control with pteromalid parasitoids: Previous research under S-1006 documented that calf areas, most often calf hutches, are the greatest source of fly breeding on dairy farms. Large, plastic covered, half-hoop structures called coveralls, are replacing calf hutches on many dairy farms. Biological control of flies on dairy farms with pteromalid wasps relies on either indigenous natural enemies or inundative releases, particularly Muscidifurax raptor (Miller and Rutz 1990, Meyer et al. 1990, Geden et al. 1992). A recent addition to the commercially available parasitoids is Muscidifurax raptorellus. This species produces multiple offspring per fly host allowing them to become established more rapidly and reducing cost. Studies in poultry facilities and cattle feedlots have documented the benefits of M. raptorellus. Sustained releases of parasitoids in poultry houses resulted in only 8% parasitism, but 50% reduction in adult house flies. The parasitoids kill many hosts by feeding without parasitizing them (Geden and Hogsette 2006).
Insecticide resistance: Permethrin was registered in the mid-1980s and Cyfluthrin in the mid 1990s and house fly resistance to both of these materials has become widespread. Studies at NY dairies show similar resistance levels for all insecticides, regardless of the spray regime used at the site (Kaufman and Rutz 2001; Scott et al. 1989). However, another study found resistance levels vary greatly between Florida, North Carolina, New York and Maine except for permethrin, which exhibits high levels of resistance in all states (Hamm et al. 2005).
An important consideration for the development of a resistance management program is to identify the size of the target area (single site, county, state or region) over which the program must be implemented in order to maximize the chances of success (i.e. determine pest mobility). Previous studies of resistance in NY suggested that house flies are quite mobile and resistance management programs must be carried out over very large areas. Insecticide use in different states produces unique patterns of resistance.
Objectives
-
Characterize dispersal and population biology of stable flies and house flies, and develop monitoring methods for use in indoor and outdoor environments.
-
Establish extent of fly-borne dispersal of human and animal pathogens.
-
Improve management tactics for stable flies and house flies.
Methods
See attachment for complete Methods section due to character limit Objective 1: Subobjective 1. Characterize stable fly origins and dispersal A. Characterize larval habitats of stable flies (NE-ARS, KS, TX, FL-ARS) The seasonal dynamics of winter hay feeding sites will be evaluated by temporal and zonal sampling of feeding sites. Core samples will be collected and macrofauna isolated. Immature stable flies will be counted and characterized by instar. Other macrofauna will be quantified and cataloged. Emergence traps will be used to evaluate zonal and seasonal stable fly emergence. These data will be used to identify the zones and seasons of primary stable fly production in hay feeding circles so control strategies may be most effectively focused. Livestock producers will be surveyed to quantify and characterize winter-feeding sites and systems. Stable fly production from different winter-feeding systems will be evaluated with emergence traps and core sampling. A survey will be conducted at the University of Nebraska Agricultural Research and Development Center (ARDC) located near Ithaca Nebraska to locate alternate larval habitats. Four sections representing 4 agroecotypes, cattle feedlot, dairy, pasture and cropland will be surveyed in early, mid and late summer. Aerial photos will be used to assist ground surveillance crews to locate and map potential stable fly larval developmental sites. Alsynite stable fly traps will be maintained semiweekly. The region within a ½ mile around traps showing rapid, isolated, increases in stable fly collections will be surveyed to identify the sources of the flies. Physical (temperature, humidity, pH, and media consistency) and biological (stable fly density, associated entomofauna and microfauna) parameters will be recorded at sites, both positive and negative for immature stable flies, to develop descriptive parameters for suitable larval developmental sites. Potential sites will be monitored throughout the year to determine their seasonal contribution to stable fly populations. In subsequent years, surveys will be conducted at other locations representing different climatic and / or cultural zones. Stable fly larval habitats identified will be characterized as were hay feeding sites. Grids of Alsynite traps will be maintained to measure the geographic and temporal distribution of adult stable fly populations associated with areas included in the surveys. Temporal correlation of adult trap collections and emergence from larval developmental sites will be used as an indicator of the contribution of given types of developmental sites to adult fly populations. B. Identify climatic factors affecting stable fly populations (MN, KS, NE-ARS, TX, FL-ARS) Records of numbers of flies caught per trap per day in each of the 60 data sets (site-years) will be analyzed using mixed regression models to relate changes in catch rates to density and antecedent weather variables. Weather data will be downloaded from the nearest NOAA station. Time scales for each series will be converted to a generational time scale, using temperature-rate data (Lysyk 1998). Catch rates will then be interpolated and matched between generations to derive sequential measures of growth from one generation to the next. Growth rates will be analyzed with a mixed model response surface approach (Goulson et al. 2005), using antecedent densities, temperatures, and precipitation as predictors. Density and weather variables will be treated as fixed effects, whereas site-year combinations will be treated as random. Results will be assembled into a spreadsheet model to calculate catch rates from daily temperature and precipitation data. Starting from an initial value for catch rate, provided by the user, the model will calculate growth rate as a function of temperature and precipitation, as deemed influential in the response surface model, and then integrate growth rates to project seasonal patterns of fly abundance. Models will be tested on new data sets as they become available. C. Dispersal of Stable Flies (ARS-NE, FL, LA, AR, TX, KS, IN, MN, NY) Historical weather and first date of stable flies appearance on traps will be evaluated to estimate when northerly dispersal of stable flies is most likely to occur. Sampling will begin 2-3 weeks prior to the estimated date and continue until local production of the second generation is observed. Alsynite traps will be deployed along the putative northeasterly dispersal routes through NW TX, and OK, KS, NE, IA, MN, IL, IN and NY. Traps will be operated semi-weekly during the dispersal period and the number of stable flies caught will be recorded. Weather will be monitored to identify frontal systems approaching the study area; traps will be operated daily at least two days before and after the arrival of frontal systems. Fresh stable fly specimens will be dissected to assess physiological status. Correlation of trap collections with weather parameters will be assessed. Three and five fold increases in trap rates (flies/trap-day) will allow two levels of discrimination of the strength of the data. The proportion of 3x and 5x traps in each of the study areas will be compared to the passage of frontal systems as confirmed by changes in barometric pressure, temperature and wind direction. Response of stable flies of different physiological states to changes in barometric pressure (Wellington 1945) will be assessed in the laboratory. Alsynite traps will be run from January to April to identify putative sources of migrating flies; traps will be run in NM, TX, and LA. Traps will be operated at representative dairies, feedlots and pastures in southern areas with high numbers of confined cattle: (a) Dairies near El Paso, TX, and along the Rio Grande in Southern New Mexico; (b) dairies in the Roswell (Chaves Co.) area in New Mexico; (c) feedlots on the Texas Panhandle; (d) confined cattle operations in Eastern-Central Texas; and (e) pasture cattle operations in Southern Louisiana. Traps will be operated semi-weekly and stable fly numbers recorded. Physiological state of flies trapped during the passage of frontal systems will be compared to that of flies collected before and after to identify characteristics of dispersing flies. Quick-Cult® test (Laboratory Diagnostics Co., Inc., Morganville, NJ) for fecal occult blood (Dryden 1998) will be used to detect blood in stable flies. The frequency of flies positive for a previous blood meal (<48h old) will be analyzed as a function of when they were trapped in relation to the passage of a frontal system. The elemental and isotopic composition of flies is expected to vary according to the characteristics of their larval habitat. The concentrations of elements in the heads of flies trapped during a frontal event will be compared to those of flies which developed locally. Elemental compositions will be determined by Neutron Activation Analysis (NAA) at Kansas State University. Movement of stable flies relative to cattle in the pasture environment will be evaluated in a 100 acre pasture removed from other cattle. Three paddocks will be created within the pasture using electric fence. A stable fly larval habitat will be created in an area central to the three paddocks by feeding round hay bales for one month prior to the study. Alsynite traps will be placed around each of the paddocks. Cattle will be rotated through the paddocks daily. The number of flies captured at each paddock and their physiological stage will be recorded. A large emergence cage will be placed over a larval habitat that has been dusted with fluorescent dye and marked flies will be released on the first day of the study to estimate the size of the stable fly population in the area and to determine if the flies remain with the cattle. D. Determine overwintering dynamics of stable fly throughout the USA (ARS-NE, ARS-FL, TX, FL, KS, MN) Stored feed and manure handling systems will be surveyed to locate overwintering stable fly larvae. Potential overwintering sites will be excavated in late winter to locate larvae. Emergence traps will be placed near potential overwintering sites to collect emerging flies. Sticky traps will be placed in the vicinity of potential overwintering sites. Physical parameters, substrate, temperature, depth & consistency, will be recorded when overwintering larvae are observed. Adults collected on sticky traps will be dissected to determine their physiological age as an indicator of whether they are migrants or locally produced. Using the physical conditions derived from natural and artificial overwintering sites, studies will be conducted to duplicate overwintering in the laboratory. Samples of stable fly larvae will be maintained at fixed temperatures. Temperature loggers will be placed in the diet / substrate to record the actual temperatures to which the larvae are exposed in addition to the ambient temperatures of the environmental chambers. Subsamples will be removed at intervals and held at 20º C for adult emergence. Reiterations will be run with smaller temperature increments until the optimal temperatures and limits for stable fly larvae to extend their larval stage and the upper limits of how long the larval stage can be extended have been identified. Experiments will be repeated with natural substrates after initial testing with laboratory diets. Overwintering temperature profiles will be compared to those observed in artificial overwintering sites fitted with temperature sensors to evaluate the potential for overwintering even if we fail to observe natural overwintering. Based upon the characteristics of positive overwintering sites, experimental sites will be constructed to evaluate time of ensilation, silage material, and time of oviposition on overwintering success. Temperature and humidity probes will be used to understand temporal and spatial dynamics of heat production and decomposition. Alternative overwintering habitats such as compost and barns will be examined for the presence of overwintering stable flies in January and February. Emergence traps will be used to monitor the emergence of overwintering stable flies from both natural and artificial overwintering sites. Seasonal populations of stable flies from northern regions of the US will be evaluated using mitochondrial and microsatellite genetic markers. Approximately 100 adult stable flies will be collected biweekly from the appearance of flies in the spring until they disappear in the fall. Four sites will be included in this study initially, TX, KS, NE, and MN. F-statistics will be used to compare temporal and geographic components of genetic variation. Subobjective 2. Improve understanding of house fly dispersal and behavior, and develop methods for monitoring them in indoor and outdoor environments. A. Dispersal of house flies (AR, ARS-FL, TX, IN, CA, TN) The effect of environmental and geographical conditions on dispersal will be evaluated using a mark-release-recapture method to examine movement of marked laboratory-reared flies under varied environments and landscapes (e.g. open agricultural areas vs. restricted terrain). Initial studies will assess movement within production facilities. Later, dispersal among agricultural and residential / urban environments will be evaluated. Different color markers will be used to determine dispersal directions and preferences. Effects of vertical barriers (e.g. tree lines) at the periphery of animal operations on fly dispersal will be evaluated. If barriers are effective, the height and density of vertical structures needed to capture dispersing flies will be evaluated. Effectiveness of treating vertical structure with residual chemical insecticides will be assessed. Passive traps that sample air space will be used in conjunction with sticky cylinder and toxic bait traps. Traps will be placed at heights, directions, and distances around developmental sites to differentially capture flies dispersing under varying environmental conditions. Captured flies will be removed hourly to provide information on diurnal variation. The age of dispersing flies will be determined by ovarian dissections and analysis of the pterin deposition in the head capsule (Mullens, personal communication). Barrier techniques will be developed and tested to reduce fly dispersal from animal operations. Barrier techniques envisioned include tree lines and covered fences with and without insecticide treatments separating animal operations from residential homes. B. Monitoring House Fly Abundance (AR, ARS-FL, CA, FL, TX, NY, IN, MN) Standardized monitoring tools will be tested both as their efficacy may vary between these two environments. Attempts will be made to characterize nuisance (or treatment) thresholds that can be used to assist animal facility managers to develop appropriate control programs. Relative house fly abundance will be measured during the early and peak periods of the house fly season. Three house fly monitoring systems will be simultaneously utilized to evaluate their ability to detect early increases in house fly abundance while still providing manageable information during periods of peak fly abundance. The three monitoring systems to be used are 1) spot card counts, 2) fly tapes, and 3) fly bait traps. At each animal facility, 10 spot cards and 5 fly tapes will be placed at fly resting sites (identified by the presence of previous spotting) in covered barns or similar animal resting and feeding areas, and 5 fly bait traps will be placed just outside structures housing animals. Monitoring devices (cards, tapes, and bait traps) will be collected and replaced weekly. A weekly count of flies (identified to species) and spots (on spot cards) will be recorded for each monitoring device. Collection data will be evaluated for variance between devices, variance between collection weeks, and correlation between the three monitoring systems. Time to deploy monitoring devices and count insects or spots will also be recorded for each monitoring system to evaluate the cost in man hours for each of these systems. Objective 2. Subobjective 1. Identification of bacteria from fecal and environmental samples. (NC, AR, KS, MN, TN, TX, ARS-FL, ARS-NE, CA) Microbial isolation and detection methods will follow those used by Szalanski et al. 2004. Cattle fecal swabs will be sampled every two weeks from each farm. Samples will be processed using thioglycollate enrichment broth supplemented with antibiotics under CO2 gas pack conditions to maximize Campylobacter growth. Cultures will be plated on Brucella blood agar and incubated at 42°C. Campylobacter presumptive cultures will be tentatively identified and stored at 70°C for subsequent PCR testing. The methods of Szalanski et al. (2004) will be used for the molecular detection of E. coli 0157:H7 and Campylobacter sp. from filth flies. DNA will be extracted from individual filth flies and re-suspended in Tris-EDTA and frozen. PCR primers MD16S1 and MD16S2 (Denis et al. 2001) will be used to detect Campylobacter sp. and primers RfbF, RfbR (Hu et al. 1999)), FLICh7F, and FLICh7R (Gannon et al. 1997) will be used to detect E. coli 0157:H7. PCR products will be run on 1% agarose gels and diagnostic PCR amplicons will be visualized using a UVP biodoc-it-system. Subobjective 2. Conduct laboratory studies to isolate and incriminate flies in the dispersal of disease agents Adult flies will be collected from five beef farms and five dairy farms (10 total) monthly using methods developed by Geden (2005) and (Likirdopulos et al. 2005). Collection devices will be placed near barns on study farms and on properties adjacent to farms (distances will be calculated from GPS coordinates for trap location) for approximately four hours. Collected dead house flies will be held on ice and taken to the laboratory where the flies will be pooled by sample site and species and frozen at 70°C until processed. Individual flies will be tested using the methods developed by Owens and Szalanski (2005). PureGene cell lysis kit (Gentra, Minneapolis, MN) will be used to extract both fly and pathogen DNA. Extracted DNA will be resuspended in 50 µl of 10 mM Tris-HCl and stored at 20°C in Eppendorf tubes. Approximately half of the frozen DNA samples will be transferred to filter papers and stored until DNA processing as described by Owens and Szalanski 2005. Remaining sample material will be returned to frozen storage and the filter paper samples will be stored at 21°C and 40% RH until processed using PCR methods described above (Szalanski et al. 2004). Subobjective 3. Develop quantitative models of fly and pathogen spread. Risk of transmission is likely to depend on (1) absolute densities (per unit surface area) of microbes and flies at the source point, (2) the frequency distribution of acquired, infectious microbes per fly (as external and internal contaminants), (3) the rate of loss per unit time of infectious microbes in the contaminated flies, and (4) the rate of spread of contaminated flies from source to recipient points. Laboratory experiments will be conducted to describe the relation between microbe density and contaminating loads per fly (relating [1] to [2]), retention of given microbes through time at different temperatures [3], and spatial spread of house flies from point sources in a variety of landscapes. Research to check current assumptions and develop the model will require collaborative efforts at the bench, the field, and the computer. Objective 3. Subobjective 1. Develop stable fly control tactics A. Control of immatures (NY, NE, TX, ARS-FL, ARS-NE, KS) Chemicals which modify the pH of larval habitat such as lime, sulfur and citric acid and biological agents such as entomopathogenic nematodes and pathogens will be bioassayed in laboratory experiments to determine their effects on stable fly larvae. Compounds which effectively reduce larval survival in the laboratory will be tested in 1 meter2 plots in natural habitats. Plots will be delineated by frames driven into the media. Emergence traps will be used to evaluate control levels. Upon completion and verification of climatically driven population models for stable flies, the effects of area wide habitat modification on populations will be evaluated. Treatments will include removal and composting of hay feeding wastes, soil incorporation of wastes and other technologies such as chemical and biological treatments as they become available. Because annual and geographic variation in stable fly populations make comparisons between area wide scale control and treatment areas difficult, treatments will be assessed by evaluating deviations from population model derived expectations. B.Control and economic impact of adults (NE, LA, FL, NY, ARS-FL, KS) Treated targets (TTs) will be evaluated on 2-4 small farms with 150 or fewer animals in each cooperating state. One meter square targets will be soaked until saturated in 0.1% lambda-cyhalothrin (Demand CS 9.7%, Wilmington, DE) or water as a control and allowed to dry. TTs will be deployed for 2-3 months once the action threshold of 100-300 stable flies / Alsynite trap / day is reached. Control and treatment farms will be determined randomly. Targets will be deployed at least 50 m apart and at the rate of one target per 25 animals. Alsynite traps and leg counts will be used to evaluate the effects of TTs on stable fly populations. Upon completion of the study, targets will be bioassayed to determine residual toxicity with a standard stable fly strain. The impact of stable flies on weight gain of yearling heifers will be measured in LA Ninety replacement heifers will be weighed and then blocked by weight and assigned to six groups of 15 each. Three groups will be treated with 1 ml of 10% permethrin per leg once per week and the other three groups will be treated with water. Weights will be recorded weekly and at the end of the 45-60 day study. The six groups will be held on 5 acre pastures with supplemental hay and rotated among the pastures weekly to remove pasture effect. The number of stable flies on the front leg of each animal will be recorded three times per week. Subobjective 2: Develop house fly control and resistance management tactics A. Biological control with pteromalid parasitoids (NY, AR, ARS-FL, FL, NC) Natural parasite populations will be monitored with screen bags of sentinel house fly pupae. Bags are placed at each farm and replaced weekly. After exposure, emerged flies will be counted and recorded. Remaining pupae will be placed individually in wells of 96 well ELISA plates and wrapped in plastic foil. After 3 weeks, emerged flies will be counted and parasites identified. Intact pupae will be dissected to determine if fly death was caused by wasps. Naturally occurring house fly pupae will be collected and transported to the laboratory each week. Pupae will be handled as above. Studies are being planned in New York to determine if a combined release of closely related, but biologically varied parasitoid species will complement or synergize each other which will then enable us to develop recommendations for dairy producers as to which species of parasitoid or combination of parasitoids to purchase for cost effective fly biological control. Releases of a 50:50 ratio of Muscidifurax raptor and Muscidifurax raptorellus will be compared to M. raptorellus-only releases. Fly population levels and parasitoid activity (successful and total parasitism) will be observed in dairy facilities before, during and after the parasitoid releases B. Chemical control (NY, AR, IN, NC, ARS-FL) Several novel insecticides will be used separately and in combination in broiler-breeder, turkey and dairy farms during the fly season to determine their efficacy in adult fly management. These novel insecticides will include Nithiazine on QuickStrike fly abatement strips, imidacloprid as an adult fly bait and Extinosad PSP applied as a residual spray to walls and curtains and to treated strings in turkey and broiler-breeder facilities as well as spot treatment to manure for larval fly management. If different or new novel insecticides become available we will include these in our studies. Flies will be collected and tested for resistance both at the beginning and at the end of the fly season to determine if resistance is developing to these insecticides. If resistance appears, rotation with other insecticides will be implemented in an attempt to minimize the development of resistance. As different or new novel insecticides become available, we will include these in our studies C. Conduct nationwide survey for insecticide resistance (NY, TX, AR, NE, MN, GA, FL, KS, IN, CA, NC, MT) Starting in the summer of 2007 we will begin a nationwide survey of insecticide resistance in house flies, using both bioassays and genotyping of the alleles involved in insecticide resistance. We anticipate evaluating one class of insecticides each year starting with pyrethroids (permethrin and cyfluthrin). The general plan is for cooperators across the USA to collect house flies (by sweep net), and obtain detailed histories of insecticide use, from one to five facilities (dairy, poultry or hog) across their state. Files would be brought back to the laboratory and eggs would be collected. Emerging flies would be used for bioassays by the lab making the collections. To standardize and facilitate the bioassays, each lab will be provided (by J. Scott) with the insecticide, and with a detailed protocol for how to carry out the tests. Each lab making collections will be required to buy a limited number of glass jars (as specified in the protocol), acetone and pipettes. Pupae (~500) from field collected flies would be sent to Cornell Univ. for genotyping. J. Scott and D. Rutz will seek additional funds to cover the sequencing costs (estimated at $800 per site). Availability of these funds will determine the number of collections for which we are able to genotype (50-100) individuals.Measurement of Progress and Results
Outputs
- This project will produce standard protocols for use in studying stable fly and house fly biology, pathogen interactions and control. Standard protocols permit data derived from different parts of the country to be directly comparable. Collaborations facilitate studies involving large geographical areas such as migration, population genetics and insecticide resistance and foster the interdisciplinary research needed to evaluate the role of flies in the epidemiology of pathogens. Few states have the expertise to conduct many of these studies alone. Recommendations for reducing stable fly and house fly populations, controlling the spread of pathogens to humans and among livestock and for maintaining the effectiveness of the available insecticides will be developed and disseminated through extension and outreach programs. Research publications on stable fly and house fly biology, relationships to disease transmission and control strategies will be produced.
Outcomes or Projected Impacts
- This project will develop biological, chemical and cultural control strategies for stable flies and house flies. Such strategies will reduce the impact of these flies on livestock producers and livestock producers impact on neighboring residential areas. Accurate models of fly population dynamics will permit livestock producers to economically implement biological and cultural controls before populations reach levels necessitating chemical controls. Characterizing the epidemiology of pathogens will permit producers to develop filth fly control strategies for reducing food borne pathogens. Models of pathogen dissemination will provide planners with quantitative tools to assist in zoning decisions. The nationwide survey of insecticide resistance will provide critical information on the extent of our fly resistance problem nationwide and will also provide a solid scientific base on which to build cost effective and environmentally sound insecticide resistance management programs.
Milestones
(0):ective 1: Years 1-3: Hay feeding sites will be characterized and surveys for alternate larval habitats will be completed. Control strategies will be tested in small plots. Stable fly population dynamics models will be completed and verified. Natural overwintering sites will be surveyed and laboratory analyses of temperature effects on larval development will be completed. Genetic analysis of temporal populations of stable flies will be completed. Long range dispersal studies will be initiated and continue through year 5. Geographic populations of stable flies will be analyzed with Nuclear Activation Analysis. Natural dispersal of house flies within animal facilities and migration to residential areas will be completed. Development of house fly monitoring protocols will be completed and recommendations made. Years 4-5: Significant alternate stable fly habitats identified in surveys will be characterized. Control strategies will be implemented on area wide basis. Populations in control zones will be evaluated against predictions of weather driven models based upon pre-control parameters to evaluate success of control strategies. Artificial overwintering sites will be constructed to verify results of laboratory studies. Dynamics of different overwintering substrates, manure, silage, etc. will be evaluated in relation to overwintering criteria developed in laboratory. Seasonal stable fly populations will be analyzed with Nuclear Activation Analysis to help determine their geographic origin and possibly larval habitat. Methods to limit house fly movement from livestock facilities to residential areas will be evaluated and recommendations made.(0):ective 2: Year 1: Identify and begin sampling flies and manure from participating farms. Standardize PCR methodology for reproducible outcomes. Identify target bacteria to be monitored. Year 2: Continue background monitoring of target bacteria on the farm. Sample fly populations to document dispersal pattern from the farms and adjacent properties. Quantify target bacteria associated with flies confirm retention time relative to environmental conditions. Develop models to characterize dispersal. Year 3: Continue background monitoring, fly population sampling and document dispersal patterns from the farms and adjacent properties. Apply and refine model to characterized dispersal. Develop intervention strategies. Year 4: Sample fly populations to document dispersal patterns from the farms and adjacent properties. Quantify target bacteria associated with flies confirm retention time. Apply intervention strategies. Apply model to characterize dispersal. Year 5: Complete analysis, develop recommendations for risk avoidance and formulate intervention strategies.
(0):ective 3: Years 1-3: Parasitoid release rates and species recommendations will be developed and made available to the livestock and poultry industry with the request for feedback on their effectiveness. Resistance development to novel fly insecticides will be determined in several sections of the US. The nationwide survey of fly insecticide resistance will be conducted and completed. Years 4-5: The biological control component, particularly the use of parasitoids, of fly IPM will be fine-tuned and made available for widespread nationwide implementation. Resistance management programs will be developed for the novel insecticides and made available to the livestock and poultry industries nationwide. Resistance management programs will be developed and made available to the livestock and poultry industries nationwide.
Projected Participation
View Appendix E: ParticipationOutreach Plan
Multiple avenues will be used to disseminate research results to livestock and poultry producers. Information will also be disseminated to the states' beef and dairy associations such as the Cattlemen's Association, to beef cattle trade publications such as "Beef," and to farm publications like the "Nebraska Farmer," and similar trade journals in the various states. Similarly, Hoard's Dairyman and other venues appropriate to dairy producers will be utilized. The information will also be provided to livestock extension educators in the various states to be used in continuing education programs. Additionally, a number of the project participants have cooperative extension assignments and will include project findings in newsletters and fact sheets aimed directly at animal producers.
In addition to the transient extension outlets listed above, comprehensive extension publications on integrated pest management of house fly and stable fly will be published for dairy and rangeland operations by university publication services; to be provided to the public as downloadable documents where possible. Publications will address IPM in light of the findings of this research; to include the best options for pest monitoring, determination of treatment thresholds, pesticide rotation programs to reduce pesticide resistance, and best practices for overall management of house fly and stable fly. Producers have routinely indicated a need for such publications during Pest Management Strategic Plan development.
Project members are currently soliciting funding to develop crop profiles for beef and dairy cattle (as well as other confined animal agriculture commodities) with the expectation that regional or national rather than state-specific crop profiles be developed and published with the USDA Regional IPM Centers Information System (http://www.ipmcenters.org/). Crop profiles would also address pest management options and include updated lists of registered pesticides for each commodity. Maintaining lists of currently registered pesticides by commodity has proven difficult and is a need that has been identified during numerous Pest Management Strategic Plans. Further such a list should be organized by chemical class of insecticide to support rotational programs to reduce resistance development. Efforts would be made by project participants to update these crop profiles annually in order to retain their value to the public. Crop profiles would provide links to appropriate university extension publications for a more comprehensive review of pest management.
Finally, project participants would be expected to sponsor or participate in state and regional workshops in conjunction with the development of new Pest Management Strategic Plans to extend project findings, to evaluate the impact of project findings on current commodity practices, and to determine future needs.
Organization/Governance
Organization will be as prescribed in the Guidelines for Multistate Research Activities. Component research projects will be planned and coordinated by the technical committee. Members with voting status will consist of a representative from each cooperating SAES who is appointed by the respective Director, and a representative from each cooperating ARS and Ag-Canada laboratory as authorized by the laboratorys administrator. The committee will also have an Administrative Advisor (non-voting status) and a consulting member representing Cooperative States Research Extension Education Service (CSREES) (non-voting status).
Officers of the Technical Committee will consist of Secretary, Chair-elect, and Chair. Officers will serve for 2-year terms. The three officers will be elected at the first meeting of the Technical Committee. Chair-elect will ascend to Chair upon the completion of the Chairs term in office. A new Chair-elect and Secretary will be elected in succeeding alternate years. The Technical Committee will meet at least once each year to discuss project results and plan subsequent work. The Chair will consult with the Technical Committee and Administrative Advisor regarding meeting dates and locations. The Advisor will authorize and announce meetings to the Technical Committee. The Chair will set meeting agendas, lead meetings, and supervise preparation of the annual report of project work. The Chair-elect will assist the Chair as requested, and serve as Chair in his or her absence. The Secretary will record minutes of meetings. Reports and minutes will be submitted to the Administrative Advisor for appropriate distribution, and will be posted on the projects web site within 60 days of each meeting.
Literature Cited
Axtell, R.C. 1986. Fly management in poultry production cultural biological and chemical. Poultry Science 65: 657-667.
Bager, F. and J. Petersen. 1991. Sensitivity and specificity of different methods for the isolation of Salmonella from pigs. Acta Vet. Scand. 32:473-481.
Beerwinkle, K.R., I.L. Berry, and S.E. Kunz. 1978. Prediction models for mortality of immature stable flies caused by cold temperatures. Environ. Entomol. 7:273-277.
Berkebile, D.R., and G.D. Thomas. 1995. Overwintering and dispersal of the stable fly. Pp. 110-118. In: Thomas, G. D., and S. R. Skoda (Eds.). The Stable Fly: A Pest of Humans and Domestic Animals. Proc. Symposium, 1992 Ann. ESA Meeting. Neb. Agric. Exp. Sta. Misc. Pub. MP 64. 148 pp.
Berkebile, D.R., G.D. Thomas, and J.B. Campbell. 1994. Overwintering of the stable fly (Diptera, Muscidae) in southeastern Nebraska. J. Econ. Entomol. 87:1555-1563.
Berry, I.L., K.W. Foerster, and J.B. Campbell. 1978. Overwintering behavior of stable flies in manure mounds. Environ. Entomol. 7:67-72.
Berry, I.L., K.W. Foerster, E.H. Ilcken. 1976. Prediction model for development time of stable flies. Trans. Am. Soc. Agric. Eng. 19:123-127.
Berry, I. L., S. E. Kunz, and K. W. Foerster. 1977. A dynamic model of the physiological development of immature stable flies. Ann. Entomol. Soc. Am. 70:173-176.
Broce, A.B., J. Hogsette, and S. Paisley. 2005. Winter feeding sites of hay in round bales as major developmental sites of Stomoxys calcitrans (Diptera: Muscidae) in pastures in Spring and Summer. J. Econ. Entomol. 98: 2307-2312.
Campbell, J.B., S.R. Skoda, D.R. Berkebile, and G.D. Thomas. 2001a. Research on stable flies and house flies at Nebraska. Res. Bull. #341, Agr. Res. Div., IANR, Univ. of Nebraska-Lincoln. 22 pp.
Campbell, J.B., R.G. White, J.E. Wright, R. Crookshank, and D.C. Clanton. 1977. Effects of stable flies on weight gains and feed efficiency of calves on growing or finishing rations. J. Econ. Entomol. 70:592-594.
Denis, M., J. Refregier-Petton, M. Laisney, G. Ermel and G. Salvat. 2001. Campylobacter contamination in French chicken production from farm to consumers. Use of a PCR assay for detection and identification of Campylobacter jejuni and Campylobacter coli. J. Appl. Microbiol. 91: 255-267.
Dryden, M.W. 1998. Laboratory evaluations of topical flea control products. In: Proc. 1998 British Veterinary Dermatology Study Group. April 1, 1998. Birmingham, United Kingdom. pp. 14-17.
Elder, R.O., J.E. Keen, G.R. Siragusa, G.A. Barkocy-Gallagher, M. Koohmaraie, and W.W. Laegreid. 2000. Correlation of enterohemorrhagic Escherichia coli O157 prevalence in feces, hides, and carcasses of beef cattle during processing. PNAS 97: 2999-3003
Gannon, V. P., S. DSouza, T. Graham, R. K. King. K. Rahn, and S. Read. 1997. Use of the flagellar H7 gene as a target in multiplex PCR assays and improved specificity in identification of enterohemorrhagic Escherichia coli strains. J. Clinic. Microbiol. 35: 656-662.
Geden, C.J. 2005. Methods for monitoring outdoor population of house flies, Musca domestica L. (Diptera: Muscidae). J. Vector Ecol. 30: 244-250.
Geden, C.J. and J.A. Hogsette [eds.]. 1994. Research and Extension Needs for Integrated Pest Management of Arthropods of Veterinary Importance. Proceedings of a Workshop in Lincoln, Nebraska (Last Updated October 2001). http://cmave.usda.ufl.edu/lincoln.html
Geden, C.J. and J.A. Hogsette. 2006. Suppression of house flies (Diptera: Muscidae) in Florida poultry houses by sustained releases of Muscidifurax raptorellus and Spalangia cameroni (Hymenoptera: Pteromalidae)
Geden, C.J., D.A. Rutz, R.W. Miller, and D.C. Steinkraus. 1992. Suppression of house flies (Diptera: Muscidae) on New York and Maryland dairies using releases of Muscidifurax raptor (Hymenoptera: Pteromalidae) in an integrated management program. Environ. Entomol. 21: 1419-1426.
Gilles J., I. Litrico, P. Sourrouille, G. Duvallet. 2004. Microsatellite DNA markers for the stable fly, Stomoxys calcitrans (Diptera : Muscidae). Molecular Ecology Notes 4: 635-637.
Goulson, D., L.C. Derwtby, M.E. Hanley, D.W. Dunn, and S.R. Abolins. 2005. Predicting calyptrate fly populations form the weather, and probable consequences of climate change. J. Appl. Ecol. 42: 795804.
Greene, G.L. 1989. Seasonal population trends of adult stable flies. Pp. 1217 in Petersen J.J. and G.L. Greene (eds), Current status of stable fly (Diptera: Muscidae) research. Misc. Publ. Entomol. Soc. Am. , No. 74. 53 pp.
Greenberg, B. 1971. Flies and disease, Vol. 1. Pinceton University Press, Princeton, New Jersey, 856 pp.
Greenberg, B. 1973. Flies and disease, Vol. II. Princeton Univ. Press, Princeton, New Jersey, 447 p.
Hall, K.D., R.L. Holloway, C.E. Hoelscher, and J.C. Paschal. 1996. Texas Beef Cattle (Cow / Calf and Stocker) 1994 Pest Control Survery. B-6045. Texas Agricultural Extension Service, Texas A&M University. 14 pp.
Hamm, R.L., T. Shono, and J.G. Scott. 2005. A North-South cline in frequency of autosomal males is not associated with insecticide resistance in housefly, Musca domestica L. J. Econ. Entomol. 98: 171-176.
Hansens, E.J. 1951. The stable fly and its effect on seashore recreational areas in New Jersey. J. Econ. Entomol. 44: 482-487.
Hinkle, N.C. and L.A. Hickle. 1999. California caged layer pest management evaluation. J. Appl. Poult. Res. 8: 327-338.
Hogsette, J.A., and J.P. Ruff. 1985. Stable fly (Diptera: Muscidae) migration in northwest Florida. Environ. Entomol. 14: 170-175.
Hu, Y., Q. Zhang, and J.C. Meitzer. 1999. Rapid and sensitive detection of Escherichia coli O157:H7 in bovine faeces by multiplex PCR. J. Appl. Microbiol. 87: 867-876.
Kaufman, P.E., and D.A Rutz. 2001. Susceptibility of house flies (Diptera: Muscidae) exposed to commercial insecticides on painted and unpainted plywood panels. Pest. Manag. Sci. 58:174-178.
Kunz, S.E., I.L. Berry, and K.W. Foerster. 1977. The development of the immature forms of Stomoxys calcitrans. Ann. Entomol. Soc. Am. 70:169-172.
Kunz, S.E., K.D. Murrel, G. Lambert, L.F. James, and C.E. Terrill. 1991. Estimated losses of livestock to pests, In D. Pimentel [ed.], CRC Handbook of Pest Management in Agriculture, vol. I. CRC Press, Boca Raton, FL. pp. 69-98.
Likirdopulos, C.A., O.D. Simmons, III, D.W. Watson, and M.D. Sobsey. 2005. Collection methods for evaluating microbial indicator concentrations of house flies (Musca domestica) on swine farms in eastern North Carolina. Proceedings on the Development of Alternative Technologies for the Processing and Use of Animal Waste. 2005 Animal Waste Symposium, Oct. 5-7 Research Triangle Park, NC http://www.cals.ncsu.edu/waste_mgt/05wastesymposium/proceedings.htm
Lole, M.J. 2005. Nuisance flies and landfill activities: an investigation at a West Midlands landfill site. Waste Management Res. 23: 420-428.
Lysyk, T.J. 1993. Adult resting and larval developmental sites of stable flies and house flies (Diptera: Muscidae) on dairies in Alberta. J. Econ. Entomol. 86:1746-1753.
Lysyk T.J. 1998. Relationships between temperature and life-history parameters of Stomoxys calcitrans (Diptera: Muscidae). J. Med. Entomol. 35: 107119.
Lysyk, T.J., and R.C. Axtell. 1996. Movement and distribution of house flies (Diptera: Muscidae) between two habitats in two livestock farms. J. Econ. Entomol. 79: 993-998.
Mead, P.S., L. Slutsker, V. Dietz, L. McCaig, J. Bresee, C. Shaprio, P. Griffin, and R. Tauxe. 1999. Food-related illness and death in the United States. Emerg. Infect. Dis. 5: 607-625.
Meyer, J.A., G.P. Georghiou, and M.K. Hawley. 1987. Housefly (Diptera, Muscidae) resistnace to permethrin on southern California dairies. J. Econ. Entomol. 80: 636-640.
Meyer, J.A., B.A. Mullens, T.L. Cyr, and C. Stokes. 1990. Commercial and naturally occurring fly parasitoids (Hymenoptera: Pteromalidae) as biological control agents of stable flies and house flies (Diptera: Muscidae) on California dairies. J. Econ. Entomol. 83: 799-806.
Miller, R.W. and D.A. Rutz. 1990. Survey of house fly pupal parasitoids on dairy farms in Maryland & New York, pp 59-66. In D.A. Rutz & R.S. Patterson (eds.), Biocontrol of Arthropods Affecting Livestock & Poultry. Westview Press, Boulder, CO. 316 pp.
Moon, R.D. 2002. Muscid Flies (Muscidae), pp. 279-303. In G. R. Mullen and L. A. Durden [eds.], Medical and Veterinary Entomology. Academic Press, San Diego.
Mullens, B.A., and N.G. Peterson. 2005. Relationship between rainfall and stable fly (Diptera: Muscidae) abundance on California dairies. J. Med. Entomol. 42: 705-708.
Owens, C.B., and A.L. Szalanski. 2005. Filter paper for preservation, storage and distribution of insect and pathogen DNA samples. J. Med. Entomol. 42: 709-711.
Petersen, J. J. and D. W. Watson. 1992. Comparison of sentinel and naturally occurring fly pupae to measure field parasitism by pteromalid parasitoids (Hymenoptera). Biological Control 2: 224-248.
Sasaki, T., M. Kobayashi, and N. Agui. 2000. Epidemiological potential of excretion and regurgitation by Musca domestica (Diptera: Muscidae) in the dissemination of Escherichia coli O157:H7 to food. J. Med. Entomol. 37: 945-949.
Scholl, P.J., J.J. Petersen, D.A. Stage, and J.A. Meyer. 1981. Open silage as an overwintering site for immature stable flies in eastern Nebraska. Southwest. Entomol. 6: 253-258.
Schurrer, J.A., S.A. Dee, R.D. Moon, K.D. Rossow, C. Mahlum, E. Mondaca, S. Otake, E. Fano, J. Collins, and C. Pijoan. Spatial dispersal of procine reproductive and respiratory syndrome virus-contaminated flies after contact with experimentally infected pigs. Amer. J. Vet. Res. 65: 1284-1292.
Scott, J.G., R.T.Roush, and D.A.Rutz. 1989. Insecticide resistance of house flies from New York dairies (Diptera: Muscidae). J. Agric. Entomol. 6: 53-64.
Scott J.G., T.G. Alefantis, P.E. Kaufman and D.A. Rutz. 2000. Insecticide resistance in house flies from caged-layer poultry facilities. Pest Manag Sci 56:147-153.
Somme, L. 1961. On the overwintering of house flies (Musca domestica (L.)) and stable flies (Stomoxys calcitrans (L.)) in Norway. Norsk. Entomol. Tidsskr. 11:191-223.
Stern, N. J., B. Wojton, and K. Kwiatek. 1992. A differential selective medium and dry-ice generated atmosphere for recovery of Campylobacter jejuni. J. Food Prot. 55:514-517.
Szalanski, A.L., C.B. Owens, T. McKay and C.D. Steelman. 2004. Detection of Campylobacter and Escherichia coli O157:H7 from filth flies by polymerase chain reaction. Med. Vet. Entomol. 18:241-246.
Thomas, G.D., and S.R. Skoda. [eds.]. 1993. Rural flies in the urban environment? Proceedings of a symposium presented at the Annual Meeting of the Entomological Society of America, December, 1989 San Antonio, Texas. Agricultural Research Division, Institute of Agriculture and Natural Resources, University of Nebraska-Lincoln, Lincoln, Neb.
Voegtline, A.C., G.W. Ozburn, and G.D. Gill. 1965. The relation of weather to biting activity of Stomoxys calcitrans (L.) along Lake Superior. Papers Mich. Acad. Sci. Arts Letters. 50: 107-114.
Wellington, W.G. 1945. Conditions governing the distribution of insects in the free atmosphere. I-IV. The Canadian Entomologist. 77: 7-15, 21-28, 44-49, 69-74.
Winpisinger, K.A., A.K. Ferketich, R.L. Berry, and M.L. Moeschberger. 2005. Spread of Musca domestica (Diptera: Muscidae), from two caged layer facilities to neighboring residences in rural Ohio. J. Med. Entomol. 42: 732-73