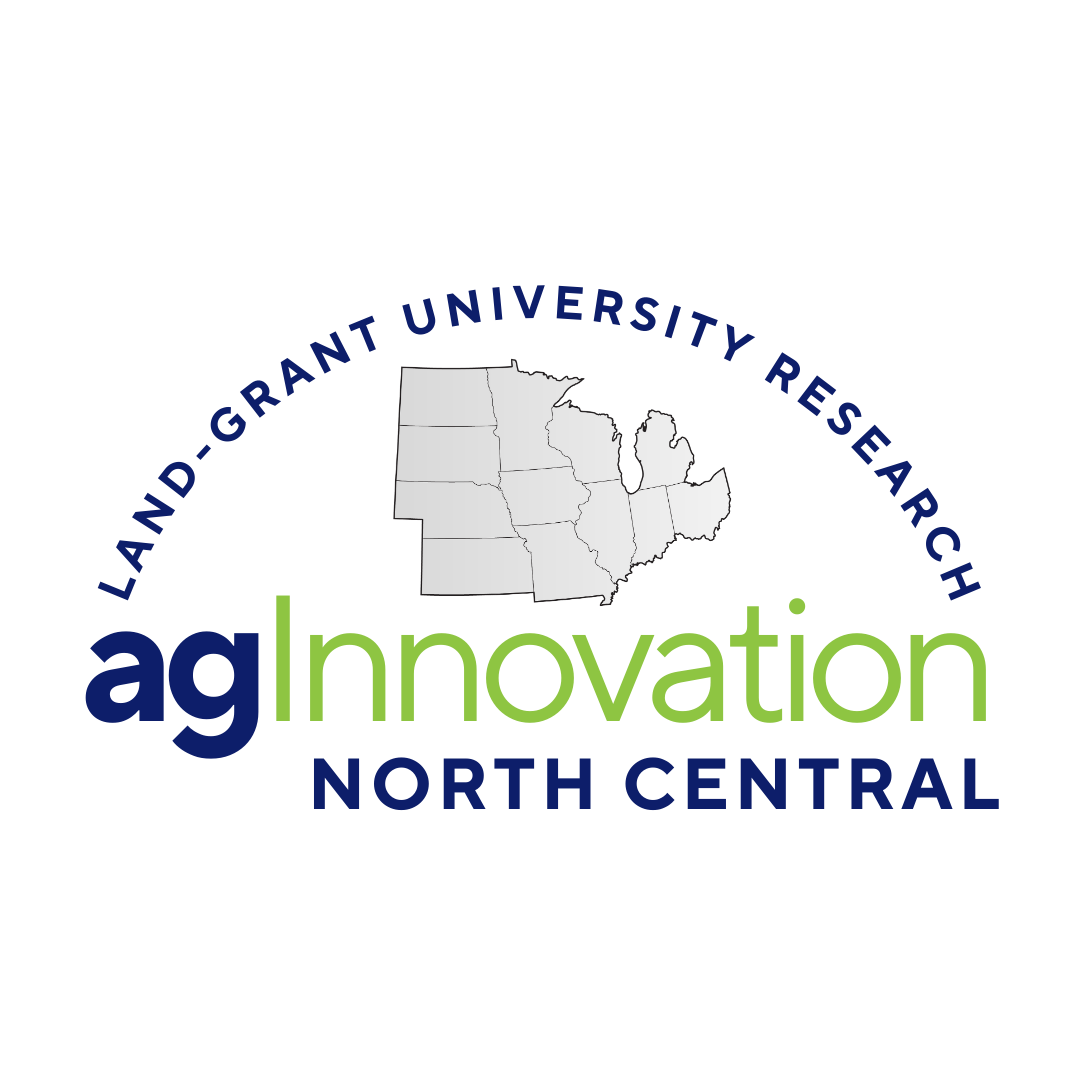
NC1017: Carbon Sequestration and Distribution in Soils of Eroded Landscapes (formerly NCT199 and NC174)
(Multistate Research Project)
Status: Inactive/Terminating
NC1017: Carbon Sequestration and Distribution in Soils of Eroded Landscapes (formerly NCT199 and NC174)
Duration: 10/01/2004 to 09/30/2009
Administrative Advisor(s):
NIFA Reps:
Non-Technical Summary
Statement of Issues and Justification
Soil erosion results in a considerable economic loss to landowners because of reduced productivity of eroded soil, and to society at large because of degradation of surface water by sediment and sediment deposition, and emission of greenhouse gases into the atmosphere. Crosson (1984) estimated a monetary loss in the U.S. from soil erosion effects on reduced cropland productivity to be 40 million dollars in a given year. In 2001, den Biggelaar et al. (2001) estimated the loss at $55.6 million. Others Crosson (1986) and Pimentel et al. (1995) have estimated the loss to be over $100 million. The results vary depending on the erosion rates and crop prices for the years covered in the study. For many soils, continued erosion results in degraded topsoil and continuing declines in crop yields as root restrictive layers, such as fragipans, subsoil horizons high in clay content, or coarse sand, become closer to the soil surface (Langdale et al., 1985).
Offsite damages to the environment caused by soil erosion and subsequent deposition of sediments in the U.S. are considerable (Pimentel, 1992). Deposition of eroded soil materials in surface water bodies such as reservoirs, lakes, rivers and streams cause a decline in water quality, and decrease the functional life expectancy of reservoirs. Eroded sediments often contain not only soil materials from the organic surface soil which are enriched in nutrients and C, but often include commercial and/or organic (animal waste) fertilizers, pesticides and agricultural pharmaceuticals.
In addition to water quality problems associated with soil erosion, the loss of organic-rich surface soil also impacts the global C balance. While there has been limited research on the impact of soil erosion on soil C balance, the soil is a major C sink and it is not always managed in a manner to take advantage of this potential. Previous work by members of NC-174 has demonstrated the importance of storing soil C (through increasing organic matter) for improving soil quality, including soil physical properties such as improved water holding capacity (Lal, 1999). However, a strong connection between soil erosion and the global C balance has not been well established. There is also a need for developing a good method for obtaining a quantitative estimate of the actual distribution of C on various eroded and noneroded landscapes in the Midwest. It was recognized during Kyoto Protocol that net emissions of greenhouse gases, such as CO2 and CH4 could be decreased by either reducing emissions or by increasing the rate of C sequestration in soils. Agricultural soils are one of the largest reservoirs of C, and thus have a great potential to mitigate the increasing concentration of CO2 in the atmosphere (FAO, 2001). Evaluation of the C pool in soils is difficult because of its heterogeneity in time and space (FAO, 2001). The global loss of C because of erosion is estimated to be in the range of 150 to 1500 million tons per year (Lal, 1995; Gregorich et al., 1998; Lal, 2003) but the processes are not well understood and we note that these values are only estimates. Erosion is a selective process involving detachment and transport of the light soil fraction consisting of soil organic carbon (SOC) and clay. The fate of eroded soil particles is complex depending on many parameters including soil properties, landscape elements and properties, and drainage net and soil management. Many of the soil particles eroded are moved downslope and may remain in the same field or watershed for a considerable length of time. However, this movement results in increased spatial variability of the landscape soil properties, especially soil organic matter and those elements of environmental concern that are associated with it - carbon and nitrogen (Schumacher et al., 1999).
Federal agencies, such as the Natural Resources Conservation Service (NRCS), state agencies involved in natural resource conservation and management, certified crop advisors, farm managers, experiment station research units, farmers, and many others have a mutual interest in better understanding the relationships among soils and landscapes as related to erosion and the effects of erosion on the amount and distribution of C in soils under different management systems. Better understanding of these relationships will improve environmental protection, soil, air, and water quality, and global warming. In addition, the research will lead to improved efficiency in use of farmer dollars in nutrients applied to the land.
This proposal outlines a project designed to help better understand C losses including soil degradation resulting from accelerated erosion and the effects of erosion on quantitative distribution of C on soil landscape. It will provide needed data on the changes in the C reservoir related to intensive land use for some of the major soils in the NC region. This study will contribute to our understanding of soil-landscape processes and has the potential to provide data that will contribute to improved management of our soil and water resources. We view this approach as a natural progression related to the past research efforts of NC-174.
Knowledge gained from the proposed research will contribute to a more quantitative understanding of agroecosystems on global C balance and increase to our understanding of the effects of erosional processes on the amounts and landscape distribution of C and organic matter. It will contribute to our knowledge leading to sustainable management of natural resources in different ecosystems.
The North Central Regional Association of Agricultural Experiment Stations has a current list of research priorities for seven cross-cutting research areas and objectives. The proposed Regional Project addresses four of these objectives for the Natural Resources and the Environment. These include: (1) Understand the ecological processes operating in human, plant and animal communities, (2) Define sustainable principles for resource management, utilization and land use, (3) Identify and apply ecosystem management principles and practices for the utilization and protection of resources, restoration of natural systems and management of rural landscapes, and (4) Assess the relationships of agricultural/forestry practices (primary production) upon soil and water systems and bio-diversity. Our project will contribute to these research priorities.