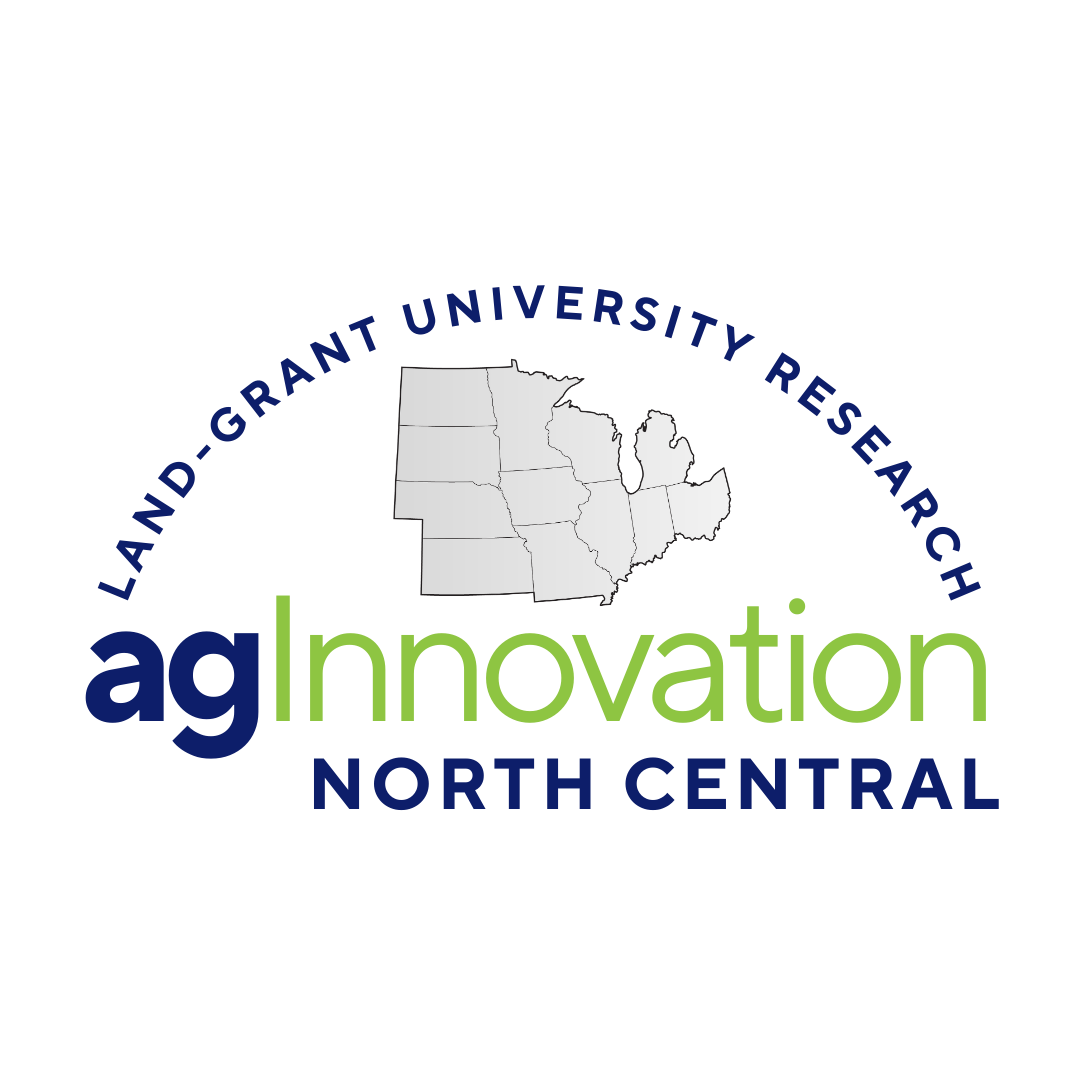
NC1167: N-3 Polyunsaturated Fatty Acids and Human Health and Disease (NC167)
(Multistate Research Project)
Status: Inactive/Terminating
NC1167: N-3 Polyunsaturated Fatty Acids and Human Health and Disease (NC167)
Duration: 10/01/2002 to 09/30/2007
Administrative Advisor(s):
NIFA Reps:
Non-Technical Summary
Statement of Issues and Justification
The need as indicated by stakeholders: The Problem: Americans are unlikely to achieve the proposed dietary recommendations of increased long chain n-3 polyunsaturated fatty acids (PUFA) by increasing fish consumption, AHA Dietary Guidelines Revision 2000 (1). For example, there is no evidence that the public would modify their dietary patterns to include a suggested four servings of fatty fish weekly and the supply of fatty fish is insufficient to meet these proposed recommendations. Therefore, agricultural-based sources of n-3 PUFA must be investigated as an alternative means of achieving equivalent health benefits (health promotion/disease prevention). However, the family of n-3 PUFA comprises a heterogeneous group of fatty acids with potentially diverse health-related functions that remains to be investigated. The overall goal of this application is to determine the effectiveness of agricultural-based n-3 PUFA as compared to marine-based n-3 PUFA to promote health and prevent disease and identify agricultural and marine sources of n-3 PUFA to meet new dietary guidelines.
This project addresses the "highest priority research" objectives as defined by the Agricultural Research, Extension and Education reform Act of 1998. This project also addresses Goal 3, Objective 3.1 (to optimize the health of consumers by improving the quality of diets, the quality of food, and the number of food choices) and Objective 3.2 (to promote health, safety, and access to quality health care) of the CSREES Strategic Plan. This project addresses three Food and Nutrition research priorities identified by the North Central Regional Association (NCRA) in Appendix A-1 of the NCRA manual, and as such, its focus on Food and Nutrition is among the highest priorities. The three priority research objectives to be addressed by NC-1167 include:
Priority Research Objective 1: Expand our understanding of the relationship between diet, health, and disease prevention with particular focus on dietary lipids.
Priority Research Objective 2: Elucidate health benefits associated with functional properties of food constituents.
Dietary guidelines for fat intakes were originally based on the formulae that predicted the impact of polyunsaturated fatty acids (PUFA), saturated fatty acids (SFA) and monounsaturated fatty acid (MUFA) on serum cholesterol levels as the cardinal biomarker of cardiovascular disease (CVD) risk (1). There has been an evolution in the dietary guidelines over the 1988-2000 period, which recognizes that while PUFA are associated with a reduction in serum cholesterol and CVD risk, not all PUFA are created equal with regards to their beneficial effects. For example, excessive consumption of n-6 PUFA is not without detrimental effects (1-3). The 2000 Revision of the American Heart Association (AHA) dietary guidelines (1) has abandoned the nutrient-based PUFA recommendation in favor of food-based recommendations of unsaturated fat consumption from fish, vegetables, legumes and nuts. These new guidelines emphasize that n-3 PUFA confer benefits over and above those attributable to improved serum lipid profiles. Despite this, consumers are unaware as to how much n-3 PUFA should be consumed, either in terms of absolute amounts, the forms of dietary n-3 PUFA, or the importance of the dietary n-3/n-6 ratio. Furthermore, consumers are unaware that different forms of dietary PUFA have differing health benefits based on their ability to impact the functional tissue long chain n-3 PUFAs, eicosapentaenoic (EPA) and docosahexaenoic acids (DHA).
Priority Research Objective 3: Design effective nutrition education programs and delivery methods that modify human behavior such that individuals, including those most at risk, choose healthier diets.
This project will enable construction of a profile that will delineate the practices used by nutrition professionals to improve consumers' n-3 intake. By profiling the n-3 counseling practices of registered dietitians, a snapshot of practitioner perceived effectiveness of altered dietary n-3 intake can be formed for conditions including but not limited to cardiovascular disease, stroke, arthritis symptoms, autoimmune disorders, neurological functions, cancer, pregnancy and birth outcomes. Then, utilizing the profile components, effective, theory-driven nutrition education programs and intervention strategies, including delivery methods, will be developed for nutrition professionals with possible adaptation for other health professions. Ultimately, this targeted nutrition education will result in improved n-3 intakes in consumers.
Importance of the work: Recommended n-3 intakes will be published for the first time by the Food and Nutrition Board, National Academy of Sciences, in 2002 and are likely to be well above current intakes. Nutrition professionals will need valid resources to implement the recommendations. Development of sound, food-based nutrition resources will 1) demonstrate the role of agricultural products in increasing n-3 intake to maintain health, 2) promote food, rather than supplement-based sources of n-3 fatty acids, and 3) ensure dissemination of accurate science-based information about n-3 fatty acids and health for health professionals and their clients. Members of NC-1167 will focus their efforts on identifying both the dietary n-3 forms and amounts that confer health promotion/disease prevention benefits.
NC-1167 maintains the focus of its predecessor projects in delineating the impact of dietary PUFA on the health of the US population with an emphasis on identifying the health benefits of the various dietary forms of n-3 PUFA, and the amounts of n-3 PUFA, as a means of establishing guidelines that will aid in setting human recommendations. NC-1167 scientists were among the first to recognize that diets formulated with n-6 PUFA-rich corn oil or safflower oil resulted in markedly elevated eicosanoid formation, particularly 2-series prostaglandins (PG) and 4-series leukotrienes (LT) while 3-series PGs and 5-series LTs were depressed (LA, CO, OH, IA, TN Stations). NC-1167 scientists have shown that aberrant eicosanoid production with high n-6 PUFA inhibits bone formation (IN, CO), and that n-3 PUFA moderates bone resorption and promotes bone formation. The NC-1167 group was among the first to report that high n-6 PUFA diets promote tumor growth (IA, WI, MN, TN) and that n-3 PUFA inhibited tumor proliferation (TN, MN). Other consequences of high n-6 PUFA diets elucidated by the NC-1167 group include increased low density lipoprotein (LDL) oxidation (NC), increased cytokine production and alterations in immune function (MO) and decreased receptor binding affinity and tissue sensitivity to insulin affecting energy metabolism (MI). NC-1167 studies have shown that increased n-3 PUFA can decrease triglyceride rich lipoproteins and CVD risk (CA, NE and TN). Recent studies (CO) have found elevations of n-6 PUFA in blood lipids of women delivering prematurely and increased n-3 PUFA decreased biomarkers of premature delivery (CO, IN). Infertility is associated with high n-6 PUFA diets, and n-3 PUFA consumption increases egg release into the oviduct (WY).
Alterations in the eating pattern of Americans have resulted in a markedly increased use of processed plant fats and oils in comparison to animal fats. PUFAs comprised approximately 7% of energy intake of US diets during the period 1987-1991 (4-6). Linoleic acid ( LA, n-6 PUFA) is the predominant PUFA in the American food supply and the average daily consumption between 1987 and 1991 was approximately 13 g/day, providing 84-89% of the total PUFA energy consumed (4,5). Thus, approximately 6% of daily energy is provided by n-6 LA acid alone. In contrast, the intake of n-3 PUFA during the 1987-1991 time period was approximately 1.6 g/day, providing approximately 0.7% of daily calories. (4-6). The major contributor to n-3 PUFA intake is -linolenic acid (LnA, 1.4 g/day, 0.6% of daily calories) with 0.1-0.2 g/day (0.05-0.1% daily calories) provided as EPA plus DHA, the functional long-chain n-3 PUFAs (4-6). Despite its high LA content, soybean oil is the major dietary source of LnA (6), but 50% is processed by hydrogenation for use in margarines, shortenings, and frying fats with increased trans fatty acid content and drastically reduced n-3 PUFA (7). The role of soybean oil as the major dietary source of n-3 PUFA in the US food supply is threatened by efforts to develop low n-3 PUFA cultivars in order to circumvent hydrogenation and assuage consumer concerns about dietary trans fatty acids (5,6,8). The conversion of dietary LnA acid to EPA and DHA, the functional n-3 PUFA in tissues, is dependent on both the amount of LnA and the dietary n-3/n-6 ratio (9). As emphasized previously, the optimal level of dietary n-6 PUFA, for wellness factors other than serum cholesterol, has yet to be determined. Work by NC-1167 scientists has shown that there are threshold levels of n-6 PUFA with increased risks for coronary thrombosis, cancer proliferation, immunosuppression, bone loss, infertility, premature delivery and perinatal health, and modest supplements of n-3 PUFA can reverse many of the detrimental effects of elevated n-6 PUFA consumption.
A crucial, and unanswered, question is whether dietary LnA acid can support tissue EPA and DHA levels at which health outcomes are improved. Evidence indicates that the current US dietary n-6/n-3 ratio of 11-12.5:1 does not allow for sufficient conversion of LnA to optimal tissue levels of EPA and DHA (6,9). The dietary n-6/n-3 ratio has remained largely unchanged over the past 40 years (10-12) and is considerably higher than the suggested optimal ratio of 2.3:1 (6). This has led to recommendations that US fish consumption be increased to approximately 4 servings of fatty fish per week in order that preformed dietary EPA and DHA intakes the functional tissue forms of n-3 PUFAs - meet the proposed daily goal of 0.65 g EPA plus DHA (13). Americans are unlikely to achieve the proposed dietary recommendations of long-chain n-3 PUFA by increasing fish consumption. There is no evidence that the public would modify their dietary patterns to include a suggested four servings of fatty fish weekly (6) and there is concern that the supply of fatty fish is insufficient to meet these proposed recommendations. The Food and Drug Administration has recently cautioned consumers to limit fish consumption because of concern with environmental heavy metal and pesticide contamination. Thus, the investigation of agricultural-based sources of dietary n-3 PUFA as alternatives to marine based n-3 PUFA for achieving health promotion and disease prevention benefits in the US population is crucial. While no single station has the expertise to investigate all the n-3 PUFA linked wellness outcomes, the expertise of the NC-1167 Committee ensures that our search for an appropriate dietary n-3 PUFA intake, both form, amount, and useful consumer information will focus on major health outcomes such as CVD, cancer, bone diseases, prematurity and perinatal health, emphasized in the Health People 2010 Report (14).
Consequences if not done: Without this research, the scientific rationale supporting the levels and types of dietary n-3 PUFA needed to reduce risk of chronic diseases, such as cancer inflammatory and cardiovascular diseases, will remain a matter of interpretation. This is important because of the prevalence of these diseases in the American population and their serious impact on the healthcare economy. It is imperative that we not lump all the n-3 fatty acids together with the assumption that they have similar biological properties.
Equally, it is a mistake to assume that it is sufficient to recommend more fish in the diet with little hope that these recommendations are achievable. The most effective methods to implement dietary behavior change are being rigorously studied, particularly in relation to reducing total fat intake. However, these educational theories have not been applied to the area of n-3 fatty acid intake and without this data success is questionable. In addition, education to increase intake of n-3 fatty acids is further complicated because it contradicts previous nutrition communications recommending dietary fat reduction.
Therefore, without these studies, n-3 PUFA as a tool for the health promotion and disease prevention will remain an interesting curiosity to the biomedical community. There is a need to determine how much and what type will result in a predictable outcomes and how to educate and motivate the public to achieve these desired outcomes.
Technical feasibility of the research: The scientists that make up the technical committee on NC-1167 are the leading authorities in their respective disciplines as it relates to the biological impact of dietary n-3 fatty acids. A review of annual reports and their publication record provides the basis for this statement. The methods outlined in this proposal are standard procedures developed and perfected by each of the investigators. As a result, each member provides a unique perspective to the problem, when seamlessly blended, will result in definitive answers. This applies to the natural as well as the social science portions of the proposal. For example, regarding the nutrition education component, this project is feasible because information will be sought from nutrition professionals who are highly organized into state and local districts. They meet on a regular basis and seek continuing education opportunities to maintain credentials. Specialized practice among nutrition professionals is common, thereby enabling us to identify practices specific to the diseases of interest. Development of an educational intervention is possible because members of the NC-1167 regional project possess n-3 fatty acid expertise and nutrition education experience, assuring scientific validity for both content and delivery components.
Advantages for doing the work as a multistate effort: The NC-1167 Research Project addresses the role of dietary n-3 PUFA in the promotion of health. No single station has the expertise or resources to investigate all the components needed to establish health driven guidelines for n-3 intakes. We will leverage our collective results into an integrative recommendation outlining the types and levels of n-3 fatty acids and their potential outcomes. We will use this information in conjunction with the nutrition education component as a means to increase n-3 PUFA consumption by Americans and to insure that the amount and types of n-3 PUFA are biologically meaningful. To accomplish this, data have to be generated using different levels and types of n-3 PUFA under a variety of experimental conditions with multiple clinical and biochemical endpoints involving conditions such as cardiovascular disease (TN, NC, CO), cancer (TN, MN), inflammation (MI, MO), diabetes (WY, MI, ND), reproductive issues (CO, WY) and bone metabolism (IN, CO). Similarly, a multistate effort (as directed by CO, KS, NE, NJ) makes it more technically feasible to have a large enough sample size of nutrition professionals for the nutrition education component. Intake of foods containing n-3 PUFA may vary by geographic locations, cultural background and demographic characteristics. A multistate effort provides the opportunity to address issues related to demographics, geography and ethnic diversity. Another advantage is the availability of a broad scope of technical and educational expertise for use in designing the intervention. A multistate effort will result in a larger sample size for the intervention. This will mean greater feedback on the nutrition education intervention practices and the ability to make statistically relevant group comparisons. This vigorous feedback will increase the likelihood that nutrition education programs designed to increase n-3 PUFA intake will be efficacious.
Likely impacts from successful completion of the work: Biomarkers will be identified thereby enabling successful educational interventions to be documented. Effective educational programs that increase nutrition practitioner n-3 PUFA counseling will increase consumption of food-based sources of n-3 fatty acids to improve the quality of life and help reduce diseases, such as heart disease and cancer. The resulting economic impacts include decreasing healthcare costs and those related to an increased need for production of agriculture-based n-3 fatty acid sources, such as flaxseed, stearadonic (SDA)-containing vegetable oils and n-3 enriched animal products. The potential for negative health and economic consequences of excessive and unsafe n-3 supplementation practices will be reduced.
Related, Current and Previous Work
NC-1167 members searched the literature in preparation for writing this section of the proposal. In addition, Dr. Melvin M. Mathias (USDA, CSREES) searched the CRIS database with a focus on polyunsaturated fatty acid, n-3 and n-6 fatty acids, linoleic and linolenic acids and fish oil. Of the sixty eight USDA CRIS projects identified in this search, twenty four were conducted by NC 1167 investigators and seven NC 1167 scientists were supported by competitive USDA funding. In addition, the ARS supports five laboratory groups in this area.
Landmark studies in the 1950s and 1960s identified SFA and PUFA as the dietary component with the greatest influence on CVD. The dietary emphasis on grouping fatty acids into classes, SFA, MUFA and PUFA, based on their cardiovascular effects has overlooked the importance of PUFA classes on many other aspects of wellness.
Dietary intakes and nutrition education intervention: Studies of Midwest populations show that intakes of n-3 fatty acids are low, particularly among at-risk groups. The mean omega-3 fatty acid consumption in women with a history of breast cancer was 610 mg/day (85% LnA, 5% EPA, 10 % DHA)(15). In a separate study, women of childbearing age (n=67) consumed 590 mg/d of n-3 fatty acids. Demographic characteristics of income and education were associated with variation in n-3 fatty acid intake with higher intakes associated with lower income and higher education levels. The highest intake documented was 890 mg/d for women with a bachelor degree or higher and the lowest intakes (390 mg/d) were in women with a high school education or lower (16). A third study documented consumption of n-3 fatty acids in low-income pregnant women (n=30). N-3 fatty acid intake was 1.06 g/day (alpha linolenic 93%, EPA; 2%, and DHA 5%) (17).
In a population of hypercholesterolemic individuals (n=25) where total fat consumption was low (21.5% of energy), n-3 fatty acid intake was 620 mg/d, approximately 0.3 % of calories (18). In another population, physically active adults (n=13) with a much higher energy intake (3015 1 161 kcals/day), fat intake was 22 percent of energy and total n-3 fatty acids intake was 887 mg/day, which is approximately 0.26 % of calories. The n-6:n-3 ratio in the diet was 11.1 (19). The proposed project will address the identified problems through the development of effective nutrition educational interventions that will ultimately lead to increased n-3 fatty acid intakes. A theory-driven educational intervention is necessary for an effective nutrition education program (20). Some nutrition education interventions are based on only one educational theory, such as the transtheoretical stage of change (21) or Social Cognitive Theory (22). An eclectic approach for theory utilization in health education has been recommended (20). Development of a nutrition education intervention requires knowledge of current practice. A survey of British dietitians to determine dietetic practices related to secondary prevention of myocardial infarction revealed that 45 percent of the 138 respondents gave dietary advice to increase oily fish intake (23). A similar study in America has not been reported and the medical nutrition therapy protocol for hyperlipidemia developed by the American Dietetic Association does not address the topic of n-3 fat intake (24). Comparative nutrition education research in The Netherlands, which included an aim for increased fish consumption, revealed the need to devise special materials to effect change rather than relying on general information encouraging a healthy diet (25).
Role of n-3/n-6 PUFA in Cardiovascular Disease: Cardiovascular disease (CVD) remains the leading cause of death in the United States and accounts for the major portion of health care costs (14). Both dietary and pharmacological approaches to lowering blood cholesterol and LDL cholesterol have been exhaustively investigated. Early studies identified n-6 LA as the major LDL-C lowering PUFA in US diets, but many studies have shown that when consumed in amounts sufficient to lower LDL-C, LA also lowers cardioprotective HDL-C. The prevailing concept that LA has unique hypocholesterolemic and cardioprotective effects has been challenged by studies showing populations consuming n-3 PUFA rich diets also had a much lower incidence of heart disease, and that n-3 PUFA also conferred additional cardioprotective benefits (27,27). The pronounced effect of n-3 PUFA on hyperlipidemia is well documented in studies where its effects are compared to both SFA and n-6 rich vegetable oil PUFA (26-30). N-3 PUFA has shown to reduce plasma triglycerides, postprandial lipemia, remnant concentrations and to inhibit triglyceride, apolipoprotein B48 and B100 synthesis in intestines and liver (18,27,28,31-33). In contrast to LA rich diets, dietary n-3 PUFA raises HDL-C (27) Dietary n-3 PUFA also prevents CVD through other diverse mechanisms such as arrhythmia prevention, inhibition of cytokine and mitogen production, increased endothelial-derived nitric oxide production, and reductions in proinflammatory eicosanoids (26,34). Several recent clinical studies have emphasized the importance of n-3 PUFA in reducing cardiovascular disease. (35-37).
Oxidized LDL accumulation by macrophage scavenger receptors leads to endothelial disruption as the first stage of hyperlipemia mediated atherogenesis (38-40). This has lead to the concern that despite their favorable effects on lipid profiles, diets relatively high in n-3 PUFA could increase in vivo oxidation and the oxidative susceptibility of LDL, thereby negating some of their cardioprotective effects. However, these studies are contradictory (41-45). In addition, LDL oxidative susceptibility studies have not resulted in support of the idea that the higher the degree of unsaturation of dietary PUFA, the greater the susceptibility of LDL to oxidation (46). In a recent human study by NC Station, postmenopausal women were provided diets rich in oleic, LA or fish oil containing both EPA and DHA. Plasma free F2-isoprostanes and malondialdehyde were lower after fish oil supplementation compared to the high LA diet. While thiobarbituric acid reactive substances were higher in the fish oil group, maximal rates of formation and concentrations of LDL phospholipid and cholesteryl ester hydroperoxides were lower. Taken together, the results suggest fish oil supplementation does not increase overall in vivo oxidation or oxidation of LDL ex vivo. Consequently, health benefits related to increased fish consumption do not appear to be offset by increased in vivo oxidation or LDL oxidative susceptibility. Further studies are needed to define the impact of n-3 PUFA, both dietary forms and amounts, on LDL oxidative susceptibility and cardioprotection.
Atherosclerosis has many of the attributes of an inflammatory disease, particularly in its early stages as monocytes/macrophages are activated (40). Inducible cyclooxygenase (COX-2) is over-expressed in sites of inflammation and in many tumor tissues (47). Activation of Toll-like receptor 4, the lipopolysaccharide receptor of macrophages, induces nuclear factor kappa B (NFB) activation and COX-2 expression. Recent work has shown that SFAs, but not unsaturated FAs, induce NFB activation and COX-2 expression in macrophages, and unsaturated FAs inhibit the COX-2 expression caused by SFAs (48). These results represent a novel, and important mechanism by which free fatty acids can activate monocytes/macrophages and COX-2 expression. Since free fatty acid pools are influenced by the type of dietary fat consumed, these results suggest a direct modulation of inflammation by dietary fat choices that may influence signaling pathways involved in CVD and tumor proliferation (48). Further studies on n-3 and n-6 PUFA, forms of n-3 PUFA and n-6/n-3 ratios are planned to delineate the inflammatory response to dietary fat choices.
AHA guidelines (1) recognize that in some individuals making food choices of high carbohydrate diets in order to reduce fat intakes can induce high circulating triglycerides that may contribute to CVD. Hypertriglyceridemia is often associated with decreased HDL-C, particularly in the cluster of metabolic risk factors collectively known as Metabolic Syndrome (Syndrome X, central body obesity) (49). Consumption of n-3 enriched eggs (300 mg LnA and 100 mg DHA per egg) by hypercholesterolemic individuals fed a low fat, low saturated fat diet decreased triglycerides by 16% and was without effect on HDL-C (18). Feeding fish oil diets to triglyceridemic subjects significantly depressed postprandial apoB100 and apo B48, very low density lipoprotein (VLDL), triglycerides and increased large, less atherogenic, LDL particles (33). The results of these studies suggest that dietary preformed long chain n-3 fatty acids may have cardioprotective effects not evident with LnA acid feeding. These studies point to the need to delineate the apparent differences in LnA and other agriculture based n-3 PUFA versus EPA/DHA on triglyceridemia, HDL-C levels and cardioprotection.
The consumption of high fat diets has been associated with the development of insulin resistance. Insulin resistance is associated with increased CVD, hypertension, dyslipoproteinemia, glucose intolerance and with obesity - the cluster of metabolic alteration described as Metabolic Syndrome (49). Preliminary evidence (MI Station) suggests that n-3 PUFA may prevent the development of insulin resistance. Mice fed n-3 PUFA (fish oil) had lower plasma insulin concentrations than mice fed a diet enriched in n-6 PUFA (corn oil). Islets from mice fed the n-3 and n-6 PUFA diets secreted similar amounts of insulin in response to glucose and acetylcholine. It appears that the beneficial effects of dietary n-3 PUFA on insulin action involve changes in peripheral sensitivity to insulin rather than changes in regulation of insulin secretion per se (50,51). These results emphasize the need for studies of long chain n-3 PUFA versus LnA and other agricultural based PUFA in the management of hypertriglyceridemia and insulin resistance.
Roles of n-3 and n-6 PUFA in cancer: Cancer is the second most common cause of death in the US (14). Colon cancer has the fourth most common incidence and second highest mortality rate of cancers in the United States. Diet, particularly dietary fat, has been implicated in explaining differences in international incidence rates. Fish oils, rich in n-3 fatty acids, have chemoprotective properties against colon cancer. In a study of 24 European countries, an inverse association between colorectal cancer mortality and current fish intake has been noted (52). In carcinogen-treated animals, high fish oil diets reduce the number of colon tumors (53,54) when fed solely in the initiation phase or in the postinitiation phase (55).
Feeding a 10% flaxseed oil (56% of fatty acids as LnA) diet reduced mammary tumor growth and metastasis in mice (56). Feeding a 1.8% flaxseed oil diet to mice reduced the tumor volume of established mammary tumors, but new tumor volume was unaffected (57). In rats treated with dimethylhydrazine, there was an 18% colon tumor incidence in animals fed 10% perilla oil (58% of fatty acids as LnA) diets compared to 47% and 41% tumor incidence, respectively, in animals fed 10% safflower or soybean oil diets (58). The number of aberrant crypt foci, a measure of precancerous lesions, in N-methyl-N-nitrosourea treated rats decreased in a linear fashion as diet perilla oil was increased from 0% to 12% (59,60). Preliminary studies (MN Station) have shown feeding 11% flax oil diets to rats significantly reduced aberrant crypts only when fed before and during carcinogen administration, i.e. the initiation phase of carcinogenesis, but not when this diet was fed during both the initiation and post-initiation phases. This suggests that LnA may alter initiation effects, such as reducing carcinogen activation or enhancing DNA repair, but at later stages may actually tend to promote the development of colon cancer.
Supplementation of n-3 LnA to a diet formulated to mimic a Western-type diet had no effect on tumorigenesis in mice that spontaneously develop intestinal tumors, but the more highly unsaturated n-3 fatty acids, stearadonic acid (SDA), EPA and DHA, significantly reduced tumor load by ~50% (61). The n-6 fatty acids LA, -linolenic acid (GLA) and AA had no effect on intestinal tumorigenesis, but supplementation of AA to EPA-containing diets rescued regressing tumors (61-63). Tumor inhibition appear to require antagonism of AA, and fatty acids with the greatest ability to antagonize AA (n-3 PUFA with 4 or more double bonds) may have the greatest impact on reducing intestinal tumor load (61,62,64-69). COX-2-derived PGE2 is important for activating downstream growth factors, such as vascular endothelial growth factor (VEGF), and that the initial up-regulation of COX-2 by tumor stromal cells inaugurates this signaling pathway. These studies suggest that the types of dietary n-3 PUFA most effective in tumor suppression are those that reduce PGE2 formation, either by reducing tissue AA content, down-regulating COX-2 expression or inhibiting COX-2 activity. Further studies are planned to determine the optimal n-3 PUFA for intestinal cancer inhibition. Hepatic tumorogenesis induced by fumonisin B1 increases hepatic PGE2 and PGF2 production and decreases natural killer cell activity. Feeding a 7% or 14% fish oil diet (menhaden oil) suppressed carcinogenesis and this suppression of was not explained by reductions in PGE2 alone (70,71). The results suggest that n-3 PUFA may act by altering natural killer cell activity (70).
Roles of n-3 and n-6 PUFA in inflammation: Approximately 25% of children in the Western world experience wheezing at some point in their life, with over 10 million individuals being screened annually for asthma. It appears that the 50% of the asthmatic population that achieves a benefit with fish oil ingestion does so by altering their leukotriene (LT) profile by elevating 5-series LT and reducing 4-series LT (72). Recent work has shown that EPA and DHA administration can reduce in vivo interleukin (IL)-12 and interferon-gamma (INF) production during an infectious challenge in mice (73-75). The current view for how fatty acids affect the immune system is centered around the ability of n-3 PUFA to diminish cytokine production and secondarily to alter eicosanoid production. For example, the consumption of fish oil has been found to decrease either ex vivo production of or circulating levels of several pro-inflammatory cytokines, such as interleukin (IL)-1, IL-6 and tumor necrosis factor (TNF) in patients with inflammatory disease and cancer (75). However, recent work with laboratory animals has shown that EPA and DHA administration can reduce IL-12 and interferon-gamma (IFN) production during an infection (73). The net effect of high n-3 PUFA intake on these mice is impaired bacterial clearance and reduced survival upon challenge with the food-borne pathogen Listeria monocytogenes (76). These effects on the immune system point to the need to determine the optimal n-3 PUFA intakes for health promotion while monitoring the potential adverse effects on infectious disease resistance.
Roles of n-3 and n-6 PUFA in bone formation: Nearly 20% of people over 50 years of age suffer from osteoporosis with over 1.5 million fractures per year attributable to this skeletal disease (14). Health care costs for osteoporosis and bone fractures were estimated at $13.8 billion per year in 1996 (14). Skeletal biology is controlled by cells of the bone microenvironment through the actions of PGs and cytokines involved in the local regulation of bone metabolism, and the actions of specific dietary PUFA have been described that impact skeletal metabolism (77,78). N-3 PUFA modulate eicosanoid biosynthesis in numerous tissues and cell types, alter signal transduction, and influence gene expression and is linked to increased bone formation in growing rats and osteoblastic activity in cell culture (79,80). Ex vivo PGE2 production was higher in rats given a diet high in n-6 PUFA (n-6/n-3 = 7.3) and serum IGF-I concentration was suppressed compared with those in the high n-3 PUFA treatment (n-6/n-3 = 1.8) and rats fed diets high in n-6 PUFA had a lower ash weight/mm bone length in humeri compared with those fed n-3 PUFA (81). These findings suggest that excessive consumption of n-6 PUFA could have a negative effect on bone metabolism by increasing PGE2 mediated bone resorption. PGE2 production in rat bone organ culture was significantly reduced by diets providing a lower dietary ratio of n-6/n-3 fatty acids (n-6/n-3 = 1.2 ~ 2.6) compared with a higher ratio (n-6/n-3 = 10 ~ 24) (82). Serum bone-specific alkaline phosphatase activity was greater in rats given a diet high in n-3 fatty acids (82). The results suggest n-3 PUFA inhibits bone resorption and favors bone formation. n-3 PUFA supplements may help maintain bone mineral content after menopause in women, and n-3 PUFA rich diets may aid in the building and maintenance of a healthy skeleton in the US population. Studies are planned to determine the optimal form and amount of n-3 PUFA needed for skeletal health and bone mass maintenance.
Roles on n-3 and n-6 PUFA in perinatal health: Birth weight and gestational age at birth are critical determinants of infant morbidity and mortality. Preterm birth resulting in low birth weight (LBW) comprises 6-10% of infant births and causes many health problems in LBW infants (83). Short gestation and LBW account for 20% of neonatal deaths (14). Supplementation with long-chain n-3 PUFA increases the length of gestation in animals and humans. DHA infusion increases time to delivery in betamethasone induced preterm labor in pregnant ewes (84). Fish oil supplementation increases gestational length in pregnant rats (85-87), with changes in PG production in reproductive tissues (86,87). In humans, supplementation with fish oil has been shown to increase the length of gestation (88-90) and to decrease the preterm delivery rate in high-risk pregnancies from 33% to 21% (91). Women who delivered prematurely have markedly elevated red blood (RBC) cell n-6 fatty acids, AA and LA, with depressed n-3 fatty acids, particularly DHA, and a 9-fold elevation of RBC n-6 docosapentaenoic acid (DPA), an indicator of n-3 deficiency (92). The dietary n6:n3 fatty acid ratio and the amount of long-chain n-3 PUFA may regulate uterine contraction through modulation of AA metabolism. DHA competes with AA for incorporation into membrane phospholipid (93,61) and can be retroconverted to n-3 EPA (94) which competes with AA for COX resulting in decreased 2-series PGs production (61) and, if provided in sufficiently high amounts, synthesis of the less potent 3-series PGs and 5-series LTs. Dietary DHA depress serum MMP-2 and proMMP-9 levels in pregnant rats at 20 days gestation (95). MMPs have been implicated in idiopathic premature rupture of membranes and in cervical maturation (ripening) that occurs at delivery. MMP production is increased by elevated PGE2, and DHA suppressed both PGF2, and PGE2 in reproductive tissues (95).
There has been an increase in reproductive infertility in women consuming typical Western diets. Rat studies conducted over the past six years indicate that egg release into the oviduct can be altered with modifications of dietary fats. N-3 PUFA consumption has repeatedly been shown to increase egg release up to 1.4-fold (96) with progressive reductions in dietary n-6 PUFA resulting in an increase in egg release until a critical minimal level is attained at which point egg release is suppressed. Furthermore, LnA appears to be equally as potent as long chain n-3 PUFA in enhancing egg release and altering ovary eicosanoid synthesis. Preliminary studies (Wyoming Station) in Alaskan natives who consume high levels of fish products indicate that ovulation, as assessed by number of children born as twins or triplets, is increased with n-3 PUFA ingestion. Suppressed fecundity impacts over 8 million women in the US annually. This research indicates that increased n-3 PUFA intake may be a means of improving reproductive success in Western populations.
Objectives
-
Evaluate the effect of different n-3 fatty acids, both form (source) and amount, on tissue functions and correlate these effects with changes in putative biomarkers relevant to health promotion and disease prevention.
-
Experimental diets used in animal studies will examine dietary levels of n-3 PUFA that are achievable in human diets, based on human equivalent amounts (allometric scaling) in rodent models.
-
Develop effective means for translating research knowledge about n-3 PUFA into consumer food choices.
-
Methods
All of the experiments proposed utilize approaches, techniques, and data analyses that have been previously subjected to peer review by independent agencies such as USDA, NIH, NCI, and other non-government funding agencies such as AICR and industry. Additionally, the publication record in peer-reviewed journals is extensive for each of the Station Representatives, indicating that the methods to be used in these experiments are routinely performed in the laboratories of the respective scientists. As such, we will be emphasizing experimental designs in this text and refer the reviewers to the publications of the scientists involved for a detailed explanation of the analytical methods to be employed. Procedures to address objectives 1 and 2: N-3 PUFA are a group of structurally related lipids with 3, 4, 5 or 6 methylene-interrupted double bonds, all in the cis configuration. However, scientists of NC 1167 have data that suggest n-3 fatty acids with less than 4 double bonds may be biologically inferior to their more highly unsaturated cousins (eg., those fatty acids with 4-6 double bonds). This is of great concern because the bulk of n-3 fatty acids in the typical diet have less than four double bonds, and these fats are found almost exclusively in vegetable oils. But there are a number of alternative sources of agricultural products that contain n-3 fatty acids with more than 3 double bonds (eg., stearadonic acid, SDA), including echium oil and a new genetically modified canola oil (14-25% SDA). SDA has been shown to have equivalent biological efficacy as that of EPA and DHA and as such has been referred to as a pro-EPA compound (61), and therefore, it is important to determine whether a terrestrially-derived SDA is a viable alternative to LnA. A more subtle but equally important issue that needs to be addressed within the scope of the project is the issue of allometric scaling to human doses. This means determining doses that are used in animal diets that correspond to doses in the human diet. This is important because the levels of n-3 PUFA that are used in animal experiments are typically 20-100 times higher than what humans typically consume. This approach will allow us to tease out the differential effects of the various n-3 PUFA under more physiologically relevant conditions. Therefore, a series of experiments will be designed to directly compare the effects of n-3 PUFA with various unsaturation indexes, viz., LnA (3 double bonds) versus SDA (4 double bonds), EPA (5 double bonds) and/or DHA (6 double bonds) on a variety of health-related biochemical and physiological endpoints. The general experimental parameters will be focusing on human achievable doses with regards to form and amount. Currently, the US diet contains ~1.6 grams/day of n-3 fatty acids of which 90% is in the form of LnA and 10% as long chain n-3 PUFA. These amounts, on an energy basis (en%), are equivalent to 0.6en% and 0.1en%, respectively. Translation of these amounts to the rodent diet, as the % of energy, will provide the framework for dosing in rodents. Therefore, the human equivalent dose of LnA in a rodent diet would also be 0.6en% (or 0.3% by weight). Since the human diet can vary, we will expand our upper achievable dose of total n-3 PUFA intake by 3 fold to 2.0en%, a reasonable and achievable dietary modification. Therefore the experimental approaches will include the following:- Investigate the form of n-3 PUFA. At current n-3 intakes, what would be the effect if all of the dietary n-3 PUFA were in the form of long chain n-3 PUFA as compared to LnA? Therefore, the comparison would be LnA versus SDA, EPA or DHA at doses relevant to current human consumption (6).
- Investigate the amount of n-3 PUFA intake. The current recommendations are to increase n-3 PUFA intake but there is uncertainty as to what these values should be (1,6). Therefore, what would be the impact if increases in the amount of n-3 PUFA occurred primarily with LnA as opposed to SDA, EPA or DHA?
Measurement of Progress and Results
Outputs
- Impact of dietary n-3 PUFA, form and amounts, on biomarkers of health promotion and disease prevention.
- Increased understanding of evolving n-3 PUFA recommendations and guidelines.
- Development of a food-based n-3 PUFA database for optimal health and disease prevention.
- Data on current n-3 intakes in diverse population groups.
- Strategies to increase human n-3 PUFA intakes for optimal health and disease prevention.
- Development of materials for education and training of practicing nutrition professionals. <LI>Dissemination of data in peer-reviewed journals and scientific/professional meetings.;8; Submission of competitive grant applications to USDA (NRI and IFAFS programs) and NIH
Outcomes or Projected Impacts
- Improved health of the US population.
- Decreased incidence of age-related and/or degenerative diseases.
- Education of clients by nutrition professional about health benefits of n-3 PUFA, both forms and amounts.
- Oil processors will be introduced to the concerns health professionals have about meeting n-3 PUFA guidelines.
- Stimulating the development of alternative sources of agriculturally-based LCn-3 PUFA.
- Stimulating the development and marketing of new and creative agriculturally-based food products containing LCn-3 PUFA. <LI>Enhanced awareness by the medical community of the benefits n-3 PUFA consumption.
Milestones
(0):ion of data to be used as preliminary data for competitive grant submissions.(0):ion of competitive grant applications to USADA and NIH.
(0):ve #3 is addressed in three consecutively dependent stages: (1) Development of Nutrition Education Intervention; (2) Implementation of the Intervention; and (3) Evaluation of Outcomes.
(0):0
Projected Participation
View Appendix E: ParticipationOutreach Plan
Discussed in Objective #3. Objective 3 is, in total, an outreach plan. A chart describing information flow, basic science to application, with a time line is included in Attachments.
Organization/Governance
The Committee, comprising scientists from each participating station, the Regional Administrative Advisor and the CSREES representative will be organized and governed as specified in the North Central Regional Association (NCRA) governance guidelines:
Literature Cited
1. AHA Dietary Guidelines. Revision 2000: a statement for healthcare professionals from the Nutrition Committee of the American Heart Association. Circulation 102: 2284-2299, 2000.
2. American Heart Association. Dietary guidelines for healthy American adults: a statement for physicians and health professionals by the Nutrition Committee. Circulation 77: 721A-724A, 1988.
3. American Heart Association. Dietary guidelines for healthy American adults: a statement for health professionals from the Nutrition Committee. Circulation 94: 1795-1800, 1996.
4. Jonnalagadda, S.S., Egan, S.K., Heimbach, J.T., Harris, S.S. and Kris-Etherton, P.M. Fatty acid consumption patterns of Americans: 1987-1988 USDA Nationwide Food Consumption Survey. Nutr. Res. 15: 1767-1781, 1995.
5. Allison, D.B., Egan, S.K., Barraj, L.M., Caughman, C., Infante, M. and Heimbach, J.T. Estimated intake of trans fatty acids and other fatty acids in the US population. J. Am. Diet. Assoc. 99: 166-174, 1999.
6. Kris-Etherton, P.M., Taylor, D.S., Yu-Poth, S., Huth, P., Moriarty, K., Fishell, V., Hargrove, R.L., Zhao, G. and Etherton, T.D. Polyunsaturated fatty acids in the food chain in the United States. Am. J. Clin. Nutr. 71(suppl): 179S-188S, 2000.
7. Enig, M.G., Atal, S., Keeney, M. and Sampugna, J. Isomeric trans fatty acids in the US diet. J. Am. Coll. Nutr. 9: 471-486, 1990.
8. Perkins, F.G. Composition of soybeans and soybean products. In: Practical handbook of soybean processing and utilization, Erikson, D.R., Ed., American Oil Chemists Society (AOCS) and United Soybean Board, AOCS Press, Champaign, IL, pp22-23, 1995.
9. Emken, E.A., Adlof, R.O. and Gulley, R.M. Dietary linoleic acid influences desaturation and acylation of deuterium-labeled linoleic and ALAs in young adult males. Biochim. Biophys. Acta. 1213: 277-288, 1994.
10. Sims, J.S. and Qi, G.H. Designing poultry products using flaxseed. In: Flaxseed in human nutrition, Cunnane, S.C and Thompson, L.U., Eds., AOCS Press, pp315-333, 1995.
11. Hunter, J.E. n-3 fatty acids from vegetable oils. Am. J. Clin. Nutr. 51: 809-814, 1990.
12. Kris-Etherton, P.M., Taylor, D.S. and Yu, S. Present estimates of n-3 fatty acid intakes in the US. World Rev. Nutr. Diet. 83: 228 (Abstract), 1998.
13. Simopoulos, A.P., Leaf, A. and Salem, N. Essentiality of and recommended dietary intakes for omega-6 and omega-3 fatty acids. Ann. Nutr. Metab. 43: 127-130, 1999.
14. Healthy People 2010. US Government Printing Office, Superintendent of Documents, Pittsburgh, PA; or http://www.health.gov/healthypeople/
15. Bridger, S., Lewis, N., Boeckner, L. and Guest, J. Dietary omega-3 and omega-6 PUFA consumption in women at risk for breast cancer. FASEB J. 12 A350, 1998.
16. Carder, J. and Lewis, N. Omega-3 fatty acid intake in midwestern women of childbearing age from different income and education levels. J. Am. Diet. Assoc. 99: A88, 1999.
17. Lewis, N.M., Widga, A.C., Buck, J.S., Frederick, A. Survey of omega-3 fatty acids in diets of Midwest low-income pregnant women. J. Agromedicine 2: 49-57, 1995.
18. Lewis, N., Schalch, K. and Scheideler, S. Serum lipid response to n-3 fatty acid enriched eggs in persons with hypercholesterolemia. J. Am. Diet. Assoc. 100: 365-367, 2000.
19. Lewis, N., Sindelar, C. and Seburg, S. Serum lipid response to inclusion of omega-3 fatty acid enriched eggs in diets of physically acitive adults. FASEB J. 14: A495, 2000.
20. Kaufman, D.M., Mann, K.V. and Jennett, P.A. Teaching and learning in medical education: How theory can inform practice. Association for the Study of Medical Education. Edinburgh, UK, 2000.
21. Ma, J., Betts, N.M. and Horacek, T. Measuring stages of change for assessing readiness to increase fruit and vegetable intake. Am. J. Health Promotion 16: 88-97, 2001.
22. Anderson, E.S., Winett, R.A., Wojcik, J.R., Winett, S.G. and Bowden, T. A computerized social cognitive intervention for nutrition behavior: Direct and mediated effects on fat, fiber, fruits and vegetables, self-efficacy, and outcome expectations among food shoppers. Annal. Behavioral Med. 23: 88-100, 2001.
23. Hooper, L. Survey of UK dietetic departments: diet in secondary prevention of myocardial infarction. J. Hum. Nutr. Dietet. 14: 307-318, 2001.
24. American Dietetic Association. Hyperlipidemia, Medical Nutrition Therapy Protocol. Medical Nutrition Therapy Across the Continuum of Care. American Dietetic Association, Chicago, IL, 1996.
25. Bemelmans, W., Broer, J., deVries, J., Hulshof, K., May, J.F. and Meyboom-de Jong, B. Impact of Mediterranean diet education versus posted leaflet on dietary habits and serum cholesterol in a high risk population for cardiovascular disease. Public Health Nutrition 3: 272-283, 2000.
26. Connor, W.E. Importance of n-3 fatty acids in health and disease. Am. J. Clin. Nutr. 71(suppl): 171S-175S, 2000.
27. Nestel, P.J. Fish oil and cardiovascular disease: lipids and arterial function. Am. J. Clin. Nutr. 71(suppl): 228S-231S, 2000.
28. Harris, W.S. Fish oils and plasma lipid and lipoprotein metabolism in humans. J. Lipid Res. 30: 785-807, 1989.
29. Hwang, D.H., Chanmugam, P.S., Ryan, D.H., Boudreau, M.D., Windhauser, M.M., Tulley, R.T., Brooks, E.R and Bray, G.A. Does vegetable oil attenuate the beneficial effects of fish oil in reducing cardiovascular disease, A. J. Clin. Nutr. 66: 89-96, 1997.
30. Nordoy, A., Hatcher, L.F., Ullmann, D.L. and Connor, W.E. Individual effects of dietary saturated fatty acids and fish oil on plasma lipids and lipoproteins in normal men. Am. J. Clin. Nutr. 57: 634-639, 1993.
31. Nestel, P.J. Effects of n-3 fatty acids on lipid metabolism. Ann. Rev. Nutr. 10: 149-167, 1990.
32. Nestel, P.J., Connor, W.E., Reardon, M.F., Connor, S., Wong, S. and Boston, R. Suppression by diets rich in fish oil of very low density lipoprotein production in man. J. Clin. Invest. 74: 82-89, 1984.
33. Tinker, L.F., Parks, E.J., Behr, S.R., Schneeman, B.O. and Davis, P.A. (n-3) fatty acid supplementation in moderately hypertriglyceridemic adults changes postprandial lipid and apolipoprotein B responses to a standardized test meal. J. Nutr. 129: 1126-1134, 1999.
34. Roche, H.M. and Gibney, M.J. Effects of long-chain n-3 polyunsaturated fatty acids on fasting and postprandial triacylglycerol metabolism. Am. J. Clin. Nutr. 71(suppl): 232S-237S, 2000.
35. Burr, M.L., Fehily, A.M, Gilbert, J.F., Rogers, S., Holliday, R.M., Sweetnam, P.M., Elwood, P.C. and Deadman, N.M. Effects of changes in fat, fish and fiber intakes on death and myocardial reinfarction: diet and reinfarction trial (DART). Lancet 2: 757-761, 1989.
36. de Lorgeril, M., Renaud, S., Mamelle, N., Salen, P., Martin, J.L., Monjaud, I., Guidollet, J., Touboul, P. and Delaye, J. Mediterranean alpha-linolenic acid-rich diet in secondary prevention of coronary heart disease. Lancet 343: 1454-1459, 1994.
37. Siskovick, D.S., Raghunathan, T.E., King, I., Weinmann, s., Bovbjerg, V.E., Kushi, L., Cobb, L.A., Copass, M.K., Psaty, B.M., Lemaitre, R., Retzlaff, B. and Knopp, R.H. Dietary intake of long-chain n-3 polyunsaturated fatty acids and risk of primary arrest. Am.J. Clin. Nutr. 71(suppl): 208S-212S, 2000.
38. Esterbauer, H., Gebicki, J., Puhl, H. and Jurgens, G. The role of lipid peroxidation and antioxidants in oxidative modification of LDL. Free Radic. Biol. Med. 9: 515-540, 1992.
39. Steinberg, D. Low-density lipoprotein oxidation and its pathobiological significance. J. Biol. Chem. 272: 20963-20966, 1997.
40. Ross, R. Atherosclerosis An inflammatory disease. N. Engl. J. Med. 340: 115- 126, 1999.
41. Assman, G., Schriewer, H., Schmitz, G. and Hagele, E.O. Quantification of high-density-lipoprotein cholesterol by precipitation with phosphotungatic acid/MgCl2. Clin. Chem. 29: 2026-2030, 1983.
42. Mata, P., Alonso, R., Lopez-Farre, A., Ordovas, J.M., Lahoz, C., Garces, C., Caramelo, C., Codoceo, R., Blazquez, E. and de Oya, M. Effect of dietary fat saturation on LDL oxidation and monocyte adhesion to human endothelial cells in vitro. Arterioscler. Thromb. Vasc. Biol. 16: 1347-1355, 1996.
43. Sorensen, N.S., Marckmann, P., Hoy, C.E., van Duyvenvoorde, W. and Princen, H.M. Effect of fish-oil-enriched margarine on plasma lipids, low-density-lipoprotein particle composition, size, and susceptibility to oxidation. Am. J. Clin. Nutr. 68: 235-241, 1998.
44. Suzukawa, M., Abbey, M., Howe, P.R. and Nestel, P.J. Effect of fish oil fatty acids on low density lipoprotein size, oxidizability, and uptake by macrophages. J. Lipid Res. 36: 473-484, 1995.
45. Janero, D.R. Malondialdehyde and thiobarbituric acid reactivity as diagnostic indices of lipid peroxidation and peroxidative tissue injury. Free Radic. Biol. Med. 9: 515-540, 1990.
46. Bonanome, A., Biasia, F., De Luca, M., Munaretto, G., Biffanti, S., Pradella, M. and Pagnan, A. n-3 fatty acids do not enhance LDL susceptibility to oxidation in hypertriacylglycerolemic hemodialyzed subjects. Am. J. Clin. Nutr. 63: 261-266, 1996.
47. Rhee, S.H. and Hwang, D. Murine TOLL-like receptor 4 confers lipopolysaccharide responsiveness as determined by activation of NFB and expression of inducible cyclooxygenase. J. Biol. Chem. 275: 34035-34040, 2000.
48. Lee, J.Y., Sohn, K.H., Rhee, S.H. and Hwang, D. Saturated fatty acids, but not unsaturated fatty acids, induce the expression of cyclooxygenase-2 mediated through Toll-like receptor 4. J. Biol. Chem. 276: 16683-16689, 2001.
49. Reaven, G.M. Role of insulin resistance in human disease. Diabetes 37: 1595-1607, 1988.
50. Lee, J.W. and Romsos, D.R. Leptin-deficient mice commence hypersecreting insulin in response to acetylcholine between 1 and 2 week of age. Exp. Biol. Med. 226: 906-911, 2001.
51. Chen, N.G., Swick, A.G. and Romsos, D.R. Leptin constrains acetylcholine-induced insulin secretion from pancreatic islets of ob/ob mice, J. Clin. Invest. 100: 1174-1179, 1997.
52. Caygill, C.P.J. and Hill, M.J. Fish, n-3 fatty acids and human colorectal and breast cancer mortality. Eur. J. Cancer Prev. 4: 329-332, 1995.
53. Deschner, E.E., Lytle, J.S., Wong, G., Ruperto, J.F. and Newmark, H.L. The effect of dietary omega-3 fatty acids (fish oil) on azoxymethanol-induced focal areas of dysplasia and colon tumor incidence. Cancer 66: 2350-2356, 1990.
54. Reddy, B.S. and Marayama, H. Effect of dietary fish oil on azoxymethane-induced colon carcinogenesis in male F344 rats. Cancer Res. 46: 3367-3370, 1986.
55. Reddy, B.S., Burrill, C. and Rigotty J. Effect of diets high in -3 and -6 fatty acids on initiation and postinitiation stages of colon carcinogenesis. Cancer Res. 51: 487-491, 1991.
56. Fritsche, K.L. and Johnson, P.V. Effect of dietary -linolenic acid on growth, metastasis, fatty acid profile and prostaglandin production of two murine mammary adenocarcinomas. J. Nutr. 120: 1601-1609, 1990.
57. Thompson, L.U., Rickard, S.E., Orcheson, L.J. and Seidl, M.M. Flaxseed and its lignan and oil components reduce mammary tumor growth at a late stage of carcinogenesis. Carcinogenesis 17: 1373-1376, 1996.
58. Hirose, M., Masuda, A., Ito, N., Kamano, K. and Okuyama, H. Effects of dietary perilla oil, soybean oil and safflower oil on 7,12-dimethylbenz[a]anthracene (DMBA) and 1,2-dimethylhydrazine (DMH)-induced mammary gland and colon carcinogenesis in female SD rats. Carcinogenesis 11: 731-735, 1990.
59. Narisawa, T., Fukaura, Y., Yazawa, K., Iskikawa, C., Isoda, Y. and Nishizawa, Y Colon cancer prevention with a small amount of dietary perilla oil high in alpha-linolenic acid in an animal model. Cancer 73: 2069-2075, 1994.
60. Onogi, N., Okuno, M., Komaki, C., Moriwaki, H., Kawamori, T., Tanaka, T., Mori, H. and Muto, Y. Suppressing effects of perilla oil on azomethane-induced foci of colonic aberrant crypts in rats. Carcinogenesis 17: 1291-1296, 1996.
61. Petrik, M.B., McEntee, M.F., Johnson, B., Obuckowicz, M. and Whelan, J. Highly-unsaturated n-3 fatty acids, but not alpha-linolenic acid, conjugated linoleic or gamma linolenic acids reduce tumorogenesis in ApcMin/+mice. J. Nutr. 130: 2434-2443, 2000.
62. Petrik, M.B., McEntee, M.F., Chiu, C.H. and Whelan, J. Antagonism of arachidonic acid is linked to antitumiorgenic effect of dietary eicosapentaenoic acid in ApcMin/+ mice. J. Nutr. 130: 1153-1158, 2000.
63. Whelan, J. and McEntee, M.F. Dietary fats and APC-driven intestinal tumorigenesis. In: Nutrition and gene expression, Berdanier, C.D. and Moustaid-Moussa, M, Eds. CRC Press, Boca Raton, FL, pp 231-260, 2001.
64. Petrik, M.B., McEntee, M.F., Obuckowicz, M., Masfarrer, J., Zwiefel, B., Chiu, C-H. and Whelan, J. Selective inhibition of delta-6 desaturase impedes APC-mediated tumorigenesis. Cancer Lett. In Press, 2001.
65. Chiu, C-H., McEntee, M.F. and Whelan, J. Discordant Effects of Nonsteroidal Antiiflammatory Drugs on Intestinal Tumor Load in Min/+ Mice. Prostaglandins, Leukot. Essen. Fatty Acids 62, 269-275, 2000.
66. Whelan, J., Chiu, C.-H. and McEntee, M.F. Intestinal Tumor Load in the Min/+ Mouse Model is not Correlated with Eicosanoid Biosynthesis. In Advances in Experimental Biology and Medicine, vol 469, (Honn, K.V., Nigam, S., Marnett, L.J. and Dennis, E., eds.) Plenum Publishing, NY pp 607-615, 1999.
67. Chiu, C-H., McEntee, M.F. and Whelan, J. Sulindac Causes Rapid Regression of Preexisting Tumors in MIN/+ Mice Independent of Prostaglandin Biosynthesis. Cancer Res. 57, 4267-4273, 1997.
68. McEntee, M.F., Chiu, C.-H. and Whelan, J. Relationship of -Catenin and Bcl-2 Expression to Sulindac-Induced Regression of Intestinal Tumors in Min Mice. Carcinogenesis. 20, 635-640, 1999.
69. Taber, L., Chiu, C-H. and Whelan, J. Assessment of the Arachidonic Acid Content in Foods Commonly Consumed in the American Diet. Lipids 33, 1151-1157, 1998.
70. Liu, H., Cunnick, J.E. and Hendrich, S. Opposing effects of prostaglandin E2 and F2 on rat liver-associated natural killer cell activity in vitro. Prostaglandins Leukotrienes and Essential Fatty Acids 63: 153-158, 2000.
71. Liu, H., Ly, Y., Haynes, J.S., Cunnick, J.E., Murphy, P. and Hendrich, S. Reaction of fumonisin with glucose prevents promotion of hepatocarcinogenesis in female F344/N rats while maintaining normal hepatic sphinganine: sphingosine. J. Agric. Food Chem. 49: 4113-4121, 2001.
72. Broughton, K.S., Johnson, C.S., Pace, B.K., Liebman, M. and Kleppinger, K.M. Reduced asthma symptoms with n-3 fatty acid ingestion are related to 5-series lipoxygenase production. J. Nutr. 65: 1011-1017, 1997.
73. Fritsche, K.L., Byrge, M. and Feng, C. Dietary fish oil reduces in vivo production of interleukin-12 in mice. Immunol. Lett. 65: 167-173, 1999.
74. Feng, C., Keisler, D.H. and Fritsche, K.L. Dietary omega-3 polyunsaturated fatty acids reduce interferon-gamma (IFN) receptor expression in mice. J. Interferon and Cytokine Res. 19: 41-48, 1999.
75. Blok, W.L., Katan, M.B. and van der Meer, J.W.M. Modulation of inflammation and cytokine production by dietary (n-3) fatty acids. J. Nutr. 126: 1515-1533, 1996.
76. Fritsche, K.L., Shahbazian, L.M., Feng, C. and Berg, J.N. Dietary fish oil reduces survival and bacterial clearance in C3H/Hen mice challenged with Listeria monocytogenes. Clin. Sci. 92: 95-101, 1997.
77. Watkins, B.A., Lippman, H.E., Le Bouteiller, L. and Seifert, M.F. Bioactive fatty acids: role in bone biology and bone cell function. Prog. Lipid Res. 40: 125-148, 2001.
78. Watkins, B.A., Li, Y., and Seifert, M.F. Nutraceutical fatty acids as biochemical and molecular modulators of skeletal biology. Am. Coll. Nutr. In Press, 2001.
79. Watkins, B.A., Shen, C-L., Allen, K.G.D. and Seifert, M.F. Dietary (n-3) and (n-6) polyunsaturates and acetylsalicylic acid alter ex vivo PGE2 biosynthesis, tissue IGF-1 levels and bone morphometry in chicks. J. Bone Miner. Res. 11: 1321-1332, 1996.
80. Watkins, B.A., Shen, C-L., McMurtry, J.P., Xu, H., Bain, S.D., Allen, K.G.D. and Seifert, M.F. Dietary lipids modulate bone prostaglandin E2 production, insulin-like growth factor-I concentration and formation rates in chicks. J. Nutr. 127: 1084-1091, 1997.
81. Li, Y., Seifert, M.F., Ney, D.M., Grahn, M., Grant, A.L., Allen, K.G.D. and Watkins, B.A. Dietary conjugated linoleic acids alters serum IGF-I and IGF binding protein concentrations and reduce bone formation in rats fed (n-6) or (n-3) fatty acids. J. Bone. Miner. Res. 14: 1153-1162, 1999.
82. Watkins, B.A., Li, Y., Allen, K.G.D., Hoffman, W.E. and Seifert, M.F. Dietary ratio of n-6/n-3 PUFA alters the fatty acid composition of bone compartments and biomarkers of bone formation in rats. J. Nutr. 130: 2274-2284, 2000.
83. McCormack, M.C. The contribution of low birth weight to infant mortality and childhood morbidity. N. Engl. J. Med. 312: 82-90, 1985.
84. Bagguma-Nibasheka, M., Brenna, J,T. and Nathanielsz, W. Delay of preterm delivery in sheep by omega-3 long chain polyunsaturates. Biol. Reprod. 60: 698-701, 1999.
85. Olsen, S.F., Hansen, H.S., Jensen, B. and Sorensen, T.J.A. Longer gestation in rats supplemented with (n-3) fatty acids than in rats fed diet supplemented with (n-6) fatty acids. Sixth International Conference on Prostaglandins and Related Compounds. Adv. Prostaglandin Thromboxane Res. 15: 89-93, 1986.
86. Leaver, H.A., Lytton, F.D., Dyson, H., Watson, M.L. and Mellor, D.J. The effect of dietary 3 and 6 polyunsaturated fatty acids on gestation, parturition and prostaglandin E2 in uterine tissues and the kidney. Prog. Lipid Res. 25: 143-146, 1986.
87. Harris, M.A., Reece, M.S., McGreggor, J.A., Manchego, J.M. and Allen, K.G.D. Possible roles of maternal and perinatal long-chain fatty acids in preterm birth. In: Lipids in Infant Nutrition, Huang, Y-S. and Sinclair, A.J., Eds., American Oil Chemists Association (AOCS) Press, Champaign, IL, pp1-18, 1998.
88. Olsen, S.F., Sorensen, J.D., Secher, N.J., Hedegaard, M., Henriksen, T.B., Hansen, H.S. and Grant, A. Randomized controlled trial of effect of fish-oil supplementation on pregnancy duration. Lancet 339: 1003-1007, 1992.
89. Olsen, S.F., Hansen, H.S., Sorensen, T.I.A., Jensen, B., Secher, N.J., Sommer, S. and Knudsen, L.B. Intake of marine fat, rich in (n-3) polyunsaturated fatty acids, may increase birthweight by prolonging gestation. Lancet 2: 367-369, 1986.
90. Olsen, S.F., Hansen, H.S., Sommer, S., Jensen, B., Sorensen, T.I.A., Secher, N.J. and Zachariassen, P. gestational age in relation to marine n-3 fatty acids in maternal erythrocytes: A study in the Faroe Islands and Denmark. Am. J. Obstet. Gynecol. 164: 1203-1209, 1991.
91. Olsen, S.F., Secher, N.J., Tabor, A., Weber, T., Walker, J.J. and Gould, C. Randomized clinical trials of fish oil supplementation in high risk pregnancies. Br. J. Obstet. Gynecol. 107: 383-395, 2000.
92. Reece, M.S., McGreggor, J.A., Allen, K.G.D. and Harris, M.A. Maternal and perinatal long chain fatty acids: possible roles in preterm birth. Am. J. Obstet. Gynecol. 176: 907-914, 1997.
93. Huang, C.M. and Craig-Schmidt, MC. Arachidonate and docosahexaenoate added to infant formula influence fatty acid composition and subsequent eicosanoid production in neonatal pigs. J. Nutr. 126: 2199-2208, 1996.
94. Conquer, J.A. and Holub, B.J. Dietary docosahexaenoic acid as a source of eicosapentaenoic acid in vegetarians and omnivores. Lipids 32: 431-345, 1997.
95. Harris, M.A., Hansen, R.A., Vidsudhiphan, P., Koslo, J.L., Thomas, J.B., Watkins, B.A. and Allen, K.G.D. Effects of conjugated linoleic acids and docosahexaenoic acid on rat liver and reproductive tissue fatty acids, prostaglandins and matrix metalloproteinase production. Prostaglandins Leukotrienes and Essential Fatty Acids 65: 23-29, 2001.
96. Trujillo, E.P. and Broughton, K.S. Ingestion of n-3 polyunsaturated fatty acids and ovulation in rats. J. Reprod. Fertil. 105: 197-203, 1995.
97. Desfaits, A.C., Serri, O. and Renier, G. Normalization of plasma lipid peroxides, monocyte adhesion, and tumor necrosis factor-alpha production in NIDDM patients after gliclazide treatment. Diabetes Care 21: 487-493, 1998.
98. Arnlich, F., Hernandez, A., Lopez-Maderuelo, D., Pena, J.M., Camacho, J., Madero, R., Vazquez, J.J. and Montiel, C. Enhanced acute-phase response and oxidative stress in older adults with type II diabetes. Horm. Metab. Res. 32: 407-412, 2000.