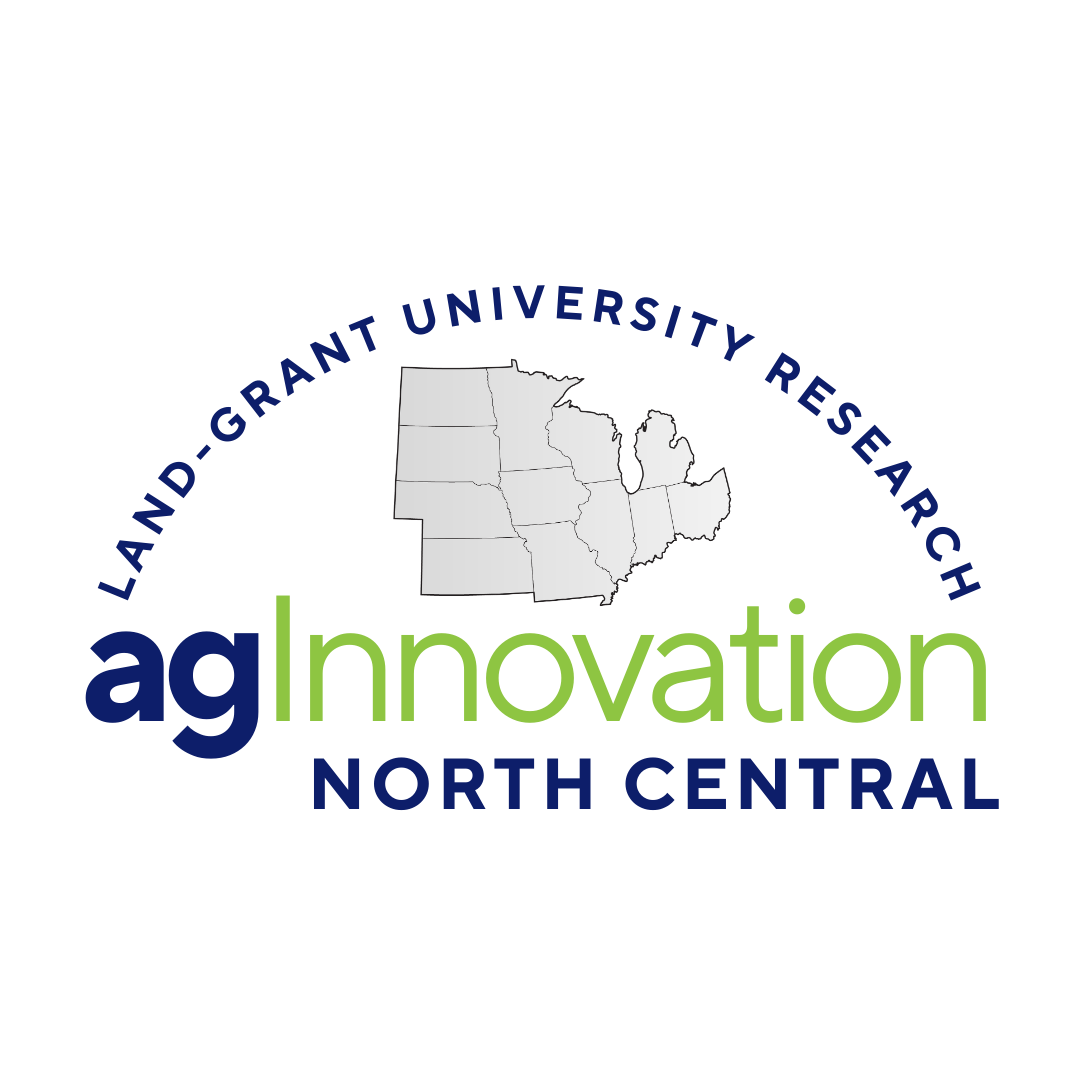
NC1182: Management and Environmental Factors Affecting Nitrogen Cycling and Use Efficiency in Forage-Based Livestock Production Systems
(Multistate Research Project)
Status: Approved Pending Start Date
NC1182: Management and Environmental Factors Affecting Nitrogen Cycling and Use Efficiency in Forage-Based Livestock Production Systems
Duration: 10/01/2024 to 09/30/2029
Administrative Advisor(s):
NIFA Reps:
Non-Technical Summary
Pastoral and forage-based ruminant production systems dominate production of meat, milk, and fiber across the globe. We propose to continue conducting complementary experiments pertaining to N-use efficiency in forage-based livestock production systems to help stakeholders make informed decisions about rural landscapes. 13 Researchers from 12 states will collaborate to conduct field research aiming to: 1) Quantify biophysical effects of grassland-based management strategies and climate change on N-use efficiency by ruminant animals, N cycling in herbage and soils, aquatic N losses, and GHG and other pollutant emissions from grassland agro-ecosystems. 2) Determine the role of plant secondary metabolites in ensuring improved pasture sustainability, enhancing animal health and performance, and decreasing the animal environmental footprint. 3) Assess the efficacy of dietary factors on N-use efficiency in ruminant animals in forage-based livestock production systems and how these practices affect composition of feces and urine and rumen microbial efficiency. Outputs from this project will included publications, presentations at professional and industry meetings, electronic newsletter articles, and extension website articles. Research findings will be delivered to producers and stakeholders by Extension Educators who are participants to this project by media including Pasture Walks, Fact-Sheets and Extension websites. Research findings will incorporated into graduate and undergraduate academic curriculum by Instructors who are participants to this project.
Statement of Issues and Justification
Pastoral and forage-based ruminant production systems dominate production of meat, milk, and fiber across the globe. However, forage-based production systems are among the most inefficient in terms of feed conversion and N-use efficiency when compared with other meat-producing production systems such as poultry. Forage-based ruminant production is often on land not suited for crop production and is important to food security (Mottet et al., 2017). Nitrogen use efficiency and improvement thereof is a recurring subject of investigation in these systems (Gerber et al., 2015). The amount of N applied annually to these systems through fertilization and/or supplemental feedstuffs can exceed plant uptake, and animal utilization of N, whether from forage or other feedstuffs, is relatively low (avg. of approximately 25%) and highly variable (10 to 40%; Calsamiglia et al., 2010). This can result in overabundance or toxic levels of N in forages and/or the ecosystem. Significant quantities of N fertilizers (mineral and animal) are applied to forage crop and grazing systems because N is often the most limiting nutrient for plant growth. Providing supplemental N to forage-based livestock production systems is expensive whether supplied as fertilizer N to the forage or as supplemental crude protein to the animal. Therefore, continued investigations into measures to improve N-use efficiency at the soil, plant, and animal interface is necessary to improve long-term, sustainable livestock production while concurrently reducing potential pollutants and greenhouse gas (GHG) emissions.
Although nutrient cycles in grazing-forage systems are less open than in systems that rely on concentrated feed such as poultry production, grazing systems cover more than a quarter of the earth’s land surface (Asner et al., 2004) and loss of N from pastures is an unsolved problem to which fertilizer N, climate and edaphic conditions, grazing density and supplemental forage and feed contribute (Zhou et al., 2017; Li et al., 2012). The magnitude of N loss and resulting positive and negative impacts on ecosystems and forage productivity are influenced by the timing, frequency, and intensity of management practices within the ecosystems. In the Midwest, loss of N from any agricultural system may contribute in a large fashion to periodic hypoxia in the Gulf of Mexico. In addition, gaseous N emissions from pastures contribute to the greenhouse effect (Gerber et al., 2016). More in-depth analysis is needed regionally (Franzluebbers, 2005) especially related to grazing management systems (Gerber et al., 2017) as grazing can improve carbon (C) and N storage depending on management (Wang et al., 2016) and environment.
Increased demand for meat products by consumers during past decades has encouraged producers to respond with an increased intensification of forage-based livestock production. Hence, there is an urgent need for scientific information to help producers make decisions about how to best manage rural landscapes and to produce agricultural commodities while maintaining soil, water, and air quality (Gerssen-Gondelach et al., 2017). The foci of our experiments will examine alternative strategies to concurrently improve N harvest efficiency while reducing N losses from forage-based livestock production systems. This overall objective will be achieved through pastoral and other forage management strategies to enhance N retention and utilization at the soil, plant, and animal level. Such strategies include improving legume establishment and persistence, alteration of spatiotemporal distribution of soil N pools, use of varied fertility practices in legume and non-legume systems, use of legumes with various secondary plant metabolites, and use of various supplementation practices while concurrently evaluating the impacts of management, climate change, and forage nutritive value on N-use efficiency. Our results will then be disseminated through coordinated extension/education activities and at national, regional and state conferences.
Expected outcomes and predictions will include advice on management strategies in terms of N use efficiency, particularly as it relates to the capture and excretion of N in the environment. The ultimate goal is to help producers adopt strategies/practices that ensure efficient use of N in order to positively influence forage and animal productivity and environmental quality. In addition, this work will facilitate the identification of forage systems that minimize N inputs and production costs. Minimizing expensive N inputs (e.g., fertilizers) in forage-based livestock production systems has tremendous potential to enhance their profitability. These impacts are most likely achieved through the development and implementation of a multiple-state project. The members of our proposed project represent a geographically diverse set of states from the Southeast through the Midwest and Great Plains to the Intermountain West. Our objectives of analyzing N use efficiency of grassland production systems will be based on a wide range of environments (humid to semi-arid) and levels of management intensity (irrigated to low-input grassland systems). The expertise, facilities and other resources required to design and conduct the proposed research are not found at a single institution. The synergy coming from a multiple-state effort in this area greatly enhances the likelihood of success in characterizing N use and developing appropriate management strategies for grassland agro-ecosystems. Furthermore, the technical feasibility of this type of research is questionable for a single university but becomes realistic when several institutions combine resources and expertise.
We propose to continue conducting complementary experiments pertaining to N-use efficiency in forage-based livestock production systems to help stakeholders make informed decisions about rural landscapes. Forage-based livestock production systems provide multiple ecosystem services including meat, milk, and fiber production as well as supporting, regulating, and cultural services. Supporting services include soil building and nutrient retention, banking, and filtering rain and melting snow waters through the soil profile. Carbon sequestration and water storage are also regulating services, and cultural services include spiritual, aesthetic, and educational factors. Forage and perennial grasslands vary greatly in their ability to provide these types of ecosystem services because of differing environmental and management characteristics. We will assess tradeoffs among these services, which should allow more informed decision-making and long-range improvements in U.S. agriculture as a result.
Related, Current and Previous Work
Our previous work illustrated that forage-based hay and livestock production systems can improve farm profitability, especially when leguminous species are utilized. We also have preliminary evidence of uneven N distribution in continuously grazed pastures and have found that much of the N losses or reduced N efficiency in these systems is related to forage type, landscape position, grazing management and associated cattle behavior. Our forage- and pasture-based management strategies were developed as appropriate for each of our regional ecosystems and demonstrate the capacity to improve C capture, reduce GHG fluxes, and increase retention and utilization of N within these soil, plant, and animal production systems. These strategies do so by redistributing, recycling, or producing N within the system at minimal cost and time to the farmer. However, what we have yet to discern is the magnitude of these services and to what degree these services change with changing environments and climate. For example, which of these forage- and pasture-based management strategies will stand-up to (resist) extreme weather events, both wet and dry. Grazing and forage systems that capture and attenuate water within the grassland soil and that can help utilize or recycle nutrients may help both the environment and the farmer but it is dependent on ecosystem and management (Abdalla et al., 2017). The negative influences of GHG emissions, eutrophication of aquatic systems, and loss of farm income may be moderated at the farm scale by adaptive management such as strategic rotational grazing management, forage choice or mixtures, and incorporation of the sensitivity of landscape to changes in these grassland management systems. The amount of change will vary depending on landscape and ecosystem. Exploring potential solutions for sustainable grassland systems throughout the US will facilitate our understanding and quantification of soil C, N use efficiencies, GHG fluxes, nutrient cycling and other measures of soil health. Differences in ecosystems (positive and negative) should be considered when developing predictions on water retention (Rojas-Downing et al., 2017) and nutrient cycling when identifying the most effective management strategies for changing climates. Pastoral systems throughout the US experience repeated droughts multiple times each decade and chronically experience patterns of very wet and very dry periods. Of additional concern is the prediction that these extreme weather events will be exacerbated in the next 25 to 50 years (IPCC, 2012).
Surface application of N fertilizers (mineral, manures, and deposited directly by cattle) to grasslands results in losses of ammonia (NH3), nitrate (NO3), and nitrous oxide (N2O) depending on environmental variables such as temperature, relative humidity, rainfall, soil texture, litter layer, and soil water content. Our previous research on Southern Piedmont pastures in Georgia has shown NH3 losses from urea-ammonium nitrate (UAN) ranging from 6 to 33% of the applied N (Vaio et al., 2008). These emissions of NH3 to the atmosphere decrease the efficiency of the applied N and the lost NH3 is eventually deposited back to soil or water with potential environmental problems. Ammonia deposition in riparian and forest areas contributes to soil acidification through nitrification and acts as a direct source of NO3 leaching or an indirect source of N2O, a potent greenhouse gas (Mosier et al., 1998). Ammonia deposition in surface waters may also lead to eutrophication in streams, rivers, and lakes (Lewis et al., 2011). Thus, identifying practices that reduce NH3 losses from grasslands is important to improve N and economic efficiency and to decrease potential environmental problems.
Another option for improving N-use efficiency is incorporation of forages with plant secondary metabolites which also adds plant species diversity and associated biodiversity and may increase in beneficial insects that predate on pests that reduce beef productivity. Legume secondary plant metabolites such as tannins and saponnins, have been shown to reduce methane (CH4) production in the rumen. Results from a preliminary meta-analysis using data from 20 studies highlighted that incorporating clover (Trifolium sp.) at levels up to 30% of dry matter in grass-based diets reduced enteric CH4 output of dairy cows by 30 g/cow/d. However, feeding clover at that rate was associated with a reduced utilization of N for milk production indicating more excretion of N in urine, which would contribute to N2O emissions from manure (Schils et al., 2013). Plant secondary metabolites such as tannins offer potential to improve this situation as tannins suppress degradation of plant protein by ruminal microorganisms (Coblentz and Grabber, 2013) and shift digestion to the small intestine (Niderkorn et al., 2011; Waghorn, 2008). This may ultimately improve animal production (Hymes-Fecht et al., 2013) or production efficiency (Aguerre et al., 2016) while reducing total urinary N and urinary urea N (Aguerre et al., 2016) if included at an optimal amount in the diet. However, this optimum level needs further evaluation because of considerable variability in the response to tannins across different studies (Animut et al, 2008; Martin et al., 2010) due to variability in response by different classes of animals (Aguerre et al., 2016) or variability in tannins from different plant sources (Animut et al., 2008; Kahn et al., 2009). Other forage phenolic compounds also reduced ruminal NH3 (Hymes-Fecht et al., 2013) and ruminal protein degradation (Grabber, 2008), and improved N utilization (Broderick et al., 2001) in dairy cows. However, as with tannins, it is likely that the optimum dose or dietary concentration will vary depending on the specific response measurement evaluated, making it necessary to evaluate a range of diets that include plants with greater phenolic compound concentrations.
Feeding tannins or other plant secondary metabolites may have additional benefits environmentally, as feeding tannin extracts substantially reduced direct NH3 emissions from dairy cattle manure (Powell et al., 2011b) or when manure was applied to soil in lab-scale chambers (Powell et al., 2011a). It is possible that the shift in digestion of forage protein from the rumen to the small intestine increased diversity in N-containing compounds in the urine. Environmentally, this is of importance because different N-containing compounds in the urine react differently when acted upon by soil microorganisms (Oenema et al., 1997; van Groenigen et al., 2005a,b). Less N in urine should result in less N volatilization as NH3 (Wachendorf et al., 2008), less leaching of N (Saarijärvi and Virkajärvi, 2009), and possibly less N loss as N2O (Oenema et al., 1997). Again, however, information of optimal dietary concentrations of tannins or other polyphenols needed to minimize environmentally negative N leaching or GHG emissions is limited.
Optimal ruminal fermentation is dependent upon microbial growth which is affected by the concurrent availability of fermentable carbohydrates and N from the breakdown of dietary protein to NH3 and numerous C-containing structures (Theodorou et al., 2006; Calsamiglia et al., 2010). This synchrony is difficult to achieve in forage-based diets however because of differential rates of microbial breakdown of the compounds that contain N and those primarily composed of cellulose. The most logical approach to optimize rumen function is to feed balanced diets to ruminants (Garg et al., 2013). Forage-based diets are not balanced generally because of fluctuations in forage quality throughout the growing season leading to great fluctuations in the C:N ratio. Means to improve fermentation efficiency by ruminant animals consuming forage-based diets are available (Gerber et al., 2013), but practical application of many of these principles is not commonly achieved (Hoekstra et al., 2007). Supplementation with concentrates or other fermentation-enhancing compounds may be used to improve the C:N ratio and improve overall animal efficiency by capturing more C and N into animal tissue and concurrently reducing N excretion, especially in urine (Dijkstra et al., 2013; Lee et al., 2014). Furthermore, fermentation may be enhanced by supplementation with different enzymes which may reduce the need for further protein supplementation. However, in many instances, supplementation practices are based on economics and feedstuff availability rather than having a primary focus of optimizing capture of C and N in the animal and reducing nitrogenous excretions by the animal.
Biological nitrogen fixation (BNF) is an important ecosystem process by which microbes transform atmospheric di-nitrogen to plant available N forms. BNF is especially important in agricultural systems, where it has the potential to reduce the need for exogenous N applications (i.e. fertilizer), which are known to have negative environmental consequences and reduce overall agricultural system sustainability (Herridge et al. 2008). In pastures worldwide, legumes are commonly deployed for their BNF capabilities, as well as their capacity to produce secondary metabolites that often improve forage quality and animal gain (Graham and Vance 2003). Legumes, such as red and white clover, form symbioses with soil rhizobia to perform BNF. Previous work has shown that legume-rhizobia BNF is controlled by nitrogen availability (King and Purcell 2005), oxygen levels (Denison 1998), carbon availability (Arrese-Igor et al. 1999), drought (Zahran 1999), and high temperatures (Whittington et al. 2013). Optimal temperatures for temperate legume-rhizobia symbiosis are 15-25oC (Aranjuelo et al. 2014). N2-fixing bacteria have a relatively low tolerance for high temperatures (Aranjuelo et al. 2014), and elevated temperatures may inhibit nodulation by reducing legume root hair formation (Hungria and Franco 1993).
Need for Research: Given that climate change is predicted to lead to warmer and drier conditions for much of the world, understanding the synergistic effects of these two stressors on legume-rhizobia BNF is critical. By evaluating plant and BNF responses to these stress factors and linking these responses to the genes controlling the legume-rhizobia symbiotic interaction, we will start to compile a comprehensive, systematic understanding of the abiotic stress responses of this important source of BNF in pastures worldwide.
Livestock waste deposition areas in grazed pastures are hot spots for atmospheric losses of carbon and nitrogen in the forms of carbon dioxide (CO2), methane (CH4), nitrous oxide (N2O), and volatilized ammonia (NH3). Waste deposition from grazing livestock is the second largest contributor to greenhouse gas emissions in the agricultural sector, with enteric fermentation emitting the greatest portion (Tubiello et al., 2013). In particular, urine depositional areas exhibit accelerated biogeochemical reaction rates and are subject to greater gaseous loss pathways than feces deposition (van der Weerden et al., 2011). Concentrated N application rates can reach 1300 kg N ha-1 per urine patch for dairy cows (Eckard et al., 2010), with excess N being lost directly through ammonia volatilization or nitrous oxide emissions. Nitrous oxide emissions are widely considered to be the most problematic greenhouse gas to control in intensively grazed pasture environments, with over 30% of total nitrous oxide emissions attributed to livestock excreta (Oenema et al., 1997). Given that global demand for meat products is expected to increase in the future (Tilman & Clark, 2014), there is a need to develop innovative strategies to mitigate greenhouse gas emissions and ammonia volatilization in livestock grazing systems.
Tactics for reducing trace gas losses in pastures are focused on limiting the risk of reactive N transformation and loss via ammonia volatilization and nitrous oxide. The majority of total N in urine patches is excreted as urea (Whitehead et al., 1989) and can be quickly volatilized as ammonia when combined with the urease enzyme, which is ubiquitous in soil. Reducing the total amount of N excreted by ruminants has long been a production goal by altering mineral rations or decreasing feed N (Dijkstra et al., 2013). Another strategy to decrease N excretions is to improve dietary protein retention in the animal, which is the main source of N in urine excretions. The rumen microbial community is diverse and includes hyper-ammonia producing bacteria (HAB), a gram-positive anaerobic guild that preferentially breaks down dietary peptides, proteins, and amino acids into ammonia. Ammonia cannot be absorbed by the animal in the rumen, so ammonia is excreted via urine and represents a loss of productive animal potential (Harlow, Flythe, et al., 2017). Supplementing cattle with biochanin A (BCA) has been shown to reduce HAB activity in the rumen (Flythe & Kagan, 2010) and the associated conversion of ruminal proteins to ammonia, thereby improving cattle growth performance and gains (Harlow, Flythe, et al., 2017). Whether the greater animal gains lead to a reduction in urinary N output is not known.
BCA is an isoflavone that is a naturally produced plant secondary metabolite in some legume species including red clover (Trifolium pratense L.) (Carlsen & Fomsgaard, 2008; Flythe & Kagan, 2010; Krizova et al., 2019). Red clover is a high-value forage for ruminants and has a long history of being integrated into grazing systems in temperate areas (Ball et al., 1991). In addition to potentially altering urinary N excretions, BCA supplementation causes urinary excretions of BCA itself and its animal-transformed breakdown products: genistein and p-ethylphenol (Batterham et al., 1971; Carlsen & Fomsgaard, 2008; Shutt et al., 1970). No information is available about how BCA, genistein, and p-ethylphenol in livestock urine affect soil microbial communities or soil trace gas emissions. Given that feeding BCA to ruminants shows promise as a strategy to improve nutrient use and animal production efficiency, an understanding of how BCA supplementation affects pasture trace gas emissions is warranted. Prior work performed in our lab demonstrated that BCA in urine significantly reduces ammonia volatilization and nitrous oxide and methane emissions from soil (Jacobs, 2022). However, this work failed to identify the mechanism by which these reductions were achieved. Thus, we propose to conduct additional work to identify the mechanisms responsible for the observed reductions in greenhouse gases.
Protecting and enhancing the capacity of temperate pasture agroecosystems to provide forage for livestock and important ecological services such as clean water and air is a high priority, given predicted shifts in climate patterns and alterations to plant growing conditions and species interactions (Tubiello et al., 2007). One such strategy is to broaden biological diversity in pasture systems, with the theory that more diverse genetic backgrounds allow species to better tolerate and/or recover from stressful conditions such as drought (Tracy et al., 2018). Greater levels of plant species diversity in non-agronomically managed grasslands have been shown to produce a range of ecological benefits that include greater aboveground net primary production (Hector et al., 1999; Naeem et al., 1994; Tilman, 1996), resilience to disturbance (Tilman & Downing, 1994), resistance to invasion by weedy species (Tracy & Sanderson, 2004), and enhancement of ecosystem services such as carbon and soil nutrient storage (Furey & Tilman, 2021).
Despite the benefits of increased plant species diversity in grasslands, integrating greater plant species diversity in pasture agroecosystems that are intensely managed for forage production is difficult. Plant diversity decreases in areas with regular nutrient and fertilizer additions (Harpole et al., 2016) and frequent biomass harvests for hay or grazing (Sanderson et al., 2004), leading to plant community simplification to two or three high-yielding grass species. The challenges of establishing competing vegetation in pasture systems suggest that efforts to increase biodiversity could be focused elsewhere besides the plant community level. Biological diversity in ecosystems may exist at many levels and scales. One method for integrating greater biological diversity into intensely managed pasture agroecosystems dominated by these highly competitive and productive grass species is to increase the genetic diversity of the primary grass species. The genetic background of individual plant species has been shown to exert considerable control over plant characteristics and associated feedbacks into soil nutrient cycles. The ability of species genetic resources to regulate ecological processes has been called the “extended phenotype”, where genes of individual species extend their sphere of influence into higher levels than that of the individual species or population level (Whitham et al., 2003).
Symbioses between species may also contribute to "extended phenotypes." For example, tall fescue, the predominant forage of the eastern half of the US (Stuedemann & Hoveland, 1988), is known to associate with the fungal endophyte Epichloë coenophiala (Bacon, 1995), which produces toxic alkaloids, the presence of which has been shown to alter a range of ecological processes (Rudgers et al., 2004). Grazing animal toxicity issues in tall fescue pastures have led to the discovery of diverse strains of E. coenophiala, each producing a distinct alkaloid profile (Takach & Young, 2014). These so-called 'novel' endophyte strains have been inserted into elite cultivars and are currently sold to producers as a single tall fescue cultivar-endophtye strain 'package.' Producers typically purchase one such 'package' and plant their fields in a symbiotic monoculture. However, these packages could be easily mixed to produce more 'symbiotically diverse' tall fescue pastures (Jacobs, 2022). Though the value of greater heterogeneity in cultivar mixtures has been recognized in improving annual crop resilience to environmental stressors (Newton et al., 2009), establishing stands containing multiple cultivars of the same forage species is not yet a common practice in pasture agroecosystems. The effects of increasing tall fescue symbiotic diversity on pasture stand resilience, productivity, species composition, alkaloid concentrations, and forage digestibility are currently unknown.
Plant litter is one of the major pools of nutrients returning into forage systems, especially under low input systems where replenishment of nutrients through fertilization is limited. Litter is defined as the “accumulation of dead detached plant material at the soil surface” (Allen et al., 2011) and it consists of partially decomposed and newly deposited senescent plant material (Dubeux et al., 2006). The litter chemical composition is defined by C, nitrogen (N), and lignin concentrations and their ratios (Prescott, 2010), and these components directly determine nutrient mineralization and immobilization processes. Environmental conditions (i.e., temperature, rainfall) play an important role in the rate of the decomposition process (Fornara et al., 2009) and management strategies directly affect forage production and nutrient pool dynamics (Dubeux et al., 2006; Liu et al., 2011). Defoliation intensity and frequency and N fertilization directly affect forage production and quality, stand persistence, and nutrient turnover. Reliance on N fertilizer increases production costs and greenhouse gas emissions associated with its production, storage, transportation, and application (Lal, 2004). Therefore, the incorporation of different functional groups, especially legumes, into forage systems is a viable option to reduce off-farm inputs while incorporating N into the system through nodule turnover, root exudation, animal excreta, and plant litter decomposition. The incorporation of legumes into grass swards improves litter quality, decomposition rate, and N release because of greater N concentration and lesser C/N ratio of legume than grass herbage (Kohmann et al., 2018; Silva et al., 2021).
For the animal production activity, the main sources of emission include methane (CH4) from livestock enteric fermentation (DeRamus et al., 2003), CH4 and nitrous oxide (N2O) emitted from excreta (Mazzetto et al., 2014), animal waste management, litter decomposition, and use of inorganic N fertilizer (Bell et al., 2016). In addition, there are impacts of CO2 emissions associated with the production, storage, transportation, and application of inorganic fertilizer (Lal, 2004), along with the use of fossil fuels for other management activities. The incorporation of legumes into forage-livestock systems is a viable practice to decrease GHG emissions since it improves diet quality and decreases the reliance on chemical N fertilizer. This can also help decrease the magnitude of emissions of N2O which are directly impacted by grazing intensity (Rafique et al., 2011), temperature, soil moisture (van der Weerden et al., 2011), and compaction (Oenema et al., 1997), pH and soil organic matter (SOM) (Bouwman, 1996), N fertilizer (Venterea et al., 2011) and excreta deposition (Giltrap et al., 2014). Many studies have focused on optimizing the rate, source, timing of application, and placement of N fertilizer (Clayton et al., 1997; Venterea et al., 2011; Bell et al., 2016). However, it has been suggested that reducing N input is the most efficient practice to reduce N2O emissions (Venterea et al., 2012).
In summary, considerable progress has been made in the understanding of the complexities of factors that affect N-use efficiency in the ruminant animal and in the environment. However, much work is needed to develop linkages between the various factors influencing N-use and associated environmental factors and to develop practical, producer-friendly management practices and decision-support tools that improve N-use efficiency in forage-based livestock production systems. In our proposed work, we look to discover more efficient means to redistribute N from animal wastes with management while concordantly reducing export of N from pastures to the atmosphere and/or ground and surface wasters. We will also further explore means to quantify the influence of a variety of forage species (both grasses and legumes) and feeding strategies to improve feed efficiency, reduce GHG emissions, and improve N-use efficiency. Much work is needed to integrate practices that improve N-use efficiency at the soil-plant-animal-environment interface. This multi-state committee is comprised of scientists with expertise in soil, forage, and animal N-use efficiency as well as environmental and economic modeling. The projects proposed herein by those scientists with the described diversity in expertise and locations will produce information for the development of guidelines that will improve N-use efficiency at all levels of the soil-plant-animal-environment interface for several ecosystems.
Networking with other Regional Projects:
A number of other regional projects are currently working on forages and may include livestock components. Overall, each of these projects currently has members on that specific committee that are also members of the current NC1182 committee or are located in the same department as a current NC1182 member. Furthermore, the focus of those committees does not align with the specific focus of NC1182 which is to ultimately improve nitrogen-use efficiency at the landscape level in forage-based livestock systems across a vast geographical region. The NC1010 project is a forage breeding project that proposes to evaluate a number of forage characteristics which include forage quality, but other than possibly using one of the cultivars released by this committee, interests are different. The NC1181 committee has somewhat similar objectives, and the NC1181 and NC1182 committees have met together on multiple occasions in recent years. However, it was determined that the focus of that committee and that of NC1182 are sufficiently different to warrant separate meetings. The NC1181 is focused more specifically on issues in the mid-western US and include a crop residue emphasis and annual forages to extend grazing using cropland. Furthermore, NC1181 and NC1182 have multiple common participants which allows sharing of information among the group without a concurrent meeting. The NCCC31 objectives are to evaluate current forage-related issues and develop collaborative efforts to address these. This objective is much broader than that of the NC1182. There are multiple members on both the NCCC31 and NC1182 committees and other NC1182 members work closely with members of the NCCC31 committee that are at their respective institutions. Because of the specific focus of the NC1182 and the general focus of the NCCC31, a common meeting would not be advantageous at the present time. The WERA1040 is another committee with the focus of reviewing western regional issues and developing collaborative research proposals and extension publications to address these issues. The WERA1040 and NC1182 committees have one common member and other members at the same institution. Based on this, and considering the tight schedules that occur at the current annual meetings, it is better use of time and resources to interact with the other committees through common members and co-workers rather than reduce the collaborative interactions of the current NC1182 meetings.
Objectives
-
Objective 1. Quantify biophysical effects of grassland-based management strategies and climate change on N-use efficiency by ruminant animals, N cycling in herbage and soils, aquatic N losses, and GHG and other pollutant emissions from grassland agro-ecosystems.
Comments: Sub-Objectives: 1.1 Use of new and novel plant materials for improved N-functionality in pasture (KY, OH, TN). 1.2 Quantification of N-cycling as affected by grazing and pasture type (GA, NE, MI) 1.3. Quantifying Ecosystem Services as measures of Sustainability in Soil Smart Grazing Systems 1.4 N-leaching and N-dynamics to improve Soil Health. (UT, SC, MI) -
Objective 2. Determine the role of plant secondary metabolites in ensuring improve pasture sustainability, enhancing animal health and performance, and decreasing the animal environmental footprint
-
Objective 3. Assess the efficacy of dietary factors on N-use efficiency in ruminant animals in forage-based livestock production systems and how these practices affect composition of feces and urine and rumen microbial efficiency.
Methods
Methods
Objective 1. Quantify biophysical effects of grassland-based management strategies and climate change on N-use efficiency by ruminant animals, N cycling in herbage and soils, aquatic N losses, and GHG and other pollutant emissions from grassland agro-ecosystems.
Sub-Objective 1
Use of new and novel plant materials for improved N-functionality in pasture (KY, OH, TN).
Experimental Design: To explore drought resistance of red clover under abiotic stress conditions, we utilized an existing field manipulative experiment at Lexington KY, where ambient temperatures are raised 3oC by infrared radiant heating and rainfall was increased by 30%, mimicking larger storms, as is predicted for our region (see McCulley et al. 2014 for more detail). This 2×2 factorial experiment provides four climate treatments: ambient control, +3oC, increased precipitation, and a combination of +3oC and increased precipitation. Each treatment was replicated five times (n=20 plots in total). In each plot, Kenland red clover seed (Trifolium pratense) was planted. Kenland is an erect, fine stemmed, early flowering, high quality, relatively old and commonly used cultivar. In 2020 and 2021, we measured clover biomass production by clipping aboveground material from 0.25m2 quadrats 4-5 times throughout the growing season (mimicking a hay operation). This material was freeze-dried, weighed, ground, and analyzed for %C, %N, δ15N, δ13C, and total phenolics (Ainsworth and Gillespie 2007).
In addition to the physiological measurements, a battery of genome-wide assays designed to query transcriptional and epigenetic changes were conducted. For each treatment and plot replicate, plants were sampled and RNA isolated. These RNA samples were used to prepare so-called transcriptomics libraries (Ma et al. 2014; Hunt 2015) and were recently submitted for sequencing on the latest Illumina platform. The sequencing data will be analyzed following the general workflows described in studies published by this group (Thomas et al. 2012; Chakrabarti et al. 2016; de Lorenzo et al. 2017). The results will be used to assemble networks consisting of differentially-expressed genes, and will also be mapped onto pre-determined networks consisting of genes known (from prior research) to be important for abiotic stress tolerance.
In a related study, Running Buffalo Clover (Trifolium stoloniferum) (RBC) is endemic to the Ohio River Valley, including West Virginia, Ohio, Kentucky, Missouri and Indiana. As a native, perennial legume, it has potential as a companion legume in native grass pastures. As a non-nitrogen fixing species, has interest as a model species to measure nitrogen cycling in pasture, or as an option to improve forage quality without increasing the risk of off-site N-losses. RBC is a rare species without information of its seed production and ecology. The goal of this study is to propagate RBC at sites throughout Ohio to increase the population of plant material and understand it population demographics.
The protocol for transplanting RBC at 12 sites in Ohio, was to collect plants from their original habitat according to the ODNR Permit, “all plants grown from collected stolon cuttings shall be re-planted to their original location in consultation with DNAP (ODNR) and the landowners”. This proposal describes planting that took place in Fall 2017 & 2018 (Oct), and Spring 2018 and 2019, and subsequent population measurement.
Each location (Bosch Hollow, Miami Whitewater Forest, and Shawnee Lookout - Miami Fort) comprised a generally uniform area of about 200 sqm (or two 100 sqm areas in close proximity). Respective locations were approximately adjacent to the original population, to minimize the possibility of disturbance of the original population from a) planting and monitoring activity, b) without threat to potential natural expansion of the original population, c) genetic drift imposed by introduction of the (upto) 25 genotypes initially sampled, d) the possibility of pests, weeds or disease in the replanted population.
Transplants were planted at a 1m grid spacing (such as 10 x 10 m, or 5 x 20 m). Half the area (approx 100 sqm) was planted in Fall 2017 (Oct, prior to freezing night temperatures), and the other half planted in Spring 2018 (March/April, as field plants show the start of growth). The grid corners were marked with a steel post (if permitted) or other prominent landmark (such as a tree). Next spring, once the grid is relocated using a planting map, each original plant will be re-identified and measured. Thus, individual plants will be monitored; comparing its size at planting with its size next spring (and hopefully again in 12 months)
Research with a third legume in Springhill TN, used Sunn hemp, a fast-growing warm-season annual legume that can provide high nutritive value for beef cattle production. Sunn hemp can be beneficial when added to tall fescue swards by increasing summer forage production and providing biologically fixed N.
Also, the addition of sunn hemp to tall fescue pastures can increase its biodiversity and reduce livestock health-associated problems that can arise when livestock graze tall fescue alone, such as tall fescue toxicosis.
The objective of this study was to evaluate and compare a cool and a warm-season legume species (red clover and sunn hemp) mixed with tall fescue and determine their ability to provide sufficient N for stockpiling tall fescue, as well as increase productivity and animal performance.
The experimental period occurred from April to December 2020 and 2021, and the experimental design was a completely randomized design (CRD) with three treatments and three replications per treatment (n = 9). The treatments were as followed: 1) tall fescue + red clover [Trifolium pratense L. ‘FSG 401rc’] (TRC); 2) tall fescue + sunn hemp [Crotalaria juncea L. ‘Tropic Sun’] (TSH); 3) tall fescue + 67 kg ha-1 N, applied in the form of bulk urea [46-0-0] (TU). Individual paddocks measured approximately 1.21 ha-1.
The grazing period for 2020 occurred from October 6 to December 14 (69 days), and for 2021 occurred from October 11 to December 15 (65 days). Twenty-seven black Angus steers [Bos taurus] were selected to graze the nine stockpiled paddocks during the grazing period. The initial and final weights of each steer were recorded to determine total weight gain (TWG) and divided by the total days of grazing to give the average daily gain (ADG).
Herbage mass, nutritive value and botanical composition were measured. Samples were collected monthly during the grazing period from a 0.1 m2 area from each paddock. Botanical composition samples were separated into grass, legume, and weed components.
- Quantification of N-cycling as affected by grazing and pasture type (GA, NE, MI)
To measure N-cycling in animal based and mineral feralization legacies on well-drained and poorly drained soils in GA, under two replicated grazing systems each with broiler litter (BL) and mineral fertilization (M) legacies, and two soil drainage classes (well-drained to moderately-well drained) we aim to:
In two replicated grazing systems each with broiler litter (BL) and mineral fertilization (M) legacies, and three soil drainage classes (well-drained to somewhat poorly drained) we aim to:
- Quantify soil C, N, P, to 90 cm;
- Quantify C and N emissions (CO2, N2O and NH3) from soils and manure during the cool wet season and the warm season and b) determine forage quality and quantity and number of hay bales needed,
- Quantify rainfall captured and runoff water quality (concentrations of C, N, P and sediments) during the cool and warm seasons,
- Quantify fly presence, biodiversity, and their influence on C and N pools in manure and in soil (0-5, 5-10 cm, 10- 20 cm).
The project area is in Putnam county Georgia and is a complex mixture of felsic, mafic, and acid crystalline rock. Landscape is gently rolling pastures surrounded by B.F. Grant Forest, and beef and dairy farms. Through preliminary intensive reconnaissance coring and first-order soil surveys we have found well-drained to somewhat poorly drained soils within the Water Quality Pastures (WQP) depending on position within each pasture. Taxonomic classifications identified in preliminary reconnaissance are Typic Kanhapludults; Aquic Hapludults; Vertic Haplualfs, and Aquultic Hapludalfs. These soils are found throughout the Southeast. Analysis of soil and water ecosystem services will be done in Franklin lab and in the Crop & Soil Sciences Community Lab.
The WQP will have 2 heifers each and hay will be fed on an as-needed-basis. During Baseline hay is fed, near shades and waterers in all ten pastures as this is status quo (SQ). In April 2023 Soil-smart (ST) grazing will be implemented. The ST grazing treatment includes planting and excluding areas 20 m above water collection points, strategic placement of hay, release of parasitic wasps and planting of refugia/exclusions. Exclusions will be planted twice a year by over-seeding in late summer with a mixture of grass/legume/brassica (August/September) and a grass/forb/legume mixture in the spring with native forbs as refugia for predatory wasps. On the WQP, hay placement will be as usual in SQ pastures, whereas in ST, hay placement will be distributed where NDVI maps indicate poorest forage and where soil N is lowest. Number of hay bales fed will be determined by farm manager and locations will be recorded. No fertilizer will be applied during the length of the project. Baseline and After soil samples will be analyzed for differences in ecosystem services by soil drainage class, fertilizer legacy, and change from 22 to 2025. Forage biomass in exclusions will be sampled just before flash grazing for yield and quality determination. Forage yield and NDVI will be determined using Sentinel satellite data and UAV sensors and validated with forage samples in exclusions and pastures seasonal soil sampling (S2) at a minimum. Cattle condition will be measured seasonally and done by technician and trained undergraduates guided by Dr. Lawton Stewart and farm managers. Steer weights will be recorded when steers are placed and removed from WQP.
At each location, about 100 transplants were planted; 20 genotypes (several of the original 25 genotypes did not propagate well, with less than 4 transplants available), x 2 plant sizes (‘large’ – approx 3-4 months old; vs ‘small’ – approx 4 weeks old) x 2 fertilities (none, vs 2 g of starter fertilizer) + 20-25 assorted duplicates.
Plant population, flowering and plant size will be measured annually in spring, when plants are flowering
In a related study, N cycling and N-use efficiency of ruminants grazing pastures with and without forage legumes will be compared in Mead NE. Measurements will include evaluation of legume cultural and management strategies emphasizing legume establishment, N cycling and use efficiency, and GHG emissions. Specific objective (ii) Compare N cycling and use efficiency of ruminants grazing pastures with and without forage legumes.
Future objectives will be to repeat the experiment evaluating grazing pastures with and without forage legumes. The experiment compares 1) N-fertilized, 2) legume-mixed, and 3) unfertilized management systems across fall-to-spring and summer production phases of triticale and pearl millet, respectively, double cropped in sequence across a 3.6-ha field subdivided into nine, 0.4-ha pastures. In the N-fertilized system, we broadcast applied granular urea at 68 kg N/ha twice a year. The first application occurred in late March-early April on triticale. The second application occurred in early June on pearl millet. In the legume-mixed system, we co-seeded triticale and pearl millet with red clover and soybean, respectively, instead of fertilizing. In the unfertilized system, the triticale and pearl millet double crops did not receive any fertilizer or legume co-seeding.
The nine pastures accommodated three replications of each system and two cross-bred steers (Bos taurus) per pasture for 30-45 days of grazing on triticale in spring. During the spring production phase, we collected aboveground biomass samples every two weeks while cattle grazed the pastures and from exclosures for measurement of aboveground biomass accumulation in the absence of grazing. During the summer production phase, we allowed aboveground biomass to accumulate across a 70-day before subsampling for aboveground biomass and harvesting the pastures for hay.
Evaluate legume cultural and management strategies emphasizing legume establishment, N cycling and use efficiency, and GHG emissions. (AR, KY, NE, UT). Specific objective (iii) Determine the impact of legumes on the GHG footprint of livestock production systems.
We addressed this objective utilizing the double crop experiment described in objective 1, specific objective ii. In 2022, we measured soil N (NO3-N and NH4-N) three times during the growing season (before fertilizer application, after grazing, and after harvesting). The measurement of GHG emissions began in 2020 and continued in 2022. The CO2, N2O, and CH4 flux data is still under processing. In FY2022, we also conducted organic matter fractionation. Soil was fractionated into three fractions, specifically free particulate organic C and N, occluded particulate organic C and N, and mineral-associated organic C and N.
In a third study at East Lansing, MI, nitrogen cycling in biodiverse perennial forage mixtures will be measured to learn more about how biological nitrogen fixation from alfalfa affects performance of forage mixes and contributes to soil health. Design is a randomized complete block with four replications at sites in East Lansing and Hickory Corners, MI. There are 15 treatments consisting of monocultures and binary mixtures of alfalfa, orchardgrass, tall fescue, and meadow fescue in a factorial arrangement. Plots were established in 2019 and managed thereafter for optimum productivity with three to five annual cuttings. Measurement of forage yield, nutritive value, and seasonal canopy dynamics will continue through 2024 with determination of biological N fixation in first cutting using the natural abundance method. In fall 2024, standard soil health measurements will be taken from the seven-year-old stands.
1.3. Quantifying Ecosystem Services as measures of Sustainability in Soil Smart Grazing Systems
In this research, we propose using systems research to quantify differences in C, N, P, water losses and retention, filth fly presence, and biodiversity in two grazing systems each with two fertilization legacies, and two soil drainage classes in the Southern Piedmont, GA. Optimized sustainable grazing systems will retain carbon, capture and filter rainfall, retain N in plant available forms and have diverse array of beneficial insects that predate on pests of forage and cattle. Each of these are ecosystem services. In this research we will focus on quantifying ecosystem services (measures of sustainability) that grazing systems can provide: capturing rainfall to bank and filter water, reduce GHG emissions, biodiversity, and readily available plant nutrients. In earlier research we found that strategic placement of hay and exclusions, with consideration of soil and location, reduced the amount of hay needed, cleaned runoff water, and increased plant available N and P. We hypothesize that the greatest amount of plant available N and P will be retained and C will move further into the soil (beyond 20 cm) in pastures fertilized with broiler litter, with strategic placement of hay and over-seeded exclusion. Hypothesis 2: by providing fly predator refugia habitat at edges of the field (within the over-seeded exclusions) we will increase biodiversity and the number of fly predators, while also providing additional forage, and a buffer system for slowing and filtering runoff water. We will therefore also explore the impact of flies (primarily horn, stable, and face flies) impact on N and C cycling and the impact of over seeding exclusions on parasitic wasps and other fly predators influence on fly abundance and pupae morbidity. Hypothesis 3: N emissions will be least in well-drain soils with greater carbon in pastures fertilized with mineral fertilizer but greater in pastures fertilized with broiler litter. Hypothesis 4: Pastures fertilized with broiler litter will retain more rainfall (less runoff) than pastures fertilized with mineral fertilizer regardless of drainage class. We expect that for a given pasture with multiple drainage classes we will be able to develop management zones for hay placement and over-seeding recommendations. The research will be done on ten gauged pastures that have been historically fertilized with either broiler litter or mineral fertilizer. We propose sampling soils before, during, and after implementation of management changes in two grazing systems to determine the retention of C, N, P and rainfall, filth fly presence and fly pupae morbidity. Foundational information on nutrient cycling and the role insects play in ecosystem services and linkages and synergies between ecosystem services will be determined.
- N-leaching and N-dynamics to improve Soil Health. (UT, SC, MI)
In the Central Mountain region (Utah), four grass species will be evaluated in an organic dairy heifer system. The four grass species will be grown as monocultures (MONO), versus grass-legume mixtures (MIX) with four breeds of dairy heifers (Holstein, Jersey, Holstein x Jersey crossbreds, and ProCross 3-way crossbreds) also being evaluated for rate of gain. A rotational grazing management system will be used. Plant samples will be collected prior to, and after grazing events and analyzed for total N. The impact on nutrient cycling will be examined by determination of the nutrients in each phase (plant, soil, and soil water). Herbage dry matter analyses and yield measurements will be utilized to calculate the nutrients being removed in the plant phase. Soil samples will be collected at three depths (0-30 cm, 30-60 cm, and 60-90 cm) at the beginning and end of each grazing season and analyzed for available N (NO3-N, ammonium NH4-N), total N, and total C. Leachate samples will be collected bi-weekly through the growing season via zero-tension lysimeters and analyzed for NO3-N.
The agronomic responses and plant litter dynamics (N) as affected by contrasting management practices will be measured in a well-established bermudagrass field located at the Edisto Research and Education Center (EREC), SC. During the cool season, the field will be overseeded with three cool-season forage mixtures and grazed throughout the Fall and Winter months. The treatments consist of combination of triticale + annual ryegrass, triticale + annual ryegrass + clovers (red + crimson), and cereal ryegrass + annual ryegrass + clovers (red + crimson) + turnip + radish. Experimental units are 1.5 acre each, and the treatments will be arranged in a randomized complete block design with two replicates. A systems perspective will be used for general defoliation management practices to be imposed. In other words, the choice of management practices will be driven primarily by the components of the systems, and not all inputs or management practices are constant across treatments. A single 50 lb/acre of N/P/K application between 45 to 60 days after planting. A control area will be left fallow and will not receive fertilizer or be overseeded with cool-season forages. This will serve as a support area to take forage and soil samples and observed differences and persistence scores in comparison to the responses to the treatments implemented.
Variables to be determined include agronomic responses (herbage mass, nutritive value, and botanical composition) and plant litter biomass, chemical composition and decomposition rate on year 2023 and 2024. On years 2025 through 2027, nitrous oxide emissions will be determined. Prior treatment imposition and after 4 yrs of management, soil samples will be collected to determine the changes in soil C, N, and K concentrations and stocks to assess the role of forage management strategies on nutrient pattern distribution. Herbage mass will be determined using the double sampling technique which associates direct and indirect measurements of herbage mass. Pre-grazing, forage samples will be harvested to determine botanical composition. Then, all forage samples collected will be dried until constant weight and weighed, ground and analyzed for neutral detergent fiber (NDF), acid detergent fiber (ADF), crude protein (CP), lignin, in-vitro digestible organic matter (IVDOM) concentrations. The litter decomposition study will be conducted for 128 days using the methodology described by Dubeux et al (2006).
A related study to measure effects on soil health from intercropping alfalfa and corn silage at East Lansing MI. The goal is to evaluate feasibility of alfalfa as a living mulch and double crop in silage corn, allowing harvest of both alfalfa and corn in a year while improving soil health. Experimental design is a randomized complete block with a split plot treatment arrangement and four replications within two sites on sandy or loam soils. Two alfalfa varieties (sativa and falcata subspecies) were established in 2022 and silage corn is planted into alfalfa each year (2022-2024). Target is alfalfa harvest in May and October with silage corn harvested in September. Forage yield and nutritive quality will be measured. Soil samples are collected annually for health measurements.
Objective 2. Determine the role of plant secondary metabolites in ensuring improved pasture sustainability, enhancing animal health and performance, and decreasing the animal environmental footprint.
Effects of increasing symbiotic diversity on tall fescue pasture species composition, soil nutrients, alkaloid concentrations, and digestibility. Hypothesis: Greater symbiotic diversity will improve tall fescue dominance, persistence, and production, thereby reducing weed presence and simultaneously building soil nutrient stocks, without negatively affecting forage quality/digestibility.
Experimental Design: Study plots are located at the Spindletop Farm, University of Kentucky and consist of 80, 8 x 8m plots of 16 symbiotic diversity treatments ranging from zero symbiotic diversity (endophyte-free tall fescue) to stands containing one, two, three, or four symbiotic mixtures. Fescue stands were established in fall 2016 and are mowed twice a year in spring and fall, with material raked off. Biomass is harvested in all plots spring and fall, sorted by species, and weighed. Fifty fescue tillers are harvested every spring, blotted to determine whether endophyte infected, and then ground and analyzed for alkaloids. Grazing by steers will be initiated in spring 2023, immediately after plot biomass harvests. Each experimental block (n=5, each consisting of 16, 8m x 8m plots and alley ways) will be grazed individually by 20 steers for 1-2 days to ensure even utilization of forage (removal to ~10cm in stubble height). Steers will be let into the block in the morning, allowed to graze all day, and removed in the late afternoon/evening. Water troughs will be set up in an adjacent buffer zone, accessible within each block. If spring grazing is successful (i.e., does not harm plots), fall grazing will occur similarly (immediately after fall biomass harvests). By measuring biomass pre- and post-grazing in each plot, we will be able to assess removal rates. Sub-samples of the endophyte- and alkaloid-tested material will be utilized for the determination of in situ rumen rate and extent of digestibility utilizing rumen-fistulated steers and procedures described by Harlow et al. (2021).
Objective 3. Assess the efficacy of dietary factors on N-use efficiency in ruminant animals in forage-based livestock production systems and how these practices affect composition of feces and urine and rumen microbial efficiency.
To measure Rumen metabolism and nitrogen use efficiency in housed lambs on supplemented forage diets, nutrient balance studies will be conducted with lambs housed in individual metabolism pens at University of Arkansas. Lambs will be offered basal diets of forages common to the southeastern and lower midwestern US along with supplements intended to modify rumen metabolism and nitrogen use efficiency. Feces and urine will be collected and analyzed to determine dietary impacts on forms of nitrogen excretion that directly affects nitrogen use efficiency at the landscape scale.
In a related study to measure the effects of Dietary Legumes on N-excretion by organic dairy cattle under grazing in the Central Mountain region (Utah), four grass species will be evaluated in an organic dairy heifer system. The four grass species will be grown as monocultures (MONO), versus grass-legume mixtures (MIX) with four breeds of dairy heifers (Holstein, Jersey, Holstein x Jersey crossbreds, and ProCross 3-way crossbreds) also being evaluated for rate of gain. A rotational grazing management system will be used. Plant samples will be collected prior to, and after grazing events and analyzed for total N. Urine and fecal samples will be collected after each rotation by grab samples. Urine samples will be analyzed for urea-N. Fecal samples will be analyzed for Total N using a combustion method.
In a third study to determine the effects of isoflavone-associated alterations of animal urine composition on soil trace gas production potential and microbial communities, in Lexington KY, we hypothesize that BCA and/or its breakdown products interacts with soil enzymes important in the production of ammonia (e.g., urease) and nitrous oxide in ways that reduce the fluxes of these gases. We also hypothesize that BCA inhibits microbes known to be important in methanogenesis and nitrous oxide production.
Experimental Design: We will utilize isoflavone diet trials happening with fistulated steers as part of the NP 101 plan. Urine will be collected for use in soil incubations from rumen fistulated steers subjected to one of the following treatments (n = 3 steers/treatment): 1) control (isoflavone-free), 2) biochanin A, and 3) red clover (matched for biochanin A concentration). Treatments will be dosed trans-ruminally (through the cannula) and diets will be balanced for both energy and protein. Levels of red clover used will be commiserate with prior work showing animal gain effects (15-30% of diet) and at levels that could be realistically achieved with pasture grazing. Urine will be analyzed for breakdown products of interest so that compositional differences and concentrations of various compounds are known prior to initiation of soil incubations. Urine generated and collected ~2-5 hrs after commencement of the feed trials will be used for this study, as that is when isoflavone effects are likely to be most pronounced. Urine will be analyzed for the presence and concentration of BCA, genistein, and p-ethylphenol using Ultra Performance Liquid Chromatography (UPLC) equipped with a mass spectrometer, as detailed in Jacobs (2022). The experimental design will comprise 3 steers × 3 feed treatments × 8 lab replicates = 48 soil incubations
For the soil incubations/microcosms, we will utilize field collected soil from Spindeltop Farm in Lexington, KY. Soil (0-15cm) will be harvested from a field that has not had large grazers on it for >5 yrs and which currently supports a tall fescue dominated plant community. Soil will be sieved, roots removed, homogenized, and air-dried. We will use a modified static incubation procedure to determine microbial and trace gas responses to the treatments (Jacobs 2022). One hundred g of soil will be weighed into 0.9 L glass mason jars, and 5 mL urine will be added 10cm above the soil surface using a perforated paper cup to facilitate even distribution of liquid over the soil surface. This level of urine addition is representative of field excretion events. Incubations will be maintained at 65% water holding capacity. Eight ‘blank’ soil incubations – receiving only water and no urine – will also be prepared. Microcosms will be immediately capped, incubated at 20oC, and headspace CO2, CH4, N2O, and NH3 concentrations will be measured over time using a Fourier transformed infrared (FTIR) gas analyzer (Gasmet DX4040; Gasmet Technologies Oy, Finland). Measurements will occur on days 1-8, 16, 23, 30, 37, 44, 51 and 60. Rates of trace gas emissions and total quantities emitted will be calculated per microcosm. We will harvest microcosms on days 1, 3, 7, 16, and 30 to quantify soil enzymes and microbial communities. Extracellular enzyme activity will be assessed using methods described in Saiya-Cork et al. (2002) and Weintraub et al. (2007). Soil microbial community composition will be analyzed using 16S and 18S DNA techniques (Fierer et al., 2005). Mixed model analysis of variance will be used to evaluate significance of main fixed effects (feed treatment, measurement day) and random effect (animal rep) on trace gas fluxes.
Measurement of Progress and Results
Outputs
- Peer Reviewed Publications Comments: The NC1182 Committee has a strong record in peer reviewed publication. As projects are completed, results and conclusions will be published in peer reviewed journals, such as Journal of Animal Science, Agronomy Journal, Journal of Environmental Science etc.
- Presentations at annual meetings Comments: The NC1182 Committee are all members of scientific societies and organizations, and meet regularly to discuss our results and methods, identifying similarities and differences, as well as opportunities for new collaborations and research. After these meetings, we will publish our findings at the state, regional and national levels, as annual reports and extension and research publications when appropriate. Our results will include findings on N efficiency relative to forage quality and productivity and animal gain, losses in runoff, leachate, and the atmosphere and will indicate where within the N-cycle, and how, efficiencies were gained.
- Extension Articles Comments: The NC1182 Committee has a strong record in peer reviewed extension publications. Traditionally this includes Fact-Sheets and Experimental Station Reports. Other non-peer reviewed articles (but with wide readership) include publications in trade magazines, newsletters, and online blogs, and extension websites.
- Extension presentations Comments: The NC1182 Committee has a strong record in peer reviewed extension presentations. Traditional extension delivery includes in-person meetings with producers and other stakeholders. More commonly today, Extension delivery includes pod-casts, online presentations, video-clips, and You-Tube presentations on extension websites.
Outcomes or Projected Impacts
- Efficient Nitrogen Use This project has potential to impact the broader public because of its emphasis on evaluating use of legumes versus nitrogen fertilizer in forage-based livestock production systems. Greater use of legumes versus nitrogen fertilizer will reduce greenhouse gas emissions and nitrogen losses to the environment, thereby reducing impact of agriculture on climate change and costs to ameliorate non-point source pollution of U.S. water systems. More efficient N-use practices will reduce off-site N-losses through reduced erosion, reduced leaching, and reductions in greenhouse gasses such as N2O, CH4 and CO2. Use of legumes in forage-based livestock production systems also has the potential to enhance habitat for wildlife such as grassland birds that depend on seeds from forbs and pollinators like bees and butterflies that use floral resources, pollen, and nectar for nutrient and energy intake.
Milestones
(2025):Objective 1. Many of the planned trials are proposed using perennial vegetation. Field Studies in KY, OH & TN will be planted and established for more than 1 year to avoid ‘establishment year’ effects in which the disturbance from establishment often results in different result than from for established pastures. Objective 1 will require identification of genome-wide assays to query transcriptional and epigenetic changes. Associated with these arrays will require successful RNA isolation. Objective 1.2 Successful measurement of N-cycling will be conducted in NE, GA and MI. Results will be compared among states to determine if site-specific responses are observed.(2026):Objective 1. Most field experiments require repeated measurements over 2-4 years to account for environmental variation. We will implement grazing of plots in Year 1 of the project, and grazing will continue once or twice a year for the entirety of the project plan. Plant measurements will occur in concert with grazing events (spring and fall). Objective 1-3. Data analysis and manuscript preparation will begin in Year 3 and continue thereafter. In many cases, this project will report on trials established prior to the project period.
(2025):In sub-objective 3.3, the steer feeding trials will occur in year 1 of the project. Urine metabolite analyses will be conducted as soon as possible upon urine collection from feeding trials. Lab incubations will occur as soon as urine composition is known. If a dosing experiment is deemed necessary, additional microcosm work will occur in year 2. Data analysis and manuscript preparation will happen in years 3 and 4. If a field trial is attempted, it will occur in year 3, with data analysis, presentations, and manuscript prep happening in years 4 and 5.
Projected Participation
View Appendix E: ParticipationOutreach Plan
Many of the NC1182 Committee have Extension appointments, specifically, Dr’s McCulley, KY, Mendez, SD, Silva, SC, and other members work closely with colleagues with Extension appointments. Information will transfer to their respective stakeholders, via in-person meetings (1 meeting per state per year), extension publications such as Fact Sheets and newsletter articles (2 articles per year), and extension websites (5 FactSheets or articles posted per year).
Outreach from this all members of NC1182 will include publications, presentations at professional and industry meetings, electronic newsletter articles, and website blog postings that disseminated research findings related to practical forage crop and grazing systems management. Extension presentations at industry meetings and workshops will target land managers and supporting industry professionals such as agricultural seed, chemical and fertilizer dealers as well as consultants, extension educators, and public agency staff (Natural Resources Conservation and Soil and Water Conservation District personnel).
Many of the NC1182 Committee have Teaching appointments and will transfer information directly to undergraduate and graduate students in their institutions, via curriculum in Forage, Soil, and Environmental Science courses.
Organization/Governance
The recommended Standard Governance for multistate research activities include the election of a Chair, a Chair-elect, and a Secretary. All officers are to be elected for at least two-year terms to provide continuity. Administrative guidance will be provided by an assigned Administrative Advisor and a NIFA Representative.
Literature Cited
Abdalla M., A. Hastings, D.R. Chadwick, D.L. Hones, C.D. Evans, M.B. Jones, R.M. Rees, P. Smith. 2018. Critical review of the impacts of grazing intensity on soil organic carbon storage and other soil quality indicators in extensively managed grasslands. Agriculture, Ecosystems, and Environment 253:62-81.
Aguerre, M. J., M. C. Capozzolo, P. Lencioni, C. Cabral, and M. A. Wattiaux. 2016. Effect of quebracho-chestnut tannin extracts at 2 dietary crude protein levels on performance, rumen fermentation, and nitrogen partitioning in dairy cows. J. Dairy Sci. 99:4476-4486.
Animut, G., R. Puchala, A. L. Goetsch, A. K. Patra, T. Sahlu, V. H. Varel, J. Wells. 2008. Methane emission by goats consuming different sources of condensed tannins. Anim. Feed Sci. Technol. 144:228-241.
Aranjuelo, I., Arrese-Igor, C., and Molero, G. (2014). Nodule performance within a changing environmental context. J. Plant Physiol. 171, 1076–1090.
Arrese-Igor C, González EM, Gordon AJ, Minchin FR, Gálvez L, Royuela M, Cabrerizo PM, Aparicio-Tejo PM: Sucrose synthase and nodule nitrogen fixation under drought and other environmental stresses. Symbiosis 1999, 27: 189–212.
Bacon, C. W. (1995). Toxic endophyte-infected tall fescue and range grasses: historic perspectives. Journal of Animal Science, 73(3), 861-870.
Batterham, T. J., Shutt, D. A., Hart, N. K., Braden, A. W. H., & Tweeddale, H. J. (1971). Metabolism of intraruminally administered 4-C-14 formononetin and 4-C-14 biochanin A in sheep. Australian Journal of Agricultural Research, 22(1), 131. https://doi.org/https://doi.org/10.1071/AR9710131
Broderick, G. A., R. P. Walgenbach, and S. Maignan. 2001. Production of lactating dairy cows fed alfalfa or red clover silage at equal dry matter or crude protein contents in the diet. J. Dairy Sci. 84:1728–1737.
Calsamiglia, S., A. Ferret, C. K. Reynolds, N. B. Kristensen, and A. M. van Vuuren. 2010. Strategies for optimizing nitrogen use by ruminants. Anim. 4:1184-1196.
Carlsen, S. C., & Fomsgaard, I. S. (2008). Biologically active secondary metabolites in white clover (Trifolium repens L.)–a review focusing on contents in the plant, plant–pest interactions and transformation. Chemoecology, 18(3), 129-170. https://doi.org/https://doi.org/10.1007/s00049-008-0402-7
Coblentz, W. K. and J. H. Grabber. 2013. In situ protein degradation of alfalfa and birdsfoot trefoil hays and silages as influenced by condensed tannin concentration. J. Dairy Sci. 96:3120–3137.
Denison R: Decreased oxygen permeability: a universal stress response in legume root nodules. Botanica Acta 1998, 111: 191–192.
Dijkstra, J., O. Oenema, J. W. van Groenigen, J. W. Spek, A. M. van Vuuren, and A. Bannink. 2013. Diet effects on urine composition of cattle and N2O emissions. Animal. 7:s2:292-302. https://doi.org/10.1017/s1751731113000578
Eckard, R., Grainger, C., & De Klein, C. (2010). Options for the abatement of methane and nitrous oxide from ruminant production: A review. Livestock science, 130(1-3), 47-56. https://doi.org/https://doi.org/10.1016/j.livsci.2010.02.010
Flythe, M., & Kagan, I. (2010). Antimicrobial Effect of Red Clover (Trifolium pratense) Phenolic Extract on the Ruminal Hyper Ammonia-Producing Bacterium, Clostridium sticklandii. Current Microbiology, 61(2), 125-131. https://doi.org/10.1007/s00284-010-9586-5
Harlow, B. E., Flythe, M. D., Kagan, I. A., & Aiken, G. E. (2017). Biochanin A (an Isoflavone Produced by Red Clover) Promotes Weight Gain of Steers Grazed in Mixed Grass Pastures and Fed Dried-Distillers' Grains. Crop Science, 57(1), 506-514. https://doi.org/10.2135/cropsci2016.07.0590
Furey, G. N., & Tilman, D. (2021). Plant biodiversity and the regeneration of soil fertility. Proceedings of the National Academy of Sciences of the United States of America, 118(49), Article e2111321118. https://doi.org/10.1073/pnas.2111321118
Garg, M.R., P.L. Sherasia, B.M. Bhanderi, B.T. Phondba, S.K. Shelke, and H.P.S. Makkar. 2013. Effects of feeding nutritionally balanced rations on animal productivity, feed conversion efficiency, feed nitrogen use efficiency, rumen microbial protein supply, parasitic load, immunity and enteric methane emissions of milking animals under field conditions. Anim. Feed Sci. Technol. 179:24–35. doi:10.1016/j.anifeedsci.2012.11.005
Gerber, P. J., A. N. Hristov, B. Henderson, H. Makkar, J. Oh, C. Lee, R. Meinen, F. Montes, T. Ott, J. Firkins, A. Rotz, C. Dell, A. T. Adesogan, W. Z. Yang, J. M. Tricarico, E. Kebreab, G. Wagnorn, J. Dijkstra, and S. Oosting. 2013. Technical options for the mitigation of direct methane and nitrous oxide emissions from livestock: a review. Anim. 7:220-234.
Grabber, J. H. 2008. Mechanical maceration divergently shifts protein degradability in condensed-tannin vs. o-quinone containing conserved forages. Crop Sci. 48:804-813.
Harpole, W. S., Sullivan, L. L., Lind, E. M., Firn, J., Adler, P. B., Borer, E. T., Chase, J., Fay, P. A., Hautier, Y., & Hillebrand, H. (2016). Addition of multiple limiting resources reduces grassland diversity. Nature, 537(7618), 93-96.
Hector, A., Schmid, B., Beierkuhnlein, C., Caldeira, M. C., Diemer, M., Dimitrakopoulos, P. G., Finn, J. A., Freitas, H., Giller, P. S., Good, J., Harris, R., Hogberg, P., Huss-Danell, K., Joshi, J., Jumpponen, A., Korner, C., Leadley, P. W., Loreau, M., Minns, A., . . . Lawton, J. H. (1999). Plant diversity and productivity experiments in European grasslands. Science, 286(5442), 1123-1127. https://doi.org/10.1126/science.286.5442.1123
Herridge DF, Peoples MB, Boddey RM: Global inputs of biological nitrogen fixation in agricultural systems. Plant and Soil 2008, 311: 1-18.
Hoekstra, N. J., R. P. O. Schulte, P. C. Struik, and E. A. Lantinga. 2007. Pathways to improving the N efficiency of grazing bovines. Europ. J. Agon. 26:363-374.
Hungria M, Franco AA: Effects of high temperature on nodulation and nitrogen fixation by Phaseolus vulgaris L. Plant Soil 1993, 149:95–102.
Hymes-Fecht, U. C., G. A. Broderick, R. E. Muck, and J. H. Grabber. 2013. Replacing alfalfa or red clover silage with birdsfoot trefoil silage in total mixed rations increases production of lactating dairy cows. J. Dairy Sci. 96:460-469.
IPCC, 2012 – Field, C.B., V. Barros, T.F. Stocker, D. Qin, D.J. Dokken, K.L. Ebi, M.D. Mastrandrea, K.J. Mach, G.-K. Plattner, S.K. Allen, M. Tignor, and P.M. Midgley (Eds.) Available from Cambridge University Press, The Edinburgh Building, Shaftesbury Road, Cambridge CB2 8RU ENGLAND, 582 pp. Available from June 2012
Jacobs, A.A. (2022). Manipulating species diversity: environmental impacts in row crop, livestock, and grassland agroecosystems. Theses and Dissertations - Plant and Soil Sciences, 156. https://uknowledge.uky.edu/pss_etds/156
Krizova, L., Dadakova, K., Kasparovska, J., & Kasparovsky, T. (2019). Isoflavones. Molecules, 24(6), Article 1076. https://doi.org/10.3390/molecules24061076
Danner, M.S. Lett, and J.K. McCarron. 2002. Rainfall variability, carbon cycling, and plant
Lee, C., G. W. Feyereisen, A. N. Hristov, C. J. Dell, J. Kaye, and D. Beegle. 2014. Effects of dietary protein concentration on ammonia volatilization, nitrate leaching, and plant nitrogen uptake from dairy manure applied to lysimeters J. Environ. Qual. 43:398–408. doi:10.2134/jeq2013.03.0083.
Lewis, W.M. Jr., W.A. Wurtsbaugh, and H.W. Paerl. 2011. Rationale for control of anthropogenic nitrogen and phosphorus to reduce eutrophication of inland waters. Envi. Sci. & Technol. 45: 10300-10305.
Martin, C., D. P. Morgavi, and M. Doreau. 2010. Methane mitigation in ruminants: from microbe to the farm scale. Anim. 4:351-365.
Mosier, A., C. Kroeze, C. Nevison, O. Oenema, S. Seitzinger, and O. van Cleemput. 1998. Closing the global N2O budget nitrous oxide emissions through the agricultural nitrogen cycling. Nutr. Cycl. Agroecosyst. 52:225-248.
Naeem, S., Thompson, L. J., Lawler, S. P., Lawton, J. H., & Woodfin, R. M. (1994). Declining biodiversity can alter the performance of ecosystems. Nature, 368(6473), 734-737. https://doi.org/10.1038/368734a0
Newton, A. C., Begg, G. S., & Swanston, J. S. (2009). Deployment of diversity for enhanced crop function. Annals of Applied Biology, 154(3), 309-322. https://doi.org/https://doi.org/10.1111/j.1744-7348.2008.00303.x
Niderkorn, V., R. Baumont, A. Le Morvan, and D. Macheboeuf. 2011. Occurrence of associative effects between grasses and legumes in binary mixtures on in vitro rumen fermentation characteristics. J. Anim. Sci. 89:1138-1145.
Oenema, O., G.L. Velthof, S. Yamulki, and S.C. Jarvis. 1997. Nitrous oxide emissions from grazed grassland. Soil Use Mgt. 13:288-295.
Powell, J. M., M. J. Aguerre, and M. A. Wattiaux. 2011a. Dietary crude protein and tannin impact dairy manure chemistry and ammonia emissions from incubated soils. J. Environ. Qual. 40:1767–1774.
Powell, J. M., M. J. Aguerre, and M. A. Wattiaux. 2011b. Tannin extracts abate ammonia emissions from simulated dairy barn floors. J. Environ. Qual. 40:907–914.
Rojas-Downing, M. Melissa, A. Pouyan Nejadhashemi, Timothy Harrigan, Sean A. Woznicki. 2017. Climate change and livestoci: Impacts, adaptation, and mitigation.
Rudgers, J. A., Koslow, J. M., & Clay, K. (2004). Endophytic fungi alter relationships between diversity and ecosystem properties. Ecology Letters, 7(1), 42-51. https://doi.org/https://doi.org/10.1046/j.1461-0248.2003.00543.x
Saarijärvi, K. and P. Virkajärvi. 2009. Nitrogen dynamics of cattle dung and urine patches on intensively managed boreal pasture. J. Agric. Sci. (Camb.) 147:479-491
Sanderson, M. A., Skinner, R. H., Barker, D. J., Edwards, G. R., Tracy, B. F., & Wedin, D. A. (2004). Plant species diversity and management of temperate forage and grazing land ecosystems. Crop Science, 44(4), 1132-1144. https://doi.org/10.2135/cropsci2004.1132
Schils, R. L. M., J. Eriksen, S. F. Ledgard, Th. V. Vellinga, P. J. Kuikman, J. Luo, S. O. Petersen and G. L. Velthof. 2013. Strategies to mitigate nitrous oxide emissions from herbivore production systems. Anim. 7:29-40.
Takach, J. E., & Young, C. A. (2014). Alkaloid genotype diversity of tall fescue endophytes. Crop Science,
Theodorou, M. K., A. H. Kingston-Smith, A. L. Winters, M. R. F. Lee, F. R. Minchin, P. Morris, and J. MacRae. 2006. Polyphenols and their influence on gut function and health in ruminants. Environ. Chem. Letters 4:121-126.
Tilman, D. (1996). Biodiversity: Population versus ecosystem stability. Ecology, 77(2), 350-363. https://doi.org/10.2307/2265614
Tilman, D., & Downing, J. A. (1994). Biodiversity and stability in grasslands. Nature, 367(6461), 363-365.
Tilman, D., & Clark, M. (2014). Global diets link environmental sustainability and human health. Nature, 515(7528), 518-522. https://doi.org/10.1038/nature13959
Tracy, B., Foster, J., Butler, T., Islam, M., Toledo, D., & Vendramini, J. (2018). Resilience in forage and grazinglands. Crop Science, 58(1), 31-42.
Tracy, B. F., & Sanderson, M. A. (2004). Forage productivity, species evenness and weed invasion in pasture communities. Agriculture, Ecosystems & Environment, 102(2), 175-183.
Tubiello, F. N., Salvatore, M., Rossi, S., Ferrara, A., Fitton, N., & Smith, P. (2013). The FAOSTAT database of greenhouse gas emissions from agriculture. Environmental Research Letters, 8(1), 015009. https://doi.org/https://doi.org/10.1088/1748-9326/8/1/015009
van der Weerden, T. J., Luo, J. F., de Klein, C. A. M., Hoogendoorn, C. J., Littlejohn, R. P., & Rys, G. J. (2011). Disaggregating nitrous oxide emission factors for ruminant urine and dung deposited onto pastoral soils. Agriculture Ecosystems & Environment, 141(3-4), 426-436. https://doi.org/10.1016/j.agee.2011.04.007
Van Groenigen, G. L. Velthrof, F. J. E. van der Bolt, A. Vos, and P. J. Kuikman. 2005b. Seasonal variation in N2O emission from urine patches: Effects of urine concentration, soil compaction and dung. Plant Soil 273:15-27.
Vaio, N., M.L. Cabrera, D.E. Kissel, J.A. Rema, J. Frank Newsome, and V.H. Calvert II. 2008. Ammonia volatilization from urea-based fertilizers applied to tall fescue pastures in Georgia, USA. Soil Sci. Soc. Am. J. 72:1665-1672.
Wachendorf, C., C. Lampe, F. Taube, and K. Dittert. 2008. Nitrous oxide emissions and dynamics of soil nitrogen under 15N-labeled cow urine and dung patches on a sandy grassland soil. J. Plant Nutr. Soil Sci. 171:171-180.
Waghorn, G. 2008. Beneficial and detrimental effects of dietary condensed tannins for sustainable sheep and goat production: Progress and challenges. Anim. Feed Sci. Technol. 147:116–139.
Whitehead, D., Lockyer, D., & Raistrick, N. (1989). Volatilization of ammonia from urea applied to soil: Influence of hippuric acid and other constituents of livestock urine. Soil Biology and Biochemistry, 21(6), 803-808. https://doi.org/https://doi.org/10.1016/0038-0717(89)90174-0
Whittington HR, Tilman D, Powers JS: Consequences of elevated temperatures on legume biomass and nitrogen cycling in a field warming and biodiversity experiment in a North American prairie. Functional Plant Biology 2013, 40:1147–1158.
Whitham, T. G., Young, W. P., Martinsen, G. D., Gehring, C. A., Schweitzer, J. A., Shuster, S. M., Wimp, G. M., Fischer, D. G., Bailey, J. K., Lindroth, R. L., Woolbright, S., & Kuske, C. R. (2003). Community and ecosystem genetics: A consequence of the extended phenotype. Ecology, 84(3), 559-573. https://doi.org/10.1890/0012-9658(2003)084[0559:Caegac]2.0.Co;2
Zahran HH: Rhizobium-legume symbiosis and nitrogen fixation under severe conditions and in an arid climate. Microbiol Mol Biol Rev 1999, 63: 968–989.