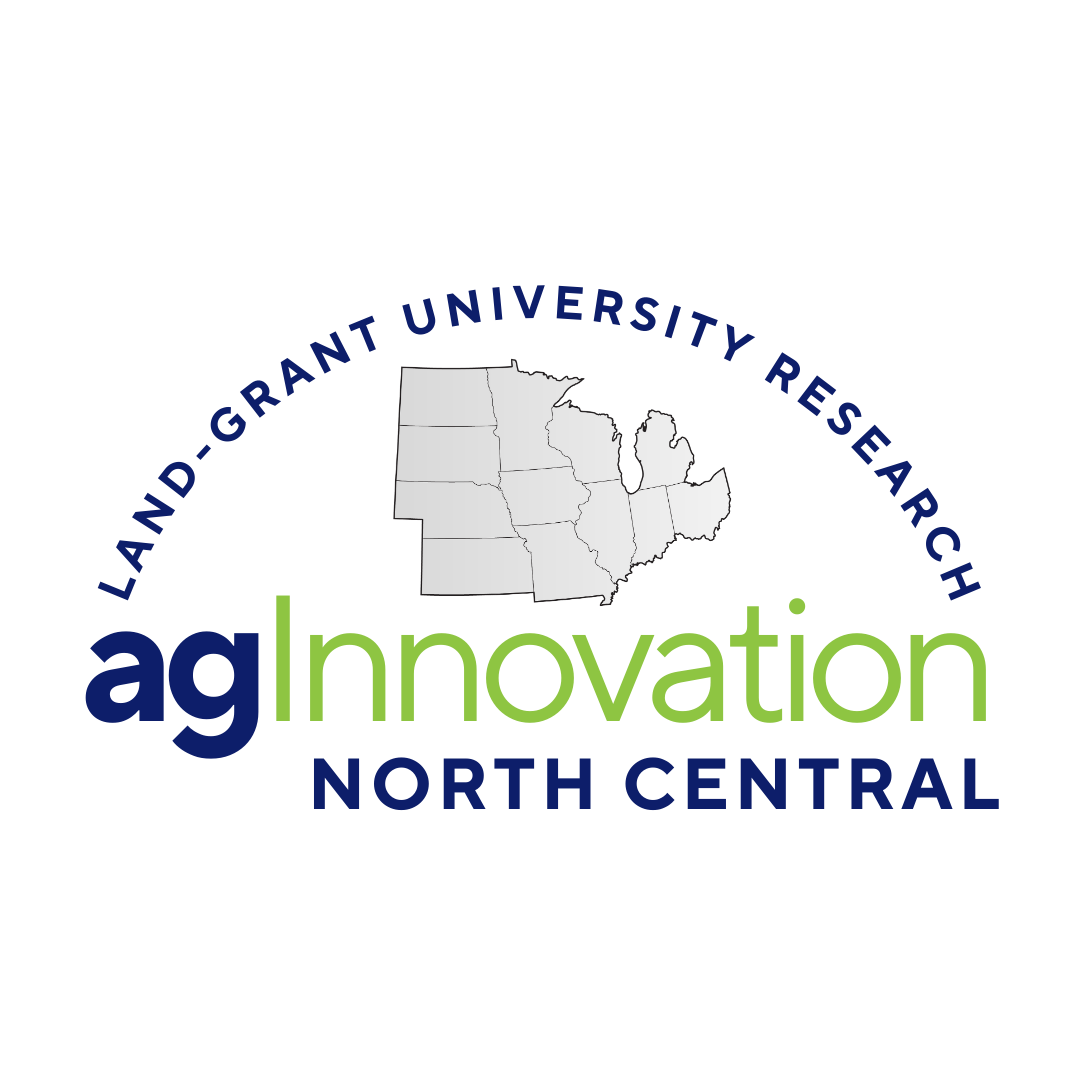
NC229: Swine Viral Diseases
(Multistate Research Project)
Status: Active
NC229: Swine Viral Diseases
Duration: 10/01/2024 to 09/30/2029
Administrative Advisor(s):
NIFA Reps:
Non-Technical Summary
NC229 membership includes representation from 16 land-grant universities, 3 federal agencies (ARS-BARC, ARS-NADC, ARS-MARC), and institutions representing 5 foreign countries (China, Canada, Spain, Cuba, and Russia). NC229 members bring diverse scientific expertise and skills to the research arena, ranging from fundamental molecular virology to epidemiology and diagnostics. Consequently, the NC229 brings an expansive array of knowledge and experience to bear on complex research projects related to swine viral diseases - far beyond the scope of possibilities for an individual investigator. In the collaborative spirit of NC229, the membership shares their latest findings at an annual Symposium in Chicago. Internationally renowned, the Symposium serves as a platform for disseminating the latest findings in the field of swine virology research. Originally designed to facilitate communications within NC229, the Symposium has evolved into a tool to deliver foundational research and practical translational insights to field practitioners, decision-makers in animal health, and biologics companies.
Statement of Issues and Justification
The NC229 project is strategically designed to address pivotal and timely cross-cutting research domains. This renewal’s goals and objectives will be highlighted in the next sections.
The North American/International PRRS Symposium, stemming from the collaborative efforts of the NC229 profoundly impacts swine virology by fostering global collaboration, showcasing innovation, and generating tangible outcomes that advance disease management, enhance swine health, and guide industry decision-making. We highlight below the impact, innovation, and outcomes that have been partially accomplished and that we hope to further develop in the next five years of this renewal.
- The NC229 research initiative will shed new insights into swine viral diseases, including PRRS and other endemic and emerging pathogens.
- Numerous new diagnostic tests and sampling strategies will be developed, collectively enhancing the timely detection and management of swine viral diseases.
- Several vaccine candidates, marked by enhanced safety and efficacy, will be generated and tested, making a substantial contribution to the successful control of crucial swine viral diseases.
- Genetic factors associated with the host’s resistance to viral diseases will be identified. This will propel the use of gene-editing technologies in the development of a novel breed of pigs resistant to viruses.
- Through the North American/International PRRS Symposium, the NC229 group will bring together researchers, veterinary practitioners, and experts from around the world, facilitating a dynamic exchange of knowledge, ideas, and experiences.
- Also through the North American/International PRRS Symposium, researchers and industry experts will continue to regularly exchange innovative strategies, interventions, and preventive measures to address the evolving challenges posed by swine viral diseases. These innovations drive progress in disease control and management; bringing cross-cutting knowledge to the end-user through extension and educational efforts.
Related, Current and Previous Work
1. Characterization of host immune responses to vaccination or natural infection
Fetus responses to PRRSV infection: We demonstrated that during fetal infection, PRRSV induces an endocrine disruption, thereby reducing animal performance. Additional studies showed that thyroid hormone levels may be promising biomarkers for genetic improvement of resilience during PRRSV challenge. We also demonstrated that PRRSV-induced hypothyroidism is not directly responsible for changes in fetal developmental processes. In addition, research groups have investigated the impact of PRRSV infection on the late gestation porcine placenta. 5-14
Swine transcriptomic response to virus infection: Using a whole transcriptome procedure, we characterized the small RNA transcriptome (sRNA-Seq) and specifically the differential microRNA (miRNA) responses in vitro in porcine alveolar macrophages (PAMs) and in vivo upon antiviral activation during viral infection.15,16
Genetic predictors of antigenic dissimilarity: We developed an in-silico machine learning algorithm that predicts antigenic dissimilarity (based on cross-neutralization data across a panel of PRRSV-1 virus and anti-sera pairs) using amino acid distances in the ectodomains of ORFs 2 to 5 and site-wise amino acid differences. The model predicts antigenic dissimilarity with 80% accuracy and could be used to develop more tailored, prescriptive immune interventions.17
New immune reagents: We developed several critical immune reagents to track cytokines and chemokines that regulate anti-viral responses.18-20
2. Antiviral host factors and the host’s innate immunity
Identification of antiviral host factors: Several antiviral RNA host factors were identified that have superior antiviral properties in terms of potentiating immune responses in pigs. Preliminary evaluation of these factors using selected interferons (IFNs) was demonstrated in vitro alone15 or through reverse genetic incorporation into a PRRSV-2 vaccine backbone.21
Innate immunity to swine RNA viruses: We examined the role of different cellular factors, including G3BP1, ZMPSTE24, and IFITM3, in PRRSV, PEDV, and SVA replication in vitro. We demonstrated the antiviral role of G3BP1 against PEDV and IFITM3 against PRRSV and SVA. We observed the effects of IFITM3 over-expression-induced autophagy in PRRSV and SVA replication. We also examined the role of DC-SIGN and L-SIGN in PRRSV and PEDV replication.22,23
PRRSV inhibits interferon-activated signaling by downregulating STAT2: Interferons are key components in innate immunity and play crucial roles against viral infection and in activating adaptive immune response via JAK/STAT signaling. STAT2 is indispensable in the JAK/STAT signaling since it is also involved in activation of antiviral activity in the absence of STAT1. PRRSV nsp11 downregulates STAT2, and the critical residue in the inhibition is K59 in the protein.24
3. Viral pathogenesis
Senecavirus A: Based on a reverse genetics system, we created a virulent and pathogenic infectious clone of Senecavirus A.25,26
New variants of PRRSV: We compared the infectivity of the recently emerged PRRSV lineage L1C 1-4-4 and confirmed that this new PRRSV variant has a higher level of infectiousness when compared to lineages L1A 1-7-9 and L9 1-4-2.
Swine Torque Teno viruses (TTSuVs): We studied the potential for cross-species transmission of TTSuVs, common contaminants of pork products and biologics. Examination of other domestic and wild animal species showed that TTSuV1 DNA was detected in 47% of equine, 70% of canine, 100% of bovine, 40% of ovine and 93% of elk sera. TTSuV1-specific antibody responses were detected only in bovine, ovine, and equine samples.27,28
4. Improve vaccine efficacy and develop new vaccines
Broadly protective PRRSV vaccines: We combined bioinformatics and molecular technologies to generate a fully synthetic PRRSV vaccine candidate that demonstrated unprecedented levels of heterologous protection. This vaccine candidate is licensed to an animal health company for commercialization.29
Novel lipid nanoparticle DNA vaccine platform: We used a DNA plasmid for vaccine delivery because it is noninfectious, nonreplicating, and safe for animals. Further, this approach is cost-effective and scalable. We enhanced its effectiveness by encapsulating it in a specialized lipid nanoparticle (LNP) formulation referred to as LNP-DNA. Pigs vaccinated with a single LNP-DNA dose containing H3N2, H1N1, or H1N2 HA genes developed high HI antibody titers within 7-14 days post-vaccination. Importantly, vaccinated pigs were fully protected against challenge infections with corresponding IAV-S strains.30
Rapid randomized mutation to attenuate RNA viruses: We pioneered a groundbreaking vaccine platform that enables the swift attenuation of newly emerging or re-emerging RNA viruses, thereby facilitating the rapid development of vaccines. Using PEDV and SIV as model viruses, we demonstrated that vaccine candidates induced robust immune responses and protection in vaccinated pigs (US Patent US20180245053A1).31,32
Minimally replicative vaccines: Although attenuated vaccines are often more effective than inactivated ones but raise concerns regarding potential reversion to virulence. To strike a balance between safety and efficacy, a precise strategy was employed to enhance the immune response and quick clearance of the attenuated vaccine strain. This was accomplished by genetically modifying serine and leucine codons so that a single mutation in these codons would result in a stop codon in the selected viral gene. This approach proved highly successful for PCV2, resulting in strong immunity, effective viral clearance, and the accumulation of stop mutations when subjected to selective pressure in vitro.33
Immune-refocused vaccines: Viruses often encode for immuno-dominant yet non-protective decoy epitopes to skew the host immune responses away from developing immunity against protective epitopes. To overcome this viral defense, a novel approach for swiftly detecting these decoy epitopes was developed.34 Reverse genetics were utilized to disrupt these identified immunodominant epitopes. Using PCV2 as a model virus, we generated an immune-refocused vaccine candidate and demonstrated that the vaccine induced broader antibody responses against heterologous field strains and provided strong protection.35
Vaccine adjuvant: We generated an innovative nanoparticle vaccine for IAV by utilizing alpha-D-glucan nanoparticles (Nano-11) in conjunction with various innate immune antagonists like poly (I:C) or STING. Our research showed that this vaccine triggered a polyfunctional and cross-protective cell-mediated immune response.36,37
Peptide-based vaccines: We developed an innovative amphiphilic inverted polymer for delivering synthetic peptide vaccine antigens, typically characterized by low immunogenicity, yet vital for eliciting precise immune responses. This vaccine delivery system proved highly efficient in the delivery of peptides that encode conserved swine influenza epitopes and exhibited a strong safety record in pigs.38
Reviews of literature: We reviewed the importance of the pig as a biomedical model.39
5. Develop new diagnostic tests
ELISA tests for ASFV: We developed a blocking ELISA for detecting antibodies against ASFV.40
ASFV surrogate assay: We developed a risk-free in situ non-animal (RISNA) surrogate assay to validate ASFV mitigation protocols.41
New sampling strategies: We assessed novel diagnostic tests and sampling methods for IAV surveillance, detection, and response during disease outbreaks. We introduced new sampling techniques, with a specific emphasis on using skin udder wipes from nursing sows to identify IAV in pig litters. Furthermore, we fine-tuned our sampling approaches to efficiently detect IAV in environmental samples, including air and farm surfaces.42,43
Metagenomics procedures to characterize viral genetic diversity: We developed a long-read sequencing pipeline to generate whole-genome sequences and conduct quasi-species analysis was developed to assess within-host diversification of PRRSV-2.
6. Swine Disease Epidemiology and Surveillance
Classification of genetic diversity of PRRSV-2: National datasets of contemporary PRRSV-2 sequences were analyzed to expand and refine the lineage-based classification system originally proposed in 2010. The diversity of PRRSV-2 wild-type and vaccine-like strains was characterized. 44-46 As a result of this recent work, lineages are now reported by all major diagnostic labs.
Understanding and predicting strain emergence and spread of PRRSV-2: Using national scale datasets, we showed that a new sub-lineage of PRRSV-2 emerges every one to five years and that the dominant sub-lineage turns over every three years. We have also documented that newly emerging sub-lineages typically harbor substitutions that result in amino acid changes in epitopes and N-glycosylation sites that may impact their fitness and ability to evade host immunity. Using these insights, we developed a statistical model that predicts the emergence potential (or future epidemiologic success) of genetic variants based on early indicators measured from phylogenetic trees. Using whole genome sequence data, we also demonstrated that the newly emerged PRRSV L1C 1-4-4 outbreak variant has a recombinant origin.44-48
Understanding risk factors for SVA exposure: We investigated the seroprevalence of SVA and risk factors associated with the U.S. breeding and growing herd pig population.49
7. Swine Disease Management and Biosecurity
PRRSV transmission via animal movements: Using genomic sequence and animal transport data, we have quantified patterns of PRRSV-2 spread within and between regions in the U.S. We showed that movement of feeder pigs, not weaned pigs, drives the dissemination of the virus at medium spatial distances.50-54 We also assessed and modeled additional transmission routes (vehicles and feed) for PRRSV.
Dynamics of PRRSV infection at farm levels: We quantified the genetic diversity of PRRSV within pigs and litters during an outbreak on a sow farm.55 Additionally, we quantified the duration that U.S. breeding farms can remain PRRS naïve.56 Finally, we investigated farm and management risk factors associated with the introduction of wild-type PRRSV introductions into wean-to-finish herds located in the Midwest in an effort to improve biosecurity practices.
Influenza A virus: We conducted genetic and antigenic characterization of new and emerging swine IAVs, including phylogenetics and network analysis. We investigated the impact of vaccination on decreasing the risk of influenza virus reassortment. Investigated farm workers' roles in introducing seasonal influenza viruses into swine farms.57,58
Characterizing the incidence of PEDV infections: We quantified the incidence of PEDV infections in growing pigs before and after biosecurity interventions. 59
8. Surveillance and Reporting Systems
The Early Regional Occurrence Warning (TEROW) tool was launched in May 2023 to alert producers and veterinarians of recent outbreaks of PRRS in their neighborhoods. https://www.swinehealth.org/regional-disease-warning-tool-results-from-shic-funding-2/
The Morrison Swine Health Monitoring Project (MSHMP) continues to provide weekly disease (e.g., PRRSV, PEDV, SVA) occurrence and elimination metrics to the U.S. industry using data from approximately 60% of the breeding population. The website has been updated with the goal of ensuring access to academia and industry: https://mshmp.umn.edu
The Swine Disease Reporting System (SDRS) has been providing monthly reports through multiple platforms (newsletters, podcasts, and YouTube videos) of the major disease trends. The collated cumulative data from the major Veterinary diagnostic laboratories also provides the opportunity to forecast the behavior of different diseases in specific production areas of the country. https://fieldepi.org/swine-disease-reporting-system/.
ARS scientists in Ames, Iowa, with APHIS colleagues, developed a publicly available interactive software system called octoFLUshow to visualize influenza A virus gene sequences collected in the USDA Influenza A virus surveillance system. When deployed alongside sampling algorithms (smot: simple manipulation of trees and flutile: flu utilities) federal scientists, academic researchers, and diagnostic labs can determine representative swine IAV genetic sequences that can be further characterized to determine phenotype. These tools will aid agricultural production and diagnostic capabilities through the identification of important changes in genetic diversity and allow for the identification of novel viruses. The software and interactive website are publicly available in repositories accessed through https://flu-crew.org
9. Outreach activities
The North American/International PRRS Symposium constitutes a significant spinoff directly derived from the NC229. As a direct continuation of the initial NC229 meetings held around the CAP 1, The North American PRRS Symposium became an essential scientific communication instrument that complemented the basic research produced by NC229 with translational technology messages targeted to field practitioners, animal health decision-makers, and biologics companies. The NC229 multistate annual meeting helped to disseminate the scientific advances contemplated within this project's objectives. More than 15 researchers shared the advances in PRRSV immunology/vaccinology and epidemiology.
The Allen D. Leman Swine Conference is an annual educational event for the global swine industry organized by faculty at the University of Minnesota College of Veterinary Medicine. It is internationally acclaimed for bringing science-driven solutions to the complex challenges facing the industry. The conference is usually attended by over 900 participants from over 20 countries, including swine veterinarians and other professionals working in swine production and animal health management.
Objectives
-
Etiology, pathogenesis (host-virus interaction, transmissibility) and genetic analysis of viruses 1a: Virus evolution, mechanisms of tropism and virulence 1b: Viral gene-protein structure and function, replication 1c: Host mechanisms associated with resistance, resilience and susceptibility
-
Epidemiological investigation of viral pathogens that affect swine population in the United States. 2a. Diagnostics and surveillance 2b. Spatial-temporal pattern and risk factors 2c. Outbreak investigations 2d. Disease prevention, control and elimination • Biosecurity-biocontainment 2e. Production/Economic impact
-
Immunology, Vaccinology, and Antiviral drugs
Comments: 3a. Immunology • Investigate innate immunity against porcine viruses and study the viral antagonism against innate immunity. • Fetal response to viral infection and immune pathways that would result in fetal resilience to PRRSV infection. • Transcriptome response and single-cell, small RNA, non-coding RNA pathway associated with acute virus infection. • Host factors contribute to immune responses against viruses. • Study the virus-induced immunopathogenesis. • Define immune markers associated with the memory responses to vaccines and virus infection. • Correlation of immune protection. • Comparative immunology to reveal immune responses to different viruses in susceptible multispecies host 3b. Vaccinology • Identification of protective antigens for vaccine design • Exploring alternate routes of vaccination such as intradermal, intranasal, oral vs intramuscular depending on the site of infection the pathogen is primarily associated with the host. • Find the approach to reduce the cost of mRNA vaccines. • Developing new vaccine platforms to enhance the duration and magnitude of immunity. • Multivalent vaccine approach • One health approach: zoonotic diseases such as coronavirus and influenza virus vaccines and use farm animals as biomedical models to understand disease pathogenesis and immune responses in humans. • Identification of immunodominant epitopes to pathogens to use in vaccines. • Innovative approaches for rapid vaccine development to respond to newly emerging viruses. • Identification and evaluation of new vaccine adjuvants to enhance the magnitude and duration of vaccine-induced immunity. • Effective vaccine delivery platforms to deliver vaccine antigens to pigs • Differentiating infected from vaccinated animals. • Microbiome Influence on vaccine efficacy. 3c. Antiviral drugs • The major limitation will be the cost to be used in farm animals, but it is possible that it can be used in animal models for the discovery of antiviral drugs that can be applied to human medicine. Also, low-cost alternatives can be explored. • Develop broad antiviral inhibitors targeting host factors that are essential for virus replication such as host kinase. • Identification of key host proteins that have broad antiviral effects, such as interferons and interferon-activated genes. • Explore nucleic acid-based antiviral approaches, such as siRNA and CRSPR/Cas system.
Methods
Objective 1: Etiology, pathogenesis, and virus-host interaction.
1a. Virus evolution, mechanisms of tropism and virulence
For Objective 1a, the NC229 consortium will use phylodynamic models to investigate the molecular clocks of swine viruses to understand the rate of evolution, and better understand and predict the emergence of new viral strains. Additionally, we will pursue research that analyzes national-level datasets to better classify genetic variability and quantifies the frequency and phenotypic consequences of recombination through genomic investigations of virus evolution both in the field and experimentally. Using both field-based and experimental approaches, we will also evaluate how evolution is shaped by immune-mediated pressures, with implications for the effectiveness of antivirus treatments and vaccination. For emerging pathogens, NC229 pursues research on the genetic factors that help viruses move between species, including their zoonotic potential. Knowledge gaps related to the mechanism of tropism that NC229 will pursue include but are not limited to, detecting and characterizing cellular receptors and attachment factors that determine the tropism of swine viruses, which will advance understanding of the viral receptor and its presence in different tissues. There is also a need to conduct more research on viral proteins that are responsible for binding to host cell receptors and how changes in these proteins alter virus entry and pathogenesis. Research will focus not only on well-characterized lab strains but also on emerging virulent viral strains, as these may have distinct phenotypes and have the most relevance to the situation in the field. Gaps in virulence factors and immunopathogenesis should be addressed as well, including identifying the specific viral and host factors that contribute to the virulence and neutralization of swine viruses. This includes studying viral proteins/epitopes and the impact of viral replication and neutralization in the host. Some swine viruses are immunosuppressive (PRRSV), and some are more pathogenic when the host is immunostimulated (PCV2, ASFV). We will also pursue more detailed investigation of molecular pathways and signaling cascades involved in virus virulence.
1b. Viral gene-protein structure and function, replication
To understand the structural characteristics that confer evolutionary advantages with regards to viral replication and evasion of the host antiviral immune system, for Objective 1b, NC229 members will employ a wide variety of classical and cutting-edge molecular methodologies. Experimental stations working in this area will focus their efforts on evaluating the functional advantage of individual viral genes, primary protein structure along with altered post-translational modification.
1c. Host mechanisms associated with resistance, resilience, and susceptibility
Work in this area will continue to focus on biological extremes selected using phenotypic data collected from large-scale challenge models. Experiments will continue to utilize archival samples from previous trials including the “PRRS host genome consortium” (PHGC), “Pregnant Gilt Model” (PGM), and Natural Disease Challenge Model (NDCM). Where suitable samples are not available to test novel hypotheses, new challenge studies will be conducted including additional planned iterations of the PGM. Samples will be shared between experimental stations to leverage the necessary molecular expertise and equipment. Ongoing multi-institutional collaborations will investigate the tissue-specific factors associated with resilience to late gestation PRRSV infection. This project will bring together the expertise and equipment necessary for Nanostring transcriptomics, with expertise in bioinformatics and developmental physiology. Using a hypothesis-driven approach, Nanostring code sets will be collaboratively designed to target specific pathways. Appropriate achieved fetal tissue samples will be from previous iterations of the pregnant gilt models, and RNA or tissues transferred for transcriptomic assessment.
Objective 2: Epidemiological investigation of viral pathogens that affect swine population in the United States.
2a. Diagnostics and surveillance
Objective 2a will include developing and implementing cutting-edge diagnostic tools and surveillance systems to enable rapid disease detection, monitoring, and response in swine populations. Achieving this objective will help accomplish five important advancements, which include enhancement of early disease detection, improvement of surveillance infrastructure, continue facilitation of data integration, improvement of rapid response protocols, and promotion of education and outreach. The first advancement will allow for improvement of disease control by enhanced diagnostic capabilities and surveillance systems that enable swine producers to identify and address disease threats more efficiently, reducing the impact of outbreaks on animal health and economic stability; while the second advancement will improve surveillance infrastructure by establishing a comprehensive, real-time monitoring system capable of collecting and analyzing data from various sources, such as farms, slaughterhouses, and veterinary clinics. The third and fourth advancements will facilitate data integration by developing further integration and analysis platforms that allow seamless sharing and interpretation of disease-related information among stakeholders, including veterinarians, producers, and regulatory agencies; implementing rapid response protocols by establishing and disseminating disease response protocols that enable swift containment and mitigation of disease outbreaks, minimizing economic losses and public health risks. This will increase food safety by promptly detecting and managing diseases, reducing the risk of contaminated pork products entering the food supply, and contributing to improving food safety and public health. It will also contribute to making the swine industry more resilient to unforeseen disease challenges, safeguarding its long-term viability. The last advancement will involve the development of educational materials and training programs to ensure that swine producers and veterinarians have the knowledge and skills to implement effective disease diagnostic and surveillance strategies, fostering a culture of proactive disease management.
2b. Spatial-temporal pattern and risk factors
Emerging and re-emerging viral diseases of swine often exhibit complex spatial and temporal patterns, and their occurrence is influenced by various risk factors. To better understand and mitigate these diseases, it is imperative to investigate and analyze the spatial-temporal dynamics and the underlying risk factors associated with their emergence and transmission. Objective 2b focuses on advancing our knowledge in this area to enhance disease management and elimination strategies within the swine industry. To achieve this objective, we will continue to advance the analyses of spatial-temporal patterns of swine viruses, identify risk factors contributing to the emergence and spread of viral diseases in swine, develop predictive models that integrate spatial-temporal data and risk factor information to forecast disease outbreaks and identify high-risk regions, and collaborate with stakeholders to ensure that research findings are translated into practical, actionable recommendations. The expected outcomes of Objective 2b aim to provide swine producers and industry stakeholders with valuable insights into the spatial-temporal dynamics of emerging viral diseases, enabling them to implement proactive disease management measures and enhance their preparedness; enable targeted interventions by identifying high-risk areas and risk factors, facilitating more efficient resource allocation for disease prevention and control efforts; reduce the economic impact of disease outbreaks by developing strategies to mitigate disease spread in high-risk regions and seasons; promote sustainable swine production practices by identifying environmentally sensitive areas and practices that may contribute to disease emergence and transmission, leading to more responsible practices; and hare research findings and predictive models with swine producers and stakeholders to foster informed decision-making and build a collaborative approach to disease management.
2c. Outbreak investigations
Outbreak investigations in swine medicine are crucial for identifying and managing diseases that affect pig populations. The goal of these investigations should be to establish a systematic process to determine the cause of the outbreak and implement control measures. Outbreak investigations often begin by detecting unusual clinical signs or spikes clinical disease in the population. However, in some instances, newly detected agents lack a "case definition". Therefore, efforts should be focused on reinforcing case definitions to accelerate investigation on new syndromes or improve those already used for field investigation. In addition, to case definition, investigators should reinforce what constitutes a disease diagnosis based on clinical signs, laboratory test results, and epidemiological criteria. Another important piece within Objective 2c will be the reinforcement of multidisciplinary teams of clinicians, epidemiologists, and laboratories that visit affected farms to collect information and samples. As such, epidemiologic and laboratory research should provide the best information possible to understand the emergence of new pathogens as well as the dynamic and impact of those already present in the swine population. Epidemiological investigations should be guided by investigators who analyze the data and help determine the disease's source and spread within and between farms/populations. Once the causative agent is identified, additional efforts to characterize strains and assess their virulence must be considered to confirm the effect these pathogens detected in the field. Finally, research findings should include recommendations and measures to prevent disease spreading. Effective communication with farmers, industry stakeholders, and regulatory authorities is essential to coordinate control efforts and prevent further spread; and this will be incorporated in the execution of this objective.
2d. Disease prevention, control, and elimination
Despite ongoing biosecurity efforts and planning, the need for comprehensive research and practical recommendations in this field remains evident. This objective focuses on enhancing biocontainment and bioexclusion strategies to fortify the swine industry's ability to prevent, control, and ultimately eliminate infectious diseases. As such, Objective 2d will investigate and implement state-of-the-art biocontainment measures within swine production facilities, develop and refine bioexclusion protocols to prevent the entry of pathogens into swine facilities, develop educational programs and resources for swine producers and industry stakeholders, develop and disseminate effective disease control strategies that encompass biosecurity, vaccination, treatment, and depopulation when necessary, and investigate strategies for the targeted eradication of specific endemic diseases. One of the main expected outcomes of this project is to minimize disease spread by strengthening biocontainment and bioexclusion measures, reducing the risk of disease introduction and transmission within swine production facilities and preventing widespread outbreaks. Furthermore, through education and outreach, we aim to foster a culture of biosecurity and disease prevention among swine producers and industry participants of all sizes, promoting responsible practices and collaborative efforts. This is expected to result in economic stability by reducing the impact of endemic diseases within the industry, and enhancement of foreign animal disease preparedness by specifically addressing biosecurity gaps/ concerns. When it comes to disease eradication, this Objective aims to explore industry-wide options for regional- or national- wide disease elimination.
2e. Production/Economic impact
Quantifying the impact and cost of viral infectious diseases on swine production requires improvements in data collection, analysis, and evaluation. Within data collection, we will be focused on establishing regional programs to evaluate disease and pathogen prevalence. Data should also include production metrics gathered from affected and non-affected swine herds. Key metrics should include growth rates, mortality rates, reproductive performance, and feed conversion ratios. Efforts will also be made toward integrating economic data, including revenue losses (e.g., reduced sales due to lower weight gain or increased mortality) and operational costs related to disease management (e.g., veterinary expenses, medication, biosecurity measures). For data analysis, we aim to establish mathematical and computational models that allow real-time production data analysis to assess the impact of viral diseases. Other analyzes include comparison of performance metrics between affected and non-affected herds, and economic modeling to estimate the overall economic impact of viral diseases in swine production. This will include calculations of losses in revenue and increased operational costs attributable to the diseases and will consider both short-term and long-term economic effects. Comparative analyses on these topics will aim to include data across regions, production systems, or management practices to identify trends and patterns in disease impact that can be used to tailor control measures to specific circumstances. Research programs will also perform a cost-benefit analyses to evaluate the economic feasibility of implementing disease prevention and control strategies and potential return on investment (ROI) of interventions, e.g., vaccination, improved biosecurity, or changes in management practices. Lastly, evaluation of data and results in this area of research will emphasize the importance of interdisciplinary programs, real-time data analysis, and economic modeling in assessing the impact of viral diseases on swine production. This comprehensive approach will provide a more accurate understanding of the challenges faced by the industry and aid in developing effective disease management and mitigation strategies. The findings provided by Objective 2e will provide a comprehensive assessment of the production and economic impact of viral diseases in swine production; information that is critical for stakeholders including farmers, veterinarians, and policymakers to make informed decisions regarding disease management and prevention.
Objective 3. Immunology, vaccinology, and antiviral drugs
3a. Immunology
Understanding host immune responses to viral infections is crucial for the rational design of safe and effective vaccines. Viruses, such as PRRSV, have evolved complex mechanisms to antagonize and evade the host immune system. For instance, viruses can suppress type-I interferon, a key component of the host's innate immune response to viral infections. Likewise, they are able to escape the induction of neutralizing antibodies, as well as T-cell responses. As viruses involved in PRDC rarely occur alone, it is also important to understand the interactions between viruses to develop effect protective measures. We will leverage cutting-edge technologies to investigate swine's immune responses to viral infections over the next five years. The following are our priority topics of study.
- Viral suppression of innate immunity and virus host interaction: We will employ molecular and biological assays to screen for viral and host genes that suppress the host's innate immune pathways, such as the STING signaling pathway, Toll-like receptors, inflammasome pathways, and the interferon signaling pathway. Subsequently, detailed mechanisms of suppression will be studied. The role of ISGs and other cellular factors in restricting virus replication and its underlying mechanisms will be examined.
- Host responses to viral infection: We will incorporate bulk- and single-cell- RNA sequencing to characterize the host transcriptome responses to viral infections. The study will be conducted on pigs at various life stages, including fetal pigs and porcine organotypic models, to identify host factors and immune pathways associated with the host’s resilience to viral infection.
- Immune correlates of protection and virus-induced pathogenesis: Vaccinated and non-vaccinated control pigs will be experimentally infected with virulent viruses and protection outcomes will be recorded. Immunological assays will be used to identify immunological parameters that correlate with vaccine-induced protection or virus-induced pathogenesis. Similarly, we will employ appropriate assays to identify immune markers associated with memory B and T cell responses to vaccines and virus infections.
3b. Vaccinology
Vaccination is the most cost-effective method for controlling and eradicating infectious diseases in livestock. An example of success is the eradication of Aujeszky's disease virus, a DNA virus, (also known as Pseudorabies) from the U.S. domestic swine population through extensive vaccination/testing campaigns. However, several other viral diseases, especially RNA viruses such as PRRSV, IAV, and PEDV, continue to cause substantial losses to the swine industry because commercial vaccines offer only limited cross-protection. Additionally, the emergence of new viruses and viral variants necessitates the rapid development of safe and effective vaccines to mitigate their impact. We will utilize holistic approaches to discover solutions that improve the safety and efficacy of existing vaccines and to develop new vaccines for emerging viruses.
- Broadly protective vaccines: RNA viruses, such as IAV and PRRSV, exhibit exceptional genetic/antigenic diversity, which represents a formidable challenge to the development of a broadly protective vaccine. We will utilize bioinformatics and immunoinformatic approaches to analyze the genetic sequences of these viruses and design vaccine antigens that potentially induce broad protection against multiple viral variants co-circulating in swine herds.
- Protective viral antigens: We will combine analysis of the host immune responses and viral structures to identify viral proteins capable of inducing protective immunity in pigs. We will also evaluate the extent to which cross-neutralization amongst viruses and anti-sera is associated with genetic variability in viral epitopes. These identified proteins as well as knowledge of the antigenic implications of genetic variability in epitopes will be critical for developing subunit vaccines.
- Novel vaccine platforms for the rapid development of vaccines against emerging viruses. We will focus on multiple vaccine delivery platforms, such as mRNA or DNA plasmids and nanovaccine delivery platforms - primarily due to their safety profile. Naked mRNA or DNA plasmids often exhibit extremely low immunogenicity because they do not enter host cells effectively. Therefore, we will develop nanoparticle formulations that can enhance the uptake of mRNA or DNA or protein antigens into the host cells and consequently augment the induction of immune responses.
- Vaccine administration. Many swine vaccines are monovalent. Thus, multiple vaccines must be administered to the pigs. We will explore approaches to combine these vaccines against multiple pathogens into a single vaccine - without compromising their immunogenicity, thus, reducing the cost and labor associated with vaccine administration. Additionally, we will explore alternative routes for vaccine delivery, such as intradermal, intranasal, and oral routes, to maximize immunity at the pathogens primary site of infection.
- Adjuvants. Adjuvants are a critical component of vaccines as they augment the magnitude and duration of vaccine-induced immunity. We will search for novel adjuvants that can enhance animal immune responses to vaccination while minimizing side effects.
- Differentiating infected from vaccinated animals (DIVA). Live-attenuated vaccines often generate immune responses that cannot be differentiated from natural infection. This can impede the use of serological tests for diagnosis. To address this issue, we will employ molecular techniques to eliminate at least one antigenic protein (known as a marker antigen) from viral vaccine strains. This modification will prevent vaccinated animals from mounting an antibody response against the marker antigen, facilitating the differentiation of naturally infected from vaccinated animals. Such vaccines are instrumental in controlling and eradicating infectious diseases, as they allow for the identification of naturally infected animals within the vaccinated population, enabling targeted measures for virus elimination.
- Influence of the microbiome on vaccine efficacy. Animals will be vaccinated and challenged with a corresponding virulent virus, and protection outcomes will be recorded. Metagenomics will be employed to analyze the microbiomes of vaccinated and protected animals following the challenge infection. The association between the microbiome compositions and vaccine efficacy will be analyzed.
3c. Antiviral drugs
Antiviral drugs are generally impractical for farm animals due to their high cost. Nevertheless, we will leverage farm animals as models for discovering antiviral drugs targeting swine viruses with zoonotic potential because such research significantly contributes to the improvement of human health. Viruses rely on the host's cellular machinery for their replication. Therefore, we will utilize biochemical and molecular virology methods to identify host factors with broad-spectrum antiviral properties, including interferons and interferon-activated genes. Building on this knowledge, we will search for inhibitors that target these critical host factors to impede virus replication. Currently, there is a vast array of chemical compounds available in commercial libraries. We will combine computational biology with viral infectivity assays to screen these libraries for compounds exhibiting broad-spectrum antiviral effects. Subsequently, we will identify host factors specifically associated with these compounds.
Measurement of Progress and Results
Outputs
- Advanced understanding of viral biology Comments: Our results will provide new insights into the viral protein structure and functions, molecular factors that control viral tropisms and virulence and host mechanisms associated with viral resistance, resilience, and susceptibility.
- Novel assays for detection and diagnosis of viral diseases: Comments: We will develop and validate new assays and sampling methods to enhance our ability to detect viruses more accurately and efficiently. These assays will contribute to our capacity to monitor and control diseases.
- Phylodynamic models for virus evolution: Comments: We will develop and utilize phylodynamic models to study the rate of evolution and genetic diversity of swine viruses, leading to a better understanding of how novel viral strains emerge.
- Novel and efficacious disease control strategies: Comments: We will develop safe and effective vaccines that provide broad protection against diverse viruses. Additionally, we will create practical biosafety measures to prevent disease incursions.
Outcomes or Projected Impacts
- Accurate disease detection and epidemiological insights: Our research will produce disease prevalence assessments and economic impact analyses, thereby empowering informed decision-making.
- Enhanced swine health and well-being: Our research will yield effective vaccines and antiviral drugs, leading to advancements in swine health, well-being, and productivity. This collective enhancement will strengthen the competitiveness of the U.S. swine industry.
Milestones
(2029):This project is planned for a 5-year duration, with 3 distinctive objectives that run concurrently. Activity/Milestone Year 1 Year 2 Year 3 Year 4 Year 5 Objective 1: Etiology, pathogenesis, and virus-host interaction. 1a. Virus evolution, mechanisms of tropism and virulence 1b. Viral gene-protein structure and function, replication 1c. Host mechanisms associated with resistance, resilience and susceptibility Objective 2: Epidemiological investigation of viral pathogens that affect swine population in the United States. 2a. Diagnostics and surveillance 2b. Spatial-temporal pattern and risk factors 2c. Outbreak investigations 2d. Disease prevention, control and elimination 2e. Production/Economic impact Objective 3. Immunology, vaccinology, and antiviral drugs 3a. Immunology 3b. Vaccinology 3c. Antiviral drugsProjected Participation
View Appendix E: ParticipationOutreach Plan
We are committed to addressing the diverse needs of our stakeholders, i.e., swine producers, practitioners, veterinary diagnosticians, researchers, and animal health regulatory agencies. To achieve this objective, we will employ a range of platforms, including peer-reviewed scientific journals, scientific conferences, and web-based methods to facilitate the effective dissemination of new knowledge to these stakeholders. The following outreach plan is formulated based on our track record of successful outreach and our commitment to collaboration.
- Scientific Peer-Reviewed Publications: We recognize the importance of peer-reviewed journal articles in disseminating our research findings. To ensure that our research reaches both the scientific community and a wider audience, we will prioritize publishing high-quality research articles in influential scientific journals.
- Scientific Conferences: The NC229 consortium actively contributes to the organization of the NAPRRS/NC229 International Conference of Swine Viral Diseases. This Symposium has gained international recognition, serving as a vital forum for uniting members of the swine disease community from around the world. Additionally, our members play a significant role in the organization of the Allen D. Leman Swine Conference, an annual educational event for the global swine industry, organized by faculty at the University of Minnesota College of Veterinary Medicine.
- Graduate Student Training: Our commitment extends to nurturing the next generation of scientists with a focus on viral swine diseases. Annually, we will provide travel awards to support 10-15 graduate students in attending the NAPRRSV/NC229 Symposium. This investment in graduate education ensures a well-prepared and knowledgeable workforce for the future.
- Extension services: We will create and distribute educational materials directly to swine producers, e.g., manuals, pamphlets, and literature on topics related to biosecurity, the implementation of biosecurity measures, and biosecurity monitoring.
Organization/Governance
The NC229 consortium will be led by an Executive Committee consisting of the Chair, Vice Chair, Secretary, and a Member-at-Large. Each position will have a two-year term, following a rotational succession plan: the Vice Chair transitions to the Chair role, the Secretary assumes the Vice Chair position, and the Member-at-Large takes on the responsibilities of the Secretary. Elections for the Member-at-Large position will occur biennially, with consortium members nominating candidates and balloting taking place at the annual meeting. During their term, the Chair and Vice Chair are responsible for organizing and overseeing the annual meeting. The Secretary's duties include documenting the meeting's progress, communicating outcomes to the membership, and preparing NC229 annual reports for submission to NIMSS. Administrative guidance will be provided by an assigned Administrative Advisor and a National Institute of Food and Agriculture (NIFA) Representative. Decision-making within the consortium will be a collaborative process, involving input from all relevant parties. Major decisions will be determined by the Executive Committee, with the Advisor providing external guidance.
Literature Cited
1 NPPC. Report Highlights Pork Industry Contributions To U.S. Jobs, Economy. https://nppc.org/capital-update/for-the-week-ending-july-15-2022/ (2023).
2 Schulz, L. L. & Tonsor, G. T. Assessment of the economic impacts of porcine epidemic diarrhea virus in the United States. J Anim Sci 93, 5111-5118.
3 Carriquiry, M., Elobeid, A., Hayes, D. & Zhang, W. Impact of African Swine Fever on US and World Commodity Markets. Iowa State University Center for Agriculture and Rural Development https://www.card.iastate.edu/ag_policy_review/article/?a=101 (2020).
4 Carriquiry, M., Elobeid, A., Swenson, D. & Hayes, D. Impacts of African Swine Fever in Iowa and the United States. CARD Working Papers. 618. https://lib.dr.iastate.edu/card_workingpapers/618 (2020).
5 Ison, E. K. et al. Compensatory mechanisms in response to induced hypothyroidism in the late gestation pig fetusdagger. Biol Reprod. 2023 May 10;108(5):731-743.
6 Ison, E. K. et al. Effects of porcine reproductive and respiratory syndrome virus (PRRSV) on thyroid hormone metabolism in the late gestation fetus. Vet Res. 2022 Sep 30;53(1):74.
7 Van Goor, A. et al. Genome wide association study of thyroid hormone levels following challenge with porcine reproductive and respiratory syndrome virus. Front Genet. 2023 Feb 9;14:1110463.
8 Mulligan, M. K. et al. Porcine reproductive and respiratory virus 2 infection of the fetus results in multi-organ cell cycle suppression. Vet Res. 2022 Feb 21;53(1):13.
9 Pasternak, J. A. et al. Thyroid hormone suppression in feeder pigs following polymicrobial or porcine reproductive and respiratory syndrome virus-2 challenge.. J Anim Sci. 2021 Nov 1;99(11).
10 Pasternak, J. A. et al. Maternal and fetal thyroid dysfunction following porcine reproductive and respiratory syndrome virus2 infection. Vet Res. 2020 Mar 30;51(1):47.
11 Barrera-Zarate, J. et al. Detection of PRRSV-2 alone and co-localized with CD163 positive macrophages in porcine placental areolae. Vet Immunol Immunopathol. 2022 Aug;250:110457.
12 Barrera-Zarate, J. A. et al. EffecAbbreviationst of porcine reproductive and respiratory syndrome virus 2 on angiogenesis and cell proliferation at the maternal-fetal interface. Vet Pathol. 2022 Nov;59(6):940-949.
13 Guidoni, P. B. et al. Effect of porcine reproductive and respiratory syndrome virus 2 on tight junction gene expression at the maternal-fetal interface. Theriogenology. 2022 May;184:162-170.
14 Malgarin, C. M. et al. Fetal hypoxia and apoptosis following maternal porcine reproductive and respiratory syndrome virus (PRRSV) infection. BMC Vet Res. 2021 May 1;17(1):182.
15 Li, J. et al. Comparative transcriptomics reveals small RNA composition and differential microRNA responses underlying interferon-mediated antiviral regulation in porcine alveolar macrophages. Front Immunol. 2022 Oct 28;13:1016268.
16 Fleming, D. S., DS, F., Miller, L. C. & LC, M. Differentially Expressed MiRNAs and tRNA Genes Affect Host Homeostasis During Highly Pathogenic Porcine Reproductive and Respiratory Syndrome Virus Infections in Young Pigs. Front Genet. 2019 Aug 2;10:691.
17 Makau, D. N. et al. Predicting Antigenic Distance from Genetic Data for PRRSV-Type 1: Applications of Machine Learning. Microbiol Spectr. 2023 Feb 14;11(1):e0408522.
18 Dawson, H. D., HD, D., Lunney, J. K. & JK, L. Porcine cluster of differentiation (CD) markers 2018 update. Res Vet Sci. 2018 Jun;118:199-246.
19 Dawson, H. D. et al. Porcine cytokines, chemokines and growth factors: 2019 update. Res Vet Sci. 2020 Aug;131:266-300.
20 Manirarora, J. N. et al. Development and Characterization of New Monoclonal Antibodies Against Porcine Interleukin-17A and Interferon-Gamma. Front Immunol. 2022 Feb 3;13:786396.
21 Fleming, D. S. et al. Transcriptomic Analysis of Liver Indicates Novel Vaccine to Porcine Reproductive and Respiratory Virus Promotes Homeostasis in T-Cell and Inflammatory Immune Responses Compared to a Commercial Vaccine in Pigs. Front Vet Sci. 2022 Mar 24;9:791034.
22 Katwal, P. et al. Role of zinc metalloprotease (ZMPSTE24) in porcine reproductive and respiratory syndrome virus (PRRSV) replication in vitro. Arch Virol. 2022 Nov;167(11):2281-2286.
23 Pandey, K. et al. GTPase-activating protein-binding protein 1 (G3BP1) plays an antiviral role against porcine epidemic diarrhea virus. Vet Microbiol. 2019 Sep;236:108392.
24 Yang, L. et al. Nonstructural Protein 11 of Porcine Reproductive and Respiratory Syndrome Virus Induces STAT2 Degradation To Inhibit Interferon Signaling. J Virol. 2019 Oct 29;93(22):e01352-19.
25 Sharma, B. et al. A Novel Live Attenuated Vaccine Candidate Protects Against Heterologous Senecavirus A Challenge. Frontiers in immunology 10, 2660.
26 Fernandes, M. H. V., de Lima, M., Joshi, L. R. & Diel, D. G. A virulent and pathogenic infectious clone of Senecavirus A. J Gen Virol 102.
27 Singh, G., G, S., Ramamoorthy, S. & S, R. Potential for the cross-species transmission of swine torque teno viruses. Vet Microbiol. 2018 Feb;215:66-70.
28 Webb, B. et al. Torque teno viruses in health and disease. Virus Res. 2020 Aug;285:198013.
29 Sun, H., Workman, A., Osorio, F. A., Steffen, D. & Vu, H. L. X. Development of a broadly protective modified-live virus vaccine candidate against porcine reproductive and respiratory syndrome virus. Vaccine 36, 66-73.
30 Nguyen, T. N. et al. A Single-Dose Intramuscular Immunization of Pigs with Lipid Nanoparticle DNA Vaccines Based on the Hemagglutinin Antigen Confers Complete Protection against Challenge Infection with the Homologous Influenza Virus Strain. Vaccines 11.
31 Singh, G. et al. A Minimally Replicative Vaccine Protects Vaccinated Piglets Against Challenge With the Porcine Epidemic Diarrhea Virus. Front Vet Sci. 2019 Oct 22;6:347.
32 Vinson, H. et al. Delivery of a thermo-enzymatically treated influenza vaccine using pulmonary surfactant in pigs. Vet Microbiol. 2019 Dec;239:108492.
33 Rakibuzzaman, A. et al. Harnessing the Genetic Plasticity of Porcine Circovirus Type 2 to Target Suicidal Replication.1676. Viruses. 2021 Aug 24;13(9):1676.
34 Ilha, M. et al. Early antibody responses map to non-protective, PCV2 capsid protein epitopes. Virology. 2020 Jan 15;540:23-29.
35 Rakibuzzaman, A. et al. Targeted Alteration of Antibody-Based Immunodominance Enhances the Heterosubtypic Immunity of an Experimental PCV2 Vaccine. Vaccines (Basel). 2020 Sep 4;8(3):506
36 Renu, S. et al. A Nanoparticle-Poly(I:C) Combination Adjuvant Enhances the Breadth of the Immune Response to Inactivated Influenza Virus Vaccine in Pigs. Vaccines (Basel). 2020 May 18;8(2):229.
37 Patil, V. et al. A split influenza vaccine formulated with a combination adjuvant composed of alpha-D-glucan nanoparticles and a STING agonist elicits cross-protective immunity in pigs. J Nanobiotechnology. 2022 Nov 11;20(1):477.
38 Singh, G. et al. An amphiphilic invertible polymer as a delivery vehicle for a M2e-HA2-HA1 peptide vaccine against an Influenza A virus in pigs. Vaccine. 2019 Jul 18;37(31):4291-4301.
39 Lunney, J. K. et al. Importance of the pig as a human biomedical model. Sci Transl Med. 2021 Nov 24;13(621):eabd5758.
40 Yuan, F. et al. Development of a Blocking Enzyme-Linked Immunosorbent Assay for Detection of Antibodies against African Swine Fever Virus. Pathogens 10.
41 Palowski, A. The Use of A Risk-Free In Situ Non-Animal (RISNA) Surrogate Assay for Evaluating Inactivation Strategies of African Swine Fever Virus in Feed Ingredients in Real-World Demonstrations. Master Thesis. (2021).
42 de Lara, A. C. et al. Effect of pooling udder skin wipes on the detection of influenza A virus in preweaning pigs. J Vet Diagn Invest. 2022 Jan;34(1):133-135.
43 Garrido-Mantilla, J. et al. Comparison of individual, group and environmental sampling strategies to conduct influenza surveillance in pigs. BMC Vet Res. 2019 Feb 14;15(1):61.
44 Paploski, I. A. D. et al. Phylogenetic Structure and Sequential Dominance of Sub-Lineages of PRRSV Type-2 Lineage 1 in the United States. Vaccines (Basel). 2021 Jun 5;9(6):608.
45 Paploski, I. A. D. et al. Temporal Dynamics of Co-circulating Lineages of Porcine Reproductive and Respiratory Syndrome Virus. Front Microbiol. 2019 Nov 1;10:2486.
46 Kikuti, M. et al. Porcine reproductive and respiratory syndrome virus 2 (PRRSV-2) genetic diversity and occurrence of wild type and vaccine-like strains in the United States swine industry. PLoS One. 2021 Nov 19;16(11):e0259531.
47 Pamornchainavakul, N. et al. Measuring How Recombination Re-shapes the Evolutionary History of PRRSV-2: A Genome-Based Phylodynamic Analysis of the Emergence of a Novel PRRSV-2 Variant. Front Vet Sci. 2022 Mar 25;9:846904.
48 Paploski, I. A. D. et al. Potential Novel N-Glycosylation Patterns Associated with the Emergence of New Genetic Variants of PRRSV-2 in the U.S. Vaccines (Basel). 2022 Nov 26;10(12):2021.
49 Preis, G. et al. Senecavirus A seroprevalence and risk factors in United States pig farms. Front Vet Sci. 2022 Oct 20;9:1011975.
50 Makau, D. N. et al. Integrating animal movements with phylogeography to model the spread of PRRSV in the USA. Virus Evol. 2021 Jul 15;7(2):veab060.
51 Pamornchainavakul, N. et al. Mapping the Dynamics of Contemporary PRRSV-2 Evolution and Its Emergence and Spreading Hotspots in the U.S. Using Phylogeography. Pathogens. 2023 May 20;12(5):740.
52 VanderWaal, K. et al. Contrasting animal movement and spatial connectivity networks in shaping transmission pathways of a genetically diverse virus. Prev Vet Med. 2020 May;178:104977.
53 Makau, D. N. et al. Temporal stability of swine movement networks in the U.S. Prev Vet Med. 2021 May 3;191:105369.
54 Makau, D. N. et al. Dynamic network connectivity influences the spread of a sub-lineage of porcine reproductive and respiratory syndrome virus. Transbound Emerg Dis. 2022 Mar;69(2):524-537.
55 Kikuti, M. et al. Porcine Reproductive and Respiratory Syndrome (PRRSV2) Viral Diversity within a Farrow-to-Wean Farm Cohort Study. Viruses. 2023 Aug 30;15(9):1837.
56 Kikuti, M. et al. Time Farms Stay Naive for Porcine Reproductive and Respiratory Syndrome. LID - 10.3390/ani13020310 [doi] LID - 310. Animals (Basel). 2023 Jan 16;13(2):310.
57 Lopez-Moreno, G. et al. Evidence of influenza A infection and risk of transmission between pigs and farmworkers. Zoonoses Public Health. 2022 Aug;69(5):560-571.
58 Li, C. et al. Vaccination decreases the risk of influenza A virus reassortment but not genetic variation in pigs. Elife. 2022 Sep 2;11:e78618.
59 Kikuti, M. et al. Growing pig incidence rate, control and prevention of porcine epidemic diarrhea virus in a large pig production system in the United States. Porcine Health Manag. 2022 Jun 7;8(1):23.