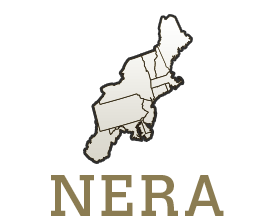
NE1501: Harnessing Chemical Ecology to Address Agricultural Pest and Pollinator Priorities
(Multistate Research Project)
Status: Inactive/Terminating
NE1501: Harnessing Chemical Ecology to Address Agricultural Pest and Pollinator Priorities
Duration: 10/01/2015 to 09/30/2020
Administrative Advisor(s):
NIFA Reps:
Non-Technical Summary
Statement of Issues and Justification
The need, as indicated by stakeholders:
Agricultural crops are valuable to the culture, economy and future of the Northeast. For example, the total value of principal crops in the Northeast was > $5.32 billion and Northeast vegetable growers harvested over 133,000 acres with a value more than $700 million. New York alone ranked 5th in the nation for vegetable production and garnered $323 million from fruit, berry and grape production (NASS 2013). Our region has numerous large cities that import food; thus, a robust regional agricultural productivity is essential for food security for these population centers.
While the value of agriculture to the Northeast is indisputable, our agricultural systems mostly remain reliant on pesticides to ensure profit. On behalf of stakeholders, the Northeast IPM Center states that they 'are enthusiastic about alternative, non-pesticidal strategies that unite several disciplines and lead to sustainable solutions'. Northeast regional priorities for fruit, vegetable and specialty crops are replete with calls for research and sustainable practices to reduce the impacts of insect pests and to protect valuable pollinators. Organic agriculture continues to grow in both demand and production and is particularly reliant on developing holistic, ecology-based systems.
Proposed objectives:
- Develop chemical ecology tools and information to support sustainable agriculture by reducing damage by pests in crops such as potatoes, brassicas, cucurbits, apples, blueberries, and sweet corn, while maintaining pollinator health in agricultural systems.
- Define variability of chemically mediated interactions between pests, crops, and beneficial organisms in terms of plant chemistry, species interactions and landscape factors in the Northeast.
- Characterize the non-target effects of pesticides on pollinators, herbivores and natural enemies of pests.
- Assess the impact of domestication on plant and animal chemical ecology in agricultural fields and identify unifying patterns of human and natural selection on chemical interactions of crop plants.
- Establish a chemical ecology analytical facility for the Northeast to allow researchers ready access to equipment and technical expertise.
- Extension to facilitate adoption and awareness of science-based chemical ecology tools to support sustainable production.
The importance of the work, and what the consequences are if it is not done:
Generally, researchers of diverse disciplines converge upon a particular crop or target pest rather than developing management models that cut across a broad range of crops and pests. We propose the reverse, to harness the intellectual breadth of chemical ecology practitioners and to focus their interests on agricultural pests and pollinators.
As the discipline of chemical ecology matures, knowledge gained in ecological, behavioral and evolutionary studies can be translated into practical and applied pest management. Thus, blending fundamental and applied research enhances the likelihood of sustainable pest management and a reduction in the quantity of pesticides released into our environment. The consequence of not pursuing sustainable, non-pesticidal management of pests is a continued reliance on insecticides and other pesticides, with potential long and short term adverse effects on our environment for future generations.
The technical feasibility of the research:
The field of chemical ecology originated nearly 50 years ago with the identification of an insect sex pheromone. That work engendered the applied practice of pheromone mating disruption and pheromone trapping to inform IPM decisions. Since then, it has become increasingly apparent that insect pests, natural enemies of pests, and pollinators respond to a complex set of chemical signals in their environment. Basic understanding of the signals that govern their interplay, especially those emitted by crop plants, will lead to the development of practical and economic tools to suppress agricultural pests and enhance pollination. Other concrete examples of applied chemical ecology are the development of non-bitter cucumbers to reduce attraction by striped cucumber beetles, the finding that pheromone monitoring can be made more effective by the addition of specific host plant volatiles and the employment of a ‘push-pull’ approach to stem borers in African maize.
Thus, we are confident of technical feasibility and fruitful knowledge as the discipline matures and as we begin to elucidate the roles of plant genetics, gene expression, and metabolic pathways that control chemical signaling among plants, pests, natural enemies and pollinators.
The advantages for doing the work as a multistate effort:
The field of chemical ecology is well represented in various land grant universities within the Northeast and while there are pest problems that are unique to the Northeast, there can be substantial overlap in pest guilds within the areas comprising the region. There is tremendous potential for unifying the various researchers within the region to address agricultural problems and there is a clear advantage to fostering a multi-state effort. We have already begun to assemble a team of researchers and recently hosted a NERA Planning Conference (Northeastern Regional Association of State Experiment Station Directors) with the express purpose of bringing together researchers from the Northeast to discuss the application of chemical ecology to agricultural pest and pollinator problems. A multistate approach would continue to provide opportunities for expedited communication and cooperation while also focusing the attention of fundamental and basic researchers on applied problems in agriculture. Additionally, several of the major crops and pests have different phenologies in different states in the region; accordingly, multi-state perspective will allow for a more comprehensive approach to understanding pest issues, especially as they may be altered by climate change.
Analytical instrumentation is increasingly a limitation for academic researchers. The equipment is expensive to purchase and maintain and requires a skilled operator which results in both high initial and per sample costs. Given the inherent similarities of many of the volatiles, pheromones, pesticides or other metabolites that would be commonly measured by chemical ecology researchers in the Northeast, a central facility would be an efficient way to enable research. It would also improve coordination among researchers by creating standard, shared methodologies that would be implemented with a single set of equipment.
What the likely impacts will be from successfully completing the work:
Chemical ecology can be defined as the study of the structure, origin and function of naturally occurring chemicals that mediate intraspecific or interspecific interactions. When applied to agricultural pests, the discipline of chemical ecology will lead to elucidating how the ubiquitous chemical signaling among pest species and their hosts can be exploited to control pests. For instance, crop breeding and selection have been cornerstones of successful agriculture for millennia; with powerful new genetic tools and new understanding of the chemical signals controlling pest behavior, we will have the opportunity to expedite plant breeding for resistance. Other likely impacts will be the ability to exploit pest behavior to our favor by targeting the communication systems upon which they depend.
Related, Current and Previous Work
The high monetary cost associated with bringing new pesticides to market, and the recognized economic, environmental and human costs of delivering pesticides to their targets are ample justification for developing alternative tactics to pesticidal control of pests. Specifically, methods that exploit the natural signaling and chemically mediated interactions that occur among crops, pests, and natural enemies can be used to improve integrated pest management and also to facilitate pollinator health and efficacy in agricultural systems. Chemical ecology generally concerns the chemically mediated interactions among organisms and their environment and covers chemical communication, chemical defense, and mutualistic interactions such as pollination. Although the discipline originated in the 1800’s with various studies by Kerner von Marilaun, Errera, and Stahl, it has rapidly expanded since the late 1950s when it was recognized that plant secondary metabolites mediate plant-insect interactions, suggesting that plant chemical diversity evolved under selection by herbivory (Hartmann 2008).
Major advances in pest management also arose in the 1950s, with the advent of identifying pest pheromones and it is widely recognized that there is still tremendous potential to apply chemical ecology to pest management. The identification of silkworm pheromone in the 1950’s by Butenandt et al. (1959) was a milestone in chemical ecology, and a 2003 review of USDA ARS research in chemical ecology shows that pheromone chemistry and utilization, for moths and beetles in particular, has long been a large and important part of the linkage between the disciplines of chemical ecology and agricultural pest control (Aldrich et al. 2003). Similarly, plant defense by naturally occurring cucurbitacins, and the interplay between resistance and susceptibility to various pests (as well as impacts on cucurbit flavor) was a milestone in breeding for resistance in the 1970s (da Costa & Jones 1971).
Chemically mediated interactions among crops and their herbivores and pollinators, largely insects and other arthropods, vary substantially and are highly dependent on the nature of the interaction. There is a wealth of research pointing to the possibility of harnessing chemical communication to assist with pest management and pollinator efficacy. Some examples relevant to agriculture are provided below.
Signaling within a species
Pheromones- Perhaps the best known application of chemical ecology to agriculture occurs with the use of pheromones to disrupt or monitor pests. Pheromones are chemicals used by animals to communicate amongst each other within a species. The use of pheromones for agricultural and forest pest management has a long history beginning with the identification of gypsy moth pheromone in the 1960’s, and published by Bierl et al. (1970). Since that time, thousands of pheromones have been identified and dozens have been used for insect monitoring and control. A search of the National Pesticide Information Retrieval System (NYPIRS) identified >100 EPA registered pheromone products that are dispensed by various methods and are typically used in monitor traps, attract and kill systems, and mating disruption (Agricultural Marketing Service 2012). And though pheromones are often used alone or as blends of pheromones, it is becoming apparent that often they are potentiated or synergized by volatile plant chemicals. This is especially evident in the aggregation pheromones of pests such as several major beetle pests: Colorado potato beetles, crucifer flea beetles and striped cucumber beetles, all chronic pests of northeastern US vegetable crops. In the case of the Colorado potato beetle and crucifer flea beetles, plant volatiles have been identified (Dickens 2006, and Soroka et al. 2005, respectively). In the case of striped cucumber beetles, the volatiles are as yet unknown, but without plant material, male beetles are unable to elicit aggregation behavior (Smyth and Hoffmann 2002).
Plant-to-plant signaling within a species has been studied and it appears to be a way for plants to help thwart insect herbivores even before they begin feeding on the plant. Early and seminal work by Baldwin & Schultz (1983) suggested that plants are primed for defense when neighbors of the same species are attacked by an insect. In an agricultural context, selection of crops that retain this evolutionary defense could help mitigate crop damage; that is, plant breeding for horticultural attributes may be detrimental to selecting for plants resistant to pests. To date, plant-to-plant signaling in defense against pests has been identified in beans, cereals, and tomato (Glinwood et al. 2009, Kost & Heil 2006).
Signaling between species Non-volatile communication- Many crops have intrinsic chemical defenses against pests, but specialist herbivores have evolved to overcome these defenses. Classic examples of this occur in chili peppers, brassicas (broccoli, cabbage, etc.) and cucurbits (squash, pumpkins, etc.). Pungent chiles are resistant to many generalist pests (Jarvis and Guthrie 1972) but are susceptible to the specialist pepper weevil (Berny-Mier y Teran et al. 2013). Brassicas often contain glucosinolates that are converted to defensive compounds, but specialist crucifer flea beetles are attracted to this these compounds (Pivnick et at. 1992). Similarly, cucurbits often contain high levels of cucurbitacins that are unpalatable to humans and many pests, but stimulate feeding by cucumber beetles (Smyth and Hoffmann 2002). So from a practical agricultural standpoint, plant breeding to reduce glucosinolates, cucurbitacins or capsaicinoids may result in greater damage by some pests while having little effect on specialist herbivores of these crops (Bodnaryk 1997, Smyth & Hoffmann 2002, Frantz et al. 2004).
Communication via volatile chemicals-While cucurbitacins, glucosinolates and capsaicins are mostly non-volatile, volatile emissions from plants are thought to play a part in virtually every aspect of a plant’s interaction with its environment. Plant volatiles can be classified as kairomones, synomones, and allomones depending on which organism derives a benefit from the signals. Kairomones mediate interactions that are adaptively favorable to the organism receiving the signal; allomones are favorable to the organism sending the signal and synomones are favorable to both. While some herbivores are specialists that are attracted to specific volatiles, most are not and their host finding appears to be in response to mixtures of chemicals that are common to many plants (Visser and Avé 1978, de Bruyne and Baker 2008, Webster et al. 2008). Thus, a generic receptor system in insects coupled with highly variable volatile cues means that using volatiles for pest management will require a detailed knowledge of insect chemical ecology (Szendrei & Rodriguez-Saona 2010)
There are numerous reports of pests’ preferences for certain varieties of a crop, but that preference has rarely been attributed to differences in constitutively produced plant volatiles. However, published research by Hoffmann et al. (1996) and subsequent unpublished research by Mazourek suggest that with the striped cucumber beetle, there is a strong attraction to certain cultivars or subspecies of Cucurbita pepo independent of the aggregation elicited by the release of a male-produced aggregation pheromone. This strongly suggests a quantitative or qualitative difference in constitutively expressed plant volatiles and introduces the possibility of manipulating these volatiles to help the crop avoid attracting an herbivore pest.
In addition to constitutively expressed plant volatiles affecting herbivores, herbivores in return can induce the production of plant volatiles and other secondary metabolites. The release of green leaf volatiles is ubiquitous and rapid when plant tissue is fed upon (Hatanaka 1993) and these volatiles have been shown to elicit or prime both direct and indirect plant defenses (Scala et al. 2013). These plant defenses are typically mediated by plant hormones such as jasmonic acid, salicylic acid and ethylene resulting in activation of metabolic pathways that produce secondary metabolites. In turn, the metabolites have effects ranging from attracting herbivores to discouraging herbivores, or that are indirectly protective by attracting parasites and predators that feed on the herbivores (Scala et al. 2013).
A particularly active area of research has been the indirect defense of plants by induced plant volatiles that alert natural enemies to the presence of herbivore pests. When plants were genetically manipulated to produce certain volatiles it resulted in the attraction of natural enemies of the herbivore that would normally induce those volatiles (Kappers et al. 2005, Schnee et al. 2006). Other researchers have found indirect defenses and the attraction of natural enemies (e. g. Agrawal et al. 2002, Thaler et al. 2002, Menzel et al. 2014). However, some argue that manipulating crops to lure natural enemies to the field early enough to be effective control agents may be difficult to achieve and may have some unwanted side effects such as the attraction of predators and parasites that attack the pests’ predators and parasites (Kaplan 2012). This must be resolved before an effective management strategy can be developed.
Aside from attracting natural enemies, plant volatiles have many other effects. Research has documented that volatiles play a role in: alerting pollinators and pests to the presence of a suitable host (Raguso 2008); alerting neighboring plants that there is an herbivore present (Baldwin et al. 2006); informing herbivores of the presence of each other (de Moraes et al. 2001); increasing or decreasing the competitiveness of neighboring plants such as weeds (Singh et al. 2003); reducing a plant’s infection by fungi and bacteria (Shiojiri et al. 2006); changing a plant’s palatability to herbivores (Halitschke et al 2004). In view of the myriad effects of constitutive and induced plant volatiles, it is obviously and area that we can exploit to improve pest management, although both laboratory as well as field trials are essential.
An area of concern in crop breeding is whether domestication and subsequent selection of cultivars for agricultural purposes has changed a crop’s chemical defenses for the better or worse. The conventional wisdom has been that there are negative trade-offs associated with yield and visual improvement of various crops (Chen et al. 2015). However, domestication imparted greater resistance in some crops but lesser resistance in other crops (Turcotte et al. 2014). In that paper, the authors found that significantly more of the 29 crops studied had a loss of resistance than not, so it remains an area of concern.
Pollinator chemical ecology as it relates to agriculture
Insect pollination provides vital ecosystem services that maintain plant biodiversity and sustain agricultural crop yields. Many agricultural crops rely completely on pollinators for successful yields and honey bees are the only managed insect used worldwide for pollination. Successful pollination can be at risk from factors including population declines and diseases, and climate change is disrupting the synchrony of bees and their hosts. Fortunately, there are often functional equivalents to carry out this valuable service. Bartomeus et al. (2013) found that a diverse assemblage of bee species allowed extensive synchrony between bee activity and apple peak bloom due to complementarity among the bee species' activity periods and system stability imparted by differential responses among the species to a warming climate. Despite the potential for successful pollination by species other than honey bees, pollination can be limiting and therefore there are several areas where the field of chemical ecology can contribute to pollinator health and abundance.
Pesticides in pollen & nectar- Although not typically considered the realm of chemical ecology, insecticides can certainly be considered a part of the ecological landscape and thus are worth of inclusion in this discussion. Pesticides in pollen and nectar have been implicated as a contributor to colony collapse disorder of honey bees (Stavely et al. 2014) as well as for adverse effects on other pollinator species such as bumblebees, flies and beetles (Easton and Goulson 2013, Gill and Raine 2014). Repellency or chronic impairment of pollinator foraging implies a chemical effect on sensory apparati and knowledge of these interactions will help guide conservation of domestic and wild pollinators.
Alteration of Floral scents- Research has shown that greater volatile emissions by unpollinated blueberry flowers resulted in greater attraction of pollinators relative to pollinated flowers (Rodriguez- Saona et al. 2011). This strategy can help ensure that bees don’t waste valuable time on flowers that have already been pollinated and thus increase crop yield and bee energetic return. Furthermore, the variation in volatile emissions from blueberry flowers depending on pollination status, plant cultivar and time of day suggests an adaptive role of floral signals in increasing pollination of flowers.
Plant secondary metabolites in pollen & nectar- Defense chemicals in plants can affect predators and parasitoids, but the impact on pollinators is not yet well studied. While most studies have found defensive secondary metabolites in vegetative structures, they have also been documented in nectar (Adler 2000) and pollen (Arnold et al. 2014, Haider et al. 2014). The effects of defense metabolites on pollinators range from pollinator avoidance (Elliot et al. 2008), to no discernible effect on their progeny (Elliot et al. 2008), to limiting pollinator larval survival (Arnold et al. 2014.)
Disease transmission from pollinators to flowers and from flowers to pollinators- Several floral microbes are known to be pathogenic to plants or to their pollinators. Attraction of floral visitors is affected by flower phenology, morphology and chemistry, and several plant pathogens manipulate floral traits to attract vectors. In plants, pathogens vectored by pollinators can result in plant disease that can be economically devastating in agricultural systems (Batra 1983; Farkas et al. 2011. Even as pollinators vector plant pathogens, flowers may transmit diseases of pollinators via fecal contamination (Durrer & Schmid-Hempel 1994), shared use of pathogen-inoculated resources such as nectar or pollen (Singh et al. 2010),or other possible mechanisms. Given the concern about pollinator declines caused in part by pathogens, the role of floral traits in mediating pathogen transmission is a key area for further research.
Floral chemical traits have also been shown to influence disease establishment in the host plant. For instance, enhanced emission of normal flower volatiles has been shown to better attract flower visitors such as moths that vector a plant disease (Dotterl et al. 2009), antimicrobial nectar has been shown to reduce bacterial wilt in wild gourds (Sasu et al. 2010), and disease induced mimicry of flower volatiles and insect pheromones caused attraction of pollinators to diseased plants (Raguso & Roy 1998, Naef et al. 2002)
Pollinator self-medication- New research also suggests that secondary compounds in flowers can reduce pathogen loads in pollinators. Honeybees for instance have prophylactic defense behavior including the foraging of antibiotic, antifungal, and antiviral compounds of plant products. Since these compounds are also fed to the bee larvae they affect colony health. Nurse bees infected with the parasite, Nosema ceranae, preferred honeys with a higher antibiotic activity (Gherman et al. 2014). This behavior might be a highly adaptive form of therapeutic medication at both the individual and the colony level and can influence the efficacy of honeybee pollinators in cropping systems.
Manson et al. (2010) found that when bumblebees were fed with a toxic flower alkaloid it did not reduce acquisition of disease but subsequent ingestion of the alkaloid did reduce pathogen loads, indicating anti-pathogen activity when consumed during infection. The importance of self-medication in insects is beginning to be recognized (de Roode et al. 2013), and this study suggests pollinators may benefit from ingesting plant secondary compounds when challenged with disease. Thus, floral chemistry may play a significant but largely unrecognized role in pollinator–pathogen infection dynamics.
Objectives
-
Develop chemical ecology tools and information to support sustainable agriculture by reducing damage by pests in crops such as potatoes, brassicas, cucurbits, apples, blueberries, and sweet corn, while maintaining pollinator health in agricultural systems.
-
Define variability of chemically mediated interactions between pests, crops, and beneficial organisms in terms of plant chemistry, species interactions and landscape factors in the Northeast.
-
Characterize the non-target effects of pesticides on pollinators, herbivores and natural enemies of pests.
-
Assess the impact of domestication on plant and animal chemical ecology in agricultural fields and identify unifying patterns of human and natural selection on chemical interactions of crop plants.
-
Establish a chemical ecology analytical facility for the Northeast to allow researchers ready access to equipment and technical expertise.
-
Extension to facilitate adoption and awareness of science-based chemical ecology tools to support sustainable production.
Methods
Nearly all projects will involve a field and laboratory component, with specific, hypothesis-appropriate experimental designs, and shared and coordinated methodologies essential for cooperative data sharing. Research will focus on potatoes,brassicas, cucurbits, apples, blueberries, and sweet corn, and the administrative leadership of the project will maintain lists of the relevant varieties and experiments in order to maximize cross validation and analyses of specific problems in multiple states. Chemical analyses, typically including liquid and gas chromatography, mass spectrometry, or spectrophotometry will be employed in nearly all research projects.Objective 1: Research will employ natural elicitors of plant resistance (jasmonic acid, salicylic acid, and related compounds), insect pheromones, and the screening of varieties for traits relevant to pest management and breeding. When chemical signaling between species is related to non-volatile chemical compounds such as cucurbitacins or similar agents, high performance liquid chromatography (HPLC) will be employed to identify and quantify the signal compounds. Plant materials of interest in a particular project will be dried and extracted with methanol or ethanol as appropriate and dried via evaporation of the solvents. Once dried, the materials will be suspended in an appropriate solvent such as chloroform or dichloromethane prior to chromatography using a column such as silica gel and gradient elution of the sample to further purify the compounds of interest. Final purification by preparative HPLC will be conducted using an appropriate mobile phase such as acetonitrile and water. Any further analyses of compounds of interest will include such methods as thin layer chromatography or mass spectrometry. Where signaling is deduced to be caused by plant or insect volatile emissions, standard and published methods typically include adsorption of candidate volatiles on carbon traps or by solid phase micro-extraction (SPME). Subsequently candidate compounds are eluted/desorbed from the collection media and passed through gas chromatography coupled with flame ionization detection with the chromatography column split to allow electroantennographic detection (EAD) by an insect pest, pollinator or natural enemy. This will allow inference regarding which classes of compounds cause neurological responses in the insect receiving the signal. Those compounds causing neurological responses in the insects are then typically identified by gas chromatography coupled with mass spectrometry. Once candidate signaling compounds have been identified by antennographic detection and subsequent determination of molecular formulae, behavioral bioassays will be conducted. This is an absolute necessity because neural stimulation does not necessarily translate into a behavioral response; it merely indicates that the insect may be affected by the signaling compound. Behavioral assays will typically run the gamut of choice assays such as y-tube, static or multi-arm olfactometers, wind tunnels, and locomotion compensators. Other choice tests will be conducted in small-plot field trials where plants with and without the signaling compounds of interests are offered as choice and no-choices options to target pests and pollinators.
Objective 2: Field experiments with replication in different states and geographic locations will be conducted to assess the variability of chemical signaling between pests, crops, and beneficial organisms in terms of plant chemistry, species interactions and landscape factors in the Northeast. Using methods described above as well as others as appropriate, trials will be conducted using methods standardized among cooperating states so that the data are robust among the regions and amenable to meaningful analyses or meta-analyses. Typically these will consist of multiple varieties, and in different agricultural contexts (in different states, or nested among different landscapes), while measuring and quantifying impacts on pests, pollinators, and natural enemies. This objective may also employ GIS-based landscape level analyses to complement experimental work.
Objective 3: We will characterize the non-target effects of pesticides on pollinators, herbivores, and natural enemies of pests including evolutionary effects that are relevant to pest management and pollinator health. Non-target effects will be conducted on farms, experimental fields, and laboratory trials. Analysis of pesticides by mass spectrometry will be critical, as will behavioral, performance, and genetic analysis of pests, pollinators, and natural enemies affected by insecticide or other pesticide exposure. In particular for pollinators such as bumblebees, there will be a coordinated multistate effort to gather pollen collected by the pollinators using methods standardized among cooperators. Subsequently, the pollen will be analyzed for pesticidal contaminants by using a common analysis facility as detailed for Objective 5. The effects of sub-lethal, chronic exposure on pollinators such as bumblebees and other non-domesticated bee pollinators will be quantified in terms of behavioral and fitness changes relative to control colonies.
Objective 4: The impact of domestication on plant and insect diversity in agricultural fields will be evaluated in replicated experimental designs that compare crops and their wild progenitors. Such trials will examine the differences between crops and wild-types and will us to quantify aspects of phytochemistry that affect the attraction of pests, pollinators, and natural enemies. Studies that employ RNAseq or other molecular genetics methods to determine which genes are affected and which are expressed will help expedite resistance breeding by focusing on specific genes and functions that control the chemical communications that occur among species. The goal of this objective is to identify novel targets and methods for manipulating crop phytochemistry for the purpose of crop resistance and pollination efficacy. Ultimately this will help expedite the breeding process, will lead to the discovery of new mechanisms of resistance to pests, and will generate breeding lines that manipulate and exploit the crops’ phytochemistry to control pests and facilitate pollination.
Objective 5: An existing small scale facility is currently available for researchers at Cornell University (www.chemicalecology.cornell.edu). Expansion is planned through a pay-for-service arrangement for multi-state researchers such that a consistent and rigorous protocol can be followed for analyses of phytochemistry relevant to breeding, pesticide residue analysis relevant to non-target effects, and analysis of volatile organic compounds relevant to attraction of pests, pollinators, and natural enemies. Additional analytical equipment that is available to participants will be inventoried and made available, if appropriate, so that resources are leveraged among cooperating institutions.
Objective 6: The Project will execute a program to disseminate relevant findings via various outlets to facilitate adoption and awareness of science-based chemical ecology tools to support sustainable production. Please see Section VII Outreach Plan for detailed description of our methods.
Measurement of Progress and Results
Outputs
- The project will result in the conversion of research-based knowledge of chemical ecology into tools useful to supporting sustainable and economically sound pest and pollinator management in agricultural systems.
- It will generate data and knowledge regarding the role of chemical communication within and among species of crops, pests, and pollinators in landscapes with an agricultural component.
- It will also generate data and applied knowledge by measuring and quantifying pesticide loads and their effects on pollinators and natural enemies of agricultural pests of importance in the Northeastern Region.
- The project will measure and quantify how crop domestication affects the interactions among agricultural crops and their pests, pollinators and natural enemies.
- The strong presence of chemical ecology researcher laboratories in the Northeast Land Grant Universities will facilitate the coordination, aggregation, sharing, and financial support of analytical equipment to be used among cooperating researchers in the Northeastern Region so that standardized methods and lower-variance data can be generated and utilized among various cooperative research projects.
- There will be annual and continued delivery of research updates to the relevant stakeholder community through open meetings, published proceedings and reports published in peer reviewed publications, trade journals, eXtension, appropriate electronic media and various other electronic avenues.
- There will be annual and continued delivery of research updates to the relevant stakeholder community through open meetings, published proceedings and reports published in peer reviewed publications, trade journals, eXtension, appropriate electronic media and various other electronic avenues.
Outcomes or Projected Impacts
- The overarching objective of this project is to develop novel, cutting-edge and economically attractive approaches that will provide options and allow growers to integrate environmentally friendly, non-pesticidal control of various agricultural pests and improve management of pollinator services.
- We will provide a greater understanding of the ecology of pests, pollinators and natural enemies and this will assist with generating recommendations for a more integrated approach to pollinator and pest management. Increased knowledge is likely to help conserve important pollinator and natural enemy populations and improve their services in agriculture and other ecosystems.
- This project represents a collaborative network of researchers. It will facilitate and expedite cooperation among researchers in the field of chemical ecology, and focus the application of fundamental and basic science on priority problems in agriculture.
- Cooperation and coordination among researchers with a common interest, shared equipment, and shared facilities will allow development of standardized protocols within particular projects and will maximize the impact of the research by generating highly consistent data that will generate meaningful inference and be suitable for future data-mining and meta-analyses.
- The project will facilitate the surveillance, collection and maintenance of population data for establishment of baselines and assessment of pollinator health in the Northeast and elsewhere.
- The knowledge generated by coordinated research will facilitate and expedite plant breeding for enhanced pest resistance and pollinator efficacy by providing explanatory mechanisms for plant’s responses to pests and pollinators. Coupled with molecular methods that identify pathways for signal chemicals, plant breeders will be able to focus on target genes during the selection and crossing process. The outcome will be horticulturally acceptable crop varieties with enhanced productivity in the face of herbivores.
Milestones
(2015): <ul><li>Roll-out of this new Multistate Project and solicitations for participation. <li>Meeting of Executive Committee and potential participants to establish project work plan. <li>Selection of model cropping systems to target for applied research. <li>Compilation and distribution of analytical equipment list to participants.</ul>(2016): <ul><li>Commencement of research in model or target cropping systems by participants beginning FY Oct. 1, 2016. <li>Establishment of standardized protocols for inter- and intrastate collaborative projects for a) pest management and b) pollinator health objectives. <li>Project participants' organizational meeting and mini-symposium to present and discuss research and developments. <li>Annual business meeting will be held to discuss developments or changes in project objectives, etc. <li>Establishment of pest management and pollinator sub-committees to guide research directions in each aspect. <li>Education and outreach efforts will be initiated pending success in research commitments.</ul>
(2017): <ul><li>Integration of any new participants into project agendas. <li>Annual business and participants meeting to discuss developments or implement changes in project execution, updates to equipment services, dovetailing of research efforts, etc. <li>Publish and disseminate via outreach avenues any relevant findings generated by project.</ul>
(2018): <ul><li>Initiate and organize symposium to present findings on integration of chemical ecology and agricultural priority issues to be combined with a relevant annual conference of entomology, ecology, pollination, etc. <li>Publish and disseminate via outreach avenues any relevant findings generated by project.</ul>
(2019): <ul><li>Conduct self-assessment and review of the project as a means to prepare for project renewal pending participant and stakeholder consensus that the project has generated sufficient returns to warrant renewal. <li>Continue publication and outreach dissemination.</ul>
Projected Participation
View Appendix E: ParticipationOutreach Plan
Several members of the project have Extension appointments and are involved in field demonstrations and outreach efforts related to the sustainable management of various agricultural pests and pollinators. Many members interact and disseminate information regularly with stakeholders from various sectors of the economy such as farmers, government agencies, agricultural professionals, commodity groups and researchers in related disciplines. Traditional extension meetings and educational programs conducted by Extension personnel will be held with producers, industry, consultants, and regulators to discuss findings and share information. Those participants with teaching appointments will be able to include current research generated by this project to inform undergraduate and graduate students of cutting edge developments in the field of chemical ecology and as it relates to agricultural issues.
Our results and conclusions will be communicated by various methods including but not limited to:
1) sharing annual reports among participants at our annual meeting;
2) refereed research publications with differing target audiences;
3) presentation in non-refereed commodity and industry publications;
4) a website maintained by Cornell University regarding the field of chemical ecology;
5) oral presentations at national and regional technical and scientific meetings.
Additionally, several members of the project have strong connections to the Northeastern IPM Center, the Pennsylvania State IPM Program, and the NY State IPM Program. The Project will cultivate an active dissemination program that utilizes the strong media presence that those agencies have already established. The Northeastern IPM Center fosters the development and adoption of integrated pest management for economic, environmental, and human health benefits. Stakeholders work with NEIPM to identify and address regional and global priorities, whether for research, education, or outreach. The Center's efforts are organized under five signature programs where leadership and advisory bodies see the greatest need. At present, the areas of focus for the programs are IPM and organic systems, rural and urban IPM, climate change and pests, next generation education, and advanced production systems. Its staff of eight people, located on the campus of Cornell University, includes a director, communication specialist, program evaluation specialist, grants and partnerships coordinator, administrative assistant, structural IPM program coordinator and trainer, and web administrator, all who work together as a team, making the organization well-suited to lead multiple IPM-related outreach efforts, including chemical ecology, pests, and pollination.
The print version of the Center’s IPM Insights newsletter reaches over 4,000 people quarterly. In the past year, we have published IPM Insights in e-book format, in addition to print, PDF, and web versions. NEIPM content is featured in media outlets including Pest Control Technology, Consumer Reports, and Entomology Today. The NortheastIPM.org website links to more than 250 IPM-related organizations while the e-mail listserv reaches approximately 3,500 people.
Organization/Governance
Organization of the project is delegated to an Executive Committee comprising an Administrative Advisor, Chair, Secretary and Representative at Large. The Administrative Advisor will be the Director of New York Agricultural Experiment Station in Ithaca NY and the remainder of the committee will be elected by and from regional project membership. Excepting the Administrative Advisor, Executive Committee members will hold a two year appointment. The Representative at Large will succeed the Secretary who will in turn succeed the Chair. It will be the responsibility of the Chair to prepare technical and executive meeting agendas, preside at meetings, and prepare an annual progress report on the research activities of the regional project. The Secretary duties will be to record the minutes of technical and executive committee meetings and perform other duties as necessary. The Representative at Large will assist both Secretary and Chair with their responsibilities as necessary. Subcommittees may be named by the Chair as needed for specific assignments such as developing new project outlines for continuing the project, to prepare publications, or other assignments. An annual meeting of the full Executive Committee will be held to summarize and critically evaluate progress, analyze results, and plan future activities, reports, and publications.
Literature Cited
Adler, L. S. 2000. The ecological significance of toxic nectar. Oikos 91:409–420. Agrawal, A. A., A. Janssen, J. Bruin, M. A. Posthumus, and M. W. Sabelis. 2002. An ecological cost of plant defence: attractiveness of bitter cucumber plants to natural enemies. Ecology Letters. 5: 377-385. Agricultural Marketing Service. 2012. Pheromones Crops Technical Evaluation Report. www.ams.usda.gov/AMSv1.0/getfile?dDocName=STELPRDC5097873 (Last Accessed January 01, 2015) Aldrich, J. R., R. J Bartelt, J. C. Dickens, A. L. Knight, D. M. Light, and J. H. Tumlinson. 2003. Insect chemical ecology research in the United States Department of Agriculture- Agricultural Research Service. Pest Management Science. 59: 777-787. Arnold, S. E. J., M. E. P. Idrovo, L. J. L. Arias, S. R. Belmain, and P. C. Stevenson. 2014. Herbivore defence compounds occur in pollen and reduce bumblebee colony fitness. Journal of Chemical Ecology. 40: 878-881. Baldwin, I. T., and J. C. Schultz. 1983. Rapid changes in tree leaf chemistry induced by damage: evidence for communication between plants. Science. 221: 277-279. Baldwin, I. T., R. Halitschke, A. Paschold, C. C. von Dahl, and C. A. Preston. 2006. Volatile signaling in plant-plant interactions: ‘talking tress’ in the genomics era. Science. 311:812-815. Bartomeus, I., M. G. Park, J. Gibbs, B. N. Danforth, A. N. Lakso, and R. Winfree. 2013. Biodiversity ensures plant-pollinator phenological synchrony against climate change. Ecology Letters. 16:1331-1338. Batra, L.R. 1983. Monilinia vaccinii-corymbosi (Sclerotiniaceae): its biology on blueberry and comparison with related species. Mycologia. 75:131–152. Berny-Mier y Teran J. C., L. Abdala-Roberts, A. Durán-Yáñez, and F. Tut-Pech. 2013. Variation in insect pest and virus resistance among habanero peppers (Capsicum chinense Jacq.) in Yucatán, México. Agrociencia. 47: 471-482. Bierl B. A., M. Beroza, and C. W. Collier. 1970. Potent sex attractant of the gypsy moth, Porthetria dispar (L): its isolation, identification, and synthesis. Science. 170: 87–89. Bodnaryk, R. P. 1997. Will low-glucosinolate cultivars of the mustard Brassica juncea and Sinapis alba be vulnerable to insect pests? Canadian Journal of Plant Science. 77:283-287. Butenandt, A., R. Beckmann, D. Stamm, and E. Hecker. 1959: Über den sexuallockstoff des seidenspinners Bombyx mori. Reindarstellung und Konstitution. Zeitschrift für Naturforschung. 14b: 283-284. Chen, Y. H., R. Gols, and B. Benrey. 2015. Crop domestication and its impact on naturally selected trophic interactions. Annual Review of Entomology. 60:35-58. da Costa, C. P., and C. M. Jones. 1971. Cucumber beetle resistance and mite susceptibility controlled by the bitter gene in Cucumis sativus L. Science. 172: 1145-1146. de Bruyne, M., and T. C. Baker. 2008. Odor detection in insects: volatile codes. Journal of Chemical Ecology. 34:882-897. de Moraes C. M., M. C. Mescher, and J. H. Tumlinson. 2001. Caterpillar-induced nocturnal plant volatiles repel conspecific females. Nature. 410: 577-580. de Roode, J.C., T. Lefevre, and M. D. Hunter. 2013. Self-medication in animals. Science. 340: 150–151. Dickens, J. C. 2006. Plant volatiles moderate response to aggregation pheromone in Colorado potato beetle. Journal of Applied Entomology. 130: 26-31. Dotterl, S., A. Jurgens, L. Wolfe, and A. Biere. 2009. Disease status and population origin effects on floral scent: potential consequences for oviposition and fruit predation in a complex interaction between a plant, fungus, and Noctuid moth. Journal of Chemical Ecology. 35: 307–319. Durrer, S., and P. Schmid-Hempel. 1994. Shared use of flowers leads to horizontal pathogen transmission. Proceedings of the Royal Society B: Biological Sciences. 258: 299–302. Easton, A. H. and D. Goulson. 2014. The neonicotinoid insecticide imidacloprid repels pollinating flies and beetles at field-realistic concentrations. PLoS ONE. 8: e54819 DOI: 10.1371/journal.pone.0054819 (Last Accessed January 27, 2015). Elliot, S. E., R. E. Irwin, L. S. Adler, and N. M. Williams. 2008. The nectar alkaloid, gelsemine, does not affect offspring performance of a native solitary bee, Osmia lignaria (Megachilidae). Ecological Entomology. 33: 298-304. Farkas, A., E. Mihalik, L. Dorgai, and T. Buban, 2011. Floral traits affecting fire blight infection and management. Trees. 26: 1–20. Frantz, J. D., J. Gardner, M. P. Hoffmann, and M. M. Jahn. 2004. Greenhouse evaluation of Capsicum accessions for resistance to European corn borer (Ostrinia nubilalis). HortScience. 39: 1336-1338. Gherman, B. I., A. Denner, O. Bobis, D. S. Dezmirean, L. A. Marghitas, H. Schluns, R. F. A. Moritz, and S. Erler. 2014. Pathogen-associated self-medication behavior in the honeybee Apis mellifera. Behavioral Ecology and Sociobiology. 68: 1777-1784. Gill, R. J., and N. E. Raine. 2014. Chronic impairment of bumblebee natural foraging behaviour induced by sublethal pesticide exposure. Functional Ecology. 28:1459-1471. Glinwood, R., E. Ahmed, E. Qvarfordt, V. Ninkovic, and J. Pettersson. (2009). Airborne interactions between undamaged plants of different cultivars affect insect herbivores and natural enemies. Arthropod-Plant Interactions. 3: 215-224. Jarvis, J. L. and W. D. Guthrie. 1972. Relation of horticultural characteristics of peppers to damage by larvae of the European corn borer. Iowa State Journal of Science. 46: 463-470. Haider, M., S. Dorn, and A. Muller. 2014. Better safe than sorry? A Fabaceae species exhibits unfavourable pollen properties for developing bee larvae despite its hidden anthers. Arthropod - Plant Interactions. 8: 221-231 Halitschke, R., J. Ziegler, M. Keinanen, and I. T. Baldwin. 2004. Silencing of hydroperoxide lyase and allene oxide synthase reveals substrate and defense signaling crosstalk in Nicotiana attenuata. Plant Journal. 40: 35-46. Hartmann, T. 2008. The lost origin of chemical ecology in the late 19th century. PNAS. 105: 4541-4546. Hatanaka, A. 1993. The biogeneration of green odour by green leaves. Phytochemistry. 34:1201–1218. Hoffmann, M. P., R. W. Robinson, M. M. Kyle and J. J. Kirkwyland. 1996. Defoliation and infestation of Cucurbita pepo genotypes by diabroticite beetles. HortScience. 31: 439-442. Jarvis, J. L., and W. D. Guthrie. 1972. Relation of horticultural characteristics of peppers to damage by larvae of the European corn borer. Iowa State Journal of Science. 46: 463-470. Kaplan, I. 2012. Attracting carnivorous arthropods with plant volatiles: the future of biocontrol or playing with fire? Biological Control. 60: 77-89. Kappers, I. F., A. Aharoni, and T. W. J. M. van Herpen, L. L. P. Luckerhoff, M. Dicke, and H. J. Bouwmeester. 2005. Genetic engineering of terpenoid metabolism attracts bodyguards to Arabadopsis. Science. 309: 2070-2072. Kost, C., and M. Heil. 2006. Herbivore?induced plant volatiles induce an indirect defence in neighbouring plants. Journal of Ecology. 94: 619-628. Manson, J.S., M. C. Otterstatter, and J. D. Thomson. 2010. Consumption of a nectar alkaloid reduces pathogen load in bumble bees. Oecologia. 162. 81–89. McArt, S. H., H. Koch, R. E. Irwin, and L. S. Adler. 2014. Arranging the bouquet of disease: floral traits and the transmission of plant and animal pathogens. Ecology Letters. 17: 624-636. Menzel, T. R., TY. Huang, B. T. Weldegergis, R. Gols, J. J. A. van Loon, and M. Dicke. 2014. Effect of sequential induction by Mamesta brassicae L. and Tetranychus urticae Koch on Lima bean plant indirect defense. Journal of Chemical Ecology. 40: 977-985. Naef, A., B. A. Roy, R. Kaiser, and R. Honegger. 2002. Insect-mediated reproduction of systemic infections by Puccinia arrhenatheri on Berberis vulgaris. New Phytologist. 154: 717–730. Pivnick, K. A., R. J. Lamb, and D. Reed. 1992. Responses of flea beetles, Phyllotreta spp. to mustard oils and nitriles in field trapping experiments. Journal of Chemical Ecology. 18: 863-873. Raguso, R.A., and B. A. Roy. 1998. ‘Floral’ scent production by Puccinia rust fungi that mimic flowers. Molecular Ecology.7: 1127–1136. Raguso, R. A. 2008. Wake up and smell the roses: the ecology and evolution of floral scent. Annual Review of Ecology, Evolution and Systematics. 39: 549-569. Rodriguez-Saona, C., L. Parra, A. Quiroz, and R. Isaacs. 2011. Variation in highbush blueberry floral volatile profiles as a function of pollination status, cultivar, time of day and flower part: implications for flower visitation by bees. Annals of Botany. 107: 1377-1390. Scala, A., S. Allmann, R. Mirabella, M. A. Haring, and R. C. Schuurink. 2013. Green leaf volatiles: a plant’s multifunctional weapon against herbivores and pathogens. International Journal of Molecular Sciences. 14:17781-17811. Schnee, C., T. G. Kollner, M. Held, T. C. J. Turlings, J. Gershenzon, and J. Degenhardt. 2006. The products of a single maize sesquiterpene synthase form a volatile defense signal that attracts natural enemies of maize herbivores. Proceedings of the National Academy of Sciences. 103: 1129-1134. Shiojiri, K., K. Kishimoto, R. Ozawa, S. Kugimiya, S. Urashimo, G. Arimura, J. Horiuchi, T. Nishioka, K. Matsui, J. Takabayashi. 2006. Changing green leaf volatile biosynthesis in plants: an approach for improving plant resistance against both herbivores and pathogens. Proceeding of the National Academy of Sciences. 103: 16672-16676. Sasu, M.A., K. L. Wall, and A. G. Stephenson. 2010. Antimicrobial nectar inhibits a florally transmitted pathogen of a wild Cucurbita pepo (Cucurbitaceae). American Journal of Botany. 97: 1025–1030. Singh, H. P., D. Batish, and R. K. Kohli. 2003. Allelopathic interactions and allelochemicals: new possibilities for sustainable weed management. Critical Reviews in Plant Sciences. 22: 239-311. Singh, R., A. L. Levitt, E.G. Rajotte, E. C. Holmes, N. Ostiguy, D. vanEngelsdorp, W. I. Lipkin, C. W. de Pamphilis, A. L. Toth, D. L. Cox-Foster. 2010. RNA viruses in Hymenopteran pollinators: evidence of inter-taxa virus transmission via pollen and potential impact on non-Apis Hymenopteran species. PLoS ONE. 5: e14357. DOI: 10.1371/journal.pone.0014357 (Last Accessed January 28, 2015) Smyth, R. R. and M. P. Hoffmann. 2002. Correspondence between rates of host plant consumption and responses to the Acalymma vittatum male-produced aggregation pheromone. Physiological Entomology. 27: 235-242. Soroka, J. J., R. J. Bartelt, B. W. Zilkowski, and A. A. Cossé. 2005. Responses of flea beetle Phyllotreta cruciferae to synthetic aggregation pheromone components and host plant volatiles in field trials. Journal of Chemical Ecology. 31: 1829-1843. Staveley J., S. Law, A. Fairbrother, and C. Menzie. 2014. A causal analysis of observed declines in managed honey bees (Apis mellifera). Human and Ecological Risk Assessment. 20: 566-591. Szendrei Z. and C. Rodriguez-Saona 2010. A meta-analysis of insect pest behavioral manipulation with plant volatiles. Entomologia Experimentalis et Applicata. 134:201-210. Thaler, J. S., M. A. Farang, P. W. Pare, and M. Dicke. 2002. Jasmonate-deficient plants have reduced direct and indirect defences against herbivores. Ecology Letters. 5: 764-774. Turcotte, M. M., N. E. Turley, and M. T. J. Johnson. 2014. The impact of domestication on resistance to two generalist herbivores across 29 independent domestication events. New Phytologist. 204:671-681. Visser, J. H., and D. A. Ave´.1978. General green leaf volatiles in the olfactory orientation of the Colorado potato beetle, Leptinotarsa decemlineata. Entomologia Experimentalis et Applicata. 24: 738–749. Webster B., T. Bruce, S. Dufour, C. Birkemeyer, M. Birkett, J. Hardie, and J. Pickett. 2008. Identification of volatile compounds used in host location by the black bean aphid, Aphis fabae. Journal of Chemical Ecology 34: 1153–1161.