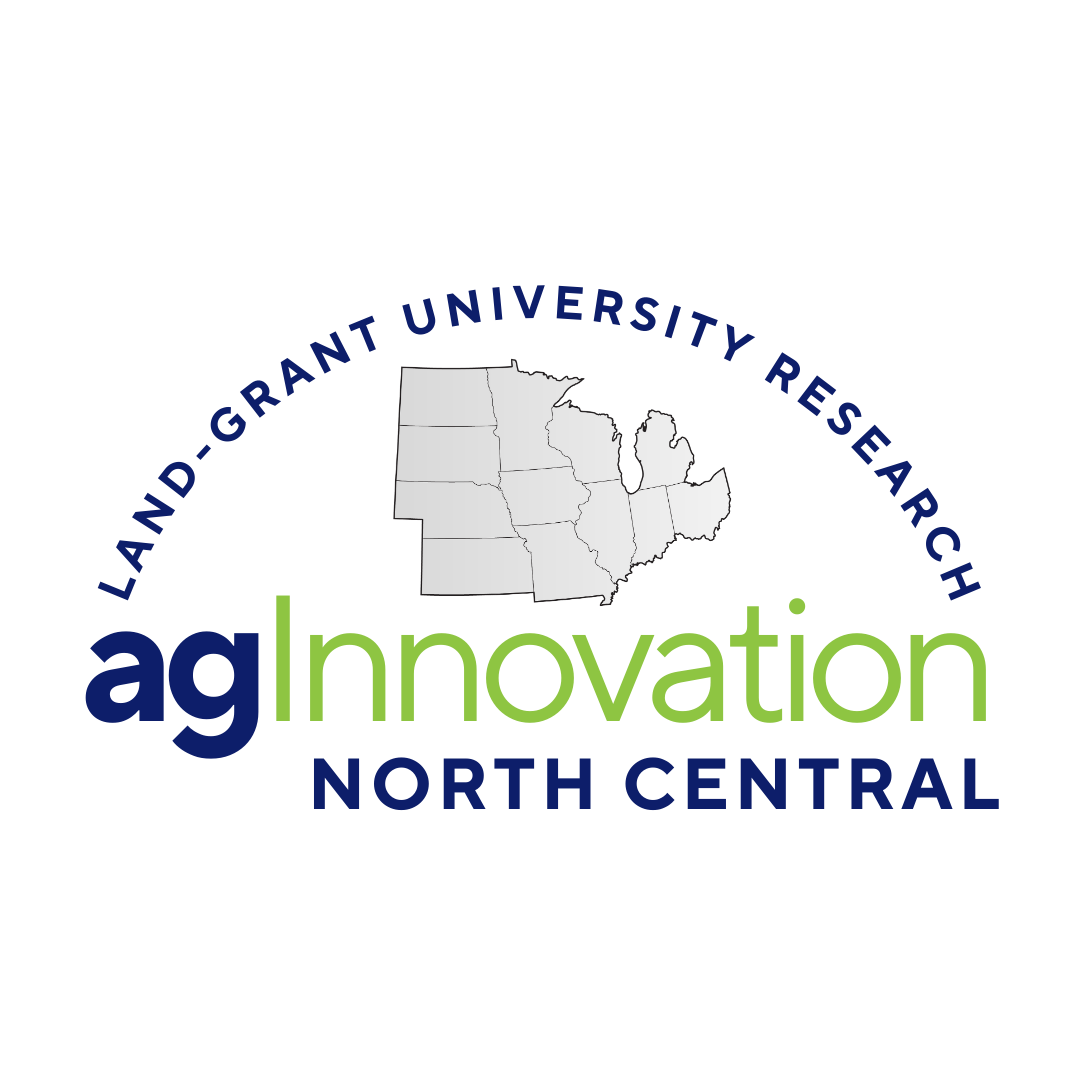
NC_old1203: Lipids In Plants: Improving and Developing Sustainability of Crops ("LIPIDS of Crops")
(Multistate Research Project)
Status: Inactive/Terminating
SAES-422 Reports
Annual/Termination Reports:
[10/13/2016] [01/05/2018] [10/23/2018] [10/04/2019] [11/05/2020] [09/20/2021]Date of Annual Report: 10/13/2016
Report Information
Period the Report Covers:
Participants
Brief Summary of Minutes
NC1203 is brand new, just started on 10/1/2016, so only meeting minutes and close-out of NCDC228 is available at this time.
Please find the business meeting notes below.
NCDC 228 Final Business Meeting 10/9/2016
Present:
Tim Durrett (KSU)
Doug Allen (USDA)
Joe Louis (UNL)
Kathryn Schrick (KSU)
Young-Jin Lee (Iowa State)
Abraham Koo (Mizzou)
Julie Stone (UNL)
Ruth Welti (KSU)
Om Parkash (Umass-Amherst)
Ed Cahoon (UNL)
Susanne-Hoffman Benning (MSU)
Rebecca Roston (UNL)
Basil Nikolau (ISU)
Sam Wong (Missouri)
This year 2016: Kansas State.
Basil Nikolau is the Chair. Iowa (Meeting 2017)
Ed Cahoon is the vice Chair. Nebraska (Meeting 2018)
Jay Thelen is the Secretary. Missouri (Meeting 2019)
Reporting Requirements:
None for this year.
Future: There will be a group report and also individual reports at each university.
- The meeting report is solicited by the annual chair preceding the time of the annual meeting. Then it is compiled with the notes of the business meeting and sent in as our annual report.
- Individual reports are summarized by the leader of the group at each university and has a CREES report like format.
When should the next meeting be?
Next September would be good.
A doodle poll will be sent around.
Ruth will forward an email list around.
Suggestions of people to invite for the next meeting would be good. Especially in skill sets - Structural biology, lipid biophysics, agricultural experts, breeders, and statisticians.
Accomplishments
Publications
Impact Statements
Date of Annual Report: 01/05/2018
Report Information
Period the Report Covers: 09/18/2016 - 09/17/2017
Participants
Brief Summary of Minutes
Accomplishments
Publications
Impact Statements
Date of Annual Report: 10/23/2018
Report Information
Period the Report Covers: 09/15/2018 - 09/16/2018
Participants
Allen, Douglas - Danforth Center/USDA-ARS; Cahoon, Edgar - University of Nebraska (UNL); Clemente, Thomas - UNL; Hoffman-Benning, Susanne - Michigan State University; Koo, Abe - University of Missouri-Columbia (UMC); Kosma, Dylan - University of Nevada; Lee, Young-Jin - Iowa State University; Minton, Ernie - Kansas State University (KSU); Narayanan, Sruthi - Clemson University; Parkash, Om - University of Massachusetts - Amherst; Roston, Rebecca - UNL; Schrick, Kathrin - KSU; Stone, Julie - UNL; Thelen, Jay - UMC; Wang, Xuemin - Danforth Center/University of Missouri - St. Louis; Welti, Ruth - KSU.Brief Summary of Minutes
The 2018 NC-1203 meeting was held September 15-16 in Columbia, Missouri at the University of Missouri. The meeting was opened by the Chair and the host, Jay Thelen, followed by remarks from KSU Interim Dean and Multistate Project Administrative Advisor Ernie Minton. A time of introductions was followed by presentations and discussions for each of the individual project aims. These were led by Ruth Welti, Jay Thelen and Ed Cahoon; accomplishments for each of the aims are summarized below. Dylan Kosma then provided a brief overview of his work on identification of transcriptional regulators of wound suberin deposition to introduce his research program to the group. This was followed by discussion of future plans for each of the aims and the timing of the 2019 annual meeting to be held in Lincoln, Nebraska. Douglas Allen was elected as secretary and the Danforth Center was chosen as the venue for the 2021 meeting.
Accomplishments
<p>Activities and accomplishments related to each of the project’s three objectives are described below. These were presented at the meeting by Ruth Welti (Objective 1), Jay Thelen (Objective 2) and Ed Cahoon (Objective 3). At the end of each presentation, group members engaged in active discussion on the challenges of each aim as well as alternative approaches to overcome these problems and to meet the remaining goals. Members also considered opportunities to work together on joint publications, either individual papers or a special issue on lipids in an appropriate journal.</p><br /> <p> </p><br /> <p><span style="text-decoration: underline;">Objective 1: Improve and extend methods for lipid characterization and measurement. </span></p><br /> <p>A new Sciex 6500+ triple quadrupole MS with differential ion mobility spectrometry, funded by an NSF MRI grant is installed at the Kansas Lipidomics Research Center (KLRC) and is functional; Ruth Welti and Tim Durrett have been trained on the instrument. The Welti lab has developed and published an abbreviated lipid extraction method, tested on Arabidopsis and sorghum leaves. The group has also reduced the time required for direct-infusion MRM analysis of ~370 lipids from 30 min to ~9 min. Polar lipid internal standard mixes are now available through the KLRC.</p><br /> <p>The Markham group has used scheduled MRMs to increase the number of analyses/time during chromatography and is working on intact ion/fragment lipidome map using accurate mass and chromatography. They are incorporating LC and prediction of retention times into their analytical strategy. Novel ceramide standards have been synthesized.The The Lee group has been developing new MALDI matrices for the visualisation and localisation of lipids. They screened various metal target deposition as a MALDI matrix, and found gold doped with sodium dramatically improves TAG signals but suppress phospholipids. Lee and Durrett submitted a NIFA proposal to study the spatiotemporal development of acetyl-TAG in genetically engineered camelina.</p><br /> <p>The Schrick lab developed and published a fluorescent labeling approach to determine the subcellular localization of choline phospholipids in plants. Data suggest choline phospholipids accumulate preferentially in guard cells. Schrick and Lee were successful in MS imaging of phosphatidylcholine (PC) in the epidermis of <em>Arabidopsis</em> leaves. Schrick received a Big 12 Faculty Fellowship to support this collaborative research.</p><br /> <p>Work in the Hoffman-Benning lab demonstrated that 14C-labeled PA moves in the phloem from source to sink, but need to confirm whether the labeled mobile compound is still PA and whether PLAFP affects its movement. They are currently designing a FRET experiment to determine whether PLAFP interacts or moves with the lipid in vivo. An NSF EAGER grant has been awarded for this work.</p><br /> <p>Wurtele is currently waiting for data sets from participants for the Plant/Eukaryotic and Microbial Systems Resource (PMR; http://metnetdb.org/PMR/). She has developed a semi-automated mechanism for upload of data that is connected to Araport. Welti reported that the LipidomeDB Data Calculation Environment will be updated from Sept. 19-21, 2018. The update includes improved ability to edit compounds and fragments, and, most importantly, ability to process MRM data with or without differential ion mobility. Processing includes isotopic deconvolution and normalization to internal standards.</p><br /> <p> </p><br /> <p><span style="text-decoration: underline;">Objecitve 2: </span><span style="text-decoration: underline;">Lipid-related metabolism and traits relevant for crop improvement</span></p><br /> <p>The Cahoon group has isolated a new DGAT1-type enzyme from <em>Cuphea avigera</em>, with improved specificity for 10:0-CoA. The Cahoon group has also demonstrated that oil from Chinese violet cress has two novel C24 dihydroxy fatty acids which gives it superior performance as a metal-on-metal lubricant. They are determining the oil structure with Kent Chapman (University of North Texas) and colleague in materials engineering and are working to improve the plant as a potential new oilseed.</p><br /> <p>A class of novel lipid-binding proteins in phloem sap has been identified by the Hoffman-Benning lab. Overexpression causes larger plants with bigger vascular bundles.</p><br /> <p>In collaboration with John Dyer (USDA-ARS), the Koo group demonstrated that that plants engineered to contain higher leaf oil content were differentially susceptible to pest attack depending on the protein that was up-regulated for oil body production.</p><br /> <p>Dylan Kosma reported the identification of 2 transcription factors in potato that appear to regulate all steps of wound suberin deposition. The same TFs also regulate other signaling and metabolic aspects of plant wound responses.</p><br /> <p>The Kutty lab showed that wheat decreases the unsaturation levels of plastidic and extraplastidic glycerolipids of leaf and/or pollen in order to adapt to heat stress.</p><br /> <p>The Schrick group has been studying the role of lipids in signaling through identification of START-domain transcription factors and their role in DNA binding, gene regulation and development of trichomes and other features of the epidermis. They have identified the nuclear localization signal for these transcription factors.</p><br /> <p>A family of negative regulators to ACCase called the BADCs was discovered by the Thelen group. These proteins resemble the BCCP subunit to ACCase. Knocking out increases oil content in Arabidopsis and the effect is currently being tested in camelina and soybean. The Thelen group also demonstrated that the alpha-carboxyltransferase subunit to ACCase is less abundant in vivo and therefore limits fatty acid synthesis; in collaboration with Koo, overexpression increases oil content in Arabidopsis and camelina.</p><br /> <p>Doug Allen reported that tobacco engineered to have 30% leaf oil because there is no transient starch. In collaboration with Durrett, he has identified soybean fast neutron mutant lines to reduce oligosaccharides and increase oil.</p><br /> <p>Research from the Wang group has explored lipid-protein interaction with cytosolic proteins, some of which are transcription factors, with interesting functions. In addition, the group has been investigating how post-translational modifications on lipid metabolic enzymes that allow attachment to the membrane, and how the stability of these interactions is important for their function.</p><br /> <p>Rebecca Roston reported that lipid changes in response to cold are equivalent to those observed in response to the diurnal cycle.</p><br /> <p> </p><br /> <p><span style="text-decoration: underline;">Aim3: </span><span style="text-decoration: underline;">Develop crops with improved yield and functionality</span></p><br /> <p>Through co-expression of AtDGAT1 and GPD1, the Parkash group has increased the oil and seed yield of camelina. They have also Identified WRI1, MGAT1, PDCT1 and SDP1 genes as key genes for further improving TAG synthesis and accumulation and are currently overexpressing these genes individually and in combination in camelina.</p><br /> <p>Allen and Wang coexpressed two Arabidopsis phospholipase genes Dz1 and Dz2 in <em>Camelina sativa</em> to test how the conversion of PC to DAG impacts TAG levels in seeds.</p><br /> <p>The Allen group has developed a new method developed to look at absolute protein quantities using LC-MS/MS and a full set of C13 amino acid standards. This approach compares well to Kjeldahl and other traditional methods.</p><br /> <p>The Durrett group has developed transgenic camelina lines that produce over 90% acetyl-TAG. These lines have been crossed with lines producing medium-chain fatty acids or ricinoleic acid to further alter the properties of acetyl-TAGs. In collaboration with Toni Wang, the viscosity and cold-temperature properties of blends of acetyl-TAG and regular TAG were quantified.</p><br /> <p>CRISPR genome editing was used by the Clemente group to modify sorghum K1C genes to create variants with reduced karfirin levels and improved protein quality and digestibility. In collaboration with Cahoon, Clemente enhanced aquaculture feed quality of soybean with EPA, Vitamin E and astaxanthin. However, increased astaxanthin results in decreased seed oil.</p><br /> <p>Roston and Clemente have collaborated to improve cold tolerance in wheat and sorghum through the expression of transcription factors. Work by Clemente and Markham to modify sphingolipid content has led to improved cold temperature tolerance in sorghum.</p><br /> <p>Sam Wang has been working to increase abiotic resistance in Arabidopsis and to some extent in rice.</p><br /> <p>The Wurtele group explored the relationship between QQS, carbon and nitrogen partitioning, and defense in a series of Arabidopsis mutants affecting starch metabolism. Results indicate potential of QQS and NF-YC4 to increase protein and improve defensive traits in crops.</p>Publications
<p>Bansal S, Kim H-J, Na G, Hamilton ME, Cahoon EB, Lu C, Durrett TP. (2018) Towards the synthetic design of camelina oil enriched in tailored acetyl-triacylglycerols with medium-chain fatty acids. <em>Journal of Experimental Botany</em> 69:4395</p><br /> <p>Belayneh HD, Wehling RL, Cahoon EB, Ciftci ON (2018) Lipid composition and emulsifying properties of <em>Camelina sativa</em> seed lecithin. <em>Food Chemistry</em> 242:139</p><br /> <p>Chikara S, Abdullah H, Akbari P, Ablordeppey K, Parkash Dhankher O. (2018) Combined expression of GPD1 and DGAT1 increased oil and seed yield in <em>Camelina sativa</em>. <em>Plant Biotechnology</em> 16:1034</p><br /> <p>Li X, Teitgen AM, Shirani A, Ling J, Busta L, Cahoon RE, Zhang W, Li Z, Chapman KD, Berman D, Zhang C, Minto RE, Cahoon EB (2018) Discontinuous fatty acid elongation yields hydroxylated seed oil with improved function. <em>Nature Plants </em>4:711</p><br /> <p>Narayanan S, Prasad PVV, Welti R. (2018) Alterations in wheat pollen lipidome during high day and night temperature stress. <em>Plant, Cell and Environment</em> 41:1749</p><br /> <p>Paper JM, Mukherjee T, Schrick K. (2018) Bioorthogonal click chemistry for fluorescence imaging of choline phospholipids in plants. <em>Plant Methods</em> 14:31</p><br /> <p>Raju SKK, Barnes A, Schnable JC, Roston RL. (2018) “Low-temperature tolerance in land plants: Are transcript and membrane responses conserved?” <em>Plant Science</em> doi: 10.1016/j.plantsci.2018.08.002</p><br /> <p>Shiva S, Enninful R, Roth MR, Tamura P, Jagadish K, Welti R. (2018) An efficient modified method for plant leaf lipid extraction results in improved recovery of phosphatidic acid. <em>Plant Methods</em> 14:1</p><br /> <p>Yu XH, Cahoon RE, Horn PJ, Shi H, Prakash RR, Cai Y, Hearney M, Chapman KD, Cahoon EB, Schwender J, Shanklin J (2018) Identification of bottlenecks in the accumulation of cyclic fatty acids in camelina seed oil. <em>Plant Biotechnology Journal</em> 16: 1926-1938</p><br /> <p>Yurchenko O, Kimberlin A, Mehling M, Koo AJ, Chapman KD, Mullen RT, Dyer JM. (2018) Response of high leaf-oil <em>Arabidopsis thaliana</em> plant lines to biotic or abiotic stress. <em>Plant Signaling and Behavior</em> 13: e1464361.</p>Impact Statements
Date of Annual Report: 10/04/2019
Report Information
Period the Report Covers: 10/01/2018 - 09/30/2019
Participants
Cahoon, Edgar - University of Nebraska (UNL); Clemente, Thomas - UNL; Durrett, Timothy - KSU; Hoffmann-Benning, Susanne - Michigan State University; Kosma, Dylan - University of Nevada Reno (UNR); Lee, Young-Jin - Iowa State University; Louis, Joe - University of Nebraska-Lincoln; Minton, Ernie - Kansas State University (KSU); Roston, Rebecca - UNL; Schrick, Kathrin - KSU; Thelen, Jay - University of Missouri - Columbia; Welti, Ruth - KSU; Om Parkash Dhankher- University of Massachusetts Amherst.Brief Summary of Minutes
The 2019 NC-1203 meeting was held September 21-22 in Lincoln, Nebraska at the University of Nebraska. The meeting was opened by representatives of the hosts: Jonathan Markham, Rebecca Roston, Ed Cahoon, Julie Stone, Joe Louis, and Tom Clemente, and included remarks from KSU Interim Dean and Multistate Project Administrative Advisor Ernie Minton. A time of introductions was followed by presentations and discussions for each of the individual project aims. These were led by Jonathan Markham, Rebecca Roston, and Ed Cahoon; accomplishments for each of the aims are summarized below. This was followed by a discussion of future plans for each of the aims and the timing of the 2020 annual meeting to be held in Manhattan, Kansas. The 2021 meeting will be held at the Danforth Center and hosted by Doug Allen and Sam Wang. Susanne Hoffmann-Benning was elected as secretary and Michigan State University was chosen as the venue for the 2022 meeting. The project renewal was discussed. Ernie Minton indicated the current project runs until September 30, 2021 but a renewal should be submitted in the fall of 2020. It was agreed that Jonathan Markham would oversee the rewrite, Ruth Welti would address Aim 1, Rebecca Roston Aim 2, and Tim Durrett Aim 3 specifically. The ongoing movement of NIFA to Kansas City was also discussed.
Accomplishments
<p>Activities and accomplishments related to each of the project’s three objectives are described below, based on talks by each member and other information presented at the meeting. At the end of each presentation, group members engaged in active discussion on the challenges of each aim as well as alternative approaches to overcome these problems and to meet the remaining goals. Members also considered opportunities to work together on joint publications, either individual papers or a special issue on lipids in an appropriate journal.</p><br /> <p><span style="text-decoration: underline;">Objective 1: Improve and extend methods for lipid characterization and measurement.<strong> </strong></span></p><br /> <p>The Welti group has worked on making all the components available for direct-infusion multiple reaction monitoring (MRM) mass spectrometry using a Sciex 6500+ triple quadrupole MS. The components include a rapid extraction method, standards available at cost, all parameters for MRM (and the data acquisition method downloadable from the web), and a complete data processing method that employs Sciex software and the online site LipidomeDB Data Calculation Environment. They have written a paper pointing users to all components. They are also updating Lipidome DB Data Calculation Environment to support collision induced fragment analysis by time-of-flight mass spectrometry; this technique can be used for fatty acid analysis at the chemical formula level. Ruth Welti, Tim Durrett, and Jonathan Markham are beginning a project to develop a lipid mass map (visualization of fragments vs. intact ion m/z) which will be used to analyze data from plants across the plant kingdom. The data will be stored in a database of complex lipid compositions. In addition, Jonathan Markham presented a cross disciplinary platform to measure lipids and model with flux balance analysis with a goal of establishing steps controlling flux through metabolism. He described challenges including a need for multiplexing and faster mass spectrometry, and that some lipid classes were more problematic than others (e.g. phosphatidic acid). In the future his group will attempt to leverage in-source ozonolysis and will create a searchable database for retention times.</p><br /> <p>The Lee group is also interested in mass spectrometry methods and continues to develop MALDI matrices for the visualization and localization of lipids with screening of metal target deposition as a MALDI matrix. Gold doped with sodium dramatically improves TAG signals but suppress phospholipids and remains a candidate of interest. Lee and Durrett submitted a NIFA proposal to study the spatiotemporal development of acetyl-TAG in genetically engineered camelina.</p><br /> <p>Others are using radiolabels as well as microscopy, FRET, and mass spectrometry to interrogate lipid metabolism. Work in the Hoffmann-Benning lab demonstrated that 14C-labeled PA moves in the phloem from source to sink, but it remains to be confirmed whether the labeled mobile compound is still PA and whether PLAFP affects its movement and is a work in progress. Currently the lab is working on FRET experiments to establish PLAFP interactions and whether it moves with the lipid in vivo. In this context the lab has generated constructs using a light-inducible promoter - PLAFP - YFP fusion for inducible and local expression of the gene. A small non-mobile protein and FT are used as negative and positive controls. Movement of the protein can be monitored using confocal microscopy and Western blotting. Movement of lipid will be monitored using mass spectrometry. Constructs are now ready for testing in protoplasts and plants. An NSF EAGER grant is funding this work.</p><br /> <p>Pertaining to enhanced data analysis, the Wurtele group developed a semi-automated mechanism for upload of data that is connected to Araport and is awaiting data sets from participants for the Plant/Eukaryotic and Microbial Systems Resource (PMR; http://metnetdb.org/PMR/). It is recognized that the analysis of complex lipid data is challenging and impedes progress related to methods being developed as part of this grant.</p><br /> <p><span style="text-decoration: underline;">Objective 2: Lipid-related metabolism and traits relevant for crop improvement</span></p><br /> <p>The Cahoon lab described an interest in looking at the extremes of fatty acid biosynthesis including medium chain fatty acid levels in Cuphea and very long chain fatty acids in various Brassicaceae. For the medium chain fatty acids, it may be a question of acyl-ACPs that are present in vivo. Since all fatty acid synthesis genes are expressed, it may be that there are structural or stoichiometric differences. The same may also be true in specialty fatty acids such as petroselinic acid production. Cahoon emphasized that gaps in our knowledge include understanding the stoichiometry of Type II fatty acid synthase components and our ability to routine measure acyl-ACP pools in plant tissues.</p><br /> <p>Welti, Durrett, and Schrick are working together to characterize the function of several plant genes that were identified in a lipid profiling screen of Arabidopsis T-DNA mutants as having complex lipid profiles that are significantly different from wild-type plants. One gene is involved in fatty acid desaturation, one is involved cutin metabolism, and one seems to be involved in lysophospholipid acylation. Welti’s lab is also working to identify genes involved in lipid metabolism that have natural variation affecting the activities of associated gene products. Particularly, they are interested in natural variation that results in altered enzyme activities during response to wounding and mild freezing stress. Some of the lipid analyses have been done in collaboration with Abe Koo. Koo also described the 2018 participants of NSF outreach program Sci-LiFT had conducted no-choice insect feeding assays on lines overexpressing WRI1 and/or those reduced in ADP-glucose pyrophosporylase generated by Sanjaya and Christoph Benning. The Lewis lab also reported on inset feeding by examining sorghum defense response to sugarcane aphids. They have screened NAM parent lines to identify resistance and are exploring the role of lignin, surface waxes, and other lipids in plant defense. The transcriptional regulation of potato tuber wound healing, in terms of the biosynthesis and deposition of the lipidic polymer suberin, is a topic being explored in the Kosma lab. Kosma is continuing characterize potato cultivars that differ in storage life and tuber wound suberin deposition. Kosma has determined that two different transcription factors, StMYB74 and StMYB102, are sufficient for the production of suberin and are likely one component of the differential wound healing capacity of potato cultivars that differ in storage life. Kosma’s group has further conducted a large comparative RNA-seq experiment on 2 potato cultivars that differ in storage life and wound suberin deposition (Snowden deposits more suberin during wound healing and has a longer storage life whereas Atlantic deposits less wound suberin and has a poor storage life). Initial observations confirm that Snowden has higher expression of suberin biosynthetic genes during the course of wound healing. Kosma has generated community resources in the form of a potato wound healing-specific coexpression network that will be released to the public in the next year.</p><br /> <p>Pertaining to abiotic stresses, the Kutty (Narayanan) lab, in collaboration with Welti, showed that wheat decreases the unsaturation levels of plastidic and extra-plastidic glycerolipids of leaf and/or pollen in order to adapt to heat stress. Lipid analysis of leaves of multiple soy lines under heat stress are underway. The Schrick group has been studying the role of lipids in signaling through identification of START-domain transcription factors and their role in DNA binding, gene regulation and development of trichomes and other features of the epidermis. Phospholipids have been identified as putative binding partners of these transcription factors. One of the family members has been identified to bind a phosphate starvation response element to negatively regulate several phospholipid catabolism genes.</p><br /> <p>The Hoffmann-Benning lab recently identified a class of novel lipid-binding proteins in phloem sap that provides motivation for a number of studies. Overexpression causes larger plants with bigger vascular bundles. In addition, the phloem lipid profile is changed. Putative interacting proteins have been identified and are investigated for their role in lipid-signaling and would be important to composition and yield.</p><br /> <p>Overall enhancements in lipid production were also described by the Thelen group. In particular, strategies for improving acetyl-CoA carboxylase performance including BADC repression, alpha-CT overexpression, and CTI repression. Like the BADCs, the CTIs are a previously uncharacterized gene family discovered by the Thelen lab to be associated with the heteromeric ACCase. The CTIs are envelope membrane proteins that anchor ACCase through direct interaction with the alpha-CT subunits. They are using multiple approaches to examine structure and derive the mechanisms that can lead to higher oil in oilseed species including Arabidopsis, Camelina, and soybean. Some of these lines will be analyzed through an NSF-Plant Genome Research project with Koo and Allen. This project will also develop a website dedicated to the study of oil production in plants called “Fat Plants” available at fatplants.net. The Allen lab is working on related projects including the analysis of repartitioning of carbon from starch to oil in trangenic tobacco plants that produce high levels of lipids, the analysis of fluxes in Chlamydomonas grown auto- or mixotrophically, and an in-silique method for isotopically labeling camelina that will be used with transgenic lines obtained from Thelen and Koo labs. The Allen lab continues to work on methods for using 13C analysis with high resolution mass spectrometry to analyze fluxes through lipid metabolism and methods to quantify acyl-acyl carrier proteins. A project with the Durrett lab includes investigations in soybean metabolism to understand the partitioning of oil late in development into other storage reserves. The Durrett group has also been exploring the role of the lipase PLIP1 in supplying FA for the synthesis of TAG in developing Arabidopsis seeds. PLIP1 is upregulated in dgat1 seed. Double mutant <em>plip1 dgat1</em> seed are green and fail to germinate. Ongoing work is testing the hypothesis that PLIP1 provides a PUFA-substrate pool necessary for the function of PDAT1. Research from Dhankher group studied the comparative transcriptome and metabolome analysis suggesting bottlenecks that limit seed and oil yields in transgenic Camelina sativa expressing Arabidopsis diacylglycerol acyltransferase 1 (DGAT1) and yeast glycerol‑3‑phosphate dehydrogenase (GPD1). They concluded that TAG production is limited by (1) utilization of fixed carbon from the source tissues supported by the increase in glycolysis pathway metabolites and decreased transcript levels of transcription factors controlling fatty acids synthesis; (2) TAG accumulation is limited by the activity of lipases/hydrolases that hydrolyze TAG pool supported by the increase in free fatty acids and monoacylglycerols.</p><br /> <p>Research from the Wang group has focused on lipid-protein interactions with cytosolic proteins, some of which are transcription factors, with interesting functions. In addition, the group has been investigating how post-translational modifications on lipid metabolic enzymes that allow attachment to the membrane, and how the stability of these interactions is important for their function.</p><br /> <p><span style="text-decoration: underline;">Objective 3: Develop crops with improved yield and functionality</span></p><br /> <p>The Durrett group has been studying the germination of seeds that produce high levels of acetyl-TAGs. Synthetic biology approaches are being used to alter the timing of EfDAcT expression to increase levels of accumulation in seeds; other projects aim to incorporate other fatty acids (e.g. ricinoleic acid) into acetyl-TAG molecules to further alter properties.</p><br /> <p>The Clemente group reported on a number of genetic modifications to alter the levels of storage proteins in soybeans, alter sulfur amino acid production, change sucrose levels, and examine QTLs for high protein. Roston, Markham, and Clemente continue to work on cold tolerance in important crops including wheat and sorghum through the expression of transcription factors and examinations in sphingolipid content. The Roston lab has identified important lipid changes cycle in response to cold and current efforts from multiple labs that appeared to be conflicting are now hypothesized to be in congruence. Multiple species have similar cycles in lipid abundance; cold tolerant species deviate from non-tolerant species at key times. They also showed that lipid responses to freezing tolerance are greatly increased in ancient lineages and suggested the hypothesis that severe stress responses may be derived from a stronger ancestral response to damage of any type. The Wurtele group explored the relationship between QQS, carbon and nitrogen partitioning, and defense in a series of Arabidopsis mutants affecting starch metabolism. Results indicate QQS and NF-YC4 can increase protein and improve defensive traits in crops. Using a mapping population of a cross between two potato cultivars that differ in terms of wound suberin deposition and storage life, Kosma’s collaborators, Dave Douches and Ray Hammerschmidt of Michigan State University, have identified 2 QTLs potentially related to better wound suberin deposition. Several of the progeny of the cross were shown to have high wound suberin deposition than either parent. These lines are being evaluated for disease resistance and storage life. The Dhankher group identified and overexpressed a bifunctional wax synthase/acyl-CoA:diacylglycerol acyltransferase (WSD1) gene, which plays a critical role in wax ester synthesis in Arabidopsis stem and leaf tissues. Gas chromatography and electron microscopy analyses of WSD1 transgenic Arabidopsis seedlings showed higher deposition of epicuticular wax crystals and increased leaf and stem wax loading in WSD1 transgenic lines as compared to wildtype (WT) plants. Transgenic plants showed strong tolerance to drought and salinity. Their results clearly show that the manipulation of cuticular waxes will be advantageous for enhancing plant productivity under changing climate. The Clemente lab reported on progress made in complex metabolic engineering to improve soybean oil composition for food and feed applications. These included the development of a high melting point soybean oil containing elevated content of palmitic acid, stearic acid, and oleic acid. The oil is a semi-solid at room temperature and is now being evaluated for animal fat-type functionality by industry. Also reported were efforts to assemble multi-gene expression cassettes in collaboration with the Cahoon lab to generate soybean oil with omega-3, astaxanthin, and high vitamin E antioxidant content for use as a sustainable aquaculture feedstock. Clemente also discussed the need for low-cost processing technology that can be deployed in rural settings to enable commercialization of high value/low acreage specialty compositional traits in soybeans and other crops. It was also noted that the Clemente and Cahoon labs have been building a cataloged repository of genetic elements (e.g., promoters, 3’UTRs) that have been domesticated for use in GoldenBraid modular gene assembly that are available for the Multistate group to facilitate metabolic engineering and crop improvement efforts. One theme that arose from several talks was reduced seed performance associated with some of the engineered oil traits and the need for a coordinated effort to understand the metabolic basis for these phenotypes.</p>Publications
<p>Abdullah HM, Chikara S, Akbari P, Schnell DJ, Pareek A, Dhankher OM. (2018). Comparative transcriptome and metabolome analysis revealed the bottlenecks for increasing the seed and oil yields in transgenic <em>Camelina sativa</em> expressing diacylglycerol acyltransferase 1 and glycerol-3-phosphate dehydrogenase. Biotechnology for Biofuels, 11 (1): 335. doi: 10.1186/s13068-018-1326-2.</p><br /> <p>Ahsan N, Wilson RS, Thelen JJ. (2018) Absolute Quantitation of Plant Proteins<em>. Curr Protoc Plant Biol</em>. 3:1-13.</p><br /> <p>Augustin JM, Brock JR, Augustin MM, Wellinghoff RL, Shipp M, Higashi Y, Kumssa TT, Cahoon EB, Kutchan TM (2019) Field performance of terpene-producing <em>Camelina sativa</em>. Industrial Crops and Products 136: 50-58.</p><br /> <p>Busta L, Yim W, LaBrant EW, Wang P, Grimes L, Malyszka K, Cushman JC, Santos P, Kosma DK, Cahoon EB (2018) Identification of genes encoding enzymes catalyzing the early steps of carrot polyacetylene biosynthesis. Plant Physiology 178: 1507-1521.</p><br /> <p>Fruehan C, Johnson D, Welti R. 2018. LipidomeDB Data Calculation Environment has been updated to process direct-infusion multiple reaction monitoring data<a href="https://www.ncbi.nlm.nih.gov/pubmed/30575037">.</a> Lipids 53, 1019-1020. doi: 10.1002/lipd.12111.</p><br /> <p>Hur M, Ware R, Park J, McKenna A, Rodgers R, Nikolau B, Wurtele E, Marshall A. 2018. Statistically significant differences in composition of petroleum crude oils revealed by volcano plots generated from ultrahigh resolution fourier transform ion cyclotron resonance mass spectra. Energy and Fuels. Fuels 32:1026–1212 DOI: 10.1021/acs.energyfuels.7b03061</p><br /> <p>Kambhampati S, Li J, Evans BS, Allen DK. 2019. Accurate and efficient amino acid analysis for protein quantification using hydrophilic interaction chromatography coupled tandem mass spectrometry. Plant Methods 15:46-58</p><br /> <p>Karanbir Aulakh and Timothy P. Durrett. 2019. The plastid lipase PLIP1 is critical for seed viability in diacylglycerol acyltransferase 1 mutant seed. Plant Physiology 180: 1962-1974. doi: 10.1104/pp.19.00600</p><br /> <p>Kim S, Nusinow DA, Sorkin M, Pruneda-Paz J, Wang X. 2019. Interaction and Regulation Between Lipid Mediator Phosphatidic Acid and Circadian Clock Regulators. Plant Cell 31:399-416.</p><br /> <p>Koenig A, Benning C, Hoffmann-Benning, S. 2020. Lipid trafficking and signaling in plants. Invited review for “Lipid Signaling and Metabolism” (Elsevier), edited by James Ntambi; planned publication date: 1/2020</p><br /> <p>Kun Wang, Timothy P. Durrett and Christoph Benning. 2019. Functional Diversity of Glycerolipid Acylhydrolases in Plant Metabolism and Physiology. Progress in Lipid Research. (in press) https://doi.org/10.1016/j.plipres.2019.100987</p><br /> <p>Li J, Wang X. 2019. Phospholipase D and phosphatidic acid in plant immunity. Plant Science 279:45-50</p><br /> <p>Li S, Jia S, Hou L, Nguyen H, Sato S, Holding D, Cahoon E, Zhang C, Clemente T, Yu B (2019) Mapping of transgenic alleles in soybean using a Nanopore-based sequencing strategy. Journal of Experimental Botany 70: 3825-3833.</p><br /> <p>Li-Beisson Y, Thelen JJ, Fedosejevs E, Harwood JL. (2019) The lipid biochemistry of eukaryotic algae. <em>Prog Lipid Res.</em> 74:31-68.</p><br /> <p>McClain S, Stevenson SE, Brownie C, Herouet-Guicheney C, Herman RA, Ladics GS, Privalle L, Ward JM, Doerrer N, Thelen JJ. (2018) Variation in Seed Allergen Content from three Varieties of Soybean Cultivated in Nine Different Locations in Iowa, Illinois, and Indiana. <em>Front Plant Sci.</em> 9:1025.</p><br /> <p>McGinn M, Phippen WB, Chopra R, Bansal S, Jarvis BA, Phippen ME, Dorn KM, Esfahanian M, Nazarenus TJ, Cahoon EB, Durrett TP, Marks MD, Sedbrook JC (2019) Molecular tools enabling pennycress (<em>Thlaspi arvense</em>) as a model plant and oilseed cash cover crop. Plant Biotechnol J 17: 776-788</p><br /> <p>Mukherjee, T., Lerma-Reyes, R., Thompson, K.A., Schrick, K. 2019. Making glue from seeds and gums: Working with plant-based polymers to introduce students to plant biochemistry. <a href="https://iubmb.onlinelibrary.wiley.com/doi/epdf/10.1002/bmb.21252?author_access_token=2UbQXTIuwvdRypz5tNM2jE4keas67K9QMdWULTWMo8MpFz1l0acm4uioeknFN00jp0XrKBx4EfyWbxhQJ18pHn5M8TBLAlxg-pRz2QduqnLmYqE6kJr58Z6pVIbq9DIK"> </a>Biochem Mol Biol Educ 47:468-475. DOI:10.1002/bmb.21252</p><br /> <p>Narayanan S, Prasad PVV, Welti R. 2018. Alterations in wheat pollen lipidome during high day and night temperature stress. Plant Cell Environ. 41, 1749-1761. doi: 10.1111/pce.13156. </p><br /> <p>Qi M, Zheng W, Zhao X, Hohenstein J, Kandel Y, O'Conner S, Wang Y, Du C, Nettleton D, MacIntosh GC, Tylka GL, Wurtele ES, Whitham SA, Li L. 2018. QQS orphan gene and its interactor NF-YC4 reduce susceptibility to pathogens and pests. Plant Biotechnology. DOI:10.1111/pbi.12961. PMID:29878511</p><br /> <p>Romsdahl T, Shirani A, Minto RE, Zhang C, Cahoon EB, Chapman KD, Berman D (2019) Nature-guided synthesis of advanced bio-lubricants. Scientific Report<em>s</em> 9: 11711</p><br /> <p>Singh U, Hur M, Dorman K, Wurtele E. 2019. MetaOmGraph: a workbench for interactive exploratory data analysis of large expression datasets. bioRxiv. 1:698969.</p><br /> <p>Vogel PA, Bayon de Noyer S, Park H, Nguyen H, Hou L, Changa T, Khang HL, Ciftci ON, Wang T, Cahoon EB, Clemente TE (2019) Expression of the Arabidopsis WRINKLED 1 transcription factor leads to higher accumulation of palmitate in soybean seed. Plant Biotechnology Journal 17: 1369-1379.</p><br /> <p>Wilson RS, Thelen JJ. (2018) In Vivo Quantitative Monitoring of Subunit Stoichiometry for Metabolic Complexes. <em>J Proteome Res</em>. 17:1773-1783.</p><br /> <p>Xie L, Cahoon E, Zhang Y, Ciftci ON (2019) Extraction of astaxanthin from engineered <em>Camelina sativa</em> seed using ethanol-modified supercritical carbon dioxide. Journal of Supercritical Fluids 143: 171-178.</p><br /> <p>Yang F, Kimberlin AN, Elowsky CG, Liu Y, Gonzalez-Solis A, Cahoon EB, Alfano JR (2019) A plant immune receptor degraded by selective autophagy. Molecular Plant 12:113-123</p><br /> <p>Zhang N, Rao RSP, Salvato F, Havelund JF, Møller IM, Thelen JJ, Xu D. (2018) MU-LOC: A Machine-Learning Method for Predicting Mitochondrially Localized Proteins in Plants. <em>Front Plant Sci</em>. 9:634.</p><br /> <p>Zhang, Q., Wijk, R., Zarza, X., Shahbaz, M., Vermeer, J.E.M., Guardia, A., Scuffi, D., García-Mata, C., Van den Ende, W., Hoffmann-Benning, S., Haring, M., Laxalt, A.M., and Munnik, T. 2018. Knock-down of Arabidopsis PLC5 Reduces Primary Root growth and Secondary Root Formation while Overexpression Improves Drought Tolerance and Causes Stunted Root Hair Growth"<a href="https://www.ncbi.nlm.nih.gov/pubmed/29309666"> Plant Cell Physiol.</a> doi: 10.1093/pcp/pcy120</p><br /> <p>Zhou X, Chen X, Du Z, Zhang Y, Zhang W, Kong X, Thelen JJ, Chen C, Chen M. (2019) Terpenoid Esters Are the Major Constituents From Leaf Lipid Droplets of Camellia sinensis. <em>Front Plant Sci.</em> 26;10:179.</p><br /> <p><strong>Software </strong></p><br /> <ol><br /> <li>MetaOmGraph.(PMR, http://metnetdb.org/PMR/ ) Java software enabling users to input and evaluate big data sets. v2019</li><br /> </ol><br /> <ol start="2"><br /> <li>Plant, Eukaryotic and Microbial Metabolomics Systems Resource (PMR, http://metnetdb.org/PMR/ ) A public database for metabolomics data and associated transcriptomics and most recently MS-imaging data from multiple species.</li><br /> </ol><br /> <ol start="3"><br /> <li>LipidomeDB Data Calculation Environment (lipidome.bcf.ku.edu:8080/Lipidomics/) An online site for data processing for direct-infusion mass spectral data.</li><br /> </ol><br /> <p> </p><br /> <p><strong>Patents</strong></p><br /> <p>Cahoon EB, Iskandarov U, Collins-Silva J, Kim HJ (2019) Novel acyltransferases and methods of using. US 10,280,431 B2. </p><br /> <p>Clemente TE, Park H, Cahoon EB, Nguyen H (2018) Method for the production of high saturated, low polyunsaturated soybean oil. US Patent # US10,100,325B2.</p><br /> <p>Timothy Durrett, Tam N.T. Tran and Catherine Kornacki (2018) Improved enzymes for the synthesis of acetyltriacylglycerols. PCT Application PCT/US2018/029590</p><br /> <p>Thelen JJ, Salie MJ (2016) Increasing plant oil content by altering a negative regulator of acetyl-CoA carboxylase. PCT/US2016/041386. Licensed to Yield10 Bioscience.</p><br /> <p>Thelen JJ (2017) Increasing plant oil content by improving activity of acetyl-CoA carboxylase. PCT/US17/40851. Licensed to Yield10 Bioscience.</p><br /> <p>Thelen JJ, Ye Y (2018) Increasing seed oil content by manipulating carboxyltransferse interactor protein expression. Disclosure filed.</p>Impact Statements
Date of Annual Report: 11/05/2020
Report Information
Period the Report Covers: 12/01/2019 - 11/30/2020
Participants
Cahoon, Edgar - University of Nebraska (UNL); Clemente, Thomas - UNL; Durrett, Timothy - KSU; Hoffmann-Benning, Susanne - Michigan State University; Kosma, Dylan - University of Nevada Reno (UNR); Lee, Young-Jin - Iowa State University; Louis, Joe - University of Nebraska-Lincoln; Minton, Ernie - Kansas State University (KSU); Roston, Rebecca - UNL; Schrick, Kathrin - KSU; Thelen, Jay - University of Missouri - Columbia; Welti, Ruth - KSU; Dhankher, Om Parkash - University of Massachusetts Amherst; Koo, Abraham - MU; Narayanan, Sruthi - Clemson University. Xuemin (Sam) Wang (Danforth Center/UMSL), Doug Allen (Danforth Center/USDA), Phil Bates (WSU), Julie Stone (UNL).Brief Summary of Minutes
The 2020 NC-1203 meeting was held virtually on September 11 through 13. The meeting was opened by representatives of the hosts: Timothy Durrett and Ruth Welti, and included remarks from Multistate Project Administrative Advisor Ernie Minton. Rather than giving detailed updates, the group discussed their research in the context of the upcoming project renewal and the respective proposed experiments and collaborations. The renewal will be submitted in fall 2020. The 2021 meeting will be held at the Danforth Center and hosted by Doug Allen and Sam Wang. The 2022 meeting will be held at Michigan State University and be hosted by Susanne Hoffmann-Benning. Jonathan Markham will oversee the rewrite, Ruth Welti and Young-Jin Lee will address Aim 1, Rebecca Roston and Susanne Hoffmann-Benning Aim 2, and Tim Durrett and Ed Cahoon Aim 3 specifically.
Accomplishments
<p>A pdf file containing the entire report and publications will be attached under "publications"</p>Publications
<p>See attached file</p>Impact Statements
Date of Annual Report: 09/20/2021
Report Information
Period the Report Covers: 09/20/2020 - 09/30/2021
Participants
Participants: Allen, Doug - Danforth Center/USDA; Bates, Philip - Washington State University (WSU); Cahoon, Edgar - University of Nebraska (UNL); Clemente, Thomas - UNL; Dhankher, Om Parkash - University of Massachusetts Amherst; Durrett, Timothy - Kansas State University (KSU); Hoffmann-Benning, Susanne - Michigan State University; Koo, Abraham - MU; Kosma, Dylan - University of Nevada Reno (UNR); Lee, Young-Jin - Iowa State University (ISU); Louis, Joe - University of Nebraska- Lincoln; Minton, Ernie - KSU; Narayanan, Sruthi - Clemson University; Nikolau, Basil - ISU; Roston, Rebecca - UNL; Schrick, Kathrin - KSU; Stone, Julie - UNL; Thelen, Jay - University of Missouri - Columbia; Wang, Xuemin (Sam) - Danforth Center/UMSL; Welti, Ruth - KSU; Yandeau-Nelson, MarnaBrief Summary of Minutes
Brief summary minutes of annual meeting: The 2021 NC-1203 meeting was held virtually on August 27. The meeting was opened by the host, Doug Allen and included remarks from Multistate Project Administrative Advisor Ernie Minton. Group members provided updates on experiments and collaborations. The renewal was submitted in fall 2020 and was accepted. The 2022 meeting will be held at Michigan State and hosted by Susanne Hoffmann-Benning. The 2023 meeting will be at University of Nebraska-Lincoln, hosted by Tom Clemente. The 2024 meeting will be hosted by Dylan Kosma at University of Nevada-Reno.
Accomplishments
<p><strong>NC-1203 2016-2021 Accomplishments.</strong></p><br /> <p>Activities and accomplishments related to each of the project’s three objectives are described below. In addition, the report communicates on the collaborative efforts among the LIPIDS members, including outcomes of these collaborations in the form of publications and/or grant activities.</p><br /> <p> </p><br /> <p><span style="text-decoration: underline;">Objective 1: Improve and extend methods for lipid characterization and measurement</span>.</p><br /> <p> </p><br /> <p>The Welti group has made all the reagents and methods available for relatively rapid (8 min/sample) direct- infusion multiple reaction monitoring (MRM) mass spectrometry using a Sciex 6500+ triple quadrupole MS (Song et al., 2020). This deliverable includes a rapid extraction method (Shiva et al., 2018), which was independently tested by Roston’s group (Mahboub et al., 2020), standards (available at cost), all parameters for MRM, including a data acquisition protocol, downloadable from the web (<a href="https://www.k-state.edu/lipid/analytical_laboratory/analysis_components/index.html">https://www.k-state.edu/lipid/analytical_laboratory/analysis_components/index.html</a>), along with a complete data processing pipeline, that employs Sciex software and the online site LipidomeDB Data Calculation Environment. The Lipidome DB Data Calculation Environment site has been updated twice: once to support lipids analysis by multiple reaction monitoring (Fruehan et al., 2018; Song et al., 2020), and once to support collision induced fragment analysis by time-of-flight mass spectrometry. This latter technique can be used for fatty acid analysis at the chemical formula level (Tamura et al., 2020). Markham’s group developed a cross disciplinary platform to measure lipids and model, with flux balance analysis, permitting the establishment of the blueprints outlining the steps controlling flux through metabolism.</p><br /> <p> </p><br /> <p>The Lee group is developing on-tissue chemical derivatization techniques to enhance the visualization and localization of low-abundance metabolites and lipids. This technique has successfully been employed, in conjunction with three derivatization methods, for primary amine, carboxylic and carbonyl functional groups that resulted in the visualization of over 600 new metabolites in maize leaf and root tissue cross sections (Objective 2). Enrichment of Arabidopsis, oxylipins, fatty acid derived signaling molecules associated with plant immune responses, in chloroplasts of FERONIA mutant, was detected employing this approach which was recently communicated (Hansen et al 2019). A collaborative effort between team members Durrett and Lee resulted in a funded USDA NIFA project to study the spatiotemporal localization of acetyl-TAG in camelina carrying a novel transgenic allele. Moreover, the Lee group archived the last ten years’ of MS imaging data at METASPACE repository (<a href="https://metaspace2020.eu/">https://metaspace2020.eu/</a>) for public access.</p><br /> <p> </p><br /> <p>The Schrick lab developed a fluorescent labeling approach to study <em>in situ</em> localization of choline phospholipids in plants (Paper et al., 2018). Data suggest choline phospholipids accumulate preferentially in guard cells. In collaboration with the Lee group they were successful in MS imaging of phosphatidylcholines in the epidermis layer of Arabidopsis leaves.</p><br /> <p>Other LIPIDS team members are using radiolabel reagents, as well as fluorescence resonance energy transfer (FRET) microscopy, and mass spectrometry tools to interrogate lipid metabolism. For example, the Hoffmann-Benning group developed an approach to reproducibly collect, purify and analyze lipids from phloem exudates (Hoffmann- Benning, 2021). They are currently assembling a set of plant usable light-switch elements (PULSE) constructs (red light induced; blue/white light repressed) which can be controlled both spatially and temporally, and allow for monitoring of lipid-binding proteins and their movement. Proof-of-concept of the PULSE constructs has been successfully shown in protoplasts and tobacco model systems. The PULSE constructs have been introduced into Arabidopsis and down-stream characterizations will be initiated shortly. The Hoffman-Benning group is also working on a FRET platform to determine location of protein-lipid interactions and translocation of the complex <em>in planta</em>. An NSF EAGER grant is funding to support this latter effort.</p><br /> <p><span style="text-decoration: underline;">Objective 2: Lipid-related metabolism and traits relevant for crop improvement</span></p><br /> <p>This component of the LIPIDS program targets two aspects of crop production i) the role of lipids in response to environmental factors, that can be translated to genetic approaches to protect yield and ii) the improvement of lipid profile and/or content in crop plants, and implementing what is learned from these activities to design genetic strategies to improve quality of output traits:</p><br /> <p>The three KSU groups, Welti, Durrett, and Schrick are collaborating on functional characterization of several plant genes that were identified in a lipid profiling screen of Arabidopsis T-DNA mutants in which the complex lipid profiles are significantly different from wild-type plants. The genes identified from this screen have annotated functions in fatty acid desaturation, cutin metabolism, and transacylase activities.</p><br /> <p>Another collaborative effort among the Welti, Schrick, and Wang groups resulted in a communication that described the changes which occur in Arabidopsis lipid composition and metabolism under heat stress (Shiva et al., 2020). While a collaboration between the Welti and Koo groups, has ongoing efforts seeking to gain insight on the genetic variation and the imapct on the phenotypic outcomes of genes involved in lipid metabolism. Particularly, they are looking at variation that impacts enzyme kinetics during response to wounding and mild freezing stress.</p><br /> <p>The Louis lab screened a maize NAM population to identify resistance towards insect predation, exploring the role of lignin, surface waxes, and other lipids in the plant defense responses upon an insect challenge. The outcome of this effort found that 12-oxo-phytodienoic acid (OPDA), an intermediate in the jasmonic acid (JA) biosynthesis pathway, promotes heightened maize resistance against corn leaf aphids, independent of the JA pathway.</p><br /> <p>More recently, Kosma has collaborated with John Cushman (non-member, UNR) to identify and characterize Camelina mutants with reduced seed mucilage content that may improve the efficacy of seed processing for oil (Lohaus et al., 2020) and with Isabel Molina (non-member) to provide an extensive characterization of the cuticular waxes, cutin, and suberin of camelina (Razeq et al., 2021). Kosma continues to collaborate on understanding mechanisms for the regulated biosynthesis of cuticular lipids (We et al., 2021).</p><br /> <p>Kosma’s main focus, however, targets understanding the gene regulatory networks controlling the wound suberin deposition with an emphasis on suberin function in potato tuber wound healing given the significant postharvest losses attributed to poor wound healing experienced by the potato industry (~33% <em>per annum</em>). Kosma has successfully identified 5 transcription factors in potato, 2 of which were described in a recent publication (Wahrenburg et al., 2021) and 4 in Arabidopsis that regulate different aspects of suberin deposition. Using an RNAseq approach, Kosma has generated community resources in the form of an eFP browser that permits exploration of global changes in the transcriptome of potato cultivars that differ in their capacity to deposit wound suberin and storage life and allows exploration of coexpression networks. Kosma has successfully used CRISPR-Cas9 to edit 3 transcription factors that regulate woud suberin biosynthesis in potato. Haplotype sequences for these 3 transcription factors were obtained using long-range PCR and PacBio SMRT sequencing from the Atlantic cultivar. Sequencing of CRISPR-Cas9 edited lines revealed substantial differences in the editing of different haplotypes. In some cases, multiple haplotypes were edited and in some cases not. Kosma is also working with Dr. Won Yim (non-member) on developing phased genomes for 4 commercial cultivars of chipping potatoes. 2 cultivars genome sequences are ~80% resolved.</p><br /> <p>Cahoon and Kosma have worked with Lucas Busta to identify genes and corresponding enzymes in the falcarin biosynthetic pathway of carrots (Busta et al., 2018). Falcarins are lipid-dervied metabolites known to function in plant defense against pathogens, to have anticancer activities, and one falcarin, falcarindiol, is known to be a major component of the bitter taste of carrots. Cahoon and Kosma recently received a USDA-NIFA grant to continue their study of the falcarin biosynthetic pathway.</p><br /> <p> </p><br /> <p>Yandeau-Nelson group is the newest member of LIPIDS of Crops joining in 2021. The team, in collaboration with the Nikolau’s group, is dissecting the metabolic and genetic networks underlying cuticular lipid deposition on various plant tissues, with an emphasis in maize silks and seedling organs. Using genetic statistical association approaches the team has identified metabolite-transcript correlations that highlight new gene candidates, with annotated functions in transcriptional regulation, fatty acid elongation, wax biosynthesis, transport, sphingolipid biosynthesis, oxylipin metabolism, and galactolipids synthesis. Her group is now characterizing these candidate genes via reverse genetic and heterologous expression approaches. Moreover, the team is exploring genotype x environment interactions that impact cuticular lipid accumulation, and will test the impacts of specific environmental parameters on cuticle</p><br /> <p>composition under controlled growth conditions. Additionally, the Nikolau group is integrating synthetic biology strategies to reconstitute the maize cuticular lipid pathway either in a single cell platform (i.e., yeast) (Campbell et al 2019) or in the model genetic plant species, Arabidopsis (Alexander et al 2020). Furthermore, in collaboration with the Lee-group, Nikolau has molecularly imaged the <em>in planta </em>deposition of cuticular lipids at a high spatial resolution (~50-µm resolution) on the leaf surfaces of maize leaves (Duenas, et al 2017) or on Arabidopsis leaves that are transgenically expressing maize cuticular lipid genes (Alexander et al. 2021). <br /><br /></p><br /> <p>To address aspects of protection of yield under abiotic stresses, the Narayanan lab, in collaboration with the Welti group, showed that decreases in the unsaturation levels of plastidic and extra-plastidic glycerolipids in leaf and/or pollen is an adaptive outcome in wheat exposed to heat stress (Narayanan et al., 2016a, 2016b, 2018). Similar lipid changes are observed in heat stress adaptation in wheat, leaves and pollen, though the lipidomes have inherently distinct compositions. This collaboration also investigated lipid metabolic changes in response to heat stress in soybean (Narayanan et al., 2020). Here an observed decrease in levels of lipids containing 18:3 acyl chains (linolenic acid) under heat stress is suggestive of a link with reduced expression of <em>Fatty Acid Desaturase </em>(<em>FAD</em>) <em>3A </em>and <em>FAD3B </em>genes. This decrease in 18:3, in heat tolerant genotypes, likely facilitates maintenance of membrane functionality upon exposure to heat. Decreases in unsaturation were also found to be associated with heat tolerance in peanut (Zoong Lwe et al., 2021) and <em>Brassica carinata</em> (Zoong Lwe et al., 2021). With funding from the USDA-NIFA, Narayanan and Welti will characterize the heat tolerance of members of a soybean recombinant inbred line population (derived from a genetic cross between a heat-tolerant and a susceptible genotype) and elite soybean genotypes by measuring physiological responses and identify molecular markers associated with heat-induced lipid metabolic changes.</p><br /> <p> </p><br /> <p>The Roston lab is also investigating aspects of protection of yield under abiotic stress. These efforts are searching for the core responses of membranes to temperature, specifically cold stress. Progress in our understanding of the role of cytosolic pH in severe cold sensing (Barnes et al. 2019), and in comparing available lipid abundance changes in related sets of species in response to cold (Yan et al., 2019, Kenchanmane et al., 2018, Zhang et al., 2017) has been accomplished. The Roston and Schrick labs started a new collaboration to investigate the roles of sterols in cold tolerance, and current data suggests that some sterol mutants have reduced freezing tolerance.</p><br /> <p> </p><br /> <p>The Schrick group has also been studying the role of lipids in signaling through identification of steroidogenic acute regulatory protein (StAR)-related lipid transfer (START)-domain transcription factors. In plants START domains are found in master regulators of cell developmental fate. Here her group is looking at their role in DNA binding, gene regulation and development of trichomes and other features of the epidermis. Lysophospholipids were identified as binding partners of these START- domain transcription factors. One family member was shown to bind a phosphate starvation response element to control the expression of several phospholipid catabolism genes. Additionally, her group has been investigating signaling functions of other lipid metabolites including sterol glucosides (Pook et al., 2017) and flavonoids (Velazhahan et al., 2020).</p><br /> <p> </p><br /> <p>Along similar lines, the Hoffmann-Benning lab identified a class of novel lipid-binding proteins (LBPs) in phloem sap with possible signaling function. Expression of genes for several of these proteins increases in response to abiotic stress. Overexpression of one LBP causes larger plants with bigger vascular bundles and a modified phloem lipid. Initial experiments also suggest increased drought tolerance (Barbaglia et al., 2016). Homologues of this LBP gene in soybean and tomato are also induced by ABA, suggesting that this protein has a broader function in plants. Computational analysis and molecular simulation modeling suggested interaction sites with phosphatidic acid, a plant lipid known to be associated with stress signaling. The lab has mutagenized the respective putative phosphatidic interaction sites and is currently investigating the effect on protein-lipid interaction, subcellular localization and functional complementation of plafp knock-down mutants.</p><br /> <p> </p><br /> <p>Research from the Wang group has focused on lipid signaling and regulation of lipid metabolism in plant growth, development, and stress responses, resulting in the identification of lipid-interacting transcription factors (Cai et al., 2019, 2020; Hoffmann-Benning, 2020).</p><br /> <p> </p><br /> <p>As communicated above, the Cahoon and Kosma labs have an ongoing collaborative effort to describe the genetic and evolutionary basis for a divergence in cuticular wax chemistry between sorghum and maize. Combining chemical analyses, heterologous expression studies, and comparative genomics approaches, they found that: (i) sorghum and maize leaf waxes are similar at the juvenile stage but, after the juvenile-to-adult transition, sorghum leaf waxes are rich in triterpenoids that are absent from maize, (ii) biosynthesis of the majority of sorghum leaf triterpenoids is mediated by a gene that maize and sorghum both inherited from a common ancestor but that is only functionally maintained in sorghum, and (iii) sorghum leaf triterpenoids accumulate in a spatial pattern that was previously shown to strengthen the cuticle and decrease water loss at high temperatures. The translational outcome of these findings that is being pursued is designing and introducing into maize the genetic variation that potentially could lead towards the resurrection of a cuticular triterpenoid-synthesizing gene in maize to create a more heat-tolerant water barrier on the plant’s leaf surfaces. To this end, working with the Clemente group and with Lucas Busta’s laboratory (U. Minnesota-Duluth) the functional sorghum allele is being introduced into maize in an attempt to generate a sorghum-like wax profile to test the hypothesis that wax triterpenoids are associated with improved water-use efficiency in sorghum.</p><br /> <p> </p><br /> <p>The Thelen group is developing genetic strategies leading towards the improvement of lipid content in oil seeds, while mitigating reduction in other seed reserves. The research targets approaches to improve acetyl-CoA carboxylase performance, including biotin/lipoyl attachment domain containing (BADC) protein repression, alpha-carboxyltransferase subunit of ACCase (alpha-CT) overexpression, and carboxyltransferase interactors (CTI) repression. Like the BADCs, the CTIs are a previously uncharacterized gene family discovered by the Thelen lab to be associated with the heteromeric ACCase (Ye et al 2020). The CTIs are envelope membrane proteins that anchor ACCase through direct interaction with the alpha-CT subunits. The group is using multiple approaches to examine structure and mechanistic outcomes of these interactions and translating the learned information for higher seed oil content with a focus on Arabidopsis, Camelina, and soybean. A subset of these large-scale biological findings will be analyzed through an NSF-Plant Genome Research project with Koo, Allen, and Bates groups. This project developed a website dedicated to the study of oil production in plants called “Fat Plants” available at fatplants.net.</p><br /> <p> </p><br /> <p>The Koo lab has developed camelina events overexpressing pea alpha-CT and RNAi events of <em>C. sativa </em>BADCs. Increased seed oil content in these events have been observed, which the Bates lab demonstrated increased rates of fatty acid synthesis in these events. Additionally, events have also been developed that carry genetic elements that will permit data to be captured through Translating Ribosome Affinity Purification RNAseq (TRAPseq) analyses. The Koo lab established and refined technical conditions (Kimberlin et al., 2021) and subsequently carried out RNAseq and TRAPseq analyses on developing seeds of WT and high oil events of Arabidopsis and camelina.</p><br /> <p> </p><br /> <p>Increases of oil in leaf tissue has been achieved through various metabolic pathway perturbation approaches in, Arabidopsis, tobacco and crop plants. The Koo lab has found positive correlation between increased oil in vegetative tissues and susceptibility of the plants to insect herbivory. Moreover, the group performed lipidomics and fatty acid profile analyses on leaf tissues from some high vegetative oil Arabidopsis events in collaboration with the Welti and Allen labs.</p><br /> <p> </p><br /> <p>The Allen lab also completed and published an acyl carrier protein (ACP) quantification method (Nam et al. 2020) which has been leveraged to analyze plant seeds and other tissues obtained in collaboration with the Koo, Thelen, and Cahoon groups. Results from these investigations are preliminary and work is ongoing. A further description of the methods was published in 2021 (Jenkins et al. 2021) and several projects are building off the method, though the pandemic has hindered some progress and skill transfer of methods within the lab.</p><br /> <p> </p><br /> <p>The Durrett and Allen labs have been collaborating to introduce genetic variation into soybeans targeting altered lipid levels by reducing the expression of lipases that are upregulated late in seed development. Work over the past several years focused on obtaining transformants and growing to homozygosity with phenotypic measurements of biomass components including lipids. Biomass from available mutants in selected gene call regions identified in a fast neutron population was assessed throughout development. These results have been communicated (Kambhampati et al. 2019). Identifying correlations between gene expression and changes in lipids, proteins and oligosaccharides is an avenue that can translate towards specific carbon partitioning outcomes in seeds. Recently, results with isotopic labeling experiments described the changes in metabolism over seed development (Kambhampati et al. 2021). Additional studies on central metabolism within a collaboration between the Allen and Clemente labs is nearing publication on the impact of enhanced malic enzyme levels in soybeans. This collaboration on carbon partitioning and flux will continue with crossing of these lines and others to improve soybean composition. Studies on soybeans will continue in the new cycle of the LIPIDS of Crops program, including further assessment of transgenics aimed at producing high value soybeans.</p><br /> <p> </p><br /> <p>The Durrett group has also investigated the role of the plastid lipase PLIP1 in supplying FA for the synthesis of TAG in developing Arabidopsis seeds. <em>PLIP1 </em>is upregulated in <em>dgat1 </em>seed. Double mutant <em>plip1 dgat1 </em>seeds are green and fail to germinate (Aulakh and Durrett, 2019). Ongoing work is testing the hypothesis that PLIP1 provides a PUFA-substrate pool necessary for the function of PDAT1.</p><br /> <p> </p><br /> <p>Research from Dhankher group uses comparative transcriptome and metabolome analysis seeking to uncover bottlenecks that limit seed and oil yields in transgenic <em>Camelina sativa </em>expressing the Arabidopsis diacylglycerol acyltransferase 1 (DGAT1) and yeast glycerol‑3‑phosphate dehydrogenase (GPD1). Their results show that TAG production is limited by (1) utilization of fixed carbon from the source tissues supported by the increase in glycolysis pathway metabolites and decreased transcript levels of transcription factors controlling fatty acids synthesis; (2) TAG accumulation is limited by the activity of lipases/hydrolases that hydrolyze TAG pool supported by the increase in free fatty acids and monoacylglycerols (Abdullah et al., 2018). Further characterization of genes, controlling these bottlenecks is in progress. Recently, Dhankher group has ectopically expressed PDCT1 and MGAT1 gene in camelina and the derived events show increased seed and oil yields, most likely due to the increased metabolic carbon flux towards TAG synthesis and accumulation in seeds. Flux analysis on these biologicals is ongoing in collaboration with Yair Shachar-Hill lab at MSU. </p><br /> <p>The Bates lab joined in 2019. The group’s work targets the analysis of lipid fluxes in <em>Physaria fendleri</em>, a burgeoning crop species that naturally accumulates unusual fatty acids with valuable industrial applications. Progress to date indicates that <em>P. fendleri</em> utilizes TAG remodeling to modify the seed oil fatty acid composition after initial synthesis (Bates & Bhandari, 2021). Ongoing efforts involves characterizing the enzymes involved in TAG remodeling and determining their value for engineering seed oil compositions, in addition the Bate’s team is in collaboration with Thelen, Allen, and Koo groups on investigating the control of fatty acids synthesis.</p><br /> <p><span style="text-decoration: underline;">Objective 3: Develop crops with improved yield and functionality</span></p><br /> <p>Acetyl-TAGs are unusual TAG molecules that possess reduced viscosity due to the presence of an sn- 3 acetate group. By expressing a high activity DAG acetyltransferase, the Durrett group developed camelina events that produced seeds accumulating over 90 mol% acetyl-TAG with minimal effect on seed size, weight and fatty acid content (Alkotami et al, 2021). To further lower the viscosity of seed oil, the Durrett group, in collaboration with the Cahoon group, generated camelina plants capable of synthesizing acetyl-TAG containing medium-chain fatty acids (MCFA). Field trials of the best transgenic events enabled viscosity testing of the acetyl-TAG derived from these lines (Bansal et al, 2018). In addition, the Cahoon and Durrett groups screened a camelina EMS mutant population and identified lines with altered seed fatty acid composition. Sequencing of candidate genes revealed causative mutations in homeologs of FAB1, FAD2, FAD3 and FAE1. Crossing to generate a fae1c/fad2a/fae1a/fad3a quadruple mutant resulted in a mid-oleic oils with nearly 40% oleic acid and reduced very long-chain (≤C20) fatty acid content. The resulting mid-oleic acid oil had improved oxidative stability due to reductions in polyunsaturated fatty acid content, increasing its utility for biofuels and other applications (Neumann et al, 2021).</p><br /> <p> </p><br /> <p>In Arabidopsis the Roston lab has identified important lipid changes cycle in response to cold and current efforts from multiple labs that appeared to be conflicting are now hypothesized to be in congruence based on recent findings from her group. Multiple plants have similar cycles in lipid abundance; cold tolerant species deviate from non-tolerant species at key times. The group also showed that lipid responses to freezing tolerance are greatly increased in ancient plant lineages, which led them to suggest that severe stress responses may be derived from a stronger ancestral response to damage, of any type.</p><br /> <p> </p><br /> <p>The Wurtele group continues to investigate the relationship between the Qua-Quine-Starch allele (QQS), and carbon and nitrogen partitioning, and along with defense, in a series of Arabidopsis mutants affecting starch metabolism. Results indicate QQS and the transcription factor, NF-YC4, can increase protein and improve defensive traits in crops.</p><br /> <p>As mentioned above Kosma is investigating methods for improving potato tuber storage life through better wound healing. The group is now well positioned to apply gene-editing approaches towards trait improvement. Kosma is working with collaborators, Dave Douches and Ray Hammerschmidt (MSU), to identify two QTLs potentially related to better wound suberin deposition.</p><br /> <p>The Dhankher group identified and overexpressed a bifunctional wax synthase/acyl- CoA:diacylglycerol acyltransferase (WSD1) gene, which plays a critical role in wax ester synthesis in Arabidopsis stem and leaf tissues. Gas chromatography and electron microscopy analyses of WSD1 transgenic Arabidopsis seedlings showed higher deposition of epicuticular wax crystals and increased leaf and stem wax loading in WSD1 transgenic events as compared to wildtype (WT) plants (Abdullah et al., 2021). Transgenic plants showed strong tolerance to drought and salinity. These results from Arabidopsis were successfully translated into Camelina and the resulting Camelina events also showed strong drought tolerance and produced better seed yield under water deficiency stress. The results clearly show that the manipulation of cuticular waxes will be advantageous for enhancing plant productivity in changing climates. Dhankher group also characterized a novel stress-associated protein 13 (SAP13) from Arabidopsis and the overexpression of SAP13 provided strong tolerance to drought and heavy metals stress (Dixit et al., 2018). They have now transferred the Arabidopsis SAP13 gene in <em>Camelina sativa </em>and <em>Brassica juncea </em>to increase productivity of oil seed crops under abiotic stress conditions. The derived transgenic <em>B. juncea</em> and camelina events are currently under investigation.</p><br /> <p> </p><br /> <p>The Clemente lab investigated soybean harboring a transgenic allele that expressed the barley homogentisate geranylgeranyl transferase (HGGT) that leads to the synthesis of very high level of tocotrienol in the seed oil (Konda et al., 2020). In collaboration with the Cahoon lab, they assembled multi-gene expression cassettes to generate soybean oil with omega-3, astaxanthin, and high vitamin E antioxidant content for use as a sustainable aquaculture feedstock. These events have advanced to field evaluation in Mead, NE in 2019 and 2020 and new events are being generated with up to 13-GoldenBraid assembled transgene cassettes per T-DNA element. These genetic designs target both higher seed oil content, in addition to aquaculture oil-quality output traits. This collaboration resulted in the generation of engineered soybean germplasm with 8- to 10-fold increases in total vitamin E tocochromanols, primarily as tocotrienols, by seed-specific expression of a barley homogentisate geranylgeranyl transferase (<em>HGGT</em>). This trait was introgressed into soybean events engineered for oils enriched in the polyunsaturated fatty acids gamma-linolenic, alpha linolenic, and stearidonic acids. Oil extracted from <em>HGGT</em>-expressing lines had ≥6-fold increase in free radical scavenging activity compared to controls. However, the oxidative stability index of oil from vitamin E-enhanced lines was ~15% lower than that of oil from non-engineered seeds and nearly the same or modestly increased in oil from the GLA, ALA and SDA backgrounds relative to controls. These findings show that soybean is an effective platform for producing high levels of free-radical scavenging vitamin E antioxidants, but this trait may have negative effects on oxidative stability of conventional oil or only modest improvement of the oxidative stability of PUFA-enhanced oil. Field trials were conducted in the summer of 2020 of engineered camelina events at the Eastern Nebraska Research and Extension Center in Mead, NE. Lines engineered for seed oils enriched in oleic acid, palmitic acid, myristic acid, lauric acid, decanoic acid, and astaxanthin were included in a ~1.9 acre planting. Seeds were harvested in July, 2020. Oils from lines with modified fatty acid composition will be used for development of new bio-based jet fuel conversion, and the astaxanthin-enriched oil will be used for development of methods for separation of carotenoids from vegetable oils. Clemente reported at the 2021 meeting that EPA and DHA production in seeds of engineered soybean events is approaching commercial relevance, and the next step is to conduct aquaculture feeding trials with extracted oil from these seeds. These events are currently being bulked by field production at ENREC in Mead, NE. Research is also ongoing to modify the protein meal quantity and quality for applications such as meat substitutes.</p><br /> <p> </p><br /> <p>The Clemente and Cahoon labs have been building a cataloged repository of genetic elements (e.g., promoters, 3’UTRs) that have been domesticated for use in GoldenBraid modular gene assembly and are available for the Multistate group to facilitate metabolic engineering and crop improvement efforts. Their collaboration has also been extended to the engineering of vegetative oil production in sorghum stems. GoldenBraid assembly of modular gene-expression elements has been used to develop multiple vectors that target enhanced vegetative oil production and the production of oils with higher value fatty acid structures. These vectors have been used for generation of engineered sorghum lines, many of which are now being subjected to the design-build-test-learn cycle to obtain iterative improvements in the targeted oil traits. Utilizing the <em>Agrobacterium</em>-mediated sorghum transformation platform developed by the research team (Guo et al., 2015) they continue to pursue genetic approaches to added valuable co-products to the biomass to complement the inherent attributes to further improve this C4 feedstock contribution to the bioeconomy through targeting both input traits (Scully et al., 2018) and output traits (Kempinski <em>et al., </em>2019). The Clemente and Cahoon labs reported at the 2021 meeting the generation of sorghum lines with >2% triacylglycerol (TAG) in leaves and stems in greenhouse-grown lines. TAG levels of up to 5% in leaves have been observed during ongoing field studies in 2021. Studies are planned to cross TAG-producing lines in grain sorghum backgrounds into sweet sorghum to test the influence of sucrose levels on TAG accumulation.</p>Publications
<p>Abdullah HM, Rodriguez J, Salacup JM, Castañeda IS, Schnell DJ, Pareek A, and Dhankher OP. (2021). Increased Cuticle Waxes by Overexpression of Wax Synthase, WSD1, Improves Tolerance Towards Osmotic Stresses in Plants. Int. J. Molecular Sciences 22(10): 5173.https://doi.org/10.3390/ijms22105173</p><br /> <p> </p><br /> <p>Alexander, L.E., Okazaki, Y., Schelling, M.A., Davis, A., Zheng, X., Rizhsky, L., et al 2020. Maize glossy2 and glossy2-like genes have overlapping and distinct functions in cuticular lipid deposition. Plant Physiol. 183:840-853.</p><br /> <p>Alkotami L, Kornacki C, Campbell S, McIntosh G, Wilson C, Tran TNT, Durrett TP. (2021) Expression of a high activity diacylglycerol acetyltransferase results in enhanced synthesis of acetyl-TAG in camelina seed oil. <em>Plant J</em>. 106: 953-964.</p><br /> <p>Bates PD (2021) The plant lipid metabolic network for assembly of diverse triacylglycerol molecular species. In Advances in Botanical Research. Academic Press (in press)</p><br /> <p>Bhandari S, Bates PD (2021) Triacylglycerol remodeling in <em>Physaria fendleri</em> indicates oil accumulation is dynamic and not a metabolic endpoint. Plant Physiology <a href="https://doi.org/10.1093/plphys/kiab294">https://doi.org/10.1093/plphys/kiab294</a></p><br /> <p>Brands M, Cahoon EB, Dörmann P (2020) Palmitvaccenic acid (Δ11-cis-hexadecenoic acid) is synthesized by an OLE1-like desaturase in the arbuscular mycorrhiza fungus <em>Rhizophagus irregularis</em>. Biochemistry 59: 1163-1172</p><br /> <p>Busta L, Schmitz EA, Kosma DK, Schnable JC, Cahoon EB (2021) A co-opted steroid synthesis gene, maintained in sorghum but not maize, is associated with a divergence in leaf wax chemistry. <em>Proceedings of the National Academy of Sciences</em>, USA 118 e2022982118</p><br /> <p>Cahoon EB, Li-Beisson Y (2020) Plant unusual fatty acids: learning from the less common. Curr Opin Plant Biol 55: 66-73.</p><br /> <p>Cahoon RE, Gonzalez-Solis A, Markham JE, Cahoon EB (2021) Mass spectrometry-based profiling of plant sphingolipids from typical and aberrant metabolism. <em>In</em> D Bartels, P Dormann, eds, Methods in Plant Lipids Springer</p><br /> <p>Cai G, Kim SC, Li J, Zhou Y, Wang X. Transcriptional Regulation of Lipid Catabolism during Seedling Establishment. Mol Plant. 2020 Jul 6;13(7):984-1000. doi: 10.1016/j.molp.2020.04.007. Epub 2020 Apr 22. PubMed PMID: 32334070.</p><br /> <p>Cai G, Fan C, Liu S, Yang Q, Liu D, Wu J, Li J, Zhou Y, Guo L, Wang X. Nonspecific phospholipase C6 increases seed oil production in oilseed Brassicaceae plants. New Phytol. 2020 May;226(4):1055- 1073. doi: 10.1111/nph.16473. Epub 2020 Mar 16. PubMed PMID: 32176333.</p><br /> <p>Chen K, Alexander LE, Mahgoub U, Okazaki Y, Higashi Y, Takano K, Loneman D, Dennison TS, Lopez M, Claussen R, Peddicord L, Saito K, Lauter N, Dorman KS, Nikolau BJ, Yandeau-Nelson MD (2021) Dynamic product-precursor relationships underlie cuticular lipid accumulation on maize silks, bioRxiv, 2021.03.31.437946.</p><br /> <p>Chopra R, Johnson EB, Emenecker R, Cahoon EB, Lyons J, Kliebenstein DJ, Daniels E, Dorn KM, Esfahanian M, Folstad N, Frels K, McGinn M, Ott M, Gallaher C, Altendorf K, Berroyer A, Ismail B, Anderson JA, Wyse DL, Ulmasov T, Sedbrook JC, Marks MD (2020) Identification and stacking of crucial traits required for the domestication of pennycress. Nature Food 1: 84-91</p><br /> <p>Davis JA, Pares RB, Bernstein T, McDowell SC, Brown E, Stubrich J, Rosenberg A, Cahoon EB, Cahoon RE, Poulsen LR (2020) The lipid flippases ALA4 and ALA5 play critical roles in cell expansion and plant growth. Plant Physiology 182: 2111-2125</p><br /> <p>Du ZY, Hoffmann-Benning S, Wang S, Yin L, Zienkiewicz K, Zienkiewicz A, (2021) "Lipid metabolism in development and environmental stress tolerance for engineering agronomic traits" Frontiers in Plant Science 12: 739786 <a href="https://doi.org/10.3389/fpls.2021.739786">https://doi.org/10.3389/fpls.2021.739786</a></p><br /> <p>Fernández-Milmanda, G. L., Crocco, C. D., Reichelt, M., Mazza, C. A., Köllner, T. G., Zhang, T., Cargnel, M.D., Lichy, M.Z., Fiorucci, A.-S., Fankhauser, C., Koo, A.J., Austin, A.T., Gershenzon, J., Ballaré, C.L. 2020. A light-dependent molecular link between competition cues and defense responses in plants. Nat. Plants 6:223-230.</p><br /> <p>Gonzalez-Solis A, Han G, Gan L, Li Y, Markham JE, Cahoon RE, Dunn TM, Cahoon EB (2020) Unregulated Sphingolipid Biosynthesis in Gene-Edited Arabidopsis <em>ORM </em>Mutants Results in Nonviable Seeds with Strongly Reduced Oil Content. Plant Cell 32: 2474-2490.</p><br /> <p>Hagely, K., Konda, A. R., Kim, J. H., Cahoon, E. B., & Bilyeu, K. (2021). Molecular-assisted breeding for soybean with high oleic/low linolenic acid and elevated vitamin E in the seed oil. Molecular Breeding, 41(1), 1-13</p><br /> <p>Hoffmann-Benning, S (2020). Beyond membranes: The evolution of plant lipid signaling. <em>Molecular Plant; </em>13, 952-954. doi: 10.1016/j.molp.2020.06.004</p><br /> <p>Hoffmann-Benning S (2021) Collection and analysis of phloem lipids. Plant Lipids: Methods and Protocols, Methods in Molecular Biology, vol. 2295, Chapter 19, p.351-361. D. Bartels and P. Dörmann (eds.), Springer Science+Business Media, LLC, part of Springer Nature 2021. <a href="https://doi.org/10.1007/978-1-0716-1362-7_19">https://doi.org/10.1007/978-1-0716-1362-7_19</a>.</p><br /> <p>Jenkins LM, Nam JW, Evans BS, Allen DK (2021) Quantification of Acyl-Acyl Carrier Proteins for Fatty Acid Synthesis using LC-MS/MS, in Plant Lipids: Methods and Protocols, Methods in Molecular Biology, Eds. Dorothea Bartels & Peter Dormann, vol. 2295, doi.org/10.1007/978-1-0716-1362-7_13.</p><br /> <p>Kambhampati S, Aznar-Moreno JA, Bailey SR, Arp JA, Chu KL, Bilyeu KD, Durrett TP, Allen DK (2021). Temporal changes in metabolism late in seed development affect biomass composition. Plant Physiology 186: 874-890</p><br /> <p>Kambhampati, S., Aznar-Moreno, J.A., Hostetler, C., Caso, T., Bailey, S.R., Hubbard, A.H., Durrett, T.P., Allen. D.K. 2020. On the inverse correlation of protein and oil: Examining the effects of altered central carbon metabolism on seed composition using soybean fast neutron mutants. Metabolites 10(1): 18; doi:10.3390/metabo10010018.</p><br /> <p>Kimberlin A, Holtsclaw RE, Koo AJ. (2021) Differential regulation of the ribosomal association of mRNA transcripts in an Arabidopsis mutant defective in jasmonate-dependent wound response. Front. Plant Sci. 12:637959.</p><br /> <p>Koenig A, Benning C, Hoffmann-Benning, S. 2020. Lipid trafficking and signaling in plants. Invited review for “Lipid Signaling and Metabolism” (Elsevier), James Ntambi ed.; Chapter 2, p. 23-44</p><br /> <p>Koenig AM, Hoffmann-Benning, S (2020) The interplay of phloem-mobile signals in plant development and stress response. Bioscience Reports 40: BSR20193329 <a href="https://doi.org/10.1042/BSR20193329">https://doi.org/10.1042/BSR20193329</a><a href="https://doi.org/10.1042/BSR20193329">.</a></p><br /> <p>Lichy, M.D., M. Z., Fiorucci, A. S., Fankhauser, C., Koo, A. J., Austin, A. T., Gershenzon, J., and Ballaré, C. L. (2020) A light-dependent molecular link between competition cues and defense responses in plants. <em>Nat Plants </em>6, 223-230</p><br /> <p>Mahboub S, Shomo ZD, Regester RM, Albusharif M, Roston RL (2021) Three Methods to Extract Membrane Glycerolipids: Comparing Sensitivity to Lipase Degradation and Yield. <em>Methods Molecular Biology</em> 2295: 15-27 doi: 10.1007/978-1-0716-1362-7_2</p><br /> <p>Moisseyev G, Park K, Cui A, Freitas D, Rajagopal D, Konda AR, Martin-Olenski M, Mcham M, Liu K, Du Q, Schnable JC, Moriyama EN, Cahoon EB, Zhang C (2020) RGPDB: database of root-associated genes and promoters in maize, soybean, and sorghum. Database 2020</p><br /> <p>Msanne J, Kim H, Cahoon EB (2020) Biotechnology tools and applications for development of oilseed crops with healthy vegetable oils. <em>Biochimie</em> 178: 4-14.</p><br /> <p>Nam, J.W., Jenkins, L.M, Li, J., Evans, B.S., Jaworski, J.G., Allen, D.K. 2020. A General Method for Quantification and Discovery of Acyl Groups Attached to Acyl Carrier Proteins in Fatty Acid Metabolism using LC-MS/MS. Plant Cell 32:820-832.</p><br /> <p>Narayanan S, Zoong Lwe ZS., Gandhi N, Welti R, Fallen B, Smith JR, Rustgi S. (2020) Comparative lipidomic analysis reveals heat stress responses of two soybean genotypes differing in temperature sensitivity. Plants 9: 457.</p><br /> <p>Neumann NG, Nazarenus TJ, Aznar-Moreno JA, Rodriguez-Aponte SA, Veintidos VAM, Comai L, Durrett TP, Cahoon EB (2021) Generation of camelina mid-oleic acid seed oil by identification and stacking of fatty acid biosynthetic mutants. <em>Industrial Crops and Products</em> 159: 113074.</p><br /> <p>O'Neill K, Lee Y, 2020, Visualizing Genotypic and Developmental Differences of Free Amino Acids in Maize Roots with Mass Spectrometry Imaging, Frontiers in Plant Science, 639. DOI:10.3389/fpls.2020.00639.</p><br /> <p>Ortiz R, Geleta M, Gustafsson C, Lager I, Hofvander P, Lofstedt C, Cahoon EB, Minina E, Bozhkov P, Stymne S (2020) Oil crops for the future. Curr Opin Plant Biol 56: 181-189</p><br /> <p>Park, K., Sanjaya, S. A., Quach, T., & Cahoon, E. B. (2021). Toward sustainable production of value-added bioenergy and industrial oils in oilseed and biomass feedstocks. <em>GCB Bioenergy</em>, 00, 1– 14. <a href="https://doi.org/10.1111/gcbb.12883">https://doi.org/10.1111/gcbb.12883</a></p><br /> <p>Romsdahl TB, Kambhampati S, Koley S, Yadav UP, Alonso AP, Allen DK, Chapman KD (2021) Analyzing Mass Spectrometry Imaging Data of 13C-labeled Phospholipids in Camelina sativa and Thlaspi arvense (Pennycress) Embryos. 11 148. doi.org/10.3390/metabo11030148</p><br /> <p>Segura, R. R., Quach, T., Gomes‑Neto, J. C., Xian, Y., Pena, P. A., Weier, S., Pellizzon, M. A., Kittana, H., Cody, L. A., Geis, A. L., Heck, K., Schmaltz, R. J., Bindels, L. B., Cahoon, E. B., Benson, A. K., Clemente, T. E., Ramer‑Tait, A. E., Stearidonic‑enriched soybean oil modulates obesity, glucose metabolism, and fatty acid profiles independently of <em>Akkermansia muciniphila</em>. <em>Mol. Nutr. Food Res. </em>2020, 64, 2000162.</p><br /> <p>Shirani A, Joy T, Lager I, Yilmaz JL, Wang H-L, Jeppson S, Cahoon EB, Chapman K, Stymne S, Berman D (2020) Lubrication characteristics of wax esters from oils produced by a genetically- enhanced oilseed crop. Tribology International 146: 106234</p><br /> <p>Shiva S, Samarakoon T, Lowe KA, Roach C, Vu HS, Colter M, Porras H, Hwang C, Roth MR, Tamura P, Li M, Schrick K, Shah J, Wang X, Wang H, Welti R (2020) Leaf lipid alterations in response to heat stress of <em>Arabidopsis. </em>Plants 9: 845.</p><br /> <p>Song Y, Vu HS, Shiva S, Fruehan C, Roth MR, Tamura P, and Welti R. (2020) A lipidomic approach to identify cold-induced changes in Arabidopsis membrane lipid composition. Editors: D. Hincha and E. Zuther. Methods Mol Biol. 2156: 187-202.</p><br /> <p>Tamura P, Fruehan C, Johnson DA, Hinkes P, Williams TD, Welti R. (2021) Fatty acid composition by total-acyl-lipid--collision induced dissociation--time-of-flight (TAL-CID-TOF) mass spectrometry. Editors: D. Bartels and P. Doermann. Methods Mol Biol. 2195, 117-133.</p><br /> <p>Velazhahan V, Glaza P, Herrera AI, Prakash O, Zolkiewski M, Geisbrecht BV, Schrick K (2020). Dietary flavonoid fisetin binds human SUMO1 and blocks sumoylation of p53. <em>PLOS One </em>15:e0234468.</p><br /> <p>Viswanathan MB, Park K, Cheng M-H, Cahoon EB, Dweikat I, Clemente T, Singh V (2020) Variability in structural carbohydrates, lipid composition, and cellulosic sugar production from industrial hemp varieties. Industrial Crops and Products 157: 112906</p><br /> <p>Wang P, Chai YN, Roston R, Dayan FE, Schachtman DP. (2021) The Sorghum bicolor root exudate sorgoleone shapes bacterial communities and delays network formation. mSystems. 6(2):e00749-20. doi: 10.1128/mSystems.00749-20.</p><br /> <p>Ye Y, Fulcher YG, Sliman DJ 2nd, Day MT, Schroeder MJ, Koppisetti RK, Bates PD, Thelen JJ, Van Doren SR. (2020) The BADC and BCCP subunits of chloroplast acetyl-CoA carboxylase sense the pH changes of the light-dark cycle<em>. J Biol Chem. </em>295:9901-9916.</p><br /> <p>Ye Y, Nikovics K, To A, Lepiniec L, Fedosejevs ET, Van Doren SR, Baud S, Thelen JJ (2020) Docking of acetyl-CoA carboxylase to the plastid envelope membrane attenuates fatty acid production in plants. <em>Nature Communications. </em>11:6191.</p><br /> <p>Zoong Lwe, Z.S., Welti, R., Anco, D., Naveed, D., Rustgi, S., Narayanan, S. 2021. Heat stress elicits remodeling in the anther lipidome of peanut. Sci Rep. 10, 22163. doi: 10.1038/s41598-020-78695-3.</p><br /> <p>Zoong Lwe Z., Sah S., Persaud L., Li J., Gao W., Reddy K.R., Narayanan S. 2021. Alterations in the leaf lipidome of <em>Brassica carinata</em> under high temperature stress. BMC Plant Biol. In Press.</p><br /> <p> </p>Impact Statements
- Impact Statements. The LIPIDS of Crops Multi-state research project has an overarching goal to increase the value of crop oilseeds by increasing seed oil content, making unusual and economically important fatty acids, finding new markets for existing or future vegetable oils and oilseed crops (e.g. camelina), and also adding value to the defatted meal particularly for niche crops like camelina. Each of these goals has the potential to impact the economy and move towards renewable energy independence. The NC- 1203 group has interacted collaboratively to achieve project milestones as indicated by “milestones”, 73 publications, 4 software tools, and 7 patents listed below and standards and protocols that have been shared amongst participants.