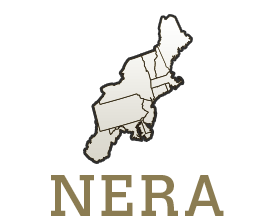
NE1027: Ovarian Influences on Embryonic Survival in Ruminants
(Multistate Research Project)
Status: Inactive/Terminating
NE1027: Ovarian Influences on Embryonic Survival in Ruminants
Duration: 10/01/2007 to 09/30/2012
Administrative Advisor(s):
NIFA Reps:
Non-Technical Summary
Statement of Issues and Justification
The need as indicated by stakeholders. Impaired reproductive performance is a major cause of reduced productivity for ruminants and of reduced profitability for dairy and meat animal producers. The focus of the NE-1007 Regional Project (and its predecessor, the NE-161 Regional Project) has been to address nutritional, management, and environmental factors that impact ovarian activity and subsequent pregnancy rates in domestic ruminants. Our current goal is to continue this important investigative work, focusing on ovarian and embryonic aspects of infertility, and to seek renewal of this multistate effort. The objectives of this proposal are consistent with the first strategic goal identified in the CSREES Strategic Plan (2004-2009), which is to "Enhance economic opportunities for agricultural producers". Under objective 1.5 [Contribute science-based information, analysis, and education to promote the efficiency of agricultural production systems], we will evaluate our progress using performance criteria 1.5.7 [Increase and improve the reproductive performance of animals; CSREES problem area 301], 1.5.8 [Enhance the efficiency of nutrient utilization for animal productivity; CSREES problem area 302], and 1.5.11 [Improve understanding of fundamental animal physiological processes; CSREES problem area 305]. Moreover, at a recent workshop, a group of more than 75 stakeholder scientists from federal, public, and private institutions across the United States recommended the following high impact areas as funding priorities for USDA/CSREES: "development of the oocyte, follicle recruitment and development, identifying genes involved in gamete quality and embryo development, uterine-conceptus interactions with emphasis on embryonic and fetal survival, reproductive immunology, and gonadal development" (Mirando and Hamernik, 2006). Our stakeholders include animal producers, the scientific community, and citizens of the region and the nation. Importance of work and consequences if it is not done. Improving fertility in ruminants requires fundamental knowledge about the influence of oocyte quality, follicular development, corpus luteum function, and uterine environment on embryonic survival. The determination of ovarian and embryonic attributes of fertility in ruminants is critical to identify the underlying causes of anovulation, fertilization failure, luteal insufficiency, and early embryonic loss. Further experimental manipulation of these identified attributes will lead to a prioritization of future studies and management strategies. By enhancing basic knowledge of the underlying biology surrounding ovarian function and embryonic survival, new strategies can be developed for application by producers and veterinarians. Application of management strategies that are not based upon drug-development or use of new drugs is economical, user and consumer friendly, and preserves food quality and safety. The technical feasibility of the work. NE-1007 has historically been one of the most productive, cohesive, and diverse multistate research groups nationwide. Technical members of NE-1007 (molecular biologists, cell physiologists, and animal scientists) have demonstrated broad expertise in reproductive physiology of domestic ruminants, using a multistate, collaborative approach to accomplish project objectives. In the four previous years of this project, the eight technical members of NE-1007 have published over 65 refereed research papers, abstracts, theses, book chapters, and technical/extension publications. Accomplishments include: developed granulosal/luteal cell models to measure the effects of heat stress on steroidogenesis in vitro; provided evidence that retention of pregnancy in ruminants is associated with both maternal age and concentration of progesterone (P4) during placentation; devised an approach to measure genetic and physiologic changes in single oocytes and steroidogenic cells; demonstrated that moderately larger, not small preovulatory follicles and greater plasma estradiol concentrations on the day of AI increased the likelihood of subsequent pregnancy at day 29 in cattle; identified molecular and cellular mechanisms that influenced luteal function and resulted in embryonic/fetal mortality; formulated a method to assess allantoic development ultrasonographically to predict pregnancy outcome; and determined that nutritional and metabolic status during late pregnancy plays a pivotal role in the outcome of the first postpartum follicular wave. The advantages for doing the work as a multistate effort. Advantages of performing this work as a multistate effort include overlapping approaches with collaborative efforts and technologies that can be directed toward several objectives simultaneously, and shared experience and data analyses that make interactions more beneficial. For example, members at different stations have contributed to unified animal protocols and exchange samples to take advantage of unique validated procedures and will continue to do so in this project. Moreover, the combination of basic biological research and innovative applied research more effectively supports outreach programs and engagement, the goal of which is to improve reproductive performance in livestock more rapidly. Likely impacts from successfully completing the work. Fulfilling the objectives of this project will provide important new information concerning declining fertility among ruminants in the face of continuous improvement in production capability. Impacts include improved understanding of how oocyte quality, follicular/luteal function, and metabolic demands of production may alter key hormonal or cellular signals necessary for oocyte competence, fertilization, and embryo survival in early pregnancy. Preventive or therapeutic approaches to treat infertility and embryonic loss will benefit consultants (Nutritionists, Veterinarians, Educators, etc) and most importantly animal producers. Improved animal reproductive performance benefits the on-farm economy and sustains an agricultural production system that is highly competitive in the global economy.
Related, Current and Previous Work
ACCCOMPLISHMENTS UNDER PREVIOUS OBJECTIVES: Objective 1. Examine events surrounding follicular development and the follicular to luteal transition that may affect embryonic/fetal mortality.
In cattle, larger pre-ovulatory diameter resulted in an increased likelihood of pregnancy to AI. Similarly, greater plasma estradiol concentrations on the day of AI significantly increased the likelihood of subsequent pregnancy at day 29. As expected, larger pre-ovulatory follicles were associated with greater pre-ovulatory estradiol production. Examination of differential gene expression between growing and persistent follicles in cows indicated that RNA can be isolated from single oocytes to analyze genes expressed at low levels, and that expression and quantity of RNA in these oocytes differed among candidate genes, including Poly (A) Ribonuclease (PARN; RNA degradation) and MSY2 (RNA masking). In granulosal cells of persistent follicles, microarray analysis revealed down regulation of many genes involved in protein metabolism, amino acid transport, and mitosis, but an up-regulation of apoptotic genes. These results indicate that granulosal cells of persistent follicles have less capacity to regulate mitosis and provide amino acids to oocytes. In ewes, relatively low dosages of P4 for estrous synchronization increased ovulation rates, but did not result in a corresponding increase in the number of lambs born per ewe. Treatment of dairy cows with a single injection of recombinant bovine growth hormone (rbST) at the time of insemination increases conception, especially in cows greater than 100 days in lactation. Objective 2. Examine mechanisms involved in insufficient or altered luteal function that may result in embryonic/fetal mortality.
In vivo studies indicated a physiological relationship between PGF2-alpha, ET-1, and nitric oxide during luteolytsis in the ewe. Multiple ET-1 injections induced luteolysis and increased uterine PGFM secretion in mid-cycle ewes; co-administration of PG or NO synthase inhibitors partially mitigated these effects. Immune and endothelial cells of the CL interact cooperatively to increase MCP-1 expression and immune cell recruitment during luteolysis. Collaborative efforts by NE-1007 representatives showed that TIMP-3 may participate in the development and regression of the CL, PGF2-alpha differentially affected MMP2 and MMP-9 in day 4 and day 10 CL, and that MMP-19 was differentially expressed over the bovine estrous cycle, with highest expression in the day 4 CL. Studies of cytokeratin-8/18 filament expression and Fas ligand-induced apoptosis indicated that cytokeratin filaments may influence cytokine-induced cell death and caspase activation during regression of the CL. Prostaglandin transporters expressed in luteal tissue may be related to acquisition of luteolytic capacity. Dendritic cells are present in the CL and may be involved in extensive tissue remodeling during luteinization and luteolysis. Indolamine 2,3 dioxygenase, as induced by interferon-gamma, is a component of the intracellular signaling cascade that results in apoptosis of luteal cells. Subclasses of endothelial cells from the bovine CL express class II MHC molecules. Osteopontin is produced within the CL and its expression pattern is consistent with a proinflammatory role during luteolysis. A method to evaluate development of the allantois ultrasonographically and to examine effects of that development on late embryonic and early fetal loss and outcome of pregnancy has been developed. A model with which to study the effects of follicular age on late embryonic loss, particularly losses of pregnancy, has been developed and used in ewes. Objective 3. Determine environmental and metabolic effects on oocyte maturation, fertilization and embryonic/fetal survival.
Collaborative in vitro studies among NE-1007 participants indicate heat treatment and PGF2-alpha induced the expression of HSP 70 and 90 in the bovine CL in a time-dependent manner, and heat shock treatment for 24 hrs upregulated HSP 70, without influencing MMP-2 or MMP-9 activity. Moreover, the effects of elevated temperature in vitro on steroid production by granulosal and thecal cells (decreased secretion of estradiol and androstenedione, and increased secretion of P4) are mediated at least in part through decreases and increases in mRNA for aromatase and 17alpha hydroxylase, and for proteins involved in P4 production (StAR, P450scc, and 3beta-HSD), respectively. Season did not affect pre-ovulatory follicular diameter in cows that become pregnant, or that become pregnant and later lose the conceptus. Progesterone concentrations were lower from day 7 onward in cows losing the conceptus during the cold season, but only from day 30 onward during the warm season. Nutritional and metabolic status during late pregnancy plays a pivotal role in the outcome of the first postpartum follicular wave. Lamb removal in combination with high dosages of P4 may improve estrus, ovulation and fertility in response to ram introduction in lactating anestrous ewes. BACKGROUND OF CURRENT OBJECTIVES: GENETIC, MORPHOLOGICAL AND PHYSIOLOGICAL ATTRIBUTES OF THE FOLLICLE/OOCYTE AND CORPUS LUTEUM
Oocyte transcripts in growing and persistent follicles: Pregnancy wastage in dairy cows has increased from 40% at first service in the 1950's (Inskeep et al., 1961) to 57% in the late 1990's (Nebel, 1999). Fertilization rate has not decreased (85% to 95%) but both early and late embryonic losses have increased significantly. A high incidence of early embryonic loss is associated with prolonged dominance of follicles, either due to protocols for synchronization of estrus or reduced function of the corpus luteum. Pregnancy rates in animals that ovulated a persistent follicle were 23.6% in cows (Cooperative Regional Research Project, NE-161, 1996) and 37% in heifers (Savio et al., 1993b). Data from our laboratory demonstrated that 86% of the embryos produced from fertilized oocytes from persistent follicles had progressed only to either the 2- to 8- cell stage (43 %) or the 9- to 15- cell stage (43 %) by day 6 (Ahmad et al., 1995).
Maternal transcripts that accumulate in the oocyte during oogenesis play important roles during initial stages of embryonic development before activation of the embryonic genome, which occurs at the 8- to 16- cell stage in cattle (Telford et al., 1990; Memili and First, 2000). Targeted disruption of maternal effect genes, such as MATER and Zar1, results in the arrest of early embryonic development (Tong et al., 2000a; Wu et al., 2003). It is likely that alterations of maternal transcripts in oocytes, as in the case of persistent follicles, may result in altered development and eventual loss of the embryos. As an initial attempt towards characterizing the changes of mRNA transcripts in oocytes from persistent follicles, we determined the mRNA levels of a number of candidate genes in oocytes from growing and persistent follicles by quantitative real time PCR analysis (Lingenfelter et al., 2005). While maternal effect genes (e.g. MATER and Zar1) did not appear to be affected, the expression of several other genes known to be important for transcription (e.g. YY1), mRNA masking (e.g. MSY2) and deadenylation (e.g. PARN) was decreased significantly in oocytes derived from persistent follicles. Although the approach yielded valuable information about alterations of gene expression in oocytes from persistent follicles, the number of genes that can be analyzed with this technique is limited.
The development of microarray technologies has permitted the analysis of thousands of genes simultaneously. Microarray resources specifically for livestock species are becoming publicly available. In particular, the development of a large bovine cDNA microarray containing 18,263 unique genes (Suchyta et al., 2003) by Michigan State University, has provided a valuable resource for functional genomics studies in cattle. Using this high density bovine cDNA array, we propose to characterize the global changes of gene expression in oocytes and early embryos derived from persistent follicles in cattle. Effects of intrafollicular estrogen levels on growing and persistent follicles: Persistent dominant follicles exhibit an altered endocrine profile that is characterized by increased circulating 17b-estradiol (E2) concentrations and increased LH pulse frequency (Inskeep, 2004). This altered endocrine profile is correlated to increased risk for early embryonic death and impaired fertility. However, the underlying molecular mechanisms of reduced developmental capacity of the oocyte within the persistent follicle have not been clearly defined. During normal follicular development, E2 regulates gonadotropin receptor expression, gap junction formation, steroid synthesis, and granulosal cell apoptosis (Rosenfeld et al., 2001). Thus, excess E2 in the follicular microenvironment may impact granulosal cell function and thereby indirectly affect oocyte growth and/or maturation. Excess E2 concentrations or altered E2 signaling is associated with duplication of centrosomes and chromosomal instability in breast cancer cells (Li et al., 2004). Recent studies indicate that centrosomes serve as multiplatform scaffolds which regulate cell cycle progression (Doxsey et al. 2005). Previous studies demonstrate that oocytes from women with Polycystic Ovary Syndrome, which is characterized by high sex steroid concentrations and persistent follicles, have increased expression of centrosome-associated genes (Wood et al., 2006). These studies suggest promoter regions of a subset of these genes contain putative nuclear receptor binding sites providing a plausible mechanism for a direct effect of E2 on oocyte maturation and early embryonic development. Oocyte quality: In recent years, fertility of high producing dairy cows, as evaluated by conception rate to insemination (AI), has steadily declined (Butler, 2003). While the causes of this decline are likely to be multi-factorial, whether or not oocyte quality is affected by the stress imposed by high production has not been evaluated rigorously. Here we propose to ascertain whether structural aspects of oocytes, such as microtubule and actin microfilament distribution, spindle formation, endoplasmic reticulum (ER) organization and inositol 1,4,5-trisphosphase receptor (IP3R-1) distribution are altered in these animals. Given that these oocyte structural elements are responsible for the organization of the metaphase II spindle and chromosome segregation as well as for the ability of oocytes to mount Ca2+ responses during fertilization, we would expect that any obvious alteration in any these variables will lead to the generation of defective zygotes. Besides structural differences, functional defects of oocyte proteins involved in the regulation of oocyte maturation and/or in the exit from MII arrest may be responsible, at least in part, for the low oocyte quality in these high producing cows. Relationship between preovulatory follicular diameter and corpus luteum dimensions and progesterone concentrations: Previous studies in beef cows (Perry et al., 2005) and dairy cows (Lopes et al., 2007) found that pregnancy rate was increased with ovulation of moderately larger versus smaller follicles. Follicular diameter on the day of AI (day 0) in dairy cows diagnosed pregnant or non-pregnant averaged 15.8 ± 0.3 mm vs. 14.5 ± 0.04 mm, respectively. In the same study, plasma estradiol was greater on the day of AI and plasma P4 concentrations became greater after day 5 in pregnant than in non-pregnant cows. Clearly, size of the CL and greater plasma P4 concentrations are correlated positively with ovulatory follicular diameter (Sartori et al., 2002a). However, high feed intake and associated increased liver blood flow in dairy cows results in more rapid metabolic clearance rate of P4 (Sangsritavong et al., 2002; Vasconcelos et al., 2003). Thus, embryo survival reflects both capacity for production and rate of clearance of P4. Activation of primordial follicles: Most of the follicles in bovine and other mammalian ovaries are at the resting, primordial stage. Utilization of this "follicular reserve" for new reproductive technologies requires development of techniques to activate primordial follicles in vitro and to promote their further growth to the stage of oocyte competence for IVM and IVF. Previously we developed an experimental model for culturing pieces of bovine and baboon ovarian cortex, obtained from late gestation fetuses. In serum-free culture with the medium supplement ITS+, almost all primordial follicles activate, but very few secondary follicles develop. More recently, we have discovered that the insulin component of ITS+ is responsible for the wholesale activation that occurs and that, in the absence of the insulin component of ITS+, most follicles remain healthy for 10 days in vitro, but activation does not occur. This new experimental model provides an exciting opportunity to test other potential stimulators of follicular activation, activators believed to be part of the normal pathway of activation and that hence, may lead to development of more secondary follicles in vitro. In cultures of newborn rat ovaries, KL ligand (KL; aka Kitlg), leukemia inhibitory factor (LIF), basic fibroblast growth factor (bFGF; aka FGF2), keratinocyte growth factor (KGF), and platelet-derived growth factor (PDGF) stimulate the primordial to primary transition; LIF, KGF, and PDGF increase mRNA for KL and thus, may exert their effects on activation through KL. Microvascular endothelial cells of the corpus luteum: The corpus luteum (CL) consists primarily of four distinct cell types: large and small steroidogenic cells, endothelial cells, and fibroblasts. Steroidogenic cells make up approximately 30% of the tissue, while the most abundant cell type of the CL is the endothelial cell (EC) population, comprising approximately 50% of the mid-cycle tissue. The vasculature is essential for the formation, maintenance, and regression of the CL. There are four distinct types of microvascular EC within the bovine CL that are morphologically and physiologically unique, and are thought to contribute to the formation, function, and regression of the microvasculature. Important distinctions based primarily upon morphologic and physiologic criteria reveal that further study is required to determine the significance of these cell types during different phases of CL function, especially with respect to angiogenesis, angiostasis and angioregression. Nitric oxide in luteolysis and peroxisome proliferators-activated receptors on luteal steroidogenesis: PGF2a increases endogenous levels of NO and ET-1 in cows and ewes. The luteolytic effects of PGF2a are reduced by ET-1 receptor and NO synthase inhibitors.
Peroxisome proliferator-activated receptors (PPARs) activation has been shown to modify steroid production in murine and bovine follicles. The effects of PPARs on luteal function have not been reported. Signal transduction mechanisms associated with luteal sensitivity to prostaglandin F2alpha: An in vitro system for examining developmental sensitivity of the bovine CL to PGF2a was developed (Choudhary et al., 2005) in which endothelial cells are removed, leaving an enriched steroidogenic cell population. Using this model, the potency of PGF2a in Day-4 steroidogenic-enriched cells was lower than in Day-10 cells in regard to the inhibitory actions of PGF2a on basal and LH-stimulated P4 accumulation and PGF2a-induced increases [Ca2+]i.
The array of PKC isozymes expressed in whole bovine CL includes a, bI, bII, e and m, and the amount of PKCe expressed in the day-10 CL is greater than in the day-4 CL (Sen et al., 2004). It is proposed that differential expression of PKCe as a function of development could play a role in PGF2a -induced luteal regression (Sen et al., 2004: Sen et al., 2006).
Preliminary observations indicate that: 1) luteal steroidogenic cells are the source of PKCe, meeting the requirement that both PGF2a-receptors and PKCe are expressed in the same luteal cell type; 2) PKCe, an isozyme expressed in CL with acquired luteolytic response to PGF2a, has a regulatory role in the PGF2a-induced Ca2+ signaling in luteal steroidogenic cells (Sen et al., 2005); and 3) this in turn affects the ability of PGF2a to inhibit LH-stimulated P4 synthesis at this developmental stage (the latter interpretation is supported by the siRNA data). 4) The cellular mechanism utilized by PKCe in mediating the developmental luteolytic actions of PGF2a might be related to regulation of expression of genes involved in Ca2+ homeostasis, an interpretation supported by data from the PKCe-specific inhibitor and calcium ionophore. These data demonstrate that an increase in [Ca2+]i is necessary and sufficient to inhibit LH-stimulated P4 accumulation regardless of the luteal developmental stage. On the other hand, increasing Ca2+ buffering capacity in day-10 CL tissue with Bapta-2AM, abrogated the inhibitory action of PGF2a on LH-stimulated accumulation of P4.
GENETIC AND METABOLIC/NUTRITIONAL ATTRIBUTES OF THE EMBRYO Gene expression in embryos: It is hypothesized that alterations in the maternal transcripts will continue through early embryonic development and alter embryonic gene expression. Embryos at 2-, 8-, and 16-cell stages derived from growing and persistent follicles will be used for this experiment. These stages represent time points when the embryo is dependent primarily on maternal transcripts (2 cell), embryonic activation (8 cell), and dependence on its own transcripts (16 cell). The stages were chosen based on when the majority of embryonic loss occurs in embryos produced from oocytes from persistent follicles (Wishart 1977; Ahmad et al., 1995). Lactation status and embryo survival: Lactation detrimentally affects embryo quality; embryos flushed from lactating cows were detectably inferior to embryos from dry cows as early as d 5 after ovulation (Sartori et al., 2006). Fertilization rate of oocytes was not different (~ 89%), but uterine flushings from 53% of lactating cows contained only non-viable embryos. These results are important to understanding low fertility rates in lactating cows, but cannot discriminate between metabolic effects on the oocyte vs. on the environment within the reproductive tract. A recent paper by Vasconcelos et al. (2006) compared pregnancy rates for lactating cows following estrus and AI (33%) vs. estrus and embryo transfer d 7(46%; P<0.01). Because the embryo donors were non-lactating cows, these results strongly indicate that oocyte competence for embryo survival is markedly decreased during high milk production. Additional effects on the uterine environment cannot be ruled out.
Objectives
-
Identify genetic, morphological and physiological attributes of the ovary considered to improve fertility in ruminants
-
Identify genetic and metabolic/nutritional attributes associated with embryo survival in ruminants.
Methods
Objective 1. Identify genetic, morphological and physiological attributes of the ovary considered to improve fertility in ruminants (Contributions from the CT, MA, NE, NH, NY, OH and WV Experiment Stations). Follicle/oocyte Global changes of maternal transcripts in oocytes produced from persistent follicles Non-lactating beef cows will be assigned randomly to four treatment groups. Ovaries will be removed via colpotomy (Casida, 1960) on the mornings of d 6 or d 8 for the cows with growing follicles and on d 13 or d 15 for cows with persistent follicles.Gene expression profiles will be analyzed in oocytes generated from growing and persistent follicles. Oocytes collected from d 6 (growing follicle; GV stage), d 8 (growing follicle; MII stage), d 13 (persistent follicle; MII stage), and d 15 (persistent follicle; MII stage) follicles will be used. The selection of these follicles should permit detection of changes in gene expression due to maturation (progression from MI to MII) or aging (persistence of a follicle) of the oocyte.
Four independent samples of 2 oocytes per sample will be collected from the above mentioned follicles. Microarray analysis will be conducted using a reference design, with amplified cDNA samples pooled from day 6 oocytes as the reference. A total of 32 microarray hybridizations will be performed, with 16 being reverse labeled cDNA samples. The 18K bovine cDNA microarray (Suchyta et al., 2003) developed by Michigan State University through the National Bovine Functional Genomics Consortium (NBFGC) will be used. Determine the effect of high estrogen concentrations on granulosa cell function. An initial experiment will be conducted to determine how E2 regulates the expression and function of gap junction proteins in granulosal cells. In vitro cultures of bovine granulosal cells and cumulus-oocyte complexes will be established and treated with increasing concentrations of E2. Chromatin immunoprecipitation (ChIP) assays will be carried out using the granulosal cell cultures to examine estrogen receptor a (ERa)- and ERb-dependent regulation of gap junction protein expression. Functional studies in the cumulus-oocyte complex cultures will be carried out to determine how altered gene expression impacts interaction of the granulosal cells with the oocyte and the ability of the granulosal cells to support oocyte growth and maturation.
A second experiment will be conducted to identify the mechanism of E2-mediated anti-apoptosis in the granulosal cell. E2 can act through both genomic and nongenomic mechanisms. To identify the genomic actions of E2, in vitro granulosal cell cultures will be treated with increasing concentrations of E2, and ChIP assays will be carried out to identify anti-apoptotic genes regulated by ERa or ERb. To define the nongenomic actions of E2 with respect to the apoptotic pathway, the activation of signal transduction pathways by E2 will be assessed. Inhibitors of signal transduction will be used to verify the importance of a given signaling pathway on inhibition of apoptosis in the granulosal cells.
Determine the mechanism of E2 regulated centrosome function and its subsequent effect on the meiotic cell cycle of the oocyte and mitotic cell cycle of the zygote. In this experiment the hypothesis is that increased E2 concentrations lead to duplication of centrosomes and chromosomal instability in the oocyte. In vitro follicle cultures will be established using a protocol described previously (Eppig and O'Brien, 1996; O'Brien et al., 2003). The follicles will be exposed to increasing concentrations of E2 and assayed for increased mRNA and protein levels of centrosome-associated genes. The functional significance of increased centrosome gene expression on the meiotic cell cycle of the oocyte as well as the the mitotic cell cycle of the pre-implantation embryo will subsequently be ascertained using in vitro follicle cultures, in vitro embryo cultures and/or transgenic animals. Do oocytes of high producing lactating dairy cows show structural differences with those of non-lactating dairy heifers? Oocytes will be obtained from large follicles (> 10 mm) using transvaginal ultrasound guided oocyte aspiration as described (Carter et al., 2002). Estrous detection will be performed twice a day and cows and heifers will be aspirated at the first sign of estrus. At this stage most oocytes will be at the GV stage, and hence oocyte maturation will be performed in vitro according to established protocols (Knott et al., 2002). Oocytes will be aspirated from pre-ovulatory follicles of 10-15 mm. Follicles will be aspirated from lactating dairy cows between 40-100 days after parturition. Heifers from the same breeds and herd will be collected in the cycles prior to their insemination to attain conception. Using this aspiration method, 60 to 70% of the medium to large-size follicles punctured result in oocytes (Carter et al., 2002). Upon returning to the laboratory, oocytes will be handled according to the assigned treatment and used for each of the analyses proposed.
For evaluation of actin distribution, microtubule and spindle organization, and IP3R-1 organization oocytes will be immediately fixed and prepared for fluorescence immunostaining. Oocytes incubated without primary antibody will serve as negative controls. Fluorescence for all the previous procedures will be examined using a Zeiss confocal laser-scanning microscope LSM 510 (CLSM) or with a Zeiss Axiovert 200M microscope.
Do oocytes of high producing lactating dairy cows show functional differences from those of non-lactating dairy heifers?
Oocytes collected from heifers or lactating dairy cows as above will be individually and simultaneously analyzed for MPF and MAPK activities, as described by our laboratory (Jellerette et al., 2004). Evaluation of the Ca2+ oscillatory ability will also be performed in individual eggs. Following aspiration, oocytes will be injected with Fura-2 dextran, which is a fluorescent Ca2+ indicator. After 30 min interval, oocytes will be injected with bovine PLCz mRNA. PLCz is the molecule from the sperm thought to be involved in the initiation of Ca2+ oscillations at fertilization (Saunders et al., 2002). Each oocyte will be injected with 0.2 to 0.5 mg/ml bPLCz mRNA, which are concentrations known to induce sperm-like Ca2+ responses (Malcuit et al., 2005). Ca2+ monitoring will start 30 to 60 min post-injection of the mRNA and will continue for 4 hr. Ca2+ monitoring will be performed as described (Malcuit et al., 2005).
Compare the effects of preovulatory follicle size and estradiol production on subsequent CL size, plasma progesterone and embryo survival in lactating dairy cows.
Lactating dairy cows will be synchronized for timed AI using a modified Ov-Synch protocol. Follicular size will be measured at the time of AI. Size of the CL will be measured on days 8 and 15 after AI. Blood samples for assay of estradiol and P4 will be collected d 0 through 8, 11, 15, 22 and 29. Pregnancy will be detected by ultrasonography on d 29 and by rectal palpation on d 42. For 15-20 cows, liver biopsies will be collected on d 8 for mRNA analysis of P4 oxidative enzymes (Lemley et al., 2006) in comparison to blood P4 profiles. Data analysis will include retrospective analyses between pregnant and non-pregnant cows. An additional group of 7 virgin heifers will be studied in the same manner as for the lactating cows to provide a control for lack of lactation related metabolic effects on plasma P4 and liver enzymes.
Develop methods for activating primordial follicles in vitro for further growth to the stage of competence for meiotic maturation and fertilization in vitro (IVM/IVF).
Ovaries will be obtained from 5-8 mo. bovine fetuses at a local slaughterhouse with age estimated by crown-rump length (Nichols, 1944). Ovarian cortical pieces will be isolated and either fixed immediately or cultured in serum-free medium supplemented with TS+ for 2, 4 or 10 days (2 cortical pieces/culture well; 2 wells/treatment/fetus) under the conditions below.
Control medium (Waymouth medium MB 752/1 + TS+)
KL (25, 50, 100, and 200 ng/ml)
ITS+ control
The proposed doses of KL bracket those effective with newborn rat ovaries. Experiments will be repeated on 4-5 separate occasions. At time 0 (uncultured control) and after 2, 4, or 10 days of culture in the treatments outlined above, cortical pieces will be fixed, embedded in LR white plastic and serially sectioned. Every twentieth section will be analyzed by histological morphometry for numbers and diameters of primordial, primary and secondary follicles. Cortical pieces cultured with ITS+ will serve as a positive control, since ITS+ has never failed to support rapid and wholesale activation of primordial follicles. The results will be analyzed by ANOVA, followed by Duncan's multiple range test if ANOVA indicates significant effects of treatment.
Corpus luteum
Isolate endothelial cells (EC) from early, mid and late cycle corpora lutea (CL).
Early, mid and late cycle CL will be collected from dairy cows either by ovariectomy (early CL) or by enucleation (mid and late cycle CL; Zhang et al. 2002).
Luteal EC will be separated from dispersed CL following dissociation using the Miltenyi AutoMacs cell separator. EC will be labeled with anti-bovine CD31 antibody (Research Diagnostics, Inc) and secondary antibody (rabbit anti-mouse IgG) conjugated to magnetic particles. Steroidogenic cells will be collected as the negative fraction; EC collected as the positive fraction (attracted to the magnet). These procedures are expected to yield highly purified steroidogenic and EC fractions not contaminated with macrophages. Purity of the preparations will be assessed by RT-PCR for StAR and immunostaining as described (Cavicchio et al. 2002). Determine gene expression profiles of EC from early, mid and late cycle CL.
Pooled EC from early, mid and late cycle CL (n=4 for each stage) will be sent to WV for microarray analysis using the 20K long oligo array developed by University of Missouri. The microarray experiments will be conducted using a reference design with pooled microvascular EC from bovine brain and kidney as the reference. Twenty micrograms of total RNA from each of the experimental samples and the reference samples will be used in a reverse transcription reaction incorporating amino-allyl dUTP into the cDNA using oligo-d (T) primer and Superscript II reverse transcriptase. The synthesized cDNAs from the experimental and reference samples will be labeled, concentrated and then injected into a Tecan HS400 automated microarray hybridization station. Following hybridization, ScanArray Express software will be used to process the array images, align spots, integrate robot-spotting files with the microarray image, and statistically analyzed. Genes with similar expression profiles will be grouped and further analyzed. Perform flow cytometric analysis to quantify and to distinguish EC subtypes by adhesion molecule expression and cytoskeletal protein. A portion of the pooled EC used for microarray analysis will be concentrated to 400,000 cells/ml and fixed in 1% paraformaldehyde solution. The fixed cells will be subsequently labeled with fluorescent antibodies to neuronal cell adhesion molecule (NCAM 140), epithelial cadherin (E-CAD) and cytokeratin 18 (CK18) to identify the 4 phenotypes of EC present within bovine CL. Determine the type of lymphocyte that is activated by luteal endothelial vs. steroidogenic cells.
Following separation, steroidogenic luteal cells or luteal EC will be placed in co-culture with T lymphocytes isolated from the same cow, as previously published (Petroff et al., 1997; Cannon et al., 2003). Negative controls for lymphocyte proliferation will include freshly isolated T cells and cultures without Con A or luteal cells. Con A activated lymphocytes will be serve as positive controls. At the end of the incubation, T cells will be collected in the culture supernatant, centrifuged, washed, and incubated with antibodies to determine which class of T cells is stimulated by EC or steroidogenic cells, and analyzed using the Guava Easy Cyte cell analyzer. Role of nitric oxide in luteolysis Experiments will be conducted to determine the effects of an exogenous inhibitor (L-NAME) or stimulator (spermine NONOate) of nitric oxide synthase on ovine CL function. The specific aims are to determine if intrauterine (IU) or intramuscular administration of L-NAME will delay spontaneous and PGF-2±-induced luteolysis, and whether IU or IM treatments with spermine NONOate increase luteal P4 production and lifespan. Additional experiments will examine the effects of these NO effectors when given in combination with a subluteolytic dose (7.5 mg) of PGF-2± at mid-cycle.
Experiments will also be conducted to determine if NO is involved in the mechanism of action of ET-1 in inducing ovine luteolysis. Various doses of NO effectors will be in combination with exogenous ET-1 during day 9 of the ovine estrous cycle. Effects of the peroxisome proliferator-activated receptors (PPARs) Preliminary results indicate that activation of PPARs can modify steroid production. Experiments will examine the effects of PPAR activators and inhibitors on the biosynthesis of basal and LH-stimulated P4 production by ovine luteal cells. In addition, the expression of PPAR ±, ³, and ´ will be determined in luteal tissue collected on days 4, 8, 12 and 15 of the estrous cycle and at frequent intervals after exogenous PGF-2±. Studies of bovine and ovine corpora lutea in relation to susceptibility to luteal regression during the etrous cycle and intraluteal mechanisms of the actions of prostaglandin (PG) F2a and endothelin-1 in luteolysis. The hypothesis that luteal expression of PKCe is a necessary component of the signal transduction associated with the FPR will be tested as follows. 1) An siRNA procedure will be used to down regulate PKCe and the ability of PGF2a to stimulate an increase in [Ca2+]i , 2) Real-time RT-PCR will be used to examine the developmental regulation of genes expected to control Ca2+ homeostasis in luteal steroidogenic cells. Three types of voltage gated Ca2+ channels (VGCCs): L-, T- and N- type and the IP3 gated calcium channels will be examined. 3) Microarray analysis will be used to profile those genes whose expression might be affected in PKCe-down regulated steroidogenic luteal cells. Endothelial cells also will be subjected to microarray analyses, because a preliminary experiment indicated upregulation from Day 4 to Day 10 of at least 10 genes likely to be present in endothelial cells (Goravanahally and Flores, unpublished). These studies will allow us to elucidate the cellular mechanisms that support PGF2a -induced increases in [Ca2+]i and that might be involved in influencing the lifespan of the CL. Objective 2. Identify genetic and metabolic/nutritional attributes of the embryo associated with survival in ruminants (Contributions from the NY and WV Experiment Stations).
Embryo Comparison of expression profiles of embryonic transcripts in early stage embryos derived from persistent and growing follicles Oocytes will be obtained as described above (A.1.iii.) using non-lactating beef cows. Cows will be assigned randomly to two superovulated groups in which either growing or persistent follicles will be allowed to ovulate and will be inseminated 12 and 24 hours after standing estrus with frozen-thawed semen from bulls of high fertility. Fifty-two, 68, or 96 hrs post insemination, oviducts will be surgically removed via a flank incision as described (Breuel et al., 1993; Lingenfelter et al., 2005). The mesosalpinx will be removed carefully and the oviduct flushed to recover all embryos. Embryos will be collected at the 2-, 8-, and 16- cell stages beginning 52 hr after ovulation. Prior to freezing, embryos will be graded as described by Bongso and Gardner (2000) using a Nikon 2000TE-S inverted microscope. Only embryos of grade 3 and above will be used in the experiment.
We will use a direct comparison design to examine gene expression profiles in embryos produced from persistent and growing follicles at the three developmental stages. For each replication, two embryos will be pooled (within stage) and lysed directly in first strand cDNA synthesis buffer. Half of the lysate (equivalent of one embryo) will be subjected to global cDNA amplification (for microarray analysis) and the other half will be subjected to T7-based linear amplification (for real time PCR confirmation of microarray data). Four replications will be used for each comparison at a specific stage. Therefore, a total of 24 embryos will be required (2 per replication, 4 replications, 3 stages) per treatment (growing or persistent follicles). Reverse labeling (dye swapping) will be performed for each comparison. Thus, a total of 24 microarray slides will be used in this experiment.
In order to confirm gene expression differences observed by microarray, quantitative real time PCR will be performed on a selected number of genes. We will select up to 20 genes identified from each microarray experiment that show significant differences between oocytes/embryos derived from growing and persistent follicles. Gene specific primers will be designed based on the sequence information of respective genes. The mRNA from equivalent of one oocyte or embryo (half of the lysate of 2 oocytes/embryos) will be linearly amplified using Ambions MessageAmp" II aRNA kit according to the manufacturers instruction Comparison of embryo survival rates following embryo transfer (ET) into lactating cows vs. virgin Holstein heifers (high fertility positive control group).
Studies will be conducted to compare effects of lactation status (lactating vs. non-lactating) on embryo survival in dairy cattle.
Embryos for transfer for both groups will be of comparable quality and from a uniform source (preferably non-lactating cows or heifers). Cows and heifers will be synchronized for day of ovulation and ET performed on d 7 of the cycle. Presence of a CL will be confirmed by ultrasonography prior to ET. Blood samples will be collected for P4 analysis. Pregnancy rate will be determined by d 42 by rectal palpation. Expected outcomes: a) a lower rate of embryo survival in lactating cows would suggest that uterine environment (P4 dependent) is impaired for supporting embryo survival and will be addressed further in this project (A.1.iv), b) Equal rates of embryo survival in lactating cows and heifers would suggest that inherently lower fertility observed in lactating cows is more related to impaired oocyte/embryo competence and will be investigated in the next experiment.
Comparisons of oocytes collected from lactating cows vs. heifers for embryo developmental capacity (IVM/IVF) to morula/blastocyst stage will be tested by subsequent transfer to recipients (2 x 2 reciprocal transfer design; lactating cows vs. heifers as donors and recipients). Following superovulation treatment procedures, oocytes will be aspirated from donor cows and heifers by ultrasound guided needles (Blondin et al., 2002). Oocytes will be matured in vitro and fertilized with semen from a single ejaculate to remove sire effects. Embryos will be cultured to the compact morula/early blastocyst stage and transferred to synchronized recipients on their cycle d 7. The presence of a CL will be verified prior to ET and blood samples collected for P4 analysis. Pregnancy rate will be determined by rectal palpation at d 42. Statistical analyses will compare main effects and interactions of donor and recipient types on embryo survival.
Measurement of Progress and Results
Outputs
- Research data on oocyte quality in growing vs. persistent follicles, high producing lactating cows vs. nonlactating dairy heifers, and in an altered steroidal milieu in published form.
- Research data on luteal cell sub-types (e.g., endothelial cells, steroidogenic cells, immune cells) that influence luteal function in published form.
- Research data on environmental and metabolic effects on embryonic/fetal survival in published form.
- Information that will help producers make informed management decisions to enhance embryonic/fetal survival in ruminants.
- Present research results at professional meetings for animal scientists, veterinarians and other agricultural related professionals.
- Models that can be used for further research.
Outcomes or Projected Impacts
- Further refine current estrous synchronization protocols and management strategies to enhance embryo survival.
- Development of herd management strategies to minimize the effects of nutritional/metabolic stress on herd fertility.
Milestones
(2008): Established genetic and structural traits of high quality oocytes and follicles.(2009): Established genetic, morphologic, and physiologic characteristics of purified endothelial cells derived from the microvasculature of the CL.
(2010): Established relationships and interactions among endothelial cells and large and small steroidogenic cells during luteal development and luteolysis.
(2011): Established methods for isolation and conditions for growth of secondary follicles in vitro
(2012): Identified influence of lactation status on oocyte quality and embryo survival.
Projected Participation
View Appendix E: ParticipationOutreach Plan
A portion of the work proposed will be basic and a portion will be applied; some of the latter will be conducted on cooperating farms. Results will be communicated in annual reports, in refereed publications, in presentations at national meetings and on the websites of the experiment stations involved. Data will be presented to experiment station advisory boards, and to extension personnel and commodity groups in short courses and at field days. In addition, some extension specialists and agents will aid in collecting applied data and selecting cooperating farms. Research workers will prepare occasional news releases or participate in interviews. Through the latter venues, stakeholder input for further work and reactions to work in progress will be obtained. Thus an interactive system for dissemination of results and guidance in applications will be maintained throughout the project.
Organization/Governance
A regional Technical Committee will be organized in accordance with the Manual for Cooperative Regional Research (CSRS-OD-1082, 1977). The voting membership of the Regional Technical Committee shall include one representative from each cooperating Agricultural Experiment Station as appointed by the respective Director, a technical representative of each cooperation USDA-ARS research division and other participating organizations. Non-voting members shall consist of the Administrative Advisor and a consulting member representing CSRS. All voting members of the Technical Committee are eligible for office. A chairperson, a secretary, and a third member of the committee will be elected for 2-year terms to compose an Executive Committee. The Technical Committee will meet at least annually. The chairperson, in consultation with the administrative advisor, will notify members of the time and place of meetings. The chairperson is responsible for the preparation of the annual report of the regional project. The secretary records the minutes and other duties as assigned by the Technical Committee. The Executive Committee may be delegated to conduct the business of the Technical Committee between meetings. Other subcommittees may be named by the chairperson as required.
Literature Cited
Ahmad, N., F.N. Schrick, R.L. Butcher, and E.K. Inskeep. 1995. Effect of persistent follicles on early embryonic loss in beef cows. Biol. Reprod. 52:1129-1135. Blondin, P., D. Bousquet, H. Twagiramungu, F. Barnes, and M.-A. Sirad. 2002. Manipulation of follicular development to produce developmentally competent bovine oocytes. Biol. Reprod. 66:38-43. Bongso A and Gardner DK. 2000. Embryo Development In: Handbook of In vitro Fertilization. Eds Alan O. Trounson and David K. Gardner. Boca Raton: CRC Press: 167. Breuel, K. F., P. E. Lewis, F. N. Schrick, A. W. Lishman, E. K. Inskeep, and Butcher RL. 1993. Factors affecting fertility in the postpartum cow: role of the oocyte and follicle in conception rate. Biol. Reprod. 48:655-661. Butler, W. R. 2003. Energy balance relationships with follicular development, ovulation and fertility in postpartum dairy cows. Livest. Prod. Sci. 83:211-218. Carter, J. Bellow, Meintjes, S., Perez, O., Fergunson, E., Godke, R.A. (2002). Transvaginal ultrasound-guided oocyte aspiration for production of embryos in vitro. Arch. Tierz., Dummerstorf 45 1, 99-108. Casida, L.E. 1960. Research techniques in physiology of reproduction in the female. In Techniques and Procedures in Animal Production Research. American Society of Animal Science Monograph. Cannon, M.J., M.G. Petroff, and J.L. Pate. 2003. Effects of prostaglandin F2¬ and progesterone on the ability of bovine luteal cells to stimulate T lymphocyte proliferation. Biol. Reprod. 69:695-700. Chen, Y, Lai M, Maeno-Hikichi Y, Zhang JF. 2006. Essential role of LIM domain in the formation of the PKCepsilon-ENH-N-type Ca2+ channel complex. Cell Signal. 18: 215-224 Choudhary C, Sen A, Inskeep EK and Flores JA. 2005. Developmental sensitivity of the bovine corpus luteum (CL) to prostaglandin F2a (PGF2a) and endothelin-1: Is ET-1 a mediator of the luteolytic actions of PGF2a or a tonic inhibitor of progesterone secretion. Biol Reprod 72:633-642. Cooperative Regional Research Project, NE-161. 1996. Relationship of fertility to patterns of ovarian follicular development and associated hormonal profiles in dairy cows and heifers. J. Anim. Sci. 74:1943-1952. Eppig JJ, O'Brien MJ 1996 Development in vitro of mouse oocytes from primordial follicles. Biol Reprod 54:197 207 Inskeep EK, Tyler WJ, Casida LE. 1961. Hereditay variation in conception rate of Holstein-Friesian cattle. J. Dairy Sci. 44:1857-1862. Iscove NN, Barbara M, Gu M, Gibson M, Modi C, Winegarden N. 2002. Representation is faithfully preserved in global cDNA amplified exponentially from sub-picogram quantities of mRNA. Nat Biotechnol. Sep;20:940-3. Jaffe, L. A., and Terasaki, M. (1994). Structural changes in the endoplasmic reticulum of starfish oocytes during meiotic maturation and fertilization. Dev Biol 164, 579-87. Jellerette, T., Kurokawa, M., Lee, B., Malcuit, C., Yoon, S.-Y., Smyth, J., Vermassen, E., De Smedt, H., Parys, J. B., and Fissore, R. A. (2004). Cell cycle-coupled [Ca2+]i oscillations in mouse zygotes and function of the inositol 1,4,5-trisphosphate receptor-1. Developmental Biology 274, 94-109. Kezele P, Nilsson EE, Skinner MK 2005 Keratinocyte growth factor acts as a mesenchymal factor that promotes ovarian primordial to primary follicle transition. Biol Reprod 73:967 73. Kline, D., Mehlmann, L., Fox, C., and Terasaki, M. (1999). The cortical endoplasmic reticulum (ER) of the mouse egg: localization of ER clusters in relation to the generation of repetitive calcium waves. Dev Biol 215, 431-42. Knott, J. G., Poothapillai, K., Wu, H., He, C. L., Fissore, R. A., and Robl, J. M. (2002). Porcine sperm factor supports activation and development of bovine nuclear transfer embryos. Biology of Reproduction 66, 1095-103. Lemly, C. O., J. M. Koch, K. P. Blemings, and M. E. Wilson. 2006. Insulin induced alteration in progesterone catabolism in murine hepatocytes appears to be mediated by CYP3A13. Biol. Reprod. 75(Suppl. 1):390. Levy N, Gordin M, Mamluk R, Yanagisawa M, Smith MF, Hampton JH, Meidan R. Distinct cellular localization and regulation of endothelin-1 and endothelin-converting enzyme-1 expression in the bovine corpus luteum: implications for luteolysis. Endocrinology 2001; 142: 5254-5260 Lingenfelter, B.M., Dailey R.A., Inskeep, E.K. Vernon, M.W., Poole, D.H., Rhinehart, J.D., and Yao J. 2005. Differential Gene Expression of Oocytes obtained from Growing and Persistent Follicles. 38th Annual Meeting, SSR 215: (Abstr 610). Lopes, A. S., S. T. Butler, R. O. Gilbert, and W. R. Butler. 2007. Relationship of pre-ovulatory follicle size, estradiol concentrations and season to pregnancy outcome in dairy cows. Anim. Reprod. Sci. (In press). Lucy, M. C., H. J. Billings, W. R. Butler, L. R. Ehnis, M. J. Fields, D. J. Kesler, J. E. Kinder, R. C. Mattos, R. E. Short, W. W. Thatcher, R. P. Wettemann, J. V. Yelich, and H. D. Hafs. Efficacy of an intravaginal progesterone insert and an injection of PGF2a for synchronizing estrus and shortening the interval to pregnancy in postpartum beef cows, peripubertal beef heifers, and dairy heifers. Journal of Animal Science 79 (4):982-995, 2001. Malcuit, C., Knott, J. G., He, C., Wainwright, T., Parys, J. B., Robl, J. M., and Fissore, R. A. (2005). Fertilization and inositol 1,4,5-trisphosphate (IP3)-induced calcium release in type-1 inositol 1,4,5-trisphosphate receptor down-regulated bovine eggs. Biol Reprod 73, 2-13. McMahon T, Andersen R, Metten P, Crabbe JC, Messing RO. 2000. Mole Pharmacol and Exp Therapeutics 57: 53-58. Memili E, First NL. 2000. Zygotic and embryonic gene expression in cow: a review of timing and mechanisms of early gene expression as compared with other species. Zygote. 8(1):87-96. Mihm, M., A. Baguisi, M.P. Boland, and J.F. Roche. 1994. Association between the duration of dominance of the ovulatory follicle and pregnancy rates in beef heifers. J. Reprod. Fertil. 102:123-130. Nebel RL. 1999. Optimizing fertility in the dairy herd. Theriogenology 51:443-452. Nichols CW Jr. 1944 The embryology of the calf: fetal growth weights, relative age and certain body measurements. Amer J Vet Res 5:135 141 Nilsson EE, Kezele P, Skinner MK 2002 Leukemia inhibitory factor (LIF) promotes the primordial to primary follicle transition in rat ovaries. Mol Cell Endocrinol 188:65 73 Nilsson E, Parrott JA, Skinner MK 2001 Basic fibroblast growth factor induces primordial follicle development and initiates folliculogenesis. Mol Cell Endocrinol 175:123 130 Nilsson EE, Detzel C, Skinner MK 2006 Platelet derived growth factor modulates the primordial to primary follicle transition. Reproduction 131:1007 15. O'Brien MJ, Pendola JK, Eppig JJ 2003 A revised protocol for in vitro development of mouse oocytes from primordial follicles dramatically improves their developmental competence. Biol Reprod 68:1682 1686 Parrott JA, Skinner MK 1999 Kit ligand/stem cell factor induces primordial follicle development and initiates folliculogenesis. Endocrinology 140:4262 4271 Perry, G. A., M. F. Smith, M. C. Lucy, J. A. Green, T. E. Parks, M. D. MacNeil, A. J. Roberts, and T. W. Geary. 2005. Relationship between follicle size at insemination and pregnancy success. Proc. Natl. Acad. Sci. U. S. A 102:5268-5273. Petroff M, Coggeshall KM, Jones LS, Pate JL. Bovine luteal cells elicit major histocompatibility complex class II-dependent T-cell proliferation. Biol Reprod 1997; 57: 887-893 Revah, I., and W. R. Butler. 1996. Prolonged dominance of follicles reduces the viability of bovine oocytes. J. Reprod. Fertil. 106:39-47. Sangsritavong, S., D. K. Combs, R. Sartori, L. E. Armentano, and M. C. Wiltbank. 2002. High feed intake increases liver blood flow and metabolism of progesterone and estradiol-17b in dairy cattle. Journal of Dairy Science 85:2831-2842. Sartori, R., R. Sartor-Bergfelt, S. A. Mertens, J. N. Guenther, J. J. Parrish, and M. C. Wiltbank. 2002. Fertilization and early embryonic development in heifers and lactating cows in summer and lactating and dry cows in winter. Journal of Dairy Science 85:2803-2812. Sartori, R., G. J. M. Rosa, and M. C. Wiltbank. 2002. Ovarian structures and circulating steroids in heifers and lactating cows in summer and lacating and dry cows in winter. Page 2813 in . Sathiyaseelan, T. and C.L. Baldwin. 2000. Evaluation of cell replication by bovine T cells in polyclonally activated cultures using carboxyfluorescein succinimidyl ester (CFSE) loading and flow cytometric analysis. Res. Vet Sci. 69:275-281. Saunders, C. M., Larman, M. G., Parrington, J., Cox, L. J., Royse, J., Blayney, L. M., Swann, K., and Lai, F. A. (2002). PLC zeta: a sperm-specific trigger of Ca(2+) oscillations in eggs and embryo development. Development (Cambridge, England) 129, 3533-44. Savio, J. D., W. W. Thatcher, G. R. Morrris, K. Entwistle, M. Drost, and M. R. Mattiacci. 1993. Effects of induction of low plasma progesterone concentrations with a progesterone-releasing intravaginal device on follicular turnover and fertility in cattle. J. Reprod. Fertil. 98:77-84. Sen A, Browning J, Inskeep EK, Lewis P, Flores JA. 2004. Expression and activation of protein kinase C isozymes by prostaglandin F 2a (PGF2a) in the early and mid-luteal phase bovine corpus luteum. Biol Reprod; 70: 379-384. Sen A, Wright M, Inskeep EK and Flores JA. 2006. Participation of specific PKC isozymes in the inhibitory effect of ET-1 on progesterone accumulation in cells isolated from early-and mid-phase corpora lutea. Domestic Anim Endo 31:284-299. Sen A, Choudhary E, Inskeep EK and Flores JA. 2005. Effects of selective protein kinase C (PKC) isozymes in PGF2a -induced Ca2+ signaling and LH-induced progesterone accumulation in the mid-phase bovine corpus luteum. Biol Reprod72:976-984. Smith TP, Grosse WM, Freking BA, Roberts AJ, Stone RT, Casas E, Wray JE, White J, Cho J, Fahrenkrug SC, Bennett GL, Heaton MP, Laegreid WW, Rohrer GA, Chitko-McKown CG, Pertea G, Holt I, Karamycheva S, Liang F, Quackenbush J, and Keele JW. 2001. Sequence evaluation of four pooled-tissue normalized bovine cDNA libraries and construction of a gene index for cattle. Genome Res. 11: 626-630. Sonstegard TS, Capuco AV, White J, Van Tassell CP, Connor EE, Cho J, Sultana R, Shade L, Wray JE, Wells KD, and Quackenbush J. 2002. Analysis of bovine mammary gland EST and functional annotation of the Bos taurus gene index. Mamm Genome. 13: 373-379. Suchyta SP, Sipkovsky S, Kruska R, Jeffers A, McNult A, Tempelman RJ, Halgren RG, Saama PM, Bauman DE, Boisclair YR, Burton JL, Collier RJ, DePeters EJ, Ferris TA, Lucy MC, McGuire MA, Medrano JF, Overton TR, Smith TP, Smith GW, Sonstegard TS, Spain JN, Spiers DE, Yao J and Coussens PM. 2003. Development and Testing of a high-density cDNA microarray resource for cattle. Physiological Genomics, 15:158-164. Taft RA. 1999. Oocyte Morphology and estrogen concentrations following a reduction in progesterone in beef cattle (Doctoral Dissertation, WVU) 34 -47. Telford NA, Watson AJ, Schultz GA. 1990. Transition from maternal to embryonic control in early mammalian development: a comparison of several species. Mol Reprod Dev. 26 (1):90-100. Tong ZB, Gold L, Pfeifer KE, Dorward H, Lee E, Bondy CA, Dean J, Nelson LM. 2000a. Mater, a maternal effect gene required for early embryonic development in mice. Nat Genet. 26 (3):267-8. Vasconcelos, J. L., S. Sangsritavong, S. J. Tsai, and M. C. Wiltbank. 2003. Acute reduction in serum progesterone concentrations after feed intake in dairy cows. Theriogenology 60:795-807. Vasconcelos, J. L., D. G. Demetrio, R. M. Santos, J. R. Chiari, C. A. Rodrigues, and O. G. Sa Filho. 2006. Factors potentially affecting fertility of lactating dairy cow recipients. Theriogenology 65:192-200. Dekel, N. (2005). Cellular, biochemical and molecular mechanisms regulating oocyte maturation. Molecular and Cellular Endocrinology 234, 19-25. Vermassen, E., Van_Acker, K., Annaert, W. G., Himpens, B., Callewaert, G., Missiaen, L., De_Smedt, H., and Parys, J. B. (2003). Microtubule-dependent redistribution of the type-1 inositol 1,4,5-trisphosphate receptor in A7r5 smooth muscle cells. Journal of Cell Science 116, 1269-77. Wandji S A, Srsen V, Voss AK, Eppig JJ, Fortune JE 1996 Initiation in vitro of growth of bovine primordial follicles. Biol Reprod 55:942 948 Wandji S A, Srsen V, Nathanielsz PW, Eppig JJ, Fortune JE 1997 Initiation of growth of baboon primordial follicles in vitro. Human Reprod 12:1993 2001 Wishart, D. F. 1977. Synchronization of oestrus in heifers using steroid (SC 5914, SC 9880 and SC 21009) treatment for 21 days: the effect of treatment on the ovum collection and fertilization rate and the development of the early embryo. Theriogenology 8:249-269. Wu X, Viveiros MM, Eppig JJ, Bai Y, Fitzpatrick SL, Matzuk MM. 2003. Zygote arrest 1 (Zar1) is a novel maternal-effect gene critical for the oocyte-to-embryo transition. Nat Genet. 33(2):187-91.