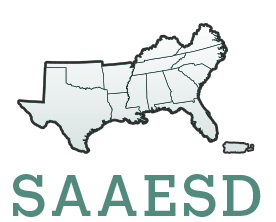
OLD S1041: The Science and Engineering for a Biobased Industry and Economy
(Multistate Research Project)
Status: Inactive/Terminating
OLD S1041: The Science and Engineering for a Biobased Industry and Economy
Duration: 10/01/2008 to 09/30/2013
Administrative Advisor(s):
NIFA Reps:
Non-Technical Summary
Statement of Issues and Justification
I. Title: The Science and Engineering for a Biobased Industry and Economy II. Statement of Issue(s) and Justification
A. The Land Grant University System, Resource Limitation, and the Impending Biological Revolution. A need for biofuels and other biobased products has been recognized as a national priority. The objectives of this project address research relating directly to SAAESD Goal 1 F (biobased products) and H (processing agricultural coproducts); research will influence Goal 5 B (rural community development and revitalizing rural economies) indirectly. The importance and extent of the problem is characterized by the fact that the U.S. must drastically reduce its dependence on petroleum. This is not the fetish of a small proportion of the population; the U.S. society as a whole recognizes the need to reduce its dependence on petroleum as a source of fuels, chemicals and other materials. If this research work is not conducted, the technical capability necessary to switch from a petroleum-based economy to a bioresource-based one will not be developed. The technical feasibility of the research is reinforced by the fact that this research will be conducted by professional researchers who currently are part of the Land Grant University system. As outlined in this project description, the Land Grant University system provides a unique capability to enable research for biobased products by providing a world class research network. Replacing petroleum products with those originating from biological sources will require significant fundamental and applied research efforts.
The Land Grant University system was established in 1862 for the purpose of providing colleges for the benefit of agriculture and the mechanic arts. This revolutionary approach to education has been remarkably successful. Since its inception, the Land Grant System has been the major driving force for the development of technology and an educated work force to use the technology that has maintained an exponential rate of increase in food production, outpacing the rate of human population increase. This success is even more remarkable in light of the fact that in recent years the increase in food production has occurred using constant land area. However, unprecedented societal changes are occurring in the 21rst century. Not only is land area called on for food production, but also for biomass production that will be converted to biofuels, biochemicals and biomaterials. In 2008 (time at which this Multi State Project was created), the price of a barrel of oil increased from $70 to over $140, emphasizing the urgent need for developing sustainable alternatives to fossil fuels. Increases in energy demand and prices are mirrored with an increase in food consumption, rendering the situation extremely challenging.
Meeting food production needs are staggering. It requires 100 fold more energy to sustain the current U.S. standard of living as it is to nourish our bodies. For example, the U.S. consumes 100 quadrillion Btu annually for food production. Of the 100 quadrillion Btu/year used, energy necessary to sustain our population is 1.2 quadrillion Btu. The other portion of the 100 quadrillion Btu is accounted by agricultural and industrial production, liquid transportation fuels, heating and lighting needs. Having the goal of supporting a population of 10 billion at the current standard of living of the U.S. will require 4,000 quadrillion Btu annually worldwide. These demands cannot be sustained with the current technology base, and alternative and sustainable technologies must be developed and refined.
Although 2008 has seen the price of a barrel of oil more than double, responses to curtail energy needs were enacted already in 2007. The Energy Independence and Security Act was signed by the President in 2007 and set a Renewable Fuel Standard of 36 billion gallons per year of biofuels by 2022. Of the 36 billion gallon per year mandated by this act, corn to ethanol contributions will be in the realm of 15 billion gallons per year, calling for the production of 21 billion gallon per year of cellulosic biofuels. To produce 21 billion gallons per year of advanced biofuels, approximately 250 million dry tons of biomass will be required. Although, the Renewable Fuel Standard specifically addresses the production of liquid fuels, unprecedented activity in renewable energy research, such as wind, solar, geothermal, tidal and battery capacity is currently underway.
To respond to these unprecedented demands, the DOE and USDA are aligning their programs and joining forces. The DOEs Office of Energy Efficiency and Renewable Energy has created three BioEnergy Research Centers: BioEnergy Science Center in Tennessee, Great Lakes BioEnergy Research Center in Wisconsin and Joint BioEnergy Institute in California. Each center represents a multidisciplinary partnership with expertise spanning the physical and biological sciences, including genomics, microbial and plant biology, analytical chemistry, computational biology and bioinformatics, and engineering to accelerate the development of sustainable bioenergy production. In addition to the BioEnergy Research Centers, DOE is pursuing energy research efforts at their National Laboratories, such as National Renewable Energy Laboratory, Pacific Northwest National Laboratory, Oak Ridge National Laboratory and Brookhaven National Laboratory. Moreover, Office of Energy Efficiency and Renewable Energys Office of Biomass has created a Multi Year Program Plan (U.S. DOE MYPP), which enumerates specific milestones to be achieved in the production and handling of herbaceous and woody biomass, of agricultural residues and of municipal wastes and in the biochemical and thermochemical energy conversion protocols of these feedstocks. The Office of Energy Efficiency and Renewable Energy is coordinating their effort with the Sun Grant Initiative.
Coordinated federal activities between the DOE and USDA are underway, leading to the development of the Biomass Research and Development Initiative, which supports research in the private sector as well as in academia. USDA also supports National Centers, such as the National Center for Agricultural Utilization Research, that have groups devoted to bioenergy research. Moreover, USDA has developed its Energy Research Education and Extension Strategic Plan which contains four goals: 1) Sustainable agriculture and natural resource-based energy production; 2) Sustainable bioeconomies for rural communities; 3) Efficient use of energy and energy conservation; and 4) Work force development for the bioeconomy. The four goals are in line and complementary to the mission of the Energy Efficiency and Renewable Energy of DOE. To attain their four goals, the USDA is aligning itself with the Land Grant Universities.
These are truly extraordinary times, where Federal Agencies are coordinating their efforts so that sustainable energy production can become a reality. The Land Grant University system is in an unprecedented position where it can coordinate its activities with those of the Federal Agencies, so that it can contribute to existing momentum. The Land Grant University system provides a mechanism for leadership and technology development to facilitate the sustainable collection of 250 million tons of dry biomass, necessary for the production of 21 billion gallons per year of cellulosic biofuels. The Land Grant University system can be a partner to DOE and USDA for attaining the energy production goals. The Land Grant University system has within its ranks expert scientists in every area related to the capture of solar energy and its transformation to food, fuel, and shelter. The Land Grant University system can assist translating research results into real-life practices and participate in the creation of a work force to support the bioeconomy.
B. Enabling New Bioindustries. This new biobased industry, be it for food, fuel, biomaterials or other coproducts is rooted in a sustainable and productive biomass production system. Perlack et al. (2005) reported that one billion dry tons of biomass can be harvested on an annual basis from U.S. land. The Office of Energy Efficiency and Renewable Energy set as a milestone in its Multi Year Program Plan (U.S. DoE MYPP) that 250 million dry tons of biomass is to be harvested annually. The term feedstock comprises agricultural residues and municipal solid wastes as well as herbaceous and woody crops. Whether one billion or 250 million dry tons, this is an enormous amount of biomass to be delivered yearly on a sustainable basis (Kumar and Sokhansanj, 2006; Cundiff and Grisso, 2008; Hoskinson etal., 2006). Creative arrangements will need to be devised to enable the production, collection, storage and transportation of this feedstock to the biorefinery. As an example, productivity will need to be increased, which calls for plant molecular biology transformations. Moreover, these feedstock production activities will need to be conducted in a sustainable fashion that respect the soil organic carbon content and minimize erosion (Lee et al. 2008). Additionally, these feedstock production and delivery activities will need to be conducted in a manner that does not hinder the existing food and forestry supply chains.
Fortunately, the Land Grant University system is not operating as a standalone organization, but can tie into existing momentum that is aimed at developing this bioeconomy. Over the past 20 years, engineers and scientists have made advances in the fractionation and bioprocessing of agriculturally based resources into raw materials that are required to obtain the principal building blocks for synthesis of new products (Corma etal, 2006; Huber, 2007; Ragauskas etal., 2006). Fractionation and bioprocessing are key to the transformation of feedstock into biofuels or other value-added products when following the biochemical conversion platform. The critical first step in biochemical conversion requires that feedstock be fractionated into constituent monosaccharides and modified to facilitate either enzymatic or microbial conversion. Several promising fractionation or pretreatment technologies have been evaluated through the work of the Consortium for Applied Fundamentals and Innovation (CAFI). Dilute sulfuric acid or sulfur dioxide, controlled pH, ammonia fiber explosion, ammonia recycle percolation and lime pretreatment have or are being evaluated on corn stover, switchgrass and hybrid poplar.
Pretreatments seem to be biomass specific, as ammonia fiber explosion appears to be the pretreatment of choice for corn stover, but dilute sulfur dioxide is preferred for biomass containing more lignin. In addition, engineering advances in bioseparation technologies are improving our ability to identify and separate reaction inhibitors and other biocompounds from the pretreated slurry (Mosier etal, 2005; Sun and Cheng, 2002). Advances in nanobiotechnology that take advantage of differences in physical and chemical properties to achieve separations of proteins, secondary metabolites and other organic compounds are only a few of the recent engineering achievements. In analytical biotechnology, chemical and biological techniques are combined and integrated into engineered devices or sensors for the detection and quantification of secondary metabolites and other organic compounds in a variety of bioprocesses.
Advanced biological conversion processes (enzymatic, microbial and physical/chemical) are important parts of the bioprocessing research agenda (Wyman, 2006; Lynd etal., 2008). Research groups throughout the U.S. are working on the development and characterization of microorganisms capable of utilizing all sugars that are present in the pretreated slurry. Better conversion organisms can be obtained by designing and inserting multifunctional enzyme complexes (cellulosomes) into the target microorganisms, enabling a faster conversion of biomass into biofuels. The bioprocess that consists of inserting cellulases into fermentation microorganisms is named consolidated saccharification and fermentation and is a possible route to enhanced biofuels production. Simultaneous saccharification and fermentation refers to combining cellulose treatment with fermentation and is another route that could enhance biofuel production (Zhang etal., 2007). Also, work aimed at reducing the energy cost of biofuel recovery from the fermentation broth is under way (Kim and Dale, 2005).
In addition to the production of biofuels, biomass can be deconstructed and transformed into valuable coproducts (Rausch and Belyea, 2006). High value phytochemicals, etc. can be extracted from the biomass prior to the biofuel conversion step, adding value to the overall biorefinery operation (Vaughn, etal, 2008; Tanko etal., 2005; Walker etal., 2006; Walker, 2002; Wang and Weller, 2006; Zhang etal, 2005). Fermentation residues can be combusted or transformed into lignin based value-added products. Furthermore, biomass can be deconstructed into basic macromolecules and reassembled into industrial materials (Rausch and Belyea, 2006; Chen etal., 2005; Reddy and Yang, 2005; Srinivasan etal., 2006;).
Agriculturally based bio-industries are driven by the enabling technologies of genomics and proteomics. Through these technologies, plant genomes can be deciphered and manipulated to produce both quantitative and qualitative changes in the organic constituents of plant biomass. Genomics and proteomics have provided us with a greater understanding of gene regulation and control of plant metabolic pathways to the point we can engineer metabolic pathways with unprecedented efficiencies and reliability. The combination of this knowledge allows engineers and scientists to be more creative and efficient in the development of novel biocatalysts, biomass conversion processes, and bio-industrial systems. Molecular biology has provided engineers and scientists with a number of tools that enable protein engineering, so that a number of traits can be expressed in microorganisms and in plants. For example, bench scale technologies show that switchgrass and poplar can be transformed through molecular biology to release their cellulose and hemicelluloses without requiring harsh pretreatments. Better conversion organisms can be obtained by designing and inserting multifunctional enzyme complexes (cellulosomes) into the target microorganisms, enabling a faster conversion of biomass into biofuels. Oil seed crops, including camelina and algae, can be engineered to contain higher lipid concentrations, increasing biodiesel yields per acre (Christi, 2007). Other bioenergy production research priorities have included biodiesel (Hanna etal., 2005) anaerobic digestion (Zhang etal., 2006) and co-firing (Turn etal., 2005).
The production of non corn starch biofuels will likely require the implementation of diverse methods of production. One such method, as an alternative to the biochemical conversion route, is the thermochemical conversion approach (Wang, etal., 2008). The greatest advantage of thermochemical conversion is the robustness of the feedstock deconstruction operation. Essentially, biomass is gasified at extremely high temperatures, in the realm of 800 ºC; and the gas is converted via fermentation or catalysis to fuel. Biomass also can be pyrolyzed in an oxygen starved environment at moderate temperature to produce bio-oils, which can be upgraded and refined to liquid fuels (Mohan, etal., 2006). Specifically, gasification produces combustible gases, tar and char. The distribution of products depends on the feedstock composition, bed temperature, gasifying media and bed pressure. Gasification is not a new technology, having been employed for several decades (Reed, 1981; McKendry, 2002). Innovative research is underway that links gasification with microbial conversion processes or with catalysis-based processes (Abu El-Rub etal., 2004). Bio-oils from biomass pyrolysis are faced with problems associated with high acid numbers and char solids and viscosity increase during storage. Research for innovative catalytic pyrolysis and catalytic treatments of bio-oils is underway. These novel reaction systems are evolving in response to the heterogeneity of most biomass resources and the realization there are important autotrophic microorganisms that are effective in converting common bioorganic compounds into more useful industrial or biomedical compounds.
Advanced biological conversion processes (enzymatic, microbial and physical/chemical) are important parts of the bioprocessing research agenda. Biological and thermochemical processes are the preferred paths for converting agriculturally based resources into industrial products (Klass, 1998). Bioprocesses tend to have higher reaction specificity, have milder reaction conditions and produce fewer toxic byproducts. These characteristics are consistent with the goal of developing industrial processes and systems that are environmentally friendly. Land Grant institutions represent a repository of scientific and engineering knowledge that can be utilized to catalyze the transition of society from a fossil fuel to a biobased economy.
Our Land Grant institutions attract some of the best young minds in this country and from the world scientific community. We need to tap this resource and begin to develop its potential for creating new and innovative systems for producing biobased products. We need to provide these students with fundamental training in the natural sciences, and engineering. They must be taught to think critically about structure and function of biobased industries and how to make these industries sustainable.
Many recent federal, industrial, and academic studies have concluded the U.S. economy of the 21st century will be biobased. During the transition from a petroleum-based economy to a biobased economy, products and processes based on biological raw materials will replace those based on fossil fuels. Biorefineries will use many types of biomass sources and produce a broad range of carbon based products, energy fuels, oils, and biochemicals, as well as a variety of biomaterials.
The educational infrastructure needed to provide such training for the nascent green collar workforce is not available in conventional academic programs, which provide a narrow focus and do not encourage interactions among students from different departments. Innovative training programs are needed that minimize these barriers and provide more integrated programs. These programs must train students to communicate effectively, solve problems, and design processes in a multidisciplinary setting (Walker etal., 2007).
Because the participants in this regional effort are dispersed geographically, a distance learning platform for courses developed as part of this regional project is recommended. Internet compatible courses can serve students in virtually any location. It is anticipated that other distance education media such as satellite communication methods could be used. In instances where internet delivery is impractical (e.g., lab based courses), conventional, on site course delivery may be the best model. In either case, the technical content of such courses should be disseminated, if possible, via publications in pedagogical journals.
Related, Current and Previous Work
This Multi State Project builds on the accomplishments of the previous Multi-State Project S-1007. The significant accomplishments as a result of participation in S-1007 include:
1) Researchers associated with S-1007 have generated approximately 30 peer reviewed and other publications annually, including numerous book chapters and in at least one case a book (Drapcho, etal., 2008), either independently or in collaboration with other experiment stations represented in S-1007.
2) Approximately 12 collaborative proposals were developed among S-1007 members during the 2005-2006 period.
3) Several members participated in review panels for the FY2006 and FY2007 Joint USDA/DOE Biomass Initiative.
4) A majority of the S-1007 members conducted 27 site visits and evaluations of projects funded by the USDA/DOE Biomass Research Initiative in FY 2003 and FY 2004. Members conducted the visits in two person teams and generated evaluation reports.
5) Consultations with industry and presentations at bioenergy conferences and professional society meetings. It is anticipated that the proposed Multi State Project will generate similar activity.
SDC-325 is cognizant of the Multi-State Project SERA-38 Biobased energy research and information exchange committee, which is an extension and outreach committee aimed at complementing SDC-325. SDC-325 is also aware of the existence of NC-506 Sustainable biorefining systems for corn in the NC region, which is devoted to examining the sustainability of corn to ethanol operations. NC-506 is complementary to SDC-325 and little duplication is reported. A CRIS data search showed the projects Management of Grain Quality and Security for World Markets and Wood Utilization Research on US Biofuels, Bioproducts, Hybrid Biomaterials Composites Production, and Traditional Forest Products, which do not present significant overlap because this current project is not devoted solely either to corn or to forestry-derived feedstock. The project New Technologies for the Utilization of Textile Materials was located on the CRIS data search and some overlap is detected in Objective C Task 4.
Objectives
-
Reduce costs of harvesting, handling and transporting biomass to increase the competitiveness of biomass as a feedstock for biofuels, biomaterials and biochemicals
-
Improve biofuel production processes
-
Identify, develop and evaluate sustainable processes to convert biomass resources into biochemicals, biocatalysts and biomaterials
-
Identify and develop needed educational resources, develop distance based delivery methods, and develop a trained work force for the biobased economy
Methods
Methods The challenges posed by this new century in terms of energy and food will require advances in technology, sustainability and work force development. It is important to note that members of the SDC-325 project will formally collaborate and disseminate their results at the following professional meetings. SDC-325 members often interact at the American Society of Biological and Agricultural Engineers (ASABE) section meetings FPE-70, BE-28, PM-23/7/12 and T-11, Institute of Biological Engineering, American Institute of Chemical Engineers, American Oil Chemists Society, American Chemical Society and the National Biodiesel Board. As a result of this collaborative work, review articles will be prepared. A review article devoted to the biodiesel properties of novel feedstock combinations will be prepared by MI, NE and ND. Similar work will be prepared by NC on the use of novel feedstock, by KS on biomaterials and by AR, IL, IN and NE on coproduct extraction. This project is building on the research and findings of the S-1007 project, and the following Objectives are based on these findings. This multi-state project proposes the following four objectives: Objective A. Reduce costs of harvesting, handling, and transporting biomass to increase competitiveness of biomass as a feedstock for biofuels, biomaterials and biochemicals. Supplying abundant and inexpensive bio-feedstocks with predictable characteristics to biorefineries involves the entire supply chain. Reduction in accumulated costs depends on reductions in step-wise costs along the supply chain, and/or streamlining the supply chain. Biomass from agricultural and forestry sources is characterized by a high moisture content, low bulk density, and variable seasonal yields. Since this is a new industry, there is potential for reducing the costs as technologies are applied to both feedstock-specific applications and biomass in general. Task 1: Quantify and characterize biological feedstocks. (CA, FL, MT, OR, TN) Work in this area will seek to quantify and characterize biological feedstocks to be grown, produced, and converted to biofuels and value-added products. The approach includes overall agronomic evaluations, site-specific crop responses, and proven and innovative, rapid chemical analyses of feedstocks. Parallel studies are underway investigating short rotation trees. Microalgae, and food processing waste streams will be characterized. Field trials of potential energy crops, including variety comparisons and development of best practices will be developed. Productivity and quality of various prairie grasses and forage crops and of grass and legume mixtures harvested from CRP fields as potential biofuel feedstock will be evaluated. Improved methods for the quantification of feedstock constituents will be developed. Fourier transform spectral techniques to rapidly quantify biomass chemistry will continue to be developed. Collaborative efforts will include evaluation of CRP land for biofuels production by MT and ND. TX and KS are collaborating to develop new sorghum hybrids for ethanol production. Improved feedstock quality analysis shared protocols will be developed and shared among participants. Task 2: Develop and evaluate harvest, process and handling methods. (CA, GA, IL, IN, KY, MS, MT, TN, TX, VA, WI ) The overall objective will be to deliver premium quality feedstock at a low cost to bioconversion facilities. New techniques for harvesting, processing, and handling include goals of assessing both packaged and bulk methods of materials handling. Knowledge of the effect of moisture content on particle breakage, evolution and their granular properties, as well as the effect of particle granular properties on flow behavior will provide relevant information and design approaches to handling biomass feedstocks. A fundamental understanding of biomass feedstock preparation and their flow behaviors, as well as focus on the preparation of switchgrass as solid fuels for cofiring with coal or alone for power generation will be developed. Characterization of binding and agglomeration properties of biomass to reduce costs associated with transport and handling and on biomass size reduction and separation of plant botanical components will be undertaken. Round bale handling techniques for perennial grass biomass energy crops will be developed. Harvest, collection, and transport options of forest residues to estimate and reduce the delivered cost and energy consumption during the entire supply chain of forest-based biomass resources will be studied. IL will investigate crop production, harvesting, transportation, storage and informatics relating to biomass feedstock production in collaboration with UC-Berkeley and Lawrence Berkeley National Laboratory. TN is collaborating with TX for efficient handling of energy cane field and pre-processing operations. Task 3: Model and analyze integrated feedstock supply and process systems. (GA, IL, IN, NE, TN, TX) The overall objective is to develop and validate computational tools to simulate feedstock supply and process systems that enable accurate decision making for assembling and evaluating supply systems. Computer models and simulations that deliver results in the form of decision support and information tools relating to crop production, harvesting, transportation and storage of biomass crops from the farm-gate to the plant-gate will be assembled. NE will work on a bioresource map for the State of Nebraska for the site selection of new biorefineries. TN and SD are addressing feedstock logistic barriers associated with supplying warm season grasses in bulk format for biofuels. Objective B. Improve biofuel production processes B.1. Biological conversion processes Task 1: Develop pretreatment methods for biological conversion processes (AR, CA, FL, IN, KS, MI, NC, NE, OK, OR, SD, UT, WI) Different pretreatment technologies will be investigated, including dilute acid, alkaline, ionic liquids, water combined with carbon dioxide mixtures, pulsed electric field, extrusion and other high shear processing, hydrothermal, steam and ammonia explosion, organosolv, sulfite and microwave pretreatments. The pretreatment technologies will be optimized and engineering analyses of the processes will be conducted. Sources of biomass investigated by AR, CA, FL, IN, KS, MI, NC, NE, OK, SD, UT and WI will include energy crops, such as switchgrass, prairie cord grass, and coastal Bermuda grass, in addition to agricultural residues, such as corn and sorghum stover, rice and wheat straw, and food processing residuals. Pretreatment effect is evaluated using common analytical protocols, which were developed by DOE so that results from the members of this group can be compared across the different laboratories. Task 2: Develop conversion processes (CA, FL, KS, IL, NC, OK, OR, SC, SD, UT, WI) Conversion of straw and food processing residuals into, biogas will be investigated. Conversion of herbaceous and woody crops into ethanol will be studied. The use of thermostabile xylanase and cellulase and fermentation of the resulting sugars with thermotolerant yeasts and xylose fermenting yeast will be examined. The synergistic roles of different enzyme catalysts in biobased deconstruction processes will be studied. Thermotolerant yeast and a high solids bioreactor for simultaneous saccharification and fermentation will be developed. Biocatalysts for the conversion of cellulose and hemicellulose to automotive fuels will be invented. Algal production, harvest and conversion systems utilizing large-scale outdoor algal production with co-culture of filter-feeding aquatic organisms for converting algal biomass into feed protein and oils for feeds and biofuels will be designed. Task 3: Develop value-added products from hemicellulose and lignin (WI, NE) Hemicellulose and/or lignin fractions from cellulosic ethanol production and lignin-based chemicals and materials and/or applications of pentoses from hemicelluloses will be investigated. B.2. Thermochemical conversion processes Task 1: Develop pretreatment methods (GA, MN) GA will study the thermochemical conversion of biomass. MN will investigate pretreatment of feedstock prior to microwave pyrolysis. Task 2: Develop conversion processes (CA, FL, GA, IN, KS, OK, MN, NE, TN, TX,) Biomass gasification and hydrothermal pyrolysis processes work will be undertaken. Catalysts to deoxygenate biomass derived oxygenates will be developed. The use of steam-air fluidized bed gasification to produce syngas with higher hydrogen content and with maximum energy efficiency will be examined. Integration of biogasification and thermochemical conversion technologies for biogas production and subsequent conversion to synthesis gas and hydrogen will be studied Task 3: Improve methods for characterization of intermediate products and process control (IN, MN, MS, SD, TN, TX) TN will work on improvement of methods for characterization of intermediate products and process control using FTIR/NIR technology. IN, MS, SD and MN are developing knowledge on pyrolysis-derived bio-oil stability and uses, such as its application for road construction. MS and TX are developing gasification technology using common feedstocks. B.3. Biodiesel production processes Task 1: Characterize new feedstocks (AZ, GA, IN, MI, MT, ND, NE, SC, UT) Production of oilseed crops, such as camelina, canola and hazelnuts, in the High Plains will be investigated in MT, NE and ND. These and other fats and oils, including those from algae and oil from corn ethanol plants, will be characterized in AZ, GA, IN, MI, MT, ND, NE, SC and UT. Task 2: Develop an understanding of fuel quality and performance issues (IL, KS, MI, MT, ND, NE, SC, UT and VA ) ND and MI will study the storage stability and cold-weather performance of canola-based biodiesel and related fuel blends. NE will provide technical assistance on engine testing of biofuels and biofuel blends to all members for-fee. KS will develop permittivity sensors and NIR models for biodiesel fuel quality monitoring and control. MI, MT, ND, NE, SC, UT and VA will jointly study the storage stability and cold-weather performance of biodiesel derived from canola, camelina, hazelnut, algae, fungi and other lipid feedstock. Relevant ASTM analytical protocols will be shared and/or implemented among participants to enhance the research capabilities among participants in order to make results comparable across laboratories. Task 3: Develop and characterize innovative processes for biodiesel production (AZ, CA, FL, SD, MN, UT) SD will determine the most robust combination of lipase enzyme, carrier and immobilization techniques for transesterification of crude vegetable oil. Methods to intensify reaction kinetics of the transesterification process and speed up separation processes will be developed. Algae production with wastewater treatment and CO2 sequestration for a more sustainable process will be examined. MN will be working on catalyically assisted fast biodiesel production from algae and biodiesel byproducts as liquefying agents for bio-oil production. Task 4: Utilize coproducts (AZ, CA, FL, KS, MI, MN, MT, ND, TN, SC, VA) Conversion of crude glycerol into highly unsaturated fatty acids via algal and fungal culture will be undertaken. TN, FL and MI will work on the development of biofuels, biofuel additives and value-added products from glycerol. ND and MT will evaluate industrial uses of camelina and/or canola meals. AZ and SC will be using coproducts of algae biomass after oil extraction for aquaculture applications. Objective C. Identify, develop, and evaluate sustainable processes to convert biomass resources into biochemicals, biocatalysts, and biomaterials (non-fuel uses) Task 1: Discover and characterize biochemicals, biocatalysts, and biomaterials in biomass. (AR, FL, HI, IL, KS, KY, NC, ND, NE, OK, SC, SD, UT, VA, WA, WI) Research groups at AR, HI, IL, NE, SD, VA and WI will work on the extraction of essential oils/antioxidants, policosanol, algae-derived oils, corn by-products, nutraceuticals, and flavonoids, respectively, from energy-designated biomass. Research groups in AR, FL, HI, KY, NC, OK, SD and WA will compare the production of fungal, bacterial and archaeal enzymes by both native and recombinant organisms. HI will develop methodologies to identify, isolate, and characterize novel enzymes and proteins in plants, microorganisms, insects, and marine organisms via proteomics. KS and UT will focus on structural and functional properties of plant proteins and oils as well as from waste streams. NE will prepare value-added green chemicals from DDGS derived hemicellulose as well as develop and characterize nanocomposite biomaterials. LA, WA and KS are characterizing plant-derived proteins for further use in biobased products. SDC-325 members that have existing industry collaborations can introduce new members to industry, expanding the academic-industry partnerships. Task 2: Develop separation processes for biochemicals, biocatalysts, and biomaterials. (AR, GA, IN, KS, SD, UT, VA) AR and GA will work on extraction and separation of phytochemicals from biomass with subcritical water prior to the energy conversion. UT and VA share organism that will convert glycerol for production of omega-3 fatty acids and bioplastics, with specific efforts in downstream processing. IN will work on fractionation processes to refine methyl esters for value-added applications in construction. SD will work with industrial partners to develop membrane-based separation processes for biochemicals. KS will pursue work on utilization of DDGS for nutritional animal feed. Task 3: Develop applications for biochemicals and biocatalysts with biological activity. (AR, HI, MI, VA) AR, VA and HI will test the effect of energy crop-extracted phytochemicals in pharmaceutical and food applications. MI will develop nanostructured bioelectronic and biomimetic interfaces with proteins incorporated into interfaces containing either polyelectrolyte multilayers or tethered lipid bilayers. Task 4: Develop enabling technologies for biochemical production. (HI, IN, KS, LA, MI, ND, SD) SD and IN will work on the development of bioreactor technology. LA, KS and ND will work on the development of industrial adhesives, coatings and composites from plant proteins, oils and chemicals derived from biomass. HI will continue to develop technologies to harvest phytochemicals responsible for the production of aroma in selected plants. MI will work on the development of routes to chemicals from biorenewables using reactive distillation. Task 5: Develop and evaluate integrated process systems for commercial feasibility. (SD, UT) SD will develop an integrated biomass pretreatment and fractionation process to separate cellulose, hemicellulose, and lignin components. UT will work on integrating algal biomass production with municipal wastewater treatment and anaerobic digester processes for fuels and high value products. Objective D. Identify and develop needed educational resources, develop distance based delivery methods, and develop a trained work force for the biobased economy. Task 1: Serve as a knowledge resource base for biobased processing and products. This regional project will serve as an information and expertise clearinghouse for biomass-related knowledge and training by interfacing with organizations involved in research and development in the biobased economy, such as USDA, DOE, NSF, and higher education institutions. Multi-State participants will contribute to existing and future biomass-related information sites, such as the SunGrant Bioweb. Task 2: Distribute new knowledge to train the work force and general public in biobased products and processing. Tasks will include identifying key areas and audiences for which training materials are critically needed, coordinating experts to create training materials in key areas, fostering educational collaborations between experts having complimentary expertise, organizing workshop/training on effective delivery methods for distance education, and assisting in assessment and quality assurance of biomass-related training materials. KY is participating in a collaborative Higher Education Challenge Grant on Biofuels and Biorenwable Resources with IA and ID. SC is leading the NSF Division of Undergraduate Education Biosystems Technology ATE Project effort. Some Stations participating in this Multi-State Project will contribute to the KS-State Institute for Academic Alliances (IAA) effort, to develop a Graduate Certificate in Biobased Materials Sciences and Engineering. Task 3: Develop and disseminate educational materials in high-priority topic areas. New, multi-disciplinary, educational materials will be developed to widely disseminate knowledge related to biobased products via distance-education methods. Examples of relevant technical topics include fundamentals of biorenewable resources, an overview of biofuels, an overview of thermochemical processing, modeling of biological systems, sustainable chemical processing, bioseparations and bioprocess engineering and design. In addition, courses will be developed to teach professional skills of particular relevance, including effective methods for distance education, and skills for working in multidisciplinary teams. The use of distance-education technologies also will allow smaller programs to leverage resources in their training process. For instance, shared lecturing by area of expertise would enable collaborations to be conducted between experts, and expand the availability of expertise in this relatively new area. Significant milestones in the education subtask area will include recruiting experts (or teams) to develop educational materials in key areas, developing educational materials suitable for distance education, organizing peer-review assessment of educational materials, and establishing a distribution method for educational materials.Measurement of Progress and Results
Outputs
- A large portion of the efforts outlined in Objectives 1 through 4 are application oriented and will be useful to develop pilot projects, demonstrations and commercialization of biomass conversion to biobased products
- Other outputs include educational materials that could be used in traditional classroom settings or for distance education and web based distribution
- Publications in peer reviewed journals, trade journals and popular magazines.
- Development of intellectual property.
- Presentations to economic development groups, legislative groups, and to the general public.
- Output 6. Development of management recommendations for producers of biobased products.
Outcomes or Projected Impacts
- The committee has served and will continue to serve as a resource for: Bioresearch and Development Initiative (BRDI), Biomass, Research and Development Board working groups, SBIR panel Biofuels 8.8, USDA/DOE Biomass Initiative Project Review Teams, NRI 71.2 panel and reviewers for the Sun Grant Initiative.
- The multi-state membership will contribute to the implementation of the REE energy science strategic plan.
- Multi-state membership will contribute to identification of funding priorities and shaping policy of Federal agencies
- Research as a result of this project will create technology adopted by industry with at least two licensed technology per year.
- Research will enable reduced dependency on foreign-based fuels and chemicals.
Milestones
(2012): To design a economical and feasible feedstock handling process for a 5 million per year biofuels plant(2012): To produce biofuels that are competitive cost-wise with fossil based fuels
Projected Participation
View Appendix E: ParticipationOutreach Plan
Research results will be made available through peer reviewed journal articles. Participants in the previous Multi-State Project S-1007 generated approximately 30 peer reviewed and other publications annually, either independently or in collaboration with other experiment stations represented by S-1007. It is expected that similar productivity will be seen in this Multi-State Project. Many articles can be accessed through individual participant web sites.
Approximately 12 collaborative proposals were developed among S-1007 members during the 2005-2006 period. It is expected that this Multi-State Project will also foster scientific collaboration in terms of collaborative proposals.
Participants will meet once per year to review progress made towards attaining Objectives A, B, C and D. Because of the multi-disciplinary nature of this Multi-State Project, participants often meet in scientific conference settings, planning committees and in scientific review panels.
Organization/Governance
The Development Committee will use a standard form of governance using a chair, vice chair and secretary as executive officers. During annual meetings, a secretary will be elected that ascends to vice chair and chair. Terms are for one year. Subcommittees will be formed as needed during annual meetings.
Literature Cited
Abu El-Rub, Z., Bramer, E.A., and Brem, G. (2004). Review of catalysts for tar elimination in biomass gasification processes. Ind. Eng. Chem. Res. 43: 6911-6919.
Chen, Y., Chiparus, O., Sun, L., Negulescu, I.I., Parikh, D.V. and Calamari, T.A. (2005). Natural Fibers for Automotive Nonwoven Composites. Journal of Industrial Textiles. 35(1): 47-62.
Chisti, Y. (2007). Research review paper: Biodiesel from microalgae. Biotechnology Advances. 25: 294-306.
Corma, A., Iborra, S. and Velty, A. (2007). Chemical routes for the transformation of biomass into chemicals. Chem Rev. 107: 2411-2502.
Cundiff, J. and Grisso, R. (2008). Containerized handling to minimize hauling cost of herbaceous biomass. Biomass & Bioenergy. 32: 308-313.
Drapcho, C., Walker, T.H. and Nghiem, N. (2008). Bioprocess Engineering for Biofuels Production. McGraw-Hill, New York. ISBN: 0071487492.
Hanna, M.A., Isom, L. and Campbell, J. (2005). Biodiesel: Current Perspectives and Future. J. Scientific and Industrial Research. 64(11): 854-857.
Hoskinson, R., Karlen, D., Birrell, S., Radtke, C. and Wilhelm, W. (2007). Engineering nutrient removal, and feedstock conversion evaluations of our corn stover harvest scenarios. Biomass & Bioenergy. 31: 126-136.
Huber, G.W. (2007). Breaking the chemical and engineering barriers to lignocellulosic biofuels: Next generation hydrocarbon biorefineries. http://www.ecs.umass.edu/biofuels/Images/Roadmap2-08.pdf.
Kim, S. and Dale, B.E. Ethanol Fuels: E10 or E85 Life Cycle Perspectives,The International Journal of Life Cycle Assessment, (Online First, 1-5).
Klass, D. (1998). Biomass for Renewable Energy, Fuels, and Chemicals,Academic Press, 525 B Street, Suite 1900, San Diego, CA 92101-4495.
Kumar, A. and Sokhansanj, S. (2006). Switchgrass (Panicum vigratum, L.) delivery to a biorefinery using integrated biomass supply analysis and logistics (IBSAL) model. Bioresource Technology. 98: 1033-1044.
Lee, H., Clark, C. and Devereaux, C. (2008). Biofuels and sustainable development: Report of an executive session on the grand challenges of a sustainable transition. San Servolo Island, Venice, Italy. May 19-20: pp. 15.
Lynd, L., Laser, M., Bransby, D., Dale, B., Davison, B., Hamilton, R., Himmel, M., Keller, M., McMillian, J., Sheehan, J. and Wyman, C. (2008). How biotech can transform biofuels. Nature Biotechnology. 26(2): 169-172.
McKendry, P. (2002). Energy production from biomass (part 3): Gasification technologies. Bioresource Technology. 83: 55-63.
Mohan, D., Pittman Jr., C. and Steele, P. (2006). Pyrolysis of wood/biomass for bio-oil: A critical review. Energy & Fuels. 20: 848-889.
Mosier, N., Wyman, C., Dale, B., Elander, R., Lee, Y.Y., Holtzapple, M. and Ladisch, M. (2005). Features of promising technologies of pretreatment of lignocellulosic biomass. Bioresource Technology. 96(6):673-686.
Perlack, R., Wright, L., Turhollow, A., Graham, R., Stokes, B. and Erbach, D. (2005). Biomass as feedstock for a bioenergy and bioproducts industry: the technical feasibility of a billion-ton annual supply. Washington DC: U. S. Department of Energy and U. S. Department of Agriculture, Forest Service. 73 p.
Ragauskas, A., Williams, C., Davison, B., Britovsek, G., Cairney, J., Eckert, C., Frederick Jr., W., Hatlett, J., Leak, D., Liotta, C., Mielenz, J., Murphy, R., Templer, R. and Tschaplinski, T. (2006). The path forward for biofuels and biomaterials. Science. 311: 484-489.
Rausch, K. and Belyea, R. (2006). The future of coproducts from corn processing. Appl. Biochem. Biotech. 128: 47-86.
Reddy, N. and Yang, Y. (2005). Biofibers from agricultural byproducts for industrial applications. Trends in Biotechnology. 23(1):22-27.
Reed, T. (1981) Biomass Gasification Principles and Technology, Energy Technology Review 67: Noyes Data Corporation, Park Ridge, NJ.
Srinivasan, R., Singh, V., Belyea, R.L., Rausch, K.D., Moreau, R.A. and Tumbleson, M.E. (2006). Economics of fiber separation from distillers dried grains with solubles (DDGS) using sieving and elutriation. Cereal Chem. 83: 324-330.
Sun, Y. and Cheng, J. (2002). Hydrolysis of lignocellulosic materials for ethanol production: A review. Bioresource Technology.83: 1-11.
Tanko, H, Carrier, D.J., Duan, L. and Clausen, E. (2005).Pre and post harvesting processing of medicinal plants. Plant Genetic Resources. 3: 304-313.
Turn, S.Q., Jenkins, B.M., Jakeway, L.A., Blevins, L.G., Williams, R.B., Rubenstein, G. and Kinoshita, C.M. (2006). Test results from sugar cane bagasse and high fiber cane co-fired with fossil fuels. Biomass & Bioenergy. 30 (6): 565-574.
U.S. Department of Energy Office of the Biomass Program (U.S. DOE MYPP). (2008). Biomass Multi-Year Program Plan. Office of the Biomass Program. Energy Efficiency and Renewable Energy. U.S. Department of Energy.
Vaughn, K., McClain, C., Carrier, D.J., Wallace, S., King, J., Nagarajan, S. and Clausen, E. (2007). Effect of Albizia julibrissin water extracts on low-density lipoprotein oxidization. Journal of Agricultural and Food Chemistry. 55(12): 4704-4709.
Walker, T.H. (2002). Bioprocessing Technologies for Production of Nutraceuticals from Food and Agricultural Byproducts. Proceedings of the AIT International Conference on Innovations in Food Processing Technology and Engineering. Bangkok, TH.
Walker, T., Allen, W.H. and Drapcho, C.M. (2007). A Zero-Based Curriculum Revision in Biological Engineering: Challenges for a New Century, Int. J Eng Ed. (Vol. 22(5) Special Issue II: Curriculum Reform, Assessment and Accreditation; Computerised Simulation Tools).
Walker, T., Patel, P. and Cantrell, K.B. (2006). Supercritical fluid extraction and other technologies for extraction of high-value food processing co-products Chapter 10 in Waste management and co-product recovery in food processing, ed. Keith Waldron, Woodhead Publishing Lmt., London, UK.
Wang, L., Weller, C., Jones, D. and Hanna M. (2008). Contemporary issues in thermal gasification of biomass and its application to electricity and fuel production. Biomass & Bioenergy. 32: 573-581.
Wang, L.J. and Weller, C.L. (2006). Recent advances in extraction of natural products from plants. Trends in Food Science and Technology.17: 300-312.
Wyman, C. (2007). What is (and is not) vital to advancing cellulosic ethanol. TRENDS in Biotechnology. 25(4): 153-157.
Zhang, C., Medina-Bolivar, F., Buswell, S. and Cramer, C.L. (2005). Purification and stabilization of ricin B from tobacco hairy root culture medium by aqueous two phase extraction. Journal of Biotechnology. 117(1): 39-48.
Zhang, R.H., El-Mashed, H.M., Hartman, K., Wang, F., Liu, G., Choate, C. and Gamble, P. (2007). Characterization of Food Waste as Feedstock for Anaerobic Digestion. Bioresource Technology. 98(4): 929-935.
Zhang, Y.H.P., Himmel, M. and Mielenz, J. (2006). Outlook for cellulose improvement: Screening and selection strategies. Biotechnology Advances. 24: 452-481.