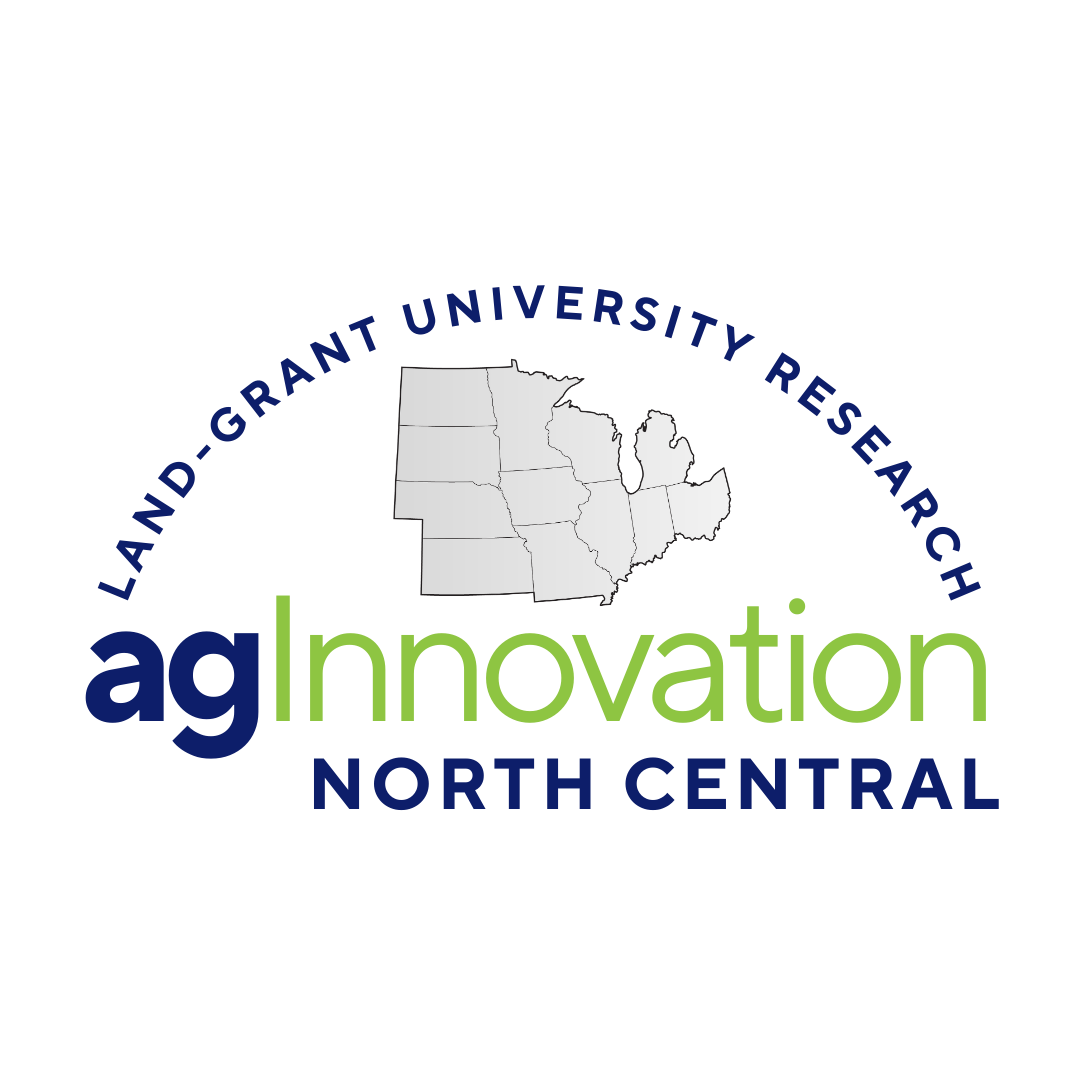
NC1040: Metabolic Relationships in Supply of Nutrients for Lactating Cows (NC-1009)
(Multistate Research Project)
Status: Inactive/Terminating
NC1040: Metabolic Relationships in Supply of Nutrients for Lactating Cows (NC-1009)
Duration: 10/01/2007 to 09/30/2013
Administrative Advisor(s):
NIFA Reps:
Non-Technical Summary
Statement of Issues and Justification
The US dairy industry is a major contributor to the diets of Americans and the economic viability of rural communities as well as many states. Our long-term goal is to improve the efficiency of milk production, cow health and longevity, and thus promote environmental and economic sustainability in the US dairy industry. Our approach to achieve this goal is to systematically identify those biological and nutritional management processes that will provide the greatest improvement and to concentrate our research efforts there. This is done partly through the construction, challenge and evaluation of computer-assisted bio-mathematical models that describe the metabolic relationships between feed inputs and milk outputs of cattle. We have chosen the term precision feeding systems in this revision to reflect the goal of being able to feed dairy cows that widely differ in genetics, environment and diets, not to reflect any particular methodology or feed delivery system. Although dairy producers, large and small, feed in a large variety of ways, a major goal is to be precise in meeting the needs of their cows. Improvement in animal and resource efficiency is slowed by a lack of clear research priorities addressing the most critical areas. This is due to a combination of factors, including: lack of quantitative data regarding absorbed nutrients and the metabolic responses of cows to those nutrients; a lack of integration of existing data into bio-mathematical models that will point out areas of greatest need; and a lack of real and enthusiastic support in the research and funding communities for cooperative, large-scale, integrated research work. In addition, integration of recent discoveries concerning genetic regulation, animal genotypes and phenotypes (genomics, gene arrays, proteomics, metabolomics), into traditional nutritional science in animal agriculture has been slow. Recent technological advancements and an appreciation for the importance of genotype in nutrient use by dairy nutritionists are now increasing the pace of that integration. This holds great promise for quantum improvements in efficiency (10 to 15 % on a herd basis as opposed to the traditional incremental increases). Our committee has addressed these lacks in knowledge steadfastly in the last 30 years, including the last 5, and the research and outreach done by this group continues to improve the understanding and efficiency of dairy production. The committee is comprised of some of the preeminent dairy scientists in the US with a broad base of specialties that encompass feed analysis; feeding management; ruminal microbial metabolism; intestinal digestion; physiology and metabolism of major organ systems; molecular and cellular biology; mathematical modeling; and the role of nutrition in health and longevity of animals. In the revision beginning in 1987, a conscious effort was made to plan and conduct experiments to provide data to improve research and practical nutritional models. Research done since then has come a long way to do that, however much remains to be done. For reasons given in the previous paragraph, improvements in complex research design, conduct and interpretation, including integrating information into model systems, has been slow. Yet this committee has continued to do excellent scientific work in practical and basic dairy nutrition. Notably, in the last few years has added several new young scientists with modern molecular biology skills as well as an appreciation for and experience in integrative biology and bio-mathematics. The committee is not dying off and wrapping up work, it is constantly changing and setting new goals, all under the umbrella of feeding and metabolism of dairy cattle. We have revised our title and our objectives to reflect the new knowledge that is available and the new research questions and field applications to address. Our first specific objective is to quantify properties of feeds that determine the availability and utilization of nutrients critical to milk production. Our second specific objective is to quantify metabolic and molecular interactions that alter synthesis of milk components. Our final objective is to use this knowledge of feed properties and metabolic and molecular quantitative relationships to challenge and refine precision feeding systems for dairy cattle. This committee has had a strong history in both basic biological research and practical application, and we intend to maintain that breadth. The overarching, ultimate goal is to do sound research directed toward finding out the most specific biological concepts and processes, and to apply that knowledge to the improvement of dairy cattle feeding in the practical work. Results from work done by this committee will be disseminated to practicing dairy nutritionists; veterinarians, extension specialists, farmers, and other scientists through regional nutrition conferences, trade and extension publications, electronic media, frequent national symposia (Hanigan et al, 2005), and applied computer ration balancing programs. Our work contributes to 1) improved accuracy of feeding standards for dairy cattle and future National Research Council publications on the nutrient requirements of dairy cattle, 2) standardization of analytical methods for feed evaluation, 3) reduced losses of nutrients to the environment from dairy cattle, 4) profitable and environmentally sustainable use of available feedstuffs, 5) continued expansion into new areas of genetics and nutrition and integrative biology and 6) continued supply of affordable, nutritious products for human consumption. JUSTIFICATION: The need as indicated by stakeholders. Over 55% of the calcium, 17% of the protein, and 15% of the energy in the US diet are supplied by dairy products; thus, the US consumer is a major stakeholder for the NC-1009 committee. Consumers want dairy products that are safe and inexpensive, but increasingly they also want an environmentally friendly dairy industry that promotes animal well-being. Recently, attention has been given to bioactive molecules in milk (in addition to Calcium) such as conjugated linoleic acids. Yet at its core the NC 1009 committee functions to do basic and applied research on the feeding and nutritional biology of dairy cattle. Major stakeholders include other scientists, practicing nutritionists, veterinarians, and farmers. The needs of these stakeholders have been addressed by the Food Animal Integrated Research group in the FAIR 2002 document. The goals of FAIR 2002 are to strengthen global competitiveness, enhance human nutrition, protect animal health, improve food safety and public health, ensure environmental quality, and promote animal well-being. Because feed inputs are a major determinant of milk yield, cow health, feed efficiency, profitability, and waste output, the work of the NC-1009 committee is critical for most of these goals. The importance of our work. Natural resources are used efficiently when milk production per unit feed and per cow is high (Baldwin, 1995; VandeHaar, 1998). To efficiently produce milk, a cow must have a well-developed mammary gland and be able to supply the gland with the nutrients it needs. Nutrition in the first year of life affects mammary gland development, and nutrition around the time of calving and throughout lactation has a major effect on the health, productivity, and efficiency of cows. Feeding for optimal nutrient intake requires not only the provision of the necessary nutrients for milk production but also consideration to the effects of diet on mammary capacity and on appetite, health, and metabolic regulation of the cow. Because feed costs account for half of all costs on a dairy farm, nutrition also significantly impacts farm expenses. The NC-1009 committee considers all of these factors for optimal feeding. For example, if we could maintain current milk production while feeding diets with 4 percentage units less total protein, we would decrease N losses to the environment in the US by 470,000 metric tons per year and save US dairy farmers $1 billion per year in feed costs. This type of progress only can be made if we take an integrated approach, with the use of mechanistic bio-mathematical models that accurately describe metabolism and production of cows. Technical feasibility. This committee has a record of making significant impacts in our knowledge of dairy cattle nutrition and metabolism and in the way that dairy cattle are fed and managed nationwide. We use the same approach that has proven effective in the past: that is to challenge and refine our models of dairy nutrition and metabolism. Computer-based, mechanistic, and quantitative metabolic models are useful in two ways: first, they help us determine critical needs in research and second they enable practical improvements in dairy cow feeding. Critical research needs are determined by using existing data from NC-1009 members or conducting new experiments to test model predictions of physiological responses to experimental diets. Examples of such responses include, rumen pH, microbial growth and function; alterations in gene expression and hormonal release of organs such as the adipose tissue; and alterations in milk fatty acid compositions. By challenging our working models in this way, we identify shortcomings that then become the basis for developing new testable hypotheses for further experimentation. Results from new experiments are incorporated into the models, and they are challenged again for further refinement. Thus, we continue to build our models so they are more mechanistic, quantitative, and accurate. These qualities enable us to improve practical feeding recommendations for dairy cattle in a variety of environmental and feeding conditions. Need for Cooperative Work. Important and complex problems require coordinated effort of many personnel. Considerable progress has been made in dairy nutrition, but practical problems remain and no single research group has the skills and resources needed to solve them alone. Only through cooperation can State Experiment Stations address the complex interactions among feed supply, nutrient use, genetic capability, and milk composition. Our committee is comprised of dairy scientists with a broad base of specialties that encompass feed analysis, feeding management, ruminal microbial metabolism, intestinal digestion, physiology and metabolism of splanchnic, adipose, muscle, and mammary tissues, endocrine regulation, molecular and cellular biology, and mathematical modeling. Furthermore, in testing and refining nutrition models for the whole country, we must consider the variation in forages and environment that exist among regions. Thus, we have scientists from every dairy region in the country. In addition, the explosion of new information in genomics, gene expression, gene array work, metabolomics and proteomics requires that we integrate this knowledge into our understanding of metabolic efficiency. Cooperation among stations is required to deal with this information and to solve problems, and will have a national impact in understanding the complex interrelationships of nutrient digestion and metabolism in lactating dairy cows and to apply this knowledge. Impacts on Science and Other Impacts. This project exemplifies the proven effectiveness of the cooperative regional approach. As detailed in the "Related Current and Previous Work" section below, results of this cooperative effort have become benchmarks of scientific progress and have led to practical feeding recommendations used worldwide. Project Leaders for the NC-185 regional project have received numerous awards for research, both basic and practical, from the American Dairy Science Association, the American Society of Animal Sciences, and industry groups. Most of the Project Leaders are in continuous demand as speakers for scientific and industry conferences in nutrition. The impact on basic and practical nutrition from Project Leaders has been profound in the areas of starch and protein chemistry and nutrition, feed processing, nutrient metabolism, and lactation biology. This group provided a major contribution to the 2001 version of the National Research Councils (NRC's) Nutrient Requirements of Dairy Cattle. Four of the 10 scientists on the NRC panel were from the NC-185 committee, and a significant portion of the data used in the latest edition came from NC-1009 committee members. In 2005, the group presented a symposium at ADSA/FASS on regulation of nutrient use in dairy cattle (references are in bibliography). Thus, this committee has had a major impact on improving the biological, economical, and environmental efficiency of the US dairy industry. We continue to recruit and support young scientists to keep the committee current and effective year to year.
Related, Current and Previous Work
General Introduction Cattle can be extremely efficient producers of quality human food. A major goal in feeding cattle is to find the optimal combination of chemical and physical properties of feeds that provides the proper amount and balance of absorbed nutrients to match the genotype of the cow or herd. This is a challenge because of the tremendous variety of feedstuffs available, the complexity of interactions among feed particles, nutrients and organisms in the rumen, genetic variance within and among herds, and the rapidly changing nutrient requirements of a cow around the time of parturition. The amount and profile of absorbed nutrients in dairy cattle are a function of rumen fermentation and intestinal digestion. Feed particles and microbes that escape the rumen can be digested in the small intestine to produce amino acids, monosaccharides, and lipids for absorption. The chemical and physical properties of feeds determine the availability of nutrients critical to the production of milk and milk components in a variety of ways. For example, the chemical composition (including total protein, nonprotein nitrogen, amino acid balance, organic acids, lipids, fiber, and non-fiber carbohydrate) dictates directly the availability of nutrients to support rumen microbial growth and the absorbed nutrients available to the animal to support milk synthesis. The physical properties of feeds, either inherent in the plant structure or altered by various processing methods, alters degradability in the rumen, and thus determines the proportion of feed fermented and used for rumen microbial growth and the proportion that passes to the small intestine. The cow is a fully integrated system (Baldwin, 1995; Hanigan et al., 2006; McNamara, 2006) in which one, even minor, change in nutrient input may lead to a variety of downstream events that alters function at the animal level. Since the last revision, we now understand more fully that this also includes changes in gene expression, and endocrine responses that were unknown or just discovered 5 to 10 years ago (IGF-1, leptin and ghrelin from the adipose tissue, for example). What follows is a brief summary of advancements since the last revision in 2002. Objective 1: To quantify properties of feeds that determine the availability of nutrients critical to milk production. Methodology Improvement Studies designed to test improved methodology have yielded improvement in isolation of ruminal protozoa. These organisms contribute significantly to the overall efficiency of the rumen, but techniques to study them have been limited. Improved isolation procedures will allow specific quantification of protozoa by real-time polymerase chain reaction, and improve prediction of rumen nitrogen flow. There appears to be minimal selective retention of protozoal genera between the rumen and omasum (i.e., the reticulum) in dairy cattle, supporting the use of omasal sampling for assessing microbial populations (Firkins et al., 2006). Dietary Carbohydrate Utilization Dietary carbohydrate fractions differ in the profiles of glucogenic and lipogenic metabolites they yield from ruminal and intestinal digestion. The amount and types of carbohydrates also impact rumen pH, which, in turn, alters fermentation and can alter the yield of nutrients, even amino acids, for absorption. Thus the various carbohydrate fractions have differential effects on the yield and composition of milk (Firkins et al. 2006; Hall, 2003, 2005). Recent improvements in methods will allow more accurate prediction of optimal amounts and ruminal availability of non-fiber carbohydrates for efficient production of milk and milk components (Hall, 2005). Rumen microbes can adapt to a number of feed types and feeding systems to increase digestibility in the rumen. Probes used include soluble sugars (sucrose, lactose), various fiber fractions, pectin and inulin. These studies continue to demonstrate the wide variability of the rumen ecosystem, increasing the need for a sophisticated bio-mathematical model to describe the rumen in a variety of situations (Firkins et al., 2006; Hanigan et al., 2006; Hall, 2005). Dietary Protein Utilization The amount and balance of absorbed amino acids also helps determine milk yield, not only milk protein synthesis. This availability of amino acids, in turn, is a function of the amount of feed protein which passes undegraded through the rumen and the amount of ruminally synthesized microbial protein that reaches the small intestine (Schwab et al. 2003). Because microbial protein has a better amino acid profile than many feed proteins, this remains an important area of study. Microbial protein yield is also a function of the amount and type of organic matter fermented (Firkins et al., 2006). Ruminal Lipid Metabolism The role of fatty acid intermediates in the rumen microbial biohydrogenation process continues to be defined. Work has demonstrated the benefits to dairy cattle efficiency, including reproductive efficiency, due to the type of fatty acids absorbed and metabolized (Mpapho et al., 2006; Qiu et al., 2004; Reveneau et al., 2005). Fat from various feedstuffs such as distillers grains, soybeans, and canola seeds influences the amount of trans fatty acids and conjugated linoleic acid (CLA) reaching the cows small intestine. The cis 9, trans 11 CLA has been shown to decrease tumor growth in animal models (Bassagany-Riera and Hontecillas, 2006). Consumer acceptance of high CLA cheese was comparable to that of cheese with lower CLA content (Khanal et al., 2003a, b). Determining optimal processing and inclusion rates of these fat sources will help increase CLA concentration in milk fat without significantly depressing fat synthesis in the mammary gland. The role of grazing fresh forages was studied to determine effects on milk CLA. Transition of cows to grazing requires more than 21 days to maximize CLA contents, but after returning to confinement feeding, CLA content decline to baseline within 8 days (Khanal et al., 2003a). Inclusion of ground canola seed in the diet can change concentrations of fatty acids isomers dramatically. Biohydrogenation (BH) intermediates that flow from the rumen influence fatty acid metabolism in the mammary gland, and work from MD has been instrumental in deriving this relationship compared with previous long-held views. Dietary factors that promote milk fat depression (high oil, high starch availability, and low rumen pH) tend to be correlated with situations in which rumen protozoa counts are decreased. Feeding Management Systems It is also critical to manage the feeding system to optimize intake through lactation and the dry period. An important time frame is the few weeks before and after calving. Substitution of digestible fiber for a portion of dietary starch improves dry matter intake during the first 90 days of lactation and milk yield after 90 days in milk (Ipharraguerre, et al., 2005a, b). Soyhulls could contribute satisfactorily up to 30% of the diet before limiting energy for milk production. Use of a wide variety of by-products and forage or grain processing methods has long been standard in the industry. While some of these have been characterized, there is still a paucity of information on their effects on ruminal digestion, absorption of nutrients and milk production efficiency. Work from the committee includes that on whole or delinted cottonseed, dried distillers grains and solubles, canola, soy, whey, sugars, glycerol from soy diesel production; steam-flaking, rolling, ensiling of forages and use of metabolic modifiers. Feeding of monensin, an ionophore, alters rumen microbial populations to improve efficiency of digestion. Feeding monensin prepartally had no effect on postpartal plasma amino acid concentrations (Williams et al., 2006). Physical form of the forage in a TMR continues to be important in the industry because we understand it has a tremendous effect on DMI and digestibility and thus ultimate efficiency. Length of chop, or particle size of forage particles is such an issue, with a wide variety of recommendations in the field. For corn silage and alfalfa haylage, the percent of DM above the 9 mm screen (on a Penn State Shaker Box) is highly correlated with the mean particle size (Armentano and Taysom, 2005). The same holds true for TMR, although the regression is different than it is for forages. The next smaller screen size (5.4 mm diagonal) also had a strong relationship. The present Molly model was constructed to include mean particle size of the diet, and this directly affects rumination and subsequent particle breakdown rate as well as rumen pH (Hanigan et al. 2006). Objective 2: To quantify metabolic interactions among nutrients that alter synthesis of milk. Synthesis of milk and milk components is a function of both the synthetic potential of the mammary gland and the supply of metabolites to the mammary gland. Supply of metabolites comes from dietary components, some of which are modified in other tissues, and from mobilization of body lipids and amino acids. There is an interaction between metabolism of body tissues, the supply of dietary nutrients and the milk production potential of the cow (as well as other animals) which has been recognized for quite some time in cattle and other animals (Baldwin, 1968; Kacser and Burns, 1979) which provide an extreme range of response of animals to even the same diet. New concepts on the interactions of nutrition and gene expression is exemplified with work at two stations: IN, at which new information on molecular control of expression of the enzymes that control gluconeogenesis in the liver are showing specific differences between the cow and other animals (Drackley et al., 2006; Velez and Donkin, 2005). At WA, work with supplemental chromium, a nutrient known to be required for many years, a positive feed intake and milk production response was obtained with supplemental chromium, along with a reduction in lipolysis and an increase in lipogenesis in adipose tissue, removing the negative effects of fatty acid mobilization on feed intake in early lactation (McNamara and Valdez, 2005). Several nutrients are now known to affect gene expression in several organs, which then alters the animal response to the diet or further changes in the diet. Newer additions to the committee (VT, MD, OH) as well as adapting previous members (IN, MI, AL, WA) have begun serious efforts in identifying genetic responses to diet and to lactation. This work falls presently into the basic aspect-providing hard data to other scientists and advanced professionals on the key interactions of genetics and diet. Major advancements have occurred in our knowledge of the interaction of metabolism and the endocrine system. Studies at AL, IN, and MI, in collaboration with other NC-1009 members, have illustrated the role of nutrition in the IGF-I system of dairy cattle (Donkin et al., 2005; Silva et al., 2002a, b; Vandehaar, 2005). Studies at IA have illustrated the role of glucagon in lipid metabolism and shown its potential benefit as a treatment for fatty liver (Bobe et al., 2003a, b, c). Work has been done on the role of leptin, which is known to alter feed intake, yet a clear understanding of the physiological role of leptin in lactation has been elusive (Silva et al., 2002a, b). A modern research front is in the genomics and phenotypic expression of key regulatory genes. Fatty acid synthesis and gluconeogenesis are critical processes in efficient dairy cattle. Genes that regulate glucose synthesis and urea cycle have been shown to be dependent on transcript variants in the dairy cow. The mechanism of control of two key enzymes, pyruvate carboxylase and phosphoenolpyruvate carboxykinase has been shown to be different for each one. Genomic DNA containing the bovine pyruvate carboxylase (PC) promoter has been cloned and the promoter identified (Rodriguez et al., 2005). This research opens up an entire new area of study to determine the genetic characteristics of the most efficient cattle. Dairy cows selected for high milk production have the ability to partition nutrients toward the mammary gland rather than to adipose tissue. Based on DNA microarray techniques, the quantitative expression of hundreds of genes was compared for MN dairy cattle selected for high milk production vs. those not selected (5500 kg/d milk production differential). Prolactin receptor in the liver had significantly greater expression in the first two weeks of lactation for the high genetic merit cattle. There was no difference between the ratio of two predominant receptor types, so they concluded that the receptors must be very sensitive to changes in blood prolactin concentration (Donkin et al., 2005). Another major variant in amino acid use is the use of amino acids by the muscle, which uses 2 to 3 times what even a very active mammary gland uses. The turnover of muscle proteins is critical to balancing out the needs of the mammary gland. AL is evaluating protein turnover in recently calved cows by use of proteomic studies and this has strong promise for identifying those cows most efficient at using amino acids for milk production. The regulation of milk fat composition is a continuing focus in dairy biology. Fatty acids such as docosahexaenoic acid and ecosapentaenoic acid have specific effects on inflammation and cellular development in a variety of species and organs. Supplementation of diets with docosahexaenoic acid (DHA) increased liver DHA concentration, regardless of ruminal protection, but also depressed dry matter intake (Donkin et al., 2005). Cows on pasture have increased conjugated linoleic acid (CLA) concentration in milk compared with feeding of conserved forages, whereas supplementation of extruded soybeans (rich in linoleic acid, the precursor to CLA) did not increase milk CLA further (Khanal et al., 2003a, b). When fed the same diets, Brown Swiss produced milk with higher CLA concentrations than did Holsteins or Jerseys. Ayrshires and Holsteins had higher CLA concentrations than did Guernseys and Jerseys. Sunflower and canola seeds are readily available oilseeds with potential to increase the concentration of CLA in milk. Both sources increased concentrations of CLA and one of its metabolic precursors, vaccenic acid, in milk. These results provide information on the improvement of healthfulness of milk for humans. Chromium supplied as chromium propionate (KemTRACE Cr® ;CrP) increased feed intake and milk production in dairy cattle (McNamara and Valdez, 2005) significantly when CrP was fed. A follow up study was done in heifers to help determine the mode of action for approval for use in ruminants. Chromium supplementation was associated with increased basal glucose and decreased basal insulin and non-esterified fatty acids (NEFA) in serum in a dose-dependent, quadratic manner (Sumner et al., 2005; McNamara, et al. 2007). Chromium increased glucose clearance rate as measured by half-life, time to nadir and area under the curve. Insulin concentrations tended to be lower in treated animals while clearance rates were unchanged. This study helps to confirm biological activity of supplementation of chromium in dairy animals, further work will define the role of altering chromium in the ration to improve energetic efficiency. Another promising method to improve lipid energy use is supplementation with rumen protected choline (RPC) or dry propylene glycol (PG). These two compounds did not significantly affect DMI, MY, milk composition, BCS, body weights or blood glucose concentrations in either the prepartum or postpartum period (Chung et al., 2006). Calf birth weights were also not affected by treatments. Based on results obtained in the present study, neither RPC nor dry PG appeared to affect intake, blood glucose concentration or production responses of multiparous periparturient Holstein dairy cows. In a second dose-response study, RPC appeared to have dose-dependent effects on reducing NEFA with 11% and 23 % reduction for cows provided 25 g and 50 g RPC vs. control, respectively. Several researchers are using a mix of in vitro and in vivo techniques to quantify fluxes of nutrients and important metabolites that influence the supply of nutrients to the mammary gland compared with other competing tissues. To study the disparity in the amount of amino acids that disappear from the small intestine and the net absorption measured by catheterization of the venous blood vessels, intestinal mucosa cells were incubated with radiolabeled glucose, glutamate, or glutamine (MD and USDA-BARC). Rumen epithelial cells metabolized a small amount of glucose in comparison with volatile fatty acids, lactate, and amino acids. About half of the glucose available from intestinal absorption or gluconeogenesis (glucose production) was used by the gastrointestinal tract in sheep. Growth of dairy heifers has a direct bearing on the efficiency and longevity of the animals as cows. Faster growth decreases feed and total costs of dairy heifer raising but also seems to decrease milk production capabilities of those heifers in subsequent lactation (Whitlock et al., 2002). Insulin-like growth factor I seems to increase, but leptin seems to decrease the proliferation of mammary parenchymal epithelial cells in prepubertal heifers. Feeding calves to gain about 700 grams/day from two to eight weeks of age enhanced mammary development. However, feeding for faster growth after weaning but before puberty increased deposition of mammary adipose tissue. Based on DNA microarray analyses of mammary gland biopsies, the hormone leptin was shown to counter-regulate insulin-like growth factor 1s ability to stimulate mammary cell growth through the expression of numerous genes. High energy diets fed to prepubertal heifers for longer durations result in a linear increase in leptin concentrations in serum and mammary tissue and leptin expression in mammary tissue (Silva et al., 2002a). Similar results were obtained with mammary tissue from heifers fed for 1200 vs 800 g/d from 4 months to puberty. Such data justified further in vivo studies to characterize the regulation of mammary development in heifers. Objective 3: To use these quantitative relationships to challenge and refine computer-based nutrition systems for dairy cattle. If we are to improve the accuracy and precision of predicting nutrient use, we must continue to improve mechanistic, dynamic models of metabolism. The newer Dairy Nutrient Requirements book (NRC, 2001) was based in large part on data from this committee. In the 5 years since its publication, it has gained great respect in the industry. However, the process of revision of this document, and the model within it, also pointed out many of the shortcomings in our current knowledge base. The new version is limited especially in predicting dietary nutrient interactions, which consequently hamper our ability to predict rumen microbial metabolism and microbial protein yield and therefore responses to rumen-undegraded protein, carbohydrate, and fat supplements. Other significant limitations are the ability to predict short term versus long-term nutritional responses and changes in body fat and protein use. More mechanistic modeling of the metabolism of the lactating dairy cow will allow for evaluation of these interactions. The most comprehensive mechanistic and dynamic model of metabolism in the dairy cow is called 'Molly', developed at CA with inputs from most NC-185 members (Baldwin, 1995). This model as well as other computer nutrition programs are soundly based on available data, yet all have weaknesses in the areas defined by Objectives 1 and 2. The rate of degradation of feedstuffs, the effect of various dietary carbohydrates on rumen fermentation and microbial protein synthesis, and quantitative data on metabolic interchanges among nutrients and body tissues limit the accuracy of these systems (Baldwin, 1995; Fadel et al., 2004, Johnson et al. 2007; McNamara, 2005, 2006; Hanigan et al, 2006). New collaborative efforts by the NC-1009 project are needed to remove these inaccuracies. Participants at WA utilized several data sets to demonstrate that understanding of adipose tissue metabolism as captured in the equations in the Molly model are correct (McNamara 2004, 2006). Work done by a newer member of the committee (Drackley et al., 2006) was used to challenge Molly for its description of energy use in the viscera. Visceral metabolism can account for the majority of maintenance requirements and can be highly variable. Errors in the model reflected a lack of knowledge of visceral metabolism in early and mid lactation. Using data generated (Donkin et al., 2006) we challenged and improved the descriptions of energy use in the model, further increasing its utility in research and application (McNamara, 2004, 2006). Also discovered during the course of this research was the newer information on the stoichiometric values for conversion of NADH and FADH to ATP in animal cells. Because the original model was constructed using parameter values of 3 ATP per NADH and 2 per FADH, this was leading to errors in describing energy use, even when the nutrient interconversions were correct. Implementing the concept of values of 2.5 and 1.5 instead, resulted in dramatic changes in several metabolic functions including weight gain (Fadel, personal communications). These improvements in description of energy use in Molly have gone a long way in improving not only its acceptance by other researcher, but its eventual adoption in more practical dairy nutrition fields. Participants from AL, CA, OH, PA, and VT have contributed data that has helped improve the parameterization of Molly. A sub-component of Molly to describe the net AA removal by the portal-drained viscera for subsequent field use has been developed. The lower bound for true digestibility of protein was determined to be 80%, but more research is needed to derive coefficients for essential AA. With prediction errors ranging from 4 to 9%, the current system now allows Molly to correct for metabolic losses in AA that are absorbed from the small intestine but not available for milk protein synthesis. Remaining limitations that this committee has been working on are in its ability to describe the rapid changes in nutrient use that occur in early lactation and in predicting physiological responses to high feed intakes or diets with atypical amino acid, fiber, or starch contents. The modeling work done spurred new research into getting those data. Molly was found to accurately predict N partition between excretion into urine, feces, and milk during short-term simulations (two weeks). The model was also able to predict the consequences of daily variation in dietary crude protein intake on milk and milk protein yield during short-term simulations (unpublished observations, CA, J. D. Heiskell). Quantitative data are still needed on the supply of milk component precursors available under different metabolic and nutritional conditions, such as early lactation. Data also are needed on the metabolic interconversions of nutrients, such as the use of amino acids for gluconeogenesis and thus milk lactose synthesis, and the partitioning of body fat and fat derived from the diet or lipogenesis for milk fat synthesis. These data will enable further refinement of current nutrition recommendations and aid in interpretation of feeding experiments.
Objectives
-
To quantify properties of feeds that determine the availability and utilization of nutrients critical to milk production.
-
To quantify metabolic and molecular interactions that alter synthesis of milk components.
-
To use this knowledge of feed properties and metabolic and molecular quantitative relationships to challenge and refine precision feeding systems for dairy cattle
Methods
Objective 1: To quantify properties of feeds that determine the availability and utilization of nutrients critical to milk production. Researchers at OH, WI, and PA will investigate feed characteristics that affect rumen microbial and protozoal nitrogen metabolism. One of the areas of effort will be to determine the effects of rumensin on these activities. Researchers at OH and PA will determine the effects of rumen pH on protein degradability in the rumen. The rumen pH affects bacterial and protozoal growth, protein passage and fiber digestibility, however, quantitative knowledge is still lacking, as methods to monitor pH accurately have only recently been developed. Work is designed in this area to quantify the effects of pH on several key ruminal processes, and that data will be used to improve our model predictions of nutrient digestibility and utilization. Ohio will evaluate the relationship between dietary factors that interact with microbial metabolism of fat, including starch availability in the rumen, forage particle size, and the presence of monensin. Based on cost projections, milk fat depression without a loss in milk volume can decrease profitability per cow by one-third. It would also help dairy farmers use greater amounts of byproducts from the bioenergy sector (such as corn distillers grains) more effectively and with less risk of milk fat depression. Trace milk fatty acids, derived from microbial synthesis, recently have been shown to have some diagnostic value in detecting volatile fatty acid patterns in the rumen and ruminal microbial protein yields. The purpose of this project is to quantify changes in microbial and milk fatty acids caused by dietary changes. Relationships with applied dietary changes will be important evaluation tools, while experiments with specific substrates will allow us to add a mechanistic interpretation that should improve the overall usefulness of the milk fatty acid information. Our end goal is to both evaluate and improve the utility of milk fatty acid analysis in interpreting results from experimental feeding trials and to introduce their use as a new troubleshooting tool for field dairy nutritionists working for commercial herds. Wisconsin will analyze samples for fatty acid composition from other collaborators as needed. Another novel effort will be a data mining exercise on relationships of branched chain milk fatty acids to determine what is happening in the rumen. Measuring ruminal events in real time is continually a challenge, and perhaps studying the present data base on these fatty acids of microbial origin may help us understand the changes in bacterial populations and activity that alter digestibility of feedstuffs. If such relationships are revealed, it may become an inexpensive way to increase our feeding systems to optimize bacterial fermentation over a range of situations. A similar effort will help to determine the effectiveness of standardization of fecal purines measurements as an estimate for microbial flows through the digestive tract. Integrating with members concentrating on Objective 3, the collaborators on this project will conduct studies to separate the bacterial and protozoal pools in the mechanistic model Molly. When this model was constructed 20 to 30 years ago (over that 10 year time span), protozoa were certainly known to be involved in ruminal digestion, but a severe lack of quantitative data precluded inclusion of specific pools of protozoa and their activity in the rumen model. Progress since then by several researchers (Firkens et al., 2006) has added to the knowledge base, and it is time to add this knowledge explicitly in the model. Researchers at MN will investigate several methods to manipulate microbial fermentation. In two sets of experiments, effects of Yucca Extract on microbial fermentation in a dual flow continuous culture system will be determined. The effect of yucca extract will be determined on protein degradation and ruminal pH. Effects of Saccahromyces cerevisae on pH, VFA and ammonia production will be studied in ruminal cannulated dairy cows. Minnesota will also conduct a dual flow continuous culture fermentation study with the aim of assessing differences in degradation pattern and ability to promote bacterial growth of the soluble protein fractions of two rapidly degraded protein sources: canola and soybean meal, and one slowly degraded protein source: fish meal. Collaborators at Ohio will expand their model measuring the transfers between N in ammonia pools to and from microbial nonammonia nitrogen pools. This information should help dairy producers reduce the amount of dietary protein with more confidence, reducing N elimination in dairy waste. We will further explain the role of rumen protozoa associated with microbial recycling in the rumen. By better characterizing the role of protozoa-bacteria interactions, we derive more precise equations to predict microbial protein flow to the duodenum, thereby improving efficiency of protein utilization. A novel and potentially far-reaching effort will begin with several members of this committee becoming collaborators and potentially members, of the new feed analysis consortium, presently called FeedAc (www.feedac.org). This organization was started after the completion of the last National Research Council Requirements of Dairy Cattle revision, by a consortium of several feed companies and private nutritionists to fill a large gap in feed data base information. Since the USDA ceased maintaining a feed data base, there has not been a central information location about feed data for agricultural animals. This remains a critical need to efficiently feed animals and use agronomic and energy resources wisely. If we do not have access to historical and recent feed chemistry and physical properties data, it becomes seriously problematic to precisely formulate rations. This knowledge is used by university scientists, private nutritionists and consultants, private industry and directly by some producers to design efficient rations. In addition, as cost of feed analysis continues to increase, and use of highly variable by products increases also, a central analytical and data storage organization becomes more important. Members of this committee will define which analyses will be done, which techniques will be used, and how information will be stored and disseminated. Funds have already been invested and will be invested by private industry, to support this endeavor. As appropriate, we will send feed samples from our research to be analyzed by techniques tested to be accurate and precise. This will allow huge leaps forward in research knowledge as lab-to-lab and university-to-university variation will decrease dramatically, increasing precision in feeding. Note that we will not be committing state or federal monies to support this group, nor using state or federal time. Instead, private industry will underwrite a large portion of the cost, and university committee members will be able to save significant dollars by using this facility for feed analysis. A direct benefit of this cost savings will be freeing up time and money to design more thorough and useful experiments. This is a novel effort whose final outcome is unknowable at this time. Yet, it has the potential to be a major new improvement in animal agricultural research. It truly meets the full spirit of regional, cooperative research and may solve one of the largest remaining problems in feeding agricultural animals, the lack of consistent analytical data for feed stuffs. In addition, it will serve Objective 3 well, as we will have excellent input data for all nutritional models, be they Molly, CPM Dairy (Cornell-Penn-Miner dairy nutrition program); or the NRC committees models. Objective 2: To quantify metabolic and molecular interactions that alter synthesis of milk components. We will use a nutri-genomics approach to identify key genes and metabolic pathways that regulate the partitioning of nutrients toward synthesis of milk protein, fat, and lactose in the mammary gland. Eventually, this information on gene expression can be combined with data on metabolite profiles and fluxes to identify novel key mechanisms influencing milk synthesis. Researchers at several stations (AL, IA, KS, IN, MI, SD, VA, WA) will, in several experiments, determine specific interactions among nutrients, hormones and physiological state that impact cow health and productivity. For example collaborative (IA, IN, SD, MI, WA) work will examine the effects of prepartal glucagon and glycerol on fatty liver, ketosis, postpartal health, and milk production. Liver will be assayed for expression of metabolic enzymes that control nutrient use Workers at WA, supported by a new NRI research grant, will be studying the role of expression of key genes in adipose tissue that regulate metabolic flux and thus milk production and efficiency. In conjunction with AL, we will take samples of muscle to determine the proteome of expression of key proteins in the muscle. We will study the effects of varied energy and protein on adipose gene expression and muscle proteome. These have direct effects on milk production, composition and thus feeding decisions. We will also directly study the variation due to genetic merit (as estimated by total production index and previous milk production) on expression of genes in adipose and muscle. Regulation of glucogenic and ureagenic genes is also important to the metabolic efficiency and health of cows. Workers in IA, SD, and KS will work to determine whether TNF± directly suppresses expression of gluconeogenic enzymes and to examine whether incidence of fatty liver in dairy cattle is associated with hepatic TNF± concentration. We will conduct specific studies that probe diet, stage of lactation and genetic merit to study specific regulatory factors (hormones, nutrients), and then integrate this knowledge with the work on adipose gene expression and muscle amino acid metabolism to build our quantitative metabolic map (MD, IN, MI, AL,VA). These metabolic patterns can be related to milk component production and expression, to help identify the most efficient patterns of metabolism in dairy cattle. This information will be used to identify the patterns of metabolism in the most efficient cattle, eventually to help select for the most efficient animals and feed them most efficiently, in other words, more precisely. Workers at KS will study the regulation of fatty acid oxidation to help determine their quantitative contribution to energy metabolism. In coordination with the studies listed above, samples will be taken to characterize the regulation of milk fat synthesis. For example, we will examine the effects of individual fatty acids on fatty acid synthesis in the mammary gland (MD). This will tie in with the work under Objective 1 as well. Work at Pennsylvania will evaluate glucogenic precursors for cows for improving cow health and performance. In addition, they will continue evaluation of ammonia emissions as affected by diet, housing and storage structures on dairy farms. Researchers at Maryland will concentrate on work funded by the USDA NRI within the Air Quality section. In collaboration with Ohio State, they will test the potential for improving N efficiency of the lactating cow by manipulation of RDP and total CP content in the diet. We are currently working to determine the effects of reduced RDP on microbial yields, ruminal digestion, urinary and fecal N output, and the ammonia volatilization rates from the manure in a controlled environment. We are planning to conduct another production study to assess the effects of 2 levels of RDP (at NRC requirement and a reduced level based on current experimentation) on ammonia emissions from the barn floor. We will conclude with a 9-month study to assess the effects of environmental factors on ammonia volatilization rates from the barn floor with cows on a single diet. This series of experiments will be used to refine current models of animal N metabolism and ammonia emissions from facilities. We are working to establish high-throughput methods of assessing metabolite concentrations in our laboratory (MD, VA). Nuclear Magnetic Resonance spectroscopy is being explored as a quantitative method to assess concentrations of a wide range of metabolites in blood and other biological fluids including tissue extra vascular space. We have collected spectra from plasma and are currently working on identification and quantification of peaks associated with the major metabolites, i.e. glucose, acetate, propionate, lactate, hydroxybutyrate, and amino acids. Software used in the human health field will be assessed for its ability to aid in quantifying gene expression in relation to metabolic flux. We have recently been granted approval to purchase a GC-MS which will complement the NMR work. These tools will help in the collection of a much greater volume of metabolite concentration data that can be paired with high-throughput gene expression data and be used to further progress in model development. We will continue to examine the molecular integration of signals controlling milk protein synthesis. Protein synthesis responds both to amino acid supply and to energy supply (either directly via metabolites or via endocrine signals). These divergent signals are apparently integrated via intracellular signaling pathways. In particular, it appears they converge at mTor, the mammalian target of rapamycin, this gene functions as a marker for translation initiation activation. The hypothesis is that the loss in milk protein yield associated with inadequate supplies of essential amino acids can be wholly or partially alleviated by increasing energy supply (or associated endocrine signals) to the mammary tissue. We are currently looking at the phosphorylation status of AKT, mTor, and 4-eBP1 in muscle and liver from calves subject to 4 different levels of energy and protein feeding. We have a production study planned to assess our treatment hypothesis at the whole animal level and plan to follow with an invasive experiment to assess mammary A-V differences and signaling protein phosphorylation via mammary biopsies. Researchers at SD will conduct experiments on the effect of omega-3 and -6 fatty acid containing feedstuffs on production response parameters in lactating dairy cows. Particular emphasis will be placed upon the influence of these fatty acids on milk composition. Transport of fatty acids in the blood, as well as partitioning of fatty acids to milk or body adipose tissues, will be examined. The effects of these dietary fatty acids on hepatic metabolism, gluconeogenesis, and endocrine regulation of hepatic metabolism in periparturient dairy cows will also be measured. Objective 3: To use this knowledge of feed properties and metabolic and molecular interactions quantitative relationships to challenge and refine precision feeding systems for dairy cattle. Work under this objective will integrate the new knowledge from the research experiments into the mechanistic model of dairy cattle digestion and metabolism. Major improvements in description of ruminal fiber and protein digestion; description of energy use in viscera; and descriptions of maintenance function have been made during the present project activity (2002-2007). Further improvements will continue to center around these critical areas, and expand out to include inclusion of specific amino acid use in the body and mammary gland. Absorption and metabolism of lysine, methionine, cysteine, histidine, branched chain amino acids (valine, leucine, isoleucine); phenylalanine and tyrosine will be included explicitly in the new version. Stations including CA, WA, AL and VA will collaborate on this model expansion. It is also our intention to begin a construction of a map of dairy cattle metabolism including gene expression, proteome and metabolomic levels. The present framework in Molly of aggregated pathway flux of protein and fat synthesis and breakdown in viscera, muscle and adipose tissues provides the framework. This validated functional description of metabolic flux will be the focus for integrating the key elements of metabolomics, proteomic, and gene expression data. Although the present model building is clearly for research purposes, long term application goals would include, for example, identifying the patterns of gene expression leading to the most efficient use of nutrients; or to identify specific dams and sires with desirable gene expression patterns to use in an advanced selection strategy. The present work on mapping the control of expression of gluconeogenic control enzymes in liver (IN) and gene expression analysis of lipogenic and lipolytic control enzymes in adipose tissue (WA, MI) are examples of the beginning of this long term process. Work at WA will focus on quantifying the contribution of metabolism and gene expression to the overall efficiency of the cow. We will determine molecular mechanisms of control of net lipolysis from the adipose tissue during the transition period of dairy cattle. We will probe the effects the transition to lactation, of genetic merit [based on sire and dam pedigree] and of dietary energy intake on control of lipogenesis and lipolysis via measuring expression of mRNA for several proteins. These include beta-adrenergic receptor subtypes (beta-1, beta-2, beta-3), hormone-sensitive lipase and the co-lipase, perilipin, insulin receptor, and the transporters GLUT1 and GLUT4. We will relate expression, as well as rates of lipogenesis and lipolysis measured in adipose tissue in vivo to DMI during the periparturient period. Data from this and other studies will be used to begin initial construction of a gene expression map, integrated into the existing metabolic flux model, following along the pattern of those made in simpler organisms. Obviously this will need to be done within each organ (liver, muscle, adipose, mammary), and then integrated into the animal model. In addition, we are building a model of nutrient use and reproductive function of the dairy cow, with the following goals and processes: 1. The goal is to construct and conduct an initial evaluation of a mechanistic, dynamic, computer-assisted model of nutrient metabolism and reproductive functions in the dairy cow; 2. This is considered a research model, along the lines of the nutrient portion is Molly. This is a dynamic (differential over time, pools can grow and shrink based on inputs and outputs (glucose, NEFA, adipose tissue, muscle, etc)) model that includes nutrients and elements of metabolic hormones. Researchers at California will collaborate with VA, WA and AKEY in model development and better partitioning of maintenance using new ATP yields. The idea here is to estimate maintenance based on datasets from private industry and CA. We will compare the three current iterative versions of the Molly (VA, WA, CA) to determine if the estimated maintenance is similar. New maintenance estimates along with the newer ATP yields should provide better models relative to energy utilization. Once the new maintenance levels are determined, further simulations would show how this impacts model outputs (with new ATP yields and new maintenance) when compared to just using the original models or using just the new ATP yields Experiments on changing N levels in the diet and subsequent flux chamber work at CA will be analyzed to test accuracy of ammonia emission predictions in current models. A better understanding of NH3 emissions will be possible if more data are available on the relationship between crude protein intake and the distribution of urea N in the milk and urine. This data will be useful in testing models for their accuracy in predicting urine urea and subsequent NH3 emissions. Workers at CA, WA, VA and AKEY will continue to work on modeling structures to improve optimization of the model. This is important because it will allow faster and more thorough testing of the effects of feeding practices, nutrient content of the feed and genetic efficiency. This will speed the outreach efforts to get research results, validated by thorough analyses, out to the industry clientele. Workers at WA, CA and VA will continue, update and expand several efforts to improve the model, integration of information, and outreach to the industry. We will complete development of the coding needed to account for trial effects for use in fitting Molly to the NRC database. We will then fit the digestive components of Molly to the entire NRC database that should improve predictions of nutrient supply to the model. The goal is to expand the past efforts of this group into a useful scientific description of the interactions of nutrient absorption, gene transcription and ultimate efficiency of dairy cows and improved precision of feeding them.Measurement of Progress and Results
Outputs
- Within three years of approval of this project, sufficient new quantitative data should be developed to allow for incorporation into the current metabolic models
- By year 5, refined models should be available and information on dietary effects on gene expression will be disseminated.
Outcomes or Projected Impacts
- Improved natural resource use efficiency
- Improved environmental impact, better utilization of N and less N waste
- Improved efficient use of bio-agriculture byproducts
- Major increase in grant support for dairy research
- Publication of a metabolic gene map integrating transcriptome, metabolomic and practical dairy outputs
- Refinement of the current nutrition programs will allow more exact formulation of diets for lactating dairy cattle. Feed costs and environmental impacts from excess dietary nutrients will be reduced for dairy farmers. Consumers will benefit from both reduced environmental impacts of food production and lower prices due to increased supply and lower input costs. Consumers will also benefit from possible alteration of the fatty acid content and composition of milk fat, resulting in a healthier diet. A more strict scientific understanding of the dairy cow will allow scientists to further refine and leverage research efforts to improve efficiency. We do not think it was due to chance that 4 of the members of this committee have been or are supported by NRI grants under the Project 42. Growth and development RFP, but because of the focus of our research on basic questions with practical applications.
Milestones
(2010): Within three years of approval of this project, sufficient new quantitative data should be developed to allow for incorporation into the current metabolic models(2012): By year 5, refined models should be available and information on dietary effects on gene expression will be disseminated.
Projected Participation
View Appendix E: ParticipationOutreach Plan
Outreach and Extension Plan: The outreach plan has two related goals: dissemination of novel research findings to a broader scientific audience, that encompasses many scientific disciplines; and dissemination of research findings of a more practical nature to audiences of producers, consultants, specialists and other allied industry leaders. This committee does cover a broad range of dairy research, and as such has many stakeholder groups. Nevertheless, individuals and collaborators in the group have targeted some very specific audiences for outreach and extension education.
For the first goal, it must be recognized that the committee is made up in large part of scientists conducting novel, fundamental basic research related to dairy cattle metabolism and nutrition. As such, we disseminate our research results at the American Dairy Science (FASS) meetings to other scientists and specialists. We also, as individuals, are invited to many national and international scientific conferences to present our research. As some examples, Dr. Gabriella Varga (PA) has spoken in the last few years at the Cornell, Intermountain and California nutrition conferences on use of dairy forages and the effects of rumensin. Dr. John McNamara (WA) has spoken at the California Nutrition Conference (03), the AMPA (Association Mexico de Production Animales) in 03, the PNW Nutrition Conference (05); and the EAAP Energy and Protein Conference in 2003 in Germany, the International Modeling in Animal Nutrition symposium in 2004 in The Netherlands, and is speaking at the Energy and Protein Conference in France in September of 2007. So is Dr. Brian Bequette (MD). Dr. Mary Beth Hall (USDA WI) has spoken at several conferences on dairy forages and rumen health over the last 5 years. In reality, there are very few committee members who have not had multiple invited talks since 2002.
As an example of a collaborative effort, in the last 13 years we have sponsored two symposia at ADSA/FASS meetings, one in 1993, and the latest in 2005. We planned and conducted a national symposium on the latest findings of our committee in conjunction with the American Dairy Science Association in 2006 (Donkin et al., 2006; Firkens et al., 2006; Hanigan et al., 2006). We intend to do this on a regular basis, likely every four to five years. In addition, we intend to work closely with the new FeedAC organization (www.feedac.org) to improve development and dissemination of good feed chemistry and utilization databases (details on this are in the proposal). We continue to publish more joint publications on our cooperative research, such as: Donkin, S., J. Knapp, M. VandeHaar, and B. Bequette. 2005. Regulation of key metabolic processes in lactation. JDS 88(Suppl.1):124; Firkins, J. L., A. N. Hristov, M. B. Hall, and G. A. Varga. 2006. Integration of ruminal metabolism in dairy cattle. J. Dairy Sci. 87-2172-2195; Hanigan, M. D., H. G. Bateman, J. G. Fadel, J. P. McNamara, and N. E. Smith. 2006. An ingredient-based input scheme for Molly. In: Nutrient digestion and utilization in farm animals: Modeling Approaches. ed. E. Kebreab, J. Dijkstra, A. Bannink, W. J. J. Gerrits, and J. France. CAB Publishing. p 328-348. Many of these publications are centered on improvements in the model discussed in the proposal.
The above type of education and outreach success can be measured by continual invitations to present at scientific meetings (as listed some examples above; the count of invited talks at meetings for the members of this group from 2002-2006 is over 60), publication in highly regarded scientific journals in biochemistry, physiology and genomics (Am J Physiology; Physiological Genomics; System & Applied Microbiology), metabolism (Endocrinology; J. Interferon Cytokine Research), nutrition (Journal of Nutrition; Animal Nutrition) and dairy and animal science (J. Dairy Science, J. Dairy Research, J. Anim. Sci, Anim. Feed Sci. Tech.) , and the continued success in obtaining research funding from private and public institutes. Four current members of this committee have earned extramural support just in the last 2 years from the USDA/CSREES National Research Initiative in Growth and Development (Project 42), a very competitive program. At least one, Shawn Donkin (IN) is presently funded by the NIH on basic metabolic regulation.
For the extension goal (dissemination of research findings of a more practical nature to audiences of producers, consultants, specialists and other allied industry leaders); each one of the collaborators on this project works in a state with extension faculty (the list includes ALL collaborators), and several collaborators also have an extension appointment (PA, MI, WI). Information from this committee has been and will continue to be disseminated to practicing dairy nutritionists, veterinarians, extension specialists, farmers, and other scientists through regional nutrition conferences, trade and extension publications, electronic media, and applied computer ration balancing programs. For example, in the Pacific Northwest, we have a tri-state Dairy Conference every other year, and a Pacific NW Nutrition Conference every year. On each program for the last many years has been one or more members of this committee, and the same is true for many other highly regarded nutrition conferences and short courses (Intermountain, Cornell, California, etc).
The members of this committee all spend considerable effort in a two-way discussion with our producers and allied industries to move forward in effective and meaningful research and application programs. At the aforementioned national, regional and local conferences, we will meet in groups and individually with producers, veterinarians, nutritional consultants and private industry scientists to discuss research results and plans. Producers and nutritionists will receive up to the minute information on the latest in practical nutritional management, through the use of extension bulletins, local and regional producer meetings, regional and national conferences, trade publications and personal conversations and correspondence.
Although the outreach and extension program is an important part of the project, the primary activity of most collaborators is in basic and systems research. The stated outcome of this basic research however includes one specific objective to improve and expand the development, refinement and use of nutritional decision support systems, or models, such as the National Research Council Nutrient requirements of Dairy Cattle, the CPM Dairy model, or the research model, Molly. This is truly the ultimate goal, that our research lead to specific, definable bio-mathematical equations that apply to the nutrition, metabolism, and thus efficiency and production of dairy cattle.
We will measure impacts of our outreach, education and extension program, not only in numbers and dollars of extramural funds and numbers of papers published and talks given, but in continued improvement and use of nutritional decision support systems that integrate our research findings. Some examples include basic research in the use of monensin to increase dairy efficiency, the use of chromium propionate to do the same, the better balancing of forage and concentrate ingredients through use of the NRC and CPM Dairy models, and better information on feeding transition cows to reduce metabolic diseases. Extension specialists and economists with more practical experience can argue about the numbers, but the track record of improving nutrient use efficiency and reduction of disease by members of this group could very conservatively be given a "floor price" of $0.25 per cow per day or a nationwide savings in resources of $730 million dollars per year.
Impacts and Benefits to stakeholder groups
Impact Statements:
Objective 1: To quantify properties of feeds that determine the availability of nutrients critical to milk production. The stakeholders of this objective will be nutritional consultants, industry specialists and producers. These impacts include management information usable today.
1. Grass hay may be included in lactating cow diets to maintain milk production and components with proper diet formulation, thus saving the cost and waste associated with higher nitrogen forages.
2. The impact of nitrogen in manure can be improved by increasing hindgut fermentation with more digestible forages.
3. The glycerol from soy biodiesel production may be fed to lactating dairy cows with no significant impact on production, up to about 2 kg/d, this may have a significant cost savings while capturing more value from bio-diesel production.
4. Wet distillers grains with solubles (DGS) may be fed at a rate up to 15% of diet dry matter with no significant impact on health, production, or composition of milk.
5. Supplemental methionine or methionine analog affects rumen bacteria differently than protozoa, which will affect the economics of feeding such products. Prior to a producer using these products, a nutritionist should be consulted to determine if there will be a significant economic return based on the diets currently being fed.
Objective 2: To quantify metabolic interactions among nutrients that alter synthesis of milk. The stakeholders of this objective will be other scientists in biology, dairy nutrition, private industry and advanced nutritional professionals. They will use this information to help explain the practical applications in the first objective, or to help provide practical suggestions for further research trials.
1. The industry"s ability to manage growth rates in developing heifers to minimize costs while maintaining future production potential has been increased. Calving heifers one month sooner saves approximately $900 per 30 heifers and increasing their size at breeding (at the same age) saves about $1600 per 30 heifers.
2. Mechanisms of conjugated linoleic acid (CLA) formation in milk were determined, improving biological and economical potential for CLA enhancement of milk to improve human health. If producers wish to increase the CLA content of milk, it can be done with a small cost. Application of this technology, however, awaits consumer demand of enhanced CLA milk.
3. Supplementing organic chromium, or with improving gluconeogenesis increased blood glucose, reduced non-esterified fatty acids, and thus improved feed intake and milk production, allowing nutritional management to help maximize the genetic potential of the cow. A two pound increase in the milk per day at peak, which is reasonable with the use of organic chromium or gluconeogenic products can return a net of $1400 for 80 cows.
4. Understanding the control of the genes in the liver that control glucose production is leading to management strategies that improve productivity, cow health and well being by reducing metabolic diseases.
Objective 3: To use these quantitative relationships to challenge and refine computer-based nutrition systems for dairy cattle. The stakeholders of this objective would also tend to be other scientists in biology, dairy nutrition, private industry and advanced nutritional professionals, and, importantly, members of this committee. They will use this information to help explain the practical applications in the first objective, or to help provide practical suggestions for further research trials.
1. Research models developed by this multistate research group more accurately describe and predict energy use by the cow. These models can now be coupled with genetics of the cow to identify the metabolic patterns that most efficiently convert feed to energy in an effort to genetically select for the most metabolic efficient animals.
2. A meta-analysis of literature data provided improve estimates for the value of various protein sources, which in practice can increase the flexibility and accuracy of feeding protein sources.
3. Accurate predictions of N partitioning to milk and urine by use of metabolic models has established a foundation for improving predictions of NH3 emissions from manure. This can lead to management decisions to alter dietary components to reduce excretion of N in manure and thus reduce the potential for environmental contamination.
4. Our improvement of existing models is leading to the next generation of models that integrate gene expression maps with our nutritional models to identify the most efficient animals on different diets, again, with the goal or more specific genetic selection that will improve biological and economical production efficiencies.
Organization/Governance
The technical committee will have a chair, secretary, and regional administrative advisor. The executive committee will consist of these three persons and the previous chair and will be the official nominating body. The chair and secretary will be elected by the voting members from within their ranks. The chair is responsible for planning and conducting the annual meeting, for submission of the project annual report, and for facilitating and ensuring effective communication and cooperation among participants. The secretary is responsible for recording minutes and distributing them prior to the chair preparing the annual report. Individual station members are responsible for preparing brief annual reports and distributing them to other participants two weeks prior to the annual meeting. Additional committees, composed of voting and non-voting members, may be appointed as needed to solve particular technical problems, to assist in communication within the project, or to report project findings to other interested parties.
Literature Cited
Arieli, A., C. M. Martinez, T. W. Cassidy, and G. A. Varga. 2006. Effects of concentration and duration of Rumensin application on milk production efficiency in multiparous Holstein cows. J. Dairy Sci. 89:126-133. Armentano, L. E. and Taysom, D. 2005 Short Communication: Prediction of Mean Baldwin, R. L. 1995. Modeling Ruminant Digestion and Metabolism. Chapman & Hall. Baldwin, R.L. Estimation of theoretical calorific relationships as a teaching technique: A review. 1968. J. Dairy Sci. 51:104-113. Bassaganya-Riera J, Hontecillas R. 2006. CLA and n-3 PUFA differentially modulate clinical activity and colonic PPAR-responsive gene expression in a pig model of experimental IBD. Clin Nutr. 2006 Jun;25(3):454-65. Bobe, E. G. Hammond, A. E. Freeman, G. L. Lindberg, and D. C. Beitz Texture of Butter from Cows with Different Milk Fatty Acid Compositions J Dairy Sci 2003 86: 3122-3127. Bobe, B. N. Ametaj, J. W. Young, and D. C. Beitz Effects of Exogenous Glucagon on Lipids in Lipoproteins and Liver of Lactating Dairy Cows. J Dairy Sci 2003a 86: 2895-2903. Bobe, B. N. Ametaj, J. W. Young, and D. C. Beitz Potential Treatment of Fatty Liver with 14-Day Subcutaneous Injections of Glucagon. J Dairy Sci 2003a 86: 3138-3147. Chung, Y. H., I. D. Girard, P. Cavassini and G. A. Varga. 2006. Effects of rumen protected choline and dry propylene glycol on production performance and blood metabolites of periparturient Holstein dairy cows. J. Dairy Sci. 89:199. Donkin, S., J. Knapp, M. VandeHaar, and B. Bequette. 2005. Regulation of key metabolic processes in lactation. JDS 88(Suppl.1):124. Drackley, J.K., S.S. Donkin, and C.K. Reynolds. 2006. Invited Review. Major advances in fundamental dairy cattle nutrition. J. Dairy Sci. 89:1324-1336. Fadel, J. G. 2004. Estimating parameters of non-linear segmented models. Journal of Dairy Science. 87:169173. Firkins, J. L., A. N. Hristov, M. B. Hall, and G. A. Varga. 2006. Integration of ruminal metabolism in dairy cattle. J. Dairy Sci. 87-2172-2195. Hall, M. B. 2003. Formulating for carbohydrates: from the ration to evaluation. Pages in 137-146 Proc. California Animal Nutrition Conference, Fresno, CA. Hall, M. B. 2005. The latest in carbohydrate nutrition & evaluating its impact on health and performance. In: Proc. Ruminant Health and Nutrition Conference, Syracuse, NY March 29, 2005. Hanigan, M. D., H. G. Bateman, J. G. Fadel, J. P. McNamara, and N. E. Smith. 2006. An ingredient-based input scheme for Molly. In: Nutrient digestion and utilization in farm animals: Modelling Approaches. ed. E. Kebreab, J. Dijkstra, A. Bannink, W. J. J. Gerrits, and J. France. CAB Publishing. p 328-348. Hanigan, M. D., H. G. Bateman, J. G. Fadel, J. P. McNamara. 2006. Metabolic models of ruminant metabolism: recent improvements and current status. Journal of Dairy Science. 89 (E. Suppl.): E52-E64. Ipharraguerre, I.R., and J.H. Clark. 2005. Varying Protein and Starch in the Diet of Dairy Cows. II. Effects on Performance and Nitrogen Utilization for Milk Production. J. Dairy Sci. 88:2556-2570. Ipharraguerre, I.R., J.H. Clark, and D.E. Freeman. 2005a. Rumen Fermentation and Intestinal Supply of Nutrients in Dairy Cows Fed Rumen-Protected Soy Products. J. Dairy Sci. 88:2879-2892. Ipharraguerre, I.R., J.H. Clark, and D.E. Freeman. 2005b. Varying Protein and Starch in the Diet of Dairy Cows. I. Effects on Ruminal Fermentation and Intestinal Supply of Nutrients. J. Dairy Sci. 88:2537-2555. Johnson, H. A. J. G. Fadel, and P. H. Robinson. 2006. Impact of variation in ration nutrient inputs on animal responses predicted by two dairy cattle metabolic models. The Professional Animal Scientist. (Submitted). Kacser, H. and J. A. Burns. 1979. Molecular democracy: Who shares the controls? Biochemical Reviews V 7: 1149-1160. Khanal, R. C., T. R. Dhiman, and R. L. Boman. 2003a. Influence of turning cows out to the pasture on fatty acid profile of milk. J. Dairy Sci. 86(Suppl. 1):356. Khanal, R. C., T. R. Dhiman, and R. L. Boman. 2003b. Influence of turning cows out to the pasture on fatty acid profile of milk. J. Dairy Sci. 86(Suppl. 1):356. Markantonatos, X., Y. Aharoni, T. Cassidy, R. K. McGuffey, L. F. Richardson, and G. A.Varga. 2006. A simulation model to integrate ruminal Volatile Fatty Acids (VFA) and blood glucose metabolism in transition dairy cows under steady state conditions. J. Dairy Sci. 89:72. McNamara, J. P. 2004. Research, improvement and application of mechanistic, biochemical, dynamic models of metabolism in lactating dairy cattle. Animal Feed Science & Technology 112:155-176. McNamara, J. P. 2006. Metabolic control: Improvement of a dynamic model of lactational metabolism in early lactation. International Workshop on Modelling Nutrient Utilization. Pp 349-365, Chapter 30 in Proceedings of 6th International workshop on modelling in farm animals. CAB International, UK. McNamara, J.P., Sumner, J. M., Valdez, F. and V. E. Sewalt. 2007. Effects of chromium propionate on response to an intravenous glucose tolerance test in growing Holstein heifers. J. Dairy Sci., submitted. McNamara, J.P., Valdez, F. 2005. Effects of dietary chromium propionate and calcium propionate on adipose tissue metabolism and milk production of dairy cattle in the transition period. J. Dairy Sci. 88: 2498-2507. Mpapho, G. S., A. R. Hippen, K. F. Kalscheur, D. J. Schingoethe 2006. Lactational performance of dairy cows fed wet corn distillers grains for the entire lactation. J. Dairy Sci. 89 (Suppl 1):1871 (abstr). New York. NRC (2001). Nutrient Requirements of Dairy Cattle, 7th revised edition. National Academy Press, Washington, DC. Particle Size and Proportion of Very Long Fiber Particles from Simplified Qiu, X., M.L. Eastridge, and J.L. Firkins. 2004. Effects of dry matter intake, addition of buffer, and source of fat on duodenal flow and concentration of conjugated linoleic acid and trans-11 C18:1 in milk. J. Dairy Sci. 87:4278-4286. Reveneau, C., C.V.D.M. Ribeiro, M.L. Eastridge, N.R. St-Pierre, and J.L. Firkins. 2005. Processing whole cottonseed moderates fatty acid metabolism and improves performance by dairy cows. J. Dairy Sci. 88:4432-4355. Rodriguez, S.M., C.A. Bidwell, and S.S. Donkin. 2005. Translational efficiency of bovine pyruvate carboxylase 5 untranslated region mRNA variants. FASEB J. 19:A317. Schwab, C. G., R. S. Ordway, and N. L. Whitehouse. 2003. Amino acid balancing in the context of MP and RUP requirements. Pages 25-34 in Proc. Four-State Applied Nutrition and Management Conf. Baraboo, WI. Silva, L. F. P., M. J. VandeHaar, M. S. Weber-Nielsen, and G. W. Smith. 2002a. Evidence for a local effect of leptin on bovine mammary gland. J. Dairy Sci. 85:3277-3286. Silva, L.F.P., M.J. VandeHaar, and M.S. Weber-Nielsen. 2002a. Intramammary infusion of IGF-I increases BrdU-labeling in mammary epithelial cells of prepubertal heifers. J. Anim. Sci. 80 (Suppl. 1):20. Silva, L.F.P., M.J. VandeHaar, B.K. Whitlock, R.P. Radcliff, and H.A. Tucker. 2002. Short communication: Relationship of body growth to mammary development in dairy heifers. J. Dairy Sci. 85: 2600-2602. Sumner, J. M. and J. P. McNamara. 2005. Effects of chromium propionate on response to an intravenous glucose tolerance test in growing Holstein heifers. J. Dairy Sci. 88(Suppl 1): VandeHaar MJ 2005 Regulation of nutrient partitioning: an overview of the hormones that are affected by nutrition or that directly alter metabolism. Pages 275-289 in Pond WG, Church DC, Pond KR, Schoknecht PA, Basic Animal Nutrition and Feeding, 5th ed., John Wiley & Sons, Inc., Hoboken, NJ. Velez, J.C. and S.S. Donkin. 2005. Feed restriction induces pyruvate carboxylase but not phosphoenolpyruvate carboxykinase in dairy cows. J. Dairy Sci. 88: 2938-2948. Whitlock, B.K., M.J. VandeHaar, and H.A. Tucker. 2002. Effect of dietary protein on prepubertal mammary development in rapidly growing dairy heifers. J. Dairy Sci. 85:1516-1525. Williams, E.L., M. M. Pickett, G. A. Varga, and S. S. Donkin. 2006. Effect of Dietary Carbohydrate and Monensin on Expression of Gluconeogenic Enzymes in Liver of Transition Dairy Cows. J. Anim. Sci. (In press)