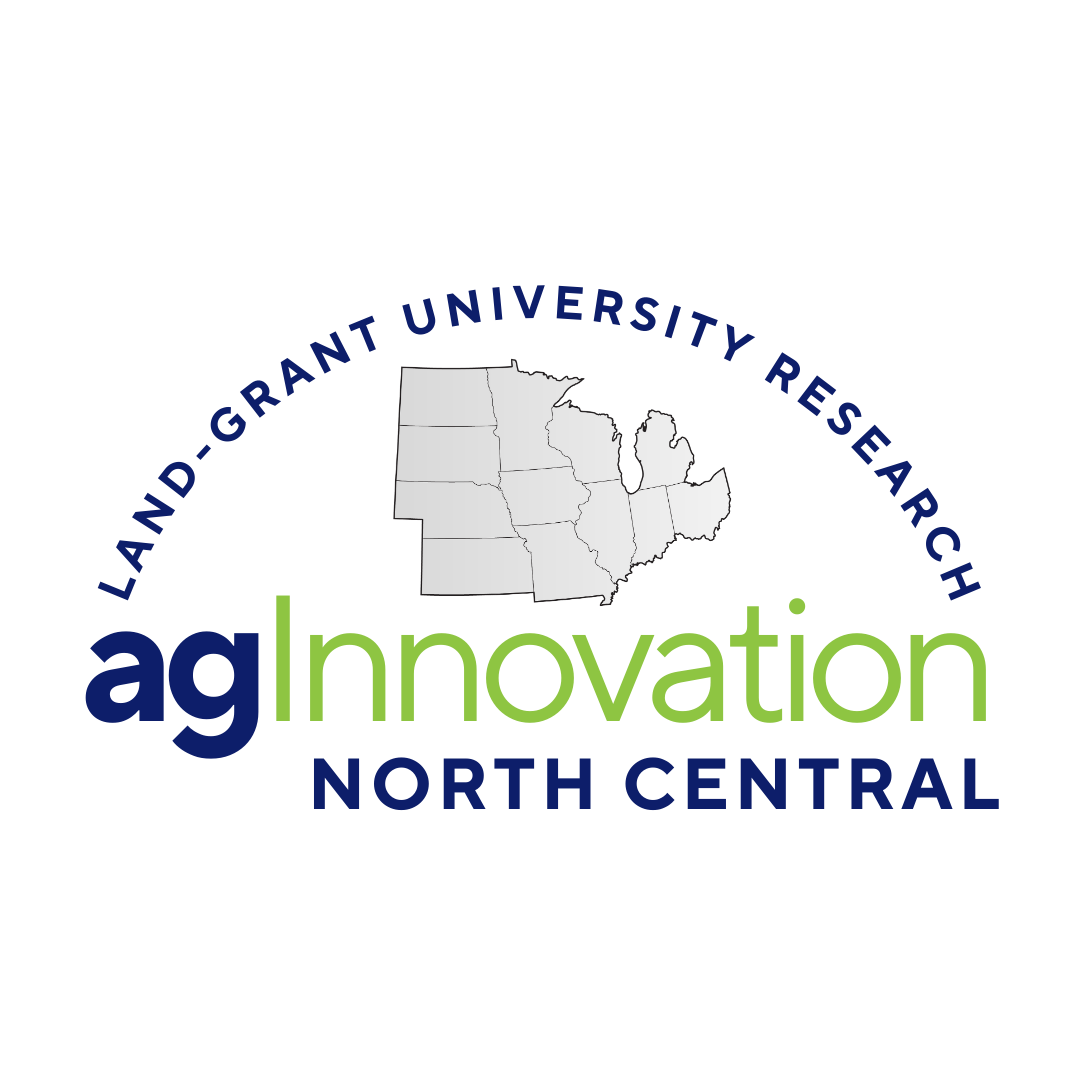
NC_OLD205: Ecology and Management of European Corn Borer and Other Stalk-Boring Lepidoptera
(Multistate Research Project)
Status: Inactive/Terminating
NC_OLD205: Ecology and Management of European Corn Borer and Other Stalk-Boring Lepidoptera
Duration: 10/01/2000 to 09/30/2005
Administrative Advisor(s):
NIFA Reps:
Non-Technical Summary
Statement of Issues and Justification
More than 80 million acres of field corn (Zea mays L.), worth more than $20 billion, is annually grown for grain in the United States. European corn borer [Ostrinia nubilalis (H|bner)] alone, among several stalk-boring pests, accounts for more than $1.85 billion in control costs and grain losses to corn growers each year. This commodity provides feed for livestock and is used as a source of raw products in the production of foods and fuels. Second only to the corn rootworm (Diabrotica spp.) in economic importance on all types of corn, European corn borer also attacks several other important crops, namely, sorghum, small grains, cotton, potatoes, snap beans, peppers, and soybeans.
The development and commercialization of Bacillus thuringiensis (Bt) transgenic corn in the past few years, specifically targeted for European corn borer, has greatly changed the paradigm for managing corn pests. One of the most significant concerns associated with this new technology is the possibility of insects developing resistance to Bt. Resistance development could threaten the future use of Bt crops, not only as a highly effective pest management alternative but also as a biological insecticide for conventional insect control. With transgenic Bt corn arising as a totally different integrated pest management (IPM) strategy, there is a critical need for new information relevant to use of this tactic. Although pertinent data applicable to pest management of stalk-boring insects has been acquired through this regional project in the past, this new paradigm has created a demand for basic economic, biological, and ecological information for European corn borer and other associated pests. This information is needed to develop models to predict resistance development and strategies for the management of resistance. Also, questions and concerns have recently surfaced on the impact of transgenic crops on beneficial organisms, such as natural enemies, and nontarget insects, including the monarch [Danaus plexippus (L.)].
We propose to address significant insect management issues associated with the adoption of transgenic crops and approaches to resistance management, especially the requirement for economic assessments, the need for more basic biological and ecological information, and the demand for information on natural enemies and other nontarget organisms. The goal of the project is to develop information leading to enhanced IPM options for corn production that economically benefit producers while minimizing environmental impact.
JUSTIFICATION:
Stalk-boring insects form a complex of pests that significantly reduce yields in field corn and other crops. European corn borer infests more than 200 species of plants, including peppers, snap beans, potatoes, sorghum, cotton, small grains, soybeans, apples, and onions (Mason et al. 1996). Other significant stalk-boring pests include stalk borer [Papaipema nebris (Guenie)], hop vine borer [Hydraecia immanis (Guenie)], potato stem borer [Hydraecia micacea (Esper)], and southwestern corn borer (Diatraea grandiosella Dyar).
Of these stalk-boring species, European corn borer has the greatest impact on corn production in North America, causing $1.85 billion in crop loss annually (Calvin 1995, Ostlie et al. 1997; this figure was modified from the cited publications by using current yields and price structures). In Iowa, a major corn-producing state, losses from European corn borer injury were estimated at 5% when current control methods (insecticides) were applied and 12.4% when no insecticides were applied. Annually, this pest costs Iowa farmers between $15 to $50/acre, even when insecticides are used.
Prior to 1996, management of European corn borer was limited to the use of rescue treatments with insecticides when other tactics (cultural, genetic, and biological) failed to maintain populations below economic levels. The use of insecticides to protect the yield of corn was only cost-effective in a few cornfields, with the highest levels of insecticide use occurring in the western Corn Belt and mid-Atlantic states where corn frequently experiences environmental stress. In many corn production areas, losses from European corn borer were accepted by growers because insecticide applications did not provide long enough residual control to be cost-effective, scouting to properly time applications was difficult and time-consuming, and improper timing of applications resulted in reduced efficacy of the products (Calvin et al. 1988, Bode and Calvin 1990).
With the introduction of transgenic corn hybrids containing the gene that enables corn plants to manufacture the crystalline protein toxin of the bacterium B. thuringiensis subsp. kurstaki, a new highly effective and relatively cheap control alternative for European corn borer was made available to corn producers. This technology lowered the economic threshold for the pest, increasing the percentage of fields that would benefit from European corn borer control from ~12 to 80% (Calvin 1995). For the first time, farmers possessed the technological capability to protect 95 to 100% of the 6 to 7% yield loss (on average) that had been caused by this pest. Because the toxins in the corn plant provide season-long protection against the pest, this technology also eliminated the requirement to scout fields or to use predictive models to optimally time insecticide applications.
During 1999, ~25% of all corn hybrids planted in the Unites States contained a Bt toxin gene (P. Davis, personal communication). Seed technology companies project that adoption levels may reach 50% by early in the next millennium. In addition, these companies are developing hybrids with stacked genes possessing multiple modes of action and that can control several species of pests. These new hybrids may enter commercial markets within the next 5 years. There is potential to select for evolution of resistance to these crystalline protein toxins in European corn borer populations because farmers can now justify treating up to 80% of their corn acreage.
Research conducted by this committee has been used to develop models predicting the rates of resistance evolution and to investigate the role of refuge structure in preventing or minimizing resistance evolution. These models indicate that a minimum refuge size of 20% is required to slow resistance development in this species. Although these models were constructed based on the best information available on the pest's biology and known population genetic relationships, a number of assumptions about pest biology were required for the simulations to be completed. In addition to addressing information gaps needed to improve these models, information is needed on nontarget impacts of Bt corn toxins. Eliminating information gaps forms the basis for several objectives of the project.
Economics. As noted, economic damage resulting from European corn borer infestations totals more than $1.85 billion in yield loss and control costs each year in the United States (Calvin 1995, Ostlie et al. 1997). Many new economic issues have arisen because of the commercial introduction of Bt corn. These issues include technology adoption, cost differences between alternative refuge configurations, and a desire to identify economic incentives that encourage growers to comply with refuge requirements. Farmers, as business owners, will make these decisions by balancing expected costs and benefits. However, because European corn borer behavior and agricultural practices differ across the Corn Belt, regional analyses are required to obtain a complete understanding of producer behavior, the factors that drive this behavior, and the policies that may modify this behavior in positive ways.
Ecology and Genetics. During the past 10 to 15 years, U.S. agriculture has been developing and implementing transgenic corn varieties that express genes originating from B. thuringiensis, alternative cropping practices, biological control, and landscape-level planning to achieve effective insect pest management. These developments are transforming management strategies of stalk-boring pests of corn. Region wide research and extension efforts are necessary if benefits are to be optimized. The dramatic management of insects via transgenic plants, however, has many scientists (and growers) concerned about high selection pressure associated with broad exposure to these toxins and the subsequent adaptation by pest insects (Gould 1988a, b; Mason et al. 1996). There is a continuing need for research to address resistance problems in stalk-boring Lepidoptera (Gould 1989) and for outreach to transfer research results to the public. There is a need to balance the desire for maintaining long-term durability of this technology with logistical and economic short-term expectations for effective and uniform management.
Resistance management for transgenic corn depends on a refuge strategy complemented by high expression of Bt protein in the plant (EPA 1998). However, there is disagreement concerning the size and placement of non-Bt refuges. Current risk assessment models have been based on incomplete biological information, particularly on dispersal and genetics, perhaps leading to unnecessary constraints as to how corn growers are permitted to use this technology. New biological information will improve the accuracy and precision of resistance management models. Improved models will allow us to investigate the consequences of reducing refuge size and of optimizing refuge placement across variable agricultural landscapes. It may be possible for dense sacrificial plantings of popcorn or nearby plantings of millets to serve as a non-Bt corn source of susceptibility genes because the insects developing within these crops probably escaped B. thuringiensis selection pressure.
European corn borer is not a single, randomly mating population even though it occurs throughout North America east of the Rockies. Clarification of population structure and genetics is necessary to model the likelihood of resistance development and to design resistance management strategies. More information for this species is needed on geographic patterns of genetic variation, voltinism, pheromone blend, sensitivity to B. thuringiensis, and the influence of host plants on genetics and population structure to develop spatial-temporal models of gene flow within an agricultural landscape. Also, little is known of the population structure and genetics of other stalk-boring insects. Acquiring such information will increase our understanding of the spectrum and mechanisms of resistance, genetic basis for resistance, status of cross-resistance, and stability of resistance.
Natural Enemies. Enhancing natural control is the first line of protection in IPM. Even though the basic biology of most of the natural enemies associated with corn has been described, the effects and value of these natural enemies in various corn cropping systems and landscapes is not well enough understood to reliably manage based on knowledge of natural controls. Key information gaps include understanding patterns of variation in natural enemy communities associated with landscapes dominated by corn and more diverse landscapes. Gaps extend to adequately quantifying the role of natural enemies in resistance evolution, improving the use of augmentative biological control agents, and characterizing the economic value of natural enemies in contemporary cropping systems.
We hypothesize that 1) natural enemy abundance and diversity may be greater in landscapes where European corn borer has historically not been a consistent significant pest compared with landscapes where it has been a pest, and 2) with wide-scale adoption of Bt corn, natural enemies that are specialized on either European corn borer or southwestern corn borer will become less abundant. Some predators and host-specific parasitoids may have such a limited food source that only those with superior host-finding behavior will persist in landscapes dominated by Bt corn.
Nontarget Effects. Management strategies with Bt corn to control European corn borer and other stalk-boring insects may have direct and indirect effects on nontarget pests, and other organisms that could result in positive or negative impacts (Ostlie et al. 1997, Schuler et al. 1999). Secondary corn pests, such as corn earworm [Helicoverpa zea (Boddie)], fall armyworm [Spodoptera frugiperda (J.E. Smith)], dusky sap beetle (Carpophilus lugubris Murray), western bean cutworm [Richia albicosta (Smith)], Banks grass mite (Oligonychus pratensis Banks, and twospotted spider mite (Tetranychus urticae Kock) are either tolerant to or not affected by the endotoxins expressed in Bt corn. With the exception of spider mites, these organisms may have reached greater economic status where Bt corn culture has resulted in reduced use of broad-spectrum insecticides (Horner et al. 1998, Dively et al. 1999). These nontarget pests require a new set of pest management practices that will need to be compatible with strategies used to delay European corn borer resistance to transgenic corn. Bt corn also may have nontarget impacts on European corn borer and other lepidopterans in noncorn host plants grown near cornfields (Losey et al. 1999). The exceptional efficacy of Bt corn may suppress local populations on diversified farms where other crops are grown that share European corn borer and corn earworm as major pests. Because most commercial Bt corn hybrids express the endotoxin in the pollen, it is possible that wind-dispersed pollen may accumulate on noncorn host plants of nontarget Lepidoptera. A regional effort among participating states is essential to provide policymakers, interest groups, and the public with unbiased, scientifically based information on the actual exposure risk of nontarget lepidopterans to Bt pollen.
IPM Education, Policy, and Regulation. Growers rely on a variety of sources for making decisions regarding implementation of traditional and transgenic pest management technologies. During the next 5 years, we expect that single management traits, such as Bt resistance to European corn borer, will no longer be available as stand-alone options. Instead, genes for European corn borer management will be stacked with other pest management and enhanced quality traits (e.g., herbicide tolerance, corn rootworm resistance, high oil or other nutritional factors). To help growers integrate these inputs and options into practical and implementable pest management programs, the results of this project must be made available in a timely fashion for use by policymakers and be packaged as unbiased recommendations for the agricultural and public sectors. It is also important to obtain feedback from growers about their constraints and willingness to adopt resistance management practices as part of their European corn borer suppression program. This input is needed to balance short-term logistical and economic expectations for effective management with the desire to maintain long-term durability of the transgenic technology.
Overall. Collectively, a multistate approach to researching these knowledge gaps and implementing effective technology transfer strategies is appropriate and necessary. Lack of knowledge has led to fears by the general public about the potential environmental and health risks associated with broad adoption of new technologies. The recent controversy about nontarget effect of the technology, particularly the potential effect of Bt corn pollen on monarch populations, has further fueled public concerns. These fears have the potential of forcing legislation to ban or slow the introduction of genetically modified organisms. Answers to questions regarding Bt corn impacts (if any) on these nontarget organisms should help focus the public's perception of this technology and, where benefits are clearly demonstrated, allow farmers to gain the dramatic pest control advantages provided by this and future technologies.
These multistate plans will be a paradigm for the development of science-based resistance management programs for other pests, other crops, and future crop protection technologies. Our efforts will provide fundamental advances in the knowledge of pest ecology, genetics, and evolution. Our work will continue to provide scientifically based assessments essential to the policy decision-making process and should help to increase the public's acceptance of these safe technologies and to identify potential negative impacts that need further investigation. We also view it as part of our responsibility to provide unbiased, scientifically based information that fosters subsequent investment in promising novel approaches to pest management. There is ample evidence that the NC-205 research group has the skills, collaborative working relationships, and commitment to provide the missing biological information and to incorporate this new information into current resistance management models.
Related, Current and Previous Work
To our knowledge, the only work with a suite of stalk-boring Lepidoptera in corn systems is that being reported by NC-205 members. With the various sustainable agriculture efforts (based largely on principles of IPM, there may be a few investigations with one or two insects, but we know of no efforts currently engaged in a regionally coordinated effort focused on the genetics, voltinism, host range, natural enemies, and management of the stalk-boring insect complex. NE-124, Genetic Manipulation of Sweet Corn Quality and Stress Resistance, has little overlap with NC-205. Five regional projects currently involve biological control of insects: W-185, Biological Control in Pest Management Systems in Plants; S-265, Development and Integration of Entomopathogens into Pest Management Systems; S-267, Biological Control of Selected Arthropod Pests and Weeds; SRDC-99-06, Development and Evaluation of Entomopathogens and Their Toxins for Control of Insect Pests; and NCR-125, Arthropod Biological Control. None of these projects deal with the entire array of natural enemies (i.e., predators, parasites, and diseases) in corn for even a single insect herbivore. Our committee has established excellent cooperative interactions with these biological control committees. NC-205 will continue to deal specifically with natural enemies of stalk-boring Lepidoptera and to coordinate efforts with other regional projects investigating biological control.
NCR-46 is a multistate committee focused on the biology and control of corn rootworms. We have held overlapping meetings with this committee and industry for the past several years to share ideas in the rapidly developing area of transgenic corn. Transgenic corn is expected to become commercially available for corn rootworm control during the next couple of years and it will be essential for us to coordinate activities with NCR-46 members. Farmers will need consistent recommendations from both groups. Several members of NC-205 attend NCR-46 meetings regularly, so effective coordination is already the norm. We suggest, however, that during the next 5 years that the two committees remain separate, in part because of the aboveground versus belowground distinctions and the massive quantities of information that each group considers annually. We will investigate the possibility of combining the committees during this proposed project cycle.
We know of no other coordinated research program that is focusing on the genetic differences among pest insect populations with taxonomic, chemical, and functional traits of resistance management. NC-94 is developing a multistate spatial analysis system of thermal unit accumulation reporting, which we may find useful in areawide management of European corn borer. Similarly, the role of weather in long-range movement of insects (NCR-148, NC-226) is of interest to NC-205 members studying management tactics in Lepidoptera.
Although some research on the economics of the value of Bt corn has been performed, this work has either been deterministic (Calvin 1995) or focused on a single, specific location (Hyde et al. 1998, Onstad and Guse 1998). The economic evaluation of insect resistance management has been limited. Hueth and Regev (1974), Taylor and Headley (1975), and Regev et al. (1976, 1983) each investigated the optimal use of synthetic pesticides with increasing resistance. The objective of our work is to understand how managing resistance can boost agricultural productivity. Although this early body of literature offers important insight into the problem of managing insect resistance to transgenic crops such as Bt corn, additional work is needed to help guide current regulatory policy. Economists and entomologists working with NC-205 have extended this earlier work focusing on the use of refuge to manage European corn borer resistance to Bt corn (Hurley et al. 1999a, b). The results of this effort are simulation models that help evaluate the merits and deficiencies of alternative refuge scenarios based on economic and ecological indicators. Further work is currently under way to assess the economics of alternative refuge configurations.
Growers rely on pesticides, fertilizers, and now transgenic crops to manage risks (Ostlie et al. 1997). In practice, growers apply extra inputs to reduce risks, rather than testing or scouting to determine actual input needs. For example, growers may prefer to use a soil-applied insecticide for corn rootworms when they do not know whether rootworms will even be a problem. This prophylactic application of insecticide is mainly for peace of mind. The Agricultural Conservation Innovation Center and Agren, Inc., are developing crop insurance as a tool to promote the wise use of biologically modified plants and IPM practices. This type of crop insurance reduces the perceived economic risks of growers by pooling risks from crop loss over several growers.
The concept of using insurance to reduce the number of insecticide applications for corn insects was proposed by Turpin (1977). The use of insurance as an economic tool to increase non-Bt corn refuge acres on farms with Bt corn is a new concept. Currently, growers have little economic incentive to plant the recommended acreages of non-Bt corn refuge. Therefore, getting growers to adopt refuge acres on a wide scale is a difficult issue to resolve with Bt corn. Insuring refuge corn acres could level the economic playing field with Bt corn. B. thuringiensis technology protects the corn crop from physical damage by European corn borer. Similarly, the non-Bt refuge corn crop could be protected financially with an insurance policy that indemnifies the producer for actual damage due to European corn borer. With insurance, growers in compliance with Bt refuge recommendations would not be at an economic disadvantage to noncompliant growers. This insurance policy could provide an economic incentive to growers to use refuge acres correctly.
Information on adult European corn borer dispersal, particularly of unmated females, is critical to predicting mixing between possible B. thuringiensis-resistant and -susceptible adults (from refuge), to estimating gene flow between these two populations, and to refuge design and placement. In 1996 and 1997, mark-recapture studies examined local dispersal of bivoltine European corn borers from release sites within cornfields (Hunt 1999). Two important findings were that corn phenology affects adult European corn borer dispersal and that unmated female European corn borers do not disperse far before they mate. Specifically, taller corn (1st flight of European corn borers) and early reproductive-stage corn (2nd flight) reduced mean dispersal distance, and 95% of all recaptured, unmated female European corn borers were trapped within ~500 m of their release site. Trapping began at the field edge, so although this information gives a general idea of where mating occurs, it lacks precision and does not provide information on movement and behavior within the field. Therefore, studies conducted in 1998 examined within-field dispersal of adult European corn borers, focusing on unmated females (Hunt 1999). Within-field dispersal was significantly different between European corn borers released in irrigated, high plant-density corn compared with those released in nonirrigated, lower plant-density corn. The irrigated, high plant-density fields had higher recapture rates, particularly near the release sites. Seventy-five percent of the unmated females were recaptured at traps nearest their release site, indicating these adults moved very little upon emergence. Few unmated European corn borers were recaptured within nonirrigated, lower plant-density corn. The effects of irrigation and plant density could not be separated in this study.
Bt corn is generally considered to have little or no impact on nontarget organisms (Orr and Landis 1997, Ostlie et al. 1997, Pilcher et al. 1997). Other studies have shown that Bt cotton and potato have no significant effect on beneficial insects or nontarget soil organisms, and pose less risk to the environment than conventional spraying with pesticides (Sims 1995, Dogan et al. 1996, Donegan et al. 1996, Yu et al. 1997, Riddick and Barbosa 1998, Riddick et al.1998). However, the novel genetic composition of these crops makes their impact on nontargets difficult to predict. To date, only a few studies have reported potential negative impacts on nontargets. Immature lacewings, Chrysoperla carnea (Hillbeck et al. 1998), and the monarch (Losey et al. 1999) may be susceptible to endotoxins expressed in Bt corn; however, these results are based on no-choice laboratory assays in which insects were exposed to high levels of transgenic tissues. It is generally agreed that the expressed toxins in pollen may represent a risk to nontargets. Most commercial Bt corn hybrids express the toxin in pollen (Fearing et al. 1997), and corn pollen is dispersed at least 60 m (Raynor et al. 1972) and possibly more than 200 m by the wind (Louette 1995). However, the potential risks must be put into proper perspective and balanced with the positive impacts of Bt corn. Currently, NC-205 members are cooperating with industry on studies addressing the monarch issue; however, a multistate effort is essential for collaboration and sharing of ideas and information.
Objectives
-
Our ultimate goal is to develop management practices for European corn borer and related stalk-boring pests that are environmentally sensitive, ecologically compatible, and economically sustainable. Our five specific objectives are designed to provide critical information needed to accomplish this goal with multistate cooperation and group validation. Several investigations are intended to be repeated over years with the experimental design modified based on findings we acquire during our on-going studies.
-
Analyze the economics of corn borer management practices.
-
Assess ecological and genetic factors that impact resistance management.
-
Evaluate the role of natural enemies of lepidopteran pests in corn-cropping systems and agricultural landscapes.
-
Assess impact of management strategies for stalk-boring insects on nontarget organisms.
-
Develop and disseminate information for use by IPM educators, policymakers, and regulators.
Methods
(Objective 1). Analyze the economics of corn borer management practicesEconomic analyses will identify factors affecting the adoption of Bt corn, develop an economic model for managing insect resistance to transgenic corn, and determine the feasibility of using crop insurance as a vehicle to increase refuge compliance. Identifying the expected economic incentives for both the adoption of Bt corn and the selection of alternative refuge programs is essential for predicting the likely behavior of producers. Due to regional differences in European corn borer pressure, cultural practices, and the presence of other pests (e.g., southwestern corn borer in southwestern states such as Kansas), a regional evaluation approach will be used.
Entomologists from Illinois will create a detailed biological simulation model of the population dynamics and genetics of the southwestern corn borer to evaluate resistance management and the economics of transgenic corn. Data for this model will come from Texas, Kansas, and Missouri. The southwestern corn borer model also will be combined with the Illinois and other European corn borer models to better understand how to simultaneously manage these two pests. This model will provide critical information to the other economic models regarding the efficacy of alternative refuge configurations and sizes.
Economists from Indiana will use dynamic programming models to determine the value of Bt corn under various growing conditions (planting dates, number of European corn borer generations and European corn borers per plant). They also will evaluate crop budgets to determine practical refuge configuration options, including sacrificial refuges. These budgets will assume a 20% refuge and will examine costs associated with labor requirements for planting the refuge, yield losses due to planting delays, and potential additional harvesting or drying costs. The sensitivity of incentive levels to field size also will be evaluated to identify whether the technology is biased towards larger (>1,000 acres) or smaller farms (<500 acres), or whether it is scale neutral. These analyses of near-term adoption decisions will rely on European corn borer behavioral data from several Corn Belt states, including Illinois, Indiana, Iowa, Kansas, Minnesota, and Nebraska.
Economists from Minnesota will work with entomologists from Iowa, Kansas, Minnesota, and Pennsylvania to develop a model describing Bt corn adoption and conventional pesticide application rates with crop budgets and information on technology fees, Bt corn adoption, and European corn borer pressure over the commercial life of the technology. The mathematical behavioral model will be integrated into a bioeconomic simulation model that compares resistance management plans based on economic, ecological, and environmental indicators such as the value of agricultural production, probability of resistance developing, and frequency of conventional pesticide applications. This model will be used to evaluate the long-term impacts of resistance management plans.
Entomologists from Iowa, Nebraska, Kansas, and Pennsylvania will work with economists from Iowa and Minnesota to evaluate the concept of using crop insurance to increase grower refuge compliance. European corn borer injury and corn-yield databases will be used to determine frequency, severity, and range of European corn borer damage and its correlation to yield loss. These data will be used to develop insurance actuarial tables and to determine whether insuring refuge corn is a viable concept. The Kansas information is important because areas infested with southwestern corn borer often have serious losses due to this pest (Whitworth et al. 1984). Per-acre insurance premiums will be estimated based on the actuarial tables.
(Objective 2). Assess ecological and genetic factors that impact resistance management
Ecological Factors. Population models have been developed in Illinois and Minnesota to predict the potential evolution of resistance and to investigate alternative resistance management strategies. Due to information gaps, these models have relied on numerous assumptions about pest biology. Key information gaps include a lack of understanding about the factors that influence adult and larval dispersal and the effects of alternative hosts on European corn borer life history characteristics. Research on these topics will provide information needed to improve the realism of these models.
Mark-recapture methods will be used to examine the temporal and spatial dispersal patterns of European corn borer adults. Nebraska, Iowa, Pennsylvania, and Delaware will release a minimum of 1,000 marked males at various distances up to 600 m from the virgin females placed within a Bt cornfield. Virgin females will be put in cages mounted on sticky traps in the same cornfield. Males released at each distance will be marked with a different colored dye. Arrival of marked males will be recorded daily. A minimum of four releases will be made in each state per year. Additional mark-recapture studies will be conducted in Nebraska and Kansas to determine the importance of irrigation, plant density, and plant phenology on adult movement. A minimum of 2,000 males and females will be released within irrigated and nonirrigated cornfields. Light traps will be placed in transects beginning at the release point (near the middle of the field) and at increasing distances to the field edge. Number, sex, and female mating status of marked adults will be recorded for each trap. These releases will be repeated for vegetative and reproductive corn and a minimum of two plant densities.
Iowa and Kansas will plant adjacent blocks of Bt (minimum of 12 rows) and non-Bt corn (four to six rows) to study movement of older larvae (European corn borer only in Iowa, European corn borer and southwestern corn borer in Kansas) from non-Bt to Bt corn plantings. A minimum of seven transects, each with six sampling points (one, two, three, four, five, or six rows from the non-Bt cornfield edge) will be sampled. Density of larvae in the non-Bt plants will be controlled by varying infestation rates. These rates will be verified by sampling 12 plants in each of the non-Bt blocks. Plants within the Bt block, along the transects, will be evaluated for evidence of larval feeding scars to determine distances larvae travel from non-Bt corn into Bt cornfields.
Pennsylvania and Iowa will evaluate the impact of host plants on European corn borer development, fecundity, and survivorship. Freshly enclosed (<12 h) neonate European corn borer will be provided with stem tissue from weeds (Pennsylvania smartweed, common ragweed, pigweed, yellow foxtail, velvetleaf) and noncorn host plants (peppers, snap beans, millet, and potatoes). These studies will be conducted under appropriate environmental conditions. A minimum of 50 larvae will be put on each host. Developmental time from eclosion to adult emergence will be determined with daily observations. In addition, percentage of survival from eclosion to adult emergence will be calculated for larvae feeding on each host. Fecundity will be determined for surviving females.
Illinois and Minnesota will use the information generated from these ecological studies to improve the realism of their models and generate predictions for use in designing insect resistance management (IRM) implementation strategies. These models also will serve as an integrating template for understanding population dynamics of the pest when new technologies become available.
Iowa and Kansas will investigate the possibility of using densely planted popcorn as sacrificial refuge. This will build on research conducted in Iowa on European corn borer that suggests 1 ha of popcorn can produce an adequate population of susceptible European corn borers for 99 ha of Bt corn. A 2B4_ density of popcorn and standard susceptible corn will be planted in grower-friendly configurations at three or more locations in each state. These plants will be dissected to determine the number of European corn borer and southwestern corn borer present in these plantings.
Population Genetic Structure. Hi-resolution landscape level maps of voltine patterns will be generated by Pennsylvania for key states in the region to investigate the relationship among climatic patterns, geographic distribution of voltine types, and their genetic relatedness. The predictions will serve as a null hypothesis about the distribution of voltine races and areas of coexistence across North America. Researchers from Pennsylvania, Delaware, Nebraska, Iowa, Kansas, Minnesota, Wisconsin, South Dakota, and Maryland will collect a minimum of 50 larvae from selected locations expected to have specific voltine races or mixtures. These larvae will be sent to Nebraska for voltine typing with molecular techniques, such as random amplified polymorphic DNA-polymerase chain reaction (RADP-PCR). The resulting voltine types will be compared with the model predictions to verify or reject ability to predict voltine patterns.
In the Eastern United States, European corn borer will be collected to evaluate their sex pheromone system. Molecular techniques will be developed to identify markers that can be used to separate races. If successfully developed, the markers will be used to evaluate geographic populations and host relationships. Attempts will be made to study the factors that influence natural hybridization. South Carolina, Delaware, Pennsylvania, Maryland, and New York will collect a minimum of 200 larvae per site for analysis. Nebraska will conduct molecular studies on larvae.
Pure colonies of the E-multivoltine, Z-multivoltine, and Z-univoltine European corn borer will be established and Nebraska will study differences in their sensitivity to B. thuringiensis toxins. In addition, Nebraska will continue to screen geographic populations from across North America for their sensitivity to the toxins. All listed participating states in this objective will provide European corn borers for the analysis. Purified insecticidal crystal proteins (Cry1Ab, Cry1Ac, Cry9C, and other Cry proteins as they become available) and transgenic Bt plants based on transformation events encoding for the same Cry toxins will be used in these experiments.
The information generated from these population genetic structure studies will be incorporated into the population dynamic-population genetic models of Minnesota, Illinois, and Pennsylvania to improve prediction of resistance development and potential spread between races. In addition, this information can be presented on a larger geographic scale.
Resistance Studies. Researchers in Kansas, Nebraska, and Minnesota will establish and maintain colonies of resistant European corn borers. The resistant colonies will be developed by selection on diets containing various Cry proteins. Purified insecticidal crystal proteins (Cry1Ab, Cry1Ac, Cry9C, and other Cry proteins) and transgenic Bt plants, based on transformation events encoding for the same Cry toxins, will be used in these experiments. These states will investigate mechanisms of resistance and cross-resistance. Studies will include comparisons of the level and profile of midgut proteinases and the affinity and density of B. thuringiensis binding receptors(s) in midgut brush border membranes between B. thuringiensis-susceptible and -resistant strains of European corn borer. Kansas and Nebraska will develop the methods to establish and maintain resistant colonies of southwestern corn borer. The same types of research will be performed on southwestern corn borer when resistant colonies are established.
To determine allele frequencies of resistance in natural populations, Minnesota will conduct an F2 screening for populations collected in Minnesota, Nebraska, and other states. Approximately 1,000 female European corn borers will be collected with a blacklight trap and screened for resistant alleles (Andow and Alstad 1998, 1999). In addition, Minnesota will establish three late-planted, 5-acre Bt sweet corn fields as sentinel plots to screen for resistance in natural populations. Isogenic non-Bt sweet corn fields will be used to determine the natural European corn borer population densities. Approximately 10,000 Bt sweet corn ears and from 100 to 200 non-Bt sweet corn ears will be sampled at each site each year to monitor for surviving European corn borers and corn earworms. Similar protocol will be applied to Bt and non-Bt sentinel field corn plots.
See attached for additional Procedures.Measurement of Progress and Results
Outputs
Outcomes or Projected Impacts
- This project will continue to serve as a focal point for research expanding management options and profitability of corn growers faced with stalk-boring insects. The scope of the project will be broad, cutting across the entire Corn Belt and surrounding states, incorporating the efforts of multiple disciplines, including entomologists, geneticists, ecologists, and economists. The project will provide needed information to multiple stakeholders, including growers, seed companies, the EPA, and the public. Expected outcomes of the project will include cutting-edge science-based reports, new proposals for research and extension, prescription of effective and cost-efficient options for corn borer management, and outreach to growers.
- A better understanding of the economics of managing stalk-boring corn insects will help regulators to design effective policies that take into account growers= incentives.
- An improved understanding of the ecology and genetics of European corn borer and southwestern corn borer will translate into better IRM recommendations and will help policymakers reach better informed decisions.
- Research on natural enemies of stalk-boring corn insects will enhance development of management tactics based on habitat management and biological control agent augmentation.
- Assessment of the impact that transgenic corn will have on nontarget insects such as monarchs will use a science-based approach to evaluation, providing needed input to the policy process.
- Grower surveys will determine the effectiveness of technology transfer, specifically IRM recommendations.<br><br> Collaboration among project participants will lead to the development of multistate, multidisciplinary proposals for research and extension funding. Such collaboration and sharing of information will lead to more efficient and effective use of public funds.<br><br> Communication among project participants and stakeholders will be enhanced. New findings will be communicated to growers, agricultural industry personnel, government agencies, and the general public through traditional grower and industry training programs, newsletters, Web pages, scientific publications, and committee position statements. The communication network fostered by the project will serve as a forum for continued research and education.<br><br> This multistate research project will enhance the development of technology and adoption of environmentally friendly, sustainable pest management by growers. Adoption will be driven by the development and dissemination of high-quality information that results from the integrated assessment of stalk-boring insect management alternatives. The efforts of the committee will focus on the specific needs of all stakeholders.
Milestones
(0):0Projected Participation
View Appendix E: ParticipationOutreach Plan
Organization/Governance
The project will be administered by a technical committee consisting of all participants of the project and all are eligible for office, regardless of sponsoring agency affiliation or the number of members per SAES. An executive committee will consist of the chairperson, secretary, and the administrative advisor. The executive committee will conduct business between meetings. Subcommittees may be named by the chair as needed for specific assignments such as developing new project outlines for continuing the project, to prepare publications, or other assignments. An annual meeting of the full technical committee will be held to summarize and critically evaluate progress, analyze results, and plan future activities, reports, and publications. The chair, in consultation with the technical committee and with the concurrence of the administrative advisor notifies the technical committee members of the time and place of meetings, prepares the agenda, and presides at meetings of the technical committee and executive committee. The administrative advisor authorizes the meeting ~90-120 days in advance. The chair prepares or supervises preparation of the annual report of the project. The secretary prepares minutes of the annual meeting and forwards them to the administrative advisory who distributes them to the regional research office, the CSREES liaison person, technical committee members, all North Central AES directors, and directors of other participating states. Procedures outlined in the manual for Cooperative Regional Research (revised 1992) will be followed.
Literature Cited
Andow, D. A., and D. N. Alstad. 1998. The F2 screen for rare resistance alleles. J. Econ. Entomol. 91:572-578.
Andow, D. A., and D. N. Alstad. 1999. Credibility interval for rare resistance allele frequency. J. Econ. Entomol. 92: 755-758.
Bode, W. M., and D. D. Calvin. 1990. Yield-loss relationships and economic injury levels for European corn borer populations (Lepidoptera: Pyralidae) infesting Pennsylvania field corn. J. Econ. Entomol. 83:1595-1603.
Calvin, D. D. 1995. Economic benefits of transgenic corn hybrids for European corn borer management in the United States. A report to Monsanto Company.
Calvin, D. D., M. C. Knapp, K. Xingquan, F. L. Poston, and S. M. Welch. 1988. Influence of European corn borer (Lepidoptera: Pyralidae) feeding on various stages of field corn in Kansas. J. Econ. Entomol. 81:1203-1208.
Dively, G .P., J. J. Linduska, and M. Ross. 1999. Timing of insecticide applications for dusky sap beetle and lepidopteran survivors ofBt sweet corn, pp. 22-25. In Proceedings, Mid-Atlantic Vegetable Workers Conference, Newark, DE, October 1998.
Dogan, E. B., R. E. Berry, G. L. Reed, and P. A. Rossignol. 1996. Biological parameters of convergent lady beetle (Coleoptera: Coccinellidae) feeding on aphids (Homoptera: Aphididae) on transgenic potato. J. Econ. Entomol. 89: 1105-1108.
Donegan, K. K., D. L. Schaller, J. K Stone, L. M. Ganio, G. Reed, P. B. Hamm, and R. J. Seidler. 1996. Microbial populations, fungal species diversity and plant pathogen levels in field plots of potato plants expressing the Bacillus thuringiensis var. tenebrionis endotoxin. Transgenic Res. 5: 25-35.
[EPA] Environmental Protection Agency. 1998. Final report of the FWRA scientific advisory panel subpanel on Bacillus thuringiensis (Bt) plant-pesticides and resistance management meeting (R. Kendall, R. Alien, M. Caprio, T. Dennehy, F. Gould, D. Hardee, R. Hellmich, R. Higgins, W. Hutchison, R. Luttrell, K. Ostlie, B. Siegfried, M. Whalon, and J. Witkowski [authors/panel members]). Feb. 9-10, 1998. www.epa.gov/pesticides/SAP/finalfeb.pdf
Fearing, P. L., D. Brown, D. Vlachos, M. Meghji and L. Privalle. 1997. Quantitative analysis of CryIA(b) expression in Bt maize plants, tissues, and silage and stability of expression over successive generation. Mol. Breed. 3: 169-176.
Gould, F. 1988a. Evolution biology and genetically engineered crops. BioScience 38: 26-33.
Gould, F. 1988b. Genetic engineering, integrated pest management and the evolution of pests. Trends Ecol. Evol. 3/TIBTECH 6: S15-S19.
Gould, F. 1989. Ecological-genetic approaches for the design of genetically engineered crops, pp.146-151. In D.W. Roberts and R. Granados [eds.], Proceedings, Biotechnology, Biological Pesticides and Novel Plant-Pest Resistance for Insect Pest Management. Boyce Thompson Institute Conference, July 1988. Boyce Thompson Institute Publications, Ithaca, New York.
Hellmich, R. L. Refuge issues. In Proceedings, EPA-USDA Workshop on Bt Crop Resistance Management. June 18, 1999, Chicago, Illinois (in press).
Higgins, R. A., and T. M. Hurley. Bt corn refuge design and deployment-High plains production areas where joint infestations of European and southwestern corn borer occur. In Proceedings EPA-USDA Workshop on Bt Crop Resistance Management, June 18,1999, Chicago, Illinois (in press).
Hilbeck, A., M. Baumgartner, P. M. Fried, and F. Bigler. 1998. Effects of transgenic Bacillus thuringiensis corn-fed prey on mortality and development time of immature Chrysoperia carnea (Neuroptera: Chrysopidae). Environ. Entomol. 27: 480-487.
Homer, T. A., G. P. Dively, and A. Herbert. 1998. Impact ofMON810-based Bt-transgenic corn on corn earworm. In Proceedings, Monsanto Academic Data Review-Research Summaries. St. Louis, MO, October 1998.
Hueth, D., and U. Regev. 1974. Optimal agricultural pest management with increasing pest resistance. Am. J. Agric. Econ. 56: 543-552.
Hunt, T. E. 1999. Dispersal and behavior of adult European corn borer in and around corn. Ph.D. dissertation. University of Nebraska-Lincoln.
Hurley, T., B. Babcock, and R. Hellmich. 1997. Biotechnology and pest resistance: an economic assessment of refuges. Center for Agricultural and Rural Development Working Paper 97-WP 183, Iowa State University, Ames.
Hurley, T., C. Secchi, B. Babcock, and R. Hellmich. 1999. Managing the risk of European corn borer resistance to transgenic corn: an assessment of refuge recommendations. Center for Agricultural and Rural Development Staff Report 99-SR 88, Iowa State University, Ames.
Hyde, J., M. A. Martin, P. V. Preckel, and C. R. Edwards, 1998. The economics ofBt corn: adoption implications. Purdue Univ. Coop. Ext. Serv. Publ. ID-219, West Lafayette, IN.
Losey, J. E, L. S. Rayor, and M. E. Carter. 1999. Transgenic pollen harms monarch larvae. Nature (Lond)399:214.
Louette, D. 1997. pp. 56-66. In J. A. Serratos, M. C. Willcox and F. Castillo [eds.]. Gene flow among maize landraces, improved maize varieties, and teosinte: implications for transgenic maize. Mexico, D.F. CIMMYT, 1997.
Mason, C. E., M. E. Rice, D. D. Calvin, J. W. Van Duyn, W. B. Showers, W. D. Hutchinson, J. F. Witkowski, R. A. Higgins, D. W. Onstad, and G. P. Dively. 1996. European corn borer ecology and management. North Central Region Ext. Publ. 327, Iowa State University, Ames.
[NC-205] North Central-205. 1998. Supplement to NCR-602. Bt corn & European corn borer: long-term success through resistance management. http://ent.agri.umn.edu/ECB/nc205doc.htm
Onstad, D. W. and C. A. Guse. Economic analysis of transgenic maize and nontransgenic refuges for managing European corn borer (Lepidoptera:Pyralidae). J. Econ. Entomol. (in press).
Onstad, D. W., and E. A. Komkven. 1999. Persistence of natural enemies of weeds and insect pests in heterogeneous environments, pp. 349-367. In B. A. Hawkins and H. V. Cornell [eds.], Theoretical approaches to biological control. Chapman & Hall, London.
Onstad, D. W., and J. V. Maddox. 1989. Modeling the effects of the microsporidium, Nosema pyraitsta, on the population dynamics of the insect, Ostrinia nubilalis. J. Invertebr. Pathol. 53: 410-421.
Orr, D. B., and D. A. Landis. 1997. Oviposition of European corn borer (Lepidoptera: Pyralidae) and impact of natural enemy populations in transgenic versus isogenic corn. J. Econ. Entomol. 90: 905-909.
Ostlie, K. R., W. D. Hutchinson, and R. L. Hellmich. 1997. Bt-com and European corn borer: strategies for long-term success of innovative technology. North Central Region Publ. NCR-602. University of Minnesota, St. Paul. http://ent.agri.umn.edu/ECB/nc205doc.htm
Pilcher, C. D. J. J. Obrycki, M. E. Rice, and L. C. Lewis. 1997. Preimaginal development, survival, and field abundance of insect predators on transgenic Bacillus thuringiensis corn. Environ. Entomol. 26:446-454.
Prokrym, D. R., D. A. Andow, J. A. Ciborowski, and D. D. Sreenivasam. 1992. Suppression of Ostrinia nubilalis by Trichogramma nubilale in sweet corn. Entomol. Exp. Appl. 64: 73-85.
Raynor, G. S., E. C. Ogden, and J. V. Hayes. 1972. Dispersion and deposition of corn pollen from experimental sources. Agron. J. 64: 420-427.
Regev, Uri, Andrew P. Gutierrez, and Gershon Feder. 1976. Pests as a common property resource: a case study of alfalfa weevil control. Am. J. Agric. Econ.: 186-197.
Regev, Uri, Haim Shalit, and A. P. Gutierrez. 1983. On the optimal allocation of pesticides with increasing resistance: the case of the alfalfa weevil. J. Environ. Econ. Manage. 10: 86-100.
Riddick, E. W., and P. Barbosa. 1998. Impact ofCry3A-intoxicated Leptinotarsa decemlineata (Coleoptera: Chrysomelidae) and pollen on consumption, development, and fecundity ofColeomegilla maculata (Coleoptera: Coccinellidae). Ann. Entomol. Soc. Am. 91: 303-307.
Riddick, E.W., G. Dively, and P. Barbosa. 1998. Effect of a seed-mix deployment ofCry3A-transgenic and nontransgenic potato on the abundance of Lebia grandis (Coleoptera: Carabidae) and Coleomegilla maculata (Coleoptera: Coccinellidae). Ann. Entomol. Soc. Am. 91: 647-653.
Schuler, T. H., G. M. Poppy, B. R. Kerry, and I. Denholm. 1999. Potential side effects of insect-resistant transgenic plants on arthropod natural enemies. Trends Biotechnol. 17: 210-216.
Sims, S. R. 1995. Bacillus thuringiensis var. kurstaki [CrylA (C)] protein expressed in transgenic cotton: effects on beneficial and other non-target insects. Southwest. Entomol. 20: 493-500.
Taylor, C. Robert, and J. C. Headley. 1975. Insecticide resistance and the evaluation of control strategies for an insect population. Can. Entomol. 107: 237-242.
Turpin, F. T. 1977. Insect insurance: potential management tool for corn soil insects. Bull. Entomol. Soc. Am. 23:181-184.
Whitworth, F. J., F. L. Poston, S. M. Welch, and D. D. Calvin. 1984. Quantification of southwestern corn borer feeding and its impact on corn yield. Southwest. Entomol. 9: 308-318.
Wiedenmann, R. N., and J. W. Smith, Jr. 1997. Attributes of natural enemies in ephemeral crop habitats. Biol. Control 10: 16-22.
Yu, L., R. E. Berry, and B. A. Croft. 1997. Effects of Bacillus thuringiensis toxins in transgenic cotton and potato on Folsomia Candida (Collembola: Isotomidae) and Oppia nitens (Acari: Orbatidae). J. Econ. Entomol. 90: 113-118.