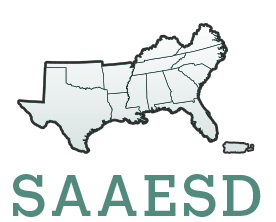
S1022: Basic and Applied Aspects of Bacterial Source Tracking
(Multistate Research Project)
Status: Inactive/Terminating
S1022: Basic and Applied Aspects of Bacterial Source Tracking
Duration: 10/01/2005 to 09/30/2010
Administrative Advisor(s):
NIFA Reps:
Non-Technical Summary
Statement of Issues and Justification
In this multistate proposal, we consider the basic and applied aspects of bacterial source tracking (BST). To understand BST, it is first necessary to understand fecal contamination of water. Water contaminated with feces has the potential to affect human health and to have adverse economic impacts. In terms of human health, it has long been known that water containing a high number of fecal bacteria is likely to contain pathogenic bacteria (Geldreich, 1970) and, if humans are exposed to these pathogenic bacteria, they can become ill. In terms of economic impacts, if an area develops a reputation for having unclean water, then people will avoid the area. If the area is a tourist area, then the economic losses can be severe. Similarly, an industry that relies on clean water, such as the shellfish industry, will suffer financial losses if the shellfish beds are closed because of fecal contamination. Therefore, it is no surprise that various federal agencies have published limits for the number of fecal bacteria permitted in fresh and marine waters (Clesceri et al., 1998; USEPA, 2002). Each state also has its own standard for the number of fecal bacteria permissible in its waters, typically varying with use (e.g., if waters are used for recreation or not). State and local communities routinely monitor fresh and marine waters to see that these standards are upheld.
If environmental waters fail to meet state standards for fecal bacteria, then these waters are out of compliance with the 1972 Federal Clean Water Act, and funds are made available for a Total Maximum Daily Load (TMDL) analysis to bring the waters into compliance. A TMDL establishes the maximum pollutant load a water body can receive and still meet water quality standards. One component of this TMDL analysis is to identify the host sources of the bacterial load. If the host sources can be identified, then it may be possible to reduce the fecal contamination such that the waters meet the state regulatory standards again. These TMDL analyses are the greatest force driving the development of BST methods in the United States.
The underlying principle of BST is that specific markers or strains of bacteria are associated with specific animal species (e.g., Amor et al., 2000). Beginning in 1995 with the development of several genotypic (DNA-based) methods to identify the host origin of Escherichia coli (Samadpour and Chechowitz, 1995; Simmons et al., 1995), BST has expanded considerably with the development of a wide array of genotypic and phenotypic (based on characteristics expressed by the bacterium) methods. Most BST research on genotypic methods has concentrated on ribotyping (Parveen et al., 1999), pulsed field gel electrophoresis (Kariuki et al., 1999), and various polymerase chain reaction (PCR)-based (Dombek et al., 2000) methods, while most BST research on phenotypic methods has concentrated on antibiotic resistance analysis (ARA; Wiggins et al., 1999). In addition, some methods have been developed that do not rely on counting fecal bacteria at all, but on detecting chemicals associated with these bacteria. For example, contamination from human activities can be identified with the chemical method, fluorometry, which measures optical brighteners from laundry detergents in water.
The adverse health and economic effects of water contaminated with feces, as well as the need for TMDL analyses, have meant that BST is consistently identified as a high priority need by various stakeholders. On a federal level, the U. S. National Oceanic and Atmospheric Administration (NOAA) and U.S. Environmental Protection Agency (USEPA) have consistently supported BST research. For example, the Cooperative Institute for Coastal and Estuarine Environmental Technology (CICEET), supported by NOAA, identifies the development of novel and cost-effective technologies and methods to identify sources of microbial contaminants as one of its four funding priorities in FY 2005. The USEPA, in conjunction with other groups such as the Southern California Coastal Water Research Project (SCCWRP) or the Water Environment Research Foundation (WERF), has offered, and continues to offer, workshops on BST methods. Because BST research has led to the development of advanced methods to detect bacterial pathogens, it belongs to one of the three priority areas identified by the American Society for Microbiology at the USDACSREES Stakeholders Workshop (USDA-CSREES, 2002). On a state level, both Water Research Institute and Coastal Zone Management (CZM) Programs consistently fund research on BST. For example, the CZM Program in Georgia identifies refinement of bacterial analysis of coastal water as one of its four funding priorities in FY 2005-2006. Bacterial source tracking is Delawares number one research priority for environmental waters (S. Myoda, pers. comm.). Therefore, BST research is a high priority for federal and state stakeholders.
When Multistate Project S-297 began in 2000, its members recognized the importance of BST research and established BST as one of their three objectives. At that time, there were no matches in the USDA-CRIS database for bacterial source tracking, microbial source tracking, or any of the genotypic, phenotypic, or chemical BST methods. Our objective was to determine the geographic and temporal variability of E. coli ribotypes in the United States. As noted in the Related, Current, and Previous Research section, members of the multistate project not only accomplished this objective, but were also responsible for a number of pioneering research articles on BST beyond this objective. As a result, members of Multistate Project S-297 are recognized as some of the leading BST researchers in the United States.
Although all BST methods have their advantages and disadvantages with respect to reproducibility, discriminatory power, ease of interpretation, ease of performance, and cost (Farber, 1996), the methods still need more development (Simpson et al., 2002). For example, a comparison of genotypic-based BST methods showed that all methods correctly identified the dominant host source in the majority of samples, but false positive rates were as high as 57% (Myoda et al., 2003). A similar study comparing seven genotypic and phenotypic methods that use Escherichia coli to identify sources of fecal contamination suggested that only one method correctly classified all test replicates to host origin, while three methods classified 48 to 62% correctly, and the remaining three classified less than 25% correctly (Stoeckel et al., 2004). Again, false positive rates were high. Therefore, more research to refine BST methods is needed. In addition, because many of us work in poor communities that cannot afford most BST technology, making BST methods less expensive is a priority. Finally, there is evidence that nearly 70% of water quality infractions are single-day events (Leecaster and Weisberg, 2001). Considering that the public is typically notified 24 to 48 hours after samples are collected, most warnings to the public about fecal contamination are already out-of-date when they are issued. Therefore, regulators continue to ask for faster BST methods as well.
This multistate project is focused on two objectives that make BST methods more accurate, less expensive, and faster. The first objective is to continue to develop fluorometry (Hagedorn et al., 2003) and detection of fecal sterols (Gilpin et al., 2003) as BST methods and to use targeted sampling (Kuntz et al., 2003) as a prelude to these methods. The second objective is to determine the stability of genes for antibiotic resistance, the principle behind the phenotypic BST method, ARA. We have already published two articles on this method (Graves et al., 2002; Hagedorn et al., 1999). The research for the two objectives is expected to take five years to complete.
There are three advantages to doing the BST research as part of a multistate effort. First, although all the members of the proposed project are microbiologists and all have conducted BST research previously, some have expertise in particular methods that others do not. For example, some are experts in phenotypic BST methods, while others have stronger chemistry backgrounds and are more skilled in chemical methods. Thus, collaboration among us ensures a diverse array of skills necessary to make the proposed research technically feasible.
Second, our objectives require waters, wastes, and soils with varying environmental conditions. The first objective requires a variety of different water conditions, from fresh to marine, with varying dissolved oxygen, organic matter, turbidity, and pH values. The second objective requires animal wastes and soils from different areas of the United States. Cooperation among laboratories at different locations in the United States is the best way to accomplish this goal. By working cooperatively, we will be able to conduct field research that could otherwise only be done with difficulty if each investigator was working separately.
Third, and most important, many researchers on the proposed multistate project have worked collaboratively for many years. Some of the researchers have collaborated on Regional (and Multistate) Projects S-112, S 170, S 226, S-262, and S-297, and their record of productivity and collaboration has been excellent. For example, members of Multistate Project S-262 and S-297 collaborated on the first and second editions of the best-selling textbook, Principles and Applications of Soil Microbiology, published as a first edition in 1998 and a second edition in 2005 (Sylvia et al., 1998, 2005). Of the 23 chapters in the first edition, 13 were written by members of Multistate Project S-262; of 23 chapters in the second edition, 12 were written by members of Multistate Project S-297. Such a publication is important and tangible evidence of the expertise, willingness, and history of cooperation among the participating scientists, and this productivity and collaboration is critical to the success of the proposed multistate project.
Related, Current and Previous Work
A recent search of the CRIS and HNRIMS databases showed six matches for the words microbial source tracking and 20 matches for the words bacterial source tracking. Of the six matches for microbial source tracking, four projects have been terminated, one belongs to Multistate Project S-297, and one is ongoing. The one ongoing project relates primarily to the performance of onsite wastewater systems and therefore pertains only indirectly to BST. Of the 20 matches to bacterial source tracking, 12 have either been terminated or have recently ended, four relate to Multistate Project S-297, and four are ongoing. Of these four ongoing projects, two pertain to other areas, one to the previously mentioned performance of onsite wastewater systems, and one to foodborne pathogens; only two pertain to the proposed Multistate project. Of these two projects, both are local studies aimed at local problems, and neither has the collaboration nor the national impact of a multistate project. Other than the ongoing Multistate Project S-297, none of the 20 projects identified under bacterial source tracking is a Multistate project except one terminated project, Multistate Project S-1001. The terminated multistate project, entitled Improving surface water quality with alternative cropping systems, relates to BST only peripherally. Therefore, other than Multistate Project S-297, there is no active multistate project on BST.
The proposed new multistate project is a partial continuation of Multistate Project S-297. This multistate project, entitled Soil Microbial Taxonomic and Functional Diversity as Affected by Land Use and Management, had three objectives: 1) to determine the geographic and temporal variability of E. coli ribotypes in the United States, 2) to determine relationships among microbial taxonomic and functional diversity, contaminant bioavailability, and remediation rates for different organic-contaminated soils, and 3) to characterize taxonomic and functional diversity of bacteria and mycorrhizae in disturbed lands and urban landscapes. As mentioned previously, the project also had a special educational objective to write a second edition to the best-selling textbook, Principles and Applications of Soil Microbiology.
Because Objective #1 of Multistate Project S-297 is the only objective pertinent to the proposed new multistate project; only the accomplishments in Objective #1 are considered here. Most importantly, the objective was completed. With respect to geographic variability of E. coli isolates, we determined that there was good ribotype separation among host animal species at one location, and that the ability to match environmental isolates to a host origin database depended on a large number of environmental and host origin isolates that were not geographically separated (Hartel et al., 2002). With respect to time, we came to a similar conclusion: the majority of ribotypes were transient and unique to each sampling time; therefore, a large number of E. coli isolates per host were necessary to establish a host origin database that was independent of temporal variability (Jenkins et al., 2003). We extended this work to flow conditions, and established that flow conditions were also important to ribotype matching (Hartel et al., 2004a). Finally, we established that an animal diet also affected the diversity of ribotype patterns (Hartel et al., 2003). Rather than continue with E. coli, we decided to test another fecal bacterium, Ent. faecalis, for changes in ribotype patterns with geography and time. Another collaborative manuscript on the changes of Ent. faecalis ribotype patterns with geography and time was completed and will be submitted for publication soon. The results were discouraging because not only did ribotype patterns of Ent. faecalis change with both geography and time, but a permanent host origin database sufficiently large to encompass these patterns would be time-consuming and expensive to construct.
In addition to these collaborative publications, researchers on the Multistate Project S-297 pursued two other collaborative projects investigating BST methodologies. The first project was to compare the various genotypic BST methodologies for their strengths and weaknesses. A manuscript was published in 2003 (Myoda et al., 2003). As previously mentioned, all methods correctly identified the dominant host source in the majority of samples, but false positive rates were as high as 57%. One S-297 researcher continued this research with another research team examining both genotypic and phenotypic methods (Stoeckel et al., 2004).
The second collaborative project was to make BST quicker, more effective, and less expensive; it led to three developments. The first development was targeted sampling as a prelude to BST (Kuntz et al., 2003). Targeted sampling is like the childrens game of hot and cold. The sampling is first divided into baseflow and stormflow conditions because runoff during stormflow typically increases fecal bacteria counts 10- to 100-fold (Solo-Gabriele et al., 2000; Hartel et al., 2004a). By combining local knowledge, sampling, and resampling of the water during one of these two flow conditions, it is possible to identify hotspots of fecal contamination quickly and easily. Targeted sampling also reduced the effects of bacterial changes with geography and time, and this reduction made existing BST methods better and less expensive by reducing the environmental complexity. At this point, targeted sampling has been conducted during both base- (Kuntz et al., 2003) and storm-flow (Hartel et al., 2004b) conditions in marine waters along the Georgia Coast, but has not yet been conducted in freshwater or in other states.
The second development in the project was to determine the limitations of fluorometry as a BST method (Hagedorn et al., 2003). Fluorometry works by detecting optical brighteners from dishwashing and laundry detergents in environmental waters because these compounds fluoresce when exposed to UV light. Therefore, fluorometry is not a bacterial source tracking method, but a chemical source tracking method. Furthermore, because these fluorescent compounds are associated with human sewage, the method discriminates only between human and nonhuman sources of fecal contamination. Preliminary tests by one multistate researcher concluded that fluorometry was an acceptable, inexpensive method to detect human sewage in fresh and marine waters (Hagedorn et al., 2003).
The third and final development in the project was to pursue enterococcal speciation as a simple method to discriminate between human and non-human fecal contamination. We extended the original research of Pourcher et al. (1991) and determined that Ent. faecalis had a host range essentially restricted to humans and wild birds (Wheeler et al., 2002; Kuntz et al., 2004). Our results suggest that unless the fecal loading rate from migratory or resident wild birds is high, water samples collected during baseflow conditions with high numbers of Ent. faecalis indicate human fecal contamination.
In the proposed new multistate project, BST work will continue on two of the three developments: targeted sampling and fluorometry. On the first objective, work will begin on combining targeted sampling with fluorometry. We have already conducted a preliminary test combining the two approaches in Georgia (Gates et al., 2004). This preliminary test was important because it was conducted in waters containing high amounts of dissolved organic matter, which fluoresce at the same wavelength as optical brighteners (Hartel, unpublished). Although fluorometry is only able to discriminate between human and nonhuman fecal contamination, the method is quick and relatively inexpensive. This discrimination is helpful because of the large number of leaking sewer lines and malfunctioning septic drainfields in the United States (e.g., Kelsey et al., 2004). The problem with septic systems is that although the states require homeowners to obtain an installation permit, inspections and maintenance are not enforced and are often neglected. Unsurprisingly, many septic systems fail. Identifying and fixing these sources of fecal contamination are a high priority for stakeholders because, unlike some other sources of fecal contamination (e.g., wildlife), they can be corrected.
Fluorometry is limited to detecting human fecal contamination, and it is important to be able to detect fecal contamination of other warm-blooded animals, especially agriculturally important animals (e. g., cattle). Therefore, we will also combine targeted sampling with the detection of fecal sterols. Like fluorometry, detection of fecal sterols is not a bacterial source tracking method, but a chemical source tracking method. The principle behind the method is that the formation of fecal sterols varies in the intestines of warm-blooded animals depending on the animals diet, intestinal microorganisms, and host metabolism. In the human intestine, cholesterol is reduced preferentially to coprostanol (5ß-cholestan-3ß-ol), whereas in the environment, cholesterol is reduced preferentially to cholestanol (5a-cholestan-3ß-ol; Leeming et al., 1996). A similar mechanism occurs in the herbivore intestine, where plant-derived 24-ethylcholesterol (24-ethylcholest-5-en-3ß-ol) is reduced preferentially to 24-ethylcoprostanol (24-ethyl-5ß-cholestan-3a-ol) and 24-ethylepicoprostanol (24-ethyl-5ß-cholestan-3a-ol), whereas in the environment, 24-ethylcholesterol is reduced preferentially to 24-ethylcholestanol (24-ethyl-5a-cholestan-3ß-ol). The ratios of various stanols (e.g., coprostanol:24-ethylcoprostanol) can not only distinguish different types of feces (e.g., Gilpin et al., 2003), but also different effluents (Gregor et al., 2002). Unlike fluorometry, detection of fecal sterols requires gas chromatography/mass spectrometry (GC/MS), instruments that are relatively expensive to own and that require a relatively high level of technical skill to operate. However, these instruments are available to us at relatively low cost and we have the technical skills to operate them. Detection of fecal sterols has been successfully used to trace fecal contamination in marine (Noblet et al., 2004), and fresh (Gilpin et al., 2003) waters. Detection of fecal sterols has never been combined with targeted sampling.
The second objective is to determine the stability of antibiotic resistance in fecal bacteria under different environmental conditions, and this objective reflects the need for further research not only to understand the underlying principle of ARA method for BST, but also to understand some basic ecological issues involving antibiotic resistance. The underlying principle for ARA is that the bacteria present in the gut of various types of animals are subjected to different types, concentrations, and frequencies of antibiotics, and that over time, selective pressure from a specific group of animals selects for bacteria that possess specific fingerprints of antibiotic resistance (Scott et al., 2002). The ARA method is among the most common of the BST methods because it is simple to conduct, is relatively inexpensive, and can successfully discriminate among different animal species (Harwood et al., 2000; Wiggins, 1996; Wiggins et al., 1999). For example, Hagedorn et al. (1999) used ARA to establish that cattle were the predominant source of fecal contamination in a rural Virginia watershed. The major disadvantages of ARA are that it is time-consuming to conduct and it requires a large host origin database (many thousands of isolates) to work well.
The underlying principle of ARA requires understanding the stability of antibiotic resistance, particularly under different environmental conditions. Antibiotic resistance is encoded on a wide variety of mobile genetic elements, including plasmids, transposons, and conjugative transposons. During the appropriate conditions, conjugation, transformation, and transduction (Kidambi et al., 1994; Davison, 1999) spread these elements (and hence, antibiotic resistance) to other bacterial lineages. For example, tetracycline resistance is encoded on plasmids (Aminov et al., 2001) and conjugative transposons (Salyers et al., 1995), and these elements have helped transfer these genes to other bacterial species (Nikolich et al., 1994). Resistance to multiple antibiotics has been observed on these readily mobilized plasmids in a diverse group of bacteria (Smalla et al., 2000). Furthermore, lateral transfer of these genetic elements, once considered to be a rare event, is now known to occur commonly (Ochman et al., 2000).
What is unclear is the extent to which antibiotic resistance lingers after antibiotic use is discontinued. If possession of antibiotic resistance does not confer a competitive advantage under certain environmental conditions, then genes encoding those mechanisms may or may not be retained or transferred. In some cases, antibiotic resistance may persist for many years. Lu et al. (2003) found gene cassettes encoding chloroamphenicol resistance in broiler litter, even though the antibiotic had been discontinued in broiler production for 15 years. Similarly, animals that had never received tetracycline or any antibiotics for prophylaxis or growth promotion still possessed bacteria with the resistance genes to tetracycline (Aminov et al., 2001). It is important to understand this stability because it affects the creation of host origin databases. For example, if the genes for antibiotic resistance persist for long periods of time, then they may represent a memory of long-past fecal contamination. Furthermore, this stability poses some basic ecological questions as well. For example, are certain resistance patterns linked to the exposure of animal populations to only a few antibiotics? Do biological responses to changes in abiotic factors alter the identification and/or prevalence of microbes expressing resistance to specific antibiotics? Answering these questions is ideally suited to multistate research because each participant offers a potentially diverse array of sites with varying environmental factors.
Objectives
-
To combine targeted sampling with fluorometry and detection of fecal sterols as BST methods to detect fecal contamination in national waterways.
-
To determine the stability of antibiotic resistance genes in fecal indicator bacteria under different environmental conditions in order to understand the movement of these genes in soils and waters receiving animal wastes, and the effect of their stability on the reliability of detection methods based on characterizing these genes.
Methods
Objective #1 Targeted sampling will be conducted at each location (AL, DE, GA, GA-USDA, MI, MN, NC, OK, VA, WV) as described by Kuntz et al. (2003). At each sampling point, the location will recorded with a hand-held global position system (GPS) device (Garmin, Olathe, KS). Turbidity will be recorded with a turbidity meter (HF Scientific, FL), and dissolved oxygen, salinity, temperature, and pH will be recorded with a Hydrolab Quanta (Austin, TX). For bacterial enumeration of estuarine and marine waters, water samples will be collected in 100-mL (4 oz.) Whirl-Pak bags (NASCO, Modesto, CA), placed on ice, and processed within 6 hours with the Enterolert System (IDEXX Labs, Westbrook, ME). For freshwater, the sampling will be the same, except the bacteria will be processed with the Colilert System (IDEXX). Enterolerts estimate the number of fecal enterococci and Colilerts estimate the number of E. coli, which are the fecal indicator bacteria of choice for marine and fresh waters, respectively (USEPA, 2002). In freshwater samples where human fecal contamination is suspected, occasional samples will also be processed through the Enterolert system to obtain fecal enterococci for analysis of human virulence factor (see below). Samples will be diluted ten-fold with sterile distilled water in sterile manufacturer-supplied polystyrene bottles. A package of Enterolert or Colilert reagent, as appropriate, will be dissolved in each bottle, which will be poured into a Quanti-tray, a sterile disposable panel containing 97 wells. Each Quanti-tray will be mechanically sealed and incubated for 24 h at the appropriate temperature. Researchers at GA, NY, and OK have Quant-tray sealers to lend to those locations without one. Fluorescing (positive) wells will be counted under a 365-nm UV light (Model EA-160, Spectronics Corp., Westbury, NY). The number of positive wells will be converted to a Most Probable Number (MPN) of fecal enterococci or E. coli based on the dilution factor and the manufacturer-supplied MPN tables. Fluorometry will be conducted during targeted sampling with a field fluorometer (Model 10-AU-005, Turner Designs, Sunnyvale, CA). The fluorometer will be set to detect long wavelength optical brighteners as described by the manufacturer. Researchers at GA have a fluorometer to lend to those locations without one. The fluorometer will be set to discrete or continuous sampling, depending on whether or not it is possible to navigate the waters. If the water cannot be navigated with a boat, then discrete samples will be obtained. If the waters can be navigated, then water will be pumped (1.9 cubic meters per hour) through the detector with a pump mounted on the boat. Background fluorescence will be determined with water distant from sewer lines and septic tanks, and the fluorometer zeroed such that these samples have fluorescent readings between -10 and +10. Any site with an optical density >100 will be considered positive (Gates et al., 2004). In the case of marine samples, they will be collected on an outgoing tide to capture land-based runoff. To confirm that the fecal bacteria are of human origin, selected Enterolert samples will be analyzed for the presence of human virulence factor in Enterococcus faecium as described by Scott et al. (2005). Approximately 100 enterococcal isolates will be obtained from separate positive Enterolert Quanti-tray wells, and spotted on a 0.45-µm membrane on a 5 cm Petri plate with mEI agar (Becton-Dickinson, Franklin Lakes, NJ). The plates will be incubated at 41±0.5 °C for 24 hours and sent overnight to Biological Consulting Service of North Florida (Gainesville, FL). Their proprietary testing determines the incidence of a human-specific virulence factor in Ent. faecium isolates. In addition, selected Enterolert samples will be analyzed in each participating laboratory to evaluate the method. In addition, where human fecal contamination is suspected, each participating laboratory will send sediment samples collected during targeted sampling to researchers in GA for solid phase extraction and analysis on a gas chromatograph/tandem mass spectrometer (GC/MS/MS; Model TraceGC/Polaris Q, Thermo Finnigan, San Jose, CA) for the presence of optical brighteners. Except for GC/MS/MS confirmation of fluorometry, the design follows the sampling and analytical procedures in Gates et al. (2004) and Hartel et al. (2004b). Locations with high bacterial counts will also have sediments analyzed for the presence of fecal sterols according to the method of Marvin et al. (2001). Briefly, sediment samples at each location will be collected in 1-L Nalgene bottles and will be placed on ice. Samples will be freeze-dried and will be passed through a 62-micrometer sieve (to remove the sand fraction). The freeze-dried samples will be sent to researchers in GA for extraction. Dry 1.0-g samples will be extracted in dichloromethane using an accelerated solvent extractor (Model ASE 100, Dionex, Sunnyvale, CA). Extracts will be cleaned up by passing the sample through an alumina column before drying under a stream of nitrogen gas. The sterol/stanol mixture will be derivatized with N-methyl-N-trimethysilyl-trifluoroacetamide and will be reconstituted in iso-octane. The derivatized products will be analyzed with PerkinElmer Auto System XL gas chromatograph (GC) interfaced with a PerkinElmer Turbo Mass Gold mass spectrometer (MS) located in the Marine Extension Laboratory, Brunswick, GA. The GC/MS is available for multistate use at minimal cost. Separation of the derivatized products will be achieved with an integrated 5-meter guard column and a 30-meter X 0.25-mm i.d. cross-linked methyl silicone (0.25 µm film thickness) fused silica capillary column (DB-5, Restek Corporation, Bellefonte, PA). Analytes will be identified by comparison of their retention times and mass spectra with those of authentic standards and augmented by the use of the TurboMass NIST/EPA/NIH Mass Spectral Database (Version 2.1). The MS analyzer will be operated in the electron ionization mode; the conditions will be ion source, 170°C; filament current, 0.25 mA; electron voltage, 70 eV; m/z range, 50-650; scan rate, and scan rate, 1 scan sec-1. Analytes will be quantified based on abundance ratios of analyte to the internal standard. Calibration curves will be established by the analysis of pure standards. Quantitative standards will also be analyzed at specified intervals to assure data quality. Objective #2 Researchers will conduct experiments integrating traditional cultivation methods and modern molecular methods (AL, AR, IN, NY, NC, OK). In terms of traditional cultivation practices, each location will obtain animal waste and soil samples, and perform 10-fold serial dilutions with 0.85% NaCl. Three serial dilutions will be plated onto 0.1X tryptic soy agar (TSA) plates containing cyclohexamide or selective media for fecal bacteria. After incubation at the appropriate temperature, the number of CFU per plate will be counted. After purifying by restreaking, each isolate will be transferred to 200 mL of sterile 0.85% NaCl contained in a 96-well microtiter plate. To test for antibiotic resistance, a few microliters from each well will be transferred with a sterile, stainless steel replica plater (Sigma) onto agar plates containing the appropriate media with known concentrations of antibiotics. Unamended plates will be used as controls. Researchers at each location will concentrate on an antibiotic appropriate to an animal industry at their location (poultry: AL, AR; swine: IN, NC; cattle: OK; dairy: NY). For example, antibiotics important to the swine industry are listed at http://www.aphis.usda.gov/vs/ceah/cahm/index.htm. Isolates will be grown and will be recorded for antibiotic resistance. Each location will determine the phylogenetic diversity of antibiotic resistant bacteria using amplified ribosomal DNA restriction analysis (ARDRA) patterns (Massol-Deya et al., 1995). Briefly, the DNA from the cultivable microorganisms will be extracted. The 16S rRNA gene will be amplified, digested with the restriction enzymes RsaI and HhaI, and products separated on 2% agarose gels. The 16S rRNA gene nucleotide sequence of at least one isolate in a group of identical RsaI and HhaI digest fingerprint patterns will be determined (LaPara et al., 2000). The gene sequences will be checked for potential chimeras with the Ribosomal Database Project II (RDPII; Cole et al., 2003) and closely related 16S rRNA gene sequences from GenBank (Benson et al., 2003). Alignments will be edited manually using appropriate programs (e.g., SeqPup, ftp://iubio.bio.indiana.edu/), and unambiguously aligned base positions will be used to construct phylogenetic trees with maximum likelihood and maximum parsimony methods using PAUP (Sinauer Associates, Sunderland MA) or other appropriate software. Sequences of 16S rRNA genes of bacterial isolates expressing antibiotic resistance will be placed in phylogenetic trees containing appropriate comparative GenBank and RDP II sequences. Because of the range of animal wastes and study sites, as well as inherent difficulties in comparing results across gels, each multistate investigator will use standards consisting of DNA from several isolates cultivated, extracted, and amplified in one lab. In addition, the isolates will also be screened to determine the location of the antibiotic genes (e.g., plasmid). The analyses will be carried out for two years, with each investigator analyzing soils and surface waters receiving wastes from the appropriate animal industry in that state. Researchers at all locations will conduct research according to Good Laboratory Practices. Quality control includes the use of fresh reagent grade chemicals for standards, blanks, duplicates, and standard reference materials. All instruments follow a preventative maintenance schedule according to the manufacturer's recommendations. All instruments will be calibrated. Performance and reference samples will be analyzed periodically; results must fall within an acceptable range defined by the laboratory precision and accuracy data, which are accumulated from duplicate sample analyses. For Objectives #1 and #2, all data will be reviewed for precision and accuracy by a biostatistician located in GA and AR, respectively. The data will be validated through review of the analytical run, calculation steps, sample acceptability, and proofing of the transcription process. If they are deemed acceptable, then the data will be analyzed on the appropriate software. For example, a Chi-squared analysis will determine the relationship between counts of fecal enterococci and fluorometric readings, as well as selected chemical and environmental variables (e.g., dissolved oxygen). All annual reports will include summaries quantifying the accuracy, precision, degree to which the sample represents a statistical population, completeness, and comparability of the experimental and control data.Measurement of Progress and Results
Outputs
- A publication reporting whether or not combining targeted sampling with fluorometry is an inexpensive yet robust BST method to detect sources of human fecal contamination (AL, DE, GA, GA-USDA, MI, MN, NC, OK, VA, WV).
- A publication reporting whether or not combining targeted sampling with fecal sterols is a robust BST method to detect human and nonhuman sources of fecal contamination (AL, DE, GA, GA-USDA, MI, MN, NC, OK, VA, WV).
- A research publication reporting a) the diversity of antibiotic-resistant bacteria in wastes and waste-amended soils, and b) the stability of the antibiotic resistance markers these bacteria harbor under varying environmental conditions (AL, AR, IN, NY, NC, OK).
Outcomes or Projected Impacts
- Combining targeted sampling with fluorometry will identify sources of human fecal contamination inexpensively. If human sources could be identified, then agricultural sources could be eliminated. Any reduction of human fecal contamination to environmental waters will have beneficial economic, health, and environmental effects
- Combining targeted sampling with fecal sterols will identify sources of human and nonhuman sources of fecal contamination. Any reduction of human and nonhuman (e.g., cattle) fecal contamination to environmental waters will have beneficial economic, health, and environmental effects.
- Determining the genetic diversity of antibiotic-resistant bacteria in wastes and waste-amended soils and characterizing their associated antibiotic-resistance genes will a) help track the survival of these bacteria, b) determine the potential for lateral gene transfer to recipient bacteria in the environment, and c) provide regulators with an understanding of the potential limitations of antibiotic resistance analysis, currently one of the more popular BST methods.
Milestones
(2006): (Objective #1) Preliminary research on combining targeted sampling and fluorometry completed (GA, VA) (Objective #2) Isolate antibiotic resistant bacteria from poultry litter, swine effluent, cattle manure, and dairy manure as appropriate for each location, and analyze their diversity by traditional and molecular genetic techniques (first year; AL, AR, IN, NY, NC, OK)(2007): (Objective #1) Complete combining targeted sampling and fluorometry in other states (AL, DE, MI, MN, NC, OK, WV). (Objective #2) Isolate antibiotic resistant bacteria from poultry litter, swine effluent, cattle manure, and dairy manure as appropriate for each location, and analyze their diversity by traditional and molecular genetic techniques (second year; AL, AR, IN, NY, NC, OK)
(2008): (Objective #1) Submit first manuscript to refereed journal (Objective #1) Preliminary research on combining targeted sampling and fecal sterols completed (GA, VA) (Objective #2) Analyze the stability of antibiotic resistance genes in bacteria from soils receiving wastes from poultry, swine, cattle, and dairies by traditional and molecular genetic techniques as appropriate for each location (first year; AL, AR, IN, NY, NC, OK) (Objective #2) Submit manuscript to refereed journal
(2009): Objective #1) Complete combining targeted sampling and fecal sterols in other states (AL, DE, MI, MN, NC, OK, WV). Objective #2) Analyze the stability of antibiotic resistance genes in bacteria from soils receiving wastes from poultry, swine, cattle, and dairies by traditional and molecular genetic techniques as appropriate for each location (second year; AL, AR, IN, NY, NC, OK)
(2010): (Objective #1) Submit second manuscript to refereed journal (Objective #2) Submit second manuscript to refereed journal
Projected Participation
View Appendix E: ParticipationOutreach Plan
The project chairperson is responsible for summarizing all the findings from the collaborative research, and ensuring that they are properly disseminated to the research and extension community. This dissemination will occur in six different ways. First, research results will be submitted for publication in refereed scientific journals (e.g., Journal of Environmental Quality) and nonrefereed proceedings (e.g., Proceedings of the Georgia Water Research Conference). Second, research will be presented at appropriate annual (e.g., American Society for Microbiology) and specialized (e.g., Microbial Source Tracking Workshop, 15-18 February 2005, San Antonio, TX) meetings. Third, because the multistate project involves BST, the results will also be submitted to Environmental Detection News. This non-refereed, nontechnical newsletter disseminates research on BST. Fourth, the research will be placed on two websites also designed for BST research, one at Virginia Tech
Organization/Governance
All members of multistate project are considered the members of the technical committee. All members of the technical committee are eligible for office, regardless of their affiliation or sponsoring agency. The technical committee will elect a project chairperson and secretary at the first meeting of the technical committee. The secretary is automatically the project chairperson-elect for the next year. Therefore, only a new secretary is elected annually after the first year. The technical committee will elect a host chairperson for the annual meeting the next year and designate a general site for the annual meeting. There will be one formal meeting per year at a designated site. The meeting will be primarily to discuss the two multistate objectives.
The project chairperson, in consultation with the administrative advisor, will: a) notify all technical committee members of the time and place for the one annual meeting, b) prepare a meeting agenda, c) preside at the meeting, and d) prepare an annual and other reports for the multistate project. The chairperson will give the project annual report to the secretary for uploading to the NIMSS website. The last chairperson of the 5-year project will prepare the final termination report. The chairperson will also consult with the administrative advisor in the conduct of her or his duties.
The secretary will: a) record the minutes of the annual meeting, b) prepare and distribute copies of the minutes to all members, and c) perform any other duties as needed by the project chairperson. In addition, the secretary will ensure that a) the minutes of the annual meeting, b) the individual annual reports, c) the overall annual project report, and d) all other necessary information and attachments are uploaded to the NIMSS website http://nimss.umd.edu.
The host chairperson will make all arrangements for the technical committee at the formal designated annual meeting site (e.g., lodging). The host chairperson is under the direction of the project chairperson.
Each of the two multistate objectives will have a chairperson. The chairperson for each objective will: a) coordinate and tie the research results together, b) report on progress for each objective at the annual meeting, and c) prepare publication of results. Because the chairperson for each objective is responsible for facilitating communication and ensuring collaboration among the multistate researchers, she or he will determine authorship for any scientific publications. It is expected that the individuals in the chair position will rotate during the course of the 5-year-life of the multistate project. The chairperson for each objective will be determined by a vote of the technical committee members at the annual meeting.
Literature Cited
Aminov, R. I., N. Garrigues-Jeanjean and R. I. Mackie. 2001. Molecular ecology of tetracycline resistance: Development and validation of primers for detection of tetracycline resistance genes encoding ribosomal protection proteins. Appl. Environ. Microbiol. 67:22-32.
Amor, K., D. E. Heinrichs, E. Frirdich, K. Ziebell, R. P. Johnson, and C. Whitfield. 2000. Distribution of core oligosaccharide types in lipopolysaccharides from Escherichia coli. Infect. Immun. 68:1116-1124.
Benson, D. A., I. Karsch-Mizrachi, D. J. Lipman, J. Ostell, and D. L. Wheeler. 2003. GenBank. Nucleic Acids Res. 31:23-27.
Clesceri, L. S., A. E. Greenberg, and A. D. Eaton. 1998. Standard methods for the examination of water and wastewater, 20th ed. American Public Health Association, American Water Works Association, and Water Environment Federation, Washington, DC.
Cole, J. R., B. Chai, T. L. Marsh, R. J. Farris, Q. Wang, S. A. Kulam, S. Chandra, D. M. McGarrell, T. M. Schmidt, G. M. Garrity, and J. M. Tiedje. 2003. The Ribosomal Database Project (RDP-II): Previewing a new autoaligner that allows regular updates and the new prokaryotic taxonomy. Nucleic Acids Res. 31:442-443.
Davison, J. 1999. Genetic exchange between bacteria in the environment. Plasmid 42:73-91.
Dombek, P. E., L.-A. K. Johnson, S. T. Zimmerley, and M. J. Sadowsky. 2000. Use of repetitive DNA sequences and the PCR to differentiate Escherichia coli isolates from human and animal sources. Appl. Environ. Microbiol. 66:2572-2577.
Farber, J. M. 1996. An introduction to the hows and whys of molecular typing. J. Food Protect. 59:1091-1101.
Gates, P., P. Hartel, K. Payne, J. McDonald, K. Austin, K. Rodgers, J. Fisher, S. Hemmings, and L. Gentit. 2004. Combining targeted sampling and bacterial source tracking to determine sources of fecal contamination to the south beach of Sea Island during calm and stormy conditions. Department of Natural Resources. 12 p.
Geldreich, E. E., 1970. Applying bacteriological parameters to recreational water quality. J. Am. Water Works Assoc. 62:113-120.
Gilpin, B., T. James, F. Nourozi, D. Saunders, P. Scholes, and M. Savill. 2003. The use of chemical and molecular microbial indicators for faecal source identification. Water Sci. Technol. 47:39-43.
Graves, A. K., C. Hagedorn, A. Teetor, M. Mahal, A. M. Booth, and R. B. Reneau, Jr. 2002. Antibiotic resistance profiles to determine sources of fecal contamination in a rural Virginia watershed. J. Environ. Qual. 31:1300-1308.
Gregor, J., N. Garrett, B. Gilpin, C. Randall, and Darren Saunders. 2002. Use of classification and regression tree (CART) analysis with chemical faecal indicators to determine sources of contamination. N. Z. J. Mar. Freshwater Res. 36:387-398.
Hagedorn, C., R. B. Reneau, Saluta, and A. Chapman. 2003. Impact of onsite wastewater systems on water quality in coastal regions. Virginia Coastal Resources Management Program Memorandum of Agreement - 50312-01-13-PT. Virginia Department of Conservation and Recreation, Virginia Department of Health, 1/28/02 - 6/30/03.
Hagedorn, C., S. L. Robinson, J. R. Filtz, S. M. Grubbs, T. A. Angier, and R. B. Reneau, Jr. 1999. Determining sources of fecal pollution in a rural Virginia watershed with antibiotic resistance patterns in fecal streptococci. Appl. Environ. Microbiol. 65:5522-5531.
Hartel, P. G., J. D. Summer, and W. I. Segars. 2003. Deer diet affects ribotype diversity of Escherichia coli for bacterial source tracking. Water Res. 37:3263-3268.
Hartel, P. G., J. D. Summer, J. L. Hill, J. V. Collins, J. A. Entry, and W. I. Segars. 2002. Geographic variability of Escherichia coli ribotypes from animals in Idaho and Georgia. J. Environ. Qual. 31:1273-1278.
Hartel, P. G., E. A. Frick, A. L. Funk, J. L. Hill, J. D. Summer, and M. B. Gregory. 2004a. Sharing of ribotype patterns of Escherichia coli isolates during baseflow and stormflow conditions. U. S. Geological Survey, Scientific Investigations Report 20045004. 10 p.
Hartel, P., K. Gates, K. Payne, J. McDonald, K. Rogers, J. Fisher, S. Hemmings, and L. Gentit. 2004b. Targeted sampling of St. Andrews Park on Jekyll Island to determine sources of fecal contamination. Department of Natural Resources. 9 p.
Harwood, V. J., J. Whitlock, and V. Withington. 2000. Classification of antibiotic resistance patterns of indicator bacteria by discriminant analysis: Use in predicting the source of fecal contamination in subtropical waters. Appl. Environ. Microbiol. 66:3698-3704.
Jenkins, M. B., P. G. Hartel, T. J. Olexa, and J. A. Stuedemann. 2003. Putative temporal variability of Escherichia coli ribotypes from yearling steers. J. Environ. Qual. 32:305-309.
Kariuki, S., C. Gilks, J. Kimari, A. Obanda, J. Muyodi, P. Waiyaki, and C. A. Hart. 1999. Genotype analysis of Escherichia coli strains isolated from children and chickens living in close contact. Appl. Environ. Microbiol. 65:472-476.
Kelsey, H., D. E. Porter, G. Scott, M. Neet, and D. White. 2004. Using geographic information systems and regression analysis to evaluate relationships between land use and fecal coliform bacterial pollution. J. Exp. Mar. Biol. Ecol. 298:197-209.
Kidambi, S., S. Ripp, and R. Miller. 1994. Evidence for phage-mediated gene transfer among Pseudomonas aeruginosa strains on the phylloplane. Appl. Environ. Microbiol. 60:496-500.
Kuntz, R. L., P. G. Hartel, K. Rodgers, and W. I. Segars. 2004. Presence of Enterococcus faecalis in broiler litter and wild bird feces for bacterial source tracking. Water Res. 38:3551-3557.
Kuntz, R. L., P. G. Hartel, D. G. Godfrey, J. L. McDonald, K. W. Gates, and W. I. Segars. 2003. Targeted sampling protocol as prelude to bacterial source tracking with Enterococcus faecalis. J. Environ. Qual. 32:2311-2318.
LaPara, T. M., C. H. Nakatsu, L. M. Pantea, and J. E. Alleman. 2000. Phylogenetic diversity of bacteria in mesophilic and thermophilic bioreactors treating pharmaceutical wastewater. Appl. Environ. Microbiol. 66:3951-3959.
Leecaster, M. K., and S. B. Weisberg. 2001. Effect of sampling frequency on shoreline microbiology assessments. Marine Pollut. Bull. 42:1150-1154.
Leeming, R., A. Ball, N. Ashbolt, and P. Nichols. 1996. Using fecal sterols from humans and animals to distinguish faecal pollution in receiving waters. Water Res. 30:2893-2900.
Lu, J., S. Sanchez, C. Hofacre, J. J. Maurer, B. G. Harmon, and M. D. Lee. 2003. Evaluation of broiler litter with reference to the microbial composition as assessed by using 16S rRNA and functional gene markers. Appl. Environ. Microbiol. 69:901-908.
Marvin, C., J. Coakley, T. Mayer, M. Brown, and L. Thiessen. 2001. Application of faecal sterol ratios in sediments and effluents as source tracers. Water Qual. Res. J. Canada 36:781-792.
Massol-Deya, A. A., D. A. Odelson, R. F. Hickey, and J. M. Tiedje. 1995. Bacterial community fingerprinting of amplified 16S and 16-23S ribosomal DNA gene sequences and restriction endonuclease analysis (ARDRA). In A. D. Akkerman, J. D. van Elsas, and F. J. de Bruijn (ed.) Molecular microbial ecology. Kluwer Academic, Dordrecht, Netherlands.
Myoda, S. P., C. A. Carson, J. J. Fuhrmann, B.-K. Hahm, P. G. Hartel, L. A. Johnson, R. L. Kuntz, C. H. Nakatsu, M. J. Sadowsky, M. Samadpour, and H. YamparaIquise. 2003. Comparison of genotypic-based microbial source tracking methods requiring a host origin database. J. Water Health 1:167-180.
Nikolich, M., G. Hong, N. Shoemaker, and A. Salyers. 1994. Evidence for natural horizontal transfer of tetQ between bacteria that normally colonize humans and bacteria that normally colonize livestock. Appl. Environ. Microbiol. 60:3255-3260.
Noblet, J. A., D. L. Young, E. Y. Zeng, and S. Ensari. 2004. Use of fecal steroids to infer the sources of fecal indicator bacteria in the Lower Santa Ana River watershed, California: Sewage is unlikely a significant source. Environ. Sci. Technol. 38:6002-6008.
Ochman, H., J. G. Lawrence, and E. A. Groisman. 2000. Lateral gene transfer and the nature of bacterial innovation. Nature 405:299-304.
Parveen, S., K. M. Portier, K. Robinson, L. Edmiston, and M. L. Tamplin. 1999. Discriminant analysis of ribotype profiles of Escherichia coli for differentiating human and nonhuman sources of fecal pollution. Appl. Environ. Microbiol. 65:3142-3147.
Pourcher, A. M., L. A. Devriese, J. F. Hernandez, and J. M. Delattre. 1991. Enumeration by a miniaturized method of Escherichia coli, Streptococcus bovis, and enterococci as indicators of the origin of fecal pollution of waters. J. Appl. Bacteriol. 70:525530.
Salyers, A. A., N. B. Shoemaker, A. M. Stevens, and L.-Y. Li. 1995. Conjugative transposons: An unusual and diverse set of integrated gene transfer elements. Microbiol. Rev. 59:579-590.
Samadpour, M., and N. Chechowitz. 1995. Little Soos Creek microbial source tracking. Report to Surface Water Management Division, King County Department of Public Works, Seattle, Washington.
Scott, T. M., T. M. Jenkins, J. Lukasik, and J. B. Rose. 2005. Potential use of a host associated molecular marker in Enterococcus faecium as an index of human fecal pollution. Environ. Sci. Technol. 39:283-287.
Scott, T. M., J. B. Rose, T. M. Jenkins, S. R. Farrah, and J. Lukasik. 2002. Microbial source tracking: Current methodology and future directions. Appl. Environ. Microbiol. 68:5796-5803.
Simmons, G. M., Jr., S. A. Herbein, and C. M. James. 1995. Managing nonpoint fecal coliform sources to tidal inlets. Universities Council on Water Resources. Water Resourc. Update 100:64-74.
Simpson, J. M., J. W. Santo Domingo, and D. J. Reasoner. 2002. Microbial source tracking: State of the science. Environ. Sci. Technol. 36:5279-5288.
Smalla, K., H. Heuer, A. Gotz, D. Niermeyer, E. Krogerrecklenfort, and E. Tietze. 2000. Exogenous isolation of antibiotic resistance plasmids from piggery manure slurries reveals a high prevalence and diversity in IncQ-like plasmids. Appl. Environ. Microbiol. 66:4854-4862.
Solo-Gabriele, H. M., M. A. Wolfert, T. R. Desmarais, and C. J. Palmer. 2000. Sources of Escherichia coli in a coastal subtropical environment. Appl. Environ. Microbiol. 66:230-237.
Stoeckel, D. M., M. V. Mathes, K. E. Hyers, C. Hagedorn, H. Kator, J. Lukasik, T. L. OBrien, T. W. Fenger, M. Samadpour, K. M. Strickler, and B. A. Wiggins. 2004. Comparison of seven protocols to identify fecal contamination sources using Escherichia coli. Environ. Sci. Technol. 38:6109-6117.
Sylvia, D. M., J. J. Fuhrmann, P. G. Hartel, and D. A. Zuberer. 1998. Principles and applications of soil microbiology. Prentice-Hall, Upper Saddle River, NJ.
Sylvia, D. M., J. J. Fuhrmann, P. G. Hartel, and D. A. Zuberer. 2005. Principles and applications of soil microbiology, 2nd edition. Prentice-Hall, Upper Saddle River, NJ.
USDA-CSREES. 2002. USDA-CSREES Stakeholders workshop. Available at
USEPA (U.S. Environmental Protection Agency). 2002. Implementation guidance for ambient water quality criteria for bacteria. EPA-823-B-02-003. U. S. Government Printing Office, Washington, DC.
Wheeler, A. L., P. G. Hartel, D. G. Godfrey, J. L. Hill, and W. I. Segars. 2002. Potential of Enterococcus faecalis as a human fecal indicator for microbial source tracking. J. Environ. Qual. 31:1286-1293.
Wiggins, B. A. 1996. Discriminant analysis of antibiotic resistance patterns in fecal streptococci, a method to differentiate human and animal sources of fecal pollution in natural waters. Appl. Environ. Microbiol. 62:3997-4002.
Wiggins, B. A., R. W. Andrews, R. A. Conway, C. L. Corr, E. J. Dobratz, D. P. Dougherty, J. R. Eppard, S. R. Knupp, M. C. Limjoco, J. M. Mettenburg, J. M. Rinehardt, J. Sonsino, R. L. Torrijos, and M. E. Zimmerman. 1999. Use of antibiotic resistance analysis to identify nonpoint sources of fecal pollution. Appl. Environ. Microbiol. 65:3483-3486.