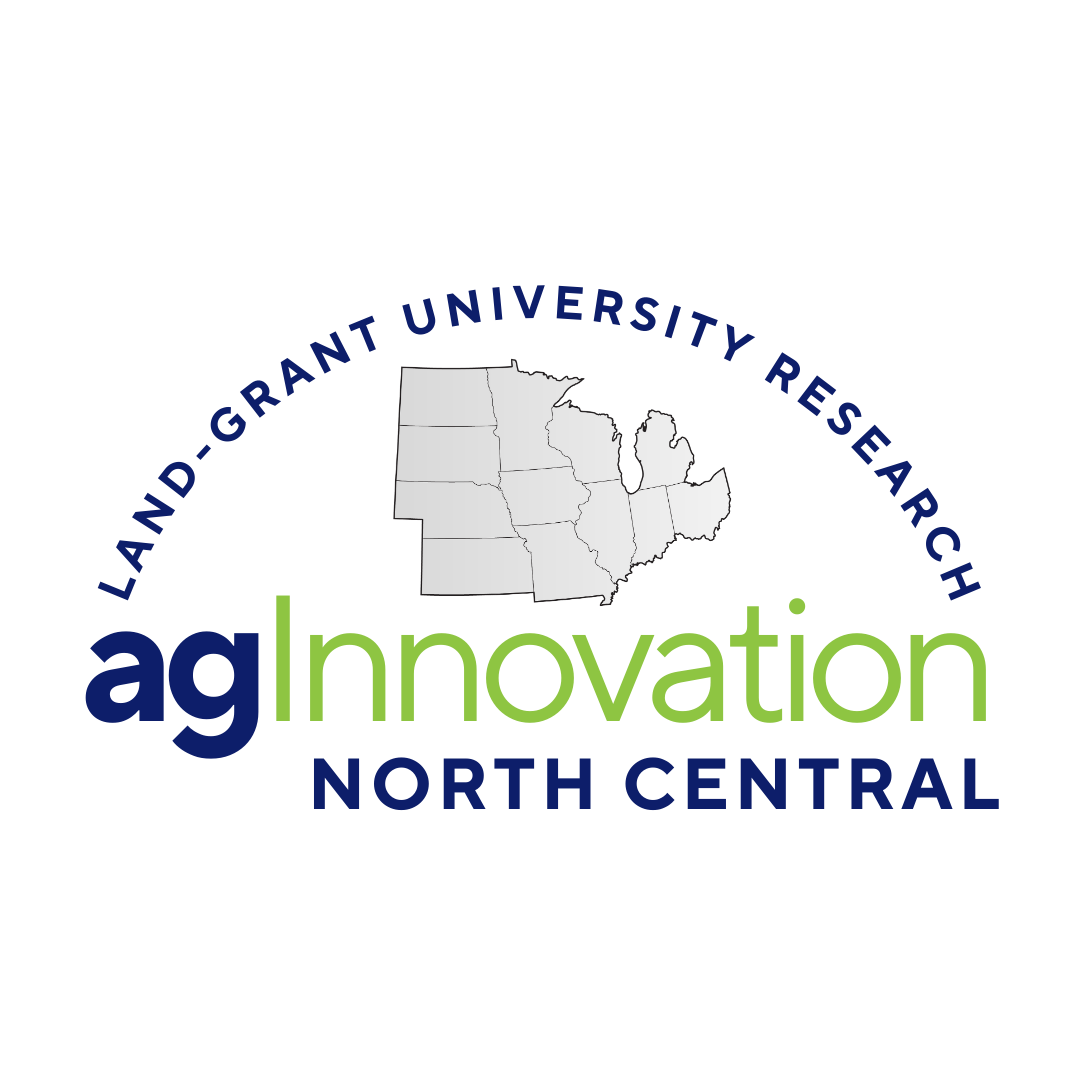
NC1022: The Chemical and Physical Nature of Particulate Matter Affecting Air, Water and Soil Quality. (NCR174)
(Multistate Research Project)
Status: Inactive/Terminating
NC1022: The Chemical and Physical Nature of Particulate Matter Affecting Air, Water and Soil Quality. (NCR174)
Duration: 10/01/2004 to 09/30/2010
Administrative Advisor(s):
NIFA Reps:
Non-Technical Summary
Statement of Issues and Justification
The need as indicated by stakeholders. The newly released National Research Council report entitled "Air Emissions from Animal Feeding Operations: Current Knowledge, Future Needs" (Ad Hoc Committee on Air Emissions from Animal Feeding Operations 2003) addresses a growing public concern about the air quality impact of animal feeding operations. This report comes as the United States Environmental Protection Agency evaluates air quality regulations for agricultural operations. Chief among the recommendations in the NRC report is a systems-based approach that places air emissions from animal feeding operations within the larger agricultural production context. Agricultural practices affect not only air quality, but also soil and water quality for both rural and urban communities. The impacts flows both ways. Activities in urban settings affect soil and water quality for rural and agricultural use, while public concern and regulatory authority to protect the environment impel efforts to preserve and improve environmental quality. Efficient management and effective regulation will optimize environmental and health protection without crippling the economy, rural or urban. The importance of the work and the consequences if it is not done. This much is certain; the demand for reliable information to guide management and regulation of rural air quality outstrips our current scientific understanding. A key agricultural air emission component is particulate matter. Some agricultural sources are very similar to urban sources including diesel engine exhaust and agricultural burning. It is well known, for instance, that agricultural use of plastic film is increasing. Much of the waste plastic film is currently disposed by on-farm burning. The rural particulate matter source that differs from urban settings is resuspended solids arising from cultivation and animal feeding operations. In urban settings resuspended particulate matter is primarily road dust. Synchrotron-based analytical methods are unrivaled in their capacity for analyzing the elemental, chemical and mineralogical composition of micrometer- and nanometer-sized particulates. This will allow researchers to characterize particulate material from agricultural operations as required for effective management and air quality regulation. By way of background, synchrotrons are large machines that produce extremely intense beams of "synchrotron light" that are useful for research in many fields of science. Synchrotron light is emitted at wavelengths from the infrared to the hard x-ray region of the electromagnetic spectrum, and the brightness synchrotron beams can be more than a billion times greater than typical laboratory sources. As a result, scientists can study smaller samples at higher spatial resolution and higher analytical sensitivity than is ever possible using normal laboratory-based instruments.The past decade has witnessed an accelerating pace in soil and water research that can be traced in no small measure to research conducted at synchrotron facilities. While synchrotron facilities offer a broad suite of experimental methods promoting these advances, the impact does not end with the instrumental capabilities. Synchrotron facilities are also institutions that foster scientific exchange within the community of soil and water researchers using the facility and between members of different scientific disciplines such as geoscience, biology, chemistry, and physics. This project would expand the community using synchrotron facilities beyond the soil and water researchers who became involved in the past decade to include a broader cross section of scientists working to support US agriculture, notability atmospheric scientists. The technical feasibility of the research. A common thread that runs through much environmental research is the importance of processes that operate simultaneously on different spatial and temporal scales. For instance, major questions surround particulate matter affecting rural air quality. The technical feasibility of applying synchrotron-based methods to a wide range of sample sizes and chemical compositions is amply supported by the current scientific literature. The advantages for doing the work as a multi-state effort. There are several advantages in doing this project as a multi-state effort. Frist, particulate matter (PM) is transported across state and regional boundaries by both air and water making it a regional rather than local problem. Second, just as urban PM emissions vary considerably from one metropolitan area to another, we can expect rural PM emissions also vary because livestock industry, crops, farming practices, soils, and water chemistry vary regionally. Third, the central focus of this project (synchrotron spectro-microscopy) demands extensive cooperation among members: sharing experience with specific facilities and microscopy techniques and sharing disciplinary expertise (soil, water and air chemistry, microbiology, etc.). Fourth, this project has two implicit strategies for reaching its objectives. It would promote soil and water chemists with synchrotron experience to acquire synchrotron spectro-microscopy experience and it would establish collaborative relationships that do not already exist between scientists studying agricultural particulates and environmental scientists with synchrotron experience. The latter logically begins with sharing samples and data for synchrotron spectro-microscopy analysis but would yield optimal progress if the collaborations went deeper. Synchrotron rsearch by soil scientists primarily focused in the past on industrial contaminants, i.e., metals and metalloids. There are many opportunities now with micro-focused techniques to study agricultural contaminants such as metals, phosphate, etc. in biosolid amended soils and biosolid materials that will link more basic soil science with applied soil science particularly in the area of nutrient management. Finally, the members have already established an excellent record of multi-state collaboration. There is no reason this will diminish. What the likely impacts will be from successfully completing the work. Recent advances in synchrotron facilities clearly demonstrate the rich chemical and physical information that can be acquired from micrometer- and nanometer-sized particles. Thus, we can anticipate developing a detailed picture of the particulate matter from agricultural emissions. Dramatic advances in soil and water chemistry over the past decade show no signs of lagging with the most notable developments coming as scientists begin to apply spectro-microscopic methods to chemically heterogeneous materials from soils and sediments. No doubt collaboration between chemists and microbiologists will grow as scientists characterize the microenvironment of microbes in situ.
Related, Current and Previous Work
The multi-state projects listed below are related to our proposed project in some manner. The potential for duplication arises either from the research focus of the project or its approach. Several projects address chemical aspects of soil and water quality but no other project integrates air, soil and water quality nor do any of these projects employ synchrotron methods. This project replaces NCR-174, which promoted synchrotron methods in soil and water quality research. broadens the scope to include the chemistry of particulate matter in air emissions from agricultural activities, and sharpens the approach to emphasize synchrotron spectro-microscopy.
Designation | Project Title |
NRSP-3 | The National Atmospheric Deposition Program |
NE-1001 | Application of Sewage Biosolids to Agricultural Soils in the Northeast: Long-term Impacts and Benefit Uses |
NCR-189 | Air Qualtiy Issues Associated with Animal Facilities |
S-219 | Systems for Controlling Air Pollutant Emissions and Indoor Environments of Poultry, Swine, and Dairy Facilities |
S-1000 | Animal Manure and Waste Utilization, Treatment and Nuisance Avoidance for a Sustainable Agriculture |
S-1011 | Water Quality Methodology for Crop Protection Chemicals |
S-1014 | Mineral Controls on P Retention and Release in Soils and Soil Amendments |
W-170 | Chemistry and Bioavailability of Waste Constituents in Soils |
W-195 | Water Quality Issues in Poultry Production and Processing |
Objectives
-
Determine the physical, chemical, and biological nature of micrometer- and nanometer-sized particulate matter from agricultural emissions as it relates to air, water and soil quality along with the associated health and environmental impacts. Participants contributing to this objective: AL, DE, GA, ID, IN, MI, MN, ND, NJ, NY, SC, TX, WA, WI.
-
Characterize particulate matter properties that control the biological availability and cycling of essential nutrients (e.g., phosphorus and sulfur) and toxic substances (e.g., heavy metals) in the air, water and soil. Participants contributing to this objective: AL, DE, GA, ID, IN, MI, MN, ND, NJ, NY, SC, TX, WA, WI.
-
Assemble microscopic-scale maps of soil and water colloids that reveal the distribution and chemical speciation of nutrients, toxicants or other compounds influencing microbial responses, at the cellular level, to environmental stress (e.g., nutrient deficiency, toxicity, lack of moisture, etc.). Participants contributing to this objective: ID, MI, MN, ND, NJ, NY, SC, TX, WA, WI.
-
Assemble microscopic-scale maps of the soil rhizosphere that reveal the distribution and chemical speciation of nutrients, toxicants or other compounds associated with plant root responses, at the cellular or tissue level, to environmental stress (e.g., deficiency, toxicity, anoxia, etc.). Participants contributing to this objective: DE, GA, IN, MI, NJ, WI.
-
Acquire the synchrotron facility resources necessary to accomplish the broad scientific goals of this project and allocate those resources to scientists involved in research that relates air, water or soil quality to US agriculture. Participants leading efforts toward this objective: DE (Sparks), IN (Schulze), GA (Bertsch), WI (Bleam, Schauer).
Methods
Atmospheric particulate matter can be divided in primary and secondary pollutants. Primary particulate matter is directly emitted from air pollution sources and includes emissions from combustion sources and resuspended soil materials. Secondary particulate matter is formed in the atmosphere by chemical reactions of gaseous pollutants that yield low vapor pressure products that condense to form particulate matter in the atmosphere. Secondary pollutants such as ammonium sulfate, ammonium nitrate, and secondary organic aerosols tend to be regional air quality problems that equally impact rural and urban locations. In contrast, primary emissions of particulate matter tend to be localized air quality problems. In the past several decades, the scientific and regulatory communities' understanding of the formation and impact of secondary particulate matter has greatly increased but only limited tools are presently available to chemical characterize the organic and trace metals in atmospheric particulate matter. Tools to characterize high molecular weight organic constituents of atmospheric particulate matter and to speciate metals in atmospheric particulate matter have not really been employed to better understand the composition and related toxicity of atmospheric particulate matter. Due to the fact that synchrotron techniques have been widely used in the aquatic and terrestrial chemistry and toxicology field for exactly these purposes, there is a great opportunity to extend these tools to the study of atmospheric particulate matter. It is now widely accepted that specific information on chemical speciation and chemical transformations is prerequisite to the development of a comprehensive understanding of toxic element and nutrient behavior in the environment. Unfortunately, our understanding of the importance of chemical speciation has advanced more rapidly than the analytical capabilities to determine it, particularly in complex systems such as soils, sediments, aerosols, and biological samples. Conventional spectroscopic and analytical methods of investigating chemical speciation often lack the sensitivity required and usually prohibit investigation under environmentally relevant conditions, for example on moist samples using minimal or no chemical pretreatment. In contrast, synchrotron-based techniques have emerged as powerful tools for determining the chemical speciation of a wide variety of toxic elements in moist soil samples, waste-forms, and biological specimens with little or no chemical pretreatment at detection limits that, on the average, exceed those of conventional methods by several orders-of-magnitude. The ability to probe matter to determine the chemical state of a system at high spatial resolution with high elemental sensitivity has been important to a number of fields, including soil and environmental sciences, biosciences, colloid and surface science, and materials science, among others. The need for such data at high spatial resolution is a result of the heterogeneous nature of samples commonly examined in these disciplines and the importance of understanding how elemental and molecular distributions influence heterogeneous chemical reactivity or, conversely, how chemical reactivity influences heterogeneous elemental and molecular distributions. Traditional spectroscopic measurements of complex samples provide volume-averaged data that obscures important spatially variable chemical information. The ability to probe homogeneous or less heterogeneous domains within complex samples at high spatial resolution with minimal sample preparation provides an opportunity for more detailed molecular level characterization. Environmental samples, including plants, soils, and aerosols are very heterogeneous and understanding the spatial and temporal patterns of elemental distributions, concentrations, and molecular forms provides significant insights into the biogeochemical processes controlling the dynamics of nutrients and contaminants in managed and natural systems. The past decade has witnessed significant advances in technologies related to spatially resolved x-ray spectroscopic techniques, both as a result of advances in x-ray optics, focusing devices, and detectors and because of greater availability of high brilliance synchrotron facilities world-wide. The result is that spatially resolved synchrotron-based x-ray fluorescence and x-ray absorption fine structure spectroscopy (XAFS) have become a mainstream techniques in a number of scientific disciplines and are providing molecular- level information not previously available using other techniques. While there exists a variety of microanalytical techniques that have long been used in these disciplines, synchrotron-based spatially resolved X-ray fluorescence spectroscopy (SXRF) and XAFS spectroscopy are emerging as important methods that compliment characterization by traditional techniques, as well as by other emerging methods, such as spatially resolved luminescence, FTIR, and Raman spectroscopy. The reasons for this are the elemental specificity, low detection limits, non-destructive nature of the measurement, the ability, in many instances, to examine samples in situ, and the ability to extract information on valence states and on specific bonding environments or molecular forms of target elements in complex matrices. X-ray fluorescence (XRF) has been long used as a technique for determining elemental concentrations in a variety of matrices (Bertsch and Hunter 2001). Synchrotron-based XRF (SXRF) techniques have decreased the detection limits by several orders-of-magnitude over conventional XRF techniques, being in the 50-100 ng g-1 range for many elements at third generation synchrotron facilities. Additionally, the enhanced brilliance of modern synchrotron facilities and advances in focusing optics, allow for spatial resolution in the 0.5 to 1 _m range for hard x-rays (>3 keV) and ~50 nm for soft X-rays (< 1 keV). The ability to access both hard and soft X-rays allows the investigation of most important nutrient elements (P, S, B, N) and C as well as transition and heavier elements. Spatially resolved SXRF imaging has become a critical complimentary technique that is a prerequisite for conducting spatially resolved XAFS spectroscopy for samples with heterogeneous distributions of target elements. Because spatially resolved SXRF is a relatively rapid method, it can be used to efficiently examine complex samples to determine if there is heterogeneous elemental distributions on a spatial scale that is resolvable by the technique. Generation of elemental distribution maps can then be used to identify localized regions of target elements with rather straightforward determination of their concentrations. Mineral phase associations and chemical speciation of target elements can also be inferred based on elemental associations elucidated in elemental maps. Recent advances in the simulation of x-ray fluorescence spectra have greatly improved the predictability of photon-matter interactions for high energy, linearly polarized monochromatic and polychromatic radiation typically available at modern synchrotron facilities. These advances in data analyses, along with specific consideration of particle topology, are greatly enhancing instrument calibration, allowing for optimization of detection geometry for specific samples, and, therefore, improving quantitative analysis of complex samples (Bertsch and Hunter 2001). Based on elemental distribution data generated by SXRF, spatially resolved XAFS experiments can be efficiently devised. XAFS spectroscopic interrogation of regions with elevated localized concentrations of target elements can then be conducted to extract information on valence states, symmetry, electronic states, and the identity, number, as well as bond lengths of neighboring atoms. Often the bulk concentrations of target elements within a sample are well below the detection limits of conventional XRF and XAFS spectroscopic techniques. If the element is localized within the sample, however, the concentration within the localized volume can be many orders-of-magnitude greater than the bulk concentration and this is often coupled to a decreased background as a result of lower than average concentrations of other common heavier elements, such as Fe, in the localized volume element. Thus, localization of elements in complex samples and the ability to analyze the localized volume often results in concentrations that are well within the detection limits of a synchrotron-based x-ray microprobe. Also, the ability to probe discrete domains containing a target element in a complex mosaic often limits the population of coordination environments, thus increasing the quality of XAFS data and facilitating data analysis for complex natural or synthesized composite samples having spatially variable composition. In much the same way, soft X-ray microscopy, which utilizes spectral features indicative of molecular structure of the dominant absorbing atoms for contrast, is often coupled with spatially resolved XANES to derive detailed information on elemental and molecular distributions in complex media. There are a number of advantages of micro-SXRF over other commonly employed microprobe technique. While electron microprobe techniques offer outstanding spatial resolution, they suffer from relatively poor sensitivity (especially for transition and heavier elements), require a UHV environment, and can result in appreciably greater beam damage to the sample. Likewise, particle induced x-ray emission (PIXE), although achieving comparable spatial resolution with SXRF, is not as sensitive for heavier elements and can impart beam damage to the sample. Advantages of SXRF over the more widely employed electron microprobe techniques include superior detection limits for (sub ug g-1) most elements, especially Z>20, the ability to analyze non-conducting materials in situ and without coating, and the non-destructive nature of the measurement. The benefits are even more dramatic for XAFS spectroscopy compared to electron energy loss spectroscopy (EELS) since valence states, which are of primary concern in most investigations, can be influenced within the electron beam and because the critical energy dose for radiation damage induced by electrons is an order-of-magnitude or more lower than for x-rays. Advances in optics and detectors coupled to the commissioning of high brilliance third generation hard and soft X-ray sources worldwide have resulted in major advances in the capabilities of X-ray microprobes and microscopes. Sub-micrometer lateral resolution is becoming routine for hard X-ray microprobes with unprecedented photon fluxes. Likewise, sub 100 nm lateral resolution is becoming routine for soft X-ray microscopes and various measurement strategies and specialized sample chambers are expanding the range of samples that can be examined. Over the past decade, and especially in the past five years, synchrotron based X-ray microprobes and microscopes have moved from being exotic techniques to techniques that are commonly employed for the characterization of complex natural and synthesized materials. These techniques are also providing unprecedented opportunities to examine samples and follow reactions under ambient conditions that are not accessible with more traditional imaging and spectroscopic methods. We expect that this trend will continue, particularly as more beamlines are commissioned at third generation sources. Micro-X-ray diffraction (XRD) capabilities are becoming common on many X-ray microprobes, allowing for SXRF imaging, micro-XAFS, spectromicroscopy, and micro-XRD in the same micrometer-sized regions within a sample. Micro-XRD maps can also be constructed from two dimensional intensity contours. Such an approach has also been used with a STXM to obtain phase and amplitude images of objects with a spatial resolution of ~45nm. Another very powerful technique that is developing rapidly, especially at third generation synchrotron radiation sources is X-ray tomography. The technique expands on the normal X-ray microprobe where a sample, in addition to being rastered in the X and Y directions, is also rotated 360 degrees. This enables three dimensional microscopic images to be obtained nondestructively on relatively large samples, with similar spatial resolution achieved on the X-ray microprobe. Imaging can be conducted with white light as well as with monochromatic radiation. In principle, spectromicroscopy can be conducted in three dimensions using this approach. This capability will greatly enhance the ability to study complex samples to examine interior pore structures, inclusions, secondary phases, and other features within the sample without the need for sectioning. Diffraction anomalous fine structure (DAFS) spectroscopy which provides site selective XAFS-like data through resonant X-ray scattering or diffraction also provides significant potential for characterizing micro-regions in complex samples. Finally, a recent development that may hold potential for future X-ray microprobes involves collection of XRF images using microchannel plate(MCP) relay optics. In this application, the whole sample is irradiated and the fluorescent X-rays are focussed onto a CCD by a MCP. This would allow for rapid generation of SXRF images of large samples. If the system could be used with a monochromator, it could provide for full XAFS spectra to be collected at each pixel simultaneously. While there are certainly a number of challenges with collecting high quality XAFS data in this manner, the possibilities for characterizing complex heterogeneous samples are intriguing. The ability to examine complex samples with high spatial resolution and chemical specificity with minimal sample manipulation is critical to detailed materials characterization. While X-ray microprobes and microscopes are not appropriate for every application, they do provide the ability to characterize many samples in a way not possible using more conventional microprobe methods. For the most demanding samples that need to be examined wet and under ambient pressure, few methods provide the versatility, the spatial resolution, and the molecular-level information provided by X-ray microscopy, micro-SXRF, micro-XAFS, and spectromicroscopy. We anticipate that these techniques will become mainstream analytical, imaging, and spectroscopic tools in a number of scientific fields over the next decade. Management Plan for Accomplishing Objective 5: The members of this project will seek additional funding from the USDA National Research Initiative and other sources to secure synchrotron time at one or more synchrotron sources. This is the essence of Objective 5. The accomplishment of Objectives 1-4 are not contingent on acquisition of this funding but success on Objective 5 will definitely enhance productivity and progress on Objectives 1-4. Objective 5, if funding is secured, will be the management of synchrotron time that leads to progress toward Objectives 1-4. The scientist awarded synchrotron time will not be restricted to official members of this multi-state project but the research allocated synchrotron time must lead to the fulfillment of Objectives 1-4 of this project. This multi-state project will manage the allocation of synchrotron time through two evaluation steps. First, the officers of the multi-state project will release a request for proposals that stipulates the synchrotron facilities where the research will be performed and the scientific priorities that the research must address (Objectives 1-4). The proposals will be submitted directly to the synchrotron facilities and undergo scientific review through the standard review process used at those facilities. This review will furnish a scientific rating for each proposal independent of the multi-state project. All of these proposals will be forwarded, along with their respective scientific ratings, to the national program staff of the USDA National Research Initiative and other agencies funding synchrotron time for a "program relevancy" review. This second review will rate proposals based on their relevancy toward Objectives 1-4 of this project. A number of the proposals processed by this project would be awarded synchrotron time at the facilities where they were submitted regardless. The proposals that would be awarded synchrotron time under the direct control of this project would fall into two categories. The first would be proposals that were awarded "general user" time but received less time than requested. We would award additional time to supplement that already awarded by the facility. The second groups would be proposals with high scientific merit but failing to "make the cut" because of insufficient "general user" time. We would award time to projects that would otherwise not be awarded synchrotron time by the facility. This approach would allow the multi-state project to use the synchrotron time we control to greatest effect: not awarding time to those projects that would receive time without our assistance, supplementing projects that receive less time than they would otherwise receive and awarding time to those projects which have high scientific merit and relevancy to the Objectives 1-4 but fail to "make the cut" when all "general user" time is awarded. The annual "all hands" meeting, publishing of a proceedings for those meetings, and annual progress reports spelled out in the "Organization" section of this proposal provide additional details on the fulfillment of Objective 5. To facilitate cooperation among project participants we will organize four working groups around classes of particulate matter and microenviroments existing in aggregated particulate matter: animal waste, air quality, rhizosphere, and soil and water colloids. Sparks (DE) will coordinate the animal-waste working group involving participants from AL, DE, ND, TX. This working group will examine animal-waste particulates to better understand chemical and biological transformations during storage, treatment to stabilize nutrients and toxicants, and following land application. Whenever feasible this working group will acquire and distribute animal-waste particulate samples to working-group members to take full advantage of participants studying related processes or elements. In particular, this group will use synchrotron spectromicroscopy to characterize phosphorus and key additives (e.g., arsenic) that pose the greatest environmental risk, seeking to develop a comprehensive understanding of biological availability in soil and water and to identify promising treatment methods to limit environmental damage or health risk. Schauer and Bleam (WI) will coordinate the air-quality working group involving participants from IN, ND, NJ, NY and WI. This working group will study airborne particulates to better understand chemical and biological properties and incipient health risks posed by airborne agricultural emissions. This working group will acquire (from national or regional air sampling networks such as CASTNET or through their own sampling efforts) and distribute airborne particulate samples to working-group members and coordinate studies to minimize duplication and maximize synergy among working-group members. This group will concentrate on the chemical speciation of metals and organic components in agricultural airborne particulate matter. Schulze (IN) will coordinate the rhizosphere working group involving participants from GA, IN, MI, NJ, and WI. This working group will characterize soil particulates in direct contact with plant roots, using spectromicroscopy to assess microenvironments that contain root tissue and microbial communities. This working group will share research techniques and, where necessary, equipment (sample cells, in particular), and coordinate research activities that characterize transformations that increase nutrient and toxicant uptake and element redistribution in the rhizosphere. Bertsch (GA) will coordinate the soil-water colloid working group involving participants from DE, GA, ID, IN, MI, MN, SC, TX, WA and WI. This working group will focus on colloids suspended in water (both surface and subsurface) and colloidal soil particles where rhizosphere processes are not dominant. This working group will collect colloidal samples and distribute them among working-group members, coordinating research activities that idenfiy colloids controlling toxicant sequestration and transport. This group will concentrate on correlating microXRD (in-situ identification of trace mineral particulates) and microXAS studies (in-situ element-specific chemical-speciation maps).Measurement of Progress and Results
Outputs
- The results of research conducted under this project will be published in peer-reviewed scientific journals and presented at scientific meetings. The multi-state committee will also compile an annual list of all research outputs by official members and users arising from the multi-state project research activities or synchrotron time allocated by the multi-state project. The research outputs compiled will consist of presentations made at scientific conferences, publications in peer-reviewed journals, and other scholarly publications.
- The immediate research product of the multi-state project will be the technical proceedings of the annual "all hands" meeting. All groups represented in the multi-state project are required to submit a research report at the annual "all hands" meeting, consisting of an abstract and either a oral or poster presentation during the technical program. If synchrotron funding is secured, each group allocated synchrotron during a given year time (referred to as a "user", see Objective 5) is required to attend the "all hands" meeting and present a research report: abstract and a presentation during the technical program. The secretary is responsible to assembling the technical proceedings: the technical program and abstracts of all presentations.
Outcomes or Projected Impacts
- The anticipated outcome is development of fundamental knowledge of the chemistry and mineralogy of particulate matter that affects soil, water and air quality. A key motivation for this project is a concern about the health and environmental effects of air emissions from animal feeding operations and other agricultural activities (Ad Hoc Committee on Air Emissions from Animal Feeding Operations 2003). Standards exist for urban emissions but since emissions form agricultural activities and settings differ substantially from urban emissions, existing air quality standards are deemed inadequate. One outcome of this project is the chemical and physical characterization of agricultural emissions that would support air quality standards suitable for these emissions.
- The scientific community investigating soil and water quality problems using synchrotron methods gained considerable experience through the efforts of NCR-174. While NCR-174 promoted the use of synchrotron methods, it did not focus the activities of its members on any particular problem. This project will coordinate and focus the efforts of a large community of scientists on a targeted set of objectives that evaluate the chemistry of particulates in air, soil and water. We anticipate no less progress by the scientists associated with this project than can be credited to NCR-174. Perhaps the best estimate of the potential impact of this project would be to imagine a level of productivity equivalent to NCR-174 but more sharply focused on and responsive to national priorities.
- Spectro-microscopy using synchrotron sources emerged in recent years, providing investigators with chemical information fundamentally equivalent to data collected in the past decade on the bulk scale. Investigators are familiar with the spectroscopy but must develop new skills, new approaches and address new challenges as they incorporate the microscopy component. This project will accelerate the application of these new methods and equip graduate students with skills and provide much needed experience that foster additional advances beyond the narrow scope of this project.
Milestones
(2005): <b>Proposal Working Group</b>: <br> An ad hoc committee appointed by the project chair will prepare and submit proposal(s) to NRI and other programs to fund synchrotron time consistent with Objective 5.(2005): <b>All Working Groups</b>: <br> Make decisions on the acquisition and sharing of particulate samples and/or equipment and develop coherent plans to coordinate specific research tasks.
(0):Animal-Waste Working Group</b>: <br> 2005: Coordinate methods development for mounting animal waste samples for spectromicroscopic analysis.<p> 2006: Examine animal waste transformations during storage or stockpiling.<p> 2007: Organize annual "all-hands" meeting in conjunction with another multi-state project studying animal waste and manure. Examine transformations affecting the biological availability of animal-waste constituents following land application.<p> 2008: Initiate evaluation of animal-waste treatment designed to reduce the environmental damage and health risk resulting from storage and land application.<p> 2009: Organize symposium during annual "all-hands" meeting to feature spectromicroscopic advances in animal-waste research. Invite stakeholders representing the animal-waste management community.
(0):>Air-Quality Working Group</b>: <br> 2005: Organize annual "all-hands" meeting in conjunction with NRSP-3 (The National Atmospheric Deposition Program), S-291 (Systems for Controlling Air Pollutant Emissions and Indoor Environments of Poultry, Swine, and Dairy Facilities) or NCR-189 (Air Qualtiy Issues Associated with Animal Facilities). Invite representatives from U.S. Environmental Protection Agency and state environmental quality agencies nationwide. Initiate spectromicroscopic analysis using both hard and soft x-ray radiation to the chemical speciation of metals.<p> 2007: Initiate soft x-ray spectromicroscopic analysis of complex organic components in air borne agricultural emissions.<p> 2008: Organize annual "all-hands" meeting in conjunction with NRSP-3, S-291 or NCR-189 with the goal of reporting results from collaborations setup during joint meeting held 2005. Invite stakeholders representing the agricultural air-quality community.<p> 2009: Organize symposium during annual "all-hands" meeting to feature spectromicroscopic advances in agricultural air-quality research. Invite stakeholders representing the agricultural air-quality community.
(0):Rhizosphere Working Group</b>: <br> 2005: Organize symposium during annual "all-hands" meeting to evaluate current status of rhizosphere and plant root research. Invite stakeholders representing the crop-production community. Coordinate methods development for mounting soil rhizosphere samples for spectromicroscopic analysis.<p> 2006: Initiate micro-XRD and micro-SXRF studies identify key minerals affected by rhizosphere conditions influencing the distribution, chemical speciation and biological availability of both nutrients and contaminants.<p> 2008: Initiate micro-XANES and micro-XAFS studies to identify changes in chemical speciation directly linked to rhizosphere conditions.<p> 2009: Organize symposium during annual "all-hands" meeting to feature spectromicroscopic advances in rhizosphere and plant root research. Invite stakeholders representing the crop-production community.
(0):-Water Colloid Working Group</b>: <br> 2005: Initiate micro-XRD and micro-SXRF studies designed to identify key trace minerals controlling nutrient and contaminant sequestration by soil oxide and silicate colloids.<p> 2006: Organize annual "all-hands" meeting in conjunction with another multi-state project studying the impact of agriculture on soil or water quality. Invite representatives from U.S. Environmental Protection Agency, Natural Resource Conservation Service, Agricultural Research Service and state environmental quality agencies nationwide. <p> 2007: Initiate micro-XRD and micro-SXRF studies designed to identify key tr
Projected Participation
View Appendix E: ParticipationOutreach Plan
The outreach plan is integrated as Objective 5. Beyond the more conventional outreach activities (presentations at professional meetings, publications in peer-reviewed journals), this project would use the targeted allocation of synchrotron time and annual "all hands" meetings to expand the scientific community working toward the objectives of this project.
Organization/Governance
Governance of the multi-state project will lie with three elected positions: chair, vice-chair and secretary. Each position is assigned specific tasks and is held for two years with officers rotating through the positions as follows: the chair steps down, the vice-chair becomes chair, the secretary becomes vice-chair and a newly elected individual becomes secretary. The persons who drafted the multi-state proposal (Will Bleam, Wisconsin; Don Sparks, Delaware; James Schauer, Wisconsin; Darrell Schulze, Purdue) will organize the first election of officers immediately upon authorization of the multi-state project. One order of business at each "all hands" meeting will be the election of a new secretary. New officers take responsibility upon conclusion of each "all hands" meeting. The chair is responsible for organizing the technical program for the upcoming "all hands" meeting. Each official group on the multi-state project is required to present a research report at each "all hands" meeting. If synchrotron funding is secured, each group allocated synchrotron time (Objective 5) during a given year is required to attend the "all hands" meeting and present a research report. The research report will consist of an abstract and either an oral presentation or a poster presentation. If synchrotron funding is secured, the chair will be the official liaison with funding source(s) and synchrotron facilities consistent with Objective 5. The vice-chair is responsible for organizing the logistics for the "all hands" meeting: selecting a venue, reserving rooms suitable for research presentations and the business meeting, arranging housing, etc. The secretary is responsible for all communication with official members of the multi-state project and, if synchrotron funding is secured, individuals allocated synchrotron time through Objective 5. The secretary is also responsible for preparing the annual report for the multi-state project which will include the proceedings of the "all hands" meeting: minutes of the business meeting, the technical program and the abstracts of all research reports. Finally, if synchrotron funding is secured the chair, vice-chair and secretary will be responsible for preparing the annual progress report for the funding source(s) and submitting any renewal proposals required for the upcoming year. This responsibility may be assigned to a committee appointed at the discretion of the officers.
Literature Cited
Ad Hoc Committee on Air Emissions from Animal Feeding Operations, C. o. A. N., National Research Council (2003). Air Emissions from Animal Feeding Operations: Current Knowledge, Future Needs. Washington, DC, National Research Council: 286. Bertsch, P. M. and D. B. Hunter (2001). "Applications of synchrotron-based X-ray microprobes." Chemical Reviews 101(6): 1809-1842. Bertsch, P. M., D. B. Hunter, et al. (1994). "In-Situ Chemical Speciation of Uranium in Soils and Sediments by Micro-X-Ray Absorption-Spectroscopy." Environmental Science & Technology 28(5): 980-984. Boese, J., A. Osanna, et al. (1997). "Carbon edge XANES spectroscopy of amino acids and peptides." Journal of Electron Spectroscopy and Related Phenomena 85(1-2): 9-15. De Stasio, G., L. Perfetti, et al. (1999). "MEPHISTO spectromicroscope reaches 20 nm lateral resolution." Review of Scientific Instruments 70(3): 1740-1742. Duff, M. C., D. B. Hunter, et al. (1999). "Factors influencing uranium reduction and solubility in evaporation pond sediments." Biogeochemistry 45(1): 95-114. Duff, M. C., D. B. Hunter, et al. (2001). "Comparison of two micro-analytical methods for detecting the spatial distribution of sorbed Pu on geologic materials." Journal of Contaminant Hydrology 47(2-4): 211-218. Duff, M. C., D. B. Hunter, et al. (1999). "Mineral associations and average oxidation states of sorbed Pu on tuff." Environmental Science & Technology 33(13): 2163-2169. Duff, M. C., D. E. Morris, et al. (2000). "Spectroscopic characterization of uranium in evaporation basin sediments." Geochimica Et Cosmochimica Acta 64(9): 1535-1550. Duff, M. C., M. Newville, et al. (1999). "Micro-XAS studies with sorbed plutonium on tuff." Journal of Synchrotron Radiation 6: 350-352. Gilbert, B., R. Andres, et al. (2000). "Charging phenomena in PEEM imaging and spectroscopy." Ultramicroscopy 83(1-2): 129-139. Gilbert, B., G. Margaritondo, et al. (2001). "XANES microspectroscopy of biominerals with photoconductive charge compensation." Journal of Electron Spectroscopy and Related Phenomena 114: 1005-1011. Gilbert, B., G. Margaritondo, et al. (2001). "Synchrotron spectromicroscopy in medicine and biology." Journal of Alloys and Compounds 328(1-2): 8-13. Hitchcock, A. P., C. Morin, et al. (2002). "Towards practical soft X-ray spectromicroscopy of biomaterials." Journal of Biomaterials Science-Polymer Edition 13(8): 919-937. Hitchcock, A. P., C. Morin, et al. (2002). "Soft X-ray microscopy of soft matter - Hard information from two softs." Surface Review & Letters 9(1): 193-201. Jacobsen, C. (1999). "Soft x-ray microscopy." Trends in Cell Biology 9(2): 44-47. Jacobsen, C., M. Feser, et al. (2003). "Cluster analysis of soft X-ray spectromicroscopy data." Journal de Physique IV 104: 623-626. Jacobsen, C., S. Wirick, et al. (2000). "Soft X-ray spectroscopy from image sequences with sub-100 nm spatial resolution." Journal of Microscopy-Oxford 197: 173-184. Kaplan, D. I., D. B. Hunter, et al. (1994). "Application of Synchrotron X-Ray-Fluorescence Spectroscopy and Energy-Dispersive X-Ray-Analysis to Identify Contaminant Metals on Groundwater Colloids." Environmental Science & Technology 28(6): 1186-1189. Koprinarov, I. N., A. P. Hitchcock, et al. (2002). "Quantitative mapping of structured polymeric systems using singular value decomposition analysis of soft X-ray images." Journal of Physical Chemistry B 106(21): 5358-5364. Lawrence, J. R., G. D. W. Swerhone, et al. (2003). "Scanning transmission X-ray, laser scanning, and transmission electron microscopy mapping of the exopolymeric matrix of microbial biofilms." Applied & Environmental Microbiology 69(9): 5543-5554. Loo, B. W., I. M. Sauerwald, et al. (2001). "A new sample preparation method for biological soft X-ray microscopy: nitrogen-based contrast and radiation tolerance properties of glycol methacrylate-embedded and sectioned tissue." Journal of Microscopy-Oxford 204: 69-86. Manceau, A., N. Tamura, et al. (2003). "Molecular-scale speciation of Zn and Ni in soil ferromanganese nodules from loess soils of the Mississippi Basin." Environmental Science & Technology 37(1): 75-80. Manceau, A., N. Tamura, et al. (2002). "Deciphering Ni sequestration in soil ferromanganese nodules by combining X-ray fluorescence, absorption, and diffraction at micrometer scales of resolution." American Mineralogist 87(10): 1494-1499. Margaritondo, G. and G. DeStasio (1997). "Synchrotron spectromicroscopy for the life sciences: General considerations and special procedures." International Journal of Imaging Systems and Technology 8(2): 188-203. Neuhausler, U., C. Jacobsen, et al. (2000). "A specimen chamber for soft X-ray spectromicroscopy on aqueous and liquid samples." Journal of Synchrotron Radiation 7: 110-112. Osanna, A. and C. Jacobsen (1999). Principal component analysis for soft x-ray spectromicroscopy. X-ray Microscopy: Proceedings of the VI International Conference, Berkeley, CA, USA, American Institute of Physics. Peak, D., J. T. Sims, et al. (2002). "Solid-state speciation of natural and alum-amended poultry litter using XANES spectroscopy." Environmental Science & Technology 36(20): 4253-4261. Roberts, D. R., A. C. Scheinost, et al. (2002). "Zinc speciation in a smelter-contaminated soil profile using bulk and microspectroscopic techniques." Environmental Science & Technology 36(8): 1742-1750. Schulze, D. G. and P. M. Bertsch (1995). Synchrotron X-ray techniques in soil, plant, and environmental research. Advances in Agronomy, Vol 55. 55: 1-66. Sutton, S. R., P. M. Bertsch, et al. (2002). Microfluorescence and microtomography analyses of heterogeneous earth and environmental materials. Applications of Synchrotron Radiation in Low-Temperature Geochemistry and Environmental Sciences. 49: 429-483. Vairavamurthy, A. and S. Wang (2002). "Organic nitrogen in geomacromolecules: Insights on speciation and transformation with K-edge XANES spectroscopy." Environmental Science & Technology 36(14): 3050-3056. Vogt, S., J. Maser, et al. (2003). "Data analysis for X-ray fluorescence imaging." Journal de Physique IV 104: 617-622. Xia, K., F. Weesner, et al. (1998). "XANES studies of oxidation states of sulfur in aquatic and soil humic substances." Soil Science Society of America Journal 62(5): 1240-1246.