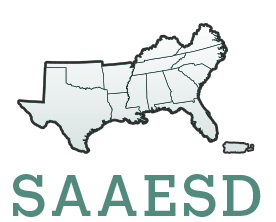
S1025: Systems for Controlling Air Pollutant Emissions and Indoor Environments of Poultry, Swine, and Dairy Facilities
(Multistate Research Project)
Status: Inactive/Terminating
S1025: Systems for Controlling Air Pollutant Emissions and Indoor Environments of Poultry, Swine, and Dairy Facilities
Duration: 02/01/2006 to 09/30/2012
Administrative Advisor(s):
NIFA Reps:
Non-Technical Summary
Statement of Issues and Justification
Advances in environmental control technologies have assisted in the intensive production of livestock and poultry resulting in improved productivity and profitability. However, the intensity of animal production and competitive economic factors have sometimes resulted in poor indoor air quality and emission of air pollutants such as odorous and hazardous gases, dusts, global atmospheric constituents (e.g., greenhouse gases like methane, carbon dioxide, nitrous oxide), and microbiological pollutants such as bacteria, fungi and endotoxin into the outdoor environment. In general, air quality and emission control technologies used for industrial sources appear to be uneconomical, and often impractical, for agricultural animal production. Further, the air emissions from animal buildings have very different characteristics from other types of facilities such as its particle size distribution and composition. However, numerous approaches to control indoor air quality and reduce air pollutant emissions have been developed which can be incorporated into existing production schemes without prohibitive costs or resource requirements. Indoor air cleaning, manure pit ventilation, manure/litter treatments, feed ration manipulation and improved indoor airflow control can benefit indoor air quality. Biological and mechanical exhaust air cleaners, dispersion devices such as stacks and windbreaks, and indoor air cleaning methods can directly reduce air pollutant emissions. In order to address the widespread and growing concerns regarding animal facility air quality and emissions, a multi-disciplinary approach involving collaborative efforts of scientists and engineers is needed. Quantification of the beneficial effects of all air emission control technologies can best be accomplished by professionals familiar with these systems and will facilitate the development of cost-effective solutions. JUSTIFICATION: Poultry and livestock production are major industries in the U.S. with 7.6 billion broilers, 301 million turkeys, and 102 million hogs marketed in the U.S. in 2003. Also animal production has been consolidating rapidly over the past 10 to 20 years. In the 15 years from 1982 to 1997, the number of US animal production operations decreased by 50% while animal production increased by 10% (Gollehon, et al., 2001). The fewer, larger and more productive facilities has magnified the influence of the indoor environments on the productivity and well-being of the animals, comfort and health of workers, air pollution emissions and effects on neighboring communities, and energy and resource use on the farm. Despite developments of some promising technologies for improving indoor environments and controlling emissions, continued cost concern by producers and the lack of targeted air pollution regulations at the animal production industry have prevented widespread adoption of these technologies. However, the explosive growth of animal production in some areas and increased migration of urban residents to rural communities have brought increased conflicts between animal producers and concerned citizens resulting in numerous lawsuits, moratoria on expansion of intensive swine farms, odor control regulations, and intensive public debates. Although much current dissatisfaction is driven by concerns about odors, air quality concerns have also focused attention on nitrogen deposition and ammonia emissions, for which animal buildings are a major source. In addition, scientists are continuing to gather and publish evidence that indoor environments are adversely affecting the health of workers and neighbors of poultry and livestock farms. Concerning emissions, the National Air Emission Monitoring Study (NAEMS) that is part of the EPA Air Quality Compliance Agreement and Final Order for Animal Feeding Operations, proposes later this year (2006) to start a comprehensive monitoring of gas and PM emissions from selected animal production sites. The National Research Council (NRC, 2003) report recommended the development of scientifically credible methodologies for estimating and mitigating air emissions from animal operation. Thus, many of the impediments to adoption of technologies for improving indoor air quality and reducing air pollution emissions are likely to change substantially in the next few years. As pressures to reduce air pollution increase while consumer demand for safe food at minimal cost continues, there will be incentives to develop cost-effective control technologies. Climatic constraints on food animal production in the U.S., especially in areas with warm, humid conditions that require substantial ventilation and removal of heat and moisture produced by the animals, will continue. Although air pollution and worker health concerns are expected to be major forces affecting animal systems in the near future, animal welfare concerns have also affected public perception of animal producers. Failure to consider animal welfare during the development and refinement of production systems could further erode public confidence and eventually result in additional constrains on production methods. A cooperative project involving engineers and scientists from a wide variety of disciplines in the intensive animal production regions will facilitate timely development and adoption of technologies and systems to meet the increased need for reducing air pollution emissions and adverse impacts of indoor environments on worker health, while sustaining animal productivity and well-being. Researchers cooperating in this project will focus on poultry, swine, and dairy due to their regional economic importance.
Related, Current and Previous Work
A search of the NIMSS database shows primarily two terminated multi-state projects, S-291 (2005) and NCR-189 (2004) and one current project S-1000 that addresses topics, related to work planned in this proposal. The new project (S-1025) will serve as a replacement for S-291. S-291 involved scientists from 17 states and several USDA-ARS laboratories. NCR-189 Air Quality Associated with Animal Facilities was terminated in 2004. This project included scientists from 15 states and focused on livestock and poultry indoor air quality concerns. The new project hopes to include scientists (university and USDA) from both the old S-291 and NCR-189 projects The S-1000 is an animal waste committee but has an air quality objective, which primarily deals with air emissions and ambient air quality downwind from animal operations. A CRIS search using animal air quality revealed 45 current and completed projects, several of which indicated an association with regional projects. A CRIS search using airborne emissions revealed 22 projects, including several from the previous search for dust-related projects. Most of the states represented in the two terminated and one existing projects are participating in this proposal. Emissions from Animal Facilities Livestock buildings and animal manures generate pollutants that include ammonia, hydrogen sulfide, methane, nitrous oxide, dust, and microorganisms. Gases are emitted from fresh and stored excreta, from the animal feed, and from the animals themselves. These aerial pollutants cause concern because of their implications in animal and human health, global warming, and forest dieback. Aerial transport of pathogens, dust and gases has implications both within the workplace and downwind of the livestock facility. Researchers are investigating methods of reducing emission of aerial pollutants through nutritional and management strategies while also providing for animal and human health and welfare and controlling environmental impacts. Methane Methane is implicated as a contributor to global warming and potential greenhouse effects. While estimates of the relative contribution from varying sectors vary, it is clear that production agriculture is an important source of methane. Johnson et al. (1992) estimates that 16.4 % of annual methane emissions, which translates to approximately 2.9% of the estimated contribution of all greenhouse gases to global warming, arises from animals, primarily ruminants, and animal manures. Jarvis et al. (1995) determined that <0.2% of total methane production from a dairy farm resulted from excreta during grazing periods, suggesting that manure storage and eructation (belching) are the primary sources in addition to feed fermentation. Dietary manipulation of livestock feeding is one method study to mitigate methane emissions. Agricultural Research Service researchers in Georgia and Texas have studied forage quality effects on methane production as well as the effects of protein and mineral supplementation on methane emission (CRIS Proj. No. 6612-13610-001-01S). Preliminary data suggest that a combination of dietary protein optimization with ionophore feeding did demonstrate emission benefits in grazing cattle. Researchers at the University of Georgia have also identified control of methane emissions from grazing cattle via forage selection as one of their research priorities (CRIS Proj. No. GEO00796). Quantification of methane emissions from manure storage facilities by using flux gradients and infrared analysis is currently a research project in Georgia (CRIS Proj. NO. 6612-13610-001-02R). Emission concentrations will be quantified as a function of season, climatic conditions, and storage conditions. Dust Organic dusts are comprised of grain and plant-derived particles, animal hair, urine, feces, microorganism and other particles (Takai et al., 1998). Dust particles may carry pollutants such as odorants, bacteria, endotoxin, and viruses, allowing for transport of potentially harmful materials within and between animal housing environments (Martin et al, 1996; Zhang, 1999). Organic dust has been suspected as a cause of work related respiratory symptoms such as asthma, organic dust toxic syndrome, and chronic bronchitis in farm workers. Researchers in Iowa, Kansas and Illinois have characterized the composition and size distribution of dust particles in swine facilities (Donham et al., 1986; Herber et al., 1988, Chen and Zhang, 1996); much of the dust is in the respirable size range and is predominantly from feces and feed. Methods for measurement of dust and other contaminants have been developed and tested by researchers at Illinois (McClure et al., 2004 and Jerez et al., 2005). Researchers in Texas are developing climate-driven models to predict dust and odor events, including ammonia emissions, associated with confined livestock production (CRIS Proj. No. TES08545), and have characterized the distribution of particle sizes emitted from cattle feedlots (Sweeten et al., 1998). Housing type and animal species greatly influence dust emissions (Takai et al., 1998). Both respirable and inhalable dust concentrations were determined to be lowest in cattle buildings followed by swine and poultry operations, respectively, based on 329 building observations. Assessment of wet scrubbers and biofilters, as part of ventilation systems, serve as outdoor odor and dust control methodologies under evaluation at the University of Illinois. Indoor air quality control measures include centrifugal dust removal systems, use of oil sprinkling (Zhang, 1997). Dust reductions attributable to indoor ozonation of a swine facility in North Carolina (Keener et al., 1999) have stimulated further evaluation of indoor ozonation systems. Fan configuration and operating conditions will be tested at Kansas State University to determine optimal conditions for reduction of respirable particle mass. Managing how air is exhausted (pit vs. wall) in a deep pit pig barn to reduce dust generation will be researched at the University of Minnesota. Previous work has demonstrated that vegetable oil or animal fat in ground feed does contribute to dust reduction (Mankell et al., 1995). Bioaerosols Air in animal production facilities often contains high concentrations of microorganisms and other biologically active substances, which are usually associated with dust particles. Such bioaerosols can cause or trigger respiratory diseases in humans and animals. Endotoxins are cell-wall components of dead Gram-negative bacteria; they are heat-stable lipopolysaccharide-protein complexes that are integral parts of the outer member and of gram-negative bacteria, of which animal waste is an important source (Windholz et al., 1976). Endotoxins are released into the environment after lysis of the bacteria cell or during active cell growth (Bradley, 1979). Endotoxins are commonly found in various agricultural setting where large amounts of organic dusts are generated (Olenchock, 1988, Martin et al., 1996). Endotoxins ranging from 4.5 to 48 mg/g of airborne dust have been detected in samples taken from swine and poultry confinement units (Thedell et al., 1908). Clark et al (1983) found average airborne endotoxin concentrations of 0.12 and 0.31 mg/m3 in Swedish swine and poultry facilities, respectively. Donham and Cumro (1999) noted that endotoxin levels typically correlate well with total dust and respirable dust levels. Symptoms of endotoxin exposure including chest tightness, cough, shortness of breath, fever, and wheezing have been found in workers in swine confinement and poultry confinement units (Donham et al., 1984; Thelin et al., 1987). Aerial pollutants have been measured downwind of swine farms. Homes et al. (1996) found that animals from a swine facility up to 200 m produced background concentrations of bacteria downwind, and viable bacteria in particles were sampled up to 300 m downwind. A European study (Seedorf et al., 1998) found that endotoxin concentrations are lowest in cattle houses and highest in poultry houses, with daytime concentrations exceeding nighttime concentrations. Research seeking to positively influence the transport characteristics of pathogens and endotoxins from poultry facilities has resulted in the prototype development of a filtration system that curtails airborne transport of Salmonella sp. by trapping the dust that serves as a carrier for Salmonella and other microorganisms (Martin, 1998). Researchers at the University of Minnesota and Purdue University are also considering animal practices and manure management influences on airborne transport of microflora (CRIS Proj. Nos. MIN-12-082 and IND051061A, respectively). Nitrogen Emissions Agricultural production results in large inputs of nitrogen (N) in the form of inorganic fertilizer and excreta to the land with subsequent loss of N to ground and surface waters (mainly as NO3-) and to the atmosphere (as N2, N2O, NO, and NH3). Nitrous oxide (N2O) emissions, largely the result of denitrification processes in soils, are of particular concern with respect to global warming and greenhouse potential. Some results that considered both seasonal effects on soil characteristic impacts on N2O emission suggest that nitrification processes also contribute to total N2O emissions (Allen et al., 1996). Watson et al. (1992) estimates that N2O accounts for 2-4% of total Greenhouse Warming Potential (GWP). Upon photolytic oxidation nitric oxide (NO) is formed which contributes to depletion of stratospheric ozone. Ammonia emissions contribute substantially to acidification and are therefore a concern with respect to acid rain formation. Estimates suggest that 45% of total acid deposition in The Netherlands that occurred during 1989 was caused by ammonia (Groot Koerkamp et al. (1998). Efforts to control ammonia emissions have demonstrated that reducing dietary crude protein concentration does reduce room ammonia concentration (Hobbs et al., 1996; Obrock et al., 1997; Sutton et al., 1998a). Diet modification work has been conducted in both swine and cattle (Kellems et al., 1979) with additional research in poultry currently underway (CRIS Proj. No. MINV-66-002). Typically, in nutritional work with monogastrics, reduced crude protein diets are achieved by including crystalline amino acids, demonstrating the impact of precision feeding on reducing nutrient excretions and emissions. Use of commercial additives, either in manure storage structures or as dietary additives, have demonstrated reduction in ammonia emissions (Heber et al., 1998; Powers et al., 1999) however, a great variability among products exists. Studies by Moore et al. (1996) and Brewer (1998) have shown that applications of alum can reduce the release of ammonia from poultry litter. Brewer (1998) estimated that whole house emissions of ammonia per flock from a commercial broiler house (12 m x 121 m) were reduced from 296 kg NH3-N to 131 kg NH3-N using alum applied at a rate of 1.22 kg/m2 compared to the untreated control. Odor Emissions Odors affect people differently based on their relationship with the odor source and their personal olfactory sensitivity. Odorous emissions can adversely affect the moods of farm neighbors and cause health effects such as eye, nose, and throat irritation, headache, and drowsiness, and possibly aggravate allergies, asthma, and bronchitis (Schiffman et al., 1995 and Schiffman, 1998). Development of methods to quantify livestock odors includes human sensory methodology (Bundy et al., 1993) as well as objective means of measurement (Zahn et al., 1997; Powers et al., 1999). Elliott et al. (1978) reviewed odor measurement approaches and recommended analytical methods such as gas chromatography in addition to olfactory techniques. Researchers in Texas developed and evaluated dynamic olfactometers for ambient odor measurements, and were able to compare olfactometer and scentometer measurements (Sorel et al, 1983; Sweeten et al., 1983). More recently, olfactometry has been developed and applied to measurement of swine odors at Iowa State University (Bundy et al., 1993), the University of Minnesota (Nicolai et al., 1997) and Duke University (Schiffman and Williams, 1999). Researchers in Minnesota, Iowa, and other universiteis are cooperatively developing a standard method of odor quantification using olfactometry and chemical analysis (CRIS Proj. NO IOW03254). Another odor sampling and measurement procedure which has proven successful in some situations with uniform, continuous odorous airflow involves exposing fabric swatches to the airflow and then presenting the swatches to human odor panelists (Miner and Licht, 1980; Schiffman and Williams, 1999). As a result of strong correlations between odor and dust measured by researchers in Iowa (Hammond et al., 1981; Hoff et al., 1997), Germany (Hartung, 1986), and identified in other literature (Parbst, 1998), analysis and quantification of the relationships between dust and odor control will be conducted in this project. Several other projects are also focusing on odor emissions from animal operations (CRIS Proj. No. ILLU-10-0355, IOW03252, MICL01888, NC00261, KAN00353, TEX08548). Researchers in Minnesota have recently developed very low-cost biofilters for treating odorous air from swine building manure pit airflows that can reduce odor and hydrogen sulfide emissions by over 90% (Nicolai and Jamni, 1998). Although the biofilters size needed for cleaning all of the air from tunnel ventilated facilities may be problematic for many farms, biofiltration of low flow, highly concentrated airflows as in cold weather or manure pit ventilation is an attractive alternative. Strategies to control odorous emissions have also included efforts to influence odors from manure storage facilities (Heber et al., 1998) and pre-excretion strategies via dietary manipulation (Bunce et al., 1995, Powers and Faust, 1998; Sutton et al., 1998b). Additional research in these areas is planned by researchers in this project and elsewhere (CRIS Proj. Nos. ILLU100202, 5438-31000-036-00D, SC1802, NEC11111, TEX08545). Animal Response to the Environment The effect of thermal environment upon animals has been the subject of many studies. Beginning in 1948 (Brody, 1948), the University of Missouri Agricultural Experiment Station began a series of research bulletins on environmental physiology. More recently, researchers have concentrated upon poultry, swine and dairy animals. Bonnet et al. (1997) found that, when exposed to high ambient temperatures, apparent metabolizable energy of broilers was significantly affected by the diet formulation. Several current projects are examining animal response to the thermal environment (CRIS Proj. Nos. ALA-50-015, FLA-AGE-03508, IND051041, IOW03355, KY005001, MIS-3150, SC01568; ARS Proj. No. 5438-32630-002-00D). Charles (1986) demonstrated that high environmental temperatures impair the growth and feed/gain ration of broiler chickens. Recently, researchers have focused on the use of evaporative cooling and air velocity to alleviate the detrimental effects of high environmental temperatures. Lacy and Czarick (1992) reported improvements in broiler weight gain in tunnel ventilated houses when compared to cross-ventilated houses. Air velocity is greater in a tunnel ventilated house that in a cross-ventilated house, with similar volumetric airflow rates. Increasing the air velocity causes a broiler to lose more heat through sensible heat loss rather than latent heat loss, without reducing total heat loss (Timmons and Hillman, 1993; Simmons et al., 1997). Lott et al. (1998) reported that as air velocity increased, body weight gain increased and feed/gain improved in male broilers. Havenstein et al. (1994) reported improvements in nutrition and genetic potential have resulted in continuous improvements in growth and feed utilization by broiler chickens since 1957. Reece and Lott (1981, 1983) reported the effects of the thermal environment on broiler chickens. May et al. (1998) reported body weight gain of male broilers was decreased as environmental temperature increased and feed/gain ration increased. Animal response to air quality has also been the subject of considerable study. Wathes (1998) summarizes air quality problems relative to poultry, swine and human health. Hamilton et al. (1996) found that the severity of turbinate atrophy in swine induced by Pasteruelli multocida was a maximum at ammonia levels of 10 to 15 ppm, and that ammonia had a synergistic effect, which has been documented for human exposure to ammonia and dust in swine buildings as well (Donham and Cumro, 1999). Jones et al. (1996) found that pigs are averse to ammonia at concentrations greater than 10 ppm. Whyte (1993) reported that dust and ammonia are the two most significant respiratory hazards to health of poultry production workers. In a later report, Whyte et al. (1998) reported a prevalence of respiratory impairment of 8% among UK poultry workers due to chronic exposure to poultry dust. Li (1997) reviewed the effects of animal building pollutants upon human health and suggested the development of multi-purpose environmental control methods. Donham (1991) reported on an epidemiologic study of swine health in Sweden and found several air contaminants (dust, ammonia, carbon dioxide, and microbes) to be correlated with swine health problems. He recommended maximum levels for these components. Zhang et al. (1998) used human subjects to test responses to typical swine housing environments, with and without canola oil treatment for dust control. Measurements immediately after five hours exposure showed significant reduction in respiratory capacity for subjects exposed to the untreated environment. Exposure to the canola oil treated environment resulted in a much smaller reduction in respiratory capacity. Reynolds et al. (1997) measured dust, endotoxins and ammonia in the environment outside swine production facilities. At 60 m from the facilities, they found that dust and endotoxin levels were near the lower detection limits. Measurable ammonia levels were detected. They suggested that ammonia might serve as a surrogate measure of other gases emitted by the facilities. Their report summarizes health concerns documented in other studies referenced in the report. Current and proposed work in the air quality area, especially ammonia and dust, includes the following projects: ARK 01602, GEO00751, ILLU-10-340, ILLU-10-345, IND046066, KAN00355, KYOO5001, MIN-12-076, NC00261, PEN03386, SC01568, TN00095, TEX08545, VA-136141, WIS03987). Optimizing Energy and Resource Use Researchers have established that dirt, dust, and moisture accumulation can significantly degrade performance of agricultural fans (Person et al., 1979). Recently, Simmons and Lott (1993, 1997b) conducted several studies of fan performance and provided suggestions for improved ventilation and reduced energy use. Heber and Li also studied fan performance and provided recommendations for predicting performance of variable speed fans (Heber and Li, 1995; Li and Heber, 1995). Stark et al. (1994) examined energy reduction options for broiler houses. They found that compact fluorescent lights resulted in a payback of less than two years. Reflective roof coatings and energy efficient motor retrofits could not be justified on energy savings alone. Bucklin et al. (1993) reached a similar conclusion regarding reflective roof coatings. Xin et al. (1993) compared energy efficiency of commercial broiler houses to the efficiency of houses designed for improved efficiency. They also compared ventilation systems and various evaporative cooling systems. Flood et al. (1998) found that ceiling fans reduced total energy requirements in broiler houses. Aho and Timmons (1991) proposed a control model for layer house ventilation based upon weather and economic factors. Several investigators (Gates and Overhults, 1991; Timmons et al., 1995) have studied application of a time integrated variable (TIV) control systems for optimizing ventilation. Gates et al. (1996) used field measurements from operating broiler houses to develop a program to estimate minimum ventilation requirement. This and related work to improve environmental control systems continues (CRIS Proj. Nos. ALA00817, KY005001, IOW03355, NC00261, NYC-123431). Zhang and Barber (1995) evaluated heating/ventilation control strategies for livestock buildings in the Canadian Prairie. Shao et al. (1997) developed a neural network model based upon animal behavior input for controlling swine comfort. They suggest that behavior-based controls would improve animal well-being and production efficiency. Klooster (1996) also proposed an animal-based ventilation control model.
Objectives
-
Develop and improve sustainable technologies and systems to measure, model and control indoor air quality and reduce air pollution emissions from poultry and livestock buildings.
-
Quantify animal response to thermal environments, develop and improve methods for providing productive thermal environments without degrading air quality or sustainability.
-
Develop and improve methods of optimizing energy and resource utilization in poultry and livestock facilities to increase profitability without degrading air quality or animal well being.
Methods
Objective 1: Develop and improve sustainable technologies and systems to measure, model and control indoor air quality and reduce air pollution emissions from poultry and livestock buildings The purpose of Objective 1 is to identify cost-effective strategies to improve indoor and outdoor air quality through housing and manure or litter management. As part of this objective, standard methods to quantify odor, gas and dust emissions will be applied and new methods will be developed. . Manure Management The MS leader will coordinate evaluations of poultry litter modifications to reduce emissions, to be conducted by AL, AR, MS, PA and SC. AL, AR and MS will determine effects of sand, paper products, cottonseed lint, and other litter amendments and alternatives on ammonia levels and emissions. Evaluations will be based on measured gas flux, bird weight gains, feed conversion, and percent mortality. The AL, AR, and MS researchers will replicate measurements of ammonia using colorimetric indicator tubes for ammonia level and emission determinations. Energy use in the houses will also be evaluated to determine if changes in ammonia levels impact energy costs and profitability. MS will also evaluate effects of litter management factors, including frequency of replacement, on ammonia levels and emissions. PA will evaluate effects of reduced indoor temperature for heating fuel conservation on indoor humidity and ammonia levels and emissions. SC will finish evaluating effects of litter aeration on indoor ammonia levels and emissions. IL will continue to develop the Nutrient Management program as part of the Certified Livestock Managers Program. Ventilation and Air Cleaning Investigators in GA, NC, SC, IA, MN and IL will measure emissions of air pollutants from ventilated buildings and evaluate air cleaning approaches as described below. Emissions from forced-ventilation housing will be evaluated using measurements of air mass flow rates and concentrations of ammonia, methane, odor-causing compounds (odorants), and odor levels from olfactometry panels, endotoxin and dust. Naturally ventilated houses used in the studies will require tracer, plume, or integrated horizontal-flux atmospheric transport techniques to quantify airflow (and thus emission) rates. The OK leader will incorporate economic information from the indoor air and exhaust air cleaning system evaluations into a spreadsheet system to assist with decision-making regarding waste management and pollution control for swine producers. As noted previously, this spreadsheet will constitute a milestone for Objective 1 and should be completed within 4 years. IA researchers will also evaluate dust and odor dispersion using air deflectors added to exhaust fans. The IL leader will evaluate exhaust airflow wet scrubbing using misting, low-pressure cyclones for dust removal, and indoor oil spraying. IL will also continue its fan testing program for evaluation of exhaust fan performance. The MN leader will evaluate dust removal of biofilters as it relates to odor and gas control from animal building exhaust streams. The SC leader will measure dust, ammonia, and odor levels and emissions for a broiler facility with an aerated litter floor, over a range of floor ventilation rates. As noted above, the NC leader will coordinate ventilation and air cleaning tasks and summarize results in annual reports of this project. Dietary Manipulations Dietary manipulation to alter or minimize excreta concentrations of compounds that metabolize to gaseous emissions of interest will be investigated by researchers in IA, NC, KY, MD, and OK. IA, NC, and KY will conduct evaluations of dietary manipulation for swine while evaluations for poultry and dairy cattle will be conducted by MD and IA, respectively. The OK leader will incorporate economic information from the dietary studies, with assistance from the IA leader, into a spreadsheet system to support decision-making regarding swine facility waste management and pollution control. Completion of the spreadsheet after the economic and technical results from the dietary study summary are incorporated, by the end of this Project, will constitute another milestone for Objective 1 that should be completed within 4 years. Emission Quantification Investigators in GA, IA, IL MN, NC, SC, TN, TX and both USDA/ARS-GA locations (Watkinsville and Athens, GA) will cooperate in quantifying air pollutant emissions from poultry and swine buildings. The NC leader will coordinate emission quantification tasks and will summarize results for annual reports of this project. Dust: Researchers in IL, GA, SC, TN, IA, MN, and NC will evaluate dust sampling and control methods and quantify dust emissions, as noted under Ventilation and Air Cleaning. Total and respirable dust levels will be measured using air sampling pumps with glass fiber filters and microbalances, with filters desiccated before and after sampling. Dust size distributions in the respirable size ranges will be quantified using optical particle counters and multi-stage impactors (for gravimetric determination of dust mass in the size ranges). Investigators in IA, IL, NC, and GA will develop a method of filtering dust from air samples and desorbing dust-borne odorants into other air samples and water. The TN leader will measure dust, ammonia, and odor emissions from swine buildings. The NC leader will coordinate evaluations of dust-borne odor measurement methods and summarizing results in a report to be published as a collaborative manuscript; establishment of recommended procedures for quantifying dust-borne odors will serve as another milestone for achieving Objective 1 and should be completed within two years. Odor: Researchers in IA, IL, MN, NC, and SC will use olfactometry to quantify odorous emissions as affected by experimental treatments. Dynamic dilution, triangular forced-choice of olfactometry with trained human panelists will be employed at Iowa State University, the University of Minnesota, Purdue University, and Duke University. Researchers in IA, MN and NC will collect replicate air and dust samples from swine building exhaust fans and send to each of these four odor measurement laboratories for odor measurements. Results will be analyzed in conjunction with results of the MN-IA project (CRIS Proj. No. IOW03254) to standardize odor measurement methods. NC will also employ unaspirated and aspirated cotton swatches and the Scentometer to measure odors at the building exhaust fans for correlation with olfactometry results from the same sampling locations and periods. TX, IA, and NC will conduct optimization of electronic nose technology, as a means of characterizing human response to livestock odors. NC will employ dust filtering and methods of desorbing odorants from captured dust to separate vaporous and dust-borne odor streams to their electronic nose, and will compare vaporous vs. dust-borne odor levels obtained using dynamic oflactometry, filtered and unfiltered fabric swatches, and the electronic nose. NH3 and odor emission rate from livestock dust: The IL researcher will develop a flux chamber to determine ammonia and odor emission rate from animal building dusts. The flux chamber is designed to prevent adsorption of gases released from dust samples. Prior to measurement of the emission rate of ammonia and odor, gas recovery efficiency will be determined by using several standard gases. Dust sample is placed on the glass plate in chamber and zero air is flown into the chamber as a carrier gas. Zero air conveys gases emitted from dust samples to NH3 analyzer (Model 17C, Thermo Environmental Instruments, TEI) and Tedlar sampling bag for odor measurement. Sampled gases for odor measurement will be analyzed by odor-calibrated experts. Gaseous samples for NH3 and odor analysis are collected after chamber equilibrium. The optimal flow rate of carrier gas and sample collection time will be determined by preliminary lab tests. All experiments will be replicated for QA/QC. Research to characterize and improve control of odorous emissions will focus on improving measurement of emissions, methods of exhaust air filtering, and aerial (wind) dispersion. Correlations between ambient conditions, ventilation rates, system types and odor will be developed. Researchers in GA, IA, and NC will identify air plumes issuing from tunnel ventilated buildings using smoke candles, sample particulates, odorants, odors, and endotoxin at several locations in the plumes, and correlate pollutant levels with particulate levels to determine if dispersion modeling of dust will enable prediction of dispersion of the correlated pollutants. NC, GA, IA, and IL researchers will evaluate effects of exhaust air filters on odor and ammonia emission rates from swine and poultry facilities, as well as effects on ventilation airflow resistance and system power requirements. The dust/odor relationships developed by project researchers, noted under the Dust section, will be applied to all odor emission calculations to determine effects of accounting for dust-borne odorants on total emissions. Analysis of the exhaust data to determine technical performance of the filtering systems; maintenance requirements, including frequency and appropriate methods of cleaning and replacing or recharging the filter media, will also be conducted for each system tested. Bioaerosols: GA, IA, and IL researchers will measure endotoxin and bacteria levels in animal facility ventilation airflows associated with field studies. The NC leader will correlate endotoxin and dust levels, bacteria and dust levels, and fungal spore and dust levels, in plumes originating from swine building exhaust fans for distances up to 300 m downwind; plumes will be visualized before and after sampling using smoke candles. Bioaerosol measurements obtained in GA, IA, IL, and NC will be summarized in each Annual Report. Chemical analyses : Researchers in USDA/ARS-GA will use tunable diode laser spectrometry or gas washing techniques to measure ammonia and methane concentrations emitted from housing systems. Gaseous emissions of chemical analytes, as affected by diet will be measured using a number of techniques. Researchers in IA, IL, NC, and GA will employ gas chromatography (GC) with differing detection systems (mass spectrometry (MS), flame ionization detection, pulsed helium ionization detection (PID), to quantify total VOCs including odorous compounds in air and dust samples. NC personnel will also use FTIR technology to identify and quantify compounds emitted during dietary modification experiments. For the total VOC analysis, preliminary tests on the most applicable detection method will be conducted since concentrations of VOCs in the air are in ppb levels requiring highly delicate sampling and analysis technique for reliable measurement. GC coupled with MS technique allows identification of most VOCs up to the ppb levels without enrichment of the samples. For the measurement of halogen and aromatic hydrocarbon compounds, however, GC coupled with either ECD or PID is more suitable. Animal and Worker Health Investigators in GA, KY, NC, SC, TN, and USDA/ARS-GA, Athens will cooperate in evaluating effects of poultry and livestock house environments on animal and human health. The USDA/ARS-GA, Athens leader will coordinate animal and worker health evaluation tasks and summarize results for the final report of the project. Transmission of disease by air will be the research focus of participants from USDA/ARS-GA, Athens station. Developments of techniques to identify the role of aerial transport in transmission of select poultry diseases and development of methods to reduce transmission of these diseases are the emphasis of the research effort. Birds will be exposed to different aerosol concentrations and their lung tissue will be examined and tested for damage, including lesions and other abnormalities, following slaughter. Researchers will incorporate evidence of respiratory damage in the evaluation of dust control approaches. The GA and NC leaders will cooperate in evaluating exposure of poultry and swine facility workers to airborne contaminants. GA will measure exposures of poultry workers, and NC will measure exposure of swine workers, to dust, ammonia and microbial contaminants. These workers will be monitored during the normal course of their work and not subjected to other environments. Monitoring of human exposures will adhere to the pertinent protocols at each station. In addition to summarizing results to quantify worker exposures and dust meter reliability, GA will analyze these data in conjunction with measured air quality levels inside buildings to determine if standard procedures for measuring indoor air quality are useful in predicting worker exposures. Objective 2: Quantify animal responses to thermal environments, develop and improve methods for providing productive thermal environments without degrading air quality or sustainability. The purpose of Objective 2 is to ensure that methods of environmental control provide adequate or improved thermal environments while addressing air quality and other concerns. Responses of the animals to thermal environments will be determined in order to develop improved thermal environment indices, improve prediction accuracy of simulation models, and better understand animal comfort ranges. Animal Response to Thermal Environments Thermal responses of poultry will be measured and analyzed to establish thermal environment indices (TEIs), which account for effects of air temperature, humidity, air speed, and thermal radiation on the animals. The project leaders for FL, GA, SC, and USDA/ARS-MS will develop TEIs for broilers. The FL leader will collaborate with Brazil in correlating dry bulb temperature, black globe temperature, and humidity with bird temperament and production parameters in warm weather, and will develop a temperature-humidity index. The USDA/ARS-MS and SC leaders will summarize results of experiments with their apparatus to control the black globe temperature, humidity, and air velocity and incorporate these three parameters into a comprehensive TEI. USDA/ARS-MS will complete wind tunnel evaluations of effects of wind speed on bird activity. The USDA/ARS-MS, SC, and FL leaders will then compare the resulting broiler TEIs with published TEIs for poultry and swine, and publish appropriate TEI models for a range of ambient conditions in a collaborative journal article. Development of these TEI models will serve as a milestone for achieving Objective 2 and should be completed within four years. IA researchers will employ computer-imaging analysis of swine behavior to determine swine responses to thermal environments and will summarize the results and indicate feasibility and effectiveness of the method. The FL leader will coordinate tasks in determining animal responses to thermal environment. Ventilation Systems and Environmental Control Improved methods of cooling stressed animals and controlling the ventilation and environmental control systems will be developed. AL and AR researchers will determine broiler performance in terms of feed efficiency, weight gain, and mortality for evaporatively cooled; tunnel ventilated housing and will summarize results to give design values of expected bird performance for such systems in a collaborative journal article. Development of design values for bird performance in evaporatively cooled, tunnel ventilated housing will serve as a milestone for achieving Objective 2 which should be completed within three years. AL researchers will also measure the performance of alternative evaporative cooling pad wetting methods in terms of pad cooling efficiency and temperature reductions, to determine design efficiencies for fogging pad systems with different nozzle arrangements. The project leaders for KY, NC, and NY will complete evaluation of time-integrating (TIV) control of ventilation and cooling. KY and NC will summarize results of TIV trials in tunnel ventilated and naturally ventilated broiler housing and NY will evaluate TIV control of tunnel ventilated dairy buildings. Evaluations will be based on effectiveness of the controller in adjusting environmental control to improve cooling when time-averaged temperatures exceed the TIV setpoint, as well as energy use and animal performance indicators. The KY leader will coordinate development of a collaborative publication summarizing results of TIV control methods, which will serve as a milestone for achieving Objective 2. Researchers in IA, IL, KY, and USDA/ARS-Athens, GA will develop electronic control systems for poultry and swine facilities based on systems control theory techniques, results of the TIV control evaluations, and incorporation or qualitative measures such as bird behavior and genetic information into fuzzy logic control. These researchers will then develop a single electronic control system incorporating these features suitable for both poultry and swine environment control, which will serve as another milestone for achieving Objective 2 and should be accomplished within the term of this project (five years). The KY leader will coordinate these tasks in developing improved ventilation and environmental control. Objective 3: Develop and improve methods of optimizing energy and resource utilization in poultry and livestock facilities to increase profitability without degrading air quality or animal well-being. The purpose of Objective 3 is to ensure that energy and resource utilization in poultry and livestock facilities remain efficient and not unduly increased by attempts to control air quality or emissions. Ventilation Efficiency Researchers in IL, IA, MN, and USDA/ARS-MS will evaluate ventilation system component performance using different methods and collaboratively summarize results. The IL researchers will develop a methodology for field measurement and troubleshooting of ventilation effectiveness for livestock buildings. The spreadsheet will enable users to be guided in making management decisions impacting energy use for animal confinement facilities. IL researchers will use their Bioenvironmental and Structural Systems (BESS) Laboratory to evaluate performance of ventilation fans, air inlets, ridges, vents, light traps, and other components used in animal confinement facilities, using the ANCI/ASHRAE standard method. The BESS Lab will also expand its testing capacity to evaluate circulation/paddling fans. USDA/ARS-MS, IA and IL researchers will then compare the spreadsheet and laboratory results to determine discrepancies and degree of concordance of fan performance determinations. The USDA/ARS-MS leader will coordinate ventilation efficiency tasks and development of a collaborative publication, with IA and IL, which compares field evaluations using anemometers with laboratory evaluations using standard methods. The comparison of field and laboratory methods and results will serve as a milestone for achieving Objective 3 and should be completed with three years. Alternative Fuels The AR leader will evaluate the efficacy of using various biomass fuels (e.g., wood pellets, sawdust, poultry litter) for poultry brooding based on costs, pollution effects, labor requirements, space requirements, and equipment requirements for the various fuels. The IL leader will employ thermochemical conversion (TCC) of animal manure in a heated enclosure to produce oil and gas. IL will then determine potential applicability of TCC fuels for poultry and livestock heating systems using the same criteria used by AR for biomass fuels. The IL leader will coordinate development of a collaborative publication with AR summarizing evaluations of biomass and TCC fuels, which will serve as a milestone for achieving Objective 3 and should be completed within four years. Energy and Management The PA leader will evaluate the potential impacts of the deregulation of electricity generation and natural gas on established management practices for animal production systems. PA will also evaluate the potential benefits of various energy strategies on poultry and livestock facilities including load management, energy management systems, energy procurement, and new technologies for on-site generation. The NC leader will provide estimates of electricity costs and requirements for odor control systems being evaluated, including windbreak walls, wet scrubbers, and indoor ozonation, as examples of alternative management systems. The PA leader will coordinate development of a publication summarizing results of the comparison of these energy management strategies, which will serve as a milestone for achieving Objective 3. SEE ATTACHED TABLEMeasurement of Progress and Results
Outputs
- This project is expected to yield designs and methods to facilitate reduction of poultry and livestock building emissions. Work to achieve Objective 1 will produce new system designs such as windbreak wall systems, dedusters, and exhaust air cleaners. Improvements in measuring techniques such as methods of quantifying both dust-borne and gaseous odorants will assist producers and regulators in accurately measuring relevant pollutant levels. New measurement technologies and protocols will be developed aimed to fill the existing EPA gaps that are primarily for atmospheric studies. The information summaries to be developed by sub-objective coordinators will assist in transferring new technology to producers and citizens; web-based and paper publications, spreadsheets, workshops, and field days resulting from research in the project will facilitate technology transfer. Information on indoor exposure to air pollutants will hopefully stimulate development of respiratory protection programs to benefit workers. Measurement of low air pollution levels in cage broiler facilities may assist in overcoming resistance to such facilities due to animal welfare or economic considerations, and stimulate caged broiler production in the U.S.
- For Objective 2, development and validation of the Thermal Environment Indexes (TEIs) will benefit researchers and equipment manufacturers by identifying optimal ranges of target indoor conditions (temperature, humidity, air velocity, and thermal radiation). Development of design values for performance of poultry in tunnel ventilated, evaporatively cooled housing will facilitate objective decision-making by producers planning to upgrade or build new facilities. The time-averaging (TIV) and other electronic control methods are expected to prove useful to producers in automating the adjustment of equipment in ways that better account for stressful conditions.
- For Objective 3, development and publication of fan test data summaries and the fan testing spreadsheet will assist fan manufacturers and poultry and livestock producers in optimizing fan specifications. Improved fan performance resulting from selection of more efficient fans would reduce energy consumption and better ensure adequate indoor thermal conditions (and animal productivity). Quality of agricultural ventilation equipment (fans, shutters, inlets, controllers, evaporative cooling pads etc) varies widely. A standard for agricultural ventilation equipment need to be developed. Alternative fuels from biomass and thermochemical conversion of animal manure to oil and gas could enhance the economic viability of livestock operation. Finally, recommendations regarding management practices to optimize the effects of electricity deregulation could benefit producers, e.g. by stimulating integrated poultry or swine production companies to incorporate electricity costs into their arrangements with contract growers.
Outcomes or Projected Impacts
- This project is expected to yield designs and methods to facilitate reduction of poultry and livestock building emissions. Work to achieve Objective 1 will produce new biological (biofilters) and physical (dedusters) catch and treat technologies. Improvements in measuring techniques such as methods of quantifying both dust-borne and gaseous odorants will assist producers and regulators in accurately measuring relevant pollutant levels. New measurement technologies and protocols will be developed aimed to fill the existing EPA gaps that are primarily for atmospheric studies including process-based models as recommended in the NRC report. The information summaries to be developed by sub-objective coordinators will assist in transferring new technology to producers and citizens; web-based and paper publications, spreadsheets, workshops, and field days resulting from research in the project will facilitate technology transfer. Information on indoor exposure to air pollutants will hopefully stimulate development of respiratory protection programs to benefit workers. Measurement of low air pollution levels in caged layer facilities may assist in overcoming resistance to such facilities due to animal welfare or economic considerations, and keep caged layer production in the U.S.
Milestones
(0):lude summaries of dietary manipulation and litter management methods and effects on air quality and emissions, development of a spreadsheet to support decision-making regarding air pollution control, development of protocols for quantifying emissions from dispersion devices and dust-borne odors, and development of a robust electronic nose which accounts for dust-borne odors For Objective 2, development and validation of the Thermal Environment Indexes (TEIs) will benefit researchers and equipment manufacturers by identifying optimal ranges of target indoor conditions (temperature, humidity, air velocity, and thermal radiation). Development of design values for performance of poultry in tunnel ventilated, evaporatively cooled housing will facilitate objective decision-making by producers planning to upgrade or build new facilities. The time-averaging (TIV) and other electronic control methods are expected to prove useful to producers in automating the adjustment of equipment in ways that better account for stressful conditions.(0):estones: Includes development of thermal environment index models for poultry and swine, design values for poultry performance in tunnel ventilated, evaporative cooled housing, summarizing methods and evaluations of time-integrated variable (TIV) electronic control for animal housing, and development of a sophisticated electronic control system for poultry and swine environments incorporating systems control techniques. For Objective 3, development and publication of fan test data summaries and the fan testing spreadsheet will assist fan manufacturers and poultry and livestock producers in optimizing fan specifications. Improved fan performance resulting from selection of more efficient fans would reduce energy consumption and better ensure adequate indoor thermal conditions (and animal productivity). Quality of agricultural ventilation equipment (fans, shutters, inlets, controllers, evaporative cooling pads etc) varies widely. A standard for agricultural ventilation equipment need to be developed. Alternative fuels from biomass and thermochemical conversion of animal manure to oil and gas could enhance the economic viability of livestock operation. Finally, recommendations regarding management practices to optimize the effects of electricity deregulation could benefit producers, e.g. by stimulating integrated poultry or swine production companies to incorporate electricity costs into their arrangements with contract growers.
(0):ncludes comparison of field and laboratory evaluations of ventilation system component performance, a summary of evaluations of alternative heating fuels from biomass and thermochemical conversion of manure, and a summary of effectiveness of alternative poultry, swine, and dairy management systems in taking advantage of the benefits of deregulation of electricity and gas.
Projected Participation
View Appendix E: ParticipationOutreach Plan
An annual project meeting will be held to summarize the progress and findings of each participating state and highlight the most significant results. The summaries will be entered into the NIMSS systems of USDA and available via each experiment station. The committee leadership will work with existing professional organizations such as ASABE and others to include results from this project in specialty conferences and meeting on the topic of environmental control for animal facilities its effect on animal performance and pollutant emission and dispersion. In addition to scientific publications, the results from this study will be summarized in fact-sheets and other extension publications that will be available on various university extension websites and distributed via the Federal Extension Services and some extension arms such as Midwest Plan Services (MWPS) (NCCC-9 committee),.
Organization/Governance
A Regional Technical Committee shall be organized in accordance with the Manual for Cooperating Regional Research. The voting membership of the Regional Technical Committee shall include one representative from each cooperating agricultural experiment station as appointed by the respective Director and a technical representative of each cooperating USDA/ARS location. Nonvoting members shall consist of other representatives of participating organizations, the Administrative Advisor, and the USDA/CSRS Representative. All voting members of the Technical Committee are eligible for office. A committee consisting of the Administrative Advisor, outgoing chair, chair, secretary, and one additional member elected from the Technical Committee shall constitute the Executive Committee and shall have the authority to act in its behalf. A member shall be elected to the Executive Committee each year and shall serve for four years. This member will become secretary the second year and the chair the third year of his or her term. No elected member of the Executive Committee may be re-elected to this committee until one year after the expiration of his or her term. The duties of the Technical Committee will be to coordinate the planning and work of the project and make such recommendations as are necessary through the Administrative Advisor to the Agricultural Experiment Station Directors. The functions of the chair will be to preside over meetings and edit the annual report. The recording secretary shall take minutes at the annual meeting and distribute these to members of the committee within a month of the meeting. Annual meetings of this committee will be held for the purpose of conducting the business connected with the project.
Literature Cited
Aarnink, A.J.A. and M.J.M. Wagemans. 1997. Ammonia volatilization and dust concentration as affected by ventilation systems in houses for fattening pigs. Transactions of the ASAE 40(4): 1161-1170. Aho, P.W. and M.B. Timmons. 1991. Minimum ventilation capacity for layer houses. Poultry Science 70(11): 2237-2245. Allen, A.G., S.C. Jarvis, and D.M. Headon. 1996. Nitrous oxide emissions from soils due to inputs of nitrogen from excreta return by livestock on grazed grassland in the U.K. Soil Biol. Biochem. 28(4/5): 597-607. Armstrong, D.V., W.T. Welchert, and F. Wiersma. 1993. Environmental modification for dairy cattle housing in arid climates. 4th International Livestock Environmental Symposium, London England, July 1993. ASAE Special Publication, St. Joseph, MI, pp. 1223-1231. Bonnet, S., P.A. Geraert, M. Lessire, B. Carre, and S. Guillaumin. 1997. Effect of high ambient temperature on feed digestibility in broilers. Poultry Science 76(6): 857-863. Bottcher, R.W., M. Czarick, III, M. P. Lacy, and G.R. Baughman. 1992. Evaporative efficiency of a fogging fan for poultry. Applied Engineering in Agriculture. 8(6): 855-860. Bottcher, R.W., J. Brake, G.R. Baughman, and J.R. Magura. 1995a. Vertically directed mixing fans for cooling floor-raised poultry. Applied Engineering in Agriculture 11(4): 591-599. Bottcher, R.W., J.R. Magura, J.S. Young, and G.R. Baughman. 1995b. Effects of tilt angles on airflow for poultry house mixing fans. Applied Engineering in Agriculture 11(5): 721-730. Bottcher, R.W. and S.J. Hoff (ed.) 1997. Livestock Environment 5: Proceedings of the fifth international symposium, Volumes 1 and 2. ASAE, St. Joseph, MI. Bottcher, R.W., G.R. Baughman, R.D. Minilla, J.L. Grimes, and E. Gonder. 1998. Development of a large paddle fan for cooling poultry. Applied Engineering in Agriculture 14(1): 87-96. Bradley, S.G. 1979. Cellular and molecular mechanisms of action of bacterial endotoxins. Ann. Rev. Microbiol. 33: 67-94. Brewer, S.K. 1998. Ammonia flux from fresh, re-used, and alum-treated broiler litter. Unpublished M.S. thesis. University of Arkansas. Brewer, S.K. and T.A. Costello. 1999. In-situ measurement of ammonia volatilization from broiler litter using an enclosed air chamber. Transactions of the ASAE (in-press). Bridges, T.C., R.S. Gates, and L.W. Turner. 1992. Stochastic assessment of evaporative misting for growing-finishing swine in Kentucky. Applied Engineering in Agriculture 8(5): 685-693. Brody, S. 1948. Physiological backgrounds. University of Missouri Agricultural Experiment Station Research Bulletin 432. Series Number I of Environmental Physiology Series. Bucklin, R.A., R.W. Bottcher, G.L. van Wicklen, and M. Czarick. 1993. Reflective roof coatings for heat stress relief in livestock and poultry housing. Applied Engineering in Agriculture 9(1): 123-129. Bunce, T.J., M.S. Kerley, and G.L. Allee. 1995. Control of swine odor through feeding fructooligosaccharides, National Pork Producers 1995 Research Report, Des Moines, IA. Bundy, D.S. and S.J. Hoff. 1998. The testing procedures and results of pit additives tested at Iowa State University. In: Animal Production Systems and the Environment, Proceedings Volume I. July 19-22, 1998, Des Moines, IA. Iowa State University, Ames, IA, pp. 279-285. Bundy, D.S., Q. Liu, and S.J. Hoff. 1993. An olfactometer for livestock odor measurement. Pap-Am-Soc-Agric-Engr. St. Joseph, MI. ASAE Paper No. 93-4522. Chen, K.H., J.T. Huber, C.B. Theurer, D.V. Armstrong, R.C. Wanderley, J.M. Simas, S.C. Chan, and J.L. Sullivan. 1993. Effect of protein quality and evaporative cooling on lactational performance of Holstein cows in hot weather. Journal of Dairy Science 76(3): 819-825. Chen, S.C., J.T. Huber, K.H. Chen, J.M. Simas, and Z. Wu. 1997. Effects of ruminally inert fat and evaporative cooling on dairy cows in hot environmental temperatures. Journal of Dairy Science 80(6): 1172-1178. Charles, D.R. 1986. Temperature for broilers. Worlds Poultry Science Journal 42: 249-258. Clark, S., R. Rylander, and L. Larsson. 1983. Airborne bacteria, endotoxin and fungi in dust in poultry and swine confinement buildings. Am. Ind. Hyg. Assoc. J. 44(7): 537-541. DeBey, M.C., D.W. Trampel, J.L. Richard, D.S. Bundy, L.J. Hoffman, V.M. Meyer, and D.F. Cox. 1994. Effect of building ventilation design on environment and performance of turkeys. American Journal of Veterinary Research 55(2): 216-220. Donham, K.J. 1990. Health effects from work in swine confinement buildings. American Journal of Industrial Medicine 17: 17-25. Donham, K.J. 1991. Association of environmental air contaminants with disease and productivity in swine. American Journal of Veterinary Research 52(10): 1723-1730. Donham, K. and D. Comro. 1999. Setting maximum dust exposure levels for people and animals in livestock facilities. Proceedings of the International Symposium on Dust Control in Animal Production Facilities, 30 May 2 June 1999, Aarhus, Denmark. Danish Institute of Agricultural Sciences, Horsens, Denmark, pp. 93-110. Donham, K.J., J.A. Merchant, D. Lassise, W.J. Popendorf, and L.F. Burmeister. 1990. Preventing respiratory disease in swine confinement workers; intervention through applied epidemiology, education, and consultation. American Journal of Industrial Medicine 18: 241-261. Donham, K.J., L.J. Scallon, W. Popendorf, M.W. Treuhaft, and R.C. Roberts. 1986. Characterization of dusts collected from swine confinement buildings. Am. Ind. Hyg. Assoc. J. 47(7): 404-410. Donham, K.J., D.C. Zavala, and J.A. Merchant. 1984. Respiratory symptoms and lung function among workers in swine confinement buildings: A cross-sectional epidemiological study. Arch. Environ. Heath 39: 96-101. Elliott, L.F., J.W. Doran, and T.A. Travis. 1978. A review of analytical methods for detecting and measuring malodors from animal waste. Trans. of the ASAE 21(1): 130-135. Flood, C.A., Jr., J.L. Koon, R.D. Trumbull, and R.N. Brewer. 1998. Energy savings with ceiling fans in broiler houses. Applied Engineering in Agriculture 14(3): 305-309. Gates, R.S. and D.G. Overhults. 1991. Field evaluation of integrated environmental controllers. ASAE Paper No. 91-4037, ASAE, St. Joseph, MI. Gates, R.S., J.L. Usry, J.A. Nienaber, L.W. Turner, and T.C. Bridges. 1991. An optimal misting method for cooling livestock housing. Transactions of the ASAE 34(5): 2199-2206. Gates, R.S., D.G. Overhultz, and S.H. Zhang. 1996. Minimum ventilation for modern broiler facilities. Transactions of the ASAE 39(3): 1135-1144. Gerrish, J.B., S.J. Mastern, H.L. Person, T. Vukinia, J.J. Wu, and M.T. Yokoyama. 1999. Odor control of urine-rich swine slurry by ozonation. In Proceedings 1999 Animal Waste Management Symposium, p. 272, G.B. Havenstein (ed.) North Carolina State University, Raleigh, NC. Groot Koerkamp, P.W.G., J.H.M. Metz, G.H. Uenk, V.R. Phillips, M.R. Holden, R.W. Sneath, J.L. Short, R.P. White, J. Hartung, J. Seedorf, M. Schroder, K.H. Linkert, S. Pederson, H. Takai, J.O. Johnsen, and C.M. Wathes. 1998. Concentrations and emissions of ammonia in livestock buildings in Northern Europe. Journal of Agricultural Engineering Research 70: 79-95. Hamilton, T.D.C., J.M. Roe, and A.J.F. Webster. 1996. The synergistic role of gaseous ammonia in the aetiology of P. multocida-induced atrophic rhinitis in swine. Journal of Clinical Microbiology 34: 2185-2190. Hammon, E.G., C. Fedler, and R.J. Smith. 1981. Analysis of particle-borne swine house odors. Agriculture and Environment 6: 395-401. Hartung, J. 1986. Dust in livestock buildings as a carrier of odours. I: Odour Prevention and Control of Organic Sludge and Livestock Farming. Nielsen, V.C., J.H. Voorburg, and P.LHermite, eds. Elsevier Applied Science Publishers, New York, NY. Pp. 321-331. Havenstein, G.B., P.R. Ferket, S.E. Scheideler, and B.T. Larson. 1994. Growth, livability and feed conversion of 1957 vs. 1991 broilers when fed "typical" 1957 and 1991 broiler diets. Poultry Science 73: 1785-1794. Heber, A.J. and J. Li. 1995. Predicting variable-speed fan performance from motor and impeller characteristics, I. Using complete motor data. Transactions of the ASAE 38(4): 1247-1252. Heber, A.J., M. Stroik, J.M. Faubion, and L.H. Willard. 1988. Size distribution and identification of aerial dust particles in swine finishing buildings. Transactions of the ASAE 31(3): 882-887. Heber, A.J., B.L. Haymore, J. Ni, and R. Duggirala. 1998. Measurements of gas emissions from commercial swine buildings. ASAE Paper No. 98-4058, ASAE, St. Joseph, MI. Hobbs, P.J., B.F. Pain, R.M. Kay, and P.A. Lee. 1996. Reduction of odorous compounds in fresh pig slurry by dietary control of crude protein. J. Sci. Food Agric. 71: 508-514. Hoff, S.J., D.S. Bundy, and X.W. Li. 1997. Dust effects an odor and odor compounds. Pp. 101-110 in: Ammonia and Odour Control from Animal Production Facilities, Proceedings of the International Symposium, Volume 1, J.A.M. Voermans and G. Monteny, eds. NVTL, Rosmalen, The Netherlands. Hoff, S.J., D.S. Bundy, and R.W. Bottcher. 1998. Strategies for reducing exhaust-air odors. In Animal Production Systems and the Environment Proceedings, Volume 2: 655-660. Iowa State University, Ames, IA. Homes, M.J., A.J. Heber, C.C. Wu, L.K. Clark, R.H. Grant, N.J. Zimmerman, M.A. Hill, B.R. Strobel, M.W. Peugh, and D.D. Jones. 1996. Viability of bioaerosols produced from a swine facility. Proceedings of the International Conference on Air Pollution from Agricultural Operations, Midwest Plan Service, Ames, IA. Pp. 127-131. Jarvis, S.C., R.D. Lovell, and R. Panayides. 1995. Patterns of methane emission from excreta of grazing animals. Soil Biol. Biochem. 27(12): 1581-1588. Jerez, S.B., Y. Zhang, J. McClure, L. Jacobson, A. Heber, S. Hoff, J. Koziel, and D. Beasley. 2005. Comparison of measured total suspended particulate matter concentration using tapered element oscillating microbalance and a TSP sampler. Journal of the Air and Waste Management Association. Pending. Johnson, D.E., T.M. Hill, and G.M. Ward. 1992. Methane emissions from cattle; global warming and management issues. In: Proceedings Minnesota Nutritional Conference, Minnesota Extension Service, University of Minnesota, St. Paul, MN. Jones, J.B., L.R. Burgess, A.J.F. Webster, and C.M. Wathes. 1996. Behavioral responses of pigs to atmospheric ammonia in a chronic choice test. Animal Science 63: 437-445. Keener, K.M., R.W. Bottcher, R.D. Munilla, K.E. Parbste, and G.L. Van Wicklen. 1999. Field evaluation of an indoor ozonation system for odor control. In Proceedings 1999 Animal Waste Management Symposium, G.B. Havenstein (ed.) North Carolina State University, Raleigh, NC. Pp. 310-313. Kellems, R.O., J.R. Miner, and D.C. Church. 1979. Effect of ration, waste composition and length of storage on the volatilization of ammonia, hydrogen sulfide and odors from cattle waste. Journal of Animal Science 48(3): 436-445. Klooster, C.E. 1996. Animal-based control algorithm for natural ventilation in pig houses. Transactions of the ASAE 39(3): 1127-1133. Lacy, M.P. and M. Gzarick. 1992. Tunnel-ventilated broiler houses: broiler performance and operating cost. Journal of Applied Poultry Research 1: 104-109. Lenhart, S.W., P.D. Morris, R.E. Akin, S.A. Olenchock, W.S. Service, and W.P. Boone. 1990. Organic dust, endotoxin, and ammonia exposures in the North Carolina poultry processing industry. Appl. Occup. Environ. Hyg. 5(9): 611-618. Leonard, S. and K. Donham. 1990. Dust and gases. National Hog Farmer. Spring 1990: 16-21. Levy, D.A. 1982. Allergic response and agricultural activities. Ann. Am. Conf. Gov. Ind. Hyg. 1982. 2: 161-170. Li, X.W. 1997. Effects of dust and contaminants in animal buildings on human health and control strategies. Journal of Environmental Science and Health 32: 2449-2469. Li, J. and A.J. Heber. 1995. Predicting variable-speed fan performance from motor and impeller characteristics. I. Using limited motor data. Transactions of the ASAE 38(4): 1253-1259. Lin, J.C., B.R. Moss, J.L. Koon, C.A. Flood, Jr., S. Rowe, J.R. Martin, B. Brady, F. DeGraves, and R.C. Smith. 1997. Effect of sprinkling over the feed area and misting free stalls on milk production. Journal of Dairy Science 80(1): 274. Lin, J.C., B.R. Moss, J.L. Koon, C.A. Flood, R.C. Smith, III, K.A. Cummins, and D.A. Coleman. 1988. Comparison of various fan, sprinkler, and mister systems in reducing heat stress in dairy cows. Applied Engineering in Agriculture 14(2): 177-182. Lott, B.D., J.D. Simmons, and J.D. May. 1998. Air velocity and high temperature effects on broiler performance. Poultry Science 77: 391-393. Maghirang, R.C., M.C. Puma, Y. Liu, and P. Clark. 1997. Dust concentrations and particle size distribution in an enclosed swine nursery. Transactions of the ASAE 40(3): 749-754. Mankell, K.O., K.A. Janni, R.D. Walker, M.E. Wilson, J.E. Pettigrew, L.D. Jacobsen, and W.F. Wilcke. 1995. Dust suppression in swine feed using soybean oil. Journal of Animal Science 73(4): 981-985. Martin, J.D. 1998. Air filtration system may reduce salmonella. Feedstuffs 71(6): 28. May, J.D., B.D. Lott, and J.D. Simmons. 1998. The effect of environmental temperature and body weight on growth rate and feed:gain of mail broilers. Poultry Science 77: 499-501. McClure, J., Y. Zhang, and S. Jerez. 2004. An isokinetic total suspended particulate sampler for measurements of emissions from confinement animal buildings. In: Proceedings of A&WMA 97th Annual Conference and Exhibition, Indianapolis, IN : 22-25 June, Air and Waste Management Association: Pittsburgh, PA (on CD-ROM). McKinney, D.B. and L.R. Wilhelm. 1998. Cold weather gas level measurements in swine houses with and without pit-ventilation. ASAE Paper No. 98-4075. ASAE, St. Joseph, MI. Means, S.L., R.A. Bucklin, R.A. Nordstedt, D.K. Beede, D.R. Bray, C.J. Wilcox, and W.K. Sanchez. 1992. Water application rates for a sprinkler and fan dairy cooling system in hot, humid climates. Applied Engineering in Agriculture 8(3): 375-379. Meyer, D.J. 1998. Winter air quality in swine buildings with different pit additives. In Animal Production Systems and the Environment Proceedings, Volume 1: 311-314. Iowa State University, Ames, IA. Miner, J.R. and L.A. Licht. 1980. Fabric swatches as an aid in livestock odor evaluations. Pp. 302-305 in: Livestock Waste: A Renewable Resource, Proc. 4th Intl. Symposium on Livestock Wastes, April 15-17, 1980, Amarillo, TX. ASAE, St. Joseph, MI. Montoya, R.E., R.A. Bucklin, R.A. Nordstedt, J. Van-Horn, Jr., and D.R. Bray. 1995. Factors affecting water usage in fan and sprinkler cooling systems for dairy cattle. Applied Engineering in Agriculture 11(1): 125-130. Moore, P.A., Jr., T.C. Daniel, D.R. Edwards, and T.C. Sauer. 1996. Evaluation of chemical amendments to reduce ammonia volatilization from poultry litter. Poultry Science 75(3): 315-320. Morris, P.D., S.W. Lenhart, and W.S. Service. 1991. Respiratory symptoms and pulmonary function in chicken catchers in poultry confinement units. American Journal of Industrial Medicine 19: 195-204. Murphy, J.P., D.A. Nichols, and F.V. Robbins. 1987. Drip cooling of lactating sows. Applied Engineering in Agriculture 3(2): 200-202. Nicolai, R. and K. Janni. 1998. Biofiltration technology for odor reduction from swine buildings. In: Animal Production Systems and the Environment, Proceedings Volume I, July 19-22, 1998, Des Moines, IA. Iowa State University, Ames, IA. Pp. 327-332. Nicolai, R.E., L.D. Jacobson, K.A. Janni, P.R. Goodrich, C.J. Clanton, and D.R. Schmidt. 1997. Development of a dynamic olfactometer lab. ASAE Paper No. 97-4019, ASAE, St. Joseph, MI. Obrock, H.C.E., P.S. Miller, A.J. Lewis, and P.K. Shoemaker. 1997. The effect of reducing dietary protein concentration on odor emission from pig buildings. Presented at American Society of Animal Science Midwestern Section, March 17-19, Des Moines, IA. Abstract Number 38. Olenchock, S.A. 1988. Endotoxins in various work environments in agriculture. Dev. Indust. Microbiol. 31: 193-197. Panti, N.K. and S.P. Clarke. 1991. Transient hazardous conditions in animal buildings due to manure gas released during slurry mixing. Applied Engineering in Agriculture 7(4): 478-484. Parbst, K.E. 1998. Evaluation of particulate removal methods for controlling odor emissions from swine housing. Unpublished M.S. thesis, North Carolina State University, Department of Biological and Agricultural Engineering, Raleigh, NC. 137 pp. Person, H.L., L.D. Jacobson, and K.A. Jordan. 1979. Effect of dirt, louvers, and other attachments on fan performance. Transactions of the ASAE 22(3): 612-616. Phillips, V.R., M.R. Holden, R.W. Sneath, J.L. Short, R.P. White, J. Hartung, J. Seedorf, M. Schroder, K.H. Linkert, S. Pedersen, H. Takai, J.O. Johnsen, P.W.G. Groot Koerkamp, G.H. Uenk, J.H.M. Metz, and C.M. Wathes. 1998. The development of robust methods for measuring concentrations and emission rates of gaseous and particulate air pollutants in livestock buildings. Journal of Agricultural Engineering Research 70(1): 11-24. Pickrell, J.A., A.J. Hebere, J.P. Murphy, S.C. Henry, M.M. May, D. Nolan, F.W. Oehme, J.R. Gillespie, and D. Schoeneweis. 1993. Characterization of particles, ammonia and endotoxin in swine confinement operations. Vet. & Human Toxicology 35(5): 421-428. Powers, W.J. and M.A. Faust. 1998. Effects of dietary bloodmeal on manure odor from weaned pigs. Journal of Animal Science 76(Suppl 1): 173. Powers, W.J., H.H. Van Horn, A.C. Wilkie, C.J. Wilcox, and R.A. Nordstedt. 1999. Effects of anaerobic digestion and additives to effluent or cattle feed on odor and odorant concentrations. Journal of Animal Science (in press). Reece, F.N. and B.D. Lott. 1981. Typical broiler chicken growth rates. Poultry Science 61: 1013-1014. Reece, F.N. and B.D. Lott. 1983. The effects of temperature and age on body weight and feed efficiency of broiler chickens. Poultry Science 62: 1906-1908. Reynolds, S.J., K.J. Donham, J. Stookesberry, P.S. Thorne, P. Subramanian, K. Thu, and P. Whitten. 1997. Air quality assessments in the vicinity of swine production facilities. J. Agromed. 4:37-45. Ryan, D.P., M.P. Boland, E. Kopen, D. Armstrong, L. Munyakazi, R.A. Godke, and R.H. Ingraham. 1992. Evaluating two different evaporative cooling management systems for dairy cows in a hot dry climate. Journal of Dairy Science 75(4): 1052-1059. Schiffman, S.S. 1998. Livestock odors: implications for human health and well-being. Journal of Animal Science 76: 1343-1355. Schiffman, S.S. and C.M. Williams. 1999. Evaluation of swine odor control products using human odor panels. Proc. of the 1999 Animal Waste Management Symposium, North Carolina State University, Raleigh, NC. Pp. 110-118. Schiffman, S.S., E.A. Sattely Miller, M.S. Suggs, and B.G. Graham. 1995. The effect of environmental odors emanating from commercial swine operations on the mood of nearby residents. Brain Research Bulletin 37(4): 369-375. Seedorf, J., J. Hartung, M. Schroder, K.H. Linkert, M.R. Holden, R.W. Sneath, J.L. Short, R.P. White, S. Pederson, H. Takai, J.O. Johnsen, J.H.M. Metz, P.W.G. Groot Koerkamp, G.H. Uenk, and C.M. Wathes. 1998. Concentrations and emissions of airborne endotoxins and microorganisms in livestock buildings in Northern Europe. Journal of Agricultural Engineering Research 70: 97-109. Shao, J., H. Xin, and J.D. Harmon. 1997. Neural network analysis of postural behavior of young swine to determine the IR thermal comfort state. Transactions of the ASAE 40(3): 755-760. Simmons, J.D. and B.D. Lott. 1993. Automatic fan control to reduce fan run time during warm weather ventilation. Journal of Applied Poultry Research 2(4): 314-323. Simmons, J.D. and B.D. Lott. 1996. Evaporative cooling performance resulting from changes in water temperature. Applied Engineering in Agriculture 12(4): 497-500. Simmons, J.D. and B.D. Lott. 1997a. Computer-controlled fogging. Applied Engineering in Agriculture 13(4): 525-530. Simmons, J.D. and B.D. Lott. 1997b. Reduction of poultry ventilation fan output due to shutters. Applied Engineering in Agriculture 13(5): 671-673. Simmons, J.D., B.D. Lott, and J.D. May. 1997. Heat loss from broiler chickens subjected to various air speeds and ambient temperatures. Applied Engineering in Agriculture 13(5): 665-669. Singletary, I.B., R.W. Bottcher, and G.R. Baughman. 1996. Characterizing effects of temperature and humidity on misting evaporative efficiency. Transactions of the ASAE 39(5): 1801-1809. Sorel, J.E., R.O. Gauntt, J.M. Sweeten, D.L. Reddell, and A.R. McFarland. 1983. Design of a 1-butanol scale dynamic olfactometer for ambient odor measurements. Transactions of the ASAE 26(4): 1201-1205, 1216. SRDC 93-03. 1994. Interior environment and energy use in poultry and livestock facilities. A regional project proposal. Preparation coordinated by Robert Bottcher, North Carolina State University. Stark, G.L., R.D. Perritt, and D. Evans. 1994. Analysis of energy conserving devices in East Texas broiler house. Applied Engineering in Agriculture 10(6): 857-861. Sutton, A.L., K.B. Kephart, J.A. Patterson, D.D. Jones, A.L. Heber, D.T. Kelly, R. Mumma, and E. Bogus. 1998a. Amino acids and carbohydrates in pig diets to reduce ammonium nitrogen and pH of manure. Journal of Animal Science 76(Suppl 1): 173. Sutton, A.L., J.A. Paterson, O.L. Adeaola, B.A. Richert, D.T. Kelly, A.J. Heber, K.B. Kephart, R. Mumma, and E. Bogus. 1998b. Reducing sulfur-containing odors through diet manipulation. In: Proceedings of the Animal Production Systems and the Environment: An International Conference on Odor, Water Quality, Nutrient Management and Socioeconomic Issues. Des Moines, IA, July 19-22, pp. 125-130. Sweeten, J.M., D.L. Reddell, A.R. McFarland, R.O. Gauntt, and J.E. Sorel. 1983. Field measurement of ambient odors with a butanol olfactometer. Transactions of the ASAE 26(4): 1206-1216. Sweeten, J.M., C.B. Parnell, Jr., B.W. Shaw, and B.W. Auvermann. 1998. Particle size distribution of cattle feedlot dust emission. Transactions of the ASAE 41(5): 1477-1481. Takai, H., S. Pederson, J.O. Johnsen, J.H.M. Metz, P.W.G. Groot Koerkamp, G.H. Uenk, V.R. Phillips, M.R. Holden, R.W. Sneath, J.L. Short, R.P. White, J. Hartung, J. Seedorf, M. Schroder, K.H. Linkert, and C.M. Wathes. 1998. Concentrations and emissions of airborne dust in livestock buildings in Northern Europe. Journal of Agricultural Engineering Research 70(1): 59-77. Thedell, T.D., J.C. Mull, M.E. Gladish, and M.J. Peach. 1980. A brief report of Gram-negative bacteria endotoxin levels in airborne and settled dust in animal confinement buildings. American Journal Ind. Med. 1,3. Thelin, A., O. Tegler, and R. Rylander. 1984. Lung reactions during poultry handling related to dust and bacterial endotoxin levels. Europ. J. Resp. Dis. 65: 266-271. Timmons, M.B. and P.E. Hillman. 1993. Partitional heat losses in heat stressed poultry as affected by wind speed. 4th International Livestock Environment Symposium, London, England. July 1993. ASAE Special Publication, St. Joseph, MI. Timmons, M.B., R.S. Gates, R.W. Bottcher, T.A. Carter, J. Brake, and M.J. Wineland. 1995. TIV algorithms for poultry environmental control. Journal of Agricultural Engineering Research 62(4): 237-245. Turner, L.W., R.C. Warner, J.P. Chastain, and H.F. Elder. 1991. Forced evaporative cooling of diary cows: on-farm demonstration results. ASAE Paper No. 91-4023. ASAE, St. Joseph, MI. Turner, L.W., J.P. Chastain, R.W. Hemken, R.S. Gates, and W.L. Crist. 1992. Reducing heat stress in dairy cows through sprinkler and fan cooling. Applied Engineering in Agriculture 8(2): 251-256. US EPA Method TO-14A, Determination of VOCs in ambient air using specially prepared canisters with subsequent analysis by GC, 2nd edition, Jan. 1997 US EPA Method TO-15, Determination of VOCs in air collected in specially-prepared canisters and analyzed by GC/MS, 2nd edition, Jan. 1997 US EPA Method TO-17, Determination of VOCs in ambient air using active sampling onto sorbent tubes, 2nd edition, Jan. 1997 Wathes, C.M. 1998. Aerial emissions from poultry production. Worlds Poultry Science Journal 54: 242-251. Watson, R.T., L.C. Meiro Filho, E. Sanhueza, and A. Janetos. 1992. Sources and sinks. In: Climate change 1992, the supplementary report to the IPPC Scientific Assessment. J.T. Houghton, B.A. Callander, and S.K. Varney, eds., pp. 25-46. Cambridge University Press, Cambridge. Whyte, R.T. 1993. Aerial pollutants and the health of poultry farmers. World's Poultry Science Journal 49: 139-156. Whyte, R.T., P.A.M. Williamson, and J. Lacey. 1993. Air pollutant burdens and respiratory impairment of poultry house stockmen. Livestock Environment IV: Proceedings of the 4th International Symposium, ASAE, St. Joseph, MI. Pp. 709-717. Whyte, R.T., P.A.M. Williamson, and J. Lacey. 1998. Comparison of the aerial environments in intensive and semi-intensive poultry laying houses and implications for stockman respiratory health. Spring conference of the International Egg Commission, London, England, 2 March 1998. Windholz, M., S. Budvari, L.Y. Stroumtsos, and M.N. Festig, eds. 1976. The Merck Index, Ninth Edition. Merck and Company, Rathway, NJ. P. 649. Xin, H., I.L. Berry, T.L. Barton, and G.T. Tabler. 1993. Energy efficiency in broiler housing systems. ASAE Paper No. 93-3015. ASAE, St. Joseph, MI. Xin, H., I.L. Berry, G.T. Tabler, and T.L. Barton. 1994. Temperature and humidity profiles of broiler houses with experimental conventional and tunnel ventilation systems. Applied Engineering in Agriculture 10(4): 535-542. Xin, H., I.L. Berry, and G.T. Tabler. 1996. Minimum ventilation requirement and associated energy cost for aerial ammonia control in broiler houses. Transactions of the ASAE 39(2): 645-653. Zahn, J.A., J.L. Hatfield, Y.S. Do, A.A. DiSpirito, D.A. Laird, and R.L. Pfeiffer. 1997. Characterization of volatile organic emissions and wastes from a swine production facility. Journal of Environmental Quality 26(6): 1687-1696. Zhang, Y. 1999. Engineering control of dust in animal facilities. Proceedings of the International Symposium on Dust Control in Animal Production Facilities, 30 May 2 June 1999, Aarhus, Denmark. Danish Institute of Agricultural Sciences, Horsens, Denmark. Pp. 22-29. Zhang, Y. and E.M. Barber. 1995. An evaluation of heating and ventilation control strategies for livestock buildings. Journal of Agricultural Engineering Research 60(4): 217-225. Zhang, Y., A. Tanaka, E.M. Barber, and J.J.R. Feddes. 1996. Effects of frequency and quantity of sprinkling canola oil on dust reduction in swine buildings. Transactions of the ASAE 39(3): 1077-1081. Zhang, Y., A. Tanaka, J.A. Dosman, A. Senthilselvan, E.M. Barber, S.P. Kirychuck, L.E. Holfeld, and T. Hurst. 1997. Respiratory responses of human subjects to air quality in a swine building. Livestock Environment V: Proceedings of the 5th International Symposium, Volume II, ASAE, St. Joseph, MI. Pp. 655-663. Zhang, Y., A. Tanaka, J.A. Dosman, A. Senthilselvan, E.M. Barber, S.P. Kirychuk, L.E. Holfeld, and T.S. Hurst. 1998. Acute respiratory responses of human subjects to air quality in a swine building. Journal of Agricultural Engineering Research 70: 367-373.