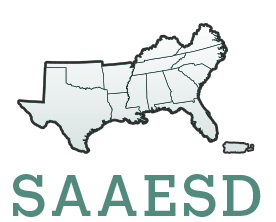
S1015: Host Resistance as the Cornerstone for Managing Plant-Parasitic Nematodes in Sustainable Agroecosystems (S-282)
(Multistate Research Project)
Status: Inactive/Terminating
S1015: Host Resistance as the Cornerstone for Managing Plant-Parasitic Nematodes in Sustainable Agroecosystems (S-282)
Duration: 10/01/2003 to 09/30/2009
Administrative Advisor(s):
NIFA Reps:
Non-Technical Summary
Statement of Issues and Justification
Project's Primary Website is at http://www.uga.edu/srel/S-1014_Multi-State_Research_Project/index.html (direct link can be found under LINKS) The proposed project is requesting approval for 5 years due to the long term nature of plant breeding programs. It often requires more than 10 years from the discovery of a resistance gene until that gene is available to growers in agonomically acceptable cultivars. The first nematode resistance cultivars in peanut (Simpson and Starr, (2001), soybean, and tomato (Starr et al., 2002) took longer than 10 years from discovery to availability to the growers. It is expected that many cultivars and new sources of resistance will be released during the life of this project because of prior achievements in this direction. The proposed project will build on the successes in discovery, characterization, and develop of resistance made under the current S-282 project. Plant-parasitic nematodes cause yield suppression in many crops species. The warm temperate to subtropical climates, abundance of favorable, coarsely-textured soils, and numerous susceptible crops all combine to make nematodes particularly important pathogens in the southern region of the United States. Among the crops with the greatest estimated losses due to nematode parasitism are cotton, cucurbits, leguminous vegetables, peanut, solanaceous vegetables, soybean, sugarcane, and tobacco (Koenning et al. 2000). Only alfalfa, hay, sorghum, rice, and wheat among the major crops were estimated to have losses of less than 1% of the yield potential. Nematode pathogens can be managed by application of various nematicides, by rotating to nonhost crops, by host resistance, and in some cases by biological control. Unfortunately, except for resistance, the use of these approaches is frequently not feasible on an economic basis due to low profit margins for most crops. There are a limited number of suitable nonhosts that can be rotated with the primary crop of interest in most cropping systems. Nematicides are costly and pose significant environmental and human health risks. There is a strong desire to further limit nematicide use. No new nematicide that has broad efficacy has been labeled for use in the past 25 years. The Food Quality Protection Act of 1996 will result in further restrictions on the use of nematicides and likely result in loss of registration for some (www.epa.gov/opppsps1/fqpa/). Increased use of host resistance to manage nematodes is attractive for several reasons. Resistance is an attractive option because it both protects crop yields from the damaging effects of nematode parasitism and resistance inhibits nematode development and reproduction (Roberts, 2002). Thus resistance, which can usually be used without any increase in production costs, also suppresses nematode population densities, and provides protection for subsequent susceptible crops. The warm temperate to subtropical environment of the southern region of the United States enables plant-parasitic nematodes to complete multiple generations during the growing season. Additionally, in some cropping systems, winter survival is enhanced not only by the relatively mild winters of the region but also by the presence of susceptible hosts throughout the year. The southern region also has an abundance of coarsely textured soils that support the highest levels of nematode activity. Thus plant nematodes cause greater crop losses in the southern region than in any other region of the country. The best documentation of these losses is for soybean (www.scnfact.org; www.ceris.purdue.edu/napis/pests/scn/facts.txt) and cotton (www.cotton.org/tech/pests/nematodes/losses.cfm). Soybean is susceptible to nine different nematode species, with the cyst nematode Heterodera glycines (SCN) being responsible for the greatest losses. SCN is present throughout the southern and midwestern states, and is found in a wide array of soils. The three root-knot nematodes (Meloidogyne arenaria, M. incognita, M. javanica ) are also widely distributed in all southern states and, whereas the distribution of these species is most common in the more coarsely textured soils, as a group they also cause substantial yield losses in soybean and many other crops. Other nematodes of more localize importance to soybean include the reniform nematode Rotylenchulus reniformis , the lance nematode Hoplolaimus columbus, lesion nematode Pratylenchus brachyurus, the stubby-root nematodes Paratrichodorus and Trichodorus, spp. and the sting nematode Belonolaimus longicaudatus. Among the other major crops of the region M. incognita and R. reniformis are important on cotton, M.arenaria is important on peanut, and all of the Meloidogynes species are important on several vegetable crops. As indicated by the numerous stakeholders listed below that support the research efforts of one or more project participants, there is broad support of the ongoing efforts for the efforts of project personnel to develop additional sources of resistance to plant-parasitic nematodes in a large number of important crop species. These stakeholders consider the efforts to identify, characterize, and deploy host resistance to be of high priority. _______________________________________________________________________ Alabama Cotton Commission Arkansas Soybean Promotion Board Cotton Incorporated Delta Pine and Land, Syngenta, and Paymaster Seeds Florida Peanut Producers Association Georgia State Support Program, Cotton Inc. Georgia Agricultural Commodity Commission for Corn Georgia Agricultural Commodity Commission for Peanut Minnesota Soybean Research and Promotion Council National Peanut Board North Carolina Soybean Producers Association Inc. North Carolina State Support Program, Cotton Inc South Carolina Soybean Board Tennessee Soybean Promotion Board Texas Peanut Producers Board United Soybean Board ______________________________________________________________________ Consequences if research not completed. Previously, plant-parasitic nematodes were controlled through the use of a variety of fumigant or non-fumigant nematicides (Whitehead, 1998). Availability of these pesticides has been increasingly restricted over the past 25 years due to increased federal regulation as concerns for human health and environmental safety increased. The Food Quality Protection Action (1996) is resulting in further restrictions on the use of nematicides. For example, the systemic nematicide phenamiphos will be withdrawn from all uses in the United States by corporate action by 2007 (personal communication, A. Lake, Bayer Crop Sciences). Additionally, because of the relatively limited market for nematicides (relative to herbicides and insecticides) and high cost of development, it is unlikely that new, safer nematicides will be developed in the near future. Thus, failure to make more effective use of currently available sources of resistance in sustainable cropping systems and to develop additional sources of host resistance will result in increased yield losses to producers as availability of nematicides declines. Another concern relative to an over reliance on nematicides for management of nematodes is the failure of these pesticide to adequately control the pest population. Resistance to insecticides (Richter, 1992) and fungicides (Staub, 1991) in target pest populations is a well-documented phenomenon. No resistance to nematicides in populations of plant-parasitic nematodes has been documented outside of experimental, laboratory circumstances (Kampfe, 1995). However several instances of increased degradation of nematicides by soil microflora have been reported. These include the fumigant 1,3 dichloropropene (Ou et al., 1995), fenamiphos (Davis et al., 1993; Ou et al., 1994), and most recently aldicarb applied to cotton for control of reniform nematodes (McLean and Lawrence, 2003). Collectively, these three nematicides (aldcarb, 1,3-dichloropropene, and fenamiphos) are the most widely used nematicides in the United States. Continued reliance on such nematicides as may remain labeled, as opposed to development of more sustainable management systems, is likely to lead to additional incidences of nematicide failures due to increased rates of microbial degradation. Although numerous soybean cultivars in a wide range of maturity groups are available that have resistance to a few races or species of H. glycines, Meloidogyne spp., and R. reniformis, this resistance is derived from relatively few genes. Thus, this resistance is vulnerable to virulence shifts in the nematode populations in response to selection pressures imposed by reliance on a limited number of resistance genes. Such shifts in virulence are well documented in the SCN/soybean system (Young and Hartwig, 1992). Of the species of root-knot nematodes that are responsible for substantial yield losses of peanut, M. arenaria has the greatest frequency distribution on peanut, but there are increasing finds of M. javanica parasitic on peanut and an as yet undescribed Meloidogyne species on peanut. Increased use of recently developed resistance to M. arenaria and M. javanica in peanut is likely to select for increased occurrence of other species as has been observed with tobacco after use of resistance to M. incognita became widespread (Fortnum et al., 1984). Currently available resistance to M. arenaria and M. javanica in peanut is due to a single dominant gene (Choi et al., 1999 ) and is available only in two cultivars that lack other necessary traits, especially resistance to the tomato spotted wilt virus (Starr et al., 2002 ). Additionally, currently there is no resistance in any peanut genotype to M. hapla, which is the most prevalent root-knot species in the NC/Va production region. The number of resistance genes available must be expanded and resistance introgressed in to a wider array of soybean and peanut cultivars. Failure to expand the numbers of resistance genes available to producers will result in increased yield losses with the development of nematode populations with virulence on currently available sources of resistance. Cotton is the second most important crop of the southern region in terms of magnitude of losses due to nematodes. Based on data from regional surveys of nematode distribution and cotton response to nematicide treatments, total annual yield losses to the 15 million acre cotton crop have been estimated at $380 million for 2001 (Blasingame, 2002). The principal nematodes causing losses to cotton are the root-knot nematode M. incognita ($159 million in losses), which is widely distributed in all cotton production areas, and R. reniformis. Prior to the 1990's, the reniform nematode was considered a minor problem with most reports of crop losses from Louisiana and Mississippi. This nematode is now documented to be present throughout the southern region and in some areas of Alabama, Louisiana, and Mississippi is causing greater losses than M. incognita. The Columbia lance nematode (H. columbus) and the sting nematode (B. longicaudatus) are aggressive pathogens with more limited distributions than root-knot or reniform nematodes. Several sources of resistance are known for M. incognita in the cotton germplasm collection, and a few cultivars have been developed. However, the only currently available cultivar is the Acala-type NemX and it is not adapted to climates or production practices outside of Arizona and California. Resistance to R. reniformis is known in diploid Gossypium species (Robinson et al., 2003; Yik and Birchfield, 1984). However, genetic incompatibility represents a formidable barrier to introgression of these resistance genes into tetraploid cultivated cotton. Failure of the cotton industry to develop and use more resistance, as compared to the soybean and tobacco industries, is due to a number of factors. Chief among these are the perceived limited importance of nematodes relative to other pathogens and pests (Mueller et al., 1996), and the lack of effective, low cost, high through-put screening methodologies needed by commericial breeding programs. Failure to develop modern marker assisted selection protocols for nematode resistance will significantly delay development of nematode-resistant cultivars of cotton. Technical Feasibility of proposed research. The identification of resistance to numerous species of nematodes is a routine but time consuming task. Resistance is usually identified based on reduced levels of nematode reproduction on the resistant hosts relative to a susceptible host genotype (Roberts, 2002). Root-gall indices are a good measure of resistance to root-knot nematodes (Hussey and Jensen, 2002) but can be complicated by the presence of N-fixation nodules on peanut and soybean. Nodules can be mistaken for galls and thus plants with both galls and nodules require more time to evaluate, decreasing the efficiency of the system. Development of females and cysts (typically referred to as a female index) is often used to assess resistance to the soybean cyst nematode (Cook and Noel, 2002). Marker-assisted selection (MAS) systems typically use one of several methods available for rapid detection of specific differences in DNA sequences between susceptible and resistant individuals (Young and Mudge, 2002). The several techniques (AFLP, RAPD, RFLP, SSR) are now routine at nearly all participating institutions, and in many of the laboratories of participating scientists. In the case of tomato, the resistance gene Mi-1 has been cloned and sequenced (Milligan et al., 1998 ), thus PCR primers that specifically amplify segments of the resistance gene can be used for MAS. With most other crops, the DNA sequence that is specific for the resistance loci is merely a sequence that is tightly linked genetically (ideally at a mapping distance of less than 5.0 cM) to the resistance locus. Such systems have already been developed for peanut (Church et al., 2002) and soybean (Young and Mudge, 2002); however, the peanut system is based on RFLP and needs additional research so that a more efficient system can be developed. Although no molecular markers linked to resistance in cotton have been reported, a molecular map of cotton has been developed (Rienisch et al., 1994) and can be used as a basis for identifying markers linked to resistance to root-knot nematodes. In cases where genetic data is more limited, techniques such as bulk segregant analysis (Michelmore et al.,1991) can be used to identify markers linked to resistance. Need for cooperative work. Currently, there are relatively few SY's devoted to plant nematology relative to the need. A coordinated, cooperative effort will increase the efficient use of these limited resources. A structure for cooperative work exists within the current S-282 Project, as demonstrated by the successful development of resistance in soybean, peanut and pepper. The proposed research on nematode resistance and how to most effectively integrate resistance with other control strategies and tactics can best be done by cooperation among the diminishing number of nematologists in the participating states and USDA ARS. Formal cooperation among participating scientists, through exchange of germplasm resources, avoidance of duplication of effort, and region-wide testing of new cultivars and cropping systems will increase the speed with which new technologies (resistance genes, resistant cultivars, MAS and cropping systems that incorporate a greater percentage of resistant crops) are developed and made available to appropriate clientele groups. The current number of nematode-resistance genes available for introgression into cultivars is relatively small and thus these genes are vulnerable to virulence shifts in nematode populations and to shifts in predominant nematode species. There is a need to identify new resistance genes to ensure durability of the resistance phenotype. Project members will cooperate in this effort by dividing the effort in terms of germplasm resources being screened and relative efforts at characterization of sources of resistance. Cooperative efforts will ensure rapid and thorough examination of available germplasm collections for new and novel sources of resistance. Most commonly grown cultivars or hybrids of soybean, cotton, and corn are developed in the private sector. Most of these private breeding companies depend on scientists from the land-grant universities and ARS to identify and characterize resistance genes. Further, most of these private companies lack the personnel and resources to effectively screen segregating breeding populations for resistance to multiple nematode species. Thus, there is an urgent need for development of DNA-based MAS systems, especially in cotton, peanut and several vegetable crops. Identification of molecular markers linked to resistance genes has been achieved in soybean and peanuts, however, some markers are based on RFLP and more efficient marker-assisted selection (MAS) systems are needed. MAS systems are needed that do not require use of 32P, but which are based on PCR technology for higher throughput (Young and Mudge, 2002). Refinement of existing MAS systems and expansion of the number of available markers will increase the ease with which private sector breeding programs can use available resistance genes. With private sector breeding programs assuming an increasingly important role in cultivar development, it is essential that we provide them not only with novel sources of resistance, but also with appropriate technology for introgression of these resistance genes into cultivars with the highest yield potentials. The communication and cooperation among participating scientists will ensure that the most urgent problems receive priority. With different laboratories focusing on different approaches (eg., RFLP, AFLP, RAPD, SSR) and different crop/nematode species systems, the greatest possible efficiency will be achieved. Finally, scientists in cooperating states will evaluate resistant germplasm along with promising strategies for the integration of resistant cultivars with biological and (or) cultural control methods under a broad range of environmental conditions to determine how broadly (or narrowly) a particular gene or cropping system might be effective. At annual research planning conferences, subcommittees organized around several research topics will meet to plan each years activities. These subcommittees will initially be orgainized around cotton, peanut, soybean, vegetables, and cropping systems. In the effort to identify new sources of resistance, the subcommittees will identify germplasm collections to be screened, intensity of screening activity, and division of effort. Similarly, once useful genes are identify, efforts to identify appropriate molecular markers will be similarly divided among the participating members such that no two participants are duplicating efforts but rather are concentrating on different resistance genes, or utilizing different approaches to identifying markers linked to the same gene. The cropping systems subcommittee will utilize a similar approach in design of experiments on optimal integration of resistance into sustaining cropping systems. Through this research planning effort, more effective use of limited SY's will achieve maximum progress across the broad objective of increased use of host resistance. Likely impacts to be derived. New vegetable, cotton, soybean, and peanut genotypes and cultivars that have durable resistance to multiple species and races of nematodes will result from the cooperative research. This resistance will allow the cultivars to achieve their genetic yield potential in fields infested with damaging population densities of plant-parastic nematodes. Resistance will be available to the growers with little or no increase in production costs and growers will be able to reduce use of costly and hazardous nematicides. Thus yields should increase with a decrease in production costs. Because resistance to nematodes typically results in a suppression of nematode reproduction, the lower nematode populations following a resistant crop will result in less nematode disease pressure on subsequent susceptible crops, as has been demonstrated in California for root-knot resistant cotton rotated with susceptible lima beans (Ogallo et al., 1999). Nematode control using nematicides typically provides only a temporary suppression of nematode population densities, such that nematicides must be applied annually. By expanding the number of crops with resistance and expanding the scope of resistance in those crops, fewer crops that support high levels of nematode reproduction will be grown. Eleven soybean cultivars or germplasm lines with resistance to one or more nematode species, three cotton breeding lines with resistance to M. incognita, three pepper germplasm lines plus two pepper cultivars with resistance to M. incognita, one cowpea cultivar with resistance to M. incognita, and two peanut cultivars and one germplasm line resistant to M. arenaria and M. javanica were released by S-282 project personnel. The proposed regional effort will build on the successes of S-282 and result in a substantial increase in the number of resistance genes identified, introgression of those genes into appropriate crop genotypes, and development of cropping systems in which overall yield losses due to nematode parasitism are reduced.
Related, Current and Previous Work
CRIS Project Review. A review of CRIS projects using plant-parasitic nematodes and host resistance revealed 26 related active projects. Fourteen of these projects were from outside the region and are focused on different cropping systems, but a minor level of redundancy is expected. Other projects were focused on other aspects of nematode biology, parasitism, and (or) management with host resistance only a small portion of the objects. Two other research projects from the southern region are focused on crops not emphasized by this proposal. Some overlap of activity between this proposed research and other research projects is necessary to ensure confirmation of research findings, fulfilling a basic tenet of science -- repeatability. To avoid excessive overlap of activitites, project members will communicate on an annual basis with members of these research projects to keep abreast of ongoing and new research activities related to activites proposed for this project. Additionally, there will be an annual review of the CRIS data base for information on related research activities and relevant new discoveries. NC -215 (Northcentral Region Technical Committee): Persistence of Heterodera glycines and other regionally important nematodes. Objectives: 1. Determine the impact of cultural factors on the attrition of nematode populations; 2. Determine the biological factors responsible for the attrition of nematode populations; 3. Characterize and understand egg dormancy. NE-171 (Northeastern Region Technical Committee): Biological and cultural management of plant-parasitic nematodes. Objectives: 1. Evaluate the effects of rotational crops, organic amendments and host crop genetics on nematode community structure; 2. Characterization of biological control agents for suppression of plant-parasitic nematodes; 3. Comparison and evaluation of IPM system managamentt of plant-parasitic nematodes based on crop rotation, organic amendments, host crop resistance and biological control agents. W-186 (Western Region Technical Committee): Genetic variability in the cyst and root-knot nematodes. Objective 1. Characterize genetic variability as related to plant-parasitic nematode responses to resistance, environment, biological processes, and morphology; 2. To determine nematode fitness and adaptability relative to environment, host plant, and host plant resistance; and 3. Design and develop management strategies for cyst and root-knot nematodes relative to genetic variability. In the previous regional research project (S282 ) several germplasm lines and cultivars with resistance to one or more nematode species were released by project scientists (Table 1). Despite this impressive accomplishment, there is need for additional effort. The range of available resistance is still limited relative to need and research is needed on integration of resistance into a variety of cropping systems. Table 1. Germplasm and cultivar releases resulting from research conducted under S-282. ______________________________________________________________________________ Boerma, H. R., R. S. Hussey, D. V. Phillips, E. D. Wood, G. B. Rowan, S. L.Finnerty and J. T. Griner. 2001. Registration of 'Prichard' soybean. Crop Sci. 41:920-921. Boerma, H. R., R. S. Hussey, D. V. Phillips, E. D. Wood, G. B. Rowan, and S.L. Finnerty. 2000. Registration of Boggs soybean. Crop Sci. 40: 294-295. Fery,R.L., and Thies,J. A. 1998. PA-353, PA-398, and PA-426: southern root-knot nematode-resistant Capsicum chinense Jacq. germplasm lines. HortScience 33:760-761. Fery, R. L., Dukes, P. D., Sr., and Thies, J.A. 1998 'Carolina Wonder' and 'Charleston Belle': southern root-knot nematode-resistant bell peppers. HortScience 33:900-902. Kays, S. J., McLaurin,W. J., Wang, Y., Dukes, P. D., Thies, J., Bohac, J. R., and Jackson, D. M. 2001. GA90-16: a nonsweet, staple-type sweetpotato breeding line. HortScience 36:175-177. Nickell, C. D., Noel, G. R., Tharp, J. E., Cary, T. R., and Thomas, D. J. 1998. Registration of LN92-11008 soybean. Crop Sci. 38:546. Nickell, C. D., Noel, G. R., Cary, T. R., and Thomas, D. J.1998. Registration of Dwight soybean. Crop Sci. 38:1398. Nickell, C. D., Noel, G. R., Cary, T. R., Thomas, D. J., and Diers, B. W. 2001. Registration of Loda soybean. Crop Sci. 41:589-590. Shipe, E. R., J. D. Mueller, S. A. Lewis, P. F. Williams, Jr., and J. P. Tomkins. 2000. Registration of Motte soybean. Crop Science 40:1497-1498. Shipe, E. R., J. D. Mueller, S. A. Lewis, P. F. Williams, JR., and J. P. Tomkins. 2000. Registration of Musen soybean. Crop Science 40:1496-1497. Sleper, D. A., Nickell, C. D., Noel, G. R., Cary, T. R., Tharp, J. E., Thomas, D. J., K. M. Clark, and Rao Arelli, A. P.1998. Registration of Maverick soybean. Crop Sci. 38:549-550 Simpson, C. E., and J. L. Starr. 2001. Regisration of COAN' peanut. Crop Science 41:918. Simpson, C. E., J. L. Starr, G. T. Church, M. D. Burow, and A. H. Paterson. 2003. Registration of NemaTAM peanut. Crop Science 43: 1561. Stalker, H. T., Beute, M. K., Shew, B. B., and Barker, K. R. 2002. Registration of two root-knot nematode-resistant peanut germplasm lines. Crop science 42:312-313. Starr, J. L., and C. W. Smith. 1999. Registration of three root-knot nematode resistant germplasm lines of upland cotton. Crop Science 39:303. Walters, S. A,and Wehner, T. C. 1997. Lucia, Manteo, and Shelby - root-knot nematode-resistant cucumber inbred lines. HortScience. 32:1301-1303. ______________________________________________________________________________
Objectives
-
Identification, characterization, and introgression of genes for resistance and tolerance to nematodes into cotton, peanut, soybean, and major fruit and vegetable crops.
-
Development of marker-assisted selection systems for more efficient introgression of multiple resistance genes into agronomically superior crop genotypes.
-
Deployment of resistance and tolerance to nematodes in sustainable cropping systems.
-
Methods
Resistance in most crops being studied will be identified based on inhibition of nematode reproduction (Roberts, 2002). Resistance to Meloidogyne spp. may also be measured based on a root-gall index (Hussey & Janssen, 2002) and resistance to Heterodera/Globodera spp. may be measured as a female index (Cook & Noel, 2002). All of these procedures are well established and data is easily shared among collaborating laboratories. Tolerance will be defined as the ability of a crop genotype to provide relatively high yield even when parasitized by a nematode (Roberts, 2002). This trait is typically measured in field tests with a split-plot design, utilizing genotype as the main plots and nematicide treatment as the subplots (Bowman & Schmitt, 1994). Genotypes that do not respond to nematicide treatment (i.e., there is no significant yield suppression due to nematode parasitism) when nematode population densities exceed the damage threshold for a susceptible cultivar will be classified as tolerant. Research subcommittees will identify particular areas of concentration and division of effort. Soybean. Although there are multiple soybean cultivars with different levels of resistance to SCN, the resistance of most cultivars is narrow with respect to that which is needed to control the numerous races of SCN. Several participants will work towards introgression of SCN resistance into traditional and transgenic soybean genotypes using cultivars which have resistance to multiple races of SCN, as resistant parents. (AR, GA, IL, NC, SC, ARS-TN) Additionally, resistance to different Meloidogyne spp. and R. reniformis will be introgressed in new soybean germplasm lines. Perennial and other exotic Glycines spp. will be screened for new sources of resistance to SCN. (IL) New sources of resistance identified will then be characterized for race specificity and rapidly made available to the other programs for testing and utilization. Efforts to identify useful levels of tolerance to H. columbus among currently available soybean cultivars will continue. (NC) One research program will focus on the bioengineering of resistance. (NC) Of particular significance are molecular signals (secretions) from nematodes that are required for penetration and feeding within host plant roots (Davis et al., 2000; Goellner et al., 2000; Goellner et al., 2001). Research within the upcoming period will focus on identifying the function(s) of SCN genes encoding putative parasitism factors, primarily by disrupting the activity of each gene or gene product. Comparison of some SCN parasitism genes to those in other plant-parasitic nematodes suggests that some mechanisms of parasitism may be conserved across nematode species (Hussey et al., 2003), indicating that mechanisms of inhibition to SCN may be applicable to multiple nematode and crop species. Cotton. No resistance to R. reniformis is known within G. hirsutum but much of the more than 4000 accessions in the USDA germplasm collection remains to be screened. A major effort of this project will be a systematic screening of these accessions for resistance to R. reniformis. (AL, ARS-TX) Resistance to R. reniformis does exist within other Gossypium spp. but its use is hampered by fertility barriers. Two cooperating projects are working to introgress resistance to R. reniformis from G. barbadense and G. longicalyx into G. hirsutum genotypes that are also resistant to M. incognita. (TX , ARS-TX) Additionally, these efforts will be coordinated with an ARS project in Mississippi that is not part of this proposal but is working to introgress resistance to R. reniformis into G. gossypium using other exotic Gossypium species (pers. commun., L. Young). Both of the G. barbadense and G. longicalyx introgression efforts have successfully developed fertile interspecific hybrids that carry resistance to the reniform nematode (Ripple, 2002; Robinson, unpub. data; Silvey et al., 2003), and future efforts will concentrate on advancing the resistance into genotypes with appropriate agronomic traits using backcross breeding programs. Variation in host reaction among geographical isolates of the reniform nematode has been observed (McGawley, unpublished data). The efforts to characterize this variation will continue and will be expanded to include popular tomato and bell pepper cultivars. (LA) Numerous isolates of R. reniformis collected from across the southern states will be increased on ?Rutgers? tomato in the greenhouse and a number of "pure" cultures will be derived from single egg masses. These populations are needed to confirm "trueness to type" and species identification, and will be used to assess host range, reproduction, and pathogenicity. Host range studies involve inoculation of host differentials used to separate species and races of root-knot nematode as well as several other leguminous and non-leguminous plant species. Selected populations will be advanced to a microplot environment for further evaluation. At harvest, plant dry weights, cotton yield, and nematode reproduction will be determined. These efforts are needed to provide a basis for development of resistance management systems that promote durability of resistance as resistance to this nematode is developed and deployed. Isolates of the reniform nematode with confirmed differences in host specificity will then be tested against different sources of resistance being developed by the introgression projects. Because resistance to R. reniformis does not currently exist among cotton cultivars but tolerance to this nematode has been identified (Cook et al., 1997), one project will focus on characterization of tolerance among modern cotton cultivars. (NC) These efforts will include economic analysis of the benefits of growing cultivars with tolerance without nematicide applications compared to growing non-tolerant cultivars with nematicide treatments (Davis et al., 2002). The identification of transgenic cotton cultivars that might contain useful levels of tolerance will provide an alternative to nematicides until high levels of resistance can be introgressed into cotton cultivars. Peanut. Although the first peanut cultivars with resistance to M. arenaria and M. javanica have recently been released, this single-gene resistance (Choi et al., 1999; Church et al. 2000) may lack durability if virulence to this resistance develops in the nematode populations. Resistance to M. arenaria derived from A. cardenasii has been introgressed into A. hypogaea by two distinct methods. The Texas germplasm is based on a synthetic triple species hybrid (Simpson,1991) that used a diploid introgression pathway, whereas the North Carolina germplasm is based on A. hypogaea x A. cardenasii using a hexaploid introgression pathway (Stalker et al., 2002). Arachis cardenasii has multiple genes for resistance (Starr & Simpson,1991; Garcia et al.,1996 ), therefore the different introgression pathways may result in genotypes with different resistance genes. Resistant genotypes from the NC introgression pathway have been crossed with the resistant cultivar COAN (from the TX introgression pathway) and progeny will be screened to determine if they have genes in common. (NC) Additionally, efforts are in progress to identify other genes for resistance derived from A. batizocoi and A. diogoi that are likely to be present in the germplasm resulting from the triple species complex hybrid (Church, 2002) from the TX introgression pathway. (TX) The tomato spotted wilt virus is a major constraint to peanut production in the southeast. Because of the explosive nature of TSWV epidemics, which may result in yield losses of greater than 50% in individual fields, management of the virus is given priority over management of root-knot nematodes by growers. Peanut cultivars with resistance to nematodes but which are not resistant to TSWV are not used by growers in the southeast. To be widely accepted by growers in this region, a nematode-resistant peanut cultivar must also have resistance to TSWV. Three programs (FL, GA, and TX) are working to introgess nematode resistance into regionally adapted TSWV resistant genotypes. These lines will be evaluated in field and greenhouse experiments for agronomic characteristics and resistance to TSWV and M. arenaria. Vegetables. The specificity of resistance to Meloidogyne spp. conferred by the N gene in Capsum annuum has been characterized; however, the mechanism(s) of resistance are not understood. Penetration and development of root-knot nematodes in roots of resistant and susceptible pepper genotypes of C. annuum will be characterized in controlled temperature experiments. (ARS-SC). The experimental design will be a randomized complete block with six replicates. Eight weeks post-inoculation, roots of the plants will be collected, egg masses on roots will be stained with red food color, and the roots will be rated for galling severity and egg mass production; eggs will be extracted from fibrous roots using NaOCl, and counted. Durability of root-knot nematode resistance in the pepper cultivars homozygous dominant for the N gene will be determined by monitoring M. incognita population development for several generations on resistant pepper plants. (ARS-SC) Two near-isogenic sets of bell pepper cultivars will be inoculated with 3,000 eggs of M. incognita race 3. Eggs will be extracted from roots, counted, and new seedlings of each cultivar will be inoculated with 3,000 eggs at 6 to 8 wk. intervals for 10 to 15 generations. The experiment will be a randomized complete block design with six replicates per cultivar. Sweet potato. The reniform nematode is a major pathogen of sweet potato in some production regions, but no cultivars with high levels of resistance are available. Two thousand accessions of sweet potato from the germplasm collection that is maintained at the University of Georgia will be screened for resistance to R. reniformis. (MS) Tobacco. Tobacco hybrids have been developed in North Carolina from parents that carry genes for resistance to two races of M. incognita, M. javanica, and G. tobacum. These hybrids will be screened to confirm resistance phenotypes among the progeny. (VA) Each year, results of the numerous screening activities will be reported at the annual meeting of project personnel. A result of these presentations will be exchange of appropriate germplasm among project personnel to ensure that newly identify sources of resistance, resistance with unique traits, and (or) tolerance is rapidly incorporated into the breeding programs of each state. Objective II. Cotton. Resistance to M. incognita is available in several wild accessions and advanced generation cotton germplasm lines Robinson & Percival, 1997; Shepherd et al.,1996; Starr and Smith, 1999) but few cultivars with resistance are available. Most modern cotton cultivars are products of the private sector and these cotton breeding efforts lack the resources for phenotypic screening of cotton for nematode resistance but expertise for marker-assisted selection (MAS) does exist. Nematode resistance will be introgressed into cultivars developed by these private companies only if they have MAS systems. Two projects will use an existing RFLP map of cotton based on the interspecific hybrid G. hirsutum x G. barbadense to find markers linked to different resistance loci (Reinisch et al., 1994). In Georgia (ARS), F2 mapping populations that are segregating for resistance to M. incognita have been developed from crosses between highly resistant G. hirsutum M120, M155, and M725, and the nematode susceptible G. barbadense germplasm Pima S-6. The TX effort to map genes for resistance to root-knot nematodes is using progeny of G. hirsutum 'M315' x G. barbadense 'Tx110', in which M315 is resistant to M. incognita whereas Tx110 is resistant to R. reniformis. The progeny of this cross will also serve as a mapping population to develop RFLP markers linked to resistance to R. reniformis. The resistance to R. reniformis is believed to be a quantitative trait (Ripple, 2002), whereas resistance to M. incognita in M315 is inherited as two genes (McPherson, 1993; Silvey et al. 2003). Bulk segregant analysis (Michelmore et al., 1991) will be used for the initial screening to identify RFLP loci putatively linked to resistance loci. These linkages will be confirmed by screening segregating populations and linkage distances calculated using the Map Marker (Lander et al., 1987). The two efforts (ARS-GA, TX) will complement each other by using different sources of nematode resistance. Peanut. Three RFLP loci linked to a locus for resistance to M. arenaria in peanut have been identified. Unfortunately, these RFLP loci all map to the same side of the resistance locus (Choi et al.,1999). An F2 population segregating for resistance is being used to screen additional RFLP loci (Burow et al., 2002) to identify flanking markers. (TX) Although RFLP markers can be used in MAS, they are more time consuming than PCR-based systems and typically require the use of 32P. Therefore the RFLP locus (R2430E) that distingishes resistant and susceptible individuals is being subcloned and sequenced. The sequence will, at a minimum, have differences in location of Eco RI restriction sites between resistant and susceptible individuals and may have additional sequence divergence. These differences will be used to design PCR primers that generate amplification products specific to resistant genotypes. Tobacco. Resistance to G. tobacum in tobacco appears to be linked to a locus that confers resistance to Phytophthora parasitica var. nicotiana (Johnson et al., 2002). RAPD markers linked to the P. parasitica nicotiana resistance locus will be evaluated for their utility as markers for the single dominant resistance gene to G. tobacum.(VA) Vegetables. High-throughput molecular markers linked to the N gene that confers root-knot nematode resistance in bell pepper (Capsicum annuum) will be developed that will allow breeders to use MAS to more rapidly develop root-knot nematode resistant pepper cultivars. PCR-based markers linked to the N gene will be identified using BC6F2 populations and placed on a genetic map. The BC6F2 populations will be scored for resistance phenotype, DNA will be extracted, and bulked segregant analysis (Michelmore et al., 1991) will be performed on F2 population DNA pools from resistant (R) and susceptible (S) plants using amplified fragment length polymorphisms (AFLP) and simple sequence repeat (SSR) markers. Putative linked-markers will be confirmed by F3 progeny tests of BC6-F2 plants. Identified AFLP markers will be converted to sequence characterized amplified regions (SCARs) and mapped. Objective III. Host-plant resistance to nematodes may be overcome through shifts in in the frequency of genes for parasitism on resistant cultivars (Kalosian et al., 1996;Young and Hartwig, 1992) or through selection of different species of nematodes (Fortnum et al., 1984). Suppression of the target nematodes population densities through the use of resistance creates a void that may be exploited by other species of nematodes. Resistance genes must be used judiciously to maximize durability. Multiple tactics for management of plant-parasitic nematodes can optimize the benefits of resistance gene deployment and prolong durability. Nematode management systems that are sustainable include rotation of resistant and susceptible crops, biologically intensive management through the use of or enhancement of pre-existing biological antagonists, and the use of soil amendments including green manures, cover crops, and as organic waste products. An important aspect of this objective is the measurement of the effects of nematode resistance on non-target nematode species. Currently more than 80% of cotton and soybean acreage is planted to transgenic cultivars. A similar trend is expected for other crops. Impact of transgenic plants on plant parasitic nematodes include: i) transgenic plants may be more susceptible to nematodes as has been demonstrate for M. incognita in some cotton cultivars (Colyer et al., 2000), ii) nematode resistance to important genera of plant-parasites may be neglected in transgenic plants, iii) transgenes may have negative impacts on nematodes, and iv) transgenic plants may result in the expansion of new crops into areas where these are not currently grown, thus encountering novel nematode problems. Because crops are affected by a number of pests, nematode-resistant cultivars will must be integrated with other pest management tactics. Experiments on the deployment of M. arenaria-resistant cultivars of peanut (TX,FL,NC), M. incognita-resistant and/or tolerant cultivars of cotton (AL,AR,GA,MS, NC,TX), SCN-resistant soybean (AR,IL,MN,NC, ARS-TN), G. tabacum-resistant tobacco (VA) and M. incognita-resistant bell pepper (GA,ARS-SC) will be conducted to optimize rotation systems for growers. Benchmarks for this aspect of the project will be the reduction in nematicide usage in these crops and the use of cultivars with different sources of resistance or cultivars with multiple, stacked resistance genes. Rotating resistant cultivars with other host and nonhost crops will reduce selection pressure on the nematode population, thus increasing the durability of the resistance. Even with highly resistant peanut cultivars, crop rotation will be recommended for management of stem rot caused by Sclerotium rolfsii, Rhizoctonia limb rot, and Cylindrocladium black rot. The effects of shorter rotations systems based on nematode-resistant cultivars will be evaluated in fields also infested with one or more of these fungal pathogens using established experimental designs. Some of the cotton and peanut cultivars that will be released in the next 8 years will likely have only moderate resistance to root-knot nematodes. Additional nematode management tactics may be needed to further reduce yield losses. Research has shown that yield of moderately-resistant cotton is increased with nematicide application in some cases (Colyer et al., 1999). The fumigant metam sodium, widely used to suppress Cylindrocladium black rot also has nematicidal activity. A field study has been initiated to determine the added benefits of metam sodium to nematode suppression and yield when a resistant, moderately resistant, and susceptible peanut are grown. (ARS-GA) Pearl millet has potential as a grain crop in the southeast because it tolerates drought and low fertility conditions, thus requiring less irrigation and fertilizer inputs, and is not susceptible to pre-harvest aflatoxin contamination. Several hybrids resistant to M. incognita and M. arenaria have been identified, one of which will be released as a cultivar in 2002 (Hanna, pers comm). Reproduction of M. incognita on resistant millet was considerably less than on corn, whereas reproduction of M. arenaria was low on both corn and the resistant millet. Thus, millet hybrids are predicted to be comparable to corn in peanut cropping systems for managing root-knot nematodes and superior to corn in cotton cropping systems. Field studies have been initiated to test this hypothesis. (ARS-GA) Biological antagonists of plant-parasitic nematodes will be evaluated, the impact of rotation and cultural practices on these nematode antagonists, and the genetic makeup of selected organisms will be determined. Also, materials that stimulate plant resistant mechanisms such as the Harpin protein and the commerical product Actiguard will be evaluated for their impacts on nematodes. Specific projects include the impact of Hirsutella spp., and other parasitic fungi and bacteria on the soybean cyst nematode (AR,MN), evaluation of fungi for management of root-knot nematodes in peanut and vegetable crops (GA,FL,SC), the effects of biologically derived pesticides on the reniform nematode in cotton and vegetable cropping systems (AL,MS), and characterization of the genome of Pasteuria spp. a bacterial parasite of nematodes (NC). The impact of numerous soil amendments on plant-parasitic nematodes will be evaluated in greenhouse and field experiments. Amendments to be evaluated include cover crops on soybean cyst nematode, and reniform nematode in cotton and vegetable crops, (AL,NC,MN,MS). Effects of soil amendments, including municipal waste on soybean cyst nematode and effects of poultry litter on reniform nematode will be conducted in field experiments (AL,GA,NC). The effects of various essential plant oils and plant herbage on reniform and root-knot nematode will be evaluated in greenhouse experiments. The influence of herbicide-resistant cotton on reniform and root-knot nematode in cotton will be evaluated in field and greenhouse experiments. (AL,MS,TN) Additionally, transgenic resistance to soybean cyst nematode will be evaluated in laboratory, greenhouse, and field experiments when it becomes available (IL, MN,NC,TN).Measurement of Progress and Results
Outputs
- Identification of new sources of resistance, release of breeding lines and cultivars with high levels of resistance to SCN, reniform, and root-knot nematodes in several crops, especially cotton, peanut, and soybean. Identification of parastism genes from SCN, functional analysis of these genes, effects of gene disruption of parasitic activities, and development of transgenic soybean with engineered resistance due to disruption of nematode parasitism genes.
- Overall, the output will be development of several marker-assisted selection (MAS) systems that aid the development of nematode-resistant cultivars. This will be especially important to private sector seed companies that typically lack the expertise or resources for phenotypic screening for nematode resistance but are increasingly able to utilize molecular techniques. This is especially true for cotton and soybean
- Improved rotation systems for management of cyst, reniform and root-knot nematodes on cotton, peanut, and soybean including use of host resistance and reduced reliance on toxic nematicides. Also the development of new cropping systems that incorporate recently identified, nematode-resistant pearl millet genotypes. Development and commerical release of more effective biological control agents for management of primarily of cyst and root-knot nematodes. Quantification of the impact of cover crops, and soil amendments on nematode populations and on populations of biological control agents (especially isolates of Hirsutella and Pasteuria ) in cropping systems with cotton, peanut and (or) soybean as the key crop.
Outcomes or Projected Impacts
- Overall, there will be an increase in grower profits due to more economical production of several key crops because of both a reduced reliance on costly nematicides and because resistance provides better protection of genetic yield potential than do other management tactics. There will be a long term increase in environmental and human health due to this reduction in pesticide use.
- Development of these MAS tools will greatly increase the availability of resistance to growers because it will enable private seed companies to introgress nematode resistance into cultivars with the greatest yield potentials and these private companies have the most effective systems for delivering these nematode management systems to growers.
- Improvement in control of plant-parasitic nematodes on cotton, peanut, soybean and other crops, resulting in improved higher yields. Increased profitability and sustainability of various cropping systems due to decreased use of costly nematicides. Because resistance, once developed, can be used at little of no increase in production costs, these technologies can be adopted by all growers, regardless of size of operation. Increased use of host resistance in these several key crops will give growers greater flexibility in crops that can be grown in rotation with the resistant crop as nematode population densities will be reduced by the resistant crop, reducing the hazard potential to subsequent crops. Similarly, improved efficacy of biological control in several cropping systems will enhance the durability of resistance genes by reducing the intensity of selection for nematode genotypes virulent on the resistance genes being deployed.