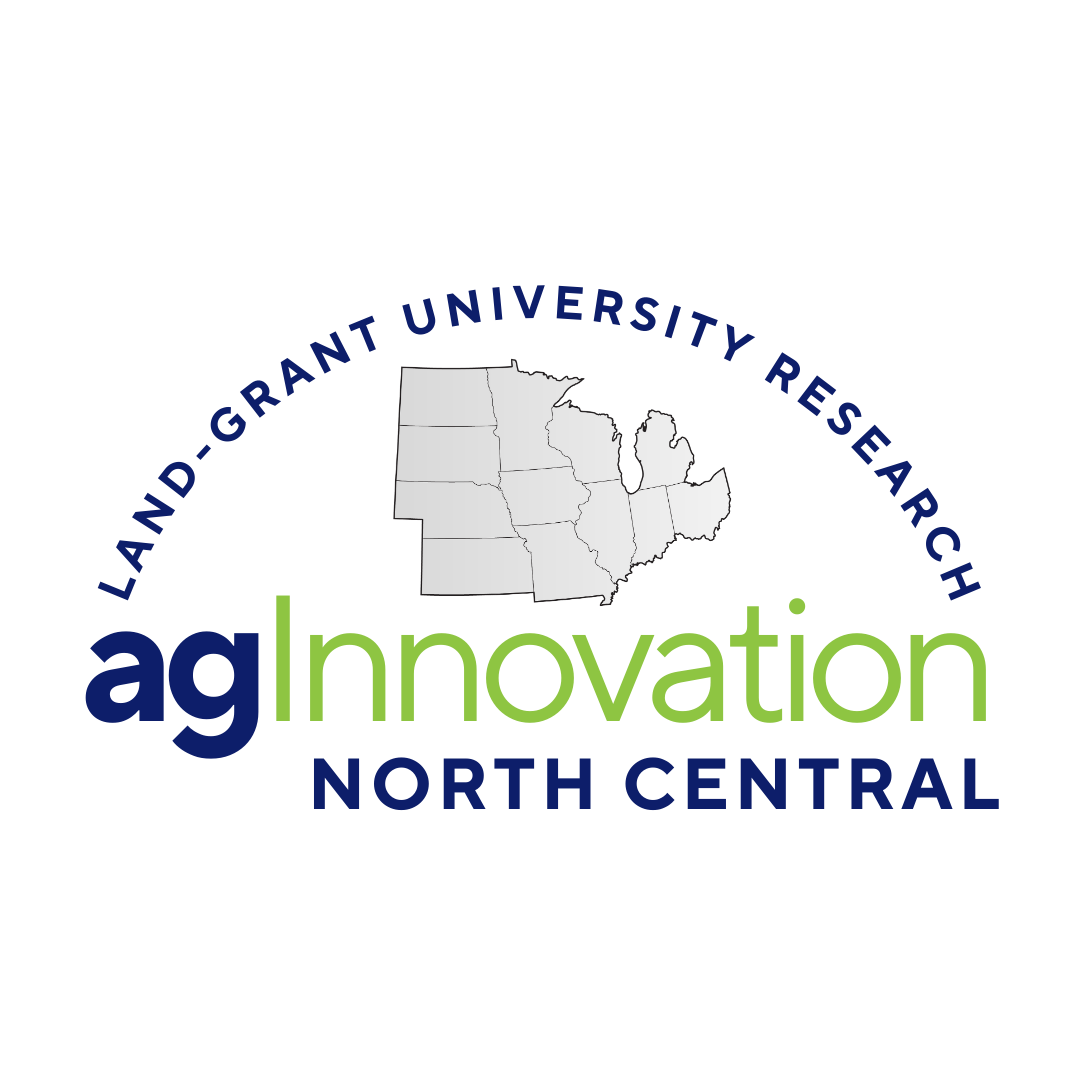
NC1142: Regulation of Photosynthetic Processes (Rev. NC-142)
(Multistate Research Project)
Status: Inactive/Terminating
NC1142: Regulation of Photosynthetic Processes (Rev. NC-142)
Duration: 10/01/2002 to 09/30/2007
Administrative Advisor(s):
NIFA Reps:
Non-Technical Summary
Statement of Issues and Justification
Photosynthesis is the primary determinant of crop productivity. It is the single process on earth that converts sunlight into biomass, sequesters atmospheric CO2 into carbohydrates, and liberates O2. Photosynthesis and the resulting yields of food and fiber products are dramatically limited by environmental constraints in agricultural contexts as reflected by the fact that average yields are typically much less than the maximal yields possible when efficiency and sustainability concerns are relaxed. Whereas agricultural productivity continues to increase slowly, the rate of increase has declined steadily since the 1960's, and it may soon plateau (Mann, 1998). Thus, there is increasing concern that future increases, essential to enhance the competitiveness of U.S. agriculture and to fulfill global demands for food and fiber, will be dependent on the development of new approaches to increase the capacity of crop plants to produce the nutrients that support the growth of harvested plant parts. New approaches will also be required to enhance efficiency and to improve agricultural sustainability. To achieve these goals, it will be essential to gain fundamental knowledge of the underlying metabolic components that control assimilate production and utilization, and hence plant growth and development. This knowledge must include an understanding of the regulation of important photosynthetic enzymes and how environmental and developmental signals affecting photosynthetic processes are perceived at the molecular and gene levels. Such knowledge will provide new opportunities for crop improvement using the techniques of both molecular genetics and classical breeding. The proposed multistate research project brings together some of the most outstanding, productive photosynthesis investigators in the country in an integrated effort to broaden our understanding of critically important areas of photosynthesis research. We propose a synergistic, cooperative research program that concentrates on four areas of photosynthetic regulation: 1. Photochemistry and the biogenesis of the photosynthetic apparatus. The purpose of this research is to understand the structure and function of the light harvesting and electron transfer components of the thylakoid membrane, with emphasis on Photosystem I and the mechanisms that control the stoichiometry between Photosystems I and II. Anterograde and retrograde pathways involved in coordinating the expression of nuclear and chloroplast genes for photosynthetic proteins will be studied, and novel photosynthetic proteins will be identified. The collaborating units include IA-AES, NE-AES and OR-AES (3 institutions, 4 research labs). 2. Photosynthetic capture and photorespiratory release of CO2. The goal of this research is to determine and modify the biochemical and regulatory factors that impact photosynthetic capture and photorespiratory release of CO2. Particular emphasis will be placed on understanding protein-protein interactions and post-translational modifications of key photosynthetic enzymes involved in primary and secondary CO2 assimilation, as well as the mechanisms that control carbon flux through primary and secondary metabolic pathways. The collaborating units include IL-ARS, KY-AES, MO-AES, NE-AES, and NV-AES (5 institutions, 6 research labs). 3. Mechanisms regulating photosynthate partitioning. The objective of this research is to gain insight into the mechanisms that regulate photosynthate partitioning into pathways of biosynthesis and use of sucrose, starch, and sugar alcohols. These studies will examine interactions between compartments of the cell, between plant parts, and the partitioning of carbohydrates between transport, storage, and stress-protective functions. The collaborating units include FL-AES, IA-AES, IL-AES, MI-AES, MN-AES, NC-AES, NE-AES, PA-AES, SC-AES, WA-AES, WI-AES (11 institutions, 13 research labs). 4. Developmental and environmental limitations to photosynthesis. The aim of this research is to analyze the limitations and environmental factors that influence photosynthetic productivity at the whole plant and canopy levels. Particular emphasis will be placed on abiotic stresses (temperature, water, and salinity), nitrogen use, and global atmospheric change. This work will integrate understanding developed here and under objectives 1 through 3 to optimize photosynthetic production and yield under current and future environmental conditions. The collaborating units include Guam-AES, IA-AES, IL-AES, KS-AES, MI-AES, MS-ARS, NE-AES, NV-AES, OR-AES, WA-AES (10 institutions, 14 research labs).
Related, Current and Previous Work
Objective 1: Photochemistry and the Biogenesis of the Photosynthetic Apparatus Radiant energy is harvested by pigment arrays on thylakoid membranes and stabilized as NADPH and ATP by the coupling of electron transport with ATP biosynthesis. These processes are carried out by membrane complexes that include photosystem I (PSI), photosystem II (PSII), the cytochrome b6/f complex and the ATP synthase. Much progress has been made toward understanding the architecture and function of these complexes, and NC-1142 contributions to this effort have been notable, particularly in the area of PSI (Chitnis, 2001). Another important area of investigation concerns the regulatory networks that modulate electron transport processes in response to varying supplies of the substrate (light), or in response to changes in the metabolic demands for the products (ATP and NADPH). As a prominent example, plants and other photosynthetic organisms, including cyanobacteria, achieve proper electron flow rates by regulating the ratio of the two photosystems. The Chitnis (IA-AES) and Rodermel (IA-AES) labs have generated mutants of Arabidopsis and cyanobacteria with altered PSI/PSII stoichiometries (Chen et al., 2000), and characterization of these mutants should lend insight into the responsible regulatory mechanisms. The biogenesis of the photosynthetic apparatus requires the concerted action of nuclear and chloroplast genes (Rodermel, 2001). Only about 100 of the estimated 2,500 chloroplast proteins are coded for by genes in the chloroplast genome; the rest are coded for by nuclear genes (Martin and Herrmann, 1998). Although many of the best-characterized biochemical pathways in plants take place in chloroplasts (e.g., photosynthetic carbon assimilation, carotenoid and chlorophyll biosynthesis), the function of most chloroplast proteins is not known. Chitnis and Rodermel have adopted a proteomics approach to identify novel thylakoid membrane proteins. Of ~150 proteins analyzed in a pilot study, 24% were derived from unidentified open reading frames. A holistic 'systems biology' approach involving mutational analysis and techniques of functional genomics will allow Chitnis and Rodermel to characterize the role of these proteins in photosynthesis. Much of the regulatory traffic that coordinates gene expression in the chloroplast and nucleus is anterograde, i.e., from the nucleus to the plastid, in the form of nuclear gene products that control the transcription and translation of plastid genes (Rodermel, 2001). Among these products are sigma factors that regulate the activity of the plastid encoded RNA polymerase (PEP) (Allison, 2000). The Allison lab (NE-AES) has shown that two plastid-localized sigma factors are expressed differentially during the process of chloroplast biogenesis in maize (Lahiri and Allison, 2000). This is consistent with the idea that sigma factor families act as global regulators of plastid gene transcription. In addition to anterograde traffic, retrograde traffic occurs between the plastid and nucleus in the form of "plastid signals" that regulate the transcription of nuclear genes for photosynthetic proteins (Rodermel, 2001). The Rodermel group (IA-AES) has used the immutans and var2 variegation mutants of Arabidopsis as models to understand these retrograde mechanisms. These mutants have green sectors with morphologically normal chloroplasts and white sectors with abnormal (non-pigmented) plastids that are blocked in the process of chloroplast biogenesis. Photosynthetic rates are markedly higher than normal in the green sectors of the mutants, and leaf morphogenesis is impaired in both, indicating a disruption in retrograde signaling (Aluru et al., 2001). Positional-cloning has revealed that IMMUTANS is a novel terminal oxidase that resides in the thylakoid membrane (Wu et al., 2000), and that VAR2 is a thylakoid membrane FtsH metalloprotease (Chen et al., 2000). Functional analyses of the IMMUTANS and VAR2 proteins by the Rodermel (IA-AES), Chitnis (IA-AES) and Daley (OR-AES) groups should lend insight into mechanisms of retrograde signaling, as well as provide information about factors that control photosynthetic rates. Objective 2: Photosynthetic Capture and Photorespiratory Release of CO2 Ribulose-1,5-bisphosphate carboxylase/oxygenase (Rubisco) initiates the major pathway of CO2 capture from the atmosphere by catalyzing the reaction between ribulose 1,5-bisphosphate (RuBP) and CO2 to produce two molecules of phosphoglycerate (Spreitzer, 1999). However, atmospheric O2 competes with CO2, and oxygenation of RuBP initiates the nonessential photorespiratory pathway that leads to the loss of CO2. Thus, if carboxylation could be increased or oxygenation decreased, a significant increase in crop plant productivity might be realized. Rubisco is comprised of eight 55-kD large subunits coded for by a single rbcL gene on the multicopy chloroplast DNA, and of eight 16-kD small subunits coded for by a family of RbcS genes in the nucleus. Because rbcL and RbcS are present in multiple copies per cell, it will be difficult to eliminate or transform engineered copies of these genes in crop plants. Despite these limitations, natural variation exists in the kinetic and regulatory properties of Rubisco from different species (Spreitzer, 1999), and thus it is possible that with advances in technology an improved higher plant enzyme might be created. For example, methods have been developed for engineering the small subunit by the Spreitzer group (NE-AES) (Spreitzer et al., 2001), and progress has been made with nuclear and chloroplast transformation of the green alga Chlamydomonas reinhardtii and of land plants by the Spreitzer groups and Allison (NE-AES) labs (Spreitzer, 1998; Daniell et al., 2002). These transformation technologies should provide new opportunities to study structure/function relationships of Rubisco. Many Rubisco X-ray crystal structures have now been solved that might also aid genetic engineering strategies (Taylor et al., 2002). Modifications in the regulation of Rubisco might result in improved plant productivity, and models have been constructed that predict patterns of CO2 uptake by the Long (IL-AES) and Portis (IL-ARS) groups (Bernacchi et al., 2001). Rubisco activase enhances the activity of Rubisco by removing inhibitory sugar phosphates from the active site, and the Portis (IL-ARS) and Spretizer (NE-AES) groups have identified the site of interaction between activase and Rubisco (reviewed by Portis, 2001). The creation of transgenic plants with altered forms of Rubisco activase by the Portis group has shown that the amount and redox control of activase are important for growth (Zhang et al., 2001). However, increased knowledge of how activation varies in different plants is necessary for ultimately improving the regulatory mechanism. As another means of Rubisco regulation, enzymes involved in Rubisco modification have been characterized by the Houtz group (KY-AES) (Ying et al., 1999; Dirk et al., 2001); altered modification enzymes might prove to be appealing targets for genetic modification of crop plants if they result in more efficient carboxylation. In C4 and Crassulacean (CAM) plants, phosphoenolpyruvate carboxylase (PEPC) catalyzes the initial fixation of atmospheric CO2 into C4-decarboxylic acids. Because this pathway concentrates CO2 in the vicinity of Rubisco, PEPC and its regulation are attractive targets for the genetic engineering of improvements in CO2 fixation. The Chollet (NE-AES) and Cushman (NV-AES) groups have shown that PEPC activity is tightly controlled by metabolites, as well as by reversible phosphorylation via PEPC kinase (PPcK) and protein phosphatase 2A (Vidal and Chollet, 1997; Taybi and Cushman, 1999). Molecular cloning of PPcK genes has allowed biochemical studies of the recombinant kinase and the development of immunological reagents for tracking its expression in various plant tissues. This work has increased our understanding of the regulation of PEPC in C/N partitioning and N2 fixation in legume nodules (Bakrim et al., 2001; Taybi et al., 2000). The Chollet group (NE-AES) has found that the chloroplast enzyme pyruvate orthophosphate dikinase (PPDK) is also controlled by phosphorylation in C4 and CAM photosynthesis (Chastain et al., 2000). The mitochondrial pyruvate dehydrogenase complex (mtPDC) controls carbon flow from glycolysis to the Krebs cycle by catalyzing the oxidative decarboxylation of pyruvate to acetyl-CoA. The Randall group (MO-AES) has found that inhibition of the mtPDC by reversible phosphorylation might reduce CO2 release from respiration (Leuthy et al., 2001). Because the mitochondrial and chloroplast forms of PDC are involved in the generation of fatty acids (oil), further analysis of the mechanism of mtPDC phosphorylation might lead to the genetic engineering of value-added crops. Objective 3: Mechanisms Regulating Photosynthate Partitioning Critical steps in the conversion of photosynthate to harvested materials can affect the photosynthetic process itself, as well as the amount of fixed carbohydrates allocated to yield. Key points in this regulation offer exciting avenues for manipulation; however, underlying mechanisms remain unclear. Feasible long-term targets for altered partitioning begin at the metabolic level (photosynthetic end-products) and extend to the whole plant level (importing plant parts). Recent work from NC-142 investigators and elsewhere indicate three especially promising avenues for regulation of photosynthate partitioning: Sucrose biosynthesis and metabolism are key components of long-distance transport of photosynthates in vascular plants, and the extent of sucrose formation, metabolism, and sites of its conversion can markedly affect exporting and importing organs at many levels. Sucrose is typically synthesized in leaves via SPS (sucrose-P synthase) and cleaved at sites of import by either SuSy (sucrose synthase, a reversible reaction) or one of the invertases (in cell walls or vacuoles). Products of the invertase and SuSy paths differ (hexoses vs. fructose + UDPG), and so too does their potential to generate sugar signals. The Koch lab (FL-AES) has shown that these signals can repress genes for photosynthesis, and also affect invertase and SuSy genes themselves (Koch, 1996; Xu et al., 1996; Koch et al., 2000). In addition, sugar signals sensed through hexokinase can potentially be amplified by repeated cycles of sucrose cleavage and re-synthesis (Moore and Sheen, 1999; Rolland et al., 2001). Invertase action and the resulting signals are typically associated with growth, expansion and cell division, whereas SuSy is more often linked with biosynthesis of cell walls and storage materials (Sturm and Tang, 1999; Koch et al., 2000). SuSy also has broadly pleiotropic effects that include tuber yield and starch content in potato (Zrenner et al, 1995); flood tolerance in maize (Ricard et al., 1998; Zeng et al., 1998, 1999); fruit set under specific conditions in tomato (D!&Aoust et al., 1999); and a critical, drought-sensitive step in N-fixing nodules of legumes (Arrese-Igor et al., 1999; Zhang et al., 1999); the Koch (FL-AES) and Chollet (NE-AES) groups have played seminal roles in these discoveries (Zeng et al., 1998, 1999; Zhang et al., 1999). Invertase and SuSy regulation extends from transcriptional to post-transcriptional mechanisms. The Koch group (FL-AES) has found that transcription of genes for both enzymes responds strongly to sugars, oxygen status and other abiotic factors, and that both are also regulated at the level of mRNA stability (Koch, 1996; Xu et al, 1996; Zeng et al., 1999; Koch et al., 2000). The Huber (NC-AES) and Chollet (NE-AES) groups have found that SuSy proteins from both maize and soybean nodules can be phosphorylated at multiple sites; CDPKs and/or SnRKs have been implicated in these phosphorylation events (Huber et al., 1996; Zhang and Chollet, 1997; Nakai et al., 1998; Anguenot et al., 1999; Zhang et al., 1999). Starch synthesis and degradation occur in chloroplasts of leaves and amyloplasts of non-green starch storage tissues (e.g., seeds and tubers). The extent of starch formation and use can be linked to both export and import of photosynthates by different plant parts. Starch is typically a composite of both amylose (unbranched a-l,4 glucan) and amylopectin (a-l,4 and a-1,6 branched glucan). Its synthesis requires three enzymes: AGP (ADP-glucose pyrophosphorylase), starch synthase, and BE (branching enzyme). In addition, a de-branching enzyme (Sugary-1 in maize) appears to be necessary for starch granule formation. AGP is an important regulatory enzyme and in many tissues it is activated by 3-P-glycerate and inhibited by inorganic phosphate. The Okita (WA-AES), Edwards (WA-AES) and Preiss (MI-AES) groups have analyzed the catalytic and regulatory sites of AGPs (Binderup et al., 2000; Frueauf et al., 2001; Sikka et al., 2001; Kavakli et al., 2001a; 2001b), and the Okita lab has found that starch content can be manipulated by altering the properties of AGP in transgenic plants (Slattery et al., 2000). The Preiss (MI-AES) and Guiltinen (PA-AES) labs have shown that starch quality depends on the properties of starch synthase and branching enzyme (Binderup et al., 2001; Blauth et al., 2001a, 2001b; Hong et al., 2001). Although little is known about the regulation of starch degradation, two amylolytic enzymes have been identified in chloroplasts of pea and Arabidopsis: one is an a-glucosidase and another is a Ca2+-independent a-amylase. The Duke group (WI-AES) has found that the latter enzyme might facilitate carbon export at night. A further understanding of the enzymes involved in starch metabolism might lead to strategies to manipulate starch quality or utilization. Partitioning of photosynthates to sugar alcohols is a central feature of several plant species that shunt recently fixed C into acyclic polyols such as mannitol and sorbitol, or cyclitols such as ononitol. In addition to their transport and storage roles, the Bohnert (IL-AES) and Loescher (MI-AES) groups have shown that these assimilates might function as osmoprotectants for plants experiencing salt and water stress (Shen et al., 1997; Sheveleva et al., 1997; Gao and Loescher, 2000; Loescher and Everard, 2000). Stress-susceptible model or crop plants have been transformed with genes involved in the synthesis of these protectants by the Bohnert and Loescher labs, and while stress resistance is often observed, more detailed studies of the mechanisms are required. Objective 4: Developmental and Environmental Limitations to Photosynthesis Factors that enhance or limit agricultural productivity do so by impacting photosynthesis. Gains in yield of the major crops over the past half-century have been predominantly through improved harvest index (HI). HI has approached the maximum achievable for many crops, and future gains will depend on increasing total production. This, in turn, will depend on improving the efficiency of crop canopy light interception and utilization. It will therefore be important to maximize these efficiencies under optimal and stress conditions, consonant with global atmospheric change. NC-1142 collaborators have been active in a number of investigations that have explored developmental and environmental limitations to photosynthesis. These include nitrogen use, water use, stress (temperature, salt, drought, CO2), and developmental factors associated with canopy architecture and leaf ontogeny. Nitrogen acquisition and use is key to increasing actual efficiencies of light interception and use; improvements in nitrogen use efficiency (NUE) will also reduce the input of fertilizer needed for high yields. The Below group (IL-AES) has been studying the Illinois Protein Strains of maize --a germplasm with a wide range of NUEs - Xand found that little genetic variation exits for NUE in corn, probably because current hybrids have been selected under high N (Gentry et al., 2001). The Markwell group (NE-AES) has found that one-carbon metabolism plays a role in water-stress; they have focused on formate dehydrogenase as a means of increasing water use efficiency at the leaf level (Olson et al., 2000). Stresses represent major limitations to photosynthesis and yield. The Jones group (MN-AES) has shown that maize kernel development is sensitive to heat stress during the first six days following pollination and is associated with decreased endogenous cytokinin levels (Commuri and Jones, 2001). Heat stress also disrupts amyloplast initiation, starch granule development, and storage protein levels. The Bohnert (IL-AES) and Cushman (NV-AES) groups have pioneered the use of microarrays, gene mining, and mutant analyses to investigate the mechanisms of drought and salt stress and the role of osmoprotectants in these processes (Cushman and Bohnert, 2000; Hasegawa et al., 2000). Field studies of the effects of global change have scaled from photosynthetic processes to yield in two agricultural systems: increased N-deposition and drought in rangeland by the Knapp group (KS-AES) (Silletti and Knapp, 2001) and increased CO2 in soybean by the Long lab (IL-AES) (Hymus et al., 2001). Both showed changes in production anticipated from prior laboratory studies, but the mechanisms differed from expectations, emphasizing the importance of interaction between field and laboratory. For soybean, significantly increased canopy duration was a major factor, whereas for rangeland an increased contribution from the C4 photosynthetic component was a key factor. Developmental factors also represent a major limitation to photosynthesis. For example, a model for light distribution within row-planted cotton canopies, based on the existing formulations for radiation transfer, has been integrated into a geometric model of the cotton canopy by the Sassenrath-Cole lab (MS-ARS). This model revealed that changes in leaf structure can enhance photosynthetic production. The Daley (OR-AES), Rodermel (IA-AES) and Edwards (WA-AES) groups have examined discontinuities in photosynthetic efficiency via fluorometry and found that these correlated with germplasm observations of dividing chloroplasts (Zheng et al., 2001a, 2001b). In addition it has been found that negative effects on photosynthesis attributed to environmental stresses are not only the direct result of decreases in photosynthesis but also a consequence of changes in plant development, e.g., that occur during the progression of leaf ontogeny (Rodermel, 1999).
Objectives
-
To examine the dynamic regulation of radiant energy capture and utilization in photosynthesis, and to study the architecture, function and biogenesis of the photosynthetic apparatus.
-
To determine and modify the biochemical and regulatory factors that impact the photosynthetic capture and photorespiratory release of CO2.
-
To understand the mechanisms that regulate photosynthate partitioning into paths for biosynthesis and use of sucrose, starch, and sugar alcohols.
-
To analyze the limitations and environmental factors that influence photosynthetic productivity at the whole plant and canopy levels.
-
Methods
Objective 1: Photochemistry and the Biogenesis of the Photosynthetic ApparatusThe Chitnis and Rodermel groups (IA-AES) will collaborate to develop tools of functional genomics analysis to define the components of the thylakoid membrane, how these membranes are synthesized, and how they function. In particular, they will identify proteins of unknown function using proteomics analysis of cyanobacterial and plant thylakoid membranes. Knockout mutants of cyanobacteria and Arabidopsis will be isolated that contain insertions in genes for the unknown proteins; the Chitnis, Rodermel and Daley (OR-AES) groups will integrate their efforts to characterize these mutants as a first step to examine protein function. The three groups will also collaborate to examine other mutants of cyanobacteria and Arabidopsis that are perturbed in thylakoid membrane biogenesis and function (such as immutans and var2 of Arabidopsis). These mutants should provide insight into retrograde signaling pathways, as well as into mechanisms that govern PSI and PSII stoichiometry. The mutant studies of Chitnis, Rodermel and Daley will set the stage for collaborations with other members of the NC-142 group, e.g., to examine how lesions in thylakoid membrane function affect downstream photosynthetic events, such as partitioning of photosynthate into sucrose versus starch (Objetctive 3). Allison (NE-AES) will develop tools of chloroplast transformation that will form the basis for future NC-142 collaborations (especially with members whose primary area of expertise is Objective 2 or 3, see below). She will also study the role of sigma factors in chloroplast biogenesis by examining the consequences of sigma factor gene disruption in insertion mutants of maize and Arabidopsis. The mutants will be analyzed by functional genomics approaches, including microarray analyses. Objective 2. Photosynthetic Capture and Photorespiratory Release of CO2
By exploiting methods of chloroplast and nuclear transformation in Chlamydomonas, the Spretizer lab (NE-AES) will investigate the importance of large and small subunit residues that differ relative to those conserved in crop plant Rubisco enzymes. The Houtz group (KY-AES) will continue to define the biochemical significance of post-translational Rubisco methylations and hydroxylations and will exploit mutant Chlamydomonas enzymes that have been engineered by the Spreitzer group (NE-AES) to promote or block such modifications. By using tobacco chloroplast transformation performed in collaboration with the Allison group (NE-AES), the KY-AES will also create amino acid substitutions to introduce post-translationally modified residues into crop plants. Knowledge of Rubisco posttranslational modifications will be important in efforts by the Portis group (IL-ARS) to replace the endogenous Rubisco in tobacco and soybeans with the Chlamydomonas enzyme (via genes provided by the NE-AES), which has more favorable kinetic properties at high CO2. The Portis and Spreitzer labs will continue to collaborate on determining the structural basis for the interaction between Rubisco and Rubisco activase using Chlamydomonas chloroplast transformation. A large subunit loop that is known to influence this interaction will be replaced with the loop from crop plants. The Portis lab will also continue studies of the impact of transforming the rca mutant of Arabidopsis activase with mutant proteins that have altered catalytic and regulatory properties. The Chollet (NE-AES) and Cushman (NV-AES) groups will continue to coordinate their biochemical, molecular, and physiological investigations into the functional contribution and metabolic regulation of PEPC, PPcK, and PPDK. Because this research has direct relevance to plant regulatory protein phosphorylation in general, collaborations with the Randall (MO-AES), and Huber (NC-ARS) groups will accelerate progress. The Chollet group will characterize the bifunctional regulatory protein involved in the (de)phosphorylation of PPDK, and begin to investigate divergent forms of PEPC as a means of gaining a deeper understanding of enzyme regulation. The Chollet and Cushman groups will coordinate and expand their investigations of PPcK in C3, C4, and CAM plants by molecular and biochemical characterization of other isozymes. Investigations into the regulation and substrate specificity of PPcK and closely-related calcium-dependent protein kinases (CDPKs) will be conducted in collaboration with the Huber group (NC-AES). The Randall group (MO-AES) will define the biochemical and regulatory properties of the protein kinase that regulates the mitochondrial PDC and coordinates photosynthetic and photorespiratory metabolism in mitochondria. The primary mechanism is reversible phosphorylation, similar to that controlling PEPC, SuSy, SPS and NR. Thus, Randall interacts and exchanges information with the Chollet, Huber and Cushman groups through efforts to understand and characterize plant protein kinases. Knockouts of kinases and phosphatases in the various systems should reveal any overlapping properties of these regulatory enzymes and their effects on photosynthesis and enhanced storage-oil synthesis. Objective 3: Mechanisms Regulating Photosynthate Partitioning
The steps involved in C-flow into and out of sucrose, starch, and sugar alcohols represent key points of control for photosynthate partitioning at the metabolic and whole plant levels. The proposed research will define mechanisms of regulation for each of these processes, and how they can be manipulated to increase productivity and yield. Regulation of sucrose biosynthesis and metabolism will be approached in several ways. Regulation can be modulated in both exporting and importing tissues: for example, at points of sucrose formation via SPS (sucrose-P synthase) or cleavage by SuSy (sucrose synthase) or invertases (vacuolar and cell wall forms). To gain insight into mechanisms of sugar sensing, the Rodermel (IA-AES) and Moore (SC-AES) groups will collaborate in using mutant and transgenic Arabidopsis with altered soluble invertase activities or with altered hexose sensing capacities. Both approaches should decrease hexose-based sugar signals. The impact of these alterations will be assessed by examining photosynthesis, plant development, diurnal carbohydrate profiles, and activities of sucrose-cycling enzymes, and by conducting isotope assays of sucrose cycling. Invertase regulation and its role in development will be further examined using transgenic maize in collaborative research by the Koch (FL-AES), Jones (MN-AES) and Huber (NC-AES) groups. In these experiments (also performed in collaboration with Pioneer Hi-Bred), several types of transgenic lines will be generated: a) invertase promoter-GUS constructs for analysis of transcriptional control (FL-AES, NC-AES); b) lines that over-express soluble invertase in specific tissues to examine the developmental role of invertase (FL-AES); and c) lines in which cytokinin metabolism is altered to assess its impact in young kernels (MN-AES). A final regulatory aspect of sucrose metabolism that will be investigated is SuSy phosphorylation in coordinated research by the Koch (FL-AES), Chollet (NB-AES) and Huber (NC-AES) groups. Stable and transient expression approaches will be used to test functionality, localization, and substrate characteristics of non-phosphorylatable SuSy enzymes. Both maize and soybean will be utilized (vegetative and nodule tissues), together with antibodies to specific sites of SuSy phosphorylation (developed by the NC- and NB-AESs). Of particular interest will be the identification of the effector kinase (a CDPK or SnRK). Regulation of starch biosynthesis and degradation will include a major focus on AGP due to its central role in starch formation. One of the approaches will be to alter the regulatory properties of AGP by modifying the binding sites for 3-PGA activation or Pi inhibition. Ongoing work by the Preiss (MI-AES), Edwards (WA-AES) and Okita (WA-AES) groups indicates that inhibition of AGP by Pi might involve both N- and C-terminal domains of the protein; mutant proteins are being constructed to test this hypothesis. If these enzymes are less sensitive to Pi inhibition, or require less activator, they could be valuable resources for increasing starch and crop yields. The Preiss, Edwards and Okita groups will coordinate their examinations of mutant AGP proteins for altered binding of the 3-PGA activator, and will evaluate and compare the physiological effects of the mutant enzymes in transgenic plants of Arabidopsis (WA-AES), rice (WA-AES), and potato (MI-AES). In these studies, rice will serve as a model system, and mutant AGPs will be targeted to leaves, or to one of two compartments in cells of the seed endosperm (cytosol or amyloplasts). This approach will allow an independent assessment of the potential for starch storage in exporting versus importing tissues. CAM mutants of Mesembryanthemum crystallinum defective in starch synthesis, which have been isolated by the Cushman group (NV-AES), also will be characterized by Preiss (MI-AES) for defects in the three starch biosynthetic enzymes. Another focus of research in this objective will be the molecular mechanisms regulating the activity of BE (branching enzyme). The Preiss group will utilize PCR random mutagenesis to alter BE structure and properties, whereas the Edwards and Okita groups will use Mu insertional mutants to study effects of altered BE enzymes in vivo and in vitro. The Duke group (WI-AES) will study the regulation of starch degradation by generating Arabidopsis mutants with defects in the chloroplast a-glucosidase and Ca2+-independent a-amylase; the mutants will be examined using procedures similar to those discussed above for the AGP mutants. The role of polyol sugars in imparting protection from drought or salt stress will be studied in coordinated efforts by the Bohnert (IL-AES) and Loescher (MI-AES) groups using stable transgenic model and crop plants. Photosynthetic characteristics of transformed tobacco and Arabidopsis plants producing mannitol, sorbitol, or ononitol will be assessed after salt stress. The importance of having cytosolic or chloroplastic polyols will be examined further. These experiments will define the protective characteristics of each polyol and the preferred compartment for its synthesis and accumulation, thereby providing insights into the use of polyols for engineering stress tolerance (see Objective 4). Recent progress in regeneration and transformation of celery (a mannitol syntheziser) and cherry (a sorbitol synthesizer) by the Loescher group (MI-AES) will allow study of genetically modified polyol biosynthesis. Objective 4: Developmental and Environmental Limitations to Photosynthesis
NC-142 collaborators will focus on the impact of six major developmental and environmental limitations to photosynthesis:
- Nitrogen use. Physiological components of NUE will be determined by the Below group (IL-AES) in field trials of different genotype x N rate treatments using resources of the Illinois Protein Strains of maize. In addition to physiological studies, the plant samples will be assayed for changes in the expression of genes known to be involved in nitrogen metabolism and storage product deposition. The NE-AES (Markwell group) will determine whether the expression of uricase, which is essential for mobilization of fixed N2, might be used as a QTL marker in different soybean breeding lines.
- Temperature stress. The Knapp group (IA-AES) will study the effects of low temperature stress on photosynthesis and yield using a range of maize genotypes that differ in their tolerance to low temperature. The transition of seedlings from heterotrophic to autotrophic growth will be characterized and genotypes screened for photosynthetic competence. The Knapp (IA-AES) and Jones (MN-AES) groups will compare tolerance to low and high temperature stresses and will provide tissue material to the Chitnis group (IA-AES) for characterization of the proteomes of the stressed plants in order to identify proteins that might be targeted for genetic manipulation.
- CO2 and ozone stress. Field studies of the impacts of global change on photosynthetic production will be expanded by the Long (IL-AES) and Knapp (KS-AES) groups. Tropospheric ozone is thought to account for $6 billion of crop losses in the US, and its continued rate of increase might eliminate any increase in production due to rising CO2. The new Free-Air Concentration Enrichment experiment (SoyFACE) will add ozone (1.5x ambient) to its elevated CO2 treatment (1.5x ambient). This will be the first field system worldwide that simultaneously treats crops in the open air with both ozone and CO2 elevated to predicted future levels. This is a resource that will be used by other NC-142 participants. For example, the Rodermel group (IA-AES) will use proteomics to characterize changes in expressed polypeptides and the Portis group (IL-USDA) will specifically examine the levels of Rubisco and activase in samples obtained from the SoyFACE facility. The KS-AES (Knapp) will conduct parallel studies of global change effects on rangeland, focusing on increased N-deposition and drought incidence.
- Photosynthetic limitations in canopies. Cotton will be used as a model system. Monitoring crop performance on the broad scale required of production agriculture is challenging, tedious, and error-prone. Within-field spatial variability affects canopy microclimate, crop growth, cotton fiber development, and final fiber quality and yield, and can cause significant reductions in the net return to farmers. The Sassenrath-Cole group (MS-ARS) will explore edaphic x microclimate effects on cotton production systems. Photosynthetic limitations in canopies will be assessed via geometric models of canopy structure, radiation transfer, and microclimate. This information will benefit efforts by the Long group (IL-AES) to develop remote sensing techniques at the Soyface facility, and will also contribute to the work in Obectives 1 and 2.
- Salt and drought stress response. The role of signal transduction in stress sensing and tolerance responses will be studied by the Bohnert group (IL-AES). Transcriptional and post-translational responses in gene regulation to varied salt concentrations will be determined. Collaborative studies with the Cushman group (NV-AES) will elucidate the physiological aspects of salt and drought tolerance in whole plants, and both groups will coordinate mechanistic studies that involve genome-wide transcript expression profiles (microarrays), gene mining, and mutant analyses (see Cushman and Bohnert, 2000).
- Water-use efficiency. The Markwell group (NE-AES) will examine whether altering one-carbon metabolism by overexpressing formate dehydrogenase in transgenic tobacco alters water-use efficiency. Plants with 25 to 50-fold elevated levels of the enzyme will be grown under water-sufficient and !Vdeficient conditions and WUE will be assess by 13C-isotope ratio mass spectrometry.
Measurement of Progress and Results
Outputs
- <B>Objective 1:</B><UL> <LI>The features of quinones and of quinone-binding sites that are essential for their function as redox centers in PSI will be determined (IA-AES). <LI>Mechanisms that control PSI and PSII stoichiometry will be identified (IA-AES, OR-AES) <LI>A map of the thylakoid proteome will be expanded and novel proteins characterized (IA-AES) <LI>Plastid transcripts that are expressed in different tissues and under different growth conditions will be identified (NE-AES). <LI>Components of plastid-to-nucleus signal transduction pathways and of thylakoid membrane biogenesis will be clarified (IA-AES, OR-AES).<P></UL> <B>Objective 2:</b><UL> <LI>The role of the Rubisco small subunit in determining Rubisco catalytic efficiency will be elucidated (NE-AES). <LI>Enzymes, mechanisms, and the significance of Rubisco post-translational modifications will be defined with respect to Rubisco synthesis, structure, and function (KY-AES, NE-AES). <LI>Structural interactions between Rubisco and Rubisco activase will be established with respect to both proteins (IL-ARS, NE-AES). <LI>Mutant forms of Rubisco activase will be created in vivo for physiological studies (IL-ARS). <LI>Signal transduction pathways for the regulatory phosphorylation of enzymes involved in C4 and CAM metabolism will be elucidated (NE-AES, NV-AES, NC-AES). <LI>The function of PPcK will be examined in transgenic C3/C4 and CAM model species (NV-AES, NE-AES). <LI>A collection of CAM-deficient mutants will be created (NV-AES). <LI>The function of the mtPDC and its associated regulatory proteins will be examined in transgenic C3 model species (MO-AES, NE-AES, NV-AES, NC-AES). <LI>Knockout mutants of Arabidopsis will be isolated and evaluated for increased carbohydrate and oil composition (MO-AES).</ul><P> <B>Objective 3:</B><ul><LI>Transgenic Arabidopsis with altered soluble invertase activity will be generated (IA-AES). <LI>Transgenic maize with altered genes for invertases (FL-AES), cytokinin oxidase (MN-AES), and sucrose synthase will be generated (Fl-AES, NC-AES). <LI>Antibodies to specific forms of phosphorylated forms of sucrose synthase from maize (NC-AES) and soybean will be produced (NB-AES). <LI>Transgenic Arabidopsis and rice plants with altered AGP and starch storage capacity in leaves and seeds will be generated (WA-AES). <LI>Maize mutants will be created with altered genes for BE and starch quality (PA-AES). <LI>Modified genes for AGP with regulatory properties that facilitate greater starch production will be produced (MI-AES, WA-AES). <LI>Modified genes for BE that allow analysis of its regulation and control of starch quality will be generated (MI-AES, PA-AES). <LI>Structural information will be produced on genes for starch biosynthesis that allows further regulatory control. <LI>Polyol transporters for sorbitol and mannitol will be identified.</ul><P> <b>Objective 4: </b><ul><LI>Improved models for optimizing light distribution in cotton canopies will be defined (MS-ARS). <LI>The role of endogenous cytokinin production in temperature response of early kernel filling in corn will be established (MN-AES). <LI>Genes or pathways will be discovered that are associated with or that potentially regulate NUE, storage product deposition, and salinity tolerance (IL-AES, NV-AES). <LI>Genotypes, including growth forms, will be identified that have increased yields under atmospheric change (IL-AES, KS-AES). <LI>The role of taxol in photosynthesis and plant development will be established (OR-AES). <LI>The significance of uricase in mobilization of fixed N2 in soybean will be established (NE-AES). <LI>The role of C-1 metabolism in photosynthetic efficiency will be elucidated (NE-AES).</ul>