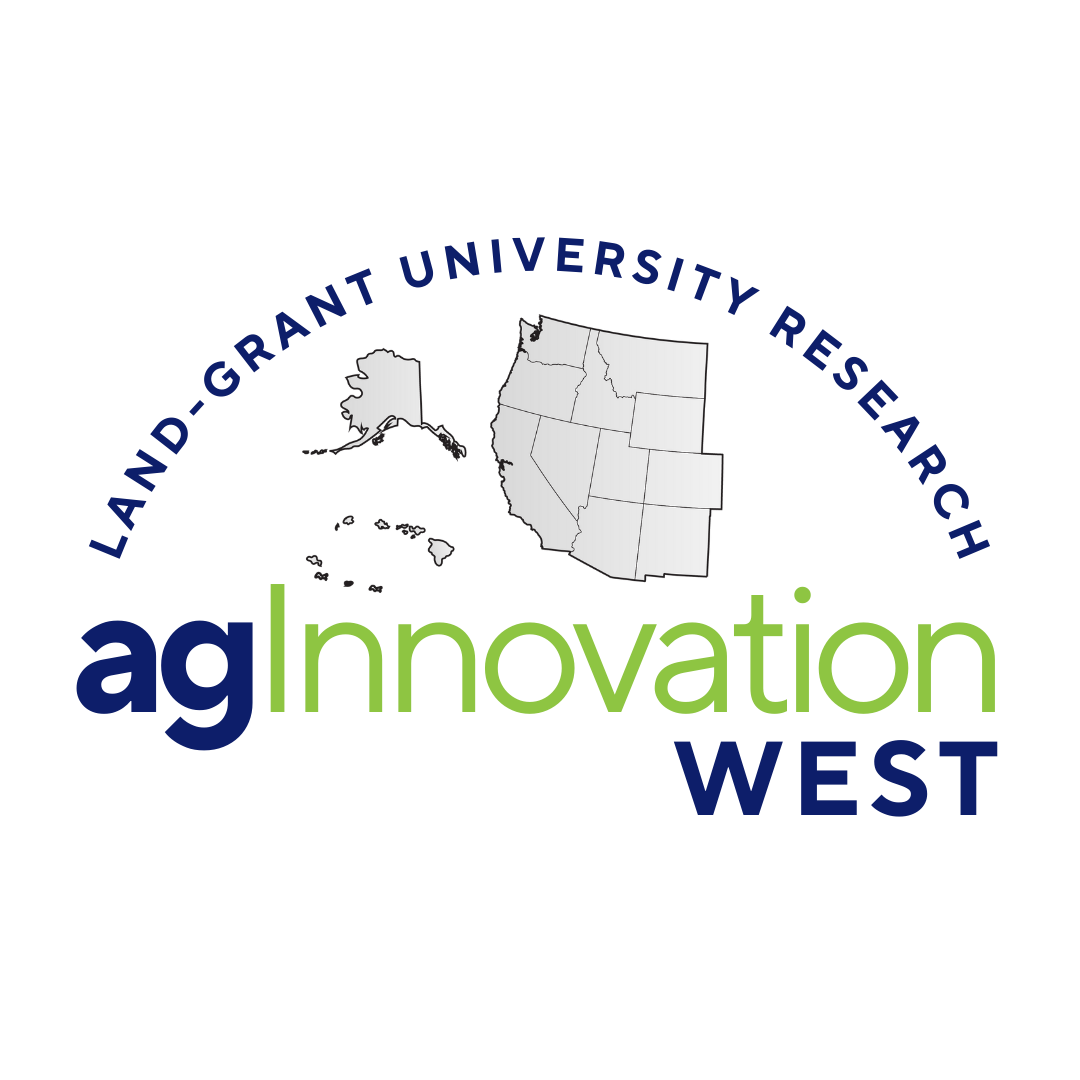
W128: Micro-Irrigation: Management Practices to Sustain Water Quality and Agricultural Productivity
(Multistate Research Project)
Status: Inactive/Terminating
W128: Micro-Irrigation: Management Practices to Sustain Water Quality and Agricultural Productivity
Duration: 10/01/1999 to 09/30/2004
Administrative Advisor(s):
NIFA Reps:
Non-Technical Summary
Statement of Issues and Justification
STATEMENT OF PROBLEM: Irrigated agriculture is facing increasing public pressure and the threat of increased regulation. These pressures include limited water resources; competition between urban, industrial, wildlife, recreation, and irrigation users; water quality degradation, and the economics. Municipal, industrial, and environmental groups are increasingly concerned with the fact that nearly 80% of the annual water supply in United States is being used for irrigated agriculture. In some instances poor irrigation practices have resulted in environmental degradation due to the transport of nutrients, pesticides, salt, and trace elements to surface and ground waters. JUSTIFICATION: Few people question that adjustments in irrigated agriculture will be required in the future (NAS, 1996, CAST, 1996). However, the scope of dependence on an irrigated agriculture base that produces 38% of farm revenue with only 15% of the land base requires that economical and technologically sound solutions be developed. Irrigation not only helps provide an economical and stable food supply for the nation but also helps to maintain prosperous and sustainable producers and rural communities. A compelling need exists for solutions that can help maintain higher levels of irrigation sustainability where water supplies can be replenished. In addition solutions are needed that will lengthen the transition period to a less irrigation intensive society in areas where replenishment is less an option. Most water planners and resource managers recognize that there will be no magic bullet that will remove all of the nations water problems. Instead there is a growing realization that it will take many tools working together to help avoid the significant disruption in the economies and societies grown accustomed to widespread irrigation use. Microirrigation is just one of the many irrigation and water management technology tools, but it is a tool that has several advantages. Microirrigation can reduce the waste of water to a negligible amount and the transport of contaminants to surface water and groundwater. Irrigation events can be fine-tuned to spoon feed water and nutrients just in time to avoid plant stress. It can optimize crop production (maximize economic yield for each unit of water) and in many cases increase the quality of agricultural products. Some scientists, water planners, and resource managers have been disappointed with the rate of adoption in microirrigation. The U.S. land area that is microirrigated varies on an annual basis but during the last 10 years has hovered in the range of 3 to 4% of the total irrigated area (Irrigation Journal, 1998). It is recognized that some crops and locations are not physically or economically suitable for microirrigation, but this is probably the exception rather than a common situation. Further efforts are justified to reduce the impediments to more widespread adoption. A cooperative approach towards technology development can be extremely fruitful as evidenced by the significant body of research developed by W128 members over the last 20 years. The cooperative approach allows for the evaluation of group hypotheses and for distillation of common results into general guidelines. It is suggested that the W128 regional project has been very successful in using this approach. The cooperative approach can also be utilized as a peer review process for hypotheses developed by individuals with the hopes of expanding or advancing the overall scientific understanding. Research to adapt or modify technologies fall into this category. Another advantage of the cooperative approach is its utilization as a strong feedback mechanism to point out where there is not enough information or theoretical understanding to develop general guidelines. In the proposed revision, W128 members will utilize the distillation process, peer review process and the feedback mechanism to accomplish the project objectives. In many cases, it will be necessary to refine and adapt microirrigation technologies for site specific conditions (crops, soils, water quality and availability, climate and irrigation system characteristics). In other cases, it will be necessary to improve and develop management strategies to take advantage of the inherent capabilities of microirrigation. To increase the adoption rate of appropriate microirrigation technologies, it will be necessary to assess and improve the decision criteria utilized in the adoption process and it will be necessary to improve technology transfer. RELATED CURRENT AND PREVIOUS WORK: Overview Microirrigation was first used in the United States in the late 1950s and early 1960s for greenhouse research and production. The first field research and demonstration studies were initiated in California in 1969 on avocados, strawberries, and tomatoes (Bucks, 1995). The USDA Western Regional project W128 concerning microirrigation followed shortly thereafter in 1972. The progress of the science of microirrigation can be partially illustrated by the periodic International Congresses that have been devoted to the subject. The First Congress in Israel in 1971 had 21 papers. The Fifth Congress was held in Orlando, Florida in 1995 with 156 presentations. Phene (1995) reflected on the historical record of research during this period and concluded that although much progress had been made, there still remained much research work to be done. Some of the prominent areas of research need listed were plant water and nutrient requirements under microirrigation; chemigation; system design and uniformity; system hardware, sensors, and automation; and modeling and decision support systems; economics, and horticulture and agronomic research to adapt to the capabilities of this relatively new irrigation method. Phene (1995) went on to point out that the bulk of the coordinated microirrigation research in the United States has been conducted by two USDA regional projects, W128 and S264 (Note: S264 was formerly S247 and S143). In comparing the subject of papers in the 1985 and 1995 International Microirrigation Congresses, Phene (1995) showed that predominant research areas remained essentially the same. This was not to criticize the progress of research but to indicate that there needs to be more continued research. This seems especially true particularly in the areas of adaptation and refinement of the technologies to remove stumbling blocks that have been encountered. The proposed W128 project seeks to develop such information. Further literature review will discuss current and previous research related to the proposed project objectives and where this research has fallen short or will be extended by the proposed W128 project. Water/Nutrient Management and Chemigation Bucks and Davis (1986) listed a number of potential advantages for microirrigation. They include increased beneficial use of water, enhanced plant growth and yield, reduced salinity hazard, improved application of fertilizer and other chemicals, limited weed growth, decreased energy requirements, and improved cultural practices. The first four potential advantages are the most important in ensuring efficient use and protection of water resources. Phene et al. (1992) listed several characteristics of SDI systems that can contribute to maximizing water use efficiency, including negligible soil evaporation, percolation, and runoff. Developing careful utilization and management procedures for these potential microirrigation advantages is a major research thrust of the proposed W128 project. Establishing and maintaining concentrated root systems near microirrigation emitters is one potential method of making more efficient use of water and nutrients. Regulated deficit irrigation (RDI) which is limited to relatively arid areas is a controlled root volume technique especially suited to microirrigation. RDI research conducted in Australia on peaches (Chalmers et al., 1981) and pears (Mitchell et al., 1984), Washington on apples (Middleton et al., 1981; Proebsting et al., 1977; Peretz et al., 1984; Evans et al., 1993, Ebel et al., 1995; Drake and Evans, 1997) and grapes (Evans et al., 1990; Wample, 1996) have produced beneficial responses. Additional work in California, Israel and other arid locations on several crops has also shown that carefully managing the severity and duration of a uniform, constant level of water stress on fruit trees and some other perennial crops can be advantageous. RDI been found to control vegetative growth, increase fruiting, advance fruit maturity, increase precocity and increase soluble solids. Annual water diversions have been shown to be reduced by 20% or more. In the proposed project, previous work will be extended to additional tree crops and through developing improved strategies to manage soil water and nutrients within the RDI context. Plant and soil water monitoring can potentially better define acceptable water stress levels and duration. A major difficulty in the estimation of water-use efficiency in irrigated agriculture is the uncertainty in the drainage calculations (Hutmacher et al., 1994). In addition to the reduction of water losses, knowledge of the drainage component is also important in the quantification of downward leaching of soluble nutrients such as nitrates. From an environmental perspective, one must assure that these chemicals are not transported below the rootzone toward the groundwater. The drainage rate can be determined from soil water potential gradients and unsaturated hydraulic conductivity values from below the root system. However, the characterization of the appropriate soil hydraulic conductivity functions is difficult. Many methods are available (Klute and Dirksen, 1986). In situ methods are preferred because results are more representative of field conditions although they are complicated and time-consuming. Recently, the inverse estimation of soil hydraulic properties has emerged as an attractive, accurate technique for laboratory experiments (Eching and Hopmans, 1994), but the utility of laboratory-measured soil hydraulic data for application of field studies is difficult and needs further study. Field studies of the pattern of root development and water uptake are especially important for the optimization of irrigation water use efficiency. Water should not be applied in areas where roots are absent, or at a rate higher than the roots can possibly take up. Thus, emitter spacing and irrigation scheduling must be flexible according to the planting pattern and crop characteristics. In general, root development under drip irrigation is constrained to the soil volume wetted by the emitters, near the soil surface with root length density decreasing with depth (Goldberg et al., 1971; Stevens and Douglas, 1994; Michelakis et al., 1993). However, recent studies have shown that root water uptake is not always in direct proportion to root length density (Clothier et al., 1990) and that plants can quickly adapt their spatial pattern of water uptake in response to irrigation water application distribution (Clothier and Green, 1994). Furthermore, it is important that empirical sink functions used in water flow and solute transport models under drip irrigation reflect the field-observed root water uptake patterns. However, few of these water uptake models have been independently tested under field experimental conditions (Molz, 1981). Studies, such as those by Coelho and Or (1996), are needed to provide accurate spatial and temporal root water uptake distribution data and models. It is hypothesized that variables such as emitter position relative to the active roots as well as irrigation amount and frequency will affect the soil water regime in general, and specifically the spatial and temporal changes in soil water content as controlled by root water uptake and leaching. Gaining a better understanding of these interrelationships, as proposed in this project, will provide alternative means for proper and efficient drip irrigation water management practices. Irrigation scheduling and water management takes on many forms (Hoffman et al. 1985). Some of these techniques are soil-based, some are plant-based, and some are climatic-based and some are a combination of two or more approaches. In specific circumstances, all of these methods can be particularly meritorious for microirrigation. Climatic-based approaches typically take the form of a water budget with a calculated reference ET multiplied by a crop coefficient. In the proposed project, efforts will be made to develop and refine crop coefficients for a number of crops under microirrigated conditions. Soil-based or plant-based methods which use sensors and controls to initiate and terminate irrigation are highly appropriate for the typical microirrigation system with its high degree of automation and application uniformity. Several of the proposed substudies will be comparing different types of sensors and controls for irrigation to more traditional irrigation scheduling techniques. A thorough review of the use of sensors for irrigation management is provided by Phene et al. (1990). Comparison of microirrigation to the more traditional forms of irrigation is an important research area because it underlies the decision process concerning adoption of microirrigation systems. Although these comparisons are important, they have often been neglected because of the difficulty in performing statistical comparisons with the scale and operating factors associated with irrigation. In a thorough review of SDI research, Camp (1998) was only able to identify 3 papers where SDI was compared to other irrigation methods. In the broader microirrigation area, only 16 papers at the Fifth International Microirrigation Congress touched on irrigation system comparisons (Lamm, editor 1995). The proposed work will extend previous work by performing some in-field statistical comparisons as well as with some larger demonstration-type studies intended to showcase the technologies to producers. The combined management of irrigation and nutrient management has long been recognized as an inherent advantage of microirrigation systems. It has received increasing research focus in recent years because of water quality concerns. Indeed, it was essentially the entire focus of the current W128 project, Microirrigation: Management Practices to Sustain Water Quality and Agricultural Productivity due to expire in September 1999. Proposed work in the new project would seek to extend these concepts to new crops and to deal with shortcomings in adapting the technology to producer operations. Some of the proposed work would be related to in-field studies while others would be broader modeling efforts. In a CRIS search of other related regional projects W-82, Pesticide and other Toxic Organics in Soil and their Potential for Ground and Surface Water Contamination was identified as having somewhat related objectives. However, the objectives of W-82 appear to be much more strongly related to modeling efforts. Microirrigation can also serve as a precisely targeted delivery system for pesticides (chemigation). Thus, microirrigation chemigation is based on the principles of precision farming where system inputs are qualitatively and quantitatively matched to the needs of the crop. Subsurface drip (SDI) and surface drip systems can be used to for the injection of systemic pesticides and some biocontrol agents while surface microsprinklers may be used to apply biocontrol agents over larger areas and on plant canopies. Use of subsurface microirrigation irrigation systems for systemic insecticide or fungicide application has the advantage of compatibility with integrated pest management principles. However, the use of pesticides through microirrigation systems is much less advanced as compared to nutrient fertigation. No papers directly related to pesticide chemigation were given at the Fifth International Microirrigation Congress (Lamm, editor, 1995). The slow advance is partially because of the increased concern about operational failures that might result in pesticide contamination of water resources. In a more recent review (Evans, 1998) did find at least eight studies where pesticide chemigation had been practiced with microirrigation. Washington State University, a member of W128, is currently involved in a NRI project concerning use of systemic insecticides through microirrigation system. The proposed W128 project will extend the relatively narrow research database on pesticide chemigation. Proposed studies are primarily related to identifying appropriate chemicals, delivery systems and evaluating their effectiveness. System Design, Operational Procedures, and Uniformity The proper design and operation has always been a high research priority, because within that design and operational strategy lies the inherent microirrigation advantage of precise and uniform application. Its high priority is partially due to microirrigations relatively short 30-40 year history and also due to the fact that precise irrigation application demands precise design and management. Camp (1998) identified 25 papers that touched on design of SDI systems, yet Lamm et al. (1995) indicated that most design guidelines are generally very specific to regions, crops and soils. Design guidelines may always necessarily contain this local aspect, but the proposed W128 project seeks to broaden these guidelines through the coordinated critical feedback members can give to each other. System characteristics and component operation are also areas in which W128 seeks to make improvements. This will include component testing as well as computer modeling to improve management. The importance of uniformity to microirrigation systems and the continued development of uniformity evaluation tools remains a major research topic. Some of the present evaluation methods (ASAE EP-458, distribution uniformity, emission uniformity) are better suited to certain types of evaluations and locales. The search for a more common or unifying evaluation tool continues. The proposed W128 research seeks to develop better evaluation tools as well as to evaluate redistribution of water and chemicals with the present uniformity evaluation tools. Decision Criteria and Economics Although there is general agreement in scientific circles that the adoption of microirrigation technology is not well understood, there is only a small amount of directly related research. Much of the identifiable research has been conducted in W128 member states of California, Florida, Hawaii, Kansas, Texas and Virginia. The proposed W128 research takes the initial first step to rectifying this information void by agreeing that all states will perform cost/benefit analyses for all microirrigation technologies they are investigating or promoting. Technology Transfer Most media experts agree that there is no single correct medium for transferring knowledge because of the vast differences in peoples abilities and learning modes. Some people are more suited to visual learning while others can easily handle auditory learning. The proposed W128 project will continue to use a wide array of technology transfer techniques. The Internet is emerging as an effective tool for technology transfer (Sistrunk, 1998, Golden et al.1994) and some special emphasis will be placed on using it during the proposed project. Transferring this knowledge to the Internet often requires some adaptation and interpretation because the new Internet media presents material on screen instead of in paper format (Ritchie and Hoffman, 1997).
Related, Current and Previous Work
Overview Microirrigation was first used in the United States in the late 1950s and early 1960s for greenhouse research and production. The first field research and demonstration studies were initiated in California in 1969 on avocados, strawberries, and tomatoes (Bucks, 1995). The USDA Western Regional project W128 concerning microirrigation followed shortly thereafter in 1972. The progress of the science of microirrigation can be partially illustrated by the periodic International Congresses that have been devoted to the subject. The First Congress in Israel in 1971 had 21 papers. The Fifth Congress was held in Orlando, Florida in 1995 with 156 presentations. Phene (1995) reflected on the historical record of research during this period and concluded that although much progress had been made, there still remained much research work to be done. Some of the prominent areas of research need listed were plant water and nutrient requirements under microirrigation; chemigation; system design and uniformity; system hardware, sensors, and automation; and modeling and decision support systems; economics, and horticulture and agronomic research to adapt to the capabilities of this relatively new irrigation method. Phene (1995) went on to point out that the bulk of the coordinated microirrigation research in the United States has been conducted by two USDA regional projects, W128 and S264 (Note: S264 was formerly S247 and S143). In comparing the subject of papers in the 1985 and 1995 International Microirrigation Congresses, Phene (1995) showed that predominant research areas remained essentially the same. This was not to criticize the progress of research but to indicate that there needs to be more continued research. This seems especially true particularly in the areas of adaptation and refinement of the technologies to remove stumbling blocks that have been encountered. The proposed W128 project seeks to develop such information. Further literature review will discuss current and previous research related to the proposed project objectives and where this research has fallen short or will be extended by the proposed W128 project. Water/Nutrient Management and Chemigation Bucks and Davis (1986) listed a number of potential advantages for microirrigation. They include increased beneficial use of water, enhanced plant growth and yield, reduced salinity hazard, improved application of fertilizer and other chemicals, limited weed growth, decreased energy requirements, and improved cultural practices. The first four potential advantages are the most important in ensuring efficient use and protection of water resources. Phene et al. (1992) listed several characteristics of SDI systems that can contribute to maximizing water use efficiency, including negligible soil evaporation, percolation, and runoff. Developing careful utilization and management procedures for these potential microirrigation advantages is a major research thrust of the proposed W128 project. Establishing and maintaining concentrated root systems near microirrigation emitters is one potential method of making more efficient use of water and nutrients. Regulated deficit irrigation (RDI) which is limited to relatively arid areas is a controlled root volume technique especially suited to microirrigation. RDI research conducted in Australia on peaches (Chalmers et al., 1981) and pears (Mitchell et al., 1984), Washington on apples (Middleton et al., 1981; Proebsting et al., 1977; Peretz et al., 1984; Evans et al., 1993, Ebel et al., 1995; Drake and Evans, 1997) and grapes (Evans et al., 1990; Wample, 1996) have produced beneficial responses. Additional work in California, Israel and other arid locations on several crops has also shown that carefully managing the severity and duration of a uniform, constant level of water stress on fruit trees and some other perennial crops can be advantageous. RDI been found to control vegetative growth, increase fruiting, advance fruit maturity, increase precocity and increase soluble solids. Annual water diversions have been shown to be reduced by 20% or more. In the proposed project, previous work will be extended to additional tree crops and through developing improved strategies to manage soil water and nutrients within the RDI context. Plant and soil water monitoring can potentially better define acceptable water stress levels and duration. A major difficulty in the estimation of water-use efficiency in irrigated agriculture is the uncertainty in the drainage calculations (Hutmacher et al., 1994). In addition to the reduction of water losses, knowledge of the drainage component is also important in the quantification of downward leaching of soluble nutrients such as nitrates. From an environmental perspective, one must assure that these chemicals are not transported below the rootzone toward the groundwater. The drainage rate can be determined from soil water potential gradients and unsaturated hydraulic conductivity values from below the root system. However, the characterization of the appropriate soil hydraulic conductivity functions is difficult. Many methods are available (Klute and Dirksen, 1986). In situ methods are preferred because results are more representative of field conditions although they are complicated and time-consuming. Recently, the inverse estimation of soil hydraulic properties has emerged as an attractive, accurate technique for laboratory experiments (Eching and Hopmans, 1994), but the utility of laboratory-measured soil hydraulic data for application of field studies is difficult and needs further study. Field studies of the pattern of root development and water uptake are especially important for the optimization of irrigation water use efficiency. Water should not be applied in areas where roots are absent, or at a rate higher than the roots can possibly take up. Thus, emitter spacing and irrigation scheduling must be flexible according to the planting pattern and crop characteristics. In general, root development under drip irrigation is constrained to the soil volume wetted by the emitters, near the soil surface with root length density decreasing with depth (Goldberg et al., 1971; Stevens and Douglas, 1994; Michelakis et al., 1993). However, recent studies have shown that root water uptake is not always in direct proportion to root length density (Clothier et al., 1990) and that plants can quickly adapt their spatial pattern of water uptake in response to irrigation water application distribution (Clothier and Green, 1994). Furthermore, it is important that empirical sink functions used in water flow and solute transport models under drip irrigation reflect the field-observed root water uptake patterns. However, few of these water uptake models have been independently tested under field experimental conditions (Molz, 1981). Studies, such as those by Coelho and Or (1996), are needed to provide accurate spatial and temporal root water uptake distribution data and models. It is hypothesized that variables such as emitter position relative to the active roots as well as irrigation amount and frequency will affect the soil water regime in general, and specifically the spatial and temporal changes in soil water content as controlled by root water uptake and leaching. Gaining a better understanding of these interrelationships, as proposed in this project, will provide alternative means for proper and efficient drip irrigation water management practices. Irrigation scheduling and water management takes on many forms (Hoffman et al. 1985). Some of these techniques are soil-based, some are plant-based, and some are climatic-based and some are a combination of two or more approaches. In specific circumstances, all of these methods can be particularly meritorious for microirrigation. Climatic-based approaches typically take the form of a water budget with a calculated reference ET multiplied by a crop coefficient. In the proposed project, efforts will be made to develop and refine crop coefficients for a number of crops under microirrigated conditions. Soil-based or plant-based methods which use sensors and controls to initiate and terminate irrigation are highly appropriate for the typical microirrigation system with its high degree of automation and application uniformity. Several of the proposed substudies will be comparing different types of sensors and controls for irrigation to more traditional irrigation scheduling techniques. A thorough review of the use of sensors for irrigation management is provided by Phene et al. (1990). Comparison of microirrigation to the more traditional forms of irrigation is an important research area because it underlies the decision process concerning adoption of microirrigation systems. Although these comparisons are important, they have often been neglected because of the difficulty in performing statistical comparisons with the scale and operating factors associated with irrigation. In a thorough review of SDI research, Camp (1998) was only able to identify 3 papers where SDI was compared to other irrigation methods. In the broader microirrigation area, only 16 papers at the Fifth International Microirrigation Congress touched on irrigation system comparisons (Lamm, editor 1995). The proposed work will extend previous work by performing some in-field statistical comparisons as well as with some larger demonstration-type studies intended to showcase the technologies to producers. The combined management of irrigation and nutrient management has long been recognized as an inherent advantage of microirrigation systems. It has received increasing research focus in recent years because of water quality concerns. Indeed, it was essentially the entire focus of the current W128 project, Microirrigation: Management Practices to Sustain Water Quality and Agricultural Productivity due to expire in September 1999. Proposed work in the new project would seek to extend these concepts to new crops and to deal with shortcomings in adapting the technology to producer operations. Some of the proposed work would be related to in-field studies while others would be broader modeling efforts. In a CRIS search of other related regional projects W-82, Pesticide and other Toxic Organics in Soil and their Potential for Ground and Surface Water Contamination was identified as having somewhat related objectives. However, the objectives of W-82 appear to be much more strongly related to modeling efforts. Microirrigation can also serve as a precisely targeted delivery system for pesticides (chemigation). Thus, microirrigation chemigation is based on the principles of precision farming where system inputs are qualitatively and quantitatively matched to the needs of the crop. Subsurface drip (SDI) and surface drip systems can be used to for the injection of systemic pesticides and some biocontrol agents while surface microsprinklers may be used to apply biocontrol agents over larger areas and on plant canopies. Use of subsurface microirrigation irrigation systems for systemic insecticide or fungicide application has the advantage of compatibility with integrated pest management principles. However, the use of pesticides through microirrigation systems is much less advanced as compared to nutrient fertigation. No papers directly related to pesticide chemigation were given at the Fifth International Microirrigation Congress (Lamm, editor, 1995). The slow advance is partially because of the increased concern about operational failures that might result in pesticide contamination of water resources. In a more recent review (Evans, 1998) did find at least eight studies where pesticide chemigation had been practiced with microirrigation. Washington State University, a member of W128, is currently involved in a NRI project concerning use of systemic insecticides through microirrigation system. The proposed W128 project will extend the relatively narrow research database on pesticide chemigation. Proposed studies are primarily related to identifying appropriate chemicals, delivery systems and evaluating their effectiveness. System Design, Operational Procedures, and Uniformity The proper design and operation has always been a high research priority, because within that design and operational strategy lies the inherent microirrigation advantage of precise and uniform application. Its high priority is partially due to microirrigations relatively short 30-40 year history and also due to the fact that precise irrigation application demands precise design and management. Camp (1998) identified 25 papers that touched on design of SDI systems, yet Lamm et al. (1995) indicated that most design guidelines are generally very specific to regions, crops and soils. Design guidelines may always necessarily contain this local aspect, but the proposed W128 project seeks to broaden these guidelines through the coordinated critical feedback members can give to each other. System characteristics and component operation are also areas in which W128 seeks to make improvements. This will include component testing as well as computer modeling to improve management. The importance of uniformity to microirrigation systems and the continued development of uniformity evaluation tools remains a major research topic. Some of the present evaluation methods (ASAE EP-458, distribution uniformity, emission uniformity) are better suited to certain types of evaluations and locales. The search for a more common or unifying evaluation tool continues. The proposed W128 research seeks to develop better evaluation tools as well as to evaluate redistribution of water and chemicals with the present uniformity evaluation tools. Decision Criteria and Economics Although there is general agreement in scientific circles that the adoption of microirrigation technology is not well understood, there is only a small amount of directly related research. Much of the identifiable research has been conducted in W128 member states of California, Florida, Hawaii, Kansas, Texas and Virginia. The proposed W128 research takes the initial first step to rectifying this information void by agreeing that all states will perform cost/benefit analyses for all microirrigation technologies they are investigating or promoting. Technology Transfer Most media experts agree that there is no single correct medium for transferring knowledge because of the vast differences in peoples abilities and learning modes. Some people are more suited to visual learning while others can easily handle auditory learning. The proposed W128 project will continue to use a wide array of technology transfer techniques. The Internet is emerging as an effective tool for technology transfer (Sistrunk, 1998, Golden et al.1994) and some special emphasis will be placed on using it during the proposed project. Transferring this knowledge to the Internet often requires some adaptation and interpretation because the new Internet media presents material on screen instead of in paper format (Ritchie and Hoffman, 1997).
Objectives
-
To evaluate and refine microirrigation management strategies to promote natural resource protection and optimal crop production
-
To improve, modify, and evaluate microirrigation system design and components for natural resource protection and optimal crop production
-
To assess and develop decision criteria for adoption of microirrigation technologies
-
To promote appropriate microirrigation technologies through formal and informal educational activities
-
Methods
Identify and characterize genes whose expression is associated with freeze-tolerance and winter survival.Measurement of Progress and Results
Outputs
- It is expected that microirrigation technologies will be further adapted and refined, so that producers can more easily utilize the technologies on their own operations. On the surface these adaptations and refinements may appear subtle, but they are essentially removing impediments to microirrigation adoption. For example, developing RDI (regulated deficit irrigation) technologies is highly appropriate to microirrigation research. It not only conserves water but also can increase water use efficiency and enhance crop quality. Thus it provides a win-win situation. Another example of benefits of the proposed work would be the lessening of foliar diseases through adoption of microirrigation. Many valuable crops are grown in the highly productive farm areas of the W128 member states, yet water resources are vulnerable to overuse and/or pollution. The combined management of water and nutrients, an integral part of the proposed research can help sustain these important crop production areas. Efforts at developing new chemigation materials and methods can result in improved product efficacy, improved worker safety, and reduced environmental hazards. Reducing human/wildlife exposure to dangerous pesticides in the environment has a tremendous value. Modeling efforts will not only assess the effectiveness of current technologies but will also identify potential new modifications to existing microirrigation technologies. For example, combining of nutrient management with water management and pesticide chemigation might help ensure optimum production and thus high efficiency from all inputs. Technology transfer is such an important aspect to the project, that Objective 4 is totally focused on it. All states agree to utilize a broad mix of traditional and non-traditional educational mediums to meet the objective. Effectiveness will be evaluated based on clientele feedback, numbers of information requests, types of information requests (i.e. basic or advanced), numbers of conference attendees, and technology adoption rates. One additional and somewhat unique aspect to the project will be the proposal of a national or international technical session concerning microirrigation technologies to one of the major US scientific bodies. This proposed session would be organized, coordinated and presented by W128 members.