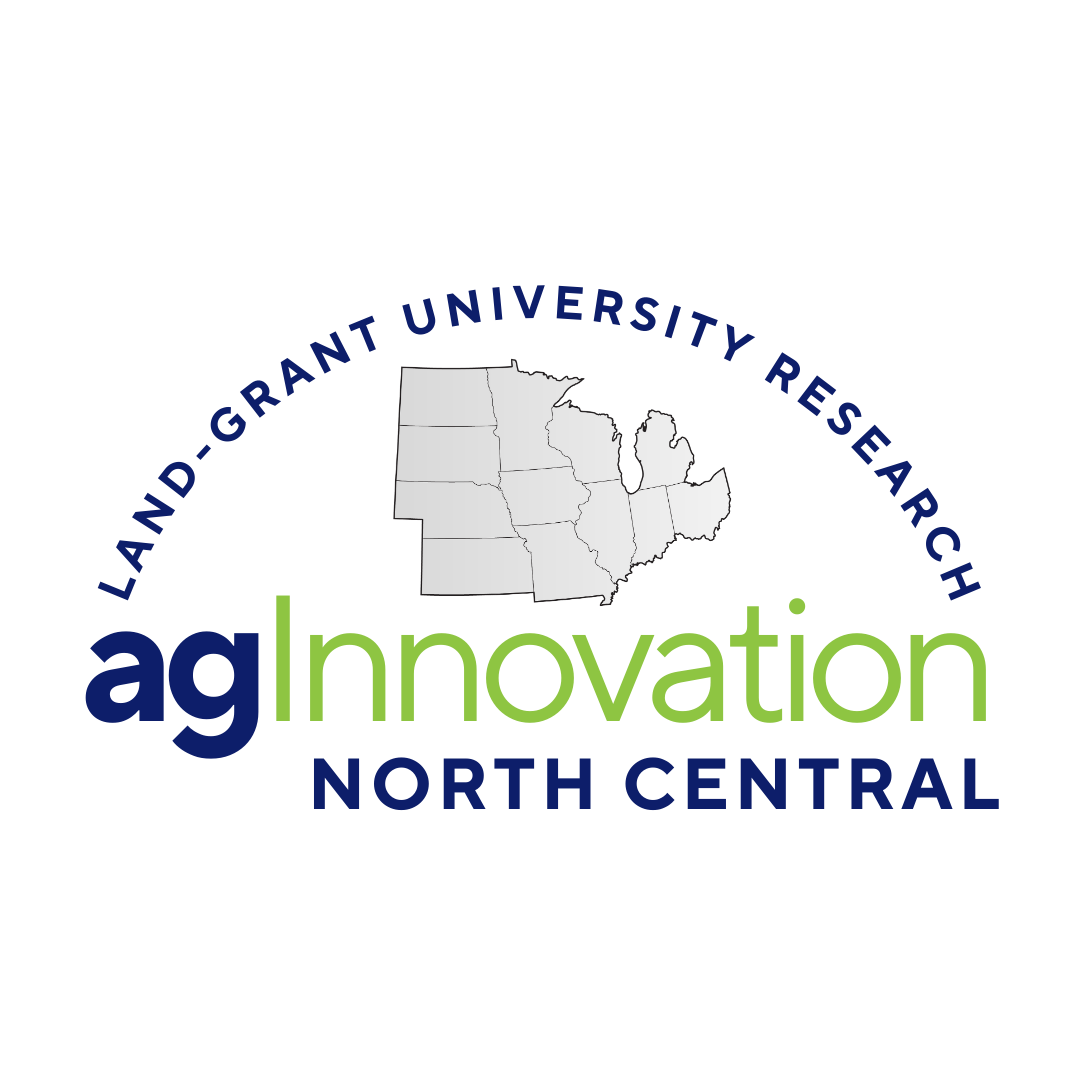
NC1004: Genetic and Functional Genomic Approaches to Improve Production and Quality of Pork
(Multistate Research Project)
Status: Inactive/Terminating
NC1004: Genetic and Functional Genomic Approaches to Improve Production and Quality of Pork
Duration: 10/01/2002 to 09/30/2007
Administrative Advisor(s):
NIFA Reps:
Non-Technical Summary
Statement of Issues and Justification
US swine producers have made tremendous improvements in the efficiency of converting feed and other inputs into pork products and genetic selection has played and will continue to play a key role in this success. Whereas production efficiency continues to be important for the U.S. swine industry, product quality, and animal health are of growing importance and form the basis of the research proposed herein. Improvement in these factors is required for US producers to remain competitive in an increasingly global market place and to compete with other meat industries and sources of protein. To this end, the National Pork Board, which represents U.S. pork producers and is one the largest U.S. livestock commodity organizations, has adopted the following mission: "to enhance opportunities for the success of U.S. pork producers and other industry stakeholders by establishing the U.S. pork industry as a consistent and responsible supplier of high quality pork to the domestic and world market, making U.S. pork the consumers meat of choice". Additionally, the mission statement of the National Swine Improvement Federation, which represents independent and company swine seedstock producers is "to advance and stimulate efforts of swine breeding stock suppliers, academic personnel, and pork industry affiliates in the research, development, and utilization of scientifically-based genetic improvement programs and associated practices for the economically efficient production of high quality, nutritious pork". The research outlined in this proposal addresses the missions of these industry organizations by elucidating the genetic control of product quality, animal health, and environmental sustainability. Furthermore, The American Society of Animal Sciences mission statement "to discover, disseminate and apply knowledge for sustainable use of animals for food and other human needs" and the Federation of Animal Science Societies stated purpose "to promote education and research." would be well served by the scientific work, publication, and outreach activities of the proposed project.
Pork quality comprises a set of key fresh meat qualities and processing characteristics that are important for the future profitability and competitiveness of the swine industry. These include intramuscular fat, cholesterol, pH, color, water holding capacity, cooking loss, tenderness, and sensory traits. Because most aspects of pork quality can only be measured on the carcass or by consumer panels, they have been difficult to improve by conventional means. However, most are at least moderately heritable and several quality-related genes have been identified. Great opportunities, therefore, exist for the use of marker-assisted selection to improve pork quality.
Improved disease resistance will enhance production efficiency, animal welfare, and producer acceptance of the pork production system. In addition, improved resistance to disease will reduce the need for antibiotics to prevent or cure disease and the potential for pork products to harbor pathogens that are of potential danger to consumers. Knowledge about the genetic aspects of resistance to disease in swine is limited.
Pork quality and disease resistance involve complex systems that require a multi-disciplinary approach to develop an understanding of the underlying genetic factors and to develop strategies for their genetic advancement. Whereas traditional approaches have concentrated on population and quantitative genetics, it is clear that a concerted effort in molecular and population/quantitative genetics is required to address these emerging issues.
Although these factors are of prime importance for the future of the U.S. swine industry, they must be accompanied by simultaneous improvement in production efficiency. Consumers expect food to be safe, of high quality and reasonably priced. This can only be achieved through a concerted effort to improve efficiency along with quality and animal health.
Over the past decade, NC-210 researchers have made substantial advances in the development and utilization of molecular and genomic technologies to map genes in the pig genome and to study their function. Researchers of NC-220 have developed expertise to integrate quantitative and molecular genetics for genetic improvement. Researchers in both groups have active collaborations with scientists with expertise in the biological and physiological aspects of disease resistance, meat quality, and production efficiency. Jointly, researchers from NC-210 and 220 are in an ideal position to successfully address the objectives of this project.
Because of the complex and multi-faceted nature of the proposed research, no single institution has sufficient resources and facilities to address the wide range and interdependent sets of research questions that must be answered. Therefore, a coordinated and integrated effort among experiment stations, as outlined in this proposal, is crucial for the success and relevance of any research effort on these aspects of pork production.