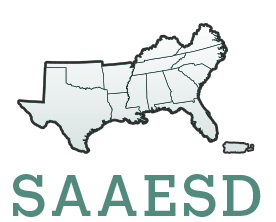
S1007: The Science and Engineering for a Biobased Industry and Economy
(Multistate Research Project)
Status: Inactive/Terminating
S1007: The Science and Engineering for a Biobased Industry and Economy
Duration: 10/01/2002 to 09/30/2007
Administrative Advisor(s):
NIFA Reps:
Non-Technical Summary
Statement of Issues and Justification
A. The Land Grant University System, Resource Limitation, and The Impending Biological Revolution. The Land-grant university system was established in 1862 for the purpose of providing colleges for the benefit of agriculture and the mechanic arts. This revolutionary approach to education has been remarkably successful. Since its inception, the land-grant system has been the major driving force for the development of technology and an educated work force to use the technology that has maintained an exponential rate of increase in food production outpacing the rate of human population increase. This success is even more remarkable in light of the fact that in recent years the increase in food production has occurred using constant land area. As the world human population continues to grow, the challenge to increase food production on a sustainable basis remains staggering. Much of the remarkable increase in food production has been made possible by the use of fossil fuels to drive the system. The shift to a fossil fuel economy has transformed agriculture linking it to energy intensive technologies. If fossil fuel use were abruptly discontinued it has been estimated (Rossi & Tiezzi, 1991) that 4 of 5 humans alive today would starve. Our dependence on fossil fuels is so extensive that we can no longer claim that agriculture converts solar energy into food energy. Rather, we are using some solar energy to convert fossil fuel to food with poor efficiency. Production, processing, storage, and distribution of food requires approximately 15% of our annual national energy supply (Pimentel & Pimentel, 1996). The time has come to direct the attention and power of the Land Grant University System toward the development of technology that can "wean" us from use of fossil fuel. As staggering as is the challenge to meet food production needs, it is meager compared to the need for energy. It requires approximately 100-fold more energy to sustain the current U.S. standard of living as it does to nourish our bodies. For example, the U.S. consumes approximately 100 quadrillion Btu annually. Food energy necessary to sustain our population is 1.2 quadrillion Btu/year of the approximately 100 quadrillion Btu (US DOE, 2000). Our population is fed with this 1.2 quadrillion Btu annually (3000 kcal/(person x day) x 365 days/year x 284,212,779 people x 1 Btu/0.252kcal = 1.2 quadrillion Btu/year). In effect, we are converting fossil fuel to food with an efficiency of about 8%. In the next 100 years world human population is projected to stabilize at about 10 billion (Caldwell, 1999). During this time, some fossil fuels may be approaching depletion (Woolsey, 2000). Having the goal of supporting a population of 10 billion at the current standard of living of the US will require approximately 4,000 quadrillion Btu annually worldwide. These demands cannot be sustained in a solar-driven world with the current technology base. However, the land-grant university system provides a mechanism for leadership and technology development to accomplish this goal. It includes expert scientists in all areas related to the capture of solar energy and its transformation to food, fuel, and shelter. We have seen national interest in biomass-based fuel and chemicals rise and fall over the past thirty years with each oil embargo or market adjustment. Thus, it is fair for one to ask "What is new about situation today that justifies a commitment by land grant institutions to a renewed investment in biomass-based fuel and chemicals research and teaching?" One of the answers to this question has to be scientific and engineering breakthroughs that are creating new opportunities for producing and processing biomass resources, plants and microorganisms, and the engineering of novel biocatalysts, reactors and industrial processes. B. Enabling The New Bioindustries A great deal of the excitement over the potential for agriculturally-based bioindustries is driven by the enabling technologies of genomics and proteomics. Through these technologies we have obtained the ability to decipher and to manipulate plant genomes to produce both quantitative and qualitative changes in the organic constituents of plant biomass. Genomics and proteomics have also provided us with a greater understanding of gene regulation and control of plant metabolic pathways to the point that we can engineer metabolic pathways with unprecedented efficiencies and reliability. The combination of this knowledge allows engineers and scientist to be more creative and more efficient in the development of novel biocatalysts, biomass conversion processes, and bio-industrial systems. For instance, one can imagine that genes coding for certain polysaccharide degrading enzyme might be introduced in plants for the large-scale production of cellulases and other polysaccharide degrading enzymes; In essence the plant will be designed to serve as the factory. Molecular biology has also provided us with a number of tools that allow us to engage in protein engineering to enhance the hydrolytic capability of a number of polysaccharide-degrading enzymes through site-directed mutagenesis or directed evolution. In addition, advances in molecular biology have provided technologies that permit metabolic engineering of microorganisms to increase enzyme copy number or to metabolize multiple carbon sources. For example, one can envision protein engineering activities leading to the development of cellulases with higher activities on crystalline cellulose with the genes for these cellulases transferable into microorganisms that can both hydrolyze cellulose to glucose and ferment glucose to ethanol; (ie, simultaneous saccharification and fermentation). Breakthroughs are not limited to only molecular biology and genetics. Over the past 15 years, engineers have made tremendous advances in the fractionation and bioprocessing of agriculturally-based resources into raw materials that are required to obtain the principal building blocks for the synthesis of new products. For example, the critical first step in bioconversion requires that ligno-cellulosic materials be fractionated into constituent biopolymers and modified to facilitate either enzymatic or microbial conversion. Several promising fractionation (pre-treatment) technologies, such as ammonia fiber explosion or alkaline pretreatment, appear promising, and additional research and development may result in improved yields and reactivity. In addition, engineering advances in bioseparation technologies are improving our ability to identify and separate secondary metabolites and other biocompounds from plant material. Advances in nanobiotechnology such as the fabrication microfluidic channels in a silicon wafer couple that take advantage of differences in physical and chemical properties such as diffusion, electrophoretic mobility, or chemical affinity to produce rapid, efficient separations of proteins, secondary metabolites and other organic compound are only a few of the recent engineering achievements. In analytical biotechnology, chemical and biological techniques are combined and integrated into engineered devices or sensors for the detection and quantification of secondary metabolites and other organic compounds in a variety of bioprocesses. Development of advanced biological conversion processes (enzymatic, microbial and physical/chemical processes) is an important part of the bioprocessing research agenda. Biological processes are the preferred paths for converting agriculturally-based resources into industrial products. Bioprocesses tend to have higher reaction specificity, milder reaction conditions and produce fewer toxic by-products. These characteristics are very consistent with the goal of developing industrial processes and systems that are environmentally friendly. Innovative research is currently underway that links physical/chemical processes, such as gasification, with microbial conversion processes. These novel reaction systems are evolving in response to the heterogeneity of most biomass resources and the realization that there are important autotropic microorganisms that are effective in converting common bio-organic compounds into more useful industrial or biomedical compounds. Land grant institutions across the USA represent a great repository of scientific and engineering know-how that can be utilized to catalyze the transition of society from a fossil fuel to a bio-based economy. Another promising application for agriculture is the production of biopharmaceuticals using plant based materials. The development of recombinant DNA technology has enabled the insertion of exogenous genes into plant genome. As eucaryotes, plants can be used to express functional human proteins. This has been shown in green plant tissues, such as tobacco leaves, and seeds, such as corn and canola. Plants offer unparalleled advantageous for scale-up production of recombinant products as compared to microbial, cell culture, and transgenic animal expression systems. For crops at risk because of health (such as tobacco), or economic related issues, the development of new applications is critical for a sustained US agricultural economy. The potential of utilizing plants as biopharmaceutical production factories has been recognized but not been utilized. A major barrier will be the development of economic processes for recombinant protein recovery and purification. It is the mission of the land grant institutions to break this barrier and to promote wider applications for various corps for a more profitable and sustainable agricultural economy. C. The Educational Infrastructure for a Bio-based Economy. Our land grant institutions attract some of the best young minds in this country and from the broader world community. We need to tap this resource and begin to develop its potential for creating new and innovative systems for producing biobased products. We need to provide these students with fundamental training in the natural sciences, economics, and engineering. They must be taught to think critically about structure and function of bio-based industries and how to make these industries sustainable. Many recent federal, industrial, and academic studies have concluded that the U.S. economy of the 21st century will be biobased (Armstrong, 1999; National Research Council, 2000). During the transition from a petroleum-based economy to a biobased economy, products and processes based on biological raw materials will replace those based on fossil fuels. Biorefineries will use many types of biomass sources and produce a broad range of carbon-based products, energy fuels, oils, biochemicals, as well as a variety of biomaterials. The educational infrastructure needed to provide such training is currently not available in conventional academic programs, which provide a narrow focus and do not encourage interactions between students from different departments. Innovative, new training programs are needed that minimize these barriers and provide more integrated programs. These programs must train students to communicate effectively, to solve problems, and to design processes in a multidisciplinary setting. Because the participants in this regional effort are geographically dispersed, a distance-learning platform for courses developed as part of this regional project is strongly recommended. Internet-compatible courses can serve students in virtually any location. It is anticipated that other distance education media such as satellite communication methods could also be used to offer these course. In instances where internet delivery is impractical (e.g., lab-based courses), conventional, on-site course delivery may the best model. In either case the technical content of such courses should be disseminated, if possible, via publications in pedagogical journals.
Related, Current and Previous Work
A wide range of activities are being conducted in the both the private and public sectors related to biobased products and biobased industries. Many of the investigators participating in this proposed project will be involved in cooperative efforts with agencies such as DOE, and USDA-ARS, as well as, private research and development programs. A summary statement of related and current activities in each of the five objectives areas is presented. A more detailed presentation of related and current activities is provided in Appendix B, (see attachments). A. Feedstock Supply Increasing Energy Security in the U.S. requires the near-term commercialization of multiple alternative energy options. Biomass energy is one alternative that is close to economic viability in many areas. Focusing on reducing the cost of harvesting, handling, storing and transporting biomass resources could increase the competitiveness of biomass energy relatively quickly. One or several of the following characteristics cast uncertainty on the orderly flow of biomass feedstock from field to a biorefinery: (a) low bulk density, (b) spoilage due to high moisture, (c) variability in physical and chemical characteristics, (d) geographical and seasonal variations in biomass, (e) conflicting demands on farm labor and machines, (f) combustibility, (g) competition with need to maintain soil fertility, (h) local regulations on storage and transport, and (i) sensitivity to price structure for other farm commodities. The objective of feedstock engineering is to address these issues through cross cutting research and development programs. B. Biofuel Production Systems and Processes. Abundant supplies of lignocellulosic materials are available for use as biofuels, either through biological conversion to liquid fuels such as ethanol or through direct thermochemical conversion processes. Current U.S. production of fuel ethanol from corn starch supplies less than 2% of our automotive fuel needs. With improved processes, which expand the range of agricultural feedstocks for ethanol production to include lignocellulose, this industry can provide a viable alternative to imported petroleum-based fuels. Technologies currently are available for all steps in the bioconversion of lignocellulose to ethanol. However, these technologies must be improved and new technologies developed to produce this renewable fuel at a price which can compete with gasoline. Feedstock costs can be minimized by initially focusing on agricultural residues and waste materials with subsequent expansion to dedicated energy crops. Other process steps, which are particularly expensive, include pretreatments to improve bioconversion, the production of enzymes for carbohydrate depolymerization, liquid/solid separations, and capital costs associated with bioconversion. These research opportunities form the basis for the proposed tasks. C. Biomaterials. A wide variety of biomass resources are available for conversion into biomaterials. These may include whole plants, plant parts (e.g., seeds, stalks), plant constituents (e.g., starch, lipids, protein, fiber), processing byproducts (distillers' grains, corn solubles), materials of marine origin (e.g., chitin, sea weed, etc), and animal byproducts (e.g. gelatin, albumen, etc). These resources may be used "as is" to create new biomaterials, but more typically they will be processed for use in biomaterial manufacture. This will require an intimate understanding of the composition of raw materials -- whether it is whole plant, constituent, or byproduct - so that desired functional elements can be obtained for biomaterial production. Equally important, however, is that this fundamental understanding will afford the opportunity to recover other potentially valuable components (biofuels, biochemicals) from these raw materials. Conversion of raw materials (or their constituents) into biomaterials will entail three steps: process design, system optimization, and model development. Processing will take the form of various biological, themochemical, and/or physical methods designed to synthesize or bioconvert new materials, recover/purify such products, and subsequently perform any needed downstream modifications. In some cases, the desired product may already exist within the raw material, thus requiring only extraction, recovery, purification, and modification steps. Once individual methods have been developed, linking them into an integrated processing system will be the next challenge. The goal will be to optimize this system in terms of yield, efficiency, productivity, energy balance, and cost. As part of this optimization work a system model will be developed to permit sensitivity analyses of process variables. Products derived from this process may be finished products or may be incorporated into multi-component products. Examples might include biopolymers, biopolyols, adhesives, resins, food/feed ingredients, etc. D. Biobased Chemicals A number of processing tools must be developed or improved to handle the demands of bio-resource based biochemical production systems. For example, reactor systems capable of efficient handling of biomass materials require development. A focus area for research is in the optimization of biological reactor designs to handle both unprocessed plant materials and the wide range of microorganisms that will be needed for desired bioconversions. Systems capable of supporting growth of algae, and filamentous organisms, and both anaerobic and aerobic bacterial systems will be needed. Studies must be conducted on the growth, decay and product formation kinetics for the variety of microbes of interest using actual biobased feedstocks. Kinetic models of the microbial growth and product formation have to be developed and optimum reactor configuration (batch vs continuous, simple CSTR vs CSTR with recycle, etc.) determined based on simulations of growth and product formation reactions. Finally, the optimum reactor design parameters and operational conditions (hydraulic retention time, cell retention time, aeration, etc.) must be maximized and verified under lab and pilot-scale conditions. Alternate fermentation systems need to be investigated. Furthermore, to isolate biopharmaceuticals expressed in plant systems, there is an urgent need for efficient, effective and economical large-scale bioseparation techniques which will be capable of handling large quantity of biomass and achieve high purity and high recovery, while maintaining the biological activity of the molecules. One such purification technique which meets these criteria is aqueous two-phase extraction (ATPE) that involves the partitioning of proteins between two immiscible phases in an aqueous system. The main advantages of using ATPE systems are that the scale up is easy and reliable, continuous processing is readily achievable, environments are friendly for proteins, separation can be made selective and rapid, and it has been proven to be more economical than most of other separation processes (Diamond and Hsu, 1992). However, since there are many factors affecting the partitioning of proteins in APTE (system inherent factors such as choice of polymers and salts, polymer molecular weight, concentration of polymers, salts and pH; and protein inherent factors such as molecular mass, charge and surface hydrophobicity), extensive studies need to be carried out in order to apply this technique to various plant expression systems for recombinant protein separation. Processes for conversion of bio-resources to useable products typically rely on the use of specific enzymes and/or microorganisms, primarily for the conversion of complex carbohydrates (starch, cellulose, etc.) to other more valuable compounds. A major drawback to the commercialization of these processes has been the lack of adequate supplies of the desired enzymes at a reasonable cost. Although enzymes in industrial quantities have become more available in the past decade, growth has been primarily in the area of proteases, where there already exists large markets in detergents. There has been limited growth in the production of carbohydrate cleaving enzymes. Although amylases are readily available, because of high volume use in the sweetener industry, commercial amounts of other carbohydrases are in short supply. Support should be given to the development of high efficiency production systems for enzymes that will be needed to transform the bio-refinery concept a reality. E. Education and Outreach The Final Report of the 2001 National Workshop on Biomass Education and Outreach identified several action items needed to meet the training needs of a fledging biobased economy (Energetics, 2001). Highest-priority action items for universities included holding workshops and developing virtual academic programs related to biomass education. Toward this end, education and outreach tasks involving development of advisory boards, and creation of targeted education programs and materials are suggested. Such activities are recommended to capitalize on the traditional strength of universities in developing effective educational programs, while integrating expertise from industry and agricultural producers to ensure relevance. Emphasis should be placed on developing educational materials in a format suitable for distance education (e.g. internet offerings) to facilitate broad dissemination.
Objectives
-
Reduce the cost of harvesting, handling, storing and transporting biomass increasing the competitiveness of biomass as a feedstock for biofuels, biomaterials and biochemicals.
-
Expand the scientific knowledge leading to significant economic improvements in biofuel production processes.
-
Develop, evaluate, and optimize integrated processes to convert biomass resources into biomaterials with commercial applications.
-
Expand the scientific knowledge for development of processes and systems for economical production of biobased speciality chemicals from agricultural feedstocks and residues.
-
Identify needed educational materials, developing those materials in distance based delivery methods and developing a trained work force to support a biobased products industry.
-
Methods
Objective 1. Reduce the cost of harvesting, handling, storing and transporting biomass increasing the competitiveness of biomass as a feedstock for biofuels, biomaterials and biochemicals.
Task 1. Feedstock quantification and characterization.
Work in this area will seek to quantify the amount and quality of biomaterial that can be collected using innovative collection equipment and techniques including development of precision farming tools for biomass management.
Work at NDSU and NE will concentrate on development of portable/field scale sensors for rapid, non-destructive and accurate characterization and quantification of feed stocks. This work will target development of intelligent and non-destructive sensing techniques for portable/on-the-go sensors for rapid and quick quantification as well as characterization of different desirable attributes of feed stocks.
Task 2. Harvest, process and handling.
The overall objective will be to deliver premium quality feedstock at a low cost to bioconversion facilities New techniques for increasing the bulk density are also critical to the success of the bioenergy systems. High density granulated biomass can be handled using conventional bulk handling equipment thus making handling of the biomass safe and inexpensive.
Researchers in California and NE will target 1) development of improved on-combine attachments for cutting and handling of residue to reduce the total number of equipment operations required in biomass harvesting and to improve the yield and quality of biomass, 2) characterization of field and economic performance of alternative grain and biomass harvesting techniques used to replace the combine harvester, 3) evaluation of techniques to vary the composition and quality of the biomass by adjustments to the harvesting system to yield different plant fractions, and 4) identification of safe storage techniques for biomass at different harvest moisture levels. Work in KY will focus on collection of corn stover and investigations into the opportunities of using precision ag to harvest specific areas of a field and changes during storage in quality and dry matter
Workers in Tennessee and NE will attempt to 1) experimentally determine the energy requirements for densifying biomaterials using pressure compaction and friction compaction, 2) quantify material characteristics, i.e., particle size, moisture content, chemical composition, and their effect on quality of compacted material and energy requirement for compacting, 3) identify process parameters, i.e., temperatures, steam quality, pressures, and hold time and their effect on quality of compacted material and energy requirement for compacting, 4) develop and validate an engineering mathematical model to be used for analysis, and optimization of compacting machines and processes.
Task 3. Modeling integrated feedstock supply and process systems. Workers in Tennessee will conduct sensitivity analysis on the cost of feedstock collection and quantify the magnitude of change in the collection cost of residue due to change in operational variables (yield, density, hours of operation, speed), investigate the current logistics of harvesting and processing operations for high density herbaceous and woody products as potential models for feedstock supply logistics, and development of operational research data (performance, service, timeliness) for field operations and for off-field operations applied to feedstock handling.
Workers in South Carolina will develop the database required to evaluate the potential of using animal manure to produce biomass crops, develop a model of an energy farm that would include animal production, manure treatment to mineralize organic nutrients (i.e. anaerobic digestion), utilization of plant nutrients to produce biomass energy crops, dewatering and drying of indigestible solids for biomass energy, and develop a model to be used to determine the economically optimum manure handling, treatment, and utilization options for energy biomass production on swine, dairy, and poultry farms.
Objective 2. Expand the scientific knowledge leading to significant economic improvements in biofuel production processes.
Task 1. Pretreatment for bioconversion processes.
Under this task, physical, chemical and biological pretreatments will be investigated to increase the ease of enzymatic digestion (FL, KS, MI, MN, NC, TX, WA). These methods will explore the full spectrum of process options: grinding and chopping, thermal/pressure treatments with acid or base, biological treatment with fungi, and chemical fractionation.
Task 2. Biological conversion processes.
Bioconversion of lignocellulose to ethanol and other chemicals has two distinct components: 1) production of enzymes for the depolymerization of cellulose (and hemicellulose) into soluble saccharides which can be metabolized by microorganisms; and 2) development of improved biocatalysts to convert these soluble saccharides into fuel ethanol. Research groups in AR, FL, KY, NC and WA will compare the production of fungal, bacterial, and archaeal (extremophile) cellulase/hemicellulase enzymes by both native and recombinant organisms. Montana State University will develop a bioreactor to convert glycerol to usable alcohol products for recycling into the further biodiesel production or utilization as biofuels.
Additional studies will focus on the development of improved biocatalysts for the conversion of saccharides into fuel ethanol and other bulk chemicals (FL, GA). Workers in FL will develop strains of Zymomonas mobilis with an enhanced potential for genetic and metabolic engineering for fuel ethanol production, and will be involved in the area of optimizing existing biocatalysts and generating new biocatalysts for the conversion of sugars to ethanol.
To improve the economics of ethanol production from renewable resources, UIUC will quantify the composition of byproducts and process streams from several corn processing plants. The research group at the University of Kentucky will evaluate the feasibility of producing plant carbohydrate-degrading enzymes in solid state culture using anaerobic thermophilic bacteria.
In California, thermophilic batch and fed batch anaerobic digestion processes will be studied for converting high solids agricultural residues into energy. Cooperators in California and South Carolina will target efforts on development of fermentation processes for conversion of micro algae biomass into hydrogen and methane biofuels.
Task 3. Development of improved thermochemical processes for biofuel production.
Three basic thermochemical conversion approaches to be evaluated include direct combustion (CA, NE), gasification (CA, OK, NE) and direct conversion to liquid fuel (WV). Problems of fouling, slagging, and boiler efficiency associated with co-firing biomass with coal will be studied (CA). A close-coupled fluidized bed biomass gasification and direct combustion system will be investigated for reduction of NOx emissions through reburning (CA). Other gasification studies will optimize production of syngas for use as a substrate for fermentation to fuel alcohol (OK). The reaction of biomass with water under conditions of high temperature and high pressure will be optimized with respect to reaction time, temperature, and water content for different types of biomass (WV).
Objective 3. Develop, evaluate, and optimize integrated processes to convert biomass resources into biomaterials with commercial applications.
Task 1. Raw feedstock evaluation.
KS and NE will characterize the rheological and thermal behaviors of starch and protein polymers as affected by their structure. SC investigators will work to evaluate traditional farm equipment for flax production and to develop and test an enzyme-retting method to replace dew-retting. Scientists from MN will pretreat lignocellulosics, followed by low temperature liquefaction to generate a mixture of hydroxyl-rich lignin and carbohydrate residues. Solutions from liquefaction will be cast to form high strength edible films or coatings (NE).
Task 2. Methodologies for producing biomaterials.
UIUC scientists will investigate a modified dry grind ethanol technology to make corn ethanol production more efficient. Scientists from KS will develop cost-effective, formaldehyde-free, protein based adhesives for producing plywood and agricultural fiber particle boards and will evaluate the adhesives for use in commercial construction
Workers in South Carolina will evaluate, adapt and further develop a recently patented algal culture technology for production of high value aquatic plant and animal biomass.
Task 3. Biomaterial applications
KS and NE will investigate the chemical and mechanical properties of blends of polylactic acid (PLA) and other commercially available biodegradable polymers with industrial grade starch, and will seek to enhance their compatibility to produce low cost, durable, and degradable plastics.
SD scientists will evaluate the mechanical properties (strength, elasticity, flexibility, etc) of microbial exopolysaccharides (gellan, scleroglucan, and polyhydroxyalkonoate) produced by fermentation of corn byproducts. SD scientists will evaluate the use of C-4 (warm season native grasses, i.e. switchgrass, big bluestem, etc.), soybean hulls, and other natural fibers as reinforcing fiber sources for use in blended plastic composites.
Objective 4. Expand the scientific knowledge for development of processes and systems for economical production of biobased speciality chemicals from agricultural feedstocks and residues.
Task 1. Biochemical Identification, Characterization and Separation from Biofeedstocks
(WA) Chemical analysis will be conducted to determine detailed composition of dairy manure in terms of carbohydrates, proteins, and minerals.
Botanicals and nutraceuticals often regulated through the Dietary Supplement Health Education Act. At present, the authorities are struggling to ensure that the botanical and nutraceutical products available to the consumers do not contain harmful components. Workers in AR and NE will study the botanical and nutraceutical extraction systems. Extraction of speciality chemicals will be scientifically documented, similar to that of edible oils, sugar, coffee and gelatin extractions.
Researchers in Hawaii will develop economical and efficient extraction methods for nutraceuticals and other bioproducts using supercritical fluids and pressurized fluids. A successful extraction system will allow selective components to be extracted in minimum volume to simplify and facilitate the subsequent purification, characterization and bioactivity screening. Metabolomic and proteomic profiling will be conducted, in conjunction sequencing and bioinformatic platforms, to elucidate metabolic pathways and significant biological and environmental regulating factors.
Investigations on how to separate or extract silymarins from milk thistle seed matrix and wax, tocols, phytosterols and polieusanols from grain sorghum will be conducted. The extraction techniques that will be evaluated consist of: traditional leaching, supercritical fluid extraction and subcritical water extraction. To evaluate extraction conditions, diffusion coefficients will be determined, setting the stage for the scale-up of possible extraction strategies. Compounds will be authenticated by Mass Spectrometry and routinely analyzed by High Pressure Liquid and Gas Chromatography.
Task 2. Process Development.
Extraction and purification of biobased chemicals, such as PUFAs and enzymes, from raw and bioconverted agricultural byproducts using superficial fluid extraction and adsorption techniques will be studied at lab and pilot-scale (LA). Enhancement of the extraction and fractionation using enzymes, adsorbents or co-solvents will be pursued. Adsorbents will be developed from agricultural wastes, such as rice hulls and shellfish waste. Steps to the design include determination of growth, decay and product formation kinetics, selection of reactor configuration (batch vs CSTR, simple CSTR vs cell recycle system) based on simulations, design optimization to maximize product formation rate or product concentration, and finally lab-scale and pilot-scale reactor operation based on design.
Investigators in Washington State will evaluate the hydrogenation process using a semi-batch reactor system equipped with continuous hydrogen feed in collaboration from the Pacific Northwest National Laboratory (PNNL) located in Richland, Washington. Products from the reactor will be analyzed by chromatography
Investigators in Washington will also study processes for lactic acid production from agricultural feedstock. The initial investigation will focus on using cull potatoes. Specific research topics include (1) developing simultaneous scarification and fermentation (SSF) process for lactic acid production with Rhizopus oryzae, (2) evaluating the performance of suspended and immobilized reactors with biomass recycle and retention, (3) improving product separation, and (4) optimizing the system efficiency based on SSF kinetics, and reactor and separation process modeling. Workers in KY will study foam fractionation, which takes advantage of the surface-active characteristics, hydrophobic/hydrophilic nature, of the protein of interest, as a means to recover, separate, and/or concentration proteins and enzymes.
Innovative catalytic processing approaches will be developed that convert dissolved sugars into a variety of valuable chemical products (WA). These processes utilize aqueous-phase catalysts to perform hydrogenation or hydrogenolysis reactions under mild thermal conditions of 100 150 oC at elevated pressures.
The University of Arizona will be involved in: (1) enhancement of extraction of biobased chemicals from raw and bioconverted agricultural byproducts using adsorbents and co-solvents; (2) determination of growth, decay and product formation kinetics; (3) selection of appropriate reactor configuration; and (4) lab-scale reactor operation based on design.
The applicability of using aqueous two-phase extraction (ATPE) in isolating recombinant proteins from various plant expression systems (such as tobacco and corn) will first be studied at lab-scale. The purification of a particular model protein from a plant system will be optimized, and the process will be scaled-up to demonstrate the scalability. Moreover, processes for the purification of a model protein will be developed based on either initial ATPE isolation or traditional protein extraction and initial column chromatographic isolation to achieve the typical protein purity required for biopharmaceuticals. The economics of the processes will be evaluated to examine the suitability of using ATPE in protein purification from plant expression systems.
Task 3. Product Application.
Odor and visual quality (color, clarity) of PUFA-enriched oil produced from fungal fermentation will be evaluated using headspace analysis using GC/MS and/or sensory panels and colorimeter measurements. These oils will be compared to currently-marketed oils extracted from fish and vegetable products (LA).
Investigators at Virginia Tech will characterize fractional pyrolysis products using GC-MS, HPLC, FTIR, and NMR. The fractional pyrolysis product will be used as phenol substitute in the preparation of phenol-formaldehyde type of resins. Researchers in NE will access the suitability of wax recovered from grain sorghum distillers dried grains (DDG) in a cosmetic product, such as lipstick, compared to other commercial waxes. Preliminary work already completed at NE will serve as the basis for the process of production and analysis.
A unique fractional catalytic pyrolysis technique being developed at Virginia Tech will be further investigated for the production of phenols and cresols from biomass feedstocks, which would be used as phenol-formaldehyde adhesive substitute (Virginia Tech. Worker in LA will use agricultural residues (bagasse, rice straw, corn stover, etc.) and plant fibers (kenaf, flax, etc.) to produce biodegradable mats and composite materials. And also will promote end-use applications of these biobased
materials in diverse industrial sectors.
Objective 5. Identify needed educational materials, develop those materials in distance based delivery methods and develop a trained work force to support a biobased products industry.
Task 1. Development of an Advisory Board for the National Resource Center.
An NRCBE Advisory board will be established, whose makeup represents the major stakeholders in the biobased economy, including representatives from the biobased-products industries as well as agricultural producers. The roles of the Advisory board will be to advise NRCBE staff on topics for which educational materials are needed, to help assess educational materials and trained students, to help arrange educational enrichment opportunities such as student internships and faculty sabbaticals, and to help place trained students in biobased industries.
Task 2. Development of Educational Materials in High-Priority Topic Areas.
New, multi-disciplinary, educational materials will be developed to widely disseminate knowledge related to biobased products via distance-education methods. Examples of relevant technical topics include an overview of engineering principles for scientists, an overview of biotechnology principles for engineers, modeling of biological systems, sustainable chemical processing, bioseparations, and bioprocess engineering and design. In addition, courses will bedeveloped to teach professional skills of particular relevance, including effective methods for distance education, and skills for working in multidisciplinary teams. The use of distance-educaiton technologies will also allow industrial expertise to be more fully integrated into the training process. For instance, videoconferencing will enable virtual collaborations to be conducted between academic and industrial experts, regardless of physical proximity.
Task 3. Development of National Resource Center for Biomass Education.
A National Resource Center for Biomass Education (NRCBE) will be established to facilitate development of a trained work force for the biobased products industry. Anticipated services to be provided by the NRCBE include identifying key areas in which training materials are critically needed, encouraging experts to create training materials in key areas, fostering educational collaborations between experts having complimentary expertise, organizing workshop/training on effective delivery methods for distance education, assisting in assessment and quality assurance of biomass-related training materials, serving as an information clearinghouse for biomass-related training materials interfacing with other organizations involved in higher education (ASEE, USDA, DOE), and organizing short courses and conferences related to biomass education.