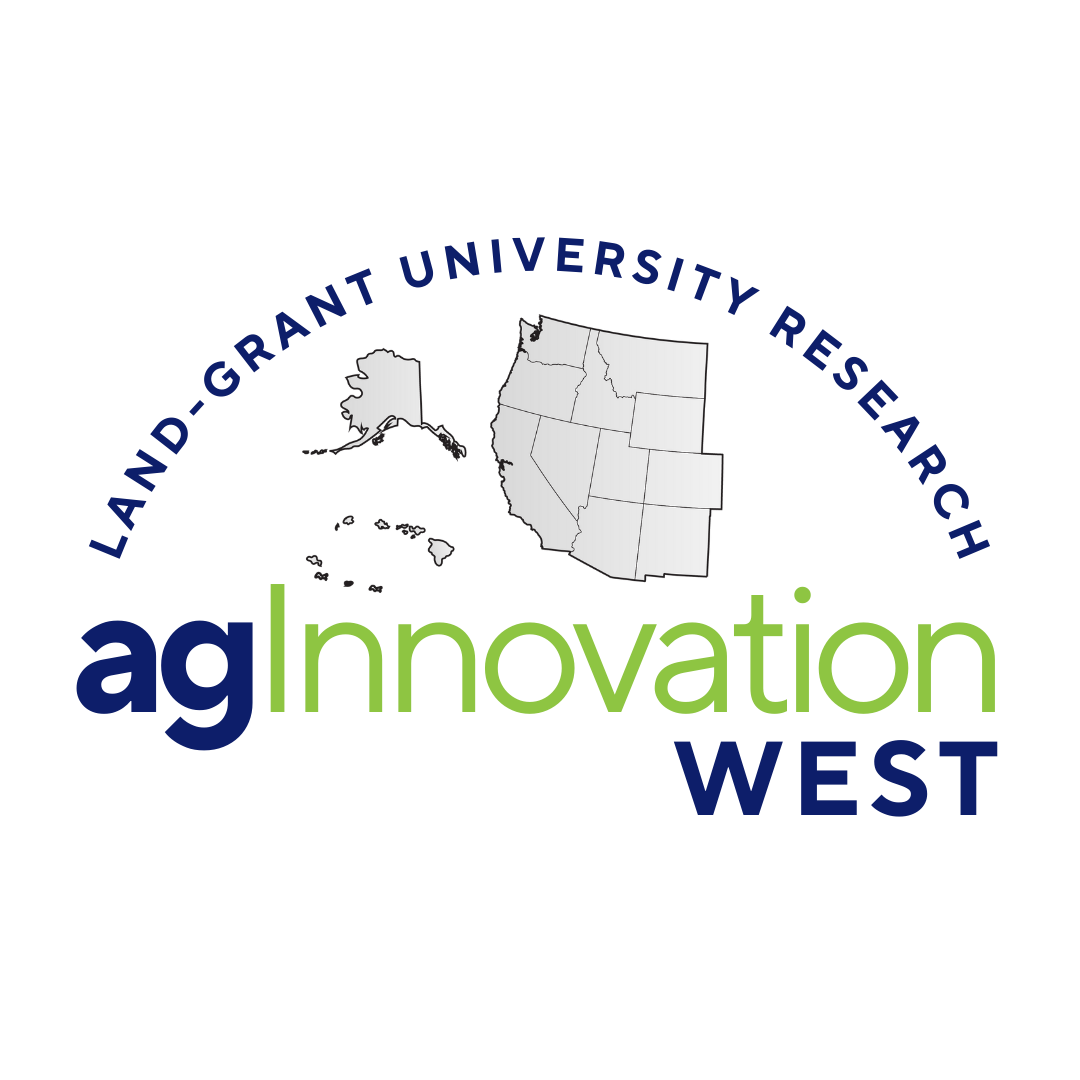
W45: Mechanisms and Mitigation of Agrochemical Impacts on Human and Environmental Health
(Multistate Research Project)
Status: Inactive/Terminating
W45: Mechanisms and Mitigation of Agrochemical Impacts on Human and Environmental Health
Duration: 10/01/2000 to 09/30/2005
Administrative Advisor(s):
NIFA Reps:
Non-Technical Summary
Statement of Issues and Justification
Economically and environmentally sustainable agriculture requires an integrated approach to pest management. Agrochemicals are one of many tools used to ensure an abundant food supply. Judicious use of agrochemicals demands practical knowledge of their fate and effects in agricultural and natural ecosystems. A mechanistic approach will allow for development of novel physical and biological analytical techniques to assist in ascertaining fate and effects. This new knowledge is required for the advancement of science-based management strategies which prevent or mitigate unacceptable adverse impacts on human and environmental health, the goal of the W-45 Committee. JUSTIFICATION: The U.S. EPA has estimated that almost 1.0 billion pounds of conventional agrochemicals (active ingredient) were used annually in the US in 1995. Use on agricultural cropland accounts for over three-quarters of the agrochemicals used annually in this country, with herbicides representing the largest (83%) percentage used in crop production. Use in the home and garden is ca. 9% of all agrochemicals used (Aspelin, 1997). Agrochemicals are initially distributed in the environment at application, with the intent of maximizing efficacy while minimizing off-site movement. The Food Quality Protection Act (FQPA) has de-emphasized efficacy and set stricter standards for acceptable risks to human and environmental health. Consequently, a better understanding of initial distribution and redistribution via airborne loss, run-off and leaching is necessary to adequately characterize both human occupational and non-occupational exposure, and assess risks to biota in surrounding ecosystems. Responsible use of soil, air and water resources for the production of food, feed and fiber must be balanced with the need to minimize impacts on human health, and preserve natural ecosystems and the biodiversity they support. As agrochemicals remain a cornerstone of pest management in U.S. agriculture, there is a continuing need to critically evaluate environmental transport processes and factors influencing fate in assessing risk and in the development of mitigation strategies. Research is needed which provides a mechanistic understanding of fate and effects, beginning at the molecular level, and including systems analysis of agroecosystems. Such efforts will allow to a greater degree science-based decision making as a basis for policy regarding the use of agrochemicals in U. S. agriculture. As no production system will be appropriate for every situation, farmers need a variety of sustainable production options. The ecological risks associated with the use of agrochemicals must be evaluated. The adoption of risk assessment and risk management strategies by state and federal regulatory agencies has resulted in more general recognition of the reality of unintentional and unavoidable agrochemical exposures by handlers, harvesters, and bystanders. Measurement of the extent of human agrochemical exposure is critical to evaluation of work practices, handling and application technologies, personal protective equipment, clothing, and strategies to reduce or minimize human exposure. Available models for the estimation of human exposure rely almost exclusively upon environmental monitoring data and human passive dosimetry. Those data are woefully inadequate due to the number of default assumptions which must be invoked to estimate or index absorbed dosage for improved risk management and risk communication. This W45 project focuses on developing tools to understand the impact, (both positive and negative) and devise mitigations when necessary or contemporary agrochemicals and related substances. The W-45 program was founded, and will continue, as a multidisciplinary venture, bringing together chemists, biologists, toxicologists and ecologists with studies ranging from the most fundamental molecular mechanisms to their applications in fields and farms. The information must be synthesized into meaningful better management practices utilizing laboratory and field experiments.
Related, Current and Previous Work
Research is needed to elucidate the processes of agrochemical function in order to maximize efficacy, determine more realistic exposure potentials to biota (including humans) from foliar, soil, water and airborne agrochemical residues, and develop exposure minimization strategies. Investigations are required to better understand the mechanisms of agrochemical loss processes and the influencing cultivation practices and environmental and matrix variables, such as temporal and diurnal terms, soil composition, moisture levels, sorption, organic carbon, solutes and abiotic and biotic transformation mechanisms. Low level chronic exposure effects will be determined, more appropriate biomarkers evaluated, and analytical methods with lower detection limits developed. Analytical Techniques. Supercritical (i.e., SC-CO2) and pressurized fluids can be used for extraction of non-polar or slightly polar compounds from complex media with considerable extraction selectivity and minimum solvent disposal, but are less effective in recovering polar molecules because of the properties of SC-CO2 and pressurized fluids (Chester et al., 1998; Westwood, 1993). Many polar solvents have been added in SC-CO2 to alter its polarity and subsequently to improve extraction efficiency of some polar herbicides from solid matrices (Reighard and Olesik, 1998). Derivatization of polar analytes or sorption sites in situ or off-line have received considerable attention (Hawthorne et al., 1992). Quantitative recoveries have been achieved for thirty polar chemicals in soils using SC-CO2 with assistance of (ethylenedinitrilo) tetraacetic acid tetrasodium salt (Na4EDTA) (Alcantara-Licudine et al., 1997; Guo et al., 1999). The procedure can also be used to recover neutral and nonpolar compounds from soils and has potential for the analysis of parent analytes and polar biomarkers simultaneously. Immunoassays are recognized as valuable alternatives to the conventional methods for monitoring agrochemical residues because they have a low detection limit, are highly specific, provide fast analysis, and are inexpensive, e.g., immunoassay analysis is $20-40 per sample compared to $150-500 per analysis for instrumental methods such as gas chromatography. (Aga and Thurman, 1997). Immunoassays and immunosensors have been developed for a number of pollutants including polycyclic aromatic hydrocarbons (PAHs) (Li et al., 1999; Liu et al., 1999a, b). Development of these methods will be invaluable for fast and large throughput monitoring of environmental samples. Weed Management. To predict and manage rangeland weed populations and to minimize negative impacts on surrounding biota characterization of crops, weeds, and herbicide interactions is needed. Broom snakeweed is a weed threatening livestock productivity. Research concerning the fate, action, and resistance of the rangeland herbicide, picloram, indicated that the extent and rate of adsorption by broom snakeweed foliar tissue was influenced by relative humidity, air temperature, solution pH, and additives (Sterling and Lownds 1992). However, herbicide differential sensitivity may be more strongly related to differences in picloram translocation or metabolism. Studies showed that picloram translocation did not parallel carbohydrate redistribution, thus, herbicide application in the fall, as is commonly hypothesized due to more herbicide being transported to the roots during the rainy season, may not improve efficacy. Furthermore, variable metabolism as a function of snakeweed species and stage of growth may be responsible for differences in whole plant sensitivity throughout the year (Hou and Sterling 1993). In other studies, the mechanism of picloram resistance in yellow starthistle may involve the auxin receptor sites in herbicide perception and signal transduction (Sabba et al., 1998; Sterling et al., 1998). Resistance was determined to not be a function of differences in foliar absorption, translocation, or metabolism (Fuerst et al., 1996). Oxidative stress is the major factor limiting plant productivity and results from environmental stresses which induce the production of active oxygen species capable of severe cell and tissue damage. Cotton tolerance variablity to the major herbicide prometryn, a photosynthetic inhibitor, is not due to differences in uptake, translocation, or metabolism or to differences at the site of action. Evidence suggests that the protective mechanisms against photo-oxidative stress, due to blocked photosynthetic electron transport caused by these herbicides, contribute to tolerance (Waldrop et al. 1996). This research will provide a basis for understanding the variation in biochemical and physiological strategies in cotton protecting it from herbicide photoinhibition. By understanding how plants avoid oxidative stress, crops will be better protected from stress and weeds better managed. Agrochemical Fate as Affected by Transport and Partitioning. Offsite movement, locally and globally, of agrochemicals can be a potential source of air, water, and soil pollution. Agrochemicals applied during the spring in the Chesapeake Bay watershed have been be measured in air and water throughout the year (Glotfelty et al. 1990; McConnell et al 1997). Regional agrochemical airborne transport from California's central valley and deposition to the Sierra Nevada Mountains has been reported (Zabik and Sieber, 1993; McConnell et al., 1998). More persistent agrochemicals, such as the organochlorine insecticides, have been found in the polar regions, areas quite remote from their application sites (Muir et al., 1990; Bidleman et al., 1990, Rice et al, 1998). Renewed interest exists in flux measurement research (Majewski et al. 1995), and the need to attempt mass balance determinations and to use multiple methods for flux estimations (Yates et al., 1996). During application, the active ingredient may move offsite as fine droplets (spray drift) particulates, or as vapor. The environmental fate of these forms can be distinct. Spray drift has been examined (Haq et al., 1983; Miller, 1993; Ware et al., 1970; Ware, et al. 1972) as well as the effect of temperature and humidity on droplet size (Freiberg and Crosby, 1986; Sundaram, 1985). However, comparatively little work has focused on the volatilization of active ingredient directly from spray droplets. For agrochemicals with low volatility, direct evaporation of active ingredient from spray droplets is expected to play a minor role compared to droplet drift but maybe significant on a regional scale. When the chemical remains within a droplet, the droplet may eventually leave the atmosphere by impacting a surface. Little information is available on the factors influencing these mechanisms or how they might be altered to improve on-target deposition. Distribution of agrochemicals by volatilization is largely controlled by the physical and chemical properties of the agrochemicals. These include vapor pressure and solubility (Henry's Law constant), organic carbon partition coefficients, and degradation rates (Jury et al.,1984a, 1984b, 1984c; Robbins 1993; Staudinger and Roberts 1996). Determination of Henry's law constants over a temperature range have been conducted (Sagebiel et al., 1992) although few studies actually examine the effect of aqueous parameters on the air/water partition coefficients (Rice et al. 1997, Staudinger and Roberts 1996) which can influence fate and transport predictions. Soil fumigants are relatively toxic to a broad spectrum of organisms and their high vapor pressures result in significant atmospheric loss. Research has focused on determining rates of volatilization and degradation under various conditions and the use of impermeable tarps following fumigation to reduce volatile losses. Preliminary results indicate that fumigant application rates can be reduced when using virtually impermeable films (VIFs) because the fumigant is held in the soil at higher concentrations for a longer period (Gan et al., 1996). Additional research is needed to develop management practices using VIFs to ensure low emissions and sufficient pest control. Agrochemical sorption to, and interactions with organic carbon, clays and minerals can alter the structure, reactivity, bioavailability, solubility, partitioning, and, ultimately, transport (Brusseau et al., 1991a, 1991b; Brusseau and Rao 1991; Gamerdinger et al., 1991; Hassett et al., 1985; van Genuchten and Waganet 1989; Wu and Gschwend 1986). In addition, the elution of freshly added agrochemicals from soil has been reported to be far greater than in aged samples (Pignatello et al., 1993). Current research result cannot adequately predict sorption. Runoff and erosion from agriculture have been identified as major contributors to water quality degradation. The U.S. EPA estimates routine agricultural activities are responsible for more than 60% of the nations surface water pollution problems (USEPA 1998). Approximately 1 to 6% of applied agrochemicals may be transported off-site by runoff and drainage depending on the slope of the field, management practices, presence or absence of subsurface drains, and the quantity and timing of rainfall after application (Bengtson et al., 1990; Leonard, 1990). Plastic mulch vegetable production systems use extensive amounts of agrochemicals and also have a greater potential for off-site movement of agrochemicals during rain events due to the use of an impervious surface. During an intense rain event, a large pulse of runoff water can quickly move into surrounding surface waters where agrochemicals in the runoff can cause potentially acute toxic effects on aquatic organisms (Rice et al, 1999). Other studies have demonstrated significant negative effects of agrochemicals on aquatic plants (Forney and Davis, 1981; Jones and Winchell, 1984) and other organisms (Clark et al., 1993; Savitz et al., 1994; Scott et al., 1990; Scott et al., 1992). Research is needed to develop technologies to mitigate these negative impacts. Transformation Processes - Photolysis. Environmental photolysis can proceed either by direct photolysis or indirect photolysis where another matrix component absorbs the solar light energy and then interacts with agrochemicals, causing decomposition via energy transfer (sensitization), direct electron transfer or other free radical processes (Zafiriou et al., 1984; Draper and Wolfe 1987; Miller and Hebert 1987). Photolytic degradation can occur in the atmosphere, in aqueous systems, within the first millimeter of soil, or on foliar surfaces. DOM (dissolved organic material), NO3 anion, and other compounds can serve as sensitizers (Zepp et al., 1981, 1987) or precursors for the production of singlet O2 (Faust and Hoigne, 1987; Haag and Hoigne 1986; Zepp et al., 1977), peroxy radicals (Faust and Allen 1992), H2O2 (Draper and Wolfe 1983), e- and OH radical (Draper and Wolfe 1981; Haag and Hoigne 1985; Faust and Allen 1993). Alternatively DOM can alter the rate of agrochemical degradation by absorbing the available light energy (Hapeman et al., 1998; Hoigne et al., 1989; Kochany and Maguire 1993; Torrents et al, 1997). Understanding the competing mechanisms is required to accurately assess the fate of agrochemicals in systems that are subjected to solar irradiation. While atmospheric chemistry is a relatively mature science for small molecules, the atmospheric fate of medium weight organic chemicals, particularly agrochemicals, is not well understood as maintaining stable agrochemical concentrations in the gas phase is difficult. Chemicals with vapor pressures less than 10-3 torr sorb on the walls of chambers and the observed chemistry is complicated by reactions on the walls. Thus, risk assessments of many agrochemicals rely on incompletely tested computer models for reactions. Both the short and long range transport of agrochemicals has been firmly established and currently represents a significant risk to humans and the environment (Majewski and Capel, 1995) Methods to assess the gas phase reactions of volatile compounds with hydroxyl radical are available (Atkinson, 1986) which utilize several compounds as stable tracers with known hydroxyl radical reactivity. Nevada scientists are continuing to develop methods for applying these methods to agrochemicals using special temperature controlled chambers and solid phase microextraction devices to reliably establish and measure pesticide gas phase concentrations (Hebert, et al., 1999a, 1999b). These methods need to be applied to many of the major agrochemicals for which risk assessments are complicated due to the paucity of data concerning their atmospheric dagradation. Initial studies have shown that herbicide photodegradation rate on leaf surfaces is influenced by epicuticular wax and the plant species. Additional photodegradation products on wax surfaces were detected relative to photodegradation on glass surfaces only (Maynard and Sterling 1996). Thus, herbicide not absorbed by the plant is subject to photodegradation, reducing plant exposure to the toxic parent herbicide and limiting product efficacy. - Other Abiotic Processes. Agrochemicals that are susceptible to environmental hydrolysis generally include esters, carbamates, organophosphates, and activated amides. Hydrolysis typically occurs in the presence of an acid, base or heterogenous catalyst usually in aqueous environments in the atmosphere or soil or at the catalytic surface. Dechlorination of the chloro-s-triazines can also be described as a hydrolytic reaction, typically occurring under acidic conditions or in the presence of an acid catalyst (Gamble and Khan 1985, 1990; Gamble et al., 1983). This process was also thought to be a hydroxy radical process but recent studies have shown that direct photolysis is more likely to be responsible for hydroxyatrazine formation (Torrents et al., 1997). Understanding the preferred degradation pathway and the environmental factors that influence transformation is required to make reasonable risk evaluations. Enhanced abiotic and biotic degradation of soil fumigants has also been observed in soils to which organic amendments have been applied. Many soil fumigants, which contain halogenated alkyl groups, can undergo abiotic degradation via nucleophilic reaction. This reaction mechanism likely occurs in unamended soil (with nucleophilic groups on soil organic matter acting as the nucleophile) and is accelerated in soil treated with nucleophilic compounds. Thiosulfate compounds, such as ammonium thiosulfate, an S fertilizer, were found to be capable of rapidly transforming several fumigants into water soluble products (Gan et al, 1998) and reduce fumigant atmospheric emissions. Optimization of this management technique demands elucidation of mechanisms and control factors. - Microbial/Enzymatic Processes. Microorganisms clearly play a vital role in the degradation and transformation of agrochemicals in soils and water (Alexander, 1999). Enhanced degradation of soil fumigant compounds observed in soils receiving repeated applications has been attributed to an increase in the rate of biological degradation although the mechanisms underlying enhanced degradation remain largely remains unexplored (Alexander, 1999; Ou, 1999). Extensive information on degradation rates of agrochemicals in root-zone soils has been generated, but much less in vadose-zone soils. . Indigenous soil and water-borne bacteria can degrade agrochemicals and may prevent carry over to next growing season, leaching into the groundwater, and volatilization into the atmosphere. Ammonia and methane oxidation bacteria has been utilized to degrade the fumigants in soil (Ou et al., 1997; Oremland et al., 1994). A strain of Arthrobacter sp. capable of extensively degrading 1,3-D, a possible alternative to methyl bromide, has been isloated from an enhanced soil. This organism can be used potentially to degrade 1,3-D from soil after fumigation is completed. This rapid degradation of the chemical will minimize volatilization into the atmosphere and percolation into the groundwater. For some agrochemicals, toxic metabolites, which have similar activity and toxicity as their parent chemicals, may accumulate in soils. For example, aldicarb sulfoxide and aldicarb sulfone, and fenamiphos sulfoxide and fenamiphos sulfone are formed in soils treated with aldicarb (Ou et al., 1985) and fenamiphos (Ou et al., 1994), respectively. Thus, it is more accurate to include these toxic metabolites for estimation of degradation rates as total toxic residue disappearance rates (parent chemical + toxic metabolites). Agrochemical Toxicity - Organophophates (OP), Carbamates, and FQPA. OP and carbamate insecticides are designed to inhibit acetylcholinesterase (AChE), the enzyme that normally hydrolyses the neurotransmitter acetylcholine in nerve and muscle tissues. In the presence of an OP, synaptic acetylcholine levels may rise to abnormally high levels resulting in repetitive stimulation of muscarinic and nicotinic receptors in target tissues. At sufficiently high exposure levels, this produces clinical signs of acute cholinergic poisoning. Recovery is possible if the individual is removed from further exposure, allowing OP clearance from the body and a return to normal levels of synaptic acetylcholine. Long-lasting neurological damage from acute high level exposure to some OPs is well documented (Karczmar, 1984). OP and carbamate insecticide fate in orchards has been primarily concerned with worker reentry hazard associated with foliar dislodgeable residues, soil, and soil dust residues because of their acute mammalian toxicity. Most of this work has been done for citrus (Spear et al., 1975; Thompson and Brooks, 1976; Gunther et al., 1973, 1977; Nigg et al., 1977; Iwata et al., 1982, 1983; Nigg et al., 1984), but studies have also been conducted on peach (Winterlin et al., 1975; Hansen et al., 1978; Spencer, et al, 1991, 1993, 1995) and apple orchards (Staiff et al., 1975; Hansen et al., 1978). Dislodgeable residues and worker exposure in strawberries has been studied (Winterlin et al., 1984; Zwieg et al., 1983, 1984; Popendorf et al., 1982). Few studies report monitoring of both dislodgeable and airborne residues (Winterlin et al., 1986, Jenkins et al., 1990). Another emerging area of interest is the potential for exposure to agrochemicals from turf (Goh et al., 1986, Sears et al., 1987, Cooper et al., 1990; Jenkins et al., 1990, 1991). From these studies and others, protective clothing requirements and reentry intervals have been established for farm workers. Despite these precautions, a heightened awareness exists for the health hazards to any person who might be exposed before, during, or after agrochemical application. This concern is due, in part, to current risk assessment guidelines under the (FQPA) that require cumulative risk from all sources of exposure (dermal, inhalation, dietary, drinking water) be considered. For those agrochemicals with a common mode of action, such as the OPs and carbamates, aggregate risk must also be considered. Also, the increased use of Integrated Pest Management (IPM), which often requires frequent human (consultants, scouts, etc.) exposure to the orchard environment, has increased the number of individuals who are potentially at risk. It is these individuals, as well as the general public, who are at the greatest risk as they are often unaware of the hazard of inhalation or dermal contact with pesticide residues and do not take the appropriate precautions. To determine exposure to specific classes of organophosphate and carbamate chemicals, California and other states require or recommend blood cholinesterase monitoring for mixer-loaders, applicators and farm workers. Methods have progressed from manometric determinations through pH assays to thiocholine ester (Ellman et al, 1961, Metcalf R.L, 1951 ; Wills, 1972). Blood cholinesterase activity does not appear to be involved in neurotransmission (the function of cholinesterases present in the blood is not known), but inhibition of these enzymes is used as a biomarker of exposure to OPs. Although a number of field studies of blood cholinesterase levels exist, the test accuracy has not been scrutinized until recently. Major inadequacies in two major commercial kits used by clinical testing laboratories have been indicated, one uses a pH and a substrate concentration that is 40% from optimum and the other uses an inappropriate substrate (Wilson et al, 1997). This has prompted a change in state regulations requiring optimum assay conditions. OP exposure can also be monitored by the presence of dialkylphosphate metabolites in urine (Drevenkar et al., 1991; McCurdy et al., 1994). While this method can detect low level exposure, the rapid elimination of urinary dialkylphosphate metabolites makes them useful for assessing only recent exposures (less than 48 hours) (McCurdy et al., 1994). Biological samples often become available only days or even weeks after exposure. Analogous procedures were recently utilized in persons who treated their residences with insecticides in the IPM of fleas. These studies with chlorpyrifos as a model compound have shown that normal exposures are low (less than 6 ug/kg) but more prolonged than predicted by available hypothetical exposure models. Clearance is maximal during the first week and declines during the subsequent weeks to levels that are at or similar to background. No adverse effects have been reported during these studies, but the availability of the indoor residue has been substantially clarified (Kreiger unpublished results). Possible consequences of chronic low-level exposures to OPs are not well characterized. Several studies have found significant behavioral changes in animals dosed with OPs at dosage levels that produced little or no cholinesterase inhibition (Hart, 1993; Kurtz, 1977; Roney et al., 1986;Dutta et al., 1993;). OPs are often applied two or three times over several months, and workers may enter treated areas more than once after these applications presenting repetitive worker exposure risks. Available OP exposure monitoring methods have limited use for detecting chronic, low-level exposure. Wide individual variation in blood cholinesterase activity requires pre-exposure testing and limits its use as a quantitative biomarker of internal dose expecially at low exposure levels (Sanz et al., 1991). - Other Agrochemicals. Some agrochemicals used currently and in the past are known to induce oxidative stress as part of their mechanism of action. Cadmium, cyanide, and mercury containing agrochemicals, for example, have been shown to induce oxidative stress (Vincent et al., 1989, Manca et al., 1991 Younes and Strubelt, 1988, Pritsos, 1997; Stacey and Kappus, 1982, Hoffman and Heinz, 1998). Cadmium has also been identified as a lung carcinogen in humans (IARC, 1993) and may be associated with hormonally mediated cancers by interactioning with steroid receptors (Antila et al., 1996; Garcia-Morales et al., 1994). Arsenic and cyanide based agrochemicals have been shown to interfere with normal mitochondrial function, inducing oxidative stress (Maupoil and Rochette, 1988, Kehrer et al., 1990). Lead-arsenal agrochemicals can affect cognitive development in children and renal function in adults (Goyer, 1989; Goyer, 1990). While many of these compounds have been studied in terms of their acute lethal toxicities, little work has been conducted to determine the biological effects of either acute or sub-chronic ingestion of non-lethal dosages. Acute biological effects of mitochondrial damaging and oxidative stress inducing agrochemicals on target and non-target organisms have been examined. Studies were conducted using potassium cyanide and sodium arsenate as model agrochemicals, rats for examining effects on a targeted organisms, and mallard ducks as a model wildlife, non-targeted organism. Mitochondrial dysfunction with subsequent tissue energy (ATP) depletion due to cyanide exposure was shown in various tissues of both rats and mallard ducks (Pritsos, 1996; Pritsos and Ma, 1997). The mallard duck was much more sensitive to cyanide than the target organism (rat). ATP levels in the mallard duck were restored to normal within 24 h of exposure and that rhodanese and 3 mercaptopyruvate sulfur-transferase were induced (Ma and Pritsos, 1997). In other studies, increased flight times of homing pigeons were observed with exposure to increasing cyanide concentrations apparently due to loss of ATP (Ross and Pritsos, unpublished work). Widely used herbicides, including the triazine herbicides atrazine, simazine and cyanazine, have been put under Special Review by EPA because experimental animal studies have shown they can cause mammary carcinogens and therefore may pose a potential cancer risk in exposed populations such as farm workers (Stevens et al., 1994; USEPA, 1994). Evidence also exists that the parent compound and degradation by products persist in the soil and low residue levels have been detected in ground and surface water in high use states (Kolpin et al., 1997; Koplin et al., 1998; Solomon et al., 1996; Thurman et al., 1998; Wall et al., 1998). Critical evaluations, comprehensive reviews and in depth evaluations of the existing published and unpublished scientific literature on relationships between specific agrochemicals and the risk of breast cancer and other cancers have been developed in concert with USDA/CSREES grant #97-34369-4005. Copies have been made available to scientists at academic institutions and federal agencies. Previously prepared technical reports on triazine herbicides (atrazine, simazine, and cyanazine) have had widespread use by prominent scientists and policy makers including the Carcinogenicity Peer Review Committee at EPA, Agency for Toxic Disease Substances Registry, and the National Institute of Environmental Health Sciences. Translation of these critical evaluations into multiple formats for the non-scientist (fact sheets and tip sheets) in both print and electronic formats (http://www.cfe.cornell.edu/bcerf/) ensures distribution to diverse audiences, including the general consumer, underserved rural populations, health and extension educators, cancer survivors, advocacy groups, grant administrators, policy makers and media representatives. Discerning Biomarkers for Determining Exposure. Some agrochemicals have been implicated as endocrine disruptors affecting the reproduction of animals including the human. For example, methoxychlor, widely used for pest control in tomato production and a component of rose dust, has been identified as an environmental estrogen mimic adversely affecting experimental animal reproductive function (Cummings, 1997; Cummings and Perreault, 1999; Danzo et al., 1997). Fecal steroids biomarkers have been used to study the reproductive state in apes, mammals, birds and even prehistoric humans and typically involve radioimmune assays (Wilson and LeBlanc, 1998; Tell, 1997; Whitten, 1995 ; Cockrem and Rounce, 1994, 1995). Recently, male and female steroids ELISA antibody assays have been developed using animal feces (Tell, 1997; Cockrem and Rounce, 1995). Such non-invasive assays are especially important when dealing with animal welfare issues and when studying wildlife, particularly endangered species (e.g., Velloso et al, 1998). An antibody to testosterone and a method to study it in feces of rodents have been developed (Billitti et al, 1998). Studies of the effects of OPs and other agrochemicals on the nervous systems and reproduction of domestic and experimental animals, wildlife and humans are in progress. The results suggest the techniques will provide useful biomarkers for studying the effects of chemical and environmental stresses. A combination of cadmium and an organophosphate was more toxic to nerve cell cultures than either chemical alone (Coatnery and Wilson, 1996). Additional studies are required to determine the combined effects on the developing nervous system of birds. Hemoglobin adducts specific for OP exposure would be useful biomarkers for monitoring OP exposed individuals because of sampling ease, dose-dependent covalent binding, and long biological lifetime (120 days in humans) (Schell, 1994). Because red blood cells have a long biological lifetime, hemoglobin contained within them can integrate an individual's cumulative exposure over that time and retrospective exposure monitoring is possible. Hemoglobin adducts have been identified for a number of agrochemicals or their metabolites including dichlorvos, pentachlorophenol, linuron, and ethylenebisdithiocarbamates (Waidyanatha et al., 1996; Sabbioni and Neumann, 1990; Pastorelli et al., 1995; Segerback and Ehrenberg, 1981). More recently, initial studies using azinphos-methyl [14C] administered by gavage to rats showed a dose-dependent and stable association of radioactivity with red blood cells and hemoglobin (Bailey and Jenkins, 1999). Future research is needed to identify the adduct and adduction site and to develop analytical methods for trace level detection. Remediation Technologies. Research is needed to provide effective and inexpensive remediation technologies for agrochemically contaminated soils and water. Oxidative and reductive radicals produced by the enzyme or fungi may be useful for the biodegradation of older agrochemicals in soils. When wood-rotting fungi, such as Phanerochaete chrysosporium are grown on a substrate containing cellulose, such as wood, they produce an enzyme that can generate very reactive radicals. This may provide a mechanism to degrade a variety of chemicals, including agrochemicals. Mineralization of lindane, DDT and PCP occurred when the fungus was grown on cellulose. Furthermore, when oxalate, a secondary metabolite of P. chrysosporium, was used as an iron chelator for the purified enzyme, it was oxidized to produce a radical which reduced bromotrichloromethane to the trichloromethyl radical. (Aust 1997). Additional mechanistic research is required so that optimal mineralization conditions can be elucidated. Constructed wetlands for agricultural runoff treatment is a relatively inexpensive alternative as compared to traditional treatment methods where the natural biodegradation processes can be optimized and exploited (Kirshner 1995). Wetlands are currently used to treat phosphorus, nitrogen, BOD, hydrocarbons, animal waste, and heavy metals from the municipal/animal, storm water, and mine wastes. Little evaluation of constructed wetlands to treat agrochemical runoff exists. This may be a function of the difficulty in analyzing complex agrochemicals and their transformation products, whereas metals, BOD, and nutrient are much easier to measure (Watanabe 1997; Thurman and Meyer 1996). A synergistic relationship exists between chemical and biological methodologies in waste treatment (Hapeman-Somich, 1992). Biodegradation can be enhanced by chemical pretreatment, particularly oxidative approaches that involve the hydroxyl radical (Qian et al., 1985; Miller et al., 1988; Leeson 1993; Acher 1994 and Hapeman et al., 1995). The reaction should result in effluent detoxification and nearly complete mineralization, the method should be convenient and able to be used in situ (in field situations), and useful reagents should be recoverable whenever possible. The effectiveness of the classic Fenton reaction and of an electrochemical Fenton reaction on the degradation have been evaluated (Dowling, 1992; Dowling and Lemley, 1995; Roe, 1996; Roe and Lemley, 1997; Pratap, 1992; Pratap and Lemley, 1994). The results provide base data for Fenton degradation of agrochemicals in distilled water using ferrous salt or by generating ferrous ion electrochemically. Further work has greatly improved the efficiency of the Fenton electrochemical system such that it works more effectively than the classic Fenton system (Pratap, 1996). Optimization of the hydrogen peroxide to ferrous iron concentration ratio and maintenance of a constant molar have resulted in this faster degradation (Pratap and Lemley, 1998). These new advances with an anodic Fenton treatment promise to provide a very effective approach to the Fenton remediation of pesticide wastes. Results of CRIS Search. A thorough search of the CRIS system was conducted to determine if the proposed research is being duplicated in any other USDA supported project. A number of projects involved the transport and fate mechanisms of agrochemicals in the environment or in biota. This project is unique because the researchers will examine molecular processes in air, water, soil processes and transport mechanisms that effect animal, plant, and microorganisms; i.e., the W-45 committee examines fate and transport from a holistic perspective. Two other regional projects are related, W-82 and, to a lesser extent, W-188. W-188 focuses primarily on modeling environmental processes, whereas W-82 is concerned with agrochemical transport and movement within soil, water and air and with the mechanisms involved. However, W-82 and W-45 groups diverge on what is the agrochemical end-target (non-intended target/sink).
Objectives
-
Identify, develop, and/or validate trace residue analytical methods, immunological procedures, and biomarkers.
-
Characterize abiotic and biotic reaction mechanisms, transformation rates, and fate in agricultural and natural ecosystems.
-
Determine adverse impacts from agrochemical exposure to cells, organisms, and ecosystems.
-
Develop technologies that mitigate adverse human and environmental impacts.
-
Methods
Identify, develop, and/or validate trace residue analytical methods, immunological procedures, and biomarkers. To characterize and quantify agrochemical exposure and effects to cells, organisms, and ecosystems, appropriate biomakers need to be elucidated and characterized. The newest measurement technologies need to be examined and optimized with respect to environmental and biological matrices. Research will be conducted at Cornell University and Universities of California at Davis and Riverside, and Hawaii. Researchers at Cornell will develop trace residue analytical methods to measure levels of agrochemicals in house dust from homes of agricultural workers and/or homes in agricultural areas. Homes in nonagricultural settings will be compared with the base sample. An agrochemical suite of those used in the dairy industry and those applied in and around homes will be examined. The preliminary list includes atrazine, metolachlor/alachor, diazinon, chlorpyrifos, and resmethrin. A mixed solvent extraction system will be developed to extract house dust samples which will be taken from vacuum bags used on carpets in homes. It is expected that all the agrochemicals will be extracted with the one method. Both NPD and ECD detectors will be used for analysis; GC/MSD will be used for confirmation of pesticide identity. ARS-Beltsville has extensive experience in multiple residue analyses and will participate in method development. Quantitative recoveries of polar and nonpolar chemicals in soils have been achieved using a recently developed Na4EDTA-assisted SFE (EDTA-SFE) procedure by University of Hawaii researchers. The chelating agent Na4EDTA may perform ligand formation, ionic displacement, and site covering, however, these hypotheses have not been confirmed. The role of Na4EDTA in enhancing recoveries of polar analytes will be defined by characterizing the interactions among Na4EDTA, soil particles and polar analytes and describing the sorption and desorption kinetics of Na4EDTA and analytes. Derivativizing reagents such as pentaflourobenzyl bromide will be used to capture released polar analytes. Other factors will include different chelators, time, soil moisture, pressure, solvent modifiers and various solid matrices. EDTA-SFE extraction method will be evaluated for different agrochemical classes, their degradation products and biomarkers. Imidacloprid, a new, systemic insecticide used to control sucking insects, inhibits insect acetycholine receptors, but has low mammalian toxicity although effects on human health and wildlife are unknown. Imidacloprid is a small molecule and cannot elicit an immune response by itself and must be attached to a large molecular weight carrier (protein). University of Hawaii researchers will follow the hapten design principles successfully proved for production of engineered antibodies and classical polyclonal and monoclonal antibodies. The mixed anhydride or active ester methods will be followed for the conjugation of proteins with haptens having a carboxyl acid group. After polyclonal antibodies are derived, immunoassays will be developed according to established procedures. Residue availability and human exposure potential as opposed to specific exposure data will be ascertained by researchers at University of California, Riverside using agrochemical biomarker clearance. Previously examined biomarkers have included pipron, malathion mono- and diacids, chlorpyrifos, captan and a variety of organophosphate agrochemicals. Only biomarkers that are cleared in urine will be considered, since the use of blood specimens are not justified in low risk activities when urine specimens can be used to document exposure. Use of urine specimens is well accepted by workers and the matrix is stable for storage and sensitive analysis. Strawberry harvesters, strawberry, citrus and apple pesticide handlers, and nursery (rose) workers have participated in this work. Biomarkers may be sensitively and specifically detected and used to form an Anticipated Occupational Exposure Level or Absorbed equivalent Daily Dosage for agrochemicals. Researchers at University of California, Davis will determine cholinesterases with micro-modifications of the thiocholine method (Ellman et al, 1961). Blood will be hemolyzed with a phosphate-Triton X 100 buffer and diluted with a phosphate saline for analysis. Inhibitors such as quinidine, BW 284c51 and iso-OMPA will be used to selectively determine plasma BuChE from red blood cell AChE (Wilson, 1999). Fecal testosterone, an exposure biomarker, will be determined in mouse feces ether extracts using a polyclonal antibody (Billitti et al, 1998). Human chorionic gonadotropin is used to fully stimulate testosterone production for a positive control, and Cd treatment is used to maximally suppress its production. The American Kestrel, a small falcon and accepted sentinel bird for wildlife research , will be used to determine the impact of agricultural practices on the animals that live in and around farms, fields and orchards. An azinphos-methyl (AZM) specific hemoglobin adduct will be investigated by researchers at Oregon State. Rats will be exposed to [14C]AZM, administered by gavage as a single dose, blood collected, and radioactivity associated with RBCs and hemoglobin determined. Brain and plasma acetylcholinesterase (AChE) activities will also be determined using the modified Elman assay developed by W-45 researchers at UC Davis. If successful, i.e, if the adduct can be identified, is dose-dependent, and is stable over the RBC lifetime, research will continue; investigating analytical methods for monitoring the adduct in humans and wildlife. Objective 2: Characterize abiotic and biotic reaction mechanisms, transformation rates, and fate in agricultural and natural ecosystems. Nearly all researchers will be involved in discerning agrochemical fate and transformation. Studies will be conducted at ARS-Beltsville and Riverside, Cornell University, New Mexico, Oregon, Utah and Washington State Universities and Universities of Florida and Nevada. Laboratory and greenhouse studies will be used by New Mexico State University researchers to investigate factors influencing selectivity of herbicides in crops and weeds at the whole plant or cellular level. Experiments will be designed to measure the absorption, translocation, metabolism, and photodegradation of herbicides. Radiolabelled herbicides will be used to follow their movement and degradation at the whole plant and cellular level. Herbicide degradation products will be isolated and elucidated using chromatography techniques. Herbicide translocation as it relates to photosynthate redistribution will be characterized. Using techniques they developed, University of Nevada researchers will use an elevated temperature approach within a 57-L reaction borosilicate chamber to establish stable gas-phase concentrations for semi to low volatility compounds. For experiments that examine the direct photolysis, compounds will be irradiated directly with a xenon arc for varying periods of time. For experiments which examine hydroxyl radical reactions, methyl nitrite will be added, along with an internal tracer of known OH reactivity, followed by irradiation with a xenon arc. In both experiments, the gas phase will be sampled using solid phase microextration devices and the remaining agrochemical and/or transformation products determined by gas chromatography. A novel laboratory apparatus was developed at Oregon State University to assess the evaporative flux of triclopyr butoxyethyl ester from simulated spray droplets of Garlon 4. Droplets are allowed to free fall through a glass column of temperature-and humidity-controlled air. Volatilized triclopyr ester was quantified by collecting acetone rinses of the column walls and extracting a polyurethane foam (PUF) air trap. Future research will continue to examine the significance of volatile loss during application and the refinement of drift models to address this environmental pathway. The degradation rate of soil fumigants and other agrochemicals under a variety of environmental conditions will be determined by researchers at ARS-Riverside and Florida. The effect of soil type, moisture, and temperature will be examined. Degradation products will be identified. Use of 14C-labeled compounds will allow for the determination of a mass balance and quantitation of bound residues. Such information will be useful for simulating fumigant fate under different soil and climatic conditions and for designing strategies to minimize their movement into non-target compartments. Scientists will also examine fumigant degradation using specific degrading bacteria previously isolated from soils and enhanced biodegradation. Organic substrates to increase degradative activity of indigenous degraders in soils will be examined and new degraders will be isolated from soils that possess different degradation pathways, affording less or non-toxic metabolites. The strain(s) of bacteria responsible for the degradation will be isolated and identified using gene sequencing. The environmental conditions (moisture, nutrients) and amendments favoring their growth and activity will be studied in an attempt to optimize the rate of degradation of soil-applied fumigants to minimize environmental contamination, while allowing for adequate pest control. Cellular and molecular evolvement of specific agrochemical degraders from soils at agricultural sites in Florida with long term repeated exposure will be examined. Bacterial isolates will be identified up to species level, presence and number of plasmids, and locations of degradative genes (plasmid and/or chromosomal DNA). Researchers at Utah State University will investigate agrochemical biodegradation by fungi and will focus primarily on peroxidases secreted by white-rot fungi. The fungi secrete laccases or cellobiose dehydrogenases and use molecular oxygen to oxidize chemicals. Cellobiose dehydrogenase can generate oxygen radicals that should oxidize agrochemicals. Representative fungi which secrete these enzymes will be investigated. In addition, conditions will be sought for fungi growth to secrete specific enzymes. The enzymes will be purified and tested for their ability to degrade several agrochemicals. The ability of the fungi to mineralize several rather recalcitrant agrochemicals will be assessed. Uptake of imidacloprid by hop vines will be measured under both laboratory and field conditions. In the lab, young hop vines will be potted in soil from the Yakima Valley, WA and Willamette Valley, OR, the two major hop growing regions of the U.S. Radiolabelled imidacloprid will be added to formulated imidacloprid and applied either on the surface or subsurface at a particular point to simulate the two types of drip irrigation practices. Pots will be held in an incubator under uniform light and humidity conditions. Soil will be watered periodically by injection at a single point on the soil surface or subsurface. Periodically, leaves of different ages will be removed, extracted and/or combusted to quantitate radiocarbon uptake. Extracts will be subjected to thin layer chromatography to elucidate metabolism rates. Field studies will be conducted in an experimental hop yard at the WSU Irrigated Agricultural Research and Extension Center in Prosser. Plot replicates will be assigned to one of two treatmentshop vines or bare soil. Imidacloprid will be injected into a subsurface drip irrigation system and leaf samplescollected from the vegetated plot along with soil samples at the depth of the drip tube emitter. Leaves will be collected from various heights on the plant and aged according to position on the arms coming off the main bine. To monitor the rate of imidacloprid movement. Researchers at ARS-Beltsville will examine the fate of agrochemicals from plastic mulches on a 16 plot field site and in lab using a rain simulator. Runoff will be collected and analyzed for sediment, agrochemical and nutrient losses. Sorption characteristics will be examined by conducting sorption/desorption experiments. A small piece of polyethylene will be weighed and placed in a conical flask containing the agrochemical/water solution, shaken, and analyzed at various intervals. In desorption experiments, treated polyethylene will be place in agrochemical-free water, shaken and analyzed. Objective 3: Determine adverse impacts from agrochemical exposure to cells, organisms, and ecosystems. Field and laboratory studies will be conducted, in conjunction with various state and local health and environmental protection agencies at New Mexico, Oregon, and Washington State Universities, and Universities of California at Davis and Nevada. From these studies, researchers will discover what the effects are and how the impacts occur. New Mexico State University researchers will continue laboratory and greenhouse studies to elucidate mechanisms of herbicide action and resistance in crops and weeds at the whole plant orcellular level. Enzyme assays will be used to evaluate sensitivity at herbicide sites of action and protective mechanisms against photo?oxidative stress induced by herbicides. Studies characterizing auxinic herbicide changes in receptor?site binding and gene expression using immunological and molecular techniques will be carried out to target protein(s) involved in the auxinic?herbicide resistance. The major inadequacies in two major commercial kits prompted a change in state regulations requiring optimum assay conditions and a request from state agencies that we assist the clinical laboratories in establishing appropriate conditions or conversion factors to make the results from one laboratory compatible with another. University of California at Davis will work with the clinical laboratories, enlist them in round robins to help standardize their assays, and develop a cholinesterase standard for each lab to use. The cholinesterase project will also examine easier methods to assay for blood cholinesterases by validating finger stick methods of sampling blood instead of venous collections requiring medical personnel. University of California, Davis will examine the fate of OPs from molecule to ecosystem, developing in vitro methods for studying the actions and impacts of agrochemicals on living systems, focusing on multiple chemical exposures and their impacts on humans, wildlife and agricultural ecosystems. Agricultural sprays applied to orchards during dormant season constituted a major part of the runoff of pesticides into the San Juaquin and Sacramento River Watersheds in California and prompted the state to call for Best Management Practices. A study of the runoff of agricultural sprays after treatment of orchards during dormant spray season continues in collaboration with aquatic toxicologist David E. Hinton and Frank G. Zalom. University of Nevada researchers have developed a homing pigeon model for testing the effect of exposure to agrochemicals by non-target migratory birds and tests the effect of exposure on the time of flight. Studies will be conducted on the biochemical effects of low-dose exposure to arsenic. The biochemical effects of low-dose arsenic exposure will be further characterized. Future testing will also measure the biological impact of arsenic exposure using our homing pigeon model. Collaborations with the California group from University of California, Davis will include testing OPs and carbamates. Use of these neurological agents as well as further refinement of our testing procedures will allow us to determine whether these neurological compounds effect the ability of these birds to navigate back to their roost. In all cases, we will attempt to relate the biological impact to a biochemical process, whether that is mitochondrial dysfunction, oxidative stress (measurement of antioxidant enzyme activities), ATP depletion or neurological damage (cholinesterase activity). FQPA has also required a greater emphasis on childrens health. This has resulted in renewed concern associated with agrochemical exposure to farm and farm worker children as a result of the close proximity of orchards to housing. Studies have been initiated in the Hood River Valley, an important tree fruit production region in Oregon, to assess pesticide exposure to children based on proximity to orchards, residue dynamics, and the potential for exposure. Hood River Basin steelhead population has drastically declined in recent years and the cause(s) are unknown, although agrochemicals, which are heavily used in the watershed have been implicated as interfering with the development of the steelhead. Researchers at Oregon State University, along with state agency scientists, will investigate the potential for exposure due to agricultural runoff, and wet or dry deposition. Juvenile steelhead trout will be obtained from Oak Springs hatchery, which currently supplies the Hood Basin's hatchery steelhead. The fish will be held at OSUs Fish and Wildlife Department Fish Laboratory and acclimated prior to experimentation. Experiments will compare juveniles and the fish during smolting transition, and examine interactions of agrochemicals on smolt body chemistry. Water concentrations of chlorpyrifos during the exposures, oxygen content, and hardness will be continuously monitored. Automated samplers and stream depth gauges will be located at 4 locations, selected with the assistance of the growers to represent various input scenarios. Rainfall, stream height, and soil moisture will be continuously monitored and will focus on runoff producing rainfall events soon after application. Objective 4: Develop technologies that mitigate adverse human and environmental impacts. Drawing from research results from above and outside collaborative efforts, economically viable management strategies will be developed and evaluated with respect to successful prevention or mitigation agrochemical contamination that preserve human and environmental health. Projects will be conducted by researchers at Cornell, Oregon State, and Washington State Universities and at ARS Laboratories in Beltsville and Riverside. Studies will typically involve extension specialists, growers, and scientists from the agrochemical business. Efforts will be made to assure the transferability of any developed risk-mitigation practices. To comply with pesticide label requirements, and given the close proximity of many farming operations to residential areas and areas frequented by the public, agricultural operations should take special care in employing best drift prevention techniques. These include: regular training of applicators, supervisors, and management in the best drift prevention techniques; use and calibration of equipment which minimize or prevent drift; monitoring of spray operations, particularly with regard to wind speed and direction; use of buffer zones and barriers; use of pesticide formulations and drift retardants that minimize or prevent drift; use of reduced rates and reduced frequency of application where feasible, priority given to non-chemical alternatives and reduced risk agrochemicals where feasible. Researchers at Oregon State and Washington State will work with grower groups in the Pacific Northwest to develop best drift prevention techniques that are tailored to regional production practices. Specific emphasis will be placed on evaluating the use of buffers and barriers in combination with other drift prevention techniques used in orchard airblast applications. Increasing concern exists that pesticide use practices will result in surface water contamination. The use of constructed wetlands for agricultural runoff treatment maybe a viable, relatively inexpensive management technique, however, little evaluation of their effectiveness in treating agrochemicals has been conducted. At Oregon State University wetland mesocosms will be used to better understand constructed wetland bioremediation capability and to elucidate mechanisms of important treatment processes. Using atrazine as a test compound, its distribution and that of its major metabolites in soil and water will be monitored over time following application. Uptake by wetland plants will be investigated using 15N enriched atrazine, assayed by isotope ratio mass spectrometry (EA-IRMS). Sorption studies designed to simulate interaction of the water column with sediments and of soil sorption in atrazine retention in the field system. In addition, Most Probable Number Assays will be used to enumerate the atrazine degrader population present in the rhizosphere and non-rhizosphere soil. Field plot studies concerning runoff mitigation will be conducted at ARS-Beltsville. Vegetable production typically utilizes polyethylene-cover beds to increase soil temperature, control moisture, reduce weed pressures, and contain fumigant emissions. Previous experiments showed that the runoff volume and soil and agrochemical losses are significant. Collaborative studies with University of Maryland, Center for Environmental Chemistry indicated that this runoff was toxic to aquatic organism. Experiments will be performed to determine the effectiveness of cover crops, in the furrows between the polyethylene-covered beds, to reduce runoff volume and the agrochemical load in the runoff. Field studies will be conducted to test the hypothesis that imidacloprid uptake efficiency is enhanced by irrigation scheduling in accordance with an optimal soil matric potential rather than on a daily timed schedule. Replicate plots at the WSU-Prosser station will be outfitted with soil moisture sensors at different depths that are connected to a system of solenoids controlling water flow only when the soil water tension falls below a prescribed level. Control plots will be irrigated daily for 4 h per day regardless of evapotranspiration rate and ambient soil water content. Two application rates will be tested, the maximum labeled rate (0.28 kg AI/ha) and an alternative minimum rate (0.05 AI/ha). Hop leaves will be collected after imidacloprid is injected into the system. Total imidacloprid residues (parent plus metabolites) will be determined derivativization and GC-MS analysis. Hop cones will be collected and analyzed to determine residue levels relative to the crop tolerance. Promising technologies to reduce the need for soil fumigants application in vegetable production and greatly decrease the atmospheric transport of fumigants will be examined by researchers at ARS-Riverside. The permeability of plastic films and the effect of ambient temperature will be measured and the results used as a basis for the development of new management practices. Field-plot studies using virtually impermeable films will be conducted to characterize the efficacy of soil fumigation while minimizing emissions. Additional field-plot studies will be conducted in growers fields to demonstrate and develop the use of thiosulfate fertilizers to minimize fumigant emissions. Researchers from Cornell will continue the study of agrochemical rinsewater remediation using an electrochemical version of the Fenton oxidation system. The newly developed Anodic Fenton Treatment (AFT) method makes use of a half cell approach as compared to the previously studied Electrochemical Fenton Treatment (EFT). AFT system optimization will require minimizing the formation of potentially toxic products and the examination of both batch and flow-through systems. Samples from AFT systems will be subjected to various toxicity tests in a separate USDA/CSREES/NRI grant with Dr. Steven Bloom at College of Veterinary Medicine at Cornell. Experiments will be conducted using a membrane to separate which would eliminate the NaCl that enters the solution from the salt bridge and make toxicity tests more efficient. Ion selective membranes investigated will allow charge transfer and be chemically stabile, thus perfluorated varieties are an excellent choice. Cation exchange membranes, such as Nafion.-, Flemion.- and Neosepta.- are less prone to precipitate fouling than anode exchange membranes [Strathmann, 1992]. Bipolar membrane arrangements will provide even less precipitate fouling if sufficient current can be applied for successful AFT. Various configurations will be evaluated with respect to efficiency of the system. Integrated Pest Management strategies rely on, and consumer attitudes are often determined by, access to agrochemical information. Work at Cornell will continue to develop the educational model, allowing the public to have access to science-based information at an appropriate level. This will be accomplished by examining and characterizing the role that agrochemicals may play in affecting human health, especially cancer incidence. Technical reports which critically evaluate the available peer-reviewed scientific literature and the gray unpublished literature will be prepared and peer-reviewed by experts in the field. Research gaps will be identified, risk reduction procedures outlined and promising avenues of future research proposed. The detailed technical reports will be translated into a variety of formats, including technical briefs for W-45 researchers and other scientists at academic institutions and federal agencies and consumer-friendly fact sheets and tip-sheets for the non-scientist. Other educational materials will be developed concerning how different agrochemical classes may affect health endpoints and what are the recommended strategies to reduce exposures. Materials will be available in multiple formats, including print and electronic formats (PageMaker, Portable Document Files [PDF], HTML). All technical and educational materials will be available in hard copy and posted on a Cornell Center for the Environment (CfE) web site (http://www.cfe.cornell.edu/bcerf/) in plain text and/or formatted down loadable files (PDFs). Efforts will continue to tract and improve evaluation of web site usage to assess audience usage and impact.Measurement of Progress and Results
Outputs
- The impacts of this project will be critical to understanding human and environmental health risks from agrochemicals in both the short and long term. Risk is defined by both exposure and hazard. The W-45 researchers will be advancing knowledge about major exposure routes and the hazardous or adverse impacts of agrochemicals to cells, organisms and ecosystems, thus completing the information necessary for understanding risks. Another major outcome of this project is the development of technologies to mitigate adverse impacts and the use of extension/outreach to communicate this information to the agricultural industry and the public. The members of W-45 have an enviable track record of publishing their work in refereed journals and presenting it at well-known venues in professional organizations. In addition, four of the research scientists in the project have extension responsibilities as part of their faculty positions. The overall outcome of the W-45 project will be a substantial increase of knowledge that will be extended to a wide variety of audiences. Some specific outcomes of individual projects are summarized below. The Hawaii project is expected to develop and improve new analytical methods for environmental compatibility, accuracy, fast analysis, and cost-effectiveness. The economic benefits to the agricultural industry in tropical ecosystems will be critical to its sustainability. The New York-Cornell project will be reducing risks of children to pesticides by determining what the exposures are in house dust and indoor air. Results from this work will immediately be translated into educational programs about practices to reduce agrochemicals in the home. The work at California-Davis is critical to measuring biomarkers by standardizing cholinesterase measurement. A significant benefit will be the ability to compare worker cholinesterase levels throughout the state, and these results will be available for the entire region and for the country. The project in New Mexico will concentrate on using mechanistic studies to minimize agricultural losses due to weeds. Annual agricultural losses due to weeds exceed $20 billion in the United States. Understanding the mechanisms involved will provide environmentally sound and cost-effective approaches to weed management. The work in Florida will generate potential biological means for reduction of volatilization and leaching of volatile and water soluble pesticides from root-zone soil. Research in Nevada and California will understand adverse impacts from agrochemicals on cells, organisms and ecosystems, making important contributions to health of people and ecosystems. <br>(continuted below)
Outcomes or Projected Impacts
- (continuted from above)<br> Development of technologies and other methods to mitigate adverse impacts will be critical to the outcomes of W-45 work. For example, Cornell researchers are developing important new technologies to treat pesticide wastewater and rinse water. Money will be saved in municipal sewer systems if water delivered for treatment has already been pretreated with an advanced oxidation method to remove toxics. Work at ARS-Beltsville and Riverside will provide usable farming systems to grow vegetables that minimize negative impacts on surrounding ecosystems. Other work at Cornell will provide critical information to scientists, health professionals, and the general public about human health effects of agrochemicals. The development of technical reports on health effects of agrochemicals and of related educational materials for the consumer will provide peer-reviewed scientific information to public policy makers and risk assessors on the relationship(s) between selected agrochemicals, cancer risk and other health risks and will provide practical strategies that can be used to reduce exposure to suspect agrochemicals to diverse audiences. These audiences include undeserved populations who may be the most vulnerable and at highest risk for exposure to pesticides and other chemicals, including the rural poor and inner city residents. In addition to the important outcomes in each state, the W-45 project will have joint outcomes as a result of the collaboration in this multistate project. A joint meeting with W-188, W-45, and W-82 in San Diego in January, 2002 is planned. The groups intend to produce a monograph that will distill key issues related to their research into a form that can be used by extension and other professionals throughout the country. Other joint projects of W-45, which can be disseminated in a variety of ways, are agrochemical management guidelines, extension educational materials, position papers and policy papers. Many of these materials can be put on the web (an excellent example of this is the BCERF web site at Cornell). Finally, the strength of W-45 committee is its scientific diversity and its commitment to superior science. As such, at its annual meeting, individual projects are rigorously reviewed from many different scientific and regional perspectives, often yielding new approaches and strategies to test. Many members are often found publishing and communicating with scientists outside their disciplines. From the results of W-45 project, human and environmental risks from agrochemicals will be reduced, food production will be more economically managed, and model risk reduction approaches will be available for use nationwide.
Milestones
(0):0Projected Participation
View Appendix E: ParticipationOutreach Plan
Organization/Governance
The W-45 Committee is composed of the members who represent the participating experiment stations. Experiment stations in other regions, state extension services, and Agricultural Research Service laboratories, as well as an Administrative Advisor, and a representative of CSREES. A contact person will also be designated for the Technical Committee to serve as liaison for the IR-4 Project and the National Agricultural Pesticide Impact Assessment Program. These two programs have closely related activities and have interacted successfully with W-45. The officers of the Technical Committee will serve for two years each, and be a chairman, vice-chairman, who will also serve as research coordinator, and a secretary. The chairman of the technical committee coordinates the annual technical committee, with consultation from the administrative advisor. The chairman prepares the agenda, presides over the annual meetings and is responsible for preparation of the annual report. The secretary is responsible for recording and distributing the minutes of the technical committee meeting and carrying out duties assigned by the technical committee or administrative advisor. The officers and the immediate past chairman comprise the Executive Committee, which is empowered to act for the Technical Committee between annual meetings.