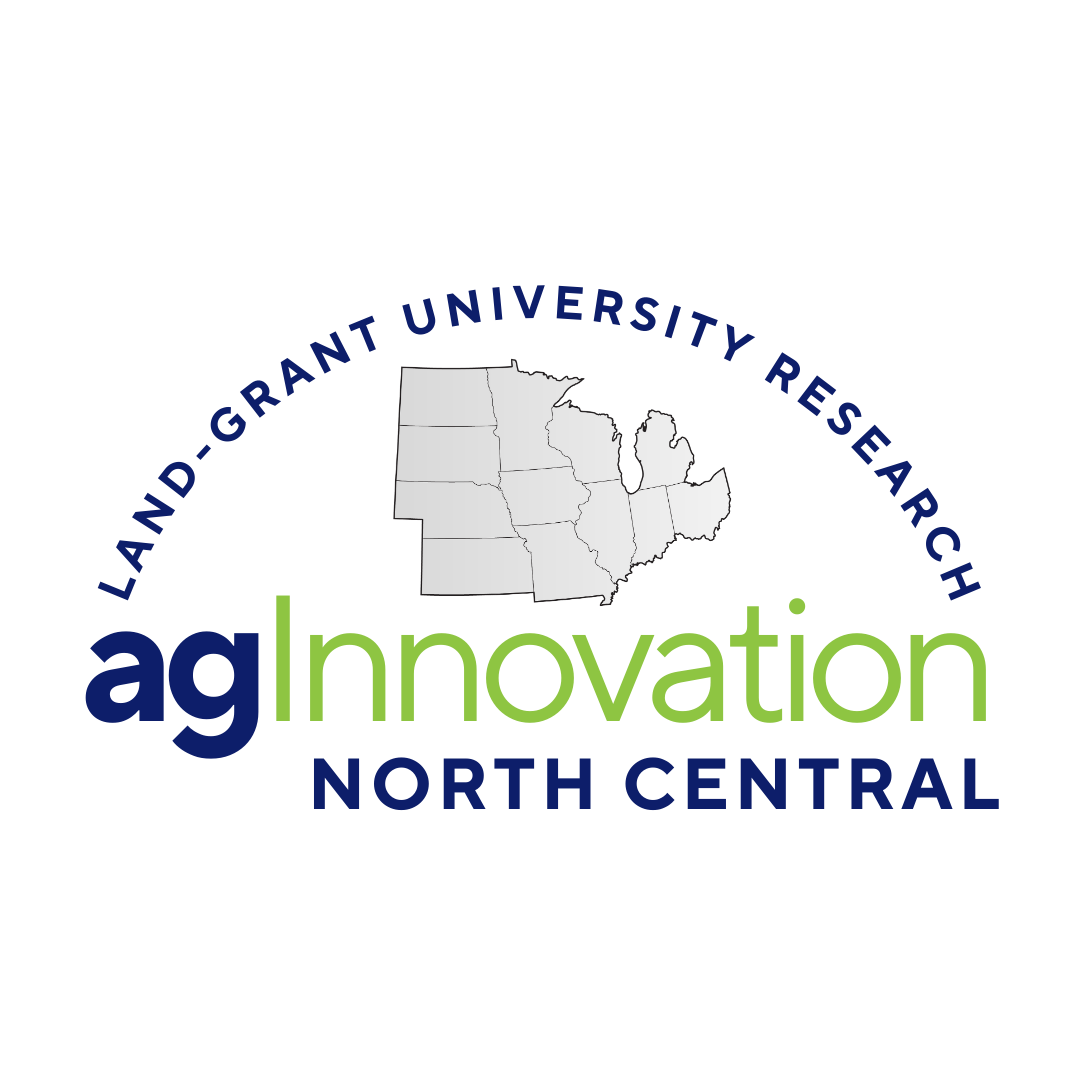
NC_temp1183: Mycotoxins in a Changing World
(Multistate Research Project)
Status: Under Review
NC_temp1183: Mycotoxins in a Changing World
Duration: 10/01/2025 to 09/30/2030
Administrative Advisor(s):
NIFA Reps:
Non-Technical Summary
Mycotoxins are ubiquitous natural toxins that can contaminate many agricultural products as a result of fungal colonization in the field or in storage. These compounds pose health risks to humans and domestic animals consuming products made from contaminated raw ingredients. They also cause serious economic consequences across the continuum of food production and consumption. As a result, mycotoxins threaten both food safety and food security for societies across the globe. Mycotoxin risk is very dependent on environmental conditions, and therefore climate change is altering the frequency and severity of mycotoxin outbreaks in ways that are not fully understood. In order to address this critical problem, we propose research and educational activities directed at four main objectives: 1. Develop data and conduct risk assessments of mycotoxin exposure in human and animal health; 2. Establish integrated strategies to manage and reduce mycotoxin contamination in cereals and in forages; 3. Increase understanding of underlying mechanisms that determine mycotoxin production potential and outcomes; and 4. Engage with stakeholders to increase knowledge transfer and workforce development. The proposed monitoring and research activities will enhance our knowledlge of mycotoxin risks and management strategies to reduce those risks. The research and outreach proposed here will address the needs of stakeholders across the spectrum of food and feed safety and security, including the research community, the seed industry, crop production professionals, the livestock industry, the grain handling and processing industries, companion animal food producers, and finally, consumers.
Statement of Issues and Justification
The need, as indicated by stakeholders
Crop producers and processors need to minimize mycotoxin contamination of food, forage, and feed to reduce the deleterious effects of mycotoxins on consumers, livestock, and domestic animals. In turn, food and feed processors must manage their sources and processes to maintain product quality and protect the health of downstream customers, while livestock producers must monitor feed sources for their herds. The presence of mycotoxins in livestock feed has led to serious issues for the industry in recent years. In particular, ingestion of zearalenone-contaminated feed can result in uterine prolapse in sows, ending their breeding life. Other mycotoxins can lead to a variety of symptoms that detract from animal well-being and the productivity of operations. Plant breeders have had success in producing plants with some resistance to mycotoxigenic fungi; however, this resistance does not always lead to reduced mycotoxin contamination. This confounding issue needs to be understood and resolved to improve plant breeding strategies and for seed producers to be able to generate high quality seed. Additionally, cost-effective methods to predict, monitor, and minimize mycotoxin production in the field, and to detoxify mycotoxins and prevent further deterioration of contaminated feed, are needed by producers of grain and livestock. The increasingly strict tolerance limits for mycotoxins in overseas markets have increased the burden for grain buyers and food processors; currently, mycotoxin levels that are acceptable for some US products are unacceptable in European and Asian markets, resulting in non-tariff trade barriers. The global trend is for regulators to impose restrictions on an increasing number of mycotoxins. This potentially will require US grain and livestock producers to be able to assess and prevent presence of these mycotoxins well below domestic guidelines. Finally, workers who are responsible for animal and human health need information about the toxicity, carcinogenicity, modes of action, and biomarkers of exposure and disease for all categories of mycotoxins. This information would be used to train veterinarians and health-care providers to identify exposure and treat related diseases, as well as to develop more accurate risk assessment.
The importance of the work, and consequences if it is not done
Mycotoxins are a serious, chronic problem throughout the agricultural regions of the U.S. If research is not applied broadly to address this problem, serious negative consequences will result. First, mycotoxins pose important health risks. Accurate risk assessments are essential in order to maintain exposures by animal and human consumers within safe limits. We propose basic research to examine the toxicity of several important mycotoxins. Without this information, it is impossible to assess the risks associated with these mycotoxins. Additionally, the presence of mycotoxins in grain is an economic concern, especially in the context of global markets. Without an aggressive research program to prevent, treat, and contain outbreaks of mycotoxins in grain, U.S. grain producers suffer the consequences of reduced marketability of their products. Furthermore, the proposed research addresses biosecurity concerns. The natural occurrence of mycotoxins in grain is an important security concern for producers and end-users of the grain globally. Without a proactive research program to find innovative ways to monitor, prevent, and treat mycotoxin contamination of grains and forage, US agriculture will be unprepared to deal effectively with a mycotoxin outbreak, regardless of its origin. Finally, the production of mycotoxins by mycotoxigenic fungi in grains, forage, and other agricultural products represents a continuing problem in agriculture that reduces food safety and security. Improving our understanding of factors that allow these fungi to colonize their hosts, and of how mycotoxin biosynthesis is regulated, will not only lead to novel treatment strategies, but will also advance our understanding of fungal pathogenesis in general. Linkages with international efforts will leverage expertise and promote mycotoxin mitigation in developing countries. In a changing world, food security is a component of political stability and US national security interests.
Technical Feasibility
The proposed research ranges from routine survey and analysis work to highly innovative strategies involving cutting-edge technology. Feasibility varies from routine to high-risk/high-reward, depending on the specific experiments proposed under each objective.
The advantages for doing the work as a multistate effort and the technical feasibility of the research
The scientists involved in this multistate, multidisciplinary research proposal bring a broad diversity of expertise on mycotoxin issues related to their respective disciplines. These include plant science and plant pathology, seed science, mycology, toxicology, epidemiology, risk assessment, public health, modeling, and economics. Just as agriculture is diverse and varies greatly from state to state (and in many instances, within a given state), the occurrence and severity of mycotoxin outbreaks vary widely across the US. A multistate effort ensures a thorough approach to investigate a complex and highly variable phenomenon that has significant impacts on both producers and consumers. Simultaneously, the differing experiences and expertise of the members are accessible to the whole, facilitating multistate collaborations that result in joint manuscripts and research proposals. Many such collaborations of long standing exist within the body of the research group. Due to the wide range of experience and expertise of the group, the proposed research is technically feasible.
What the likely impacts will be from successfully completing the work
The research and outreach proposed here will address the needs of stakeholders across the spectrum of food and feed safety and security, including the research community, the seed industry, crop production professionals, the livestock industry, the grain handling and processing industries, companion animal food producers, and finally, consumers. Outputs will include information on the action of mycotoxins in livestock and animal models. This information will be applicable to the risk assessment process. Genetically improved crop varieties and potential biological control agents will be generated to address management of mycotoxin formation in the field. Information will be generated to address the need for management practices that help prevent mycotoxin-related problems during grain and forage production, handling, storage, processing, and consumption. Finally, we will generate important basic knowledge about major groups of mycotoxigenic fungi, and the biochemical and molecular factors that regulate the biosynthesis of aflatoxins, endophyte-associated alkaloid toxins, and Fusarium-associated mycotoxins including deoxynivalenol, fumonisins, and zearalenone. This will reveal critical points in the biosynthesis pathways where targeted controls can be developed. Students will be trained to contribute to the scientific workforce with expertise in mycotoxicology and a broad perspective through interaction with the multi-state group composed of scientists in multiple disciplines.
Related, Current and Previous Work
Research on mycotoxin risks and management in relation to climate change is an active area of research among the participants of this project, with collaborating organizations such as USDA-ARS, and externally in other parts of the world (Anelli et al., 2024; Casu et al., 2024; Nesic et al., 2015; Perrone et al., 2020). Existing surveillance programs have been conducted in a non-systematic way, resulting in a patchwork of available data for risk assessment and prioritization of management practices. International experts have assessed and prioritized available management practices, and many innovative management tools have been proposed (Logrieco et al., 2021. Munkvold et al., 2019). A variety of modelling approaches aimed at assessing risk of specific mycotoxins have been developed and applied in different parts of the world, with mixed success (Branstad-Spates et al., 2024; Cui et al., 2022; de Wolf and Paul, 2014; Froment et al., 2011; Marin et al., 2021; Wang et al., 2022). The abundance and nature of mycotoxins present in a commodity or region changes from year to year based on numerous factors, such as local weather, crop genotype and regional cropping patterns, and shifts in populations of mycotoxigenic fungi (e.g., the replacement of 15-ADON with 3-ADON Fusarium graminearum genotypes across two clines in Canada and in portions of Minnesota and the Dakotas; the appearance of Fusarium boothii in Texas, Nebraska, and South Dakota) (Okello et al. 2019, Valverde-Bogantes et al. 2019).
While toxicological studies on individual mycotoxins have been conducted for many years (EFSA 2013; NTP 2001; WHO, 2002, 2011), there are many knowledge gaps in terms of understanding the risks of multi-mycotoxin exposure, and exposure to lesser known or emerging mycotoxins. Human and animal health risks are determined by a variety of factors, including abundance of mycotoxins in the diet, other dietary inputs and options, possible synergy between mycotoxins (especially aflatoxin and fumonisin, which often occur in maize), and socioeconomic factors and long-standing tradition (e.g. populations in Central America have a deep cultural association with maize and consume proportionally much more than populations in the US while, conversely, US corn is subject to much more stringent quality regulations – and therefore lower mycotoxin levels – prior to human consumption).
Strategies to reduce mycotoxin risk range from genetic resistance to pre-harvest management to post-harvest management and detoxification methods (Logrieco et al., 2021. Munkvold et al., 2019). Studies on mycotoxin biosynthesis pathways are very promising toward designing genetic tools to interfere with the ability of fungi to produce mycotoxins in planta (Johnson et al.,2018; Kim et al., 2020). Meanwhile, great strides are being made toward identifying and improving biological control opportunities through microbial screening methods, fungal genomics and metabolomics
Participants in this project have published multiple papers recently on various topics related to the current objectives (Branstad-Spates et al., 2024; Davis et al., 2023; Deng et al., 2023; Dor et al., 2024; Du et al., 2023; Jones et al., 2024; Kaur et al., 2024a, 2024b; Liu et al., 2023; Luciano-Rosario et al., 2023a, 2023b; Maguay-Gonzalez et al., 2023; Mandap et al., 2024; Munkvold et al., 2021; Nava-Ramirez et al., 2024; Singh et al., 2023; Yang et al., 2024; Yu et al., 2024)
Objectives
-
Develop data and conduct risk assessments of mycotoxin exposure in human and animal health
Comments: • Conduct surveillance of crops, food, and feed for the presence of mycotoxins and mycotoxigenic fungi • Determine sources of exposure for human and animal populations exhibiting mycotoxin intoxication symptoms • Assess the role of environmental factors in mycotoxin development • Develop predictive and practical methods to assist in managing mycotoxin outbreaks • Assess human and animal health risks from mycotoxin exposure in the US -
Establish integrated strategies to manage and reduce mycotoxin contamination in cereals and in forages
Comments: • Employ biotechnology and conventional breeding strategies to develop crop cultivars that are resistant to mycotoxigenic fungi or can detoxify mycotoxins • Develop and enhance high-throughput screening methods for phenotyping germplasm • Conduct screening to characterize germplasm for resistance/susceptibility to mycotoxin contamination and improve methodology for breeding for resistance • Identify and test microbial detoxification approaches to reduce mycotoxin contamination. -
Increase understanding of underlying mechanisms that determine mycotoxin production potential and outcomes
Comments: • Identify genetic factors contributing to mycotoxin production in the pathogen and resistance in the host • Evaluate the role of microbe-microbe interactions and the host microbiome in the production of mycotoxins -
Engage with stakeholders to increase knowledge transfer and workforce development
Comments: • Train the next generation of mycotoxin researchers/food system practitioners • Disseminate mycotoxin-related knowledge to stakeholders across the farm-feed-food continuum
Methods
Objective 1: Develop data and conduct risk assessments of mycotoxin exposure in human and animal health
1.1 Conduct surveillance of crops, food, and feed for the presence of mycotoxins and mycotoxigenic fungi
The KSU veterinary toxins laboratory routinely assays grain and animal feed samples for mycotoxins via GC/MS on a fee for service basis for producers. Toxins assayed are commonly aflatoxin, and derivatives, and the typical spectrum of Fusarium toxins. Corn, sorghum, wheat and soybeans are the usual crops of interest. Samples are submitted by producers across the upper midwest. Some international samples also are analyzed as part of USAID projects in Africa and Nepal.
Fusarium-infected field samples of wheat and corn in Nebraska and the upper Midwest will be collected, the Fusarium species identified by PCR and the mycotoxin profile by PCR genotyping (3-ADON, 15-ADON, and NIV) and/or GC-MS. The upper Midwest (MN, IA, ND, SD, NE, KS) is an area of interest based on movements of pathogens and mycotoxins over the previous decade.
KS and NE have active and ongoing international survey and surveillance projects through the USAID Feed the Future Innovation Lab for the Reduction of Post-Harvest Losses and Borlaug Fellowships at UNL (e.g. Mendoza et al. 2017).
VA conducts DON testing for several states participating in the US Wheat and Barley Scab Initiative. The Schmale Lab at Virginia Tech provides diagnostic testing services for DON for stakeholders in the US. These services are vital to the development of new varieties of wheat and barley with reduced mycotoxin potential and are necessary to identify and/or exclude appropriate strategies for managing FHB. While methods are standardized for wheat and barley, these methods do not translate satisfactorily to sorghum (matrix effects) so new methods have been developed and validated (McMaster et al. 2019); method development will continue with other host substrates. This testing, and its ongoing improvement, benefits participants in many states.
MO offers mycotoxin testing (aflatoxin, ochratoxin A, zearalenone, vomitoxin, and fumonisin) in feed for states across the US and their metabolites testing in animal tissues and biofluids (Du et al. 2023). New methods for trichothecenes including nivalenol, vomitoxin, 3-acetyl vomitoxin, and 15-acetyl vomitoxin in various matrices using immuno-affinity column cleanup are undergoing in the laboratory. New methods for fumonisin exposure diagnosis to measure the sphinganine and sphingosine ratio in animal serum are under development.
NJ On the plant side, NJ has used CRISPR-CAS 9 editing in Arabidopsis to create a platform for validating candidate mycotoxin response genes from wheat and barley using a functional complementation assay. This approach allows the efficacy of these genes to be tested, prior to CRISPR-CAS9-mediated editing of these genes in barley and wheat.
The roundworm Caenorhabditis elegans and the waxworm caterpillar Galleria mellonella provide inexpensive and easy to rear models of animal response. NJ has expertise in using C. elegans to reveal cellular targets of DON (Di et al. 2018) and will continue this work to test better understand how mycotoxins disrupt normal cellular function and to devise and test intervention strategies. The development of comparatively rapid growing and inexpensive plant and animal models will benefit all mycotoxin researchers and will be applicable to other genes, mycotoxins, and host-pathogen systems.
In WI, Kaur corn agronomy lab, is focused on surveying mycotoxins in grain and silage corn. The goals for next three years involve collecting ear and stalk rot ratings at multilocation corn field trials and grower fields and quantifying multiple mycotoxins occuring in grain and whole plant. Additionally, we are aiming at building a database exploring correlations among different mycotoxins to leverage the presence of one toxin as an indication for the presence of another to provide a quick and cheap identification tool to the growers.
1.2 Determine sources of exposure for human and animal populations exhibiting mycotoxin intoxication symptoms
Work by KS and NE through USAID in Honduras, Nepal, Malawi and Guatemala includes working with human populations experiencing chronic exposure to aflatoxins and fumonisins in excess of the provisional maximum tolerable daily intake (Mendoza et al. 2018; Sabillón et al., 2023). In Honduras, up to 25% of children are considered stunted (diet consists of maize, with high levels of fumonisins), while in one district in Nepal 95% of pregnant women tested positive for aflatoxin exposure. In Nepal, maize and groundnut have high levels of aflatoxin but are irregularly consumed, while chilies and extruded soybean nuggets have intermediate aflatoxin levels but are frequently consumed. Continuing work will tease out the contributions and synergies between mycotoxins, and continue to identify sources that might not initially be suspected (e.g. chili peppers). Preliminary data shows common features across broadly different populations exposed to mycotoxins, and outcomes will guide further international efforts.
1.3 Assess the role of environmental factors in mycotoxin development
UW-Madison Keller lab has initiated a project to address the consequences of climate change on mycotoxin production. The design of this study will determine if aflatoxigenic Aspergillus flavus isolates increase or decrease in numbers when in competition against aflatoxin null strains under environmental conditions associated with climate change including but not limited to temperature fluctuations, humidity, and microbiome shifts. Recent computational studies have predicted an increase in aflatoxin contamination of corn in the Midwest and the UW-Madison study is aimed at addressing these risk assessments.
The Kaur lab at UW-Madison is working to identify the weather patterns in the last three decades and how has it affected mycotoxin occurrence (type and quantity) in corn. The project is being designed to use the data from NASS and USDA to identify problem years and the recorded weather events in those years to create predictive models going forward. We also plan to leverage grower surveys and grower field sample collection to identify how the different environments across the state affected the quality of both grain and silage corn.
Aside from field and greenhouse trials, it is possible to impute the impact of climatic factors on fungal growth and mycotoxin production through examining the USDA RMA database for aflatoxin-related crop insurance claims, and determining the conditions that caused the greatest amount of aflatoxin risk. In the Wu lab at Michigan State University, we will use these USDA RMA data to model the temperature and moisture conditions, as well as grower practices, that are likely to either increase or decrease the risk of aflatoxin contamination in crops that is enough to trigger farmers to file crop insurance claims.
NE is studying DON persistence and production, and fungal viability, under long-term storage of wheat grain at different water activities. Initial results show active trichothecene biosynthetic genes and DON production even at 120 days of storage in untreated and strobilurin-treated wheat. These studies have implications nationally and internationally for best practices in grain management.
Conditions for ZEN production need to be better defined in particular in the context of sources of this mycotoxin in swine feed. Multiple stations will be examining this aspect and sharing data.
IL is identifying environmental factors associated with GER severity and mycotoxin accumulation. We are growing hybrids across multiple environments across the Eastern US by collaborating with Genomes to Fields and industry partners. These locations represent very diverse geographical environments with varying disease pressures. Our hypothesis is that distinct environmental covariates (ECs) and their timing are drivers for ear rot and mycotoxin incidence. We are quantifying ear rot severity in each environment and mycotoxin concentrations in samples from each environment. We are curating 60 environmental covariates from each environment and are using this dataset to understand how weather impacts ear rot severity and mycotoxin accumulation.
1.4 Develop predictive and practical methods to assist in managing mycotoxin outbreaks
Predictive models allow us to better prepare for mycotoxin contamination in US crops in the near and long-term future. Our past work has used aflatoxin-related crop insurance claims from the USDA Risk Management Agency (RMA) to develop a model of how aflatoxin risk changes as a function of climatic factors and grower practices; including but not limited to maximum and minimum daily temperatures, precipitation, relative soil moisture, irrigation, transgenic Bt corn planting, and state and year. We will refine this model to determine how aflatoxin risk may spread in US corn, particularly in the major corn-producing states in the Corn Belt, over the next 10, 20, and 30 years.
At the moment, it is not possible to use RMA data to develop similar climate models predicting the risks of other mycotoxins; because these mycotoxins - fumonisins, DON, ZEN, OTA - are not specifically accounted for in crop insurance claims. However, similar principles could be used to develop climate predictive models based on our data on optimal growth and toxin-producing conditions for each of the causative fungi (Fusarium, Penicillium, Aspergillus), including temperature ranges, water activity, substrates, and the effectiveness of transgenic hybrids and other control methods to reduce mycotoxin risk.
At Iowa State University, data collection and modeling efforts continue with the target of predicting risk for several mycotoxins in corn. Grain samples were collected from small plot trials that have been established as part of the National Predictive Modelling Tool Initiative. The samples were sent to the USDA-ARS SRRC (New Orleans) for quantification of mycotoxins (aflatoxin, DON and fumonisins). The data will be used to develop mycotoxin prediction models for the U.S. Dr. Hennessy has obtained data from a large grain processing company on mycotoxin incidence averages in corn intake by toxin and state over about 10 years. These data will be used to analyze risk factors for mycotoxin occurrence in corn.
NDSU aims to develop a rapid and non-destructive model for identifying Fusarium damaged kernels (FDKs) of wheat, barley and the FDKs resilient during the malting process by rapidly quantifying mycotoxin production in single kernels. They propose to develop a hyperspectral imaging method for rapidly and nondestructively quantifying FDKs of barley, wheat and their malt. Link the hyperspectral imaging/wavelengths to toxigenic fusarium biomass (Tri5 DNA) and/or DON and other mycotoxin contents of FDKs. For this, the growth of fusarium and mycotoxin production in FDKs during malting will be monitored to anticipate fusarium and mycotoxin presence in bulk malt by identifying FDKs in grains. The quantification model will be interpreted and validated by measuring the mycotoxin contents and fusarium Tri5 DNA in the discriminated FDKs and asymptomatic kernels.
1.5 Assess human and animal health risks from mycotoxin exposure in the US
Human health risk assessment for foodborne chemicals and toxins was codified in the 1983 National Academy of Sciences publication Risk Assessment in the Federal Government: Managing the Process. Essentially, the risk of chemicals to our health depends on:
- Hazard identification: linking the particular chemical (in this case, each mycotoxin) to specific human health effects - diseases, conditions, symptoms - that are sufficiently well-established in toxicological and epidemiological literature. For example, over 60 years of data exist that causatively link aflatoxin exposure to hepatocellular carcinoma (liver cancer).
- Dose-response assessment: understanding the dose of a chemical or toxin that triggers the disease. In most cases, there is a threshold dose, below which humans could consume a small amount daily and not expect to compromise health. If the threshold dose is exceeded, however, health risks could result.
- Exposure assessment: determining how much of a particular chemical or toxin people encounter daily on average in their food, water, air, or other source.
- Risk characterization: comparing the amount to which people are exposed to a particular chemical or toxin with the amount that is considered “safe” per the dose-response assessment. If the amount people are exposed to is below the threshold, then the chemical is not likely to cause human health risk. If the amount exceeds the threshold of safety, then risk of disease may be present.
We will determine the US populations’ risk for particular mycotoxins for which we have data on well-established health effects, and doses that are considered safe (tolerable daily intake, or using a FDA action level or industry guideline). We will then estimate exposure through the Centers for Disease Control and Prevention (CDC) National Health and Nutrition Examination Survey (NHANES) by age group of different foodstuffs, and contamination levels of mycotoxins in these foodstuffs. Then we can determine which populations - age groups, consumers of particular foodstuffs - may be most at risk from adverse health effects of mycotoxins. In the case of aflatoxin, there is a confounding factor of liver infection with one of the hepatitis viruses; chronic infection with a hepatitis virus increases the risk of liver cancer caused by aflatoxin. We will account for this based on the US prevalences of chronic HBV and HCV infection, in determining aflatoxin-induced liver cancer risk.
KS cooperation with BOKU in Austria through the EU FoodSafeR project is developing a database and web site on food safety with readily accessible materials for producers, shippers, retailers and consumers. The web site has a European focus but will contain information usable worldwide. Mycotoxins are a major food safety concern in Europe and will have a prominent place on the web site. The foods of interest will be much broader than usually considered within NC-1183 and will include raisins and other dried fruits, peanuts, cocoa and coffee in addition to coarse and small grains. Up to 30 mycotoxins will be evaluated in various European labs participating in the FoodSafeR project. Work with the EU MycoKey and MyToolBox consortia has resulted in several publications on mycotoxin management at the policy and practical application levels (Krska et al., 2022; Leslie et al., 2020, 2021; Logrieco et al., 2021)
The roundworm Caenorhabditis elegans and the waxworm caterpillar Galleria mellonella provide inexpensive and easy to rear models of animal response. NJ has expertise in using C. elegans to reveal targets of DON (Di et al. 2018) and will continue work with model systems. The development of comparatively rapid growing and inexpensive plant and animal models will benefit all mycotoxin researchers and will be applicable to other genes, mycotoxins, and host-pathogen systems.
Objective 2: Establish integrated strategies to manage and reduce mycotoxin contamination in cereals and in forages
We (MN) are developing a set of high-resolution melt (HRM) probes that enable the tracking of shifts in mycotoxigenic fungal populations. Combined with our ongoing efforts to determine the mycotoxin production profiles of Fusarium and Aspergillus species, these efforts will allow for population-specific toxin mitigation efforts in a changing climate.
Iowa State University will continue to investigate the impact of interactions between insects and mycotoxigenic fungi in the field and in storage. Studies will continue to explore how transgenic insect-resistance traits can contribute to mycotoxin management.
IL will conduct management trials to test current and novel Fungicide formulations as well as different levels of wheat resistance to control DON contamination in wheat. Novel fungicide application methods such as the use of drones will be tested.
Kaur corn agronomy lab at WI, is focused on identifying the patterns between weather, growers decisions, and mycotoxin occurrence (primarily the toxins produced by Fusarium spp.) to explore integrated management options. We are aiming to investigate the efficiency and impact of insect and disease resistant traits, planting time variability, plant density, residue management, and harvest timing for mycotoxin management. We are also focused on evaluating susceptibility of novel corn concepts such as short stature corn to fungal infections and mycotoxin contamination. We are aiming to conduct multi location small plot and on-farm trials to test various management decisions (starting from hybrid selection to harvest and storage) and strategize an integrated management program for short stature corn to aid the growers in decision making. Furthermore, we are aiming to identify and address any potential insect-pest resurgence or resistance to current Bt traits.
Hermetic storage is one of the best ways to reduce mycotoxin production in storage. KS and Malawi have been working to evaluate hermetic storage bags under conditions where they can be implemented by subsistence and commercial farmers in Malawi and elsewhere in sub-Saharan Africa. Grain stored in hermetic bags has less mycotoxin contamination than grain stored in conventional jute or plastic bags, since the lack of oxygen in these bags limits fungal growth and often survival. Most of the work so far has been done with maize, but expansion to other crops is being considered (Ngoma et al., 2024).
DON testing services at Virginia Tech will provide analytical services necessary to develop new cultivars of wheat and barley with reduced potential for DON contamination, and improve chemical and cultural practices necessary to reduce DON contamination in wheat and barley.
2.1 Employ biotechnology and conventional breeding strategies to develop crop cultivars that are resistant to mycotoxigenic fungi or can detoxify mycotoxins
NJ – In a proof-of-principle approach, two candidate genes proposed to condition FHB susceptibility have been edited in Arabidopsis using by CRISPR/Cas 9 technology. Editing of these candidate genes resulted in plants with reduced susceptibility to Fusarium graminearum. These functionally validated genes are currently the target of CRISPR/Cas 9 editing in barley.
In a complementary approach, NJ will use CRISPRa to activate the expression of endogenous genes involved in broad immunity to infection by Aspergillus flavus in maize. A number of gene targets, shown to be effective by over-expressing the transgenes have been identified in maize and will be the subject of efforts to enhance expression and disease resistance.
Because regeneration of gene-edited barley and maize plants is much less efficient than in Arabidopsis, NJ has undertaken a number of strategies to improve this bottleneck. These include: (i) The development of guide RNA (sgRNA) and dual tRNA-based CRISPR-gene editing vectors to target multiple loci within a single gene (thus, ensuring gene disruption) or within separate genes; (ii) A systematic assessment of transformation and regeneration protocols to identify optimal tissue culture strategies; (iii) The use of so-called morphogenes, whose inclusion in gene-editing vectors and expression in transformed cells and tissues promotes somatic embryogenesis, greatly increases the number of candidate plants generated and provides a simple morphological basis for selecting gene-edited plants.
These strategies will be applied to create barley and maize plants with enhanced immunity and decreased susceptibility to FHB and to A. flavus. These plants will be tested for disease resistance, mycotoxin contamination and other morphological and agronomic properties. If successful, these plants will be shared with other members of the consortium for testing under field conditions.
MS is working with corn inbred line Mp313E which confers plant resistance to Aspergillus flavus infection and exhibits low levels of aflatoxins in mature kernels, comparing near-isogenic lines and conducting field trials (Windham et al. 2018).
NJ – three genes likely involved in FHB susceptibility have been edited in Arabidopsis using CRISPR/Cas 9 technology as proof-of-principle, with reduced Fusarium graminearum infection confirmed in two of the three. As genes are validated in Arabidopsis, they are edited in the relevant FHB host plant, barley, and the phenotypes are confirmed. Vectors for CRISPR editing of wheat and rice are also under investigation.
NDSU proposes to improve FHB resistance in wheat by site-directed mutagenesis of genes for FHB susceptibility through wheat × maize hybridization coupled with CRISPR/Cas9 genome editing. Previous studies identified several genes responsible for FHB susceptibility in wheat, including TaHRC (Su et al. 2019), TaLpx1 (Nalam et al. 2015), TaNPR3 and TaNPR4 (Zhang et al. 2006; Ding et al. 2018; Zhou et al. 2023). To knock out these FHB susceptibility genes, the wheat x maize hybridization combined with genome editing approach as described by Karmacharya et al. (2023) will be used. Haploid embryos are rescued in vitro from the wide crosses to generate haploid plants. PCR amplification and sequencing of the target genes in the haploid plants will confirm the mutations at the target sites. Doubled haploid (DH) plants with the mutations will be produced by colchicine treatment (Niu et al. 2014) and evaluated for FHB resistance in the greenhouse. It is anticipated that DH lines with the susceptibility gene mutated will have improved resistance to FHB.
2.2 Develop and enhance high-throughput screening methods for phenotyping germplasm.
There is a need to conduct higher throughput screening of grain for fungal infection, as screening is laborious and time-consuming. There is an opportunity to employ high-throughput phenotyping methods to quantify ear rot severity. IL has developed high-throughput field-based methods for silk channel inoculations, kernel inoculations, and scoring GER. Using these methods we are able to score thousands of ears per season. We are exploring using imaging and machine learning to increase phenotyping throughput.
2.3 Conduct screening to characterize germplasm for resistance/susceptibility to mycotoxin contamination and improve methodology for breeding for resistance
SD has conducted inoculated FHB disease nurseries for winter and spring barley and evaluated the 29 and 32 entries in replicated trials for disease severity and post-harvest DON content to identify the potential resistant germplasm. Winter barley is a unique promising option for current crop rotations and adds an advantage of ground cover in the winter months for soil conservation.
IL has screened 317 diverse inbred lines in two replications across two years (four total replications) for GER severity and DON and ZEA concentration. From this diverse panel, we have selected 27 resistant, intermediate, and susceptible lines, which we used as parents for the creation of 108 hybrids with varying levels of resistance. Along with collaborators, we have evaluated these hybrids across multiple environments in the Eastern United States and assessed disease severity and mycotoxin accumulation for these hybrids. In addition, we are phenotyping these genotypes for biochemical traits that may be involved in resistance to F. graminearum. We are using these datasets to improve breeding methods for GER and mycotoxin accumulation by developing improved prediction models. We have also identified stable sources of resistance based on these trials.
NDSU aims to identify and characterize genes responsible for resistance or susceptibility to Fusarium head blight (FHB)/mycotoxins in wheat. They propose to isolate resistant genes from the wheat line PI 277012 using the MutChromSeq method (Steuernagel et al. 2017). Initial molecular mapping study detected two QTLs conferring the FHB resistance in PI 277012 (Chu et al. 2011). One of them was fine-mapped and . the whole genome of PI 277012 was sequenced. They generated EMS mutants from GP112 (an FHB-resistant recombinant inbred line selected from the cross between Grandin and PI 277012) and identified FHB-susceptible mutant lines. Flow-sorting and sequencing of chromosome 5A from GP112 and its six EMS mutants that are susceptible to FHB will be conducted. Through the MutChromSeq analysis (Steuernagel et al. 2017), they anticipate identifying candidate genes for the second QTL that will be further characterized and verified using in planta gene expression, targeted mutagenesis by genome editing, and plant transformation.
To identify genes related to FHB susceptibility in durum wheat, they have screened a population of EMS mutants derived from Kronos. 1500 EMS mutagenized individuals from Kronos were generated and mutations in protein-coding regions of each EMS mutant were identified through targeted genome sequencing (Krasileva et al. 2017). 500 EMS mutants along with the parent were evaluated for FHB resistance in the greenhouse and 11 EMS mutants were identified with less susceptibility to FHB compared to the wild type. The genes with mutations in those EMS mutants have been detected using the gene annotation database available for Kronos and its mutants (Krasileva et al. 2017). Those genes with mutations across multiple HB-resistant mutant lines will be selected as candidates for FHB susceptibility. Site-directed mutagenesis by wheat × maize hybridization coupled with CRISPR/Cas9 genome editing as described in (Karmacharya et al. 2023) will be used to confirm if they confer susceptibility to FHB in durum wheat.
2.4 Identify and test microbial detoxification approaches to reduce mycotoxin contamination.
Microbial detoxification offers an eco-friendly and effective solution to mitigate mycotoxin contamination in food and feed. MO conducts In Vitro evaluation testing for microbial detoxification. Specifically, MO has provided adsorption assays to evaluate the ability of microorganisms to bind mycotoxins to their cell walls or extracellular polysaccharides. Meanwhile, MO has helped the In Vivo testing for animal feeding trials with researchers in other institutions in the US (Nava-Ramirez et al. 2024, Maguey-Gonzalez et al. 2023, Deng at al. 2023).
VA and MI (see also below) are conducting studies on microbes from grain crop fields. VA has found microbes capable of tolerating, transporting and/or detoxifying DON.PA has a collection of microbes with the ability to both inhibit the Fusarium Head Blight pathogen Fusarium graminearum and to reduce DON in culture media. This relates to work by PA and MI described below (Objective 3).
Objective 3: Increase understanding of underlying mechanisms that determine mycotoxin production potential and outcomes
There is still much to be learned about the factors influencing mycotoxin accumulation. Work during the 2015-2020 period of NC-1183 by numerous labs has demonstrated clearly that fungal biomass does not correlate tidily with mycotoxin quantity, nor does mycotoxin biosynthetic gene expression. This has very important implications in mycotoxin mitigation suggesting, among other things, that simply trying to reduce or control mycotoxigenic fungi in crops is not an adequate strategy.
3.1 Identify genetic factors contributing to mycotoxin production in the pathogen and resistance in the host
KY has been developing heterothallic mating tester strains of the normally homothallic fungus Fusarium graminearum to conduct controlled crosses and evaluate marker associations for traits contributing to high levels of toxigenicity across varying environments and management protocols. Fusarium graminearum is the causal agent of Fusarium Head Blight on wheat and of Gibberella Ear Rot on corn, and both diseases result in contamination of grains with trichothecene or estrogenic mycotoxins. Although mycotoxin profiles are primarily regulated by the well-characterized TRI gene cluster, different strains that share the same TRI genes nonetheless vary significantly, both quantitatively and qualitatively, in their capacity to produce these mycotoxins under different conditions. Controlled crosses among divergent strains result in Mendelian segregation of SNP markers, and association mapping will reveal QTLs outside the TRI cluster that contribute significantly to variation across different environmental extremes related to climate change (e.g. drought or high temperatures) and different management strategies (e.g. different host genotypes or fungicide treatment protocols). Some of these QTLs may be useful for development of new markers for tracking pathogen population shifts, and for local and regional risk assessment. These genetic analysis protocols will also be useful for developing a deeper understanding of fungal adaptation to different hosts which could lead to more effective management of rotation schemes.
Ongoing transcriptomic studies of A. flavus are revealing genes outside of known mycotoxin biosynthetic pathways that impact mycotoxin production.
Using the diversity panel IL screened for GER severity and DON and ZEA concentration, we are identifying regions of the genome associated with resistance to GER, as well as loci associated with resistance to mycotoxin accumulation. We are also evaluating near-isogenic lines to confirm regions of the genome that we have identified as being involved in resistance to GER. These NILs can also be useful for breeding for resistance.
3.2 Evaluate the role of microbe-microbe interactions and the host microbiome in the production of mycotoxins
MI has been characterizing the root, stalk, leaf, and seed microbiomes of a corn/soy/wheat rotation. Endophytes demonstrating protection against FHB (three identified to date) are being readied for field trials. PA has data on Fusarium species in corn plants differentiated by plant organs and across different cultivars. There is potential to leverage these studies for synergistic activities. The microbiota isolated from different fields may be very site-specific, with novel microbes occurring in each field surveyed; however, there is potential to develop pathogen-inhibiting and mycotoxin-detoxifying microbes for broad application.
Keller lab UW Madison is collaborating on a project on the interactions between AHL quorum sensing gram negative bacteria and Penicillium expansum. P. expansum contaminates various fruits with the lactone mycotoxin patulin. The current study examines if patulin inhibits bacterial quorum sensing, if bacteria degrade and possibly induce patulin.
KY and WV are collaborating on a project to assess the diversity and roles of defensive alkaloids, including lolines, indole-diterpenes, and ergot alkaloids, in wild and cultivated grasses. These alkaloids are produced by mycotoxigenic endophytic fungi and commonly confer a benefit on the host grass (e.g. drought or stress tolerance) while being detrimental to grazing animals, including domesticated hoofstock. The biosynthesis and potential host-protective nature of similar ergot alkaloids found in symbionts of Convolvulaceae and in root and rhizosphere-associated fungi found affiliated with a variety of plants also will be investigated. Proposed experiments will focus on roles of ergot alkaloids in interactions of the producing fungi and their hosts with insects and other microorganisms.
IL is investigating the effect of the microbial community on ear rot severity and mycotoxin accumulation. We are conducting experiments to examine whether microbiome treatments can alter GER severity.
PA plans to examine foliar fungal endophytes in maize leaves with an emphasis on the Fusarium endophyte community, and on relationships between the occurrence of Fusarium species and other fungal endophytes. The overall aim is to better understand the field ecology of mycotoxigenic Fusarium species to inform management approaches. Assessment of the general fungal community will be done using sequences from a portion of the internal transcribed spacer of the ribosomal RNA genes, and assessment of Fusarium will be done using sequences from a portion of the elongation factor 1-alpha gene (Boutigny et al., 2019, Cobo-Diaz et al., 2019). Data analysis will describe species abundance and diversity, as well as associations amongst fungi if they exist. We will also examine, to the extent possible, ecological roles of the fungi. The foliar fungal endophyte populations will be determined for maize grown under drought conditions compared with maize grown with adequate soil moisture using a high tunnel system to control moisture. Field studies will also be conducted to assess the fungal foliar endophytes communities following fungicide, herbicide, and fertilizer treatments that are standardly used in PA maize production.
Objective 4: Engage with stakeholders to increase knowledge transfer and workforce development
4.1 Train the next generation of mycotoxin researchers/food system practitioners
Project participants will continue to actively mentor M.S. and PhD students and postdoctoral researchers working on project objectives. Mycology and Toxicology courses taught by Iowa State University participants incorporate mycotoxin fundamentals and case studies. Lectures on mycotoxin production, symptoms and detection are a routine part of the annual Fusarium Laboratory Workshops taught through KSU. Lectures on mycotoxins are taught annually by Schmale in graduate and undergraduate courses at Virginia Tech. At least two undergraduate students support mycotoxin testing services each year in the Schmale Lab.
4.2 Disseminate mycotoxin-related knowledge to stakeholders across the farm-feed-food continuum
Through venues such as the annual NC-1183 meeting, other scientific meetings (the annual U.S. Wheat and Barley Scab Initiative (USWBSI) Forum, biennial Fusarium workshops conducted in conjunction with the Fungal Genetics Conference, meetings of the Mycological Society of America and the American Phytopathological Society, etc.), and peer-reviewed publications, other stations and stakeholders are kept informed of this work and can respond accordingly in their own states and reach out to the group to share data and resources.
The committee will prioritize creating contributed sessions at appropriate scientific conferences as the group identifies such opportunities. Refereed journal publications will be an important outreach tool for all the listed outcomes. Many of the publications will be on applied research. Also, members who have extension activities will transfer information to grain and food producers. With respect to the outcomes anticipated for Objective 3, several members will present their findings at the annual USWBSI meeting.. Attendees to the forum include growers, millers, representatives of industry, and scientists. Members are involved in international programs sponsored by the USDA and USAID, conducting surveys and workshops and training personnel in Central America, Africa, Nepal, and Afghanistan. We will coordinate with other global efforts in Europe and Africa. Furthermore, the outcomes derived from Objective 1 will be reported at the annual meetings of the Institute of Food Technologists and Experimental Biology, which are major venues for communicating on food toxicology and nutrition toxicology. Strategies for disseminating information to stakeholders outside the scientific community have been developed as part of the KS/NE collaborations on USAID projects (Leslie and Morris, 2020; Leslie et al., 2023) and are readily adaptable to other outreach campaigns.
Measurement of Progress and Results
Outputs
- Information disseminated in the form of peer reviewed literature and presentations at scientific meetings. Comments: This information will include survey results (what practices elevate or reduce risk of mycotoxin contamination of food and feed? What is the prevalence of mycotoxin-associated morbidity in populations? What can be done to ameliorate the problem?); and data on a wide range of subjects (optimal mycotoxin detection and management strategies in different regions and for different foodstuffs; any movements of mycotoxins or mycotoxin-producing fungi; detailed description of host-mycotoxin interactions; environmental, genetic and epigenetic impacts on mycotoxin production). .
- New methods and protocols, such as rapid detection methods for mycotoxins and mycotoxin-producing fungi.
- Plant material resistant to mycotoxin accumulation, such as gene-edited plants or plants infected with beneficial endophytes
- New management tools, such as biological control agents
Outcomes or Projected Impacts
- Implementation of best practices to prevent post-harvest mycotoxin formation in materials such as grains and improve human and animal health. This may be as simple as adequate drying of grains and secure, moisture-controlled storage in places lacking advanced infrastructure. Impact: Reduced risk of mycotoxins in agricultural products Impact: More efficient crop production with fewer losses to mycotoxin contamination
- Deployment of mycotoxin-resistant and/or -detoxifying cereals for human and animal consumption, and resistant forage grasses Impact: Reduced risk of mycotoxins in agricultural products Impact: More efficient crop production with fewer losses to mycotoxin contamination
- Genetic resources related to strain diversity and genetic markers, including characterized strain collections, genome sequences, and mapping populations which will be made available to the community. This information will be helpful in developing a protocol for strain profiling that will allow work with different local strains by partners in different states and countries to be more easily compared. Impact: faster development of genetic resistance to mycotoxin-producing fungi or their mycotoxins.
Milestones
(5):As each objective and sub objective involves different groups of workers and no objective depends on the completion of any other objective before it can be implemented, all objectives (and sub objectives) will follow a similar timeline, proceeding from experiments or surveys to data collection and data analysis, and write-up and summary of results. It is anticipated that data will give rise to new questions and avenues of exploration, which will fall into the scope of existing objectives. Publications will be generated steadily throughout the life of the project, with the first ones coming from areas with preliminary data, and an increase as the project matures in years 3-5.Projected Participation
View Appendix E: ParticipationOutreach Plan
See activities under Objective 4: Engage with stakeholders to increase knowledge transfer and workforce development
Organization/Governance
The executive committee will consist of a chair, vice-chair, secretary and past chair. The executive committee will be elected by the technical committee. Each year a new secretary will be elected and the vice-chair will advance to chair, with the chair; becoming past chair. This committee will conduct business as necessary for the whole committee, between meetings of the technical committee.
The technical committee meeting will be called once a year by the Administrative Adviser. At these meetings, work at the participating stations will be reviewed for progress and for areas needing further effort. When advantageous, efforts will be made to provide for exchange of representatives with other technical committees. Publication of results will be in the form of scientific publications, extension reports or technical bulletins, as appropriate. Attendance at the annual meeting and participation with the group will be monitored on a yearly basis. The committee will discuss with the Administrative Advisor possible remedies for delinquent members.
Duties of Members of the Executive Committee:
Chair - establish location of meeting and coordinate the date with the Administrative Adviser. Notify technical committee members of dates, times and location of meeting and assist members in making accommodations. Call the meetings to order and preside during the meeting. Will become past Chair following Annual Meeting adjournment.
Vice-Chair - will function as the Chair in his/her absence. Becomes chair immediately following the Annual Meeting. Is responsible for writing, getting approval and disseminating the Annual Report.
Secretary - will take minutes for all meetings of the Executive Meeting and the Annual Meeting at which he/she is elected. Is responsible for disseminating copies of the minutes to all Technical Committee members following approval by the Administrative Adviser. Becomes vice chair for the next Annual Meeting.
Literature Cited
Anelli, P., Haidukowski, M., Ferrara, M., Kisikkaya, A., Pembeci, C., Ozer, H., Mulè G., Loi M., Moretti A. & Susca, A. 2024. Monitoring fungi and mycotoxin potential in pistachio nuts of Turkish origin: a snap-shot for climate change scenario. Fungal Biology. DOI: 10.1016/j.funbio.2024.07.009
Boutingny, A.-L., Gautier, A. Basler, R., Dauthieux, F.,Leite, S., Valade, R., Aguayo, J., Ioos, R., and Laval, V. 2019. Metabarcoding targeting the EF- alpha region to assess Fusarium diversity on cereals. PLoS ONE 14(1): e0207988. https://doi.org/10.1371/journal.pone.0207988.
Branstad-Spates, E., Castano-Duque, L.M., Mosher, G., Hurburgh, Jr, C., Rajasekaran, K., Owens, P.R., Winzeler, H.E., Bowers, E. 2024. Predicting fumonisins in Iowa corn: gradient boosting machine learning. Cereal Chemistry.
https://doi.org/10.1002/cche.10824.DOI: https://doi.org/10.1002/cche.10824
Casu, A., Camardo Leggieri, M., Toscano, P., and Battilani, P. 2024. Changing climate, shifting mycotoxins: A comprehensive review of climate change impact on mycotoxin contamination. Comp Rev Food Sci Food Safe 23:e13323. https://doi.org/10.1111/1541-4337.13323.
Chu, C., Niu, Z., Zhong, S., Chao, S., Friesen, T.L., Halley, S., Elias, E.M., Dong, Y., Faris, J.D. and Xu, S.S., 2011. Identification and molecular mapping of two QTLs with major effects for resistance to Fusarium head blight in wheat. Theoretical and Applied Genetics, 123, pp.1107-1119
Cobo-Diaz, J. F., Baroncelli, R., Le Flock, G., and Picot, A. 2019. A novel metabarcoding approach to investigate Fusarium species composition in soil and plant samples. FEMS Microbiology Ecology 95(7) fiz084. https://doi:10.1093/femsex/fiz084.
Cui, H., Wang, S., Yang, X., Zhang, W., Chen, M., Wu, Y., Li, S., Li, L., Cai, D., Guo, B., Ye, J., and Wang, S. 2022. Predictive models for assessing the risk of Fusarium pseudograminearum mycotoxin contamination in post-harvest wheat with multi-parameter sensors. Food Chem. X 16:100472. https://doi.org/10.1016/j.fochx.2022.100472.
Davis, K.A., Jones, A.M., and Panaccione, D.G. 2023. Two satellite gene clusters enhance ergot alkaloid biosynthesis capacity of Aspergillus leporis. Applied and Environmental Microbiology 89:e00793-23.
de Wolf, E., and Paul, P. A. 2014. Predicting Mycotoxin Contamination in Wheat. eds. J. F. Leslie and A. F. Logrieco. Oxford: Blackwell Science Publ.
Deng, Z; Jang, K. B.; Jalukar, S; Du, X.; Kim, S. W. “Efficacy of Feed Additive Containing Bentonite and Enzymatically Hydrolyzed Yeast on Intestinal Health and Growth of Newly Weaned Pigs under Chronic Dietary Challenges of Fumonisin and Aflatoxin”, Toxins. 2023, 15, 433.
Di R, Zhang H, Lawton MA. 2018. Transcriptome analysis of C. elegans reveals novel targets for DON cytotoxicity. Toxins 10:E262.
Ding, Y., Sun, T., Ao, K., Peng, Y., Zhang, Y., Li, X., Zhang, Y. 2018. Opposite roles of salicylic acid receptors NPR1 and NPR3/NPR4 in transcriptional regulation of plant Immunity. Cell, 173, 1454–1467.
Dor S, Nudel K, Eagan JL, Cohen R, Hull CM, Keller NP, Prusky D, Afriat-Jurnou L (2024) Bacterial-fungal crosstalk defined by fungal lactone mycotoxin and its degradation by a bacterial lactonase. Appl Environ Micro. 18;90(6):e0029924
Du, X.; Schrunk, D. E.; Imerman, P. M.; Tahara, J.; Tkachenko, A.; Guag, J.; Reimschuessel R.; Rumbeiha, W. K. “Extensive Evaluation of a Method for Quantitative Measurement of Aflatoxins B1 and M1 in Animal Urine Using High-Performance Liquid Chromatography with Fluorescence Detection”, J. AOAC Int. 2023, 106, 645-651.
EFSA Panel on Contaminants in the Food Chain. (2013) "Scientific Opinion on Risks for Animal and Public Health Related to the Presence of Nivalenol in Food and Feed." EFSA Journal 11, (6), 3262-62
Froment, A., Gautier, P., Nussbaumer, A., and Griffiths, A. 2011. Forecast of mycotoxins levels in soft wheat, durum wheat and maize before harvesting with QualimStreA®. J. Verbrauch. Lebensm. 6:277–281. https://doi.org/10.1007/s00003-010-0655-2.
Johnson, E. T., Proctor, R. H., Dunlap, C. A., and Busman, M. 2018. Reducing production of fumonisin mycotoxins in Fusarium verticillioides by RNA interference. Mycotoxin Res. 34:29–37. https://doi.org/10.1007/s12550-017-0296-8.
Jones, A.M., Davis, K.A., and Panaccione, D.G. 2024. A major facilitator superfamily transporter contributes to ergot alkaloid accumulation but not secretion in Aspergillus leporis. Applied Microbiology 4:406-417.
Karmacharya, A., Li, D., Leng, Y., Shi, G., Liu, Z., Yang, S., Du, Y., Dai, W. and Zhong, S., 2023. Targeting disease susceptibility genes in wheat through wide hybridization with maize expressing cas9 and guide rna. Molecular Plant-Microbe Interactions, 36(9), pp.554-557.
Kaur, H., DiFonzo, C., Chilvers, M., Cassida, K., and Singh, M. P. 2024. Planting time and seeding rate impact insect feeding, ear rots, and forage quality in silage corn. Agronomy Journal, 1-13. https://doi.org/10.1002/agj2.21620
Kaur, H., Durst, P., Kaatz, P., and Singh, M.P. 2024. Occurrence and associated agronomic factors of mycotoxin contamination in silage maize in the Great Lakes region. World Mycotoxin Journal. https://doi.org/10.1163/18750796-bja10005
Kim, H.-S., Lohmar, J. M., Busman, M., Brown, D. W., Naumann, T. A., Divon, H. H., Uhlig, S., and Proctor, R. H. 2020. Identification and distribution of gene clusters required for synthesis of sphingolipid metabolism inhibitors in diverse species of the filamentous fungusFusarium. BMC Genomics 21:510. https://doi.org/10.1186/s12864-020-06896-1.
Krasileva, K.V., Vasquez-Gross, H.A., Howell, T., Bailey, P., Paraiso, F., Clissold, L., Simmonds, J., Ramirez-Gonzalez, R.H., Wang, X., Borrill, P. and Fosker, C., 2017. Uncovering hidden variation in polyploid wheat. Proceedings of the National Academy of Sciences, 114(6), pp. E913-E921.
Krska R, Leslie JF, Haughey S, Dean M, Bless Y, McNerney O, Elliott C, Spence M. 2022. Effective approaches for early identification and proactive mitigation of aflatoxin in peanuts – An EU-China perspective. Comprehensive Reviews in Food Science and Food Safety 21: 3227-3243. DOI: 10.1111/1541-4337.12973.
Leslie JF, Morris JB. 2020. Talking about mycotoxins. Frontiers in Sustainable Food Production:Agro-Food Safety 3: 109. DOI: 10.3389/fsufs.2019.00109.
Leslie JF, Poschmaier B, van Egmond H, Malachová, A, de Nijs M, Bagi F, Zhou J, Jin Z, Wang S, Suman M, Schatzmayr G, Krska, R. 2020. The MyToolbox EU-China partnership – Progress and future directions in mycotoxin research and management. Toxins 12(11): 712. DOI: org/10.3390/toxins12110712.
Leslie, J. F., Moretti, A., Mesterházy, Á., Ameye, M., Audenaert, K., Singh, P. K., Richard-Forget F, Chulze SN, Del Ponte EM, Chala A, Battilani P, Logrieco AF. 2021. Key global actions for mycotoxin management in wheat and other small grains. Toxins 13(10): 725. DOI: 10.3390/toxins13100725.
Leslie JF, Morris, JB, Gurung, JK, Harvey, JJW, Ayalew A, Baker R, Zhang G. 2023. Mycotoxin communications: Managing messages for different audiences. Frontiers in Sustainable Food Systems 6: 1095256. DOI: 10.3389/fsufs.2022.1095256.
Liu X, Wang L, Choera T, Fang X, Wang G, Chen W, Lee YW, Mohamed SR, Dawood DH, Shi J, Xu J, Keller NP (2023) Paralogous FgIDO genes with differential roles in tryptophan catabolism, fungal development and virulence in Fusarium graminearum. Microbiol Res Apr 6;272:127382. doi: 10.1016/j.micres.2023.127382.
Logrieco, A., Battilani, P., Leggieri, M. C., Jiang, Y., Haesaert, G., Lanubile, A., Mahuku, G., Mesterházy, A., Ortega-Beltran, A., Pasti, M., Smeu, I., Torres, A., Xu, J., and Munkvold, G. 2021. Perspectives on Global Mycotoxin Issues and Management From the MycoKey Maize Working Group. Plant Disease 105:525–537. https://doi.org/10.1094/PDIS-06-20-1322-FE.
Luciano-Rosario D, Peng H, Gaskins VL, Fonseca JM, Keller NP, Jurick WM 2nd. (2023) Mining the Penicillium expansum Genome for Virulence Genes: A Functional-Based Approach to Discover Novel Loci Mediating Blue Mold Decay of Apple Fruit. J Fungi (Basel) 9(11):1066. doi: 10.3390/jof9111066.
Luciano-Rosario D, Barda O, Tannous J, Frawley D, Bayram O, Edward Sionov E, Dov Prusky D, Keller NP (2023) The histone demethylase KdmB is part of a trimeric protein complex and mediates virulence and patulin production in Penicillium expansum Fungal Genetics Biol. doi: 10.1016/j.fgb.2023.103837. Online ahead of print. PMID: 37722619
Maguey-Gonzalez, J. A.; Nava-Ramirez, M. J.; Gomez-Rosales, S.; Angeles, M. L.; Solis-Cruz, B.; Du, X.; Hargis, B. M.; Tellez-Isaias, G. “Evaluation of the efficacy of humic acids to counteract the toxic effects of aflatoxin B1 in turkey poults”, Front. Vet. Sci. 2023, 10, 1-11.
Mandap, J.A.L., Hellmich, R.L., Busman, M., Maier, D.E., and Munkvold, G.P. 2024. Aspergillus flavus and stored grain insect interactions in stored conventional and transgenic maize hybrids. J. Stored Prod. Res. 106. https://doi.org/10.1016/j.jspr.2024.102258
Marin, S., Freire, L., Femenias, A., and Sant’Ana, A. S. 2021. Use of predictive modelling as tool for prevention of fungal spoilage at different points of the food chain. Curr. Opin. Food Sci. 41:1–7. https://doi.org/10.1016/j.cofs.2021.02.006.
McMaster N, Acharya B, Harich K, Grothe J, Mehl H, Schmale DG. 2019. Quantification of the mycotoxin deoxynivalenol (DON) in sorghum using GC-MS and a stable isotope dilution assay (SIDA). Food Anal Methods 12:2334-2343.
Mendoza JR, Sabillón L, Martinez W, Campabadal C, Hallen-Adams HE, Bianchini A. 2017. Traditional maize post-harvest management practices amongst smallholder farmers in Guatemala. J Stored Products Res 71:14-21.
Mendoza JR, Rodas A, Oliva A, Sabillón L, Colmenares A, Clarke J, Hallen-Adams HE, Campabadal C, Bianchini A. 2018. Safety and quality assessment of smallholder farmers’ maize in the Western Highlands of Guatemala. J Food Prot 81:776-784.
Munkvold, G.P., Arias, S.L., Taschl, I., and Gruber-Dorninger, C. 2019. Mycotoxins in Corn – Occurrence, Impacts, and Management. Ch. 9 in Corn Chemistry and Technology, 3rd Edition. Serna-Saldivar, S., Ed. Am. Assoc. Cereal Chemists, St. Paul, MN
Munkvold, G.P., Proctor, R.H., and Moretti, A. 2021. Mycotoxin production in Fusarium according to contemporary species concepts. Annu. Rev. Phytopathol. 59: 373–402 https://doi.org/10.1146/annurev-phyto-020620-102825
Nalam, V.J., Alam, S., Keereetaweep, J., Venables, B., Burdan, D., Lee, H., Trick, H.N., Sarowar, S., Makandar, R. and Shah, J., 2015. Facilitation of Fusarium graminearum infection by 9-lipoxygenases in Arabidopsis and wheat. Molecular Plant-Microbe Interactions, 28(10), pp.1142-1152.
Nava-Ramirez, M. J.; Maguey-Gonzalez, J. A.; Gomez-Rosales, S.; Hernandez-Ramirez J. O.; Latorre, J. D.; Du, X.; Lopez-Coello, C.; Hargis, B. M.; Tellez-Isaias, G.; Vazquez-Duran, A.; Mendez-Albores, A. “Efficacy of powdered alfalfa leaves to ameliorate the toxic effects of aflatoxin B1 in turkey poults”, Mycotoxin Res. 2024, 40, 269-277.
Nesic, K., Milicevic, D., Nesic, V., and Ivanovic, S. 2015. Mycotoxins as one of the foodborne risks most susceptible to climatic change. In 58TH INTERNATIONAL MEAT INDUSTRY CONFERENCE (MEATCON2015), Procedia Food Science, eds. I. Nastasijevic, R. Fries, and S. Avery. Amsterdam: Elsevier Science Bv, pp. 207–210. https://doi.org/10.1016/j.profoo.2015.09.058.
Niu, Z., Jiang, A., Abu Hammad, W., Oladzadabbasabadi, A., Xu, S.S., Mergoum, M. and Elias, E.M., 2014. Review of doubled haploid production in durum and common wheat through wheat× maize hybridization. Plant Breeding, 133(3), pp.313-320.
NTP (2001) Technical Report on the Toxicology and Carcinogenesis Studies of Fumonisin B1 (CAS NO. 116355-83-0) in F344/N Rats AND B6C3F1 MICE (Feed Studies). NTP TR 496.( U.S. Dept. of Health and Human Services, NIH Publication No. 01-3955) EFSA 2011b, 'Scientific opinion on the risks for public health related to the presence of zearalenone in food', EFSA journal, vol. 9, no. 6, pp. 2197-n/a.
Okello PN, Petrović K, Kontz B, Mathew FM. 2019. Eight species of Fusarium cause root rot of corn (Zea mays) in South Dakota. Plant Health Progress 20:38-43.
Pack, E., Meyerhoff, K., Schmale, D.G. 2021. Tracking zearalenone and type-B trichothecene mycotoxins in the commercial production of beer and brewers’ spent grains. Journal of American Society of Brewing Chemists, DOI: 10.1080/03610470.2021.1938489.
Pack, E., Weiland, S., Musser, R., Schmale, D.G. 2021. Survey of zearalenone and type-B trichothecene mycotoxins in swine feed in the United States of America. Mycotoxin Research, 37: 297–313.
Perrone, G., Ferrara, M., Medina, A., Pascale, M., and Magan, N. 2020. Toxigenic Fungi and Mycotoxins in a Climate Change Scenario: Ecology, Genomics, Distribution, Prediction and Prevention of the Risk. Microorganisms 8:1496. https://doi.org/10.3390/microorganisms8101496.
Sabillón, L., Alvarado J, Leiva A, Mendoza R, Espinal R, Leslie JF, Bianchini A. 2023. Presence, co-occurrence, and daily intake estimates of aflatoxins and fumonisins in maize consumed in food-insecure regions of western Honduras. Toxins 15(9): 559. DOI: 10.3390/toxins15090559.
Singh, M.P., DiFonzo, C., Fusilier, K., Kaur, H., and Chilvers, M. 2023. Insect ear-feeding impacts gibberella ear rot and deoxynivalenol accumulation in corn grain. Crop, Forage, and Turfgrass Management. 10(1). https://doi.org/10.1002/cft2.20258
Soffa, D. R., Stewart, J. W., Pack, E. D., Arneson, A. G., De Vita, R., Knight, J. W., ... and Rhoads, M. L. 2023. Short-term consumption of the mycotoxin zearalenone by pubertal gilts causes persistent changes in the histoarchitecture of reproductive tissues. Journal of Animal Science, 101, skac421.
Steuernagel, B., Vrana, J., Karafiatova, M., Wulff, B.B. and Doležel, J., 2017. Rapid gene isolation using MutChromSeq. Methods Mol Biol., 1659, pp231–243.
Su, Z., Bernardo, A., Tian, B., Chen, H., Wang, S., Ma, H., Cai, S., Liu, D., Zhang, D., Li, T. and Trick, H., 2019. A deletion mutation in TaHRC confers Fhb1 resistance to Fusarium head blight in wheat. Nature Genetics, 51(7), pp.1099-1105.
Valverde-Bogantes E, Bianchini A, Herr JR, Rose DJ, Wegulo SN, Hallen-Adams HE. 2019. Recent population changes of Fusarium head blight pathogens: drivers and implications. Can J Plant Pathol 2019 https://doi.org/10/1080/07060661.2019.1680442.
Wang, X., Liu, C., and van der Fels-Klerx, H. J. 2022. Regional prediction of multi-mycotoxin contamination of wheat in Europe using machine learning. Food Res. Int. 159:111588. https://doi.org/10.1016/j.foodres.2022.111588.
WHO. (2002) Evaluation of Certain Mycotoxins in Food: Fifty-Sixth Report of the Joint Fao/Who Expert Committee on Food Additives. WHO Technical Report Series, 906: 62 pp
WHO (2011) Safety evaluation of certain contaminants in food: 72nd report of the joint FAO/WHO expert committee on food additives. Geneva: World Health Organization (WHO Food Additives Series 63)
Windham GL, Williams WP, Mylroie JE, Reid CX, Womack ED. 2018. A histological study of Aspergillus flavus colonization of wound-inoculated maize kernels of resistant and susceptible maize hybrids in the field. Front Microbiol 9:799.
Yang K, Luo Y, Sun T, Qiu H, Geng Q, Li Y, Liu M, Keller NP, Song F, Tian J (2024) Nitric oxide-mediated regulation of Aspergillus flavus asexual development by targeting TCA cycle and mitochondrial function J Hazard Mater. 5;471:134385.
Yu, J., Hennessy, D.A., and Wu, F. 2024. Bt corn and cotton planting may benefit peanut growers by reducing aflatoxin risk. Plant Biotechnology Journal (2024), pp. 1–9. https://doi.org/10.1111/pbi.14425
Zhang, Y., Cheng, Y.T., Qu, N., Zhao, Q., Bi, D., Li, X. 2006. Negative regulation of defense responses in Arabidopsis by two NPR1 paralogs. Plant J 48, 647–656.
Zhou, P., Zavaliev, R., Xiang, Y. and Dong, X., 2023. Seeing is believing: Understanding functions of NPR1 and its paralogs in plant immunity through cellular and structural analyses. Current Opinion in Plant Biology, 73, p.102352.