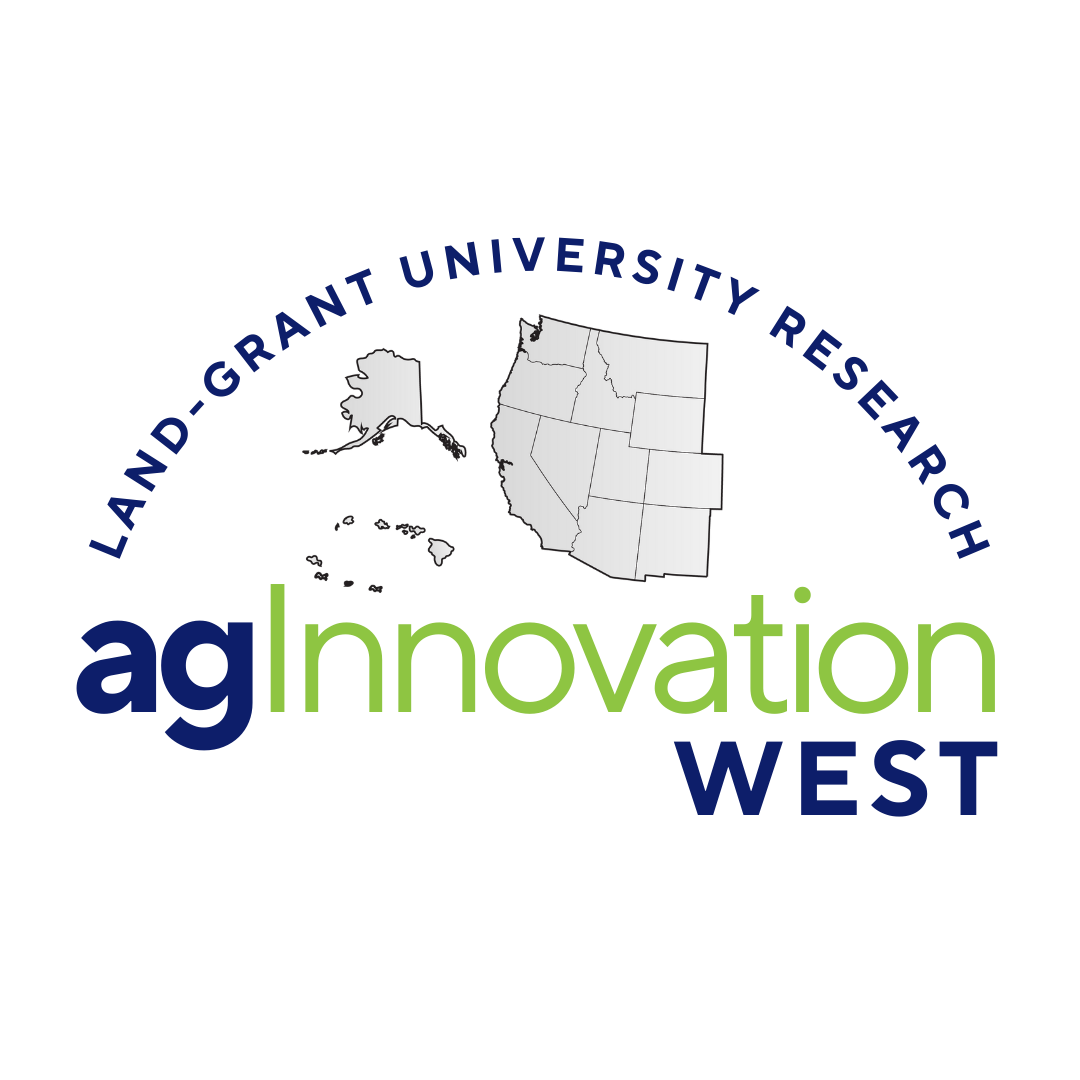
W5170: Beneficial Use of Residuals to Improve Soil Health and Protect Public, and Ecosystem Health
(Multistate Research Project)
Status: Approved Pending Start Date
W5170: Beneficial Use of Residuals to Improve Soil Health and Protect Public, and Ecosystem Health
Duration: 10/01/2024 to 09/30/2029
Administrative Advisor(s):
NIFA Reps:
Non-Technical Summary
According to the USEPA, over 292 million tons of residual by-products, such as urban wastes (biosolids, recycled water, food scraps and other municipal solid waste), agricultural waste (manure), industrial sludges and waste byproducts are currently produced in the U.S. On average, approximately 50% of biosolids, 98% of food scraps, and 45% of yard trimmings are currently disposed in landfills or incinerated at a substantial cost. Thus, increasing the reuse of various residual materials for land application as soil amendments can potentially reduce disposal costs while increasing their beneficial agronomic and environmental uses. There is a high demand for wastewater recycling and reuse for agricultural production. In addition, treated liquid wastes, such as wastewater effluent, recycled water and other non-potable waters, present opportunities for beneficial reuse in lieu of their discharge into surface waters or expensive treatment. There have been many obstacles to the optimized use of residuals. These include a lack of research to optimize residual-based product development, conflicting regulations or the absence of regulations on residuals use, and lack of public outreach and communication. The project will aim to enable the development of guidelines for the sustainable use of a wide variety of residuals and residual by-products, maximizing benefits while minimizing the potential for unintended negative consequences.
Statement of Issues and Justification
STATEMENT OF ISSUES
Over 292 million tons of municipal solid waste are generated in the U.S., with approximately 1/3 utilized for recycling and composting (both of which include land application; US EPA, 2023). According to the US EPA (2018), approximately 48% of these materials are either composted, combusted for energy recovery or recycled, respectively, while the remaining 52% are landfilled. Specifically in terms of material disposal, ~50% of biosolids, 98% of food scraps and 45% of yard trimmings are currently landfilled or incinerated at a substantial cost to the industry and public (King et al., 2011). Thus, increasing reuse of various residual materials such as valuable soil amendments offers the potential to replace disposal costs with beneficial agronomic and environmental uses. It is predicted that 52% of the world’s population will live in regions under water stress by 2050 (Schlosser et al., 2014), resulting in high demand for wastewater recycling and reuse for agricultural production. In addition, treated liquid wastes, such as wastewater effluent, recycled water and other non-potable waters, present opportunities for beneficial reuse in lieu of surface water discharge or expensive treatment. However, there are consistently numerous obstacles that prevent the full beneficial utilization of the above materials.
Obstacles that limit the beneficial use of residual products include conflicting regulations or the absence of regulations on residuals use, lack of public outreach and communication, and limited research on optimizing residuals-based product development. Fortunately, current and increasing evidence exits exemplifying that land application of a variety of residuals do provide agronomic and environmental benefits, which were either not previously well understood or are critical to addressing emerging environmental issues (e.g., system resiliency in the face of changing environmental factors highlighted in Brown et al., 2011a,b). The W4170 workgroup, who consists of some of the best minds and resources within and outside the State Agricultural Experiment Station System (e.g., US EPA, municipality personnel, private entities), proposes to continue to conduct research towards sustainable use of residual products. The W4170 proposes to continue investigating the biogeochemical cycling of plant nutrients, movement and potential adverse impacts of trace element and emerging contaminants within ecosystems and the food chain, their long-term bioavailability in residual-amended soils, and soil health effects from application of residuals. These investigations will generate knowledge needed to develop and promote residuals recycling practices that are protective of human health and the environment. Outcomes will provide data for continuing one-health based risk assessment required by the US EPA Part 503 Rule for biosolids land-application as well as for developing regulations for land-based recycling of residuals and reclaimed water. Research will also focus on reuse benefits including field and watershed scale effects on soil quality/health, plant drought response, soil carbon sequestration, water quality, greenhouse gas emissions and climate change impacts associated with soil-based residuals and reclaimed water reuse. We will also explore the potential for residual based product usage in urban and agriculture systems, and green stormwater infrastructures as well in restoration of degraded lands. W4170 members are currently conducting and synthesizing both short- and long-term research that will enable the development of guidelines for the sustainable use of a wide variety of residuals and residual by-products: maximizing benefits while minimizing the potential for unintended negative consequences.
JUSTIFICATION:
The beneficial use of residuals requires an understanding of both potential hazards and the value of constituents in the by-products. Investigation of the fate and behavior of contaminants and nutrients in biosolids-amended soils has been the historic research focus of the W4170 multistate workgroup. Research conducted previously by the workgroup formed the basis for the U.S. EPA Part 503 biosolids rule, which is one of the few rules to include bioavailability assessments in the development of limits for critical contaminants (National Research Council, 2009). Two subsequent NAS reviews, focused on the 503 rule, confirmed that the scientific basis for this rule and risk assessment were valid. Members of the W4170 group have contributed research and technical expertise that has led to guidance that permit beneficial reuse of many industrial and municipal byproduct for land application or as feedstocks in manufactured soil blends, including spent foundry sands (U.S. EPA, 2014), water treatment waste residuals, and many other residuals. Research conducted by W4170 members included evaluating the occurrence of per- and polyfluoroalkyl substances (PFAS), a large family of chemicals of high concern in biosolids including comparing with non-biosolids-based compost (Lazcano-Kim et al., 2020; Schaefer et al, 2022; Bolan et al., 2021) as well as the effect of treatment on PFAS presence in biosolids (Lazcano-Kim et al., 2019; Li and Koosaletse-Mswela, 2023). PFAS analysis in municipal solid waste composts from across the country (Choi et al., 2019) contributed to the successful passage in 2018 of the State of Washington’s Healthy Food Packaging Act which bans the use of the entire class of PFAS in paper food packaging after January 1, 2022 assuming suitable alternatives are identified (HB2658-2017-18). This type of law will help prevent the use of products from other countries where PFAS-related bans may not exist and will prevent unwanted contaminants in our food, reduce their input into wastewater treatment plants, thus biosolids, and will minimize their entry into compostable materials where their presence has been pervasive. Research by members included PFAS presence and release from drinking water treatment residuals and their subsequent potential to be applied with biosolids to reduce PFAS leaching and crop uptake (Graveson et al., 2023; Broadbent et al, 2023). Additional work included synthesizing data on plant uptake of PFAS and uptake mechanisms, which guided current research underway (Costello et al., 2023; Wang et al., 2020). More recently, W4170 members also performed laboratory and field studies to quantify the occurrence, persistence, fate and plant uptake of PFAS. Studies to date highlight the importance and magnitude of perfluoroalkyl acid (PFAA) precursors such as the polyfluoroalkyl phosphate esters (diPAPs) present in biosolids when land-applied serve as a long-term source of more mobile PFAA after land-applied. Also, field data consistently show low to negligible precursors in the soil profile within one year after biosolids have been applied, an accumulation of the longer-chain PFAS in the upper 1-2 feet of soil, although some are leached, and migration of shorter-chain PFAS through the soil profile (manuscripts in preparation). Data gaps include what level of PFAS in soils will lead to levels below concern in plants and mitigate strategies from crop selection and management to sorbent additions, particularly in heavily PFAS-contaminated agricultural fields.
Recently, the U.S. EPA Office of Inspector General released their report “EPA unable to assess the impact of hundreds of unregulated pollutants in land-applied biosolids on human health and the environment” (U.S. EPA, 2018). The U.S. EPA “identified 352 pollutants in biosolids but cannot yet consider these pollutants for further regulation due to either a lack of data or risk assessment tools. Pollutants found in biosolids can include pharmaceuticals, steroids, and flame retardants.” The W4170 committee is “the” USDA NIFA Multistate Committee that can continue to provide data and technical expertise to U.S. EPA for addressing the regulatory needs they identified in the U.S. EPA OIG report. U.S. EPA Office of Water and Office of Research and Development personnel continue to be active members of W4170. Our university members are recognized internationally as leaders in research on the environmental fate of emerging contaminants, e.g, pharmaceuticals, steroids, chemicals from personal care products, flame retardants, microplastics, and PFAS, that can enter the environment with land-applied biosolids. In 2020, the W4170 group wrote a comprehensive review to respond to the request of biosolids stakeholders for W4170 to provide a scientific review of the OIG Report. The review (available at: https://www.nimss.org/system/ProjectAttachment/files/000/000/502/original/W4170%20Response%20to%20OIG%20Report%20July%2023%202020%20final.pdf) addressed chemical, antibiotic, and pathogen issues raised in the OIG report. The W4170 team concluded that OIG report was flawed and that the science of beneficial use of biosolids was lacking. Addressing U.S.EPA OIG data and research gaps will be a priority in the proposed W4170 renewed project.
Human and ecological risk assessment (HERA) science continues to mature with biogeochemical availability-based risk assessment frameworks being developed and/or considered for implementation in the U.S., Canada, the European Union, Australia and other countries. Continued research is needed to provide the scientific basis for risk-based methods and to evaluate residuals and residual-treated soils for adoption by HERA frameworks. Research needed to evaluate contaminants in residuals includes the following: (i) trace element speciation by advanced spectroscopic methods and wet chemical speciation methods; (ii) chemical lability and plant uptake potential of frequently detected trace elements and emerging contaminants in residuals-amended soils in the field; (iii) use of bioavailability to adjust human and ecological exposure in risk assessment frameworks by using inexpensive novel bioaccessibility methods and in vivo methods; and (iv) total combined effects of residuals on soil physical, chemical, nutrient and biological components in order to ascertain alterations in soil quality/health following residuals land application.
Several members of the W4170 were extensively involved in the development of the Part 503 regulation and continue to be involved in the development of risk assessment for other residuals (e.g., the EPA risk assessment for land application of cement kiln dust and foundry sand) and for other pollutants not initially regulated or considered in Part 503 (e.g., barium, PFAS, microplastics, and other emerging contaminants). The scientific approach used in the development of Part 503 has been applied by this group to an expanded variety of residuals, contaminants and receptors. As the understanding of bioavailability is expanding, the group is broadening its focus to develop linkages between quantitative understanding of the contaminant forms and their bioavailability to a range of receptors.
In the past, our group has conducted cooperative projects involving laboratory incubation, greenhouse studies and X-ray absorption spectroscopy to elucidate the role of organic and inorganic components of biosolids and other residuals in binding metals (Brown et al., 2003a; Hettiarachchi et al., 2003a, b, 2006; Ippolito et al., 2009, 2013; Obrycki et al., 2016; Ryan et al., 2003, 2004a; Scheckel et al., 2004). We have demonstrated that biosolids and other soil amendments can reduce the bioavailability of metals in contaminated systems (e.g., Attanayake et al., 2014; 2015; 2017; Barbarick et al., 2015, 2016, 2017; Basta et al., 2001; Brown et al 2003b, 2007; DeVolder et al., 2003; Hettiarachchi and Pierzynski, 2002; Ippolito et al., 2010, 2014; Meiman et al., 2012), while developing various extractants to assess bioavailability of mineral and bioavailable fractions of inorganic contaminants (e.g., Basta and Juhasz, 2014; Basta et al., 2003; Brown et al., 2004; Rodriguez et al., 2003; Ryan et al., 2004; Schroder et al., 2003; Whitacre et al., 2017).
Our W4170 group members have conducted research on occurrence, sorption, transformation, plant uptake, and remediation of PFAS and pharmaceuticals in the environment (Chen et al., 2010; Choi et al., 2023; Ding et al., 2011; Dodgen et al., 2015; Fu et al., 2019; Fu et al., 2016; Gravesen et al., 2023; Kim et al., 2022; Lazcano et al., 2020; Lazcano et al., 2019; Li et al., 2022a; Li et al., 2022b; Song et al., 2010; Wang et al., 2009; Wang et al., 2021; Wang et al., 2022; Wu et al., 2014; Wu et al., 2013). The trace-levels of antibiotics (e.g. tetracyclines) could exert selective pressure on E. coli to express antibiotic resistant genes, and the magnitude of selective pressure is determined not only by the concentration but also by the surrounding environmental chemistry and speciation tetracycline in environmental matrices (Chen et al., 2015; Zhang et al., 2014a, b). Soil-sorbed tetracyclines are still bioavailable to bacteria to evoke the expression of antibiotic resistance as long as the bacteria are closely attached to soil surfaces (Chen et al., 2017; Zhang et al., 2018). Smaller-sized pharmaceuticals demonstrate a higher translocation potential to plant leaves (Chuang et al., 2019). Pharmaceuticals in soil pore water are the major fraction in soils bioavailable to plants for uptake (Li et al., 2020; Li et al., 2019; Li et al., 2022c).
Our group’s research has broadened to encompass a range of measurement endpoints as we realize and understand the potential for metals to affect a range of receptors. The goal of this research is to evaluate functions of restored ecosystems utilizing tools such as in vivo and in vitro assays, toxicity assays and measures of microbial community dynamics (Alasmary et al., 2020; 2021; Alexander et al., 2003; Basta and Juhasz, 2014; Bastaand Wragg, 2013; Brown et al., 2004; Hettiarachchi et al., 2003a; Schroder et al., 2003; Sullivan et al., 2005). As a result of cooperative research conducted by members of W4170, in situ remediation options, including biosolids and other residuals, have been utilized at several EPA Superfund NPL (National Priority List) sites, contaminated urban soils, mine lands, forest fire burned areas and overgrazed and degraded ecosystem sites. The tools developed for this research have broadened our understanding on the functioning of soils amended with biosolids or residuals.
The sustainability of biosolids application to agricultural lands has also been demonstrated by evaluating the effects of biosolids application on various aspects of soil functionality, recently termed “soil health.” While the implications of this research are being recognized in agroecosystems and remediation of contaminated sites, such research is also needed for rehabilitation of urban landscapes. Among the greatly expanding potential markets for exceptional quality (EQ) biosolids are used for urban agriculture, turfgrass and other vegetated urban landscapes on anthropogenic soils.
Among the challenges in urban agriculture and other anthropogenic soil landscapes are their commonly degraded nature and potential presence of contaminants in such soils. In contrast to natural soils, human activities strongly influence urban soils through the removal of topsoil, physical disturbances, heavy vehicle traffic and addition of foreign materials (e.g., asphalt, bricks, glass, plastic, etc.), which ultimately result in a highly impaired soil (Craul, 1985; Gregory et al., 2006; Beniston and Lal, 2012; Chaganti and Crohn, 2014). These disturbed soils are characterized by soil compaction, increased bulk density, reduced water infiltration rates and aeration, low water holding capacity, low organic matter content and low fertility, which ultimately limit crop growth and yields and create major constraints for productive urban agriculture (Gregory et al., 2006; Beniston and Lal, 2012; De Sousa et al., 2023).
EQ biosolids can provide organic matter and nutrients necessary to improve anthropogenic soils and promote vegetative establishment and growth. EQ biosolids are by-products of wastewater treatment generated by Processes to Further Reduce Pathogens (PFRPs) and possess low vector attraction and pollutant concentrations. Such products are suitable amendments for landscapes having high public access. Research needs for these newly developed products include quantifying N and P availability and C sequestration potential in anthropogenic soils. Initial work on these questions has been performed (Alvarez-Campos et al., 2018; Alvarez-Campos and Evanylo, 2019a; Brown et al., 2016c), but additional research is needed to ensure appropriate EQ biosolids management for urban landscapes. Biosolids blended with compost and/or other residuals have been shown useful in reducing exposure to Pb contaminated soil, improve soil health and protect public health (Obrycki et al., 2017b). The multistate research group will continue research to improve our understanding of this in field studies, focusing on changes in soil attributes that may have implications for altering soil quality/health (e.g., soil physical, chemical, nutrient, and biological characteristics).
In particular, some of the greatest challenges for expanding the beneficial use of treated wastewater effluent and residuals (such as biosolids) are concerns associated with PFAS compounds. Based on their extensive use in a myriad of products, their existence in the environment is ubiquitous. Land application of biosolids has been shown to disseminate PFAS in soils and subsequently be assimilated by crops and leached to groundwater (Bizkarguenaga et al., 2016; Blaine et al., 2014a, b; Lee et al., 2014; Lindstrom et al., 2011; Sepulvado, et al., 2011; Navarro et al., 2017; Wang et al., 2020). Biological degradation processes that often time reduce trace organic chemicals loads in wastewater treatment and in land-applied biosolids do not alleviate PFAS load since any microbially degradation that occurs leads to persistent perfluoroalkyl acids (PFAAs) (Liu et al., 2017). Even small contributions of PFAAs to water sources can be problematic from a management standpoint given the very low thresholds (at ppt levels) being proposed (4 ppt, USEPA) or already being implemented as screening levels or actual health advisory levels by some states (e.g., 14 ppt in NY). On March 22, 2019, Maine’s Department of Environmental Protection (DEP) released a memorandum requiring sludge/biosolids program licensees and sludge/biosolids composting facilities to test their materials for PFAS. Any material exceeding the screening standards set for three PFAAs (2.5 mg/kg PFOA, 5.2 mg/kg PFOS or 1,900 mg/kg PFBS) may not be land-applied until DEP approves otherwise.
Another area of concern for the W4170 workgroup is irrigation with water resource recovered from and/or reuse of wastewaters. This particularly holds true due to the fact that fresh water sources for irrigation are limited, and impending water shortages are motivating the intentional reuse of degraded waters (Blaine et al., 2014b). While the application of treated or partially treated wastewater effluents to cropped and forested lands has long been practiced, other sources of degraded water (stormwater, irrigation return flow, gray water, and CAFO effluents) are available to meet anticipated shortfalls. In conjunction with degraded water use, soil application of residual products may, in fact, have positive effects on drought-stressed crops. Several researchers have noted improvements in crop performance with the biosolids land application (e.g., Cogger et al., 2013; Heckman et al., 1987; Lu et al., 2020), with speculation geared towards improvements in soil water relations (Brown et al., 2011a,b). Since many of the major reuse opportunities involve water applications to soil systems (e.g., irrigation), with or without residuals application, addressing the benefits, risks, and sustainability of degraded water reuse logically fits within the scope of the proposed research project. As resource limitations spur development of reuse technologies and renewable commodities, wastewater should be viewed as a renewable resource that contains valuable raw materials. Indeed, many resource-stressed communities have begun to adopt resource recovery systems (RRS), particularly for water. Adoption of resource recovery from, and reuse of, wastewater depends on technological innovations that increase technology efficiencies and lower capital and operational costs. Members of our team are working with academics and professional engineers on valorization of carbon, nutrients (N&P), and water from agricultural, municipal, and industrial wastewaters and their beneficial reuse potential in agricultural systems (Heronemus et al., 2021; Awasthi et al., 2023; Kannan et al., 2023).
In summary, there is a continued need for research on contaminant mobility and bioavailability in both residual-amended soils and in contaminated soils restored with residuals. New ecological endpoints must be investigated to improve risk assessment to ensure human health and environmental safety. Research is also needed to maximize yields via the use of residuals and reclaimed wastewater. Both offer potential alternatives to current, sometimes environmentally detrimental, agricultural and land restoration practices. The experience and expertise of W4170 in addressing these issues are widely recognized and W4170 is well positioned for continued success. The W4170 membership offers the advantage of including institutions and entities (universities, USDA-ARS, US EPA, municipal government utilities) from across the entire US. Such collaboration enables discoveries to account for widespread differences in climate, soils and residual types and sources, whose fate, transport and impact will vary due to across these factors.
Related, Current and Previous Work
W4170 Objective 1. Evaluate the short- and long-term chemistry and bioavailability of PFAS, pharmaceuticals and personal care products (PPCPs), persistent organic contaminants, microplastics, and pathogens in residuals, reclaimed water, and biosolids-amended soils in order to assess the environmental and human health risk-based effects of their application at a watershed scale.
Early work on the effect of residuals application to agricultural or undisturbed soils focused on changes in total metal and plant-available metal concentrations. As our understanding of ecosystem function and nutrient cycling has become increasingly sophisticated, additional measures that include a wider range of endpoints are being included in research efforts. W4170, the premiere US residuals reuse workgroup, has continued to focus efforts on cutting-edge topics such as reductions in emerging contaminants associated with beneficial use of residuals (e.g., solids, semi-solids, reclaimed waters, wastewaters) in amended soils.
Fate of residuals-borne emerging contaminants in wastewaters and residuals-amended soils. Classes of emerging contaminants include estrogenic compounds, pharmaceuticals and personal care products (PPCPs) and per- and polyfluoroalkyl substances (PFAS) (Codling et al., 2018; Dalahmeh et al., 2018; Stahl et al., 2013; Sepulvado et al., 2011; Xia et al. 2010). Quantifying the persistence and fate of emerging contaminants in soils and evaluating their impacts to soil health and the ecosystem are critical areas requiring additional research. The W4170 workgroup has been consistently diligent in studying the fate and effects of emerging contaminants for a wide array of scenarios.
Microplastic particles (size of 1 μm - 5 mm) are a contaminant of emerging concern in wastewater and biosolids. In CA, fundamental and applied research was carried out to advance microplastics field sampling, laboratory processing and analysis, and data analysis techniques in service of this objective through projects funded by NOAA Marine Debris Research and the California State Water Board. Collaborators include the Southern California Coastal Water Research Project, the Santa Ana Regional Water Quality Control Board, Orange County Sanitation District, Orange County Environmental Resources, and the Los Angeles County Department of Public Works. Collaborative research among W4170 members from CA and IN and University of Cincinnati resulted in a funded project through USEPA’s National Priorities – Evaluation of Pollutants in Biosolids program. This project is expected to expand the team research on TOrCs to consider processes, risks and management in land applications of biosolids.
Antibiotics are frequently found in soil and water environments, thus the potential to exert selective pressure on surrounding bacteria for development and enrichment of antibiotic resistant strains. Members of the W4170 demonstrated that manure application methods did not affect dissipation rates of antibiotics in soil with some antibiotics remained detectable in soils 180 days after manure application. Field investigation and simulated rainfall studies conducted in VA to monitor distribution and dissipation of pirlimycin, tylosin, chlortetracycline, and sulfamerazine in soil following either surface application or subsurface injection of liquid dairy manure demonstrated that subsurface manure injection reduced surface runoff output of antibiotics, antibiotic-resistant fecal coliform bacteria, and resistant genes to the aquatic environment while still allowing access to the nutrients in manure.
Minimizing the presence of emerging contaminants coming in our wastewater or treating wastewater streams prior to their discharge or use as well as knowing when treatment is needed are all important ways to minimize environmental contamination. Research in PA evaluated the potential of biochar materials as potential adsorbents for removing pharmaceuticals from reclaimed water prior to irrigation of food crops. Results demonstrated that increase in biochar pyrolysis temperature from 350C to 700C led to an increase in pH, surface area, and hydrophobicity. The team concluded that biochars could potentially serve as low-cost adsorbents for reducing pharmaceuticals in treated wastewater prior to beneficial reuse by careful selection/modification of biochar properties to sorb compounds most likely to impact health though dietary intake of consumed crops. In a similar study in MI designed to evaluate biochar potential to mitigate the uptake of 15 pharmaceuticals by radish (Raphanus sativus), researchers reported that accumulation of acetaminophen, carbamazepine, sulfadiazine, sulfamethoxazole, lamotrigine, carbadox, trimethoprim, oxytetracycline, tylosin, estrone, and triclosan in radish grown in the soil amended with 1.0% of biochar significantly decreased by 33–83% relative to control (no biochar) treatments. While soils amended with 1.0% of biochar increased sorption of all 15 pharmaceuticals, the persistence (half-life) of 7 pharmaceuticals in the soil were prolonged, including caffeine, sulfadiazine, sulfamethoxazole, lincomycin, estrone, 17 β-estradiol and triclosan. The reduced plant uptake of pharmaceuticals was attributed mainly to the fact that biochar could lower the pharmaceutical concentrations in soil pore water. The estimated daily intake data suggest that biochar amendment could potentially decrease the human exposure to a mixture of pharmaceuticals (Li et al., 2020) .
In the wastewater treatment (WWT) process, many emerging contaminants become concentrated in WWT residuals and thus enter the environment when residuals are land-applied for their nutrient and carbon value. Dr. Linda Lee and her team (Purdue Univ.) analyze 33 emerging contaminantsn commercially available biosolids-based and non-biosolids-based fertilizer products. Emerging contaminants included synthetic musks and fragrances (SMFs), PFAS, and PPCPs. Triclosan and triclocarbon, typically present in many PPCPs, were found at the highest levels of 285-20,000 mg kg-1 and 190-17,800 mg kg-1, respectively. BPA alternatives were found in all products with BPA being highest (468 - 68,200 mg kg-1) followed by BPS, TBBPA and BPAF. In aerobic soil biodegradation studies, BPS degraded rapidly with t1/2 <1 d; however, BPAF persisted (t1/2 ~4.5-6 weeks). The pharmaceuticals found included diphenhydramine > miconazole, cimetidine > ciprofloxacin > ibuprofen. Only one estrogen was detected, estrone (the primary estradiol metabolite) along with two UV filters used in sunscreens and as a UV protectant in food-grade plastics. For the SMFs, tonalide and galaxolide were observed at high concentrations. Nonylphenol ranged from limited detection in composted biosolids to several thousand in some of the other products. Parabens, which are naturally occurring, were observed in all products.
Lee and coworkers (Purdue) did more detailed studies focused on the occurrence of perfluoroalkyl acids (PFAAs) and other PFAS in the waste-based fertilizers including biosolid-based materials and the composted (organic fraction) municipal solid wastes (OFMSW), e.g., yard trimmings, food wastes, food packaging, etc. Total PFAA loads in OFMSW composts were about half of what was observed in biosolids-based products (Choi et al., 2019; Lazcano Kim et al., 2019a,b). Perfluorooctanoic acid (PFOA) and perfluorooctanesulfonate (PFOS) were detected in all biosolids-based fertilizers and all OFMSW composts; however, OFMSW composts were dominated by short-chain PFAAs (>64%) of which > 68% were perfluoroalkyl carboxylates, particularly the C6 PFCA. Of the total PFAA load in the OFMSW composts, 25−49% was released to porewater (∼1 g/2 mL 48-h static contact time) (Choi et al., 2019), which was generally similar to what was observed with the biosolids-based fertilizer. PFAA porewater concentrations versus PFAA loads as well as OC-normalized sorption coefficients versus the number of PFAA CF2 units are strongly correlated (R2 > 0.85) and similarly for the biosolids-based fertilizers (R2 > 0.8). Heat-treatment, composting, blending, and thermal hydrolysis of biosolids did not reduce PFAA loads except for blending due to dilution (Lazcano Kim et al., 2019a,b). Total PFAA concentrations increased in heat-treated and composted products, which was likely due to the breakdown of PFAA precursors during post-treatment. Several PFAA precursors were identified in samples before post-treatment. PFAA levels change with different post treatment processes.
Routine application of biosolids, which are nutrient-and carbon-enriched materials, to soils improves fertility and other characteristics, but the presence of emerging contaminants in amendments raises questions about potential adverse effects on biota and in the case of antibiotics, the development of ARGs. Bioavailability and hazard risks are largely dictated by sorption-desorption and diffusion behavior in amendments. Researchers from KY and VA are conducting research on microbial genomics that could elucidate effects of such constituents. Researchers in KY are developing state-of-the-art methods, a version of metatranscriptomics termed Reference Sequencing (RefSeq), which can quantify abundance of all genes expressed by all bacteria in the soil. The UKY researchers are using RefSeq to determine the effects of biosolids application on microbial community composition in soils that have been treated with and without biosolids. Using the RefSeq approach, UKY researchers discovered that application of biosolids did not affect the number of transcriptionally-active genera (average richness=3600 genera) but did cause a significant shift in genus composition (Multiple Response Permutation Procedure, p-value<0.05), including significant reductions in Shannon and Simpson Diversity (p<0.05), significant increases in several “potential” pathogens including Bacillus, Staphylococcus, Pseudomonas, Tissierella, and Clostridium at the expense of significantly more transcriptionally-active Luteitalea, Pedosphaera and Verrucomicrobia in unamended soil (log 2-fold changes>3, p<0.05).
Many of the changes in bacterial community composition in biosolids-amended soils also occurred in soils amended with plant litter, suggesting that changes were simply due to organic matter inputs, not pathogens in the biosolids. In VA, spectroscopic analyses, eDNA degradation and the associated alterations in DNA secondary structure were investigated by exposing DNase I to tested DNA in the presence of chlorpyrifos, a commonly used organophosphate pesticide. Molecular dynamics simulation was used to explore the weak interactions between the tested DNA and chlorpyrifos. Both spectroscopic and molecular simulation results indicated that chlorpyrifos significantly enhanced DNA degradation without affecting the enzyme activity of DNase I in an aqueous system. The findings provided novel insight into the genotoxicity and ecotoxicity of chlorpyrifos and chlorpyrifos-methyl, as well as their impacts on DNA persistence in aquatic environments. The Virginia Tech researchers employed an integrated, high-resolution examination of the effects of prior antibiotic use, composting, and a 120-day wait period on soil resistomes on manure-amended soil, demonstrating that all three management practices have measurable effects and should be taken into consideration in the development of policy and practice for mitigating the spread of antibiotic resistance.
W4170 members from numerous states (AZ, FL, IN, MI, FL, PA) performed laboratory and field studies quantifying the occurrence, persistence, fate and human health effects of emerging contaminants fate and transport. Results collected from monitoring wells at Penn State’s Living Filter site showed that long-term (40+ years) of spray-irrigation activities did not lead to groundwater PFAS levels above the federal guidelines for PFAS in potable water; however, some of the monitoring well data collected at Penn State’s Living Filter site did exceed the state guidelines. This project also demonstrated that that PFAS are entering livestock feed due to elevated levels of short-chain PFAS compounds in corn silage and fescue grown at a site where treated wastewater is spray-irrigated, and suggest that more research is needed to understand the impacts of wastewater irrigation activities on livestock feed quality, with potential implications for human health (meat and milk consumption).
A field study conducted in Pima County, AZ was designed to investigate the impact of long-term land application of biosolids on PFAS presence in soils. The study involved multiple agricultural plots that have received annual land application of Class B biosolids from 1984 to 2019. No PFAS analytes were detected at any of the three sampling depths for the soil samples collected from undisturbed sites with no history of agriculture, irrigation, or biosolids application (background control sites). Concentrations of PFAS ranging from non-detect to 1.8 μg/kg were measured in soil samples collected from sites that were used for agriculture and that received irrigation with groundwater, but never received biosolids. Similar levels of PFAS were measured for soils collected from the agricultural fields that received biosolids applications. PFOS was observed at the highest concentrations, followed by PFOA. PFAS concentrations in soil attenuated dramatically with depth. Overall, attenuation was >90% at the 6 foot soil depth regardless of the biosolid loading rate. These results indicate that biosolids and irrigation water both are important sources of PFAS present in the soils for all of the study sites. This study resulted in the moratorium being lifted, and significant cost reduction associated with biosolids management.
A mesocosms study in IN demonstrated that PFAS leaching from biosolids-amended soils was sustained throughout the 6-month field study with total PFAS concentrations ranging from 10s to 100s of nanogram per liter (ng/L) in the leachate. Transformation of precursor compounds accounted for the vast majority of PFAS present in the leachate from the biosolids.
Another PFAS leaching study in a land-reclamation field study in VA where biosolids were applied at either the typical N-based rates for crops or five times that rate demonstrated that PFAS leached (captured by 15-cm depth) was in the order of 100s to 1000s of nanogram per liter (ng/L) depending on timing of rain after application. Results from this study also indicated that rainfall intensity and rainfall frequency, and biosolids application rate affected PFAS leaching potential.
Ongoing research in IN and FL are currently evaluating the presence of PFAS in drinking water treatment residuals as a first step in evaluating their potential as an amendment for mitigating PFAS release from biosolids. Preliminary data from IN indicated that alum WTRs showed 7 PFAS detected at a total of 84 ± 4.2 mg/kg dry weight with the dominant PFAS being perfluoropentanoic, and perfluorohexanoic, and perfluorooctanoic carboxylic acids (C5, C6, and C8), and perfluorooctane sulfonate (PFOS). Similarly, W4170 members in FL are also investigating characteristics and wastewater treatment plant management practices associated with PFAS partitioning to drinking water treatment residual (WTRs) and biosolids, as well as how amending biosolids with WTRs affects plant uptake of PFAS and P. Preliminary results indicated PFAS partitioning in biosolids is likely to be related to Al, Fe, and OM content, and thus, likely to be influenced by WWTP management practices affecting biosolids Al and Fe content (e.g., use of biological phosphorus removal, inclusion of WTRs in WWTP influent, etc.). In GA, studies are focused on developing and evaluating wastewater and residuals treatment strategies to reduce pathogens, antibiotics and per- and polyfluoroalkyl substances, with emphasis on using electrochemical approaches. Such novel technologies are expected to have major impacts on beneficial reuse of biosolids and wastewater.
Per- and polyfluoroalkyl substances are present in biosolids due to input from variety of sources including residential homes from the use and care of numerous PFAS-containing consumer products. Research group in IN reported an increased number of PFAS they were able to quantify for which 50% or more appear to be polyfluoroalkyl phosphate esters (diPAPs) similar to what has been found in household dust. These diPAPs will degrade to more mobile and more commonly monitored poly- and perfluoroalkyl acids particularly during aerobic processing or after being land-applied. Researchers in IN are also evaluating PFAS release from pyrolyzed (350 C, low oxygen) biosolids reported that although PFAS concentrations shifted some in the biochar relative to the biosolids, overall PFAS content was still high. However, PFAS release to porewater dropped from hundreds of ppt leaching from the biosolid was negligible (only low single digit ppt of short chain PFAAs) leaching from the biochar.
The W4170 workgroup efforts also focused on microplastics, pathogens, and other emerging contaminants. Microplastic particles (size of 1 μm - 5 mm) are a contaminant of emerging concern in wastewater and biosolids. In CA, fundamental and applied research was carried out to advance microplastics field sampling, laboratory processing and analysis, and data analysis techniques in service of this objective through projects funded by NOAA Marine Debris Research and the California State Water Board. Collaborators include the Southern California Coastal Water Research Project, the Santa Ana Regional Water Quality Control Board, Orange County Sanitation District, Orange County Environmental Resources, and the Los Angeles County Department of Public Works. Collaborative research among W4170 members from CA and IN and University of Cincinnati resulted in a funded project through USEPA’s National Priorities – Evaluation of Pollutants in Biosolids program. This project is expected to expand the team research on emerging contaminants consider processes, risks and management in land applications of biosolids.
Manure-associated antibiotics are often concentrated in manure injection slits, however, research in VA demonstrated that manure application methods did not affect dissipation rates of antibiotics in soil with some antibiotics remained detectable in soils 180 days after manure application. Field investigation and simulated rainfall studies were conducted in VA to monitor distribution and dissipation of pirlimycin, tylosin, chlortetracycline, and sulfamerazine in soil following either surface application or subsurface injection of liquid dairy manure. Liquid dairy manure spiked with eight antibiotics from four classes (sulfonamides, tetracyclines, macrolides and lincosamides) at 500 μg/kg was applied to field plots at 56 Mg/ha in spring and to different plots the following fall. Rainfall simulations were conducted on the plots at 1 or 7 day(s) after manure application. The absolute abundance (CFU/mL/g) of antibiotic-resistant fecal coliform bacteria (ARFCB) in surface runoff and soils were monitored. Results from these efforts suggested that subsurface injection, rather than surface application, should be the method of manure application to reduce surface runoff output of antibiotics, antibiotic-resistant fecal coliform bacteria, and resistant genes to the aquatic environment while still allowing access to the nutrients in manure.
W4170 Objective 2. Evaluate the uses and associated environmental benefits for residuals and wastewaters in various ecosystems (e.g., agricultural, urban, recreational, forest, rangeland, mine-impacted, disturbed, degraded) with respect to changes in soil physical, chemical, biological, nutrient, and trace/heavy metals with respect to soil quality/soil health.
Some of the major soil properties that influence contaminant solubility, bioavailability, and exposure to ecological and human receptors include physical (soil bulk density, clay type and content, aggregate stability, and porosity; chemical (pH, salts, non-essential heavy metal(oid)s, and redox potential; microbial (microbial biomass C, mineralizable N content, macrofauna and microbial activity and function; plant-available essential nutrients with organic matter being the soil physical/chemical/biological/nutrient nexus. The premiere US residuals reuse workgroup, W4170, has continued to focus efforts on cutting edge topics related to beneficial use of residuals, reclaimed waters, wastewaters, and amended soils associated with agronomic and environmental benefits to aid in developing a soil health framework with respect to residuals land application.
The application timing, fate and effects on above ground plant growth of land-applied, residuals-based nutrients continues to be studied under a variety of environmental scenarios. Research in CO, FL, and WA demonstrated that land application of biosolids to various crops (wheat, corn, pastures, tree plantation) have multiple agronomic and environmental benefits including improvement in soil health as compared to inorganic fertilizers. Results from field and laboratory trials and a rainfall simulation study in FL (Silveira et al., 2017) suggested a significant lower risk of N and P losses via runoff and leaching with the application of biosolids than commercial inorganic fertilizer. Negligible (0.1 to 0.2% of applied P) P leaching occurred from biosolids-amended soils during a 3-yr field study in FL. In 2020, the OH team in collaboration with the City of Columbus established long-term experimental areas on local farms in OH to evaluate the effect of land application biosolids on soil health.
Newly developed EQ biosolids products specially tailored for urban soil were compared with established products to assess their capability to support germination and plant growth, as well as to quantify their plant available nitrogen (N) and phosphorus (P) (Alvarez-Campos & Evanylo et al., 2019a, 2019b). Seven EQ biosolids products derived from treatment processes such as thermal drying, com-posting, and blending with complementary organic and mineral materials and an inorganic fertilizer control were compared in greenhouse bioassays employing soybean (Glycine max L.) and tall fescue (Festuca arundinacea Schreb.). The biosolids applied at estimated agronomic N rates enabled adequate germination and plant growth. EQ biosolids were compared to a synthetic fertilizer on urban soil properties and vegetable yield in a field study (Alvarez-Campos & Evanylo, 2019b). Compared to the inorganic fertilizer, the biosolids increased soil C by 37% to 84% after two years; lowered soil N availability likely due to low N mineralization OC was low and clay concentrations high; and increased yields of cabbage and kale yields (fall 2017). Despite limiting soil physicochemical conditions, biosolids addition at reclamation rates showed greater potential to increase vegetable yield after two years of application.
In addition to the multiple agronomic benefits, biosolids offer several environmental advantages compared with inorganic fertilizers because nutrients are less soluble and, therefore less likely subject to losses. Research in FL demonstrated that long-term biosolids application reduced P leaching compared with control treatments (no biosolids addition). This response was due to the addition of Fe and Al with biosolids and subsequent positive effects on soil P storage capacity. Research findings in KS also concluded that high-Fe biosolids reduced bio accessible lead in alkaline soils that are not responding effectively to conventional P fertilizer treatments.
Ippolito (2015) completed a study on the beneficial reuse of Al-Water Treatment Residuals (WTR) in an engineered urban wetland in Boise, ID. Al-WTR application significantly increased P capture and lessened offsite P movement into adjacent waterbodies. Banet et al. (2020a, b), Zohar et al. (2020, 2018), Litaor et al. (2019), and Massey et al. (2018) worked on a similar project with Al-WTR from the City of Fort Collins, CO and from Eshkol Reservoir in Israel, studying Al-WTR’s ability to sorb organic P from swine and dairy wastewater containing > 7000 mg organic P L-1. Almost all organic P (99.9%) was sorbed within 1 day resulting in the resulting Al-WTR composite having the potential to release 380 to 485 mg total P kg-1. A subsequent greenhouse trial was performed, proving that this Al-WTR-organic P composite material performed equally as well as common P fertilizer.
Ippolito and his research team (Colorado) found that certain biochars can stabilize extracellular enzymes necessary for nutrient cycling and maintain or enhance microbial community activities when applied with or without manure (Elzobair et al., 2016a,b), improve nutrient aspects, hydraulic properties, and reduce greenhouse gas emissions when applied along or in conjunction with manure (Ippolito et al., 2016a,b; Kammann et al., 2017; Laird et al., 2017; Spokas et al., 2017), and sorb/sequester heavy metals, improve plant productivity, and overall soil health in biochar amended systems (Mehmood et al., 2017), including mine spoils (Novak et al., 2016; Ippolito et al., 2017).
The carbon benefits associated with biosolids use has been well documented (Brown et al., 2011, Brown and Beecher, 2020; Leonard et al., 2021; Trlica et al., 2013). Multiple studies have shown enhanced soil carbon storage with biosolids based amendments. This has been a research focus for several members of the W 4170 committee. Work is now considering the role of municipal biosolids in the broader context of sustainability (Brown et al., 2020). A component of this is nutrient capture and reuse. Nutrient circularity is key to sustainable agricultural systems. A recent paper co-authored by a member of the group considered the quantities of N and P that are present in municipal residuals including food scraps and wastewater (Brown et al., 2023). The study found that sufficient N and P enters the wastewater systems in Tacoma and Seattle, WA to meet the crop (non -meat and non- diary) needs of the populations of the two cities. However, only a fraction of these are captured in biosolids with the majority (particularly N) in the effluent. The study then further considered the importance of community gardens within those cities for their ability to provide a significant source of vegetables for each city. For this estimate, arable land in the gardens along with yield, with or without the use of residuals -based soil amendments were considered (Niedzwiecki et al., 2022). Use of amendments in the urban soils was able to enhance production for the more disturbed urban soils.
The fate of land-applied residuals-borne trace and heavy metals, and metalloids, have also been extensively studied decades. For example, the long-term benefits of biosolids additions to dryland winter wheat agroecosystems has been studied for 37 years in CO. At agronomic rates based on N needs of wheat, Barbarick et al. (2015) found that uptake coefficients for nutrients and trace metals in biosolids-amended soils were much lower than those used for risk analysis by the US EPA. Furthermore, when biosolids land application occurs in dryland agroecosystems, biosolids tend to concentrate Cu and Zn in the soil surface preventing appreciable downward movement (Ippolito et al., 2015). More importantly, Zn addition may be beneficial in semi-arid cropping systems such as those found in eastern CO, as plant-available Zn soil concentrations may be close to or below sufficient concentrations for plant growth. Barbarick et al. (2016) furthered their biosolids research to determine direct and indirect influences on grain concentrations and cumulative uptake of Cu, Fe, Ni, P, and Zn and that Zn application across biosolids amended sites may offset potential Zn deficiency symptoms present in semi-arid soils consistent with Ippolito et al. (2015).
Hettiarachchi’s team at KSU, in conjunction with other W4170 members (Brown, UW; Chaney, USDA-ARS; Basta, OSU; Scheckel, USEPA), evaluated the long-term bioavailability of As, Cd, Pb, and Zn in mildly contaminated urban brownfields soils and mine waste materials amended with different organic amendments (manures, various compost types, composted and non-composted class A biosolids products). X-ray absorption spectroscopy and in vitro bioaccessibility extraction tesst (also known as Physiologically-Based Extraction Test) showed that the dominant Pb species in the urban soils were Pb adsorbed to Fe oxy(hydr)oxides (Pb-Fh) and Pb adsorbed to organic C (Pb-Org C), which increased as soil-compost mixture aged in the field. During the in vitro extraction test that Pb initially sorbed to Fe oxy(hydr)oxides dissolved, and Pb-org C and hydroxypyromorphite were formed. Aged soil-compost mixture reduced Pb-Fh dissolution during the in vitro extraction (Defoe et al; 2014; Attanayake et al., 2015; Brown et al., 2016a; Henry et al., 2015; Attanayake et al., 2017).
Land application of biosolids can increase soil organic and also help mitigate greenhouse gas emissions. Despite the vast literature on the agronomic value of biosolids, there is still limited information on its potential benefits soil carbon sequestration potentials. Understanding the impact of biosolids end use on C emissions can help municipalities achieve C neutrality. A systematic literature review and meta-analysis was conducted in VA to quantify carbon sequestration potential of land-applied biosolids. The VA team reported growing evidence that biosolids may be used to restore mine soils in VA. Similarly, research in WA suggested that use of biosolids for commercial tree plantations can be a beneficial end use for tree response and for carbon storage. Although land application of biosolids for commercial tree plantations is a common practice in the Pacific Northwest, scientists in WA concluded this practice could also provide multiple benefits in other regions such as loblolly pine plantations in the Southeast U.S. In addition to promoting soil C sequestration, research in FL also demonstrated reduced nitrous oxide and carbon dioxide emissions associated with biosolids compared with commercial fertilizer. Brown et al. (2017) co-authored a book chapter summarizing carbon accounting associated with mine land reclamation. Examples included biosolids, coal bottom ash, and pulp sludge for restoring a range of disturbed sites. Carbon accounting typically considers increases in soil carbon as a result of mine site restoration as well as fugitive emissions avoidance for diverting organics from landfills to beneficial use. Benefits of inorganic materials such as coal ash for reclamation was included in this work.
Arsenic is one of the most common contaminants of concern exceeding risk criteria with with As-soil contaminated soil ingestion being the primary human health risk driver at many urban, military, U.S. Brownfields and CERCLA sites. Use of total contaminant content instead of bioavailability is often overly conservative and can result in costly and unnecessary soil remedial action. Basta et al. (2016), Obrycki et al. (2017a) and Whitacre et al. (2017) completed several projects that determined the ability of in vitro bioaccessible (IVBA) methods to predict relative bioavailable (RBA) As, which resulted in four primary conclusions. First, total soil As concentration was not correlated with RBA as determined by adult mouse (r2=0.24) or the juvenile swine (r2 = 0.09) bioassays. Secondly, all of the IVBA methods generally were predictive of RBA for both bioassays. Thirdly, IVBA arsenic gastric extraction GE) is a better and more conservative predictor than IVBA arsenic from the intestinal extraction. Lastly, the California Bioaccessibility (CAB) method was developed to provide a conservative estimate of RBA As on CA mining site soils, which was was an accurate predictor of swine RBA As for soils with high oxide content and soil As concentrations <1,200 mg As/kg.
Developing management recommendations for contaminated urban systems is necessary to address public questions regarding best practices for using urban soil for food and recreation. Brown and co-workers (Brown et al., 2016c, 2016d) have co-edited a 2-volume series on urban agriculture entitled Sowing Seeds in the City. The first focuses on ecosystem and municipal services. It includes chapters on use of alternative water (stormwater, grey water and reclaimed water), residuals use (biosolids, food and yard waste) and greenhouse gas implications of residuals use in urban agriculture. The second focuses on human health and includes contributed chapters from Basta (OSU) and Hettiarachchi (KSU).
Brown et al. (2016b) have been defining standards for green stormwater infrastructure in urban areas. Green stormwater infrastructure relies on enhancing soil/plant systems’ ability to both allow stormwater to infiltrate and to remove contaminants from stormwater. These systems are being used both alone and in combination with grey or engineered systems as a way to limit combined sewer overflows in urban areas. They are also likely to enhance off peak stream flow and reduce the flashiness of urban streams. Current standards for these systems vary widely by location and appear to be more qualitative than quantitative. This research tested the importance of compost feedstock for removal of nutrients and metals and found that feedstock (manure, biosolids, yard/food scraps) was a poor predictor of system performance. They also tested the potential for the phosphorus saturation index (PSI) to serve as a better predictor of P movement in these systems. Furthermore, the team also demonstrated that Fe-containing water treatment residuals were beneficial in these systems. This builds on the literature and research done within the W4170 group (e.g., Basta – OSU; Elliott – PSU; O’Connor, Silveira – UF; Ippolito - CSU) on the PSI as a tool for predicting P movement in biosolids amended soils.
Brown (2016) and Jay et al. (2017) tested the importance of compost feedstock in predicting the performance of stormwater bioretention soil mixtures in a replicated greenhouse trial using biosolids/yard waste-, animal manure/sawdust-, and food/yard waste-based composts. They investigated the movement of P (compared to the PSI predictions), N, metals and PAHs. This work builds on the literature and research done within the group, e.g., Basta (OSU), Elliott (PSU), O’Connor (UF) on the PSI as a tool for predicting P movement in biosolids amended soils as well as work done by Evanylo (VT) and Ippolito (CSU) on bioretention soil mixtures. Morris et al. (2017) worked with Dr. Sally Brown, as part of a team tasked by the Oregon DEQ to evaluate the impact of different food waste management alternatives. Composting, in-sink disposal, direct anaerobic digestion and combustion were considered. In addition to traditional Life cycle assessment parameters, this study included a section on the soil impact of each end use/disposal method (Morris et al., 2017).
Brown authored a pamphlet on how compost production and use can impact Climate Change per request of the US Compost Council in 2017, which provides an easy to understand guide on how land application of composts and other organics such as municipal biosolids can be an effective tool to both reduce fugitive gas emissions and to sequester carbon. Brown (2017) authored a chapter on the potential to create soils from urban wastes for a book on urban soil. The benefits and obstacles for diverting residuals to beneficial use were discussed and materials that would be suitable as feedstocks for soils and soil amendments were described.
Hacheney and Brown (2017) started a cooperation with inmates at the Monroe Correctional Facility in WA State. The inmates compost a majority of the food waste that is generated in the prison using Vermi -composting, Bokashi fermentation and black soldier fly composting. Dr. Brown co-authored an article for Biocycle with a prison inmate leading the compost program based on the data he generated. The cooperation is continuing with field plots with select garden vegetables where the relationship between soil health, yield and nutrient concentrations will be explored.
Basta et al. (2015), Carlson et al. (2015), Henry et al. (2015), Kaiser et al. (2015), Li et al. (2015), Nelson et al. (2016) and Obrycki et al. (2016) have performed focused investigations on the addition of phosphates to lead-contaminated soils as a management technique for reducing risk of Pb exposure in children. Pb contaminated soils (790 to 1,300 mg Pb kg-1) from a garden and a city lot in Cleveland, OH were incubated in a bench scale experiment for 1 year. Six phosphate amendments including bone meal (BM), fish bone (FB), poultry litter (PL), monoammonium phosphate (MAP), diammonium phosphate (DAP), and triple super phosphate (TSP) were added to pots at two application rates. Six phosphate amendments showed mixed results on their ability to reduce soil Pb bioaccessibility (IVBA Pb) and exposure risk to children. Soil amendments were largely ineffective in reducing IVBA Pb in these two urban soils when using EPA Method 1340. However, P treatments were much more effective when evaluated using modifications of EPA Method 1340. The greatest reductions in IVBA Pb were found at pH 2.5. Reductions in bioaccessible Pb from soil treatment ranged from 5-26% for the pH 2.5 extractions. A modified EPA Method 1340 that does not contain glycine and uses pH 2.5 rather than 1.5 has potential to predict efficacy of P soil amendments to reduce bioaccessible and bioavailable Pb.
University of Minnesota researchers (Jelinski et al.) screened 58 houses and over 2,500 soil samples from households across the Twin Cities for soil Pb via pXRF and wet chemical methods. Over half of investigated cores now have their maximum Pb concentrations at depths deeper than 10 cm, and in some cases to 20 or 40 cm, suggesting ongoing physical or chemical processes which are redistributing Pb in urban soils likely through biotic and abiotic processes. Therefore, decades-old predictions of the surficial Pb concentrations may now overestimate the current loading and risk of Pb at the surface. Understanding the depth distribution of Pb is also important for making better recommendations for urban agricultural uses of soil, where contaminant screening in the top portion of the soil may not accurately reflect the total Pb loadings available once the soil is mixed more deeply, or if plant roots reach those soil depths. The researchers are planning to select a 220-samples epresenting a moderate to high range in soil Pb and a range of depth and locations from an existing 600+ sample household soils archive. This subset will be assayed for soil Pb bioaccessibility by PBET, RBALP 1.5 and RBALP 2.5 methods as well as for soil organic matter, P and pH resulting in for bounding the range in variability of soil Pb bioaccessibility at the household scale and soil characteristics, which can be utilized to predict bioaccessibility.
Brown et al. (2016a) and Laidlaw et al. (2017) cooperated with a large group to address the hazards associated with Pb contamination in urban soils. Research summarized the use of biosolids to reduce Pb availability in situ. Potential transfer of Pb from soils to food crops was evaluated using peer reviewed literature. Direct ingestion of soil with elevated Pb was also considered. The authors developed practical guidelines to minimize any potential risk of exposure. Beyer et al. (2017) reviewed use of soil amendments including municipal biosolids and other residual based materials to reduce metal toxicity and restore ecosystem function to metal contaminated waste materials at mining sites. Conclusions drawn suggest that wider spread use of biosolids in urban areas has the potential to offer protection against Pb and other heavy metal exposure.
Hettiarachchi and her team evaluated the uses and associated agronomic and environmental benefits for residuals in agricultural and urban systems using lab, greenhouse and field research. Their research continues to be designed to assess the ability of residuals rich in C, P and Fe (for example non-composted or composted Class A biosolids) to reduce contaminant bioavailability in Brownfields. Chemical methods are used to evaluate reduction in risk from contaminants to human and ecological receptors. Research to date indicates that the potential exposure pathway of concern is direct exposure of contaminated soils to humans with the pathway from contaminated soil to plant to human being insignificant (Attanayake et al., 2015, 2017; Brown et al., 2016; Henry et al., 2016; Karna et al., 2016; Wijesekara et al., 2016).
Thermal treatment of biosolids to create a partially (i.e., biochar) or fully (i.e., ash) combusted byproduct is becoming more common, but the properties and potential benefits of such residuals are not as well-understood as the uncombusted biosolids. Members of W4170 in CO, FL, HI, and NE continue to study land application biochar on heavy metal mitigation, soil health, carbon sequestration, and nutrient availability. In FL, research on the co-application of biochar with organic residuals has provided evidence that that biochar may improve the efficiency of nutrients in biosolids. Results from laboratory and field, including rainfall simulation, trials suggested significantly lower risk of N and P losses via runoff and leaching than commercial inorganic fertilizer. Research in HI evaluated the impact of biochar (either surface applied or incorporated) and chicken manure compost applied at 4% v/v/ on crop responses. Soil pH, total N, and extractable P, Ca, and Mg concentrations increased in response to biochar application. Chinese cabbage biomass was 14-70% higher in biochar treatments than unamended soil, while papaya biomass increased by 19-23%. Results indicated that surface co-application of biochar and compost could be an effective farm-scale practice with potential benefits to annual and perennial crops. In NE, research is evaluating low-cost animal manure management options, including production of manure-derived char. In a greenhouse study evaluating co-application of dairy slurry and swine lagoon wastewater with biochar and coal char, they reported significant reduction in P leaching (15-24% for biochar vs. 38-50% char). The study also demonstrated that unlike biochar, some char-N is plant available, which could potentially have agronomic benefits.
Soil quality/health. The term “soil quality” was initially defined by Mausel (1971) who stated that “soil quality is the ability of soils to yield corn, soybeans and wheat under conditions of high-level management”. Unfortunately, this definition solely emphasized crop yields under agriculture management (Bünemann et al., 2018). In 1997, the Soil Science Society of America (SSSA, 1997) described soil health as “the capacity of a soil to function within ecosystem boundaries to sustain biological productivity, maintain environmental quality, and promote plant and animal health”. This phrase was restated and simplified by (Karlen et al. (1997) as “the capacity of soil to function”. The most recent soil health description by the USDA-NRCS (2023) is “the continued capacity of soil to function as a vital living ecosystem that sustains plant, animals, and humans”. The metamorphosis of soil health definitions clearly outlines our general understanding of soil functionality, yet these definitions do not specifically guide us towards how to quantify soil health.
Much like human health, which is never directly measured and only quantified via a series of metrics (e.g., physical strength, blood chemistry, gut microbiome), soil health is also never directly measured but quantified via a series of soil physical, chemical, and biological indicators. Understanding the intimate linkages between soil physical, chemical, and biological characteristics within any given system, and how these characteristics may be altered for improvements in ecosystem services, is the audacious goal of soil health programs globally. Furthermore, understanding that soil health is related to soil function is important for discerning how to specifically quantify soil health. When soils are functioning at an optimal capacity for a specific outcome (e.g., maximum crop yields, minimal erosion losses, improved water retention, increased biodiversity), soil characteristics are likely optimized as well. But which soil characteristics and what exactly do they look like when optimized?
In soil health quantification programs, soil characteristics typically fall into three categories: 1) physical; 2) chemical; and 3) biological soil characteristics. These characteristics are oftentimes referred to as indicators of soil health. Physical soil health indicators typically encompass bulk density (or penetration resistance), aggregate stability, available water content, soil texture and clay content. Chemical soil health indicators encompass macro- and micro-nutrient availability (and sometimes trace/heavy metal concentrations), pH, electrical conductivity, and sodium adsorption ratio. Biological soil health indicators encompass organic C content (SOC) (or soil organic matter content [SOM]), microbial biomass C, potentially mineralizable N, soil respiration (e.g., CO2 burst), soil protein content, water extractable C and N, a suite of enzymatic assays (e.g., β-glucosidase, nitrogenase, phosphatase, arylsulfatase, etc.), and on occasion have included PLFA, metagenomics, and metabolomics.
Optimization of soil health indicators often follows the more is better, less is better, or somewhere in the middle is better approach. The “more is better” approach is followed by soil indicators such as aggregate stability, available water content, extractable K, SOC (and SOM), potentially mineralizable N, microbial biomass C, soil respiration, and enzymatic activity. The “less is better” approach is followed by soil indicators such as bulk density, electrical conductivity, and sodium adsorption ratio. The “somewhere in the middle” approach is followed by pH and extractable P. Regardless of the approach, each indicator is scored on a unitless scale (e.g., from 0 to 1, or 0 to 100), with indicator scores added together or weighted to derive final soil health scores. The greater the soil health score, theoretically the “better” the soil is with respect to being healthy. Of course, this approach should be interpreted with respect to what the ultimate outcome is within the context of site management.
Residuals application, and specifically biosolids application, as a land management strategy has occurred globally. Biosolids-receiving lands have received a great deal of attention with respect to the quantification soil physical (e.g., Orndorff et al. (2015), Yarwood et al. (2015), Daniels et al. (2016), Clark et al. (2017)), chemical (e.g., Ippolito et al., (2015), Barbarick et al. (2016, 2017), and biological (e.g., Meinhardt et al. (2015), Bertagnolli et al. (2016), Lauriault et al. (2018)) alterations following application, yet the quantification of overall soil health has attracted little attention.
Of the attention that has focused on the combined soil physical, chemical, and biological alterations following biosolids land application, positive as well as negative responses have been noted. For example, Jin et al. (2015) evaluated the effects of “mid-term” biosolids surface applications (0, 20, 40, 60 Mg ha-1, applied every year for 8 years) or a “long-term” biosolids surface application (20 Mg ha-1, applied every year for 20 years) to no-till perennial coastal bermudagrass fields. Total SOC increased with increasing biosolids application rates and were greater in the long-term fields as compared to the mid-term fields at comparable application rates. Total N stocks, available water capacity, and soil volumetric water content at both wilting point and field capacity were all greater with biosolids as compared to controls. Soil bulk density was similar across application rates and depths, except for in the 0-10 cm depth where bulk density increased with increasing biosolids application rate; bulk density did not exceed 1.36 g cm-3, below the ~ 1.7 g cm-3 threshold where roots might suffer. A decrease in water stable aggregates with increasing biosolids application rates was also noted, opposite of most (e.g., Ojeda et al. (2008), Wallace et al. (2009), Nicolás et al. (2014)), but not all (e.g., Abiven et al. (2009); Diacono and Montemurro (2010)) previous, similar works. Jin et al. (2015) did note that above- and below-ground organic inputs often increase microbial activity, exudation, and turnover, yet these constituents were not directly measured in their study.
Nicholson et al. (2018) studied the long-term effects of biosolids land application (2.9 to 3.4 Mg ha-1, applied every year over 20 years), as compared to a control that received only inorganic fertilizers, on soil health at four locations that raised winter wheat. The authors noted increases in SOM, available water capacity, water infiltration, aggregate stability (opposite of Jin et al., 2015), total N, extractable P, and earthworm numbers and weight. The authors noted that biosolids land application is important for helping to maintain/improve SOM concentrations, leading to additional positive responses, and ultimately maintaining sustainable agroecosystems.
Urra et al. (2019) utilized a 24-year field experiment (under a cereal-cereal-non cereal rotation) where 40 and 80 Mg ha-1 of biosolids were applied every 1, 2, or 4 years; a control was also utilized. Soil organic matter content and extractable Cu, P, and Zn concentrations were greater in biosolids amended soils as compared to the control. The 80 Mg biosolids ha-1 application rate had greater plant-available N as compared to all other treatments. Soil microbiological parameters, including phosphatase, arylsulfatase, β-glucosidase, β-glucosaminidase, microbial biomass C, respiration, and potentially mineralizable N were all greater in plots receiving biosolids as compared to the control. Urra et al. (2019) then determined a soil health index utilizing all studied microbiological indicators, finding that microbiological soil health was statistically greater in biosolids amended plots as compared to control plots.
Ippolito et al. (2021) compared biosolids (0 to 11.2 Mg ha-1) to inorganic N fertilizer (0 to 112 kg ha-1) applied every other year over 22 years to a wheat-fallow agroecosystem. The authors investigated the use of the Soil Management Assessment Framework (SMAF), which is an excel-based tool to determine soil health changes based on changes in soil indicators. Increasing N fertilizer rates had little effect on soil indicators or soil health. However, increasing biosolids application rates increased SOC, potentially mineralizable N, microbial biomass C, and improvements in soil pH. Biosolids improved soil chemical health, biological health, and overall soil health as determined via a weighted average of soil physical, chemical, and biological health scores.
Buchanan and Ippolito (2021) studied alterations in soil health via biosolids applied at 0, 2.5, 5, 10, 21, and 30 Mg ha-1 once in 1991 or again in 2002 to an overgrazed rangeland. These authors also utilized SMAF to ascertain difference in soil health. There was no evidence of changes in soil physical or nutrient health indices (which included bulk density, aggregate stability, and extractable P and K indicators). Chemical soil health (pH and EC indicators) was greatest when biosolids were applied at < 30 Mg ha-1 and with the single as compared to the repeated biosolids rates. Biological soil health (SOM, potentially mineralizable N, microbial biomass C, and β-glucosidase indicators) improved with increasing biosolids application rates, was greater in the repeated as compared to the single biosolids application, and was maximized at 30 Mg ha-1. Buchanan and Ippolito (2021) then compared all soil health scores to past plant community shifts as affected by increasing biosolids application rates, and suggested that soil health and plant community structure, which would benefit the ecosystem and grazing animals, appeared to be optimized at 10 Mg ha-1 of biosolids.
Una et al (2022) evaluated soil health on urban soils. Soils had been amended with two biosolids based products, a vermicompost produced from vegetable scraps or with traditional fertilizers. Changes in physical, chemical and biological characteristics were measured. Garden vegetables were grown on the soils in field and greenhouse trials. Yield and nutritional density were recorded. On the undisturbed urban soils, amendments increased soil N and P, showed a beneficial impact on at least one of the physical characteristics measured (water infiltration rate and bulk density) but had no impact on soil biological measures (POX-C). In contrast, on the disturbed urban soil, amendments improved soil across all measures. Plant yield in the disturbed soil was close to zero for all crops with conventional fertilization. All organic amendments significantly increased yield with one of the biosolids products and the vermicompost increasing yields to higher than those measured in the healthy urban soils
Desjardins (2022) connected biosolids land application to soil health in a no-till wheat-fallow rotation that received 4.5, 6.7, and 10 Mg ha-1 applied every four years since 1996; unfertilized and fertilized plots were used for additional comparisons. The author found that biosolids helped reduce soil bulk density, and that the 6.7 and 10 Mg ha-1 application rates increased soil water holding capacity. However, aggregate stability and hydraulic conductivity did not change with biosolids application rates. Additional soil health metrics are currently being collected.
The above examples of biosolids effects on soil physical, chemical, and biological attributes supports the need for continued site-specific management decisions that can contribute to improvements in soil health, as soil health quantification in biosolids-amended soils is lacking. Continued illustration of the positive effects regarding biosolids land application will support land application programs nationwide, an approach followed and highlighted by W170 through W4170 workgroups.
Residuals land application and the use of soil quality assessment tools is currently in its infancy. Ippolito et al. continues to investigate the use of the SMAF to determine soil health changes at several locations, including: 1) The scientists are ascertaining soil health differences at an eastern Colorado dryland site that has received agronomic biosolids or inorganic fertilizer applications since 1999 (up to ~ 90 Mg biosolids ha-1 total). Soil health alterations are being investigated over topographical location (e.g., hill, side, or toe slope positions) and over cropping rotation (e.g., wheat-fallow or wheat-corn-fallow); 2) Ippolito et al. are determining alterations in soil health (and reseeded mixed grass prairie nutrient/metal concentrations) at the Tri-State Mining District (near Joplin, MO) where composted biosolids land applications have occurred in 2016 at rates of 0, 180, and 360 Mg ha-1 to a site contaminated with Cd, Pb, and Zn. An undisturbed area is being utilized as the benchmark site to potentially strive towards with this mine land reclamation project; 3) At the Tri-State Mining District, Ippolito et al. are comparing soil health (and plant health) at three locations that received 360 Mg ha-1 of composted biosolids in either 2016, 2018, or 2021; 4) Within the city of Joplin, MO, Ippolito et al. will be comparing soil health across plots that received biosolids and/or P fertilizer in an effort to reduce Pb bioavailability and improve plant growth; 5) currently, Ippolito et al. are studying alterations in soil and plant health at a Superfund site in Leadville, CO, where lime and biosolids were applied at 224 Mg ha-1 each in 1998 to reduce acidity, reduce heavy metal bioavailability, and to improve plant growth.
Objectives
-
Evaluate the short- and long-term fate, bioavailability and persistence of emerging contaminants with an emphasis on per- and polyfluoroalkyl substances (PFAS), microplastics, metals, and pathogens in residuals, reclaimed water, and amended soils to aid in assessing and minimizing environmental and human health risks from their application at a watershed scale.
Comments: Specific tasks: i) Quantify and evaluate the uptake, accumulation and transport of emerging contaminants in residuals, wasteways and residuals- and wastewater-treated soils (e.g., agricultural, urban and brownfields); ii) Predict the long-term bioavailability, persistence and toxicity of emerging contaminants in residuals- and wastewater-amended soils; iii) Evaluate ecological effects of emerging contaminants from soils amended with residuals and reclaimed wastewaters; and iv) Evaluate long-term effects of residuals and wastewater application on the emergence/spread of antibiotic resistance. -
Evaluate and optimize the uses and associated environmental benefits of residuals and wastewaters applied to various ecosystems (e.g., agricultural, urban, recreational, forest, rangeland, mine-impacted, other anthropogenic) on soil physical, chemical, and biological properties and plant nutrition, health, and yield.
Comments: Specific tasks: i) Quantify the effects of biosolids and other municipal, industrial, and agricultural residuals on indicators of soil health; ii) Quantify the effects of biosolids and other residuals on pollutants (including emerging contaminants and metals/metalloids) availability, assimilation, phytotoxicity, and remediation; and iii) Develop and evaluate treatment strategies of residuals and wastewaters to reduce contaminant or pathogen loads.
Methods
Objective 1. Evaluate the short- and long-term fate, bioavailability and persistence of emerging contaminants with an emphasis on per- and polyfluoroalkyl substances (PFAS), microplastics, metals, and pathogens in residuals, reclaimed water, and amended soils to aid in assessing and minimizing environmental and human health risks from their application at a watershed scale.
Specific tasks: i) Quantify and evaluate the uptake, accumulation and transport of Emerging contaminants in residuals, wastewaters and residuals- and wastewater-treated soils (e.g., agricultural, urban and brownfields); ii) Predict the long-term bioavailability, persistence and toxicity of emerging contaminants in residuals- and wastewater-amended soils; iii) Evaluate ecological effects of emerging contaminants from soils amended with residuals and reclaimed wastewaters; and iv) Evaluate long-term effects of residuals and wastewater application on the emergence/spread of antibiotic resistance.
We are interested in a science-based understanding of fate and environmental and human impact of emerging contaminants, persistent organic contaminants, and pathogens in land-applied residuals and reclaimed waters. Contaminant reaction products will be identified, occurrence of antibiotic resistance will be evaluated, and contaminant bioavailability to plants, animal (including humans), and other ecological receptors will be measured. This will be accomplished via US EPA’s fate, transport and transformation test standard guidelines (US EPA, 1998; US EPA2007a, bVent, 2008a, 2008b) in conjunction with methods previously established by W4170 workgroup members (Bhalsod et al., 2018; Li et al., 2019). Studies will be conducted in laboratory, greenhouse, and field settings. This research will be done collaboratively between MI, IN, FL, IL, CO, OH, PA, VA, and GA. Specific subgroups are identified in the descriptions of specific research covered under Objective 1.
Tasks i-iii: i) Quantify and evaluate the uptake, accumulation and transport of emerging contaminants in residuals, wastewaters and residuals- and wastewater-treated soils (e.g., agricultural, urban and brownfields); ii) Predict the long-term bioavailability, persistence and toxicity of emerging contaminants in residuals- and wastewater-amended soils; iii) Evaluate ecological effects of emerging contaminants from soils amended with residuals and reclaimed wastewaters, iv. Evaluate long-term effects of residuals and wastewater application on the emergence/spread of antibiotic resistance
Most published studies on the environmental fate and transport of trace organic compounds (emerging contaminants), except for those of W4170 members, have been conducted using experimental design of laboratory tests in soil-only systems in accordance with standard guidelines that have been established for pesticides. It has become evident that those standard guidelines that are derived from direct application of pesticides are not appropriate due since many emerging contaminants enter a soil system in residuals-partitioned states; residuals-partitioned emerging contaminants behave differently than a direct chemical application, and residuals can alter the physical, chemical and biological properties of soil, thus influences environmental fate of emerging contaminants. Therefore, we will continue to employ comprehensive laboratory and field investigations to gain further systematic and mechanistic understandings of fate and transport of residuals-borne emerging contaminants. The information generated will be utilized to better understand the environmental behavior of residuals-borne emerging contaminants at the macro- and field-scale in a wide range of residuals-amended soil systems. We will also develop and use molecular technologies to detect and identify microbial pathogens from animal, human, and natural sources and characterize microbial populations and communities contributing to applied remediation processes, such as the bioremediation of organic contaminants. Ultimately, knowledge gained will help site managers and environmental engineers design better residuals land management systems to minimize environmental impact of residuals-borne emerging contaminants.
With the increasing awareness and concerns of PFAS in wastewater and treatment residuals, we will focus considerable attention on developing a better understanding of the occurrence and fate of PFAS in agricultural and urban landscapes and watersheds from application of wastewater and treatment residuals. We will conduct research at the laboratory, greenhouse and field scale to better ascertain the influence of land-applied residuals and wastewaters on PFAS inputs to water sources and accumulation in plants including under more realistic agricultural production settings. We will evaluate PFAS load in biosolids and quantify and correlate PFAS leaching potential from biosolids as a function of biosolids characteristics including iron, aluminum and organic matter (OM) content, production practices and PFAS properties. We will evaluate the uptake and accumulation of PFAS in vegetables and grain crops from the soils amended with biosolids. PFAS can be released from biosolids, transport with water flow from soils to vegetables/crops and accumulate in plants. Accumulation will be related to plant type and vary in different plant tissues and as a function of growth condition as well as soil water distribution and flow patterns in soil-water-plant systems. Soils will be amended with biosolids samples, and investigate plant uptake of selected PFASs from the soils. Lettuce or kale and radish or turnips will be used as model vegetables and corn will represent grain crops. Approaches will include (1) characterizing of the major PFAS present in the biosolids received, (2) investigating the uptake of representative PFAS in selected vegetables and grain crop under relevant environmental settings, and (3) evaluating plant constituents and soil water influencing the uptake and distribution of PFAS. The results obtained from these studies will provide more reliable information to evaluate the potential accumulation of PFAS in grain crops and vegetables produced in soils amended with biosolids. PFAS transformation kinetics, metabolites, plant uptake, bioconcentration factors, their potential to leaching and environmental factors affecting the fate and impact of PFAS will be characterized.
Objective 2. Evaluate and optimize the uses and associated environmental benefits of residuals and wastewaters applied to various ecosystems (e.g., agricultural, urban, recreational, forest, rangeland, mine-impacted, other anthropogenic) on soil physical, chemical, and biological properties and plant nutrition, health, and yield. Specific tasks: i) Quantify the effects of biosolids and other municipal, industrial, and agricultural residuals on indicators of soil health; ii) Quantify the effects of biosolids and other residuals on pollutant (metals/metalloids) availability, assimilation, phytotoxicity and remediation; and iii) Develop and evaluate treatment strategies of residuals and wastewaters to reduce contaminant or pathogen loads.
Task i) Quantify the effects of biosolids and other municipal, industrial, and agricultural residuals on indicators of soil health (AZ, CO, FL, KS, MN, OH, PA, VA, WA)
We will determine residuals land application effects on indicators of plant and on soil health itself by utilizing tools such as the Soil Management Assessment Framework. The beneficial use of residuals for land application has been demonstrated by our W4170 members. Residuals land application can improve soil physical, chemical, and biological attributes in a variety of ecosystems that have been degraded by anthropogenic activities, such as those found in agricultural, urban, overgrazed rangelands, and historically mined settings (Ippolito and Barbarick, 2022; Natasha et al., 2021; Ducey et al., 2021; Joseph et al., 2021; Buchanan and Ippolito, 2021; Cui et al., 2021; Trippe et al., 2021; Lu et al., 2020a, b; Ippolito et al., 2020; Banet et al., 2020a, b).
However, one of the main challenges with residuals land application is determining a proper application rate. Residual application rates should maximize soil health while minimizing any detrimental environmental effects. Proper application rates are not completely established and often vary depending on site characteristics. Establishing a target residual application rate will depend on whether the purpose of the residual is to improve soil physical (tilth, water holding capacity, aggregate stability, infiltration, porosity, bulk density), chemical (cation exchange capacity, pH, essential plant nutrient supply, C sequestration), or microbiological (enzymatic activity, overall microorganism populations or bacterial to fungal ratios, respiration rates, mineralization of necessary plant nutrients) properties (or a combination of physical-chemical-microbiological).
A secondary challenge to residuals land application for improving soil health is the use of new residuals products. Biosolids that have been pyrolyzed or have undergone wet gasification, in an effort to remove PFAS compounds, may not act similar to the initial biosolids when land applied. These new products may be used as-is, or blended with other materials to help facilitate improvements in soil health. These areas of research are novel, relatively new to the scientific community, with only a few published studies (e.g., Paz-Ferreiro et al., 2018; Shanmugam et al., 2021; Novotny et al., 2023) focused on material characterization. We are unaware of any literature that focuses attention on these new biosolids products as a means to improve soil health. Thus, Ippolito, Basta, and Borch will focus attention on biosolids biochar creation for the removal of PFAS. A subsequent study by Ippolito and Basta will focus attention on soil health improvements via the use of biosolids-biochar.
The W4170 workgroup proposes to: 1) collect soil physical, chemical, biological, and nutrient characteristics under current and new residuals and wastewater scenarios; and 2) investigate the ability of residuals and wastewaters to improve soil health and carbon sequestration. Laboratory, greenhouse, and field research designed to assess the ability of residuals and wastewaters to alter various soil characteristics and ultimately soil health, will be conducted. Soil bulk density, water stable aggregates, infiltration, plant available water, pH, carbon, EC, inorganic trace elements, microbial biomass, potentially mineralizable N and beta-glucosidase activity will be compared among residuals- and fertilizer-amended soils. These soil attributes and others as they come on-line (e.g., microbial community structure profiling techniques) will be analyzed separately and collectively in order to create a framework for understanding how residuals applications to soils affect various aspects of soil health, and ultimately how soil health is linked to plant, animal, human, and environmental health.
Collaborative research among W4170 members at Colorado State University (Borch, Ippolito), Washington State University (Griffin-Lahue), Virginia Tech (Chaganti), Kansas State University (Hettiarachchi) and Ohio State University (Basta, and now Ippolito) will determine long-term benefits of biosolids land application to agricultural and disturbed soils with respect to soil health.
Task ii) Quantify the effects of biosolids and other residuals on pollutant (emerging contaminants and metals/metalloids) availability, assimilation, phytotoxicity, and remediation (FL, IN, KS, OH, WA)
At various sites located in Kansas City, MO, Hettiarachchi et al. will determine the feasibility of using Class A biosolids amendments for reducing risk associated with common urban and brownfields soil contaminants. These sites will be monitored to determine the effect of soil amendments on bioaccessibility and speciation of soil Pb and other contaminants, soil health, and soil-plant transfer of contaminants. In Washington, Brown et al. will use develop soil blends from Class A biosolids with other materials including foundry sand, woody debris, biochar, water treatment residuals to create end products suitable for broad use in urban areas including urban agriculture and green stormwater infrastructure, ranging in scale from rain gardens to larger scale bioretention systems. The impact of biosolids blends on urban soil contaminants including Pb, As, and emerging contaminants and on urban soil physical characteristics, and growth response and nutritional value of crops grown in urban gardens will be investigated in this work. Lee from IN and Judy from FL will collaborate with Brown (WA) and Hettiarachchi et al. (KS) to specifically evaluate PFAS presence and impact associated with their field studies.
Further research is needed to develop and evaluate advanced spectroscopic methods to predict the long-term bioavailability and toxicity of trace elements in residuals and residual-amended soils. Our ability to measure the form of a contaminant in soil may provide a more accurate assessment of the long-term fate and bioavailability of contaminants in amended soils. The research gaps on trace element bioavailability are (i) development and application of methods to evaluate the soil ingestion (i.e. human and ecological) exposure pathway, (ii) development and evaluation of methods used to evaluate trace element bioavailability to ecological receptors and risk-based ecological endpoints, and (iii) development and application of advanced solid phase spectroscopic methods.
In-situ (on site) soil remediation methods, where inexpensive materials (e.g., municipal, industrial residual byproducts) are added to contaminated soil to reduce the mobility and bioavailability of heavy metal and toxic organic chemical contaminants, offer a cost-effective alternative to traditional dig and haul approaches. Incidental ingestion is an important exposure pathway for assessing human health exposure and risk associated with trace-element contaminated soils. The bioavailability of Pb, As and other soil contaminants can be determined using in vitro gastrointestinal (IVG) methods that have been developed that simulate human gastrointestinal conditions in lieu of expensive animal models. However, there is currently no information on IVG methods for evaluating soil ingestion in a HERA framework for residuals (Juhasz et al., 2013). In order to define cleanup and remediation standards for contaminated sites, site-specific data. Relative bioavailability (RBA), a type of IVG, is a measure of the amount of the contaminant that is absorbed by a receptor as a result of contaminated soil exposure (i.e. incidental ingestion of soil). It is dependent on mineralogy, soil properties and the residence time of the contaminant in the soil (Ruby et al. 1999). RBA is often less than the assumed 100%. This may have a significant influence on human exposure and risk assessment. Adjustments to RBA may be achieved by conducting in vivo bioavailability or in vitro bioaccessibility studies. Over the past 2 decades, a limited number of studies have established the relationship between in vivo RBA and in vitro bioaccessibility for As, Cd, and Pb (Basta et al. 2007; Bradham et al., 2011; Denys et al., 2012; Hettiarachchi et al., 2003a; Juhasz et al. 2007b; Juhasz et al. 2009, 2010, 2011; Rodriguez et al. 1999; Ryan et al., 2004; Smith et al., 2011). Currently, the Relative Bioaccessibility Leaching Procedure (RBALP) developed by Drexler and Brattin (2007) is accepted for use in human health risk assessment (USEPA, 2007b). However, the viability for this test for sites outside of the CERCLA or NPL programs has not been demonstrated. Basta (2013) reported that RBALP did not predict reductions in bioavailable Pb for soil treatments previously shown to reduce bioavailable Pb (Scheckel et al., 2009). This is a serious obstacle because in situ remediation methods cannot be evaluated without an accurate in vitro method to access their ability to reduce Pb bioavailability and human exposure. We will develop and evaluate in vitro (including chemical speciation) and novel in vivo methods to correlate human and ecological health responses with risk-based bioavailability of trace elements in residuals and residual-treated soils.
Task iii) Develop and evaluate treatment strategies of residuals and wastewaters to reduce contaminant or pathogen loads. (FL, IN, IL)
W4170 members have already been developing and testing treatment strategies associated with nutrients, metalloids and pathogens, which will continue. The aspect of treatment will be focused on PFAS. Opportunities exist to decrease PFAS concentrations in both WWTP effluent and sludge from which biosolid-based fertilizers originate, by treating influent inputs of smaller volume but higher PFAS loads such as the dewatering solution/filtrate (centrate) resulting from the dewatering of sludge that is recycled back into the WWTP and landfill leachate. To address removal of PFAS from WWTP centrate, we will determine the technical and economic feasibility using a treatment train approach consisting of nanofiltration followed by electrochemical oxidation of the PFAS present in the nanofiltration retentate solutions. We will evaluate PFAS load in biosolids (Objective 1) and potential treatment options her in Objective 2. We will determine the reduction in PFAS leaching from biosolids after amending biosolids with sorbents at different rates, including drinking water treatment residuals (WTRs) and biochars. We will also evaluate if low temperature O2-free pyrolysis may reduce PFAS lability in laboratory and greenhouse studies. We will use a treatment train consisting of ultrafiltration (UF) followed by nanofiltration (NF) and subsequent electrochemical oxidation using microporous and UF REMs to reduce PFAS concentrations in centrate and targeted landfill leachates. We will quantify PFAS release from amended and unamended biosolids as a function of specific PFAS loads and characteristics, aluminum, iron, OM, and water-soluble carbon concentrations. This research will be done collaboratively between Indiana (Lee, PU), Florida (Judy, UF), Illinois (Brose, Kumar – MWRD).
For pyrolysis treatment of biosolids, we propose the use of low temperature (350 °C – 600 °C) low to no O2 pyrolysis as a technology to reduce PFAS release associated with biosolids by entrapment and sorption but retain the value of the biosolids. We will evaluate if biochar produced from biosolids under low temperature pyrolysis of biosolids can lead to PFAS entrapment thereby reducing PFAS leachability and bioavailabilty and if biosolid-derived biochar can serve as a sorptive sink for PFAS released from untreated biosolids, thus allowing the mix to maintain key fertilizer properties while minimizing PFAS mobility and plant uptake. These hypotheses will be tested using both laboratory- and greenhouse-based studies. Our ultimate goal is to target the biosolids with high PFAS loads for pyrolysis andshow that a mix of this biochar with untreated biosolids could be safe for field-application. This would prevent biosolids from ending up in landfills and incineration and allow them to continue to be used as a rich nutrient source to improve soil and crop health. This research will be done collaboratively between Indiana (Lee, PU), Florida (Judy, UF), Illinois (Brose, Kumar – MWRD and Zheng, UI).
Measurement of Progress and Results
Outputs
- • We will improve our understanding of the fate and transport of emerging contaminants (with an emphasis on PFAS) and pathogens in residuals, reclaimed water, and amended soils to assess the environmental and human health risk-based effects of their application.
- • We will evaluate the capability of residuals and wastewaters to improve soil physical, chemical, and biological attributes, including the development of testing protocols and comparison of management practices that provide accurate nitrogen and phosphorus application rates, ameliorate heavy metal contaminated soils, increase carbon sequestration, and identify beneficial microbial communities.
- • We will develop strategies to minimize the presence and/or mobility and bioavailability of PFAS in biosolids- and waste-based fertilizer products.
- • We will develop strategies to minimize nutrients in treated wastewaters, while finding ways for safe and beneficial reuse of recovered nutrients and treated water in agricultural systems.
Outcomes or Projected Impacts
- • Improved mechanistic understanding of transformation of contaminants in residuals and reclaimed waters and in amended soils; their plant and human bioavailability; and human health risk and exposure associated with contaminants.
- • Sound decisions for use of residuals and wastewaters in a variety of ecosystems to improve soil health, air and water quality, and vegetative growth and quality. The research results will enable scientists to provide policy makers with information for developing residuals’ use practices across the US.
Milestones
(2024):Data generation from field and lab studies.(2025):Compilation of information on bioavailability, chemistry, transport, and toxicology of residuals- and wastewater-borne emerging contaminants and their effects on soil health.
(2026):Compilation of information on the effects of biosolids and other residuals on pollutant availability, assimilation, phytotoxicity, and remediation.
(2027):Compilation of information on treatment and reduction of PFAS mobility and bioavailability in biosolid- and waste-based products
Projected Participation
View Appendix E: ParticipationOutreach Plan
The Wx170 group traditionally includes members from industry such as Metropolitan Water Reclamation District of Greater Chicago (MWRDGC) and the CA Assoc. of Sanitation Agencies (CASA) as well as government (EPA). The group maintains close relationships with other water and wastewater utilities related associations, e.g., Water Environment Federation (WEF); Northwest Biosolids Management Association; North East Biosolids and Residuals Association (NEBRA); Mid Atlantic Biosolids Association; Midwest Biosolids Association, National Association of Clean Water Agencies. Through direct participation in conferences and workshops as well as webinar opportunities (e.g., those hosted by EPA, NEBRA, WEF), research findings will be reported to industry cooperators. The Wx170 group will continue to publish collaborative research reviews that are available to industry and academic partners, publish findings in scientific journals and present at national and international meetings. We will continue to participate in training sessions organized by local wastewater treatment agencies to provide their members with relevant information from our workgroup. Regional/national level outreach activities will be organized during our annual meetings as well as national/international conferences (e.g., ASA, SSSA, ACS, SETAC, ICOBTE, ICHMET).
Our members who hold extension appointments (representing CO, FL, IN, VA, OH) with help from the other members will develop and provide educational programming including extension publications beneficial to educators, regulators, local government decision makers, the concerned public, other scientists, and stakeholders from entities that generate potentially recyclable wastes. We will continue to convey the science on the risks and benefits of constituents in land-applied residuals and reclaimed waters through written publications, field demonstrations, workshops, webinars, and direct interaction with stakeholders and clientele.
Organization/Governance
The W4170 technical committee will consist of project leaders for the contributing states, the administrative advisor, and NIFA representatives. Voting membership includes all persons with contributing projects; however, only one vote is permitted for each research location.
Co-chairpersons, one from the western and one from the other regions, will be elected at the first authorized committee meeting after the renewal project has been accepted. The co-chairpersons will serve multiple years, if so desired by project participants, and will be responsible for meeting arrangements, the annual reports, coordination of research activities, compilation of regional research data, and preparation of the renewal proposal. One representative each from the western and the other regions and a secretary will be elected annually. Office terms for annually elected positions begin immediately after completion of the annual committee meeting. An Executive Committee, consisting of the co-chairs, the secretary, and the two regional representatives, will serve as a guidance body in matters such as new project participant additions and meeting agenda.
In addition to the official project representatives, other researchers with activities that contribute to the project have been and will continue to be invited to participate on a regular basis.
Land Grant Participating States/Institutions: AZ, CA, CO, DE, FL, GA, HI, IN, KS, KY, MA, MI, MN, NE, NM, OH, OK, OR, PA, TN, TX, VA, WY
Non Land Grant Participating States/Institutions: Black & Veatch Inc., California Association of Sanitation Agencies, Denali Water Solutions, Lystek International Limited USA Operations, Metropolitan Water Reclamation District of Greater Chicago, MWRD-Chicago, Orange County Sanitation District, San Francisco Public Utilities Commission, University of Massachusetts, University of Washington
The California Association of Sanitation Agencies (CASA), along with Northwest Biosolids (NW Biosolids), North East Biosolids and Residuals Association (NEBRA), the Midwest Biosolids Association (MBA), The Mid Atlantic Biosolids Association (MABA), Virginia Biosolids Council (VBC), the Southeast Biosolids Association (SEBA), the National Association of Clean Water Agencies (NACWA), and the Water Environment Federation (WEF) strongly support the continuation of the W4170 multi-state research committee. W4170 and all of its earlier iterations has been the premier scientific authority on biosolids, and other organic amendments, for the past 5+ decades. They have produced the seminal research products for these invaluable renewable resources. The regulatory and regulated communities rely on the credibility of the research produced by this distinguished committee. The next 5 years will be especially critical to ensure credible research is done which will be necessary to inform regulatory development and public opinion on the fate and transport of emerging constituents such as PFAS and microplastics. The Associations listed above appreciate the ability to participate directly in meetings and on research projects with W4170 committee members and in their willingness to explain findings and interpret research conclusions. Thank you very much for the continued support for this essential committee.
Literature Cited
Abiven, S., S. Menasseri, C. Chenu. 2009. The effects of organic inputs over time on soil aggregate stability—a literature analysis. Soil Biol. Biochem. 41:1–12.
Alasmary, Z., G.M. Hettiarachchi, K. L. Roozeboom, L.C. Davis, L.E. Erickson, T. Todd, V. Pidlisnyuk, T. Stefanovska, and J. Trogl. 2021. Phytostabilization of a Contaminated Military Site Using Miscanthus and Soil Amendments. J. Environ. Qual. 50: 1220-1232.
Alasmary, Z., T. Todd, G.M. Hettiarachchi, T. Stefanovska, V. Pidlisnyuk, K. Roozeboom, L. Erickson, L. Davis, and O. Zhukov. 2020. Effect of soil treatments and amendments on the nematode community under miscanthus growing in a lead contaminated military site. Agronomy 10, 1727.
Alvarez-Campos, O., and G.K. Evanylo. 2019a. Plant available nitrogen estimation tools for a biosolids-amended, clayey urban soil. Soil Sci. Soc. Am. J. (Accepted Feb 22, 2019)
Alvarez-Campos, O., and G.K. Evanylo. 2019b. Biosolids improves urban soil properties and vegetable production in urban agriculture. Urban Agriculture & Regional Food Systems. (Accepted with minor revisions, May 17, 2019)
Alvarez-Campos, O., M. Badzmierowski, and G.K. Evanylo. 2018. Development and assessment of exceptional quality biosolids products for urban gardens. Compost Science & Utilization 26(4): 234-245.
Alvarez-Campos, O., M. Badzmierowski, G.K. Evanylo, K. Bamber, H-C Yu. 2018. Development and testing of exceptional quality biosolids-based by-products for urban landscapes. Compost Science & Utilization 26(4): 234-245.
Attanayake, C.P., G.M. Hettiarachchi, A. Harms, D. Presley, S. Martin, and G.M. Pierzynski. 2014. Field Evaluations on Soil Plant Transfer of Lead from an Urban Garden Soil. J. Environ. Qual. Vol. 43, 475-487.
Attanayake, C.P., G.M. Hettiarachchi, Q. Ma, G.M. Pierzynski, M.D. Ransom. 2017. Lead speciation and in vitro bioaccessibility of compost-amended urban garden soils. J. Environ. Qual. 46:1215-1224.
Attanayake, C.P., G.M. Hettiarachchi, S. Martin, G.M. Pierzynski. 2015. Potential bioavailability of lead, arsenic, and polycyclic aromatic hydrocarbons in compost-amended urban soils. J. Environ. Qual. 44:930-944.
Awasthi, M.K., P. Ganeshan, N. Gohil, V, Kumar, V. Singh, K. Rajendran, S. Harirchi, M.K. Solanki, R. Sindhu, P. Binod, and Z. Zhang, 2023. Advanced approaches for resource recovery from wastewater and activated sludge: A review. Bioresource Technology, p.129250.
Banet, T., I. Zohar, I.M. Litaor, M. Massey, J.A. Ippolito. 2020b. Phosphorus removal from swine wastewater using aluminum-based water treatment residuals. Res. Conserv. Recycl. X. 6:100039.
Banet, T., M. Massey, I. Zohar, I. Litaor, J.A. Ippolito. 2020a. Assessing modified aluminum-based water treatment residuals as a plant-available phosphorus source. Chemosphere. 247: 125949.
Barbarick, K.A., J.A. Ippolito, J. McDaniel. 2016. Path Analysis of grain P, Zn, Cu, Fe, and Ni in a biosolids-amended dryland wheat agroecosystem. J. Environ. Qual. 45:1400-1404.
Barbarick, K.A., J.A. Ippolito, J. McDaniel. 2017. Meta-analysis of biosolids effect in dryland wheat agroecosystems. J. Environ. Qual. 46:452-460.
Barbarick, K.A., J.A. Ippolito, J.P. McDaniel. 2015. Uptake Coefficients for Biosolids-Amended Dryland Winter Wheat. J. Environ. Qual. 44:286-292.
Basta, N.T., A. Juhasz. 2014. Chapter 9: Using In Vivo Bioavailability and/or In Vitro Gastrointestinal Bioaccessibility Testing to Adjust Human Exposure from Soil Ingestion. In : R.J.Bowell, J. Majzlan and C.Alpers (eds.) Geochemistry, Mineralogy and Microbiology of Arsenic in Environment, Reviews in Mineralogy and Geochemistry, Mineralogical Society of America.
Basta, N.T., D.M. Busalacchi, L.S. Hundal, K. Kumar, R.P. Dick, R.P. Lanno, J. Carlson, A.E. Cox, T.C. Granato. 2015. Restoring ecosystem function in degraded urban soil using biosolids, biosolids blend and compost. J. Environ. Qual. Special Issue: Soil in the City. 45:74-83.
Basta, N.T., D.M. Busalacchi, L.S. Hundal, K. Kumar, R.P. Dick, R.P. Lanno, J. Carlson, A.E. Cox, T.C. Granato. 2016. Restoring ecosystem function in degraded urban soil using biosolids, biosolids blend and compost. Special Issue: Soil in the City. J. Environ. Qual. 45:74-83.
Basta, N.T., R. Gradwohl, K.L. Snethen, J.L. Schroder. 2001. Chemical immobilization of lead, zinc, and cadmium in smelter-contaminated soils using biosolids and rock phosphate. J. Environ. Qual. 30:1222-1230.
Basta, N.T. 2013. Using Biosolids to Restore and Revitalize Soil Ecosystem Services to Degraded Soils in the Calumet Region. Ecological Restoration Seminar and Workshop. Metropolitan Water Reclamation District of Greater Chicago, Cicero, IL. Jan. 18, 2013.
Basta, N.T., and J. Wragg. 2013. Advances in Bioaccessibility Methodology. Journal of Environmental Science and Health, Part A. 48(6):593.
Basta, N.T., J. N. Foster, E.A. Dayton, R. R. Rodriguez, and S.W. Casteel. 2007. The effect of dosing vehicle on arsenic bioaccessibility in smelter-contaminated soils. Invited manuscript for the special JEHS publication “Bioaccessibility and human bioavailability of soil contaminants” J. Environ. Health Sci. Part A. 42:1275-1281.
Beniston, J., R. Lal. 2012. Improving Soil Quality for Urban Agriculture in North Central U.S. In R. Lal, and B. Augustin (eds), Carbon Sequestration in Urban Ecosystems. Springer Dordrecht Heidelberg London New York, p. 173-196.
Bertagnoli, A.D., K.A. Meinhardt, M. Pannu, S. Brown, S. Strand, S.C. Fransen, D.A. Stahl. 2016. Influence of edaphic and management factors on the diversity and abundance of ammonia-oxidizing thaumarchaeota and bacteria in soils of bioenergy crop cultivars. Environ. Microbiology Reports. 7:2:312-320.
Bhalsod, G.D., Y.H. Chuang, S. Jeon, W.J. Gui, H. Li, E.T. Ryser, A.K. Guber, W. Zhang. 2018. Uptake and Accumulation of Pharmaceuticals in Overhead- and Surface-Irrigated Greenhouse Lettuce. J. Agric. Food Chem. 66:822-830.
Bizkarguenaga, E., I. Zabaleta, L. Mijangos, A. Iparraguirre, L.A. Fernandez, A. Prieto, O. Zuloaga. 2016. Uptake of perfluorooctanoic acid, perfluorooctane sulfonate and perfluorooctane sulfonamide by carrot and lettuce from compost amended soil. Sci. Total Environ. 571:444-451.
Blaine, A.C., C.D. Rich, E.M. Sedlacko, K.C. Hyland, C. Stushnoff, E.R.V. Dickenso, C.P. Higgins 2014b. Perfluoroalkyl Acid Uptake in Lettuce (Lactuca sativa) and Strawberry (Fragaria ananassa) Irrigated with Reclaimed Water. Environ. Sci. Technol. 48:14361-14368.
Blaine, A.C., C.D. Rich, E.M. Sedlacko, L.S. Hundal, K. Kumar, C. Lau, M.A. Mills, K.M. Harris, C.P. Higgins. 2014a. Perfluoroalkyl Acid Distribution in Various Plant Compartments of Edible Crops Grown in Biosolids-Amended soils. Environ. Sci. Technol. 48:7858-7865.
Brown, S. 2016. Greenhouse gas accounting for landfill diversion of food scraps and yard waste. Compost Sci. 24:11-19.
Brown, S. and N. Beecher. 2020 Carbon accounting for compost use in urban areas. Compost Sci. & Utilization. 27:227-239 https://doi.org/10.1080/1065657X.2019.1674224
Brown, S. L., R.L. Chaney, G.M. Hettiarachchi. 2016a. Lead in urban soils: a real or perceived concern for urban agriculture? J. Environ. Qual. 45:26-36.
Brown, S. L., R.L. Chaney, J. Hallfrisch, J.A. Ryan, W.R. Berti. 2004. In situ soil treatments to reduce the phyto- and bioavailability of lead, zinc, and cadmium. J. Environ. Qual. 33:522-531.
Brown, S., J.A. Ippolito, L. Hundal, and N.T. Basta. 2020 Municipal biosolids- a resource for sustainable communities. Current Opinion in Environmental Science & Health. 14:56-62
Brown, S., K. Kurtz, A. Bary, and C. Cogger. 2011a. Long-term effects of organic amendments on soil carbon storage and physical properties. Environ. Sci. & Tech. dx.doi.org/10.1021/es2010418
Brown, S., K. Kurtz, A. Bary, C. Cogger. 2011b. Quantifying benefits associated with land application of organic residuals in Washington State. Environ. Sci. Technol. 45:7451-7458.
Brown, S.L. 2017. Making Soils from Urban Wastes. In Advances in Soil Science: Urban Soils R. Lal and B.A. Stewart (Ed) Taylor and Francis Publishers.
Brown, S.L., A. Corfman, K. Mendrey, K. Kurtz, D. Grothkopp. 2016b. Stormwater Bioretention systems- testing the phosphorus saturation index and compost feedstocks as predictive tools for system performance. J. Environ. Qual. 45:98-106.
Brown, S.L., A. Trlica, J. Lavery, M. Teshima. 2017. Carbon sequestration potential on mined lands. In Mine Site Rehabilitation and Restoration. N. Bolan, M.B. Kirkham and Y. Ok (eds.) Taylor and Francis Publishers.
Brown, S.L., D. Butman and K. Kurtz. 2023. Steps to circularity: impact of resource recovery and urban agriculture in Seattle and Tacoma, Washington. J. Environ. Manage. In Review
Brown, S.L., K. McIvor, E. Snyder (Eds). 2016c. Sowing seeds in the city: Ecological and Municipal Considerations. Springer Publishers.
Brown, S.L., K. McIvor, E. Snyder (Eds). 2016d. Sowing seeds in the city: Human Dimensions. Springer publishers.
Brown, S.L., R.L. Chaney. 2016. Use of amendments to restore ecosystem function to metal mining impacted sites: tools to evaluate efficacy. Current Pollution Reports. 2:91-102
Buchanan, C.M., and J.A. Ippolito. 2021. Long-term biosolids applications to overgrazed rangelands improve soil health. MDPI Agronomy. 11: 1339.
Bünemann, E. K., Bongiorno, G., Bai, Z., Creamer, R. E., De Deyn, G., de Goede, R., Fleskens, L., Geissen, V., Kuyper, T. W., Mäder, P., Pulleman, M., Sukkel, W., van Groenigen, J. W., & Brussaard, L. (2018). Soil quality – A critical review. Soil Biology and Biochemistry, 120, 105–125. https://doi.org/10.1016/j.soilbio.2018.01.030.
Carlson, J., J. Saxena, N. Basta, L. HUndal, D. Busalacchi. 2015. Application of organic amendments to restore degraded soil: effects on microbial properties. Environ. Monit. Assess 187(3):1-15.
Chaganti, V.N. and D.M. Crohn. 2014. Evaluation of compost blankets for erosion control and runoff water quality on a constructed hillslope in southern California. Transactions of the ASABE, 57(2), pp.403-416.
Chen, W. R., Ding, Y. J., Johnston, C. T., Teppen, B. J., Boyd, S. A., and Li, H. (2010). Reaction of Lincosamide Antibiotics with Manganese Oxide in Aqueous Solution. Environmental Science & Technology 44, 4486-4492.
Chen, Z., Zhang, Y., Gao, Y., Boyd, S. A., Zhu, D., and Li, H. (2015). Influence of Dissolved Organic Matter on Tetracycline Bioavailability to an Antibiotic-Resistant Bacterium. Environmental Science & Technology 49, 10903-10910.
Chen, Z. Y., Zhang, W., Wang, G., Zhang, Y. J., Gao, Y. Z., Boyd, S. A., Teppen, B. J., Tiedje, J. M., Zhu, D. Q., and Li, H. (2017). Bioavailability of Soil-Sorbed Tetracycline to Escherichia coli under Unsaturated Conditions. Environmental Science & Technology 51, 6165-6173.
Choi, Y. J., Lee, L. S., Hoskins, T. D., Gharehveran, M. M., and Sepulveda, M. S. (2023). Occurrence and implications of per and polyfluoroalkyl substances in animal feeds used in laboratory toxicity testing. Science of the Total Environment 867.
Choi, Y., R. Kim Lazcano, P. Yousefi, H. Trim and L.S. Lee. 2019. Perfluoroalkyl Acid Characterization in U.S. Municipal Organic Solid Waste Composts, Environ. Sci. Technol. Letters. Accepted and published online ez-2019-00280t.
Chuang, Y.-H., Liu, C.-H., Sallach, J. B., Hammerschmidt, R., Zhang, W., Boyd, S. A., and Li, H. (2019). Mechanistic study on uptake and transport of pharmaceuticals in lettuce from water. Environment International 131.
Clark, E.V., C. Zipper, W.L. Daniels, Z.W. Orndorff, M.J. Keefe. 2017. Modeling Patterns of Total Dissolved Solids Release from Central Appalachia, USA Mine Spoils. J. Environ. Qual. 46:55-63.
Codling, G., S. Hosseini, M.B. Corcoran, S. Bonina, T. Lin, A. Li, N.C. Sturchio, K.J. Rockne, K. Ji, H. Peng, J.P. Giesy. 2018. Current and historical concentrations of poly and perfluorinated compounds in sediments of the northern Great Lakes - Superior, Huron, and Michigan. Environ. Pollut. 236:373-381.
Cogger, C.G., A.I. Bary, E. A. Myhre, A. Fortuna. 2013. Biosolids applications to tall fescue have long-term influence on soil nitrogen, carbon and phosphorus. J. Environ Qual. 42:516-522.
Craul, P.J. 1985. A description of urban soils and their desired characteristics. J. Arboriculture 11:330-339.
Cui, L., J.A. Ippolito, M. Noerpel, and K.G. Scheckel. 2021. Nutrient alterations following biochar application to a Cd-contaminated solution and soil. Biochar. 3:457-468. https://doi.org/10.1007/s42773-021-00106-1.
Dalahmeh, S., S. Tirgani, A.J. Komakech, C.B. Niwagaba, L. Ahrens. 2018. Per- and polyfluoroalkyl substances (PFASs) in water, soil and plants in wetlands and agricultural areas in Kampala, Uganda. Sci. Tot. Environ. 631-632:660-667.
Daniels, W.L., C.E. Zipper, Z.W. Orndorff, J. Skousen, C.D. Barton, L.M. McDonald, M.A. Beck. 2016. Predicting total dissolved solids release from central Appalachian coal mine spoils. Env. Pollut. 2016:371-379.
De Sousa, C., A.M.M. Carroll, S. Whitehead, L. Berman, S. Coffin, L. Heberle, G. Hettiarachchi, S. Martin, K. Sullivan, and J. Van Der Kloot. 2023. A review of brownfields revitalisation and reuse research in the US over three decades, Local Environment, https:// doi.org/10.1080/13549839.2023.2248625
Defoe, P.P, G.M. Hettiarachchi, C. Benedict, S. Martin. 2014. Safety of Gardening on Lead- and Arsenic-Contaminated Urban Brownfields. J. Environ. Qual. 43:2041- 2078.
Desjardins, M. 2022. Bathroom reading: connecting biosolids to soil health. Soil Health. Washington State University. Available at: https://soilhealth.wsu.edu/2022/10/28/bathroom-reading-connecting-biosolids-to-soil-health/
Diacono, M., F. Montemurro. 2010. Long-term effects of organic amendments on soil fertility. A review. Agron. Sustain. Develop. 30:401–422.
Ding, Y., Zhang, W., Gu, C., Xagoraraki, I., and Li, H. (2011). Determination of pharmaceuticals in biosolids using accelerated solvent extraction and liquid chromatography/tandem mass spectrometer. J. Chromatgra A. 1218, 10-16.
Dodgen, L. K., Ueda, A., Wu, X., Parker, D. R., and Gan, J. (2015). Effect of transpiration on plant accumulation and translocation of PPCP/EDCs. Environmental Pollution 198, 144-153.
Drexler, J.W., and W.J. Brattin. 2007. An in vitro procedure for estimation of lead relative bioavailability: with validation. Human Ecol. Risk Assess. 13:383–401.
Ducey, T.F., G.C. Sigua, J.M. Novak, J.A. Ippolito, K.A. Spokas, and M.G. Johnson. 2021. Microbial response to phytostabilization in mining impacted soils using maize in conjunction with biochar and compost. Microorganisms. 9:2545. https://doi.org/10.3390/microorganisms9122545.
Elzobair, K.A., M.E. Stromberger, J.A. Ippolito, R.D. Lentz. 2016b. Contrasting effects of biochar versus manure on soil microbial communities and enzyme activities in an Aridisol. Chemosphere. 142:145-152.
Elzobair, K.A., M.E. Stromberger, J.A. Ippolito. 2016a. Stabilizing effect of biochar on soil extracellular enzymes after a denaturing stress. Chemosphere. 142:114-119.
Fu, Q., Malchi, T., Carter, L. J., Li, H., Gan, J., and Chefetz, B. (2019). Pharmaceutical and Personal Care Products: From Wastewater Treatment into Agro-Food Systems. Environmental Science & Technology 53, 14083-14090.
Fu, Q., Wu, X., Ye, Q., Ernst, F., and Gan, J. (2016). Biosolids inhibit bioavailability and plant uptake of triclosan and triclocarban. Water Research 102, 117-124.
Gravesen, C. R., Lee, L. S., Alukkal, C. R., Openiyi, E. O., and Judy, J. D. (2023). Per- and polyfluoroalkyl substances in water treatment residuals: Occurrence and desorption. Journal of Environmental Quality.
Gregory, J.H., M.D. Dukes, P.H. Jones, G.L. Miller. 2006. Effect of urban soil compaction on infiltration rate. J. Soil and Water Conservation 61:117-124.
Hacheney, N., S. Brown. 2017. Correctional facility adopts multiple food scrap practices. Biocycle. 58:9:34.
Heckman, J.R., J.S. Angle, R.L. Chaney. 1987. Residual effects of sewage sludge on soybeans: II. Accumulation of soil and symbiotically fixed nitrogen. J. Environ. Qual. 16:118-124.
Henry, H., M.F. Naujokas, C. Attanayake, N.T. Basta, Z. Cheng, G.M. Hettiarachchi, M. Maddaloni, C. Schadt, K.G. Scheckel. 2015. Bioavailability-based in situ remediation to meet future lead (Pb) standards in urban soils and gardens. Environ. Sci. Technol. 49:8948–8958.
Heronemus, E., K.H.H. Gamage, G.M. Hettiarachchi, and P. Parameswaran. 2021. Efficient recovery of phosphorus and sulfur from Anaerobic Membrane Bioreactor (AnMBR) permeate using chemical addition of iron and evaluation of its nutrient availability for plant uptake, Sci. Total Environ. 146850. https://doi.org/10.1016/j.scitotenv.2021.146850.
Hettiarachchi, G. M., G. M. Pierzynski. 2002. In situ stabilization of soil lead using phosphorus and manganese oxide: Influence of plant growth. J. Environ. Qual. 31: 564-572.
Hettiarachchi, G.M., G.M. Pierzynski, F.W. Oehme, O. Sonmez, J.A. Ryan. 2003a. Treatment of contaminated soil with phosphorus and manganese oxide reduces absorption of lead by Sprague-Dawley rats. J. Environ. Qual. 32: 1335-1345.
Hettiarachchi, G.M., J. A. Ryan, K. G. Scheckel, S. R. Sutton, M. Newville. 2006. µ-XANES and µ-XRF investigations of metal binding mechanisms in biosolids. J. Environ. Qual. 35: 342-351.
Hettiarachchi, G.M., J.A. Ryan, R.L. Chaney, C.M. La Fleur. 2003b. Adsorption of cadmium onto different fractions of biosolids-amended soils. J. Environ. Qual. 32: 1684-1693.
Ippolito, J.A. 2015. Aluminum-based water treatment residuals use in a constructed wetland for capturing urban runoff phosphorus: Column study. Water, Air, and Soil Pollut. 226:334. DOI 10.1007/s11270-015-2604-2.
Ippolito, J.A., and K.A. Barbarick. 2022. The Clean Water Act and biosolids: A 45-year chronological review of biosolids land application in Colorado. J. Environ. Qual. 51:780-796. http://doi.org/10.1002/jeq2.20376.
Ippolito, J.A., C.M. Berry, D.G. Strawn, J.M. Novak, J. Levine, A. Harley. 2017. Biochars reduce mine land soil bioavailable metals. J. Environ. Qual. 46:411-419.
Ippolito, J.A., Cui, L., C. Kammann, N. Wrage-Monnig, J.M. Estavillo, T. Fuertes-Mendizabal, M. Cayuela, G. Sigua, J. Novak, K. Spokas, and N. Borchard. 2020. Feedstock choice, pyrolysis temperature and type influence biochar characteristics: A comprehensive review. Biochar. 2:421-438.
Ippolito, J.A., K.A. Barbarick, M.W. Paschke, R.B. Brobst. 2010. Infrequent composted biosolids applications effect semi-arid grassland soils and vegetation. J. Environ. Management. 91:1123-1130.
Ippolito, J.A., K.A. Barbarick, R.B. Brobst. 2014. Copper and zinc speciation in a biosolids-amended, semiarid grassland soil. J. Environ. Qual. 43:1576-1584.
Ippolito, J.A., K.G. Scheckel, K.A. Barbarick. 2009. Selenium adsorption to aluminum-based water treatment residuals. J. Colloid Interf. Sci. 338:48-55.
Ippolito, J.A., M.E. Stromberger, R.D. Lentz, R.S. Dungan. 2016a. Hardwood biochar and manure co-application to a calcareous soil. Chemosphere. 142:86-91.
Ippolito, J.A., T.F. Ducey, K. Diaz, and K.A. Barbarick. 2021. Long-term biosolids land application influences soil health. Science of the Total Environment. 791:148344.
Ippolito, J.A., T.F. Ducey, K.B. Cantrell, J.M. Novak, R.D. Lentz. 2016b. Designer, acidic biochar influences calcareous soil characteristics. Chemosphere. 142:184-191.
Ippolito, J.A., D.G. Strawn, and K.G. Scheckel. 2013. Investigation of copper sorption by sugar beet processing lime waste. J. Environ. Qual. 42:919-924.
Jay, J.G., S.L. Brown, K. Kurtz, F. Grothkopp. 2017. Predictors of phosphorus leaching from bioretention soil media. J. Environ. Qual. 46:1098-1105
Jin, V.L., K.N. Potter, M-V. V. Johnson, R.D. Harmel, J.G. Arnold. 2015. Surface-Applied Biosolids Enhance Soil Organic Carbon and Nitrogen Stocks but Have Contrasting Effects on Soil Physical quality. Appl. Environ. Soil Sci. http://dx.doi.org/10.1155/2015/715916.
Joseph, S., A. Cowie, L. Van Zwieten, N. Bolan, A. Budai, W. Buss, M.L. Cayuela, E. Graber, J. Ippolito, Y. Kuzyakov, Y.S. Ok, K. Palansooriya, J. Shepherd, S. Stephens, Z. Weng, Y. Luo, and J. Lehmann. 2021. How biochar works and when it doesn’t: A review of mechanisms controlling soil and plant response to biochar. Global Change Biology Biochem. 13:1731-1764. Doi: 10.1111/gcbb.12885.
Juhasz, A.L., E. Smith, J. Weber, M. Rees, A. Rofe, T. Kuchel. 2007. Comparison of in vivo and in vitro methodologies for the assessment of arsenic bioavailability in contaminated soils. Chemosphere. 69:961–966.
Juhasz, A.L., J. Weber, E. Smith, R. Naidu, M. Rees, A. Rofe, T. Kuchel, L.Sansom. 2009. Assessment of four commonly employed in vitro arsenic bioaccessibility assays for predicting in vivo relative arsenic bioavailability in contaminated soils. Environ. Sci. Technol.43 (24):9487-9494.
Kaiser, M.L., M.L. Williams, N. Basta, M. Hand, S. Huber. 2015. When vacant lots become urban gardens: Characterizing the perceived and actual food safety concerns of urban agriculture in Ohio. J. Food Protect. 78(11):2070-2080.
Kammann, C., N. Borchard, M. Cayuela, N. Hagemann, J. Ippolito, S. Jeffery, J. Kern, D. Rasse, S. Sanna, H-P. Schmidt, K. Spokas, N. Wrage-Mönnig. 2017. Biochar as a tool to reduce the agricultural greenhouse burden – Knowns, unknowns and future research needs. J. Environ. Engineer. Landscape Management. 25:114-139.
Kannan, A., J. Dillavou, K.H.H. Gamage, G.M. Hettiarachchi, and P. Parameswaran. 2023. Recovery of high-quality Calcium phosphate fertilizer products from anaerobic membrane bioreactor treated swine wastewater. Chem. Eng. J. 453, 139539.
Karlen, D.L., M.J. Mausbach, J.W. Doran, R.G. Cline, R.F. Harris, G.E. Shuman. 1997. Soil quality: A concept, definition, and framework for evaluation (A guest editorial). Soil Sci. Soc. Am. J. 61:4-10.
Karna, R., G.M. Hettiarachchi, M. Newville, C-J Sun, Q. Ma. 2016. Synchrotron-Based X-Ray Spectroscopy Studies for Redox-Based Remediation of Lead, Zinc, and Cadmium in Mine Waste Materials. J. Environ. Qual. 45:1883-1893.
Kim, J., Xin, X., Mamo, B. T., Hawkins, G. L., Li, K., Chen, Y., Huang, Q., and Huang, C.-H. (2022). Occurrence and Fate of Ultrashort-Chain and Other Per- and Polyfluoroalkyl Substances (PFAS) in Wastewater Treatment Plants. Acs Es&T Water.
Lazcano, R. K., C. de Perre, M.L. Mashtare and L.S. Lee. 2019a. Characterizing Per- and Polyfluoroalkyl Substances in Commercially Available Biosolid- versus Nonbiosolid-based Organic Fertilizers, In submission to Environmental Science & Technology.
Lazcano, R. K., C. de Perre, M.L. Mashtare and L.S. Lee. 2019b. Per- and Polyfluoroalkyl Substances in Commercially Available Biosolid-Based Fertilizer Products: The Effect of Post-treatment Processes, Water Environ. Research, WER-2019-04-0209
Lazcano, R. K., Choi, Y. J., Mashtare, M. L., and Lee, L. S. (2020). Characterizing and Comparing Per- and Polyfluoroalkyl Substances in Commercially Available Biosolid and Organic Non-Biosolid-Based Products. Environmental Science & Technology 54, 8640-8648.
Lazcano, R. K., de Perre, C., Mashtare, M. L., and Lee, L. S. (2019). Per- and polyfluoroalkyl substances in commercially available biosolid-based products: The effect of treatment processes. Water Environment Research 91, 1669-1677.
Laidlaw, M.A.S., G.M. Filippelli, S. Brown, J. Paz-Ferreiro, S.M. Reichman, P. Netherway, A. Truskewzcz, A.S. Ball, H.W. Mielke. 2017. Case studies and evidence-based approaches to addressing urban soil lead contamination. Appl. Geochem. 83:14-30.
Laird, D.A., J.M. Novak, H.P. Collins, J.A. Ippolito, D.L. Karlen, R.D. Lentz, K.R. Sistani, K. Spokas, R.S. Van Pelt. 2017. Multi-year and multi-location soil quality and crop biomass yield responses to hardwood fast pyrolysis biochar. Geoderma. 289:46-53.
Lauriault, L.M., M.K. Darapuneni, G.K. Martinez, J. Box1, P.L. Cooksey, J. Jennings, S. Jenings & A. Williams. 2018. Impact of Water Source on Alfalfa Establishment and Production, https://tucumcarisc.nmsu.edu/documents/2018-annual-report.pdf
Lee, H., A.G. Tevlin, S.A. Mabury, S.A. Mabury. 2014. Fate of Polyfluoroalkyl Phosphate Diesters and Their Metabolites in Biosolids-Applied Soil: Biodegradation and Plant Uptake in Greenhouse and Field Experiments. Environ. Sci. Technol. 48:340-349.
Leonard, E. J. Bodas, S. Brown and B. Axt. 2021. Carbon balance for biosolids use in commercial Douglas Fir plantations in the Pacific Northwest. J. Environ. Manage. 295:113115.
Li, C., Wang, Y., Wang, Y., Wang, Z., and Huang, Q. (2022a). Electrochemical oxidation combined with UV irradiation for synergistic removal of perfluorooctane sulfonate (PFOS) in water. Journal of Hazardous Materials 436.
Li, G., Dunlap, J., Wang, Y., Huang, Q., and Li, K. (2022b). Environmental Life Cycle Assessment (LCA) of Treating PFASs with Ion Exchange and Electrochemical Oxidation Technology. Acs Es&T Water 2, 1555-1564.
Li, J., K. Li, X-Y. Cui, N.T. Basta, L-P. Li, H-B. Li, L.Q. Ma. 2015. In vitro bioaccessibility and in vivo relative bioavailability in 12 contaminated soils: Method comparison and method development. Sci. Total Environ. 532:812-820.
Li, Y., He, J., Qi, H., Li, H., Boyd, S. A., and Zhang, W. (2020). Impact of biochar amendment on the uptake, fate and bioavailability of pharmaceuticals in soil-radish systems. Journal of Hazardous Materials 398.
Li, Y., Sallach, J. B., Zhang, W., Boyd, S. A., and Li, H. (2019). Insight into the distribution of pharmaceuticals in soil-water-plant systems. Water Research 152, 38-46.
Li, Y., Sallach, J. B., Zhang, W., Boyd, S. A., and Li, H. (2022c). Characterization of Plant Accumulation of Pharmaceuticals from Soils with Their Concentration in Soil Pore Water. Environmental Science & Technology.
Li, Y., J.B. Sallach, W. Zhang, S.A. Boyd, H. Li. 2019. Insight into the Distribution of Pharmaceuticals in Soil-Water-Plant Systems. Water Res. 152:38-46.
Lindstrom, A.B., M.J. Strynar, A.D. Delinsky, S.F. Nakayama, L. McMillan, E.L. Libelo, M. Neill, L. Thomas. 2011. Application of WWTP Biosolids and Resulting Perfluorinated Compound Contamination of Surface and Well Water in Decatur, Alabama, USA. Environ. Sci. Technol. 45:8015-8021.
Litaor, M.I., S. Schechter, I. Zohar, M.S. Massey, and J.A. Ippolito. 2019. Making phosphorus fertilizer from dairy wastewater with aluminum water treatment residuals. Soil Sci. Soc. Am. J. 83:649-657.
Liu, Z.Y., Y.L. Lu, Y.J. Shi, P. Wang, K. Jones, A.J. Sweetman, A.C. Johnson, M. Zhang, Y.Q. Zhou, X.T. Lu, C. Su, S. Sarvajayakesavaluc, K. Khan. 2017. Crop bioaccumulation and human exposure of perfluoroalkyl acids through multi-media transport from a mega fluorochemical industrial park, China. Environ. Int. 106:37-47.
Lu. Y.G, Silveira, M.L., Vendramini, J.M.B., Erickson, J.E., Li, Y. 2020. Biosolids and biochar application effects on bahiagrass herbage accumulation and nutritive value. Agronomy Journal 112, 1330-1345.
Lu. Y.G, Silveira, M.L., Cavigelli, M., O’Connor, G.A., Vendramini, J.M.B., Erickson, J.E., and Li, Y.C. 2020a. Biochar impacts on nutrient dynamics in subtropical grassland soil: 2. Greenhouse gas emissions. Journal of Environmental Quality 49:1421-1434. DOI: 10.1002/jeq2.20141.
Lu. Y.G, Silveira, M.L., O’Connor, G.A., Vendramini, J.M.B., Erickson, J.E., Li, Y.C., and Cavigelli, M. 2020b. Biochar impacts on nutrient dynamics in subtropical grassland soil: 1. Nitrogen and phosphorus leaching. Journal of Environmental Quality 49:1408-1420. DOI: 10.1002/jeq2.20139.
Massey, M.S., I. Zohar, J.A. Ippolito, and I.M. Litaor. 2018. Phosphorus sorption to aluminum-based water treatment residuals reacted with dairy wastewater: 2. X-ray absorption spectroscopy. J. Environ. Qual. 47:546-553.
Mausel, P. W. (1971). Soil Quality in Illinois—An Example of a Soils Geography Resource Analysis∗. The Professional Geographer, 23(2), 127–136. https://doi.org/10.1111/j.0033-0124.1971.00127.x
Mehmood, K., E. Chávez Garcia, M. Schirrmann, B. Ladd, C. Kammann, N. Wrage-Mönnig, C. Siebe, J.M. Estavillo, T. Fuertes, M. Cayuela, G. Sigua, K. Spokas, A.L. Cowie, J. Novak, J.A. Ippolito, N. Borchard. 2017. Biochar research activities and their relation to development and environmental quality. A meta-analysis. Agron. Sustain. Dev. 37:22.
Meiman, P.J., N.R. Davis, J.E. Brummer, and J.A. Ippolito. 2012. Riparian shrub metal concentrations and growth in amended fluvial mine tailings. Water, Air, Soil Pollut. 223:1815-1828.
Meinhardt, K.A., A. Bertagnolli, M. Pannu, S.E. Strand, S.L. Brown, D.A. Stahl. 2015. Evaluation of revised polymerase chain reaction primers for more inclusive quantification of ammonia-oxidizing archaea and bacteria. Environ. Microbiology Reports, 7:2:354-363.
Morris, J., S. Brown, M. Cotton, H.S. Matthews. 2017. Life-cycle assessment harmonization and soil science ranking results on food-waste management methods. Environ. Sci. Technol. 51:5360-5367.
Natasha, N., M. Shahid, S. Khalid, I. Bibi, M.A. Naeem, N.K. Niazi, F.M.G. Tack, J.A. Ippolito, and J. Rinklebe. 2021. Influence of biochar on trace element uptake, toxicity, and detoxification in plants and associated health risks: A critical review. Critical Reviews in Environmental Science and Technology. 52:2803-2843. Available at: https://www.tandfonline.com/doi/full/10.1080/10643389.2021.1894064.
National Research Council. 2009. Urban stormwater management in the United States. Washington, DC: Press. Available at: https://www.nap.edu/catalog/12465/urban-stormwater-management-in-the-united-states.
Navarro, I., A. de la Torre, P. Sanz, M.A. Porcel, J. Pro, G. Carboneb, M.D. Martinez. 2017. Uptake of perfluoroalkyl substances and halogenated flame retardants by crop plants grown in biosolids-amended soils. Environ. Res. 152:199-206.
Nelson, W.B., N.T. Basta, R. Chaney, P.F.P. Henry, T. May, D. Mosby, B.A. Rattner, K.G. Scheckel, D. Sprague. 2016. Bioaccessibility tests accurately estimate bioavailability of lead to quail. Environ. Toxicol. Chem. 35:2311-2319.
Nicholson, F., N., A. Bhogal, M. Taylor, S. McGrath, and P. Withers. 2018. Long-term effects of biosolids on soil quality and fertility. Soil Science. 183:89-98.
Nicolás, C., J. N. Kennedy, T. Hernández, C. García, and J. Six. 2014. Soil aggregation in a semiarid soil amended with composted and non-composted sewage sludge—a field experiment. Geoderma. 219-220:24–31.
Niedzwiecki, A., N. Kates, S. Brown and K. McIvor. 2022. Quantifying use in a community garden program with extensive resource provision to gardeners. Urban Ag. Regional Food Sys. DOI: 10.1002/uar2.20032
Novak, J.M., J.A. Ippolito, R.D. Lentz, K.A. Spokas, C.H. Bolster, K. Sistani, K.M. Trippe, M.G. Johnson. 2016. Soil health, crop productivity, microbial transport, and mine spoil response to biochars. Bioenerg. Res. 9:454-464.
Novotny, M., M. Markovic, J. Racek, M. Sipka, T. Chorazy, I. Tosic, and P. Hlavinek. 2023. The use of biochar made from biomass and biosolids as a substrate for green infrastructure: A review. Sustainable Chemistry and Pharmacy. 32:100999.
Obrycki, J.F., N.T. Basta, K. Scheckel, A. Juhasz, B.N. Stevens, K.K. Minca. 2016. Phosphorus amendment efficacy on soil Pb depends upon bioaccessible method conditions. J. Environ. Qual. Special Issue: Soil in the City. 45(1): 37-44.
Obrycki, J.F., N.T. Basta, R.S. Wilson. 2017b. Evaluating public and regulatory acceptance for urban soil management approaches. J. Environ. Qual. 46: 20-26.
Obrycki, John F., Nicholas T. Basta, Steven W. Culman. 2017a. Management Options for Contaminated Urban Soils to Reduce Public Exposure and Maintain Soil Health. J. Environ. Qual. 46:420-430.
Ojeda, G., J. M. Alcañiz, and Y. Le Bissonnais. 2008. Differences in aggregate stability due to various sewage sludge treatments on a Mediterranean calcareous soil. Agriculture, Ecosystems & Environment. 125:48–56.
Orndorff, Z., W. Daniels, C. Zipper, M. Eick, M. Beck. 2015. A column evaluation of Appalachian coal mine spoils’ temporal leaching behavior. Environ. Pollut. 204:39-47.
Paz-Ferreiro, J., A. Nieto, A. Mendez, M.P.J. Askeland, and G. Gasco. 2018. Biochar from biosolids pyrolysis: A review. International Journal of Environmental Research and Public Health. 15:956.
Ruby, M.V., R. Schoof, W. Brattin, M. Goldade, G. Post, M. Harnois, D.E. Mosby, S.W. Casteel, W. Berti, M. Carpenter, D. Edwards, D. Cragin, and W. Chappell. 1999. Advances in evaluating the oral bioavailability of inorganics in soil for use in human health risk assessment. Environ. Sci. Technol. 33(21): 3697-3705.
Rodriguez, R.R., N.T. Basta, S.W. Casteel, F.P. Armstrong, D.C. Ward. 2003. Chemical extraction methods to assess bioavailable As in contaminated soil and solid media. J. Environ. Qual. 32:876-884.
Scheckel, K.G., R.L. Chaney, N.T. Basta, and J.A. Ryan. 2009. Advances in assessing bioavailability of metal(loid)s in contaminated soils. Adv. Agron. 104:1–52.
Schlosser, C.A., K.M. Strzepek, X. Gao, A. Gueneau, C. Fant, S. Paltsev, B. Rasheed, T. Smith-Greico, E. Blanc, H.D. Jacoby, J.M. Reilly. Joint Program Report Series. Environmental Science & Technology, 2014, pp. 30.
Schnell, R.W., D.M. Vietor, C.L. Munster, R.H. White, T.L. Provin. 2010. Effect of turfgrass establishment practices and composted biosolids on water quality. J. Environ. Qual. 39:697-705.
Sepulvado, J. G., Blaine, A. C., Hundal, L. S., and Higgins, C. P. 2011. Occurrence and fate of perfluorochemicals in soil following the land application of municipal biosolids. Environmental science & technology, 45(19):8106-8112
Sepulvado, J. G.; Blaine, A. C.; Hundal, L. S.; Higgins, C. P. 2011. Occurrence and Fate of Perfluorinated Compounds in Soil Following Land Application of Biosolids. Environ. Sci. Technol. 45:8106–8112.
Shanmugam, S., S.N. Jenkins, B.S. Mickan, N.M. Jaafar, F. Mathes, Z.M. Solaiman, and L.K. Abbott. 2021. Co-application of a biosolids product and biochar to two coarse-textured pasture soils influenced microbial N cycling genes and potential for N leaching. Scientific Reports. 1:955.
Silveira, M.L., G.A. O’Connor, J. Vendramini. 2017. Utilization of biosolids in forage production systems in Florida. SL444 (EDIS # SS658).
Song, W. L., Ding, Y. J., Chiou, C. T., and Li, H. (2010). Selected Veterinary Pharmaceuticals in Agricultural Water and Soil from Land Application of Animal Manure. Journal of Environmental Quality 39, 1211-1217.
Spokas, K.A, R. Weis, G. Feyereisen, D.W. Watts, J.M. Novak, T.J. Lee, J.A. Ippolito. 2017. Biomass or biochar – Which is better at improving soil hydraulic properties? Acta Horticulturae. 1146.31:235-242.
SSSA. (1997). Glossary of soil science terms. Soil Science Society of America.
Stahl, T., R.A. Riebe, S. Falk, K. Failing, H. Brunn. 2013. Long-Term Lysimeter Experiment To Investigate the Leaching of Perfluoroalkyl Substances (PFASs) and the Carry-over from Soil to Plants: Results of a Pilot Study. J. Agric. Food Chem. 61:1784-1793.
Sullivan, T.S., M.E. Stromberger, M.W. Paschke, J.A. Ippolito. 2005. Long-term impacts of infrequent biosolids application on chemical and microbial properties of a semi-arid rangeland soil. Biol. Fert. Soils. 42:258-266.
Trippe, K.M., V.A. Manning, C.A. Reardon, A.M. Klein, C. Weidman, T.F. Ducey, J.M. Novak, D.W. Watts., H. Rushmiller, K.A. Spokas, J.A. Ippolito, and M.G. Johnson. 2021. Phytostabilization of acidic mine tailings with biochar, biosolids, lime, and locally-sourced inoculum: Do amendment mixtures influence plant growth, tailing chemistry, and microbial composition? Applied Soil Ecology. 165:103962.
Trlica, A. and S. Brown. 2013. Greenhouse gas emissions and the interrelation of urban and forest sectors in reclaiming one hectare of land in the Pacific Northwest. Environ. Sci. Technol., 2013, 47 (13), pp 7250–7259
Una, T., J. Hernandez, A. Beebe, and S. Brown. 2022. How does your garden grow? Impact of residuals-based amendments on urban soil health, vegetable yield and nutritional density. Urban Forestry Urban Greening 77:127742
Urra, J., I. Alkorta, I. Mijangos, L. Epelde, and C. Garbisu. 2019. Application of sewage sludge to agricultural soil increases the abundance of antibiotic resistance genes without altering the composition of prokaryotic communities. Science of the Total Environment. 647:1410-1420.
US EPA. 1998. Fate, Transport and Transformation Test Guidelines: Sediment and Soil Adsorption/Desorption Isotherm. EPA 712–C–98–048.
US EPA. 2007a. Method 1694: Pharmaceuticals and Personal Care Products in Water, Soil, Sediment, and Biosolids by HPLC/MS/MS. U.S. Environmental Protection Agency, Office of Water, Office of Science and Technology Engineering and Analysis Division (4303T), Washington, DC. EPA-821-R-08-002.
US EPA. 2007b. Method 1614: Brominated Diphenyl Ethers in Water Soil, Sediment and Tissue by HRGC/HRMS. U.S. Environmental Protection Agency, Office of Water, Office of Science and Technology Engineering and Analysis Division (4303T), Washington, DC. EPA-821-R-07-005.
US EPA. 2008a. Fate, Transport and Transformation Test Guidelines: OPPTS 835.0001 Principles and Strategies Related to Biodegradation Testing of Organic Chemicals under the Toxic Substances Control Act (TSCA).
US EPA. 2008b. Fate, Transport and Transformation Test Guidelines: OPPTS 835.1230 Adsorption/Desorption (Batch Equilibrium).
US EPA. 2014. Risk assessment of spent foundry sands in soil-related applications. Available at: https://www.epa.gov/sites/default/files/2016-03/documents/risk_assessment_sfs_in_soil.pdf (verified 1/1/24).
US EPA. 2018. National overview: Facts and figures on materials, wastes and recycling. Available at: https://www.epa.gov/facts-and-figures-about-materials-waste-and-recycling/national-overview-facts-and-figures-materials (verified December 14, 2018).
USDA-NRCS. 2023. Soil health. Available at: https://www.nrcs.usda.gov/conservation-basics/natural-resource-concerns/soils/soil-health.
Wallace, B.M., M. Krzic, T. A. Forge, K. Broersma, and R. F. Newman. 2009. Biosolids increase soil aggregation and protection of soil carbon five years after application on a crested wheatgrass pasture. Journal of Environmental Quality. 38:291–298.
Wang, C., Ding, Y., Teppen, B. J., Boyd, S. A., Song, C., and Li, H. (2009). Role of interlayer hydration in lincomycin sorption by smectite clays. Environ. Sci. Technol. 43, 6171-6176.
Wang, W., Rhodes, G., Ge, J., Yu, X., and Li, H. (2021). Uptake and accumulation of per- and polyfluoroalkyl substances in plants. Chemosphere 261, 127584.
Wang, W. F., Rhodes, G., Zhang, W., Yu, X. Y., Teppen, B. J., and Li, H. (2022). Implication of cation-bridging interaction contribution to sorption of perfluoroalkyl carboxylic acids by soils. Chemosphere 290, 133224.
Whitacre, S.D., N.T. Basta, B.N. Stevens, V. Hanley, R.H. Anderson, K.G. Scheckel. 2017. Modification of an Existing In vitro Method to Predict Relative Bioavailable Arsenic in Soils. Chemosphere 180:545-552.
White, R.E., S.I. Torri, R.S. Correa. 2011. Biosolids soil application: Agronomic and environmental implications. Appl. Environ. Soil Sci. http://dx.doi.org/10.1155/2011/928973.
Wijesekara, H., N.S. Bolan, M. Vithanage, Y.Xu, S. Mandal, S.L. Brown, G.M. Hettiarachchi, G.M. Pierzynski, L. Huang, Y. S. Ok, M.B. Kirkham, C. Saint. A. Surapaneni. 2016. Utilization of biowaste for mine spoil rehabilitation. Adv. Agron. 138:97-173.
Wu, X., Conkle, J. L., Ernst, F., and Gan, J. (2014). Treated Wastewater Irrigation: Uptake of Pharmaceutical and Personal Care Products by Common Vegetables under Field Conditions. Environmental Science & Technology 48, 11286-11293.
Wu, X., Ernst, F., Conkle, J. L., and Gan, J. (2013). Comparative uptake and translocation of pharmaceutical and personal care products (PPCPs) by common vegetables. Environment International 60, 15-22.
Xia, K., L.S. Hundal, K. Kumar, K. Armbrust, A.E. Cox, T.C. Granato. 2010. Triclocarban, triclosan, polybrominated diphenyl ethers, and 4-nonylphenol in biosolids and in soil receiving 33-year biosolids application. Environ. Toxic. Chem. 29:597-605.
Xu, H., X. Qu, H. Li, C. Gu, D. Zhu. 2014. Sorption of Tetracycline to Varying-Sized Montmorillonite Fractions. J. Environ. Qual. 43:2079-2085.
Yarwood, S., A. Wick, M. Williams, W. Daniels. 2015. Parent material and vegetation influence early soil microbial community establishment following 30-years of rock weathering. Microbial. Ecol. 69:383-94.
Zhang, Y., Boyd, S. A., Teppen, B. J., Tiedje, J. M., Zhang, W., Zhu, D., and Li, H. (2018). Bioavailability of tetracycline to antibiotic resistant Escherichia coli in water-clay systems. Environmental Pollution 243, 1078-1086.
Zhang, Y. J., Boyd, S. A., Teppen, B. J., Tiedje, J. M., and Li, H. (2014a). Organic Acids Enhance Bioavailability of Tetracycline in Water to Escherichia coli for Uptake and Expression of Antibiotic Resistance. Water Research 65, 98-106.
Zhang, Y. J., Boyd, S. A., Teppen, B. J., Tiedje, J. M., and Li, H. (2014b). Role of Tetracycline Speciation in the Bioavailability to Escherichia coli for Uptake and Expression of Antibiotic Resistance. Environmental Science & Technology 48, 4893-4900.
Zohar, I., Litaor, M.I., J.A. Ippolito, M. Massey. 2018. Phosphorus sorption characteristics in aluminum-based water treatment residuals reacted with dairy wastewater, 1: Isotherms, XRD and SEM-EDS analysis. J. Environ. Qual. 47:538-545.
Zohar, I., N.B. Rose, J.A. Ippolito, I. Litaor. 2020. Phosphorus pools in Al and Fe-based water treatment residuals (WTRs) following mixing with agro-wastewater - a sequential extraction study. Environ. Technol. Innovation. https://doi.org/10.1016/j.eti.2020.100654.