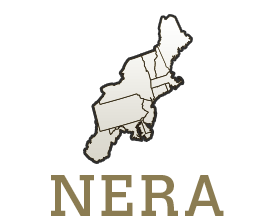
NE2334: Genetic Bases for Resistance and Immunity to Avian Diseases
(Multistate Research Project)
Status: Active
NE2334: Genetic Bases for Resistance and Immunity to Avian Diseases
Duration: 10/01/2023 to 09/30/2028
Administrative Advisor(s):
NIFA Reps:
Non-Technical Summary
Statement of Issues and Justification
The Ongoing Need for this Work, as indicated by stakeholders
Poultry consumption has increased worldwide at a steady rate since 1960. Estimates for American per capita consumption of poultry are 113 pounds for 2022 with an expected increase to 114.5 pounds in 2023 (National Chicken Council, 2022). In addition, per capita poultry (chickens, turkeys) consumption in the US equals the combined per capita consumption of beef and pork. United Nations (UN) forecasters project the 2050 world population to be 9.7 billion people, increased from the 2022 population of 8.0 billion people [United Nations, 2022]. Producing protein to feed this number of people will require economical systems that have environmental sustainability. Poultry fit both criteria because of high feed efficiency. In order to meet this ever-increasing food demand for the growing human population, poultry breeders, scientists and producers seek to provide consumers with a safe, wholesome product with higher production efficiency, lower production cost combined with high welfare standards.
Disease remains a significant issue for the poultry industry. Morbidity, poor performance, and mortality cause significant economic losses combined with the human health threat attributable to bacterial and viral zoonotic pathogens. The impact of diseases is one major impediment for sustained productivity despite advances in poultry pathogen control. Economic impacts of disease in the poultry sector can be divided into production and prevention. Poultry production impact includes losses due to mortality, decreased meat and egg production, and condemnations at processing, Prevention impact encompasses the increased costs in vaccinations, biosecurity, and eradication programs for exotic diseases. Maintaining sustainable production by understanding and optimizing immune function has reached critical importance due to increased focus on antibiotic free (ABF) or organic production as well as the re-emergence of previously controlled pathogens.
The project goal of improving poultry health will reduce the need for antibiotics and other drugs which aligns with the producers’ goal of judicious antibiotic use to produce poultry meat and eggs. Animal production, the environment, and poultry wellbeing are challenged by the use of pharmacological agents (e.g., antibiotics) to treat disease. For organic production, the project aligns with the goal of healthier animals, reducing disease incidence and ameliorating welfare issues.
Meat and egg producers place high priority on protecting poultry flocks against endemic and exotic diseases. Two U.S. outbreaks of highly pathogenic avian influenza (HPAI) in 2015 and the current one in 2021-2022 reminds us of system vulnerabilities. Avian influenza has been detected in commercial and backyard chickens across 39/50 states during the current outbreak. A major outbreak is expected for the 2022-2023 season due to the amount of virus in wild birds. In addition, the risk for exotic disease introduction is also elevated by consumer preferences driving commercial production systems to be more open (free range and cage free). The U.S. Poultry and Egg Association (USPEA) defines multiple research priorities for controlling disease and ensuring food safety in poultry. These priorities seek to reduce antibiotic, pesticide and anti-parasitic drug use through a focus on disease prevention, genetics, welfare, nutrition and hatchery management.
This project addresses the genetic bases of disease resistance and immunity in poultry. The work also seeks mechanistic understanding of innate and adaptive immune processes; issues having fundamental importance. Poultry breeding, vaccine, and allied animal health industries are the most immediate users of these data, reagents, and tools generated from project research. These primary stakeholders are frequent participants through attendance at annual technical committee meetings as well as their many collaborative research efforts with the members. Interaction as described signifies the high value that stakeholders ascribe to this project.
The importance of the work
A sustainable poultry industry which can increase production to feed a growing world population needs disease prevention and control strategies. Natural selection produces genetic variability in populations. This multistate project fills this crucial need by enhancing stakeholders’ knowledge of the genetics of resistance and immunity to poultry diseases. Stakeholders can improve their stocks by applying this knowledge of genetic variability and disease resistance to augment desired traits while eliminating unwanted characters. Genetic responses to disease may be controlled by one or a few genes. The major histocompatibility complex (MHC) is the primary example. Responses may be more complex because many genes contribute to the overall response. Low heritability, a consequence of multigene control makes improvement through traditional selection.
Knowledge gaps about polymorphic loci and their interactions persist, despite completion of multiple chicken genome sequences. This project effort will facilitate understanding of immune responses to common poultry pathogens. The knowledge gained will enhance development of targeted responses providing protection.
Both large-scale and small-scale poultry production face multiple limiting factors. Disease impacts in both systems have been exacerbated by efforts to reduce antibiotic use plus managing birds in less-controlled environments. Production has been disrupted by recurrence of diseases that once seemed to be controlled along with emerging pathogens. Without this project’s work to study the important issues of environmental and physiologic factors that impact immune system development, optimal immune function and disease resistance, disease will increase, production efficiency will decrease, food safety will be greatly compromised, and export markets will be closed to products.
Unlike model species in biomedical research, few poultry research reagents are available through commercial sources. In addition, genetic resources, such as stocks that enable experiments to answer critical questions, are limited. The multistate objective focuses on resource development (methods, reagents, specialized genetic stocks) that will facilitate immune system assessment. Morbidity and mortality could increase if immune development is not monitored to prevent deterioration of immune responses to vaccines and disease organisms. Poultry breeding companies will have direct application for methods to address genetic and environmental factors that impact the immune system. Production companies will realize lower production costs benefit through improved health and effective responses to vaccination.
The Technical Feasibility of This Project
Technical committee members for NE-1834 cross multiple disciplines including genetics, genomics, immunology, infectious diseases, microbiology, virology, poultry medicine, physiology, kinomics, nutrition, biochemistry, and molecular biology. The committee’s expertise enables individual and collaborative research which is essential for the future of poultry production. Methods that have demonstrated success in other species, i.e. humans, have been adapted to poultry work. Development of other sophisticated methods have enabled examination of gene expression and interaction that may affect disease resistance. Defined genetic stocks can be studied with techniques such as next generation sequencing and gene editing to understand responses against disease. Project participants are leaders in their respective specialties. The work is technically feasible, and the necessary infrastructure exists for members’ success in completing the work. A final necessary component is adequate financial support for the proposed effort.
Advantages for doing the work as a multistate effort (The Essential Collaborative Nature of this Project)
Project members conduct studies on genetic resistance to disease across multiple disciplines. Operating through the multistate system offers the advantage of shared resources that synergize to address critical scientific questions. No one station or participating laboratory has the range of expertise, facilities, equipment and biological resources to investigate the crucial scientific questions for the project. The effort is enhanced by the unique skills of the project members. Some stations have specialized genetic stocks or reagents (antibodies, cell lines, pathogen stocks), that are shared with the scientific community. Genetic stocks (inbred lines, congenic lines, selected lines) have facilitated many experiments that have answered key questions on disease resistance and the immune response. The value of these resources cannot be overstated. The regular interaction through the multistate committee enhances research opportunities for each participant. Networking has brought about multiple collaborations.
The current NE-1834 group of scientists each addressing complementary aspects of the genetics of resistance and immunity to avian diseases includes 23 independent laboratories representing 13 institutions across 12 U.S. states [AL, AR, CA (2), DE (3), GA (2), IL, IA, MA, MD (2), NC, OH (2), WU, and WV (2)]. Two USDA scientists (ADOL, FFSRU) and one international researcher in the Netherlands (NL) collaborate on the project. Conducting this work as a multistate effort allows for the greatest efficiency of resource use among these scientists.
Participating stations and scientists have authored 165 refereed publications and 203 abstracts, a very productive effort for the project period. Forty-three publications have joint-authorship illustrating the truly essential, cooperative, multidisciplinary nature among the NE-1834 project members. Technical committee members and collaborators from NE-1834 contributed to reference works such as Avian Immunology (Kaspers, et al, 2022) co-edited by a project member plus authorship in 7 of the 22 chapters and one of the two appendices.
In addition, five project members hold endowed faculty positions indicting the prestigious nature of their research. The Poultry Science Association (PSA) and the American Association of Avian Pathologists, scientific organizations tied most closely to the project, have awarded their highest honors to project scientists. Five project members are Fellows of the Poultry Science Association. Other awards presented to project members include the Bayer Snoeyenbos New Investigator Award. Embrex Fundamental Science Award, Evonik Degussa Award for Achievement in Poultry Science, Hy-Line International Poultry Science Research Award, Novus International, Inc. Teaching Award, PSA Early Achievement Award for Research, and two US Poultry Distinguished Poultry Industry career awards.
Synergies exist with other multistate projects. The NE-1834 project members cooperate with relevant multistate projects including NC1170: Advanced Technologies for the Genetic Improvement of Poultry and NC1180: Control of Endemic, Emerging and Re-emerging Poultry Respiratory Diseases in the United States. Multiple NE-1834 technical committee members also participate in either NC1170, NC1180 or both. One participant has been active in the NE-1834 and NC1170 technical committees for more than 34 years. Excellent communication and coordination are the primary benefits of their active participation in both projects. Duplication across projects is avoided thereby enhancing efficient resource use.
Outcomes and Impacts of this Project
Greater fundamental understanding of how the avian immune system functions, improved comprehension of immune responses to specific antigens, higher efficacy of pharmaceutical agents including vaccines; advanced vaccine programs for controlling existing as well as emerging diseases; increased knowledge of genetic selection consequences on poultry health and production; insight into polymorphic loci effects; and breeding strategies that enhance disease resistance and robustness in poultry are among the impacts expected for this project. Production efficiency, animal health and welfare will increase through improved disease resistance and better prevention strategies. These advances will produce immediate as well as long-term benefit. Consumers will favor improved poultry product safety and reduced antibiotic use. Valuable resources for stakeholders and project participants will be generated as scientific knowledge, both basic and translational, combined with new or modified tools and reagents.
References:
Avian Immunology, 3rd Ed. 2022. B. Kaspers, K. Schat, T. Göbel, and L. Vervelde, eds. 692 pages. Elsevier, Amsterdam. ISBN: 9780128187081; https://doi.org/10.1016/C2018-0-00454-5
National Chicken Council. 2022. Per-capita-consumption-of-poultry-and-livestock. https://www.nationalchickencouncil.org/about-the-industry/statistics/per-capita-consumption-of-poultry-and-livestock-1965-to-estimated-2012-in-pounds/
United Nations Department of Economic and Social Affairs, Population Division (2022). World Population Prospects 2022: Summary of Results. UN DESA/POP/2022/TR/NO. 3.
Related, Current and Previous Work
Objective 1. To identify and characterize genes and their relationship to disease resistance in poultry with emphasis on the major histocompatibility complex as well as other genes encoding alloantigens, communication molecules, and their receptors and other candidate systems.
CA – Indigenous ecotypes in Africa were challenged with Newcastle disease virus and genome-wide association studies were used to identify genetic markers associated with increased immunity, reduced viral load, and enhanced survival time.
IA – At Iowa State University, the splenic transcriptome of birds infected with avian pathogenic E. coli (APEC) revealed innate immune pathways associated with response to APEC. Differentially expressed genes were identified in the splenic transcriptome of birds after infection with Newcastle disease virus (NDV) at IA. Targeted gene expression assays and gene knock-down studies at IA demonstrated the involvement of the eukaryotic translation initiation factor (eIF2) gene family and the oligoadenylate synthase-like (OAS) gene in response to NDV.
IL – Illinois studies host genetics in horizontal transmission of Marek’s disease virus (MDV). Preliminary studies in chicken lines at UI showed significant differences in disease incidence and transmission between three chicken lines. Our work has focused on identifying cellular proteins that interact with MDV proteins and the allelic variations in these host factors.
MD – Maryland explored some statistical methods in epigenomic and systems biology in poultry. We inoculated inbred lines of White Leghorn chickens with infectious bursal disease virus (IBDV) and evaluated clinical signs, gross and histopathology, viral replication, and gene expression in the bursa of Fabricius (BF) by RNASeq. We determined that birds with more severe disease had more inflammation that correlated with an increased number of macrophages/monocytes in the BF.
WV – DNA sequences and SNP analyses of samples from chickens with defined alloantigen genotypes to identify candidate genes for chicken alloantigens. Linked alloantigens A and E on chromosome 26 were C4BPM (complement component 4 binding protein membrane) and FCAMR, Fc fragment of IgA and IgM receptor, respectively. Alloantigen D, found on chromosome 1, was revealed to be CD99, part of the Xg blood system. Chromosome 23 contained RHCE (human: Rh blood group CcEe antigens), the candidate gene for alloantigen I. Two independent analyses showed a chromosome 4 region associated with alloantigen L. A gene has yet to be identified in this area. Allele frequencies for alloantigens A, E, B, and D differed significantly between Wageningen University and Virginia Tech high antibody (HA, HAS) lines versus their corresponding low antibody (LA, LAS) lines. The distribution for alloantigen I differed between the Virginia Tech HAS vs LAS lines but not the Wageningen lines.
WU – Western University investigated genes involved in differential disease resistance of B2/B2 and B19/B19 MHC haplotypes. RNA sequencing has shown that genes are largely dysregulated in B19/B19 during differentiation and after stimulation, leading to decreased immune function. T-cells did not have as much influence as macrophages. Focusing on specific genes such as Cluster Homolog of Immunoglobulin-like Receptors (CHIR), we completed phylogenetic analysis and re-annotation of CHIR, which was submitted to NCBI. CHIR-B silencing affects haplotypes differentially. Single-cell sequencing of the reproductive tract show differences in cellular populations and CHIRs in B2 and B19 haplotypes.
Objective 2 "To Identify Factors and Agents Affecting Poultry Immune Development, Function, Dysfunction and Pathology"
The group has made significant accomplishments in characterization of innate and adaptive immune responses, factors that influence these activities, and mechanisms of dysfunction and pathology. Research approaches included use of genetic and divergently selected lines, whole animal experiments, novel cellular and molecular analyses, including analyses at the transcriptome, proteome, kinome, and metabolome level.
Using genetic lines that spontaneously develop multifactorial, non-communicable diseases, AR further defined the cellular and molecular mechanisms involved in the immunopathology associated with development of vitiligo in Smyth chickens, demonstrated autoimmune memory in Smyth line vitiligo, and identified suppressive activities in tolerance maintenance in vitiligo-susceptible, pigmented parental Brown line controls. Additionally, AR demonstrated aberrant innate responses in scleroderma-prone UCD200/206 chickens, especially heightened TGF-β, IL-17 and IFN-γ responses following intradermal injection of killed Mycobacterium butyricum. In vivo studies by AR on the inflammatory response to various microbial components revealed different leukocyte recruitment profiles to products of Gram-negative versus Gram-positive bacteria, reduced local and systemic inflammatory responses with heat-stress, and heterophil dominated responses to autogenous Salmonella bacterin vaccines in pullets.
NC increased the characterization of the adaptive immune functions and the influence of genetic and environmental factors contributing to these processes by studying High Antibody Selected (HAS) and Low Antibody Selected (LAS) White Leghorn lines that continuously have been divergently selected for 5-day post-injection antibody titer to injection with sheep red blood cells (SRBC) for nearly 50 years. Differential gene expression was analyzed combining traditional statistics and machine learning to obtain signature gene lists for functional analysis, which revealed differences in energy production and cellular processes between lines and with SRBC injection.
DE compared immune responses of ACRB and modern broilers. This work revealed that the modern broilers displayed greater inflammatory response to CpG administration and showed continuous response to Cocci vaccination, while ACRB show increased inflammation and apoptosis to challenge.
Through detailed examination of blood cell films from various poultry species and environmental/health conditions, MA demonstrated heterogeneity among plasmacyte (PC) series, cells known for antibody secretion. The capacity to recognize differences among primitive PC and derived types is an important step in understanding the complexities of immune reactions and factors influencing these activities. Atypical plasmacytes include Mott cells recognized by the presence of retained as unsecreted antibodies (Russell bodies) and Dutcher bodies (nuclear vacuoles). Recognition of Türk cells as members of the avian proplasmacyte cell series adds to basic immune function. These developmental cells are typically large with deep basophilic cytoplasm and sometimes a nucleolus. Many retain a capacity for division. They are not widely known in the veterinary immunity or pathology community but are found in chickens, ducks, and turkeys either as components of the circulation or in the bone marrow. MA also continued the study of reactive thrombocytes and their relation to stress.
DE studied the immunometabolic response in the broiler chicken to pathogens and immune modulatory agents. Their results showed that postbiotic fermentates are anti-inflammatory and improve outcomes to a necrotic enteritis challenge. DE also demonstrated that administration of essential oils and antioxidants resulted in reduction in inflammatory state beneficial for growth during early stress by activating pathways via FOXO, and that butyrate displays pro-inflammation activities in the context of Salmonella infection, which may be the mechanism butyrate aids in clearance. Furthermore, Salmonella studies by DE showed that calmodulin signaling is an important pathway of response, whereby signaling is through TLR, NOD, cytokine and chemokine PRRs and Jak-STAT pathways. Additionally, DE observed a greater inflammatory response in chickens with early post-hatch Salmonella inoculation.
NC expanded our understanding of how diet, environment, and the microbiome influence the host’s immune response and how this impacts the colonization of pathogens, like Salmonella. NC’s work demonstrated that the microbiome regulates the phenotype of Salmonella and the immune system, and the microbiome is controlled through diet and environmental conditions.
CA found perturbation in metabolic pathways related to arginine and proline metabolism associated with Salmonella infection, and that the TCA cycle contributed to Salmonella persistence in chickens. Furthermore, chickens from a knockout line (KO) lacking B lymphocytes were examined by CA for their resistance to a challenge with Salmonella enterica. When challenged, the KO chickens lost less body weight than the controls, had similar mortality, but higher indices of intestinal inflammation.
VA/GA investigated necrotic enteritis (NE) which continues to present critical challenges to the poultry industry. They revealed key microbiome signatures associated with an attenuated immune response and a modification of tight junction proteins, which would promote better gut integrity. VA/GA explored of the host response during NE identified new markers of disease progression, including inflammasome and autophagy markers that were differentially expressed in NE birds at 3 weeks of age (time of NE outbreak in commercial birds). VA/GA also found signature host responses to two NE-inducing isolates of Clostridium perfringens, which is aiding in elucidating their pathogenicity.
OH showed age-related effects on the role of pioneer colonization of the GI-tract in neonatal birds, especially with regards to immune tolerance and innate immune function. Generally, Gram-negative bacteria decreased ability of birds to respond to inflammatory events and lactic acid bacteria promoted colonization with segmented filamentous bacteria, thought to support beneficial innate immune function. OH demonstrated that early inoculation with Gram-negative bacteria increased gut permeability and susceptibility to necrotic enteritis. Gram-negative inoculation promoted dendritic cell migration to gut tissue, decreased HNF1-alpha, decrease pathways associated with D-glucose, and Fcγ receptor dependent phagocytosis. Conversely, lactic acid bacteria promoted gluconeogenesis, B cell receptor signaling, Class I MHC antigen processing and IL-1, while downregulating heterophil degranulation and MHC Class II antigen presentation. MD evaluated the structure and movement of the intracellular replication complexes of infectious bursal disease virus (IBDV) and how they form.
DE examined the molecular pathogenesis of Marek’s disease virus (MDV), resulted in obtaining and analyzing transcriptomes and proteomes of exosomes purified from the serum of vaccinated and protected chickens and tumor-bearing chickens. DE also patterned macrophage cell line HD11 to become mature macrophages, dendritic cells and polarized dendritic cells. DE also found that key drivers of the MDV evolution of virulence are at the level of innate immune selection of the oncoprotein Meq and mutations that affect specific Meq-binding partners.
Research conducted by IL on identification of cellular proteins important for horizontal transmission of MDV, included cloning of the putative chicken complement receptors (CR) 1 (CR1), CR2, and CR1-like (CR1L) from established chicken cell lines and two chicken lines. Variations of protein coding sequences were identified.
The emergence of antibiotic resistant pathogens from food animal production has restricted the application of antimicrobial compounds in the poultry industry. Removing antimicrobial compound application has increased the incidences of poultry gut pathogen infections, namely coccidiosis, necrotic enteritis, salmonellosis and campylobacteriosis. GA has identified that probiotics stimulate the immune responses, competitively exclude pathogenic microbes, stimulate digestive enzymes, and produce antibacterial substances. We have synthesized and characterized Salmonella and Clostridium perfringens nanoparticles for cytotoxicity, morphology, protein loading efficiency and pH stability. The synthesized vaccines successfully decreased the enteric pathogen loads in poultry intestine.
Objective 3 To develop and employ genetic stocks, methods, reagents and other tools to assess basic immune function, characterize immune evolutionary processes, guide genetic selection, and increase resistance to or protection against avian diseases
To increase disease resistance and protect poultry from disease, our working hypothesis is that the ideal model system and powerful tools can help facilitate excellent scientific research to enhance poultry health and welfare. The avian immune community has put tremendous efforts into developing and employing new genetic stocks, methods, reagents, and advanced tools to assess basic immune function, characterizing immune evolutionary processes, guiding genetic selection, and providing valued information for continued research on the genetic bases of resistance and immunity to avian health and welfare. Multiple stations (AR, CA, GA, IL, IA, OH, MD, NC, WU, WVU) have developed, maintained, characterized, and made available unique genetic resources to the NE-1834 project members and the entirety of the poultry research community.
IA maintained, characterized, and shared eight unique genetic lines of chickens and their biological products (fertile eggs, chicks, DNA, RNA, tissues, blood, etc.) for research on genetics and genomics of immune response and disease resistance. They studied the expression levels over time of host-defense peptide (HDP) genes in fibroblasts and bone-marrow derived cells from Fayoumi (resistant) and Leghorn (susceptible) lines, after exposure to immune stimulants, and demonstrated innate and post-exposure differences in HDP expression between the chicken lines and demonstrated differences between the ISU chicken genetic lines in MHC class I or beta2 microglobulin cell-surface proteins. Their research will determine the genetics associated with response to infections with APEC and NDV.
Histomoniasis (blackhead disease) is a perennial problem in the poultry industry, particularly in turkey poults, where it inflicts substantial losses but is also problematic in broiler breeders. GA recently developed a lateral transmission model of the parasite Histomonas meleagridis in poults raised in floor pens. This research model closely resembles commercial field conditions and affords a much-needed platform for conducting detailed research on histomoniasis. The newly established lateral transmission model in floor pens is a key system to study this disease, its progression, and potential mitigation strategies. In mechanisms, GA conducted a study to identify the effects of Salmonella enterica ser. Enteritidis and Heidelberg on host CD4+CD25+ regulatory T cell suppress immune responses in chickens. Besides, GA also recognized if a Salmonella chitosan nanoparticle vaccine administration is protective against S. Enteritidis in broiler birds. Chitosan nanoparticle-vaccinated birds had 0.9 Log10 CFU/g decreased SE cecal loads compared to the control (P<0.05). The vaccine under study did not adversely affect the bird’s BWG and FCR or the IL-1β, IL-10, IFN-γ, or iNOS mRNA expression levels. They have found that the CNP vaccine, either as a first dose or as a booster vaccination, is an alternative vaccine against Salmonella in poultry.
In genetics and epigenetic marks, MD characterized copy number variations (CNV) and epigenome modifications in the host genome in the resistance to Marek’s disease. Most importantly, MD also developed a novel reverse genetics rescue system and a novel neutralization assay using only chicken B cells to rescue molecular clones of IBDV and quantify antibody responses against them. They then used these methods to engineer a panel of viruses containing hypervariable regions (HVRs) belonging to diverse genogroups and screened serum from vaccinated birds to evaluate the breadth of antibody responses. Finally, the group at IL examined complement and complement receptors in chickens. They have developed reagents monoclonal antibodies (mAbs) against chicken CR1L and have cloned chicken CR1, CR2, C3, and C4 to produce mAbs against these proteins, which will provide tools to address resistance to MDV infection.
DE has cloned for expression chicken innate immune genes, as well as other cellular gene products that interact with MDV proteins. The work focused on forms of the Meq oncoprotein of MDV (pathotypic point mutations and splice variants). The collection of cloned chicken genes in expression vectors has been made available to all participants in this project and is accessible in a shared Google folder.
Objectives
-
To determine how genetics, epigenetics and gene regulation influences innate and acquired immune functions in poultry.
-
To identify factors and agents affecting poultry immune development, function, dysfunction and pathology
-
To develop and employ genetic stocks, methods, reagents and other tools to assess basic immune function, characterize immune evolutionary processes, guide genetic selection, and increase resistance to or protection against avian diseases
Methods
NE1834 Objective 1. To determine how genetics, epigenetics and gene regulation influences innate and acquired immune functions in poultry.
CA – RNA-seq, whole-genome sequencing, ChIP-seq and ATAC-seq will be utilized by University of California-Davis to identify genetic variants affecting immunity and disease resistance to poultry pathogens in diverse genetic lines in chickens.
MD – Maryland will characterize epigenetic modifications and copy number variations (CNV) within the host genome in lines that are resistant or susceptible to Marek’s disease. Tools developed, such as monoclonal antibodies and a novel reverse genetics rescue system will be available for community use.
IL – The University of Illinois will continue exploring host genetics in horizontal transmission of Marek’s disease virus (MDV). We will identify cellular proteins important for MDV transmission and examine allelic variations within these proteins by cloning and sequencing the putative genes.
IA – Iowa State University will complete analyses and dissemination of studies on genetics associated with response to avian pathogenic E. coli and avian influenza virus. IA will collaborate with CA on studies of genomics of response of African ecotypes of chickens to Newcastle disease virus.
WV – DNA sequences from chickens with defined alloantigen genotypes will be examined to identify a chromosomal region associated with alloantigens L and C. Individual and pooled samples from matings with segregating L and C alleles will be analyzed for SNP associations with alloantigen type. Bioinformatic analyses will focus on genes that are expressed on cell surfaces where SNP in exons alter the protein product (WV). Chickens segregating for different alloantigen alleles will be tested for responses against sheep red blood cells. Alloantigen type will be tested for effect on responses against pathogens: Eimeria tenella and Marek’s disease.
WU – WU will continue genome-wide annotation of cis-regulatory elements in the chicken: Characterization of cis-regulatory elements (such as enhancers and others) by performing several genomic and epigenomic assays for chicken tissues and cells. Generation of data and bioinformatic analysis. We will investigate epigenetic regulation in coronavirus infection in different haplotypes, using RNA-sequencing and ATAC-sequencing. We will identify the role of CHIRs in infectious disease through using small interfering RNA and detecting aberrant changes in immune response in disease susceptible and resistant line (B2/B2 and B19/B19). The project will evaluate for differences in cellular activation and inflammation markers expression. We will characterize the tissue distribution of CHIRs in the reproductive tract in disease susceptible and resistant lines (B2/B2 and B19/B19) to provide the genetic biomarkers for selective breeding of chickens with high production traits and a robust immune system.
NE1834 Objective 2 To identify factors and agents affecting poultry immune development, function, dysfunction and pathology
A variety of approaches will be used to address this objective. AR will use chicken lines that spontaneously develop multifactorial, non-communicable diseases, such as scleroderma/systemic sclerosis (a fibrotic disease), autoimmune-vitiligo, and -hypothyroidism to study mechanisms of immune dysfunction, immunopathology, and chronic inflammation.
MA is working on further expanding diagnostic insights that can be gained by microscopic examination of blood cells when studying poultry immune system function and pathology. Specifically, MA is working on a three-tiered system or strategy for examining stained blood films from avian samples. Tiers are cellularity, given as the percentage of leukocytes occupying space in a microscopic field; cytology, as the sorting scheme used to differentiate leukocytes as lymphocytes, granulocytes, and others; and, behavior, the third tier, includes both intracellular and intercellular facets.
Intercellular behavior may also include leukocyte/erythrocyte interactions and interactions between blood cells and microorganisms such as phagocytosis and other cell-microbe relations. AR, DE, NC, OH, and GA will focus on studying immune system function and development in the context of nutritional, environmental, microbiome, genetic selection, and/or microbial infections. AR will use minimally invasive in vivo procedures and molecular/cellular ex vivo analyses to conduct longitudinal studies of cellular innate and adaptive immune responses in chickens reared in different nutritional and environmental conditions and/or divergently selected for genetic traits, like water efficiency.
DE will characterize at a molecular and proteomic level, chicken-disease pathogenesis and potential feed treatments and therapeutics. Recently developed techniques such as qPCR, kinome peptide arrays, Seahorse metabolic flux analysis and in vitro and in vivo trials will be employed. DE is working to further develop applications of digital PCR to poultry health, including detection of host-effects from subclinical bacterial infection in broilers. Other projects planned by DE include comparisons of gut immunometabolic responses of ACRB and modern broilers to a variety of pathogens and interventions; in vivo and in vitro infections of chickens with Salmonella with and without treatment and antibiotics to determine mechanism of action and disease pathogenesis; and, research towards refining and characterizing alternatives to antibiotics, including probiotics and eukaryotic cell fractions.
NC will use next generation sequencing, metabolomics, and data analytics to establish mechanistic links between the influences of diet, environment, and the microbiome on the host’s immune response and colonization of pathogens, like Salmonella. Additionally, research by NC will focus on determining metabolites produced by the microbiome and how they modulate immune cell activity, identify the key members of the microbiome producing these metabolites, and develop novel ways to use these mechanisms to better control immunity of production birds.
OH will investigate the molecular and cellular mechanisms of intestinal immune responses to enteric diseases (coccidiosis and necrotic enteritis) in chickens, as well as the metabolic role of intraepithelial lymphocytes in progression of wooden breast syndrome in broilers. Cell population analysis by flow cytometry will be used to identify immune cells that confer resistance to diseases, particularly in the intestinal mucosa. This will be followed RNA-seq and/or scRNA-seq on sorted immune cells that confer protection to the target diseases. OH also plans to continue studying the role of neonatal exposure to bacteria on gut function and immune development, especially how early exposure to Enterobacteriaceae affects susceptibility to opportunistic diseases like necrotic enteritis, Salmonella and avian pathogenic E. coli.
GA will expand their investigations on the impact of in ovo and in vivo delivered supplements (e.g., postbiotics) on the gut microbiota and the development of the immune response in poultry (broilers) during progression of critical diseases (e.g., coccidiosis, necrotic enteritis), and examine their impact on response and resistance to such enteric challenges. GA also will conduct research on detailed immune responses of heritage and modern breeds to better characterize their responsiveness to such challenging enteric diseases in order to better design mitigation strategies.
AL will assess how infections with coccidia and avian reoviruses (ARV) interact with each other and the immune system. Coccidia are among the most important intestinal pathogens in chickens. ARV are one of the possible causes of runting-stunting syndrome. ARV also are known to be immunosuppressive. There are indications that co-infections with coccidia and ARV act synergistically. AL seeks to explore how these two parasites interact and how better prevention against one pathogen, e.g., by improved management or vaccination strategies, can mitigate the damage done by the other pathogen. Towards this goal, AL will first establish a model for oral infections of chickens with ARV and characterize the expression of a panel of immune genes and lesions in various organs. Based on these data, ARV isolate, infection dose, time points and target genes will be selected, and birds will be co-infected with coccidia before, with and after the ARV infection to test for synergism of the pathogens.
Research towards a better understanding of immune system function and dysfunction in protection and transmission of Marek’s disease virus (MDV) and infectious bursal disease virus (IBDV) will be carried out at DE and IL, and MD, respectively. DE plans to further investigate serum exosomes as these relate to the patterning of innate immune cells (monocyte, macrophages, dendritic cells and polarized dendritic cells). In the case of vaccine-associated exosomes, DE plans to determine their effects on eliciting anti-MDV vaccine responses through the treatment of dendritic cells with exosomes and co-cultivation with MHC-matched peripheral blood mononuclear cells from vaccinated chickens. Furthermore, DE plans to examine the effects of exosome treatment on innate and acquired immune responses using kinomic, proteomic and targeted transcriptomic analysis in cell culture and on vaccine responses in vivo. IL will focus efforts on identification of cellular proteins important for horizontal transmission of MDV. They will develop reagents to test potential cellular receptors for MDV infection using protein-protein interaction studies.
MD will define the processes that occur in the intracellular IBDV replication complexes and evaluate whether these structures play a role in antagonizing antiviral immune responses, for example through the sequestration of proteins involved in innate immune pathways. MD will use the novel IBDV rescue system and neutralization assay to assess the individual contribution mutations in the HVR make to immune escape to determine which HVR mutations should be included in vaccines to ensure optimal disease control.
GA will apply commercially available nutritional supplements or nanoparticle vaccine to control coccidiosis, necrotic enteritis, Salmonella, Campylobacter infections of poultry. In vivo experiments will be conducted to study the efficacy of probiotics, synbiotics, and vaccines to decrease the loads of gut pathogens in poultry. Production performances, gut integrity indices and immune parameters will be measured. Nanoparticle vaccines will be designed to deliver antigens against intestinal pathogens can deliver vaccine payload through an oral route and will ideally elicit protective mucosal immunity against gut pathogens. Serum, cloacal swabs, and bile samples from vaccinated and unvaccinated chicks will be analyzed of anti-CP specific IgA and IgG antibodies. Splenocytes will be analyzed for recall response, and liver and cecal tonsils will be analyzed for cytokines.
NE1834 Objective 3 To develop and employ genetic stocks, methods, reagents and other tools to assess basic immune function, characterize immune evolutionary processes, guide genetic selection, and increase resistance to or protection against avian diseases
AR continues to maintain autoimmune disease models such as the Smyth (SL), UCD 200/206, and the Obese Strain (OS) lines of chicken as models for human disease as well as for multifactorial non-communicable diseases in poultry and to study immune system development and function of the avian immune system in health and disease. Interestingly, AR has updated the growing feather as an “in-vivo test tube and window into cellular/tissue responses in vivo to intradermally injected test materials. The particular focus is on the valuable techniques for refining the method and demonstrating its uses. WVU also maintains two inbred lines, four congenic lines, and four line crosses typed at the MHC. Stocks typed for other alloantigen systems are maintained for station research and collaboration.
CA holds twelve lines with different MHC types. Eight of these lines are congenic to each other on the background of inbred line UCD 003. The station also maintains inbred Red Jungle fowl Line UCD 001, the reference line for the original chicken genome sequence. Multiple lines having single gene developmental mutants are also available.
Unique genetic stocks are preserved at IA. These stocks include six lines which have been inbred between highly inbred between 80 to 110 generations while maintaining defined MHC types. In addition to these egg-type lines, other stocks are Fayoumi, Spanish and broiler type. An advanced intercross line (AIL) from the cross of a single outbred broiler male with Fayoumi females is produced annually.
WU continues functional annotation of the chicken genome on 20 tissues/cells. They have completed DNA methylome analysis for reproductive and intestinal tissues/ peripheral immune cells and transcriptome with RNA-seq for all tissues and peripheral blood cells. In the future, WU will annotate CHIR receptors in various haplotypes and employ spatial sequencing to further locate tissue and cell distribution. Meanwhile, OH uses RNA-seq to discover and characterize new immune cell populations in intestinal mucosa that confer resistance to enteric diseases in chickens, specifically in intraepithelial-lymphocyte subpopulations.
DE will continue to provide access to the cloned chicken genes collected as well as determine the relevance of specific protein-binding to Meq isoforms, in terms of cellular localization and changes in function.
The knowledge of associations of biomarkers with specific immune traits, MHC haplotypes, and segregating alloantigen alleles will allow genetic selection to enhance innate disease resistance in poultry, thus improving bird health and production. Enhance poultry health by identifying physiological and immune response markers key to improving host resistance to pathogens. This information will facilitate the development of intervention and mitigation strategies. Identifying cellular factors will allow better techniques to prevent and control infectious diseases and provide marker-assisted selection and breeding.
Measurement of Progress and Results
Outputs
- Genetic variants associated with immune response, virus infection in diverse chicken population were investigated. Peer-reviewed abstracts and papers were published. Participating regional, national and international scientific conferences, workshops, symposiums and disseminating gap knowledge.
- Identification of cellular factors important for transmission of MDV and assessment of allelic variations in these genes. This project will clone specific immune-related cellular genes important for MDV infection in chickens.
- This project will determine the genetics associated with response to infections with APEC and NDV and disseminate the results in peer-reviewed journal publications and at scientific conferences.
- Evaluate the effect of alloantigen allelic variation on response against bacterial, viral or parasite pathogens or other immune stimuli. Disseminate information to stakeholders and the public through refereed publications, symposia, invited lectures and informal discussions at regional, national and international workshops and meetings.
- Apply genetic, epigenetic and immunological approaches to define the role of individual genes on disease resistance or susceptibility in different B-haplotypes. Results will be disseminated via publications and conferences.
- New knowledge on temporal, qualitative, and quantitative aspects of innate and adaptive immune responses to microbial components and vaccine antigens in genetic lines and commercial poultry.
- Disseminate our findings to stakeholders at regional (NECAD), national (AAAP) and international (Marek’s disease Symposium) meetings, via publications, and through our undergraduate outreach program (UD Envision).
- Characterize the immunometabolic interaction of various gastrointestinal pathogens with the broiler chicken.
- Study putative treatment/therapeutic compounds against gastrointestinal pathogens and their mechanism of action in broiler chickens.
- Solutions to replace antimicrobial products in poultry production will be provided through refereed publications, symposia, lectures and informal discussions at regional, national and international meetings.
- Evaluate the role microbiome associated metabolites have on avian immune function using transcriptomics and metabolic analysis, along with in vitro and in vivo assays.
- Continue microscopic studies of the plasmacyte series by describing variant cells with comparisons to classic types that should allow for a better interpretation of complex hemograms and their relation to disease stress
- Elucidate the protective role of T-cell subpopulations in the intestinal mucosa against Eimeria infection
Outcomes or Projected Impacts
- Identification of genes that are associated with resistance to heat stress and Newcastle disease virus and can be used to genetic enhancement of disease resistance of chicken in adaption to hot climate.
- Identification of cellular factors important for transmission of MDV will allow better strategies to prevent and control MDV in poultry houses, as well as provide marker-assisted selection and breeding.
- Knowledge of associations of biomarkers with specific immune traits will allow genetic selection to enhance innate disease resistance in poultry, thus improving bird health and production.
- Identification of alloantigen genes that improve the immune response to vaccine and pathogens, facilitating breeding for improved immune response in poultry and other species.
- Characterization of genes, and their regulation, in disease susceptible and resistant lines that will provide genetic biomarkers for selective breeding of chickens with high production traits and a robust immune system.
- Assessing the impact of microbial components and vaccine antigens on the direction and quality of the immune response, for application to modulate and optimize immune system function.
- Classifying fundamental mechanisms of virulence evolution of Marek’s disease virus via exosome studies that are essential to the understanding how these vesicles affect systemic immunity.
- Interpreting the obtained results to better determine how disease occurs, identify the most effective means of prevention or mitigation at the tissue or cellular level and to develop targeted intervention.
- Establish mechanistic links between the microbiome, its metabolites and immune function to facilitate new ways of controlling the immune response of production birds
- Identifying the mechanisms, magnitude, dose and duration of probiotics, acidifiers, and vaccine schedules to decrease incidence of important enteric or foodborne pathogens of poultry
- Designing an effective vaccine against coccidiosis using T data for -cell subpopulations and their functions during coccidia infection in chickens
- Publishing refereed papers, symposia, invited lectures and informal discussions at regional, national and international workshops and meetings to disseminate information to stakeholders and the public.
Milestones
(2023):Publication of four peer-reviewed journal papers disseminating the results of studies on genetics associated with response to pathogens. Develop tools to address MDV transmission in chickens. Identify genetic variations between chicken lines related to MDV transmission. Establish genetic lines with altered immune system function to produce experimental animals for collaborative work with multiple stations. Determine expression changes elicited by serum exosomes. Identify pathogenesis mechanisms and compounds related to disease in vivo. Identify the dose and duration of probiotics, acidifiers, and vaccine schedules decrease incidence of enteric pathogens of poultry Prepare and submit papers for publication Establish a list of 10 metabolites most likely involved in regulating immune function(2024):Detection of distinct alloantigen genotypes and their interactions that affect immune function. Completion of functional annotation of chicken genome in selected cells and tissues. Establishing immune response profiles to microbial components for phenotyping innate immune response capabilities in poultry populations. Determining how mutations in Meq increase MDV virulence. Establishing an in vitro system for evaluating identified metabolites’ ability to regulate function of individual immune cells. Measuring the dPCR response after infection. Identifying the mechanism through which probiotics, acidifiers, and vaccine schedules decrease incidence of enteric pathogens of poultry Submitting papers for publication.
(2025):Examining data to better comprehend the range of factors and agents affecting the poultry immune system. Establishing a definition of the CHIR role in infectious disease and reproduction. Modeling key effectors of MDV virulence in vitro and in vivo. Characterizing markers of immune response Developing an in vivo system for validating the impact of identified metabolites on immune system regulation. Identifying the magnitude of protection of probiotics, acidifiers, and vaccine schedules against enteric pathogens of poultry Publishing papers in peer-reviewed journals
(2026):Characterizing gene regulation in infectious disease, differentiation of immune cells and reproduction, specifically enhancers. Developing strategies to block MDV spread in poultry houses. Targeting key immune responses with feed interventions Characterizing the immunometabolism of negative responses. Submitting papers for publication.
Projected Participation
View Appendix E: ParticipationOutreach Plan
The Technical Committee invites industry stakeholders to attend the annual project meetings. The frequent attendance of these stakeholders facilitates information exchange. Project members learn of the emerging problems which can be examined through experiments. Poultry breeding companies can learn of genetic impacts in the immune system. The information exchange often leads to successful collaborations. Additional extension personnel and other potential collaborators will be invited. The project scientists will disseminate their new data using refereed publications, symposia, invited lectures, informal discussions and online data bases of genetic lines and genome/transcriptome information. Participants will work to develop a symposium at a national meeting. The cooperative effort among project members and other researchers will include sharing scientific expertise and genetic resources held at numerous project stations will continue. This cooperation has enabled members to make significant scientific contributions to the improvement of poultry immune responses as well as the genetics of disease resistance.
Project research will assess the effects of genetic variation on responses to immune stimuli including specific pathogens. Multiple stations will examine the major histocompatibility complex (MHC) which has significant impact on immune responses. Other stations study alloantigens or various molecules that modulate immunity via enhancement or suppression. All these approaches seek to improve poultry health in production environments that employ limited medications.
Poultry immune responses are influenced by many genetic, environmental, nutritional, physiological, management, and microbial factor. Technical committee members of NE-1834 are leaders in research to understand basic mechanisms as well as the unique features of the avian immune response. Furthermore, members have worked to develop effective means to promote poultry health and production. Project participants have published 165 refereed publications and 203 abstracts. Grants have been awarded from multiple agencies and businesses. This productive research focused on genetic factors, agents that affect immunity and toll development, to increase our knowledge and understanding of the immune response.
Collaboration and group interaction remain hallmarks of the group. The diversity of expertise among the project members contribute to project success. Resources are shared among project members. Genetic stocks including highly inbred chicken and turkey lines, sets of MHC-congenic lines, random breed lines and lines with distinct phenotypes combined with research techniques such as SNP analysis, qPCR, and next generation sequencing allow the assessment and selection of functional genetic elements that are related to immune function. Continuous communication and collaboration will occur through direct collaboration of participants and / or by presentation and diffusion of the results at scientific meetings, including our annual meeting. This constant contact allows to strategize and plan in order to avoid research topics duplication.
Organization/Governance
The Multistate Technical Committee has the responsibility for planning and supervision of the Multistate Research Project. Committee membership of this shall consist of an Administrative Advisor, which this time will be an active member of this project, a technical representative of each participating agency or experiment station, and a representative of the USDA National Institute of Food and Agriculture (NIFA). The voting membership shall consist of the Technical Committee Representatives. The Technical Committee shall be responsible for review and acceptance of contributing projects, preparation of reviews, modification of the multistate project proposal, and preparation of an annual report for transmittal by the Administrative Advisor upon approval to NIFA. Annual written reports will be prepared by each technical committee member and distributed prior to the annual meeting. The Technical Committee will meet annually.
At the annual meeting, the Technical Committee will elect a secretary, who will serve the year after election and as the chairperson the following year. An Executive Committee will be formed to conduct all business of the Technical Committee between annual meetings. The Executive Committee shall consist of the current Technical Committee Chairperson, the Secretary, and the two immediate Past Chairpersons. The chairperson may name other subcommittees as needed to perform specific assignments. They may include subcommittees to develop procedures, manuals, and phases of the multistate project, to review work assignments; to develop research methods, to prepare publications, and to write proposals. Other agencies and institutions may participate and vote at the invitation of the Administrative Advisor. Minimum expectations for Technical Committee members are submission of a written annual report every year, and attendance at an annual meeting including presentation of research results at least every other year. Collaborators may include emeritus members with an interest in attending annual meetings, scientists who wish to contribute by virtue of having special expertise or interest, and those who engage in research interactions with an individual Technical Committee member. Collaborators should submit a written report every year and present their progress when attending the annual meeting. Guests who attend an annual meeting through special connection to the Technical Committee (i.e. host institution) are invited to make a brief presentation of their interests and ongoing research.
Literature Cited
REFEREED JOURNAL ARTICLES (165)
* = Collaborative publication among multiple NE-1834 participants
43 journal articles (26%) are collaborative
Acevedo-Villanueva, K.Y., S. Renu, R. Shanmugasundaram, G.O. Akerele, R.J. Gourapura, and R.K. Selvaraj. 2021. Salmonella chitosan nanoparticle vaccine administration is protective against Salmonella Enteritidis in broiler birds. PLoS One. 16: e0259334
Alber, A., K. M. Morris, K. J. Bryson, K. Sutton, M. S. Monson, C. Chintoan-Uta, D. Borowska, S. J. Lamont, C. Schouler, P. Kaiser, M. Stevens, L. Vervelde. 2020. Avian pathogenic Escherichia coli (APEC) strain-dependent immunomodulation of respiratory granulocytes and mononuclear phagocytes in CSF1R-reporter transgenic chickens. Front. Immunol. 10:3055.
Asfor A. S., Nazki S., Reddy V., Campbell, E., Dulwich, D. L., Giotis, E. S., Skinner, M. A., and Broadbent A. J. 2021. Transcriptomic analysis of inbred chicken lines reveals infectious bursal disease severity is associated with greater bursal inflammation in vivo and more rapid induction of pro-inflammatory responses in primary bursal cells stimulated ex vivo. Viruses. 18;13(5):933 doi: 10.3390/v13050933
*Aston E. J., Y. Wang, K. E. Tracy, R. A. Gallardo, S. J. Lamont, H. Zhou, H. 2021. Comparison of cellular immune responses to avian influenza virus in two genetically distinct, highly inbred chicken lines. Vet. Immunol. Immunopathol. 235:110233.
*Aston, E. J., Y. Wang, K. E. Tracy, R. A. Gallardo, S. J. Lamont, and H. Zhou. 2021. Comparison of cellular immune responses to avian influenza virus in two genetically distinct, highly inbred chicken lines. Vet Immunol Immunopathol 235:110233. doi: 10.1016/j.vetimm.2021.110233
Aylward, B.A., C.N. Johnson, F. Perry, R. Whelan, C. Zhang, and R.J. Arsenault. 2022. Broiler chickens with 1950s genetics display a more stable immune profile as measured by kinome, mRNA expression, microbiome and metabolism when stimulated early in life with CpG. Poult. Sci. 101(5):101775-101788.
Azcarate-Peril, M. A., N. Butz, M. B. Cadenas, M. Koci, A. Ballou, M. Mendoza, R. Ali, and H. Hassan. 2018. An attenuated Salmonella enterica serovar typhimurium strain and galacto-oligosaccharides accelerate clearance of Salmonella infections in poultry through modifications to the gut microbiome. Appl Environ Microbiol 84. doi 10.1128/AEM.02526-17
Bai H, He Y, Ding Y, Chang S, Zhang H, Chen J, Song J. 2019. Parent-of-origin has no detectable effect on survival days of Marek's disease virus infected White Leghorns. Poult Sci. pii: pez209. doi: 10.3382/ps/pez209.
Bai H, He Y, Ding Y, Chu Q, Lian L, Heifetz EM, Yang N, Cheng HH, Zhang H, Chen J, Song J. 2020. Genome-wide characterization of copy number variations in the host genome in genetic resistance to Marek's disease using next generation sequencing. BMC Genet. 21(1):77. doi: 10.1186/s12863-020-00884-w.
Bai Y, Yuan, P., Zhang, H. Ramachandran, Yang, N., Song J. 2020. Adiponectin and its receptor genes expression in response to MDV infection of White Leghorns. Poultry Science. DOI: 10.1016/j.psj.2020.06.004
*Bai, H.; He, Y.; Ding, Y.; Carrillo, J.A.; Selvaraj, R.K.; Zhang, H.; Chen, J.; Song, J. 2019. Allele-Specific Expression of CD4+ T Cells in Response to Marek’s Disease Virus Infection. Genes 10, 718.
*Biswas, S., A. Nazmi, M. Pitesky, R.A. Gallardo, P. Pandey. 2019. Thermal inactivation of Escherichia coli and Salmonella typhimurium in poultry carcass and litter at different thermophilic temperatures. J. Appl. Poult. Res. 28:307-317. https://doi.org/10.3382/japr/pfy072
Bortaluzzi, C., L. Lahaye, F. Perry, R.J Arsenault, E. Santin, D.R. Korver, and M.H. Kogut. 2021. A protected complex of biofactors and antioxidants improved growth performance and modulated the immunometabolic phenotype of broiler chickens undergoing early life stress. 2021. Poult. Sci. 101176-101185.
*Cadena, M., L. Froenicke, M. Britton, M. L. Settles, B. Durbin-Johnson, E. Kumimoto, R. A. Gallardo, A. Ferreiro, T. Chylkova, H. Zhou, and M. Pitesky. 2019. Transcriptome Analysis of Salmonella Heidelberg after Exposure to Cetylpyridinium Chloride, Acidified Calcium Hypochlorite, and Peroxyacetic Acid. J Food Prot 82(1):109-119. doi: 10.4315/0362-028X.JFP-18-235
*Chanthavixay, G., C. Kern, Y. Wang, P. Saelao, S. J. Lamont, R. A. Gallardo, G. Rincon, and H. Zhou. 2020. Integrated transcriptome and histone modification analysis reveals NDV infection under heat stress affects bursa development and proliferation in susceptible chicken line. Front. Genetics 11:567812.
*Chanthavixay, G., C. Kern, Y. Wang, P. Saelao, S. J. Lamont, R. A. Gallardo, G. Rincon, and H. Zhou. 2020. Integrated Transcriptome and Histone Modification Analysis Reveals NDV Infection Under Heat Stress Affects Bursa Development and Proliferation in Susceptible Chicken Line. Front Genet 11:567812. doi: 10.3389/fgene.2020.567812
Chen H, Jin, K., Song J, Zuo Q, Yang H, Zhang Y, Li B. 2018. Functional characterization of the Sox2 , c-Myc , and Oct4 promoters. Journal of Cellular Biochemistry. DOI: 10.1002/jcb.27374
Cheng S, Wang M, Wang Y, Zhang C, Wang Y, Song J, Zuo Q, Zhang Y, Li B. 2018. RXRG associated in PPAR signal regulated the differentiation of primordial germ cell. J Cell Biochem. May 8. doi: 10.1002/jcb.26891.
Chu, Q.; Ding, Y.; Cai, W.; Liu, L.; Zhang, H.; Song, J. 2019. Marek’s disease virus infection-induced mitochondria changes in chickens. Int. J. Mol. Sci. 20:3150.
Coe, C., T. Boltz, R. Stearns, P. Foster, R. L. Taylor, Jr., J. S. Moritz, J. Jaczynski, A. Freshour, and C. Shen. 2022. Thermal inactivation of Salmonella typhimurium and the surrogate Enterococcus faecium in mash broiler feed in a laboratory scale circulated thermal bath. Poult. Sci. 101:101976 https://doi.org/10.1016/j.psj.2022.101976
Collisson, E., L. Griggs, and Y. Drechsler. 2017. Macrophages from disease resistant B2 haplotype chickens activate T lymphocytes more effectively than macrophages from disease susceptible B19 birds. Dev Comp Immunol 67:249-256. doi 10.1016/j.dci.2016.09.013
Conradie, A. M., L. D. Bertzbach, J. Trimpert, J. N. Patria, S. Murata, M. S. Parcells, and B. B. Kaufer. 2020. Distinct polymorphisms in a single herpesvirus gene are capable of enhancing virulence and mediating vaccinal resistance. PLoS Pathog 16:e1009104. doi 10.1371/journal.ppat.1009104
Conradie, A. M., L. D. Bertzbach, N. Bhandari, M. Parcells, and B. B. Kaufer. 2019. A Common Live-Attenuated Avian Herpesvirus Vaccine Expresses a Very Potent Oncogene. mSphere 4:e00658-00619. doi 10.1128/mSphere.00658-19
Cotter P. F. 2022. A microscopic study of the morphology of reactive thrombocytes of the duckling. J. World Poult. Res., 12 (3): ---. DOI: https://dx.doi.org/10.36380/jwpr.2022.-- (in press)
Cotter P. F. 2022. Cytogenetics of reactive bone marrow associated with a fungal infection (Hemomycetes avium) of ducklings. World J Vet Sci.;4(1): 1018 http://www.medtextpublications.com/open-access/cytogenetics-of-reactive-bone-marrow-associated-with-a-fungal-infection--1071.pdf
Cotter P. F. 2022. Plasmacyte heterogeneity of lame ducklings. J Immunological Sci. 6(1): 7-14 doi: 10.29245/2578-3009/2022/1.1225
Cotter P. F. 2022. Stress assessment by the hemogram method - circulating cells complicating reliance on heterophil/lymphocyte (H/L) ratio. J Vet Med Res 9(1): 1224. https://www.jscimedcentral.com/VeterinaryMedicine/veterinarymedicine-9-1224.pdf
Council for Agricultural Science and Technology (CAST) [Long., J. H. Blackburn, A. Martin, F. Silversides, R. L. Taylor, Jr. and C. Youngs]. 2019. Protecting food animal gene pools for future generations—A paper in the series on The Need for Agricultural Innovation to Sustainably Feed the World by 2050. Issue Paper 65. CAST, Ames, Iowa. https://www.cast-science.org/publication/protecting-food-animal-gene-pools-for-future-generations/
*da Silva, A. P., E. J. Aston, G. H. Chiwanga, A. Birakos, A. P. Muhairwa, B. B. Kayang, T. Kelly, H. Zhou, and R. A. Gallardo. 2020. Molecular Characterization of Newcastle Disease Viruses Isolated from Chickens in Tanzania and Ghana. Viruses 12(9)doi: 10.3390/v12090916
*da Silva, A. P., R. Hauck, S. R. C. Nociti, C. Kern, H. L. Shivaprasad, H. Zhou, and R. A. Gallardo. 2021. Molecular Biology and Pathological Process of an Infectious Bronchitis Virus with Enteric Tropism in Commercial Broilers. Viruses 13(8)doi: 10.3390/v13081477
Deist, M. S., and S. J. Lamont. 2018. What makes the Harderian gland transcriptome different from other chicken immune tissues? A gene expression comparative analysis. Front. Physiol. 9:492.
*Deist, M. S., R. A. Gallardo, D. A. Bunn, T. R. Kelly, J. C. M. Dekkers, H. Zhou, and S. J. Lamont. 2018. Novel analysis of the Harderian gland transcriptome response to Newcastle disease virus in two inbred chicken lines. Sci. Rep. 8:6558
*Deist, M. S., R. A. Gallardo, J. C. M. Dekkers, H. Zhou, and S. J. Lamont. 2020. Novel Combined Tissue Transcriptome Analysis After Lentogenic Newcastle Disease Virus Challenge in Inbred Chicken Lines of Differential Resistance. Front Genet 11:11. doi: 10.3389/fgene.2020.00011
*Deist, M. S., R. A. Gallardo, J. C. M. Dekkers, H. Zhou, and S. J. Lamont. 2020. Novel combined tissue transcriptome analysis after lentogenic Newcastle disease virus challenge in inbred chicken lines of differential resistance. Front. Genet. 11:11.
Del Vesco, A. P., H. J. Jang, M. S. Monson, and S. J. Lamont. 2021. Role of chicken oligoadenylate synthase like gene during in vitro Newcastle disease virus infection. Poult. Sci. 100:101067.
*Del Vesco, A. P., M. G. Kaiser, M. S. Monson, H. Zhou, and S. J. Lamont. 2020. Genetic responses of inbred chicken lines illustrate importance of eIF2 family and immune-related genes in resistance to Newcastle disease virus. Sci Rep 10(1):6155. doi: 10.1038/s41598-020-63074-9
*Del Vesco, A. P., M. G. Kaiser, M. S. Monson, H. Zhou, and S. J. Lamont. 2020. Genetic responses of inbred chicken lines illustrate importance of eIF2 family and immune-related genes in resistance to Newcastle disease virus. Sci. Rep. 10:6155.
Erf, G. F. 2019. Multifactorial, non-communicable diseases in poultry: the importance of conserving genetic models for autoimmune diseases. 68th Annual National Breeders Roundtable, St. Louis, MO: pp 1-7.
Erf, G. F., G. Le Pape, S. Rémy, D. M. Falcon, and C. Denesvre. 2020. Mardivirus infection and persistence in feathers of a chicken model harboring a local autoimmune response. Microorganisms. 8(10):1613. doi: 10.3390/microorganisms 8101613
Felfoldi, B., H. Wang, N. Nuthalapati, R. L. Taylor, Jr., J. D. Evans, S. L. Branton, and G. T. Pharr. 2021. Expression of chicken leukocyte cell-derived chemotaxin 2 in the embryonic bursa of Fabricius. Int. J. Poult. Sci. 20: 43-47 https://doi.org/10.3923/ijps.2021.43.47
*Figueroa, A., T. Derksen, S. Biswas, A. Nazmi, D. Rejmanek, B. Crossley, P. Pandey, R.A. Gallardo. 2021. Persistence of low and highly pathogenic avian influenza virus in reused poultry litter, effects of litter amendment use and composting temperatures. J. Appl. Poult. Res. 30,100096. https://doi.org/10.1016/j.japr.2020.09.011
French, C. E., M. A. Sales, S. J. Rochell, A. Rodriguez, and G. F. Erf. 2020. Local and systemic inflammatory responses to lipopolysaccharide in broilers: new insights using a two-window approach. Poult. Sci. 99:6593-6605. doi: 10.1016/j.psj.2020.09.078.
Fulton. J. E. W. Drobik-Czwarno, A. Wolc, A. M. McCarron, A.R. Lund, C. J. Schmidt and R. L. Taylor, Jr. 2022. The chicken A and E blood group systems are due to variation in proteins encoded by genes within the chicken RCA syntenic gene region. J. Immunol. 209:1-10 https://doi.org/10.4049/jimmunol.2101010
*Gallardo, R. A., A. P. Da Silva, S. Egana-Labrin, S. Stoute, C. Kern, H. Zhou, G. Cutler, and C. Corsiglia. 2020. Infectious Coryza: Persistence, Genotyping, and Vaccine Testing. Avian Dis 64(2):157-165. doi: 10.1637/0005-2086-64.2.157
Gilbert, I. M, J. M. Santamaria, and G. F. Erf. 2022. Time-course investigation of dermal leukocyte response to lipoteichoic acid in chickens. Discovery 23:44-50.
Glenn, H., Mullinex, G., and G. F. Erf. 2021. Effects of low crude protein diet with and without Spirulina platensis inclusion on white blood cell profiles in broilers. Discovery 22:38-44.
Hassan, H. M., M. Mendoza, M. Rezvani, M. D. Koci, A. N. Dickey, and E. H. Scholl. 2020. Complete genome sequences of Lactobacillus strains C25 and P38, isolated from chicken cecum. Microbiol Resour Announc 9. doi 10.1128/MRA.00501-20
He Y, Han B, Ding Y, Zhang H, Chang S, Zhang L, Zhao C, Yang N, and Song J. 2019. Linc-GALMD1 Regulates Viral Gene Expression in the Chicken. Front Genet. Nov 14;10:1122. doi: 10.3389/fgene.2019.01122. eCollection 2019.
He Y, Zuo Q, Edwards J, Zhao K, Lei J, Cai W, Nie Q, Li B, Song J. 2018. DNA Methylation and Regulatory Elements during Chicken Germline Stem Cell Differentiation. Stem Cell Reports. Apr 17. pii: S2213-6711(18)30145-0. doi: 10.1016/j.stemcr.2018.03.018.
He, H., R. J. Arsenault, K. Genovese, C. Swaggerty, C. Johnson, D. Nisbet, and M. H. Kogut. 2019. Inhibition of calmodulin increases intracellular survival of Salmonella in chicken macrophage cells. Vet. Microbiol. 232:156-161.
He, H., R. J. Arsenault, K. J. Genovese, C. Johnson, and M. H. Kogut. 2018. Chicken macrophages infected with Salmonella (S.) Enteritidis or S. Heidelberg produce differential responses in immune and metabolic signaling pathways. Vet. Immunol. Immunopathol. 195:46-55.
Hoek, K.H., M.J. Greer, K. McClanahan, A. Nazmi, K. Singh, M.P. Piazuelo, K.T. Wilson, D. Olivares-Villagόmez. 2021. Granzyme B prevents aberrant IL-17 production and increased pathogenicity in CD4+ T cells. Mucosal Immunol. 14(5):1088-1099. https://doi.org/10.1038/s41385-021-00427-1
Hughes, R. A., R. A. Ali, M. A. Mendoza, H. M. Hassan, and M. D. Koci. 2017. Impact of dietary galacto-oligosaccharide (GOS) on chicken's gut microbiota, mucosal gene expression, and Salmonella colonization. Front Vet Sci 4:192. doi 10.3389/fvets.2017.00192
*Irizarry, K. J. L., A. Chan, D. Kettle, S. Kezian, D. Ma, L. Palacios, Q. Q. Li, C. L. Keeler, and Y. Drechsler. 2017a. Bioinformatics Analysis of Chicken miRNAs Associated with Monocyte to Macrophage Differentiation and Subsequent IFNgamma Stimulated Activation. Microrna 6:53-70. doi 10.2174/2211536605666161129122803
*Irizarry, K. J. L., E. Downs, R. Bryden, J. Clark, L. Griggs, R. Kopulos, C. M. Boettger, T. J. Carr, Jr., C. L. Keeler, E. Collisson, and Y. Drechsler. 2017b. RNA sequencing demonstrates large-scale temporal dysregulation of gene expression in stimulated macrophages derived from MHC-defined chicken haplotypes. PLoS One 12:e0179391. doi 10.1371/journal.pone.0179391
Jang, H.-J., M. Monson, M. Kaiser, and S. J. Lamont. 2020. Induction of chicken host defense peptides within disease-resistant and -susceptible lines. Genes 11:1195.
Jiang J, Chen C, Cheng S, Yuan X, Jin J, Zhang C, Sun X, Song J, Zuo Q, Zhang Y, Chen G, Li B. 2021. Long Noncoding RNA LncPGCR Mediated by TCF7L2 Regulates Primordial Germ Cell Formation in Chickens. Animals (Basel) 11(2):292. doi: 10.3390/ani11020292. PMID: 33498947; PMCID: PMC7912682.
Jin K, Chen C., Sun, X., Zhu, C., Ahmed, M. Zuo, Q., Song J, Li B. Identification and generation of transgenic mice and goats with Capra hircus SCD1 Gene. Pakistan Journal of Zoology DOI: 10.17582/journal.pjz/20181013161028
Jin K, Li D, Jin J, Song J, Zhang Y, Chang G, Chen G, Li B. 2020. C1EIP Functions as an Activator of ENO1 to Promote Chicken PGCs Formation via Inhibition of the Notch Signaling Pathway. Front Genet. Jul 24;11:751. doi: 10.3389/fgene.2020.00751. PMCID: PMC7396672.
Jin K, Zhou J, Zuo Q, Song J, Zhang Y, Chang G, Chen G, Li B. 2020. Transcriptome Sequencing and Comparative Analysis of Amphoteric ESCs and PGCs in Chicken (Gallus gallus). Animals (Basel). Nov 27;10(12):E2228. doi: 10.3390/ani10122228. PMID: 33261034.
Jin K, Zuo Q, Song J, Zhang Y, Chen G, Li B. 2020. CYP19A1 (aromatase) dominates female gonadal differentiation in chicken (Gallus gallus) embryos sexual differentiation. Biosci Rep. Oct 30;40(10):BSR20201576. doi: 10.1042/BSR20201576.
Jing, Y., Y. Yuan, M. Monson, P. Wang, F. Mu, Q. Zhang, W., Na, K. Zhang, Y. Wang, L. Leng, Y. Li, P. Luan, N. Wang, R. Guo, S. Lamont, H. Li and H. Yuan. 2022. Multi-omics association reveals the effects of intestinal microbiome-host interactions on fat deposition in broiler lines divergently selected for abdominal fat content. Front. Microbiol. 12:815538.
Johnson, C., M. Kogut, K. Genovese, H. He, S. Kazemi, R. Arsenault. 2019. Administration of a postbiotic causes immunomodulatory responses in broiler gut and reduces disease pathogenesis following challenge. 2019. Microorganisms. 7(8): 268-287.
Johnson, C.N., M.M. Hashim, C.A. Bailey, J.A. Byrd, M.H. Kogut and R.J. Arsenault. 2020. Feeding of yeast cell wall extracts during a necrotic enteritis challenge enhances cell growth/survival and immune signaling in the jejunum of broiler chickens. Poult. Sci. 99(6):2955-2966.
Kaiser, M., J. Hsieh, P. Kaiser, and S. J. Lamont. 2022. Differential immunological response detected in mRNA expression profiles among diverse chicken lines in response to Salmonella challenge. Poult. Sci. 101:101605.
Kim, T. H., and H. Zhou. 2018. Overexpression of Chicken IRF7 Increased Viral Replication and Programmed Cell Death to the Avian Influenza Virus Infection Through TGF-Beta/FoxO Signaling Axis in DF-1. Front Genet 9:415. doi: 10.3389/fgene.2018.00415
Kim, T. H., C. Kern, and H. Zhou. 2020. Knockout of IRF7 Highlights its Modulator Function of Host Response Against Avian Influenza Virus and the Involvement of MAPK and TOR Signaling Pathways in Chicken. Genes (Basel) 11(4)doi: 10.3390/genes11040385
Kim, T., H. D. Hunt, M. S. Parcells, V. van Santen, and S. J. Ewald. 2018. Two class I genes of the chicken MHC have different functions: BF1 is recognized by NK cells while BF2 is recognized by CTLs. Immunogenetics. doi 10.1007/s00251-018-1066-2
Kogut, M., K.J. Genovese, J.A. Byrd, C. Swaggerty, H. He, Y. Farnell, and R. Arsenault. 2022. Chicken-Specific Kinome Analysis of Early Host Immune Signaling Pathways in the Cecum of Newly Hatched Chickens Infected with Salmonella enterica Serovar Enteritidis. Front. Cellular Infect. Microbiol. 12:857-874.
Krieter, A., H. Xu, H. Akbar, T. Kim, and K. W. Jarosinski. 2022. The conserved Herpesviridae protein kinase (CHPK) of Gallid alphaherpesvirus 3 (GaHV3) is required for horizontal spread and natural infection in chickens. Viruses 14. doi:https://doi.org/10.3390/v14030586.
Krieter, A., N. Ponnuraj, and K. W. Jarosinski. 2020. Expression of the conserved herpesvirus protein kinase (CHPK) of Marek's disease alphaherpesvirus in the skin reveals a mechanistic importance for CHPK during interindividual spread in chickens. J Virol 94:e01522-01519. doi:https://doi.org/10.1128/JVI.01522-19.
Lai B, Tang Q, Jin W, Hu G, Wangsa D, Cui K, Stanton BZ, Ren G, Ding Y, Zhao M, Liu S, Song J, Ried T, Zhao K. Trac-looping measures genome structure and chromatin accessibility. Nat Methods. 2018 Aug 27. doi: 10.1038/s41592-018-0107-y
Lee, A., G.C. Dal Pont, M. Battaglia, R. Arsenault, and M. Kogut. 2021. Role of JAK-STAT pathway in chicks fed with chestnut tannins. Animals. 11(2): 337-347.
Lee, A., G.C. Dal Pont, M.B. Farnell, S. Jarvis, M. Battaglia, R. Arsenault, and M. Kogut. 2021. Supplementing chestnut tannins in the broiler diet mediates a metabolic phenotype of the ceca. Poult. Sci. 100(1): 47-54.
Litvak, Y., K. K. Z. Mon, H. Nguyen, G. Chanthavixay, M. Liou, E. M. Velazquez, L. Kutter, M. A. Alcantara, M. X. Byndloss, C. R. Tiffany, G. T. Walker, F. Faber, Y. Zhu, D. N. Bronner, A. J. Byndloss, R. M. Tsolis, H. Zhou, and A. J. Baumler. 2019. Commensal Enterobacteriaceae Protect against Salmonella colonization through oxygen competition. Cell Host Microbe 25(1):128-139 e125. doi: 10.1016/j.chom.2018.12.003
Liu L, Wang D, Mi S, Duan Z, Yang S, Song J, Xu G, Yang N, Yu Y. 2019. The different effects of viral and bacterial mimics maternal stimuli on ethology of hens and reproduction of their offspring. Poult Sci. Apr 15. pii: pez189. doi: 10.3382/ps/pez189.
Liu L, Yang N, Xu G, Liu S, Wang D, Song J, Duan Z, Yang S, Yu Y. 2018. Transgenerational transmission of maternal stimulatory experience in domesticated birds. FASEB J. Sep 27:fj201800762RR. doi: 10.1096/fj.201800762RR.
Markazi, A., A. Luoma, R. Shanmugasundaram, G.R. Murugesan, M. Mohnl, and R.K. Selvaraj. 2019. Effect of acidifier product supplementation in laying hens challenged with Salmonella. J. Appl. Poul. Res. 28: 919-929.
Meyer, M. M., S. J. Lamont, and E. A. Bobeck. 2022. Mitochondrial and glycolytic capacity of peripheral blood mononuclear cells isolated from diverse poultry genetic lines: optimization and assessment. Front. Vet. Sci. 8:815878.
Mon, K. K. Z., C. Kern, G. Chanthavixay, Y. Wang, and H. Zhou. 2021. Tolerogenic Immunoregulation towards Salmonella Enteritidis Contributes to Colonization Persistence in Young Chicks. Infect Immun 89(8):e0073620. doi: 10.1128/IAI.00736-20
Mon, K. K. Z., Y. Zhu, G. Chanthavixay, C. Kern, and H. Zhou. 2020. Integrative analysis of gut microbiome and metabolites revealed novel mechanisms of intestinal Salmonella carriage in chicken. Sci Rep 10(1):4809. doi: 10.1038/s41598-020-60892-9
Monson, M. S. and S. J. Lamont. 2021. Genetic resistance to avian pathogenic Escherichia coli (APEC): current status and opportunities. Avian Pathol. 50:392-401.
*Monson, M. S., A. G. Van Goor, C. M. Ashwell, M. P. Persia, M. F. Rothschild, C. J. Schmidt, and S. J. Lamont. 2018. Immunomodulatory effects of heat stress and lipopolysaccharide on the bursal transcriptome in two distinct chicken lines. BMC Genomics 19:643.
Monson, M. S., A. G. Van Goor, M. E. Persia, M. F. Rothschild, C. J. Schmidt, and S. J. Lamont. 2019. Genetic lines respond uniquely within the chicken thymic transcriptome to acute heat stress and low dose lipopolysaccharide. Sci. Rep. 9:13649.
Monson, M. S., B. L. Bearson, M. J. Sylte, T. Looft, S. J. Lamont, and S. M. D. Bearson. 2021. Transcriptional response of blood leukocytes from turkeys challenged with Salmonella enterica serovar Typhimurium UK1. Vet. Immunol. Immunopathol. 232:110181.
Mullenix, G. J., E. S. Greene, N. K. Emami, G. Tellez-Isaias, W. G. Bottje, G. F. Erf, M. T. Kidd, S. Dridi. 2021. Spirulina platensis inclusion reverses circulating pro-inflammatory (chemo)cytokine profiles in broilers fed low-protein diets. Front. Vet. Sci.8:640968. Doi:10.3389/fvets.2021.640968
*Mushi, J. R., G. H. Chiwanga, E. L. Mollel, M. Walugembe, R. A. Max, P. M. Msoffe, R. A. Gallardo, T. R. Kelly, S. Lamont, J. Dekkers, H. Zhou, and A. Muhairwa. 2021. Antibody response, viral load, viral clearance, and growth rate in Tanzanian free-range local chickens infected with lentogenic Newcastle disease virus. J Vet Med Animal Health 13:98-105.
*Mushi, J. R., G. H. Chiwanga, E. N. Amuzu-Aweh, M. Walugembe, R. A. Max, S. J. Lamont, T. R. Kelly, E. L. Mollel, P. L. Msoffe, J. Dekkers, R. Gallardo, H. Zhou, and A. P. Muhairwa. 2020. Phenotypic variability and population structure analysis of Tanzanian free-range local chickens. BMC Vet Res 16(1):360. doi: 10.1186/s12917-020-02541-x
Nath Neerukonda, S., N. A. Egan, J. Patria, I. Assakhi, P. Tavlarides-Hontz, S. Modla, E. R. Munoz, M. B. Hudson, and M. S. Parcells. 2019. Comparison of exosomes purified via ultracentrifugation (UC) and Total Exosome Isolation (TEI) reagent from the serum of Marek's disease virus (MDV)-vaccinated and tumor-bearing chickens. J Virol Methods 263:1-9. doi 10.1016/j.jviromet.2018.10.004
Nazmi, A., K. G. McClanahan, D. Olivares-Villagόmez. 2021. Unconventional intestinal intraepithelial lymphocytes in health and disease. Crit. Rev. Immunol. 41(4):23-38. DOI: 10.1615/CritRevImmunol.2021039957
Nazmi, A., K.H. Hoek, M.J. Greer, M.B. Piazuelo, N. Minato, D. Olivares-Villagόmez. 2019. Innate CD8αα+ cells promote ILC1-like intraepithelial lymphocyte homeostasis and intestinal inflammation. PLoS One. 10:14(7):e0215883. https://doi.org/10.1371/journal.pone.0215883
Nazmi, A., M.J. Greer, K.H. Hoek, M.B. Piazuelo, J.H. Weitkamp, D. Olivares-Villagόmez. 2020. Osteopontin and iCD8α cells promote intestinal intraepithelial lymphocyte homeostasis. J. Immunol. 1;204(7):1968-1981. https://doi.org/10.4049/jimmunol.1901168
Neerukonda, S. N., P. Tavlarides-Hontz, F. McCarthy, K. Pendarvis, and M. S. Parcells. 2019b. Comparison of the Transcriptomes and Proteomes of Serum Exosomes from Marek's Disease Virus-Vaccinated and Protected and Lymphoma-Bearing Chickens. Genes (Basel) 10. doi 10.3390/genes10020116
Neerukonda, S. N., U. K. Katneni, M. Bott, S. P. Golovan, and M. S. Parcells. 2018. Induction of the unfolded protein response (UPR) during Marek's disease virus (MDV) infection. Virology 522:1-12. doi 10.1016/j.virol.2018.06.016
Neerukonda, S. N., U. K. Katneni, N. Bhandari, and M. S. Parcells. 2019a. Transcriptional Analyses of Innate and Acquired Immune Patterning Elicited by Marek's Disease Virus Vaccine Strains: Turkey Herpesvirus (HVT), Marek's Disease Virus 2 (strain SB1), and Bivalent Vaccines (HVT/SB1 and HVT-LT/SB1). Avian Dis 63:670-680. doi 10.1637/aviandiseases-D-19-00117
Niu Q, Zhang T, Xu L, Wang T, Wang Z, Zhu B, Zhang L, Gao H, Song J, Li J, Xu L. 2021. Integration of selection signatures and multi-trait GWAS reveals polygenic genetic architecture of carcass traits in beef cattle. Genomics 113(5):3325-3336. DOI: 10.1016/j.ygeno.2021.07.025. Epub Jul 25. PMID: 34314829.
Nuthalapati, N., J. D. Evans, R. L. Taylor, Jr., S. L. Branton, B. Nanduri, and G. T. Pharr. 2019. Transcriptomic analysis of early B-cell development in the chicken embryo. Poult. Sci. 98:5342-5354 https://doi.org/10.3382/ps/pez354 published online: 06/25/19
Nuthalapati, N., T. A. Burks, R. L. Taylor, Jr., P. B. Siegel, and G. T. Pharr. 2021. Protein tyrosine kinase gene expression profiles in the embryonic bursa of Fabricius of chicken lines selected for high and low antibody responses. Int. J. Poult. Sci. 20:173-178 https://doi.org/10.3923/ijps.2021.173.178
Overbey, E. G., T. T. Ng, P. Catini, L. M. Griggs, P. Stewart, S. Tkalcic, R. D. Hawkins, and Y. Drechsler. 2021. Transcriptomes of an Array of Chicken Ovary, Intestinal, and Immune Cells and Tissues. Frontiers in Genetics 12. doi 10.3389/fgene.2021.664424
Pagano, G., C. Johnson, C. Hahn, and R.J. Arsenault. 2018. A new tool for studying waterfowl immune and metabolic responses: molecular level analysis using kinome profiling. Ecology and Evolution. 8(16): 8537-8546.
Perry, F., C.N. Johnson, B.A. Aylward, and R.J. Arsenault. 2020. The Differential Phosphorylation-Dependent Signaling and Glucose Immunometabolic Responses Induced during Infection by Salmonella Enteritidis and Salmonella Heidelberg in Chicken Macrophage-like cells. Microorganisms. 8(7):1041-1061.
Ponnuraj, N., Y. T. Tien, W. Vega-Rodriguez, A. Krieter, and K. W. Jarosinski. 2019. The Herpesviridae Conserved Multifunctional Infected-Cell Protein 27 (ICP27) Is Important but Not Required for Replication and Oncogenicity of Marek's Disease Alphaherpesvirus. J Virol 93:e01903-01918. doi:https://doi.org/10.1128/JVI.01903-18.
Raddatz, G., R.J. Arsenault, B. Aylward, R. Whelan, F. Böhl, and F. Lyko. 2021. A chicken DNA methylation clock for the prediction of broiler health. Communications Biol.. 4(1):1-8.
Ramírez, G. A., E. Richardson, J. Clark, J. Keshri, Y. Drechsler, M. E. Berrang, R. J. Meinersmann, N. A. Cox, and B. B. Oakley. 2020. Broiler Chickens and Early Life Programming: Microbiome transplant-induced cecal bacteriome dynamics and phenotypic effects. bioRxiv:2020.2008.2013.240572. doi 10.1101/2020.08.13.240572
Reddy, V., Campbell, E. A., Wells, J., Simpson, J., Nazki, S., Hawes, P. C., and Broadbent, A. J. 2022. Birnaviridae virus factories show features of liquid-liquid phase separation, and are distinct from paracrystalline arrays of virions observed by electron microscopy. J. Virol. 23;96(6):e0202421 doi: 10.1128/jvi.02024-21.
Reddy, V., Nazki, S., Brodrick, A. J., Asfor, A., Urbaniec, J., Morris, Y., and Broadbent, A. J. 2022. Evaluating the breadth of neutralizing antibody responses elicited by infectious bursal disease virus (IBDV) genogroup A1 strains using a novel chicken B-cell rescue system and neutralization assay. J. Virol. 28;96(18):e0125522 doi: 10.1128/jvi.01255-22
Redweik, G.A.J., M.H. Kogut, R.J. Arsenault, and M. Mellata. 2020. Oral Treatment with Ileal Spores Triggers Immunometabolic Shifts in Chicken Gut. Front. Vet. Sci. 7(629):1-14.
Redweik, G.A.J., M.H. Kogut, R.J. Arsenault, M. Lyte, and Mellata M. 2021. Reserpine improves Enterobacteriaceae resistance in chicken intestine via neuro-immunometabolic signaling and MEK1/2 activation. Communications Biol. 4(1):1-11.
Renu, S., Y. Han, Y. Lakshmanappa, S. Ghimire, N. Feliciano-Ruiz, S. Senapati, B. Narasimhan, R.K. Selvaraj, and R.J. Gourapura. 2020. Chitosan-adjuvanted Salmonella subunit nanovaccine for poultry delivered through drinking water and feed. Carbohydrate Polymers. Carbohydrate Polymers 243: 116434
Rocchi, A., J. Ruff, C. J. Maynard, A. J. Forga, R. Señas-Cuesta, E. S. Greene, J. D. Latorre, C. N. Vuong, B. D. Graham, X. Hernandez-Velasco, G. Tellez Jr., V. M. Petrone-Garcia, B. M. Hargis, G. F. Erf, C. M. Owens, and G. Tellez-Isaias. 2022. Cyclic heat stress model alters intestinal permeability, bone mineralization, and meat quality in broiler chickens. Animals 12:1273 doi: 10.3390/ani12101273.
*Rowland, K., A. Wolc, R. A. Gallardo, T. Kelly, H. Zhou, J. C. M. Dekkers, and S. J. Lamont. 2018. Genetic analysis of a commercial egg laying line challenged with Newcastle Disease Virus. Front. Genetics 9:326.
*Rowland, K., P. Saelao, Y. Wang, J. E. Fulton, G. N. Liebe, A. M. McCarron, A. Wolc, R. A. Gallardo, T. Kelly, H. Zhou, J. C. M. Dekkers, and S. J. Lamont. 2018. Association of candidate genes with response to heat and Newcastle disease virus. Genes 9:560.
*Rowland, K., P. Saelao, Y. Wang, J. E. Fulton, G. N. Liebe, A. M. McCarron, A. Wolc, R. A. Gallardo, T. Kelly, H. Zhou, J. C. M. Dekkers, and S. J. Lamont. 2018. Association of Candidate Genes with Response to Heat and Newcastle Disease Virus. Genes (Basel) 9(11)doi: 10.3390/genes9110560
*Saelao, P., Y. Wang, G. Chanthavixay, V. Yu, R. A. Gallardo, S. J. Lamont, J. M. Dekkers, T. Kelly, and H. Zhou. 2018. Integrated proteomic and transcriptomic analysis of differential expression of chicken lung tissue in response to NDV infection during heat stress. Genes 9:579.
*Saelao, P., Y. Wang, G. Chanthavixay, R. A. Gallardo, A. Wolc, J. C. M. Dekkers, S. J. Lamont, and H. Zhou. 2019. Genetics and genomic regions affecting response to Newcastle disease virus infection under heat stress in layer chickens. Genes 10:61.
*Saelao, P., Y. Wang, G. Chanthavixay, R. A. Gallardo, A. Wolc, J. C. M. Dekkers, S. J. Lamont, T. Kelly, and H. Zhou. 2019. Genetics and Genomic Regions Affecting Response to Newcastle Disease Virus Infection under Heat Stress in Layer Chickens. Genes (Basel) 10(1)doi: 10.3390/genes10010061
*Saelao, P., Y. Wang, G. Chanthavixay, V. Yu, R. A. Gallardo, J. C. M. Dekkers, S. J. Lamont, T. Kelly, and H. Zhou. 2021. Distinct transcriptomic response to Newcastle disease virus infection during heat stress in chicken tracheal epithelial tissue. Sci. Rep. .11:7450.
*Saelao, P., Y. Wang, G. Chanthavixay, V. Yu, R. A. Gallardo, J. C. M. Dekkers, S. J. Lamont, T. Kelly, and H. Zhou. 2018. Integrated Proteomic and Transcriptomic Analysis of Differential Expression of Chicken Lung Tissue in Response to NDV Infection during Heat Stress. Genes (Basel) 9(12)doi: 10.3390/genes9120579
*Saelao, P., Y. Wang, G. Chanthavixay, V. Yu, R. A. Gallardo, J. C. M. Dekkers, S. J. Lamont, T. Kelly, and H. Zhou. 2021. Distinct transcriptomic response to Newcastle disease virus infection during heat stress in chicken tracheal epithelial tissue. Sci Rep 11(1):7450. doi: 10.1038/s41598-021-86795-x
*Saelao, P., Y. Wang, R. A. Gallardo, S. J. Lamont, J. M. Dekkers, T. Kelly, and H. Zhou. 2018. Novel insights into the host immune response of chicken Harderian gland tissue during Newcastle disease virus infection and heat treatment. BMC Vet Res 14(1):280. doi: 10.1186/s12917-018-1583-0
Sato, J., S. Murata, Z. Yang, B. B. Kaufer, S. Fujisawa, H. Seo, N. Maekawa, T. Okagawa, S. Konnai, N. Osterrieder, M. S. Parcells, and K. Ohashi. 2022. Effect of Insertion and Deletion in the Meq Protein Encoded by Highly Oncogenic Marek’s Disease Virus on Transactivation Activity and Virulence. Viruses 14:382.
Schilling, M. A., R. Katani, S. Memari, M. Cavanaugh, J. Buza, J. Radzio-Basu, F. N. Mpenda, M. S. Deist, S. J. Lamont, and V. Kapur. 2018. Transcriptional innate immune response of the developing chicken embryo to Newcastle disease virus infection. Front. Genetics 9:61
Schilling, M., S. Memari, M. Cavanaugh, R. Katani, M. S. Deist, J. Radzio-Basu, S. J. Lamont, J. J. Buza, and V. Kapur. 2019. Conserved, breed-dependent, and subline-dependent innate immune responses of Fayoumi and Leghorn chicken embryos to NDV infection. Sci. Rep. 9:7209.
*Silva, A. P. D., R. Hauck, C. Kern, Y. Wang, H. Zhou, and R. A. Gallardo. 2019. Effects of Chicken MHC Haplotype on Resistance to Distantly Related Infectious Bronchitis Viruses. Avian Dis 63(2):310-317. doi: 10.1637/11989-103118-Reg.1
Song, J. He, Y, Ding, Y. Tian, F. Zhao, K., Zhang, H., Yu, Y., Yang, N., Lian, L., Luo, J., Mitra, A. 2021. The Epigenetics and Plasticity of CD4+ T Cells in Poultry Health, Journal of Animal Science, 99(Suppl 3): 55, https://doi.org/10.1093/jas/skab235.098
Sorrick, J., W. Huett, K. A. Byrne, and G. F. Erf. 2022.Autoimmune activities in choroids of visually impaired Smyth chickens with autoimmune vitiligo. Front. Med. 9:846100. doi:10.3389/fmed.2022.846100.
Su, S., K. B. Miska, R. H. Fetterer, M. C. Jenkins, S. J. Lamont, and Wong, E.A. 2018. Differential expression of intestinal nutrient transporters and host defense peptides in E. maxima infected Fayoumi and Ross chickens. Poult. Sci. 97: 4392-4400
Sun C, Jin K, Zhou J, Zuo Q, Song J, Yani Z, Chen G, Li B. 2021. Role and function of the Hintw in early sex differentiation in chicken (Gallus gallus) embryo. Anim Biotechnol. 21:1-11. DOI: 10.1080/10495398.2021.1935981. Epub ahead of print. PMID: 34153202.
Sun C, Jin K, Zuo Q, Sun H, Song J, Zhang Y, Chen G, Li B. 2021. Characterization of Alternative Splicing (AS) Events during Chicken (Gallus gallus) Male Germ-Line Stem Cell Differentiation with Single-Cell RNA-seq. Animals (Basel)11(5):1469. doi: 10.3390/ani11051469. PMID: 34065391; PMCID: PMC8160964.
Sun C, Wang Y, Jin K, Song J, Zuo Q, Zhang Y, Chen G, Li B. 2018. Study on immortal conditions of chicken embryonic stem cells. J Cell Biochem. 2018 Nov 16. doi: 10.1002/jcb.27173.
Swaggerty, C.L., J.A. Byrd, R.J. Arsenault, F. Perry, C.N. Johnson, K.J. Genovese, H. He, M.H. Kogut, A. Piva, and E. Grilli. 2022. A blend of microencapsulated organic acids and botanicals reduces necrotic enteritis via specific signaling pathways in broilers. Poult. Sci. 101(4): p.101753-101763.
Swaggerty, C.L., R.J. Arsenault, C. Johnson, A. Piva, and E. Grilli. 2020. Dietary supplementation with a microencapsulated blend of organic acids and botanicals alters the kinome in the ileum and jejunum to enhance growth and feed efficiency in broilers. PLOS One. 15(7), e0236950.
Taylor, R. L., Jr. 2020. A Year of Change. Poult. Sci. 99:6291–6292 https://doi.org/10.1016/j.psj.2020.09.018
Taylor, R. L., Jr. 2021. The 100 most cited papers from Poultry Science’s centennial. Poult. Sci. 100:101256 https://doi.org/10.1016/j.psj.2021.101256
Taylor, R. L., Jr. 2022. The 50 most downloaded articles from Poultry Science in 2021. Poult. Sci. 101: 101818 https://doi.org/10.1016/j.psj.2022.101818
Taylor, R. L., Jr. and D. Jones. 2021. A century of progress 1921-2021. Poult. Sci. 100:101073 https://doi.org/10.1016/j.psj.2021.101073
Tilley, J. E. N., J. L. Grimes, M. D. Koci, R. A. Ali, C. R. Stark, P. K. Nighot, T. F. Middleton, and A. C. Fahrenholz. 2017. Efficacy of feed additives to reduce the effect of naturally occurring mycotoxins fed to turkey hen poults reared to 6 weeks of age. Poult Sci 96:4236-4244. doi 10.3382/ps/pex214
Tong, Z. W. M., A. C. Karawita, C. Kern, H. Zhou, J. E. Sinclair, L. Yan, K. Y. Chew, S. Lowther, L. Trinidad, A. Challagulla, K. A. Schat, M. L. Baker, and K. R. Short. 2021. Primary Chicken and Duck Endothelial Cells Display a Differential Response to Infection with Highly Pathogenic Avian Influenza Virus. Genes (Basel) 12(6)doi: 10.3390/genes12060901
Troxell, B., M. Mendoza, R. Ali, M. Koci, and H. Hassan. 2020. Attenuated Salmonella enterica serovar typhimurium, Strain NC983, is immunogenic, and protective against virulent typhimurium challenges in mice. Vaccines (Basel) 8. doi 10.3390/vaccines8040646
*Tudeka, C. K., G. K. Aning, A. Naazie, P. K. Botchway, E. N. Amuzu-Aweh, G. K. Agbenyegah, B. Enyetornye, D. Fiadzomor., P. Saelao, Y. Wang, T. R. Kelly, R. Gallardo, J. C. M. Dekkers, S. J. Lamont, H. Zhou, and B. B. Kayang. 2022. Response of three local chicken ecotypes of Ghana to lentogenic and velogenic Newcastle disease virus challenge. Tropical Anim. Health Prod. 54: 134.
*Tudeka, C. K., G. K. Aning, A. Naazie, P. K. Botchway, E. N. Amuzu-Aweh, G. K. Agbenyegah, B. Enyetornye, D. Fiadzomor, P. Saelao, Y. Wang, T. R. Kelly, R. Gallardo, J. C. M. Dekkers, S. J. Lamont, H. Zhou, and B. B. Kayang. 2022. Response of three local chicken ecotypes of Ghana to lentogenic and velogenic Newcastle disease virus challenge. Trop Anim Health Prod 54(2):134. doi: 10.1007/s11250-022-03124-8
Vega-Rodriguez, W., H. Xu, N. Ponnuraj, H. Akbar, T. Kim, and K. W. Jarosinski. 2021b. The requirement of glycoprotein C (gC) for interindividual spread is a conserved function of gC for avian herpesviruses. Scientific reports 11:7753. doi:https://doi.org/10.1038/s41598-021-87400-x.
Vega-Rodriguez, W., N. Ponnuraj, M. Garcia, and K. W. Jarosinski. 2021a. Ther equirement of glycoprotein C for interindividual spread is functionally conserved within the alphaherpesvirus Genus (Mardivirus), but not the host (Gallid). Viruses 13. doi:https://doi.org/10.3390/v13081419.
*Walugembe, M., E. N. Amuzu-Aweh, P. K. Botchway, A. Naazie, G. Aning, Y. Wang, P. Saelao, T. Kelly, R. A. Gallardo, H. Zhou, S. J. Lamont, B. Kayang, and J. Dekkers. 2020. Genetic basis of response of Ghanaian local chickens to infection with a lentogenic Newcastle disease virus. Front. Genetics 11:739. doi: 10.3389/fgene.2020.00739
*Walugembe, M., J. Mushi, E. Amuzu-Aweh, G. Chiwanga, P. Msoffe, Y. Wang, P. Saelao, T. Kelly, R. Gallardo, H. Zhou, S. Lamont, A. Muhairwa, and J. Dekkers. 2019. Genetic analyses of Tanzania local chicken ecotypes challenged with Newcastle disease virus. Genes 10: 546. doi: 10.3390/genes10070546
*Wang, Y., P. Saelao, C. Kern, S. Jin, R. A. Gallardo, T. Kelly, J. M. Dekkers, S. J. Lamont, and H. Zhou. 2020. Liver transcriptome responses to heat stress and Newcastle Disease virus infection in genetically distinct chicken inbred lines. Genes 11:1067. doi: 10.3390/genes11091067
*Ward, T.L., B.P. Weber, K.M. Mendoza, J.L. Danzeisen, K. Llop, K. Lang, J.B. Clayton, E. Grace, J. Brannon, I. Radovic, M. Beauclaire, T.J. Heisel, D. Knights, C. Cardona, M. Kogut, C. Johnson, S.L. Noll, R. Arsenault, K.M. Reed, and T.J. Johnson. 2019. Antibiotics and host-tailored probiotics similarly modulate effects on the developing avian microbiome, mycobiome, and host gene expression. mBio. 10(5): 02171-19.
Wilkinson, N. G., R. T. Kopulos, L. M. Yates, W. E. Briles, and R. L. Taylor, Jr. 2020. Major histocompatibility (B) complex recombinant R13 antibody response against bovine red blood cells. Poult. Sci. 99:4804-4808 https://doi.org/10.1016/j.psj.2020.06.069
Wilkinson, N. G., R. T. Kopulos, L. M. Yates, W. E. Briles, and R. L. Taylor, Jr. 2021. Research Note: Rous sarcoma growth differs among congenic lines containing major histocompatibility (B) complex recombinants. Poult. Sci. 100:101335 https://doi.org/10.1016/j.psj.2021.101335
*Xu, L., Y. He, Y. Ding, G. E. Liu, H. Zhang, H. H. Cheng, R. L. Taylor, Jr., and J. Song. 2018. Genome-wide assessment of inbred chicken lines indicates genomic signatures of resistance to Marek’s Disease. J. Anim. Sci. Biotech. 9:65-75 doi 10.1186/s40104-018-0281 published online: 09/13/18)
You Z, Zhang Q, Liu C, Song J, Yang N, Lian L. 2019. Integrated analysis of lncRNA and mRNA repertoires in Marek's disease infected spleens identifies genes relevant to resistance. BMC Genomics. Mar 28;20(1):245. doi: 10.1186/s12864-019-5625-1.
Yuan X, Zhang C, Zhao R, Jiang J, Shi X, Zhang M, Sun H, Zuo Q, Zhang Y, Song J, Chen G, Li B. 2021. Glycolysis Combined with Core Pluripotency Factors to Promote the Formation of Chicken Induced Pluripotent Stem Cells. Animals (Basel) 11(2):425. DOI: 10.3390/ani11020425. PMID: 33562170; PMCID: PMC7915628.
Zhang C, Zuo Q, Wang M, Chen H, He N, Jin J, Li T, Jiang J, Yuan X, Li J, Shi X, Zhang M, Bai H, Zhang Y, Xu Q, Cui H, Chang G, Song J, Sun H, Zhang Y, Chen G, Li B. 2020. Narrow H3K4me2 is required for chicken PGC formation. J Cell Physiol 236, 1391-1400. Aug 4. doi: 10.1002/jcp.29945.
*Zhang, J., M. G. Kaiser, M. S. Deist, R. A. Gallardo, D. A. Bunn, T. R. Kelly, J. C. M. Dekkers, H. Zhou, and S. J. Lamont. 2018. Transcriptome analysis in spleen reveals differential regulation of response to Newcastle disease virus in two chicken lines. Sci. Rep. 8:1278.
*Zhang, J., M. G. Kaiser, R. A. Gallardo, T. R. Kelly, J. C. M. Dekkers, H. Zhou, and S. J. Lamont. 2020. Transcriptome analysis reveals inhibitory effects of lentogenic Newcastle disease virus on cell survival and immune function in spleen of commercial layer chicks. Genes 11:1003. doi: 10.3390/genes11091003
*Zhang, J., R. M. Goto, A. Psifidi, M. P. Stevens, R. L. Taylor, Jr., and M. M. Miller. 2022. Research Note: MHCY haplotype and Campylobacter jejuni colonization in a (Line N x Line 61) x Line N backcross population. Poult. Sci. 101:101654 https://doi.org/10.1016/j.psj.2021.101654
*Zhang, J., R. M. Goto, C. F. Honaker, P. B. Siegel, R. L. Taylor, Jr., H. K. Parmentier, and M. M. Miller. 2022. Association of MHCY genotypes in lines of chickens divergently selected for high or low antibody response to sheep red blood cells. Poult. Sci. 101:101621 https://doi.org/10.1016/j.psj.2021.101621
Zhang, S., S. J. Lamont, and M. Persia. 2018. Differential growth performance and intestinal immune gene expression in diverse genetic lines of growing chickens fed a high concentration of supplemental phytase. J. Agricultural Sci.1-7.
Zhao R, Zuo Q, Yuan X, Jin K, Jin J, Ding Y, Zhang C, Li T, Jiang J, Li J, Zhang M, Shi X, Sun H, Zhang Y, Xu Q, Chang G, Zhao Z, Li B, Wu X, Zhang Y, Song J, Chen G, Li B. 2021. Production of viable chicken by allogeneic transplantation of primordial germ cells induced from somatic cells. Nat Commun. 12(1):2989. DOI: 10.1038/s41467-021-23242-5. PMID: 34017000; PMCID: PMC8138025.
Zhao, C.F., Li, X., Han, B., Qu, L.J., Liu, C.J., Song, J., Yang, N., Lian, L. 2020. Knockdown of the Meq gene in Marek's disease tumor cell line MSB1 might induce cell apoptosis and inhibit cell proliferation and invasion. J. Integrative Agriculture 19:2767-2774 doi.org/10.1016/S2095-3119(20)63321-4
Zuo Q, Jin J, Jin K, Sun C, Song J, Zhang Y, Chen G, Li B. 2019. Distinct roles of retinoic acid and BMP4 pathways in the formation of chicken primordial germ cells and spermatogonial stem cells. Food Funct. Nov 1;10(11):7152-7163. doi: 10.1039/c9fo01485c.
Zuo Q, Jin J, Jin K, Zhou J, Sun C, Song J, Chen G, Zhang Y, Li B. 2020. P53 and H3K4me2 activate N6-methylated LncPGCAT-1 to regulate primordial germ cell formation via MAPK signaling. J Cell Physiol. doi: 10.1002/jcp.29805.
Zuo Q, Jin K, Song J, Zhang Y, Chen, G. and Li B. 2018. Interaction of the primordial germ cell-specific protein C2EIP with PTCH2 directs differentiation of embryonic stem cells via HH signaling activation. Cell Death Dis. 9:497 doi:10.1038/s41419-018-0557-2
Zuo Q, Jin K, Song J, Zhang Y, Li B. 2018. Cloning, expression pattern analysis, and subcellular localization of Capra hircus SCD1 gene with production of transgenic mice. J Cell Biochem. Feb;119(2):2240-2247. doi: 10.1002/jcb.26386.
Zuo Q, Zhang, C., Jin K, Jing, J., Sun, C., Ahmed, M., Song J, Zhang Y, Chen, G. and Li B. 2018. NICD-mediated notch transduction regulates the different fate of chicken primordial germ cells and spermatogonial stem cells. Cell Biosci 8:40 https://doi.org/10.1186/s13578-018-0238-y
BOOK CHAPTERS (10)
Aggrey, S. E., H. Zhou, M. Tixier-Boichard and D. D. Rhoads (Eds). 2020. Advances in poultry genetics and genomics Burleigh Dodds Science Publishing ISBN:978-1-78676-324-2
*Alders, R., Costa, R., Gallardo, R.A., Sparks, N., Zhou, H. 2019. Smallholder Poultry: Contributions to Food and Nutrition Security. In: Ferranti, P., Berry, E.M., Anderson, J.R. (Eds.), Encyclopedia of Food Security and Sustainability, vol. 3, pp.292–298. Elsevier. ISBN: 9780128126875
*Alders, R., Costa, R., Gallardo, R.A., Sparks, N., Zhou, H., 2019. Smallholder Poultry: Leveraging for Sustainable Food and Nutrition Security. In: Ferranti, P., Berry, E.M., Anderson, J.R. (Eds.), Encyclopedia of Food Security and Sustainability, vol. 3, pp.340–346. Elsevier. ISBN: 9780128126875
Cheng, H. H. and S. J. Lamont. 2020. Genetics of disease resistance. Pages 90-108. In Diseases of Poultry, 14th ed. D. E. Swayne, M. Boulianne, C. M. Logue, L. R. McDougald, V. Nair, and D. L. Suarez, eds. Wiley-Blackwell, Hoboken.
Erf, G. F. Autoimmune diseases of poultry. 2021. Pages 437-455 in: Avian Immunology, 3rd edition. B. Kaspers., K. A. Schat, T. Göbel T., L. Vervelde, eds. Elsevier, Academic Press, San Diego, CA.
Erf, G. F., and I. C. Le Poole. 2019. Animal models. Pages 205-223 in Vitiligo, 2nd edition. M. Picardo, A. Taieb, eds. Springer, SPi Global.
Jarosinski, K. W. 2017. Interindividual Spread of Herpesviruses. Adv. Anat. Embryol. Cell Biol. 223:195-224. doi:https://doi.org/10.1007/978-3-319-53168-7_9.
*Lamont, S. J., J. C. M. Dekkers, A. Wolc, and H. Zhou, H. 2022. Immunogenetics and the mapping of immunological functions. Pages 277-297. In Avian Immunology, 3rd ed. B. Kaspers, K.A. Schat, T. Gobel, L. Vervelde, eds., Elsevier, London, San Diego, Cambridge, Oxford.
Pinard-van der Laan, M.-H., J. Kaufman, A. Psifidi, H. Zhou, M. Fife. 2020. Genetics and genomics of immunity and disease traits in poultry species. In S. E. Aggrey, H. Zhou, M. Tixier-Boichard and D. D. Rhoads (Eds). 2020 Advances in poultry genetics and genomics. Burleigh Dodds Science Publishing ISBN:978-1-78676-324
Webb, K. C., S. W. Henning, G. F. Erf, and I. C. Le Poole. 2019. Autoimmune pathology of vitiligo. Pages 285-301 in Vitiligo, 2nd edition. M. Picardo, A. Taieb, eds. Springer, SPi Global.
Technical Reports: 10
Abstracts: = 203
MS Theses: 5
PhD Dissertations: 14
Undergraduate Honors Theses: 3