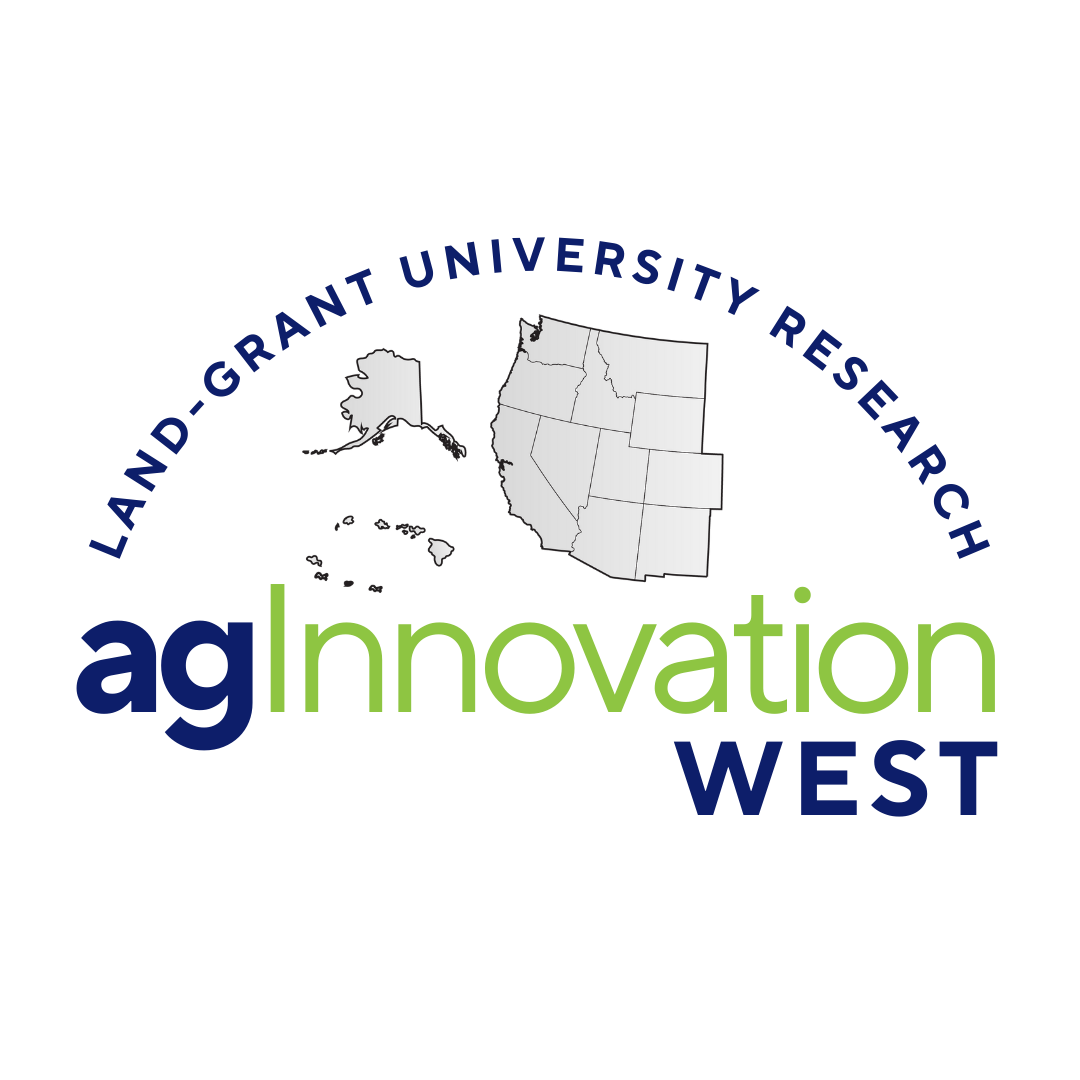
W5186: Variability, Adaptation and Management of Nematodes Impacting Crop Production and Trade
(Multistate Research Project)
Status: Active
W5186: Variability, Adaptation and Management of Nematodes Impacting Crop Production and Trade
Duration: 10/01/2023 to 09/30/2028
Administrative Advisor(s):
NIFA Reps:
Non-Technical Summary
Statement of Issues and Justification
The Need as Indicated by Stakeholders:
Estimates over the last 35 years indicate that plant parasitic nematodes cause 10-14% average annual yield losses among the world's major crops [1], and within the United States, there are losses ranging from minimal in some localities to as high as 50% in other areas [2, 3]. More recent surveys of nematode-associated crop losses indicate that these estimates remain at similar levels. In economic terms, these estimated annual crop losses translate to at least $8 billion in the United States and $80 - $157 billion worldwide [4-6]. These numbers are likely to be under-estimates since plant parasitic nematodes are belowground and invisible to the naked eye, and because the disease symptoms are often non-specific, growers may be unaware of the presence of these pests [4]. Therefore, in many production systems, incipient losses are probably missed. In addition, there are significant economic losses associated with the costs of nematicides. A recent cost estimate from a representative commercial potato farm in central Michigan, USA, indicated that nematicides can cost $1,000 or more an acre [7, 8]. The costs associated with nematode management include the price of the nematocidal products, the labor costs for application, and their efficacy [8]. In addition to the production costs incurred by nematode management, the presence of plant parasitic nematodes may be associated with trade embargos due to actual or suspected quarantine nematode infestations.
Increasingly, scientific evidence and public awareness have heightened concerns about environment quality, food quality, and human health and safety relative to pest management in agricultural production. The need for alternative and integrated nematode management has been propelled by the actions triggered by the Montreal Protocol and the Food Quality Protection Act (FQPA) of the 1990’s. The phase-out of methyl bromide and the loss of several highly effective organo-phosphate and carbamate nematicides has occurred over the last decade [9, 10]. In addition to methyl bromide, the use of fenamiphos and aldicarb have become severely restricted [11]. Moreover, the soil fumigant nematicide 1,3-dichloropropene (Telone II) is a B2 carcinogen, reviewed under FQPA and must be used under more rigorous restrictions. Together with another fumigant, metam-sodium, the fumigant nematicides have been identified by California EPA as the largest agricultural source of VOCs (volatile organic compounds) that contribute to air pollution by ground level ozone formation. US EPA Phase 2 labeling for all soil fumigants, implemented in 2012, establishes mandatory buffer zones surrounding treated fields that further restricts their use. More recently, safer nematicide chemistries have become available and can be tested as components of integrated nematode management programs [9]. Both locally and nationally, the agricultural community (our stakeholders) have a critical need for viable, sustainable alternatives to traditional chemical-based nematode control.
World travel and commerce have accelerated the dissemination of pest species, including plant parasitic nematodes. Climate change is contributing to enhanced disease severity in crops, and it is also leading to the northward migration of plant parasitic nematodes into new regions and agricultural systems [12, 13]. Development and application of new diagnostic protocols for accurate identification of nematode species are imperative for national and international regulatory and quarantine agencies [14]. For example, the presence of quarantine-status of cyst or root-knot nematodes in US potato production areas has led to restrictions on international trade and movement of potatoes within the country [15, 16].
The nematology community has repeatedly advocated the need for funding support focused on the basic and applied research required to advance agro-ecologically sustainable alternative management approaches and accurate nematode detection and diagnostic tools. Each member of this project is engaged with stakeholders (commodity boards, farm advisors, and industry experts), and a large driver of this research is the continued need from the various stakeholders for better nematode management tools. Outreach with commodity leaders, farm field days, and one-on-one meetings with growers have helped members devise the major milestones of the objectives. Our members of the grant with extension appointments will continue to gauge the stakeholder interest during these interactions. Building on advances made in the W-4186 project over the last 5 years, this proposed project renewal, called W-5186, addresses these needs directly for important groups of plant parasitic nematodes.
Importance of, and Consequences Without, the Work:
Cyst, root-knot, root-lesion and other nematode species included in this project are the most important groups of plant parasitic nematodes in the United States and globally [4]. Management of these nematodes in US agriculture has been largely via the application of broadly efficacious nematicides [17]. Nematicidal activity, especially of soil fumigants, is generally non-discriminating between nematode species and genera. Historically, growers would “spray and pray” that the chemicals they used would solve the problems with nematodes and other soil borne pathogens. But this approach also sterilized the soil of beneficial organisms [18]. A more targeted approach towards nematode control would be beneficial to soil health, and such an approach is now possible in the “omics” age. An understanding of the genetic variability and adaptation potential among nematodes will be important factors for the development of effective and target-based agrichemicals [9]. Additionally, desirable alternative nematode management approaches involve combinations of crop rotation, host plant resistance, cultural manipulations, and biological control. All of these tactics may have specific genotype-level interactions with nematodes and are influenced by production practices and environmental conditions. Preliminary evidence indicates that the promising new nematicide products show differential efficacy among main nematode groups (e.g., root-knot versus cyst nematodes). Hence, variability and adaptation in nematode populations must be considered to successfully develop and deploy new management strategies.
This multistate project was initiated because the membership recognized the increasing importance of characterizing the genetic variation in nematode populations and its influence on the success of alternative management strategies. For example, many years of research went into the development of cyst-nematode resistant soybeans and root-knot nematode resistant tomatoes, cotton, and potatoes but in some of these cases rapid selection of resistance-breaking nematode isolates has eroded their management efficacy [19]. The biological processes in nematodes are complex and ultimately will influence the development of effective management strategies. The multiple generations and interactions between nematode, host plant and environment can finally be addressed using a combination of applied and basic research. Although the effects of climate change will not be studied in this proposed research as a specific driver of nematode genetic variability and adaptation, we cannot ignore the contemporary changes in climates that may ultimately affect plant parasitic nematodes and their hosts. As a result, this multistate effort will provide a snapshot of the major plant parasitic nematodes and their current genetic repertoire that will no doubt become a resource for future genetic adaptation studies. A renewal of the project as requested herein is critical for continuing the research and application required to meet the overall project goals outlined below.
Research conducted under the current W-4186 multistate project has provided considerable evidence that genetic and parasitic variability in nematodes is important to management populations within production systems. Results from current work indicate that genetic variability and adaptation potential in nematode populations are responsible for the aberrant and inconsistent results of many experiments assessing resistance, crop rotations, host ranges, cover/trap cropping, biological control, and new nematicides. The plasticity of nematode responses to environmental factors such as the microbiome, soil structure, temperature/moisture, host nutrient status, and other biotic/abiotic factors stems from genetic variability. Greater understanding of nematode genetic response and adaptation to biotic/abiotic factors will be important in optimizing the design of cultural management tactics such as manipulations of planting and harvest times, wet or dry fallow, and soil solarization. The potential for invasive nematode pests to establish in our agricultural production systems also can be better determined from studies on genetic responses and adaptation to local environments.
Knowledge gained from our focus on cyst, root-knot and other nematodes of agricultural significance will be applied and tested between nematode groups within the project matrix (see Table 1 in Attachments). This will strengthen the overall scientific scope of the research activities and will broaden the impact of the findings to benefit agriculture in multiple states.
Strength of the Group in Technical Feasibility of the Research:
Recent advances in molecular and genetic methodologies and knowledge will facilitate the study of nematode genetic variability and adaptation. Access to genomes will promote diagnostic protocols with much greater resolution than has been possible. Some of these protocols have been developed and tested under the current project. For example, shared root-knot and cyst populations led to characterization of a mitochondrial cytochrome oxidase I (COI) gene that promises to alleviate current ambiguities in molecular species identification within these difficult-to-identify genera [20, 21]. In addition, several diagnostic tools for Meloidogyne chitwoodi were developed under the current project, including markers for a virulent, resistance-breaking pathotype [22]. Rapid advances in DNA-based diagnostics and genetic analyses generated by the current and other projects and their coupling to on-line databases and knowledge-based systems will assist in information transfer to user groups in the relevant agricultural communities.
This group of scientists has strong expertise across nematodes and applying basic and applied science approaches. Three groups of nematodes are the primary focus for this project: Group I - The warm-temperature root-knot species (Meloidogyne incognita, M. javanica, M. arenaria, M. enterolobii); Group II - The temperate root-knot species (M. chitwoodi and M. hapla); Group III - The cyst species (Globodera, Heterodera). These nematodes are the subject of research efforts in the designated participating states. Within these groups, the quarantined nematodes in Globodera (G. pallida) and Meloidogyne (M. enterolobii) are worked on in the states and labs that are permitted to work with such nematodes (Table 1).
The project participants share strong common interests that will provide the central focus for both project members and other collaborators. In addition, parallel studies will be made by some participants on other parasitic nematodes, such as reniform nematode (Rotylenchulus reniformis), sting nematode (Belonolaimus longicaudatus), and root-lesion nematodes (Pratylenchus spp.). This will maximize both the scientific scope of the project and its multi-state impact in agricultural and natural ecosystems.
Characterizing genetic variability – requisite for novel management strategies:
The unifying theme of this project is that genetic variability is a critical biological feature that complicates management and enhances the pest status of nematode species and populations. W-4186 participants and others have been documenting the extent of genetic variability within populations and the agro-environmental factors that influence it. Rapid developments in genomic techniques and their application through this project will continue to increase our understanding of the genetic processes involved. Failure of current nematode management practices, such as the breakdown of resistance, can be resolved through greater understanding of the underlying genetic and biological processes in parasitic nematode populations vis a vis management.
Genetic variability can impact both the effectiveness and longevity of alternative nematode-management strategies based on host plant resistance, crop rotation, cultural manipulations, and biological control. Therefore, continuing the knowledge development in these systems should provide rational guidance for the design and development of nematode management strategies. The project focus is on understanding nematode variability and adaptation, such that it can be identified, characterized, and managed or manipulated to benefit agricultural production systems. This requires research on the genetic variability and gene frequencies, including aspects of stability and adaptability, of host range, response to resistance, response to environmental conditions, biological processes (e.g. fecundity) and morphology. This approach is being complemented and aided by the development of markers to identify variability by molecular, histochemical, and morphological polymorphisms. The development of molecular techniques with greater efficiency, predictability and ease of use will expedite nematode genetic analyses and design of management strategies.
Current and previous work under W-4186 has allowed participants to make advances on these research goals. However, this work cannot be considered “complete,” and pressure for alternatives to existing nematicides has increased. Regarding our objectives, it is exciting that the arsenal of established and new tools used to address our applied research questions is increasing rapidly.
Below are four key nematode genetic variability-based considerations that are central to the development and deployment of alternative management strategies as proposed under this multistate project:
- Host plant resistance – The genetic composition of nematode populations is changed by the selection pressure imposed by growing resistant cultivars. The changes include shifts in species composition and shifts in presence and frequency of nematode virulence alleles matching specific resistance genes in crop cultivars [23-26]. Similar potential shifts may occur in response to nematode-resistant trap crops [27]. Little is known of the existing frequency of virulence alleles, the frequency with which new alleles are generated, or the underlying mechanisms that regulate changes in genetic variability in root-knot, cyst and other nematode populations. New genomic resources have provided information about nematode populations, the genes underlying nematode parasitism, and the molecular pathways that link the nematode and host plant [28, 29]. In addition, as more sources of resistance are bred into cultivars, knowledge of gene frequency and stability effects assumes greater importance in determining the direction and requirements of breeding for nematode resistance, and the effective long-term deployment of available resistant cultivars [27, 30-33].
- Host range for rotations and cover-cropping - The host ranges of important nematode species have been defined within general limits, but the extent of variability in host range among populations within species is not well-characterized. Much of this host range information has been compiled from numerous tests and observations based on non-standardized host testing procedures, and in most cases with only one or a few isolates per species. Standardized conditions are needed to determine whether differences are due to variability in nematode populations or to differences in susceptibility in the plant lines used.
- Cultural and Biological controls - Genetic variability in nematodes for responses to temperature and moisture has been demonstrated, but little is known about underlying mechanisms or stability. Soil amendments (compost, green manures, various bio-products) show promise for nematode suppression and improving soil health in some systems and require further study [34-37]. Potential biological control agents of cyst and root-knot nematodes are known to have specific host ranges among target nematode species [38]. Such specificity may be controlled by genetic factors, and thus, variability in Meloidogyne may influence the potential of biological control agents.
- Nematicide controls – Several new nematicides have been shown by our current group and others to have high nematicidal efficacy and are in final testing phases to support new use registrations. Active ingredients include fluopyram, fluensulfone, fluazaindolizine, spirotetramat, and cyclobutrifluram, among others. They represent important tools for integration into nematode management programs, including use as seed treatments and in combinations with bio-products and biocontrol organisms. However, nematode responses to such new nematicides may be influenced by nematode genetic factors. Research efforts in this hatch project include investigating the efficacy of new nematicide treatments while also looking at the effects of genetic variability that may underlie the efficacy.
Advantages of a Multistate Effort:
Under the current project, research to apply emerging methodologies to obtain knowledge of the variability and adaptation potential in nematode populations is in various stages of advancement. The W-4186 membership proposes to continue and extend these efforts to identify and characterize the variability in important nematode pests. The participants share research interests on primary nematode pests and bring complementary expertise and resources to the project.
The group is a cross section of nematologists that span both basic and applied research on nematodes of major agronomic importance. Our research expertise will help to determine gene frequencies, genetic stability, and adaptation and fitness, such that genetic variability can be managed and manipulated in agricultural production systems by appropriate alternative management strategies. The diversity in cropping systems and rank of importance of nematode groups among participating states clearly provides opportunities for conducting meaningful collaborative research on major nematode pathogens. The participants utilize the opportunity to collaborate in ways that enhance the benefits accrued from the research, as opposed to pursuing individual projects within limited geographic boundaries. For example, the warm climate root-knot species will be studied by participants from a majority of the participating institutions (see Attachment Table 1) – a group effort that will pay large dividends in understanding nematode variability relative to management.
Despite retirements of key members in the W-4186 working group, this multistate project has attracted several new members. These members include nematologists asking very basic research questions about variability in nematode biology, effectors, and host resistance. In addition, several new members also have extension appointments. Their goal in the multistate effort will not only be to contribute to the excellent scientific research, but to also enhance the group’s outreach to growers and stakeholders, which is a focus of this project.
This team approach enables a pooling of scientific expertise and resources to maximize the amount and quality of the information that can be generated. This multistate project is unique in that it provides a necessary forum for rapid scientific advancement in aspects of both basic and applied research directed toward nematode diagnostics and development of management strategies. For example, some participants have programs devoted to molecular research on nematodes, which can be applied across all states for diagnosis and to assess nematode genetic variability. This project has ongoing molecular-based programs in some states (e.g. California, Hawaii, Indiana, Nebraska, Florida, Washington) that can facilitate research by other participant states. In turn, those states focusing on phenotypic differences in nematode populations can provide nematode populations and isolates for molecular analysis. This coordinated approach minimizes unnecessary duplication of research programs, and provides fertile opportunities for a seamless, interactive approach to advancing nematode management. The end result is that the project has enhanced the quality and applicability of the research findings across geographic locations and agricultural production systems.
Likely Impacts of Work:
The stakeholders (agricultural community) will be positively impacted by application of the project findings in expediting the development of new, environmentally benign management strategies to minimize losses due to nematodes. This in turn should help boost the international competitiveness of our agricultural production systems. The project will also benefit the diagnosis and response process required when invasive nematode pests are suspected or found in production fields or in traded agricultural products.
Related, Current and Previous Work
Related, Current and Previous Work: Accomplishments (2018-2023):
A full listing of the 224 research publications of the W-4186 project from 2018-2022 and Annual Progress Reports for W-4186 are available through the NIMSS site for more in-depth descriptions of related work progress. The following is a summary of examples that highlight areas of significant impact in addressing project goals, with comments concerning the need for additional research.
Overall, the project scientists have been very productive in efforts to develop improved techniques for nematode diagnostics, understanding of nematode diversity and genetic variability, processes of nematode fitness and adaptation, and the incorporation of this knowledge into the design and analysis of improved integrated nematode and soil health management strategies. These findings directly benefit all participating states and more broadly other states whose crop production systems are compromised by cyst, root-knot and other nematode infestations and invasions. Moreover, many of the accomplishments have resulted from our close collaboration within W-4186, which has allowed members to share knowledge, techniques and materials.
Under Objective 1 (characterize genetic and biological variation in nematodes relevant to crop production and trade), nematode (a)virulence and nematode population genetic diversity were elucidated in several important nematodes. For example, in Idaho, a project to investigate the genetic diversity found in Globodera spp. was initiated. Understanding genetic diversity will allow the Idaho scientists to find appropriate sources of resistance to encompass this nematode population diversity and which will allow for the development of resistant potatoes to a broad swathe of cyst nematode populations. Variation in virulence and pathogenicity of G. pallida is known to occur, and different populations are characterized into pathotypes based on their ability to reproduce on a set of potato genotypes with known resistance genes. The pathotypes of 10 populations from Peru were characterized using a set of potato differential lines containing different resistance genes. According to the pathotype scheme, the Idaho G. pallida population has been identified as a pathotype 2/3 (Pa2/3) [39]. Pathotypes with different virulence is a constraint towards the development of fully resistant potato varieties. Continuous use of resistance has selected for populations with increased virulence. Resistance derived from Solanum tuberosum spp. andigena is more readily overcome than resistance from S. vernei [40, 41] and may select for more virulent populations [42], which can be studied at the genomic/transcriptomic level. Moreover, recent evidence suggests individuals within a cyst may have different virulence characteristics and these individuals may contribute to erosion of resistance. Understanding genetic factors that contribute to virulence would help to maintain durability of resistance genes.
Studies on root-knot nematode genomes/transcriptomes have led to insights on the genetic factors controlling nematode parasitism and virulence. Transcriptome analyses of M. chitwoodi was performed to identify nematode parasitism genes (i.e. effector genes) that facilitate infections of potato [43]. Comparing this list of M. chitwoodi effector genes to other root-knot nematode effector genes indicated that M. chitwoodi contains many pioneer genes. Other accomplishments within this project Objective include high-quality reference genomes for two pathotypes of another root-knot nematode called M. javanica. The genetic variation between these M. javanica strains will help elucidate how the nematode has evolved to avoid immune detection in resistant plants. Altogether, the information will be key in understanding variations in plant-nematode interactions, how nematodes manipulate plant responses, and changes in nematode populations that contribute to their virulence on previously resistant plants.
Molecular approaches to nematode identification within and between species have also been advanced under this objective. Molecular techniques have been developed to differentiate nematode species, races and populations [14, 44, 45]. Some of the major accomplishments include simple molecular assays for the identification of M. chitwoodi, including specific races/pathotypes of this nematode [46]. This information will help growers make management decisions in potato production. Other major accomplishments include advances in nematode identification by molecular ‘barcoding’ approaches (e.g., [47, 48]). Continued efforts are being made to refine and define the conditions and limitations of DNA barcoding using the COI mitochondrial gene. A DNA barcoding reference database called NemaTaxa was developed as a reference data base of nematodes in US agriculture [47]. Work from Nebraska has supported a field device for rapid identification of cyst nematode juveniles, which can accelerate the time of species identification and reduce expenses. Continued work in detecting and diagnosing the presence of plant parasitic nematodes provides valuable management insights to regional agricultural communities.
Under Objective 2 (determine nematode adaptation processes to hosts, agro-ecosystems and environments), several systems have been analyzed, revealing high levels of nematode adaptation to parasitic ability on resistant host plants, alternative hosts (weeds), seasonal climatic differences, co-infection of plants by fungal pathogens, and soil conditions. Variation in parasitic ability among root-knot nematodes was described for species parasitizing grain legumes such as cowpea and Lima bean [49], common beans, cotton, tomato, and potato cultivars and wild relatives.
Focusing on host resistance to nematodes, W-4186 researchers found resistance markers against M. hapla in the carrot cultivar “Homs.” Although the M. hapla avirulence gene(s) in this interaction need to be identified, the new knowledge on natural host resistance traits can be adopted by plant breeding programs to benefit growers by producing nematode resistant carrot varieties. W-4186 scientists at UC Davis found that the host-range of the tomato Mi-resistance breaking M. javanica strain seems to be different from its progenitor strain. Resistance-breaking nematodes are becoming increasingly problematic to growers, and these studies in Davis demonstrate the need for broad-based forms of resistance for use in tomato. Moving to potato, members of the project continue to screen potato clones that are being developed to determine their resistance level to G. pallida [50, 51]. In addition to development of resistance through traditional breeding efforts, a project was initiated to understand plant defenses of the trap crop Solanum sisymbriifolium [52]. This will lead to new knowledge on natural host resistance traits to manage nematodes in field and vegetable crops.
Understanding nematode parasitic variability (PV) in diverse cropping systems and developing integrated soil health and nematode management strategies therein has been limited by lack of decision-making tools and models that can translate basic and complex science into practical applications. Therefore, one accomplishment of the W-4186 project focused on modeling the PV of soybean cyst nematodes and Northern root-knot nematodes in various soil conditions [53]. Field studies in Michigan showed that soil texture influences H. glycines population density [54] and M. hapla reproductive potential, with adaptation to mineral or muck soils [53-55]. It was shown that there are clusters and specificity of PV in M. hapla populations isolated from mineral and muck soils with disturbed and degraded soil health conditions. Populations from degraded mineral soils had significantly higher PV than those from disturbed mineral and disturbed and degraded muck soils, and PV within the populations from the degraded mineral soils varied [56]. The information derived from this research has been utilized to develop models of nematode populations in various soil types, and in the future, it will help scientists understand how nematodes adapt to changes in cultural practices and in environmental factors.
Nematodes can also adapt to new environments and a major issue is that plant parasitic nematodes can be introduced into new areas where they adapt and infect important crop plants. Identifying such infestations is critical; potato cyst nematodes G. rostochiensis and G. pallida are significant threats to potato production, and a comprehensive detection survey documented absence of G. rostochiensis but confirmed the presence of G. pallida in Idaho. Project members also determined that a new ring nematode species, Mesocriconema nebraskense, endemic to the Central Plains has adapted to cause serious damage to bent grass on numerous golf courses in New Mexico. Lastly, within this objective, new nematode records have been reported. There was a first report of Ditylenchus dipsaci from alfalfa in New Mexico [57]. At a value of $125 million, New Mexico alfalfa hay production is ranked as the fourth largest cash commodity in the state. It is a critical agricultural commodity that supports the two top agricultural industries, dairy and cattle production, in New Mexico. In addition, the alfalfa cyst nematode Heterodera medicaginis was found in samples from Kansas, Montana, and Utah. This is the first time that the alfalfa cyst nematode has been found in North America [58]. The cactus cyst nematode, Cactodera cacti, was found in Idaho and Colorado, a first for these two states [59]. There was a first report of Cactodera milleri from Quinoa fields in Colorado [60]. First reports are the starting point in obtaining nematodes and understanding how nematodes adapt to new environments.
Another aspect of Objective 2 has been to look at nematodes in terms of adaption to potential new hosts. For example, the University of Hawaii has surveyed native plants in areas around University of Hawaii Campus for susceptibility to root-knot and reniform nematodes. For reniform nematodes, the native plant Ipomoea appeared to be a good host, but most native plants seemed to be poor hosts to these nematodes. The observed variation of resistance in seedlings of native plants may reflect the variation in the seed germplasm rather than a genetic variation in the tested nematode species.
Under Objective 3 (develop and assess nematode management strategies in agricultural production systems), root-knot, cyst, and other nematode management systems have been studied, developed, or improved for multiple cropping systems and production areas. Several examples highlight nematode-cropping systems that have been and will continue to be a project focus, targeting the most damaging nematode problems.
In Idaho, cropping systems using a combination of resistant varieties and trap crops are being developed for control of G. pallida. Toxic metabolites from the trap crop S. sisymbriifolium are being purified and assessed for development of a ‘smart’ nematicide against potato cyst nematodes [61]. There is a continued need for new safe, effective, and inexpensive nematode control options in potato and beyond.
Work on root-knot nematode management has included host plant resistance studies. In carrot, high resistance was found in carrot lines from Brasilia, South Africa and India to Meloidogyne incognita and M. javanica. Resistance to M. hapla in a carrot entry from Syria was found effective against nine out of ten different M. hapla isolates collected from different cropping systems and agro-ecologies. In cowpea, analysis was continued to study the genome-level organization of root-knot nematode resistance traits, and the study identified resistance loci on four of the 11 cowpea chromosomes. Work in Southern US has shown the new cotton cultivars with root-knot and reniform resistance are doubling cotton yields in fields infested with these nematodes.
Nematicides offer an effective means of nematode management, but as companies develop new nematicides, it is important to investigate the effectiveness of their formulations or their application methods. Working directly with local commercial producers to evaluate new nematicides in locally relevant cropping systems aids growers in making informed management decisions. For example, investigations into the seasonality of M. incognita populations in drip-irrigated, wine-grape vineyards in southern New Mexico has aided in determining the most effective timing for nematicide applications. For management of reniform nematode, allyl isothiocyanate holds promise as a preplant treatment. However, the reniform nematode reduced susceptible cotton yield potential in half even with nematicide applications. Numerous studies were also performed on M. chitwoodi, allowing for specific recommendations be made to potato growers, such as using Velum Prime with in-furrow application rather than oxamyl [62].
Developing predictive tools to aid in nematode management has also formed part of the project effort, including improving a fertilizer-use efficiency model by incorporation of concurrent changes in nematode community population density, plant growth and soil environment [63], and use of spatial information regarding nutsedge and M. incognita populations as predictors in modeling the nematode/nutsedge pest complex [64].
These are selected examples of many advances in nematode management being made under W-4186 participation in which the collective knowledge gained in one system is being applied to other systems. These nematode management systems are at various stages of development and require additional study and modification under a project renewal.
Areas Needing Further Investigation:
Efforts under W-4186 have laid the foundations for integrating new approaches to nematode management that consider the genetic variability in nematode species. Areas needing further investigation include using DNA sequence-based technologies and advances in molecular protocols for the ease of nematode identification. It is imperative to understand the genetic variability of these complex organisms in the context of diverse soil ecosystems. We must also continue to study the efficacy of nematicides on different species and on populations within a species so that growers are aware of the best management practices for their region. Lastly, another area that need further investigation is studying the efficiency of crop rotations on species and their adaption to crops under current climatic conditions. We must take advantage of new resources and apply them to the nematode-crop systems and invasive nematode threats that the project members are familiar with and for which representative samples are available. A new generation of novel, highly efficacious and less toxic nematicides has become available for testing, and these need to be researched and adapted into our focus nematode management systems. Farmers’ limited knowledge of the cause-and-effect relationships between agricultural practices, soil health, and nematodes adversely affect crop yield. Therefore, this multistate project has expanded to increase the number of members with extension programs. This will help promote our research efforts and contribute to higher rates of adoption of 'best' practices.
Other Regional Projects: There are three multistate projects that are regional in scope and with minimum overlap with the current multistate proposed project.
NC-1997: Practical Management of Nematodes on Corn, Soybeans and Other Crops of Regional Importance. This project is mostly focused on the US Midwest.
S1092: Biology, ecology, and management of emerging nematode threats in the Southern United States. This multistate project focuses primarily on nematode threats to agriculture in the Southern region. It is much more limited in regional scope. It emphasizes invasive nematodes and infestations of endemic nematodes on new crops. Thus, while portions of its goals are similar to our proposal, it is limited to a defined geographical region and focuses on approaches to mitigate emerging nematode problems.
NE-2140: Sustainable Management of Nematodes in Plant and Soil Health Systems. This project is focused on developing ecologically based nematode control practices rather than understanding the genetic variability of the host and nematode parasites that our multistate project (here) does.
Objectives
-
Characterize genetic and biological variation in nematodes relevant to crop production and trade.
-
Determine nematode adaptation processes to hosts, agro-ecosystems and environments.
-
Develop and assess nematode management strategies in agricultural production systems.
Methods
The research focus of each participating state is given as a matrix in Table 1. The nematode group and main crop areas are indicated, together with the procedural research emphasis, as covered under the three objectives. Research coordination will ensure that standardized procedures are generally followed, and research findings can be compared within and across nematode, plant and subject area categories. Plant germplasm (accessions, breeding lines, cultivars), nematode isolates of representative species and populations with GPS location data, and DNA primer and other sequence information for markers will be available among the participants.
Experimental protocols and procedures are structured according to the main target nematode groups under the three objectives. All objectives have a common focus of addressing critical aspects of nematode variability, adaptation, and management, with the application and extension of the findings tailored to meet individual state and sub-regional needs, in addition to those at the project-wide level. While findings under all objectives are considered important to each state, duplication will be avoided by partitioning individual state research activities. This structure will also ensure that all phases of the objectives are being met, while addressing local state needs, a structure that has worked well in the W-4186 project and its previous versions. Following are examples: tropical root-knot and reniform nematodes will be considered in Alabama, Arkansas, California, Louisiana, Florida, Maryland (USDA-ARS), and North Carolina. Cool-climate root-knot species (Meloidogyne chitwoodi and M. hapla) will be studied in detail in California, Michigan, Nebraska, New Mexico, and Washington. M. hapla will also be studied in Florida, where it is commonly found in strawberry fields during winter. Cyst nematodes will be studied in Indiana, Florida, Hawaii, Michigan, Arkansas, and Nebraska. Idaho will consider impacts specifically of G. pallida on potato production systems.
Objective 1:
This objective, to characterize genetic and biological variation in nematodes, requires diverse species and populations of nematodes. First, phenotypic assessments will be made on isolates of nematode populations that are collected from field sites. The assessments will include nematode host range and virulence/avirulence on known resistant crops. This information will provide important background considerations for the level and nature of any phenotypic differences detected in comparative experiments. In many programs, investigators either have a partial or nearly complete collection of live cultures for genetic comparisons. For example, for Group I warm-climate root-knot populations, 40 isolates have been assembled at California-Riverside and a collection of group II cool-climate root-knot nematodes M. chitwoodi and M. hapla isolates are maintained and expanded in Washington. For cyst nematodes, numerous populations of H. glycines are being cultured in Michigan and Nebraska, and a collection of H. schachtii and H. cruciferae geographic and host-selected isolates is under culture in Hawaii. The Idaho population of G. pallida is being cultured and maintained in Idaho, and additional populations from South America and Europe are being assembled. Work in Michigan applying various models that identify the soil environment in which the nematodes exist and how, will help W-5186 members relate soil health to nematode populations across the study areas. Based on the outcomes, Michigan will establish nematode cultures for testing parasitic variability for M. hapla and a database accessible to all collaborators.
In this objective, the scientists are taking comparative genomics and transcriptomic approaches to understand the genetic variability of species/populations. For example, UC Davis plans to sequence the genome of M. floridensis, a quarantine pathogen, and WSU will be performing transcriptome analysis of M. chitwoodi gland cells. The scientists within the hatch project have previously published nematode genome and transcriptome datasets; they will continue to follow the standard, published methods for these analyses [28,43]. The availability of diagnostic tools for species and pathotypes within a species will facilitate the development of alternative management strategies, including the identification of appropriate rotation crops.
Members of W-5186 are in a uniquely advantageous position to develop rapid and robust molecular diagnostic tools for simultaneous analysis of multiple nematode species. W-5186 will play a central role in the development of new markers and identification assays based on genetic variations within and between species. It necessitates a team approach due to the need to identify multiple species/or haplotypes from multiple geographic regions, coupled with the testing of multiple genetic markers. Different states will focus on particular species in a collaborative framework: e.g., M. incognita populations from states, including Alabama, Arkansas, California, Indiana, and New Mexico; M. chitwoodi and M. hapla in the potato-small-grain-alfalfa systems of Washington and Idaho; H. schachtii, H. glycines, and H. cruciferae from California, Hawaii, Michigan and Nebraska. Excitingly, development of diagnostic systems that feature the simultaneous analysis of multiple species based on DNA extracted from soil provides new opportunities. Members of the project will use mtDNA, ribosomal DNA, and nuclear DNA sequence and markers [66, 67] for initiating race/pathotype studies. Comparative genomics will also help to identify polymorphic DNA regions for marker development. Methods for such development of race specific root-knot nematode markers have been published [68] and can serve as a guide for future studies. In the future, this level of analysis will no doubt be required for phytosanitary certification. As costs of nematode surveys and identifications are increasing with the demands for greater specificity, high-throughput approaches to nematode diagnostics will be useful.
W5186 is uniquely positioned to address major regulatory and emerging nematode pest issues. Several programs are focused on defining species boundaries and developing rapid identification methods, both from a regulatory perspective and a management concern. For example, as soybean production moves west across the Great Plains, soybean cyst nematode is expanding its western boundaries into dry bean, (an SCN host) as well as sugar beet and alfalfa production regions. With an overlapping distribution with sugar beet and alfalfa cyst nematodes, differentiation of these three closely related species becomes increasingly important. To enhance the ability of W5186 to deal with potentially regulated nematodes, we will receive support from Dr. Sergei Subbotin, a nematologist with the California Department of Food and Agriculture, who has extensive experience in identifying nematodes (see letter of support).
W5186 members are developing molecular and AI assisted identification protocols. Although specific methods vary by research group, one approach is for nematode classification is to use captured images of different, known nematodes, which are used to train and validate machine learning algorithms. The image preprocessing and analysis pipeline can be developed in an open-source programming language like Python. The collection of nematode images can be shuffled and split into training and test sets. A convolution neural network (CNN), a type of deep learning algorithm which takes raw images as an input file, can be used to train the model. There are usually three types of layers in a typical CNN architecture- convolutional layers, max-pooling layers, and fully connected layers. The TensorFlow framework in Python can be used to implement deep learning algorithms. In brief, the CNN architecture can be comprised of four convolutional layers, two max-pooling layers, and three fully connected layers. 3 x 3 and 2 x 2 striding filters can be used for convolutional and max-pooling layers, respectively. ‘relu’ activation function will be applied after convolutional layers. The Softmax activation function, which calculates the relative probabilities, will be used in the last layer. The model with the trained parameters will then be tested on the previously unseen dataset, also called the test set. Evaluation metrics like accuracy, sensitivity, and specificity will be calculated to assess the model performance. The model that can classify each nematode genera with high accuracy will be selected for future use.
Using AI and molecular diagnostics will facilitate W5186 scientist(s) dealing with another nematode challenging regulatory decisions: Xiphinema americanum-group, a complex of 60 putative species with regulatory and quarantine status in North America and European Union respectively (EPPO, 2018) due to the ability of some species to transmit plant viruses in the genus Nepovirus. Although evidence suggests that only seven members of the X. americanum group transmit viruses, difficulties in discrimination and potential vectoring capabilities, US trading partners take a conservative approach in considering all 60 members of the group as a regulatory concern.
One of the exciting aspects of looking at genetic variation in plant parasitic nematodes is its potential usefulness in developing novel biotechnology for crop protection [69, 70]. Genetic variability in nematodes will be leveraged for generating transgenic resistant plants for future use. For example, members will study nematode parasitism gene diversity for the identification of nematode genes that could be specifically targeted for silencing by a process called RNA interference (RNAi) [71]. Parasitism genes (namely effectors encoding nematode secreted proteins) are usually genes whose inactivation/targeting impair the success of parasitism. Several members of W-5186 (Washington, California, Indiana, Florida, Maryland [USDA], and Arkansas) are in the unique position in having the knowledge and expertise in using sequencing and molecular approaches that leverage genetic diversity between parasitic and non-parasitic nematode in order to develop targeted nematode controls using biotechnology, including RNAi.
One important way to control Meloidogyne and other plant parasitic nematodes is the deployment of nematicidal compounds that are either natural or synthetic in origin. The use of these compounds may enhance the sustainability of planting nematode-resistant crop varieties by interfering with the ability of nematode populations to evolve a resistance-breaking phenotype. However, to know whether this will indeed be the case, we need a better understanding of the genetic and phenotypic variation in their populations with regards to their responses to natural and synthetic nematicidal compounds and how populations may respond evolutionarily to the deployment of such compounds. Our goal is to understand patterns of phenotypic and genome-wide genetic variation in nematode responses to natural and synthetic nematicidal compounds such as glucosinolates and their isothiocyanate derivatives, glycoalkaloids, and other compounds. The diversity of root-knot and cyst nematode populations will be useful. The cyst nematodes are excellent "model" nematode systems for genetic studies because they reproduce sexually. Conversely, many root-knot species are parthenogenetic (asexual). Their genetics will be studied using isofemale or single descent lineages to track inheritance, variability and adaptation, and via Mendelian approaches using species that can reproduce sexually, such as M. hapla and M. chitwoodi. The knowledge of costly trade-offs, for example by diminishing the success of toxin-insensitive nematodes in infecting a variety of potential host plants, will be key for developing new management approaches and minimizing loss of crop yield.
Objective 2:
There is a need for a clear understanding of environmental pressures and parameters which contribute to genetic and biological variation so that effective control strategies can be implemented. Phenotypic characterization and genetic markers developed under Objective 1 will provide the basis of understanding the nematode adaptation processes in response to diverse hosts, geographic (climatic) and soil (abiotic) environments. The goal of Objective 2 is for W5186 scientists to link differential responses to host and/or environmental conditions to the genetic variations identified in Objective 1. The idea is to link genetic variability to nematode fitness on different plants and in different environments. For example, effects of non-agronomic hosts on nematode fitness will be examined, such as studies on the effects of weed hosts on root-knot nematodes in cropping systems, which will be expanded to include weed populations from other geographic areas to better understand the implications of population adaptation. Participants also will include comparisons with invasive root-knot nematode species, such as M. enterolobii (Group I), which have heightened aggressiveness and broad host range. Adaptation and fitness also will be studied by imposing continuous selection for virulence in wild-type populations. Changes in reproductive capacity will be assessed to measure virulence frequencies and genetic stability of virulence. Tests of selected isolates will be made on resistant or nonhost plants other than those used to impose the original selection pressure. For example, studies of resistance breaking populations of M. javanica [72] and M. chitwoodi [73] will be conducted to investigate how a genetic adaptation to plant resistance may influence the nematode’s host range and determine whether there is stability of virulence with and without host selection pressure.
Some of the questions to be addressed are how nematode populations respond to changes in the hosts. Assays of (a)virulence responses to resistant lines and cultivars and of host range will be made under greenhouse and controlled environment conditions using well-established experimental procedures. The host range and virulence testing for the root-knot species (Groups I and II) will include standard sets of host differential plants and also differential cultivars and crop plants applicable to their local cropping systems, e.g., on potatoes in the Pacific Northwest. A specific example of the future research under this project is based on a recent survey which detected resistance-breaking isolates of group I nematodes (M. incognita and M. javanica) in 27 out of 30 tomato fields exhibiting symptoms typical of nematode infection throughout California [65]. Comparative genomics will be used to identify genetic factors that contribute to resistance outbreaks in tomato fields. Host range assays will be made under greenhouse and controlled environmental conditions. The genetic factors that contribute to a potential fitness cost of virulence will also be investigated. In addition to root-knot nematodes, Heterodera, Globodera and other nematode populations will be typed phenotypically for (a)virulence with respect to resistance genes from different crop plant species and close wild relatives, for host range, and for biological traits including responses to abiotic soil conditions. In all cases, the genomic data will be used to develop rapid reliable methods to identify resistance-breaking nematode isolates from fields.
A combination of controlled, large field scale studies will be conducted to understand nematode adaptation for nematodes such as M. incognita, M. javanica, M. hapla, H. glycines and R. reniformis, in cropping systems. We will evaluate survival and reproduction of M. javanica, H. glycines and R. reniformis, and potential to cause damage to their hosts under different conditions.
Quantifying nematode adaptability to changes in soil-nutrient environments will be extended as a major emphasis of work in Michigan, enhancing understanding of possible factors contributing to nematode adaptations. For example, in order to inform potential mechanisms of parasitic variability in M. hapla, we will explore links or variations in biophysicochemical conditions across the clusters of parasitic variability [73] and data to be generated under Objective 1. Associations and links to parasitic variability will be identified using proteomic and genetic approaches for M. incognita, M. javanica, M. hapla, H. glycines, and R. reniformis [74, 75]. Second tier follow up experiments will determine the implications of what this variation does and could play in nematode adaptation to changes in cultural practices and in environmental factors. In addition, we will continue with our long-term study on H. glycines establishment subjected to typical Midwest agricultural practices [76] and effects of agricultural practices on nematodes soil health and nutrient cycling. We will apply a combination of the concepts of the “Soil Food Web (SFW)”, “Fertilizer Use Efficiency (FUE),” and “Integrated Production Efficiency” models as diagnostic and decision-making tools to describe the experimental soil conditions and/or sustainability of the outcomes [56, 74, 76-78].
Objective 3:
The goal of Objective 3 is to develop and assess nematode management strategies in agricultural production systems. The overarching aim is to link the nematode genetic variability studied in Objectives 1 and 2 and link this information to the development and deployment of alternative management strategies. A diversity of features and methods will be considered in the design of nematode management strategies encompassed in Objective 3. This objective will address integrated nematode management including host plant resistance, tillage and reduced to no tillage practices, new pre- and post-plant nematicides, and impact of biological antagonists in crop rotation sequences. Cover, trap, and green manure crops will be considered in practical combinations and multiple locations. These field-based studies will employ standard experimental designs (randomized complete block, split-block, split-plot) with positive and negative control treatments and 4 to 6-fold replications under current production practices. Parameters measured in these experiments will be expanded to include soil properties, nematode community structure and function, sampling strategies tied with opportunities afforded by precision agriculture technologies for site-specific management. The development of biotyping schemes for cyst and root-knot nematode populations for reaction to host and nonhost crops and to resistant cultivars and accompanying practical marker systems under Objective 1 will provide important information for resistance and rotation implementation in annual crops. For example, soybean cyst nematode, reniform nematode and root-knot nematode rotation experiments will be conducted with contemporary highly resistant, resistant and susceptible soybean, cotton, and vegetable varieties, because new varieties are coming to market every year and we must continually evaluate new varieties in production systems. Crop rotation utilizing only nonhosts in production or cover crops that are grown in rotation with hosts will be evaluated for success in nematode management and profitability. Knowledge on nematode fitness and adaptability developed under Objective 2 will aid in the optimization of durable nematode management strategies. Climatic and soil conditions, different nematode species, and variability in host ranges among different populations will require unique rotations for different growing regions.
Several cropping systems being evaluated currently under W-4186 and described earlier have multi-year horizons and crop sequence combinations that require additional analysis. Rotation schemes will be assessed in microplots or infested field sites within the relevant localities. Techniques will be coordinated among participants to facilitate direct comparisons of results. The rapidly changing demands on crop production present new challenges to the use of crop rotation for nematode management. For example, demand for ethanol has resulted in the shift from wheat to corn in potato rotations. While wheat is a host for M. chitwoodi and M. incognita, it is harvested early in the summer and supports limited reproduction in northern areas but can increase populations in the southern regions. In some areas it can also be followed by green manure cover crops in the fall that significantly suppress nematode populations. In contrast, when corn is grown, it will support several generations of M. chitwoodi or M. incognita and eliminate the opportunity for suppression of population increases using cover crops. However, corn can vary greatly in its host status to M. chitwoodi and different cultivars need to be screened for resistance to inform growers about which varieties would limit population increases. Grower interest in green manure crops and cover crops for nematode suppression is increasing but little is known about the host status of many of these plants. This needs to be determined so that effective recommendations can be made. In the case of sugar beet cyst nematode, H. schachtii, and cereal cyst nematode H. avenae, and the soybean cyst nematode, fallow or planting with a green manure crop incorporated after eight weeks will be tested in rotations with non-host crops such as corn, bean, onion or potato between main crops. In Idaho, the trap crop S. sisymbriifolium has been found to greatly reduce populations of G. pallida [61]. A comprehensive management plan for deployment is being developed.
As stated earlier, there are several new pre- and post-plant nematicides with reduced environmental toxicity available to growers across production systems. The W-5186 research group is uniquely situated to evaluate these nematicides across a range of environments, crops, and nematode targets. For example, Meloidogyne incognita and M. enterolobii are the species of nematicide field trials of Alabama, Arkansas, Florida, Louisiana, North Carolina, and New Mexico, most often in cotton, corn, soybean, peppers, vegetables, and fruit crops. Rotylenchulus reniformis has also been spreading across the cotton belt but is also a production problem in Hawaii. Many new nematicide products that are characteristically lower in mammalian toxicity are becoming available. They have active ingredients such as cyclobutrifluram, fluopyram, fluensulfone, fluazaindolizine, or spirotetramat. These will be evaluated as tools for use in nematode management programs, including use as seed treatments and in combinations with bio-products and biocontrol organisms. New biological products are also increasingly available and often found in combinations with chemicals and varieties. The diverse crops and nematode pests investigated by project participants present a major opportunity for incorporation of these new tools into nematode management systems.
To enhance understanding of spatio-temporal relationships among nematode populations, soil conditions, biological antagonists, and production practices, a combination of the SFW [79] and FUE [63]) models will be utilized to assess agro-biological and ecological suitability of cultural practice-based nematode management strategies. The SFW and FUE models are nematode community analysis based and use quadrant systems to describe best-to-worst case scenarios for outcomes of soil biophysicochemical changes and potential sustainability of the agronomic practices, respectively. Both models will be utilized to assess how any specificity and variable outcomes observed under Objective 2 relate to agricultural and ecological efficiencies of cultural practice-based nematode management strategies. In doing so, we will be developing bridges suitable for multi-disciplinary approaches beyond nematology and critical to solving agricultural problems [80].
Measurement of Progress and Results
Outputs
- Basic knowledge on biological, ecological and genetic processes underlying the success of cyst, root-knot, and other nematodes as parasites of crop plants.
- New or improved, and safer tactics for the management of nematodes that affect crop production and trade in US agriculture.
- Holistic approaches for integrating different nematode management strategies into IPM systems.
- Advanced assessment of management practices for control of plant parasitic nematodes of major agricultural significance.
Outcomes or Projected Impacts
- Increased knowledge base in plant-nematode biology, ecology and genetics for use in identifying novel targets for nematode control.
- Implementation of nematode management tactics that complement reduced or softer pesticide usage, benefitting human health and the environment.
- Promotion of sustainable management practices through new nematode management tactics.
- More efficient and effective response for nematodes with implications in trade.
- Producers and consumers realizing increased yield through reduction in nematode management costs and food production.
Milestones
(2025):Development and validation of new nematode diagnostic and detection protocols (related to Objective 1)(2027):Development of new research-based knowledge on nematode variability and adaptation relative to abiotic and biotic parameters in target cropping systems (related to Objective 2).
(2026): Evaluation of new nematode management tactics under appropriate experimental conditions (related to Objective 3).
(2028):Transfer of knowledge to stakeholders on new nematode management tactics and new diagnostic and detection protocols (related to outreach).
(0):Concerning the milestones indicated above, the research will provide information necessary to implement nematode management alternatives. Research in each nematode - cropping system combination is at various stages of maturity along a sequence of progressive steps based on the order of the objectives. These include developing new basic knowledge, from which a control strategy or diagnostic protocol is developed, followed by testing and implementation by field experimentation and demonstration, and transfer to stakeholders. For example, identification of resistance in a crop, advancement of the trait into commercial varieties and testing its effectiveness in a cropping system rotation will be made. Each nematode-crop plant combination and cropping system will have its own timeline for completion, but typically will require completion of the objectives.
Projected Participation
View Appendix E: ParticipationOutreach Plan
Throughout these efforts, researchers will interface with growers and other agricultural professionals through outreach efforts to partner in managing nematode diseases. The project has a successful track record of disseminating the new knowledge and information created by participants and co-operating colleagues. It is planned that the traditional outlets for transferring project results will continue to be utilized, including peer-reviewed journals, annual progress reports, scientific meeting presentations, and websites. Basic aspects of this research (i.e., resistance/parasitism gene discovery and characterization) will be published in refereed journals such as the Journal of Nematology, Nematology, Phytopathology, Molecular Plant-Microbe Interactions, Plant Molecular Biology, and PLoS ONE. Applied research will be published in refereed journals such as Plant Disease Management Reports and Plant Health Progress. Social media, blogs, and trade journal articles will be used to disseminate educational material and recent findings to a mass audience in the national and international community.
Outreach and extension goals also include translating research findings into recommendations for agricultural professionals and engaging in dialogue with agricultural professionals to improve and direct research efforts. In addition, team members routinely provide progress to growers and other stakeholders at the state level annually. In order to increase the dissemination of the project accomplishments, we have invited several new researchers with extension appointments to join as W-5186 members. This includes new members from Florida, Louisiana, Indiana, and Arkansas. Many W-5186 participants have a strong applied research component to their programs and routinely participate in extension-based activities (meetings, presentations, publications) with our agricultural stakeholders. They host and attend field days and talk to growers on a regular basis. Our extension members host weekly podcasts which have a wide reach and have won some awards for their influence. This is an excellent tool for communication to the end users. Twitter accounts also allow us an immediate announcement by phone that can be tracked for numbers of users. Everyone in today’s world has a phone and being able to reach them on that communication level allows our research finding to be in everyone’s hand quickly! The project members will also use web-based delivery vehicles to reach the broad stakeholder base that will benefit from objective, science-based knowledge and information about important plant parasitic nematode biology, diagnosis, management and regulation. Examples include GenBank for depositing most DNA sequences, the Barcode of Life Database (BOLD) for DNA sequences of COI barcodes together with associated metadata, NemaTaxa (new ITS nematode barcoding database), and the international database of quarantine organisms (QBOL). Among our stakeholders we have identified growers and food processors, national and international trade partners, USDA-APHIS and state regulatory agencies, national and state legislators, fellow scientists, and K -16 teachers and students.
Organization/Governance
The organization and governance of this Multistate Research Project will conform to the guidelines presented in the United States Department of Agriculture's publication "Manual for Cooperative Regional Research". Committee officers include a Chair, a Vice-Chair, and a Secretary. A new secretary will be elected at each annual meeting of the Technical Committee with the current secretary assuming the position of Vice-Chair. The Vice-Chair assumes the position of Chair at the end of the annual meeting. In the event that an Executive Committee is needed, the officers are authorized to serve in that role. For organization of each annual meeting, one member of the Technical Committee will be selected to coordinate and oversee local arrangements for the meeting. The meeting coordinator will be chosen based on the consensus selection of the meeting site, and typically will be the member from the state or institution hosting the meeting. The Technical Committee will rotate the annual meetings between representative Experiment Stations who will be participating in the project. Members will benefit from site visits of research and extension facilities at the host Experiment Station institution. Administrative guidance will be provided by an assigned Administrative Advisor.
Literature Cited
- Sasser J: A world perspective on nematology: the role of the society. Vistas on Nematology 1987:7-14.
- Koenning S, Overstreet C, Noling J, Donald P, Becker J, Fortnum B: Survey of crop losses in response to phytoparasitic nematodes in the United States for 1994. Journal of Nematology 1999, 31(4S):587.
- Committee SoNCLA: Bibliography of estimated crop losses in the United States due to plant parasitic nematodes. Journal of Nematology 1987, 19(Annals 1):6.
- Jones JT, Haegeman A, Danchin EGJ, Gaur HS, Helder J, Jones MGK, Kikuchi T, Manzanilla-López R, Palomares-Rius JE, Wesemael WML et al: Top 10 plant parasitic nematodes in molecular plant pathology. Molecular Plant Pathology 2013, 14(9):946-961.
- Nicol JM, Turner SJ, Coyne DL, Nijs Ld, Hockland S, Maafi ZT: Current Nematode Threats to World Agriculture. In: Genomics and Molecular Genetics of Plant-Nematode Interactions. Edited by Jones J, Gheysen G, Fenoll C. Dordrecht: Springer Netherlands; 2011: 21-43.
- Smiley R: Plant parasitic nematodes affecting small grain cereals in the Pacific Northwest. 2015.
- Dyrdahl-Young R, Cole E, Quintanella-Tornel M, Weldon R, DiGennaro P: Economic assessment of nematode biological control agents in a potato production model. Nematology 2020, 22:1-9.
- Talavera-Rubia M, Vela-Delgado MD, Verdejo-Lucas S: A cost-benefit analysis of soil disinfestation methods against root-rnot rematodes in Mediterranean intensive horticulture. Plants 2022, 11(20):2774.
- Desaeger J, Wram C, Zasada I: New reduced-risk agricultural nematicides - rationale and review. Journal of Nematolog 2020, 52.
- Chen J, Li QX, Song B: Chemical nematicides: Recent research progress and outlook. J Agric Food Chem 2020, 68(44):12175-12188.
- EPA: Fenamiphos; Amendment to use deletion and product cancellation order, available at: https://www3.epa.gov/pesticides/chem_search/reg_actions/reregistration/frn_PC-100601_10-Dec-08.pdf. In.; 2010.
- Skendžić S, Zovko M, Živković IP, Lešić V, Lemić D: The impact of climate change on agricultural insect pests. Insects 2021, 12(5).
- Palomares-Rius JE, Hasegawa K, Siddique S, Vicente CSL: Editorial: Protecting our crops - approaches for plant parasitic nematode control. Frontiers in Plant Science 2021, 12.
- Bogale M, Baniya A, DiGennaro P: Nematode identification techniques and recent Advances. Plants (Basel) 2020, 9(10).
- Mburu H, Cortada L, Haukeland S, Ronno W, Nyongesa M, Kinyua Z, Bargul JL, Coyne D: Potato cyst nematodes: A new threat to potato production in East Africa. Frontiers in Plant Science 2020, 11.
- Kantor M, Handoo Z, Kantor C, Carta L: Top ten most important U.S.-regulated and emerging plant parasitic nematodes. Horticulturae 2022, 8(3):208.
- Brown RH, Kerry BR: Principles and practice of nematode control in crops. 1987.
- Jeschke P: Progress of modern agricultural chemistry and future prospects. Pest Management Science 2016, 72(3):433-455.
- Brown CR, Mojtahedi H, Zhang LH, Riga E: Independent resistant reactions expressed in root and tuber of potato breeding lines with introgressed resistance to Meloidogyne chitwoodi. Phytopathology 2009, 99(9):1085-1089.
- Matczyszyn JN, Harris T, Powers K, Everhart SE, Powers TO: Ecological and morphological differentiation among COI haplotype groups in the plant parasitic nematode species. Journal of Nematology 2022, 54(1):-.
- Bai M, Qing X, Qiao K, Ning X, Xiao S, Cheng X, Liu G: Mitochondrial COI gene is valid to delimitate Tylenchidae (Nematoda: Tylenchomorpha) species. Journal of Nematology 2020, 52(1):1-12.
- Zhang L, Gleason C: Loop-mediated isothermal amplification for the diagnostic detection of Meloidogyne chitwoodi and M. fallax. Plant Disease 2019, 103(1):12-18.
- Petrillo MD, Matthews WC, Roberts PA: Dynamics of Meloidogyne incognita virulence to resistance genes Rk and Rk in cowpea. J Nematol 2006, 38(1):90-96.
- Varypatakis K, Véronneau PY, Thorpe P, Cock PJA, Lim JT, Armstrong MR, Janakowski S, Sobczak M, Hein I, Mimee B et al: The genomic impact of selection for virulence against resistance in the potato cyst nematode, Globodera pallida. Genes (Basel) 2020, 11(12).
- Fournet S, Kerlan MC, Renault L, Dantec JP, Rouaux C, Montarry J: Selection of nematodes by resistant plants has implications for local adaptation and cross-virulence. Plant Pathology 2013, 62(1):184-193.
- Beniers JE, Nöllen Y, van Eck HJ, Schouten HJ: Increased virulence of Globodera pallida during repeated rearing on different resistant potato cultivars explained by a simple model. Plant Pathology 2019, 68(3):445-453.
- Djian-Caporalino C, Palloix A, Fazari A, Marteu N, Barbary A, Abad P, Sage-Palloix AM, Mateille T, Risso S, Lanza R et al: Pyramiding, alternating or mixing: comparative performances of deployment strategies of nematode resistance genes to promote plant resistance efficiency and durability. BMC Plant Biology 2014, 14:53.
- Siddique S, Radakovic ZS, Hiltl C, Pellegrin C, Baum TJ, Beasley H, Bent AF, Chitambo O, Chopra D, Danchin EGJ et al: The genome and lifestage-specific transcriptomes of a plant parasitic nematode and its host reveal susceptibility genes involved in trans-kingdom synthesis of vitamin B5. Nature Communications 2022, 13(1):6190.
- Montarry J, Mimee B, Danchin EGJ, Koutsovoulos GD, Ste-Croix DT, Grenier E: Recent advances in population genomics of plant parasitic nematodes. Phytopathology 2021, 111(1):40-48.
- Starr JL, Koenning SR, Kirkpatrick TL, Robinson AF, Roberts PA, Nichols RL: The future of nematode management in cotton. Journal of Nematolog 2007, 39(4):283-294.
- Ali MA, Azeem F, Li H, Bohlmann H: Smart parasitic nematodes use multifaceted strategies to parasitize plants. Frontiers in Plant Science 2017, 8.
- Blok VC: Apomictic, polyphagous root-knot nematodes: Exceptionally successful and damaging biotrophic root pathogens. Annual Review of Phytopathology 2001, 39(1):53-77.
- Kokalis-Burelle N, Rosskopf EN: Susceptibility of several common subtropical weeds to Meloidogyne arenaria, M. incognita, and M. javanica. Journal of Nematolog2012, 44(2):142-147.
- Habteweld A, Brainard D, Kravchenko A, Grewal P, Melakeberhan H: Effects of plant and animal waste-based compost amendments on the soil food web, soil properties, and yield and quality of fresh market and processing carrot cultivars. Nematology 2017, 20.
- Li Q, Jiang Y, Liang W, Lou Y, Zhang E, Liang C: Long-term effect of fertility management on the soil nematode community in vegetable production under greenhouse conditions. Applied Soil Ecology 2010, 46(1):111-118.
- Rosskopf E, Gioia FD, Hong JC, Pisani C, Kokalis-Burelle N: Organic amendments for pathogen and nematode control. Annual Review of Phytopathology 2020, 58(1):277-311.
- Habteweld A, Brainard D, Kravchencko A, Grewal PS, Melakeberhan H: Effects of integrated application of plant-based compost and urea on soil food web, soil properties, and yield and quality of a processing carrot cultivar. J Nematol 2020, 52.
- Davies KG, Rowe JA, Williamson VM: Inter- and intra-specific cuticle variation between amphimictic and parthenogenetic species of root-knot nematode (Meloidogyne spp.) as revealed by a bacterial parasite (Pasteuria penetrans). International Journal for Parasitology 2008, 38(7):851-859.
- Phillips M, Blok V: Biological characterisation of Globodera pallida from Idaho. Nematology 2012, 14:817-826.
- Gartner U, Hein I, Brown LH, Chen X, Mantelin S, Sharma SK, Dandurand L-M, Kuhl JC, Jones JT, Bryan GJ et al: Resisting potato yst nematodes with resistance. Frontiers in Plant Science 2021, 12.
- Phillips M, Blok V: Selection for reproductive ability in Globodera pallida populations in relation to quantitative resistance from Solanum vernei and S. tuberosum ssp. andigena CPC2802. Plant Pathology 2008, 57(3):573-580.
- Varypatakis K, Véronneau P-Y, Thorpe P, Cock PJA, Lim JT-Y, Armstrong MR, Janakowski S, Sobczak M, Hein I, Mimee B et al: The genomic impact of selection for virulence against resistance in the potato cyst nematode, Globodera pallida. Genes 2020, 11(12):1429.
- Zhang L, Gleason C: Transcriptome analyses of pre-parasitic and parasitic Meloidogyne Chitwoodi Race 1 to identify putative effector genes. Journal of Nematolology 2021, 53.
- Powers T, Harris T, Higgins R, Mullin P, Powers K: An 18S rDNA perspective on the classification of Criconematoidea. Journal of Nematolology 2017, 49(3):236-244.
- Powers TO, Bernard EC, Harris T, Higgins R, Olson M, Lodema M, Mullin P, Sutton L, Powers KS: COI haplotype groups in Mesocriconema (Nematoda: Criconematidae) and their morphospecies associations. Zootaxa 2014, 3827(2):101-146.
- Shengwei H, Franco J, Bali S, Chavoshi S, Brown C, Mojtahedi H, Quick R, Cimrhakl L, Ingham R, Gleason C et al: Diagnostic molecular markers for identification of different pathotypes of Columbia root knot nematode. Phytofrontiers under review.
- Baker HV, Caballero JRI, Gleason C, Jahn CE, Hesse CN, Stewart JE, Zasada IA: NemaTaxa: A new taxonomic database for analysis of nematode community data. Phytobiomes Under review.
- Blok V, Powers T: Biochemical and molecular identification. In Root Knot Nematodes, 1st ed.; Perry, R.N., Moens, M., Star, J., Eds.; CABI International: London, UK, 2009; pp. 98–112
- Huynh B-L, Matthews WC, Ehlers JD, Lucas MR, Santos JRP, Ndeve A, Close TJ, Roberts PA: A major QTL corresponding to the Rk locus for resistance to root-knot nematodes in cowpea (Vigna unguiculata L. Walp.). Theoretical and Applied Genetics 2016, 129(1):87-95.
- Dandurand LM, Zasada IA, Wang X, Mimee B, De Jong W, Novy R, Whitworth J, Kuhl JC: Current status of potato cyst nematodes in North America. Annual Review of Phytopathology 2019, 57:117-133.
- Whitworth JL, Novy RG, Zasada IA, Wang X, Dandurand LM, Kuhl JC: Resistance of potato breeding clones and cultivars to three species of potato yst nematode. Plant Diease 2018, 102(11):2120-2128.
- Dandurand LM, Zasada IA, LaMondia JA: Effect of the trap crop, Solanum sisymbriifolium, on Globodera pallida, Globodera tabacum, and Globodera ellingtonae. Jounral of Nematology 2019, 51:1-11.
- Melakeberhan H, Wang W: Proof-of-concept for managing Meloidogyne hapla parasitic variability in carrot production soils. Nematology 2013, 15(3):339-346.
- Melakeberhan H, Wang W, Kravchenko A, Thelen K: Effects of agronomic practices on the timeline of Heterodera glycines establishment in a new location. Nematology 2015, 17(6):705-713.
- Melakeberhan H, Wang W: Suitability of celery cultivars to infection by populations of Meloidogyne hapla. Nematology 2012, 14(5):623-629.
- Lartey I, Kravchenko A, Marsh T, Melakeberhan H: Occurrence of Meloidogyne hapla relative to nematode abundance and soil food web structure in soil groups of selected Michigan vegetable production fields. Nematology 2021, 23(9):1011-1022.
- French JM, Beacham J, Garcia A, Goldberg NP, Thomas SH, Hanson SF: First report of stem and bulb nematode Ditylenchus dipsaci on garlic in New Mexico. Plant Health Progress 2017, 18(2):91-92.
- Powers T, Skantar A, Harris T, Higgins R, Mullin P, Hafez S, Handoo Z, Todd T, Powers K: DNA barcoding evidence for the North American presence of alfalfa cyst nematode, Heterodera medicaginis. Journal of Nematology 2019, 51:1-17.
- Skantar AM, Handoo ZA, Kantor MR, Hult MN, Hafez SA: First report of the cactus cyst nematode, Cactodera cacti, from a cactus garden in Idaho. J Nematol 2019, 51:1-6.
- Skantar AM, Handoo ZA, Kantor MR, Hafez SL, Hult MN, Kromroy K, Sigurdson K, Grabowski M: First report of Cactodera milleri Graney and Bird, 1990 from Colorado and Minnesota. Journal of Nematology 2021, 53.
- Dandurand L-M, Knudsen GR: Effect of the trap crop Solanum sisymbriifolium and two biocontrol fungi on reproduction of the potato cyst nematode, Globodera pallida. Annals of Applied Biology 2016, 169(2):180-189.
- Ingham R: Nematode management in the face of short supply of Telone and Vydate In: Potato Progress. vol. X X I 2021.
- Melakeberhan H, Avendaño F: Spatio-temporal consideration of soil conditions and site-specific management of nematodes. Precision Agriculture 2008, 9(6):341-354.
- Vetter J, Ou Z, Murray L, Thomas S, Schroeder J: Determining the effectiveness of including spatial information into nematode/nutsedge pest complex model. Conference on Applied Statistics in Agriculture 2012.
- Kaloshian I, Williamson V, Miyao G, Lawn D, Westerdahl B: “Resistance-breaking” nematodes identified in California tomatoes. California Agriculture 1996, 50(6):18-19.
- Powers T: Nematode molecular diagnostics: from bands to barcodes. Annual Review of Phytopathology 2004, 42:367-383.
- Bhat KA, Mir RA, Farooq A, Manzoor M, Hami A, Allie KA, Wani SM, Khan MN, Sayyed RZ, Poczai P et al: Advances in nematode identification: A journey from fundamentals to evolutionary aspects. Diversity 2022, 14(7):536.
- Hu, S., Franco, J., Bali, S., Chavoshi, S., Brown, C., Mojtahedi, H., Quick, R., Cimrhakl, L., Ingham, R., Gleason, C., Sathuvalli, V: Diagnostic Molecular Markers for Identification of Different Races and a Pathotype of Columbia Root Knot Nematode PhytoFrontiers, 2023, 00:ja.
- Eves-van den Akker S: Plant–nematode interactions. Current Opinion in Plant Biology 2021, 62:102035.
- Bali S, Zhang L, Franco J, Gleason C: Biotechnological advances with applicability in potatoes for resistance against root-knot nematodes. Current Opinion in Biotechnology 2021, 70:226-233.
- Abd-Elgawad MMM: Understanding molecular plant-nematode interactions to develop alternative approaches for nematode control. Plants (Basel) 2022, 11(16).
- Gleason CA, Liu QL, Williamson VM: Silencing a candidate nematode effector gene corresponding to the tomato resistance gene Mi-1 leads to acquisition of virulence. Molecular Plant Microbe Interactions 2008, 21(5):576-585.
- Bali S, Hu S, Vining K, Brown C, Mojtahedi H, Zhang L, Gleason C, Sathuvalli V: Nematode genome announcement: Draft genome of Meloidogyne chitwoodi, an economically important pest of potato in the Pacific Northwest. Molecular Plant Microbe Interactions 2021, 34(8):981-986.
- Lartey I, Kravchenko A, Bonito G, Melakeberhan H: Parasitic variability of Meloidogyne hapla relative to soil groups and soil health conditions. Nematology 2022, 24:1-10.
- Martins ACQ, Mehta A, Murad AM, Mota APZ, Saraiva MAP, Araújo ACG, Miller RNG, Brasileiro ACM, Guimarães PM: Proteomics unravels new candidate genes for Meloidogyne resistance in wild Arachis. Journal of Proteomics 2020, 217:103690.
- Melakeberhan H, Maung Z, Lee C-L, Poindexter S, Stewart J: Soil type-driven variable effects on cover- and rotation-crops, nematodes and soil food web in sugar beet fields reveal a roadmap for developing healthy soils. European Journal of Soil Biology 2018, 85:53-63.
- Melakeberhan H, Bonito G, Kravchenko AN: Application of nematode community analyses-based models towards identifying sustainable soil health management outcomes: A review of the concepts. Soil Systems 2021, 5(2):32.
- Habteweld A, Kravchenko AN, Grewal PS, Melakeberhan H: A Nematode community-based integrated productivity efficiency (IPE) model that identifies sustainable soil health outcomes: A case of compost application in carrot production. Soil Systems 2022, 6(2):35.
- Ferris H, Bongers T, de Goede RGM: A framework for soil food web diagnostics: extension of the nematode faunal analysis concept. Applied Soil Ecology 2001, 18(1):13-29.
- Melakeberhan H: Assessing cross-disciplinary efficiency of soil amendments for agro-biologically, economically, and ecologically integrated soil health management. Journal of Nematology 2010, 42(1):73-77.