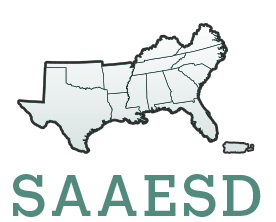
S1076: Arthropod Management in Animal Agriculture Systems and Impacts on Animal Health and Food Security
(Multistate Research Project)
Status: Active
S1076: Arthropod Management in Animal Agriculture Systems and Impacts on Animal Health and Food Security
Duration: 10/01/2023 to 09/30/2028
Administrative Advisor(s):
NIFA Reps:
Non-Technical Summary
Statement of Issues and Justification
Federal funding priorities are focused on major issues of national concern, including climate change, food safety, food security, biofuels, and obesity. We will only be able to meet these challenges through a one health approach where we recognize that the health of people is closely connected to the health of animals and our shared environment. Entomologists play a key and vital role in helping to solve many of these national concerns by evaluating the potential impact of climate change on arthropod populations and how these changes can threaten the health and well-being of humans and animals, ultimately compromising a safe and secure food supply for the nation.
External parasites and flies are among the most important pests in animal agriculture which includes (but is not limited to) beef, cervid, dairy, equine, poultry, and swine systems. These organisms include ticks, mites, fleas, lice, and both biting and nuisance flies. Ectoparasites can cause a range of damage including blood loss, skin inflammation and pruritus, toxic and allergic responses, disturbance, self-wounding, and may transmit pathogens causing disease. These can affect production, and social relationships, and cause animal welfare concerns (Machtinger et al. 2021). Flies, in particular, are responsible for damage and control costs in excess of a billion dollars per year in the United States (e.g., see Machtinger et al. 2021; https://doi.org/10.1093/jipm/pmab026). In addition to the direct damage these flies inflict upon livestock, their presence as a byproduct of confined livestock and poultry operations has been repeatedly cited as a nuisance, especially when flies
enter the vicinity of human habitations and urban environments. Lawsuits, zoning limitations and animosity between farmers and homeowners have resulted (Thomas and Skoda 1993). In spite of the importance of these pests, knowledge of the biology of these groups of pests is seriously wanting and available control technologies remain inadequate. The recent sequencing of the genomes of several of these common pest species, taken together with new molecular tools, integration of technology, and other novel methodologies offer great potential for the identification of novel opportunities for managing these pests.
The Southern Region Information Exchange Group for IPM (SERA3) listed several identified priorities in 2021 including improving linkages and partnerships, enhancing federal, state, and territory support for IPM, flies on agricultural animals, ticks affecting animals, arthropod-borne diseases, pesticide resistance, and invasive arthropods, among others as priority areas based on the selection criteria
of strong stakeholder identified need, addressing economic, environmental, and human health issues, as well as regional relevance
(https://bugwoodcloud.org/CMS/mura/sipmc/assets/File/SERA%20Priorities%202021.pdf). The Western IPM Center has listed identification and management of invasive pests, addressing climate change impacts on pests, and improving animal health by minimizing arthropod impacts as priorities. Finally, the North Central IPM Centers have listed invasive species, new technologies for managing pests, IPM and ecosystem services, and biological control of pests as areas of investment. All of these priorities from the respective centers and others from related working groups are addressed with the strengths of this collaborative multi-state group.
In 2001, Research and Extension Needs for Integrated Pest Management for Arthropods of Veterinary Importance that were identified as part of a USDA sponsored workshop in Lincoln, Nebraska (Geden and Hogsette 1999) were reevaluated (http://www.ars.usda.gov/Services/docs.htm?docid=10139). This document describes the IPM needs of eight animal commodity groups including poultry, dairy, beef cattle, and swine. This document was revised again into a special collection IPM of Pest Flies in Agriculture in the Journal of IPM (https://academic.oup.com/jipm/pages/ipm-of-fly-pests-in-animal-agriculture) that highlighted current research and Extension needs for five species of pest flies as well as economic considerations. The working groups developing these recommendations noted the direct, indirect, and peripheral impacts arthropod pests have on agricultural animals and made strong recommendations for increased research and Extension efforts to reduce the considerable losses resulting from pest activities. This workgroup also noted the decline to critical levels of
Extension personnel nationally, particularly related to domestic animal production. For years, we have noted a need for increasing coordination, collaboration, and education among veterinary entomologists to more efficiently disseminate research findings and management recommendations (Mullens et al. 2018).
Successful completion of this revised multistate project will provide a better understanding of the interactions among animals, animal agriculture production systems, and the biology of pest arthropods. Exploitation of these interactions will provide economically feasible and environmentally friendly technology for reducing the impact of pest arthropods on animal agriculture and human health. Results from the project will provide quantitative data to analyze vector-borne spread of pathogens within and from animal production systems into residential areas. Outcomes from the project will include the development and assessment of new control technologies for pest arthropods that will assist with monitoring pest resistance to insecticides that are currently available or under development. New technological innovations and comprehensive pest management information will be disseminated to stakeholders through a multistate coordinated effort to provide the broadest reach for project outcomes thereby increasing the health and quality of animals and reducing the economic impact of these pests.
The expertise to accomplish the objectives of this project exists within the university and USDA-ARS systems; however, expertise is widely dispersed with few states having more than one veterinary entomologist and many having none (Mullens et al. 2018). A revised Multistate Project will serve to coordinate this research effort, maximizing synergy and minimizing duplicated effort.
This project “Arthropod Management in Animal Agriculture Systems and Impacts on Animal Health and Food Safety” project will replace the existing Multistate Project S-1076: Fly Management in Animal Agriculture Systems and Impacts on Animal Health and Food Safety (2018-2023).
Related, Current and Previous Work
Arthropod pests, such as flies, mites, and ticks, are a concern in animal facilities. These pests can also serve as vectors for diseases agents affecting animals and humans. In recent years, extensive research has been conducted to develop effective control strategies to manage these pests and reduce their negative impact on animal health and welfare. Research has explored the effects of environmental factors, such as temperature and humidity, on their abundance and distribution in animal facilities. Various pest management methods have been examined for efficacy, including chemical, biological, and physical control measures. Ongoing research in this area is essential for developing sustainable pest management strategies to promote the health and well-being of animals in facilities.
To effectively manage pest populations, it is imperative to have current knowledge of their ecology, biology, and life history. With shifting climates and land use patterns, the historical range of many pest species is changing, necessitating ongoing attention. Ticks are expanding their distribution and introducing new pathogens to previously unaffected areas, as exemplified by the Asian longhorned tick and Theileria orientalis. Updated ecological data and occurrence information are critical for many tick and insect species. Various multi-state projects have been established to address the abundance and distribution of arthropods, and arthropod-borne pathogens, particularly ticks and mosquitoes. However, these initiatives are often regionally specific. They may not focus on pests relevant to livestock health. For example, NE1943 aims to map tick abundance, infection rates, and transmission risks in the eastern United States. At the same time, WERA1017 coordinates integrated pest management research and extension programs in the Western States and Pacific Basin Territories. These projects do not prioritize arthropods of livestock concern. Combining research efforts that address similar questions across various pest systems is crucial to develop a more comprehensive understanding of livestock-pest-pathogen interactions. The proposed multi-state project aims to do just that, without regional limitations, by integrating diverse research areas.
There is a need to focus on the fundamental biology and behavior of pests essential for maintaining sustained pest and pathogen control. Over the past 15 years, the genomes of several arthropod pests with veterinary significance have been sequenced, providing valuable insight into host-seeking behavior, pesticide resistance, and vector competence. However, there are still many pests without this valuable information.
Monitoring and trapping are critical tools for detecting and managing arthropod pests in animal facilities. Establishing accurate methodology and technology is crucial for providing livestock producers with the knowledge to execute treatments when pest populations reach or exceed economic thresholds. Recently, digital imagery has been used to capture real-time fly population densities (Smythe et al. 2020). Developments in neural networks are promising pathways to automate counts from images (Psota et al. 2021). Using SMART (specific, measurable, achievable, relevant, and time-Bound)technologies to observe animal behavior and monitor animal health are promising alternatives to pest scouting and enumeration, ultimately guiding treatment decisions (e.g., Murillo et al. 2020).
Various traps have been established for arthropod pests, especially pest flies. Bruce traps (Bruce 1938) mechanically control horn flies by brushing them from cattle moving through a dark, enclosed walk-through corridor. The efficacy of Bruce and Cow Vac traps (Spalding Laboratories, Reno, NV., https://spalding-labs.com/products/fly_control_products/cow_vac/default.aspx) have been compared. Both traps significantly reduced on-animal horn fly numbers. Still, in areas where horn fly numbers are typically very high, the additional efficacy of the Cow Vac trap may be needed to protect productivity. Traps for other arthropod pests, such as poultry red mites, northern fowl mites, ticks of medical and veterinary importance, and other arthropod pests, have not been as extensively investigated.
Push-Pull is an integrated pest management strategy that prevents insect host-seeking behavior by repelling insects from protected hosts and pulling them toward alternative hosts or traps (Cook et al. 2006, Pickett et al. 2014). This eco-friendly tactic (Bhattacharyya 2017) has been recognized for managing arthropod pests of animals (Zhu et al. 2015). An application of push-pull to stable flies on pasture cattle (Boxler et al. 2011) has been completed by members of S1076. It is currently in peer review for publication in an international journal. Several vector management systems have been tested (Midega et al. 2018, Takken 2020), including a tsetse fly push-pull currently in Use (Saini et al 2017).
Insecticides have long been the standard method of controlling arthropod pests. However, the development of insecticide resistance has been documented in various pest species, including the house fly, mosquitoes, and ticks, over the past 15 years (Scott 2017; Sun et al. 2017; Freeman et al. 2019; Burgess et al. 2022; Eidan et al. 2015). Moreover, the limited registration of novel chemistries for managing arthropod pests of livestock in recent years poses an additional challenge for managing insecticide resistance. Only a few new chemistries, such as cyantraniliprole and broflanilide, have been developed and registered for managing house and filth flies (Geden et al. 2021). Developing new chemistries with novel modes of action is complicated by overlapping resistance mechanisms, even in pests that are initially naive to a given chemistry. For example, during selection experiments, resistance mechanisms were already present in in-house flies towards fluralaner, a new insecticide from the isoxazoline class (Norris et al. 2023). To avoid complete product failure, constant surveillance is necessary to overcome the challenges of pesticide resistance.
Biopesticides, including essential oils, are gaining attention as promising alternatives to traditional pesticides (Khater and Geden 2019). Mullens et al. (2018) reported that 2% geraniol provides short-term horn fly control by reducing blood-feeding success and reproductive fitness relative to untreated controls. Fatty acid-based biopesticides have also been investigated for their pest-control properties (Brewer et al. 2021). Given the urgent need to manage current and future pests and supplement existing registered pesticides, developing novel chemistries is essential. Exploring plant-based and other biopesticides needs expansion, and standardized testing protocols should be implemented to assess their efficacy. Ticks and mites are also important pests that require attention in developing new chemistries and biopesticides.
Precision agriculture technology is increasingly used for pest and vector surveillance in animal agriculture. Recent studies have demonstrated that SMART traps can effectively monitor pests and vectors (Potamitis et al. 2017, Staunton et al. 2021). Digital imagery can be used for pest monitoring (Smythe et al. 2020, Psota et al. 2021). Sensors enable real-time analysis of individual animal responses, such as feed and water consumption, behaviors, and movements (Barriuso et al. 2018, Oliveira et al. 2018, Martínez Rau et al. 2020, Murillo et al. 2020, Herlin et al. 2021, Spiegal et al. 2020, Neethirajan 2017). Previous studies have explored using digital photography and deep learning to automate fly counting (Smythe et al. 2020). Algorithms to detect pests on poultry and cattle (Psota et al. 2021). In addition, research has shown that pests can alter animal behavior (El Ashmaway et al. 2019, El Ashmaway et al. 2020, Aboli et al. 2020) and that imagery and producer decisions can be used in pest control (Smythe et al. 2020, Psota et al. 2021, El Ashmawy et al. 2021). There is a need to investigate the effectiveness of artificial intelligence in monitoring and predicting pest populations, using data obtained from wireless sensor technology and functionality within prediction algorithms, and establishing dynamic thresholds accounting for breed and genetic selection. Although similar multi-state projects are present in the CRIS database, none specifically mention pests.
Stakeholder education is crucial for the successful management of arthropod pests of livestock. In response to stakeholder needs, project members developed an online searchable pesticide database using grant funds from the Western IPM Center. This database was subsequently made available to the public through the multi-state project website (Veterinary Entomology: www.veterinaryentomology.org), an essential outreach tool for the project. Project members have created pest surveillance and management videos linked to the website to support stakeholder education further. They could be utilized as educational products for youth farming organizations such as 4H and FFA.
One extension objective of the previous project was to update the Research and Extension Needs for IPM of Arthropods of Veterinary Importance (Geden and Hogsette 1994), produced as a Proceedings of a USDA-sponsored workshop in 1994 and was last updated in 2001. Recognizing the outdated nature of this document, project members collaborated to develop pest reviews that included discussions of extension needs for each of the common fly pests of livestock and poultry. Reviews introduce fly impacts on animal production (Machtinger et al. 2021) and then specifically discuss important pests, including the house fly (Geden et al. 2021), little house fly (Murillo et al. 2021), stable fly (Rochon et al. 2021), horn fly (Brewer et al. 2021), and face fly (Trout Fryxell et al. 2021). These reviews were published through a special issue arrangement with the Journal of Integrated Pest Management, where they are more easily accessible relative to the older 1994 Proceedings document.
In addition, project members have worked with regional partners to develop pest management guidelines and other documents to support pest management. Many examples of pest management guidelines or pest notes can be found on the project website. Members continue to work on improving these guidelines to address changes in industry practices, such as increases in organic animal production and animal welfare concerns. To this end, some members have successfully pursued funding from regional IPM Centers to update animal "Crop Profiles" and develop new Pest Management Strategic Plans that address current producer concerns regarding pest management. These plans are developed in close partnership with livestock and poultry producers, animal scientists, agricultural engineers, and other stakeholders to ensure the timely dissemination of information across the USA.
Objectives
-
1. Investigate the distribution, ecology and biology of arthropods of veterinary concern
Comments: a. Determine life history and ecology patterns of arthropod pests b. -Omics of arthropod pests c. Investigate arthropod-microbe interactions and their impact on food security d. Quantify arthropod physiological and behavioral responses to a changing environment -
2. Develop and implement Pest Management Systems to Protect Animal Health, Welfare, and Productivity
Comments: a. Developing new IPM tactics and tools such as competitive exclusion to reduce pest population development in larval habitats, selection for virulence in entomopathogens, improving fly trap efficacy, and integration into IPM systems. b. Provide IPM solutions for production systems, commodities, and species management, including invasive species. c. Determine baseline efficacy for novel pesticides and monitor pesticide resistance levels for key pests and currently used pesticides. -
3. Develop and strengthen insecticide resistance surveillance of arthropod pests of veterinary concern and associated arthropod-transmitted pathogens
Comments: a. Insecticide resistance detection and management b. Xenosurveillance c. Ecological epidemiology -
4. Develop precision innovations for economically beneficial management of arthropods of veterinary concern
Comments: a. New/developing approaches to managing pests i. Vaccination technologies including anti-tick vaccines ii. Automation and precision in monitoring (Technology, automation, and remote sensing of arthropods) b. Improved economic modeling (Identify successful models of producer behavior change and technology adoption emphasizing the critical decision drivers and thresholds) i. E.T.s / EILs ii. Animal Response to pest pressure iii. Production expenditures and losses/costs iv. Modeling economic impacts of arthropod pests. -
5. Develop and deliver science-based educational materials focused on the management of arthropods of veterinary concern
Comments: a. Professional development for multi-state members and stakeholders. b. Website workshop c. Assessment Coordinator d. Impact speakers e. Connecting with veterinarian groups f. Education of Stakeholders, Sells/Co-op, Extension, next generation of livestock entomologist
Methods
Objective 1. Investigate the distribution, ecology and biology of arthropods of veterinary concern
Distribution studies will survey and trap arthropods directly from the field/vertebrate host while taking environmental measurements to determine ecological niches and preferences. Participants from different locations will collaborate to collect data over a wide geographic range. All the arthropods studied here have broad geographic ranges, and collaborative collections are essential for accurate and representative ecological studies. Whole organism laboratory and field experiments will address questions relating to arthropod choice, behavior, and responses to stimuli and may involve replicating experiments in different laboratories with different colonies. As this objective focuses on a wide range of arthropod pests, collaboration is essential as not all researchers can access colonies or equipment to conduct experiments. In addition, many projects within this objective will couple whole organism experiments with omics approaches such as genomics, transcriptomics, proteomics, epigenomics, and metabolomics. The wide range of researchers participating in this study will share expertise, data, equipment, and materials resulting in successful multidisciplinary studies.
Objective 2. Develop and implement Pest Management Systems to Protect Animal Health, Welfare, and Productivity
Biological Control. Black soldier fly larvae have the potential to exclude stable fly larvae from potential larval habitats. Lab bioassays using a RCB design will test larval development of both species in media at variable ratios of black soldier fly to stable fly larvae and to the introduction to the media at different timelines. If initial results are positive, a series of field trials will be done at regional locations.
Commodity, Species, and Invasive Management. As new production practices are implemented and as pest landscapes change, there is a continuing need to develop and implement IPM strategies for specific species. Three examples follow.
The invasive Asian Longhorn tick is progressively expanding its range in the eastern U.S. and in Arkansas and Missouri. It has a wide host range and vectors a number of zoonotic diseases. Multiple sites in the U. S. will evaluate current and novel control chemistries, including biopesticides on livestock to establish treatment efficacy, frequency, and cultural guidelines for the management of this invasive pest species.
Second, there is a need for alternative control products for the northern fowl mite. Current lab testing of plant essential oil biopesticides will be done to identify promising products. Testing will be done with an olive oil surfactant at high and low rates applied to the vent region at two time periods. Mite populations will be monitored weekly. The best products will then be trialed at different locations and in various production systems.
Third, there is a concern of Culicoides susceptibility to current control products. Adult and larval Culicoides will be bioassayed using multiple application methods. Cultural controls, habitat modification, will also be tested to determine their effect on Culicoides populations.
Fly Stress Management. With an emphasis on smart technologies, measurements of cattle stress and behavioral changes will be related to multispecies fly counts. One goal is to develop multispecies, stress focused injury levels to guide treatment decisions. A second goal is to produce a multiple species IPM system that integrates within a Smart Ranching System. Testing will be done at multiple locations and environments.
Trap Improvement. Recent testing has demonstrated efficacy of a tubular stable fly trap and of walk-though horn fly traps. The stable fly trap is both visually and olfactorily attractive. Lab assays to identify new stable fly attractants and release strategies to improve trap capture rates in a push-pull strategy. Field trials will then be done at regional locations and in different production systems. Walk-through horn fly traps will be tested in different environments and production systems as standalone treatments to reduce fly loads, to improve cattle comfort, and to protect productivity. Integrated horn fly rescue treatments emphasizing low-impact biopesticides will also be done.
Insecticide Resistance Management. Developing horn fly resistance to current labeled chemistries is threatening control failure. The focus of this project is to develop standard protocols and evaluation procedures to determine current baseline LC50 and LC90 levels for regional horn fly populations and to monitor for changes over time. Information will be shared with stakeholders to encourage and guide adoption of insecticide resistance management plans to reduce horn fly impact, improve animal welfare and producer profitability and sustainability. Similar procedures and monitoring strategies will be developed for other species as necessary.
Novel Chemistries and Modes of Action. Developing resistance to currently registered control products for horn, face, and stable flies is threatening quality production and animal welfare. Novel chemistries including biopesticides will be field evaluated on pastured cattle at multiple sites across the U.S. using various application technologies. Efficacy guidelines at environments and in production systems will be evaluated.
Alternate Sources of Radiation for Screwworm Eradication Efforts. Lab trials to assess the feasibility of Xray and eBeam radiation to replace Cobalt-60 for production of sterile screwworms (Cochliomyia hominivorax) for use in Sterile Insect Technique eradication programs will be done. Treated specimens will be assessed for eclosion success, adult gonad development, longevity, and reproduction. Results will be compared across radiation types and dose levels to determine the relative quality of the screwworm flies produced. If successful, the new SIT production technology could be trialed on other species and field testing could be implemented if approvals are obtained.
Objective 3. Develop and strengthen insecticide resistance surveillance of arthropod pests of veterinary concern and associated arthropod-transmitted pathogens
House flies. Resistance monitoring efforts will again be carried out in many states with an effort to establish baseline susceptibility to new and existing fly control products, document resistance levels, reversion of resistance, and the evolution of resistance to current insecticides, as well as new insecticides that become available for fly control over the next five years. In addition to bioassay methods, molecular techniques (sequencing of PCR products, multiplex PCR, etc.) will be used to evaluate the frequency of essential resistance alleles in the house fly (Rinkevich et al. 2007; Kozaki et al. 2009).
Work will continue on the behavioral resistance to imidacloprid. We have selected resistant lines to confirm the existence of behavioral resistance. The next step will be to conduct additional behavioral and neurophysiological assays to point us toward the receptor(s) driving this behavioral resistance. Finally, a genomics approach will be utilized to identify the altered alleles that support this phenotype. Ultimately we hope to identify the mutation responsible for it and determine its frequency in natural populations.
Reduced cuticular penetration has been isolated to chromosome III in pyrethroid-resistant house flies (Patil and Guthrie 1979) and was recently discovered as a resistance mechanism in a house fly strain pressured with fluralaner, a new isoxazoline class insecticide (Norris et al. 2023). We will work to uncover the structural, molecular, and biochemical mechanisms underlying reduced cuticular penetration seen in this strain.
Stable flies. We will continue to collect data on stable fly permethrin resistance through the distribution of resistance kits. The original University of Florida permethrin-susceptible stable fly colony (UFD) was established in 2007. Thus we are currently establishing a new stable fly colony (Pitzer et al. 2010). Comparisons will be made between two newly established strains for the presence of the kdr mutations. Should we move the resistance to a higher level than the original permethrin resistant stable fly strain, we will identify if additional resistance mechanisms emerge as metabolic resistance or additional sodium channel mutations.
Alphitobius diaperinus. Elucidating resistance mechanisms will be done similar to the house fly methods mentioned above. In brief, molecular, and biochemical mechanisms underlying insecticide resistance will be characterized and molecular based tools, including PCR and its variants, will be developed to enable resistance surveillance in this important poultry pest.
Ticks. PCR and tick bioassays will be used to monitor and/or confirm resistance to permethrin in different species and lineages of ticks. When possible, after the characterization of the molecular mechanism of target site insensitivity is completed, studies to investigate the mechanisms of resistance will occur (e.g., PCR high-resolution melting point analysis). Ticks of interest include Rhipicephalus microplus, R. sanguineus, and Haemphysalis longicornis. Data from projects will help with the Cattle Fever Tick Eradication Program and clarify basic science questions.
Objective 4. Develop precision innovations for economically beneficial management of arthropods of veterinary concern
Precision ectoparasite surveillance. Current management practices rely on producers evaluating their animals in terms of the number of each fly or tick species present, as well as any occurrence of disease events. The surveillance for presence of ectoparasites has been primarily accomplished by on-host visual inspection, as well as off-host trap or drag counting and immature population counting. These traditional methods are not feasible for many cattle operations. Smythe et al. (2020) demonstrated the use of digital imagery to enumerate horn fly populations within a cattle herd, while Psota et al. (2021) applied computer vision and deep learning to identify and enumerate flies from digital images acquired from individual cattle hosts. Further, most economic impacts in veterinary entomology are measured by production outputs like weight gains, milk output, etc. In mature animals, this may not be the most accurate way to determine stress effects of parasitism by biting and nuisance flies or by ticks. Correlating animal behavior responses to intensity of parasitism could be more indicative of the degree to which management interventions are required and, once implemented, their effectiveness.
In this objective, we will employ sensors, monitoring, analysis, and reporting (SMART) approaches to improve ectoparasite surveillance and correlate animal behavior responses with level of parasitism. We will extend the work of Psota et al (2021) and use images (digital or video) with both fly and tick pests on livestock. Machine learning will be used to build an artificial intelligence-driven application to identify and/or count pest flies and ticks on hosts. Once trained, we will host the algorithm either on a website or within an app that can receive images uploaded from producers for analysis. Images could be those acquired by digital cameras, drones, cell phones, and/or game/stationary cameras. The analysis output would then be provided to the producer to inform their management approach. In parallel, current and novel wearable, wireless biosensor technologies that measure animal behavior variables and temperature will be evaluated. Classical indicators of animals that are parasitized by flies include tail flicking, leg stamping, head throwing, skin twitching, and ear flicking, and these have traditionally been monitored in herds by casual observation. Wearable, wireless sensors will be used to record these specific animal behaviors and general cattle movement, and these data will be coupled with digital imagery (video observations) to relate the sensor outputs for these behaviors to the level of fly or tick parasitism, assessed using digital imagery analysis. These data will be incorporated into training machine learning algorithms to more accurately model and predict the onset of ectoparasite levels that may impact animal health and welfare.
Developing a new sampling model for horn flies. The US has the largest fed-cattle industry in the world, and beef production is a prominent economic driver of agricultural production enterprises in the US, especially in states like Oklahoma, Texas, Kansas, and Nebraska which are in the top ten states for cattle production. Production efficiency is essential to maintain long-term profitability and sustainability in this sector, and extensive research has enabled genetic selection of traits to improve the performance of beef production animals that optimize the needs of the operation; however, the negative impacts of ectoparasites impact this efficiency. Horn flies are of particular significance because of their obligate blood-feeding behavior causing blood loss, loss in average daily gains, and more negative effects. A static economic threshold of 200 horn flies per animal was suggested in the late 1980s (Gordon et al. 1984, Kunz et al. 1984, Schreiber et al. 1987), but dynamic thresholds are needed that consider parameters such as cattle breed or changes in production performance that have occurred within beef cattle systems. In this objective, we will develop a new sampling model for horn flies that incorporates breed and genetic selection as a component. Further, we will determine if new economic thresholds should be established based on genetically selected cattle performance enhancements that are present in a herd.
Improving decision-making methods for producers using indicators. Spiegal et al. (2020) reported that seven different states identified ‘precision ranching through sensor-driven technology’ as the most adaptable strategy to increase the sustainability of beef operations and implementing this real-time analysis will be beneficial to producers. The combined improvements to sensor technologies and to computational algorithms that synthesize parameters associated with ectoparasite burden (i.e., ectoparasite detection and the host response to parasitism) will ultimately enable producers to make informed treatment decisions. These decisions could be based on calendar, animals’ current market value, climate, environment, and/or costs of treatment approaches. In the studies outlined above, we plan to evaluate new technologies for measuring and assessing selected parameters associated with hosts burdened by parasitic flies and ticks. In this objective, we will use ecological, epidemiological, and economical data streams to identify indicators associated with pest/pathogens of cattle. Ecological inputs from sensors acquiring data for biotic and abiotic (meteorological) factors will be incorporated along with animal health data and cost data, which can be extensive and challenging to capture. Costs include losses from production efficiency, expenditures from control measures, and reductions in animal welfare. Examples of losses in production efficiency include, but are not limited to, reduced average daily gain, disease damages, and treatment costs (e.g., pinkeye). Control measure expenditures include items such as the cost of labor for treating flies and ticks and the cost of insecticides/acaricides. We aim to develop a dynamic managing platform that incorporates these data into a decision-making model to identify a problem before it starts. For example, Ghafoor and Sitkowska (2021) describe a machine learning application to predict bovine mastitis in cattle using automated milking system sensor data, enabling detection in the milking parlor before cases occur. The dynamic managing platform would be designed to also help a producer choose, for example, if it is currently economical or sustainable to treat a pest population or if the population is tolerable.
Objective 5. Develop and deliver science-based educational materials focused on the management of arthropods of veterinary concern.
To achieve the objective of developing and delivering science-based educational materials focused on the management of arthropods of veterinary concern, the following methods could be used:
Professional development for multi-state members and stakeholders: We will organize training sessions or workshops for members and stakeholders from different states to enhance their knowledge in IPM for pests of veterinary concern. These workshops could include lectures, hands-on demonstrations, and group discussions on the latest techniques, tools, and best practices in arthropod management.
Website workshop: We will create an interactive website with educational resources accessible to a wide audience. The website could include videos, fact sheets, infographics, and other materials on arthropod management. The website could also host webinars and virtual workshops to engage stakeholders and encourage participation.
Assessment Coordinator: An assessment coordinator will be invited to monitor and evaluate the effectiveness of the educational materials and workshops. This person will gather feedback from participants, assess the impact of the materials on knowledge and practices, and suggest improvements.
Connecting with veterinarian groups: We will engage with veterinary groups and associations could help reach a larger audience and build partnerships. Collaborating with these groups could also help ensure that the educational materials are relevant and targeted to the needs of the veterinary community.
Education of Stakeholders, Sells/Co-op, Extension, next generation of livestock entomologists: We will engage with key stakeholders such as sellers, co-ops, extension agents, and the next generation of livestock entomologists to help spread the word about the initiative and ensure that the educational materials reach a wider audience. This engagement could involve training, mentoring, and knowledge sharing to enhance their understanding of arthropod management and the importance of science-based approaches.
Measurement of Progress and Results
Outputs
- Field surveys will be conducted to determine the distribution, species diversity, habitat preference, host preference, and feeding location of pest arthropods. In particular, the study will focus on generating distribution and ecological predictors for various tick species, such as blacklegged, lone star, brown dog, cattle fever, and Asian longhorned ticks. Additionally, the diversity, larval habitats, and ecology of biting midges will be determined, as well as the feeding habits of the stable fly, including host preference. Field surveys will also identify the seasonality patterns and locations and larval habitats of pest flies. The data collected will help better understand the behavior and ecology of these arthropod pests and can be used to develop effective control strategies.
- Data on arthropod physiology, behavior, and vector-borne pathogens will be generated, particularly focusing on tick and fly species. This will enable a better understanding of the microbial ecology of these species and their potential to act as vectors of pathogens. The data collected will help identify the key factors that influence the transmission of pathogens by these arthropods.
- Control approaches for non-model arthropods (e.g., understudied, neglected vectors or pests) will be developed or validated using standard and novel gene editing technologies. Proposals for updates to current control methods will also be based on data derived from studies
- Pest monitoring techniques for existing and new invasive pests of poultry, horses, and cattle will be developed and validated, focusing on developing new action thresholds for new invasive species.
- New and standardized tools for pesticide resistance surveillance will be established for pest arthropods, and important aspects of physiology and origin that explain mechanisms of resistance that are understudied will be uncovered.
- Systems and tactics will be developed to gauge the magnitude of animal stress expressed as pest-induced changes in animal behaviors.
- Novel chemistries and modes of action will be evaluated for managing poultry, horse, cattle, and sheep ectoparasites.
- The use of Sterile Insect Technology for the control of arthropod pests will also be reexamined using newer eBeam and X-ray technologies.
- A new decision-making model for vector-pathogen complexes will be developed.
- New biochemical/physiological pathways will be explored and evaluated to impact pest species selectively, and cultural control tools will be evaluated to help manage hemorrhagic disease transmission to livestock.
- AI-driven algorithms will be developed to identify and enumerate pest flies or ticks. Digital imagery will be used and uploaded to a website/phone app platform, and algorithms will be created to interpret sensor data relating animal movements to fly or tick parasitism.
- Results of experiments will be published in peer-reviewed journals, and informational material will be generated to convey results to the public.
- Graduate students will be trained in veterinary entomology, contributing to the future of the field.
- Extension material will be created and distributed to reach target stakeholders and interest groups and shared among participating institutions to increase reach.
- The impact of the black soldier fly Hermetia illucens (L.) on developing stable fly larvae populations will be measured to reduce pesticide use at dairies and other confined livestock operations across the U.S.
Outcomes or Projected Impacts
- Improved understanding of the impact of black soldier fly Hermetia illucens (L.) on stable fly numbers.
- Improved arthropod population monitoring systems for livestock will enable better decision-making and more efficient control tactics.
- Identifying biochemical/physiological pathways to selectively reduce arthropod pests and developing cultural control tools such as source reduction and pasture management to reduce hemorrhagic disease transmission to livestock.
- Establishment of guidelines regarding animal behavior and physiology that describe the impact of fly species and population numbers on animal productivity and welfare.
- Establishing protocols and efficacy data for horn fly, stable fly, and face fly traps will enable producers to make informed decisions about pest control.
- Standardization and development of insect resistance protocols for multiple pests and species with testing at a participating network at regional test locations will improve pest control efficacy.
- Evaluation of existing and novel fly control products and new actions against arthropod pests affecting livestock in various animal facilities will help producers make informed decisions and likely reduce the number of pesticide applications.
- Establishing new treatment technologies associated with Sterile Insect Technology will enable more efficient and effective pest control.
- The development of new tools for pesticide resistance surveillance will aid in the surveillance of pesticide resistance and provide data, information, and guidelines for overcoming these mechanisms if proven possible.
- Establishment of insecticide-resistant colonies of select arthropod pests, which will be made available to help other researchers.
- Improved GenBank sequence data will be made available to other researchers.
- Improved use of sensors for automation of animal management, greater efficiency of ectoparasite surveillance, and a platform for submission of digital files (imagery/video) to assess animal behaviors in response to parasitism. This will enable producers to make more informed decisions about pest control and reduce the number of pesticide applications.
- More accurate assessment of control tactics for horn fly control in cattle systems and an improved understanding of horn fly impacts on current cattle systems.
- Students trained in veterinary entomology will ensure producers have a reliable knowledge base for continued assessment and implementation of these new technologies, likely resulting in improved production potential.