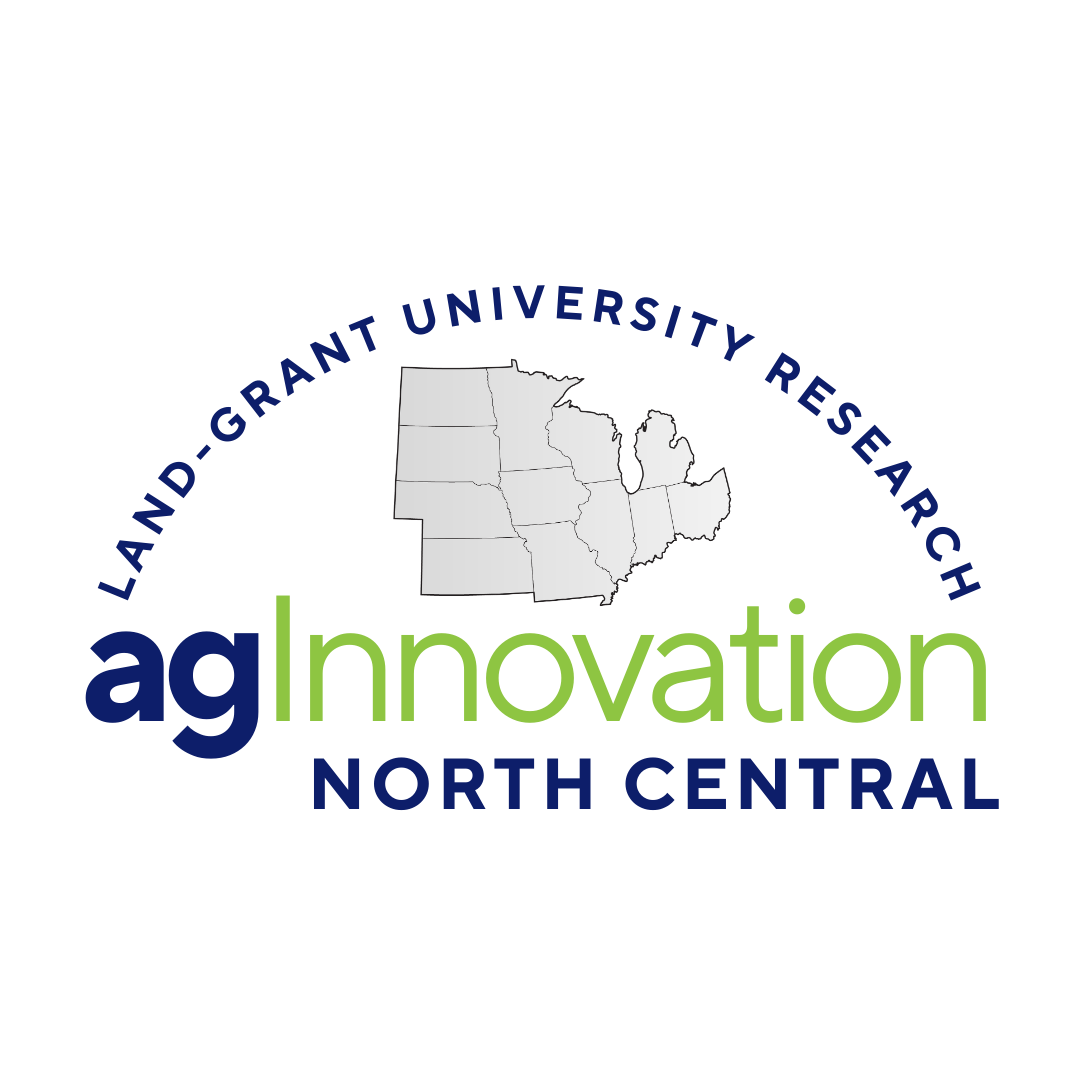
NC1206: Antimicrobial Resistance
(Multistate Research Project)
Status: Active
NC1206: Antimicrobial Resistance
Duration: 10/01/2022 to 09/30/2027
Administrative Advisor(s):
NIFA Reps:
Non-Technical Summary
Statement of Issues and Justification
Antimicrobial Resistance (AMR) is one of the most daunting challenges to human, animal, and environmental health, and thus should be approached with a One-Health perspective. Increasing focus has been given to the wide-ranging impact AMR will have on our global community. A recent report estimates that by 2050 AMR will not only be dangerous to human health, with annual death tolls reaching 10 million, but may reduce global GDP by as much as 3.8%[1–3]. An alarming increase in AMR to the “last resort” antibiotics used to treat severe infection is being observed, and the World Health Organization (WHO) reported resistance in up to 54% of human pneumonia isolates with an unfortunate lack of new drugs in development [4,5].
According to the Centers for Disease Control & Prevention (CDC) 2019 AMR Threats report, more than 2.8 million antimicrobial-resistant infections occur in the United States each year, 35,000 of which prove fatal [6]. A major concern of the CDC has been in surveilling the emergence and spread of these new forms of resistance, which do not arise only because of medical treatment. While neither antimicrobial use (AMU) in clinical settings nor in agriculture are solely to blame for the increase in the selective pressure for the rise of AMR, efforts must be taken in all sectors to decrease the use of antimicrobials and mitigate the risks associated with AMR.
Researchers and public health officials alike have been monitoring AMR for decades and in response to concerns about this issue, the National Antimicrobial Resistance Monitoring System for Enteric Bacteria (NARMS) was established. The NARMS program is a collaboration of the U.S. Food and Drug Administration (FDA), the CDC, the U.S. Department of Agriculture (USDA), state and local health departments, and universities established to monitor trends in AMR among enteric bacteria from humans, retail meats, and animals in the U.S. [7]. While the U.S. food supply chain is among the safest in the world, foodborne infections are still a concern, and many of these may arise from antimicrobial-resistant bacteria. Hundreds of thousands of Americans still become ill due to resistant Campylobacter or Salmonella each year [8,9], indicating that current surveillance methods are not sufficient for the appropriate application of mitigation strategies, and more efforts are needed to understand the ecology of AMR for effective mitigation interventions to be developed.
The use of antibiotics in animal agriculture is integral to the treatment of and protection against disease. It is imperative that stakeholders in animal agriculture and animal health address the important public health concern of bacterial resistance before antibiotic drugs either become ineffective or are further restricted for use in animals. In fact, according to NIFA’s stakeholder feedback [10], substantial support was demonstrated on the topic areas targeting AMR; furthermore, the development of rapid diagnostic tools, rapid identification tools and techniques, and the role of the microbiome in antimicrobial resistance were specifically stated as areas of interest. Stakeholders also suggested that efforts must be taken to leverage data and knowledge generated from other sources, including Federal activities.
The CDC and USDA action plans are comprehensive in their approaches to combat antibiotic resistance. The plans call for applied and basic research to:
- enhance surveillance and monitoring of antibiotic resistance,
- better understand the epidemiology and ecology of antibiotic resistance,
- develop new antibiotics and alternatives to antibiotics,
- determine the mechanisms involved in resistance and transmission of resistance,
- determine and/or model patterns and practices that impact the use of antibiotics in animal agriculture
- develop improved diagnostic tests and vaccines.
Described as a ‘wicked’ problem [11], AMR issues will not be solved by any one group or perspective. Antimicrobial resistance is a One-Health [12] phenomenon by definition, and in order to make a difference, all sectors - veterinary medicine, food science, human medicine, basic sciences, farmers, and educators - must be involved. Achieving these goals require interdisciplinary and transdisciplinary collaborations, which are greatly facilitated by multistate collaborations enabled by this project. More specifically, the objectives of this project will include performing research that will allow for the development of practices and recommendations to reduce the development and spread of AMRs. The objectives of this multistate project also aim to track and evaluate the effectiveness of these practices and programs.
Related, Current and Previous Work
Wherever more than one state is noted for a single project in the section below, the work described has been carried out as a collaborative project under the auspices of the current multistate project NC1206 – Antimicrobial Resistance (2017-2022).
Objective 1: Develop knowledge and tools to improve antimicrobial stewardship
Surveillance and monitoring of antimicrobial resistance
As part of the current Antimicrobial Resistance multistate project (NC1206 – 2017-2022) Investigators at MD, NY and PA carried out a collaborative project that quantified AMR in various manure systems across states. Investigators performed a literature review of concentrations of antibiotics and antibiotic resistant bacteria in dairy farm manures and compared manure management methods, finding that antibiotic concentrations often do not correlate with the abundance of antibiotic resistant bacteria [13]. An on-farm antibiotic degradation study revealed that antibiotic degradation during composting in sick cow bedding can vary between what is reported in the literature, done at laboratory scale, vs. what happens on farm, likely due to difference in management, time of composting, and temporal conditions. Researchers evaluated different manure management practices in controlling spread of antimicrobial resistance from 11 dairy farms across NY, PA, and MD at all points of the manure system (digestion, composting, solid-liquid separation, lagoon storage) over 18 months with 8 sampling events. Antimicrobial resistance gene (ARG) prevalence varied based on location and time, but did not correlate to antimicrobial usage or tetracycline concentrations across farms and collection dates. Antibiotic residuals in dairy manure digesters were often at or close to zero, but there were spikes with cow treatments, up to 45,000 ng/g dry weight [14].
Furthermore, evaluation of transformations of antibiotics and ARGs during anaerobic digestion of dairy manure revealed that >95% reduction of sulfa based (SDM) drugs could be achieved during digestion, but removal of tetracycline (TC) was observed to be inconsistent (0-80%) due to the adsorptive nature of TC. The reductions of antibiotic residuals in manure did not correspond with reductions of all ARG, with Sul1 genes having lesser reductions than TetM genes. No significant decreases were observed in methane production with addition of the highest levels of antibiotic residuals seen on-farm (1 mg/L), and when the concentration was increased to 10 mg/L of tetracycline and sulfamethoxine, less than 10% reduction in biogas production was observed [15].
Investigators from NY enhanced surveillance and monitoring of AMR and developed improved diagnostic tests. Using classical statistical methods, they have assessed the variation of multidrug resistance (MDR) in the publicly available NARMS data. As an alternative to classical methods, a graphical network method was developed, and current work focuses on machine learning. Additionally, an analytic pipeline was developed for using association rule mining to identify MDR patterns and trends. Integrated graphical network diagnostics with association rule mining output was successfully used to capture trends in MDR, and a modified procedure to control false discovery rate during association rule mining was used. The major advantage of these methods was shown to be recognition of the pattern of MDR in “big data” ultimately without human decision (machine learning). Specifically, the association rule mining method can efficiently analyze high-dimensional data (p>n) and return multivariate results. Methods developed by NY researchers can be used to quantify animal health, public health, social, economic, and environmental impacts of antimicrobial interventions in food production systems. From a sustainability perspective, a socio-ecological framework was used to assess AMU in animal agriculture, which included an assessment of the costs of AMU restrictions on the U.S. dairy market [16].
A four-year collaborative project between NE and HI was initiated in 2017 with support from USDA NIFA, aimed at applying a risk-based, systems approach to identify critical control points and evaluate potential intervention strategies to mitigate public health risks imposed by livestock-originated AMR. Beef cattle were used as the model system. Multiple interventions implemented on feedlot surfaces, during manure storage, and at land application were identified and their efficacies were quantified by inactivation of antimicrobial resistant bacteria and genes and reduced human exposure through consumption of fresh produce grown in the field where livestock manure was applied [17,18].
Investigators from CA characterized a total of 242 Salmonella isolates retrieved from 9,162 bovine fecal samples submitted to the University of California Davis Veterinary Medical Teaching Hospital from 2002 to 2016. These isolates were tested for antimicrobial susceptibility using a standardized broth dilution panel. Multidrug resistance to three or more classes of antimicrobials was observed in 50.8% of isolates, and the most common MDR pattern was amoxicillin-ampicillin-cefoxitin-ceftiofur-ceftriaxone-chloramphenicol-streptomycin-tetracycline (23.2%). There were significantly greater odds for AMR to aminoglycosides (OR: 2.03, 95% CI: 1.1-3.7), penicillins (OR: 1.87, 95% CI: 1.007-3.5), and tetracyclines (OR: 1.87, 95% CI: 1.017-3.4) for the 2002-2009 period when compared to the 2010-2016 period. The most prevalent MDR serotypes were Newport (100% MDR, n = 52), Typhimurium (100%, n = 20), and Dublin (71% MDR, n = 46). Risk factors associated with higher odds for isolating MDR Salmonella included isolates from calves when compared to adult cattle (OR: 22.0; 95% C.I.: 3.9-125.7), and isolates obtained from cattle suspected of having salmonellosis versus from the infectious disease control surveillance program (OR:13.7; 95%C.I.: 2.8-66.8). Despite an overall temporal trend for reduced AMR to most antimicrobial drug classes, resistance to important drug classes such as cephalosporins was not different in the 2002-2009 period when compared to the 2010-2016 period (OR: 1.6, 95% CI: 0.87-3.1), and a temporal increase in resistance to quinolones drugs (P value 0.004) highlighted the relevance of AMR surveillance in cattle with Salmonella infections with the aim of targeting future prophylactic interventions [19].
Determining the ecology and mechanisms involved in resistance and transmission of resistance
Researchers from PA and IN investigated the impact of heavy metals on AMR in swine and cattle. Investigators from IN used a highly parallel qPCR array (WaferGen) to determine the abundance of hundreds of ARGs in feces from pigs fed Zn and Cu, a growth promoter (Carbadox), or no additive. Each of these treatments resulted in unique ARG profiles and some enriched ARGs. More ARGs co-occurred with mobile genetic elements in the group that received growth promoter than in the control group samples. In this study, community composition could not be linked to resistome in the growth promoter group samples, indicating a potential decoupling of ARGs and phylogeny [20]. Researchers from PA investigated the impact of heavy metal-contaminated drinking water on antibiotic resistance genes in dairy cattle. Heavy metal-contaminated drinking water affected the fecal microbiome to a greater extent than the ruminal or nasopharyngeal microbiomes, and an increased the number of antibiotic resistant bacteria was observed in the fecal microbiome of animals exposed to heavy metal-contaminated drinking water [21].
Investigators from IN and IA studied the effects of bacitracin methylene disalicylate (BMD), an in-feed antibiotic that continues to be used for feed efficiency as well as for disease treatment in turkeys, on intestinal microbial communities over 14 weeks. BMD had immediate and lasting impacts on the microbiota structure, reducing species richness in the BMD-treated turkeys through the end of the study. Members of the microbiome that were enriched due to BMD included members of Lachnospiraceae and Ruminococcaceae families, while Lactobacillus and members of the TM7 phylum were eliminated or decreased due to BMD [22]. Antibiotic resistance genes (n= 155) were annotated in the assembled metagenomic contigs, some of which contained multiple classes of AMR (i.e., aminoglycoside and streptothricin resistance genes) or a resistance gene(s) with a mobile genetic element. Complete type G vancomycin resistance operons (vanRSHGXY) were detected together on contigs and were enriched due to BMD. This project demonstrated that that BMD appears to co-select for many types of ARGs, including many important for human medicine.
Investigators from CA, IA, IL, IN, KS, MD, MI, MN, NC, ND, NE, NH, NY, OH, PA, RI and SD worked together on a variety of projects aimed at alternative approaches and potential mitigation of ARG transmission. One study evaluated the impact of added Cu alone or in combination with an antibiotic selection pressure in the form of a feed-grade antimicrobial (chlortetracycline; CTC) on growth performance, Cu resistance gene (tcrB) prevalence, and AMR of fecal Enterococci in weaned piglets. The impact of added Cu and CTC on tcrB was assessed through the development, validation, and utilization of real-time, quantitative PCR (qPCR) to quantify the abundance of tcrB in fecal community DNA. Other projects investigated the impact of alternatives to antibiotics and route of antibiotic administration as potentially effective mitigation strategies to diminish the transfer of AMR in food animal production systems [23–26].
Antimicrobial-resistant bacteria and genes may be present in cattle production environments, persist through manure storage and its application on crop lands, and contaminate the environment causing risks for human health. To this end, three treatments - control (no antibiotic use), Tylan, and CTC - were introduced to beef cattle in feed and the prevalence and concentration of resistant bacteria including E. coli, Salmonella and Enterococcus were determined in feces, cattle hides, pen surface materials, manure stockpiles, and in manure amended soils. In addition, bio-aerosol samples were collected in the upwind and downwind environment of the beef cattle pens, manure stockpiles and manure application sites to evaluate the transfer of antimicrobial-resistant bacteria through air and/or dust. The major findings of this study are (i) Salmonella was not detected on beef cattle feedlot pen surfaces, manure stockpiles or amended soil throughout the project; (ii) no significant difference was observed in the level of bacteria or resistant bacteria in feces and on hides collected at the feedlot among the three treatment groups; (iii) during a 3-month manure stockpiling, the concentration of generic E. coli and Enterococcus in stockpiled manure dropped more than 100 times and by the end of the storage period, no resistant bacteria were detected or enumerated in almost all samples; (iv) resistant E.coli, Salmonella, or Enterococcus in amended soils were all below the level of quantification; (v) generic E.coli and Enterococcus can be transmitted in air over a distance of up to 80 m at feedlot, manure stockpiles and land application sites, but resistant bacteria were only detected at the manure storage sites at a very low level.
Developing improved diagnostic tests
Investigators from MN have released bioinformatics tools to identify and quantify ARG from next-generation sequencing datasets with high quality, hand-curated reference sequences (MEGARes) and a carefully crafted hierarchical annotation scheme along with AmrPlusPlus software to perform the analysis [27]. A second version of the database has also been released, with updated reference sequences for ARGs as well as metal and biocide resistance genes [28]. Other projects done by this group include work on integrative and conjugative elements, improved analytical tools, and the use of whole genome sequencing to link genotype and phenotype of pathogens involved in bovine respiratory disease [29–31]
Bovine respiratory disease (BRD) is one the most significant and economically costly diseases of cattle production and has a multi-factorial etiology, including viral and bacterial pathogen components. Antimicrobial resistance is an emerging concern relative to BRD as resistance has recently been reported in several BRD-associated pathogens. Investigators from NE, IN, CA and MN have all put efforts toward the investigation of BRD in various projects. A real time multiplex PCR assay (rtPCR) was designed by investigators from NE that detects four genes conferring macrolide erm(42), mph(E), msr(E)) or tetracycline (tetH) resistance in Mannheimia haemolytica. Overall detection of resistance genes by rtPCR had mostly moderate levels of agreement with isolation of resistant M. haemolytica from lung tissues. The presence of other integrative conjugative element (ICE)-containing organisms within the lung may have increased the level of disagreement between qPCR detection and bacterial isolation [32,33]. Investigators from IN developed loop-mediated isothermal amplification primers to target M. haemolytica, Pastuerella multocida, and Histophilus somni with high accuracy and short time to detection [34].
Objective 2: Develop and evaluate antimicrobial use, resistance transmission, mitigation strategies, and stewardship programs in food systems from a One-Health perspective.
Antimicrobial use and stewardship
Investigators from KS and collaborators from IA studied antibiotic selection and route of administration as mitigation strategies to reduce AMR in food animal production systems. They investigated the association between antimicrobial drug selection for treating BRD and outcomes in feedlot cattle. Antimicrobial pharmacodynamic (bactericidal vs. bacteriostatic) impacted outcomes upon re-treatment for BRD. Consecutive treatments with bactericidal drugs resulted in better health and production outcomes when compared to treatment with bacteriostatic drugs [25]. Studies on the impact of oxytetracycline on swine intestinal microbial diversity and ARG abundance found that fecal ARG abundance was higher in animals treated with in-feed antimicrobials compared to parenteral antimicrobial administration [26]. Other studies from that group include the examination of the association of fluoroquinolone usage with the development of AMR in Campylobacter and how the antibiotic treatment influences the microbiota balance in the intestinal tract.
Researchers from CA, PA, and NY have collaborated to understand differences in milk microbiota between cows treated with antimicrobials at dry-off and those not treated with antibiotics. The results of this work were presented at the Conference of Research Workers in Animal Disease (CRWAD) in 2018 [35]. They also sought to understand whether method of selective dry cow therapy (culture- or algorithm-based) reduced the prevalence of new intramammary infections and observed no significant differences between methods[36].
Another team comprised of investigators from MN, IA, and NY completed a project to develop and disseminate effective selective dry cow therapy (SDCT) strategies to reduce AMU at dry-off in dairy cows. This included a multi-location, non-inferiority randomized clinical trial and stochastic modeling to evaluate two different SDCTs and their effect on cow health, performance, AMU, and economics. The results demonstrated that SDCT was successful in maintaining animal health while reducing AMU at dry-off by more than 50%, bringing significant economic savings to dairy producers [36–38]. They also evaluated three rapid diagnostic tests for intramammary-infection at dry-off, finding that none had both a high sensitivity and specificity [39]. Finally, they examined the impact of AMU in uninfected cows on the milk microbiome, udder health, and early lactation performance and found no difference between treated and untreated cows, although sample size was smaller than expected. The results of this work were disseminated through a podcast, articles in the lay press, and statewide dairy associations.
The economics of using genomic selection to reduce AMU for clinical mastitis and metritis was the focus of a study that included collaborators from NY and FL. They compared four genomic selection strategies, including selection for disease resistance, and predicted the future (15-year) genetic and economic performance for dairy farms that adopt these strategies. They found that selecting for resistance to mastitis and metritis was less profitable than selecting for lifetime net merit [40].
Knowing the perceptions of dairy farmers, veterinarians, and consumers about barriers to and motivations for judicious AMU in dairy operations is crucial to guide our efforts in AMR mitigation. A team from NY, IN, CA, and MD collaborated to understand these perceptions through a survey of adults on purchasing decision when it pertains to AMU at the farm [8]. This study revealed that perceptions of AMU have no effect on purchasing decision, and that consumers familiar with the Veterinary Feed Directive are more likely to oppose the organic law. Fifty percent of consumers would be willing to purchase milk with responsible AMU certification, but they would not pay a premium for it [41]. The group will use these results in a mathematical model to determine how much AMR would decrease with a reduction in AMU on dairy farms. Investigators from NE are joining this effort with the goal of investigating stakeholder perceptions of AMR prevalence and conveyance in the environment.
Investigators from MS and MN built a mathematical model of diseases in poultry production systems to investigate antimicrobial-resistant foodborne pathogens and AMU on farms. This model has been made available to producers and other stakeholders who can use it to compare the efficacy and cost of different strategies for reducing AMU and mitigating AMR [42].
AMR transmission and mitigation strategies
Research from NY and NC used machine learning and statistical models to monitor AMR in meat from slaughter to retail. They assessed variation in MDR in publicly available NARMS data using classical statistical methods, graphical networks, and machine learning approaches. Many differences were observed in the MDR patterns of bacteria collected from slaughterhouses (carcasses) and retail meats. It was concluded that their machine learning method agreed with the other approaches but provided greater flexibility for studying MDR in large datasets [16].
A team from MN collaborated with investigators in TX and DE to determine how antibiotics and probiotics work and their effects on animal growth and immune system modulation in commercial turkeys. The work included a comprehensive and systems approach to identify relationships between (1) natural turkey intestinal microbiome succession and host development and (2) intestinal microbiome modulations and host status. They also studied the genetic aspects of host adaptation to turkeys in Lactobacillus johnsonii. Finally, they developed a 4-strain probiotic cocktail that enhanced turkey performance [43].
Investigators from NC and CA worked together to understand emergence, horizontal gene transfer (HGT), and resilience of AMR in agricultural ecosystems. Their main findings were that MDR Campylobacter extensively colonized turkeys grown in close proximity to other turkey farms and was uncommon in turkeys grown at a distance from other farms [44], and that survival of Campylobacter in turkey feces and water depended on genotype and MDR profile [45]. The team also discovered that commercial probiotics and prebiotics protected the ileal mucosa of poultry exposed to Salmonella and Campylobacter [46]. Separately, they found that ceftiofur treatment did not substantially impact the prevalence or ceftiofur resistance of Campylobacter species in beef cattle [47]. Finally, they investigated the escape of AMR pathogens from animal agriculture operations due to floodwaters associated with hurricane Florence [48].
Understanding the mechanisms of AMR and ARG in dairy farms was the objective of the work carried out by a team including investigators from CA and NC. They aimed to identify locations where ARG accumulate and could be reservoirs for dissemination, specifically examining cattle in different production stages and management units. In this study, they found that E. coli and Enterococcus were most often resistant to tetracycline and macrolides-lincosamides, respectively. Dairy cattle of different ages and in different units were found to harbor bacteria with different AMR phenotypes and genotypes [49,50].
A team from NE collaborated with HI researchers to evaluate potential intervention strategies to mitigate livestock-origin AMR. They have been investigating beef cattle systems, studying interventions on feedlot surface, manure storage, and land application [17,51]. The group also developed a metagenomic sequencing approach that can achieve high-throughput and gene quantification in environmental samples. This can be a useful tool for monitoring AMR and ARG in agroecosystems [52,53].
Researchers from IA, TN, KS, and GA studied microbial ecologic and metagenomic strategies to reduce AMR in foodborne pathogens. They have examined the microbiomes of swine and turkeys with and without antibiotic or non-antibiotic feed additives or treatments. They found that oxytetracycline fed to post-weaned pigs had a larger impact on microbiota than parenteral oxytetracycline administration [26,54]. They also examined the interaction of the intestinal immune system and commensal bacteria, and characterized intra-epithelial T cells that play a critical role in barrier integrity and immune defense in swine intestines [55]. Another study demonstrated shifts in the cattle enteric microbiome following vaccination for E. coli O157:H7 [56]. Finally, they evaluated environmental and host influences on enteric bacterial ecology and foodborne pathogen control strategies and identified poultry litter characteristics and microbiota that could exclude or reduce the growth of Salmonella Heidelberg [57].
Finally, plasmid-mediated transfer of antibiotic resistance genes to Enterococcus faecalis JH2-2 in poultry litter was investigated by a group from IN and IA. In this study it was observed that Enterococcus faecalis JH2-2 usually acquires two resistance genes at a time (manuscript in preparation).
Objective 3: Create and deliver programs on antibiotic stewardship in food production systems through education and outreach.
A group comprised of investigators from NE and IA collaborated to create a One-Health certification program focused on responsible AMU audited by the USDA Agricultural Marketing Service Process Verified Program [58]. To be certified, participating farms are expected to report entire AMU and outcomes on an annual basis, along with information used to calculate sustainability metrics.
Findings from AMR studies were communicated to people working in food production systems in an online course developed by a team from IN. The course is divided into different commodity production types with a specific course for dairy systems that highlights AMR dry-cow therapy research outcomes, using similar structures for other commodities. Additionally, the expanded Purdue Food Animal Education Network program uses web and digital based experiential learning to disseminate AMR information to consumers who have little to no connection to livestock production.
Researchers from MD, NY, and NE worked in collaboration with USDA Agricultural Research Service, the University of Maryland – Baltimore County and Ithaca College to create video, web and print information on AMR prevalence, treatment, stewardship and perceptions. This group also investigated the language used surrounding AMR in One-Health domains through text data mining and natural language processing [59]. In addition, MD, NY, MI and NE worked together to host a national workshop of AMR social science research leaders to discuss approaches to improving AMR communication using science communication[60,61].
A comprehensive education and extension effort, iAMResponsible, was developed by a team from MD, NY, MI and NE [62,63]. This group has a rapidly growing social media presence on Twitter [61], YouTube, Instagram, and Facebook and focuses on AMR related to food safety issues.
With the objective of addressing prioritized gaps in knowledge related to AMU, stewardship, and resistance, a multi-organization consortium was founded by Iowa State University, University of Nebraska Lincoln, the Association of Public and Land-Grant Universities, and the Association of American Veterinary Medical Colleges. The National Institute of Antimicrobial Resistance Research and Education (NIAMRRE) was established in early 2019 and includes participating members from IA, KS, NC, IL, OH, CA, FL, GA and NE [64]. Housed at Iowa State, this organization currently has over 30 members including these academic institutions. The program works to address education and extension by providing regular webinars, starting conversations around key topics, and by providing an online community that encourages discussion and collaboration. To date, an online community connecting members has been developed, an annual conference occurred on April 2021, and several roundtables, webinars, and research updates have been developed and hosted.
Objectives
-
Develop knowledge and tools to improve antimicrobial stewardship.
Comments: This will be accomplished by enhancing surveillance and monitoring of antibiotic resistance, developing improved diagnostic tests, and determining the ecology and mechanisms involved in resistance and transmission of resistance. -
Develop and evaluate antimicrobial use, resistance transmission, mitigation strategies, and stewardship programs in food systems from a One-Health perspective.
Comments: This will be accomplished by investigation of several aspects of animal health, public health, social, economic, and environmental impacts of antimicrobial interventions in food production systems. -
Create and deliver programs on antibiotic stewardship in food production systems through education and outreach.
Comments: This will be accomplished by the creation and delivery of various forms of instructional material targeted at diverse audiences, and specifically tailored for each. Additionally, public engagement will be achieved through various mechanisms, including social media content, extension activities, and meetings.
Methods
Objective 1: Develop knowledge and tools to improve antimicrobial stewardship
Despite the extensive work performed in the studies outlined above, several questions remain unanswered when it pertains to the ecology and mechanisms of ARG selection and transmission across the food supply, particularly under different management conditions. Furthermore, knowledge on AMR transmission encompassing animals, humans, and the environment as a single interconnected system continues to be scarce In Objective 1, collaborative projects will mainly focus on a One-Health approach for the surveillance and monitoring of antibiotic resistance, development of improved diagnostic tests, and determination of the ecology and mechanisms involved in resistance and transmission of resistance across the food supply.
Surveillance and monitoring of antimicrobial resistance
Research teams will apply an AMR profiling method (WaferGen) to determine the abundance of hundreds of ARGs in production animals from a farm-to-fork perspective [20]. Investigators from PA and NY will evaluate the composition and presence of ARGs across various livestock production systems and identify management practices that can be adopted to decrease the prevalence of pathogenic bacteria and the spread of ARGs across the food supply [21]. Specifically, they will apply a recently developed, highly multiplexed amplicon sequencing platform to track ARGs from farm-to-fork. Additionally, they will compare the effects of antibiotics, probiotics, prebiotics, and placebo in broilers in a randomized, controlled trial.
The team from IN will perform comprehensive and integrated risk-based research and outreach to improve the safety of food from farm to fork. Specifically, they aim to understand the prevalence and frequencies of pathogens and AMR within the environment, food products, and food production processing, distribution, and consumer systems. Toward that goal they will employ a functional metagenomic approach to identify AMGs in microbial reservoirs [65,66].
Determining the ecology and mechanisms involved in resistance and transmission of resistance
Researchers from CA will investigate the prevalence of AMR in cow-calf operations on pasture and the potential role of recycled flush and lagoon water as a reservoir and mode of transmission of antibiotic residues and antimicrobial-resistant organisms. The impact of antimicrobial therapy for BRD on resistance of fecal E. coli in neonatal and pre-weaned dairy calves will be determined by 1) characterizing the AMR phenotype of fecal E. coli , 2) characterizing the ARGs of fecal E. coli, and 3) estimating and calculating the risk of phenotypic and genotypic resistance to antimicrobial therapy for BRD. Investigators from PA will determine the role of microbiome assembly and succession in the rise and establishment of AMR in poultry production systems.
Developing improved diagnostic tests
Investigators from MN will continue to improve and develop bioinformatics tools to identify and quantify ARGs from next-generation sequencing datasets with high quality, hand-curated reference sequences [27]. A special focus will be placed on the role of selection of heavy metal resistance mechanisms to ARGs, as well as biocide resistance genes [28]. This group will continue to work on integrative and conjugative elements, improved analytical tools, and the use of whole genome sequencing to link genotype and phenotype of pathogens involved in BRD [29–31]
Objective 2: Develop and evaluate antimicrobial use, resistance transmission, mitigation strategies, and stewardship programs in food systems from a One-Health perspective.
Further work is necessary to address remaining questions on the impact of different management strategies and AMU in diverse production systems. In Objective 2, collaborative projects will focus on the influence of AMU and transmission of resistant microorganisms and determinants throughout the food supply chain and associated environments. They will also facilitate the development of effective mitigation strategies and stewardship programs in food systems for combating AMR from a One-Health perspective. The projects across multiple states will collectively contribute to the proactive and concerted efforts for AMR mitigation.
Antimicrobial Use and Stewardship
The team from NY will investigate different economically and ethically justifiable antimicrobial stewardship programs to reduce antibiotic use. Specifically, the investigators will 1) adapt an existing whole-herd, agent-based simulation model to incorporate pathogen-specific mastitis incidence and associated production losses at the cow level; 2) devise effective and practical antimicrobial stewardship programs that combine genomic selection of replacements with therapeutic and preventive pathogen-specific mastitis management programs, and 3) compare herd performance under the devised antimicrobial stewardship programs to find the programs which optimize antibiotic use relative to pathogen-specific mastitis incidence and farm profits. A promising alternative to AMU that will be evaluated by the team is the pre-conditioning of weaned calves to a dry feed diet prior to sale, from both animal health and economic impact perspectives.
Investigators from CA will determine the AMR profiles of fecal E. coli in response to common BRD antimicrobial drug therapies used in pre-weaned dairy calves. Specifically, the team will characterize the AMR phenotype and genotypes of fecal E. coli isolated from dairy calves at birth and weaning for calves receiving antimicrobial therapy for BRD, and estimate and correlate the risk of phenotypic and genotypic resistance to antimicrobial therapy for BRD in pre-weaned dairy calves. In addition, the team will employ a collaboration approach between calf operators and researchers to provide recommendations on effective, realistic, and prudent antibiotic treatments of diarrheic pre-weaned calves.
The researchers from NE, IA, MD, NY, and MI will continue their collaborative education and extension efforts to disseminate AMR-related knowledge across the food production industry, academic community and the general public through workshops, a media initiative (iAMResponsible), and the activities organized by National Institute of Antimicrobial Resistance Research and Education (NIAMMRE).
AMR transmission and mitigation strategies
Investigators from NE and HI are applying risk-based, systems approach for the identification of critical control points and evaluation of potential intervention strategies to mitigate public health risks imposed by livestock-originated AMR [67]. Beef cattle are used as the model system. Multiple interventions used on feedlot surfaces, during manure storage, and at land application are identified and their efficacies are being evaluated. Inactivation of antimicrobial-resistant bacteria, genes, and human exposure through consumption of fresh produce grown in the field treated with livestock manure are being quantified.
A novel approach to control poultry respiratory diseases, including the development of new vaccines and alternatives to antimicrobials, is being developed by investigators from OH, MD, SD, and IA. These alternatives are designed to inhibit enzymatic reactions without exerting selective pressure on the targeted bacterium. Toward this end, the group has identified several novel small molecules that inhibit avian pathogenic E. coli and Mycoplasma gallisepticum with low toxicity [68,69]. They also identified new antibacterial targets in these pathogens [70].
Collaborators from NY, MI, and NE are developing a mathematical modeling study to identify new and improved approaches to control AMR in heifer raising operations. Their goals are to (i) evaluate the dynamics of AMR in generic Escherichia coli; (ii) evaluate the dynamics of AMR and spread of Salmonella Dublin; and (iii) develop and disseminate education materials about control of AMR and associated infections in dairy farming. Preliminary results suggest that increased nutrients from deposited manure may support the proliferation of AMR irrespective of antibiotic use.
Manure treatment technologies to mitigate AMR in dairy operations are being investigated by collaborators in NY and MD. They found that composting does not completely degrade antibiotics in manure [71]. A study of on-farm solid-liquid separator and rotary drum composter manure treatment systems revealed variable effects on different antimicrobial classes and ARG, with the rotary drum significantly reducing ARGs [72]. This effort was further developed by the joining of investigators from MI, who examined anaerobic digestion, and found substantial reductions in sulfadimethoxine but variable reductions in tetracycline concentrations [15]. This collaborative project will continue investigating strategies to reduce ARGs in manure with the objective of decreasing the environmental impact and transmission of ARGs.
Investigators from MN, TX, CO and MO will conduct a field trial to investigate the impacts of post-processing commingling and a high-fiber, post-weaning diet on AMR dynamics after metaphylaxis in swine. Through multivariable modeling and network analysis of time-series metagenomic data, the investigators will identify when and how commingling and diet reduce AMR mechanisms and which microbial taxa significantly alter AMR dynamics. This group will develop a discriminative tool for source tracking and attribution of AMR in the environment based on resistome fingerprints generated using metagenomic data. The group at UMN is collaborating with industry partners from CA to develop a method for tracking and source attribution of AMR in environmental systems using metagenomic data and computational algorithms. Thus far, they have developed highly validated metagenomic and synthetic datasets and completed a systematic review of current bioinformatics tools [73].
Investigators from CA, ID, MI, NC, and SC will use whole genome sequencing to identify AMR determinants and the transposons that may harbor some of these determinants in Campylobacter and Listeria strains from food animals. In a laboratory setting, the capacity of inter-strain transfer of tetracycline resistance will be evaluated through the employment of conjugations and transformations. In addition, resistance transfer will be evaluated in real-world matrices, including surface water and food contact surfaces during processing.
Investigators from CA will determine the role of recycled lagoon water in disseminating resistant bacteria and antibiotic residues in the dairy production-related environment. This group will disseminate knowledge regarding control of antimicrobial drug residues during manure management through extension education programs.
Investigators from PA and NY will evaluate the composition and presence of ARGs across various livestock production systems and identify management practices that can be adopted to decrease the prevalence of pathogenic bacteria and the spread of ARGs across the food supply [21]. Specifically, they will apply a recently developed, highly multiplexed amplicon sequencing platform to track ARGs from farm-to-fork. Additionally, they will compare the use of antibiotics, probiotics, prebiotics, and placebo in broilers in a randomized, controlled trial.
The team from IN will perform comprehensive and integrated risk-based research and outreach to improve the safety of food from farm to fork. Specifically, they aim to understand the prevalence and frequencies of pathogens and AMR within the environment, food product and processing, distribution, and consumer systems. Toward this goal they will employ a functional metagenomic approach to identify ARGs in microbial reservoirs [65,66].
The team from MI will address gaps in the knowledge of AMR ecology of enteric bacteria in food animals, develop effective interventions to reduce AMR in enteric bacteria in livestock, and explore alternatives to AMU for treatment of mastitis in dairy cattle. Specifically, their goals are to describe temporal changes in AMR in dairy cattle, determine if probiotics can be used to reduce AMR in pre-weaned dairy calves, and determine if Manuka honey is a practical and safe alternative to antibiotic treatment for mastitis in dairy cattle.
Objective 3: Create and deliver programs on antibiotic stewardship in food production systems through education and outreach.
Education and outreach are critical aspects of improving antimicrobial stewardship in food production systems. The current lack of connection between human, animal, and environmental health in AMR education is a prime example of work needed in this realm. Moreover, currently available educational materials are often siloed to a single group and very little cross-training exists between stakeholders involved in aspects of human, animal, and environmental health.
In developing education and outreach materials, there is a wide variety of audiences that need to be considered. Certainly, materials that assist antibiotic prescribers (i.e., veterinarians) in making good antibiotic stewardship decisions are critical, and this has been a primary focus of stewardship efforts in human medicine. Resources such as antibiograms, client education materials, continuing education opportunities, and tools that allow veterinarians to benchmark and compare their prescribing habits all aid in this effort. The collective body of this team will work together to identify, prioritize, and develop these materials through several avenues. Additionally, education and outreach efforts focused on antibiotic users, antibiotic decision makers (i.e., farm personnel who may be responsible for using standard operating procedures to use prescribed antibiotics when necessary), policy makers, and the general public are important. Finally, effective translation of knowledge gained through this project from the research laboratory to the field is critical and will be a driver of education and outreach efforts. As results are reported, the team will evaluate which audiences need to hear the message and develop mechanisms to effectively disseminate information to those audiences.
Many of these efforts will be developed as project-specific outcomes, targeted to the most appropriate audience and message. We will use a variety of channels to disseminate information, including but not limited to social media, extension and outreach publications, field days, and online modules. The collective expertise of our institutions in these educational efforts will be leveraged to broaden the message by disseminating information from multiple institutions when appropriate. Evaluation of outcomes will be monitored using a variety of channels and audience- specific metrics and will include (when feasible) the number of stakeholders reached, the level of engagement with the information, and the perceived value or benefit of the information.
In addition to our broader effort, we will also utilize two existing and growing collaborative mechanisms to foster education and outreach related to our work. The first of these is the iAMResponsible effort that is a collaboration of MD, NY, MI, and NE. This is a social media-based education and outreach platform with over 4000 followers across channels on Twitter, Facebook, Instagram, and YouTube. The audience is primarily the general public with an interest in food safety. We will actively monitor this project for messages appropriate to the audience and develop messaging to disseminate research outcomes, foster improved antimicrobial stewardship, and educate the public.
The second focused effort will include members from IA, KS, NC, IL, OH, CA, FL, GA and NE through the National Institute of Antimicrobial Resistance Research and Education (NIAMRRE, niamrre.org). NIAMRRE will undertake two significant education and outreach focused efforts as part of this program: one focused on AMR learning outcomes and objectives, and a second focused on inter-professional education.
The recent release of the Presidential Advisory Council for Combating Antimicrobial Resistant Bacteria (PACCARB) report entitled Bridging the Gap: Improving Antimicrobial Access and Use Across One Health [74] highlights the need for a harmonized and shared AMR curriculum. In 2015, the Association of Public and Land Grant Universities collaborated with the American Association of Veterinary Medical Colleges to develop an AMR Learning Outcomes document with outcomes appropriate to veterinarians, undergraduate animal scientist and 4-H/FFA. However, this document was focused on animal agriculture as opposed to taking a broader One-Health perspective. The team at NIAMRRE will work to update these outcomes (APLU and AAVMC have endorsed this effort) to better reflect a One-Health perspective, broadening them to include human and environmental health components and providing the framework for the type of harmonized and shared curriculum recommended by PACCARB. Following finalization of the updated learning outcomes, we will develop and implement a plan for benchmarking how effective our institutions are in teaching these outcomes, as well as online materials to help educators assure that these outcomes are accessible and understandable to a diversity of audiences.
In a second report released by PACCARB entitled Advancing Interprofessional Education and Practice to Combat Antimicrobial Resistance [75] the committee highlighted the need for improving AMR integration into inter-professional education efforts. This includes harmonizing perspectives of One-Health and inter-professional education which are commonly siloed into veterinary and human health respectively. While many such inter-professional programs exist, the committee found that almost none of them use AMR as an exemplar in their programs despite the clear benefit of during so for improving stewardship. In addition, many programs fail to effectively engage professionals across One-Health. Taking a One-Health approach includes engaging audiences that have previously not been considered “standard” in the inter-professional health efforts including extension agents, veterinary pharmacists, animal health antibiotic decision makers (i.e., producers or employees making decisions about specific animals to treat), and feed store owners. The team at NIAMRRE will work to develop a hybrid online training and virtual discussion community prototype to demonstrate the ability to deliver this type of education to geographically distributed participants in an effective manner. Once developed, the group will work to broaden participation in the activity.
Measurement of Progress and Results
Outputs
- A comprehensive catalogue of ARGs and resistance-gene variants in pathogens of importance in agricultural production systems. Comments: Research findings and collaborative projects will be reported on a yearly basis throughout the NC 1206 program lifetime.
- Evidence on how treatment with antimicrobial drugs impacts the host/pathogen interface and how it affects the resistome and selection and dissemination of AMR Comments: Research findings and collaborative projects will be reported on a yearly basis through the NC 1206 program lifetime.
- Direct fed microbials, dietary formulations, phytochemicals, new vaccines, and novel therapeutic agents to improve gut health and reduce pathogen colonization and AMR. Comments: Research findings and collaborative projects will be reported on a yearly basis through the NC 1206 program lifetime.
- Biological indicators, assays, and models to evaluate the impact of different AMU scenarios in food-producing animals on animal, human health, environmental protection, and related social-economic effects. Comments: Research findings and collaborative projects will be reported on a yearly basis through the NC 1206 program lifetime.
- Population studies evaluating the impact of different AMU strategies in food-producing animals and their results on AMR dissemination through the food supply chain. Comments: Research findings and collaborative projects will be reported on a yearly basis through the NC 1206 program lifetime.
- Educational programs tailored to diverse audiences, using a variety of channels to disseminate information on AMR including but not limited to social media, extension and outreach publications, field days and online modules. Comments: Research findings and collaborative projects will be reported on a yearly basis through the NC 1206 program lifetime.
- Interprofessional educational programs using AMR as the focus will be developed and delivered with a One-Health perspective. Comments: This program will aim at breaking barriers of inter-professional education which are commonly siloed into veterinary and human health and provide a more seamless platform for antimicrobial resistance education. Research findings and collaborative projects will be reported on a yearly basis through the NC 1206 program lifetime.
- Practices and recommendations to reduce the development and spread of AMR in various sections of the farm-to-fork continuum. Research findings and collaborative projects will be reported on a yearly basis through the NC 1206 program lifetime. Comments: Research findings and collaborative projects will be reported on a yearly basis through the NC 1206 program lifetime.
Outcomes or Projected Impacts
- Improved tracking and identification of current and newly identified resistance genes and variants will result in better antibiotic stewardship methods.
- Development and implementation of new platforms for studying the resistome in animals will facilitate monitoring of AMR, the development of practical means to reduce AMR and its transmission among livestock, the environment, and humans.
- Novel non-antibiotics developed through our collaborative efforts will lower the amount of antibiotics used in food production systems and hence reduce the development of drug resistance in enteric bacteria
- Providing scientific evidence to food animal producers, food processors and other members of the food value chain, extension educators and policy makers will maximize the judicious use of antimicrobials in food-producing animals by weighing benefits and risks of their uses in animal, human health, and environmental sustainability.
- Delivering scientifically sound evidence to all parties in the food supply chain will maximize the judicious use of antimicrobial drugs and reduce AMR in food-producing animals.
- Providing inter-professional educational programs that use AMR as the focus and present information with a One-Health perspective will enhance the understanding of the multifactorial nature of AMR.
Milestones
(0):The agricultural systems in which AMR emerge, evolve, accumulate, and disseminate are exceedingly complex. Hence, these problems dictate a trans-institutional and interconnected trans-disciplinary approach creating collaborations among people with different and overlapping areas of expertise. We have brought together a group of investigators under the umbrella of AMR with noticeably diverse areas of expertise ranging from molecular biology/microbiology, modeling, epidemiology, statistics, infectious diseases, AMR mitigation, applied research and education, and extension/outreach. Research activities described in the above-described objectives represent extensive inter- and intra-disciplinary collaboration amongst and between objectives. Progress in meeting our objectives will be measured on an annual basis in terms of current and new collaborations within and across objectives; work completed; publications (peer reviewed and otherwise); outputs in terms of students graduated; students mentored; development of outreach materials; presentations of research at professional and other meetings; and additional funding secured on the basis of the research and extension activities generated.(2023):The first inter-professional educational programs that use AMR as the focus and present information with a One-Health perspective will be delivered by 2023.
(2025):New platforms for characterization of AMR will be developed and shared between project members by 2025. This will facilitate the investigation of management practices that can be used as tools to improve antimicrobial stewardship.
Projected Participation
View Appendix E: ParticipationOutreach Plan
Outreach and education are critical elements in efforts to control and mitigate AMR on livestock premises, and they are advocated for by national and international organizations. The stakeholders, with respect to AMR in livestock, are many and diverse with different perspectives and often conflicting agendas. Therefore, our basic message needs to be clear and consistent with room for customization for each unique audience. We will develop a coordinated effort to create communication and appropriate messages for all involved parties. Our outreach and extension program will engage students, veterinarians, extension personnel, producers, and their employees to increase understanding of the importance of antibiotic management decisions necessary to implement antibiotic stewardship and AMR mitigation programs. Our messages will be delivered to audiences through the previously established media platform - iAMResponsible, a social media-based education and outreach platform with informational pages and educational videos that has just over 4000 followers (primarily the general public) across channels on Twitter, Facebook, Instagram, and YouTube. The group at the National Institute of Antimicrobial Resistance Research and Education (NIAMRRE, niamrre.org) will work on educational courses, and materials will be tailored to communicate important information about AMR, animal and human health, and environmental sustainability to various audiences. Additionally, findings from studies conducted as part of this program will be presented at national scientific and producer organization meetings and workshops and through undergraduate and graduate level courses at participating universities. We will document all outputs, activities, and impacts related to NC 1206, and we will measure where capacity to improve antimicrobial stewardship and AMR control has been strengthened.
Organization/Governance
The recommended Standard Governance for multistate research activities include the election of a Chair, a Chair-elect, and a Secretary. All officers are to be elected for at least two-year terms to provide continuity. Administrative guidance will be provided by an assigned Administrative Advisor and a NIFA Representative.
Literature Cited
1. ANTIMICROBIAL RESISTANCE Global Report on Surveillance;
2. SECURING NEW DRUGS FOR FUTURE GENERATIONS: THE PIPELINE OF ANTIBIOTICS;
3. Drug-Resistant Infections: A Threat to Our Economic Future Available online: https://www.worldbank.org/en/topic/health/publication/drug-resistant-infections-a-threat-to-our-economic-future (accessed on 3 April 2020).
4. WHO | ANTIBACTERIAL AGENTS IN CLINICAL DEVELOPMENT. WHO 2017.
5. WHO | ANTIBACTERIAL AGENTS IN CLINICAL DEVELOPMENT Available online: https://www.who.int/medicines/areas/rational_use/antibacterial_agents_clinical_development/en/ (accessed on 3 April 2020).
6. CDC Antibiotic Resistance Threats in the United States; Atlanta, GA, 2019;
7. (FDA), F. and D.A. NARMS Now Available online: https://www.fda.gov/animal-veterinary/national-antimicrobial-resistance-monitoring-system/narms-now-integrated-data.
8. CDC What CDC Is Doing: Antibiotic Resistance (AR) Solutions Initiative Available online: https://www.cdc.gov/drugresistance/solutions-initiative/ (accessed on 4 April 2020).
9. CDC About Food and Antibiotic Resistance Available online: https://www.cdc.gov/drugresistance/solutions-initiative/food.html (accessed on 3 April 2020).
10. USDA AFRI Stakeholder Feedback - Food Safety. 2016.
11. Wilson, J.B.; Salman, M.; Janzen, E.; Sparagano, O.; Speer, N.; Pantaleon, L.; La Jeunesse, C.; Häsler, B.; Wills, M.; Rielander, D.; et al. Community Network Integration: An Approach to Alignment of One Health Partners for Solutions to ‘Wicked’ Problems of Antimicrobial Resistance. Preventive Veterinary Medicine 2020.
12. Prestinaci, F.; Pezzotti, P.; Pantosti, A. Antimicrobial Resistance: A Global Multifaceted Phenomenon. Pathogens and Global Health 2015, 109, 309–318.
13. Oliver, J.P.; Gooch, C.A.; Lansing, S.; Schueler, J.; Hurst, J.J.; Sassoubre, L.; Crossette, E.M.; Aga, D.S. Invited Review: Fate of Antibiotic Residues, Antibiotic-Resistant Bacteria, and Antibiotic Resistance Genes in US Dairy Manure Management Systems. Journal of dairy science 2020, 103, 1051–1071, doi:10.3168/JDS.2019-16778.
14. Hurst, J.J.; Oliver, J.P.; Schueler, J.; Gooch, C.; Lansing, S.; Crossette, E.; Wigginton, K.; Raskin, L.; Aga, D.S.; Sassoubre, L.M. Trends in Antimicrobial Resistance Genes in Manure Blend Pits and Long-Term Storage Across Dairy Farms with Comparisons to Antimicrobial Usage and Residual Concentrations. Environmental science & technology 2019, 53, 2405–2415, doi:10.1021/ACS.EST.8B05702.
15. Schueler, J.; Lansing, S.; Crossette, E.; Naas, K.; Hurst, J.; Raskin, L.; Wigginton, K.; Aga, D.S. Tetracycline, Sulfadimethoxine, and Antibiotic Resistance Gene Dynamics during Anaerobic Digestion of Dairy Manure. Journal of environmental quality 2021, 50, 694–705, doi:10.1002/JEQ2.20211.
16. Cazer, C.L.; Al-Mamun, M.A.; Kaniyamattam, K.; Love, W.J.; Booth, J.G.; Lanzas, C.; Gröhn, Y.T. Shared Multidrug Resistance Patterns in Chicken-Associated Escherichia Coli Identified by Association Rule Mining. Frontiers in microbiology 2019, 10, doi:10.3389/FMICB.2019.00687.
17. Staley, Z.R.; Tuan, C.Y.; Eskridge, K.M.; Li, X. Using the Heat Generated from Electrically Conductive Concrete Slabs to Reduce Antibiotic Resistance in Beef Cattle Manure. The Science of the total environment 2021, 768, doi:10.1016/J.SCITOTENV.2020.144220.
18. Staley, Z.R.; Schmidt, A.M.; Woodbury, B.; Eskridge, K.M.; Durso, L.; Li, X. Corn Stalk Residue May Add Antibiotic-Resistant Bacteria to Manure Composting Piles. Journal of environmental quality 2020, 49, 745–753, doi:10.1002/JEQ2.20017.
19. Davidson, K.E.; Byrne, B.A.; Pires, A.F.A.; Magdesian, K.G.; Pereira, R. v. Antimicrobial Resistance Trends in Fecal Salmonella Isolates from Northern California Dairy Cattle Admitted to a Veterinary Teaching Hospital, 2002-2016. PloS one 2018, 13, doi:10.1371/JOURNAL.PONE.0199928.
20. Muurinen, J.; Richert, J.; Wickware, C.L.; Richert, B.; Johnson, T.A. Swine Growth Promotion with Antibiotics or Alternatives Can Increase Antibiotic Resistance Gene Mobility Potential. Scientific Reports 2021 11:1 2021, 11, 1–13, doi:10.1038/s41598-021-84759-9.
21. Gaeta, N.C.; Bean, E.; Miles, A.M.; Carvalho, D.U.O.G. de; Alemán, M.A.R.; Carvalho, J.S.; Gregory, L.; Ganda, E. A Cross-Sectional Study of Dairy Cattle Metagenomes Reveals Increased Antimicrobial Resistance in Animals Farmed in a Heavy Metal Contaminated Environment. Frontiers in Microbiology 2020, 11, doi:10.3389/FMICB.2020.590325/FULL.
22. Johnson, T.A.; Sylte, M.J.; Looft, T. In-Feed Bacitracin Methylene Disalicylate Modulates the Turkey Microbiota and Metabolome in a Dose-Dependent Manner. Scientific reports 2019, 9, doi:10.1038/S41598-019-44338-5.
23. Capps, K.M.; Amachawadi, R.G.; Menegat, M.B.; Woodworth, J.C.; Perryman, K.; Tokach, M.D.; Dritz, S.S.; DeRouchey, J.M.; Goodband, R.D.; Bai, J.; et al. Impact of Added Copper, Alone or in Combination with Chlortetracycline, on Growth Performance and Antimicrobial Resistance of Fecal Enterococci of Weaned Piglets. Journal of animal science 2020, 98, doi:10.1093/JAS/SKAA003.
24. Kuralayanapalya, S.P.; Patil, S.S.; Hamsapriya, S.; Shinduja, R.; Roy, P.; Amachawadi, R.G. Prevalence of Extended-Spectrum Beta-Lactamase Producing Bacteria from Animal Origin: A Systematic Review and Meta-Analysis Report from India. PloS one 2019, 14, doi:10.1371/JOURNAL.PONE.0221771.
25. Coetzee, J.F.; Cernicchiaro, N.; Sidhu, P.K.; Kleinhenz, M.D. Association between Antimicrobial Drug Class Selection for Treatment and Retreatment of Bovine Respiratory Disease and Health, Performance, and Carcass Quality Outcomes in Feedlot Cattle. Journal of animal science 2020, 98, doi:10.1093/JAS/SKAA109.
26. Ricker, N.; Trachsel, J.; Colgan, P.; Jones, J.; Choi, J.; Lee, J.; Coetzee, J.F.; Howe, A.; Brockmeier, S.L.; Loving, C.L.; et al. Toward Antibiotic Stewardship: Route of Antibiotic Administration Impacts the Microbiota and Resistance Gene Diversity in Swine Feces. Frontiers in veterinary science 2020, 7, doi:10.3389/FVETS.2020.00255.
27. Lakin, S.M.; Dean, C.; Noyes, N.R.; Dettenwanger, A.; Ross, A.S.; Doster, E.; Rovira, P.; Abdo, Z.; Jones, K.L.; Ruiz, J.; et al. MEGARes: An Antimicrobial Resistance Database for High Throughput Sequencing. Nucleic Acids Research 2017, 45, D574–D580, doi:10.1093/NAR/GKW1009.
28. Doster, E.; Lakin, S.M.; Dean, C.J.; Wolfe, C.; Young, J.G.; Boucher, C.; Belk, K.E.; Noyes, N.R.; Morley, P.S. MEGARes 2.0: A Database for Classification of Antimicrobial Drug, Biocide and Metal Resistance Determinants in Metagenomic Sequence Data. Nucleic Acids Research 2020, 48, D561–D569, doi:10.1093/NAR/GKZ1010.
29. Noyes, N.R.; Weinroth, M.E.; Parker, J.K.; Dean, C.J.; Lakin, S.M.; Raymond, R.A.; Rovira, P.; Doster, E.; Abdo, Z.; Martin, J.N.; et al. Enrichment Allows Identification of Diverse, Rare Elements in Metagenomic Resistome-Virulome Sequencing. Microbiome 2017, 5, 142, doi:10.1186/s40168-017-0361-8.
30. Muggli, M.D.; Bowe, A.; Noyes, N.R.; Morley, P.S.; Belk, K.E.; Raymond, R.; Gagie, T.; Puglisi, S.J.; Boucher, C. Succinct Colored de Bruijn Graphs. Bioinformatics (Oxford, England) 2017, 33, 3181–3187, doi:10.1093/BIOINFORMATICS/BTX067.
31. Owen, J.R.; Noyes, N.; Young, A.E.; Prince, D.J.; Blanchard, P.C.; Lehenbauer, T.W.; Aly, S.S.; Davis, J.H.; O’Rourke, S.M.; Abdo, Z.; et al. Whole-Genome Sequencing and Concordance between Antimicrobial Susceptibility Genotypes and Phenotypes of Bacterial Isolates Associated with Bovine Respiratory Disease. G3: Genes, Genomes, Genetics 2017, 7, 3059–3071, doi:10.1534/G3.117.1137/-/DC1.
32. Dutta, E.; Loy, J.D.; Deal, C.A.; Wynn, E.L.; Clawson, M.L.; Clarke, J.; Wang, B. Development of a Multiplex Real-Time PCR Assay for Predicting Macrolide and Tetracycline Resistance Associated with Bacterial Pathogens of Bovine Respiratory Disease. Pathogens (Basel, Switzerland) 2021, 10, 1–21, doi:10.3390/PATHOGENS10010064.
33. Loy, J.D.; Leger, L.; Workman, A.M.; Clawson, M.L.; Bulut, E.; Wang, B. Development of a Multiplex Real-Time PCR Assay Using Two Thermocycling Platforms for Detection of Major Bacterial Pathogens Associated with Bovine Respiratory Disease Complex from Clinical Samples. Journal of Veterinary Diagnostic Investigation 2018, 30, 837–847, doi:10.1177/1040638718800170.
34. Mohan, S.; Pascual-Garrigos, A.; Brouwer, H.; Pillai, D.; Koziol, J.; Ault, A.; Schoonmaker, J.; Johnson, T.; Verma, M.S. Loop-Mediated Isothermal Amplification for the Detection of Pasteurella Multocida, Mannheimia Haemolytica, and Histophilus Somni in Bovine Nasal Samples. ACS Agricultural Science & Technology 2021, 1, 100–108, doi:10.1021/ACSAGSCITECH.0C00072.
35. Biscarini, F.; Cremonesi, P.; Castiglioni, B.; Stella, A.; Bronzo, V.; Locatelli, C.; Moroni, P. A Randomized Controlled Trial of Teat-Sealant and Antibiotic Dry-Cow Treatments for Mastitis Prevention Shows Similar Effect on the Healthy Milk Microbiome. Frontiers in Veterinary Science 2020, 7, 581, doi:10.3389/FVETS.2020.00581/BIBTEX.
36. Rowe, S.M.; Godden, S.M.; Nydam, D. v.; Gorden, P.J.; Lago, A.; Vasquez, A.K.; Royster, E.; Timmerman, J.; Thomas, M.J. Randomized Controlled Trial Investigating the Effect of 2 Selective Dry-Cow Therapy Protocols on Udder Health and Performance in the Subsequent Lactation. Journal of dairy science 2020, 103, 6493–6503, doi:10.3168/JDS.2019-17961.
37. Rowe, S.M.; Godden, S.M.; Nydam, D. v.; Gorden, P.J.; Lago, A.; Vasquez, A.K.; Royster, E.; Timmerman, J.; Thomas, M.J. Randomized Controlled Non-Inferiority Trial Investigating the Effect of 2 Selective Dry-Cow Therapy Protocols on Antibiotic Use at Dry-off and Dry Period Intramammary Infection Dynamics. Journal of dairy science 2020, 103, 6473–6492, doi:10.3168/JDS.2019-17728.
38. Rowe, S.M.; Nydam, D. v.; Godden, S.M.; Gorden, P.J.; Lago, A.; Vasquez, A.K.; Royster, E.; Timmerman, J.; Thomas, M.J.; Lynch, R.A. Partial Budget Analysis of Culture- and Algorithm-Guided Selective Dry Cow Therapy. Journal of dairy science 2021, 104, 5652–5664, doi:10.3168/JDS.2020-19366.
39. Rowe, S.; Godden, S.; Nydam, D. v.; Gorden, P.; Lago, A.; Vasquez, A.; Royster, E.; Timmerman, J.; Thomas, M. Evaluation of Rapid Culture, a Predictive Algorithm, Esterase Somatic Cell Count and Lactate Dehydrogenase to Detect Intramammary Infection in Quarters of Dairy Cows at Dry-Off. Preventive veterinary medicine 2020, 179, doi:10.1016/J.PREVETMED.2020.104982.
40. Kaniyamattam, K.; de Vries, A.; Tauer, L.W.; Gröhn, Y.T. Economics of Reducing Antibiotic Usage for Clinical Mastitis and Metritis through Genomic Selection. Journal of dairy science 2020, 103, 473–491, doi:10.3168/JDS.2018-15817.
41. Bulut, E.; Stout, A.; Wemette, M.; Llanos-Soto, S.; Schell, R.C.; Greiner Safi, A.; Shapiro, M.A.; Moroni, P.; Ivanek, R. How Does Public Perception of Antibiotic Use on Dairy Farms Contribute to Self-Reported Purchasing of Organic? Journal of food science 2021, 86, 2045–2060, doi:10.1111/1750-3841.15720.
42. Galarneau, K.D.; Singer, R.S.; Wills, R.W. A System Dynamics Model for Disease Management in Poultry Production. Poultry science 2020, 99, 5547–5559, doi:10.1016/J.PSJ.2020.08.011.
43. Ward, T.L.; Weber, B.P.; Mendoza, K.M.; Danzeisen, J.L.; Llop, K.; Lang, K.; Clayton, J.B.; Grace, E.; Brannon, J.; Radovic, I.; et al. Antibiotics and Host-Tailored Probiotics Similarly Modulate Effects on the Developing Avian Microbiome, Mycobiome, and Host Gene Expression. mBio 2019, 10, doi:10.1128/MBIO.02171-19.
44. Niedermeyer, J.A.; Ring, L.; Miller, W.G.; Genger, S.; Lindsey, C.P.; Osborne, J.; Kathariou, S. Proximity to Other Commercial Turkey Farms Affects Colonization Onset, Genotypes, and Antimicrobial Resistance Profiles of Campylobacter Spp. in Turkeys: Suggestive Evidence from a Paired-Farm Model. Applied and environmental microbiology 2018, 84, doi:10.1128/AEM.01212-18.
45. Good, L.; Miller, W.G.; Niedermeyer, J.; Osborne, J.; Siletzky, R.M.; Carver, D.; Kathariou, S. Strain-Specific Differences in Survival of Campylobacter Spp. in Naturally Contaminated Turkey Feces and Water. Applied and environmental microbiology 2019, 85, doi:10.1128/AEM.01579-19.
46. Rahimi, S.; Kathariou, S.; Fletcher, O.; Grimes, J.L. The Effectiveness of a Dietary Direct-Fed Microbial and Mannan Oligosaccharide on Ultrastructural Changes of Intestinal Mucosa of Turkey Poults Infected with Salmonella and Campylobacter. Poultry science 2020, 99, 1135–1149, doi:10.1016/J.PSJ.2019.09.008.
47. Fan, S.; Foster, D.; Miller, W.G.; Osborne, J.; Kathariou, S. Impact of Ceftiofur Administration in Steers on the Prevalence and Antimicrobial Resistance of Campylobacter Spp. Microorganisms 2021, 9, 1–16, doi:10.3390/MICROORGANISMS9020318.
48. Niedermeyer, J.A.; Miller, W.G.; Yee, E.; Harris, A.; Emanuel, R.E.; Jass, T.; Nelson, N.; Kathariou, S. Search for Campylobacter Spp. Reveals High Prevalence and Pronounced Genetic Diversity of Arcobacter Butzleri in Floodwater Samples Associated with Hurricane Florence in North Carolina, USA. Applied and environmental microbiology 2020, 86, 1–14, doi:10.1128/AEM.01118-20.
49. Jeamsripong, S.; Li, X.; Aly, S.S.; Su, Z.; Pereira, R. v.; Atwill, E.R. Antibiotic Resistance Genes and Associated Phenotypes in Escherichia Coli and Enterococcus from Cattle at Different Production Stages on a Dairy Farm in Central California. Antibiotics (Basel, Switzerland) 2021, 10, doi:10.3390/ANTIBIOTICS10091042.
50. Li, X.; Aly, S.S.; Su, Z.; Pereira, R. v; Williams, D.R.; Rossitto, P.; Champagne, J.D.; Chase, J.; Nguyen, T.; Atwill, E.R. Phenotypic Antimicrobial Resistance Profiles of E. Coli and Enterococcus from Dairy Cattle in Different Management Units on a Central California Dairy. 2018, doi:10.4172/2327-5073.1000311.
51. Staley, Z.R.; Woodbury, B.L.; Stromer, B.S.; Schmidt, A.M.; Snow, D.D.; Bartelt-Hunt, S.L.; Wang, B.; Li, X. Stockpiling versus Composting: Effectiveness in Reducing Antibiotic-Resistant Bacteria and Resistance Genes in Beef Cattle Manure. Applied and environmental microbiology 2021, 87, 1–16, doi:10.1128/AEM.00750-21.
52. Li, B.; Li, X.; Yan, T. A Quantitative Metagenomic Sequencing Approach for High-Throughput Gene Quantification and Demonstration with Antibiotic Resistance Genes. Applied and environmental microbiology 2021, 87, 1–11, doi:10.1128/AEM.00871-21.
53. Li, B.; Yan, T. Next Generation Sequencing Reveals Limitation of QPCR Methods in Quantifying Emerging Antibiotic Resistance Genes (ARGs) in the Environment. Applied microbiology and biotechnology 2021, 105, 2925–2936, doi:10.1007/S00253-021-11202-4.
54. Mou, K.T.; Allen, H.K.; Alt, D.P.; Trachsel, J.; Hau, S.J.; Coetzee, J.F.; Holman, D.B.; Kellner, S.; Loving, C.L.; Brockmeier, S.L. Shifts in the Nasal Microbiota of Swine in Response to Different Dosing Regimens of Oxytetracycline Administration. Veterinary microbiology 2019, 237, doi:10.1016/J.VETMIC.2019.108386.
55. Wiarda, J.E.; Trachsel, J.M.; Bond, Z.F.; Byrne, K.A.; Gabler, N.K.; Loving, C.L. Intraepithelial T Cells Diverge by Intestinal Location as Pigs Age. Frontiers in immunology 2020, 11, doi:10.3389/FIMMU.2020.01139.
56. Mir, R.A.; Schaut, R.G.; Allen, H.K.; Looft, T.; Loving, C.L.; Kudva, I.T.; Sharma, V.K. Cattle Intestinal Microbiota Shifts Following Escherichia Coli O157:H7 Vaccination and Colonization. PloS one 2019, 14, doi:10.1371/JOURNAL.PONE.0226099.
57. Bucher, M.G.; Zwirzitz, B.; Oladeinde, A.; Cook, K.; Plymel, C.; Zock, G.; Lakin, S.; Aggrey, S.E.; Ritz, C.; Looft, T.; et al. Reused Poultry Litter Microbiome with Competitive Exclusion Potential against Salmonella Heidelberg. Journal of environmental quality 2020, 49, 869–881, doi:10.1002/JEQ2.20081.
58. Certified Responsible Antibiotic Use | Agricultural Marketing Service Available online: https://www.ams.usda.gov/services/auditing/crau (accessed on 9 November 2021).
59. Wind, L.L.; Briganti, J.S.; Brown, A.M.; Neher, T.P.; Davis, M.F.; Durso, L.M.; Spicer, T.; Lansing, S. Finding What Is Inaccessible: Antimicrobial Resistance Language Use among the One Health Domains. Antibiotics 2021, Vol. 10, Page 385 2021, 10, 385, doi:10.3390/ANTIBIOTICS10040385.
60. Antimicrobial Resistance Workshop | Environmental Science & Technology Available online: https://enst.umd.edu/research/antimicrobial-resistance-workshop (accessed on 9 November 2021).
61. IAMR (@i_AMResponsible) / Twitter Available online: https://twitter.com/i_AMResponsible (accessed on 9 November 2021).
62. IAMResponsible – Livestock and Poultry Environmental Learning Community Available online: https://lpelc.org/tag/iamresponsible/ (accessed on 9 November 2021).
63. I(AM)Responsible: A Nationwide Antimicrobial Resistance (AMR) Education Network | UNL Water Available online: https://water.unl.edu/article/animal-manure-management/iamresponsible-nationwide-antimicrobial-resistance-amr-education (accessed on 9 November 2021).
64. Home - NIAMRRE | National Institute of Antimicrobial Resistance Research and Education Available online: https://www.niamrre.org/ (accessed on 9 November 2021).
65. Yarlagadda, V.; Medina, R.; Johnson, T.A.; Koteva, K.P.; Cox, G.; Thaker, M.N.; Wright, G.D. Resistance-Guided Discovery of Elfamycin Antibiotic Producers with Antigonococcal Activity. ACS infectious diseases 2020, 6, 3163–3173, doi:10.1021/ACSINFECDIS.0C00467.
66. Wickware, C.L.; Johnson, T.A.; Koziol, J.H. Composition and Diversity of the Preputial Microbiota in Healthy Bulls. Theriogenology 2020, 145, 231–237, doi:10.1016/J.THERIOGENOLOGY.2019.11.002.
67. Zhang, Y.; Schmidt, J.W.; Arthur, T.M.; Wheeler, T.L.; Wang, B. A Comparative Quantitative Assessment of Human Exposure to Various Antimicrobial-Resistant Bacteria among U.S. Ground Beef Consumers. Journal of food protection 2021, 84, 736–759, doi:10.4315/JFP-20-154.
68. Helmy, Y.A.; Kathayat, D.; Ghanem, M.; Jung, K.; Closs, G.; Deblais, L.; Srivastava, V.; El-Gazzar, M.; Rajashekara, G. Identification and Characterization of Novel Small Molecule Inhibitors to Control Mycoplasma Gallisepticum Infection in Chickens. Veterinary microbiology 2020, 247, doi:10.1016/J.VETMIC.2020.108799.
69. Helmy, Y.A.; Deblais, L.; Kassem, I.I.; Kathayat, D.; Rajashekara, G. Novel Small Molecule Modulators of Quorum Sensing in Avian Pathogenic Escherichia Coli (APEC). Virulence 2018, 9, 1640–1657, doi:10.1080/21505594.2018.1528844.
70. Kathayat, D.; Antony, L.; Deblais, L.; Helmy, Y.A.; Scaria, J.; Rajashekara, G. Small Molecule Adjuvants Potentiate Colistin Activity and Attenuate Resistance Development in Escherichia Coli by Affecting Pmr AB System. Infection and drug resistance 2020, 13, 2205–2222, doi:10.2147/IDR.S260766.
71. Schueler, J.; Naas, K.; Hurst, J.; Aga, D.; Lansing, S. Effects of On-Farm Dairy Manure Composting on Tetracycline Content and Nutrient Composition. Antibiotics (Basel, Switzerland) 2021, 10, doi:10.3390/ANTIBIOTICS10040443.
72. Oliver, J.P.; Hurst, J.J.; Gooch, C.A.; Stappenbeck, A.; Sassoubre, L.; Aga, D.S. On-Farm Screw Press and Rotary Drum Treatment of Dairy Manure-Associated Antibiotic Residues and Resistance. Journal of environmental quality 2021, 50, 134–143, doi:10.1002/JEQ2.20161.
73. Kaufman, J.H.; Terrizzano, I.; Nayar, G.; Seabolt, E.; Agarwal, A.; Slizovskiy, I.B.; Noyes, N. Integrative and Conjugative Elements (ICE) and Associated Cargo Genes within and across Hundreds of Bacterial Genera. bioRxiv 2020, 2020.04.07.030320, doi:10.1101/2020.04.07.030320.
74. Presidential Advisory Council on Combating Antibiotic-Resistant Bacteria BRIDGING THE GAP: IMPROVING ANTIMICROBIAL ACCESS AND USE ACROSS ONE HEALTH A REPORT WITH RECOMMENDATIONS. 2021.
75. Presidential Advisory Council on Combating Antibiotic-Resistant Bacteria ADVANCING INTERPROFESSIONAL EDUCATION AND PRACTICE TO COMBAT ANTIMICROBIAL RESISTANCE A REPORT WITH RECOMMENDATIONS. 2021.