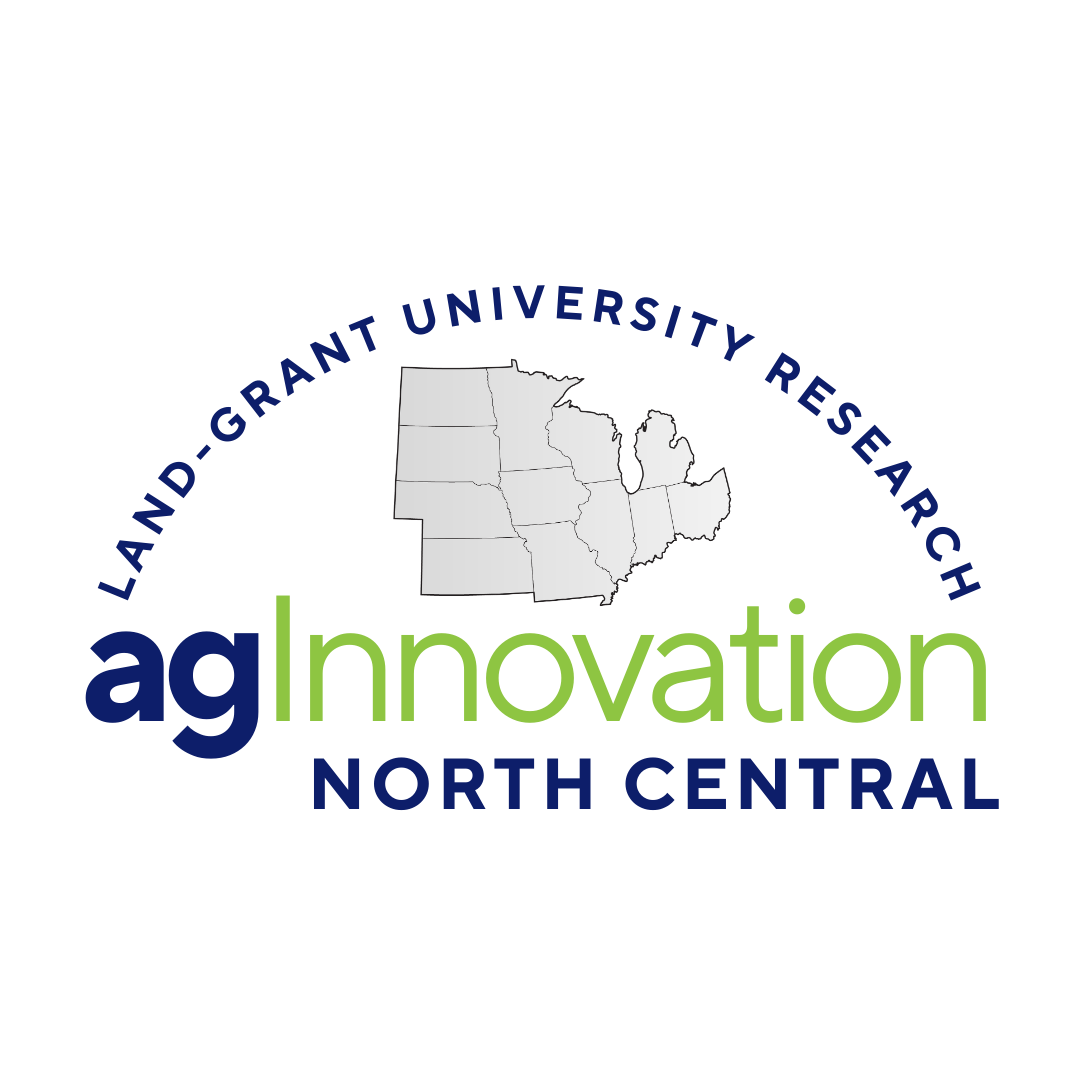
NC1213: Sources and fate of ammonia across the landscape
(Multistate Research Project)
Status: Active
NC1213: Sources and fate of ammonia across the landscape
Duration: 10/01/2020 to 09/30/2025
Administrative Advisor(s):
NIFA Reps:
Non-Technical Summary
Statement of Issues and Justification
Ammonia (NH3) is a gas released into the air from a variety of biological, industrial and combustion processes. It is the principle basic gas in the atmosphere and can be found at varying levels across the US. The 2014 U.S. National Emissions Inventory (NEI) indicated that emissions of NH3 decreased 2% since 2002 while that of nitrogen oxides (NOx) decreased by 48% (USEPA, 2017). These changes have resulted in making ammonium (NH4+) the primary component of nitrogen (N) wet (precipitation) deposition in most of the US (Li et al., 2016). Around 80% of NH3 emissions is estimated to come from animal waste and commercial fertilizer applications (Paulot et al., 2014). These sources are highly variable in both space and time (Walker et al., 2004; van Damme et al., 2015) and the models of transport, deposition and transformations of emitted NH3 cannot explain the distributions of measured NH3 (Van Damme et al., 2014).
An increased understanding of sources, transport and sinks of NH3 in the atmosphere and across the landscape will greatly enhance our ability to address a number of environmental and health issues associated with farming in the 21st century. Ammonia is a detriment to animal, human and ecosystem health. Inhalation of NH3 at varying concentrations and durations can causes pulmonary edema and pulmonary inflammation (Carson et al., 1981). Ammonia is the leading basic gas in the atmosphere creating fine particulate matter, particles with aerodynamic diameters ≤ 2.5 μm (PM2.5) (USEPA, 2011; Weber et al., 2016). These inorganic salts comprise half or more of the PM2.5 in the eastern United States (Hand et al., 2012). PM2.5 is associated with premature mortality, chronic bronchitis, and asthma complications (Dockery et al., 1993; McCubbin et al., 2002; Pope et al., 2009). In the US alone, health costs associated with agricultural NH3 are estimated at $36 billion (2006 USD) annually. PM2.5 also scatters light creating visibility-reducing haze. Ammonia also contributes to haze by affecting aerosol water content and the formation of secondary organic aerosols from biogenic VOC emissions (Carleton et al., 2010, Carleton and Turpin, 2013).
Reduced nitrogen (NR; NH3 + NH4+) is a significant contributor NR deposition throughout the United States. With the successful reduction in NOx due partly to emission reductions driven by the Clean Air Act, NH3 is now the predominate form of NR deposition in many ecosystems (Schichtel and Walker, 2015; Li et al., 2016, Due et al., 2015). Reactive nitrogen deposition in many ecosystems is already causing adverse effects (the ‘critical load’ defines the threshold for adverse effects). These effects include acidification and nutrient enrichment in aquatic and terrestrial ecosystems (Pardo et al., 2011; US EPA 2011) and reduced fish and aquatic insect populations (Bulger et al., 2000). Nutrient enrichment can disrupt the nutrient balance leading to reduced biodiversity (Nordin, et al., 2005), increases in invasive species, and increased biological production or eutrophication of fresh and coastal waters (Bergstrom et al., 2006). It can also increase susceptibility of vegetation to drought, freezing, and insect infestations (Bailey et al., 2004).
Better understanding agriculturally-produced NH3 nitrogen losses from farms and the nitrogen additions to the surrounding environments will assist in the development of management practices that will support a sustainable agricultural system in the US. Four major areas of understanding are required to address NH3 in agriculture: 1) NH3 emissions, 2) NH3 transport, 3) NH3 transformations in the atmosphere, and 4) NH3 deposition. Although transport, transformation, and deposition occur both in the atmosphere and hydrosphere, this project will focus solely on the atmosphere and land-atmosphere interactions
NH3 Emissions.
In the United States, about 80% of the NH3 emissions are from agricultural activities (Reis et al., 2009). However, other source categories such as wildfires, mobile and natural sources can also be important in some locations (Paulot et al., 2013; Zhang et al., 2018; Fenn et al., 2018). The large contributions of NH3 by agricultural activities makes emission characterization and quantification a critical activity. These emissions are dependent on diverse and varied activities and conditions making current estimates uncertain.
Emissions from livestock feed yards, dairies, hog farms, and broiler and laying chicken farms were extensively studied in the National Air Emissions Monitoring Study (Ni et al., 2011). High variability in emission appeared to result from a wide range of management practices, climate, animal feed, and a multitude of other factors (eg. Grant et al, 2016; Ni et al, 2018). The importance of the farm management on the emission rates (eg. Rotz et al., 2013) illustrates the difficulty in determining national-scale agricultural emissions of NH3 from bottom-up approaches.
NH3 emissions from livestock waste is a result of the biological breakdown of urea and ureic acid (Li et al., 2012) and varies widely depending on species, feed composition, and climate (Bouwman et al, 2002; Hristov et al., 2010; Ni, 2012; Schaefer et al., 2012; Grant and Boehm, 2013b, 2015a).
NH3 emissions from inorganic fertilizer, a lesser source of emissions, are a function of the fertilizer type, application type (injection or surface), application rate, soil type and climate (Bouwman et al., 2002). Up to 50% of the NH3 is volatilized from fertilizer applications occurs in the first few hours and can represent up to 50% of the application (Sommer and Christensen, 1992).
High temporal variability of NH3 emissions from agricultural production makes annual assessment of emission difficult. The high spatial variability of agricultural operations further complicates the situation. The estimation of NH3 emissions from and deposition to individual farms requires knowledge of the ambient background levels (Grant et al., 2020). This has been identified as a significant knowledge gap for estimating farm-scale NH3 emissions (Powers et al., 2014) and has been partially addressed through the National Atmospheric Deposition Program (NADP)-established Ammonia Monitoring Network (AMoN) measurements made across the US.
Regional NH3 emissions have been estimated at two very tall towers located in Colorado and the upper Midwest. Measurements made in Colorado indicated that a land surface can be either a source or sink depending on the surface (vegetation, soil, litter) NH3 concentrations, ambient NH3 in the boundary layer, and local meteorology (Tevlin et al, 2017). Tall tower observations from the US Corn Belt indicate that net ecosystem NH3 exchange was greatest in spring and fall (Griffis et al., 2019).
To assess the effects of agricultural activities on NH3 emission and how this loss impacts agricultural profits and downwind sensitive receptors there is a critical need to better understand and quantify NH3emissions from crop and livestock agriculture across spatial scales.
NH3 Transport and transformation.
Gaseous NH3 has a high deposition rate and can be quickly deposited to surfaces limiting the spatial extent of emissions. However, it can be revolatilized from the surface, i.e. bi-directional flux, and transported further down wind (Walker et al., 2019). This NH3 can be transported downwind and directly deposited on the landscape or may neutralize acid inorganic and organic gases and form PM2.5 that can reside in the atmosphere for days to weeks and be transport 1000s of kilometers (Hidy, 1994). Approximately 25% of NR deposited in Rocky Mountain NP comes from sources more than 1000 km away (Thompson et al., 2015).. Similar results were found for a study in Grand Teton, NP (Zhang et al., 2018). Consequently, NH3 emission have local to regional to hemispheric impacts and mitigation of negative NH3 emission effects requires a multistate approach.
In many regions in the US ammonium nitrate (NH4NO3) PM2.5 formation is limited by low NH3 concentrations, and with local NH3 emissions present, aerosol formation can occur quickly (Pinder et al., 2007). Given the dominance of agricultural activities in the emission of NH3 and the rapid formation of NH4NO3 in high NH3 environments, PM formation is also enhanced by agricultural operations. While PM2.5 has the greatest health risk, all inhalable PM (with aerodynamic diameters ≤10 μm; PM10) can cause adverse health effects. The 2014 NEI indicated that PM10 and PM2.5 emissions increased by 16% and 14% respectively (USEPA, 2017). Reductions in emissions of sulfur and nitrogen oxides and their secondary PM2.5 products in many regions of the US have resulted in decreased PM2.5 concentrations (Hand et al., 2014).
The potential for addressing the impacts of NH3 emissions has increased in recent years with improvements in the measurement and modeling NH3 emission, transformation, and deposition (Walker et al., 2019). Recent trends in wet deposition and air quality indicate that the relative role of reduced (NH3, NH4+) vs NOx is changing (Pinder et al., 2011; Li et al., 2016). In addition, due primarily to very steep declines in emissions of sulfur oxides the fraction of PM2.5 that is NH4NO3 has increased in most of the eastern US. Consequently, it is expected that NH3 will be playing a larger role in PM formation in the future (Heald et al, 2012) and understanding the changing chemistry of PM formation associated with agricultural production will assist in effective measures to limit PM and improve human and animal health.
With the continued changing emissions and atmospheric chemical environment throughout the United States there is a need to resolve the impact of atmospheric NH3 on PM formation and NR deposition. This information is vital to develop the most effective mitigation strategies of elevated PM2.5 concentrations.
NH3 Deposition.
Atmospheric deposition of reduced N (NHx = NH4+ + NH3) occurs through precipitation (wet deposition) and dry particulate (NH4+) and gaseous (NH3) deposition. Deposition fluxes may be derived from measurements, site-specific inferential models, gridded chemical transport models (CTMs) or hybrid methods combining measurements with models (Walker et al., 2019). Ammonia may be rapidly deposited or emitted depending on the equilibrium between the NH3 surface concentration and ambient air known as the compensation point (Mattson and Schjoerring, 2007; Massad, et al., 2010). Data from the National Atmospheric Deposition Program/National Trends Network (NADP/NTN), a 40+ year wet deposition network with over 250 sites across the U.S., have shown an increasing trend in NH4+ concentration within precipitation in the central U.S and other regions with abundant agricultural sources (i.e. Utah, California, and North Carolina). In many areas, NHx dry deposition contributes significantly to atmospheric N deposition (Schwede and Lear, 2014; Li et al. 2016). This has been shown to be true for a number of U.S. national parks and wilderness areas (Ellis et al., 2013; Benedict et al., 2013a,b; Schichtel et al., 2019).
Areas with the highest ambient atmospheric concentrations (measured in AMoN) and the highest wet deposition fluxes (measured in NTN) are both found in the Midwest and Plains states.
Understanding the contribution of reduced nitrogen, versus oxidized forms that are subject to regulatory control, to total deposition of nitrogen is important for developing effective ecosystem protection through the Secondary National Ambient Air Quality Standards for NOx and sulfur oxides (SOx) and critical load frameworks (Ellis et al., 2013, Walker et al., 2019). Assessing NH3 dry deposition can substantially assist in the protection of ecosystems when incorporated into developing models of critical loads.
The technical feasibility of the research.
In recent years, the information about NH3 emissions from agricultural sources has greatly increased. While traditionally NH3 emissions have been estimated using closed or open chambers, incorporation of these new analytical methods into micrometeorological flux approaches have begun to give real-world flux estimates (Walker et al, 2019).
Measuring ambient concentrations and the bi-directional flux of NH3 has been commonly limited by the complexity and cost of analytical methods. The accurate measurement of NH3 concentrations has greatly improved with the utilization of commercially-available annular denuder systems (Walker et al., 2013), passive samplers (Puchalski et al., 2011), open-path tunable diode lasers (TDL; Grant et al., 2013a,b) and Fourier transform infrared spectrometers (FTIR; Grant et al., 2018), and differential optical absorptionspectrometers (UVDOAS; Mount et al., 2002). Direct measurement of NH3 fluxes are limited to micrometeorological techniques (Shah et al, 2006). Accounting for the bi-directional nature of NH3 exchange (combined deposition and emission) has significantly improved our ability to predict NH3 concentrations (Fletchard et al., 2013; Walker et al., 2013), however improvements are limited by available measurements to evaluate the model results. To fully evaluate the CTMs measurements, NH3 flux measurements under a wide range of chemistry and physical characteristics of exchange surfaces and meteorological conditions are needed (Walker et al., 2019; Baum and Ham, 2009; Sintermann et al., 2011; Farrara et al., 2012; Nelson et al, 2017).
The Satellite-based systems such as the Infrared Atmospheric Sounding Interferometer (IASI) and the Tropospheric Emission Spectrometer (TEM) provide the first spatially complete atmospheric column NH3 measurements (Van Damme et al, 2014). These measurements provide spatial variability to the emission sources but are limited in use by their columnar measurement: comparisons of the estimated surface NH3 to measurements have only had fair success (Pinder et al, 2011).
The advantages for doing the work as a multistate effort.
Characterizing NH3 emissions, transport, transformation and deposition is complex- requiring a broad range of expertise working across the entire agricultural sector. Since the number of researchers working in this area is small (typically only a few per state) and the need for a broad diversity of researchers to address this topic across the wide diversity of agricultural sources and climatological conditions, scientists and engineers from many disciplines within agricultureneed to have a mechanism to collaborate. Thus far, collaboration has typically involved only two of three scientists studying small portions of the overall issue. A multistate effort is needed to broaden the collaborative effort and move the science forward to address this serious issue.
The need as indicated by stakeholder.
Stakeholders include research scientists, land owners, agricultural producers, and government agencies responsible for emissions inventories, air quality standards, land conservation and preservation, and emissions regulation. Results of a recent stakeholder workshop (Connecting Stakeholder and Science Perspectives to Better Understand the Linkages Between Agriculture and Reactive Nitrogen Deposition) held 4 Nov, 2019 by the National Atmospheric Deposition Program (NADP) indicated a need for improved and increased communication across the stakeholder groups, monitoring near NH3 sources to ground-truth satellite measurements, measurements of NHx in routine monitoring networks, and improved methods for a more accurate emissions inventory. Losses of NH3 from agricultural production systems were also discussed as they represent reduced farm profits through loss of purchased N inputs. Improved understanding of the factors controlling the emissions will also increase farm profitability through improved best management practices (Rotz and Oenema, 2006).
Related, Current and Previous Work
The NIMSS data base of existing active projects was interrogated for existing projects with goals that overlap foreseen objectives relating to the proposed project (Table 1). The only project with potentially overlapping objectives with the proposed committee was NC1187. The project’s overlapping objectives were: 1) Determine the physical, chemical, and biological nature of particulate matter, including nanoparticles, derived from agricultural practices, processes, and operations and from the production, use, and disposal of consumer products, as they impact air, water, and soil quality and associated health, economic, and environmental impacts, including ecological sustainability and agricultural production; 2) Determine those particulate matter properties that control the cycling, biological availability, and uptake of nanoparticles, nutrients, carbon, and toxic substances in air, water, and soil systems. The only USDA project with clear objectives involving NH3 was NRSP003. The project’s overlapping objectives were: 1) Characterize geographic patterns and temporal trends in chemical or biological atmospheric (wet and dry) deposition; and 2) Support research activities related to: (a) the productivity of managed and natural ecosystems; (b) the chemistry of surface and ground waters, including estuaries; (c) critical loads in terrestrial and aquatic ecosystems; (d) the health and safety of the nation's food supply; and (e) source-receptor relationships. Within the NADP, there is a background NH3 monitoring effort (AMoN; http://nadp.slh.wisc.edu/AMoN/) that would be supporting the proposed MSRP. Within the NADP, there is a Total Deposition Science committee that meets to address the uncertainties in measurement and modeling techniques used to provide fluxes of dry and total deposition of NH3 among other chemicals.
Table 1: Related Projects extracted from the NIMSS data base
Project | Title | Period of operation |
NC1195 | Enhancing nitrogen utilization in corn based cropping systems to increase yield, improve profitability and minimize environmental impacts | 10/01/2016 - 09/30/2021 |
WERA103 | Nutrient Management and Water Quality | 10/01/2015 - 09/30/2020 |
NE1544 | Dairy Production systems: C,N, and P management for production, profitability and the environment. | 10/01/2015 - 09/30/2020 |
NC1187 | The Chemical and Physical Nature of Particulate Matter Affecting Air, Water and Soil Quality | 10/01/2015 - 09/30/2020 |
NRSP003 | The National Atmospheric Deposition Program (NADP) | 10/01/2019 - 09/30/2024 |
The Total Deposition Science Committee has indicated their interest in this MRSP and multiple meetings have occurred during the proposal development process. The Total Deposition Science Committee also sponsored a workshop before the NADP Fall 2019 Technical Committee Meeting on ‘Connecting Stakeholder and Science Perspectives to Better Understand the Linkages Between Agriculture and Reactive Nitrogen Deposition’ to facilitate the communication between stakeholders in this issue.
The proposed project will utilize the AMoN network as a platform to provide spatial gradients of NH3 concentrations at or near agricultural sources. The AMoN currently has 104 sampling locations in the United States and Canada. Many of the current AMoN sites are located east of the Mississippi River, while significant spatial gaps exist in the Mid-west and West Coast. These gaps often occur in regions with significant agricultural activities, such as plains states and the San Joaquin Valley of California. The NADP wet deposition network has identified these regions as having either high or increasing NH4+ wet deposition values. Western states often see these NH4+ deposition trends at locations with sensitive ecosystems, in areas with PM air quality concerns, or in areas impacted by wildfire emissions. The current network can be expanded to establish sites operated by stakeholders to provide information on seasonal changes in NH3 concentrations, atmospheric transport, and the variability in concentrations due to management or application systems. AMoN is complemented in select regions by regional NH3 monitoring networks such as in NE Colorado (Li et al., 2017), an active livestock production region where NH3 emissions can impact sensitive high elevation ecosystems in Rocky Mountain National Park (Benedict et al., 2013c).
GRACEnet (Greenhouse gas Reduction through Agricultural Carbon Enhancement network), supported by the USDA Agricultural Research Service since the early 2000s, also measures reactive N gas emissions from a wide range of cropping and grazing management practices using soil chambers (https://catalog.data.gov/dataset/gracenet-greenhouse-gas-reduction-through-agricultural-carbon-enhancement-network). This effort is aligned with our objective of determining differences in the emissions of reactive N due to different management approaches however it is focused on N2O, not NH3.
Objectives
-
To better understand and quantify ammonia emissions from crop and livestock across spatial scales.
-
To characterize the magnitude and variability of ammonia deposition in natural and agricultural landscapes.
-
To understand the transport and fate of NH3 emissions and its impact on PM formation and reactive nitrogen deposition
Methods
Methods for Objective 1:
The temporal and spatial variability in emission sources in agricultural systems and the complexity of deposition surfaces requires both measurement and modeling at multiple scales. Two major approaches will be used to determine the NH3 exchanges:
- A top-down approach where the emissions from the agricultural landscape is estimated from integrated column measurements using satellite and profile measurements on tall towers (greater than 100 m).
Top down approaches approximate surface emissions from accumulated NH3 concentrations in the atmospheric column. They include micrometeorological and remote sensing/modeling approaches. The advantage to such approaches is the ability to estimate emissions from large domains including emission from not only agricultural operations, but other sources such as from urban areas and wildfires. The main disadvantage is that individual sources cannot be identified with confidence.
Regional NH3 emissions will be estimated using aerodynamic profile methods from Tall tower (> 100 meters above ground level) measurements. Although there are many tall towers in the NOAA Earth System Research Laboratory Global Monitoring Division (ESRL/GMD) network for greenhouse gas measurements, they are not instrumented for NH3 concentration measurements. Tall towers have been instrumented for NH3 concentration measurements (Griffis et al., 2019a,b; Tevlin et al., 2017) to associate emissions with wind conditions and calculate emissions of upwind ground source areas. Land use of upwind source areas will be assessed using GoogleEarth® images and county-based USDA National Agriculture Statistics Service (NASS) annual farm census data.
Regional NH3 emissions and deposition will also be constrained by combining the tall tower concentration data, the Weather Research and Forecasting Chemistry (WRF-Chem) model and state-of-the-art emission inventories to simulate the sources and transport of NH3. These simulated NH3 concentrations will be compared to the observations and objective cost function analyses used to optimize the agreement between the observations and simulated values. This will provide a best-estimate of regional NH3 emissions and deposition and will also identify important uncertainties related to emissions, transport processes, and chemical transformations occurring during long-range transport.
Regional emissions estimated from satellite NH3 concentration measurements will utilize the Infrared Atmospheric Sounding Interferometer (IASI) on the MetOp-A satellite (van Damme et al., 2014, 2018), the Tropospheric Emission Sounder (TES) on the Aura satellite (Pinder et al., 2011), and the Cross-Track Infrared Sounder Satellite (CrIS) on the Suomi National Polar-orbiting Partnership (SNPP) platform (Kharol et al. 2017; Shephard et al., 2020). The CrIS retrievals have been shown by Kharol et al. (2017) to be valid down to 0.2-0.3 ppbv at a single 14 km pixel. While the CrIS satellite only passes over North America twice a day, results have shown elevated NH3 concentrations in the Central Valley of California, portions of Idaho and Washington, and several states in the central U.S. (e.g. Nebraska, Kansas, Oklahoma and Northern Texas) where agriculture dominates NH3 emissions. Kharol et al. (2017) used concentrations from the CrIS satellite to calculate dry deposition of NH3 across North America. Using a uni-directional model, they showed high deposition rates seasonally (spring fertilizer application) and spatially (higher over agricultural regions). Additional efforts will be made to relate surface and tall tower measurements with the NH3 atmospheric column measurements (Luo et al., 2015).
An expanded ground-based network of AMoN passive samplers will be established to assist in ground-truthing satellite measurements and characterize spatiotemporal patterns of NH3 concentrations. The network study area will be located based on prior satellite observations. Where possible, sites will be chosen to collocate with existing measurements from climatological (i.e., state climate networks; NOAA climate reference network), ecological (e.g., NEON, Ameriflux), agricultural (state agricultural extension, USDA) and atmospheric chemistry (EPA/CASTNET, NADP/NTN, EPA/NCore) networks to facilitate integration of datasets for modeling efforts describe under Objectives 2 and 3.
- A bottom-up approach where the emissions from diverse agricultural land uses are measured at or near the ground surface.
This includes micrometeorological within the lowest 100m of the atmosphere and chamber measurements at the surface. The advantage to such approaches is the ability to estimate emissions from known surface land use or agricultural operation. The main disadvantage is that regional emissions cannot be derived from the individual sources due to limited land use and local air and soil climate information.
Since agricultural emissions often span spatial scales of hundreds of square meters (e.g., waste lagoons) to hectares (e.g., fertilized fields), micrometeorological approaches utilizing modified Bowen ratio methods (Walker et al., 2013), aerodynamic flux gradient methods (Wichink et al., 2006), relaxed eddy accumulation (Meyers et al., 2006), eddy covariance (Sintermann et al., 2011; Sun et al, 2015), and backward Lagrangian (Grant et al. 2013a,b) methods will be employed to directly quantify net emission rates. An advantage of micrometeorological methods is that the bidirectional exchange of NH3 over vegetated surfaces may also be resolved.
Ammonia concentrations will be measured with non-intrusive open cell technologies including TDL (Grant et al., 2103a; Sun et al., 2015) and FTIR (Grant et al., 2018). Intrusive NHx concentrations will be made using an annular denuder/filterpack system (Walker et al., 2013; Benedict et al., 2013c). Field-scale studies should consider ways to segregate advected NH3 and the local production of NH3. Such advection can be addressed utilizing the AMoN network as well as adding samplers upwind, within and downwind sources. Additionally, while the open path measurements may operate continuously, often there is downtime over the course of a sampling period. The AMoN passive samplers can help fill in gaps in the continuous measurements.
While emissions inventories are on an annual basis, the estimation of yearly emissions is difficult. Emissions of NH3 from agricultural operations vary widely over the year and most surface-based measurements are not continuous throughout the year. Emissions from livestock waste lagoons vary with respect to temperature, wind speeds, lagoon crusting (Grant et al, 2013a,b; Grant et al, 2015a). Emissions of NH3 from land applications of waste are, among other factors, related to maximum temperatures or time since land application (Grant et al, 2018). Since most measurements are made over relatively short periods of time, care must be made to measure the relevant conditions influencing emission so process models can be checked and adjusted according to real-world conditions. Measurements will be made over the range of conditions that occur over the course of a year and at both experimental research and commercial cropping and livestock farms. Many agricultural operation components are relatively small domains and can more readily be measured at many farms. Whole farm measurements will require careful selection of farms to be measured to fulfill the assumptions used in the emissions estimation and to position equipment within the producer’s land.
Bottom-up approaches using direct field-scale flux measurements will be made by CO, IN, MN, PA, WI and EPA in a variety of environments: corn-soybean cropping systems, forests, and beef and dairy farms. Top-down approaches, combining tall tower NH3 observations with WRF-Chem modeling and satellite observations to constrain regional emissions and deposition, will be conducted by CO, IN, MN, WI, and EPA.
Methods for Objective 2:
While some progress has been made in validating the modeling of NH3 transport and deposition, much work is still needed to improve the accuracy of these models to capture changes in daily deposition rates. Given the complexity of fugitive NH3 dispersion and the variety of deposition surfaces, a combination of direct flux measurements and bi-directional flux modeling will be used to quantify NH3 dry deposition and bi-directional exchange in agricultural and natural landscapes. The dispersion and deposition of NH3 is influenced not only by local emissions but by meteorology, dew formation, plant residue, soil and leaf nitrogen content and pH, soil temperature and moisture, and the composition of leaf litter (Walker et al, 2013; Flechard et al, 2013; Wentworth et al, 2016). Dew, a common event in the midwestern US evenings, appears to store NH3 as NH4+ from one day to the next (Wentworth et al, 2016). There is also often a release of NH3 from a plant surface as the tissue dries and the moisture evaporates. In landscapes with a patchwork of agricultural and natural ecosystems, variability in NH4+ concentrations and pH of soils and vegetation creates large spatial variability in the NH3 surface emission potentials. All of these factors influence the NH3 compensation point of the surface, which influences the direction and magnitude of the NH3 flux to or from the atmosphere (i.e., bidirectional exchange).
Micrometeorological flux methods will be employed to quantify NH3 air-surface exchange in natural ecosystems (e.g., forests and grasslands) targeting a range of atmospheric chemical conditions representative of the high and low concentrations of NH3 to examine the role of compensation points. Flux measurements will be conducted during intensive periods to examine air-surface exchange processes, including the influence of meteorology, surface wetness, atmospheric and biogeochemistry on soil and vegetation and net canopy-scale fluxes to improve bidirectional exchange models. Because continuous flux measurements are resource intensive, these shorter-term measurements will be supplemented with low-cost direct flux measurements that will operate year-round.
Deposition of fugitive NH3 emissions are often in regions that are inaccessible to measurement equipment due to power needs and terrain. Consequently, direct measurements of NH3 deposition must be supplemented with field-scale models and satellites or chemical transport models to characterize deposition patterns at landscape to regional scales. Passive NH3 concentration measurements will be used to drive the bidirectional flux model to generate point estimates of dry deposition across the landscape- allowing for a more detailed characterization of spatial patterns of dry deposition at the landscape scale than capable with direct flux measurements. These passive systems, termed Conditional Time-Integrated Average Gradient Systems (COTAG) will be deployed to quantify dry deposition of NH3, HNO3, NH4+ and NO3- on a bi-weekly time-scale (Famulari et al., 2010; Tang et al. 2009). Data from these systems will provide seasonal and annual estimates of deposition for development of total deposition budgets and for evaluation of dry deposition estimates from field-scale models and CTMs.
Bi-directional exchange measurements will be conducted by IN, WI and EPA. Dry deposition measurements and field-scale modeling will be conducted by CO and EPA using passive NH3 measurements collected under objective 1.
Methods for Objective 3:
Two critical issues associated with the transport and fate of NH3 emissions on a national and regional scales are the role of NH3 in PM2.5 concentrations and NH3 contribution to NR in sensitive ecosystems. Understanding the transport and fate of NH3 emissions over broad spatial scales is primarily a modeling project, but also requires coordination and collaboration with groups that can take ambient measurements (NH3, PM2.5 speciation, SO2, meteorology) to evaluate the model and to bias correct the modeling results through inverse and hybrid modeling methods (Schwede and Lear, 2014; Malm et al., 2016).
To address these issues process based regional CTMs such as CAMx and CMAQ will be used to simulate NH3 emission, transport and fate. Models will be operated at 12 km grids over the US with 1-4 km nested grids in select locations to capture important mechanisms. Emissions will be based on the 2016 NEI developed by EPA for regional haze modeling. These will incorporate the advancements made by the Western Regional Modeling and Analysis Platform (http://views.cira.colostate.edu/iwdw/). CTM will include the latest mechanism to simulate NH3 bidirectional flux (Objective 2). The role of dew processes in diurnal deposition and re-emission of NH3 (Wentworth et al., 2016) will be explored and incorporated in CTM if this mechanism can be understood.
Past work has revealed biases in available NH3 emissions and modeled precipitation patterns that affect NH3 deposition and source apportionments (Zhang et al., 2018). Uncertainties and potential biases in CTM emissions or modeled meteorological fields will be determined by evaluation of modeled concentrations and depositions of all nitrogen compounds (with a focus on NH3) against available measurements. Methods will be developed to further identify and reduce model biases using satellite-derived NH3 concentrations and animal feeding operation locations (Whitburn et al., 2016) to constrain NH3 emissions rates, measured precipitation to adjust the meteorological inputs or scaling of wet deposition rates (Appel et al., 2011), and hybrid modeling (Malm et al., 2016). This objective will take advantage of ongoing National Monitoring efforts including EPA/CASTNET, EPA/NCore, IMPROVE, and NADP (NRSP-3) networks.
Some measurements described in Objectives 1 and 2 will improve estimates of emissions and dry deposition and used to improve CTM. CTM will help address the following questions: 1) how much of the Midwest is NH3 versus acid gas limited with respect to NH4+ aerosol production and how does this change spatially and seasonally, 2) how much of the NH4+ and N deposition in the Midwest originates from agricultural NH3 sources, 3) how might large-scale implementation of BMPs to reduce agricultural NH3 emissions effect NH4+ aerosol formation and reactive N deposition in the US. Other measurements will be situated in areas with known PM2.5 issues to capture the processes impacting particle formation near agricultural sources to identify conditions that cause exceedances.
CO will examine the impacts and fate of ammonia emissions studying the thermodynamic partitioning of ammonia/ammonium between the gas and particle phases in the presence of sulfuric and nitric acids, mineral dust, sea salt, and organic acids and studies of oxidized and reduced nitrogen deposition to various ecosystems, particularly in the western United States. The NPS-CIRA group (NPS) will conduct modeling studies to assess the impact of agricultural activities in the United States on PM, haze and reactive nitrogen deposition in national parks and wilderness areas.
Measurement of Progress and Results
Outputs
- A structured database including measurements of NH3 emissions, meteorological conditions, source information (cropping or livestock production management characteristics) and land surface (water, soil, crop, vegetation) conditions from a diversity of agricultural sources.
- Peer-reviewed scientific publications derived from the studies addressing the proposed Objectives. The publications will describe ecosystem specific NH3 deposition measurements and the parameters driving the atmospheric exchange processes. Agricultural stakeholders may use the results to improve estimates of nitrogen loss from the system. The publications will also help prioritize new research areas.
- Improvements to the emission factors used to inventory agriculturally-originating ammonia emissions
- Maps and data products of land-use specific ammonia deposition linking measured and modeled ground-based fluxes, satellite retrievals and CTMs over agricultural regions where local and regional sources are changing on a daily time-scale.
- Observations and trend analyses of long-term flux measurements at agriculturally impacted sites, including within the source region and downwind of the region to capture the transport, fate and direction of net canopy-scale ammonia fluxes.
Outcomes or Projected Impacts
- Outcomes of Objective 1: • Greater understanding of the relationship of ammonia emissions to climatic and surface variables (i.e. vegetation type, soil characteristics). • Improvement of the national inventory of ammonia emissions from agricultural operations. • Increased awareness of producers of the influence of fertilization timing for fertilizer applications as it relates to the N losses as ammonia. • Improved products from satellites and chemical transport models to better characterize emissions and air concentrations across agricultural regions • Improved routines in process-based NH3 emissions models
- Outcomes of Objective 2: • Greater understanding of the relationship between ammonia transport and deposition to environmental variables. • The improvement of the critical loads of ammonia deposition originating from agricultural operations. Include results from long-term measurements in CTM updates to improve air-surface exchange algorithms and biogeochemistry parameterizations. • The awareness of land managers of the likely impacts of agriculturally-produced ammonia in the atmosphere. • Identify areas where estimates of NH3 concentrations and deposition fluxes are highly uncertain and prioritize these locations for surface monitoring to better understand deposition impacts and critical loads in managed areas. • Improve understanding of the times and regions where NH3 is a limiting ingredient to PM2.5 formation.
- Outcomes of Objective 3: • Identification of areas where agricultural NH3 emissions contribute a significant fraction of the PM2.5 in the United States. • Improved understanding of the sensitivity of PM2.5 to changes agricultural NH3 emissions and location sensitive to increases or decreases in these emissions. • Model estimates of the contribution of agricultural NH3 emissions to total reactive nitrogen deposition in sensitive ecosystems throughout the United States. • Evaluation of impacts due to shifts in agricultural methods on reduced nitrogen deposition given changes in food demand, best management practices, and a changing climate. Information from the long-term measurements should be used to better understand shifts in nitrogen transport, atmospheric exchanges processes and chemistry, and carbon-nitrogen cycling.
Milestones
(2023):Complete field-scale bidirectional NH3 flux measurements in a range of environments for bidirectional model improvements by 2024.(2024):Complete field-, farm- and region-scale NH3 measurements for inclusion of local climate influences on process-based NH3 emissions/transport and deposition models for use in impact assessments in managed areas in 2025.
(2024):Complete model development to further understand the influence of changes in agricultural NH3 emissions on PM formation across the US.
Projected Participation
View Appendix E: ParticipationOutreach Plan
Research will be regularly reported in scientific conferences and workshops and documented in popular trade articles and peer-reviewed scientific journal articles. Measurement data will be provided in a publicly available database.
Organization/Governance
The multistate research project will use the standard form of governance. We will elect a chair, a chair-elect, and a secretary on two-year terms. Administrative guidance and management oversight will be provided by an assigned Administrative Advisor represent the sponsoring regional association. All decisions by a committee will be made in an open and democratic process.
Literature Cited
Baum, K.A.; J.M. Ham. 2009. Adaptation of a speciation sampling cartridge for measuring ammonia flux from cattle feedlots using relaxed eddy accumulation. Atmos. Environ. 43, 1753–1759
Benedict, K.B.; S.M. Kreidenweis; B. Schichtel; W.C. Malm; C. Carrico; J.L. Collett, Jr. 2013a. A seasonal nitrogen deposition budget for Rocky Mountain National Park. Ecol. Appl., 23, 1156-1169.
Benedict, K. B.; X. Chen; A.P. Sullivan; Y. Li; D. Day; A.J. Prenni; E.J.T. Levin; S.M. Kreidenweis; W.C. Malm; B.A. Schichtel; J.L. Collett Jr. 2013b. Atmospheric concentrations and deposition of reactive nitrogen in Grand Teton National Park, J. Geophys. Res. 118, 11,875–11,887, doi:10.1002/2013JD020394.
Benedict, K.B.; D. Day; F.M. Schwandner; S.M. Kreidenweis; B.A. Schichtel; W.C. Malm; J.L Collett. 2013c. Observations of atmospheric reactive nitrogen species in Rocky Mountain National Park and across northern Colorado. Atmos. Env. 64, 66-76, doi:10.1016/j.atmosenv.2012.08.066.
Bouwman, A. F.; D.S. Lee; W.A.H. Asman; F.J. Dentener; K.W. Vander Hoek; J.G.J. Olivier. 1997. A global high-resolution emission inventory for ammonia, Glob. Biogeochem. Cy., 11, 561–587.
Bulger, A. J.; Cosby, B. J.; Webb, J. R. Current, Reconstructed Past, and Projected Future Status of Brook Trout (Salvelinus fontinalis) Streams in Virginia. Can. J. Fish Aquat. Sci. 2000, 57, 1515-1523.
Carson, B L; H.V. Ellis; C. M. Beall; and L. H. Baker; 1981. Ammonia Health Effects. Environmental Protection Agency, Emission Control Technology Division, 2565 Plymouth Road Ann Arbor, MI 48105 USA, EPA/460/3-81-027 Final Rpt., 181pp.
Dockery, D. W.; C.A. Pope; X.P. Xu; J.D. Spengler; J. H. Ware; M.E. Fay; B.G. Ferris; F.E. Speizer. 1993. An association between air-pollution and mortality in 6 United-States cities, N. Engl. J. Med., 329, 1753–1759, 1993.
Donham, K. J.; D. Cumro; S. Reynolds. 2002. Synergistic effects of dust and ammonia on the occupational health effects of poultry production workers. J. Agromedicine, 8, 57-76.
Ellis, R. A.; D.J. Jacob; M. Payer; L. Zhang; C.D. Holmes; B.A. Schichtel; T. Blett; E. Porter; L.H. Pardo; J.A. Lynch. 2013. Present and future nitrogen deposition to national parks in the United States: Critical load exceedances. Atmos. Chem. Phys. Discussions, 13: 9151–9178.
Famulari, D., Fowler, D., Nemitz, E., Hargreaves, K.J., Storeton-West, R.L., Rutherford, G., Tang, Y.S., Sutton, M.A., Weston, K.J. (2010) Development of a low-cost system for measuring conditional time-averaged gradients of SO2 and NH3. Environ. Monit. Assess., 161, 11-27.
Ferrara, R.M.; B. Loubet; P. Di Tommasi; T. Bertolini; V. Magliulo; P. Cellier; W. Eugster; G. Rana. 2012. Eddy covariance measurement of ammonia fluxes: comparison of high frequency correction methodologies. Agric. For. Meteorol., 158–159, 30-42,
Flechard, C.R.; R.-S. Massad; B. Loubet; E. Personne; D. Simpson; J.O. Bash; E.J. Cooter; E. Nemitz; M.A. Sutton. 2013. Advances in Understanding, Models and Parameterizations of Biosphere-Atmosphere Ammonia Exchange. Pp 11-84 in R.-S. Massad and B. Loubet (eds.), Review and Integration of Biosphere-Atmosphere Modelling of Reactive Trace Gases and Volatile Aerosols, Springer, Versailles cedex, France, DOI 10.1007/978-94-017-7285-3_2, 235p.
Grant, R.H.; M.T. Boehm; A.J. Lawrence. 2013a. Comparison of backward-Lagrangian Stochastic and Vertical Radial Plume Mapping methods for estimating animal waste lagoon emissions. Agric. Forest Meteorol.180: 236-248.
Grant, R.H.; M.T. Boehm; A.J. Lawrence; A.J. Heber. 2013b. Ammonia Emissions from Anaerobic Treatment Lagoons at Sow and Finishing Farms in Oklahoma. Agric. Forest Meteorol. 180: 203-210.
Grant, R.H.; M.T. Boehm; A.J. Heber. 2016. Ammonia emissions from anaerobic waste lagoons at pork production operations: influence of climate. Agric. Forest Meteorol. 228, 73-84.
Grant, R.H.; C.-H. Lin; C.T. Johnston. 2018. Ammonia volatilization associated with anhydrous ammonia nitrogen applications to cropped land. Extended Abstract # 410765 in Proc. Ann. Meeting, Air Waste Management Assn., Hartford, CT. (CD)
Grant, R.H.; M.T. Boehm; G.R. Haagevoort. 2020. Ammonia emissions from a western open-lot dairy. Atmos. 11, 913; doi:10.3390/atmos11090913
Griffis, T.; C. Hu; J. Baker; J. Wood; D. Millet; M. Erickson; Z. Yu; M.J. Deventer; C. Winker; Z. Chen. 2019a. KCMP Minnesota Tall Tower Ammonia Observations and Emission Estimates Dataset 2017-2018. United States: N. p., 2019. Web. doi:10.15485/1550921.
Griffis, T.; C. Hu; J. Baker; J. Wood; D. Millet; M. Erickson; Z. Yu; M.J. Deventer; C. Winker; Z. Chen. 2019b. Tall tower ammonia observations and emission estimates in the US Midwest. J. Geophys. Res.-Biogeo, 124, 3432-3447 https://doi.org/10.1029/2019JG005172
Hand, J.L; B.A. Schichtel; W.C. Malm; S. Copeland; J.V. Molenar; N. Frank; M. Pitchford. 2014. Widespread reductions in haze across the United States from the early 1990s through 2011. Atmos Environ 94, 671-679. https://doi.org/10.1016/j.atmosenv.2014.05.062
Heald, C.H.; J. L. Collett Jr., T. Lee, K. B. Benedict, F. M. Schwandner, et al.. 2012. Atmospheric ammonia and particulate inorganic nitrogen over the United States. Atmos. Chem. Phys. 12,10295-10312. ⟨10.5194/acp-12-10295-2012⟩.
Kharol, S.K.; Shephard, M.W.; McLinden, C.A.; Zhang, L.; Sioris, C.E; O’Brien, J.M., Vet, R.; Cady-Pereira, K.E.; Hare, E.; Siemons, J.; Krotkov, N.A. 2017. Dry deposition of reactive nitrogen from satellite observations of ammonia and nitrogen dioxide over North America. Geophyiscal Research Letters 45, 1157-1166. doi: 10.1002/2017GL075832
Li, Y.; B.A. Schichtel; J.T. Walker; D.B. Schwede; X. Chen; C.M.B. Lehmann; M.A. Puchalski; D.A. Gay; J.L. Collett, Jr., J.L. 2016. Increasing importance of deposition of reduced nitrogen in the United States. Proc. Nat. Acad. Sci. 113, 5874-5879, doi:10.1073/pnas.1525736113.
Li, Y.; T.M. Thompson; M. Van Damme; X. Chen; K.B. Benedict; Y. Shao; D. Day; A. Boris; A.P. Sullivan; J. Ham; S. Whitburn; L. Clarisse; P.-F. Coheur; J.L. Collett, Jr. 2017. Temporal and spatial variability of ammonia in urban and agricultural regions of northern Colorado, United States, Atmos. Chem. Phys. 17, 6197–6213, doi:10.5194/acp-17-6197-2017.
Lin, C.-H.; C.T. Johnston; R.H Grant; A.J. Heber. 2019a. Application of Open Path Fourier Transform Infrared Spectroscopy (OP-FTIR) to Measure Greenhouse Gas Concentrations from Agricultural Fields. Atmos. Meas. Tech. 12, 3403-3415.
Lin, C.-H.; C.T. Johnston; R.H Grant; A.J. Heber. 2019b. Sources of error in Open Path Fourier Transform Infrared Spectroscopy (OP-FTIR) Measurements of N2O and CO2 emitted from agricultural fields. Atmos. Meas. Tech. Discussions, https://www.atmos-meas-tech-discuss.net/amt-2019-263/.
Luo, M.; M.W.Shephard; K.E. Cady-Pereira; K.H. Daven, Z. Liye; J.O Bash, R.W. Pinder; S.L. Capps; J.T. Walker; M.R. Jones. 2015. Satellite observations of tropospheric ammonia and carbon monoxide: Global distributions, regional correlations and comparisons to model simulations. Atmos. Environ. 106, 262-277.
Malm, W. C., M. A. Rodriguez, B. A. Schichtel, K. A. Gebhart, T. M. Thompson, M. G. Barna, K. B. Benedict, C. M. Carrico and J. L. Collett, Jr. (2016). "A hybrid modeling approach for estimating reactive nitrogen deposition in Rocky Mountain National Park." Atmos. Environ. 126: 258-273.
Mount, G H; B. Rumberg; J. Havig, B. Lamb, H. Westberg, D. Yonge, K. Johnson, R. Kincaid. 2002. Measurement of atmospheric ammonia at a dairy using differential optical absorption spectroscopy in the mid-ultraviolet. Atmos. Environ. 36, 1799-1810.
McCubbin, D. R.; B.J. Apelberg; S. Roe; F. Divita. 2002. Livestock ammonia management and particulate-related health benefits. Environ. Sci. Technol., 36, 1141-1146.
Meyers T.P.; W.T. Luke; J.J. Meisinger. 2006. Fluxes of ammonia and sulfate over maize using
relaxed eddy accumulation. Agric. Forest Meteorol. 136,203–213
NARSTO. 2004. Particulate Matter Science for Policy Makers: A NARSTO Assessment, Cambridge, UK.
Nelson, A.J.; S. Koloutsou-Vakakisa; M.J. Rood; L. Myles; C. Lehmann; C.L Bernacchi; S. Balasubramaniana; E. Jooe; M. Heuer; M. Vieira-Filho; J. Lin. 2017. Season-long ammonia flux measurements above fertilized corn in central Illinois, USA, using relaxed eddy accumulation. Agric. Forest Meteorol. 239, 202–212
Ni, J.-Q.; E.L. Cortus; A.J. Heber. 2011. Improving Ammonia Emission Modeling and Inventories by Data Mining and Intelligent Interpretation of the National Air Emission Monitoring Study Database. Atmosphere 2, 110-128.
Ni, J.-Q.; A.J. Heber; E.L. Cortus; T.T. Lim; B.W. Bogan; R.H. Grant; M.T. Boehm. 2012. Ammonia Emissions from Swine Facilities in the U.S.: Application of knowledge from experimental research. Environ. Sci. Policy 22, 25-35.
Ni, J.-Q., A. J. Heber, and T.-T. Lim. 2018. Ammonia and hydrogen sulfide in swine production. In: Air Quality and Livestock Production, Banhazi, T., A. Aland, and J. Hartung (Eds). London, UK: CRC Press: 29-47.
Paulot, F.; D.J. Jacob; R.W. Pinder; J.O. Bash; K. Travis; D.K. Henze. 2014. Ammonia emissions in the United States, European Union, and China derived by high-resolution inversion of ammonium wet deposition data: Interpretation with a new agricultural emissions inventory (MASAGE_NH3). J. Geophys. Res.: Atmos. 119, 4343-4364.
Paulot, F. & Jacob, D. J. 2014. Hidden cost of U.S. agricultural exports: Particulate matter from ammonia emissions. Environ. Sci. Technol. 48, 903–908.
Pinder, R. W.; K.W. Appel; R.L. Dennis. 2011. Trends in atmospheric reactive nitrogen for the Eastern United States. Environ. Pollut. 159, 3138–3141, doi:10.1016/j.envpol.2011.04.042.
Pope, C. A.; M. Ezzati; D.W. Dockery. 2009. Fine-Particulate Air Pollution and Life Expectancy in the United States, N. Engl. J. Med., 360, 376–386.
Powers, W., B. Auvermann, N.A. Cole, C. Gooch, R. Grant, J. Hatfield, P. Hunt, K. Johnson, A. Leytem, W. Liao and M. Powell, 2014. Chapter 5: Quantifying Greenhouse Gas Sources and Sinks in Animal Production Systems. In: Eve, M., D. Pape, M. Flugge, R.Steele, D. Man, M. Riley‐Gilbert, and S. Biggar, Eds., Quantifying Greenhouse Gas Fluxes in Agriculture and Forestry: Methods for Entity‐Scale Inventory. Office of the Chief Economist, U.S. Department of Agriculture, Tech. Bull. 1939. Washington, DC. 606 p. July 2014.
Puchalski, M.A.; M.E. Sather; J.T. Walker; C.M.B. Lehmann; D.A. Gay; J. Mathew; W.P. Robarge. 2011. Passive ammonia monitoring in the United States: comparing three different sampling devices. J. Environ. Monit. 13,3156-3167.
Puchalski, M.A.; J.T. Walker; G.M. Beachley; M.A. Zondlo; K.B. Benedict; R.H. Grant; B.A. Schichtel; C.M. Rogers; A.B. Leytem; J. Rice; K. Morris; J.J. Schauer; R. Wang. 2019. Need for improved monitoring of spatial and temporal trends of reduced nitrogen. AWMA, Em Magazine (June) http://pubs.awma.org/flip/EM-July-2019/emjuly19.pdf
Rotz, C.A.; F. Montes; S.D. Hafner; A.J. Heber; R.H. Grant. 2013. Ammonia Emission Model for Whole Farm Evaluation of Dairy Production Systems. J. Environ. Qual., doi:10.2134/jeq2013.04.0121.
Rotz, C.A. and Oenema, J., 2006. Predicting management effects on ammonia emissions from dairy and beef farms. Transactions of the ASABE, 49(4), pp.1139-1149.
Schichtel, B.A; Gebhart, K.A.; Morris, K.H.; Cheatham, J.R.; Vimont, J.; Larson, R.S.; Beachley, G. 2019. Long-term trends of wet inorganic nitrogen deposition in Rocky Mountain National Park: Influence of missing data imputation methods and associated uncertainty. Science of the Total Environ. 687, 817-826, doi: 10.1016/j.scitotenv.2019.06.104.
Schwede, DB; G.G. Lear. 2014. A novel hybrid approach for estimating total deposition in the United States. United States Environmental Protection Agency Papers. Paper 219. http://digitalcommons.unl.edu/usepapapers/219.
Shah, S.B.; P.W. Westerman; J. Arogo. 2006. Measuring ammonia concentrations and emissions from agricultural land and liquid surfaces: a review. J. Air Waste Man. Assoc. 56, 945-960.
Shephard, M. W., Dammers, E., Cady-Pereira, K. E., Kharol, S. K., Thompson, J., Gainariu-Matz, Y., Zhang, J., McLinden, C. A., Kovachik, A., Moran, M., Bittman, S., Sioris, C. E., Griffin, D., Alvarado, M. J., Lonsdale, C., Savic-Jovcic, V., and Zheng, Q.: Ammonia measurements from space with the Cross-track Infrared Sounder: characteristics and applications, Atmos. Chem. Phys., 20, 2277–2302, https://doi.org/10.5194/acp-20-2277-2020, 2020.
Sintermann; J.; C. Spirig; A. Jordan; U. Kuhn; C. Ammann; A. Neftel. 2011. Eddy covariance flux measurements of ammonia by high temperature chemical ionisation mass spectrometry. Atmos. Meas. Tech., 4, 599-616
Sun, K.; Tao; L; Miller; D.J.; Zondlo; M.A.; Shonkwiler; K.B.; Nash; C.; Ham; J.M. 2015. Open-path eddy covariance measurements of ammonia fluxes from a beef cattle feedlot. Agric. Forest Meteorol. 213, 193–202
Tang, Y.S.; Simmons, I.; van Dijk, N.; Di Marco, C.; Nemitz, E.; Dammgen, U.; Gilke, K.; Djuricic, V.; Vidic, S.; Gliha, Z.; Borovecki, D.; Mitosinkova, M.; Hanssen, J.E.; Uggerud, T.H.; Sanz, M.J.; Sanz, P.; Chorda, J.V.; Flechard, C.R.; Fauvel, Y.; Ferm, M.; Perrino, C.; Sutton, M.A. 2009. European scale application of atmospheric reactive nitrogen measurements in a low-cost approach to infer dry deposition fluxes. Agric., Ecosy.& Environ. 133, 3-4, 183-195. Doi: 10.1016/j.agee.2009.04.027
Tevlin, A.G.; Y. Lin; J.L. Collett; E.E. McDuffie; E.V. Fischer; J.G. Murphy. 2017. Tall tower vertical profiles and diurnal trends of ammonia in the Colorado front range. J. Geophys. Res: Atmos. 122, 12468-12487.
USEPA, 2011. Reactive Nitrogen in the United States: An Analysis of Inputs, Flows, Consequences and Management Options. A Report of the EPA Science Advisory Board (EPA-SAB-11-013), 172 p. August 2011.
USEPA, 2017, 2014 Profile of version 1 of the 2014 National Emissions Inventory, U.S. EPA 2014 NEI Version 1.0, Office of Air Quality Planning and Standards, Emissions Inventory and Analysis Group.
Van Damme, M.; L. Charrisse; C.L. Heald; D. Hurtmans; Y. Ngadi; C. Clerbaux; A.J. Dolman; J.W. Erisman; P.F. Coheur. 2014. Global distributions, time series and error characterization of atmospheric ammonia (NH3) from IASI satellite observations. Atmos. Chem. Phys. 14, 2905-2922.
Van Damme, M.; J.W. Erisman; L. Charrisse; E. Dammers; S. Whitburn; C. Clerbaux; A.J. Dolman; P.F. Coheur. 2015. Worldwide spatiotemporal stmospheric ammonia (NH3) columns variability revealed by satellite. Geophys. Res. Let. 42, 8660-8668.
Walker, J.T.; D.R. Whitall; W. Robarge; H.W. Paerl. 2004. Ambient ammonia and ammonium aerosol across a region of variable ammonia emission density. Atmos. Environ. 38, 1235-1246.
Walker, J.T.; M.R. Jones; J.O. Bash; L. Myles; T. Meyers; D. Schwede; J. Herrick; E. Nemitz; W. Robarge. 2013. Processes of ammonia air-surface exchange in a fertilized Zea mays canopy. Biogeosci. 10, 981-998.
Walker, J.T.; G.M. Beachley; H.M. Amos; J.S. Baron; J. Bash; R. Baumgardner; M.D. Bell; K.B. Benedict; X. Chen; D.W. Clow. A. Cole; J.G. Coughlin; K. Cruz; R.W. Daly; S.M. Decina; E.M. Elliott; M.E. Fenn; L. Ganzeveld; K. Gebhart; S.S. Isil; B.M. Kerschner; R.S. Larson; T. Lavery; G.G. Lear; T. Macy; M.A. Mast; K. Mishoe; K.H. Morris; P.E. Padgett; R.V. Pouyat; M. Puchalski; H.O.T. Pye; A.W. Rea; M.F. Rhodes; C.M. Rogers; R. Saylor; R. Scheffe; B.A. Schichtel; D.B. Schwede; G.A. Sexstone; B.C. Sive; P.H. Templer; T. Thompson; D. Tong; G.A. Wetherbee; T.H. Whitlow; Z. Wu; Z. Yu; L. Zhang; 2019. Science needs for continued development of total nitrogen deposition budgets in the United States. U.S. Environmental Protection Agency, Washington, DC, EPA 601/R-19/001.
Wentworth, G.R.; J.G. Murphy; K.B. Benedict; E.J. Bangs; J.L. Collett Jr. 2016. The role of dew as a night-time reservoir and morning sources for atmospheric ammonia. Atmos. Chem. Phys. 16, 7435-7449.
Wichink, R.J.K; W.A.J. van Pul; R.P. Otjes; P. Hofschreuder; A.F.G. Jacobs; A.A.M. Holtslag. 2007. Ammonia fluxes and derived canopy compensation points over non-fertilized agricultural grassland in The Netherlands using the new gradient ammonia- high accuracy- monitor (GRAHAM). Atmos. Environ. 41, 1275-1287.