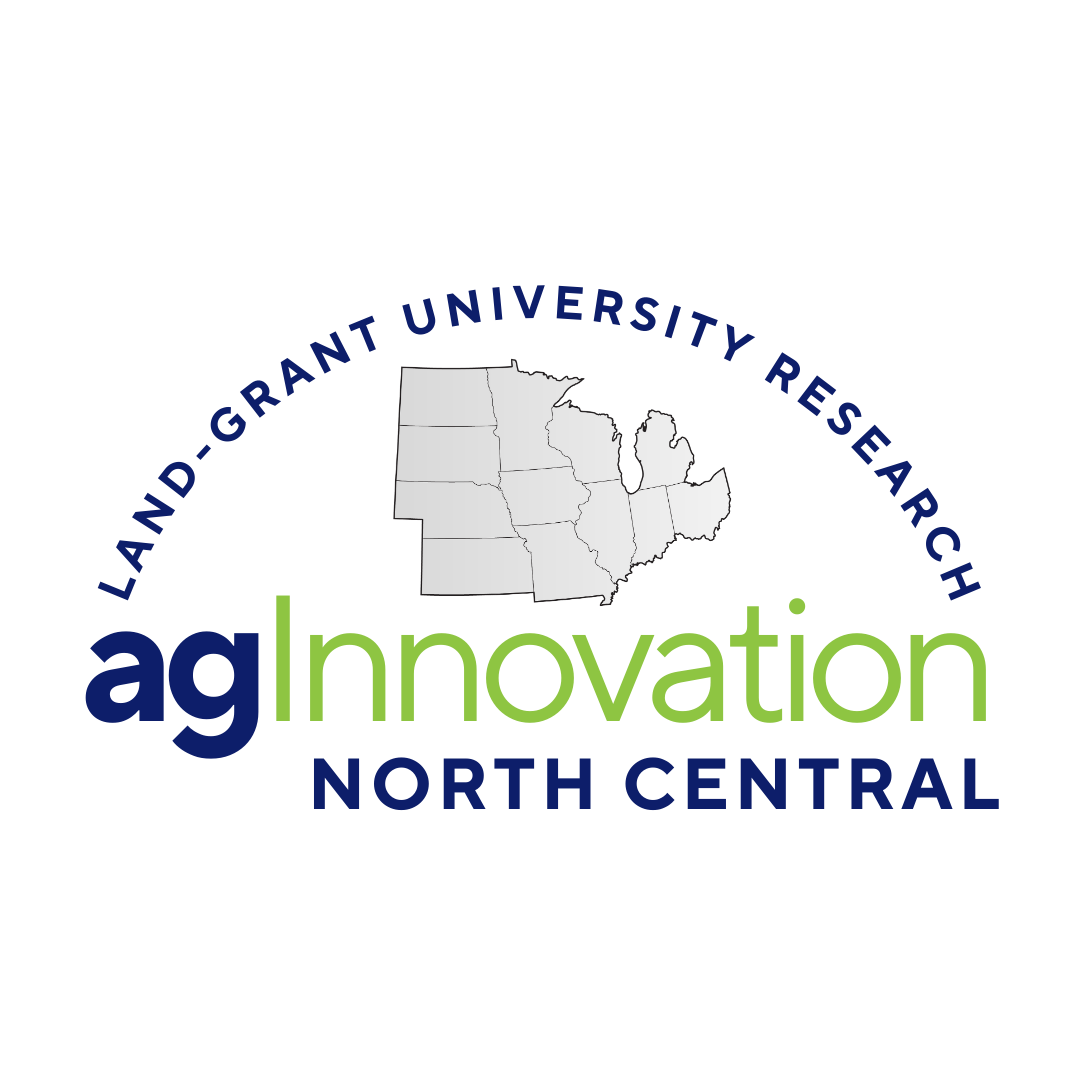
NC_old246: Ecology and Management of Arthropods in Corn
(Multistate Research Project)
Status: Active
NC_old246: Ecology and Management of Arthropods in Corn
Duration: 10/01/2020 to 09/30/2025
Administrative Advisor(s):
NIFA Reps:
Non-Technical Summary
Statement of Issues and Justification
Over 80 million acres of field corn (Zea mays) and 600,000 acres of sweet corn, worth about $65 billion and $1 billion, respectively, are grown in the United States each year. The European corn borer (ECB) (Ostrinia nubilalis) and western corn rootworm (WCR) (Diabrotica virgifera virgifera) account for over $1 billion each in terms of total annual costs of control and yield loss. Rootworms are especially problematic due to their propensity to evolve resistance to crop rotation and other management tactics (Gray et al. 2009) including insecticides (Meinke et al. 1998; Pereira et al. 2015) and genetically-modified (GM) hybrids with transgenic expression of one or more Bacillus thuringiensis (Bt) toxins (Gassmann et al. 2011, 2014, 2016; Ludwick et al. 2017). Most recently, resistance to the Bt Cry1F protein was documented among ECB in Nova Scotia, Canada (Smith et al. 2019a).
Other insect pests also cause significant economic loss to U.S. corn producers. The corn earworm (CEW) (Helicoverpa zea) and fall armyworm (FAW) (Spodoptera frugiperda) cause significant foliage and ear feeding damage in the southeast (Buntin et al. 2004), even when Bt corn hybrids are used. Resistance to corn producing the Cry1F protein has been reported for FAW populations in Puerto Rico, Florida and North Carolina (Storer et al. 2010; Huang et al. 2014). Field evolved resistance to sweet corn producing the Cry1Ab protein was described for CEW in Maryland (Dively et al. 2016). The western bean cutworm (WBC) (Striacosta albicosta) is a perennial pest in the western Corn Belt (Archibald et al. 2017; Smith et al. 2019b), but due to range expansion is also now a serious corn pest in areas of the Great Lakes region of the United States and Canada (Smith et al. 2018), in part due to resistance to the Bt Cry1F toxin (Ostrem et al. 2016; Smith et al. 2017). The northern corn rootworm (NCR) (D. barberi) is a perennial pest in much of the Corn Belt, and has evolved resistance to multiple Bt toxins (Calles-Torrez et al. 2019). The southern corn rootworm (SCR) (D. undecimpunctata howardi) causes occasional significant economic damage in the southeast. Wireworms (Elateridae) and white grubs (Scarabaeidae) can cause serious local problems. The common prophylactic use of neonicotinoid seed treatments to control these soil pests is not sustainable due to inconsistent economic returns and increasing environmental concerns (Mullin et al. 2005; Krupke et al. 2012; Tooker et al. 2017).
The current NC246 committee was formed in 2016 by merging the former NC205 (Ecology and Management of European Corn Borer and Other Lepidopteran Pests of Corn) and NCCC46 (Development, Optimization and Delivery of Management Strategies for Corn Rootworms) committees. These parent committees have a long history of addressing critical pest issues. NC205 was established in 1953 to address issues relating to the control of ECB, but expanded to include a broader array of corn pests. NCCC46 was established in 1964 as WCR was spreading eastward from the Great Plains into the Central Corn Belt (Gray et al. 2009). These two committees began co-locating their meetings in the 1990’s and collaborations between the two became increasingly valuable with the rapid adoption of Bt transgenic corn, the stacking of Bt traits targeting both corn rootworm and lepidopteran pests in the same plant, and the discovery of WCR field resistance to Cry3Bb1 toxin (Gassmann et al. 2011). Due to these increasing interactions, NC205 and NCCC46 committees were merged following a majority vote by both and a joint proposal for renewal submitted in 2015. The newly combined NC246 project was initiated in 2016. The formal merging of the committees has been scientifically and logistically beneficial, allowing the committee to address the larger corn insect pest complex, encompassing both above- and below-ground pests.
Since commercialization of Bt-transgenic corn targeting ECB in 1996 and rootworms in 2004, Bt corn acreage in the U.S. has increased from 8% in 1997 to 83% in 2019 (USDA NASS 2019). This has sparked a fundamental change in the management of corn insect pests by stakeholders. Since then, seed companies continue to develop GM crops with transgenic expression of crop protectants. Most current commercial GM hybrids express multiple Bt toxins targeting both Lepidoptera and Coleoptera. Initially, the use of GM crops often eliminated the need to apply insecticides for pest control, improving insect management for growers (Rice 2004; Sappington 2014). However, the development of resistance by target pests threatens the sustainability of these transgenic technologies.
Investigate the ecology, biology, evolution, genetics, genomics, and behavior of corn arthropods.
Dispersal of adults and gene flow within a species are critical factors for modeling the development and spread of resistance to Bt corn, as well as for implementing and monitoring the efficacy of IRM strategies. Regardless, these factors remain poorly understood for most corn pest species. Adult dispersal is high within ECB (Showers et al. 2001). Gene flow was also shown to be high between ECB pheromone strains, except at loci under sexual selection for strain differentiation (Coates et al. 2019). Genes involved in diapause differences were identified (Kozak et al. 2019), and their allele frequencies associated with increasing number of generations across latitudes (Levy et al. 2015). An inversion on the Z chromosome housing genes determining diapause variation is at highest frequency in populations differing in both pheromone and voltinism traits (Kozak et al. 2017), wherein allelic combinations within the inversion were suggested to be “optimized” for local conditions (Coates et al. 2018). A genetic marker was developed to differentiate pheromone strains based on specific alleles at the female pheromone production locus, the pheromone gland fatty acyl-reductase gene (pgfar; Coates et al. 2013a), but analogous markers are needed to determine and estimate gene flow between voltinism ecotypes. A region of the Z chromosome containing four genes was linked to differences in male pheromone response (Koutroumpa et al. 2016), but the specific gene needs to be determined and genetic markers developed to track this trait in field populations.
There is a high beetle dispersal rate in WCR (Spencer et al. 2009), which is density dependent (Yu et al. 2019a). Correspondingly, no genetic differentiation has been detected within WCR populations (Kim and Sappington 2005; Coates et al. 2009). A recent study corroborated the homogenization of WCR across the Corn Belt to the Atlantic coast, but also determined the ancestral source of the United States population was located in Mexico (Lombaert et al. 2018). Within this genetically homogenized United States population, no variation was predicted between WCR soybean variants compared to non-variants (Miller et al. 2006). Analogously, high levels of gene flow were reported between NCR populations with 1-year compared to 2-year extended diapause (Krafsur 1995). It remains a challenge to detect variation among variant phenotypes in corn rootworm populations. Although a relatively large number of SNP markers were developed for WCR (Coates et al. 2016), there still is a need to apply these or other markers within population genetic analyses or genome-wide association studies. Spatial and temporal aspects of adult movement have proven difficult to characterize. Additional population genetics studies and the development of molecular genetic tools are critically needed to more accurately estimate dispersal distances of corn pest insects.
The high dose refuge strategy has been implemented within IRM plans to delay the onset of Bt resistance among WCR and ECB, wherein non-Bt refuges were planted in a structured block design. This scenario changed in 2010 when regulatory decisions allowed the use of blended refuges consisting of either 5 or 10% of non-Bt seed to be planted in the Corn Belt, referred to as “refuge in a bag” (RIB) products. RIB had the advantage of facilitating grower refuge compliance. Structured refugia did not facilitate the expected movement of mate-seeking adults from refuge and Bt corn blocks; WCR abundance was distributed uniformly across fields planted with RIB products (Hughson and Spencer 2015). Mating between WCR from refuge and Bt corn was more likely in seed blends than in block refuges; however, the low abundance of refuge-produced WCR in seed blends likely limits their contribution to delaying resistance (Taylor and Krupke 2018). There has been a relatively recent evolution of Bt resistance among several ear-feeding lepidopteran pest species including CEW, FAW, and WBC (Tabashnik and Carrière, 2017), but the cause of some of these events remains uncertain. Resistance in FAW seems to be linked to alterations in receptor genes for the Cry1F and Cry1Ab proteins, and current evidence suggests the frequency of these resistance alleles may be high in some locations (Banerjee et al. 2017). Larvae may be exposed to varying levels of one or more Bt toxin when feeding on kernels of cross-pollinated refuge plants, which is exacerbated via intra-plant movement (Mallet and Porter 1992; Tabashnik 1994). Field studies show higher larval densities on non-Bt refuge corn plants within structured compared to blended refuges for ECB (Oyediran et al. 2016) and CEW (Burkness et al. 2015), but not for the sugarcane borer (Wangila et al. 2013). Larval behaviors influencing differences in exposure and survival observed between species remain unknown, but research is needed to investigate timing of larval movements, tissues fed upon, and Bt toxin exposures across instars. Moreover, while exposure to sublethal levels of Bt proteins in lepidopteran larvae alters their migratory behaviour as adults (Jiang et al. 2013), there is no available data on this process or how it may affect resistance management tactics in CEW, FAW or ECB populations. Understanding these factors is crucial for evaluating the efficacy of current IRM strategies and product durability for ear-feeding pest species of Lepidoptera.
Genomic tools have been developed and applied. For instance, WCR, ECB and FAW genome and transcriptome sequence assemblies have been produced through international and multi-institutional efforts involving several NC246 members. Application of these genomic resources will facilitate probing the genetic basis of many important pest traits, including resistance to insecticides and Bt toxins (Coates 2016; Banerjee et al. 2017; Abdelgaffar et al. 2019), behaviors relevant to pest status, and insect-plant interactions. To date, applications of large numbers of genetic markers have resulted in identification of genes or genomic regions controlling organophosphate resistance in WCR (Coates et al. 2016) and Cry1F resistance in ECB (Coates and Siegfried 2015). Differential gene expression studies suggest candidate genes involved in Bt resistance in WCR (Rault et al. 2018) and ECB (Vellichirammal et al. 2015; Yao et al. 2017), WCR rotation resistance (Chu et al. 2015), and insecticide resistance (Wang et al. 2013; Coates et al. 2016). Transcriptome profiling has allowed the identification of field-evolved alleles for resistance to Cry1F and Cry1Ab in FAW (Banerjee et al. 2017). The effect of these resistance alleles on the metabolism of FAW larvae have been studied to identify resistance markers (Abdelgaffar et al. 2019). Additionally, genomic research has led to the identification of genes controlling differences in diapause duration between univoltine and bivoltine ECB (Kozak et al. 2019), and estimation of gene flow among ECB populations (Coates et al. 2019).
Develop and assess Integrated Pest Management systems for the arthropod complex in corn. Promotion of IPM-based approaches has reached a critical cross-road, as resistance to some toxins has led to recommendations from various quarters to layer multiple control tactics on Bt corn, including conventional insecticides, without assessing actual need. Since 2004, the percentage of U.S. corn acreage with seed-applied neonicotinoids has increased dramatically, and they are now used on virtually all non-organic corn acres (Douglas and Tooker 2015; Tooker et al. 2017; Papiernik et al. 2018). Changes in pest insect biology, pest management technologies, and regulatory issues have illustrated the fact that much of our past information and research about the corn cropping system must be re-evaluated. However, across most of the Corn Belt the distributions of targeted early-season pest species are sporadic and patchy, and most crop fields do not experience annual economic infestations. Furthermore, neonicotinoids have potential roles in pollinator declines and contamination of surface waters (Goulson 2013; van der Sluijs et al. 2014). Seed-applied active ingredients can persist in soil for up to two years and move into aquatic systems where they can reduce aquatic invertebrate communities (Laurent and Rathahao 2003; Krupke et al. 2012; Van Dijk et al. 2013). A recent Canadian study revealed substantial ground water contamination with thiamethoxam (Main et al. 2014). Clearly, an evaluation of the effects of seed treatments upon a range of non-target insects in and around cornfields is warranted.
Characterize and monitor pest resistance and assess Insect Resistance Management and mitigation strategies. More information is needed regarding sensitivity to Bt, and the influence of host plants. Furthermore, the impact of Bt corn on the ecology and behavior of lepidopteran target pests, such as CEW, FAW, and WBC is important, especially in relation to Bt resistance of these pests and the worldwide range expansion of FAW. The Committee has an interest in the use of Bt and other insect control tactics in an economically viable and sustainable manner that will prevent or delay the onset of insect resistance. Ensuring long-term durability of these tools requires IRM strategies that are compatible with production practices.
Monitoring for resistance among pest insects targeted by GM corn-incorporated insecticidal toxins remains a United States Environmental Protection Agency (US EPA)-mandated component of IRM plans (Siegfried and Spencer, 2012). Being able to detect insecticide resistance is necessary to determine if control failures occur because of resistant insects or other factors, determine the extent of the resistance, and design resistance mitigation programs. This is particularly important for WCR field populations, which have confirmed resistance to, and cross-resistance among Cry3Bb1, eCry3A, mCry3A (Gassmann et al. 2011, 2014), as well as resistance to Cry34/35Ab1 (Gassmann et al. 2016, Ludwick et al. 2017). Analogous resistance has been shown in populations of NCR (Calles-Torrez et al. 2019). Recently, field-evolved resistance to Cry1F for WBC (Smith et al. 2017) and FAW (Storer et al. 2010; Huang et al. 2014), as well as field-evolved resistance in CEW to Cry1A.105 and Cry2Ab2 in sweet corn (Dively et al. 2016) and field corn (Bilbo et al. 2019), have been reported. Although no field failures are documented, alleles conferring FAW resistance to Vip3A corn were identified in FAW field populations (Yang et al. 2019). Identification of resistance alleles to Cry1F in FAW has allowed preliminary efforts at developing DNA-based resistance monitoring tools with increased sensitivity and amenability to high throughput (Banerjee et al. 2017). This research is opening the door to the development of more sensitive, cheaper and high throughput resistance monitoring methods. However, to realize this vision, there is an urgent need to characterize the mechanism(s) of resistance among corn pests, and develop and deliver IPM recommendations utilizing multiple pest control strategies to mitigate the development of resistance. There is also need to assess the geographic distribution of Bt susceptibilies, as well as a proactive plan to address the risk of resistance to Vip3A and other insecticides. As additional pests become primary targets of transgenic technology, monitoring methodologies and programs will have to accommodate these new target pests.
Damage by ear-feeding Lepidoptera has increased during the last decade partly due to a range expansion of the WBC (e.g., Archibald et al. 2017; Smith et al. 2019b), and the development of resistance to Bt hybrids among CEW, FAW, and WBC that is associated with increased aflatoxin levels in the grain. The corn pest complex includes several Lepidoptera, but also coleopteran and hemipteran pests, which presents significant management and research complications. Basic issues such as accurate quantification of injury and the effects of sequential feeding require characterization, as well as the broader issues relating to IPM and IRM.
Employ traditional and innovative delivery methods to disseminate information. This includes information and educational materials related to sustainable management of corn arthropod pests delivered to farmers, industry, academic colleagues, agricultural professionals, educators, and policy makers. Packaging unbiased results for agricultural and public sector stakeholders remains critical. Although traditional methods for information delivery are still needed, the use of timely, interactive and mobile accessible digital formats is in increased demand.
A Multi-State Approach. Collectively, a multi-state approach to researching the knowledge gaps described above, developing IPM tools and programs, assessing IRM strategies, and implementing technology transfer is appropriate and necessary. Geography plays an important role in how pests interact with other organisms in their environment, and how IPM and IRM strategies are designed and employed. This significant spatial effect of population and community dynamics makes a regional project necessary. Lack of knowledge has led to fears by the general public about the potential environmental and health risks associated with adoption of new technologies. Controversy about the effect of GM and seed treatment technology on non-target organisms and human health has fueled public concerns. These fears have the potential of forcing legislation to ban or slow the introduction of these technologies. Answers to questions regarding new technologies should help focus the public's perception of them and allow growers to gain the pest control advantages provided by future technologies. Timely, directed, and relevant national research efforts that span multiple climates and cropping systems is facilitated through coordination of the NC246 multistate research committee.
The proposed multi-state plans will continue to be a model for the development of science-based resistance management programs and risk assessment for other pests, other crops, and future crop protection technologies. Our efforts will provide fundamental advances in the knowledge of pest ecology, genetics, behavior, and evolution. Our work will continue to provide science-based assessments essential to the policy decision-making process, which should help to increase the public's acceptance of environmentally friendly technologies while identifying potential negative impacts that need further investigation. Our work also will continue to lead to more sustainable pest management systems for corn pest complexes. There is ample evidence that the NC246 research group has the skills, collaborative working relationships, and commitment to provide the missing biological information and to incorporate this new information into evolving IPM programs and IRM models.
Related, Current and Previous Work
A CRIS search indicates that there are 118 active projects related to ‘corn Lepidoptera,’ 61 for ‘corn Diabrotica,’ and 617 for ‘corn insects.’ Of these, 69 are directly associated with NC246. Several USDA-ARS units conduct research on insect pests of corn, including units at Tifton, GA, Stoneville, MS, Gainesville, FL, Mississippi State, MS, Fargo, ND, Brookings, SD, Columbia, MO, and Ames, IA. The Corn Insects and Crops Genetics Research Unit at Ames, IA conducts considerable research on corn pests, and several of its research scientists are members of NC246.
A NIMSS search indicates three active Multistate Research Projects other than NC246 that involve corn arthropod pests: NE-1332 Biological Control of Arthropod Pests and Weeds; S-1052 Working Group on Improving Microbial Control of Arthropod Pests; and W-3185 Biological Control in Pest Management Systems in Plants. None of these projects deal with the entire array of natural enemies for even a single corn insect pest, so NC246 will continue to deal specifically with natural enemies of corn pests. We have established cooperative interactions with members of these committees and we will continue to coordinate efforts with other regional projects.
There are two active Coordinating Committees that involve corn arthropod pests: NECC-1008 Improving Sweet Corn: Genetics & Management and NECC-029 Northeastern Corn Improvement Conference. NECC-029 is quite broad, addressing a few facets of corn rootworm resistance management only in the northeast region. NECC-1008 provides a forum for information exchange on current and emerging sweet corn pests.
There are several active Education/Extension and Research Activity Committees that, in some part, address corn arthropod pests. Some are based largely on the principles of IPM (e.g., SERA-003 Southern Region Exchange Group for IPM), but these are very broad and address corn arthropod pests only in brief. Others address biological control or resistance management (e.g., NCERA-220 Biological Control of Arthropods and Weeds, WERA-060 Management of Pesticide Resistance), but their primary purpose is information exchange and awareness, not in-depth and collaborative research. We know of no other research projects engaged in regionally coordinated research that focus on the ecology, evolution, genetics, behavior, and management of corn arthropod pests.
Investigate the ecology, biology, evolution, genetics, genomics, and behavior of corn arthropods
NC246 members have developed WCR genomic resources, including a large-insert bacterial artificial chromosome library, DvvBAC1 (Coates et al. 2012), single nucleotide polymorphism (SNP) markers (Coates et al. 2009), and transcriptome assemblies (Siegfried et al. 2005; Knolhoff et al. 2010; Adedipe et al. 2019). Using these resources, a gamma-aminobutyric acid (GABA) receptor mutation was found causal of organochlorine resistance in WCR (Wang et al. 2013), and differential expression of an esterase was associated with organophosphate resistance (Coates et al. 2016). Despite the WCR genome being large (2.56 Gbp) and highly repetitive (Coates et al. 2012, 2014), the Diabrotica Genetics Consortium, which includes several NC246 scientists, publicly released a draft genome assembly (GenBank accession PXJM00000000.2). This assembly was based on an inbred WCR strain developed at ARS Brookings. Analogously, an ECB genome was released by an NC246 member (Coates et al. unpublished; GenBank SWFO00000000.1). In ECB, differential expression of midgut genes (Vellichirammal et al. 2015) and a single quantitative trait locus (QTL) were linked to Cry1F resistance (Coates et al. 2011; Coates and Siegfried 2015). Also, Cry1Ab resistance in ECB is controlled by two QTL modify the expression of an unlinked aminopeptidase N1receptor (Coates et al. 2013b). These studies are pertinent given the recent documentation of ECB resistance to Cry1F hybrids in Nova Scotia, Canada (Smith et al. 2019a).
Z-race ECB is widespread east of the Rocky Mountains, but E-race is restricted to the Northeast U.S. (Mason et al. 2018). E- and Z-races are sympatric in the Northeast and show partial genetic isolation (Dopman et al. 2005). Two ECB ecotypes differ in the initiation and termination of diapause. Variation in daylength and temperature influence post-diapause development, Pdd, times and results in differences in number of mating generations per year (voltinism). Univoltine ECB are restricted to northern latitudes and have obligatory diapause after a single mating generation. In contrast, multivoltine ECB are more geographically dispersed where Pdd responds to local environmental cues, such that >1 annual mating generation may occur. Newly emerged multivoltine females engage in migratory behavior (Reardon et al. 2006; Dorhout et al. 2008), with indirect evidence suggesting 1st and 2nd generations may behave similarly (Sappington 2018). However, effect of larval diapause on flight behavior has not been tested. Longer Pdd times among univoltine individuals results in delayed adult emergence compared to multivoltine counterparts (Dopman et al. 2005; Levy et al. 2015), which may lead to between-location or year variation in mating asynchrony and gene flow (Dopman et al. 2010). Transition across one, two, and three annual reproductive generation regions is correlated with cyclical changes in allele frequency at period, per, gene locus (Levy et al. 2015). Recently genetic mapping indicated that per and dpfr genes interact to determine Pdd differences between ECB voltinism ecotypes (Kozak et al. 2019).
Resistance by FAW to corn producing the Cry1F protein originally emerged in Puerto Rico (Storer et al. 2010), but was later found in Florida and North Carolina (Huang et al. 2014). Migratory models for FAW in North America suggest movement of FAW from the Caribbean into an overwintering FAW population in FL, which migrates northward yearly along the eastern US seaboard (Nagoshi et al. 2017). Identification of the allele linked with FAW resistance to Cry1F in Puerto Rico and subsequent genotyping of field collected samples (Banerjee et al. 2017), support that resistance in Puerto Rico and FL represent independent resistance events and are not a result of resistance migratory dispersal. Rapid evolution of FAW resistance to Cry1F compared to ECB (Vélez et al. 2016), lack of fitness costs associated with FAW resistance (Vélez et al. 2013a; Jakka et al. 2014), and information from allele frequency estimations (Vélez et al. 2013b; Banerjee et al. 2017) support that resistance in FAW emerges locally and is accelerated by the resistance gene being highly polymorphic. Moreover, resistant FAW adults display increased flight tendency (Jurat-Fuentes et al, unpublished data), and the implications for this behavioral alteration in IRM practices needs examination.
Develop and assess IPM systems for the arthropod complex in corn
Economic injury levels, economic thresholds, and sampling tools have been developed for WBC (Paula-Moraes et al. 2011, 2012, 2013). However, the utility of these tools can be compromised by the presence of other ear-feeding pests, particularly non-lepidopteran species. There are several kinds of insects that feed on corn ears besides Lepidoptera, including multiple species of stink bug (Pentatomidae) and sap beetles (Nitidulidae). Several species may occupy the ear at the same time, and current research is being designed and conducted to address differentiating injury types, the effects of competition, and consequent effects on IPM and IRM. Economic thresholds for adult WCR and NCR in corn and for crop-rotation resistant WCR in soybean were recently revised (Dunbar and Gassmann 2013). Despite availability of IPM strategies, there remains little practical integration of corn pest management tactics in the Corn Belt (Gray 2011).
WCR populations are not genetically differentiated in the Corn Belt as a consequence of range expansion (Kim and Sappington 2005; Flagel et al. 2014), but population genetics analyses indicate genetic structuring exists in eastern Colorado and western Kansas. This has allowed estimates of spatial gene flow and genetic neighborhood radius (Kim et al., unpub.). Considerable progress has been made in estimating short-range WCR dispersal behavior (Spencer et al. 2009, 2013). Understanding both short- and long-range dispersal of WCR is critical to developing effective insecticide resistance management and mitigation strategies (Miller and Sappington 2017). Overwintering populations of FAW host races in FL and TX re-infest the northern and eastern U.S. each summer via long-distance migration. Host races are distinguishable based on mitochondrial DNA haplotypes. Recent research extended the haplotype map to Mexico and South America and confirmed that regional differences are stable over time (Nagoshi et al. 2015).
Without a corresponding increased threat from insect pests, neonicotinoid seed treatments are now used on virtually all non-organic corn acres. These seed treatments are deployed outside of an IPM framework (i.e., field histories or pest infestation levels do not drive their use). The early-season pest species targeted by these seed-applied insecticides are generally considered secondary pests and by definition are sporadic and patchily distributed. As a result, the large majority of crop fields do not annually experience economically significant populations of the pest species; thus, the insecticide has little opportunity to provide a benefit (Higley and Boethel 1994; Pedigo and Rice 2008; Papiernick et al. 2018). Most producers appear to be unaware that neonicotinoids only target a subset of the pest complex for a relatively brief period (2-3 weeks maximum) in each growing season (Alford and Krupke 2017) and that the pests targeted are infrequently encountered and often require no intervention (Papiernick et al. 2018). Research on neonicotinoid seed treatments has recently been directed at their potential role in pollinator declines and for evidence suggesting that neonicotinoid contamination of surface waters can be negatively correlated with abundance of some vertebrate and invertebrate species (Goulson 2013; Van Dijk et al. 2013; van der Sluijs et al. 2014). Mammals and migratory bird species may also incur non-target effects of these ubiquitous chemicals (Berheim et al. 2019; Eng et al. 2019). It is clear that there is a poor understanding of the potential influence of such widespread adoption of insecticides with such a high toxicity. The role of planter dust has received attention recently (Krupke et al. 2012, 2017), and although it is possible to mitigate this exposure route with planter and/or seed lubricant alterations, to date there is no progress on this front. Only a minority of the seed-applied active ingredient is actually absorbed by the plant and the material left behind can persist in soil for up to two years and move into groundwater where it can simplify aquatic invertebrate communities and significantly retard decomposition (Laurent and Rathahao 2003; Van Dijk et al. 2013).
Characterize and monitor pest resistance and assess IRM and mitigation strategies
Field evolved resistance by WCR to insecticides is well documented. Resistance has been confirmed to insecticides in the cyclodiene, carbamate, and organophosphate classes (Ball and Weekman 1962, 1963; Meinke et al. 1998) and recently the pyrethroid class (Pereira et al. 2015). Data collected to date indicate that repeated exposure of adult WCR to foliar applications of pyrethroid insecticides led to resistance evolution. Adult resistance monitoring in the U.S. Corn Belt with a diagnostic dose of bifenthrin indicated that resistance was limited to the western Corn Belt (especially SW Nebraska and SW Kansas, Pereira et al. 2015). Follow-up work using simulated aerial application methodology confirmed that formulated bifenthrin was ineffective at the highest registered rate (Souza et al. 2019a). Results of replicated field trials indicated that formulated soil-applied WCR pyrethroid insecticides were also ineffective in areas where adult pyrethroid resistance had been documented (Souza et al. 2019b). Cross-resistance and synergism bioassays suggested that both detoxifying enzymes and target site insensitivity might be involved as resistance mechanisms (Pereira et al. 2017). Follow-up biochemical assays using model substrates as well as High-Throughput RNA Sequencing revealed that insecticide-related metabolism in pyrethroid-resistant WCR populations was significantly enhanced compared to pyrethroid-susceptible populations (Souza 2019).
With the advent of pyramided Bt corn (corn with two or more Bt toxins with different modes of action targeting one or closely related insect species), refuge has evolved from a structured refuge approach to an unstructured refuge approach that uses a blend of Bt and non-Bt corn seed (i.e., refuge in a bag). Theoretically, pyramids require less refuge (Gould et al. 2006; Zhao et al. 2003), and EPA has allowed reduced refuge for some pyramided corn (Coons 2009). However, recent modeling suggests larval biology and behavior, such as dispersal between Bt and non-Bt corn, may compromise seed blends as a resistance management strategy (e.g., Goldstein et al. 2010; Razze et al. 2011; Yang et al. 2014). ECB neonate dispersal is greater on Bt corn compared with non-Bt corn (Razze and Mason 2012). Recent research has indicated that larvae of several lepidopteran species (e.g., ECB, FAW) exhibit more plant-to-plant movement than was expected, particularly in early instars.
Annual assessments of the susceptibility of target pest species such as ECB and FAW have been ongoing. This research has involved diagnostic bioassays (Marçon et al. 2000; Siegfried et al. 2007), F1 and F2 screening approaches (Vélez et al. 2013a,b; Siegfried et al. 2014), and more recently the use of DNA-based genotyping for known FAW resistance alleles (Banerjee et al. 2017) to isolate resistance alleles and assess frequencies. In instances where resistance has been identified, specific research to identify inheritance patterns, fitness costs (Crespo et al. 2010; Pereira et al. 2011; Vélez et al. 2013a; Jakka et al. 2014; Petzold-Maxwell et al. 2014), and molecular mechanisms of resistance have been conducted (Crespo et al. 2011; Coates et al. 2011, 2013a; Coates and Siegfried 2015; Jakka et al. 2016; Banerjee et al. 2017; Abdelgaffar et al. 2019). Bioassays with Bt toxins have relied on standard methods involving surface treatment of artificial diet with toxin preparations (Marçon et al. 1999) and have provided consistent assessments of susceptibility for more than a decade (Siegfried et al. 2007; Siegfried and Hellmich 2012; Siegfried and Spencer 2012). A variety of biochemical, molecular, and genomic tools have been developed to assess mechanisms of resistance (Coates et al. 2011; Crespo et al. 2011; Jakka et al. 2016) and identify resistance alleles (Banerjee et al. 2017). Both CEW (Dively et al. 2016; Bilbo et al. 2019) and FAW (Huang et al. 2014; Li et al. 2016; Jakka et al. 2014, 2015) show resistance to more than one Bt toxin, which reduces producer ability to control associated damage. WBC resistance to Bt Cry1F toxin has been documented in Ontario, Canada (Smith et al. 2017). NCR is a perennial pest in much of the Corn Belt and has evolved resistance to multiple Bt toxins (Calles-Torrez et al. 2019).
Resistance to Cry3Bb1 corn was initially documented in Iowa (Gassmann et al. 2011, 2012). Cry3Bb1 resistant WCR were shown to be cross-resistant to mCry3A (Gassmann et al. 2014) and eCry3.1Ab (Jakka et al. 2016). More recently, WCR resistance to Cry34/35Ab1 was documented in Iowa (Gassmann et al. 2016) and Minnesota (Ludwick et al. 2017), which exhibits no cross-resistance to Cry3Bb1 (Zhao et al. 2016). Analysis of WCR collected from other regions confirmed resistance to “Cry3” (Cry3Bb1, mCry3A, and eCry3.1Ab) corn across the Midwest (Wangila et al. 2015; Zukoff et al. 2016; Schrader et al. 2017; Reinders et al. 2018). A WCR resistance management strategy based on the “high dose/refuge” concept has been shown to be ineffective for currently available rootworm Bt events which produce less than a high-dose of Bt toxin (Andow et al. 2016). Because commercially available rootworm events are not high dose, this allows for some larval survival and adult emergence (Hibbard et al. 2011; Frank et al. 2015; Hitchon et al. 2015; Keweshan et al. 2015; Head et al. 2017). Limited data has been collected on fitness of adults emerging from Bt events. To date, few WCR fitness costs have been associated with resistance to Bt events (Oswald et al. 2012; Meihls et al. 2012; Hoffmann et al. 2015; Ingber and Gassmann 2015). Cry3Bb1 resistance persists over time in the field (Gassmann et al. 2014; Wangila and Meinke 2017) and when frequency of Cry3Bb1 individuals is high in a population the average delay in adult emergence from Cry3Bb1 commonly reported in the literature (e.g., Murphy et al. 2010; Clark et al. 2012; Hitchon et al. 2015; Keweshan et al. 2015) can be substantially reduced (Wangila and Meinke 2017). A single QTL on LG8 was linked to Cry3Bb1 resistance in WCR and screening of field populations with markers within the QTL were modestly predictive of resistance (Flagel et al. 2015). Additional data on resistant WCR fitness and mechanisms of resistance are needed.
A series of experiments have been conducted to develop an improved, standardized artificial diet for western corn rootworm that can be publically available. An initial diet (WCRMO-1) that improved upon an older published WCR diet (Pleau et al. 2002) was published by Huynh et al (2017). WCR development and survival were significantly different when proprietary WCR diets, the older published diet (Pleau et al. 2002) and the new diet were compared (Meihls et al. 2018). A number of improvements have been made to the WCRMO-1 diet (Huynh et al. 2019a) including removal of corn root powder (WCRMO-2, Huynh et al. 2019b). An artificial diet for NCR has also recently been developed (Huynh et al. 2019c). The WCRMO-1 diet has been shown to be compatible with rootworm Bt proteins and can differentiate resistant WCR colonies from susceptible colonies (Ludwick et al. 2018). These new diets will enable standardization of diet-overlay bioassays across labs to monitor for WCR or NCR resistance.
As noted above, questions have arisen with respect to larval movement, and the efficacy of seed blends in slowing the development of resistance. Associated with movement are the consequent effects of larval feeding on corn ears that are the result of cross-pollination between Bt corn and non-Bt corn (e.g., Chilicutt and Tabashnik 2004; Burkness et al. 2011; Burkness and Hutchison 2012; Kang et al. 2012; Yang et al. 2014). Reduced mortality was observed by ECB larvae feeding on kernels of Bt corn expressing Cry1Ab toxin (Burkness et al. 2011), and recent studies have indicated that some species (e.g., corn earworm) may exhibit differential feeding on kernels expressing different, or no, Bt toxin. Differential expression of Bt toxins in corn ears has been suggested to affect the evolution of resistance in Lepidoptera that feed primarily in the ear (e.g., corn earworm, western bean cutworm) (Burkness et al. 2011; Onstad et al. 2011). Using purple seeded corn as a marker, studies (Dively et al. 2019) showed that outcrossing of pollen from the refuge plant in a seed blend averaged <1% in neighboring Bt ears, but was much greater from Bt pollen in the opposite direction, averaging 18.6% in refuge ears. Laboratory bioassay further showed that consumption of a meridic diet containing 6% refuge kernel tissue collected from a 95:5% seed blend field caused significant larval growth inhibition of CEW and ECB larvae.
Objectives
-
Investigate the ecology, biology, evolution, genetics, genomics, and behavior of corn arthropods.
Comments: A. Characterize dispersal of arthropod corn pests, and assess its implications for Integrated Pest Management (IPM) and for resistance development, spread, and mitigation. B. Improve rearing and bioassay methodologies, including development of arthropod pest artificial diets and standardized procedures. C. Investigate aspects of ecology, including gene flow, among spatially or temporally differentiated corn pest subpopulations or races/strains/biotypes. D. Assess effects of seed blend refuge in Bacillus thuringiensis (Bt) transgenic corn on biology, development, behavior, and genetics of multiple lepidopteran pest species. E. Examine the potential role of microbial associates on important pest traits, including insecticide resistance, behaviors relevant to pest status, and insect-plant interactions. F. Develop genomics tools for key corn pests, including assembled and annotated genome and transcriptome sequences, genetic markers, and quantitative trait locus maps of important traits. -
Develop and assess IPM systems for the arthropod complex in corn.
Comments: A. Coordinate early detection and mitigation networks for invasive species. B. Assess the relationships between pest management technologies with respect to the agricultural landscape and producer profitability. C. Assess the need for and efficacy of insecticidal agents, including chemical insecticides, Bt toxins, and RNA interference (RNAi) molecules. D. Evaluate possible effects of pest management interventions on non-target organisms. E. Investigate the potential for the use of biological control and bio-based insecticides, including conservation of natural enemies. F. Assess the extent to which the availability of management tools, including Bt traits and seed treatments, affects grower choice and IPM and Insect Resistance Management (IRM) adoption. -
Characterize and monitor pest resistance and assess IRM and mitigation strategies.
Comments: A. Establish resistance monitoring networks for corn pests, using laboratory diet bioassays or field sentinel plots. B. Characterize the geographic range, demographics, and nature of arthropod pest resistance to insecticidal agents (e.g., chemical insecticides, Bt toxins, and RNAi molecules) C. Identify genes, genome regions, and cellular and biochemical mechanisms involved in resistance to insecticidal agents. D. Develop region-specific bioeconomic models to assess refuge and IPM strategies for managing pest resistance to Bt corn. E. Use data generated in the above efforts to develop and evaluate IPM and IRM strategies. -
Employ traditional and innovative delivery methods to disseminate information related to sustainable management of corn arthropod pests to farmers, industry, colleagues, agricultural professionals, and policy makers.
Methods
Obj. 1. Ecology, biology, evolution, genetics, genomics, and behavior of corn arthropods.
A) Characterize dispersal of arthropod corn pests, and assess its implications for IPM and IRM.
Work in IL will include the characterization of rootworm attraction and orientation to volatiles from trap crop plantings to develop more effective trap crops using biotechnological approaches. Analyses of WCR engaged in flight will reveal the proportion dispersing beyond the field and their respective physical and reproductive characteristics. Multiple states will participate in a study led by FL to map seasonal migration of lepidopterans, such as FAW, into the central and eastern U.S. using a network of pheromone traps and molecular genetic markers, including Bt-resistance genes. Several states (MI, OH, NY) and six Canadian provinces will collaborate in the Great Lakes and Maritimes Pest Monitoring Network where pheromone traps will monitor for shifts in the distribution and phenology of important lepidopteran corn pests and indicate when scouting and management decisions are required. The network will be used in conjunction with sentinel plots and pest collections for bioassays to determine resistance development. Several states (PA, MD, DE) will conduct pheromone trap monitoring to assess the spread of WBC into the mid-Atlantic region. In MD, CEW and FAW will also be monitored at multiple sites. Flight mill experiments (IA) will compare ECB adults emerging after larval diapause with those reared from non-diapause larvae. Diapause of laboratory colony insects maintained by USDA (IA) will be manipulated in growth chambers, and both sexes tested using flight mill methods described previously (Dorhout et al. 2008; Rovnyak et al. 2018) on a new state of the art flight mill system (Yu et al. 2019a,b). In TN, the effect of larval feeding on Bt corn in FAW migratory behaviour will be tested using flight mills and include comparisons with Cry1F-resistant strains to determine effects of resistance genotype on flight behaviour.
B) Improve rearing and bioassay methodologies, including artificial diets.
MO will be working with Frontier Scientific to develop and evaluate diet formulations for WCR and NCR larvae that are commercially available to all. The diets initially are intended for toxin overlay assays in resistance management and new toxin evaluations. Eventually, it may be possible to rear WCR all the way to beetle emergence. We will evaluate changes in microbiota with the WCR associated with improved diets and wild WCR and NCR. IA and NE will investigate WBC dietary nutrition and rearing conditions to improve laboratory fecundity. Use of non-commercial single batch Bt toxins will be explored in efforts to standardize bioassays.
C) Ecology and gene flow among pest races/strains/biotypes.
Characterize races of corn pests, including ecology of races in sympatry. IA will lead studies, in collaboration with states such as DE and PA, on the characterization of races of corn pests. Temporal genetic variation will be assessed in geographic regions with sympatric ECB voltinism ecotypes using SNP markers inclusive of those in development for genes linked to voltinism variation (Kozak et al. 2019). Estimates of genetic differentiation among previously genotyped samples of sympatric voltinism and pheromones strain ECB (Coates et al. 2019) will be re-evaluated following integration of genetic markers defining voltinism and/or male response phenotypes. The role of an inversion on the Z chromosome of ECB, differing in frequency within populations differing in both voltinism and pheromone strain (Kozak et al. 2017), will be assessed by determination of haplotype variation at genes influencing these traits. A project led by TN will develop next generation sequencing to determine dispersal among FAW populations of Bt resistance alleles and their frequency. Genomic resources generated from FAW collections from pheromone traps by multiple states will be used to determine migratory movement of overwintering populations. This information will help resolve questions on gene flow between FAW populations, its role in spreading candidate Bt resistance alleles and facilitate the development of predictive models for the movement of resistant FAW.
D) Effects of Bt seed blend refuge on lepidopteran pest species.
The rate of cross-pollination between Bt and non-Bt refuge plants will be estimated within block and integrated refuges at NE and KS locations, using PCR-based transgene detection methods developed in IA. Quantitative ELISA will be used to estimate the level of Bt toxin expression in different corn tissues, including kernels of cross-pollinated refuge plants, and used to estimate potential exposures and impacts on larval development within refuges. Plant-insect interactions, including larval movement and low-dose exposure, may contribute to the accumulation of Bt resistance traits within pest populations. WBC move plant-to-plant and at different periods during larval stages. Studies will be conducted in NE to determine movement potential of WBC during instars that correspond to three larval movement periods in low and high density field scenarios. With documented resistance in H. zea to Cry toxins, there is increasing evidence that inhibition of cannibalistic behavior may allow more larvae to feed together and survive in a Bt ear. Studies (MD) on H. zea pupae extracted from soil beneath refuge and Bt plants will determine relative survival and fitness characteristics of adult moths.
E) Role of microbial associates on important pest traits.
The rate of microsporidia infection will be estimated among WBC populations, and any difference in Bt resistance measured between infected and non-infected cohorts.
F) Genomics tools for key corn pests, including genome and transcriptome sequences, genetic markers, and QTL maps of important traits.
The draft WCR genome assembly (GenBank accession PXJM00000000.2) will be annotated, and loaded into the i5K.nal.usda.gov database. Transcriptome resources will be developed for NCR and SCR. Mapping of Cry3Bb1 and Cry34/35Ab1 resistance in WCR pedigrees and populations will be completed. A new WCR BAClibrary will be screened for candidate Bt resistance genes. Fine mapping of a QTL for organophosphate resistance (Coates et al. 2016) will narrowed number of candidate genes in genome interval. RNAi and CRISPR gene editing will validate gene functions (Adedipe et al. 2019). ECB population genomic and pedigree studies will investigate roles of per and pdfr genes in voltinism (Kozak et al. 2019), refine interactions with pheromone strains (Coates et al. 2019), and identify genes involved in male pheromone response. Markers will be added to two ECB QTL previously linked to Cry1Ab resistance (Coates et al. 2013b ). Genetic markers will be integrated into ECB genetic maps for Cry1F resistance (Coates and Siegfried, 2015 ) and used to evaluate field resistance. Genetic markers will be developed to estimate gene flow, and track putative Bt resistance genes in the field populations of corn pests. Genome sequence and transcriptome assemblies will be generated from CEW and WBC, and other corn pests according to needs and resources. In CEW, genomic studies to better understand the genes involved in CEW resistance to Bt and the dominance of the traits are underway (MD), and will be used to develop markers of resistance as well as better inform IRM strategies. Genomes of FAW collected from multiple states are being sequenced at TN and used to determine population structure in FAW. These genomic resources include Bt-resistant FAW samples and will be used in genome wide association studies to identify genetic loci involved in resistance to Cry1F and Vip3Aa proteins. In TN, molecular and biochemical characterization of resistance to Cry1F and Vip3Aa in FAW will include sequencing of candidate resistance genes, transcriptome profiling and functional tests with identified alleles. Information from these studies will be included in efforts to develop targeted multiplexed next generation sequencing tools for high throughput monitoring for resistance to Cry1 and Vip3 proteins in FAW. These tools will also be expanded to CEW, SWCB and ECB, as appropriate.
Obj. 2. Develop and assess IPM systems for the arthropod complex in corn.
A) Coordinate early detection and mitigation networks for invasive species.
The Helicoverpa armigera early detection and mitigation network will serve as an example for coordinated multi-state invasive species monitoring. We will be well positioned to respond to new invasive species threats as they arise.
B) Relationships between pest management technologies with respect to the agricultural landscape and producer profitability.
Relationships between neonicotinoid seed treatments, target pests, and crop damage and yield are ongoing in IN and VA. The overall goal of the work is to assess when and where seed treatments provide the most value to producers across the Corn Belt. Outdoor tethered flight of monarchs on a modified flight mill system (IA) will be used to measure flight direction relative to wind direction, and distance to moveable habitat patches (potted flowers for nectaring and milkweed for oviposition). Together with colleagues’ telemetry research, these experiments will provide insight on habitat-perception distance of foraging monarchs to inform strategic placement of habitat patches in the agricultural landscape. Lab experiments with milkweed receiving treatments with neonicotinoid insecticide taken up from water (simulating worst-case runoff from seed treatments) or applied as a foliar spray (simulating worst-case drift) will determine whether ovipositing monarchs avoid treated plants. The results will provide information useful to regulators in determining minimum separation distances between monarch habitat patches and crops treated with neonicotinoids.
C) Need for and efficacy of insecticidal agents, including chemical insecticides, Bt toxins, and RNA interference (RNAi) molecules.
Multiple states conduct annual University and/or on-farm efficacy trials comparing controls with various insecticide treatments and Bt corn hybrids. Measuring of insect emergence, root injury, lodging, ear feeding damage, yield, etc. provide data that is locally relevant and available to producers. Research in TN aims at improving RNAi efficacy against FAW as a potential control alternative.
D) Effects of pest management interventions on non-target organisms.
IN, PA, and MD will lead U.S. efforts to evaluate the effects of seed treatment upon a range of beneficial insects, including pollinators, predators, and detritivores. Abundance and diversity studies of non-target insects using a combination of survey techniques will be conducted. Candidates for further study may be subjected to more detailed dose/response studies in laboratory settings to characterize susceptibility to various doses and routes of exposure, including some estimates of sublethal effects.
E) Biological control and bio-based insecticides, including conservation of natural enemies.
The potential for native entomopathogenic nematodes (EPNs) to persist across multiple growing seasons from a single inoculation will be studied in multiple regions of the Corn Belt and fringe areas (e.g., TX, NM, KS, NE, MI, PA,VT and NY). Field data will be collected to evaluate if sufficient populations of EPNs can provide CRW mortality independent of other management strategies, including GE events. In various locations with failing GE events, the potential of persistent EPNs to be deployed as a mitigation strategy will be investigated as a tool to extend the durability of all management strategies.
F) Does availability of management tools, including Bt traits and seed treatments, affects grower choice and IPM and IRM adoption.
By surveying seed dealer/cooperators across the region, we are developing a tool to compare and assess prices of Bt hybrids with respect to the incidence of the target pests. Currently, the entire corn belt is relatively uniform in adoption of Bt hybrids. But we know the pest distributions are not uniform. This project will seek to uncover where the mismatches occur, and whether farm profitability, IPM and IRM could both be better addressed with an adjustment to the approach.
Obj. 3. Characterize and monitor pest resistance and assess IRM and mitigation strategies.
A) Establish resistance monitoring networks for corn pests.
Collections from across the Corn Belt and diagnostic bioassays will be conducted annually to assess the susceptibility of target pest species (e.g. WCR, ECB, FAW, and WBC) using established methodology (Marçon et al. 1999, 2000; Siegfried et al. 2007; Dyer et al. 2013). Multiple US states and Canadian provinces will conduct field visits to identify suspected cases of Bt or other insecticide resistance. Fields will be visited in response to concerns by farmers and/or crop consultants. A gene check will be used to confirm the type of Bt corn planted in the field, feeding injury evaluated, and arthropods collected to generate populations for bioassays. For corn rootworm, single-plant bioassays, following Gassmann et al. (2014), along with other bioassay methods, such as Nowatzki et al. (2008), will be applied to measure the level of resistance to various Bt events. Continuation of a multistate network of sweet corn sentinel plots will monitor for further lepidopteran resistance development to the Bt toxins and determine whether regional control efficacy differences exist among the toxins expressed in Bt sweet corn. Since ‘practical resistance’ in CEW to Cry1Ab and Cry1A.105+Cry2Ab2 toxins has already been documented, a major focus of the monitoring network will be to detect changes in CEW susceptibility to the Vip3Aa toxin. The findings will also demonstrate the utility of the sentinel plot monitoring approach and enable improvements in the design of resistance monitoring for Bt crops.
B) Characterize the geographic range, demographics, and nature of arthropod pest resistance to insecticidal agents.
Inheritance experiments will involve reciprocal crosses of resistant and susceptible parental populations to establish an F1 generation and dominance determined based on the response curve of heterozygous individuals. Backcross of the F1 heterozygotes to one of the parental strains will be used to estimate the number of genetic factors that contribute to resistance. A variety of biochemical, molecular and genomic tools will be used to assess mechanisms of resistance (Coates et al. 2011, 2013a, 2016; Crespo et al. 2011; Wang et al. 2013; Jakka et al. 2016; Banerjee et al. 2017). IL will evaluate the effects of open (free-flying WCR adults from fields) and closed (adults emerging and mating within screenhouses enclosing pure-stand Bt plots) collection circumstances on Bt resistance in subsequent single plant Bt resistance bioassays. IL will use single plant Bt resistance bioassay methods to document Bt resistance in WCR populations from multiple statewide locations.
C) Identify genes, genome regions, and cellular and biochemical mechanisms involved in resistance to insecticidal agents.
QTL mapping in WCR pedigrees will be completed to identify genome regions linked to Cry3Bb1 and Cry34/35Ab1 resistance in WCR. The bacterial chromosome library (DvvBAC2) clones will be identified carrying candidate Bt resistance genes. A WCR genome region with a QTL for organophosphate resistance (Coates et al. 2016) will be saturated with additional markers and candidate genes predicted. Additional markers will be added to two independent ECB genome regions which were each previously shown to carry a QTL for Cry1Ab resistance (Coates et al. 2013a). Genetic markers will also be added to ECB genetic maps previously identifying a QTL for Cry1F resistance (Coates and Siegfried 2015). Differential transcript expression studies will be conducted between Bt resistant and susceptible ECB, or Bt exposed and unexposed WCR and WBC. MD will use genomic approaches to determine the number, location, effect sizes, and dominance of quantitative trait loci (QTL) associated with CEW resistance to Cry1Ab as well as Cry1A.105+Cry2Ab2 using diet bioassay phenotyped field-collected populations. In TN, protein binding assays with radiolabeled Cry1 and Vip3 proteins will be used to test for reduced binding in resistant populations. Candidate receptor genes will be sequenced and their levels of expression quantified to identify mutations and indels linked with resistance. DNA-based genotyping tests and targeted next generation sequencing tools will be developed based on the obtained information and used to examine the frequency of known and new candidate resistance alleles. Gene editing by CRISPR/Cas9 will be used as a functional tool to establish Cry1F resistance alleles in FAW and test their potential effects in cross-resistance to synthetic pesticides.
D) Develop region-specific bioeconomic models to assess strategies for managing and mitigating pest resistance to Bt corn.
Existing bioeconomic models (Hurley et al. 2001, 2002; Onstad et al. 2003; Mitchell and Onstad 2005; Saikai et al. 2019) will be extended to incorporate multiple insect targets with multiple modes of actions for each target and to examine both IRM and mitigation policies. Model extensions will include assessing the impacts of pyramiding and stacking of GE corn traits for both below-ground and above-ground target pests and more sophisticated modeling of human behavior using agent-based modeling, building on the work of Saikai et al. (2019) currently in progress. Key topics to examine will include mitigation policies to respond to the development of resistance (e.g., localized Bt seed bans, areawide spray applications, Bt seed taxes, increased refuge), and impacts of using homogeneous trait packages (stacked pyramids for lepidopteran and coleopteran pests) when pressure from the target pests varies substantially. MN and WI will lead model development, working with committee members to parameterize the yield loss and pest population dynamics models with regionally appropriate values.
E) Use data generated in the above efforts to develop and evaluate IPM and IRM strategies.
The results for the above models will include economic assessments to project the social surplus generated by the alternative IRM strategies for resistance management and mitigation. Following previous research (Hurley et al. 2001, 2002; Onstad et al. 2002; Mitchell and Onstad 2005; Saikai et al. 2019), these measures will largely be based on farmer returns, accounting for major cost categories related to insect management, including technology fees for seed and insecticide costs, as well as taxes or other revenue collected. The purpose of these social surplus metrics is to provide a measure for comparing and ranking alternative management and mitigation strategies. MN and WI will lead efforts in these analyses, building on the work in the previous sub-objective, 3D.
Obj. 4. Employ traditional and innovative delivery methods to disseminate information related to sustainable management of corn arthropod pests to farmers, industry, colleagues, agricultural professionals, and policy makers.
Traditional in-person and electronic extension meetings and educational programs will be held with producers, industry, consultants, and regulators to discuss findings and share information and ideas to enhance policy and regulatory decisions compatible with the economic, environmental, health and social needs of both the agricultural and non-agricultural communities. Newsletters, traditional extension materials, Web pages, Blogs, Ag Twitter and other social media, videos, interactive training modules, scientific publications, surveys and focus groups, and position statements will be used to disseminate information to the agricultural and public sectors.
Measurement of Progress and Results
Outputs
- 1) The assembled genome sequence of WCR and FAW, and reference transcriptomes of WCR, ECB, and WBC. Identification of genes involved in Bt toxin and insecticide resistance in WCR, ECB, CEW, FAW and WBC.
- 2) Annual assessments of pest susceptibility provided to regulatory agencies and to sponsoring industry organizations, as well as refereed publications that document changes in susceptibility and additional studies related to inheritance and identification of resistance mechanisms.
- 3) Improved artificial diet(s) that can be used for diet toxicity evaluations with all rootworm toxins.
- 4) Data and refereed publications documenting the ability of neonicotinoids to control secondary below-ground insect pests and an increased understanding of the effects of these compounds beyond the planted field, as well as clarification of whether seed-treatment neonics are of universal value in corn.
- 5) Data and refereed publications on the movement and kernel feeding of key lepidopteran pests in seed blend refuges.
Outcomes or Projected Impacts
- 1) Corn producers and other stakeholders (e.g. regulatory bodies) will have the necessary information to make informed decisions on topics such as seed blend refuge strategy and neonicotinoid seed treatment use and regulation.
- 2) Improved IPM recommendations that increase input efficiency and reduce non-target effects, resulting in more resilient corn production. Improved IRM policies that reduce the risk of arthropod pest resistance and result in improved product and technology stewardship.
- 3) Resistance monitoring and characterization of resistance in field populations of corn pests will provide critical information to federal agencies that regulate the use of this technology and help ensure that the technology is used in a sustainable manner.
- 4) Advances in our understanding of pest ecology, behavior, movement, rearing, and genetics will be used to improve research approaches and models, increasing our capacity to respond to management issues.
Milestones
(2020):Initiation of new NC246 project. Coordination of research teams and initiation of research projects for Objectives 1, 2, and 3.(2021):Data gathering, field work, bioassay completion, methods troubleshooting, and project expansion for Objectives 1, 2, and 3. Sharing of data and results from these efforts will be completed for Objective 4. Initial outputs of data, publications, and presentations will begin.
(2022):Ongoing data gathering, field work, bioassay completion, methods troubleshooting, and project expansion for Objectives 1, 2, and 3. Sharing of data and results from these efforts will be completed for Objective 4. Outputs of data, publications, and presentations will continue. New projects in response to previous results may be developed.
(2023):Ongoing data gathering, field work, bioassay completion, methods troubleshooting, and project expansion for Objectives 1, 2, and 3. Sharing of data and results from these efforts will be completed for Objective 4. Outputs of data, publications, and presentations will continue. New projects in response to previous results may be developed.
(2024):Ongoing data gathering, field work, bioassay completion, methods troubleshooting, and project expansion for Objectives 1, 2, and 3. Sharing of data and results from these efforts will be completed for Objective 4. Outputs of data, publications, and presentations will continue. New projects in response to previous results may be developed. Evaluation of project completion and future directions in preparation for resubmission of multi-state project proposal for the next 5 years.
Projected Participation
View Appendix E: ParticipationOutreach Plan
Members of NC246 are involved in a wide variety of outreach efforts related to the sustainable management of corn arthropod pests. They recommend IPM-based approaches that exploit multiple strategies for suppressing pests to avoid growers' reliance on any one practice or tactic, and associated rapid selection for pest resistance. Many committee members interact regularly with farmers, media, government agencies, state commodity boards, and other Ag professionals regarding IPM, IRM, and pest biology. In addition to helping farmers and the public understand IRM programs, committee members with extension appointments will provide guidance about how transgenic crops and IRM programs fit into a larger field corn IPM program. Members provide growers with specific, science-based guidance to make informed decisions about the management of corn insect pests.
Traditional in-person and electronic extension education will be held for producers, industry, consultants, and regulators to discuss findings and share information and ideas to enhance policy and regulatory decisions compatible with the economic, environmental, health and social needs of both the agricultural and non-agricultural communities. Feedback from growers will be used to help direct research activities of the committee. Newsletters, traditional extension materials, Web pages, Blogs, Ag Twitter and other social media, videos, interactive training modules, scientific publications, surveys and focus groups, and position statements will be used to disseminate information to the agricultural and public sectors. These efforts are encompassed in Objective 4. NC246 activities will also focus on expanding the land-grant university research and Extension presence and leadership roles in the traditional and electronic media arenas to provide unbiased science-based IPM and IRM decision support to clientele.
Organization/Governance
The project will operate following the “Guidelines for Multistate Research Activities” document developed by the State Agricultural Experiment Station Directors in cooperation with USDA NIFA and ESCOP, available on the NIMSS website.
The project will be administered by a Technical Committee consisting of all Appendix E participants of the project; all are eligible for office, regardless of sponsoring agency affiliation or the number of members per SAES. An Executive Committee will consist of the Past Chair, Chair, Vice Chair, and Secretary (each serving 1-year terms), as well as the Administrative Advisor. The Executive Committee will conduct business during and between annual meetings (e.g. annual reports, NIMSS entries, project rewrites). Other important standing committees include Local Arrangements and Nominating Committee, the membership of which will vary from year to year.
An annual meeting of the full Technical Committee will be held to summarize and critically evaluate progress, analyze results, and plan future activities, reports, and publications. The Executive Committee, in consultation with Local Arrangements, the Technical Committee, and with the concurrence of the Administrative Advisor, notifies the Technical Committee members of the time and place of meetings, prepares the agenda, and presides at meetings. The Administrative Advisor authorizes the annual meeting 90-120 days in advance. The Executive Committee, in coordination with Local Arrangements, will be responsible for organizing, setting the agenda, and presiding over an annual Open Meeting with Industry, EPA, and other invited guests, which will be scheduled as part of the regular annual Technical Committee meeting.
An incoming Secretary will be nominated by the Nominating Committee and voted upon by the full Technical Committee at the annual meeting. The Secretary will serve a 1-year term culminating at the close of the annual meeting. The Secretary will rotate into the Vice Chair position after the first year, then Chair, and finally Past Chair. Therefore, election as Secretary implies a 4-year commitment as an Executive Committee officer, although alterations to this pattern can be addressed as needed.
Literature Cited
Abdelgaffar H, Tague ED, Gonzalez HFC, Campagna SR, Jurat-Fuentes JL. 2019. Midgut metabolomic profiling of fall armyworm (Spodoptera frugiperda) with field-evolved resistance to Cry1F corn. Insect Biochem. Mol. Biol. 106: 1-9.
Adedipe F, Grubbs N, Coates BS, Wiegmann B, Lorenzen M. 2019. Structural and functional insights into the Diabrotica virgifera virgifera ATP-binding cassette (ABC) transporters gene family. BMC Genomics 20: 899.
Alford A, Krupke CH. 2017. Correction: Translocation of the neonicotinoid seed treatment clothianidin in maize. PLOS ONE 12(10): e0186527. https://doi.org/10.1371/journal.pone.0186527
Andow DA, Pueppke SG, Schaafsma AW, Gassmann AJ, Sappington TW, Meinke LJ, Mitchell PD, Hurley TM, Hellmich RL, Porter RP. 2016. Early detection and mitigation of resistance to Bt maize by western corn rootworm (Coleoptera: Chrysomelidae). J. Econ. Entomol. 109: 1-12.
Archibald WR, Bradshaw JD, Golick DA, Wright RJ, Peterson JA. 2017. Nebraska growers’ and crop consultants’ knowledge and implementation of Integrated Pest Management of western bean cutworm. Journal of Integrated Pest Management 9: 1-7.
Ball HJ, Weekman, GT. 1962. Insecticide resistance in the adult western corn rootworm in Nebraska. J. Econ. Entomol. 55: 439-441.
Ball HJ, Weekman GT. 1963. Differential resistance of corn rootworms to insecticides in Nebraska and adjoining states. J. Econ. Entomol. 56: 553-555.
Banerjee R, Hasler J, Meagher R, Nagoshi R, Hietala L, Huang F, Narva K, Jurat-Fuentes JL. 2017. Mechanism and DNA-based detection of field-evolved resistance to transgenic Bt corn in fall armyworm (Spodoptera frugiperda). Scientific Reports 7: 10877.
Berheim EH, Jenks JA, Lundgren JG, Michel ES, Grove D, Jensen WF. 2019. Effects of neonicotinoid insecticides on physiology and reproductive characteristics of captive female and fawn white-tailed deer. Scientific Reports 9: 4534.
Bilbo TR, Reay-Jones FPF, Reisig DD, Green JK. 2019. Susceptibility of corn earworm (Lepidoptera: Noctuidae) to Cry1A.105 and Cry2Ab2 in North and South Carolina. J. Econ. Entomol. 112: 1845-1857.
Buntin GD, All JN, Lee RD, Wilson DM. 2004. Plant-incorporated Bacillus thuringiensis resistance for control of fall armyworm and corn earworm (Lepidoptera: Noctuidae) in corn. J. Econ. Entomol. 97: 1603-1611.
Burkness EC, O’Rourke PK, Hutchison WD. 2011. Cross-pollination of nontransgenic corn ears with transgenic Bt corn: Efficacy against lepidopteran pests and implications for resistance management. J. Econ. Entomol. 104: 1476-1479.
Burkness EC, Hutchison WD. 2012. Bt pollen dispersal and Bt kernel mosaics: Integrity of non-Bt refugia for lepidopteran resistance management in maize. J. Econ. Entomol. 105: 1477-1487.
Burkness EC, Cira TM, Moser SE, Hutchison WD. 2015. Bt maize seed mixtures for Helicoverpa zea (Lepidoptera: Noctuidae): Larval movement, development, and survival on non-transgenic maize. J. Econ. Entomol. 108: 2761-2769.
Calles-Torrez V, Knodel JJ, Boetel MA, French BW, Fuller BW, Ransom JK. 2019. Field-evolved resistance of northern and western corn rootworm (Coleoptera: Chrysomelidae) populations to corn hybrids expressing single and pyramided Cry3Bb1 and Cry34/35Ab1 Bt proteins in North Dakota. J. Econ. Entomol. 112: 1875-1886.
Chilcutt CF, Tabashnik BE. 2004. Contamination of refuges by Bacillus thuringiensis toxin genes from transgenic maize. Proc. Natl. Acad. Sci. U.S.A. 101: 7526-7529.
Chu CC, Zavala JA, Spencer JL, Curzi MJ, Fields CJ, Drnevich J, Siegfried BD, Seufferheld MJ. 2015. Patterns of differential gene expression in adult rotation-resistant and wild-type western corn rootworm digestive tracts. Evol. Appl. 8: 692-704.
Clark TL, Frank DL, French BW, Meinke LJ, Moellenbeck D, Vaughn TT, Hibbard BE. 2012. Mortality impact of MON863 transgenic maize roots on western corn rootworm larvae in the field. J. Appl. Entomol. 136: 721-729.
Coates BS. 2016. Bacillus thuringiensis toxin resistance mechanisms among Lepidoptera: Progress on genomic approaches to uncover causal mutations in the European corn borer, Ostrinia nubilalis. CurR. Opin. Insect Sci. 15: 70-77.
Coates BS, Siegfried BD. 2015. Linkage of an ABC transporter to a single QTL that controls Ostrinia nubilalis larval resistance to the Bacillus thuringiensis Cry1Fa toxin. Insect Biochem. Molec. 63: 86-96.
Coates BS, Sumerford DV, Miller NJ, Kim KS, Sappington TW, Siegfried BD, Lewis LC. 2009. Comparative performance of single nucleotide polymorphism and microsatellite markers for population genetic analysis. J. Hered. 100: 556-564.
Coates BS, Sumerford DV, Lopez MD, Wang H, Fraser LM, Kroemer JA, Spencer T, Kim KS, Abel CA, Hellmich RL, Siegfried BD. 2011. A single major QTL controls expression of larval Cry1F resistance trait in Ostrinia nubilalis (Lepidoptera: Crambidae) and is independent of midgut receptor genes. Genetica 139: 961-972.
Coates BS, Alves AP, Wang H, Walden KKO, French BW, Miller NJ, Abel CA, Robertson HM, Sappington TW, Siegfried BD. 2012. Distribution of genes and repetitive elements in the Diabrotica virgifera virgifera genome estimated using BAC sequencing. J. Biomed. Biotechnol. 2012: Article ID 604076, 9pp.
Coates BS, Sumerford DV, Siegfried BD, Hellmich RL, Abel CA. 2013a. Unlinked genetic loci control the reduced transcription of aminopeptidase N1 and 3 in the European corn borer and determine tolerance to Bacillus thuringiensis Cry1Ab toxin. Insect Biochem. Mol. Biol. 43: 1152-1160.
Coates BS, Johnson H, Kim KS, Hellmich RL, Abel CA, Mason C, Sappington TW. 2013b. Frequency of hybridization between Ostrinia nubilalis E- and Z-pheromone races in regions of sympatry within the United States. Ecol. Evol. 3: 2459-2470.
Coates BS, Fraser LM, French BW, Sappington TW. 2014. Proliferation and copy number variation of BEL-like long terminal repeat retrotransposons within the Diabrotica virgifera virgifera genome. Gene. 534: 362-370.
Coates BS, Alves A, Wang H, Zhou X, Nowatski T, Chen H, Rangasamy M, Robertson HM, Whitfield CW, Walden KK, Kachman SD, French BW, Meinke LJ, Hawethorne D, Abel CA, Sappington TW, Siegfried BD, Miller NJ. 2016. Genetic mapping and functional genomics link a carboxyesterase with organophosphate resistance in the western corn rootworm, Diabrotica virgifera virgifera. Insect Mol. Biol. 25:1-15.
Coates BS, Dopman EB, Wanner KW, Sappington TW. 2018. Genomic mechanisms of sympatric ecological and sexual divergence in a model agricultural pest, the European corn borer. Curr. Opin. Insect Sci. 26: 50-56.
Coates BS, Kozak GM, Seok Kim K, Sun J, Wang Y, Fleischer SJ, Dopman EB, Sappington TW. 2019. Influence of host plant, geography and pheromone strain on genomic differentiation in sympatric populations of Ostrinia nubilalis. Mol. Ecol. 28: 4439-4452.
Coons R. 2009. U.S., Canadian regulators OK SmartStax, reduce refuge requirements, Chemical Week. pp. 1.
Crespo ALB, Spencer T, Tan SY, Siegfried BD. 2010. Fitness costs of Cry1Ab resistance in a field-derived strain of Ostrinia nubilalis (Lepidoptera: Crambidae). J. Econ. Entomol. 103: 1386-1393.
Crespo ALB, Rodrigo-Simón A, Siqueira HAA, Pereira EJG, Ferre J, Siegfried BD. 2011. Cross-resistance and mechanism of resistance to Cry1Ab toxin from Bacillus thuringiensis in a field-derived strain of European corn borer, Ostrinia nubilalis. J. Invert. Pathol. 107: 185-192.
Dively GP, Venugopal D, Finkenbinder C. 2016. Field-evolved resistance in corn earworm to Cry proteins expressed by transgenic sweet corn. PLoS ONE 11(12): e0169115. doi:10.1371/journal.pone.0169115.
Dively GP, Huang F, Oyediran I, Burd T, Morsello S. 2019. Evaluation of gene flow in structured and seed blend refuge systems of non-Bt and Bt corn. J. Pest Sci. https://doi.org/10.1007/s10340-019-01126-4.
Dopman EB, Perez L, Bogdanowicz SM, Harrison RG. 2005. Consequences of reproductive barriers for genealogical discordance in the European corn borer. Proc. Natl. Acad. Sci. USA. 102: 14706-14711.
Dopman EB, Robbins PS, Seaman A. 2010. Components of reproductive isolation between North American pheromone strains of the European corn borer. Evolution 64:881-902.
Dorhout DL, Sappington TW, Rice ME. 2008. Evidence for obligate migratory flight behavior in young European corn borer (Lepidoptera: Crambidae) females. Environ. Entomol. 37: 1280-1290.
Douglas MR, Tooker JF. 2015. Large-scale deployment of seed treatments has driven rapid increase in use of neonicotinoid insecticides and preemptive pest management in US field crops. Environ. Sci. Technol. 49: 5088-5097.
Dunbar MW, Gassmann AJ. 2013. Abundance and distribution of western and northern corn rootworm (Diabrotica spp.) and prevalence of rotation resistance in Eastern Iowa. J. Econ. Entomol. 106: 168-180.
Dyer JM, Sappington TW, Coates BS. 2013. Evaluation of tolerance to Bacillus thuringiensis toxins among laboratory-reared western bean cutworm (Lepidoptera: Noctuidae). J. Econ. Entomol. 106: 2467-2472.
Eng ML, Stutchbury BJ, Morrissey CA. 2019. A neonicotinoid insecticide reduces fueling and delays migration in songbirds. Science 365: 1177-1180.
Flagel LE, Bansal R, Kerstetter RA, et al. 2014. Western corn rootworm (Diabrotica virgifera virgifera) transcriptome assembly and genomic analysis of population structure. BMC Genomics 15: 195.
Flagel LE, Swarup S, Chen M, Bauer C, Wanjugi H, Carroll M, Hill P, Tuscan M, Bansal R, Flannagan R, Clark TL. 2015. Genetic markers for western corn rootworm resistance to Bt toxin. G3: Genes, Genomes, Genetics 5: 399-405.
Frank DL, Kurtz R, Tinsley NA, Gassmann AJ, Meinke LJ, Moellenbeck D, Gray ME, Bledsoe LW, Krupke CH, Estes RE, Weber P, Hibbard BE. 2015. Effect of seed blends and soil-insecticide on western and northern corn rootworm emergence from mCry3A+eCry3.1Ab Bt maize. J. Econ. Entomol. 108: 1260-1270.
Gassmann AJ, Petzold-Maxwell JL, Keweshan RS, Dunbar MW. 2011. Field-evolved resistance to Bt maize by western corn rootworm. PLoS ONE 6(7): e22629.
Gassmann AJ, Petzold-Maxwell JL, Keweshan RS, Dunbar MW. 2012. Western corn rootworm and Bt maize: Challenges of pest resistance in the field. GM Crops and Food 3: 235-244.
Gassmann AJ, Petzold-Maxwell JL, Clifton EH, Dunbar MW, Hoffmann AM, Ingber DA, Keweshan RS. 2014. Field-evolved resistance by western corn rootworm to multiple Bacillus thuringiensis toxins in transgenic maize. Proc. Natl. Acad. Sci. USA 111: 5141-5146.
Gassmann AJ, Shrestha RB, Jakka SR, Dunbar MW, Clifton EH, Paolino AR, Ingber DA, French BW, Masloski KE, Dounda JW, St. Clair CR. 2016. Evidence of resistance to Cry34/35Ab1 corn by western corn rootworm (Coleoptera: Chrysomelidae): root injury in the field and larval survival in plant-based bioassays. J. Econ. Entomol. 109: 1872-1880.
Goldstein JA, Mason CE, Pesek J. 2010. Dispersal and movement behavior of neonate European corn borer (Lepidoptera: Crambidae) on non-Bt and transgenic Bt corn. J. Econ. Entomol. 103: 331-339.
Gould F, Cohen MB, Bentur JS, Kennedy GG, Van Duyn J. 2006. Impact of small fitness costs on pest adaptation to crop varieties with multiple toxins: A heuristic model. J. Econ. Entomol. 99: 2091-2099.
Goulson D. 2013. An overview of the environmental risks posed by neonicotinoid insecticides. J. Appl. Ecol. 50: 977-987.
Gray ME. 2011. Relevance of traditional Integrated Pest Management (IPM) strategies for commercial corn producers in a transgenic agroecosystem: A bygone era? J. Agr. Food Chem. 59: 5852-5858.
Gray ME, Sappington TW, Miller NJ, Moeser J, Bohn MO. 2009. Adaptation and invasiveness of western corn rootworm: Intensifying research on a worsening pest. Annu. Rev. Entomol. 54: 303-321.
Head GP, Carroll M, Evans S, Rule DM, Willse A, Clark T, Storer N, Flannagan R, Samuel L, Meinke LJ. 2017. Evaluation of SmartStax and SmartStax PRO maize against western corn rootworm and northern corn rootworm: Efficacy and resistance management. Pest Manag. Sci. 73: 1883-1899.
Hibbard BE, Frank DL, Kurtz R, Boudreau E, Ellersieck MR, Odhiambo JF. 2011. Mortality impact of Bt transgenic maize roots expressing eCry3.1Ab, mCry3A, and eCry3.1Ab plus mCry3A on western corn rootworm larvae in the field. J. Econ. Entomol. 104: 1584-1591.
Higley LG, Boethel DJ. 1994. Handbook of Soybean Insect Pests. Entomological Society of America, 136 pp.
Hitchon AJ, Smith JL, French BW, Schaafsma AW. 2015. Impact of the Bt corn proteins Cry34/35Ab1 and Cry3Bb1 alone or pyramided on western corn rootworm (Coleoptera: Chrysomelidae) beetle emergence in the field. J. Econ. Entomol. 108: 1986-1993.
Hoffmann AM, French BW, Hellmich RL, Lauter N, Gassmann AJ. 2015. Fitness costs of resistance to Cry3Bb1 maize by western corn rootworm. J. Appl. Entomol. 139: 403-415.
Huang F, Qureshi JA, Meagher RL, Reisig DD, Head GP, Andow DA, Ni X, Kerns D, Buntin GD, Niu Y, Yang F, Dangal V. 2014. Cry1F resistance in fall armyworm Spodoptera frugiperda: Single gene verus pyramided Bt maize. PloS ONE 9(11): e112958.
Hughson SA, Spencer JL. 2015. Emergence and abundance of western corn rootworm (Coleoptera: Chrysomelidae) in Bt cornfields with structured and seed blend refuges. J. Econ. Entomol. 108: 114-125.
Hurley TM, Babcock BA, Hellmich RL. 2001. Bt corn and insect resistance: An economic assessment of refuges. J. Agr. Resour. Econ. 26: 176-194.
Hurley TM, Secchi S, Babcock BA, Hellmich RL. 2002. Managing the risk of European corn borer resistance to Bt corn. Environ. Resour. Econ. 22: 537-558.
Huynh MP, Meihls LN, Hibbard BE, Lapointe SL, Niedz RP, Ludwick DC, Coudron TA. 2017. Diet improvement for western corn rootworm (Coleoptera: Chrysomelidae) larvae. PLoS ONE 12(11): e0187997.
Huynh MP, Bernklau EJ, Coudron TA, Shelby KS, Bjostad LB, Hibbard BE. 2019a. Characterization of corn root factors to improve artificial diet for western corn rootworm (Coleoptera: Chrysomelidae) larvae. J. Insect Sci. 19(2): 20.
Huynh MP, Hibbard BE, Vella ME, Lapointe SL, Niedz RP, Shelby KS, Coudron TA. 2019b. Development of an improved and accessible diet for western corn rootworm larvae using response surface modeling. Sci. Rep. 9: 16009.
Huynh MP, Hibbard BE, Lapointe SL, Niedz RP, French BW, Pereira AE, Finke DL, Shelby KS, Coudron TA. 2019c. Multidimensional approach to formulating a specialized diet for northern corn rootworm larvae. Sci. Rep. 9: 3709.
Ingber DA, Gassmann AJ. 2015. Inheritance and fitness costs of resistance to Cry3Bb1 corn by western corn rootworm (Coleoptera: Chrysomelidae). J. Econ. Entomol. 108: 2421-2432.
Jakka SR, Knight VR, Jurat-Fuentes JL. 2014. Fitness costs associated with field-evolved resistance to Bt maize in Spodoptera frugiperda (Lepidoptera: Noctuidae). J. Econ. Entomol. 107: 342-351.
Jakka SR, Shrestha RB, Gassmann AJ. 2016. Broad-spectrum resistance to Bacillus thuringiensis toxins by western corn rootworm (Diabrotica virgifera virgifera). Sci. Rep. 6: 27860.
Jiang XF, Chen J, Zhang L, Sappington TW, Luo LZ. 2013. Increased long-flight activity triggered in beet armyworm by larval feeding on diet containing Cry1Ac protoxin. PLoS One 8(5): e63554.
Kang J, Onstad DW, Hellmich RL, Moser SE, Hutchison WD. 2012. Modeling the impact of cross-pollination and low toxin expression in corn kernels on adaptation of European corn borer (Lepidoptera: Crambidae) to transgenic insecticidal corn. Environ. Entomol. 41: 200-211.
Keweshan RS, Head GP, Gassmann AJ. 2015. Effects of pyramided Bt corn and blended refuges on western corn rootworm and northern corn rootworm (Coleoptera: Chrysomelidae). J. Econ. Entomol. 108: 720-729.
Kim KS, Sappington TW. 2005. Genetic structuring of western corn rootworm (Coleoptera: Chrysomelidae) populations in the U.S. based on microsatellite loci analysis. Environ. Entomol. 34: 494-503.
Knolhoff LM, Walden KKO, Ratcliffe ST, Onstad DW, Robertson HM. 2010. Microarray analysis yields candidate markers for rotation resistance in the western corn rootworm beetle, Diabrotica virgifera virgifera. Evol. Appl. 3: 17-27.
Koutroumpa FA, Groot AT, Dekker T, Heckel DG. 2016. Genetic mapping of male pheromone response in the European corn borer identifies candidate genes regulating neurogenesis. Proc. Natl. Acad. Sci. USA 113 : E6401-E6408.
Kozak GM, Wadsworth CB, Kahne SC, Bogdanowicz SM, Harrison RG, Coates BS, Dopman EB. 2017. A combination of sexual and ecological divergence contributes to rearrangement spread during initial stages of speciation. Mol. Ecol. 26: 2331-2347.
Kozak GM, Wadsworth CB, Kahne SC, Bogdanowicz SM, Harrison RG, Coates BS, Dopman EB. 2019. Genomic basis of circannual rhythm in the European corn borer. Curr. Biol. 29(20): 3501-3509.e5.
Krafsur ES. 1995. Gene flow between univoltine and semivoltine northern corn rootworm (Coleoptera: Chrysomelidae) populations. Ann. Entomol. Soc. Am. 88: 699-704.
Krupke CH, Hunt GH, Eitzer BD, Andino G, Given K. 2012. Multiple routes of pesticide exposure for honey bees living near agricultural fields. PLoS ONE 7(1): e29268. doi:10:1371/journal.pone.0029268.
Krupke CH, Holland J, Long E, Eitzer B. 2017. Planting of neonicotinoid treated maize poses risks for honey bees and other non-target organisms over a wide area without consistent yield benefit. J. Appl. Ecol. 54: 1449-1454. https://doi.org/10.1111/1365-2664.12924
Laurent FM, Rathahao E. 2003. Distribution of [(14)C]imidacloprid in sunflowers (Helianthus annuus L.) following seed treatment. J. Agric. Food Chem. 51: 8005-8010.
Levy RC, Kozak GM, Wadsworth CB, Coates BS, Dopman EB. 2015. Explaining the sawtooth: Latitudinal periodicity in a circadian gene correlates with shifts in generation number. J. Evol. Biol. 28: 40-53.
Li G, Reisig D, Miao J, Gould F, Huang F, Feng H. 2016. Frequency of Cry1F non-recessive resistance alleles in North Carolina field populations of Spodoptera frugiperda (Lepidoptera: Noctuidae). PloS ONE 11(4): e0154492.
Lombaert E, Ciosi M, Miller NJ, Sappington TW, Blin A, Guillemaud T. 2018. Colonization history of the western corn rootworm (Diabrotica virgifera virgifera) in North America: Insights from random forest ABC using microsatellite data. Biol. Invasions 20: 665-677.
Ludwick DC, Meihls LN, Ostlie KR, Potter BD, French L, Hibbard BE. 2017. Minnesota field population of western corn rootworm (Coleoptera: Chrysomelidae) shows incomplete resistance to Cry34Ab1/Cry35Ab1 and Cry3Bb1. J. Appl. Entomol. 141: 28-40.
Ludwick DC, Meihls LN, Huynh MP, Pereira AE, French BW, Coudron TA, Hibbard BE. 2018. A new artificial diet for western corn rootworm larvae is compatible with and detects resistance to all current Bt toxins. Sci. Rep. 8: 5379.
Main AR, Headley JV, Peru KM, Michel NL, Cessna AJ, et al. 2014. Widespread use and frequent detection of neonicotinoid insecticides in wetlands of Canada’s Prairie Pothole Region. PLoS ONE 9(3): e92821. doi:10.1371/journal.pone.0092821.
Mallet J, Porter P. 1992. Preventing insect adaptation to insect-resistant crops—Are seed mixtures or refugia the best strategy. Proc. R. Soc. B Biol. Sci. 250: 165-169.
Marçon PCRG, Young LJ, Steffey KL, Siegfried BD. 1999. Baseline susceptibility of European corn borer (Lepidoptera: Crambidae) to Bacillus thuringiensis toxins. J. Econ. Entomol. 92: 279-285.
Marçon, PCRG, Siegfried BD, Spencer T, Hutchison WD. 2000. Development of diagnostic concentrations for monitoring Bacillus thuringiensis resistance in European corn borer (Lepidoptera: Crambidae). J. Econ. Entomol. 93: 925-930.
Mason CE, Rice ME, DiFonzo CD, Porter RP, et al. 2018. European Corn Borer Ecology and Management, and Association with Other Corn Pests. NCR 0327. Ames: Iowa State University Extension and Outreach.
Meihls LN, Higdon ML, Ellersieck MR, Hibbard BE. 2012. Greenhouse-selected resistance to Cry3Bb1-producing corn in three western corn rootworm populations. PLoS ONE 7: e51055.
Meihls LN, Huynh MP, Ludwick DC, Coudron TA, French BW, Shelby KS, Hitchon AJ, Smith JL, Schaafsma AW, Pereira AE, Hibbard BE. 2018. Comparison of six artificial diets for western corn rootworm bioassays and rearing. J. Econ. Entomol. 111(6): 2727-2733.
Meinke LJ, Siegfried BD, Wright RJ, Chandler LD. 1998. Adult susceptibility of western corn rootworm (Coleoptera: Chrysomelidae) populations to selected insecticides. J. Econ. Entomol. 91: 594-600.
Miller NJ, Sappington TW. 2017. Role of dispersal in resistance evolution and spread. Curr. Opin. Insect Sci. 21: 68-74.
Miller NJ, Kim KS, Ratcliffe ST, Estoup A, Bourguet D, Guillemaud T. 2006. Absence of genetic divergence between western corn rootworms (Coleoptera: Chrysomelidae) resistant and susceptible to control by crop rotation. J. Econ. Entomol. 99: 685-690.
Mitchell PD, Onstad DW. 2005. Effect of extended diapause on the evolution of resistance to transgenic Bacillus thuringiensis corn by northern corn rootworm (Coleoptera: Chrysomelidae). J. Econ. Entomol. 98: 2220-2234.
Mullin CA, Saunders II MC, Leslie TW, Biddinger DJ, Fleischer SJ. 2005. Toxic and behavioral effects to Carabidae of seed treatments used on Cry3Bb1- and Cry1Ab/c-protected corn. Environ. Entomol. 34: 1626-1636.
Murphy AF, Ginzel MD, Krupke CH. 2010. Evaluating western corn rootworm (Coleoptera: Chrysomelidae) emergence and root damage in a seed mix refuge. J. Econ. Entomol. 103: 147-157.
Nagoshi RN, Rosas-García NM, Meagher RL, Fleischer SJ, Westbrook JK, Sappington TW, Hay-Roe M, Thomas JMG, Murúa GM. 2015. Haplotype profile comparisons between Spodoptera frugiperda (Lepidoptera: Noctuidae) populations from Mexico with those from Puerto Rico, South America, and the United States and their implications to migratory behavior. J. Econ. Entomol. 108: 135-144.
Nagoshi RN, Fleischer S, Meagher RL, Hay-Roe M, Khan A, Murúa MG, Silvie P, Vergara C, Westbrook J. 2017. Fall armyworm migration across the Lesser Antilles and the potential for genetic exchanges between North and South American populations. PLoS One 12(2): e0171743.
Nowatzki TM, Lefko SA, Binning RR, Thompson SD, Spencer TA, Siegfried BD. 2008. Validation of a novel resistance monitoring technique for corn rootworm (Coleoptera: Chrysomelidae) and event DAS-59122-7 maize. J. Appl. Entomol. 132: 177-188.
Onstad DW, Crowder DW, Mitchell PD, Guse CA, Spencer JL, Levine E, Gray ME. 2003. Economics versus alleles: Balancing IPM and IRM for rotation-resistant western corn rootworm (Coleoptera: Chrysomelidae). J. Econ. Entomol. 96: 1872-1885.
Onstad DW, Mitchell PD, Hurley TM, Lundgren JG, Porter RP, Krupke CH, Spencer JL, DiFonzo CD, Baute TS, Hellmich RL, et al. 2011. Seeds of change: Corn seed mixtures for resistance management and IPM. J. Econ. Entomol. 104: 343-352.
Oswald KJ, French BW, Nielson C, Bagley M. 2012. Assessment of fitness costs in Cry3Bb1-resistant and susceptible western corn rootworm (Coleoptera: Chrysomelidae) laboratory colonies. J. Appl. Entomol. 136: 730-740.
Oyediran I, Dively G, Huang F, Burd T. 2016. Evaluation of European corn borer Ostrinia nubilalis (Lepidoptera: Crambidae) larval movement and survival in structured and seed blend refuge plantings. Crop Prot. 81: 145-153.
Papiernik SK, Sappington TW, Luttrell RG, Hesler LS, Allen K. 2018. Overview: Risk factors and historic levels of pressure from insect pests of seedling corn, cotton, soybean, and wheat in the United States, Journal of Integrated Pest Management 9: 18. https://doi.org/10.1093/jipm/pmx026
Paula-Moraes SV, Burkness EC, Hunt TE, Wright RJ, Hein GL, Hutchison WD. 2011. Cost-effective binomial sequential sampling of western bean cutworm, Striacosta albicosta (Lepidoptera: Noctuidae), egg masses in corn. J. Econ. Entomol. 104: 1900-1908.
Paula-Moraes SV, Hunt TE, Wright RJ, Hein GL, Blankenship EE. 2012. On-plant movement and feeding of western bean cutworm (Lepidoptera: Noctuidae) early instars on corn. Environ. Entomol. 41: 1494-1500.
Paula-Moraes SV, Hunt TE, Wright RJ, Hein GL, Blankenship EE. 2013. Western bean cutworm survival and the development of economic injury levels and economic thresholds in field corn. J. Econ. Entomol. 106: 1274-1285.
Pedigo LP, Rice ME. 2008. Entomology and Pest Management. 6th ed. Waveland Press, Inc. 816 pp.
Pereira EJG, Storer NP, Siegfried BD. 2011. Fitness costs of Cry1F resistance in laboratory-selected European corn borer (Lepidoptera: Crambidae). J. Appl. Entomol. 135: 1-6.
Pereira AE, Wang H, Zukoff SN, Meinke LJ, French BW, Siegfried BD. 2015. Evidence of field-evolved resistance to bifenthrin in western corn rootworm (Diabrotica virgifera virgifera LeConte) populations in western Nebraska and Kansas. PLoS One 10(11): e0142299.
Pereira AE, Souza DS, Zukoff SN, Meinke LJ, Siegfried BD. 2017. Cross-resistance and synergism assays provide evidence for multiple mechanisms of resistance to pyrethroids in western corn rootworm populations. PLoS ONE 12(6): e0179311.
Petzold-Maxwell J, Siegfried BD, Hellmich RL, Abel CA, Coates BS, Spencer TA, Gassmann AJ. 2014. Effect of maize lines on fitness costs of Cry1F resistance in the European corn borer. J. Econ. Entomol. 107: 764-772.
Pleau MJ, Huesing JE, Head GP, Feir DJ. 2002. Development of an artificial diet for the western corn rootworm. Entomol. Exp. Appl. 105: 1-11.
Rault LC, Siegfried BD, Gassmann AJ, Wang H, Brewer GJ, Miller NJ. 2018. Investigation of Cry3Bb1 resistance and intoxication in western corn rootworm by RNA sequencing. J. Appl. Entomol. 142: 921-936.
Razze JM, Mason CE. 2012. Dispersal behavior of neonate European corn borer (Lepidoptera: Crambidae) on Bt corn. J. Econ. Entomol. 105: 1214-1223.
Razze JM, Mason CE, Pizzolato TD. 2011. Feeding behavior of neonate Ostrinia nubilalis (Lepidoptera: Crambidae) on Cry1Ab Bt corn: Implications for resistance management. J. Econ. Entomol. 104: 806-813.
Reardon BJ, Sumerford DV, Sappington TW. 2006. Dispersal of newly-eclosed European corn borer adults (Lepidoptera: Crambidae) from corn into small-grain aggregation plots. J. Econ. Entomol. 99: 1641-1650.
Reinders JD, Hitt BD, Stroup WW, French BW, Meinke LJ. 2018. Spatial variation in western corn rootworm (Coleoptera: Chrysomelidae) susceptibility to Cry3 toxins in Nebraska. PLoS ONE 13(11): e0208266.
Rice ME. 2004. Transgenic rootworm corn: assessing potential agronomic, economic, and environmental benefits. Plant Health Progress, http://www.plantmanagementnetwork.org/sub/php/review/2004/rootworm/
Rovnyak AM, Burks CS, Gassmann AJ, Sappington TW. 2018. Interrelation of mating, flight, and fecundity in navel orangeworm females. Entomol. Exp. Appl. 166: 304-315.
Saikai Y, Mitchell PD, Hurley TM. 2019. An agent-based model of insect resistance management and mitigation for Bt maize: A social science perspective. In review at Pest Management Science.
Sappington TW. 2014. Emerging issues in Integrated Pest Management implementation and adoption in the North Central USA. Pp. 65-97. In: R. Peshin, and D. Pimental (eds.), Integrated Pest Management – Experiences with Implementation, Global Overview, Vol. 4. Springer Science+Business Media, Dordrecht.
Sappington TW. 2018. Migratory flight of insect pests within a year-round distribution: European corn borer as a case study. J. Integrative Agric. 17: 1485-1505.
Schrader PM, Estes RE, Tinsley NA, Gassmann AJ, Gray ME. 2017. Evaluation of adult emergence and larval root injury for Cry3Bb1-resistant populations of the western corn rootworm. J. Appl. Entomol. 141: 41-52.
Showers WB, Hellmich RL, Derrick-Robinson ME, Hendrix III WH. 2001. Aggregation and dispersal behavior of marked and released European corn borer (Lepidoptera: Crambidae) adults. Environ. Entomol. 30: 700-710.
Siegfried BD, Spencer T, Crespo AL, Storer NP, Head GP, Owens ED, Guyer D. 2007. Ten years of monitoring for Bt resistance in the European corn borer: What we know, what we don’t know and what we can do better. Amer. Entomol. 53: 208-214.
Siegfried BD, Hellmich RL. 2012. Understanding successful resistance management: The European corn borer and Bt corn in the United States. Special Issue of GM Crops and Foods 3: 184-193.
Siegfried BD, Spencer T. 2012. Bt Resistance Monitoring in European Corn Borers and Western Corn Rootworms. In: M. Oliver and Y. Li, eds. Plant Gene Containment. Elsevier, NY.
Siegfried BD, Rangasamy M, Wang H, Haridas CV, Tenhumburg B, Sumerford D. 2014. Estimating the frequency of Cry1F resistance in field populations of the European corn borer (Lepidoptera: Crambidae). Pest Manag. Sci. 70: 725-33.
Siegfried BD, Waterfield N, ffrench-Constant RH. 2005. Expressed sequence tags from Diabrotica virgifera virgifera midgut identify a coleopteran cadherin and a diversity of cathepsins. Insect Mol. Biol. 14: 137-143.
Smith JL, Rule DM, Lepping MD, Farhan Y, Schaafsma AW. 2017. Evidence for field-evolved resistance of Striacosta albicosta (Lepidoptera: Noctuidae) to Cry1F Bacillus thuringiensis protein and transgenic corn hybrids in Ontario, Canada. J. Econ. Entomol. 110: 2217-2228.
Smith JL, Baute TS, Sebright MM, Schaafsma AW, DiFonzo CD. 2018. Establishment of Striacosta albicosta (Lepidoptera: Noctuidae) as a primary pest of corn in the Great Lakes region. J. Econ. Entomol. 111: 1732-1744.
Smith JL, Farhan Y, Schaafsma AW. 2019a. Practical resistance of Ostrinia nubilalis (Lepidoptera: Crambidae) to Cry1F Bacillus thuringiensis maize discovered in Nova Scotia, Canada. Sci. Rep. 9: 18247.
Smith JL, DiFonzo CD, Baute TS, Michel AP, Krupke CH. 2019b. Ecology and management of the western bean cutworm (Lepidoptera: Noctuidae) in corn and dry beans - Revision with focus on the Great Lakes region. Journal of Integrated Pest Management. doi: 10.1093/jipm/pmz025.
Souza D. 2019. Characterization of pyrethroid resistance in the western corn rootworm Diabrotica virgifera virgifera LeConte. Ph.D dissertation, University of Nebraska-Lincoln.
Souza D, Vieira BC, Fritz BK, Hoffmann WC, Peterson JA, Kruger GR, Meinke LJ. 2019a. Western corn rootworm pyrethroid resistance confirmed by aerial application simulations of commercial insecticides. Sci. Rpts. 9: 6713.
Souza D, Peterson JA, Wright RJ, Meinke LJ. 2019b. Field efficacy of soil insecticides on pyrethroid-resistant western corn rootworms (Diabrotica virgifera virgifera LeConte). Pest Manag. Sci. online: DOI 10.1002/ps.5586
Spencer JL, Hibbard BE, Moeser J, Onstad DW. 2009. Behaviour and ecology of the western corn rootworm (Diabrotica virgifera virgifera LeConte). Agric. For. Entomol. 11: 9-27.
Spencer J, Onstad D, Krupke C, Hughson S, Pan Z, Stanley B, Flexner L. 2013. Isolated females and limited males: Evolution of insect resistance in structured landscapes. Entomol. Exp. Appl. 146: 38-49.
Storer NP, Babcock JM, Schlenz M, Meade T, Thompson GD, Bing JW, Huckaba RM. 2010. Discovery and characterization of field resistance to Bt maize: Spodoptera frugiperda (Lepidoptera: Noctuidae) in Puerto Rico. J. Econ. Entomol. 103: 1031-1038.
Tabashnik BE. 1994. Delaying insect adaptation to transgenic plants: seed mixtures and refugia reconsidered. Proc. R. Soc. B Biol. Sci. 255: 7-12.
Tabashnik BE, Carrière Y. 2017. Surge in insect resistance to transgenic crops and prospects for sustainability. Nat. Biotechnol. 35: 926.
Taylor S, Krupke C. 2018. Measuring rootworm refuge function: Diabrotica virgifera virgifera emergence and mating in seed blend and strip refuges for Bacillus thuringiensis (Bt) maize. Pest Manag. Sci. 74: 2195-2203.
Tooker JF, Douglas MR, Krupke CH. 2017. Neonicotinoid seed treatments: Limitations and compatibility with integrated pest management. Agricultural & Environmental Letters 2: 170026.
United States Department of Agriculture National Agricultural Statistics Service. 2019. ‘Acreage.’ https://www.nass.usda.gov/Publications/Todays_Reports/reports/acrg0619.pdf
Van der Sluijs JP, Amaral-Rogers V, Belzunces LP, Bijleveld van Lexmond MFIJ, Bonmatin J-M, Chagnon M, Downs CA, Furlan L, et al. 2014. Conclusions of the worldwide assessment of neonicotinoids and fipronil to biodiversity and ecosystem functioning. Environ. Sci. Pollut. Res. DOI 10.1007/s11356-014-3229-5.
Van Dijk TC, Van Staalduinen MA, Van der Sluijs JP. 2013. Macro-invertebrate decline in surface water polluted with imidacloprid. PLoS ONE 8: e62374. doi:10.1371/journal.pone.0062374
Vélez AM, Spencer TA, Alves AP, Crespo ALB, Siegfried BD. 2013a. Fitness costs of Cry1F resistance in fall armyworm, Spodoptera frugiperda. J. Appl. Entomol. 138: 315-325.
Vélez AM, Spencer TA, Alves AP, Moellenbeck D, Meagher RL, Chirakkal H, Siegfried BD. 2013b. Inheritance of Cry1F resistance, cross-resistance and frequency of resistant alleles in Spodoptera frugiperda (Lepidoptera: Noctuidae). Bull. Entomol. Res. 103: 700-7013.
Vélez AM, Vellichirammal NN, Jurat-Fuentes JL, Siegfried BD. 2016. Cry1F resistance among lepidopteran pests: A model for improved resistance management? Curr. Opin. Insect Sci. 15: 116-124.
Vellichirammal NN, Wang H, Eyun SI, Moriyama EN, Coates BS, Miller NJ, Siegfried BD. 2015. Transcriptional analysis of susceptible and resistant European corn borer strains and their response to Cry1F protoxin. BMC Genomics 16: 558.
Wang H, Coates BS, Chen H, Sappington TW, Guillemaud T, Siegfried BD. 2013. Role of a gamma-aminobutryic acid (GABA) receptor mutation in the evolution and spread of Diabrotica virgifera virgifera resistance to cyclodiene insecticides. Insect Mol. Biol. 22: 473-484.
Wangila DS, Leonard BR, Ghimire MN, Bai Y, Zhang L, Yang Y, Emfinger KD, Head GP, Yang F, Niu Y, Huang F. 2013. Occurrence and larval movement of Diatraea saccharalis (Lepidoptera: Crambidae) in seed mixes of non-Bt and Bt pyramid corn. Pest Manag. Sci. 69: 1163-1172.
Wangila DS, Gassmann AJ, Petzold-Maxwell JL, French BW, Meinke LJ. 2015. Susceptibility of Nebraska western corn rootworm (Coleoptera: Chrysomelidae) populations to Bt corn events. J. Econ. Entomol. 108: 742-751.
Wangila DS, Meinke LJ. 2017. Effects of adult emergence timing on susceptibility and fitness of Cry3Bb1-resistant western corn rootworms. J. Appl. Entomol. 141: 372-383.
Yang F, Kerns DL, Head GP, Leonard BR, Levy R, et al. 2014. A challenge for the seed mixture refuge strategy in Bt maize: Impact of cross-pollination on an ear-feeding pest, corn earworm. PLoS ONE 9(11): e112962. doi:10.1371/journal.pone.0112962 .
Yang F, Williams J, Porter P, Huang F, Kerns DL. 2019. F2 screen for resistance to Bacillus thuringiensis Vip3Aa51 protein in field populations of Spodoptera frugiperda (Lepidoptera: Noctuidae) from Texas, USA. Crop Prot. 126: 104915.
Yao J, Zhu YC, Lu N, Buschman L, Zhu K. 2017. Comparisons of transcriptional profiles of gut genes between Cry1Ab-resistant and susceptible strains of Ostrinia nubilalis revealed genes possibly related to the adaptation of resistant larvae to transgenic Cry1Ab corn. Int. J. Mol. Sci. 18: 301.
Yu EY, Gassmann AJ, Sappington TW. 2019a. Effects of larval density on dispersal and fecundity of western corn rootworm, Diabrotica virgifera virgifera LeConte (Coleoptera: Chrysomelidae). PloS One 14(3): p.e0212696.
Yu EY, Gassmann AJ, Sappington TW. 2019b. Using flight mills to measure flight propensity and performance of western corn rootworm, Diabrotica virgifera virgifera (LeConte). J. Vis. Exp. (152), e59196, doi:10.3791/59196.
Zhao JZ, Cao J, Li YX, Collins HL, Roush RT, Earle ED, Shelton AM. 2003. Transgenic plants expressing two Bacillus thuringiensis toxins delay insect resistance evolution. Nature Biotechnology 21:1493-1497. DOI: 10.1038/nbt907.
Zhao JZ, O’Neal MA, Richtman NM, Thompson SD, Cowart MC, Nelson ME, Pan ZQ, Alves AP, Yamamoto T. 2016. mCry3A-selected western corn rootworm (Coleoptera: Chrysomelidae) colony exhibits high resistance and has reduced binding of mCry3A to midgut tissue. J. Econ. Entomol. 109: 1369-1377.
Zukoff SN, Ostlie KR, Potter B, Meihls LN, Zukoff AL, French L, Ellersieck MR, French BW, Hibbard BE. 2016. Multiple assays indicate varying levels of cross-resistance in Cry3Bb1-selected field populations of the western corn rootworm to mCry3A, eCry3. 1Ab, and Cry34/35Ab1. J. Econ. Entomol. 109: 1387-1398.