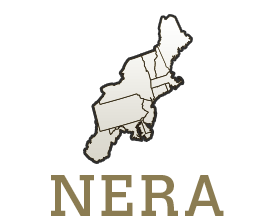
NE1943: Biology, Ecology & Management of Emerging Disease Vectors
(Multistate Research Project)
Status: Inactive/Terminating
NE1943: Biology, Ecology & Management of Emerging Disease Vectors
Duration: 10/01/2019 to 09/30/2024
Administrative Advisor(s):
NIFA Reps:
Non-Technical Summary
Statement of Issues and Justification
The need as indicated by stakeholders. Infectious diseases are on the rise worldwide and account for a quarter of all human mortality and morbidity despite extraordinary medical advances. Diseases once thought to menace only remote tropical regions are now spreading extensively due to dramatic social and environmental changes, including globalization, land use modification, and climate change. Of particular concern are those disease-causing pathogens that are transmitted by arthropod vectors. These diseases can spread rapidly and have complex epidemiologies that are susceptible to changing environmental conditions, and cause unpredictable costly outbreaks. Mosquitoes and ticks are the most important disease vectors in the U.S. and around the world. Worldwide, mosquito-borne diseases have killed more people than all the wars in history combined. Malaria probably killed more Americans than any other disease in the 1800s, except tuberculosis, cholera, and dysentery (Hong 2007, Urban 2010). More recently, West Nile virus (WNV) caused widespread fear in the U.S. when it was first detected in New York in 1999 and rapidly spread across the country within 5 years. WNV has emerged as the most important mosquito-transmitted disease in the U.S., with over 41,000 diagnosed cases and 1,700 deaths to date (CDC 2018a). Within the last 10 years outbreaks of emerging mosquito-borne diseases, such as chikungunya, dengue, Zika, and Rift Valley fever viruses, have revealed new vulnerabilities to the U.S. and around the world. Tick-borne Lyme disease is currently the most commonly reported vector-borne disease in the U.S. with an annual estimate of 300,000 cases, mostly in the northeastern and upper midwestern regions (CDC 2018b). Ticks also transmit a number of other microbial, protozoan, and viral diseases, some of which have only recently been identified and whose impacts on humans, livestock, and wildlife may be profound (e.g., Bourbon virus, heartland virus) (Savage et al 2013, Kosoy et al 2015). Overall, the CDC has reported that disease cases from infected mosquitoes, ticks, and sand flies in the U.S. have tripled in the last 13 years but that 80% of vector control organizations lack critical prevention and control capacities (CDC 2018c). There is also a need for better vector-human contact monitoring tools. These are helpful in calculating the risk of disease and potential pathogen emergence events.
The importance of the work and consequences if not done. With few available vaccines and new vector-borne diseases emerging at a steady rate, minimizing human exposure to disease vectors and managing vector populations remain the primary methods for reducing mosquito- and tick-borne infections. Lyme disease continues to present the largest vector-borne disease burden in the U.S. with 30,000 cases reported annually to the CDC, but approximately 300,000 total human infections per year (CDC 2018b). In the past decade, several new tick-borne infections have been identified (e.g., Borrelia miyamotoi, B. mayonii, Heartland virus), and well-known infections have re-emerged such as Powassan virus, a potentially lethal tick-borne pathogen (Savage et al 2013, Kosoy et al 2015, Mansfield et al 2017). Other dangerous native pathogens include the mosquito-borne LaCrosse encephalitis virus, responsible for numerous cases of childhood encephalitis every year, eastern equine encephalitis (EEE) with about a 50% case fatality rate, and even pathogens that affect companion animals, such as the fatal dog heartworm.
Our ability to properly control these and other disease threats are limited by our understanding of vector ecology. Historically and recently, investigations into basic science questions in vector biology have led to innovative control strategies. However, invasive species threaten to alter the epidemiology and transmission dynamics of existing and new diseases, and ultimately the health of humans, pets, wildlife, and livestock. This presents a “moving target” that requires regular scientific investigation. Mosquito invaders include the yellow fever mosquito (Aedes aegypti) and the Asian tiger mosquito (Aedes albopictus), well established in the southeastern U.S. and recently reported in California as rapidly spreading (Metzger et al 2015). The Asian tiger mosquito is moving northward and newer mosquito species have also arrived, such as the Asian bush mosquito (Ae. japonicus japonicus), a cold weather adapted species that is becoming increasingly common in the urban and suburban landscapes along the eastern seaboard, as well as in the northwest and Canada (Kaufman & Fonseca 2014, Jackson et al 2016, Peterson et al 2017), and the Australian Aedes notoscriptus in southern California (Paterson and Campbell 2015). Even within the last year, we have witnessed the invasion and spread of the Asian longhorned tick (Haemaphysalis longicornis), which was first discovered on a sheep farm in New Jersey in November 2017 and has since been detected in seven other states as of September 2018 (Beard et al. 2018, Rainey et al. 2018). The Asian longhorned tick can be self-cloning and is known to transmit several human disease agents in its native range in Asia, including spotted fever rickettsiosis and a potentially deadly virus (Severe Fever with Thrombocytopenia Syndrome Virus (SFTSV)) (Heath 2016). Experts expect that it is only a matter of time before this invasive species begins to transmit pathogens to Americans. Its immediate threat, however, is toward the country’s livestock. The Asian longhorned tick is known to multiply rapidly on livestock in Australia and New Zealand and can kill young animals by exsanguination (Heath 2016).
The U.S. will likely continue to experience the arrival of new vector species and pathogenic agents. Consider chikungunya virus, a dangerous pathogen that causes crippling arthritic damage to survivors, that infected more than 2.5 million people in the Indian Ocean region in a massive eruption in 2004-2005 and more than 350,000 people in the Caribbean in the first recorded outbreak outside of tropical Africa and Asia (Weaver et al 2018). Or the 2015-2016 outbreak of Zika virus, which infected tens of thousands of people in the Americas, causing severe birth defects as well as neurological problems in over 3,000 cases. The U.S. was affected during both outbreaks. Over 2,800 chikungunya cases were recorded in the U.S. in 2014 (CDC 2018d). Over 5,000 Zika cases were recorded in the U.S. in 2016, including 224 that were transmitted by local mosquitoes in the continental U.S. Ultimately, if infectious patients meet competent vectors then the disease can establish, amplify, and spread (CDC 2018e). The U.S. not only is a travel and immigrant destination for large numbers of people from around the world but also has highly competent mosquito vectors that include Ae. aegypti and Ae. albopictus.
The economic impact of arthropod-borne illness is devastating. For example, Lyme disease – America’s most common vector-borne disease - has been estimated to cost the U.S. healthcare system between $712 million and $1.3 billion annually in total doctor visits and testing one year after the first diagnosis, as symptoms of fatigue, musculoskeletal pain, and memory problems persist (Adrion et al 2015). The cost of treating WNV infections has been considerable, with Louisiana estimating $70 million for 2002 alone (Zohrabian, 2005). This estimate was made early in the outbreak and more recent estimates have indicated suggested that costs are much higher with total cumulative expenses related to reported hospitalized cases from 1999 through 2012 to be $778 million (95% confidence interval $673 million–$1.01 billion) (Staples et al 2014). The estimated cost per human case of EEE is $3 million in 1995 dollars (Villari et al. 1995). EEE and WNV both threaten the nation’s multi-billion-dollar equine industry. The mortality rate of horses infected with WNV is 34%; the rate for those with EEE approaches 100%. For example, in 2000, the estimated loss in New Jersey alone due to equine cases of WNV was $6 million. Tourism, which increases human exposure to mosquitoes, is similarly impacted by outbreaks of mosquito-borne disease. The recent outbreak of Zika virus, although generally mild in adults, has been conclusively linked to severe birth defects, which carry a tremendous economic and emotional burden on affected families. Recent computational analyses estimate that even a mild Zika outbreak could cost the U.S. in excess of $183 million in medical costs and productivity losses, whereas a severe outbreak could cost up to $1.2 billion.
The lessons learned from new and emerging mosquito- and tick-borne disease risks serve as an impetus for bolstering U.S. vector surveillance and management (Anyamba et al 2014). Encouragingly, important advances are being made in areas that include new methods and tools for monitoring and the control of mosquitoes and ticks. However, the budgets for research and abatement programs have been substantially reduced. In a report released in early 2018, the CDC estimated that 80% of the nation’s vector control organizations lack the critical prevention and control capacities needed to combat the tripling of disease cases from mosquitoes, ticks, and sand flies reported over the past 13 years (CDC 2018). Improved information sharing and coordination and the standardization of monitoring and control tools are needed for researchers and control professionals to make informed decisions and better utilize limited resources.
The technical feasibility of the research. Our research will focus on critical gaps in our understanding of the biology of mosquito and tick disease vectors of disease agents in the U.S. These include Ae. aegypti, Ae. albopictus and Culex pipiens mosquitoes, which are the three most important mosquito vectors nationally, and tick species (e.g., blacklegged tick, Ixodes scapularis; American dog tick, Dermacentor variabilis; lone star tick Amblyomma americanum) that are vectors of human pathogens. We will also gather vital knowledge on the ecology of other invasive vectors, with a focus on the newly invasive Asian longhorned tick, H. longicornis, in the early stages of its invasion and spread into the U.S., as well as encourage the knowledge infrastructure to deal with other potential invasive species. Our project will have three clear objectives:
- Develop and strengthen effective surveillance and monitoring of disease vectors at local and regional scales, including the development and testing of novel trapping and vector/pathogen identification techniques. Under this objective, project participants will leverage and strengthen existing surveillance programs in a coordinated fashion to yield robust comparable data across large geographic scales.
- Determine the ecology and geographic distribution of invasive and native disease vectors under changing environmental conditions to enhance our ability to predict conditions leading to existing and novel animal and human diseases.
- Develop novel control and management interventions and test their impacts on the transmission of human and animal diseases.
Under each of these objectives, we have 3-4 sub-objectives to focus our project, which is described in the Methods section. Our objectives are technically feasible, but only if done by a coordinated team. Our team is composed of 20 top entomologists who study tick and mosquito biology, with the required experience and facilities and we are confident we can succeed with the proposed research.
The advantages of doing the work as a multistate effort. This work cannot be completed without a multistate effort. In a rapidly changing landscape, the experience and guidance of one region are critical to others. As a specific example, both Ae. aegypti and Ae. albopictus are new to California, and California abatement districts have benefited from the experience of those in the east coast states where these species are often the most common mosquito species. Conversely, researchers in Florida and Texas bring to the table experience working with populations affected with dengue and Zika, a set of skills that may be needed more broadly across the U.S.
What the likely impacts will be from successfully completing the work. The project will build a highly collaborative network of scientific expertise to deal with existing and newly invasive tick and mosquito species. The project will benefit all U.S. residents by understanding, assessing, and mitigating the threat posed by ticks and mosquitoes of public health, veterinary, and wildlife importance. Our efforts will strengthen capacity to detect and predict outbreaks of vectors and associated diseases and evaluate the effectiveness of existing and novel control interventions under different environmental and social conditions. The project further provides for and encourages environmentally sound, scientifically based, and professional control by public health and pest control agencies. Our proposed research directly builds on a prior multistate project (NE1443). Since 2014, we have met and exchanged published and unpublished results, have generated novel hypotheses from group participants and discussed coordination in sampling effort. We have produced numerous accomplishments and deliverables and garnered some competitive grants from newly formed collaborations. For this revision/replacement, we are now ready to expand our collaborative network to develop and strengthen a more coordinated region-wide and multi-state research effort.
Related, Current and Previous Work
Some of the key outcomes of the prior NE-1443 project include:
- Compiled a comprehensive catalog of disease vector resources that includes more than 70 strains of mosquitoes, mosquito cell lines, muscoid flies, sand flies, sand fly cell lines, Culicoides, and Culicoides cell lines, ticks and tick cell lines.
- Reviewed and compiled new shipping/regulatory information regarding the interstate transfer of live arthropod vectors.
- Compiled a comprehensive database of training courses within the field of Medical and Veterinary Entomology, identifying pedagogical issues and content requiring attention.
- Developed the first egg identification guide for Aedes container-breeding mosquitoes in New York.
- Evaluated the spatial distribution and environmental risks of Powassan (POWV) arbovirus in Connecticut from multi-year tick field surveys.
- Tracked the genetic diversity of POWV in field-collected mice (vertebrate host) and ticks (arthropod vector) to yield empirical evidence supporting observed gradual, long-term evolutionary trends of POWV.
- Elucidated spatial and temporal infection dynamics of the Lyme disease spirochete (Borrelia burgdorferi) using resident submitted tick specimens and physician-reported Lyme disease cases.
- Conducted and reported evaluations of various tick-associated disease prevention and integrated tick management methods, including personal protective measures, habitat modification, applications of biological and natural compounds, and host-targeted control measures.
- Identified and tested natural and registered compounds for mosquitocidal activity.
- Conducted genomic and deep sequencing investigations of two species of microsporidia (Edhazardia aedis and Vavraia culicis) and the mosquito immune response to improve our knowledge of mosquito microsporidia and our understanding of host-pathogen interactions.
- Developed a simulation model for chikungunya virus outbreaks transmitted by Ae. aegypti and Ae. albopictus and public health interventions in the U.S. Monitored mosquito-associated arboviruses across regions to allow better predictions of arboviral disease incidence.
- Preliminary experiments to better understand winter egg survival of Ae. albopictus under different temperatures and within different container habitats.
- Tested and reported the effects of ultraviolet radiation (UVR) on mosquito populations from direct exposure and indirectly through the degradation of tire material and the toxicity of tire compounds.
- A comprehensive review of barriers to the publishing of mosquito information by states, highlighting that available funding was the most important predictor.
- Conducted and reported Wolbachia trials to control Ae. albopictus in California.
- Tested the application of dsRNA technologies for potential mosquito control efforts.
- Elucidated key socio-ecological factors and processes driving the production of Ae. aegypti and Ae. albopictus in urban areas.
- Evaluated the ability of unmanned aerial vehicles (UAVs) to identify potential Ae. albopictus larval habitat in urban areas, and machine learning of resultant data for long-term monitoring of Ae. albopictus populations.
Related Multistate Projects: A search of the national database website reveals no other current project that addresses similar objectives and scope as this project.
Objectives
-
Develop and strengthen effective surveillance and monitoring of disease vectors at local and regional scales, including the development and testing of novel trapping and vector/pathogen identification techniques.
Comments: Under this objective, project participants will leverage and strengthen existing surveillance programs in a coordinated fashion to yield robust comparable data across large geographic scales. -
Determine the ecology and geographic distribution of invasive and native disease vectors under changing environmental conditions to enhance our ability to predict conditions leading to existing and novel animal and human diseases.
-
Develop novel control and management interventions and test their impacts on the transmission of human and animal diseases.
Methods
Objective 1: Develop and strengthen effective surveillance and monitoring of mosquito and tick disease vectors at local and regional scales, including the development and testing of novel trapping and vector/pathogen identification techniques.
Vector-based surveillance efforts may be used in conjunction with human and domestic animal case reporting as a part of a comprehensive surveillance program. Specifically, surveillance efforts based on sampling host-seeking ticks and mosquitoes from natural areas and testing them for infection is one of the most effective ways to discern trends and monitor changes in risk. These activities provide 1) sensitive and early evidence of pathogen transmission, 2) information on the abundance, distribution, identity and infection status of potential vectors, and 3) information on the risk of human infection to warn the public and guide disease prevention and control measures in real-time. In addition, testing for human antibodies against the main salivary proteins associated with infections from arthropod vectors (e.g., D7 long in Aedes and agTRIO in Anopheles mosquitoes) may give estimations of individual human-vector contact and disease risk within at-risk communities. Moreover, a vector surveillance program can monitor long-term changes in vector and pathogen distribution anticipated under climate change and provides the first line of defense against the introduction of exotic ticks, mosquitoes, and pathogens through early detection of these threats. Under this objective, project participants seek to improve current monitoring practices by developing and testing novel trapping and pathogen testing techniques. The long-term goal is to leverage and strengthen existing surveillance programs by providing data on the best practices for vector surveillance and pathogen testing and to encourage standardized data collection to yield robust comparable data across large geographic scales. Under this objective, we propose the following four sub-objectives:
(1.1) Map tick abundance, infection status, and related transmission risks in the eastern United States.
The rise of tick-borne diseases in the U.S. is driven largely by the proliferation and range expansion of the black-legged tick (I. scapularis) and lone star tick (A. americanum) in the eastern half of the country. In addition, the Asian longhorn tick (H. longicornis) poses a new threat after it was first discovered in the U.S., in New Jersey, in 2017, and has since been found in nine states. Consequently, we need to understand where these tick species are located, in addition to mapping their abundance, infection status, and human exposure risk to their pathogens. As a part of this sub-objective, ticks will be sampled seasonally at a minimum of 20-30 sentinel sites located in the eastern U.S. using standard “drag” procedures (i.e. dragging flannel cloth over the vegetation along fixed-distance transects). The density of questing ticks and pathogen infection rates will be obtained. Entomological risk indices will be estimated and compared to reported human case incidences at the county scale. In addition, we will use these data to map the range expansion of ticks and their pathogens. We will compare these data to information obtained from passive tick surveillance and data from state and commercial tick-testing laboratories where ticks are submitted by residents, health departments, or physicians’ for identification and pathogen testing. This will help us determine which ticks parasitize residents and how frequently they are exposed to their pathogens.
(1.2) Develop improved diagnostic screening tools for tick-borne pathogens of public health and veterinary importance.
Ticks transmit a diverse array of bacterial, protozoan, and viral pathogens in the U.S. and new tick-borne pathogens are continually being discovered which necessitates the development of new diagnostic assays. We propose to develop a panel of PCR-based diagnostic assays to screen for diverse pathogens from each of the major human-biting tick species. Multiplex assays will be developed for the pathogen complexes transmitted by I. scapularis, A. americanum, and Dermacentor variablis, and will include newly discovered pathogens such as Borrelia mayonii, Bourbon virus, and Heartland virus. Nucleic acid extraction methods and PCR reaction conditions will be optimized and tested against known standards before being applied to field-collected specimens. From this, we will develop best-practice recommendations and support the adoption of standardized methods for diagnosis and testing of ticks.
(1.3) Better predict the distribution and density of the Ae. albopictus mosquito in relation to habitat type and climate conditions using data from surveillance programs throughout the eastern U.S.
The Asian tiger mosquito (Ae. albopictus) is an invasive species and an important arbovirus vector that is expanding its range in the U.S. Winter temperature is an important constraint to its northward expansion with potential overwintering range limits located between the 0o and -5o C cold month isotherm. Under this sub-objective, we will monitor Ae. albopictus populations in the eastern U.S. to determine where populations can stably persist. We hypothesize that Ae. albopictus populations will be constrained to urban heat-islands that serve as refugia at the northern limits of its range. We will use data obtained from surveillance programs in the eastern U.S. to predict Ae. albopictus distribution and density in relation to habitat type and climate conditions to determine its thermal and habitat requirements. We will compare these observations with direct measurements on the overwintering survival of Ae. albopictus eggs placed in different microhabitats in both urban and rural habitats along a latitudinal gradient. Temperature and humidity will be monitored and diapause-conditioned eggs will be hatched the following spring to assess overwintering survival.
(1.4) Develop and test new chemical formulations and lures to improve the efficiency of mosquito trapping and surveillance programs
Current operational trapping methodologies for mosquito and arbovirus monitoring in the U.S. rely primarily on the deployment of CO2-baited CDC light and gravid traps. CDC light traps are effective for collecting a diversity of Anopheline and Culicine mosquito species, whereas gravid traps are more effective for monitoring WNV activity via the collection of gravid Culex species that have already ingested at least one blood meal. However, neither trap type is particularly effective for collecting diurnal feeding and container-breeding species such as Aedes triseriatus, an important vector of La Crosse virus, and three important invasive human-biting species of concern: Ae. aegypti, Ae. albopictus and Ae. japonicus. BG-Sentinel traps have shown promise for collecting host-seeking Ae. albopictus and Ae. aegypti but further improvements are needed to enhance the collection of these and other container-breeding species. We propose to evaluate the efficacy of new chemical lures developed by commercial and government laboratories, including those in Bedoukian Research and USDA ARS Beltsville and will compare them to combinations of standard BG-lures and CO2 to attract (invasive and native) Aedes and to compare overall species catch diversity. These studies will be conducted in at least 2 experimental field sites in CT and 2 urban residential areas in MD, each with established overwintering populations of Ae. albopictus and Ae. japonicus using different lure combinations. Trapping will occur 3 times a week from May through September when populations are at their peak abundance. In CT, traps will be placed at least 10 m apart and randomly rotated. In MD, traps will be deployed in residential urban landscapes at least 150 m apart, and collections made prior and after the addition to lures in a Before-After-Control Intervention design to help control for site effects. Linear models and pairwise post-tests will be performed to compare the number of mosquito species collected by each lure. Preliminary observations have also suggested that some of these lures may also have attractant effects on gravid Aedes and be toxic to their larval offspring. Mosquitoes will be identified in the laboratory, and in a subset of adult-trapping trials, sorted into pools for virus testing in Vero cell culture. From this, we will compare the mosquito trap efficiency, overall species richness, and arbovirus infection rates for mosquitoes collected by each lure/compound. Separate oviposition experiments will compare the attractant effects of lure compounds to standard plant infusions that are typically used to bait oviposition traps in a Latin-square design. All trials will ensure that that traps are spatially independent and located in appropriate landscapes for the target Aedes species (i.e., urban, forested etc). Laboratory-based dose-response trials will test the toxicity of lure compounds on newly hatched larvae. Statistical comparisons among treatments will be made using linear models.
Objective 2: Research the ecology and geographic distribution of invasive and native disease vectors under changing environmental conditions to enhance our ability to predict conditions leading to existing and novel animal and human diseases.
The cornerstone of the effective prevention of human and animal disease transmission by arthropods is a precise understanding of their species-level biology. In particular, the ecology of a vector species and its distribution inform effective implementation of control and the generation of accurate predictions of future risk. For many pathogen-vector-host systems, the vector is the easiest target to interrupt transmission, therefore understanding its ecology and distribution has a direct impact on animal and human health. Under this objective, we propose the following four sub-objectives:
(2.1) Determine the distribution of native tick vectors of Lyme disease, rickettsial disease, and Heartland virus.
This sub-objective has two essential elements, the distribution of ticks and their potential as vectors of disease. Ostensibly, the collection methods follow the recommendations of the CDC and will include (but will not be limited to) standardized procedures collected at our sentinel sites established under sub-objective 1.1. We will utilize data from six standard methods of tick collection: tick flagging/dragging, tick collection off human researchers (walking), CO2 traps, collecting from dead deer, collecting from live, small animals, and opportunistic collection from pets or animals. These are focused on I. scapularis, and we will add collections from livestock as a seventh approach to capture ticks that may be important as pests or vectors of animal pathogens.
With the first year if the project, we will identify sites within the landscape likely to harbor tick populations. These might include forests, woodlots, pasture, ecotones, and other natural habitats. Our goal is to drag sample sites twice a month, covering a linear distance of ~500m, and checking drags every 10m. All ticks will be collected in 80% ethanol and subsequently morphologically identified. Specimens will be saved in alcohol for identification confirmation and pathogen testing.
Tick specimens will be tested for pathogens using molecular approaches. For bacterial and protozoan pathogens, real-time PCR will generally be used because it offers the best combination of accuracy and ease of use, and current multiplexed approaches allow for the detection of multiple pathogens in a single assay. However, more specialized assays may also be used to address particular questions about specific pathogens. For example, testing for viruses (especially RNA viruses like Powassan or Heartland) may require more careful handling. In general, these will follow guidelines from the CDC, in which a given assay is: 1. Compared to a panel of positive and negative control DNA/RNA, 2. Targeted to specific, multiple sequences, increasing specificity of the assay, and 3. Positive results are confirmed by sequencing and BLASTing of sequences.
(2.2) Make novel discoveries of the ecology and distribution of container-breeding Aedes vectors responsible for the transmission of human arboviruses (dengue, Zika, chikungunya), as well as ornithophilic vectors responsible for transmission of zoonotic viral encephalitides (WNV, western equine encephalitis, and EEE viruses).
Mosquitoes are responsible for the transmission of a number of pathogens in the U.S., which can be roughly divided into human-to-human pathogens transmitted by container Aedes spp. and zoonotic pathogens, mostly transmitted by Culex spp. While the potential for human-to-human transmission likely represents the greatest potential for large human epidemics (1000s to 100000s of new and overall cases) (Habálek 2003), zoonotic arboviruses have long been responsible for greater morbidity and mortality in the continental U.S. (Calisher 1994). Surveillance of these species focuses on trapping host-seeking adults, egg-laying females, or eggs. No trapping methodologies are completely unbiased, but most host-seeking traps can capture both container Aedes spp. and Culex spp. We will trap host-seeking adults with off-the-shelf traps, baited with CO2 and/or a chemical lure, BG-Sentinel (focused on Aedes spp.) or CDC light (a more general trap) traps (Silver 2008). Gravid trapping is usually more species-specific, although both Culex spp. and Aedes spp. that inhabit containers are often caught in standard gravid traps, with Culex spp. often more abundant (CDC or Reiter-style) (Silver 2008). We will ovitrap with black plastic cups, filled with water and baited with standard leaf infusion (Silver 2008). These approaches are effective for documenting the presence and relative abundance of these species and generate consistent data allowing for meaningful comparisons (see sub-objective 2.4). From these surveillance data, we will make inferences about phenology, spatial variation, population genetics/genomics, and population dynamics (e.g., Little et al. 2017, Reed et al. 2018, Hopperstad and Reiskind 2016). Surveillance of Culex spp. vectors usually focus on gravid female capture and follow similar methodologies and data generation as studies on container-breeding Aedes mosquitoes. Whenever possible, we will save biological specimens for potential subsequent data collection (e.g. DNA extraction, microbiome analyses, etc.). Replicate numbers of traps will vary among indvidual trials depending on the specific study system and be subject to statistical power analyses to detect potential differences among treatment levels.
We will make novel discoveries about the ecology of these container species from hypothesis-driven experiments and observations. These can vary in scale from landscape population responses to semi-field studies to microcosm studies of larval or adult behavior to physiological or biochemical studies on individual mosquitoes (e.g., Piermarini et al. 2013, Couret et al. 2014, Freed et al. 2014).
(2.3) Conduct surveillance for novel vectors, including range changes in resident species and invasive, exotic, or understudied species.
Of paramount importance to the utility of this multi-state project is the ability to coordinate and disseminate information about novel vector distributions, either because of a change in the range of a resident species, or the emergence of an invasive species.
We will take a number of approaches to discover new species. As mentioned above, for container-breeding mosquitoes and ticks, sampling approaches are generally well-known and universally applied (although region-wide systematic and coordinated surveillance programs are still lacking). However, to effectively search for novel species we need to employ creative monitoring approaches and sampling regimes. For example, the Invasive Mosquito Project (http://www.citizenscience.us/imp/) and Texas A&M University effort on triatomine bugs (http://kissingbug.tamu.edu) (Curtis-Robles et al 2015) have provided high-quality data on the distribution of important vectors using unconventional sampling approaches and citizen scientists. These approaches use schools (IMP) or citizen submissions of data (kissingbug.tamu.edu) to improve our understanding of vector distribution, as opposed to relying strictly on active, planned surveys as might be done by a mosquito control agency or individual professional researcher. Likewise, the discovery of the Asian longhorned tick in the U.S. (H. longicornis) was from a citizen reporting an odd tick, along with a local vector-control specialist making the effort to assist the victim and experts at the state land-grant institution (Rutgers University) confirming identification. These cases exemplify the effectiveness of various interacting entities: citizens, local government, and academic institutions, at discovering novel vectors. As such, we work with the various federal (CDC, NSF National Ecological Observatory Network), state (e.g. Departments of Health, Departments of Environmental Quality), and local (e.g. mosquito or vector control districts), to assist in the detection and confirmation of range expansions, novel species, and population increases of important species. These various projects often result in similar data products, and we will provide standard data formats and a network for these disparate projects that will allow participants in NE1943 to leverage the data.
(2.4) Synthesize historical and contemporary datasets, either bespoke by researchers or from various federal, state, and local agencies, to examine trends in vector distributions.
Increasingly, region-wide data involving vectors are being collected, substantial barriers exist preventing their consolidation and utilization. One limitation in the utility of these data is a lack of expertise in their analysis. Another is the reluctance of multiple groups to share data. This multi-state project is well positioned to serve as a nexus for the analysis of these data sets to provide value-added products. Datasets can be either historical and/or contemporary, and collected at various scales, from local, specific data collected by individual researchers for a particular question, up through centrally coordinated efforts like that at the National Ecological Observatory Network (NEON) on ticks and mosquitoes. The methodologies for data analysis will vary, but we will establish pipelines for a given dataset and share analyses through online data sharing portals, like DataDryad or Github.
Objective 3. Develop novel disease vector control (management) interventions and test their impacts on the transmission of human and animal diseases.
The global emergence and re-emergence of mosquito and tick-borne pathogens are on the rise due to many factors, including urbanization and sprawl, globalization, and climate change. Current control tools are often ineffective and require innovation and evolution to adapt to the vector-borne disease system, the local ecology, and changing environment. The aim of this objective is to develop and evaluate mosquito intervention approaches. This research occurs along the spectrum from basic science needed to develop new tools to observational studies evaluating interventions to randomized control trials of contemporary tools (Wilson et al. 2015). We propose the following three sub-objectives:
(3.1) Evaluate intervention campaigns for Aedes aegypti using autodissemination stations of pyriproxyfen.
We will evaluate the efficacy of SpringStar’s Autodissemination Stations (ADS) for the control of Aedes aegypti. The ADS works by attracting oviposition-seeking females who are unable to reach the water in the 5-gallon black bucket and instead pick-up a gel containing pyriproxyfen as they exit. This technology exploits mosquito behavior to distribute toxic concentrations of larvicide to cryptic containers and is ideally suited to Ae. aegypti that exhibit skip-oviposition behavior. This ADS approach originated by the vector biologists at Rutgers Center for Vector Biology and SpringStar Inc. is working on continued R&D and commercialization of this product. We are now partnering with SpringStar to use the current prototypes in field intervention studies in South Texas. Several technical experts from the Centers for Disease Control and Prevention, including Roberto Barrera, is overseeing this work.
We will conduct field trials in South Texas deploying about 500 ADS units in two neighborhoods from August to December. The mosquito outcome variable will consist of vector abundance data from BG-Sentinel 2 traps distributed in the ADS intervention neighborhoods as well as nearby reference (‘control’) neighborhoods receiving no intervention. Each intervention community consists of about 100 homes and we will have about 12 BG traps. About 6 BG traps will be placed in the nearby reference communities receiving no intervention. Each trap location will be sampled one time per week by placing the trap in the morning on day one and retrieving the trap and contents in the morning on day two (24 hours). We determine the ability of the ADS intervention to reduce the vector population in low-income communities of South Texas with previous local transmission of dengue, Zika, and chikungunya virus transmission.
(3.2) Evaluate the effect of truck-based adulticide on Culex quinquefasciatus abundance and infection with West Nile virus.
A partnership with Harris County Mosquito and Vector Control Division and Texas A&M University is allowing a retrospective study to evaluate the effectiveness of adulticides for WNV management. We are using a database from 2002 to 2017. Over this time period, 36,685 spray events have been recorded which represent a truck delivering adulticide (ULV) to a known operational area. To complement these data, we have the Culex abundance and infection data for the same operational areas and over the same time period. Over 11 million female Culex quinquefasciatus were collected during this period and about half of these were tested for WNV. Out of 152,661 pools of Cx. quinquefasciatus tested for WNV, 5,018 were positive. We will build a statistical model evaluating how the quantity of product deployed in each operational area predicts the Culex abundance and infection with WNV. We will interrogate multiple time lags to measure how long the effect of the intervention lasted.
(3.3) Evaluate the effectiveness of community-based habitat management on the abundance of urban mosquitoes and West Nile virus transmission in neighborhoods that vary in socio-economic status
In partnership with residents within focal urban areas in the northeast region (e.g., Baltimore, MD and New York City, NY) we will evaluate the effectiveness of low-cost mosquito management approaches that aim to manage larval habitat, reduce vector populations, and lower related nuisance and infection risks. Agency-based mosquito control professionals have a long history of partnering with local communities to manage vector species and related public health threats. But many urban communities in the northeast region receive little or no publicly-funded control and lack the resources to afford services from private vendors. Moreover, the knowledge and attitudes of residents toward mosquitoes and the environment, in general, have been shown to vary considerably and unpredictably along socio-economic gradients and in response to education programs (e.g., Bartlett-Healy et al 2011, Dowling et al 2013, Bodner et al 2016). There is a need to investigate cost-efficient approaches that help urban communities reduce mosquito-related issues over sustained periods with minimal ongoing intervention from mosquito-control professionals. Under this sub-objective, we will engage communities in 3 field sites (Baltimore MD, Greenbelt/University Park MD, Washington DC) to formulate management strategies tailored for local conditions, and then evaluate those strategies for measurable declines in vector populations, nuisance burdens, and infection rates. Approaches will involve (but not necessarily limited to) workshops, train-the-trainer programs, and community-based habitat removal interventions. Changes in resident knowledge, attitudes, and practices will be accessed by various social-science instruments appropriate for the particular study conditions and might include focus groups, semi-structured interviews, and pre- and post-surveys with a range of qualitative (e.g., thematic and pattern analyses) and quantitative (e.g., Q-sort, multiple regression) analyses. Numbers of participants in each study will vary, but appropriate for the methodological instrument being used to detect meaningful differences, if any. Dissemination of information related to mosquitoes and their control (useful, ineffectual, or detrimental) will be accessed with social network analyses. Changes to vector populations and infection rates will be sampled by scientific surveys of larval habitats and adult populations using standard approaches (e.g., surveys of resident containers, ovitrapping, adult trapping).
Measurement of Progress and Results
Outputs
- Improved understanding of the thermal and expansion range limits of invasive mosquito species (Obj. 1,2)
- Assessment of mosquito trap lures to strengthen and improve operational surveillance (Obj. 1)
- Evaluation of passive and active surveillance data to track geographic spread and risk of tick-borne pathogens (Obj. 1,2)
- Develop and evaluate PCR assays to detect diverse pathogens carried by invasive and native ticks (Obj. 1)
- Better evidence for the efficacy of autodissemination of pyriproxyfen for the control of Ae. aegypti (Obj. 3)
- Improved understanding of the effects of adulticide applications on Culex abundances and WNV infection rates (Obj. 3).
Outcomes or Projected Impacts
- An interactive and dependent network of scientific expertise to deal with new mosquito- and tick-borne disease outbreaks.
- Enhanced capacity to detect, predict, and respond to outbreaks of vectors and associated human, livestock and wildlife diseases.
- Develop, test, implement, and encourage novel control (management) interventions that reduce transmission of human and animal diseases using environmental sound and scientifically-based approaches.
Milestones
(1):Assess overwintering survival of Ae. albopictus eggs in field sites (Obj. 1) Establish test plots for mosquito trap lure study (Obj. 1) Establish a network of surveillance sites for tick collection (Obj. 1) Optimize multiplex PCR assays for pathogen testing (Obj. 1) Coordinate existing mosquito and tick surveillance efforts by project participants (Obj. 2) Collect baseline ecological and distribution data of invasive and native mosquito and tick vectors to identify key areas of collaboration (Obj. 2) Generate data integration schemes to leverage diverse surveillance data of invasive and native vectors, including citizen-based data collection (Obj. 2) Establish data standardization protocols for combining and comparing vector ecology and distribution data across studies (Obj. 2) Combine 2019 vector ecology and distribution data, and generate hypotheses for 2020 (Obj. 2) Develop key areas of vector ecology and distribution of study on collaboration for external grant submissions (Obj. 2). Conduct autodissemination interventions (Obj. 3) Build statistical models to see how truck-based ULV product quantity predicts the Culex abundance and infection with WNV (Obj. 3) Bidirectional engagement with urban communities to formulate community-based mosquito control approaches (Obj. 3)(2):Field test the efficacy of different chemical lures (Obj. 1) Continue assessment of overwintering survival of mosquito eggs (Obj. 1) Develop models to predict Ae. albopictus distribution and abundance (Obj. 1) Collect and test ticks from surveillance sites (Obj. 1) Develop standardized protocols for pathogen testing of ticks (Obj. 1) Produce a review article identifying important strengths, weaknesses, opportunities, and challenges for understanding future vector ecology and distribution changes (Obj. 2) Analyze data comparing Ae. aegypti abundance in neighborhoods with autodissemination station intervention and control neighborhoods with no intervention (Obj. 3) Communication results of studies evaluating vector control interventions (Obj. 3) Development, Implementation, and testing of community-based interventions (Obj. 3)
(3):Optimize attractant lures for mosquito traps (Obj. 1) Continue assessment of overwintering survival of mosquito eggs (Obj. 1) Continue tick surveillance from field sites (Obj. 1) Continued grant production efforts, with a goal of at least three grant proposals involving multiple states (Obj. 2) Leverage existing surveillance efforts and data to submit multi-institutional grants on invasive and native disease vectors under changing environmental conditions (Obj. 2) Write proposals to build on previous and current work evaluating vector control interventions (Obj. 3) Development, Implementation, and testing of community-based interventions (Obj. 3)
(4):Large-scale field testing of new chemical trap lures (Obj. 1) Continue tick surveillance from field sites (Obj. 1) Report the results of community-based interventions to scientific audiences and citizen stakeholders (Obj. 3)
(5):Evaluate the efficacy of passive and active tick surveillance data (Obj. 1) Predict the geographic spread and risk of mosquito- and tick-borne diseases based on surveillance data (Obj. 1) Final report preparation (Obj. 1-3)
Projected Participation
View Appendix E: ParticipationOutreach Plan
Several of the universities represented in this multistate group of researchers have formal extension programs while some do not. However, most researchers and all institutions interact closely with health officers, mosquito and tick professionals or the public at large (Table 1). Many of us conduct a variety of outreach activities and create documents or web-based information. In order to summarize a large amount of information in the limited space available in this proposal, we are providing in Table 1 links to the web pages where descriptions of the outreach programs as well as PDFs of fact sheets, publications, lecture summaries and curricula, standard operating procedures, etc. can be found and downloaded.
Additionally, we will hold an annual meeting to promote cooperation among participants, which can lead to enhance research opportunities. Results of our meetings and activities will be available to all interested parties via the NIMSS website. All publications, both refereed and non-refereed will be listed on NIMSS. Informational meetings will be held to brief appropriate state and county mosquito control personnel on our progress, particularly via oral presentations at national (e.g., American Mosquito Control Association), regional (Northeastern Mosquito Control Association) and local (e.g., New Jersey Mosquito Control Association) meetings that are designed to bring together scientists and mosquito control practitioners.
Table 1. Critical outreach activities provided by researchers at the institutions participating in this multistate collaboration. Click on the web pages to access and download materials and obtain lists of publications and course curricula.
Institution |
Serve as a consulting resource to state and local agencies |
Provide non-University outreach |
Provide services and Standard Operating Procedures (SOPs) |
Develop fact sheets and other education materials |
Cornell University |
Public Health, Vector Control, Department of Environmental Conservation, State legislature, County-level Cooperative Extension, 4-H, USDA |
Pest control applicators; K-12 educators; ScienceCenter |
Training for PCO, Mosquito/vector control units, certification credit training webinars, vector biology boot camp |
Fact sheets for ticks (e.g., http://neregionalvectorcenter.com/longhorned-tick) and mosquitoes, online webinars and other educational materials available via the website |
Iowa State University |
Iowa Department of Public Health, local public health, and vector control agencies |
Pest control applicators, Science Outreach events |
Mosquito surveillance: https://mosquito.ent.iastate.edu/ Fact sheet for ticks: https://store.extension.iastate.edu/Product/Ticks-and-Tick-borne-Diseases-in-Iowa-PDF |
|
North Carolina State University |
North Carolina Department of Health and Human Services |
North Carolina Museum of Natural Sciences, North Carolina Mosquito and Vector Control Association |
Training at all levels, from CEUs for PCOs to helping establish Mosquito Control Districts |
|
University of Idaho |
Idaho Department of Health and Welfare |
Northwest Mosquito and Vector Control Association; Idaho Mosquito Abatement Districts |
For students, postdocs, faculty, professionals: 6-day annual “Biology of Vector-borne Diseases (BVBD)” course (Center for Health in the Human Ecosystem, UI) |
BVBD course materials provided to all participants |
University of Kentucky |
Kentucky Department of Public Health |
Pest control applicators, Science Outreach events; K-12 educators |
Training for PCOs |
|
University of Maryland |
Health, Mosq. Control |
Rotary Club; K-12; Landscape Professionals |
Fact sheets for mosquitoes |
|
University of Wisconsin |
Wisconsin Department of Health Services, County Public and Environmental Health, Vector Control |
Pest control applicators, Science Outreach events |
Certification for public health entomology; workshops for current pest control practitioners |
Educational materials for ticks and mosquitoes |
Organization/Governance
This multistate research project will be established in accordance with the format suggested in the “Manual for Cooperative Regional Research”. One person at each participating institution or agency will be designated, with the approval of the institution’s or agency’s director, as the voting member of the Technical Committee. Other individuals and interested parties are encouraged to participate as non-voting members of the committee. There will be elections of a Chair, Chair-elect, and a Secretary. All officers are to be elected for two-year terms to provide continuity. Administrative guidance will be provided by an assigned Administrative Advisor and a CSREES Representative.
Note: This proposal has purposefully been written to define the project in broad research terms. This is intended to best position the Multistate Project to cast a broad net to secure an expanded membership, and therefore expanded opportunities for community building and collaboration. First, an expansion of membership will be sought geographically so that the Multistate Project can become a truly national project. Second, the intended expansion will be aimed at securing participants beyond the traditional agricultural experiment station and USDA-ARS mosquito biologists to involve mathematical modelers, community ecologists, and others with special skills regardless of whether or not they study mosquitoes and/or ticks.
Literature Cited
Adrion, E. R., J. Aucott, K. W. Lemke, and J. P. Weiner. 2015. Health Care Costs, Utilization and Patterns of Care following Lyme Disease. PLoS One 10: e0116767.
Anyamba, A., J. L. Small, S. C. Britch, C. J. Tucker, E. W. Pak, C. A. Reynolds, J. Crutchfield, and K. J. Linthicum. 2014. Recent weather extremes and impacts on agricultural production and vector-borne disease outbreak patterns. PLoS One 9: e92538.
Bartlett-Healy, K., G. Hamilton, S. Healy, T. Crepeau, I. Unlu, A. Farajollahi, D. Fonseca, R. Gaugler, G. G. Clark, and D. Strickman. 2011. Source reduction behavior as an independent measurement of the impact of a public health education campaign in an integrated vector management program for the Asian Tiger mosquito. Int J Env Res Pub He 8: 1358-1367.
Bodner, D., S. L. LaDeau, D. Biehler, N. Kirchoff, and P. T. Leisnham. 2016. Effectiveness of print education at reducing urban mosquito infestation through improved resident-based management. PLoS One 11: e0155011.
Beard C. B., J. Occi, D. L. Bonilla et al. 2018. Multistate Infestation with the Exotic Disease–Vector Tick Haemaphysalis longicornis — United States, August 2017–September 2018. MMWR Morb Mortal Wkly Rep 2018;67:1310–1313. DOI: http://dx.doi.org/10.15585/mmwr.mm6747a3.
Calisher, C. H. 1994. Medically important arboviruses of the United States and Canada. Clinical Microbiology Reviews 7: 89-116.
CDC. 2018a. West Nile Virus. Centers for Disease Control and Prevention. Available at: https://www.cdc.gov/westnile/index.html [Accessed Jan 2, 2019].
CDC. 2018b. Lyme Disease. Centers for Disease Control and Prevention. Available at: https://www.cdc.gov/lyme/index.html [Accessed Dec 27, 2018].
CDC. 2018c. Vital Signs. Illnesses of the Rise. Centers for Disease Control and Prevention. Available at: https://www.cdc.gov/vitalsigns/vector-borne/index.html [Accessed Dec 20, 2018].
CDC. 2018d. Chikungunya Virus. Centers for Disease Control and Prevention. Available at: https://www.cdc.gov/chikungunya/index.html [Accesses Dec 28, 2018].
CDC. 2018e. Zika Virus. Centers for Disease Control and Prevention. Available at: https://www.cdc.gov/zika/index.html [Accesses Dec 28, 2018].
Couret J., E. Dotson, and M. Q. Benedict. 2014. Temperature, larval diet, and density effects on development rate and survival of Aedes aegypti (Diptera: Culicidae). PLoS One 9: e87468 https://doi.org/87410.81371/journal.pone.0087468.
Dowling, Z., P. Armbruster, S. L. LaDeau, M. DeCotiis, J. Mottley, and P. T. Leisnham. 2013. Linking mosquito infestation to resident socioeconomic status, knowledge, and source reduction practices in suburban Washington, DC. EcoHealth 10: 36-47.
Freed, T. Z., B. Kesavaraju, and P. T. Leisnham. 2014. Effects of competition and predation by native mosquitoes on the North American invasion of Aedes japonicus japonicus (Diptera: Culicidae). J. Med. Entomol. 51: 1159-1167.
Heath, A. 2016. Biology, ecology and distribution of the tick, Haemaphysalis longicornis Neumann (Acari: Ixodidae) in New Zealand. NZ Vet J. 64(1): 10-20.
Hubálek, Z. 2003. Emerging human infectious diseases: anthroponoses, zoonoses, and sapronoses. Emerging infectious diseases 9: 403-404.
Hong, S.K. 2007. The burden of early exposure to malaria in the United States, 1850-1860: Malnutrition and Immune Disorders. J Econ Hist. 67(4): 1001–1035.
Hopperstad, K. A., and M. H. Reiskind. 2016. Recent Changes in the Local Distribution of Aedes aegypti (Diptera: Culicidae) in South Florida, USA. J. Med. Entomol. 53: 836-842.
Jackson, M., P. Belton, S. McMahon, M. Hart, S. McCann, D. Azevedo, and L. Hurteau. 2016. The First Record of Aedes ( Hulecoeteomyia ) japonicus (Diptera: Culicidae) and Its Establishment in Western Canada. J Med Entomol 53: 241-244.
Kaufman, M. G. and D. M. Fonseca. 2014. Invasion biology of Aedes japonicus japonicus. Ann Rev Entomol 59: 31-49.
Kosoy O. I., A. J. Lambert, D. J. Hawkinson, D. M. Pastula, C. S. Goldsmith D. C. Hunt et al. 2015. Novel thogotovirus associated with febrile illness and death, United States, 2014. Emerg Infect Dis. 21: 760–764.
Little, E., D. Biehler, P. T. Leisnham, R. Jordan, S. Wilson, and S. L. LaDeau. 2017. Socio-ecological mechanisms supporting high densities of Ae. albopictus (Diptera: Culicidae) in Baltimore, MD. J Med Entomol 54: 1183-1192.
Mansfield, K. L., L. Jizhou, L. P. Phipps, and N. Johnson. 2017. Emerging tick-borne viruses in the Twenty-First century. Front Cell Infect Microbiol 7: 298. https://doi.org/10.3389/fcimb.2017.00298
Metzger, M. E., M. H. Yoshimizu, M. Hardstone; K. A. Padgett, A. Kerry, R. et al. 2017. Detection and Establishment of Aedes aegypti and Ae. albopictus (Diptera: Culicidae) Mosquitoes in California, 2011-2015. J Med Entomol 54(3): 533-543
Peterson, A. T., and L. P. Campbell. 2015. Global potential distribution of the mosquito Aedes notoscriptus, a new alien species in the United States. J. Vector Ecol 40: 191-194.
Petersen, W. H., M. Lyons, M. Westlind, A. Duffy, D. Kangiser, and E. A. Dykstra. 2017. New distribution records of mosquitoes in Washington state. J Am Mosq Control Assoc 33(1): 60-63. DOI: 10.2987/16-6630.1. PubMed PMID: 28388317.
Rainey, T., J. L. Occi, R. G. Robbins, and A. Egizi. 2018. Discovery of Haemaphysalis longicornis (Ixodida: Ixodidae) parasitizing a sheep in New Jersey, United States. J Med Entomol 55(3): 757-759.
Reed, E. M. X, B. D. Byrd, S. Richards, M. Echkart, C. Williams, and M. H. Reiskind. 2018. A statewide survey of container Aedes spp. (Diptera: Culicidae) in North Carolina, USA 2016: A multiagency response to Zika Using ovitraps. J Med Entomol: DOI: 10.1093/jme/tjy190.
Rochlin, I. 2018. Modeling the Asian longhorned tick (Acari: Ixodidae) suitable habitat in North America. J Med Entomol. DOI: 10.1093/jme/tjy210.
Savage H. M., M. S. Godsey, A. Lambert, N. A. Panella, K. L. Burkhalter, J. R. Harmon et al. 2013. First detection of heartland virus (Bunyaviridae: Phlebovirus) from field collected arthropods. Am J Trop Med Hyg 89(3): 445-52.
Silver, J. B. 2008. Mosquito Ecology: Field Sampling Methods, 3rd ed. Springer, New York.
Staples J., M. Shankar, M. Sejva, M. Meltzer, M. Fischer. 2014. Initial and long-term costs of patients hospitalized with West Nile virus disease. Am J Trop Med Hyg 90: 402–409.
Urban, L. 2010. U.S. Malaria Deaths 1870. The Scientist.
Villari, P., A. Spielman, N. Komar, M. McDowell, and R. J. Timperi. 1995. The economic burden imposed by a residual case of eastern encephalitis. Am J Trop Med Hyg 52(1): 8-13.
Wilson, A. L., M. Boelaert, I. Kleinschmidt, M. Pinder, T. W. Scott, L. S. Tusting, and S. W. Lindsay. 2015. Evidence-based vector control? Improving the quality of vector control trials. Trends Parasitol 31: 380-390.
Weaver, S. C., C. Charlier, N. Vasilakis, and M. Lecuit. 2018. Zika, Chikungunya, and Other Emerging Vector-Borne Viral Diseases. Ann Rev of Med 69: 395-408.
Zohrabian A, M. I. Meltzer, R. Ratard, K. Billah, N. A. Molinar, K. Roy, R. D. Scott, L. R. Petersen 2002. West Nile virus economic impact, Louisiana. Emerg Infect Dis 10:1736–1744.