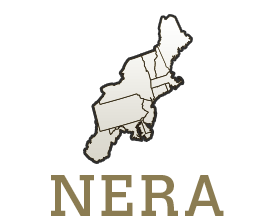
NE1942: Enhancing Poultry Production Systems through Emerging Technologies and Husbandry Practices
(Multistate Research Project)
Status: Inactive/Terminating
NE1942: Enhancing Poultry Production Systems through Emerging Technologies and Husbandry Practices
Duration: 10/01/2019 to 09/30/2024
Administrative Advisor(s):
NIFA Reps:
Non-Technical Summary
Statement of Issues and Justification
The United States Poultry and Egg Association, the worlds’ largest and most active poultry industry organization, has identified the need for incorporating engineering and technology to enhance energy/resource efficiencies for layers, broilers, and turkeys. In addition, this non-profit organization considers its strategic objectives to be responsive to the changing needs of the poultry industry, to advance research in poultry science and technology, and to address the safety of processed poultry and poultry products. Past and ongoing research from this multi-state organization is in direct alignment with these objectives in terms of providing a collaborative approach to enhance poultry research in the area of nutrition, environmental control, air quality, housing systems, lighting, automation and robotics, food safety and security, health and bird welfare. Moreover, the United Egg Producers (UEP), a laying hen industry-wide commodity group has identified the need for the development of enhanced technology to address management techniques to improve laying hen performance, behavior, and well-being in alternative housing systems (cage-free aviaries). Furthermore, industry organizations such as the Egg Industry Center also supports collaborative research and outreach activities to find appropriate solutions to the questions faced by the poultry and egg industry.
Due to an increasing global population, there is a need for sustainably, efficiently, and safely produced and processed protein, such as poultry. Adapting Precision Livestock Farming (PLF) techniques to poultry production and processing systems will further enhance the industry’s capability to meet the growing demand. Consumer and retail demands of the poultry industry have resulted in an expansion in a variety of production and feeding systems with differing constraints and knowledge base. The collaborative research efforts outlined here will further expand the concept of PLF, using automated continuous monitoring of animals to allow producers to record and assess in real-time, the health and welfare status of their animals. The approach includes the use of automation and robotics, equipment efficiency, facility design, energy, and resource allocation. In addition, improvements in antimicrobial intervention technologies and processing methods will mitigate the risk of foodborne pathogens in poultry and poultry products.
Poultry production systems must be optimized to be more energy/resource efficient, minimize their carbon footprint and sustainable through infrastructure development throughout the blockchain of production, that is, breeder, hatchery, producer, processor, and consumer. Collaborative research can help tie together the different components of the system and their relationships. The consequences of not renewing the project will result in the loss of the poultry industry’s position in the global marketplace and the ability to provide safe and nutritious poultry products to consumers throughout the world.
This multi-state poultry research team is comprised of environmental physiologists, behaviorists, animal welfare scientists, nutritionists, engineers, extension scientists, microbiologists, and economists. These collaborators have access to commercial-type, pilot scale, and laboratory-scale facilities with the equipment and expertise necessary to work in the proposed areas of research. Collaboration has been documented in the past with successful outcomes reported. Additionally, this multi-state group has several leading industry experts that are active participants. These industry connections provide valuable input and link researchers with commercial operations/birds/equipment to maximize the relevance and feasibility of research efforts.
Objectives:
Collaborators at the experimental stations in AL, AR, CA, GA, GA-ARS, HI, IA, IL, IN, IN-ARS, KY, MD, MI, MN, MS, MS-ARS, NE, NC, PA, TX, VA, WI, and Bern (Switzerland), will work on research related to the following objectives:
- Integrating technological advances into poultry systems:
Emphasis on collaborative and translational research incorporating science, engineering, and technology to enhance system efficiency and sustainability through infrastructure development of blockchain production.
- Establishing and adopting husbandry practices to a changing industry landscape:
Collaborative research will encompass a multi-disciplinary approach to create a resilient poultry production system through optimal management of inputs and outputs in an ethically responsible manner.
Some of the unique instruments and facilities that will be used and shared are emission chambers (IA, IL, and PA), portable air monitoring units (IL), multi-channel telometric body temperature sensors systems (IA), multi-station individual bird feeding units (IA), environment chambers and animal calorimeters (CA, IA, IL, PA, MS, and VA). We also have state of the art commercial poultry production facilities which will allow us to conduct research (AL, IA, IN, IL, MN, MS, NC, PA, and VA). In addition, some of our collaborators possess biosafety level (BSL) 2 pathogen containment facilities for conducting challenge experiments in all production birds (AL, MN, MS, CT, and VA). Facilities with fully functioning processing plants for converting birds to food (GA, AL, and WI) and BSL 2 poultry processing research (GA and AL) are available for use. The specialized equipment located at these stations can enhance collaborative research efforts and could bring synergistic outcomes. Therefore, the collaborative use of these facilities by participating universities and USDA stations will maximize research productivity.
Due to the complexity of the poultry industry needs, research objectives addressed by this multi-state project could not be accomplished at any single station/university. Each of the researchers has expertise and facilities to address some component(s) of poultry production efficiency, well-being, nutrition, environmental concerns, facility management, and/or technical monitoring of poultry environments and poultry processing. To best comprehensively address the challenges facing the poultry industry identified in this project, a collaborative effort is necessary and will eliminate duplication of effort and conserve resources.
Data generated from these collaborative research efforts will enhance the resiliency of the poultry industry through real-time monitoring (digital, thermal, spectral, and spatial) of facilities, leading to improved poultry welfare, performance, food safety and security throughout the blockchain of poultry production.
The successful completion of the objectives outlined in this proposal will lead to:
- Incorporation of advanced science, engineering and technology into poultry production facilities to enhance system efficiency, and improve production efficiency and poultry well-being.
- Identification of relationships between environmental, nutritional, and disease factors that affect poultry well-being, food safety, and economics.
- Establishment and adoption of current and future poultry husbandry practices and development of a trained scientific workforce to address a changing industry landscape.
- Development of new antimicrobial intervention technologies or processing methods to control foodborne pathogens in poultry and poultry products.
Related, Current and Previous Work
A CRIS search on poultry environment or production system did not identify any active multi-state projects, other than the existing NE-1442.
Contributions of NE-1442 investigators.
NE-1442 was founded in 1978 to facilitate research in the area of poultry production recognizing collaboration and communication amongst the research institutions would advance poultry management strategies. NE-1442 has grown from 11 universities in 1999 to 18 universities and two USDA-ARS units for the 2014 renewal. The multistate has expanded to 25 universities, three USDA-ARS units, and several additional international partners for this 2019 rewrite. An annual meeting is held each year to discuss research results and plans collaborative projects for the coming year. The NE-1442 philosophy is to help implement sustainable practices in poultry production for the industry from a multi-state collaborative approach. Recognizing that more advances can be made as a collaborative group rather than working as a single investigator-initiated grant. The complexities of animal-environment interactions overlaid with animal welfare and consumer demands require a multi-discipline, collaborative approach to identifying ways to best achieve the producer and societal goals.
Examples of sustained endeavors by NE-1442 participants include:
- History of publication in the scientific literature. Over the last 4 project years of this 5-year project, this regional poultry research group has published 412 abstracts, 119 peer-reviewed journal articles, 10 popular-press articles, 9 peer-reviewed extension reports, and 55 proceedings at national and international meetings.
- Success with joint proposals demonstrates the capacity of NE-1442 to coordinate ideas, resources, and execute multi-state research projects.
- The multistate research group has secured over $22 million in grants to conduct research with the NE-1442 project during the last project cycle (5-year) period.
Collaborative research previously conducted and currently being worked on by these research stations is listed below.
Collaborative efforts in exchanging ideas and research by MI, MS, IN, NC, NE, and the University of Bern have progressed in developing better management techniques to increase the performance efficiency of laying hens in cage-free aviaries. Problems exist in aviary housing, such as feather pecking, skin lesions, keel bone damage, laying eggs on the litter floor, worker and bird exposure to particulate matter, noxious gases, mortality, and other general management problems of maintaining hens. These research stations, along with various international experts in the United Kingdom discussed how to minimize these problems at the meeting in Switzerland in 2016. Out of this meeting, came ideas for developing several research projects designed to minimize the above problems. Continued research in this area is needed going forward. The U. of Bern (CH), MI, CA and IN are collaborating on keel bone damage of hens in aviaries in an effort to better prepare pullets for aviaries and enhance workforce development. CA has also been collaborating with IA and with U. of Bristol (UK) on keel bone damage issues.
MI collaborated with other institutions and completed research on turkeys relative to fearfulness and the impact on meat quality and mitigation of pale, soft, and exudative (PSE) meat. The stations that conducted research in this area were CA, IN, USDA-ARS, NC, and U. of Bern.
VA worked with NC on projects to minimize the impacts of subclinical disease on broiler performance using antibiotic alternatives.
Several stations have researched air quality in commercial and alternative poultry facilities. PA collaborated with other stations to contribute to the PA HPAI Task Force with preparedness documents, websites, and presentations to the backyard and commercial poultry producers on flock plans, virus testing, biosecurity, flock depopulation, and bird disposal.
PA and members of NE-1442 from MN, MI, and IN collaborated on a NIFA grant addressing production and animal welfare of turkeys. A study is evaluating heavy hen density and litter material on the welfare of hens (growth, foot-pads, litter score, walking ability, and behavior).
Members from MS, AL, and USDA-ARS have collaborated to investigate radiant heater efficiencies, thermal performance of broiler house insulation, and the feasibility of rainwater capture and reuse on commercial broiler farms.
IA, through the Egg Industry Center (EIC), has been collaborating with several members of this multi-state project team (AL, CA, GA, IN, MI, MS, and PA) to address issues facing the egg industry. In particular, EIC has provided considerable funding (~$1 million) to the researchers, which has led to leverage of additional external funding (~$3.5 million). The IA team works closely with commercial egg producers to provide solutions to challenges associated with alternative hen housing systems. The EIC has been an effective conduit of communicating and disseminating research findings to egg producers, allied industries, government agencies, and consumers. One of such communication avenues is the EIC’s Annual Industry Issues Forum that moves around the country.
IN, USDA-ARS and IL demonstrated the utility of cooled perches for heat stress relief for laying hens, as well as how to engineer these systems, including the use of heat in winter.
Current Work: Literature Review
According to the United Nations, the global population will reach 9.9 billion in 2050; this rapid growth necessitates a dramatic increase in the world’s food supply, requiring 60 to 110% more agricultural products produced. However, the yield projected based on the current increasing rates are insufficient to meet the 2050 demand. Therefore, farmers worldwide will have to boost agricultural production, either by increasing the amount of agricultural land/facilities or by enhancing productivity with existing resources. The objectives of this multistate project aim to help in achieving this growth in agricultural production.
OBJECTIVE 1
1a. Engineering and Technology
Producers will inevitably rely on advanced tools and technologies to handle challenges in the process of production expansion. Compared to other industrial sectors, agriculture has been a sector tardy in applying high-tech engineering systems. In the early '80s, scientists promoted the concept of 'precision agriculture,' which aims to optimize agricultural returns on inputs and preserve resources by observing, measuring and responding to inter and intra-field variabilities with advanced technologies. Since then, precision agriculture has been exhibiting significant impacts to sustainable agricultural production. While most efforts in precision agriculture have been dedicated to crop production, its application and research on livestock and poultry are scarce. Real-time monitoring of metrics (i.e., ventilation, air quality, lighting, acoustics, microbial, and others) within the production system needs to be at both a flock and individual bird basis; this allows for welfare elements and distinctions to be balanced for the flock and individuals, which is a critical component of precision agriculture.
Currently, poultry producers have a limited ability to identify and address problems within in a production system rapidly. This is because more real-time monitoring systems have been directed at larger animals, such as swine or dairy. The discrepancy in innovation efforts directed at poultry likely reflects monitoring difficulties caused by the small size of the birds, and related to tracking similarly looking individuals within very large flocks (up to 100,000 plus per poultry house). While there has been some development of sensor technology to track hen movement, the existing methods are not without problems. Many rely on video to track patterns of the flock (therefore, do not provide information at the individual level) or electronic technologies that are incompatible with poultry housing systems (e.g., other birds and objects within the environment interfere with signals). The development of hen-mounted acoustic sensors and acoustic monitoring systems offers a novel approach with the potential for mitigating these problems. For instance, sensors can be placed under the feathers, reducing damage from pecking, and allow continuous recording of hen location compared to sensors that require readers in fixed locations. These devices can also be quite small, easily automated, and do not need external readers, compared to devices used now.
A need for objective determination of poultry welfare is of utmost importance as guidelines transition from resource-based allocations to outcome-based inspections of the birds in their housing environment (Hester, 2005). Currently, outcome-based welfare assessments are performed by subjective evaluation of trained individuals; however, this can still lead to different interpretations of the numerous indicators of welfare, such as, overall plumage score, cleanliness, keel deformation, comb pecking, footpad dermatitis, claw length, skin lesions, beak trimming, and toe damage. Furthermore, the scoring of these indicators is discrete without any discretion for intermediate scores. Advances in technology have led to machine vision systems capable of advanced pattern recognition needed to identify and classify different indicators of bird welfare accurately. The use of technology to aid in welfare assessments could substantially decrease observer-observer differences and allow for a rapid and widespread application of welfare assessments to advance the industry at the rate needed to match demand. This is especially relevant in the layer industry, where legislation in states (California) has mandated for certain alternative housing standards. Due to consumer demand and this legislation, producers have had to change to these alternative hen housing systems, without knowing the impact on bird health and welfare.
1b. Environmental Control and Management
As novel technologies, new housing, and system designs emerge, there is a need for evaluation and optimization of these systems to establish best management practices for producers. Particular interest is focused on perches, lighting, system layout, and ventilation (including equipment, thermal and air quality) depending on poultry production system. The provision of perches in hen housing systems could still lead to many detrimental effects (e.g., keel bone deformities, foot disorders, and bone fractures) that would negatively impact production and welfare of the birds. Considerable efforts are still needed towards optimizing perch design (e.g., shape, size, texture, material, and temperature), spatial arrangement (e.g., height, angle, and relative position), and management (e.g., timing of bird’s introduction to perches). Furthermore, lighting is of critical importance and a crucial environmental factor that affects behavior, development, production performance, health, and well-being of poultry (Lewis and Morris, 2000; Parvin et al., 2014). Recently, LED lights have become more readily available and affordable to poultry producers; these lights are promoted as being more energy-efficient, readily-dimmable, and long-lasting. However, the existing lighting guidelines/recommendations were mainly established based on the traditional incandescent or CFL lights and may not accurately reflect the operational characteristics and impact of the LED lights on birds. In addition, despite anecdotal claims about advantages of some commercial poultry-specific LED lights over traditional incandescent or fluorescent lights on poultry performance and behavior, data from controlled lab and controlled comparative studies are insufficient (Long et al., 2016a, b).
Although layer aviary systems have existed in the US for the last decade, the ventilation and environmental control requirements are vastly different from the conventional housing (Hayes, 2012; Zhao et al., 2015a). This situation is in part due to the lower stocking density and access to litter on the floor. Mainly during the winter, ventilation for indoor air quality at the lower stocking density requires supplemental heat, which must be properly distributed throughout the house. Further challenges in aviary systems include design ventilation rates, indoor air quality, heat, and moisture production of the birds and the surroundings, fuel usage, and the birds’ preference for winter temperature-ammonia combinations (Zhao et al., 2013; Ni et al., 2012). Another concern with these housing systems is that a portion of manure from the birds is held in the house on the floor as litter. Litter on the floor impacts indoor air quality and can lead to elevated ammonia and dust concentrations (Zhao et al., 2015b, Shepherd et al., 2015). Also, with the aviary system, the birds can be more active, potentially leading to increased heat and moisture production rates of these birds.
Increases in wind speed to improve convective cooling, particularly with heavy broilers (≥ 3 kg) and turkeys, requires increased ventilation capacity. The additional fans and evaporative pad area provide increased opportunity for ambient light access, which can lead to increased temporal and spatial variability in light intensity. Non-uniform light intensities can impair the ability to control photoperiod, introduce variations in feed and water consumption, decrease carcass quality (scratching), and decrease litter quality from excess bird density in some areas of the house (Czarick and Fairchild, 2016). Given these results, a better understanding of the spatial and temporal variability of light intensity in broiler houses is necessary to achieve proper prescriptive lighting programs and dynamic management of lighting systems in broiler houses. Newly developed technology such as non-restrictive fan shades and smart lighting systems could both be employed to improve prescriptive control of the lighting environment in commercial broiler housing.
Many environmental factors affect the growth of broilers, turkeys, and egg production in laying hens in a commercial setting. These factors include temperature, humidity, and ventilation air exchange, which can positively and negatively affect growth rate, uniformity, feed consumption, and feed conversion. Of these factors, proper ventilation rates in poultry houses are critical to the success of optimizing growth and other vital parameters. An essential part of maintaining proper ventilation is properly balancing carbon dioxide (CO2) within the house (Xin et al., 2009). In addition, heat and moisture production of poultry adds to the calculation of adequate ventilation rates (Li et al., 2005; Chepete et al., 2004). Many commercial broiler and turkey houses still use conventional propane-fueled heaters to brood birds during their early stages of life. Brooder buildings may also have suboptimal air exchange during the cold months of the year because of the need to reduce ventilation to conserve heating costs. When these conditions exist, oxygen availability is reduced under the brooder stoves, and levels of CO and CO2 may also increase. Thus, increased CO2 levels may have deleterious effects on bird health, particularly during the brooding phase.
It has been documented that turkey poults exposed to levels of over 25 to 30 ppm CO for just a few hours may have a higher risk of spontaneous turkey cardiomyopathy (STC; round heart disease; Frame et al., 2010). In addition to the adverse effect of increased CO, increased levels of CO2 are also of concern when conventional brooders are used during cold weather conditions. The previous researchers also documented that CO2 levels above 2500 ppm may be linked to increased mortality resulting from STC. If air circulation and fresh air ventilation rates are diminished during cold winter months, then a buildup of CO2 will result at the bird level (Frame et al., 2010). Since CO2 is denser than air, it will displace the air at the level of a bird. In addition to the CO2 formed as a by-product of combustion, CO2 is also exhaled from the birds, which adds to the overall air CO2 concentration. Frame et al. (2010) also commented that pockets of increased levels of the CO2 buildup of over 3000 ppm might exist in a brooder building if birds are crowded together, but much lower levels might exist just a few feet away. Thus, based on this research finding, increased CO2 levels may have deleterious effects on turkey health, particularly during the brooding phase.
Exposure of poults to excessive levels of CO2 may also affect their metabolism. Donaldson et al. (1995) subjected tom turkey poults to either ambient air (contains about 400 ppm CO2) or 4000 ppm CO2 for 16 or 40 h post-hatch. The results of subjecting the poults to 4000 ppm CO2 caused a reduction in body glycogen and plasma T4 concentrations. In addition, the increased CO2 levels made the poults very lethargic and sleepy. In the research published by Donaldson et al. (1995), the reduced plasma T4 concentration suggests that high atmospheric CO2 levels negatively affect thyroid function. Since thyroid activity is related to appetite control, reduced thyroid function may produce lower feed intake which would negatively affect body weights. Moreover, a lower thyroid activity would decrease metabolic processes which might lead to reduced nutrient absorption. While research in this area has been conducted, there is still a need, as the brooding environment for growing turkeys and broilers is so critical to achieving optimum growth performance. Ultimately, the goal will be to generate data to these industries that define the proper atmospheric conditions to promote optimum growth.
Vegetative buffers have been a useful tool at the urban/rural interface to deflect nuisance complaints from neighbors and mute the odors and dust associated with commercial poultry production. These buffers have effectively served as a screen and landscape for the farm and buildings to give them a more pleasing appearance in the neighborhood. However, less documented are their effects on the climate around the poultry houses, that is, shade, cooling the barns in summer and windbreaks, protecting the birds from winds and snow in winter. More reports in the literature and more companies are utilizing biomass crops like Miscanthus, switchgrass, and willows for renewable bedding options instead of pine or wood shavings (Mayer et al., 2016). Further research is needed on the merits of vegetative cover for outdoor access birds provided by buffers. For instance, does the vegetation result in more birds accessing the outdoor spaces provided; is bird welfare improved, and is flock mortality from aerial and terrestrial predators reduced? These are all important considerations that must be determined for farmers that produce poultry that requires outdoor access. All of these factors contribute to the health and welfare of the bird and must be considered when a farmer is producing poultry under one of these alternative housing systems.
OBJECTIVE 2
2a. Nutrition Strategies and Feed Manufacture
Understanding the way in which chickens and turkeys partition their nutrient intake in different production systems is mostly unknown. Therefore, research is needed on the breadth of poultry feeding programs by examining feed form, dietary ingredients, feed additives, and individual nutrients and how these influence not only the bird performance but also other elements of the production system such as well-being, environment, health, and food safety. This research will create replicated data to investigate the relationship between physical activity and production uses of nutrients in the current egg and meat-type bird genotypes and bird performance and health. Additionally, identification of bird strain and feed form effects on bird performance and nutrient utilization will be determined.
In Ovo Strategies. There is an increasing interest in ways to improve the developing embryo through early nutritional manipulation, such as in ovo administration of nutrients. This technique is used to enhance early gut development and may abate the challenges chicks encounter during the first few hours post-hatch when a delay in feeding or long periods of fasting are experienced. Although the amount of research on supplementing breeder diets with different nutrients has been increasing, perinatal nutrition has also been gaining recognition.
During the early 1980s and 1990s, most in ovo studies focused on the delivery of vaccines to the developing embryo. Sharma and Burmester (1984) showed that in ovo technology could be successful in vaccinating embryos against Marek’s virus. These investigators developed the basis of the in ovo injection procedures in use today. From the early work of Uni and Ferket (2003), there has been an increased interest in pre-hatch feeding. To date, sources of protein, carbohydrates, vitamins and minerals (Foye, et al., 2007; Tako et al., 2005; Ohta and Kidd, 2001) have been administered to the developing embryo via the amnion, air cell or yolk sac to improve embryo energy status, hatchability, BW, and post-hatch performance. In addition, early feeding may help prepare the chick gut transition from yolk-derived nutrition to external diet. Also, the enhancement of intestinal transporters due to in ovo administration of nutrients, such as HMB may aid in early gut maturation leading to the increased nutrient absorptive capacity of intestinal cells (Foye et al., 2007). In a research study conducted by Macalintal (2012), it was reported that injection of selenium into the egg yolk on the 10th day of incubation might be a useful tool in enhancing the selenium status of embryonic and post-hatch chick development.
More recently, raffinose (as a prebiotic) was delivered in ovo to investigate its effect on growth performance and gut health parameters of broilers (Berrocoso et al., 2017). The increasing dose of raffinose increased the villus height and villus height: crypt depth ratio and immune-related genes (CD3 and chB6) expression positively, suggesting its role in the potential of improving gut health. Similarly, mannan-oligosaccharide fractions (derived from the yeast cell wall) and probiotic (live nonpathogenic microorganisms that can create favorable gut microenvironment in the host) have been delivered into the amnion of fertile eggs on the 18th day of incubation to investigate their effects on early embryonic development as they relate to the health status and productive performance during post-hatch growth. These data demonstrated that mannan-oligosaccharide fractions, as well as probiotic (i.e., lactic acid bacteria) could be delivered in ovo without negatively affecting the hatchability of chicks (Macalintal et al. 2017 a, b). In the case of mannan-oligosaccharide fraction injection, gene expression on small intestinal segments showed increased intestinal cell proliferation, improved epithelial barrier function, and decreased stress level. These are important characteristics that support gut health development of chicks. On the other hand, in ovo probiotic administration (lactic acid bacteria), demonstrated a positive response in reducing Salmonella counts in the cecal contents of day-old and day 3 hatched chicks. These results warrant further research in this area, such as the extended physiological and production value of in ovo application of these products, as well as others. These studies are important as consumers demand poultry without the use of antibiotics.
Alternative Ingredients and Feedstuffs. Due to fluctuations in feed costs and variability of ingredients, there is a need to establish viable alternative feed ingredients for commercial poultry diets. This need has become more pertinent in isolated areas primarily where the economy relies on the livestock sector for employment, income, and food and nutrition security. One example of this is in Hawaii and the Pacific Islands, an isolated geographic region that has limited affordable cultivable land area. Hawaii imports over 85% of its food from the U.S. mainland and other countries (Leung and Loke, 2008), while the State of Hawaii set a goal of doubling food production by 2030. Moreover, communities across Hawaii and the Pacific Islands want to increase the local food supply to become less reliant on imports and also desire to lower their carbon footprint via reducing imports and using local inputs. With chicken being more environmentally friendly, producing eight times less greenhouse gas than beef (7 vs 56.6 kg CO2-eq/kg of meat produced; Gerber et al., 2013) and a feed conversion ratio of chicken is four times lower than beef (Eshel et al., 2014), it is cost effective and less competitive with human food.
Researchers in Hawaii have been working on a project entitled “Evaluation of local feedstuffs for sustainable animal farming in Hawaii” and have been able to develop a large dataset of nutrient profiles of local feedstuffs (e.g., macadamia nut cake, microalgae, cassava root chips, sweet potato, taro, yam, wheat bran, barley brewer’s grain, and others) to test productivity and efficiency of animals (Berrocoso et al., 2017; Malabad et al., 2016; Tiwari and Jha, 2015, 2016, 2017; Yadav and Jha, 2017; Yadav et al., 2017). These data reveal that some feedstuffs are rich in energy and protein, which can serve as potential feedstuffs for poultry diets when appropriately formulated. However, these alternative feedstuffs are typically rich in fiber. The fiber is not well utilized by endogenous enzymes of monogastric animals and reduces the utilization of other nutrients as well (Jha and Berrocoso, 2015). The fiber in the feedstuffs, however, can be degraded by use of exogenous enzymes to enhance its nutritional value and gut health (Jha et al., 2015; Tiwari et al., 2018), leading to improved performance. However further concerns include the effects on the health of the poultry; there are limited data on the impact of alternative feedstuffs and pasture-based poultry production on the intestinal microbiome and gut health parameters. This is an important area for future research as they play a crucial role in immune regulation, digestion, metabolism, and general health and well-being (Jha and Berrocoso, 2016).
The nutritional database generated in Hawaii may serve other sectors of the poultry industry as well, such as niche markets in the U.S. Specifically, the organic poultry industry is in desperate need of a viable, concentrated, highly available sources of methionine. While it is true that the National Organic Standards Board (NOSB) approved the utilization of synthetic methionine for poultry in 2015 (McEvoy, 2015) at new limits between 2 and 3 lbs./ton, the board also committed to phasing-out synthetic methionine for organic poultry production. The board has encouraged aggressive research on natural alternative sources of methionine. To these ends, research is still needed to identify sustainable and bioavailable sources of methionine for organic poultry production in order to retain this market, bird welfare, and the environment.
Use of Exogenous Enzymes. The poultry industry has adopted the practice of utilizing combinations of exogenous carbohydrases or carbohydrase(s) with phytase enzymes in order to improve feed efficiency and bird performance (Olukosi et al., 2007). As such, nutrient digestibility in broilers has been improved with a cocktail of enzymes such as xylanase, amylase, and protease when included with phytase (Olukosi et al., 2007). There are two major mechanisms by which nonstarch polysaccharide (NSP)-degrading enzymes are thought to improve performance: 1) eliminating the nutrient encapsulating effect of the cell wall and 2) reducing viscosity problems associated with NSPs, such as β-glucans and arabinoxylans (O’ Neill et al., 2014). More research is warranted in this area to test new, evolving enzyme technologies, as well as in varying feed formulations. Though, the most significant gap in knowledge exists for these enzymes and their efficacy in laying hen diets. As such, previous research utilizing layers is scarce and has produced inconsistent results. A combination of xylanase and phytase in wheat-based diets were fed to white and brown laying hens (Silversides et al., 2006). This study reported that in Phosphorus (P) - adequate diets, xylanase improved egg and albumen weight, while in the P-reduced diet with xylanase, the level of phytase increased egg and albumen weight. Also, research investigating reduced P layer diets, phytase has shown an incremental improvement on both Ca and P digestibility (Liu et al., 2007). However, the role of such enzymes to release energy and P to contribute in both early and later stage of egg production of laying hens, as well as manure P control has not been investigated.
Feed Manufacturing. Feed and feed manufacture represent the largest production cost (60-70%) for a commercial integrator. All commercial broilers and most turkeys are fed diets that are pelleted due to improvements in body weight gain, feed efficiency, and feed intake; with these benefits being more pronounced as feed form improves (Behnke 1996; Lilly et al., 2011; Lemons et al.; 2016; Sellers et al., 2017). Therefore, research relating to feed manufacture and feed quality has the potential to have a dramatic impact on commercial integrator production costs and ultimately reduce the cost for the consumers. During the pelleting process, mash feed is subjected to conditions of high moisture, pressure, and temperature; thus, adding additional costs and variables to the feeding process. Commercial poultry feed mills (in general) utilize the same equipment to accomplish the pelleting process. However, due to variations in equipment manufacturers, the age of the mill, ingredients in the diet, feed throughput demands, ambient temperature, and others, the quality of the feed produced at a given mill and at a given time may vary (Buchanan et al., 2010). Feed quality, also referred to as durability or pellet quality is defined as how durable a pellet responds to intense handling and transportation from the time the feed is manufactured to the point of consumption. This is important to consider because finished feed at the feed mill will have to be loaded onto a truck, transported to a farm, augered into a feed bin, and finally augered throughout the feed system at a house before reaching the point of consumption. The delivery process adds severe stress on the feed and pellets often dramatically deteriorate before being consumed, placing more importance on creating high-quality feed at the feed mill.
The starter growth phase represents a critical stage in a broiler’s lifecycle. During this time, gastrointestinal tract formation is rapid and extremely critical for improvements in digestion to maximize performance (Lilburn and Loeffler, 2015; Willemsen et al., 2008). While ample research on feed quality improvement on broiler performance exists, research pertaining to feed quality presented in the early growth phases is lacking. Determining the optimal starter feed particle size may optimize starter performance and impact the overall performance of the bird (Lemons et al., 2016).
Layers and Egg production. To increase the laying persistency with high-quality eggshell, researchers in Hawaii have been working on the genetic and hormonal regulation of egg production and discovered several novel genes and biological pathways involved in the eggshell biomineralization (Sah et al., 2018). Further, we are conducting studies on the nutritional programming to mitigate the heat stress in laying hens. PI has been developing collaborative research with the team of NE-1942 to investigate the effects of different housing, lighting and nutritional interventions on the oviductal functions.
Recently, for layers, there has been increased interest in feeding varied particle sizes of a Ca source to laying hens in order to maximize Ca absorption. Calcium is one of the essential minerals that determines the eggshell quality. There has been an increased interest in research on the Ca metabolism of layers in their second production cycle. Especially, when hens are molted and produce egg size much larger than the previous, which is not preferred by consumers. Also, the presence of soft-shells, cracks, breakage, and irregularities in eggs lead to significant economic losses. Therefore, the Ca requirement, as well as metabolism, becomes significant. Until now, the inclusion of Ca in a fully-grown laying hen is as high as 5%. However, research has shown that we cannot achieve a higher distribution of Ca in the bones or the eggshell by increasing the Ca inclusion level higher than 5%. Therefore, strategies must investigate how to maximize Ca utilization; one approach is to feed several particle sizes of the Ca source (fine, medium and large) and carrying out feeding two times a day. It is known that hens have a preference for larger particle sizes. When larger limestone particles with lower solubility are used, the layer digestive tract can maintain Ca blood levels even during the night, due to its gradual solubilization and availability (Pizzolante et al., 2011). However, research in this area is lacking, and it is unknown whether providing fine Ca particles at a different time of the day will provide an equivalent egg quality to that of a hen provided a fine Ca particle provided in a single meal.
Along a similar thread, supplying a single meal to hens has often resulted in the selection of larger particles thus leaving fine particles in the evening meal. When this occurs, hens cannot have longer retention of Ca in their gizzard that allows assisting in eggshell formation. Also, Ca intake is higher later in the day. Hens will have higher protein and energy requirements during the morning when albumen is started to form and a higher Ca requirement during the evening and night when eggshell formation takes place. Phosphorus is also required mostly during the morning as it is mainly needed to reabsorb Ca to the medullary bone. When hens are allowed to have their meals separately in the morning, containing 40% of the daily amounts with higher energy, protein, and P (lower Ca) and evening, containing 60% of the daily amount with higher Ca (lower AME, CP, and P) (Ahmad and Balander, 2003). The potential impact of split feeding will involve a reduction in nitrogen, P and Ca in hen excreta due to the adequate supply of the nutrients.
Although the feed provides most of the eggshell calcium, about 25-40% is from skeletal stores (Mueller et al., 1964). At the onset of sexual maturity, calcium reserves in the medullary bone are rapidly turned over at a rate of 1.6 g/day until the end of the laying cycle (Whitehead and Fleming, 2000). As the hen ages, medullary bone is significantly expanded at the expense of structural bone. Depletion of structural bone damages skeletal quality by reducing bone mineral density and eventually causing osteoporosis (OS) in laying hens (Thorp, 1994). Osteoporosis increases skeletal fragility and risk of fracture, leading to broken bones and reducing welfare. Broken bones associated with OS can occur in 30% of hens during their lifetime (Budgell and Silversides, 2004; Fleming, 2008). The condition (OS) is responsible for 20-35% of all mortality among aged hens (Anderson, 2002; Whitehead, 2004). Management techniques, such as providing perches or diet modification, have been used in attempts to prevent OS, with limited success. For instance, keel bone fracture is in approximately 90% of caged hens regardless of perch usage (Hester et al., 2013). Gut microbiota can regulate the host’s health through the integrity of metabolic, immune, endocrine and neural activity (Kelly et al., 2015; Yarandi et al., 2016). In addition, it has been proposed that OS is not caused by Ca deficiency in chickens; and various nutritional treatments, including Ca supplementation, increase the amount of medullary bone, but have little impact on the loss of structural bone (Whitehead and Fleming, 2000; Webster, 2004). Multiple factors have been involved in increased osteoclastic resorption. In general, gut microbiota positively impacts skeletal health and prevents pathological damage such as OS in rodents (McCabe et al., 2015; Villa et al., 2015; Hernandez et al., 2016), and may have similar functions in poultry (Abdelqader et al., 2013). In a pilot study, the hippocampus levels of IL-6, a pro-inflammatory cytokine promoting osteoclast formation (osteoclastogenesis) and function, was reduced, but the bone mineral densities in both the tibia and femur were increased, and gait was improved in Bacillus subtilis fed chickens following heat stress at 32oC for 28 days. Therefore, these data suggest that more research is warranted to explore the potential relationship of commercial probiotics and their use to potentially reduce OS in commercial laying hens; these data are even more vital as more hens are being reared in alternative housing systems, where welfare may be compromised (i.e., keel bone fracture).
Although dietary energy is the most expensive component of laying hen diets, energy requirements have not been recently validated in the caged-layer system, let alone in the newer (higher activity) housing systems that are being implemented in the US. Therefore, we propose to re-validate laying hen energy requirements using modern genetics and varied housing conditions using a combination of egg production, feed conversion and body composition to better understand the fate of dietary energy in the egg production cycle and to use those data to streamline evaluation of dietary manipulation of laying hen diets including the use of non-traditional feed ingredients and feed additives.
2b. Disease Control and Microbiology
Avian Influenza (AI) and Depopulation. The depopulation and more importantly, the need for timely depopulation (USDA 2015) is one of the most critical control measures outlined in “The Red Book” to contain a highly pathogenic avian influenza (HPAI) outbreak. Delayed depopulation is a primary predictive factor in determining the size of an outbreak based on the 2003 H7N7 highly pathogenic avian influenza outbreak in the Netherlands (Le Menach et al. 2006). As such, the poultry industry is examining all methods to depopulate infected birds and those at the same complex within 24 to 48 h in a manner to minimize animal suffering (Eberle-Krish et al., 2018; Eberle et al., 2018). In the Egg Industry, there is no current methods of CO2 Kill Carts, CO2 infusion of an entire production house, and firefighting foam to asphyxiate the flock that will accomplish this. In addition, each of these systems can become quickly overwhelmed in the face of millions of turkeys or broilers in need of being depopulated. The AVMA and AAAP have indicated the need for definitive and decisive depopulation measures to end the suffering of diseased poultry and to prevent the infection of healthy poultry flocks. Depopulation is a crucial factor in eradicating this disease from the U.S. effectively.
Dr. John Clifford, Deputy Administrator of Veterinary Services for USDA APHIS, supported ventilation shutdown (VSD) as the fastest and the most humane method for depopulation (USDA, 2015). There is a reality that in order to ensure the health of non-infected animals and minimize the potential of HPAI to spread to other species, rapid depopulation is essential. It should be a primary goal that depopulation (euthanasia) methods strive to meet the highest standards within the context of the extreme need in which VSD would be used.
Webster (2007) reviewed potential depopulation methods which could be employed in emergencies with the conclusion that there does not appear to be a suitable method for mass depopulation. Existing methods required extensive human-bird interaction while the birds were in an infective state; thus, increasing the transmission potential. Additionally, the existing methods required on-site resource availability for the 40,000 gallons of water for foam or 24,000 lb. of liquid CO2 to depopulate a moderate size cage layer facility. Also, of concern, natural disasters which have resulted in power outages and failure of backup generator systems have illustrated the effectiveness of VSD+ (supplementation of heat or CO2) in mass death of a flock (Eberle-Krish et al, 2018; Anderson, 1982, personal experience). VSD+ also can meet the 24-hour depopulation goal set forth by the memorandum distributed by USDA, (9/18/2015). The VSD+ methods need to be evaluated in a manner similar to the data procurement for different gas mixtures (Gerritzen et al., 2004), high expansion foam (McKeegan et al., 2013) and liquid CO2 (Turner et al., 2012; Mitchell, 2015). The work on ventilation shut down and carcass disposal on commercial large hen farms will help us manage the difficult reality of catastrophic disease outbreaks that require the depopulation and disposal of large number of birds. In 2014 and 2015, when AI destroyed over 50 million birds in the U.S., smaller flock sizes of meat birds in floor houses could be euthanized using firefighting foam, and composted in-house. However, large hen complexes with a million or more birds had no viable options at the time for either euthanasia or disposal. Therefore, it is of the utmost importance to evaluate the merits and pitfalls of these procedures (VSD, VSD+, and ensiling) and to document the equipment, personnel, and costs associated with these practices to ensure those in the poultry industry are prepared for the next catastrophic disease event.
Bacterial Infections of Poultry and Control. Poultry is the second most commonly consumed meat worldwide, and consumption of poultry is predicted to increase more quickly than any other major meat (Chai et al., 2016). The United States is the largest consumer of poultry meat; each inhabitant consumes 49.8 kg per year (Rouger et al., 2017). In poultry, bacterial infection is one of the leading causes of mortality and morbidity, and it threatens poultry welfare and food security with associated economic losses in the poultry industry throughout the world (Zhuang et al., 2014). The most common infections are associated with E. coli, Clostridia, Eimeria and others. The economic losses of bacterial infections in poultry are mainly due to decreased hatching rates and egg production, lowered production, increased mortality, increased carcass condemnation at slaughter, and increased costs associated with treatment and prophylaxis (Guabiraba and Schouler, 2015).
Poultry are major reservoir hosts for many foodborne pathogens, such as Salmonella and Campylobacter (Mor-Mur and Yuste, 2010; Kiess et al., 2010). Therefore, the microbial safety of poultry meat products is an important issue for human health, when considering the increases in consumption and production. Reducing the populations of these pathogens in the chickens, turkey, and layer intestinal tract would lead to reduced contamination of poultry and products. Due to the problem of antibiotic resistance in bacteria, the industry is currently exploring several alternatives to antibiotics to control these pathogens. Since humans can contract these pathogens by consuming contaminated chicken meat and eggs, multifaceted approaches are required to control them, with steps being investigated from before hatch, in the house, as well as in the processing environments.
The genetic diversity of a pathogen population can strongly influence disease severity and spread (Kennedy and Dwyer, 2018). Different isolates may harbor different virulence factors; any one of these may induce severe infections. Therefore, the diversity among strains of pathogenic bacteria has been a major obstacle for scientists trying to understand pathogenesis (Dziva and Stevens, 2008). This gap in knowledge also makes it challenging to design drugs that target all pathogens and diagnose the impact those drugs have on the pathogenic strains. An understanding of the mechanisms determining pathogen variation might, therefore, lead to novel interventions, reducing the toll of infectious diseases.
Biochar has also been studied extensively as a biofilter for the removal of E. coli from stormwater and soils. One study found that sand amended with 5% by weight of biochar retained up to three orders of magnitude more E. coli when compared to sand only and it also prevented mobilization during successive intermittent flows (Mohanty et al., 2014). Transport of E. coli through the water-saturated soil was five orders of magnitude lower than control when amended with 10% by weight of biochar (Bolster and Abit, 2011). Overall, the literature suggests that biochar is effective at binding E. coli in both stormwater and soil applications (Abit et al., 2014; Mohanty et al., 2014). Studies that focus on the ability of biochar to prevent the mobilization of the E. coli in poultry houses needs to be explored.
Footpad Dermatitis and Control. Litter moisture plays a crucial role in poultry performance and health. Excessive moisture levels in the litter have been documented to produce more severe footpad dermatitis in poultry (Costa, et al., 2014; Mayne et al., 2007). Footpad dermatitis has been identified as a welfare issue in poultry production systems (Erasmus, 2018; Wyneken et al., 2015) but flocks vary in the level of severity (Krautwald-Junghanns et al. 2009). While controlling litter moisture seems relatively straightforward, litter condition is the result of a set of complex conditions intertwined, including ventilation, heating, feed, nutrition and gut health (Shepherd and Fairchild, 2010). With the Veterinary Feed Directive (VFD) limitations on feed use of antibiotics and consequent increase in antibiotic-free (ABF) production, disturbances to the gut and excreta composition/moisture probably will increase (Collett, 2012). In bedded systems, the extent to which moisture removal can occur via ventilation is limited. Modifying litter composition through feed and litter treatment is another option. For feed, examining the role of feed, especially relative to ingredient/mineral content is essential. Work by Hoeven-Hangar et al. (2013) has indicated the following feed minerals, and nutrients were associated with excreta moisture: Na, P, and Ca, along with protein.
Litter moisture can also be modified with litter treatments, as many have a drying effect and modify microbial composition. Likewise, products administered through feed/water can help stabilize gut health and improve litter conditions (Reisinger et al., 2011). Another way to reduce litter moisture is to collect excreta separately. This can be done with the use of slotted flooring. However, past work had indicated difficulty raising poultry on slotted-only flooring. Other previous research has indicated that a partial slotted flooring is possible for use in rearing turkeys (Chen et al. 1991; Noll et al. 1997). These data suggest that more research needs to be done for broilers and turkeys to determine economically feasible ways to manage litter moisture in ABF production systems.
Red Mite Control. As the commercial layer industry in the U.S. moved to cage production, many improvements were seen, especially in the reduction of pests, such as mites. The red mite, Dermanyssus gallinae, is a devastating ectoparasite of domestic poultry. In Europe (e.g., reviewed in Sparagano et al., 2014; Sigognault Flochlay et al., 2017), Dermanyssus mites flourish in cage-free poultry operations. While several factors have been attributed to the increase in red mite infestations in Europe, such as climate change and removal of chemical treatments, the rapid change of laying hen housing systems from cages to cage-free is considered the primary cause (Höglund et al., 1995; Sparagano et al., 2009; Sigognault Flochlay et al., 2017). Several European countries have recently reported infestation rates over 90% (Mul, 2013), and economic losses have been estimated around €231 million (over $285 million; Sigognault Flochlay et al., 2017).
Dermanyssus gallinae live off-host in cracks and crevices (perches and nest boxes) and travel at night to feed on the blood of birds. A blood meal is required for immature and adult life stages to develop and reproduce. Wire cages that have housed commercial egg-layers for decades do not offer the proper habitat for these mites. However, Dermanyssus mites did not completely disappear from the U.S.; they have persisted in wild bird nests and also in backyard chicken flocks (Murillo and Mullens, 2016). With the rapid changes currently in the U.S. egg industry of moving from cages to cage-free housing, infestations like those seen in Europe are highly probable. Therefore, it is imperative to understand how to identify, treat and eradicate these mites from flocks before an infestation.
2c. Physiological Response to Environment and Welfare
Litter Restriction in Cage-Free Aviaries. An increasing number of producers in the U.S. laying hen industry is transitioning from conventional cages to cage-free housing systems, in response to customer and legislative demands for eggs from welfare-friendly production systems. Cage-free systems come in many forms; however, a common style is the aviary, which consists of elevated (sometimes enclosed) tiers, nests, and perches, and provides access to a litter-covered floor area in which hens can perform behaviors such as foraging and dust bathing (Campbell et al., 2016; Odén et al., 2002). A major concern specific to cage-free housing systems is the undesirable consequence of eggs laid in the litter areas, which results in lower quality eggs, more labor required to collect eggs, and prolonged contact time between hens and eggs that lead to more damaged eggs. Initial exclusion from litter helps train birds to use nests as they develop egg laying routines, minimizing floor-laid and damaged eggs later when hens are given litter access (Alm et al., 2014). Partial litter restriction is permitted by several industry guidelines in the U.S., including those of the United Egg Producers (UEP, 2016). Several studies (Gunnarsson et al., 2000; Bestman et al., 2009) have suggested that litter restriction may have negative effects on bird welfare; however, a recent study examining partial litter restriction following 4 weeks of initial restriction found no adverse impacts on physical indicators of hen welfare (Oliveira and Xin, 2018).
Keel-bone Fractures in Cage-Free Birds. Keel bone fractures have been identified as a serious welfare concern due to their high reported prevalence and association with pain (Nasr et al. 2012a; Nasr et al. 2013a; Nasr et al. 2015) and reduced egg production efficiency and quality (Nasr et al. 2012b; Nasr et al. 2013b). With only a handful of U.S.-based research teams working to understand and mitigate risk factors associated with the development of keel bone fractures, minimal information currently exists on the extent of the issue in U.S. cage-free systems. However, over the next 7 years, the U.S. egg industry is projected to move away from its reliance on conventional cage housing (associated with a relatively low keel bone fracture incidence rate) toward cage-free housing (associated with keel bone fracture rates of up to 90% by 60 to 70 weeks hen age). Understanding the causes of keel bone damage and management strategies that may mitigate keel bone fracture risk will assist US producers with successfully implementing cage-free systems in their operations while reducing keel bone fracture risk.
Role of Space on Behavior. Aviaries typically house large groups of hens together, offering more space per bird in comparison to conventional cages as well as resources intended to meet hen behavioral needs (Cooper and Albentosa, 2003). However, merely providing more space does not ensure hens will be able to perform essential behaviors. Therefore, it is important to understand factors that may interfere with hens’ abilities to use resources and perform key behaviors, which would reduce potential welfare benefits of transitioning hens to cage-free housing.
In a production setting, hen housing guidelines and codes of practice frequently include recommendations on the amount of space or resource allocation per hen with the intent of allowing expression of behavioral needs such as standing, lying, perching, wing flapping, and dust bathing. If hens cannot perform these behaviors, possible results are frustration, injury, or deprivation (Vestergaard et al., 1997). For example, hens will work to gain perch access, particularly at night (Olsson and Keeling, 2000), and if sufficient perch space is provided for each hen, hens may spend 100% of their night perching (Appleby et al., 1993; Olsson and Keeling, 2000). Dust bathing is also well documented as having a positive benefit for hens. This “high priority behavior” (EFSA, 2015) is a maintenance behavior that can improve feather condition and dislodge skin parasites (Weeks and Nicol, 2006). Dust bathing is regarded as a comfort behavior, and situations that do not allow hens to dust bathe may cause stress (Vestergaard et al., 1997; Weeks and Nicol, 2006).
However, relatively little research has directly examined the amount of space required for laying hens to perform these key behaviors (Dawkins and Hardie, 1989; Mench and Blatchford, 2014; Spindler et al., 2016) or postures, such as standing and lying, in which hens spend much of their day (Keeling, 1994; Channing et al., 2001). Recently, we developed a unique approach to determine the physical space required by hens to perform key behaviors in commercial style aviaries and reported differences in space requirements between four different strains to perform certain behaviors (Riddle et al., 2018). We found that popular commercial hen strains such as Hy-Line W36, Bovan Brown, Hy-Line Brown, and DeKalb White required more physical space to perform key behaviors such as standing, lying, dust bathing, wing flapping and perching than proposed by housing guidelines and codes of practice or reported by previous research (Dawkins and Hardie, 1989; Mench and Blatchford, 2014; UEP, 2017; Spindler et al., 2016). For instance, United Egg Producer (UEP) standards recommend 15 cm perch space per bird and that 50% of the flock be able to perch simultaneously on elevated perching structures (UEP, 2016). In our study, hens occupied more than 15 cm of perch width, with the narrowest of the strains (DeKalb White) we assessed occupying an average of 18 cm.
However, there is more to a hen being able to perform a behavior than just the amount of space physically taken up by her body during the behavior. For example, we must also consider how many hens will engage in a given behavior simultaneously, a phenomenon called flock synchrony (Odén et al., 2002; Appleby, 2004; Mench and Blatchford, 2014) as well as how much space a hen will place between herself and others in her group when performing a behavior (Keeling, 1994; Collins et al., 2011). For example, hens have more inter-bird space when walking than when standing, and when pen space per hen decreases beyond a certain point hen decrease the amount of walking (Keeling, 1994). Aviary systems are designed to promote behaviors shown by hens and provide more space per enclosure and per hen than conventional cages, this larger space allowance. However, to understand if the amount and type of space provided by aviaries allow hens to meet behavioral needs, space needs to be considered in the context of the presence of other birds, the frequency at which behaviors are performed, and behavioral space.
Broiler Enrichment. An area of animal welfare with poultry even less explored is broiler enrichment. Although several animal welfare certification programs have encouraged the scattering of feeding as a form of broiler enrichment, there is little evidence to support that broilers would benefit from this practice. The idea that the scattering of whole grains or other food items can be used as a form of environmental enrichment is grounded in the assumption that foraging behavior is important for broilers and will be readily expressed if the scattered substrate is provided. However, what constitutes a normal behavior (i.e., a behavior that is important to the animal) can be altered by selective breeding and environmental conditions. It has been suggested that along with selection for fast growth the broiler behavioral repertoire has shifted towards the performance of behaviors that allow the birds to conserve their energy. For example, previous research has shown that broiler chickens are less willing to work for food when a freely available food source is available as compared to laying hens or red jungle fowl, their wild counterparts (Linqvist et al., 2002; 2006). Additionally, provision of foraging or pecking materials as an enrichment in the presence of freely available food has not been associated with benefits, such as increased locomotion or improvements in leg condition (Guy and Wright, 2003; Pichova et al., 2016). Together, the results of these previous studies put into question whether scatter feeding has the potential to be an effective form of broiler enrichment. If scattering of feed is to be recommended as a form of environmental enrichment for broilers its implications for broiler welfare must first be examined.
Heat Stress. Climate change-associated hot weather is an increasing threat to the animal production industries (Hajat et al., 2010). When ambient temperature increases toward the critical upper thermal limits in animals, heat gain exceeds heat loss, resulting in hyperthermia. As one of the critical reasons causing pathophysiological damage, hyperthermia leads to oxidative stress, i.e., disturbance in the pro-oxidant/antioxidant balance in favor of producing reactive oxygen species (ROS; Droge, 2002). Increased ROS contributes to cytotoxicity, causing cell death, tissue injury and organ damage resulting in increased morbidity and mortality (Solymosi et al., 2010). Estimated heat stress (HS) related losses for livestock was estimated at $2.4 billion in the U.S. (St-Pierre et al., 2003).
Heat stress is of great concern for poultry operations, as chickens have been continuously selected for fast growth with much heavier harvest weight (broilers) or increased egg production (layers), which may increase susceptibility to HS by shifting energy from maintenance in response to stressors to productivity (Geraert et al., 1993; Berong and Washburn, 1998). When the temperature exceeds the comfort level of a bird, behavioral and physiological changes occur that are accompanied by changing physiological homeostasis and oxidative balance (Mujahid et al., 2005; Lin et al., 2006). These changes reduce feed intake and nutrient metabolism while increasing morbidity and mortality. Thus, it is critical to increasing their heat tolerance. Gut microbiota reacts to various internal and external stimuli, influencing brain function in regulating the host’s health via the gut-brain axis (Kelly et al., 2015; Yarandi et al., 2016). Some probiotics have demonstrated beneficial effects on alleviating oxidative stress and related tissue damage in rodents (Pandey et al., 2015; Lei et al., 2015). These data suggest that supplementing probiotics may provide a new strategy to inhibit HS-associated damage to health and welfare in poultry.
Housing Systems. At the moment, the modern laying hen industry is exploring alternative types of hen raising systems, cage-free that includes both aviary and floor pen. With the demand of changing the egg industry to cage-free by 2025, as well as interest from consumers, hens have begun to be provided more space in addition to access to outdoors (Mench et al., 2011). However, there needs to be a more substantial amount of research data that includes production, feed conversion and health of hens that will allow making a broader comparison between hen housing types before the world embraces cage-free as its best option (Anderson, 2018). Egg parameters (i.e., egg mass) and hen parameters (i.e., mortality and hen weight) have been found to vary between the three types of housing: floor pen, traditional, and enriched cage, providing conflicting results (Hidalgo et al., 2008; Singh et al., 2009; Sherwin et al., 2010). Along with the production, bone health and bone biology are the two significant concerns to the poultry industry. Bone weakness is an important issue for the egg layer industry, as it directly relates to hen pain and affects the egg production (Webster, 2004). Research has shown that hens housed in cage-free aviary have a higher rate of keel bone damage due to failure in landing and collision with housing structures (Campbell et al., 2016). There is a scarcity of data on keel and tibia bone health in hens housed in floor pens and the other hen house types.
Aspects of production systems interact with the physiology of the bird and subsequent performance and well-being. Feather pecking (FP) and cannibalism occur in all the current egg production systems, including cage and free range, which is an important cause of mortality in untrimmed chickens. Beak trimming (BT) is a common practice to prevent FP and cannibalism. Light spectrum appears to have an impact on FP and Cannibalism. Research needs to be conducted to develop a bird-friendly method for preventing FP and cannibalism, eliminating BT. Another area of concern in laying hen production systems are bone fractures, and the effect of pullet rearing on skeletal quality during egg laying has not been extensively studied. Additionally, perch use/design has not been extensively studied for its impact on bone mineralization during rearing.
Next Section - "To Be Continued" in attachments section.
Objectives
-
Integrating technological advances into poultry systems.
Comments: This will include collaborative research on incorporating engineering and technology to enhance system efficiency and sustainability through infrastructure development of blockchain production. -
Establishing and adopting husbandry practices to a changing industry landscape.
Comments: This collaborative research will encompass a multi-disciplinary approach to create a resilient poultry production system through optimal management of inputs and outputs in an ethically responsible manner.
Methods
Objective 1
1a. Engineering and Technology
Studies will establish methods that use novel technologies to enhance poultry production systems by integrating engineering, physiology, and ethology. State-of-the-art sensors, current data acquisition systems, and modern data analysis technologies (e.g., image processing and data mining) will be employed to better understand and improve housing ventilation, acoustic conditions, air quality, and lighting environments. Real-time monitoring systems using electronic and visual sensing systems will be developed to autonomously monitor poultry environmental interactions, bird behavior, and welfare. Radio-Frequency Identification (RFID) technology to individually identify bird will be used to develop methods for commercial applications. In addition, a new style of perch will be developed such that the antenna will be integrated directly into the perch so that perches at different locations and heights within the cage-free housing system can be instrumented. Hen behavior at different locations (perch, drinker, nest box, feeder, etc.) will be recorded.
Wearable sensor technology for laying hens will be developed and evaluated in alternative housing systems. Varying behavioral states will be validated through video recordings and used to correlate individual differences in behavior correlated to parasite infestation. Prototype acoustic sensors will also be used to track the use of space by laying hens housed in alternative housing systems, particularly in free-range and pasture-based systems.
A new research farm at Iowa State University will enable pilot-scale testing of technologies and provide a platform for studying and demonstrating the different management strategies under controlled conditions with commercially relevant laying hen environment and housing styles. Prior to populating, this farm will undergo an extensive commissioning process to ensure the ventilation, heating, and cooling systems are all performing to their designed specifications. In addition to the commissioning process, the laying hen rooms will be instrumented with various types of gas, particulate matter, and thermal environment sensors to provide robust feedback to researchers and enable accurate comparison of different treatments. The addition of these air quality and thermal parameters will add a new level of insight into potential linkages between nutrition, genetics, well-being, and environment interactions. Sensors will be placed where the fresh air enters the rooms, throughout the room to capture the spatial distribution, and at the room exhaust. After completion, this technologically advanced poultry research facility will help further research in management schemes, air quality, behavior, and welfare.
1b. Environmental Control and Management
Management strategies will be evaluated to determine their effectiveness at reducing floor eggs, maintaining flock uniformity, laying percentage, mortality, improving the well-being of the hens. Such strategies to be tested include, but not limited to, lighting intensity, spectrum, and photoperiod, litter depth, and moisture, robotics, feeding events, system layouts, and thermal conditions. The performance of high-efficiency fan shades in reducing light leakage and spatial variation of illuminance in commercial broiler houses will also be evaluated. Studies to mimic the effective photoperiod due to light ingress through tunnel fans and evaluate subsequent broiler performance are also proposed.
Mitigation strategies for gas (mainly ammonia) and particulate matter (dust) emissions and generation will be developed and tested using existing technologies. For example, bio-aerosol samplers will be used to quantify airborne pathogens and examine their biological responses to environmental stimuli. In addition, portable monitoring units will be used to determine the effects of atmospheric concentrations of CO2 on turkey and broiler production performance, particularly during the brooding phase. Several studies will compare higher than normal CO2 levels vs. levels typically seen in a commercial broiler or turkey brooder unit. A unique comparison of these atmospheric conditions within cage-free aviaries will be determined.
Studies will also evaluate the following conservation advantage of vegetative buffers: 1) improved air quality, 2) improved water quality, 3) screen and/or landscape the poultry farm at the urban/rural interface, 4) improve farm energy efficiency via shade, windbreaks, and snow fences, 5) provide biomass for bedding and fuel, and 6) provide cover/shelter for outdoor access birds. Vegetative buffers have been a useful tool at the urban/rural interface to deflect nuisance complaints from neighbors and mute the odors and dust associated with commercial poultry production. However, future studies will attempt to determine their merits for modifying the climate around the poultry houses (shade, cooling the barns in summer and windbreaks, protecting the birds from winds and snow in winter).
Ventilation shut down (VSD) in commercial laying hens will be studied as a means to control disease during catastrophic events such as AI. Landfilling, rendering and ensiling of the hen carcasses will be examined to better understand the logistics, labor and equipment requirements associated with these actions and their potential merits, shortcomings, and costs. The effects of brooding temperature on turkey poult and broiler growth parameters will be researched as well.
Objective 2
2a. Nutrition Strategies and Feed Manufacture
The NOSB approved the utilization of synthetic methionine for poultry in 2015 at new limits between 2 and 3 lbs./ton. Recently, they have also committed to phasing-out synthetic methionine for organic poultry production and encourage aggressive research on natural alternative sources of methionine. Studies will be conducted to explore novel organic feed ingredients and feeding strategies that can help satisfy the methionine requirement of poultry in the face of dietary limits on synthetic methionine established by the National Organic Program. Research identifying viable, concentrated, highly available sources of methionine for poultry production is essential for the sustainability of the organic market, bird welfare, and the environment.
Poultry nutrition programs using alternative feedstuffs and pasture-based production systems will also be examined with the goal of reducing the cost of poultry production and its environmental impact while enhancing overall sustainability. The feeding of all-vegetable diets to poultry presents another set of challenges such as the need for high inclusion levels of plant protein sources (soybean meal). Plant protein sources typically are less concentrated in essential amino acids and contain many different types of anti-nutritional factors. Research will be conducted to examine nutritional needs with all-vegetable diets. Also, feeding trials with insect meals and other novel feedstuffs for both broiler and laying hen diets will be studied for their nutritional constituents, availability, and impact on production, economics and poultry product quality.
Additionally, researchers will investigate the optimal feed particle size for broilers in the starter period in order to improve overall performance. Diets will be of common formulation and initially pelleted at one time, and particle size will be manipulated via roller mill. Self-adaptation to varying particle sizes will be investigated, as well as broiler performance (i.e., feed intake, feed conversion, average bird weight, mortality, and others), gastrointestinal tract morphometry, as well as the rate of digestion. After the feeding the experimental diet for 14 days, birds will be fed a nutritionally common diet until day 63 (to observe carryover performance effects of starter feed particle size).
The role that nutrients play in egg size, eggshell strength, and internal egg quality is critical to the layer industry. In response, egg production, egg quality, and bone quality (strength, ash, and dimensions) in White Leghorns W-36 and Hy-Line Brown housed in conventional, enriched, and floor pen with outdoor access will be determined. Researchers will also investigate the role of Ca particle size and source (limestone and oyster shell) in eggshell quality, split feeding of Ca in laying hens and its role on egg and eggshell quality, and Ca and P digestibility in hens at different phases of production. Energy requirements and utilization will be re-evaluated for laying hen production systems. The effect of traditional broiler and layer diets (corn and soybean meal-based) versus feed supplemented with varying combinations of carbohydrase and phytase enzymes will be studied for their effect on production, litter condition, and energy utilization.
Osteoporosis in laying hens increases skeletal fragility and risk of fracture, leading to broken bones and reducing welfare. In addition, heat stress in broiler chickens can lead to pathophysiological damage, hyperthermia, cell death, tissue injury, and organ damage. Heat stress has resulted in an estimated as a $2.4-billion-dollar loss for livestock in the U.S. Researchers in this project have proposed to study the effects of probiotics on the gut microflora laying hens and broiler chickens and associated improvements in skeletal health and heat tolerance. Hens will be evaluated for mortality, body weight, egg production, feed efficiency, skeletal health, behavioral, immune and physiological response. Broilers will be evaluated for mortality, body weight, feed efficiency, skeletal health, behavioral, immune and physiological response.
Based on the results and success of the earlier studies, researchers in this in ovo proposal will evaluate the effectiveness of different lactic acid bacteria (probiotic) either singly or in combination given at 18 days of incubation to fertile broiler or layer eggs on parameters as indicated. Fertile eggs will be prepared according to the methodology described (Macalintal et al., 2017a, b). The scope of the project will be expanded to include not only the assessment of gut health but more importantly, immune competence during immunosuppressive conditions such as bacterial, viral, or even coccidial challenges. This is crucial because of the non-AGP movement that the poultry and livestock industry is facing. At day of hatch, hatch parameters will be evaluated including hatch of fertile eggs and mortality. All hatched chicks will be fed either a wheat or a corn-soy based diets throughout the duration of the study i.e. 42 days. Production performance including body weight and feed intake will be assessed on a weekly basis such that feed efficiency can be calculated. Blood samples will be collected at different time points to assess immunoglobulin titers and lymphoblastogenic responses. To determine the influence of in ovo probiotic injection on bacterial population growth, in vitro Salmonella, E. coli, or Campylobacter challenges will be performed. Finally, post-hatch chicks will be evaluated post-slaughter at Day 42 to determine the extent to which in ovo administration of probiotic can help mitigate the immune challenges and antibiotic use.
2b. Disease Control and Management
The red mite, Dermanyssus gallinae, is a devastating ectoparasite of domestic poultry. Understanding the host-parasite interactions of the chicken red mite, as well as potential treatments that would be effective in alternative housing systems is critical. Flocks will be experimentally infested with red mites, and feeding habits, mite locations, hen welfare, and behavioral measures will be measured. The effects of treatments on parasite populations through mite counts will also be studied.
The inter-relationships of footpad dermatitis with turkey performance and health as modified through manipulation of feed and litter will be determined. Commercial market turkeys will be reared on feeds varying in nutrient density and supplements that improve nutrient digestibility and gut health. Within a factorial design, litter condition will be monitored or modified with different litter management techniques or treatments. In addition, a partial slotted flooring system will be designed based on varying the type of flooring and bird density. Turkey toms will be reared on different types of flooring constituting 25% of the floor area with the remaining area being bedded with wood shavings. Rearing density space will be varied around commercial industry standards to assess the impact on remaining bedding area.
Pre- and post-harvest interventions to control and/or eliminate pathogens, including multidrug-resistant bacteria, is vital to the overall safety of our poultry food supply. As a pre-harvest strategy, new antimicrobials to eliminate foodborne bacteria of zoonotic importance in poultry will be investigated (Nair and Kollanoor Johny 2018a, b). Innovative approaches will be developed, or existing approaches refined and characterized for their functionality in poultry (Nair et al., 2018a, b; Nair and Kollanoor Johny 2018a, b). As post-harvest antimicrobial interventions, phytochemicals and emerging alternatives will be studied to improve the microbiological safety of poultry meat and eggs. Results from these studies will lead to developing interventional food safety models in poultry. Moreover, interventions to alleviate dysbiosis caused by the pathogens in the gut will be characterized and developed for translation.
Biochar has been studied extensively as a biofilter for the removal of E. coli from stormwater and soils. One study found that sand amended with 5% by weight of biochar retained up to three orders of magnitude more E. coli when compared to sand only and it also prevented mobilization during successive intermittent flows (Mohanty et al., 2014). Based on its past success at pathogen removal, lab-scale and live studies will be conducted to evaluate the effects of biochar as a litter amendment on E. coli in the broiler litter matrix. The addition of biochar to an E. coli-colonized litter matrix and its effects on E. coli transmission to live birds will also be examined.
2c. Physiological Response to Environment and Welfare
Litter restriction during the middle of layer hen flocks as a means of reducing floor-laid and damaged eggs will be examined. Welfare measures, such as body weight, keel bone damage, foot health, and utilization of nest and litter areas will be measured for the litter-restricted birds. Birds’ litter access will be restricted at 54 weeks of age when ~60% of all eggs are being laid in the litter, at which time partial litter restriction will be implemented for a 4-week period. Under this scheme, doors to the litter areas will open automatically every day at 11:30 and close at 13:00. At the end of the 4-week restriction, hens will be again granted unrestricted access to evaluate if any effects of retraining are long-lasting. Egg production (i.e., total eggs laid in nests, enclosure, and litter; number of laid eggs versus number of damaged eggs), nest occupancy (number of hens in nest), litter utilization, and physical measures of hen’s welfare before, during, 1 week and 4 weeks after the litter restriction period will be evaluated.
Researchers will evaluate the influences of flock synchrony (number of hens simultaneously use a specific resource and performing the same behavior) and inter-bird distance (space a hen will place between herself and others in her group when performing a behavior), on hens’ ability to perform specific behaviors uninterruptedly (frequency and duration of behavior bouts). Video recordings made of hens on the litter will be used to capture hens while performing specific behaviors such as ‘lying’, ‘standing’, ‘perching’ and ‘dust bathing’ and ‘wing flapping’ on litter area and perches, while in their day-to-day aviary housing environment at manufacturer’s recommended stocking density. The number of hens on litter area and the number of hens performing selected behaviors at those times will be recorded from still images. Length and width of floor space, or width of perch space occupied by focal hens while performing selected behaviors, and inter-bird distances between focal and surrounding nearest hens during dust bathing will be recorded from each image (see Riddle et al., 2018 for details).
The prevalence of keel bone fractures among laying hens in U.S. cage-free production systems will be evaluated in relation to rearing and housing. The effects of vertical complexity during pullet rearing on the development of spatial acuity and keel bone fracture development will be tested in a series of experiments conducted at a poultry facility at UC Davis. The development of spatial abilities will be evaluated using a combination of cognitive tests, behavioral assessments, and evaluations of space use. Keel integrity of individual birds will be evaluated via palpation. In addition, practices that have been proposed as viable environmental enrichments for poultry will be evaluated. The willingness of broiler chicks to forage for feedstuff will be evaluated throughout the growing period. The effects of foraging enrichment provision on general activity and growth of the birds will also be evaluated.
2d. Housing Systems
The microbial profile in laying hens and pullets raised in cage house and cage-free by traditional culture and molecular method will be assessed. The profile of both live and euthanized hens will be studied. Traditional and molecular techniques will be used to assess the bacterial profile in manure samples and tissue samples from the ceca and ileum. In addition, a series of challenge studies will be performed in hens and pullets raised in cage house and cage-free environments using E. coli and Clostridium as pathogen models. Feed will be supplemented with prebiotics, probiotics, and essential oils. Respiratory and digestive organs will be periodically collected and tested, and overall bird health and performance will be evaluated between the two housing systems.
Economic analysis methods will be used to estimate demand and supply models for all type of eggs and predict the effects of imposing stricter animal welfare standards for producing eggs on the industry structure and the welfare of market participants (eggs consumers and producers). To evaluate the effects of the proposed regulation on market participants, a structural model for table eggs demand and supply will be developed. The proposed model will be a heterogeneous-product oligopoly model where egg producers compete with one another to sell differentiated table eggs to consumers. Table eggs in the model will be differentiated by the producer as well as how the laying hens are treated during production. Depending on how laying hens are treated, table eggs will be classified as conventional, free-range, cage-free, and certified organic. On the supply side of the model, producers compete with one another in a heterogeneous-product oligopoly fashion, and each producer decides the average price for each line of their table eggs in each time period, which will be one week in our analysis. On the demand side, consumers will choose which egg products to purchase. Once the model is constructed, and the parameters are estimated, various regulatory policy (counterfactual) scenarios will be simulated.
Measurement of Progress and Results
Outputs
- Enhanced collaborative research and development of research proposals for submission to federal agencies
- Successful extramural funding from industry and government programs that target specific collaborative research goals outlined in the project
- Support and training for undergraduate, graduate and post-doctoral students
- Data published in peer-reviewed high impact journals and presented at major scientific venues including association conferences such as Poultry Science, Worlds Poultry Science, International Poultry Scientific Forum, Institute of Food Technologists, International Association for Food Protection, and American Society of Agricultural and Biological Engineers
- Results are translated and available for various stakeholders including government agencies, poultry associations, poultry, and food industry and consumers. Different formats used include various media (print, web-based, webinar and other media specific to the target audience
- Optimize poultry house environments, monitoring and management will improve producer economic situations
- Performance and well-being data generated from alternative housing and pasture-raised studies will improve decision-making in a rapidly changing industry
- Data generated from nutrition trials utilized by producers for feed formulations, feed milling and feed suppliers
- Producers will have access to data related to poultry well-being to supplement decisions related to management practices
- Economic analyses of poultry production systems will improve industry profitability and consumer demand for poultry products
- Industry adoption of recommendations generated from research findings
Outcomes or Projected Impacts
- Improved productivity in broiler, turkey and layer chickens (feed conversion, weight gain, dozens of eggs produced)
- Improved disease management and food safety as a result of feed supplements or management practices
- Adoption of new engineering strategies and technology for reducing energy consumption and improve poultry house environments and food safety
- Improved poultry well-being through advanced monitoring systems and precision livestock farming
- Management recommendations on alternative housing systems for laying hens and other poultry that will assist producers in making sound business decisions on which systems are most suitable to their operations; and identify best practices to be adopted to allow these systems to function to full potential
- Strategized feeding programs with the use of alternative feed additives or feed ingredients or under constrained ingredient use while maintaining or improving broiler, turkey, and/or hen performance, well-being, and environment parameters
Milestones
(2020):• Complete lab-scale biochar study on E. coli mobilization in the litter • Complete performance study of high-efficiency fan shades in reducing light leakage and spatial variation of illuminance in commercial broiler houses • Complete study on litter restriction during the middle of layer hen flocks as a means of reducing floor-laid and damaged eggs • Complete evaluation of the influences of flock synchrony and inter-bird distances on hen behavior • Publish lab-scale biochar study on E. coli mobilization in the litter • Determination of optimal starter feed particle size for broilers • Development of rugged, portable monitoring units to determine the effects of atmospheric CO2 on turkey and broiler production performance • Conduct studies on mitigation strategies for gas (mainly ammonia) and particulate matter (dust) emissions and generation • Complete the examination of real-time monitoring systems using RFID technology and visual sensing systems to autonomously monitor poultry environmental interactions, bird behavior, and welfare • Development of a new perch system such that the antenna will be integrated directly into the perch • Commissioning of a new state-of-the-art poultry teaching and research facility at Iowa State University(2021):• Complete the live bird trials using biochar as a litter amendment • Studies to mimic the effective photoperiod due to light ingress through tunnel fans and evaluate subsequent broiler performance • Conduct studies using portable gas monitoring systems to compare higher than normal CO2 levels vs. levels typically seen in a commercial broiler or turkey brooder unit • Compare atmospheric conditions within cage-free aviaries using portable gas monitoring system • Publish information on real-time monitoring of poultry environmental interaction using RFID technology and visual sensing • Determination of optimal grower feed particle size for broilers • Complete studies on VSD in commercial laying hens as a means to control disease during catastrophic events such as AI. • Evaluate egg production, egg quality, and bone quality in White Leghorns W-36 and Hy-Line Brown housed in conventional, enriched, and floor pen with outdoor access • Conduct studies on the split feeding of Ca in laying hens and its role on egg and eggshell quality • Complete studies on antimicrobials to eliminate foodborne bacteria of zoonotic importance in poultry • Complete footpad dermatitis study in turkeys
(2022):• Evaluate the conservation advantage of vegetative buffers • Complete studies on Ca particle size and source (limestone and oyster shell) in eggshell quality • Complete challenge studies in hens and pullets raised in cage house and cage-free environments using E. coli and Clostridium as pathogen models • Complete the studies on host-parasite interactions of the red mite in chickens in alternative housing systems • Determination of optimal finisher feed form for broilers • Evaluate the effects of probiotics on the gut microflora of laying hens and broiler chickens and associated improvements in skeletal health and heat tolerance. • Complete the investigation into feeding strategies that that can help satisfy the methionine requirement of organic poultry
(2023):• Ca and P digestibility in hens at different phases of production • Complete the investigation into prototype acoustic sensors to track the use of space by laying hens in alternative housing systems • Identify optimal poultry nutrition programs using alternative feedstuffs and pasture-based production system • Determination of optimal finisher feed form for broilers • Complete feeding trials with insect meals and other novel feedstuffs for both organic broiler and laying hens
(2024):• Complete study on viable environmental enrichments for poultry • Complete feed supplement studies investigating carbohydrates and phytase enzymes on production, litter condition, and energy utilization • Determination of optimal withdrawal feed form for broilers • Develop economic analysis methods to estimate demand and supply models for all type of eggs and predict the effects of imposing stricter animal welfare standards on the industry structure and the welfare of market participants (eggs consumers and producers).
Projected Participation
View Appendix E: ParticipationOutreach Plan
Study findings will be disseminated promptly to the academic communities, industry stakeholders, and the general public through press releases, web publications, extension reports, presentations at professional conferences and industry educational workshop such as the Annual Industry Issues Forum organized by the Egg Industry Center (www.eggindustrycenter.org). The results will also be disseminated via extension publications (e.g., Animal Industry Report produced at ISU, NCLP and MT Reports at http://poultry.ces.ncsu.edu/layer-performance/), graduate student theses and dissertations, and peer-reviewed journal articles. While some of the outreach will be passive (user identifies information through web searching or other means), other outreach will be active (planned "events"). The "events" will tend to be planned by the Extension specialists participating in the project including seminars, field days, and workshops at which results of the research technologies will be discussed/disseminated to poultry producers. On-farm demonstrations will be conducted when feasible to allow farmers to see firsthand the results of the research in conjunction with traditional producer education programs. Members of the group will identify relevant information to share with interested clientele groups within their states or regions. Engagement of the poultry industry stakeholders (UEP, National Chicken Council, National Turkey Federation, USPEA, and others) will allow for input on the relevance of research being conducted and provide feedback on strategic ways to actively share information as well as provide ideas on future research topics. We believe a partnership between industry, government, and academia must exist to improve poultry production systems and well-being.
Organization/Governance
The Technical Committee is responsible for the planning and supervision of the Multi-State Research Project. The membership of this committee shall consist of an Administrative Advisor, a technical representative of each participating agency or experiment station, and representative of the USDA Cooperative States Research Service. Each participating agency or experiment station is entitled to one vote. The Technical Committee shall be responsible for review and acceptance of contributing projects, preparation of reviews, modification of the regional project proposal, and preparation of an annual report. Each technical committee member will prepare annual written reports and distributed at the annual meeting. Annual reports will be compiled and distributed to Technical Committee members and Agricultural Experiment Station Directors. The Technical Committee will meet yearly and conduct an election for the office of Junior Executive. The position should alternate between Poultry Scientists and Agricultural Engineers. The person elected to serve as Junior Executive will rotate through the remaining offices of Senior Executive and Secretary and will serve as Chair in the fourth year. All voting members of the Technical Committee are eligible for office. The Chair prepares the meeting agenda and presides at meetings. The Chair is responsible for the preparation of the annual report. The Secretary records minutes and assists the Chair. The Senior and Junior executives help with policy decisions and nominations. The Technical Committee functions as a unit with sub-committees formed as necessary, which is, preparing nominations for elections.
Literature Cited
Abdelqader, A., A. R. Al-Fataftah, and G. Das. 2013. Effects of dietary Bacillus subtilis and inulin supplementation on performance, eggshell quality, intestinal morphology and microflora composition of laying hens in the late phase of production. Anim. Feed Sci. Tech. 179:103-111.
Abit, S. M., C. H. Bolster, K. B. Cantrell, J. Q. Flores, and S. L. Walker. 2014. Transport of Escherichia coli, Salmonella Typhimurium, and microspheres in biochar-amended soil with different textures. J. Environ. Qual. Abstract - Groundwater Quality 43:371-388.
Ahmad, H. A., and R. J. Balander. 2003. Alternative feeding regimen of calcium source and phosphorus level for better eggshell quality in commercial layers. J. Appl. Poult. Res. 12:509-514.
Allender, W. J., and T. J. Richards. 2010. Consumer Impact of Animal Welfare Regulation in the California Poultry Industry. J. Agric. Res. Econ. 35:424-442.
Alm, M., H. Wall, L. Holm, A. Wichman, R. Palme, and R. Tauson. 2015. Welfare and performance in layers following temporary exclusion from the litter area on introduction to the layer facility. Poult. Sci. 94:565-573.
Anderson, K. E. 2002. Final report of the 34th North Carolina layer performance and management test. North Carolina Cooperative Extension Service, North Carolina State University, Raleigh.
Anderson, K. E. 2018. Single Cycle Report of the Fortieth North Carolina Layer Performance and Management Test. Vol. 40, No. 4, http://poultry.ces.ncsu.edu/layer-performance.
Appleby, M. 2004. What causes crowding? Effects of space, facilities and group size on behaviour, with particular reference to furnished cages for hens. Anim. Welfare. 13:313-320.
Appleby, M., S. Smith, and B. Hughes. 1993. Nesting, dust bathing and perching by laying hens in cages: effects of design on behaviour and welfare. Br. Poult. Sci. 34:835-847.
Behnke, K. C. 1996. Feed manufacturing technology: current issues and challenges. Anim. Feed Sci. and Tech. 62:49-57.
Berong, S. L., and K. W. Washburn. 1998. Effects of genetic variation on total plasma protein, body weight gains, and body temperature responses to heat stress. Poult. Sci. 77:379-85.
Berrocoso, J. D., R. Kida, A. K. Singh, Y. S. Kim, and R. Jha. 2017. Effect of in ovo injection of reffinose on growth performance and gut health parameters of broiler chicken. Poult. Sci. 96:1573-1580.
Berrocoso, J. D., S. Yadav, and R. Jha. 2017. Nitrogen-corrected apparent metabolizable energy value of macadamia nut cake for broiler chickens determined by difference and regression methods. Anim. Feed Sci. Tech. 234:65-71.
Bestman, M., P. Koene, and J. P. Wagenaar. 2009. Influence of farm factors on the occurrence of feather pecking in organic reared hens and their predictability for feather pecking in the laying period. Appl. Anim. Behav. Sci. 121:120-125.
Blackmore, D. K. 1993. Euthanasia; not always Eu. Aust. Vet. J. 70:409-413.
Bolster, C. H., and S. M. Abit. 2011. Biochar Pyrolyzed at two temperature affects Escherichia coli transport through a sandy soil. J. Environ. Qual. Abstract - Groundwater Quality 41:124-133.
Buchanan, N. P., K. G. S. Lilly, C. K. Gehring, and J. S. Moritz. 2010. The effects of altering diet formulation and manufacturing technique on pellet quality. J. Appl. Poult. Res. 19:112-120.
Budgell, K. L., and F. G. Silversides. 2004. Bone breakage in three strains of end-of-lay hens. Can. J. Anim. Sci. 84:745-747.
Campbell, D. L. M., S. L. Goodwin, M. M. Makagon, J. C. Swanson, and J. M. Siegford. 2016. Failed landings after laying hen flight in a commercial aviary over two flock cycles. Poult. Sci. 95:188-97.
Campbell, D. L. M., M. M. Makagon, J. C. Swanson, and J. M. Siegford. 2016. Litter use by laying hens in a commercial aviary: dust bathing and piling. Poult. Sci. 95:164-175.
Chai, S. J., D. Cole, A. Nisler, and B. E. Mahon. 2016. Poultry: The most common food in outbreaks with known pathogens, United States, 1998–2012. Epidemiol. Infect. 145: 316-325.
Chang, J. B., J. L. Lusk, and F. B. Norwood. 2010. The price of happy hens: A hedonic analysis of retail egg prices. J. Agric. Res. Econ. 35:406-423.
Channing, C. B. Hughes, and A. Walker. 2001. Spatial distribution and behaviour of laying hens housed in an alternative system. Appl. Anim. Behav. Sci. 72:335-345.
Chen, F., S. L. Noll, C. J. Clanton, K. A. Janni, and D. A. Halvorson. 1991. Market turkey performance affected by floor type and brooding method. Appl. Eng. Agric. 7:606-612.
Chepete, H. J., H. Xin, M. Puma, and R. S. Gates. 2004. Heat and moisture production of pullets and layers with or without the contribution of feces (4720 RP-1044). ASHRAE Transactions 110:286-299.
Collett, S. R. 2012. Nutrition and wet litter problems in poultry. (Special Issue: Nutrition and pathology of non-ruminants). Anim. Feed Sci. Tech. 173:65-75.
Collins, L. M., L. Asher, D. U. Pfeiffer, W. J. Browne, and C. J. Nicol. 2011. Clustering and synchrony in laying hens: The effect of environmental resources on social dynamics. Appl. Anim. Behav. Sci. 129:43-53.
Cooper, J. J., and M. J. Albentosa. 2003. Behavioural priorities of laying hens. Avian Poult. Biol. Rev. 14:127-149.
Da Costa, M. J., J. L Grimes, E. O. Oviedo-Rondon, I. Barasch, C. Evans, and M. Nixon. 2014. Footpad dermatitis severity on turkey flocks and correlations with locomotion, litter conditions, and body weight at market age. J. Appl. Poult. Res. 23:268-279.
Czarick, M., and B. Fairchild. 2016. Is light from your tunnel fans determining your birds' day length. In Poultry Housing Tips. Vol. 28, No. 2. Athens, GA: University of Georgia Cooperative Extension Service.
Dawkins, M. S., and S. Hardie. 1989. Space needs of laying hens. Br. Poult. Sci. 30:413-416.
Dewey-Mattia, D., K. Manikonda, A. Hall, M. Wise, and S. Crowe. 2018. Surveillance for Foodborne Disease Outbreaks—United States, 2009–2015. MMWR Surveill. Summ. 67:1.
Donaldson, W. E., V. L. Christensen, J. D. Garlich, J. P. McMurtry, and N. C. Olson. 1995. Exposure to excessive carbon dioxide: A risk factor for early poult mortality. J. Appl. Poultry Res. 4:249-253.
Droge, W. 2002. Free radicals in the physiological control of cell function. Physiol. Rev. 82:47-95.
Dziva, F., and M. P. Stevens. 2008. Colibacillosis in poultry: Unravelling the molecular basis of virulence of avian pathogenis Escherichia coli in their natural hosts. Avian. Pathol. 37:355-366.
Eberle-Krish, Krista. N., Michael P. Martin, Ramon D. Malheirow, Sanjay B. Shah, Kimberly A. Livingston, and Kenneth E. Anderson. 2018. Evaluation of ventilation shutdown in a multi-level caged system. J. Appl. Poult. Res. 27:555-563.
Eberle, K. N., M. P. Martin, S. Shah, R. D. Malheiros, K. A. Livingston, and K. E. Anderson. 2018. A novel non-invasive method for evaluating electroencephalograms on laying hens. Poult. Sci. 97:860-864.
EFSA, E. A. 2015. Scientific Opinion on welfare aspects of the use of perches for laying hens. EFSA J. 197:1-23.
Erasmus, M. A. 2018. Welfare issues in turkey production. In “Advances in Farm Animal Welfare Series” Ed. Mench J. A., Woodhead Publishing, Duxford, UK.
Eshel, G., A. Shepon, T. Makov, and R. Milo. 2014. Land, irrigation water, greenhouse gas, and reactive nitrogen burdens of meat, eggs, and dairy production in the United States. Pro. Natl. Acad. Sci. USA. 111:11996-12001.
Fleming, R. H. 2008. Nutritional factors affecting poultry bone health. Proc. Nutr. Soc. 67:177- 183.
Foye, O. T., P. R. Ferket, and Z. Uni. 2007. The effects of in ovo feeding arginine, betahydroxy-beta-methyl-butyrate, and protein on jejunal digestive and absorptive activity in embryonic and neonatal turkey poults. Poult. Sci. 86:2343-2349.
Frame, D. D., R. E. Buckner, and G. L. Anderson. 2010. Causes and control of spontaneous cardiomyopathy or roundheart disease in Utah turkeys. Fact sheet. Utah State University Cooperative Extension.
Gates, R. S., H. Xin, K. D. Casey, Y. Liang, and E. F. Wheeler. 2005. Method for measuring ammonia emissions from poultry houses. J. Appl. Poult. Res. 14:622-634.
Geraert, P. A., S. Guillaumin, and B. Leclercq. 1993. Are genetically lean broilers more resistant to hot clomate? Br. Poult. Sci. 34:643-653.
Gerber, P. J., H. Steinfeld, B. Henderson, A. Mottet, C. Opio, J. Dijkman, A. Falcucci, and G. Tempio. 2013. Tackling climate change through livestock – A global assessment of emissions and mitigation opportunities. Food and Agriculture Organization of the United Nations (FAO), Rome.
Gerritzen. M. A., B. Lambooij, H. Reimert, A. Stegeman, and B. Spruijt. 2004. On-farm euthanasia of broiler chickens: Effects of different gas mixtures on behavior and brain activity. Poult. Sci. 83:1294-1301.
Guabiraba, R., and C. Schouler. 2015. Avian colibacillosis: still many black holes. FEMS Microbiol. Lett. 362:fnv118.
Gunnarsson, S., L. R. Matthews, T. M. Foster, and W. Temple. 2000. The demand for straw and feathers as litter substrates by laying hens. Appl. Anim. Behav. Sci. 65:321-330.
Guy J. H., and A. N Wright. 2003. Effect of enrichment with Pecka-Blocks™ on the behaviour and feather condition of commercially-reared broilers. Br. Poult. Sci. 44:29-30.
Hajat, S., M. O'Connor, and T. Kosatsky. 2010. Health effects of hot weather: From awareness of risk factors to effective health protection. Lancet. 375:856-863.
Hayes, Morgan Davis, "Environmental and energy assessment of an aviary laying-hen housing system in the Midwestern United States". 2012. Graduate Theses and Dissertations. 12601. https://lib.dr.iastate.edu/etd/12601.
Heng, Y., H. H. Peterson, and X. Li. 2013.Consumer attitudes toward farm-animal welfare: The case of laying hens. J. Agric. Res. Econ. 38:418-434.
Hernandez, C. J., J. D. Guss, M. Luna, and S. R. Goldring. 2016. Links between the microbiome and bone. J. Bone Miner. Res. 09:1638-46.
Hester, P. Y. 2005. Impact of science and management on the welfare of egg laying strains of hens. Poult. Sci. 84:687-696.
Hester, P. Y., S. A. Enneking, K. Y. Jefferson-Moore, M. E. Einstein, H. W. Cheng, and D. A. Rubin. 2013. The effect of perches in cages during pullet rearing and egg laying on hen performance, foot health, and plumage. Poult. Sci. 92:310-320.
Hidalgo A., M. Rossi, F. Clerici, and S. Ratti. 2008. A market study on the quality characteristics of eggs from different housing systems. Food Chem. 106:1301-1308.
Hoeven-Hangoor, E. van der Paton, N. D. Linde, I. B. van de Verstegen, M. W. A. Hendriks, and W. H. 2013. Moisture content in broiler excreta is influenced by excreta nutrient contents. J. Anim. Sci. 91:5705-5713.
Höglund, J., H. Nordenfors, and A. Uggla. 1995. A prevalence of the poultry red mite, Dermanyssus gallinae, in different types of production systems for egg layers in Sweden. Poult. Sci. 74:1793-1798.
Jha, R., T. A. Woyengo, J. Li, M. R. Bedford, T. Vasanthan, and R. T. Zijlstra. 2015. Enzymes enhance degradation of the fiber-starch-protein matrix of distillers dried grains with solubles as revealed by a porcine in vitro fermentation model and microscopy. J. Anim. Sci. 93:1039-1051.
Jha, R., and J. D. Berrocoso. 2015. Review: Dietary fiber utilization and its effects on physiological functions and gut health of swine. Animal. 9:1441-1452.
Jha, R., and J. F. D. Berrocoso. 2016. Dietary fiber and protein fermentation in the intestine of swine and their interactive effects on gut health and on the environment: A review. Anim. Feed Sci. Technol. 212:18-26.
Ji, B., W. Zheng, R. S. Gates, and A. R. Green. 2016. Design and performance evaluation of the upgraded portable monitoring unit for air quality in animal housing. Comput. Electron. Agric. 124:132-140.
Keeling, L. 1994. Inter-bird distances and behavioural priorities in laying hens: the effect of spatial restriction. Appl. Anim. Behav. Sci. 39:131-140.
Kelly, J. R., P. J. Kennedy, J. F. Cryan, T. G. Dinan, G. Clarke, and N. P. Hyland. 2015. Breaking down the barriers: the gut microbiome, intestinal permeability and stress-related psychiatric disorders. Front. Cell Neurosci. 9:392.
Kennedy, D. A., and G. Dwyer. 2018. Effects of multiple sources of genetic drift on pathogen variation within hosts. PLOS Biol. 16:e2004444.
Kiess, A. S., H. Parker, and C. D. McDaniel. 2010. Evaluation of different selective media and culturing techniques for the quantification of Campylobacter ssp. from broiler litter. Poult. Sci. 89:1755-1762.
Kollanoor Johny, A., J. G. Frye, A. Donoghue, D. J. Donoghue, S. Porwollik, M. McClelland, and K. Venkitanarayanan. 2017. Gene expression response of Salmonella enterica serotype Enteritidis phage type 8 to subinhibitory concentrations of the plant-derived compounds trans-cinnamaldehyde and eugenol. Front. Microbiol. 8:1828.
Krautwald-Junghanns, M. E., R. Ellerich, R. Bohme, J. Cramer, K. DellaVolpe, A. Mitterer-Istyagin, H. Ludewig, M. Fehlhaber, K. Schuster, E. Berk, J. Aldehoff, D. Fulhorst, D. Kruse, W. Dressel, A. Noack, and U. Bartels. 2009. Examination of rearing standards and health status in turkeys in Germany. Berl. Munch. Tierarztl. Wochenschr. 122:271-283.
Lei, K., Y. L. Li, Y. Wang, J. Wen, H. Z. Wu, D. Y. Yu, and W. F. Li. 2015. Effect of dietary supplementation of Bacillus subtilis B10 on biochemical and molecular parameters in the serum and liver of high-fat diet-induced obese mice. J. Zhejiang Univ. Sci. B. 16:487-95.
Le Menach, A., E. Vergu, R. F. Grais, D. L. Smith, and A. Flahault, 2006. Key strategies for reducing spread of avian influenza among commercial poultry holdings: lessons for transmission to humans. Proc. Biol. Sci. 2006 Oct 7; 273:2467-2475. doi:10.1098/rspb.2006.3609.
Lemons, Mark, Christopher McDaniel, Joseph Moritz, and Kelley Wamsley. 2016. Influence of feed form (FF) and diet phase effects on 0-46 d broiler performance. Poult. Sci. 95(E-Suppl. 1): 230.
Lemons, M. E., and J. S. Moritz. 2016. The effect of feeder space access and crumble- or pellet-to-fine ratio on 38 day-day-old broiler performance. J. Appl. Poult. Res. 25:12-20.
Leung, P., and M. Loke. 2008. Economic Impacts of Increasing Hawaii’s Food Self- Sufficiency. CTAHR Extension Series EI-16. CTAHR, UH Mānoa.
Lewis, P. D., and Morris, T. R. 2000. Poultry and coloured light. Worlds Poult. Sci. J. 56:189-207.
Li, H., H. Xin, Y. Liang, R. S. Gates, E. F. Wheeler, and A. J. Heber. 2005. Comparison of direct vs. indirect ventilation rate determination rates in layer barns using manure belts. Transactions of the ASAE 48:367-372.
Lilburn, M. S., and S. Loeffler. 2015. Early intestinal growth and development in poultry. Poult. Sci. 94:1569-1576.
Lilly, K. G. S., C. K. Gehring, K. R. Beaman, P. J. Turk, M. Sperow, and J. S. Moritz. 2011. Examining the relationships between pellet quality, broiler performance, and bird sex. J. Appl. Poult. Res. 20:231-239.
Lin H., E. Decuypere, and J. Buyse. 2006. Acute heat stress induces oxidative stress in broiler chickens. Comp. Biochem. Physio.l A Mol. Integr. Physiol. 144:11-17.
Lindqvist, C. E, K. E. Schütz, and P. Jensen. 2002. Red jungle fowl have more contrafreeloading than White Leghorn layers: effect of food deprivation and consequences for information gain. Behav. 139:1195-1209.
Lindqvist C. E., P. Zimmerman, and P. Jensen. 2006. A note on contrafreeloading in broilers compared to layer chicks. Appl. Anim. Behav. Sci. 101:161-166.
Liu, N. G., H. Liu, F. D. Li, J. S. Sands, S. Zhang, A. J. Zheng, and Y. J. Ru. 2007. Efficacy of phytases on egg production and nutrient digestibility in layers fed reduced phosphorus diets. Poult. Sci. 86:2337-2342.
Liu, K., and H. Xin. 2017. Effects of horizontal distance between perches on perching behaviors of Lohmann hens. Appl. Anim. Behav. Sci. https//dx.doi.org/10.1016/j.applanim.2017.05.001.
Liu, Kai. 2017. "Behavior and production responses of pullets and laying hens to enriched housing and lighting". Graduate Theses and Dissertations. 16164. https://lib.dr.iastate.edu/etd/16164.
Long, H., Y. Zhao, T. Wang, Z. Ning, and H. Xin. 2016a. Effect of light-emitting diode (LED) vs. fluorescent lighting (FL) on laying hens in aviary hen houses: Part 1 – Operational characteristics of lights and production traits of hens. Poult. Sci. 95:1-11.
Long, H., Y. Zhao, H. Xin, H. Hanses, Z. Ning, and T. Wang. 2016b. Effect of light-emitting diode (LED) vs. fluorescent (FL) lighting on laying hens in aviary hen houses: Part 2 – Egg quality, shelf life and lipid composition. Poult. Sci. 95:115-124.
Macalintal, L. M. 2012. In Ovo Selenium (Se) Injection of Incubating Chicken Eggs: Effects on Embryo Viability, Tissue Se Concentration, Lipid Peroxidation, Immune Response and Post Hatch Development. PhD Dissertation, University of Kentucky, Lexington, KY, USA.
Macalintal, L. M., A. J. Pescatore, T. Ao, R. Xiao, M. J. Ford, R. Power, and K. A. Dawson. 2017. Intra-amnion delivery of mannose or mannose-based oligosaccharides in fertile broiler eggs at 18d of incubation. In: International Poultry Scientific Forum Abstracts, Atlanta, GA, USA, January 30-31. P300.
Macalintal, L. M., A. J. Pescatore, T. Ao, P. Glenney, M. J. Ford, and K. A. Dawson. 2017. Exploring the novel approach of probiotic delivery into the gut of pre-hatch chicks. In: International Poultry Scientific Forum Abstracts, Atlanta, GA, USA, January 30-31. P301.
Malabad, C., K. Balagso, A. Haygood, K. Neupane, and R. Jha. 2016. Effects of in vitro fermentation of alternative feedstuffs on intestinal microbiota of chicken analyzed using 16S ribosomal DNA typing. Proceedings of the 28th Annual CTAHR/COE Student Research Symposium (April 8-9, 2016), Honolulu, HI.
Malone, T., and J. L. Lusk. 2016. Putting the chicken before the egg price: An ex post analysis of California’s battery cage ban. J. Agric. Res. Econ. 41: 518-532.
Mayer, A., P. Patterson, R. Hulet, and M. Hile. 2016. Chopped Miscanthus grass vs. softwood shavings as a bedding material for broiler grow-out. Poult. Sci. 95(Suppl. 1):219.
Mayne, R. K., R. W. Else, and P. M. Hocking. 2007. High litter moisture alone is sufficient to cause footpad dermatitis in growing turkeys. Br. Poult. Sci. 48:538-545.
McCabe, L., R. A. Britton, and N. Parameswaran. 2015. Prebiotic and probiotic regulation of bone health: role of the intestine and its microbiome. Curr. Osteoporos. Rep. 13:363-371.
McEvoy, M. 2015. Memorandum to the National Organic Standards Board. https://www.ams.usda.gov/sites/default/files/media/NOSB%20Memo%20Response%20to%20Rec%20from%20April%202015%20Meeting.pdf.
McKeegan, D. E. F., H. G. M. Reimert, V. A. Hindle, P. Boulcott, J. M. Sparrey, C. M. Wathes, T. G. M. Demmers, and M. A. Gerritzen. 2013. Physiological and behavioral responses of poultry exposed to gas-filled high expansion foam. Poult. Sci. 92:1145-1154.
Mench, J. A., D. A. Summer, J. T. Rosen-Molina. 2011. Sustainability of egg production in the United States-The policy and market context. Poult. Sci. 90:229-240.
Mench, J. A., and R. A. Blatchford. 2014. Determination of space use by laying hens using kinematic analysis. Poult. Sci. 93:794-798.
Mitchell, B. 2015. Layer flock depopulation exercise. http://prezi.com/iguofwpmzi7k/?utm_ campaign=share&utm_medium=copy.
Mor-Mur, M., and J. Yuste. 2009. Emerging Bacterial Pathogens in Meat and Poultry: An Overview. Food Bioprocess. Tech. 3:24.
Mohanty, S. K., and A. B. Boehm. 2014. Escherichia coli removal in biochar augmented biofilter: Effect of infiltration rate, initial bacterial concentration, biochar particle size, and presence of compost. Environ. Sci. Tech. 48:11535-11542.
Mohanty, S. K., K. B. Cantrell, K. L. Nelson, and A. B. Boehm. 2014. Efficacy of biochar to remove Escherichia coli from stormwater under steady and intermittent flow. Water Res. 61:288-296.
Mueller, W. J., R. Schraer, and H. Schraer. 1964. Calcium metabolism and skeletal dynamics of laying pullets. J. Nutr. 84:20-26.
Mujahid, A., Y. Yoshiki, Y. Akiba, and M. Toyomizu. 2005. Superoxide radical production in chicken skeletal muscle induced by acute heat stress. Poult. Sci. 84:307-314.
Mul, M. 2013. Fact Sheet: The poultry red mite, Dermanyssus gallinae: A small pest that packs a big punch. Wageningen University and Research.
Mullally, C., and J. L. Lusk. 2015. Happy hens, sad consumers? The economic impact of restrictions on farm animal housing in California. Unpublished Manuscript. Available online at http://agecon.okstate.edu/faculty/publications/5115.pdf.
Murillo, A., and B. Mullens. 2016. Diversity and prevalence of ectoparasites on backyard chicken flocks in California. J. Med. Entomol. 53:707-711.
Nair, D. V. T., and A. Kollanoor Johny. 2018a. Characterizing the antimicrobial function of a dairy-originated probiotic, Propionibacterium freudenreichii, against multidrug-resistant Salmonella enterica serovar Heidelberg in turkey poults. Front. Microbiol. 9:1475.
Nair, D. V. T., and A. Kollanoor Johny. 2018b. Food grade Pimenta leaf essential oil reduces the attachment of Salmonella enterica Heidelberg (2011 ground turkey outbreak isolate) on to turkey skin. Front. Microbiol. 8:2328.
Nair, D. V. T., J. V. Thomas, S. Noll, R. Porter Jr., and A. Kollanoor Johny. 2018a. Effect of various inoculum levels of multidrug-resistant Salmonella enterica serovar Heidelberg (2011 ground turkey outbreak isolate) on cecal colonization, dissemination to internal organs, and deposition in skeletal muscles of commercial turkeys after experimental oral challenge. Front. Microbiol. 8:2680.
Nair, D. V. T., K. Venkitanarayanan, and A. Kollanoor Johny. 2018b. Antibiotic-resistant Salmonella in the food supply and the potential role of antibiotic alternatives for control. Foods 7:167.
Nasr, M. A. F., C. J. Nicol, and J. C. Murrell. 2012a. Do laying hens with keel bone fractures experience pain? PLoS ONE 7:e42420.
Nasr, M. A, J. Murrell, L. J. Wilkins, and C. J. Nicol. 2012b. The effect of keel fractures on egg production parameters, mobility, and behaviour in individual laying hens. Animal Welfare 21:127-135.
Nasr, M. A, W. J. Browne, G. Caplen, B. Hothersall, J. C. Murrell, and C. J. Nicol. 2013a. Positive affective state induced by opioid analgesia in laying hens with bone fractures. Appl. Anim. Behav. Sci. 147:127-131.
Nasr, M.A., J. Murrell, and C. J. Nicol. 2013b. The effect of keel fractures on egg production, feed, and water consumption in individual laying hens. Br. Poult. Sci. 54:165-170.
Nasr, M. A., C. J. Nicol, L. Wilkins, and J. C. Murrell. 2015. The effects of two non‐steroidal anti‐inflammatory drugs on the mobility of laying hens with keel bone fractures. Vet. Anaesth. Analg. 42:197-204.
Ni, J.-Q., L. Chai, L. Chen, B. W. Bogan, K. Wang, E. L. Cortus, A. Heber, T. Lim, and C. A. Diehl. 2012. Characteristics of ammonia, hydrogen sulfide, carbon dioxide, and particulate matter concentrations in high-rise and manure-belt layer hen houses. Atmos. Environ. 57:165-174. Retrieved 2/5, 2018, from http://sciencedirect.com/science/article/pii/s1352231012003536.
Noll, S. L., K. A. Janni, D. A. Halvorson, and C. J. Clanton. 1997. Market turkey performance, air quality, and energy consumption affected by partial slotted flooring. Poult. Sci. 76:271-279.
Norwood, B. F., and J. L. Lusk. 2009. The Farm Animal Welfare Debate. Choices 24: 1–7. Available online at http://www.choicesmagazine.org/magazine/article.php?article=89.
Norwood, B. F., and J. L. Lusk. 2011. Compassion, by the Pound: The Economics of Farm Animal Welfare. New York: Oxford University Press.
Odén, K., L. Keeling, and B. Algers. 2002. Behaviour of laying hens in two types of aviary systems on 25 commercial farms in Sweden. Br. Poult. Sci. 43:169-181.
Ohta, Y., and M. T. Kidd. 2001. Optimum site for in ovo amino acid injection in broiler breeder eggs. Poult. Sci. 80:1425-1429.
Oliveira J., and H. Xin. 2018. Responses of laying hens to full vs. partial litter access in aviary housing. 10th International Livestock Environment Symposium (ILES X), 25-27 September 2018, Omaha, Nebraska USA. ILES18-013:1-8.
Olsson, I. A. S., and L. J. Keeling. 2000. Night-time roosting in laying hens and the effect of thwarting access to perches. Appl. Anim. Behav. Sci. 68:243-256.
Olukosi, O. A., A. J. Cowieson, and O. Adeola. 2007. Age-related influence of a cocktail xylanase, amylase, and protease or phytase individually or in combination in broilers. Poult. Sci. 86:77-86.
O’Neill, H. V. M., J. A. Smith, and M. R. Bedford. 2014. Multicarbohydrase enzymes for non-ruminants. Asian-Australasian J. Anim. Sci. 27:290-301.
Pandey, S., A. Singh, N. Chaudhar, L. P. Nampoothiri, and G. N. Kumar. 2015. Protection against 1, 2-di-methylhydrazine-induced systemic oxidative stress and altered brain neurotransmitter status by probiotic Escherichia coli CFR 16 secreting pyrroloquinoline quinone. Curr. Microbiol. 70:690-7.
Parvin, R., M. M. Mushtaq, M. J. Kim, and H. C. Choi. 2014. Light emitting diode (LED) as a source of monochromatic light: a novel lighting approach for behaviour, physiology and welfare of poultry. World’s Poult. Sci. J. 70:543-556.
Pichova, K, J. Nordgreen, C. Leterrier, L. Kostal, and R. O. Moe. 2016. The effects of food-related environmental complexity on litter directed behaviour, fear and exploration of novel stimuli in young broiler chickens. Appl. Anim. Behav. Sci. 174:83-89.
Pizzolante, C. C., S. K. Kakimoto, E. S. P. B. Saldanha, C. Laganá, H. B. A. Souza, and J. E. Moraes. 2011. Limestone and oyster shell for brown layers in their second egg production cycle. Br. Poult. Sci. 13:103-111.
Reisinger, N., T. Steiner, S. Nitsch, G. Schatzmayr, and T. J. Applegate. 2011. Effects of a blend of essential oils on broiler performance and intestinal morphology during coccidial vaccine exposure. J. Appl. Poult. Res. 20:272-283.
Richards, T. J., W. Allender, and D. Fang. 2013. Media Advertising and Ballot Initiatives: The Case of Animal Welfare Regulation. Contemporary Economic Policy 31:145–162. doi: 10.1111/j.1465-7287.2011.00292.x.
Riddle, E. R., A. B. Ali, D. L. Campbell, and J. M. Siegford. 2018. Space use by 4 strains of laying hens to perch, wing flap, dust bathe, stand and lie down. PloS ONE 13: e0190532.
Rouger, A., O. Tresse, M. Zagorec. 2017. Bacterial Contaminants of Poultry Meat: Sources, Species, and Dynamics. Microorganisms 5:50.
Sah, N., D. N. Kuehu, V. S. Khadka, Y. Deng, K. Peplowska, R. Jha, and B. Mishra. 2018. RNA sequencing-based analysis of the laying hen uterus revealed the novel genes and biological pathways involved in the eggshell biomineralization. Sci. Rep. 8:16853.
Sellers, R. B., P. B. Tillman, J. S. Moritz, and K. G. S. Wamsley. 2017. The effects of strain and incremental improvements in feed form on d 28 to 42 male broiler performance. J. Appl. Poult. Res. 26:192-199.
Sharma, J. M., and B. R. Burmester. 1984. Disease control in avian species by embryonal vaccination. U.S. Patent no. 4,458,630.
Shepherd, E. M., and B. D. Fairchild. 2010. Footpad dermatitis in poultry. Poult. Sci. 89:2043-2051.
Shepherd, T. A., Y. Zhao, H. Li, J. P. Stinn, M. D. Hayes, and H. Xin. 2015. Environmental assessment of three laying-hen housing systems. Part II: Ammonia, greenhouse gas, and particulate matter emissions. Poult. Sci. 94:534-543.
Sherwin C. M., G. J. Richards, and C. J. Nicol. 2010. A comparison of the welfare or layer hens in four housing systems in the UK. Br. Poult. Sci. 51:488-499.
Sigognault Flochlay, A., E. Thomas, and O. A. E. Sparagano. 2017. Poultry red mite (Dermanyssus gallinae) infestation: a broader impact parasitological disease that still remains a significant challenge for the egg laying industry. Parasites and Vectors. 10:357-362.
Silversides, F. G., T. A. Scott, D. R. Korver, M. Afsharmanesh, and M. Hruby. 2006. A study on the interaction of xylanase and phytase enzymes in wheat-based diets fed to commercial white and brown egg laying hens. Poult. Sci. 85:297-305.
Singh R., K. M. Cheng, and F. G. Silversides. 2009. Production performance and egg quality of four strains of laying hens kept in conventional cages and floor pens. Poult. Sci. 88:256-264.
Solymosi, N., C. Torma, A. Kern, A, Maroti-Agots, Z. Barcza, L. Konyves, O. Berke and J. Reiczigel. 2010. Changing climate in Hungary and trends in the annual number of heat stress days. Int. J. Biomet. 54:423-431.
Sparagano, O. A. E., D. R. George, D. W. J. Harrington, and A. Giangaspero. 2014. Significance and control of the poultry red mite, Dermanyssus gallinae. Ann. Rev. Entomol. 59:447-466.
Sparagano, O. A. E., A. Pavlicevic, T. Murano, A. Camarda, H. Sahibi, O. Kilpinen, M. Mul, R. Van Emous, S. le Bouquin, K. Hoel, and M. A. Cafiero. 2009. Prevalence and key figures for the poultry red mite Dermanyssus gallinae infections in poultry farm systems. Exp. Appl. Acarol. 48:3-10.
Spindler, B., M. Giersberg, A. Briese, N. Kemper, and J. Hartung. 2016. Spatial requirements of poultry assessed by using a colour-contrast method (KobaPlan). Br. Poult. Sci. 57:23-33.
St-Pierre, N. R., B. Cobanov, and G. Schnitkey. 2003. Economic losses from heat stress by US livestock industries. J. Dairy Sci. 86:E52-E77.
Tako, E., P. R. Ferket, and Z. Uni. 2005. Changes in chicken intestinal zinc exporter mRNA expression and small intestinal functionality following intra-amniotic zinc-methionine administration. J. Nutr. Biochem. 16:339-346.
Turner, P. V., H. Kloeze, A. Dam, D. Ward, N. Leung, E. E. L. Brown, A. Whiteman, M. E. Chiappetta, and D. B. Hunter. 2012. Mass depopulation of laying hens in whole barns with liquid carbon dioxide: Evaluation of welfare impact. Poult. Sci. 91:1558-1568.
Thorp, B. H. 1994. Skeletal disorders in the fowl: A review. Avian Pathol. 23:203-236.
Tiwari, U. P., and R. Jha. 2015. Nutrient profile and in vitro digestibility of fresh and ensiled cassava in swine. Proceeding of the 3rd International Seminar on Animal Industry (Sept 17-18, 2015), Bogor, Indonesia. pp. 252-253.
Tiwari, U. P., and R. Jha. 2016. Nutrient profile and digestibility of tubers and agro-industrial coproducts determined using an in vitro model of swine. Anim. Nutr. 2:357-360.
Tiwari, U. P., and R. Jha. 2017. Nutrients, amino acid, fatty acid and non-starch polysaccharide profile and in vitro digestibility of macadamia nut cake in swine. Anim. Sci. J. 888:1093-1099.
Tiwari, U. P., H. Chen, S. W. Kim, and R. Jha. 2018. Supplemental effect of xylanase and mannanase on nutrient digestibility and gut health of nursery pigs studied using both in vivo and in vitro model. Anim. Feed Sci. Technol. 245:77-90.
Tucker, C., A. R. Green-Miller, R. S. Gates, S. Myint, and J. Salak-Johnson. 2018. Behavioral responses of laying hens to atmospheric ammonia in an environmental preference chamber. Paper ILES18-106, 25-27 September. 10th Intl. Livestock Env. Symp, Omaha NE, USA.
Parvin, R., M. M. Mushtaq, M. J. Kim, and H. C. Choi. 2014. Light emitting diode (LED) as a source of monochromatic light: a novel lighting approach for behaviour, physiology and welfare of poultry. Worlds Poult. Sci. J. 703:543-556.
UEP. 2017. United Egg Producers Animal Husbandry Guidelines for US Egg Laying Flocks. Retreived from https://uepcertified.com/wp-content/uploads/2017/11/2017-UEP-Animal-Welfare-Guidelines-Cage-Housing-11.01.2017-FINAL.pdf.
Uni, Z., and P. R. Ferket. 2003. Enhancement of development of oviparous species by in ovo feeding. U.S. Patent no. 6,592,878 B2.
Vestergaard, K. S., E. Skadhauge, and L. Lawson. 1997. The stress of not being able to perform dustbathing in laying hens. Physiology and Behavior 62:413-419.
Villa, C. R, W. E. Ward, and E. M. Comelli. 2015. Gut Microbiota-bone Axis. Crit. Rev. Food Sci. Nutr. 57:1664-1672.
Webster, A. B. 2004. Welfare implication of avian osteoporosis. Poult. Sci. 83:184-192.
Webster, A.B. 2007. Depopulation methods for a commercial layer flock: Part 1 and 2. The Poultry Site, Poultry Digital #51; Health and Disease. http://www.thepoultrysite.com/articles/843/depopulation-methods-for-a-commercial-layerflock-part-1-and-2.
Weeks, C., and C. Nicol. 2006. Behavioural needs, priorities and preferences of laying hens. Worlds Poult. Sci. J. 62:296-307.
Whitehead, C. C. 2004. Overview of bone biology in the egg-laying hen. Poult. Sci. 83:193-199.
Whitehead, C. C., and R. H. Flemming. 2000. Osteoporosis in cage layers. Poult. Sci. 79:1033-1041.
Willemsen, H., N. Everaert, A. Witters, L. De Smit, M. Debonne, F. Verschuere, P. Garain, D. Berckmans, E. Decuypere, and V. Bruggeman. 2008. Critical assessment of chick quality measurements as an indicator of posthatch performance. Poult. Sci. 87:2358-2366.
Wyneken, C. W., A. Sinclair, A. Veldkamp, T. Vinco, and L. J. Hocking. 2015. Footpad dermatitis and pain assessment in turkey poults using analgesia and objective gait analysis. Br. Poult. Sci. 56:522-530.
Xin, H., H. Li, R. T. Burns, R. S. Gates, D. G. Overhults, and J. W. Earnest. 2009. Use of CO2 concentration difference or CO2 balance to assess ventilation rate of broiler houses. Transactions of the ASABE 52:1353-1361.
Yadav, S., and R. Jha. 2017. Cassava chips as an alternative feedstuff for broiler chickens: effect on growth performance and ileal morphology. Poult. Sci. 96(E-Suppl. 1):180.
Yadav, S., K. Neupane, and R. Jha. 2017. Macadamia nut cake as an alternative feedstuff for broiler chickens: effect on growth performance and gut microbiota profile. Poult. Sci. 96(E-Suppl. 1):186.
Yarandi, S. S., D. A. Peterson, G. J. Treisman, T. H. Moran, and P. J. Pasricha. 2016. Modulatory effects of gut microbiota on the central nervous system: How gut could play a role in neuropsychiatric health and diseases. J. Neurogastroenterol. Motil. 22:201-212.
Zhao, Y. T. A. Shepherd, J. Swanson, J. A. Mench, D. M. Karcher, and H. Xin. 2015a. Comparative evaluation of three laying-hen housing systems: Description of the production systems and management practices. Poult. Sci. 943:475-484.
Zhao, U., T. A. Shepherd, H. Li, J. P. Stinn, M. D. Hayes, and H. Xin. 2015b. Environmental assessment of three laying-hen housing systems – Part I: Monitoring system and indoor air quality. Poult. Sci. 94:518-533.
Zhao, Y., H. Xin, T. A. Shepherd, M. D. Hayes, J. P. Stinn, and H. Li. 2013. Thermal environment, ammonia concentrations, and ammonia emissions of aviary houses with white laying hens. Transactions of the ASABE. 56:1145-1156.
Zheng, W., R. S. Gates, and K. Koelkebeck. 2015. Assessing cold weather ventilation using intelligent portable monitoring units (iPMU) in a layer barn. International Symposium of Ambience and Engineering for Sustainable Livestock Production, Vicosa – Minas Gerais State, Brazil.
Zhuang, Q. Y., S. C. Wang, J. P. Li, D. Liu, S. Liu, W. M. Jiang, and J. M. Chen. 2014. A clinical survey of common avian infectious diseases in China. Avian Dis. 58:297-302.