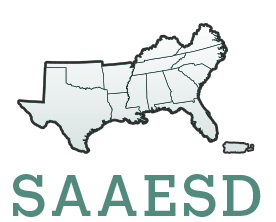
OLD S1075: The Science and Engineering for a Biobased Industry and Economy
(Multistate Research Project)
Status: Inactive/Terminating
OLD S1075: The Science and Engineering for a Biobased Industry and Economy
Duration: 10/01/2018 to 09/30/2023
Administrative Advisor(s):
NIFA Reps:
Non-Technical Summary
Statement of Issues and Justification
- The Land Grant University System, Resource Limitation, and the Impending Biological Revolution
Increased use of economically-viable and sustainable processes will require deliberate development and refinement of novel technologies. Implementation of economically viable and sustainable processes is urgent due to three converging issues: (1) decrease in productive agricultural land due to urbanization pressures; (2) using unsustainable methods to clear land for agricultural production; and (3) increasing world population with a rising per capita energy use and consumption of animal protein. One billion hectares of land will be cleared by 2050, resulting in the release of 3 Gt/year of greenhouse gases (Tilman et al., 2011). Global population will reach 9 billion by 2050, resulting in rising food demand from 2005 to 2050 (Tilman et al., 2011; Hochman et al. 2014). The breadth of these intersecting problems is so vast that constructive solutions can only be developed and implemented through collaborations that cross traditional disciplinary boundaries. Replacing existing petroleum-based energy and products with those that are stemming from biomass will require research and development (Dale et al. 2014). Additionally, in order to determine whether novel processes and ensuing technology are actually making advances in sustainability, quantitative sustainability metrics need to be developed. These metrics are urgently needed to guide science and/or engineering approaches to increase sustainability of agricultural production and processing.
Specifically, the objectives of this project are to address research relating directly to the Southern Association of Agricultural Experiment Station Directors Goal 1.6 (biobased products) and Goal 1.8 (processing agricultural byproducts). Proposed research will influence Goal 5.2 (rural community development and revitalizing rural economies) indirectly. Because renewable energy systems and by-product production stem from biomass production systems requiring large land areas, these processing facilities are typically located in rural areas which promote economic development of these communities. Moreover, transitioning away from fossil-fuel systems and toward economically-viable, biomass-based systems will require research. Without research, the technical capacity to switch from a fossil fuel-based economy to a diversified bioresource-based economy will be severely limited by unanswered questions, undeveloped technologies, and suboptimal bioresource production and utilization. Research proposed herein is designed to help address these limitations with the expertise of professional scientists and engineers primarily associated with the Land Grant University system. The Land Grant University system also partners with important policy-setting agencies such as the United States Departments of Agriculture (USDA), Energy (US DOE) and Defense (US DOD), as well as the National Science Foundation (NSF) and various state agencies to meet its research mission.
- How S-1041 enabled new renewable energy industries.
Increasing the breadth of economically-viable renewable energy and by-product production implies the production of power and second-generation liquid biofuels, as well as bioderived chemicals that can be petroleum additions or event replacements. Researchers in the S-1041 Multistate project are advancing this goal through research into most facets of production systems for bioenergy and associated co-products, as detailed below.
B.1. Feedstock
Efficient feedstock supply chains are needed at commercial scale to enable the successful deployment of biobased systems. Progress was made in securing an abundant, economical supply of biomass feedstock delivered with predictable specifications that meet conversion needs. Biological feedstocks from areas representative of the various Land Grant University partners were evaluated for biomass yield, composition, and other characteristics. Feedstocks included switchgrass, giant miscanthus, energy cane and sweet sorghum with dry matter yields of 36-41 Mg/ha, 30 Mg/ha, 83 Mg/ha and 12-22 Mg/ha, respectively (West and Kincer (2011); Heaton et al. (2008); Heaton et al. (2010); Bischoff et al. (2008); Aita et al. (2011); and, Singh et al. (2012)). Camelina and other oilseed crops were evaluated as potential feedstock for biodiesel and aviation fuels (Chen et al. 2015). This effort included the development of camelina cultivars for higher yield and improved oil content/profile as well as cropping system development (Chen et al. 2015). The yield potential of biomass was evaluated for different climate and geographic regions with an aim to increase yield while maintaining sustainable production with lower environment and wildlife habitat impacts (Mochizuki et al. 2015). Growing condition impacts were determined for feedstocks such as prairie cordgrass (Kim et al. 2015). Inter-cropping practices were examined to determine influence on soil health and biomass yield (Shao et al. 2015). Woody crop growth was modeled in the Pacific Northwest (Hart et al. 2015).
A study of the impact of soil type and fertility treatment on productivity and composition of perennial grasses determined that composition was influenced by both species and treatment, with fewer differences due to soil type. Biomass composition prediction was improved using Fourier transform near infrared (FT-NIR) spectroscopy coupled with multivariate analysis. A generalized, single broad-based predictive model for multiple types of biomass feedstock was developed using corn stover and switchgrass to predict concentrations of glucan, xylan, galactan, arabinan, mannan, lignin, and ash. Both cross-validation and independent validation supported a single FT-NIR model for both species, and results indicated the method's potential for wheat straw (Liu et al. 2010). NIR technology was used to characterize structural polysaccharides and soluble sugars in sorghum biomass (Xu et al. 2015). Innovative methods for biomass physical property measurement were developed. Image analysis was used to identify chopped biomass stem nodes and internodes; this information may impact both biochemical and thermochemical conversion platforms (Pothula et al. 2015). High resolution broadband sum frequency generation vibrational spectroscopy techniques were developed to determine the structures of cellulose microfibrils (Zhang et al. 2015)
Bulk density, a major logistics factor in handling biomass, is a strong function of the size and shape of the particle and particle density. Loose-filled bulk densities were determined to be 67.5±18.4 kg/m3 for switchgrass, 36.1±8.6 kg/m3 for wheat straw, and 52.1±10.8 kg/m3 for corn stover (Chevanan et al. 2011). Pressure and volume relationship of chopped biomass during compression with application of normal pressure was observed to fit the Walker and Kawakita and Ludde models well. Parameters of the Walker model correlated with compressibility at a Pearson correlation coefficient greater than 0.9.
Densification of biomass feedstocks was applied to switchgrass and woody biomass following fast pyrolysis, and woody biomass fractionated into lignin, cellulose and hemicellulose streams. Densified switchgrass, wheat straw, giant miscanthus, elephant grass, cotton gin trash, and softwood forest trimmings were produced through pelleting and briquetting with resultant heating values ranging from 17,908 to 18,839 kJ/kg. Biomass specifications, energy, and costs for feedstock densification were identified particularly for pellets, cubes, and grinds. Minimum-pressure densification was investigated due to recognition of the high cost and inefficient pressure application associated with the pelleting process. Specific energy for minimum-pressure densification ranged from 0.25 to 2 kJ/kg, which was significantly less than the typical energy requirement to produce pellets. An integrated biomass pretreatment (AFEX) and densification process using corn stover, switchgrass and prairie cordgrass indicated that AFEX-pretreated billets hold together well without added binders. Biomass pellets have also been shown to improve pretreatment efficacy, which can reduce costs and environmental impacts (Nahar and Pryor, 2014; Nahar and Pryor, 2017).
Flowability of chopped switchgrass, wheat straw, and corn stover at pre-consolidation pressures of 3.80 kPa and 5.02 kPa indicated Mohr-Coulomb failure. Results of measured angle of internal friction and cohesive strength indicated that typical chopped biomasses cannot be handled by gravity alone. Unconfined yield strength and major consolidation strength used for characterization of bulk flow materials and design of hopper dimensions were 3.4 and 10.4 kPa for chopped switchgrass; 2.3 and 9.6 kPa for chopped wheat straw and 4.2 and 11.8 kPa for chopped corn stover (Chevanan et al. 2009). These results are useful for development of efficient handling, storage, and transportation systems for biomass in biorefineries.
Although, great strides were made in terms of predicting biomass crop yields and quality on selected areas, knowledge gaps still remain in: 1) expected yields of these energy crops throughout US available land; 2) spatial and temporal variations in yield and supply due to climate and soil conditions; 3) harvesting and handling of these low bulk density materials; and, 4) identifying target physical properties important at the interface of processing and conversion.
B.2 Conversion
B.2.1. Thermochemical
Cellulosic biomass conversion to industrial chemicals and fuels is performed via thermochemical, biochemical or a combination of these platforms. Unfortunately, there is still no clear technology winner and both conversion platforms have tradeoffs. The thermochemical platform is robust in terms of feedstock processing, but somewhat complicated in terms of the resulting product portfolio (Sharara et al. 2012). Thermochemical conversion includes the initial steps of gasification, pyrolysis or a combination of these technologies. The effects of furnace temperature, steam to biomass ratio and equivalence ratio on gas composition, carbon conversion efficiency and energy conversion efficiency of the product gas were investigated with temperatures of 650, 750 and 850 °C, steam to biomass ratios of 0, 7.30 and 14.29 and equivalence ratios of 0.07, 0.15 and 0.29. Gasification temperature was determined to be the most influential factor on hydrogen and methane contents, carbon conversion, and energy efficiencies. A steam to biomass ratio of 7.30 resulted in maximum carbon conversion and energy efficiencies. Effect of initial biomass composition on furnace temperature profile and resultant gas composition was modeled; predicted flow rates of hydrogen were similar to experimental values, (Kumar et al. 2008; Kumar et al. 2009). Gasification studies were conducted for wood chips and agricultural residues (Gautam et al. 2011a), pelletized biomass (Gautam et al. 2011b) and coal/biomass mixtures (Brar et al. 2013). Use of a fluidized bed gasifier with wheat straw feedstock was tested (Mac and Bhaird et al. 2014). Downdraft gasifier technology was used to produce char from sorghum and eastern red cedar for further study (Qian et al. 2015). Downdraft gasifier technology was evaluated for 11.6% dry basis switchgrass, where the lowest heating value of gas was 1,566 kcal/Nm3, CO, H2 and CO2 concentrations were 23%, 12% and 9%, respectively, and the corresponding average specific gasification rate was 663 m3 dry gas/h-m2 of combustion zone area (Patil et al. 2011).
Wang et al. (2012) investigated the possibility of fermenting bio-oil sugars. New pyrolysis systems were developed and tested at the pilot scale (Wan et al. 2009a). Corn stover and aspen wood pellets were pyrolyzed using microwave heating with or without addition of catalysts (Moen et al. 2010). Pyrolysis studies were conducted using corn stover (Capunitan and Capareda 2012), switchgrass (Iman and Capareda 2012), and sweetgum (Wang et al. 2012).
Catalytic-based processes were developed by S-1041 members (Street et al. 2012a; Street et al. 2012b; Street and Yu 2011). The upgrade, via catalysis, of synthesis gas to useable fuels was investigated (Yan et al. 2012a; Yan et al. 2012b). Synthesis of gasoline-range hydrocarbons from nitrogen-rich syngas over a bi-functional catalyst was investigated (Lu et al. 2015). Dimethyl ether (DME) was synthesized in a one-step process from syngas on CuZnAl/zeolite catalyst (Xie et al. 2015). Microalgae, corn stover, poultry litter, peanut, pinewood and switchgrass, were tested as microwave assisted pyrolysis (MAP) feedstocks (Du et al. 2011; Wan et al. 2010; Wan et al. 2009b). Microalgae-produced MAP bio-oils displayed better quality in terms of heating value, oxygen content, viscosity and density than those obtained from lignocellulosic biomass. Enhanced quality of microalgae bio-oils could be due to the higher concentration of hydrocarbons and lower concentration of oxygenated compounds compared to other bio-oils (Wan et al. 2010).
Effect of catalyst loading and nature of support was examined on the water gas shift reaction (Haryanto et al. 2011). The use of biomass was investigated in the Fischer-Tropsch reaction (Hu et al. 2012: Lu et al. 2015). Catalytic conversion of syngas to mixed alcohols over catalysts was studied (Lu et al. 2012). Synthesis of alkylated aromatic hydrocarbons from CO/H2 mixtures over molybdenum/ZSM/water gas shift catalysts was examined (Wijayapala et al. 2014). Pyrolysis vapors were co-processed with bio-chars with an upgrading goal (Jin et al. 2015). Catalytic cracking of non-edible sunflower oil over ZSM-5 catalyst for bio-jet fuel production was reported (Zhao et al. 2015).
Although, great advances were made in terms of designing pyrolysis, gasification and catalysts, knowledge gaps still remain related to integration of these technologies to produce thermochemically-based and economically-viable energy systems.
B.2.2. Biochemical
As opposed to thermochemical processing, biochemical processing does not typically require temperatures above 200 °C. On the other hand, this processing platform is based on the saccharification of plant cell wall structural carbohydrates to produce high quality sugar streams that can be converted to biobased fuels or chemicals. To produce fuels using biochemical processing technologies, feedstocks must be reduced in size, pretreated, hydrolyzed with enzymes, and fermented (Lynd et al. 2008). Specific pretreatment methods attack different components of the cell wall. Alkaline treatments result in the disruption of lignin, while acidic pretreatments are effective primarily because of hemicellulose solubilization (Kumar and Wyman 2008). Pretreatment efficacy is rated according to the production of reactive fiber, utility of the hemicellulose fraction, and limitation of hydrolysis or fermentation inhibitors in the pretreated material (Singh et al. 2015). High-temperature pretreatments can lead to the generation of inhibitory products such as lignin derivatives and xylose degradation products (Du et al. 2010).
Members of S-1041 are actively involved in pretreatment research using a variety of technologies. Dilute acid pretreatment was examined on alfalfa stems (Zhou et al. 2014; Djioleu and Carrier 2016). The effect of Fenton chemistry on pretreatment was examined by Kato et al. (2014) and He et al. (2015). Ionic liquids as pretreatment agents were also examined (Zhang et al. 2014). AFEX pretreatment was researched (Sundaram et al. 2015; Karki et al. 2015; Rijal et al. 2014). Solid state fermentation pretreatment with various fungal organisms was examined as illustrated in Yao and Nokes (2014) and Flythe et al. (2015). Pryor has studied soaking in aqueous ammonia pretreatment (Nahar and Pryor, 2014; Nahar and Pryor, 2017).
Pretreatments open tightly woven biomass plant cell walls, increasing internal surface area. With more available surface area, enzymes have access to carbohydrate polymers, hydrolyzing hemicellulose into five-carbon sugar xylose and cellulose into six-carbon sugar glucose. The hydrolysis of the treated cellulose is performed with an enzymatic cocktail containing xylanases, β-glucosidases, endo-cellulases and exo-cellulases. These enzymes hydrolyze hemicellulose, cellobiose, as well as the middle and extremities of the cellulose polymer, respectively. It is critical to digest the plant cell wall polysaccharides with the least amount of cellulase and xylanase enzymes; these biocatalysts account for a significant cost component of biochemical processing and have a sizable influence on the economic viability of the biorefinery. Although pretreatment is critical, this processing step unfortunately can lead to the production of compounds that inhibit enzymatic hydrolysis. This saccharification step is obstructed mainly by three groups of compounds: lignin derivatives that cause non-productive binding of the saccharification cocktail (Berlin et al. 2006); xylose-derived compounds inhibiting the enzyme cocktail (Cantarella et al. 2004); and, oligomers and phenolic-derived compounds deactivating the enzymes over time (Kumar and Wyman 2008; Berlin et al. 2006 and Ximenes et al. 2011). To lessen the effect of these inhibitory compounds and minimize the amount of enzymes used, inhibitors must be removed by separation methods such as washing the pretreated biomass with successive volumes of water (Hodge et al. 2008). Members of S-1041 are actively involved in determining the effects of specific inhibitory compounds produced during pretreatment in order to guide and evaluate pretreatment research. By knowing exactly which compounds have the greatest influence, pretreatment processing parameters can be adjusted to minimize their concentration (Rajan and Carrier 2016; Rajan and Carrier 2014).
Although great strides were made in pretreatment and enzymatic hydrolysis research, knowledge gaps remain related to integration of these technologies to produce second-generation production systems that are economically viable energy systems.
Economically feasible biorefinery scenarios must use all the components from the biomass to produce a diverse mix of fuels, materials, and chemicals according to their highest value (Ragauskas et al. 2014). Lignin is the second largest renewable polymer after cellulose and is the largest source of aromatic compounds. However, due to lignin’s amorphous structure and cross-linked nature, its valorization is challenging and requires additional research.
Lignin products have been converted to many products including carbon and composite fibers (Lora and Glasser 2002), adhesives (Yang et al. 2014), concrete plasticizers (Yu et al. 2013), dispersants, surfactants (Thakur et al. 2014), and resins (Kadla et al., 2002). Additionally, lignin derivatives have been used as a feedstock to produce liquid fuels or burned to power the biorefinery.
Challenges that exist for lignin utilization include both separation and purification from biorefinery streams. The chemistry of these streams significantly impacts isolation processes (Fatehi et al. 2011). After extraction and purification, lignin may also require additional modification to achieve the desired properties in material application.
Great progress has been made over the last five years in creating viable feedstock supply chains, deciphering between important and trivial pre- and post-harvest parameters, integrating thermochemical energy production systems, and designing fermentable sugar streams. Knowledge gaps remain in understanding the system environmental impacts, including carbon footprint.
Sustainability metrics such as socioeconomic and environmental indices are needed to assess biorefinery systems (McBride et al., 2011). Effective metrics can help to identify and quantify the sustainability attributes of biorefinery processes and products. However, methodologies and outputs need to be adapted to produce reliable and publically-understandable results. Additionally, tradeoffs between different socioeconomic and environmental indicators need to be considered. Conventional indicators describe both environmental impacts, such as GHGs, eutrophication, net energy, and fossil fuel usage, as well as socioeconomic impacts, such as social well-being, energy security, trade, profitability, and acceptability (Dale et al. 2015; Kline et al., 2017). This suite of indicators will need to apply to the full biorefinery supply chain including: feedstock production and logistics; conversion to fuels, chemicals and materials; and end uses.
- Funding landscape: Challenges and Opportunities for the next five years
Historically, industrial support of biobased energy has fluctuated with energy prices. Following a period of mostly high prices beginning in 2008, low oil prices since 2015 have moderated interest in biobased industry development. Although research funding for the field has also tightened recently, such fluctuations are common and interest is expected to rise again with energy demand and energy prices. Although many biobased industrial development opportunities lie outside of the energy field specifically, many of these markets still compete with petrochemicals or are otherwise tied to energy prices.
Despite the relatively lower funding opportunities for research related to biobased industry development, some level of federal funding has been consistent across a range of agencies for 10 years or longer. A variety of program funding has been available through AFRI (USDA NIFA), BRDI (USDA and DOE), Sun Grant Initiative (USDA, DOE, DOT), NSF, as well other initiatives through the Departments of Agriculture, Energy, Transportation, or Defense. The 2015 AFRI annual review lists bioenergy as the second highest funded program area ($33 million) after food security. A recent grants.gov search listed 9 funding programs posted in 2017 with specific relevance to biomass and bioproducts development. S1041 members see this committee as an important mechanism for maintaining connection, continuity, and versatility in meeting research demands in a dynamic funding environment
Related, Current and Previous Work
This proposed Multi-State Project builds on the accomplishments of previous Multi-State Projects S-1007 and S-1041. The significant accomplishments resulting from participation in S-1041 include:
1) Researchers associated with S-1041 annually generate, on average, over 100 peer reviewed and other publications annually. They have also supervised over 35 MS or PhD theses, published over 8 book chapters and books. Many book or journal publications result from collaboration between experiment stations represented in S-1041.
2) Collaborative funded projects developed among S-1041 members include:
Pryor, S.W., D. Grewell, I. Cannayen. 2016. Use of Pelleted Biomass to Reduce Pretreatment Severity. US Department of Agriculture, North Central Sun Grant Initiative. $150,000.
Wiesenborn, D.P., S.W. Pryor, D. Ripplinger, I. Cannayen, W. Gibbons. 2014. Energy beets: a new industrial sugar source for the production of advanced biofuels. US Department of Transportation, North Central Sun Grant Initiative. $248,099
Wilkins, M.R., D.J. Carrier et al. 2014. Investigating fungal degradation of switchgrass in a controlled storage environment. US Dept. of Agriculture, South Central Sun Grant Initiative. $110,000.
Wilkins, M.R., D.J. Carrier et al. 2013. Decreasing severity of switchgrass pretreatment through biological pretreatment. US Dept. of Agriculture, South Central Sun Grant Initiative. $171,994.
- Hashimoto, S. Khanal, G.S. Murthy et al. 2012. Conversion of high-yield tropical biomass into sustainable biofuels. Biomass Research and Development Initiative. $6.0 million.
- S. Sun, D. Wang, C. Chen et al. 2012. Enhancing economic viability of camelina as biofeedstock: optimization and demonstration of the production system and byproduct development. Biomass Research and Development Initiative $5.079 million
3) Thirty-six S-1041 members conducted 17 site visits and evaluations of projects funded by the USDA/DOE Biomass Research Initiative (BRDI) in FY 2012, FY 2013 and FY 2016. Members conducted the visits in teams and generated project evaluation reports. The reports documented progress, challenges, outcomes and impacts of each project and were submitted to USDA, DOE, Office of Management and Budget, and Congressional leadership to provide an update on funded projects and to guide overall program management activities.
4) Consultations with industry and presentations at bioenergy conferences and professional society meetings. Also, industry representatives presented at several S-1041 annual meetings and networked with members at these meetings. It is anticipated that the proposed Multi-State Project will generate similar activity.
S-1041 is cognizant of the Multi-State Project S-1054, Biobased Fibrous Materials and Cleaner Technologies for a Sustainable and Environmentally Responsible Textile Industry. Objective 1 of the current S-1054 project has some overlap with Objective B (Tasks 2 and 3) in that both committees are looking at polymer production; however, S-1054 focuses on textile applications while S-1041 will include many applications beyond textiles.
Objectives
-
Develop deployable biomass feedstock and supply knowledge, processes and logistics systems that economically deliver timely and sufficient quantities of biomass with predictable specifications to meet efficient handling, storage and conversion process requirements
-
Research and develop technically feasible, economically viable and environmentally sustainable technologies to convert biomass resources into chemicals, energy, materials in a biorefinery methodology including developing co-products to enable greater commercialization potential.
-
Perform system analysis to support and inform development of sustainable multiple product streams (chemicals, energy, and materials) and use the insights from the systems analysis to guide research and policy decisions
Methods
This project is building on the research and findings of the previous cycles of the S-1041 project (2008 – 2018), and the following Objectives and Tasks are based on these findings. This multi-state project proposes the following set of tasks that relate to each of the three objectives (Note: Two-letter state abbreviations identify the agricultural experiment stations that will conduct various tasks):
Objective A: Develop deployable biomass feedstock and supply knowledge, processes and logistics systems that economically deliver timely and sufficient quantities of biomass with predictable specifications to meet efficient handling, storage and conversion process requirements.
Biomass type and availability is dependent on local climate and soil conditions. Cellulosic biomass from agricultural and forestry sources is characterized by a high moisture content, low bulk density, and variable seasonal yields. This objective seeks to identify and evaluate biomass feedstock type and availability, characterize biomass properties, and develop engineered systems that harvest and deliver biomass, to supply biorefineries with abundant and inexpensive biomass feedstocks with predictable characteristics. Overall, this objective leads to production of technology and data for designing scalable supply systems to provide abundant quantities of cost-competitive biomass.
Task 1: Identify and evaluate biomass type and availability for selected geographic regions based on economic, agronomic, and climate conditions (KY, MT, ND, OH).
KY will use combined agronomic, experimental, and economic approaches to evaluate regionally available biomass feedstocks (e.g. industrial hemp and kenaf varieties, switchgrass and algae) in comparison to other energy crops. MT will conduct field and laboratory research to identify and evaluate cellulosic, oilseed, and sugar beet yield and production systems appropriate for the state of Montana. ND will use a technology called Phenocam to determine biomass availability remotely and image analysis to determine plant health, yield, and weed presence throughout the season. Additionally, ND will develop a data-driven, web-based, drought management forage prediction tool. OH will evaluate the availability of corn stover, perennial energy crops (e.g., switchgrass, miscanthus), and oilseeds in Ohio.
Task 2: Characterize feedstock physical and chemical properties throughout the supply chain (AL, CA, IN, MT, ND, OH, OK)
AL, CA, OH, and OK will characterize the physical and chemical properties of various biomass types grown or proposed to be grown in their respective regions. IN will investigate flow behavior of biologically active particulates and determine other feedstock quality parameters necessary for processing and handling solid fuels. MT will characterize the properties of cellulosic biomass and oilseeds that grow well in Montana. MT will also perform genetic engineering and agronomic technology to improve physical and chemical properties of these feedstocks to enhance processing of these feedstocks. ND will evaluate the most useful physical properties (e.g., densities, shear and tensile stresses, calorific value, moisture absorption) of feedstocks new to North Dakota using standard instruments and procedures. Also, ND will characterize particle size distributions (PSD) for biomass feedstocks throughout the processing chain using image processing software tools.
Task 3: Develop harvest, pre-processing, handling, densification, storage, and transportation methods for specific biomass feedstock end-users (AL, KY, ND, OH, OK, PA, TN, WI)
AL, KY, ND, OH, OK, PA and TN will develop biomass pre-processing, storage, and transportation strategies for various types of biomass with the goal of economically delivering timely and sufficient quantities of biomass with predictable specifications to meet conversion process-dictated feedstock tolerances. ND will develop low-cost methods for energy beet juice storage for subsequent conversion to biofuels or bioproducts. WI will research equipment and methods for one pass baling of corn stover and water resistant pellet production.
Objective B: Research and develop technically feasible, economically viable and environmentally sustainable technologies to convert biomass resources into chemicals, energy, and materials in a biorefinery. This objective includes developing co-products to enable greater commercialization potential.
First generation energy (energy produced from oilseeds, sugar crops, grains, and animal waste) production must continue to improve to remain economically competitive and improve sustainability. Despite efforts to advance conversion technologies, development of industrial scale second generation biorefineries, using either biochemical or thermochemical platforms, is still lagging. Improving sugar, bioenergy and/or bioproduct yields will be valuable to enable development. Integration between feedstock supply and conversion could be an important step in improving yields. The multi-state community can facilitate the integration between feedstock logistics and conversion by providing systems-level insight between conversion and biological and physical properties of biomass feedstocks.
Task 1: Develop and assess technologies to produce valuable products from lipids and residuals from lipid processing (AL, CA, IN, KS, MN, ND, SC, SD, TN).
AL will develop robust microbial strains and bioprocesses for converting crude glycerol from biodiesel production to value-added bioproducts. CA will evaluate liquid effluent from anaerobic digestion of animal waste for production of algal biomass and lipids for biodiesel production, develop advanced anaerobic digestion and digestate processing technologies to produce bioenergy and biofertilizers, and advance fungal production and flocculation technologies used for algae and bacterial harvesting. IN will develop wood sealants based on soybean methyl esters. KS will develop and increase the performance of adhesives produced from oilseed proteins and other plant-based proteins. MN will develop and evaluate extended aquaponics systems that integrate a novel anaerobic digestion process to strip ammonia from animal wastewater, a microalgae process to control nutrient balance, and a black soldier fly culture for fish feed. ND will evaluate new, low-value lipids for conversion to methyl esters (biodiesel) and evaluate the quality of those esters. SC will use natural antioxidants from cottonseed oil to improve stability of biodiesel fuels made from cottonseed oil and waste vegetable oils catalyzed by free-enzyme solutions. SC will also investigate replacing expensive nitrogen sources (e.g., yeast extract and/or peptone) with soybean meal and meat bone meal for marine protist fermentation to produce antioxidants. SD will develop an innovative catalytic cracking process to upgrade non-food vegetable oils into “green” hydrocarbon diesel and/or bio jet fuel. TN will investigate the use of immobilized lipases to make biobased surfactants and detergents, particularly sugar-fatty acid esters.
Task 2: Develop and assess technologies to produce valuable products from cereal grains, other starchy crops and food waste (CA, HI, IL, IA, MN, NJ, SC, WI).
CA will evaluate by-products from food processing as alternatives to chemical soil fumigants. HI will investigate producing carbon feedstock from food waste to support immobilized cells in microbial reactors. IL will develop novel processing strategies for producing ethanol and other products from corn. IA will characterize flowability of pig feeds containing corn distillers’ dried grains with solubles (DDGS). IA will produce biopolymer films via cast and extrusion technology using starch from various sources, including cassava byproducts peels. MN will develop innovative biomass cultivation systems that utilize municipal and agricultural wastewaters, electro-flocculation harvest methods, and biomass pretreatment processes. NJ will screen different strains of duckweed (a plant with high starch content) and characterize their physical and chemical properties. SC will study biocementation technologies to produce construction materials and valuable antioxidants from food waste and human waste for use in deep space exploration. WI will develop extraction techniques to isolate hemicellulose and cellulose from corn DDGS.
Task 3: Develop and assess biological conversion technologies to produce valuable products from carbohydrates in cellulosic biomass (AL, CA, HI, IL, IN, IA, KY, NE, ND, OH, OK, PA, SC, SD, WI).
AL will develop microbial strains through metabolic engineering strategies to enhance utilization of low-value feedstocks, improve bioproduct yield and production, and generate new high-value bioproducts. AL will also employ systematic characterization and optimization of bioprocesses to enhance efficiency of biological conversion. CA will: 1) characterize microbiomes from thermophilic decomposition systems to identify new organisms and metabolic pathways for biological conversion, 2) develop new catalysts, including recombinant strains and enzymes, and fermentation processes to convert cellulosic biomass to biofuels and value added products, 3) integrate fermentation and separation technologies to produce volatile fatty acids and biopolymers, and 4) develop new methods to evaluate the efficacy of thermochemical pretreatment on improving enzymatic digestibility of biomass. HI will engineer microbial strains, microbial consortia, and enzymes/proteins for improved biochemical conversion of cellulosic biomass and waste lipid feedstock to biofuels and/or high-value biochemicals. IN will develop extraction and conversion technologies for producing modified nano-cellulose products from hemp bast fiber. IL will develop pretreatment strategies for preparing polysaccharides in cellulosic biomass for enzymatic or acid hydrolysis to produce sugars. IA will investigate pretreatments, including dilute acid and ultrasonication, on soybean hulls and switchgrass for the production of microbial biosurfactants and analogs via Bacillus fermentation and enzyme technology. KY will 1) evaluate products such as essential oils and foods extracted from the grain of industrial hemp, 2) develop new ionic-solvent-based fractionation technologies to extract lignin and facilitate biological conversion of the cellulosic components, 3) investigate conversion of stalks and bagasse to bioderived products to replace traditional pesticides and synthetic chemicals/fertilizers, and 4) examine microbe-root-soil interactions in Kentucky-specific crop and forage systems. NE will investigate cell culture control schemes for biological production of polyhydroxyalkonoates (PHAs) using glucose and xylose from hydrolysis of cellulose and hemicellulose as the substrate. ND will develop and characterize low-severity and low-cost pretreatment options for densified cellulosic biomass and develop systems for enzyme immobilization and reuse to reduce hydrolysis costs. OH will investigate liquid and solid state anaerobic digestion, as well as ethanol and butanol fermentation using lignocellulosic feedstock. OK will develop biochemical conversion processes to convert cellulosic biomass to butanol with high yield and carbon conversion using novel biocatalysts. PA will investigate and develop sustainable technologies to produce chemicals, energy, materials and other value added products from cellulosic biomass, and investigate and develop biomethane as a near term biofuel for natural gas vehicles. SC will develop bioproducts from industrial hemp including “hempcrete” made from lower-energy or solar-powered concrete processing and hemp byproducts and investigate anaerobic digestion to convert food waste, biosolid, crop residues and crude glycerol into biogas as fuel for combined heat and power production. SD will develop effective processes to produce high performance nanocomposites from lignocellulosic biomass for smart packaging applications. WI will develop new pretreatments for anaerobic digestion and ethanol fermentation from lignocellulosic biomass.
Task 4: Develop and assess technologies to produce valuable products from lignin (AL, IN, KS, KY, NE, SC TN, WI).
AL, IN, KY, NE, TN and WI will develop novel catalytic and/or biological conversion methods to upgrade lignin to high value chemicals. KS will develop adhesives derived from lignin. KY will develop technologies to produce carbon composite materials for energy storage applications from lignin. SC will develop novel biotechnology to transform lignin into high-value nutraceuticals and pharmaceuticals as well as lipid feedstock for biodiesel.
Task 5: Develop and assess thermochemical conversion technologies to produce valuable products from cellulosic biomass (AL, KY, MN, OH, OK, TN, TX, WV, WI).
AL, OK, TN, TX, and WV will research gasification, pyrolysis and intermediate upgrading for production of liquid fuels, power and other products. KY will research the efficiency and effectiveness of using white rot fungi as a treatment prior to converting lignocellulosic biomass into bio-oil. MN will develop and assess scalable microwave assisted conversion and upgrading processes to convert a wide range of solid feedstocks to liquid and gaseous fuels and research integrated catalytic reforming and upgrading processes to convert intermediates to high value hydrocarbons. OH will study torrefaction of cellulosic biomass. WI will develop heterogeneous catalysts for conversion of both sugars and bio-oil to produce high-value chemicals.
Task 6: Integrate thermochemical and biological conversion processes to produce valuable products from lignocellulosic biomass (KY, OH, OK, TX).
KY will determine the large-scale feasibility of using algae cultures to mitigate CO2 levels from coal-fired power plants with subsequent utilization of algal lipids as a feedstock for biodiesel production. OH and OK will investigate syngas fermentation processes to produce valuable chemicals from synthesis gas. TX will integrate thermochemical and biological conversion processes to generate fuels, high-value chemicals, and co-products from cellulosic biomass.
Objective C: Perform system analysis to support and inform development of multiple sustainable product streams (chemicals, energy, and materials) and use systems analysis insights to guide research and policy decisions.
Development of environmentally sustainable and economically viable sources of bioenergy and bioproducts is driven by the need to reduce the environmental impact of the energy industry. For this, supply chains capable of providing ample biomass for production of advanced biofuels will be necessary. These supply chains will need to be integrated with commercial scale conversion facilities and connected to viable distribution systems. This objective includes utilization of existing system analysis technology to assist in the development of usable frameworks to facilitate the biorefinery development.
Task 1. Develop system models and data to assess sustainability of integrated conversion platforms (IA, NE, ND, OH, TN, WI).
IA, NE, ND, OH and WI will use process- and LCA-modelling to determine techno-economic and sustainability impacts of conversion platforms. NE will develop empirical, mechanistic, and process control models for hydrolysis and fermentation to optimize the biochemical conversion of cellulose and lignin into value-added products. TN will develop multiscale, multiphysics and reduced order models to better inform biomass preprocessing treatment, handling and conversion in reactors and gasifiers.
Task 2. Develop integrated system models to configure, analyze and optimize bioenergy and biofuel production systems (AL, CA, IN, KY, NJ, NY, OH, OK, PA, SC, WI).
AL, OH, and WI will conduct life cycle analysis of bioenergy/bioproducts production systems for sustainability evaluation. CA will develop decision support systems for spatial analysis and optimization of forestry-based feedstock and bioproduct supply chains. IN, OH, OK and WI will develop feedstock logistics models for supplying agricultural residues, energy crops, and oilseeds to biorefineries. KY will develop integrated agronomic, techno-economic models to evaluate regionally available biomass feedstocks such as different industrial hemp and kenaf varieties in comparison to other energy crops. NJ will develop a pilot system linking duckweed production to aquaculture and analyze the system to determine if duckweed is an environmentally sustainable and economically viable source for bioproducts. NY will define the productivity and environmental impacts of perennial grass bioenergy production on marginal soils in New York on a large-scale, long-term (7+ year) experimental bioenergy system growing switchgrass and miscanthus. NY will also develop risk management strategies specific to bioenergy and bioproducts that are accessible to industrial scale producers. Tasks include uncertainty analysis, conversion process modeling, and development of optimal policies for facility operations under various market conditions. PA will develop systems approaches and models to support development of sustainable biomass production and conversion to bioenergy and bioproducts. They will also evaluate soil and water quality benefits of producing perennial biomass crops on environmentally vulnerable parts of the landscape. SC will develop a system model for a regional integrated biofuels network in the Southeast with emphasis on sustainability measures and coupled models with transportation, power networks, and water systems
Measurement of Progress and Results
Outputs
- A web site (https://probe.osu.edu/s1041) that was launched during the current project will be updated and maintained.
- A large portion of the efforts outlined in Objectives A through C are application oriented and will be useful to develop pilot projects, demonstrations and commercialization of biomass conversion to biobased products.
- Publications in peer reviewed journals, trade journals and popular magazines
- Patents and patent applications
- Presentations to economic development groups, legislative groups, and to the general public
- Posters and oral presentations at scientific and engineering conferences
Outcomes or Projected Impacts
- The committee will continue to serve as a resource for the Biomass Research and Development Initiative (BRDI) (or similar program included in the next Farm Bill), Biomass, Research and Development Board working groups, USDA and NSF SBIR, NSF, NIFA and DOE panels
- Multi-state membership will contribute to identification of funding priorities and shaping policy of Federal agencies through participation in working groups, advisory groups, and workshops
- The proposed research will enable reduced dependency on foreign petroleum
- The proposed research will encourage investment in the agricultural sector and in rural communities where the feedstocks required for biobased industry are located.
Milestones
Projected Participation
View Appendix E: ParticipationOutreach Plan
The newly designed web site will be critical to disseminate the results and information that the group has generated. The Newsletter also will be essential to synthesize and disseminate the group’s progress. Tackling a yearly problem and inviting specific industry stakeholders, economists, and social scientists to present and attend the Symposium will be essential to build important leverages with industrial partners.
Research results will be made available through peer-reviewed journal articles. Participants in the previous Multi-State Project S-1041 generated over 100 peer reviewed and other publications annually, either independently or in collaboration with other experiment stations represented by S-1041. It is expected that similar productivity will be seen in this Multi-State Project. Many articles can be accessed through individual participant web sites. Workshops and demonstrations will be organized by participating stations for feedstock production, harvesting, storage and conversion.
It is expected that this Multi-State Project will continue to foster scientific collaboration for collaborative proposals. Participants will meet once per year to review progress made towards attaining Objectives A, B and C. Because of the multi-disciplinary nature of this Multi-State Project, participants also often meet in scientific conference settings, planning committees, and in scientific review panels.
Organization/Governance
The Development Committee will use a standard form of governance using a chair, vice chair and secretary as executive officers. During annual meetings, a secretary will be elected that ascends to vice chair, then chair. Terms are for one year. Subcommittees will be formed as needed during annual meetings.
Literature Cited
Aita G, Salvi D and Walker M. 2011. Enzyme hydrolysis and ethanol fermentation of dilute ammonia pretreated energy cane. Bioresource Technology 102: 4444–4448.
Berlin A, Balakshin M, Gilkes N, Kadla J, Maximenko V, Kubo S and Saddler J. 2006. Inhibition of cellulase, xylanase and β-glucosidase activities by softwood lignin preparations. Journal of Biotechnology 125:198-209.
Bischoff K, Gravois K, Reagan J, Hoy J and Kimbeng C. 2008. Registration of L 79–1002 sugarcane. Journal of Plant Registrations 2: 211–217.
Brar J, Singh K, Zondlo J, Wang J and Kumar S. 2013. Co-gasification of coal and biomass- A review. Biomass & Bioenergy 2:25-40.
Cantarella M, Cantarella L, Gallifuoco A, Spera A and Alfani F. 2004. Effect of inhibitors released during steam-explosion treatment of poplar wood on subsequent enzymatic hydrolysis and SSF. Biotechnology Progress 20: 200-206.
Capunitan J and Capareda S. 2012. Assessing the potential for biofuel production of corn stover pyrolysis using a pressurized batch reactor. Fuels 95: 563-572.
Chen C, Bekkerman A, Keshavarz-Afshar R and Neil K. 2015. Intensification of dryland cropping systems for bio-feedstock production: Evaluation of agronomic benefits of Camelina sativa. Industrial Crops and Products 71:114-121.
Chevanan N, Womac A, Bitra V and Sokhansanj S. 2011. Effect of particle size distribution on static and tapped densities of selected biomass after knife mill size reduction. Applied Engineering in Agriculture 27: 631-644.
Chevanan N, Womac A, Bitra V, Yoder D and Sokhansanj. 2009. Flowability parameters for chopped switchgrass, wheat straw and corn stover. Powder Technology 193:79-86.
Dale B, Anderson J, Brown R, Csonka S, Dale V, Herwick G, Jackson R, Jordan N, Kaffka S, Kline K, Lynd L, Malmstrom C, Ong R, Richard T, Taylor C and Wang M. 2014. Take a closer look: biofuels can support environmental, economic and social goals. Environmental Science and Technology 48:7200-7203.
Dale V, Efroymson R, Kline K, and Davitt M. 2015. Framework for selecting indicators of bioenergy sustainability. Biofuels, Bioproducts and Biorefining 9: 435-446.
Djioleu A and Carrier DJ. 2016. Effects of dilute acid pretreatment parameters on sugar production during biochemical conversion of switchgrass using a full factorial design. ACS Sustainable Chemistry and Engineering 4: 4124-4130.
Du B, Sharma L, Becker C, Chen S-F, Mowery R, van Walsum P and Chambliss CK. 2010. Effect of varying feedstock pretreatment chemistry combinations on the formation and accumulation of potentially inhibitory degradation products in biomass hydrolyzates. Biotechnology and Bioengineering 107: 430-440.
Du Z, Wan Y, Li Y, Chen Q, Lin X, Chen P and Ruan R. 2011. Microwave-assisted pyrolysis of microalgae for biofuel production. Bioresource Technology 102: 4890-4896.
Flythe M, Elia N, Schmal M and Nokes S. 2015. Switchgrass (Panicum virgatum) fermentation by Clostridium thermocellum and Clostridium beijerinckii sequential culture: effect of feedstock particle size on gas production. Advances in Microbiology 5:311-316.
Fatehi P, Ni Y. Integrated forest biorefinery-prehydrolysis/dissolving pulping process. In: Zhu J, Zhang X, Pan X, editors. Sustainable production of fuels, chemicals, and fibers from forest biomass, American chemical society symposium series, NY, 1067. Washington, DC: American Chemical Society; 2011. p. 475–506.
Gautam G, Brodbeck C, Adhikari S, Bhavnani S, Fasina O and Taylor S. 2011a. Gasification of wood chips, agricultural residues and waste using a downdraft gasifier in a commercial gasifier. Transaction of ASABE 54:1801-1807.
Gautam G, Adhikari S, Gopakumar S, Brodbeck C, Bhavnani S and Taylor S. 2011b. Tar analysis in syngas derived from pelletized biomass in a commercial stratified downdraft gasifier. BioResources 6: 4652-4661.
Hart Q, Tittmann P, Bandaru V and Jenkins M. 2015. Modeling poplar growth as a short rotation woody crops for biofuels in the Pacific Northwest. Biomass & Bioenergy 79:12-27.
Haryanto A, Fernando S, To S, Steele P, Pordesimo L and Adhikari S. 2011. High temperature water gas shift reaction over nickel catalysts for hydrogen production: effect of supports, GHSV, metal loading, and dopant materials. Journal of Thermodynamics & Catalysis 2: 106 doi:10.4172/2153-0645.1000106
He Y, Ding Y, Xue Y, Yang B, Liu F, Wang C, Zhu Z, Qing Q, Wu H, Zhu C, Tao Z and Zhang D. 2015. Enhancement of enzymatic saccharification of corn stover with sequential Fenton pretreatment and dilute NaOH extraction. Bioresource Technology 193: 324–330.
Heaton E, Dohleman F and Long S. 2008. Meeting US biofuel goals with less land: the potential of Miscanthus. Global Change Biology14: 2000-2014.
Heaton E, Dohleman F, Miguez A, Juvik J, Lozovaya V, Widholm J, Zabotina O, McIssac G, David M, Voight T, Boersma N and Long S. 2010. Miscanthus: a promising biomass crop. Advances in Botanical Research 56: 76-137.
Hochman G, Rajagopal D, Timilsina G and Zilberman D. 2014. Quantifying the causes of the global food commodity price crisis. Biomass & Bioenergy 68:106-114.
Hodge D, Karim N, Schell D and Macmillan J. 2008. Soluble and insoluble solids contributions to high-solids enzymatic hydrolysis of lignocellulose. Bioresource Technology 99: 8940-8948.
Hu J, Yu F and Lu Y. 2012. Application of Fischer-Tropsch synthesis in biomass to liquid conversion. Catalysts 2:303-326.
Iman T and Capareda S. 2012. Characterization of bio-oil, syngas and bio-char from switchgrass pyrolysis at various temperatures. Journal of Analytical and Applied Pyrolysis 93:170-177.
Karki B, Muthukumarappan K, Wang Y, Dale B, Balan, V and Gibbons W. 2015. Physical characteristics of AFEX-pretreated and densified switchgrass, prairie cord grass, and corn stover. Biomass & Bioenergy 78: 164-174.
Kline K, Msangi S, Dale V, Woods J, Souza G, Osseweijer P, Clancy J, Hilbert J, Johnson F, McDonnell P and Mugera H. 2017. Reconciling food security and bioenergy: priorities and action. Global Change Biology Bioenergy 9: 557-576.
Jin W, Singh K and Zondlo J. 2015. Co-processing of pyrolysis vapors with bio-chars for ex-situ upgrading. Renewable Energy 83:638-645.
Kadla J, Kubo S, Venditti R, Gilbert R, Compere A and Griffith W. 2002. Lignin-based carbon fibers for composite fiber applications. Carbon 40:2913–20.
Kato D, Elia N, Flyth M and Lynn B. 2014. Pretreatment of lignocellulosic biomass using Fenton chemistry. Bioresource Technology 162: 273–278.
Kim S, Guo J, Kwak S, Jin Y, Lee D and Singh V. 2015. Effects of genetic variation and growing condition of prairie cordgrass on feedstock composition and ethanol yield. Bioresource Technology 183: 70-77.
Kumar R and Wyman C. 2008. Effect of enzyme supplementation at moderate cellulose loadings on initial glucose and xylose release from corn stover solids pretreated by leading technologies. Biotechnology & Bioengineering 102:457-467.
Kumar A, Wang L, Yuris D, Jones D.D, and Hanna M. 2008. Thermogravimetric characterization of corn stover as gasification and pyrolysis feedstock. Biomass & Bioenergy 32:460-467.
Kumar A, Eskridge K, Jones D and Hanna M. 2009. Steam-air fluidized bed gasification of distiller’s grains: effects of steam to biomass ratio, equivalence ratio and gasification temperature. Bioresource Technology 100:2062-2068.
Liu L, Ye X, Womac A and Sokhansanj S. 2010. Variability of biomass chemical composition and rapid analysis using FT-NIR techniques. Carbohydrate Polymers 81: 820–829.
Lora JH and Glasser WG. 2002. Recent industrial applications of lignin: a sustainable alternative to nonrenewable materials. Journal of Polymers and the Environment 10:39–48.
Lu Y, Zhou P, Han J and Yu F. 2015. Fischer-Tropsch synthesis of liquid hydrocarbon over mesoporous SBA-15 supported cobalt catalysts. RSC Advances. 5:59792-59803.
Lu Y, Yu F, Hu J and Liu J. 2012. Catalytic conversion of syngas to mixed alcohols over Zn-Mn promoted Cu-Fe based catalyst. Applied Catalysis A, General. 429430: 48-58.
Lynd L, Laser M, Bransby D, Dale B, Davison B, Hamilton R, Himmel M, McMillan J, Sheehan J and Wyman C. 2008. How biotech can transform biofuels. Nature Biotechnology 26:169-172.
Mac an Bhaird S, Eilin W, Hemmingway P, McDonnell K et al. 2014. Analysis of bed agglomeration during gasification of wheat straw in a bubbling fluidized bed gasifier using mullite as bed material. Powder Technology 254: 448-459.
McBride A, Dale V, Baskaran L, Downing M, Eaton L, Efroymson R, Garten Jr C, Kline K, Jager H, Mulholland P, Parish E, Schweizer P and Storey M. 2011. Indicators to support environmental sustainability of bioenergy systems. Ecological Indicators 11:1277-1289.
Moen J, Yang C, Zhang B, Lei H, Hennessy K, Wan Y, Le Z, Liu Y, Chen P and Ruan R. 2010. Catalytic microwave assisted pyrolysis of aspen. International Journal of Agricultural and Biological Engineering 2:70-75.
Mochizuki J, Coffman M and Yanagida J. 2015. Market, welfare and land implications of lignocellulosic bioethanol in Hawai’i. Renewable Energy 76: 102-114.
Nahar N and Pryor S. 2017. Effects of reduced severity ammonia pretreatment on pelleted corn stover. Industrial Crops and Products 109C:163-172.
Nahar N and Pryor S. 2014. Reduced pretreatment severity and enzyme loading enabled through
Patil K, Bhoi P, Huhnke R and Bellmer D. 2011. Biomass downdraft biomass gasifier with internal cyclonic combustion chamber: design, construction, and experimental results. Bioresource Technology 102: 6286-6290.
Pothula A, Igathinathane C and Kronberg S. 2015. Profile based image analysis for identification of chopped biomass stem nodes and internodes. Industrial Crops and Products 70:374-382.
Qian K, Kumar A, Bellmer D, Yuan W, Wang D and Eastman M. 2015. Physical properties and reactivity of char obtained from downdraft gasification of sorghum and eastern red cedar. Fuel 143: 383-389.
Ragauskas A, Beckham G, Biddy M, Chandra R, Chen F, Davis M, Davison B, Dixon R, Gilna P, Keller M, Langan P, Naskar A, Saddler J, Tschaplinski T, Tuskan G and Wyman C. 2014. Lignin valorization: improving lignin processing in the biorefinery. Science 344 doi: 10.1126/science.1246843.
Rajan K and Carrier DJ. 2016. Insights into exo-cellulase inhibition by the hot water hydrolyzates of rice straw. ACS Sustainable Chemistry and Engineering 4:3627–3633.
Rajan K and Carrier DJ. 2014. Characterization of rice straw prehydrolyzates and their effect on the hydrolysis of model substrates, using a commercial endo-cellulase, β-glucosidase and cellulase cocktail. ACS Sustainable Chemistry and Engineering 2:2124–2130.
Rijal B, Biersbach G, Gibbons W and Pryor S. 2014. Effect of initial particle size and densification on AFEX-pretreated biomass for ethanol production. Applied Biochemistry and Biotechnology 174:845–854.
Sharara M, Clausen C and Carrier DJ. 2012. An overview of biorefinery technology in biorefinery co-products: phytochemicals, primary metabolites and value-added biomass processing (Wiley Series in Renewable Resource). Bergeron C, Carrier DJ and Ramaswamy S. (eds). John Wiley & Sons. Pp.1-18.
Shao X, DiMarco K, Richard T and Lynd L 2015. Winter rye as a bioenergy feedstock: impact of crop maturity on composition, biological solubilization and potential revenue. Biotechnology for Biofuels 8:35
Singh S, Cheng G, Sathitsuksanoh N, Wu D, Varanasi P, George A, Balan V, Gao X, Kumar R, Dale B, Wyman C and Simmons B. 2015. Comparison of different biomass pretreatment techniques and their impact on chemistry and structure. Frontiers in Energy Research https://doi.org/10.3389/fenrg.2014.00062
Singh M, Erickson J, Sollenberger L, Woodard K, Vendramini J and Fedenko J. 2012. Mineral composition and biomass partitioning of sweet sorghum grown for bioenergy in the southeastern USA. Biomass & Bioenergy 47:1-8.
Street J, Yu F, Warnock J, Wooten J and White MG. 2012a. Design and testing of a LabVIEW controlled catalytic packed-bed reactor system for production of hydrocarbon fuels. Transactions of the ASABE. 55: 1047-1055.
Street J, Yu F, Wooten J, Columbus E, White MG and Warnock J. 2012b. Gasoline-range hydrocarbon production using biomass derived synthesis gas over Mo/H+ZSM-5. Fuel. 96:239–249.
Street J and Yu F. 2011. Production of high-value products including gasoline hydrocarbons from thermochemical conversion of syngas. Biofuels 2:677-691.
Sundaram V, Muthukumarappan K and Kamireddy S. 2015. Effect of ammonia fiber expansion (AFEX™) pretreatment on compression behavior of corn stover, prairie cord grass and switchgrass. Industrial Crops and Products 74: 45-54.
Thakur V, Thakur M, Raghavan P and Kessler M. 2014. Progress in green polymer composites from lignin for multifunctional applications: a review. ACS Sustainable Chemistry and Engineering 2:1072–92.
Tilman, D, Blazer C, Hill J and Befort B. 2011. Global food demand and the sustainable intensification of agriculture. Proceedings of the National Academy of Sciences 108: 20260-20264.
Wan Y, Chen P, Zhang B, Yang C, Liu Y, Lin X and Ruan R. 2009a. Microwave-assisted pyrolysis of biomass: Catalysts to improve product selectivity. Journal of Analytical and Applied Pyrolysis. 86:1611.
Wan Y, Liu Y, Lin X, Yang C, Zhang B, Chen P, Lei H and Ruan R. 2009b. Microwave assisted pyrolysis of corn stover pellets with catalysts for bio-oil production and its component. Transactions of the Chinese Society of Agricultural Engineers 25:190- 195.
Wan Y, Wang Y, Lin X, Liu Y, Chen P, Li Y and Ruan R. 2010. Experimental investigation on microwave assisted pyrolysis of algae for rapid bio-oil production. Transactions of the CSAE 26:295-300.
Wang, H., D. Livingston, R. Srinivasan, Q. Li, P. Steele, and F. Yu. 2012. Detoxification and fermentation of pyrolytic sugar for ethanol production. Applied Biochemistry and Biotechnology. 168:1568–1583.
Wang H, Srinivasan R, Yu F, Steele P, Li Q, Mitchell B and Samala A. 2012. Effect of acid, steam explosion, and size reduction pretreatments on bio-oil production from sweetgum, switchgrass, and corn stover. Applied Biochemistry and Biotechnology. 167: 285-297.
West D and Kincer D. 2011. Yield of switchgrass as affected by seeding rates and dates. Biomass & Bioenergy 35:4057-4059.
Wang Z and Cheng J. 2011. Lime pretreatment of coastal Bermuda grass for bioethanol production. Energy & Fuels 25: 1830-1836.
Wijayapala R, Pittman C, Yu F and Mlsna T. 2014. Synthesis of alkylated aromatic hydrocarbons from CO/H2mixtures over Molybdenum/ZSM/ water gas shift catalysts. Applied Catalysis A, General 480:93-99.
Xie Q, Chen P, Peng P, Liu S, Peng P, Zhang B, Cheng Y, Wan Y, Liu Y and Ruan R. 2015. Single-step synthesis of DME from syngas on CuZnAl/zeolite bifunctional catalyst: the influence of zeolite type. RSC Advances 5:26301–26307.
Ximenes E, Kim Y, Mosier N, Dien B and Ladisch M. 2011. Deactivation of cellulases by phenols. Enzyme and Microbial Technology 48:54-60.
Xu F, Zhou L, Zhang K, Yu J and Wang D. 2015. Rapid determination of both structural polysaccharides and soluble sugars in sorghum biomass using near infrared spectroscopy. BioEnergy Research 8: 130-136.
Yao W and Nokes S. 2014. Phanerochaete chrysosporium pretreatment of biomass to enhance solvent production using solid-substrate cultivation. Biomass & Bioenergy 62:100-107.
Yan Q, Yu F, Cai Z and Zhang J. 2012a. Catalytic upgrading nitrogen-rich wood syngas to liquid hydrocarbon mixture over a Fe–Pd/ZSM-5 catalyst. Biomass & Bioenergy. 47: 469-473.
Yan Q, Yu F, Liu J, Street J, Gao J, Cai Z and Zhang J. 2012b. Catalytic conversion wood syngas to synthetic aviation turbine fuels over a multifunctional catalyst. Bioresource Technology.127:281-290.
Yang D, Li H, Qin Y, Zhong R, Bai M, and Qiu X. 2014. Structure and properties of sodium lignosulfonate with different molecular weight used as dye dispersant. Journal of Dispersion Science and Technology 36:532–539.
Yu G, Li B, Wang H, Liu C and Mu X. 2013. Preparation of concrete superplasticizer by oxidation-sulfomethylation of sodium lignosulfoanate. BioResources 8: http://ojs.cnr.ncsu.edu/index.php/BioRes/article/view/3435
Zhang L, Lu Z, Verlarde L, Fu L, Pu Y, Ding S and Ragauskas A. 2015. Vibrational spectral signature of cellulose microfibrils using high resolution broadband sum frequency generation vibrational spectroscopy (HR-BB_SFG-VS), Cellulose 22:1469-1484.
Zhang X, Xu J and Cheng J. 2011. Pretreatment of corn stover for sugar production with combined Alkaline Reagents. Energy & Fuels 25: 4796-4802.
Zhang J, Feng L, Wang D, Zhang R, Liu G and Cheng G. 2014. Thermogravimetric analysis of lignocellulosic biomass with ionic liquid pretreatment. Bioresource Technology 153:379-382.
Zhang C and Runge T. 2011. Fractionating pentosans and hexosans in hybrid Poplar. Industrial & Engineering Chemistry Research 51: 133-139.
Zhao X, Wei L, Julson J, Qiao Q, Dubey A and Anderson G. 2015. Catalytic cracking of non-edible sunflower oil over ZSM-5 for hydrocarbon bio-jet fuel. New Biotechnology 32:300-312.
Zhou S, Weimer P, Hatfield R, Runge T and Digman M. 2014. Improving ethanol production from alfalfa stems via ambient-temperature acid pretreatment and washing. Bioresource Technology 170: 286–292.