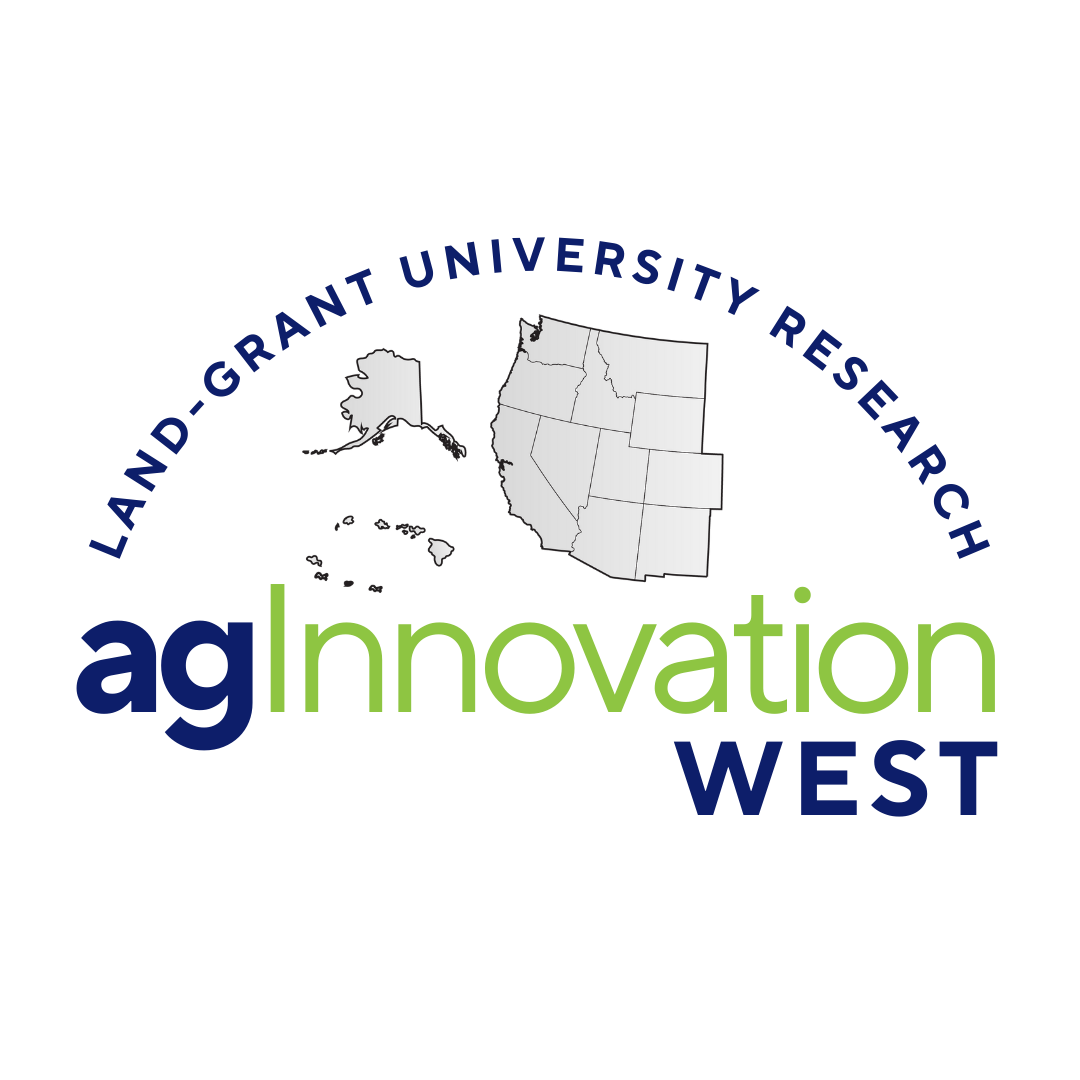
W4186: Variability, Adaptation and Management of Nematodes Impacting Crop Production and Trade
(Multistate Research Project)
Status: Inactive/Terminating
W4186: Variability, Adaptation and Management of Nematodes Impacting Crop Production and Trade
Duration: 10/01/2018 to 09/30/2023
Administrative Advisor(s):
NIFA Reps:
Non-Technical Summary
Statement of Issues and Justification
The Need as Indicated by Stakeholders:
Estimates over the last 30 years indicate that plant-parasitic nematodes cause 10-14% average annual yield loss among the world's major crops (Sasser and Freckman, 1987), and losses ranging from minimal in some localities to as high as 15% in other areas in United States’ major crops (Koenning et al., 1999; McSorley et al., 1987). More recent surveys of nematode-associated crop losses indicate that these estimates remain at similar levels. In economic terms, these estimated annual crop losses translate to at least $8 billion in the United States and $80 billion worldwide (Jones et al., 2013; Nicol et al., 2011; Smiley, 2005). These are likely to be under-estimates since in many production systems incipient losses are probably missed. In addition, economic loss associated with increasing material and application costs of nematicides and associated with trade embargos due to actual or suspected quarantine nematode infestations exacerbate nematode problems in agriculture. Increasingly, scientific evidence and public awareness have heightened concerns about environment quality, food quality, and human health and safety relative to pest management in agricultural production. The need for alternative, integrated nematode management has been propelled by the actions triggered by the Montreal Protocol and the Food Quality Protection Act (FQPA) of the 1990’s. Phase-out of methyl bromide and the loss of several highly effective organo-phosphate and carbamate nematicides has occurred over the last decade. In addition, the soil fumigant nematicide 1,3-dichloropropene (Telone II) is a B2 carcinogen, reviewed under FQPA and must be used under more rigorous restriction and recently supplies have been limited. Together with another fumigant, metam-sodium, the fumigant nematicides have been identified by California EPA as the largest agricultural source VOC (volatile organic compound) contributors to air pollution by ground level ozone formation. Recent US EPA Phase 2 labeling for all soil fumigants, implemented in 2012, establishes mandatory buffer zones surrounding treated fields that further restricts use. However, novel, safer nematicide chemistries are now available for testing as components of integrated nematode management programs.
Both locally and nationally, the agricultural production community (our stakeholders) is scrambling to find viable, sustainable alternatives to traditional chemical-based soil pathogen and nematode control. In addition, world travel and commerce have accelerated the dissemination of pest species, including plant-parasitic nematodes. Development and application of new diagnostic protocols for accurate identification of nematode species are imperative for national and international regulatory and quarantine agencies relative to free trade and economics, and new protocols are available for testing or use. Trade restrictions on movement of potatoes due to findings of quarantine-status cyst or root-knot nematodes in US production areas such as Idaho and the Pacific Northwest provide examples. The nematology community has repeatedly advocated the need for funding support focused on the basic and applied research required to advance agro-ecologically sustainable alternative management approaches and accurate nematode detection and diagnostics. This proposed project renewal addresses these needs directly for the most important groups of plant parasitic nematodes, by building on advances made in the W-3186 project over the last 5 years.
Importance of, and Consequences Without, the Work:
Cyst, root-knot, lesion and other nematode species included in this project are the most important groups of plant-parasitic nematodes in the United States and globally (Jones et al., 2013). Management of these nematodes in US agriculture has been largely via the application of broadly efficacious nematicides. Nematicidal activity, especially of soil fumigants, is generally non-discriminating between nematode species and genera. Therefore, understanding the genetic variability and adaptation potential among nematodes was not important for effective nematode control. In contrast, desirable alternative nematode management strategies involve combinations of crop rotation, host plant resistance, cultural manipulations, and biological control. All of these tactics may have specific genotype-level interactions with nematodes and are influenced by the production practices and environmental conditions. In addition, preliminary evidence indicates that the promising new nematicide products show differential efficacy among main nematode groups (e.g., root-knot versus cyst nematodes). Hence, variability and adaptation in nematode populations must be considered to successfully develop and deploy alternative management strategies. This multistate project was initiated because the membership recognized the increasing importance of characterizing the genetic variation in nematode populations and its influence on success of alternative management strategies. For example, many years of research went into the development of cyst-nematode resistant soybeans and root-knot nematode resistant tomatoes, but in both cases rapid selection of resistance-breaking nematode isolates has eroded their management efficacy. The biological processes in nematodes that influence the development of effective management strategies are complex, involving changes over multiple generations and interactions between nematode, host plant and environment. A renewal of the project as requested herein is critical for continuing the research and application required to meet the overall project goals.
An important difference from traditional broad-spectrum nematicides is that the alternatives are influenced directly by genetic variability existing in target nematode field populations. Hence, the successful use of alternatives requires more information to implement than nematicide-based strategies. This project will advance the assessment and characterization of variability and adaptation extant in nematode populations which in turn will assist in the successful development and application of alternative management approaches. For example, knowing the frequency of virulence genes in a nematode population will allow deployment of corresponding resistance genes and promotion of resistance durability.
Research conducted under the current W-3186 multistate project has provided considerable evidence that genetic variability in nematode populations is important in nematode management systems. Results from current work indicate that genetic variability and adaptation potential in nematode populations are responsible for the aberrant and inconsistent results of many experiments assessing resistance, crop rotations, host ranges, cover/trap cropping, biological control, and new nematicides. The plasticity of nematode responses to abiotic environmental factors such as temperature, moisture, soil conditions, and host nutrient status stems from genetic variability, and such responses require additional characterization. Greater understanding of nematode genetic response and adaptation to abiotic factors will be important in optimizing the design of cultural management tactics such as manipulations of planting and harvest times, wet or dry fallow, and soil solarization. The potential for invasive nematode pests to establish in our agricultural production systems also can be better determined from studies on genetic response and adaptation to local environments.
Without the proposed work continuing in a coordinated manner, the participants believe that effective nematode management alternatives will be developed more slowly, with success coming more on an ad hoc basis and with economic inefficiencies and a high likelihood of short-term failure of new products or management approaches. Knowledge gained from our main focus on cyst, root-knot and other significant nematodes will be applied and tested between nematode groups within the project matrix (see Table 1 in Attachments). This will strengthen the overall scientific scope of the research activities and will broaden the impact of the findings to benefit agriculture in multiple states.
Technical Feasibility of the Research:
Recent advances in molecular and genetic methodologies and knowledge will facilitate the study of nematode genetic variability and adaptation and promote diagnostic protocols with much greater resolution than has been possible. Some of these protocols have been developed and tested under the current project. For example, shared root-knot and cyst populations led to characterization of a mitochondrial cytochrome oxidase I (COI) gene that promises to alleviate current ambiguities in molecular species identification within these difficult-to-identify genera. Rapid advances in DNA-based diagnostics and genetic analyses generated by the current and other projects and their coupling to on-line databases and knowledge-based systems will assist in information transfer to user groups in the relevant agricultural communities.
The root-knot and cyst nematodes are distributed throughout the United States and are damaging pathogens, parasitizing a wide range of important crops. Three groups of nematodes are the primary focus for this project: Group I - The warm-temperature root-knot species (Meloidogyne incognita, M. javanica, M. arenaria); Group II - The temperate root-knot species (M. chitwoodi and M. hapla); Group III - The cyst species (Heterodera schachtii, H. cruciferae, H. glycines, Globodera pallida, G. ellingtonae). These nematodes are the subject of research efforts in the designated participating states. Current research is addressing many areas of management for these three groups, including: development and deployment of nematode-resistant plants; rotation to reduce population densities of these pathogens; cover crops, trap crops and soil amendments including green manures to reduce population densities; the role of weed hosts in bringing about phenotypic changes in nematode populations; characterization of resistance genes and host resistance responses; the development of molecular diagnostic protocols for nematode identification and the reference databases necessary for their implementation. Thus, the project participants share strong common interests that will provide the central focus for both project members and other collaborators. In addition, parallel studies will be made by some participants on other endoparasitic nematodes, reniform nematode (Rotylenchulus reniformis), lesion nematodes (Pratylenchus spp.), stem and bulb nematodes (Ditylenchus spp.), and important ectoparasitic nematodes including stubby root (Paratrichodorus spp.), dagger (Xiphinema spp.), and ring (Mesocriconema) nematodes. This will maximize both the scientific scope of the project and its multi-state impact in agriculture.
Characterizing genetic variability – requisite for novel management strategies:
The unifying theme of this proposal is that genetic variability is a critical biological feature that complicates management and enhances the pest status of nematode species and populations. W-3186 participants and others have been documenting the extent of genetic variability within populations, and the agro-environmental factors that influence it. Rapid developments in genomic techniques and their application through this project will continue to increase our understanding of the genetic processes involved. Failure of current nematode management, such as breakdown of resistance, and successful development of novel approaches can be resolved through greater understanding of the underlying genetic and biological processes in parasitic nematode populations vis a vis management. For example, the importance of mutation compared to maintained variability in field populations is unclear, and this is a research area that will be pursued.
Genetic variability can impact both the effectiveness and longevity of alternative nematode-management strategies based on host plant resistance, crop rotation, cultural manipulations and biological control. Therefore, continuing the knowledge development in these systems should provide rational guidance for the design and development of management strategies. The project focus is on understanding nematode variability and adaptation, such that it can be identified, characterized, and managed or manipulated to benefit agricultural production systems. This requires research on the phenotypic and genotypic characterization of variability and gene frequencies, including aspects of stability and adaptability, of host range, response to resistance, response to environmental conditions, biological processes (e.g. fecundity) and morphology. This approach is being complemented and aided by development of markers to identify variability by molecular, histochemical, and morphological polymorphisms. The development of molecular techniques with greater efficiency, predictability and ease of use will expedite nematode genetic analyses and design of management systems.
Current and previous work under W-3186 has allowed participants to make advances on these research goals. However, this work cannot be considered “complete” and pressure for alternatives to existing nematicides has increased. Relative to our objectives, it is exciting that the arsenal of established and new tools used to address our applied research questions is increasing rapidly.
Four key considerations based on nematode genetic variability are central to development and deployment of alternative management strategies as proposed under this multistate project:
- Host plant resistance – The genetic composition of nematode populations is changed by the selection pressure imposed by growing resistant cultivars. The changes include shifts in species composition, and shifts in presence and frequency of nematode virulence alleles matching specific resistance genes in crop cultivars (Petrillo et al., 2006). Similar potential shifts may occur in response to nematode-resistant trap crops. Little is known of the existing frequency of virulence alleles, the frequency with which new alleles are generated, or the underlying mechanisms that regulate changes in genetic variability in root-knot, cyst and other nematode populations. As more sources of resistance are bred into cultivars, knowledge of gene frequency and stability effects assumes greater importance in determining the direction and requirements of breeding for nematode resistance, and the effective long-term deployment of available resistant cultivars (Starr et al. 2008).
- Host range for rotations and cover-cropping - The host ranges of important nematode species have been defined within general limits, but the extent of variability in host range among populations within species is not well-characterized. Thus, although most cyst nematodes have narrow host ranges and are amenable to control by non-host rotation programs, less is known about the extent of reported hosts outside the typical host taxa, the likelihood of shifts in host range, or the host ranges of new species. For example, although sugarbeet cyst nematode hosts are found almost entirely within the Brassicaceae and Chenopodiaceae, tomato (Solanaceae) has been reported to host this nematode in California and Utah, with potentially serious consequences for rotation planning in western sugarbeet production areas. For the new cyst species Globodera ellingtonae in Oregon, little host range data are available. Evidence for genetic adaptations and modification of nematode host range has also been presented whereby local nematode populations are better adapted to local weed populations. The processes involved in these interactions are poorly understood.
In contrast to cyst nematodes, root-knot nematode species have broad host ranges of more than 2000 plant species from diverse plant families. Much of this host range information has been compiled from numerous tests and observations based on non-standardized host testing procedures, and in most cases with only one or a few isolates per species. Standardized conditions are needed to determine whether differences are due to variability in nematode populations or to differences in susceptibility in the plant lines used. For example, there is evidence that local weed populations influence the behavior of root-knot nematode populations on subsequent crops (Trojan et al., 2007). Resolving the true levels and stability of host range relationships will be critical to development and implementation of non-host crops in rotation and cover-cropping programs, and for determining the role that host weed species play in maintaining nematode population levels.
- Cultural and Biological controls - Manipulating abiotic effects on nematode populations to suppress nematode activity or infection are key strategies. Examples include wet or dry fallowing so nematodes starve while active (wet fallow) or die from extreme moisture stress (dry fallow). Soil solarization involves natural heating of soil under plastic cover to attain the thermal death point of nematodes. Avoidance may include changes in planting and harvest dates, such as delaying planting in the fall to avoid infection activity, and early planting or late harvest of crops to avoid additional nematode generations. Genetic variability in nematodes for response to temperature and moisture has been demonstrated, but little is known about underlying mechanisms or stability. Soil amendments (compost, green manures, various bio-products) show promise for nematode suppression and improving soil health in some systems and require further study (Habteweld et al., 2017; Lie et al., 2010; Mennan and Melakeberhan, 2010).
Potential biological control agents of cyst and root-knot nematodes are known to have specific host ranges among target nematode species, such as the bacterium Pasteuria penetrans among root-knot nematode species and populations. Such specificity may be controlled through surface protein binding and recognition between bacterium and nematode, suggesting variability in Meloidogyne may influence the potential of this and similar organisms as useful biological control agents.
- Nematicide controls – Several new nematicides have been shown by our current group and others to have high nematicidal efficacy and are in final testing phases to support new use registrations. Active ingredients include fluopyram, fluensulfone, fluazaindolizine, spirotetramat, and abamectin, among others. These materials offer much lower mammalian and avian toxicities than the current suite of fumigant, organo-phosphate and carbamate nematicides, thus being safer to apply, less damaging to the environment, and with less re-entry and residue issues. They represent important tools for integration into nematode management programs, including use as seed treatments and in combinations with bio-products and biocontrol organisms.
Advantages of a Multistate Effort:
Under the current project, research to apply emerging methodologies to obtain knowledge of the variability and adaptation potential in nematode populations is in various stages of advancement. The W-3186 membership proposes to continue and extend these efforts to identify and characterize the variability in important nematode pests. The participants share research interests on primary nematode pathogens and bring complementary expertise and resources to the project. We will determine gene frequencies, genetic stability, and adaptation and fitness, such that genetic variability can be managed and manipulated in agricultural production systems by appropriate alternative management strategies. The cyst and root-knot species are of primary importance as major nematode pathogens and as actual or potential invasive nematodes in most agricultural production areas and cropping systems, as reflected in the proposed contributions from participating states across the country. The diversity in cropping systems and rank of importance of nematode groups among participating states clearly provides opportunities for conducting meaningful collaborative research on major nematode pathogens. The participants utilize the opportunity to collaborate in ways that enhance the benefits accrued from the research, as opposed to pursuing individual projects within limited geographic boundaries. For example, the warm climate root-knot species will be studied by participants from a majority of the participating institutions (see Attachment Table 1) – a group effort that will pay large dividends in understanding nematode variability relative to management.
This team approach enables a pooling of scientific expertise and resources to maximize the amount and quality of the information that can be generated. The resources available to researchers working within the Agricultural Experiment Stations have been declining in recent years, especially for nematology programs. Conversely, the demands and expectations for new, environmentally friendly management tactics have never been greater. This multistate project provides a necessary forum for rapid scientific advancement in aspects of both basic and applied research directed toward nematode diagnostics and development of management strategies. For example, some participants have programs devoted to molecular research on nematodes, which can be applied across all states for diagnosis and to assess nematode genetic variability. This project has ongoing molecular-based programs in some states (e.g. California, Hawaii, Mississippi, Nebraska, New Mexico, Washington) that can facilitate research by other participant states. In turn, those states focusing on phenotypic differences in nematode populations can provide nematode populations and isolates for molecular analysis. This coordinated approach minimizes unnecessary duplication of research programs, and provides fertile opportunities for a seamless, interactive approach to advancing nematode management. Most importantly, the project also enhances the quality and applicability of the research findings across geographic locations and agricultural production systems.
Likely Impacts of Work:
The stakeholders (production agriculture) will be positively impacted by application of the project findings in expediting the development of new, environmentally benign management strategies to minimize economic losses from nematodes. This in turn should help boost the international competitiveness of our agricultural production systems. The project will also benefit the diagnosis and response process required when invasive nematode pests are suspected or found in production fields or in traded agricultural products.
Related, Current and Previous Work
Accomplishments (2013-2018):
A full listing of the 270 research publications of the W-3186 project from 2013-2018 and Annual Progress Reports for W-3186 are available through the NIMSS site for more in-depth descriptions of related work progress. Following is a summary of example findings that highlight areas of significant impact in addressing project goals, with comments concerning the need for additional research.
Overall, the project scientists have been very productive in efforts to develop improved techniques for nematode diagnostics, understanding of nematode diversity and genetic variability, processes of nematode fitness and adaptation, and the incorporation of this knowledge into the design and analysis of improved nematode management strategies. These findings directly benefit the 13 participating states and more broadly other states whose crop production systems are compromised by cyst, root-knot and other nematode infestations and invasions. Moreover, many of the accomplishments have resulted from our close collaboration within W-3186, through which shared knowledge, techniques and materials provide important synergies.
Under Objective 1 (characterize genetic and biological variation in nematodes relevant to crop production and trade), nematode (a)virulence and plant resistance gene interactions were elucidated in several important nematode crop combinations. These included root-knot nematode interactions with resistance gene(s) and nonhost determinants in coffee, cotton, cowpea, common and Lima beans, potato, tomato, wheat, and chile pepper, plus cyst nematode interactions for (a)virulence matching host resistance defined to varying levels in sugarbeet, soybean, wheat, and more recently potato. Within these interactions, new resistance specificities were identified, such as the Rkn genes in cotton and new root-knot resistance in chile pepper, together with the existence and frequency of matching virulence in nematode populations and their geographic distribution. Variation within and between Meloidogyne spp. was found for ability to parasitize a panel of resistant carrot germplasm (Roberts et al., 2016). These studies have been used to guide plant breeders, for planning crop rotations, and for cultivar selection. Variation was found in H. glycines isolates used for resistance screening and additional studies are needed to determine the extent to which this affects routine resistance screening procedures in breeding programs. Molecular studies on root-knot nematode and host genes have also led to insights on the genetic factors controlling nematode parasitism (Gleason et al., 2017; Gleason et al., 2008: Teixeira et al., 2016). This information will be key in understanding variations in plant-nematode interactions, how nematodes manipulate plants responses, and changes in nematode populations that contribute to their virulence on previously-resistant plants.
Molecular approaches to nematode identification within and between species have also been advanced under this objective. Molecular techniques have been applied to or developed for identifying target nematode species, races and populations (e.g., Powers and Ramírez-Suárez, 2012; Powers et al, 2014; Powers et al., 2017), including molecular ‘barcoding’ approaches (e.g., Blok and Powers, 2009). Data on hatching, host range, and a multigene phylogenetic analysis revealed G. ellingtonae as more similar to G. rostochiensis than G. pallida. Key biological features supporting this conclusion are the rapid hatch of G. ellingtonae in potato root diffusate, inability to reproduce on potato varieties with gene Ro1 for resistance to G. rostochiensis, and a highly supported phylogenetic analysis based upon 6,933 bp from 11 genes grouping G. ellingtonae with G. rostochiensis and G. tabacum, separate from G. pallida. (Zasada et al. 2013, 2015). In field studies G. ellingtonae did not reduce yield of ‘Désireé’ and reduced yield of ‘Russet Burbank’ only slightly, while 5 potato varieties inoculated with 80 G. ellingtonae eggs/g soil had no top weight or tuber yield reduction compared to non-inoculated controls (Ingham et al. 2015a).
Under Objective 2 (determine nematode adaptation processes to hosts, agro-ecosystems and environments), several systems have been analyzed, revealing high levels of nematode adaptation to parasitic ability on resistant host plants, alternative hosts (weeds), seasonal climatic differences, co-infection of plants by fungal pathogens, and soil conditions. Variation in parasitic ability among root-knot nematodes was described for species parasitizing grain legumes such as cowpea and Lima bean (Huynh et al., 2015), common beans, cotton, tomato, and potato cultivars and wild relatives. These studies demonstrated the need for broad-based forms of resistance for use in crop cultivars and cover and trap crops, for example for M. incognita in cotton (Wang et al., 2017). Earlier, we found that M. incognita populations virulent to resistance in cowpea had reduced fitness (lowered fecundity and increased extinction rates) (Petrillo et al., 2006), contributing to our understanding of nematode adaptation rates and guiding the breeding efforts for ‘Blackeye’ beans in California and other states (Roberts et al., 2017). In addition, project scientists identified local fitness and adaptation variations in M. chitwoodi and M. hapla populations to temperature regimes during the potato season in the Pacific Northwest, demonstrating the need to adjust predictive models for completion of nematode generations that influence decisions on time of nematicide applications. Temperature did not have an appreciable influence on hatch of G. ellingtonae eggs stored dry but hatch of eggs stored in moist soil was significantly higher than in dry soil (Ingham et al. 2015b). Also in the Pacific Northwest, it was demonstrated that white wine grape varieties are 3x better hosts than red wine grape varieties for M. hapla, leading to immediate planting recommendations for viticulturists (Howland et al., 2015). Field studies in Michigan showed that soil texture influences H. glycines population density (Melakeberhan et al., 2015) and M. hapla reproductive potential, with adaptation to mineral or muck soils (Melakeberhan and Wang, 2012, 2013; Melakeberhan et al., 2012).
Plant-parasitic nematodes can be introduced into new areas and identifying such infestations is critical; potato cyst nematodes G. rostochiensis and G. pallida are significant economic threats to potato production, and a comprehensive detection survey documented absence of G. rostochiensis in Idaho but confirmed the presence of G. pallida in Idaho, and also the absence of these species in Oregon but the presence of a new species, G. ellingtonae. Project members determined that a new ring nematode species, Mesocriconema nebraskense, endemic to the Central Plains has adapted to cause serious damage to bent grass on numerous golf courses in New Mexico (Thomas et al., 2017). Description of the new cyst nematode G. ellingtonae in Oregon is an important project outcome with implications for potato production and trade. These discoveries require further survey and study.
Meloidogyne incognita is adapting to new crops that are being introduced in the southern US. Common turmeric (Curcuma longa), a spice crop native to India used in foods, cosmetics and pharmaceuticals, is being evaluated as niche crop for Alabama. Root-galling was observed on multiple lines of C. longa being increased for dispersal across the southeast, and all seven tested lines were good hosts for M. incognita (Hall et al., 2017). Likewise, cultivars and breeding lines of the increasingly used herbaceous forage Lotus corniculatu (birdsfoot trefoil) were all susceptible to M incognita (Moye et al., 2017).
Under Objective 3 (develop and assess nematode management strategies in agricultural production systems), root-knot, cyst, and other nematode management systems have been studied, developed or improved for multiple cropping systems and production areas. Several examples highlight nematode-cropping systems that have been and will continue to be a project focus, targeting the most damaging nematode problems. Sugarbeet production systems in California and Idaho have been adjusted based on W-3186 studies to utilize trap cropping, green manures and modified rotation sequences for cyst nematode management. Various soil amendments and solarization have been assessed in Hawaiian cropping systems relative to impacts on soil nematode communities (Chan et al., 2016). In California, annual field and vegetable crop systems have been enhanced for root-knot nematode management utilizing host plant resistance in tomato, cotton, carrot and grain legume crops. In New Mexico, annual weeds were found to be unaffected by Verticillium dahliae and M. incognita and enhanced pressure on chile pepper from both pathogens (Sanogo et al., 2013). Susceptibility of biofumigant cover crops to M. incognita was also found important when attempting to suppress fungal pathogens in chile pepper (Rudolph et al., 2015). In the Pacific Northwest, potato production systems have been modified to optimize the choice of rotation crops and green manure crops with strategic timing of nematicide applications for managing M. hapla and M. chitwoodi. Growing ‘Sordan 79’ sudangrass reduced M. chitwoodi egg populations by 98% and incorporating shoot biomass at 15 to 39 Tons/acre suppressed reproduction by 49 to 99%. Growing ‘Terra Nova’ radish in greenhouse pots to simulate a fall planted cover crop reduced populations to near zero. Microbial amendments with species that may be antagonistic toward nematodes may also be a useful tactic; treating soil with microbial amendments (e.g., MeloCon, Hyper Galaxy, Bio Blend, BioFit N) significantly suppressed M. chitwoodi reproduction. Raspberry producers in the Pacific Northwest have received extensive information on management alternatives to standard broadcast fumigation, enabling transition to other management methods that suppress Pratylenchus spp. but reduce the amount of fumigants applied on a landscape scale (Walters et al., 2017). The regional utility of these management approaches will be tested on both cyst and root-knot nematode targets in different cropping systems.
In soybean, isolation of H. glycines-induced syncytia by laser microdissection from resistant and susceptible lines led to identification of hundreds of genes that are expressed during the resistance response (Klink and Thibaudeau, 2014), and some were tested in a genetic transformation platform to determine their function (Klink et al., 2009). Soybean genes proven to function in resistance to H. glycines have then been expressed in cotton, resulting in suppressed parasitism by M. incognita (McNeece et al., 2017). One such gene (NDR1), known to be activated by the bacterial effector protein harpin, led to topical treatments of soybean seeds with harpin preparations which suppressed parasitism levels of H. glycines, R. reniformis and M. incognita (AlJaafri et al., 2017). Xiang et al. (2017a,b) worked with industry to develop new biological nematicides for M. incognita and H. glycines management. Spore-forming plant growth-promoting rhizobacteria are being added to nematicide seed treatments to increase management options and reduce the pesticide footprint for cotton and soybean growers.
Developing predictive tools to aid in nematode management has also formed part of the project effort, including improving a fertilizer-use efficiency model by incorporation of concurrent changes in nematode community population density, plant growth and soil environment (Melakeberhan and Avendaño, 2008), and use of spatial information regarding nutsedge and M. incognita populations as predictors in modeling the nematode/nutsedge pest complex (Vetter et al., 2014). These are selected examples of many advances in nematode management being made under W-3186 participation in which the collective knowledge gained in one system is being applied to other systems. These nematode management systems are at various stages of development and require additional study and modification under a project renewal.
Current W-3186 project findings were incorporated into updates of the online resource NEMAPLEX (Ferris, 2012). Our findings were presented at national meetings attended by producers, scientists, and professionals. Presentations were made to state and federal regulators and at field days and extension meetings. Information was extended to smaller groups through facility tours, email, telephone conversations, and traditional hard copy. Several project participants have extension appointments and activities.
Areas Needing Further Investigation: Many of the cropping systems we are studying involve perennial crops or multi-year rotations requiring several years of experimentation to test the various permutations of cropping sequences and tillage systems, durability of resistance, and nematode adaptation to environment and to other control measures. Efforts under W-3186 have laid the foundations for integrating new approaches to nematode management that consider the genetic variability extant in nematode species. Additional study and modification of these systems for managing nematodes is necessary in order to design and optimize new integrated strategies. Coupled with these efforts, DNA sequence-based technologies have developed rapidly in the last five years and their versatility and knowledge value has increased tremendously, while their costs of application to diagnostics and research into nematode variation has dropped considerably. These advances in molecular protocols are also applicable for selection of nematode resistance traits in crop plants for plant breeding. We must take advantage of these new resources and apply them to the nematode-crop systems and invasive nematode threats that the project members are familiar with and for which representative samples are available. A new generation of novel, highly efficacious and less toxic nematicides has become available for testing, and these need to be researched and adapted into our focus nematode management systems.
Other Regional Projects: The only current multistate project with potential overlap to this proposal is S-1066: ‘Development of sustainable crop production practices for integrated management of plant-pathogenic nematodes,’ which focuses primarily on cotton, peanut and soybean cropping systems of the southeastern U.S. It emphasizes resistant variety development and has greater emphasis on soybean cyst nematode and especially reniform nematode, in contrast to the current proposal. Thus, while portions of its goals are similar to the current proposal, it focuses primarily on a different set of cropping systems to the ones proposed herein. During the current W-3186 project, two joint annual meetings of W-3186 and S-1066 were held for sharing and coordination of the relevant research interests and we plan to do this again with the S-1066 group during the next phase.
Objectives
-
1. Characterize genetic and biological variation in nematodes relevant to crop production and trade.
-
2. Determine nematode adaptation processes to hosts, agro-ecosystems and environments.
-
3. Develop and assess nematode management strategies in agricultural production systems.
Methods
The research focus of each participating state is given as a matrix in Table 1. The nematode group and main crop areas are indicated, together with the procedural research emphasis, as covered under the three objectives. Research coordination will ensure that standardized procedures are generally followed and research findings can be compared within and across nematode, plant and subject area categories. Plant germplasm (accessions, breeding lines, cultivars), nematode isolates of representative species and populations with GPS location data, and DNA primer and other sequence information for markers will be available among the participants.
Experimental protocols and procedures are structured according to the main target nematode groups under the three objectives. All objectives have a common focus of addressing critical aspects of nematode variability, adaptation, and management, with the application and extension of the findings tailored to meet individual state and sub-regional needs, in addition to those at the project-wide level. While findings under all objectives are considered important to each state, duplication will be avoided by partitioning individual state research activities. This structure will also ensure that all phases of the objectives are being met, while addressing local state needs, a structure that has worked well in the W-3186 project. Following are examples: Heterodera schachtii will be considered in California, Hawaii, Nebraska, and Idaho; Heterodera glycines will be considered in Alabama, Arkansas, Michigan, Mississippi, and Nebraska, while the Group II cool-climate root-knot species (Meloidogyne chitwoodi and M. hapla) will be studied in detail in California, Michigan, Nebraska, New Mexico, Oregon, Idaho, and Washington. Idaho and Oregon will consider impacts of G. pallida and G. ellingtonae on potato production systems. In terms of crops, Oregon, Idaho, and Washington will focus on potato, perennial crops, and (or) small grain interactions, New Mexico will focus on specialty crop interactions, while Washington, Oregon and California will focus on host-plant resistance in several crops. Oregon, Idaho, and New Mexico will address host range and rotations, while California, Idaho, and Washington will address cover-cropping and green manure treatments. Similar partitioning of research activity will be made for the other nematode groups.
Objective 1:
Phenotypic assessments will be made on isolates of nematode populations that are collected following survey and documentation of habitat, locality, and agronomic or cropping history of the collection site. This information will provide important background considerations for the level and nature of any phenotypic differences detected in comparative experiments. In most programs, investigators either have a partial or nearly complete collection of live cultures for genetic comparisons. For example, for Group I warm-climate root-knot populations, 40 isolates have been assembled at California-Riverside and a group of isolates is being assembled in Arkansas, while collections of group II cool-climate root-knot nematodes M. chitwoodi and M. hapla isolates are maintained and expanded in California, Oregon and Washington. For cyst nematodes, numerous populations of H. glycines are being cultured in Arkansas, Michigan, and Nebraska, and a collection of H. schachtii and H. cruciferae geographic and host-selected isolates is under culture in Hawaii. The Idaho population of G. pallida is being cultured and maintained in Idaho, and additional populations from South America and Europe are being assembled.
Assays of (a)virulence response to resistant lines and cultivars and of host range will be made under greenhouse and controlled environment conditions using well-established experimental procedures. The host range and virulence testing for the root-knot species (Groups I and II) will include standard sets of host differential plants and also differential cultivars and crop plants applicable to their local cropping systems, e.g., on grapes in the Pacific Northwest. The biotype scheme for root-knot nematode virulence will be expanded using known accessions, breeding lines, and cultivars of critical crops. For cyst nematodes, H. glycines will be examined on resistant soybean differentials and H. schachtii on sugarbeet breeding lines with resistance, while R. reniformis will be examined on pineapple lines in Hawaii and on grain legumes or cotton in Alabama, Arkansas, Hawaii and Mississippi. The pathotype system used for G. pallida is under study to potentially include virulence characteristics.
The cyst nematodes are excellent "model" nematode systems for genetic studies because they reproduce sexually. Conversely, many root-knot species are parthenogenetic (asexual). Their genetics will be studied using isofemale or single descent lineages to track inheritance, variability and adaptation, and via mendelian approaches using species that can reproduce sexually, such as M. hapla and M. chitwoodi. This work was developed under the previous project and several valuable segregating populations are now available. This work will take advantage of both established and new molecular techniques, including whole genome sequence assemblies. Techniques for multi-locus genomic and mtDNA analysis are well established in several participants' laboratories (Oliveira et al., 2011; Powers and Ramírez-Suárez, 2012). PCR and sequencing based techniques will be combined with transmission genetics of phenotypes of interest to conduct analyses of markers and associated traits. Meloidogyne, Heterodera, Globodera and other nematode populations will be typed phenotypically for (a)virulence with respect to resistance genes from different crop plant species and close wild relatives, for host range, and for biological traits including responses to abiotic soil conditions. Molecular analyses of these populations may lead to stable marker systems for nematode (a)virulence phenotypes, host range determinants (including changes in phenotypic response associated with prior parasitism of weeds), and geographical variants for diagnostic purposes, using mtDNA, ribosomal DNA, and nuclear DNA sequence and markers (Powers and Ramírez-Suárez, 2012). They also will provide stronger and more accurate diagnostic protocols. This will also allow us to monitor changes in gene frequency in fields, as we subject field populations to different cropping sequences. From a perspective of diagnostics, high resolution DNA markers will facilitate standardization of identifications and aid in analysis of hypothesized pathways for dispersal that may explain current species or haplotype distributions.
Members of W-3186 are in a unique position to address the question of Meloidogyne haplotype distribution across the western and central regions of North America. High resolution markers are available for the mitochondrial genome of Meloidogyne and most nematology laboratories in W-3186 participating states are PCR capable. Most participants have ready access to low cost DNA sequencing facilities. In addition to GenBank, DNA sequences of COI barcodes can be deposited in the Barcode of Life Database (BOLD) together with associated metadata as well as the international database of quarantine organisms (QBOL). A standardized geographic map of Meloidogyne haplotypes, ranked the most economically important nematode genus (Jones et al., 2013), can be constructed and made available online. This approach will allow the monitoring of changes in haplotype compositions with changing agricultural practices or climate changes, enable comparisons of haplotype diversity within fields, and also allow detection of new emerging pathogens or recent invasive nematodes. For instance, if M. enterolobii were introduced to California, it could be detected readily in routine molecular assays.
Excitingly, development of diagnostic systems that feature the simultaneous analysis of multiple species based on DNA extracted from soil provides new opportunities. To be effective, the DNA reference database of plant parasitic nematodes must be enhanced and validated as a goal of the project. In the future, this level of analysis will no doubt be required for phytosanitary certification. As costs of regulatory surveys and identifications are increasing with the demands for greater specificity, this high-throughput metagenomic approach to nematode diagnostics which identifies multiple species could eventually counteract those cost increases. W-4186 will play a central role in the development of this approach. It necessitates a team approach due to the need to identify multiple species/or haplotypes from multiple geographic regions, coupled with the testing of multiple genetic markers. Different states will focus on particular species in a collaborative framework: e.g., M. incognita populations from cotton-producing states, including Alabama, Arkansas, California, Mississippi and New Mexico; M. chitwoodi and M. hapla in the potato-small-grain-alfalfa systems of Oregon, Washington, and Idaho; H. schachtii, H. glycines, and H. cruciferae from California, Hawaii, Michigan and Nebraska.
Objective 2:
Phenotypic characterization and genetic markers developed under Objective 1 will provide the basis for selecting candidate populations that show adaptation to increased virulence and parasitism, and for variants associated with geographic (climatic) and soil abiotic isolation and adaptation. The fitness of isolates virulent for specific resistance genes will be assessed by controlled culturing on susceptible plants for multiple generations. Effects of non-agronomic hosts on fitness will also be examined, such as studies on the effects of weed hosts on root-knot nematodes in cropping systems, which will be expanded to include weed populations from other geographic areas to better understand the implications of this adaptation. Participants also will include comparisons with invasive root-knot nematode species, such as M. enterolobii (Group I) and M. fallax (group II), which have heightened aggressiveness and broad host range.
Adaptation and fitness also will be studied by imposing continuous selection for virulence in wild-type populations. Changes in reproductive capacity will be assessed to measure virulence frequencies and genetic stability of virulence. Tests of selected isolates will be made on resistant or nonhost plants other than those used to impose the original selection pressure. For example, studies of M. incognita (a)virulence matching resistance genes in cowpea will be continued (Petrillo et al., 2006) as will M. hapla virulence to various hosts using the root-knot nematode genomic resources. In the field, changes in virulence in H. glycines towards soybean cultivars carrying specific resistance genes will be monitored. Likewise, in Oregon pathogenicity of root-knot, lesion and ectoparasitic nematodes on small fruit genotypes as well as pathogenicity of G. ellingtonae on different genotypes of potato and weeds will continue to be evaluated (Howland et al., 2015; Zasada and Moore, 2014). In Idaho, where selection pressure on G. pallida has been limited, potential shifts in virulence of the Idaho population will be assessed on different genotypes of potato.
In addition, fitness and adaptability of H. glycines races, M. hapla, M. incognita and Pratylenchus to changes in soil nutrition and soil physio-chemical environments will be tested, focusing on sources of nitrogen and types and levels of nutrients and their effects on host plant status. Quantifying nematode adaptability to changes in soil-nutrient environments will be extended as a major emphasis of work in Michigan (Melakeberhan and Avendano, 2008; Melakeberhan and Wang, 2013), enhancing understanding of possible factors contributing to nematode adaptations.
Objective 3:
A range of factors and approaches will be considered in the design of nematode management strategies under Objective 3 - soil ecology and other soil properties, nematode community structure and function, sampling strategies tied with opportunities afforded by precision agriculture technologies for site-specific management. The integration of host resistance, cover, trap, and green manure crops, tillage practices, new post-plant nematicides, and impact of biological antagonists in crop rotation sequences will be considered in practical combinations and locations. These field-based studies will utilize standard experimental designs (randomized complete block, split-block, split-plot) with control treatments and 4 to 6-fold replications under current production practices. The development of biotyping schemes for cyst and root-knot nematode populations for reaction to host and nonhost crops and to resistant cultivars, and accompanying practical marker systems under Objective 1 will provide important information for resistance and rotation implementation in annual crops. For example, soybean cyst nematode rotation experiments will be conducted with contemporary highly resistant, resistant and susceptible soybean varieties, because the data we have presently is severely outdated for production areas in several states. Crop rotation utilizing only nonhosts as production or cover crops that are grown in rotation with hosts will be evaluated for success in nematode management and profitability. Knowledge on nematode fitness and adaptability developed under Objective 2 will aid in the optimization of durable nematode management strategies. Climatic and soil conditions, different nematode species, and variability in host ranges among different populations will require unique rotations for different growing regions.
Several cropping systems being evaluated currently under W-3186 and described earlier have multi-year horizons and crop sequence combinations that require much additional analysis. New ones, such as lesion nematode in raspberry production in the Pacific Northwest will be addressed through integrated management studies. Rotation schemes will be assessed in microplots or infested field sites within the relevant localities. Techniques will be coordinated among participants to facilitate direct comparisons of results. The rapidly changing demands on crop production present new challenges to the use of crop rotation for nematode management. For example, demand for ethanol has resulted in the shift from wheat to corn in potato rotations. While wheat is a host for M. chitwoodi, it is harvested early in the summer and supports limited reproduction. In some areas it can also be followed by green manure cover crops in the fall that significantly suppress nematode populations. In contrast, corn is grown into the fall, supporting several generations of M. chitwoodi and eliminating the opportunity for suppression of population increases with cover crops. However, corn can vary greatly in its host status to M. chitwoodi and different cultivars need to be screened for resistance to inform growers about which varieties would limit population increases. Grower interest in green manure crops and other cover crops for nematode suppression is increasing but little is known about the host status of many of these plants. This needs to be determined so that effective recommendations can be made. In the case of sugarbeet cyst nematode, H. schachtii, and cereal cyst nematode H. avenae, fallow or planting with a green manure crop incorporated after eight weeks will be tested in rotations with non-host crops such as corn, bean, onion or potato between main crops. In Idaho, the trap crop Solanum sisymbriifolium has been found to greatly reduce populations of G. pallida (Dandurand and Knudsen, 2016). However, a comprehensive management plan for its deployment needs to be developed.
The long-term economic productivity of U.S. agriculture depends upon the ability to respond in a timely, economical, and environmentally sound way to invasive agricultural pests such as the recent introductions and discovery of the regulated cyst nematodes in the genus Globodera. Risks and damage from introductions of these types of invasive pests can be minimized when appropriate tools are available for their control. For invasive and potentially spreading pests, critical first steps toward deployment of effective control strategies include rapid detection methods, and a clear understanding of environmental pressures and parameters which contribute to genetic and biological variation so that effective control strategies can be implemented.
As stated earlier, there are several new post-plant nematicides with reduced environmental toxicity available to growers across production systems. The W-3186 research group is uniquely situated to evaluate these nematicides across a range of environments, crops, and nematode targets. For example, M. hapla and M. chitwoodi will be the targets of field trials evaluating nematicides in Idaho, Oregon, and Washington in potato, sugarbeets, onion, and wine grapes. These newly-emerging, less toxic nematicides with active ingredients including fluopyram, fluensulfone, fluazaindolizine, spirotetramat, and abamectin, will be evaluated as tools for use in nematode management programs, including use as seed treatments and in combinations with bio-products and biocontrol organisms. The diverse crops and nematode pests investigated by project participants present a major opportunity for incorporation of these new tools into nematode management systems that have been a central focus of this project.
To enhance understanding of spatio-temporal relationships among nematode populations, soil conditions, biological antagonists, and production practices, a combination of the soil food web (SFW, Ferris et al., 2001) and fertilizer use efficiency (FUE, Melakeberhan and Avendaño, 2008) will be utilized to assess agro-biological and ecological suitability of cultural practice-based nematode management strategies. The SFW and FUE models are nematode community analysis based and use quadrant systems to describe best-to-worst case scenarios for outcomes of soil biophysio-chemical changes and potential sustainability of the agronomic practices, respectively. Both models will be utilized to assess how any specificity and variable outcomes observed under Objective 2 relate to agricultural and ecological efficiencies of cultural practice-based nematode management strategies. In doing so, we will be developing bridges suitable for multi-disciplinary approaches beyond nematology and critical to solving agricultural problems (Melakeberhan, 2010).
Measurement of Progress and Results
Outputs
- Basic knowledge on biological, ecological and genetic processes underlying the success of cyst, root-knot, and other nematodes as parasites of crop plants.
- New or improved, and safer tactics for the management of nematodes that affect crop production and trade in US agriculture.
- Holistic approaches for integrating different nematode management strategies into IPM systems. A diagnostic DNA barcoding reference data base of major plant parasitic nematodes that impact US agriculture
- Economic impact and ecological assessment of management approaches for control of plant parasitic nematodes of major agricultural significance.
Outcomes or Projected Impacts
- Increased knowledge base in plant-nematode biology, ecology and genetics for use in identifying novel targets for nematode control.
- Implementation of nematode management tactics that complement reduced or softer pesticide usage, benefitting human health and the environment.
- Promotion of sustainable farm management practices through new nematode management tactics.
- More efficient and effective response and mitigation capabilities for invasive and trade product contamination issues.
- Economic benefits to producers and consumers through reduction in nematode management costs and food production.
Milestones
(2019):Development of new research-based knowledge on nematode variability and adaptation relative to abiotic and biotic parameters in target cropping systems.(2021):Development and validation of new nematode diagnostic and detection protocols.
(2022):Evaluation of new nematode management tactics under appropriate experimental conditions.
(2023):Transfer of knowledge to stakeholders on new nematode management tactics and new diagnostic and detection protocols.
Projected Participation
View Appendix E: ParticipationOutreach Plan
The project has a successful track record of disseminating the new knowledge and information created by participants and co-operating colleagues. It is planned that the traditional outlets for transferring project results will continue to be utilized, including peer-reviewed journals, annual progress reports, scientific meeting presentations, and websites. Extension presentations and publications also will be made; both by participants with extension appointments, and by AES and ARS participants, most of whom have a strong applied research component to their programs and who routinely participate in extension-based activities (meetings, presentations, publications) with our agricultural stakeholders. The project members will use web-based delivery vehicles to reach the broad stakeholder base that will benefit from objective, science-based knowledge and information about important plant-parasitic nematode biology, diagnosis, management and regulation. Examples include GenBank for depositing most DNA sequences, the Barcode of Life Database (BOLD) for DNA sequences of COI barcodes together with associated metadata, and the international database of quarantine organisms (QBOL). Project findings also will be incorporated into updates of the online resource NEMAPLEX (Ferris, 2012; http://plpnemweb.ucdavis.edu/nemaplex). Among our stakeholders we have identified growers and food processors, national and international trade partners, USDA-APHIS and state regulatory agencies, national and state legislators, fellow scientists, and K -16 teachers and students.
Organization/Governance
The organization and governance of this Multistate Research Project will conform to the guidelines presented in the United States Department of Agriculture's publication "Manual for Cooperative Regional Research". Committee officers include a Chair, a Vice-Chair, and a Secretary. A new secretary will be elected at each annual meeting of the Technical Committee with the current secretary assuming the position of Vice-Chair. The Vice-Chair assumes the position of Chair at the end of the annual meeting. In the event that an Executive Committee is needed, the officers are authorized to serve in that role. For organization of each annual meeting, one member of the Technical Committee will be selected to coordinate and oversee local arrangements for the meeting. The meeting coordinator will be chosen based on the consensus selection of the meeting site, and typically will be the member from the state or institution hosting the meeting. The Technical Committee will rotate the annual meetings between representative Experiment Stations who will be participating in the project. Members will benefit from site visits of research and extension facilities at the host Experiment Station institution. Administrative guidance will be provided by an assigned Administrative Advisor.
Literature Cited
Aljaafri, W.A.R., B.T. McNeece, B.R. Lawaju, K. Sharma, P.M. Niruala, S.R. Pant, D.H. Long, K.S. Lawrence, G.W. Lawrence, and V.P. Klink. 2017. A harpin elicitor induces the expression of a coiled-coil nucleotide binding leucine rich repeat (CC-NB-LRR) defense signaling gene and others functioning during defense to parasitic nematodes. Plant Physiology and Biochemistry 121:161–175.
Blok, V.C. and T.O. Powers. 2009. Biochemical and molecular identification. In Root-knot Nematodes, Eds Perry, Moens, and Starr. Publisher: CABI Publishing, UK
Chan, K.D., B. Sipes, K.H. Wang, and P.S Leung. 2016. Mentha spicata: A potential living mulch for conservation agricultural practices in tropical climates. Journal of Nematology 48:309.
Dandurand, L.M., and G.R. Knudsen. 2016. Effect of the trap crop Solanum sisymbriifolium and two biocontrol fungi on reproduction of the potato cyst nematode, Globodera pallida. Annals of Applied Biology 169:180-189.
Ferris, H. 2012. NEMAPLEX - Nematode-Plant Expert Information System. http://plpnemweb.ucdavis.edu/nemaplex.
Ferris, H., T. Bongers, and R.G.M. De Goede. 2001. A framework for soil food web diagnostics: extension of the nematode faunal analysis concept. Applied Soil Ecology 18:13–29. DOI: 10.1016/S0929-1393(01)00152-4
Gleason, C.A., Q.L. Liu and V.M. Williamson. 2008. Silencing a candidate nematode effector gene corresponding to the tomato resistance gene Mi-1 leads to acquisition of virulence. Molecular Plant-Microbe Interactions 21:576-585.
Gleason C, F. Polzin, S.S. Habash, L. Zhang, J. Utermark, F.M. Grundler, and A. Elashry. 2017. Identification of two Meloidogyne hapla genes and an investigation of their roles in the plant-nematode interaction. Molecular Plant-Microbe Interactions 30(2), 101-112
Habteweld, A. W., D.C. Brainard, A.N. Kravchenko, P.S. Grewal, and H. Melakeberhan. 2017. Effects of plant and animal waste-based compost amendments on soil food web, soil properties, and yield and quality of fresh market and processing carrot cultivars. Nematology 19: 1 -22.
Hall, M., K. Lawrence, W. Groover, D. Shannon, and T. Gonzalez. 2017. First Report of the Root-Knot Nematode (Meloidogyne incognita) on Curcuma longa in the United States. Plant Disease 101 (10):1826. https://doi.org/10.1094/PDIS-03-17-0409-PDN
Howland, A.D., P.A. Skinkis, J.H. Wilson, E. Riga, J.N. Pinkerton, R.P. Schreiner, and I.A. Zasada. 2015. Impact of grapevine (Vitis vinifera) varieties on reproduction of the northern root-knot nematode (Melodidogyne hapla). Journal of Nematology 47:141-147.
Huynh, B.-L., W.C. Matthews, J.D. Ehlers, M. Lucas, J.P. Santos, A. Ndeve, T.J. Close and P.A. Roberts. 2015. A major QTL corresponding to the Rk locus for resistance to root-knot nematodes in cowpea (Vigna unguiculata L. Walp.). Theoretical and Applied Genetics 129: 87-95. DOI 10.1007/s00122-015-2611-0
Ingham, R E., W.S. Phillips, A. Peetz, N.M. Wade, and I. A. Zasada. 2015a. Effects of the cyst nematode Globodera ellingtonae on potato. Journal of Nematology 47:247.
Ingham, R.E., D. Kroese, and I.A. Zasada. 2015b. Effect of storage environment on hatching of the cyst nematode Globodera ellingtonae. Journal of Nematology 47:45-51.
Jones, J.T., A. Haegeman, E.G.J. Danchin, H.S. Gaur, J. Helder, M.G.K. Jones, T. Kikuchi, R. Manzanilla-Lopez, J.E. Palomares-Rius, W.M.L. Wesemael, and R.N. Perry. 2013. Top 10 plant-parasitic nematodes in molecular plant pathology. Molecular Plant Pathology 14(9):946-961. DOI: 10.1111/mpp.12057
Klink, V.P., K.-H. Kim, V.E. Martins, M.H. MacDonald, H.S. Beard, N.W. Alkharouf, S.-K. Lee, S.-C. Park, and B.F. Matthews. 2009. A correlation between host-mediated expression of parasite genes as tandem inverted repeats and abrogation of the formation of female Heterodera glycines cysts during infection of Glycine max. Planta 230:53-71.
Klink, V. P. and G. Thibaudeau. 2014. Laser microdissection of ultrathin sections for studying plant-pathogen developmental processes at single cell resolution. Journal of Plant Interactions 9:610–617.
Koenning, S.R., C. Overstreet, J.W. Noling, P.A. Donald, J.O. Becker, and B.A. Fortnum. 1999. Survey of crop losses in response to phytoparasitic nematodes in the United States for 1994. Journal of Nematology 31:587-618.
Lie, Q., Y. Jiang, W. Liang, Y. Lou, E. Zhang, and C. Liang. 2010. Long-term effect of fertility management on the soil nematode community in vegetable production under greenhouse conditions. Applied Soil Ecology 46, 111-118. DOI: 10.1016/j.apsoil.2010.06.016
McNeece, B.T., S.R. Pant, K. Sharma, P.M. Nirula, G.W. Lawrence, and V.P. Klink. 2017. A Glycine max homolog of NON-RACE SPECIFIC DISEASE RESISTANCE 1 (NDR1) alters defense gene expression while functioning during a resistance response to different root pathogens in different genetic backgrounds. Plant Physiology and Biochemistry 114:60-71.
McSorley, R. et al. 1987. Bibliography of estimated crop losses in the United States due to plant-parasitic nematodes. Annals of Applied Nematology 1:6-12.
Melakeberhan, H. 2010. Cross-disciplinary efficiency assessment of agronomic and soil amendment practices designed to suppress biotic yield-limiting factors. Journal of Nematology 42: 73-77.
Melakeberhan, H. and M. Avendaño. 2008. Spatio-temporal consideration of soil conditions and site-specific management of nematodes. Precision Agriculture 9:341-354.
Melakeberhan, H., D. Douches, and W. Wang. 2012. Interactions of selected potato cultivars and populations of Meloidogyne hapla adapted to the US Midwest soils. Crop Science 52:1-6.
Melakeberhan, H. and W. Wang. 2012. Suitability of celery cultivars to populations of Meloidogyne hapla. Nematology 14: 623-629.
Melakeberhan, H. and W. Wang. 2013. Proof-of-concept for managing Meloidogyne hapla parasitic variability in carrot production soils. Nematology 15:339-346.
Melakeberhan H., W. Wang, A.N Kravchenko, and K. Thelen. 2015. Effects of agronomic practices on the timeline of Heterodera glycines establishment in a new location. Nematology 17:705-713.
Mennan, S. and H. Melakeberhan. 2010. Effects of biosolid amendment on populations of Meloidogyne hapla in soil with different textures and pHs. Bioresource Technology 101: 7169-7175.
Moye, H.H., W.L. Groover, E. van Santen, N. Xiang, and K.S. Lawrence. 2017. First report of the root-knot nematode (Meloidogyne incognita) on Birdsfoot Trefoil (Lotus corniculatus L.) in the southern United States. Plant Disease. DOI 10.1094/PDIS-08-17-1299-PDN
Nicol, J.M., S.J. Turner, D.L. Coyne, L. den Nijs, S. Hockland and Z.T.Maafi. 2011. Current nematode threats to world agriculture. Pp. 21–44 in J.T. Jones, G. Gheysen and C. Fenoll, eds. Genomics and Molecular Genetics of Plant–Nematode Interactions. Heidelberg: Springer.
Oliveira, C.M.G., A.R. Monteiro, and V.C. Blok. 2011. Morphological and molecular diagnostics for plant-parasitic nematodes: working together to get the identification done. Tropical Plant pathology 36(2):65-73.
Petrillo, M.D., W.C. Matthews, and P.A. Roberts. 2006. Host influence on Meloidogyne incognita virulence to resistance genes Rk and Rk2 in cowpea. Journal of Nematology 38:90-96.
Powers, T.O., E.C. Bernard, T. Harris, R. Higgins, M. Olson, M. Lodema, P. Mullin, L. Sutton, and K.S. Powers. 2014. COI haplotype groups in Mesocriconema (Nematoda: Criconematidae) and their morphospecies associations. Zootaxa 3827 (2): 101–146.
Powers, T.O., T. Harris, R. Higgins, P. Mullin, and K. Powers. 2017. An 18S rDNA perspective on the classification of Criconematoidea. Journal of Nematology 49: 236-244.
Powers. T.O. and A. Ramírez-Suárez. 2012. Molecular diagnostics of nematodes: some practical considerations. Pp. 131-145. In Practical Plant Nematology. 2012. Manzanilla-López, R.H. and Marbán-Mendoza, N. (Eds). Colegio de Postgraduados and Mundi-Prensa, Biblioteca Básica de Agricultura. Printing Arts Mexico, Guadalajara, Jalisco, México. xxx + 883 pp.
Roberts, P.A., B.-L. Huynh, N. E. Clark, W.C. Matthews, and C.A. Frate. 2017. Blackeye varietal improvement: University of California Dry Bean Research 2016. California Dry Bean Advisory Board, Dinuba, CA. Pp. 1-7.
Roberts, P. A., W.C. Matthews, P.S. Simon, and T.T. Duong. 2016. Variation among Meloidogyne spp. isolates on a panel of resistant carrot genotypes. Journal of Nematology 48:365-366.
Rudolph, R.E., C. Sams, R. Steiner, S.H. Thomas, S. Walker, and M.E. Uchanski. 2015. Biofumigant performance of four Brassica crops in a green chile pepper (Capsicum annuum) rotation system in southern New Mexico. HortScience 50:247-253.
Sanogo, S., J. Schroeder, S. Thomas, L. Murray, N.E. Schmidt, J. Beacham, C. Fiore, C., and L. Liess. 2013. Weed species not impaired by Verticillium dahliae and Meloidogyne incognita interactions that damage chile pepper. Plant Health Progress doi:10.1094/PHP-2013-0920-01-RS.
Sasser, J.N., and D.W. Freckman. 1987. A world perspective on nematology: the role of the society. Pp. 7-14 in J. A. Veech and D. W. Dickson (eds.). Vistas on Nematology. Society of Nematologists.Smiley, R. 2005. Plant-parasitic nematodes affecting wheat yield in the PNW. Oregon State University Extension Service Pp.1-6.
Starr, J.L., S.R. Koenning, T.L. Kirkpatrick, A.F. Robinson, P.A. Roberts, and R.L. Nichols. 2008. The future of nematode management in cotton. Journal of Nematology 39:283-294.
Teixeira, M.A., L. Wei, and I. Kaloshian. 2016. Root-knot nematodes induce pattern-triggered immunity in Arabidopsis thaliana roots. New Phytologist 211: 276–287. doi: 10.1111/nph.13893
Thomas, S.H., J. Beacham, and T.O. Powers. 2017. Suppression of Criconematid-induced injury to golf course greens in New Mexico. Journal of Nematology 49: (in press).
Trojan, J.M., S.H. Thomas, J. Schroeder and L.W. Murray. 2007. The influence of Meloidogyne incognita inoculum source and competition on nematode virulence on chile pepper. Journal of Nematology 39:97.
Vetter, J., Z. Ou, L. Murray, S.H. Thomas, and J. Schroeder. 2014. Determining the effectiveness of including spatial information into a nematode/nutsedge pest complex model. Proceeding of the 24th Annual Kansas State University Conference on Applied Statistics in Agriculture: 109-124.
Walters, T.W., M. Bolda, and I.A. Zasada. 2017. Alternatives to current fumigation practices in western states raspberry. Plant Health Progress 18:104-111.
Wang, C., M. Ulloa, T.T. Duong, P.A. Roberts. 2017. QTL analysis of transgressive nematode resistance in tetraploid cotton reveals complex interactions on chromosome 11 regions. Frontiers in Plant Science 8: 1979 p.1-12. doi: 10.3389/fpls.2017.01979
Xiang, Ni, K.S. Lawrence, J.W. Kloepper, P.A. Donald, and J.A. McInroy. 2017a. Biological control of Heterodera glycines by spore-forming plant growth-promoting rhizobacteria (PGPR) on soybean. PLOS ONE 12(7): e0181201. https://doi.org/10.1371/journal.pone.0181201.
Xiang, N., K.S. Lawrence, J.W. Kloepper, P.A. Donald, J.A. McInroy, and G.W. Lawrence. 2017b. Biological control of Meloidogyne incognita by spore-forming plant growth-promoting rhizobacteria on cotton. Plant Disease 101: 774-784. http://apsjournals.apsnet.org/doi/pdf/10.1094/PDIS-09-16-1369-RE.
Zasada, I.A., R.E. Ingham, and W.S. Phillips. 2015. Biological insights into Globodera ellingtonae. Aspects of Applied Biology 130:1-9.
Zasada, I.A. and P.P. Moore. 2014. Host status of Rubus species and hybrids for the root lesion nematode, Pratylenchus penetrans. HortScience 49:1128-1131.
Zasada, I.A., A.B. Peetz, N. Wade, R.A. Navarre, R.A., and R.E. Ingham. 2013. Host status of different potato (Solanum tuberosum) varieties and hatching in root diffusates of Globodera ellingtonae. Journal of Nematology 45:195-201.