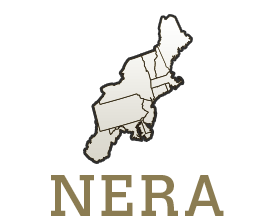
NE1835: Resource Optimization in Controlled Environment Agriculture
(Multistate Research Project)
Status: Inactive/Terminating
NE1835: Resource Optimization in Controlled Environment Agriculture
Duration: 10/01/2018 to 09/30/2023
Administrative Advisor(s):
NIFA Reps:
Non-Technical Summary
Statement of Issues and Justification
Statement of Issues
Agriculture is threatened by its sensitivity to climate change and irregular weather patterns. Accurate projection of yields and harvest time are essential to pair production with market demands and forecast financial margins. In controlled environment agriculture (CEA), farmers can closely control most aspects of the growing environment and adjust production to weather and market fluctuations. The ability to control environmental and agricultural inputs results in increased resource efficiency per production area and reduced shrinkage compared with outdoor field production. Hence optimized efficiency and use of low–cost alternative resources is fundamental for the successful future of CEA operations. Adaptation to climate change to overcome potential risks demands strategies that match current and projected conditions.
CEA is an economically important sector in agriculture in the U.S. Despite the economic crisis in 2008, the USDA Census reported that the number of operations and sales in specialty crops increased by 7.5% and 17%, respectively, from 2009 to 2014. IBISWorld reported that the hydroponic industry is on the growth stage in the market, which is characterized by many new companies entering the market, rapid technology change, growing acceptance by consumers, and rapid introduction of products and brands. CEA is particularly important in northern climates where year–round production is only possible in protected agriculture, and in urban areas where space is limited.
While we have advanced the understanding of energy, light, and water utilization in CEA, we still must further understand the potential of emerging technologies on energy and water optimization. The results of our previous project expanded knowledge on (1) the effect of irrigation practices and nutrient management on plant health, (2) the energy footprint and efficiency of different type of lights and production systems, and (3) sensors to monitor energy use. For the next project, we propose evaluating new greenhouse lamps, wavelengths of light, and layout designs and their effect on plant growth. We also aim to evaluate and design low energy alternatives to ventilate high tunnels, cool and heat greenhouses, and sensors to monitoring environmental conditions and plant growth. We will evaluate organic fertilizers for production of edible crops and test alternative growing media for container production. Finally, we will evaluate alternative irrigation methods and water sources to reduce the amount of fresh water for crop production. We will evaluate non-chemical alternatives to control diseases. Ultimately, we aim to measure energy and water consumption and develop saving strategies. We will deliver recommendations to commercial growers.
Our team consists of 24 members who represent 18 different experiment stations. Four members (FL, NH, IN, IL), are representatives of three new stations, who joined our group in the 2016–2017 period. Five new members (2 OH, IA, NC, MI) joined our group in this upcoming period. Our team has produced a total of 158 scholarly outputs which include 11 dissertations/theses, 1 book, 10 book chapters, 45 refereed journal articles, 20 symposium proceedings, and 71 presentation papers. Our team has also dedicated to translate research outcomes to stakeholders via 170 outputs, including 72 popular articles, 28 sponsored-workshops, 28 participations in workshops, and 42 other creative works. We have a long standing history of collaboration and productivity and the continuous enrollment of new and young faculty reflects the steady growth of our field of research.
The environmental conditions in each of our stations vary by location, nonetheless by conducting research as a multistate group we are able to develop robust models and then tailor research projects and recommendations to our local stakeholders. Moreover, our team brings together a complementary knowledge base that is essential for optimizing resource management in CEA. Members include greenhouse engineers, plant scientists, and an economist, all with practical experience in solving problems in multidisciplinary environments and direct contact with stakeholders.
Justification
A major benefit of growing plants in CEA operations is the ability to use sensors to monitor the production environment to make informed decisions about fertilization, irrigation, heating, cooling, and lighting. Over the last decade, new technologies have emerged in the industry with the potential to provide growers with alternatives to improve production efficiency and profit margins. However, science–based guidelines are needed for growers to make informed–decisions about the feasibility of implementing these technologies in their operations. Growers can achieve consistent production (i.e. increase crop cycles per year, reduce time from seed to harvest, and improve flowering regulation) by reducing temporal and spatial variation in the greenhouse environment. Our team believes that sensors and control strategies are essential for efficient production in CEA operations. CEA production systems range in technological complexity from high tunnels to highly controlled environments (e.g. plant factories). Strategies to control fertilization, irrigation, heating, cooling, and lighting will vary by CEA production systems. We aim to work with the whole range of CEA operations.
Heating, cooling and lighting options determine energy consumption and are strongly correlated with plant growth rate. Therefore, the efficiency of heating, cooling, and lighting have a direct impact in the bottom–line of businesses. Fan performance and ventilation alternatives to achieve homogeneous temperatures and humidity, temperature prediction models using thermal environment in high tunnels and greenhouses, and alternative lighting wavelengths, intensity and duration can be used to regulate plant growth and maximize outputs (production) per input (energy).
Water management is closely tied to nutrient management, particularly in greenhouse production where plants receive nutrients via fertigation. Automated–irrigation scheduling using sensor–based set–point irrigation has the potential to reduce water volume significantly. Despite the low–cost of water, we anticipate that economic benefits may result in terms of reduced labor, fertilizer injection, and disease incidence. Fertilizer reduction can also lower the environmental impact related to fertilizer runoff into natural habitats, and fertilizer mining and processing. CEA facilities can be designed or easily retrofitted to maximize water use efficiency, while at the same time minimize or eliminate leachate from contaminating the outdoor environment (e.g., recirculating ebb and flood irrigation). We propose to determine set–point irrigation control of different irrigation species in propagation and evaluate how alternatives substrates and water sources impact water use in container production.
In 2017, the National Organic Standard Board voted to allow USDA Organic certification of hydroponic production systems. This news provides CEA operations a gateway to a growing market. However, high efficiency in organic greenhouse production in not possible yet. Matching nutrient availability with crop demand is a major challenge in organic greenhouse production, which can have negative effects in plant quality and productivity. Nutrient release from organic compounds is linked to microbial activity, hence associated with environmental conditions and cultural practices that affect microbiological processes. We propose to develop nutrient release curves of organic–sources of fertilizer under multiple environmental conditions and provide recommendations on how to improve predictability of nutrient release.
Even though the U.S. is a pioneer in CEA, there is still a lot of room for resource optimization. For example, the water footprint global average (gal per pound) for tomato production is 25.6, the average in the U.S. is 15.2 which is 15 times more than the average in the Netherlands where the majority of crops are grown in greenhouses. Our team believes that optimization of resources used in CEA can result in significant reduction of agricultural inputs (water, fertilizer, and energy), increased production efficiency (days to harvest, crops per year, yields per square foot greenhouse), and lowered production costs.
Related, Current and Previous Work
Our approach has been to conduct applied research that is relevant to industry needs. We have provided valuable greenhouse engineering technology to the industry throughout our history through technology transfer. Some examples include the use of air–inflated double–layer polyethylene films as greenhouses cover material, advances in hydroponic production systems and supplemental lighting, floor heating for greenhouses, and the use of energy curtains. Many of the technologies originally developed by members of this project are now industry standards for improving sustainability and conserving energy. Our team has trained several professionals who are now leaders in the CEA industry.
Alternative Energy Sources and Energy Conservation
Energy is the second largest input cost in greenhouse production. Unheated high tunnels were found to be a suitable energy-efficient alternative to heated greenhouses for finishing cold-tolerant bedding plants in the spring in northern U.S. locations (IA, IN, NJ, NY) and, in some cases, crops finished in high tunnels led to high plant quality (Currey et al., 2014; Gerovac et al., 2015). The energy efficacy (light output per unit electricity consumed) of supplemental lights has increased greatly in the past 20 years. Some, but not all, light emitting diodes (LED) have higher energy efficacy than traditional high-pressure sodium (HPS) fixtures (Wallace and Both, 2016). The decision on when to add supplemental light to the greenhouse is often based on a time clock approach or instantaneous light threshold. However, a coordinated daily predictive approach to achieve a target daily light integral (DLI) can reduce lighting energy costs by more than 30 percent compared with the light threshold. As LEDs improve in energy efficiency and initial cost, commercial adoption will become more widespread. However, more research is needed to: test energy efficacy of fixtures, optimize light spectrum modification to maximize crop growth, and control lighting.
Research on supplemental lighting (Mitchell et al., 2015; Kubota et al., 2016; Wallace and Both, 2016) has resulted in a proposed product label (Both et al., 2017) for lamps designed to deliver photosynthetically active radiation to promote crop production (FL, NJ, OH). The label provides information about operating characteristics that will be useful to growers considering using a supplemental lighting system and we hope manufacturers will adopt it. Work on measurement standards (Both et al., 2015) has resulted in a set of measurement guidelines researchers can use for research and reporting of those measurements in the scientific literature. A collaboration with researchers in Japan (Ishii et al., 2015) has further improved our understanding of natural ventilation in open-roof greenhouses. As a result of the rapid developments in the lighting industry (especially regarding LED lamps), work on supplemental lighting is continuing and includes the evaluation of lamp operating characteristics as part of a collaborative project with researchers from Cornell and Rensselaer Polytechnic Institute (GLASE project) (NY, NJ). Additional work on ventilation will continue as part of a multi-institutional SCRI grant that investigates berry production in high tunnels (IL, NJ, OH).
We evaluated plant growth response to the environmental parameters in lettuce and spinach grown in heated greenhouses in hydroponics with control of the nutrient solution (CT, NH). Lettuce had a greater contribution of irradiance, and lesser contributions from temperature and solution nitrate to relative growth rate compared with spinach (Gent, 2017). In other words, lettuce was affected more by irradiance and spinach was affected by temperature and solution nitrate concentration. On a separate experiment, we (NH) evaluated hydroponic lettuce growing in naturally sunlit greenhouses was subjected to two temperature treatments (NH). Minimum temperatures were 20° or 10°C, and ventilation temperatures were 26 and 16oC, for warm and cool treatments, respectively. Warm compared to cool temperature increased relative growth rate by 85% for small plants of 2 to 5 g fresh weight (fw) compared to 30% for medium plants of 20 to 60 g fw. Warm temperature increased specific leaf area for plants grown under a low light integral of 4.5 mol.m-2.d-1, compared to a high light integral of 15 to 17 mol.m-2.d-1. Dry matter content was 20% greater for plants grown under cool compared to warm conditions. Total reduced nitrogen was 7% less under cool temperature. The concentration of nitrate was 40% lower, while the concentration of sugars was about 50% higher, under cool compared to warm temperature. Cool temperature increased the tissue concentrations of malic acid and potassium, but had no effect on phosphorus.
We (CT) evaluated the effect of daily light integral on the composition of hydroponically grown lettuce (Gent, 2014). We identified that except for nitrate, metabolite concentrations on a fresh weight basis increased with irradiance, and the changes resulting from irradiance were greater when harvested in the afternoon than in the morning. Our results suggest that lettuce for human consumption is best harvested in the afternoon after growth under high light, when it has the least nitrate and more of other nutrients. On spinach, sugars, sucrose, glucose, and fructose, had a binary response with high values in the day and low values in the night (Gent, 2016). Spinach grows slower in the fall compared with summer because of the effect of low temperature on metabolism of sugars and nitrate.
Virginia Cooperative Extension provided access to and funding for energy audits and renewable energy feasibility studies for 66 agricultural operations in Southside and Southwest Virginia. Between 2014 and 2016, 71 farms were accepted into the energy program, 5 farms withdrew, 64 of the 66 remaining farms completed an energy audit with 2 farms delaying their audits, 31 farms have used approximately $214,000 in grant funding along with over $610,000 in individual funds to implement energy retrofits, and 35 farms have funds remaining in their energy accounts for additional improvements. The 64 completed farm energy audits identified potential annual energy savings of 873,968 kWh in electricity and 429,847 gallons of propane with efficiency improvements resulting in a projected 3,151 MTCO2e greenhouse gas emissions reductions and an annual energy-cost savings of $850,734. Approximately 46% of the energy conservation measures had a payback period of less than 5 years. The Agriculture Energy Efficiency Initiative has delivered 20 educational programs on energy efficiency practices and technologies. Partnerships are in place with USDA Rural Development, Virginia Department of Mines, Minerals and Energy, Old Dominion Electric Cooperative, and other organizations to support this project.
Water and Nutrient Management
While fertilizers and fertigation practices have improved agricultural productivity, nutrients that are not taken up by the crops and run off into the environment have caused environmental concerns that put at risk the long term sustainability of water bodies and soils. Our approach has been to evaluate alternatives to (1) reduce initial water use while maintaining plant health and quality (CT, ME), (2) use non-traditional water sources such as recycled or recirculated water (CT), (3) improve the efficiency of fertilizer applications (CT, ME, NH, NY,OH). We investigated several parallel approaches frequently sharing the results and incorporating feedback from other members into new research.
ME built a capacitance sensor automated fog system for propagating stem cuttings (ME). This novel system allows growers to more precisely control the environment during propagation. We tested this system and found that the amount of water applied during propagation impacts root quantity and length of the native plant, Twinflower (Linnaea borealis). We also determined that the environment in which stock plants are grown affects root quantity and length (Foster et al., 2017). We acknowledge that we need to evaluate more crops and we also need to understand how we should adjust light in propagation settings to make it a more comprehensive propagation method.
ME developed water guidelines for herbaceous perennial plants using a capacitance sensor automated irrigation system. These guidelines allow growers to determine the right amount of water to apply to important ornamental crops. For example, the herbs rosemary (Rosmarinus officinalis) and English lavender ‘Munstead’ and ‘Hidcote’ (Lavandula angustifolia) were larger when grown at high soil moisture contents (Zhen et al., 2014; Zhen and Burnett, 2015). Although both of these plants are considered to be drought tolerant, survival was poor at low soil moisture contents (volumetric water content = 10%). English lavender produced more flowers when grown at a volumetric water content of at least 30%. Growth of the perennials cheddar pink (Dianthus gratianopolitanus ‘Bath’s Pink’) and columbine (Aquilegia canadensis) was also greater when plants were grown with more water (Zhen et al., 2014). However, these plants survived in drier soils and appeared to be more drought tolerant than English lavender and rosemary.
NE conducted a 3-year study to determine if final biomass of basil could be predicted based on electronic sensor inputs and other factors. Five basil (Ocimum spp.) cultivars were grown using a capillary mat (CapMat) fertigation bench system that consisted of a sandwich of white reflective, black plastic polyethylene top, capillary mat, black 6 mm polyethylene, in a double polyethylene-glazed Quonset style greenhouse (ENT-3). We installed Decagon GS3® electronic sensors at the root balls of the containers to sense and monitor volumetric water content, electrical conductivity, and media temperature. We used height as the response parameter, which we collected weekly. There were three plants per pot in this study. Plant height responses did not follow a classical sigmoidal growth model. We concluded that approximate reasoning modeling (Fuzzy Logic discussed under objective 2) followed the growth process closely.
CT experiment stations (Storrs and New Haven) collaborated to study the effects of salinity and irrigation management on zinnia, pansy and poinsettia. We demonstrated that partial saturation is an effective water management regime even when raw water quality is poor as represented by elevated salinity (Gent et al., 2016; Macherla, 2014; Macherla and McAvoy, 2017). Whether using a partial-saturation or full-saturation irrigation management regime, dissolved sodium (Na) has dramatic deleterious effects on zinnia health that become visually evident as the electrical conductivity approaches 10 mS/cm and tissue Na concentrations approach 8,000 ppm.
VA team surveyed nursery and greenhouse operators to evaluate BMPs use and barriers to implement new BMPs. We are currently using these results to develop publications and resources to assist producers in improving production efficiency and reduce environmental impact (Mack et al., 2017).
High diversity and incidence of plant pathogens have been reported in low-quality water resources (i.e. recycled and recirculated water) (Hong, 2014). Growers inject chemical sanitizers in the irrigation system to prevent spread of propagules. However, plant sensitivity to sanitizers is in general unknown. CT-Storrs estimated the phytotoxicity threshold of Impatiens walleriana to residual concentrations of free chlorine in the irrigation water (Raudales, 2017). We applied chlorine between 2 and 32 mg/L of free chlorine in every irrigation. Plants irrigated with 2 mg/L were no different from plants irrigated with no chlorine residues. Plants irrigated with 4 mg/L of free chlorine were smaller by weight and did not present any visual lesions on the foliage or flowers. Plants irrigated with 8-32 mg/L presented clear phytotoxicity symptoms. Results indicate that irrigation water with chlorine levels greater than 4 mg/L affect quality and yield of Impatiens walleriana is 2 mg/L.
Fertilizer Optimization
In previous research, alternatives to constant liquid fertilizer (CLF) for bedding plants and vegetable transplants were investigated to reduce nutrient leaching as well as develop fertilization strategies for organic crops (NY). Controlled release fertilizers incorporated into the potting mix could reduce leaching of garden mums by 5-fold (Mattson et al., 2014). Use of controlled- and slow- release fertilizers was most effective to replace CLF for bedding plants and vegetable transplants with low to moderate fertility (Mattson, 2014). For heavier feeders (ex: petunias, garden mums) additional liquid fertilizer appears necessary for optimal plant growth (Mattson et al., 2014). Commercial substrates vary greatly in their base fertility (starter nutrient charge), pH, and physical properties and growers should obtain media analysis before using new products (Li and Mattson, 2016). Substrate amendments can further enhance plant performance but must be examined on a crop-by-crop basis. For example, seaweed extract applied as a weekly drench (but not foliar spray) enhanced post-production quality of petunias and tomatoes (Li and Mattson, 2015). We summarized research and commercial practices for organic fertilization practices (Burnett et al. 2016, ME, NY, VA). For vegetable transplants, organic amendments added to the substrate may provide decent fertility for 4 to 6 weeks, but must be further amended or supplied with liquid organic fertilizers after this time. With increased interest in hydroponic vegetable production, more research is needed to further optimize fertilization of conventional and organic hydroponic production systems.
Microgreens (young leafy greens harvested at the emergence of a true leaf) are a relatively new profitable niche crop for greenhouse operations. However, very little information is available in the scientific literature regarding cultural practices. The influence of liquid fertilizer (applied at 0, 50, 100, 150, and 200 ppm N from a complete liquid fertilizer) was evaluated on three brassica microgreens (arugula, mustard and mizuna) (Allred, 2017). All species exhibited increased fresh weight as fertilization increased from 0 to 200 ppm N, with fresh weight about double at 200 ppm N than the unfertilized control. Seeds were sown in a peat/perlite based soilless media. Substrate depth within a flat was also studied and significantly enhanced yields were found when a flat was filled nearly to the top (as opposed to shallow filling to save on substrate).
Container-grown ornamental and edible crops are at the highest quality at the end of production in the greenhouse or nursery. Plant quality often declines in retail and for consumers, typically resulting from stress, insufficient watering or fertilization. In retail, marketable plant quality lasts on average three to six days, resulting in crop losses near 15% (Healy, 2009). Even though problems occur during post-production, poor plant experiences result in dissatisfied customers and lower repeat business. In addition, contract growers only receive payment for plants sold in retail, and have greater incentive to promote plant quality post-production. Unless growers take action to extend plant quality into post-production, they will likely encounter more negative customer experiences, increased shrink (plants not sold), and reduced profitability. Past research has shown that providing fertilizer with residual activity and agrichemicals that prevent wilt or increase substrate water-holding capacity improve the post-production performance of container crops (Argo and Biernbaum, 1993; Armitage, 1992; Blanchard and Runkle, 2007; Million et al., 2001; Nell, 1994; van Iersel, 2009). We (NH) have focused on understanding how plant genotypes affect the pH of the growing media (Dickson et al., 2017). We further conducted a pilot study (NH, unpublished) that has shown potential to manipulate nutrient concentrations and form, application method, and type for improved post-production performance and added value. There is a need for further research on optimizing new fertilizer and agrichemical strategies for post-production, focusing on practicality and cost for growers.
Costs of soilless substrates used in greenhouse crop production have increased significantly over the past decade. In addition, key components such as sphagnum peat moss are considered non-sustainable. New tree wood materials offer potential to reduce substrate costs and decrease dependence on peat (Caron, 2017; Jackson et al., 2009; Schmilewski, 2017). New manufacturing processes have improved the consistency and quality of wood chip and wood fiber products as well as decrease material cost. Pine trees are also a renewable resource and in some cases produced locally (Barrett et al., 2016). Wood materials have potential to immobilize nitrogen, influence substrate-pH buffering capacity and water-holding capacity. Using new wood fiber and wood chip substrates will likely influence fertilizer and irrigation practices for the grower, in retail, and for consumers. Project results indicated that peat-based substrates amended with wood fiber and coconut coir may require growers to adjust their fertilizer and irrigation program. Recommendations on fertilizer nitrogen form, substrate lime type and rate, and irrigation frequency for different blends of peat, coir, and wood fiber blends were developed.
Education
Over the years, members of this project have collaborated formally and informally in many education programs.
The results of light research that we have conducted over the years has been summarized in a new book where six of our members (IA, MI, NC, NJ, NY, OH) were first authors and co-authors of multiple chapters (Lopez and Runkle, 2017).
In 2015, team members from AZ, OH, and NJ received funding from the USDA-NIFA Higher Education Challenge Grant Program. We used these funds to develop a series of “Controlled Environment Plant Production Engineering/Technology Education Modules”. We uploaded all the modules in the “Horticultural Engineering Technology” YouTube channel, that we created (https://www.youtube.com/channel/UCsD2oKzVv1B_GtumyKKE8LA/videos). Up to Jan 2018, the channel had a total of 15,456 views.
Many of our members have partial extension appointments and organize annual greenhouse workshops and short courses in AZ, CT, IA, NH, NJ, NY, OH. In all of these instances, we invite members from other stations to participate as speakers.
CT, MA & NH are conducting an eight-part webinar series focused on root-zone management to cover local needs of greenhouse growers in the northeastern US.
Virginia Tech and CT-Storrs conducted a workshop on hydroponic production of greenhouse vegetables that included lectures and hands-on demonstrations for about 40 people in each location. Comments after the workshop included appreciation for the training and decision support tools. As a result of the workshop, one attendee has joined our team as an online graduate student starting this fall. All presentations have been posted on the Fresh Produce Food Safety YouTube channel.
Maine presented information on water requirements of various herbaceous perennials at New England Grows, which is a large regional trade show. This information was a summary of research in Maine over the past ten years regarding how much water a variety of perennial plants need for growth and development. These recommendations were developed by growing perennials in a moisture sensor automated irrigation system at various soil moisture levels (varying from 5% to 40% soil moisture).
A series of three extension articles regarding visual symptoms of nutrient deficiencies in hydroponic lettuce, basil, and arugula was developed (NY). The project was completed by a female undergraduate student who grew plants in hydroponic nutrient solutions lacking particular elements of interest. Photos and written symptoms of nutrient deficiencies were documented. The article series has been published both at e-Gro.org and Inside Grower magazine.
Objectives
-
Objective 1. To evaluate and develop strategies to improve energy efficiency in controlled environment agriculture.
-
Objective 2. To reduce fresh water use and evaluate alternative fertilizers and growing substrates for the production of greenhouse crops.
-
Objective 3. To train growers and students to utilize emerging controlled environment agriculture technologies.
Methods
Objective 1. Evaluate and develop strategies to improve energy efficiency in controlled environment agriculture.
We will study several approaches to improve energy efficiency in controlled environment agriculture, with a focus on lighting and air quality (temperature and humidity).
AZ, FL, MI, NC, NY, NJ and OH will collaborate to determine energy efficacy of commercial lighting systems. Several commercially available horticultural LEDs are on the market but their energy efficiency varies widely. The best fixtures are twice as efficient as the lowest efficiency fixtures. LED fixtures also vary in their light spectrum with two typical approaches: 1) primarily red/blue light in different ratios and 2) broad spectrum (white) light (obtained from phosphor-coated blue LEDs). The ratio of red/blue can impact plant height and yield. NY will study effect of red/blue ratios on model vegetable and flower crops as well as compare plant performance of several higher efficacy LEDs (both red/blue and broad spectrum) compared to baseline HPS lamps. Data on crop yield, installation, maintenance, ongoing energy costs, and life expectancy will be used to determine economics of LED adoption.
We will use a 2-meter integrating sphere and a dark room to evaluate the efficacy and light distribution of a variety of lamps designed for horticultural applications. Testing reports will be generated and made available to growers and the research community. Outreach activities will be conducted toward the lamp manufacturers in order to encourage them to use the proposed product label. We will continue to contribute to lighting standard development efforts focused on the green industry that are currently undertaken by several organizations such as ASABE, UL, DLC, and IES.
We will also develop a computational fluid dynamics (CFD) model to evaluate various ventilation strategies in high tunnels. Several novel ventilation designs have been installed at a research facility operated by colleagues at Penn State, and these designs will be used to develop and further test the CFD model. The final model should be able to help determine the optimum ventilation strategies for crops grown in high tunnels. Improved ventilation strategies will improve the overall efficiency of this popular production system.
Several strategies to make supplemental lighting decisions given a background of sunlight will be compared, including: time clock (lights on for a fixed number of hours daily), light threshold (lights turn on/off when instantaneous light reaches a set threshold), daily light integral (DLI, coordinated control of light and shade curtains to reach a target DLI). A greenhouse energy model simulation (previously developed by NY) will be used to determine potential energy costs when using each of the 3 control methods using example floriculture, lettuce, and tomato crops. We will then compare crop uniformity/quality using the three methods to grow a model floriculture and lettuce crop in our greenhouses. Data on crop yield/quality, control system costs, and energy costs will be compiled to determine economics of various control strategies.
We will develop dynamic light recipes to improve lettuce growth rate and quality (morphology and phytochemical content) in indoor production systems. Most research has focused on characterizing plant responses when exposed to a single light recipe (static spectrum). We will contribute by researching and the matching spectrum to the different plant growth stages (lag, log, and plateau) to maximize output (nutrient, flavor, growth) and improve system energy efficiency. We called this the dynamic spectrum. This research project is focused on finding dynamic light recipes to improve lettuce growth and quality.
We will investigate the interactions between daily light integral (DLI) and CO2 concentration to improve propagation efficiency of grafted vegetable seedlings. Indoor nursery using electrical lighting is a standard commercial technology for pre- and/or post-grafting processes in Asian and European countries that have advanced nursery technologies. The advantages include 1) uniform plant growth, 2) higher density production, 3) highly contained environment, 3) complete independence from outside climate, and 5) conditioned flowering for earliness; yet the disadvantages are 1) high capital costs, higher energy consumption and 3) limited availability of systems. The objective of this project is to maintain high plant quality while reducing electricity demands from electrical lighting by increasing concentration of supplemental CO2 in order to increase plant grow efficacy (g per kWh).
We will work on the optimization of strawberry nursery production in full controlled environment systems. The current open-field strawberry nursery production system is a multi-year and multi-location operation and limited to select regions in the U.S. The current system has inherit risks. For example, disease contamination of strawberry nursery material; and lack of plant uniformity and supply due to variable environmental conditions. In this research project, we aim to designing precision indoor propagation (PIP) tools to optimize strawberry nursery production and to develop disease-free propagation material. The project main objective is to optimize the environment to increase runner/tip production while reducing production cost. Current efforts are focused on photoperiod, light intensity, and temperature optimization.
We will work on optimizing site-specific solar radiation modelling for its application in the horticultural, agricultural and photonics industries. The objective of this project is to measure irradiance conditions under a range of structural materials, different locations, sites, and times of day/year. Measurements will be performed with partners, in Finland, the US, UK and mainland Europe. The objective of the project is to predict light quality inside the greenhouse based on outside irradiance using modelling. Validation of models is done by measuring under various scenarios and comparing measurements to models of what the irradiance is predicted to be.
We aim to improve environmental optimization to increase phytochemicals in non-commercial plant species. The objective of this research program is to increase the affordability of Vertical farm products by providing more nutritionally dense produce by significantly increasing the phytochemical contents and biomass accumulation while reducing the resource inputs. We will characterize the responses of exotic germplasms grown under optimized indoor conditions in hopes to have a greater content of beneficial phytochemicals than commercial cultivars.
Objective 2: Reduce fresh water use and evaluate alternative fertilizers and growing substrates for the production of greenhouse crops.
We (CT, IN, ME, MN, NH, NY, OH) aim to develop alternatives to preserve fresh water use for production of greenhouses by studying irrigation methods to reduce fresh water use, treatment options to use low-quality water, mechanisms to improve fertilizer efficiency, and enhance conditions to promote beneficial microbiomes.
One primary objective is to reduce the amount of water used during vegetative propagation of ornamental plants in the horticulture industry. We are exploring two approaches that will provide growers with alternatives to over-head mist, the traditional approach. Both of these would reduce water use during this phase of production. One of these systems is a sensor-automated mist system, which we plan to further explore. We would like to use our sensor automated mist system to help growers refine over-head mist systems and more accurately determine how much water is truly needed during propagation. The second system is called submist; in this system, water is applied to the base of cuttings that are in enclosed tubs rather than overhead. Preliminary results indicate that roots form more quickly when plants are propagated in this system compared to traditional overhead mist.
We will evaluate the feasibility of using phytoremediation in line in hydroponic production systems. We will assess if halophytes can be used in hydroponic systems to remove sodium and chloride from reclaimed water. We will evaluate crops that not only have the potential to remove salts inline, but also have a commercial value. Our first experiment will consist in growing lettuce and purslane in hydroponic systems where nutrient solutions contain a range of chloride and sodium levels.
Several commercially available conventional and organic hydroponic nutrient solutions will be tested for production of greenhouse leafy greens and fruit-bearing crops. Daily pH and EC measurements and periodic nutrient solution elemental analysis will be taken to determine potential for salt buildup and nutrient imbalances of the given crops. Based on initial findings, one conventional and one organic nutrient solution will be further tested for hydroponic lettuce grown using nutrient film technique (NFT). Different strategies for nutrient management (frequencies of replacing nutrient solution) will be undertaken to test several salt thresholds for nutrient solution replenishment and impact on crop yield.
Our team will also study the microbial activity in hydroponic solutions. We will evaluate if microbes in the solution can be primed to accelerate nitrification, used to improve nutritional quality of the crop for human consumption and resistance to plant diseases.
A range of fertilizer and application strategies designed to provide nutrients during post-production will be evaluated at the University of New Hampshire. Fertilizer types include various controlled release (single and dual polymer coat), slow release organic, and water-soluble fertilizer formulations. Effects on substrate-pH, substrate nutrient level, plant performance (leaf greenness, growth, flowering, size and compactness), and longevity will be evaluated in both simulated and actual retail environments. Production simulations and partial economic analyses will be used to evaluate fertilizer strategy practicality and potential cost-benefit.
New wood chip and fiber substrates will be evaluated for potential nitrogen drawdown and effects on plant performance for container-grown crops. Projects will test substrates varying proportions and types of wood materials blended with sphagnum peat in combination with different fertilizer strategies. Fertilizer strategies will consist of a range of applied nitrogen concentrations and form (ammonium and nitrate). Nitrogen immobilization will be measured in substrates using VDLUFA standard methodology. Plant performance (plant growth, leaf greenness, flower number, size and compactness), substrate-pH buffering, and nitrogen immobilization will be measured during production and simulated retail environments to determine the effects of wood materials for growers and consumers.
We will develop best nutrient management practices for hydroponic and bioponic production systems and provide growers with crop production options that will improve yield, quality, and profitability, while significantly reducing fertilizer use and minimizing discharge (IN). Our activities include reducing the use of nitrogen and phosphorus fertilizers, determining the efficiency of open and closed systems, enhancing food safety, and conducting cost-benefit analysis in different production scenarios.
We will develop best nutrient management strategies for economically and environmentally viable hydroponic and bioponic food-production systems. The proposed research gives producers significant tools to make decisions from start-up through production and sales. Best production management practices, economic and food safety analysis will increase the likelihood of successful operation producing local food for Indiana and beyond. It will also significantly increase the diversification of food crops and production systems, while protecting its fragile environment.
Objective 3: Train growers and students to utilize emerging controlled environment agriculture technologies.
All team members have committed to involve undergraduate in independent-research related to this project. We strongly believe that the future of the industry depends on highly-qualified individuals.
We will continue to train graduate students and involve them in this project. This year we will begin the NE-1385 travel grant scholarship to incentivize graduate student attendance to our annual meeting.
We will continue to host short courses on energy efficiency, water management, and general controlled environment production practices. Our members with partial extension appointments will continue to organize annual greenhouse workshops and short courses in AZ, CT, IA, NH, NJ, NY, OH. In all of these instances, we will invite members from other stations to participate as speakers. Upcoming events include Northeast Greenhouse Conference and Cultivate with attendance over 800 individuals.
Many of our members have outfitted greenhouse sections with several alternative production systems (horizontal and vertical hydroponic growing systems, as well as traditional pot-and-media based systems) that the students use to learn about different growing techniques. We all have agreed to do more hands-on training sessions in CEA-related courses to increase practical training.
We are currently collaborating on a seven-part article series on Urban Agriculture. The articles will be published in Produce Grower Magazine, a well–known trade journal with a readership of 9,749. We agreed to use the proceeds of these publications to provide travel grants to graduate students to attend to our annual meetings.
Measurement of Progress and Results
Outputs
- We will develop recommendations for optimal lamp choices and layouts for greenhouses and indoor production facilities
- We will improve ventilation alternatives for high-tunnels that result in better cooling in the summer and reduced heat loss in the winter.
- We will develop practical production guidelines to increase the efficiency of organic fertilizers in production of container-grown ornamentals and hydroponically-grown vegetables.
- We will develop practical management guidelines yield and quality of vegetables grown in recirculating hydroponics and aquaponics systems.to improve production efficiency and increase
- We will develop recommendations for application of flexible wavelength lighting and selective cover materials or shading elements for greenhouses.
- We will develop strategies to reduce water use in propagation of ornamentals and vegetables.
- We will accelerate propagation timing by reducing water use.
- We will generate new knowledge about environmental management practices that enhance beneficial microbes in hydroponic solutions.
- We will develop management guidelines to use low-quality water for irrigating greenhouse crops.
- We will production guidelines to adjust nutrient programs to non-peat-based substrates.
- We will organize education programs that target CEA growers around the US, our target populations will include Hispanics, Native Americans, and new farmers.
- We will publish a hydroponic production book and an eight-part article series on urban agriculture.
- We will enhance undergraduate research training in the area of controlled environment plant production to prepare the students for independent studies.
- We will submit at least 3 grants to enhance our collaboration within the team.
- We will produce at least 10 scholar outputs every year.
Outcomes or Projected Impacts
- Improved ventilation, lighting, heating, cooling and dehumification strategies will improve energy efficiency in greenhouses. This will allow growers to reduce their energy consumption by 10-30%, resulting in significant savings.
- Production time (day to harvest) will reduce by at least 15% and therefore annual yields will also increase by at least 10%. This gain in production time is expected in response to tighter environmental controls and more production cycles per year (more yields per area per year).
- The water footprint of CEA-grown products will reduce by at least 30%. We expect these results in response to increase water recirculation within the farm, increase recycling water from other sources (i.e. reclaimed water), and reduce use of fresh and potable water.
- Fertilizer recommendations for hydroponic production will reduce leaching and fertilizer usage by 10-20% and allow growers to remain competitive in this rapidly growing field.
- The development of the proposed guidelines for controlled agriculture and hydroponics in will become part of a joint publication article series on Urban Agriculture planned for Produce Grower, and a short book on hydroponics. These publications will help to keep growers competitive and aware of the latest research in controlled environment agriculture.
- Information gathered and shared through this project will empower growers to improve production practices through the use of (remote) monitoring and control of the greenhouse environment and plant status. The realtime and web based greenhouse and climate control platforms developed will help growers to reduce 10% of their labor use dedicated to greenhouse climate monitoring, recording, and management.
Milestones
(2018):Teach undergraduate course on controlled environment crop production practices.(2018):Transfer basic knowledge and newly generated results to local, regional, and national stakeholders.
(2018):Test several lamps that are specifically designed for horticultural applications.
(2018):Develop a list of herbaceous and woody plants that can be propagated in sub–mist irrigation.
(2018):Evaluate non-chemical control options to manage waterborne pathogens and algae in hydroponic systems.
(2018):Finish first draft of hydroponic book.
(2019):Contribute to lighting standard developments. Promote the use of the lighting label.
(2019):Develop a preliminary ventilation model for high tunnels.
(2019):Evaluate phytoremediation as an alternative to remove sodium and chloride from reclaimed water in hydroponic systems.
(2019):Compare root formation timing and plant physiological traits under sub–mist compared with overhead mist.
(2019):Refine a ventilation model for high tunnels. Develop ventilation recommendations for high tunnel growers.
(2019):Develop guidelines on managing conventional and organic fertilizer source, and substrates for hydroponics and container-grown crops during production and post-production.
(2019):Develop guidelines on managing on nutrition when using alternative growing-media substrates.
(2019):Evaluate silicon supplementation for control of root-borne pathogens in soilless substrates and hydroponic systems.
(2019):Compare yield and system efficiency between hydroponics and bioponics.
(2020):Conduct a series of outreach efforts to promote the results of the studies on ventilation, water treatments, irrigation, and nutrient management.
(2020):Develop Si application guidelines for growers.
(2020):Develop practical management guidelines and economic analysis of nutrient programs for promoting retail and consumer success with container crops.
(2020):Evaluate technical and financial characteristics of wood chip and fiber substrates in container production.
(2020):Compare LED fixtures with HPS fixtures in terms of energy efficiency, crop response, and financial benefits as compared with the 2016 HPS baseline.
(2020):Develop production guidelines and best management practices for hydroponics/bioponics of major greenhouse vegetables
(2021):Guidelines for managing salt build–up and nutrient imbalances for NFT production of hydroponic lettuce to reduce water usage while maintain crop yield/quality.
(2021):Develop propagation protocols for appropriate moisture content for annuals plants using sensor automated propagation system.
(2021):Develop guidelines on using plant growth regulators, surfactants, and adsorbents to prevent wilt and promote container–crop longevity during post–production and retail.
(2021):Develop production guidelines and best management practices for hydroponics/bioponics of many greenhouse vegetables
(2022):Conduct research determining whether microbial inoculants are beneficial in hydroponic production and if the presence of these microbes affects the nutritional quality for consumers. Transfer results to producers.
(2022):Develop models predicting the impact of irradiance, temperature and CO2 concentration on leafy green and ornamental crop photosynthesis. Integrate these models into the USDA–ARS ‘Virtual Grower’ software program available to all growers.
(2022):Develop and test lighting control strategies that reduce energy usage as compared to timeclock/threshold while maintaining crop quality/yield.
(2022):Conduct a series of outreach efforts to promote the use of LED lamps for horticultural applications.
(2022):Develop guidelines on non-chemical alternatives to prevent clogging caused by biofilms in irrigation systems.
(2022):Develop models predicting the impact of irradiance, temperature and CO2 concentration on leafy green and ornamental crop photosynthesis. Integrate these models into the USDA–ARS ‘Virtual Grower’ software program available to all growers.
(2022):Evaluate potential phytotoxicity of various agrichemicals used to promote post–production performance of container crops.
(2022):Evaluate the effect of residual fertilizer and agrichemical strategies when using wood fiber substrates on plant performance during production and post–production at commercial growers and retail operations.
(2022):Cost-benefit analysis as a decision tool for hydroponic/bioponics crop production.
Projected Participation
View Appendix E: ParticipationOutreach Plan
We work closely with the controlled environment agricultural industry throughout the United States. Several team members have partial Extension appointments, providing additional connections with grower associations, industry suppliers, and the Land-grant Extension network. We use these connections with the industry to develop questions that are based on actual industry needs and to communicate the new knowledge that we generate.
We plan to continue sharing the results of our research through a variety of methods to reach both our peers at research institutions, as well as greenhouse growers, industry suppliers, and Extension personnel. Results will be published in refereed articles and Extension publications.
A large number of our members organize annual education programs for greenhouse growers. In this venues, we collaborate with our peers and cross state borders to share our knowledge.
Our team is currently collaborating on a seven-part article series on Urban Agriculture. The articles will be published in Produce Grower Magazine, a well–known trade journal with a readership of 9,749. In addition, we plan to publish a book on Hydroponic Production for greenhouse growers that includes the results of our previous work on water and energy savings, ventilation and cooling, and alternative energy use.
We will work closely with Cultivate has an attendance of over 10K, and the Northeast Greenhouse Growers Association and the Michigan Growers Association annual meetings which have an attendance of over 800. We will also write articles for trade magazines with readerships of >18K (Greenhouse Grower, GrowerTalks and GPN Magazine) and repost in HortiDaily which has a readership of > 20K world-wide.
Over the years, members of this project have provided valuable greenhouse engineering technology to the industry throughout our history through technology transfer. Some examples include the use of air–inflated double–layer polyethylene films as greenhouses cover material, advances in hydroponic production systems and supplemental lighting, floor heating for greenhouses, and the use of energy curtains. Many of the technologies originally developed by members of this project are now industry standards for improving sustainability and/or conserving energy. Several key industry members received part of their education at the institutions (and in some cases were instructed by members of our team: involved in this project.
We are distinctively qualified to develop strategies that address resource management in controlled environment agriculture. Our group consists of plant scientists, agricultural engineers, and an agricultural economist. Our group includes early, mid, and late career researchers. The extent of diversity in terms of area of expertise, career stage, demographics, and location provides a valuable contribution to the industry in which practical and research-based solutions are quickly developed and spread in time and space.
Organization/Governance
The technical committee has organized itself by annually appointing an incoming secretary, who then serves as the secretary for the following year (including the next annual meeting). The secretary will complete a one–year term and then serve as the committee chair the following year. We do not have the position of vice chair (chair elect). Therefore, officers served for two–year terms. This limited the time commitment requested from incoming officers and since the committee was small and informal, sufficient institutional memory could be tapped in case procedural questions came up. Annual meetings were organized on a rotating basis after the membership was polled for availability and interest. This organizational model has worked well for many years, and we plan to continue with it if our proposal is approved for continued funding. Many of the participating members meet each other at other scientific meetings throughout the year, ensuring sufficient opportunity for interaction in addition to the annual project meetings.
Literature Cited
Allred, J.A. 2017. Environmental and cultural practices to optimize the growth and development of three microgreen species. M.S. Thesis. Cornell University.
Altland, J. E., L. Morris, J. Boldt, P.R. Fisher, R.E. Raudales. 2015. Sample container and storage for paclobutrazol monitoring in irrigation water. HortTechnology, 25(6):769-773.
Argo, W.R. and J.A. Biernbaum. 1993. Factors affecting garden performance of flowering plants in hanging baskets. Bedding Plants Foundation, Inc., Research report. No. F-061A.
Armitage, A.M. 1992. Bedding plants: prolonging shelf performance, postproduction care and handling. Ball Publishing, Batavia, IL.
Barrett, G.E., P.D. Alexander, J.S. Robinson, N.C. Bragg. 2016. Achieving environmentally sustainable growing media for soilless plant cultivation systems—a review. Sci. Hort. 212: 220-234.
Blanchard, M.G. and E.S. Runkle. 2007. Exogenous applications of abscisic acid improved the postharvest drought tolerance of several annual bedding plants. Proc. IC on Qual. Manag. Supply Chains of Ornamentals. Acta Hort. 755.
Both A.J. , B. Bugbee, C. Kubota, R.G. Lopez, C. Mitchell, E.S. Runkle, C. Wallace. 2017. Proposed product label for electric lamps used in the plant sciences. HortTechnology 27(4):544-549
Both, A.J., L. Benjamin, J. Franklin, G. Holroyd, L.D. Incoll, M.G. Lefsrud, and G. Pitkin. 2015. Guidelines for measuring and reporting environmental parameters for experiments in greenhouses. Plant Methods 11(43). 18 pp.
Burnett, S.E., Mattson, N.S., & Williams, K.A. 2016. Substrates and fertilizers for organic container production of herbs, vegetables, and herbaceous ornamental plants grown in greenhouses in the United States. Scientia Horticulturae. 208:111-119. doi:10.1016/j.scienta.2016.01.001
Caron, J and J.-C. Michel. 2017. Overcoming physical limitations in alternative growing media with and without peat, pp. 413 In: (Eds.) A. Baumgarten, B. Carlile, M. Raviv, Proc. Intern. Symp. On growing media, composting, and substrate analysis. Acta. Hort.
Currey, C.J., R.G. Lopez and N.S. Mattson. 2014. Finishing bedding plants: a comparison of unheated high tunnels versus heated greenhouses in two geographic locations. HortTechnology 24(5):527-534.
Dickson, R.W., P.R. Fisher, S.R. Padhye, and W.R. Argo. 2016. Evaluating calibrachoa (Calibrachoa ×hybrida Cerv.) genotype sensitivity to iron deficiency at high substrate pH. HortScience 51(12):1452-1457
Dickson, R.W., P.R. Fisher, and W.R. Argo. 2017. Quantifying the acidic and basic effects of fifteen floriculture species grown in peat-based substrate. HortScience 52(8):1065-1072
Foster, J.J., S. Burnett, and L.B. Stack. 2017. Effects of light, soil moisture, and nutrition on greenhouse propagation of Linnaea borealis. HortTechnology 27:782-788.
Gent, M.P.N. 2014. Effect of daily light integral on composition of hydroponic lettuce. HortScience 49(2):173–179.
Gent, M.P.N. 2016. Effect of irradiance and temperature on composition of spinach. HortScience 51:133-140.
Gent, M.P.N. 2017. Factors affecting relative growth rate of lettuce and spinach in hydroponics in a greenhouse. HortScience 52(12):1742-1747.
Gent, M.P., W.H. Elmer, K. Macherla, and R.J. McAvoy. 2016. Effects of salinity and irrigation management on growth and nutrient concentrations in poinsettia. HortScience 51(4):427-434.
Gerovac, J.R., R.G. Lopez and N.S. Mattson. 2015. High tunnel versus climate-controlled , greenhouse: transplant time and production environment impact growth and morphology of cold-tolerant bedding plants. HortScience. 50:830-838.
Harbick, K., Albright, L.D., and Mattson, N.S. Electrical savings comparison of supplemental lighting control systems in greenhouse environments. 2016 ASABE Annual International Meeting. American Society of Agricultural and Biological Engineers, 2016. 8 pp. DOI: 10.13031/aim.20162460478
Healy, W. Piles of money. Grower Talks, March 2009.
Hong, C. 2014. Component Analyses of Irrigation Water in Plant Disease Epidemiology in: C. Hong, G.W. Moorman, W. Wohanka, C Büttner (eds.) Biology, Detection, and Management of Plant Pathogens in Irrigation Water., pp.111-121. APS Press, St. Paul, MN.
Ishii, M., L. Okushima, H. Moriyama, S. Sase, N. Fukuchi, and A.J. Both. 2015. Experimental study of natural ventilation in an open-roof greenhouse during the summer. Acta Hort. 1107:67-74.
Jackson, B.E., R.D. Wright, M.M. Alley. 2009. Comparison of fertilizer nitrogen availability, nitrogen immobilization, substrate carbon dioxide efflux, and nutrient leaching in peat-lite, pine bark, and pine tree substrates. HortScience (44); 781-790.
Kubota, C., M. Kroggel, A.J. Both, J.F. Burr, and M. Whalen. 2016. Does supplemental lighting make sense for my crop? – Empirical evaluations. Acta Hort. 1134: 403-412.
Li, Y. and N.S. Mattson. 2015. Effects of seaweed extract application rate and method on post-production life of petunia and tomato transplants. HortTechnology. 25:505-510.
Li, Y. and N.S. Mattson. 2016. Effect of different commercial substrates on growth of petunia transplants. HortTechnology. 26(4):507-513.
Lopez R., and E.S. Runkle. 2017. Light management in controlled environments. Meister Media Worldwide. Willoughby, OH
Macherla, K.L. The effect of salinity on the growth and nutrient status of ornamental crops grown under partial- and full-saturation sub-irrigation management. (2014). Master's Theses. 645. http://opencommons.uconn.edu/gs_theses/645
Macherla, K. and R.J. McAvoy. 2017. The effect of salinity on the growth and nutrient status of zinnia grown under short- and long-cycle subirrigation management. 52(5):770-773
Mack, R., J.S. Owen, A.X. Niemiera, and J. Latimer. 2017. Virginia nursery and greenhouse grower survey of best management practices. HortTechnology 27(3):368-392
Mattson, N. 2014. Comparing substrate fertilizer amendments for spring bedding plants. Greenhouse Grower Magazine. 32(13):48-54.
Mattson, N.S., M. Bridgen, and N. Catlin. 2014. Using controlled-release fertilizer to produce garden mums. Greenhouse Grower Magazine. 32(9):72-74.
Million, J.B., J.E. Barrett, T.A. Nell, and D.G. Clark. 2001. Late-season applications of media surfactant improve water retention and time to wilt during postproduction. Proc. VII Int. Symp. On postharvest Physiology Ornamentals. Acta Hort. 543.
Mitchell, C.A., J.F. Burr, M.J. Dzakovich, C. Gómez, R. Lopez, R. Hernández, C. Kubota, C.J. Currey, Q. Meng, E.S. Runkle, C.M. Bourget, R.C. Morrow, and A.J. Both. 2015. Light-Emitting Diodes in horticulture. Horticultural Reviews 43:1-87. John Wiley & Sons, Inc., Hoboken, NJ.
Nell, T.A. 1994. Potted plants: prolonging shelf performance, postproduction care and handling. Ball Publishing, Batavia, IL.
Quagrainie, K.K., R.M.V.Flores, H.J. Kim, and V. McClain. 2017. Economic analysis of aquaponics and hydroponics production in the U.S. Midwest. Journal of Applied Aquaculture 30 (1):1-14.
Raudales R.E. 2016. Nutritional Disorders of Florists’ Crops in: RJ McGovern, WH Elmer (eds.), Handbook of Florist's Crops Diseases, Handbook of Plant Disease Management, DOI 10.1007/978-3-319-32374-9_2-1 Springer Meteor, Switzerland
Raudales R.E. 2017. Sensitivity of Impatiens walleriana to chlorine in irrigation water. HortScience 52(9):S450 (Abtr.)
Raudales R.E., C.R. Hall, and P.R. Fisher. 2017. The cost of irrigation sources and water treatment in greenhouse production. Irrigation Sci. 35(1): 43-54
Schmilewski, G. 2017.Growing media constituents used in the EU in 2013, (Eds.) A. Baumgarten, B. Carlile, M. Raviv In: Proc. Intern. Symp. On Growing Media, Composting, and Substrate Analysis. Acta. Hort. Pp. 85
van Iersel, M.W., K. Seader, and Sue Dove. 2009. Exogenous absisic acid application effects on stomatal closure, water use,and shelf life of hydrangea. J. Environ. Hort. 27(4): 234-238.
Wallace, C and A.J. Both. 2016. Evaluating operating characteristics of light sources for horticultural applications. Acta Hort. 1134:435-444.
Wallace, C. and Both, A.J. 2016. Evaluating operating characteristics of light sources for horticultural applications. Acta Hort. (ISHS) 1134:435-444. https://doi.org/10.17660/ActaHortic.2016.1134.55
Wongkiew, S., B.N. Popp, H.J. Kim, and S.K. Khanal. 2017. Fate of nitrogen in floating-raft aquaponic systems using natural abundance nitrogen isotope. International Biodeterioration & Biodegradation 125:24-32.
Zhen, S. and S. Burnett. 2015. Effects of substrate volumetric water content on English lavender morphology and photosynthesis. HortScience 50:909-915.
Zhen, S., S. Burnett, M.E. Day, and M.W. van Iersel. 2014. Effects of substrate water content on morphology and physiology of rosemary, Canadian columbine, and cheddar pink. HortScience 49:486-492.