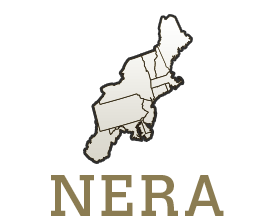
NE1834: Genetic Bases for Resistance and Immunity to Avian Diseases
(Multistate Research Project)
Status: Inactive/Terminating
NE1834: Genetic Bases for Resistance and Immunity to Avian Diseases
Duration: 10/01/2018 to 09/30/2023
Administrative Advisor(s):
NIFA Reps:
Non-Technical Summary
Statement of Issues and Justification
a) The Ongoing Need for this Work, as indicated by stakeholders
The US per capita poultry (chickens, turkeys) consumption equals the combined per capita consumption of both beef and pork. The consumption of poultry worldwide has steadily increased since 1960. Given the high feed efficiency of poultry, this food source represents one of the most economically- and environmentally-sustainable means to provide protein to the growing human population. United Nations projects the 2050 world population to be 9.7 billion people up from the 7.3 billion people in 2015. Poultry breeders and producers seek to provide consumers with a wholesome product with higher production efficiency and lower production cost to meet this ever-increasing demand.
Disease remains a major issue for the poultry industry. Economic losses due to morbidity, poor performance, and mortality are significant with the added threat that some bacterial and viral zoonotic pathogens can cause human illness or death. While advances have been made in controlling many poultry pathogens, the impact of diseases is one major impediment for sustained productivity. The total disease impact on poultry production not only includes losses due to mortality, decreased meat and egg production, and condemnations at processing, but encompasses the increased costs in prevention (i.e. vaccinations, biosecurity, and eradication programs for exotic diseases). Moreover, with a major focus on antibiotic free (ABF) production, understanding and optimizing immune function has become of paramount importance to maintaining sustainable levels of production.
This project aligns with the producers’ goal of the judicious antibiotic use in poultry meat and egg production, since healthier birds will reduce the events in which these drugs are necessary. The use of pharmacological agents (e.g., antibiotics) to treat disease poses its own challenges to animal production, the environment, as well as the wellbeing of poultry and the consumer.
Protection of poultry flocks against endemic and exotic diseases is a priority for meat and egg producers. The 2015 outbreak of highly-pathogenic avian influenza (HPAI) in the U.S. (Washington, Wisconsin, Minnesota, and Iowa) and subsequent smaller outbreaks in Montana, Tennessee, Alabama, Kentucky and Georgia, remind us of the vulnerability of these systems. In addition, consumer preferences are also driving commercial production systems to be more open (free range), elevating the risk for exotic disease introduction. The U.S. Poultry and Egg Association defines 28 critical needs for controlling disease and ensuring food safety in poultry. These needs include a focus on prevention of diseases, while decreasing the use of antibiotics, pesticides and anti-parasitic drugs.
This project addresses the genetic bases of disease resistance and immunity in poultry, as well as mechanistic understanding of innate and adaptive immune processes; issues having fundamental importance. Primary stakeholders (the most immediate users of these data, reagents, and tools generated in this project) are poultry breeding, vaccine, and allied animal health industries. Their frequent participation in the annual Technical Committee meetings, and their many collaborative research with the members, clearly indicate the high value that stakeholders ascribe to this project.
b) The Essential Nature of this Project
This work is essential to advance disease prevention and control strategies that ensure a sustainable poultry industry with increased production for a growing world population. Genetic variability is inherent within populations of species and is a product of natural selection. This project addresses the important issues of genetic bases of resistance and immunity to diseases in poultry providing stakeholders with a better understanding of genetic variability within their stocks in order to produce future populations with sustainable, desired traits. Disease resistance as a genetic trait is very multifaceted. Sometimes one or a few genes, such as the major histocompatibility complex, affect disease responses. Other instances are more complex with many contributory genes, making heritability low, and difficult to improve by traditional genetic selection methods. Although there is a chicken genome sequence, much remains to be learned about loci that are naturally polymorphic and about the functional outcomes of interactions among polymorphic loci that are increasingly under directed selection. We will also be able to better understand immune responses to common poultry pathogens through this project. This information is crucial in order to strategize novel preventative strategies, which in some cases can be cross protective. Disease is one of the major limiting factors in large-scale and small-scale poultry production. Shifts toward managing birds in less-controlled environments, and reduction of antibiotics use in animal production are exacerbating disease issues. Currently pathologies that seemed to be controlled are recurring thereby disrupting commercial production. This project addresses the important issues of environmental and physiologic factors that regulate or affect immune system development, optimal immune function and disease resistance in poultry. If this work is not done, disease will increase, production efficiency will decrease, food safety will be greatly compromised, and as a result export markets will be closed. This project also addresses the need for development of methods, reagents and specialized genetic stocks to be able to assess, monitor and modulate immune system development, patterning and function. In contrast to biomedical model species, such as mice, few of the reagents needed for poultry research are commercially available. If immune development is not monitored, immune responses to vaccine and disease organisms could deteriorate, thereby increasing morbidity and mortality. Understanding immune system development as affected by genetic and environmental factors will find direct application in the breeding poultry stocks that have improved health and effective responses to vaccination. These improvements will contain production costs.
c) The Technical Feasibility of This Project
The NE-1334 Technical Committee collectively possesses a spectrum of scientific expertise to execute the collaborative research essential for the future of poultry production. Their a range of expertise encompasses many disciplines; including: immunology, infectious diseases, genetics, genomics, virology, kinomics, poultry medicine, physiology, nutrition, biochemistry, microbiology, and molecular biology. The work is technically feasible as it is rooted in methodologies that have been demonstrated as successful when used in other species, most notably human medicine. In the past decade, next generation sequencing, gene editing and many highly sophisticated methods have become now available to examine the expression and interactions of genes important in disease resistance. The techniques can be readily applied in investigations of disease especially in genetically defined experimental lines. The researchers work on the leading-edge of science, have demonstrated their expertise with the requisite infrastructure to successfully complete the described work, if sufficient financial support is made available.
d) Advantages for doing the work as a multistate effort (The Essential Collaborative Nature of this Project)
Conducting this work as a multistate project offers the advantage of pooling and sharing resources to address critical scientific questions. The members of the NE-1334 committee are well-established scientists conducting research in a range of disciplines to examine disease resistance at all levels. In addition, participants have unique skills or specialized resources such as genetic stocks and poultry-specific reagents that are needed to conduct the work. The multistate effort is required for the synergistic and collaborative conduct of research that is based upon the combination of biological materials (experimental lines of birds, antibodies, cell lines, pathogen stocks), facilities, equipment and expertise from multiple stations. No single station possesses all of these to address the major scientific issues for the project. Conducting this work as a multistate effort allows for the greatest efficiency of resource use from 27 independent laboratories from 17 U.S. states [AL (1), AR (1), CA (6), DE (1), GA (1), IA (1), IN (1), MD (1), MI (USDA-ADOL, 1), MO (1), NC (2) , NY (1), OH (1), TX (1), VA (1), WI (1), WV (1)], and 2 other countries [Canada (2, ON, PEI) and the Netherlands (2, NL)] in the current NE-1334 group of scientists - each addressing complementary aspects of the problem. The truly essential, cooperative, multidisciplinary nature of the project is illustrated by the many joint-authored publications among participating stations. Between 2015 and 2016, the NE-1334 project members have produced 119 refereed publications and 145 abstracts many of which feature joint authorship among multiple stations. The extensive expertise of the NE-1334 Technical Committee members and collaborators is also very clearly illustrated by the members’ contributions to books such as Avian Immunology (published in 2013) co-edited by a NE-1334 member plus authorship in 7 of the 22 chapters plus one of the two appendices. In addition, contributions of NE-1334 Technical Committee members and collaborators have been recognized by the Poultry Science Association (PSA) and the American Association of Avian Pathologists: PSA Early Achievement Award for Research, Hy-Line International Poultry Science Research Award, Embrex Fundamental Science Award, Evonik Degussa Award for Achievement in Poultry Science, Novus International, Inc. Teaching Award, two US Poultry Distinguished Poultry Industry career recognitions, Induction as Poultry Science Association Fellow and Bayer Snoeyenbos New Investigator Award.
In addition, this project synergizes and collaborates closely with other projects. There is very strong cooperation between NE1334 and the other most relevant multi-state project: NC1170, Advanced technologies for the genetic improvement of poultry. Multiple Technical Committee members of NE1334 are also members of NC1170 (including one who has been an active member of both projects for over 34 years), and their active participation in both projects allows for excellent coordination between the two multi-state project, ensuring good communication and collaboration while avoiding duplicative efforts.
e) Outcomes and Impacts of this Project
Impacts are expected to include but not be limited to: a better understanding of polymorphic loci and the consequence of selection on poultry health and production; new breeding strategies to produce more robust, disease resistant lines of poultry; improved efficacy of vaccines and other pharmaceutical agents; new vaccine programs for controlling existing as well as emerging diseases; a better comprehension of immune responses to specific antigens and a better fundamental understanding of how the avian immune system functions. These impacts aid future scientists by facilitating prevention or control strategies for current issues plus new problems that will arise. Improved disease resistance and enhanced prevention strategies will boost production efficiency, animal health and hence welfare. Reduced antibiotic use and improved poultry product safety will have favorable consumer reception. Much new knowledge in the basic and translational sciences, as well as, reagents and tools generated by this project, will constitute valuable resources to the stakeholders.
Related, Current and Previous Work
ACRONYMS
AR = Arkansas
UCD = Univ of California-Davis
DE = Univ of Delaware
GA = Univ of Georgia
IA = Iowa State
BRI = Beckman Research Institute, City of Hope
UMD = Univ of Maryland
NC = North Carolina State Univ
NL = Wageningen Univ
OH = Ohio State Univ
PEI = Prince Edward Island Univ
UA = University of Alberta
ADOL = United States Department of Agriculture, Avian Disease and Oncology Laboratory
VT = Virginia Tech
WU = University of Washington
WV = West Virginia University
WVC = Western University of Health Sciences
The NE-1834 multistate research project scientists design, create, maintain, and study unique poultry genetic lines. Some members carry out all of these functions and others a subset as an integral part of our research. These efforts have been our contribution and our responsibility to achieve the project objectives of understanding the genetic bases for immunity to avian diseases. Special genetic lines, established over the last 80 years, are at risk at many research stations. If lost, these unique avian genetic resources (e.g., congenic, recombinant, and inbred lines) are unlikely to be recreated. Since member scientists share these genetic resources in collaborative research, their elimination will impact the project, collaborators and the avian research community at large. The Technical Committee recognizes the imperative to conserve the resources currently available. Several Technical Committee members served on the Avian Genetic Resources Task Force and are now part of the National Animal Germplasm Program, Poultry Committee. The members are committed to the establishment of a national system of networked researchers and a site for orphaned stock conservation to support our objectives of understanding and improving resistance to diseases in poultry. Innovative technologies such as candidate gene identification, applications of recombinant DNA, monoclonal antibodies, DNA probes, QTL analysis, global and targeted transcriptome analysis, gene sequencing and other novel molecular approaches have been effectively used to identify and characterize many facets of disease resistance or immune function. These techniques expand upon the pioneering work conducted by NE-1834 members throughout the project history. Project results continue to be important and readily applicable in both research and industry. Commercial poultry breeders lead other animal breeders in terms of improvement of a variety of economic traits, including genetic resistance to disease. Further research on new methods to select for disease resistance in poultry must, and will, continue in the proposed renewal project for NE-1834. Recent scientific advances in understanding the immune system and enhanced knowledge about poultry pathogens promise imminent and significant improvements in poultry health, production efficiency, food safety and animal wellbeing through genetic selection. Extensive publication searches indexed in the comprehensive databases (Agricola, Biosis, CAB, CRIS, Health Index, and Medline) for the last five year period revealed substantial scientific contributions that NE-1834 members have made in genetics of disease resistance and immune response in poultry. A significant number of the current Technical Committee members are also part of the NC- 1170 multi-state project on advanced technologies for the genetic improvement of poultry. Through interactions with NC-1170, which focuses their research efforts more on genomics and system biology of poultry and elucidation of genetic mechanism that underlie economic traits, duplication can be avoided. The two groups overlap in the creation and sharing of poultry research populations and research tools, including gene transfer technologies, hence maximizing resources. Compared to other multi-state projects that focus on specific diseases [NC-1202; Enteric diseases of food animals; enhanced prevention, control and food safety] or animal welfare beyond animal health [NE-1042; Optimizing poultry welfare], the uniqueness of the NE-1834 group lies in examining genetic bases of resistance to diseases in avian species in the context of all levels of immune system development and function. Working relationships, either formal or informal, exist between NE-1834 stations and the international laboratories conducting similar research. This, too, assures coordination of efforts and avoidance of unnecessary research duplication. Most of the members also participate in meetings of the Avian Immunology Research Group which is an international conference that brings together researchers in avian immunology from all over the world to share advances in this field, build collaborations and avoid duplicate efforts. Participation of international contributors to NE-1834 from institutions in Canada (UA, PEI) and the Netherlands (NL) demonstrates the stature of the project. To highlight accomplishments achieved by the NE-1834 investigators during the past 4 years of this project, a summary of major contributions under each Objective and the need for continuation are presented below.
Objective 1 "To Determine how Allelic Variation Influences the Efficacy of Innate and Acquired Immune Functions"
Efforts by UCD, BRI, NL yielded a more extensive description of the organization and sequences of the B- and Y-MHC regions and MHC-B and MHC-Y haplotypes. Within this effort, the correct order of MHC genes on gga-16 was further defined revealing the presence of a here-to-fore unknown gene segment within the MHC. The nucleolus organizer region (NOR) was found to be tightly linked to the MHC-Y and separated from the telomeric MHC-B by a GC-rich region. Hence, the lack of linkage between the MHC-Y and -B is not the result of being separated by the NOR. This work debunked the untested hypothesis of the NOR being responsible for the lack of linkage of Y from B and opened up an entirely new avenue of research to explore the content of the GC-rich region, which likely includes repeat elements and other genes having important physiologic function relevant to the immune response and resistance or susceptibility to disease. Extensive sequence data for 14 MHC-B haplotypes, as well as, detailed definitions of the binding motifs for two MHC-B class I (BF) antigen-presentation molecules and candidate viral peptides, opened new venue for the study of target cell recognition by Tc. This research also led to the discovery that only few of the MHC-YF class I proteins are expressed in chickens and that at least one of these may represent a new type of antigen presentation molecule with a hydrophobic binding groove able to present non-peptide molecules. Molecular definition of MHC haplotypes was extended beyond the use of the LEI0258 microsatellite marker based on finding micro-variation in the BF1 or BLb2 gene exons. For reliable molecular rather than serological MHC-typing, further characterization of gene exon variation is required in addition to the molecular MHC typing using the LEI0258 microsatellite marker. AR, UCD, BRI, WVC, DK, IA, ON, and VT investigators studied the sequences, expression and function of the products of other genes playing a role in immune function. Molecular characterization of chicken natural killer (NK) cells, heterophils and macrophages, as well as, cloning and characterization of avian cytokines and receptors (e.g. IL-19, IL-22, IL22BP, MIF, Nod1/2, TLRs, scavenger receptors) greatly increased our understanding of genes and their products involved in avian immune system development and function. The effort initiated and led by VT to sequence, annotate and analyze the turkey genome and immune related genes provides the much needed basic knowledge and tools for the study of disease resistance and immunity in turkeys. Further, part of the mannose-binding-lectin (MBL) promoter was cloned and sequenced to identify polymorphism in two inbred and various commercial and experimental lines. In total, 14 SNPs were identified which resulted in identification of six different promoter alleles. The allele A1 was found to be associated with low MBL in serum and it was found in inbred lines as well as in commercial lines. The phenotypic consequences of MHC-haplotypes in terms of immune system function and disease resistance were examined through live-bird challenges, quantitative trait locus mapping, single nucleotide polymorphisms (SNP) analyses, establishment of linkage maps based on microsatellite markers and SNPs, global microarray and qPCR assessment of gene expression (UCD, WVC, DK, IA, ADOL, NH). Using these approaches, many genes, genomic regions and signaling pathways associated with the host response to infection with pathogens such as E. coli, Salmonella, and Campylobacter in the chicken have been identified providing important direction for further study into these economically important bacterial infections. Similarly, microRNAs and signaling pathways related to avian influenza virus infection in chickens have been identified using high-throughput technology including microarray and next-generation sequencing. Other major contributions to our understanding of viral infections in poultry include NH’s observation that Marek’s disease incidence was affected by a single locus BG1 3’-untranslated region difference identified in congenic lines 003.R2 and 003.R4 with serologically identical MHC recombinant haplotypes. DK established that different MHC haplotypes were associated with different amounts of antibodies to infectious bursal disease virus (IBDV) and lower disease severity after experimental infection of chickens. A similar association of MHC-haplotype with antibody levels and pathology was also found in Newcastle disease virus (NDV) infected chickens and for parasite egg burden after a challenge with Ascaridia galli. Greater resistance to clinical illness and better viral clearance in infectious bronchitis virus (IBV) infected chicks with the B2/B2 compared to the B19/B19 MHC-haplotype were also reported by CA-WU and collaborators. These studies offer new opportunities for characterization of genetic regulation of resistance and immunity to pathogens in poultry.
Objective 2 "To Identify Factors and Agents Affecting Poultry Immune Development, Function, Dysfunction and Pathology"
The group has also made significant accomplishments regarding basic characterization of innate and adaptive immune functions and examination of the influence of genetic, environmental, nutritional and physiological factors on these processes. For these studies, availability of genetic lines with optimal and suboptimal immune responses to an experimental antigen has been very helpful. Lines selected for high or low antibody responses to SRBC were shown by NH to exhibit differential bursal gene-expression profiles revealing biomarkers unique for high and low SRBC-Ab responders as early as day 15 of incubation. Embryonic testosterone propionate exposure, which results in bursal ablation, influenced distinct pathways in birds from the high and low SRBC-Ab responder lines. USDA-ADOL compared MDV-susceptible (7-2) and resistant (6-3) lines based on expression analysis with a panel of immune-related genes revealing a much more vigorous, especially T cell-mediated, immune response in line 6-3 than line 7-2. Additionally, transient paralysis could be observed in both lines with high pathogenic strains of MDV. Using MHC-defined lines of chickens, known to respond differently to infection with pathogens or to have different innate immune activity to PAMPs or other immunostimulants, several members (BRI, WVC, UCD) were able to better define the nature of an effective or ineffective immune response. BRI and WVC in collaboration with others have helped to elucidate mechanisms underlying the activation of natural killer cells, T lymphocyte responses and macrophages. Evaluation of chicken monocytes as a factor in disease resistance showed that B2/B2 monocytes differentiated more readily into macrophages, were stimulated to significantly greater levels with either poly I:C or IFNgamma, and exhibited differential upregulation of at least 9 pathways compared to B19 stimulated macrophages. Genetic lines prone to non-communicable diseases with immune system involvement (e.g. autoimmune disease, ascites, skin disorders, lameness, etc.) were used by AR, ADOL and NH for comparison of aberrant versus normal immune activities. AR identified IL-21, IL-10 and IFNgamma as the signature cytokine profile associated with autoimmune vitiligo onset and progression in susceptible Smyth Line (SL) chickens. Global gene-expression analysis of the target tissue (feather) before and throughout SL vitiligo development established a role of innate and adaptive immunity, as well as, cellular stress. Independent of serotype, MDV infection administered at hatch was reliably associated with vitiligo expression in susceptible SL chicks. Based on HVT administration, the ability of MDV to trigger SL vitiligo is limited to infection during the first 6 weeks of life. Susceptibility to autoimmune SL vitiligo appears to be manifested in part in target cell (melanocyte) defects. Studies by NH using an atherosclerosis model also reports differentiallyexpressed genes and soluble proteins found in aortic smooth muscle cells in atherosclerosissusceptible White Carneau and atherosclerosis-resistant Show Racer pigeons. Immune activity and pathogenic mechanisms initiated as a result of PAMP administration, viral, bacterial, or parasitic infections were also investigated in selected lines of chickens, commercial layers, broilers and turkeys by members and collaborators of NE-1034 (AR, UCD, DE, DK, NC, NL, NY, ON, PEI and VT). These studies yielded critical new knowledge regarding impact of physiological factors on aspects of disease susceptibility, disease progression, activities and interrelationships of innate and adaptive immune systems, virulence of pathogens, immunodominant epitopes, nature of effective or ineffective host responses and approaches for disease intervention and prevention. An eight year longitudinal survey of SPF flocks at NY infected with immunosuppressive chicken infectious anemia virus (CIAV) showed that antibody development to CIAV started in general on or after sexual maturity with significant differences in levels of seroconversion during this period. These findings suggest that the infection may remain latent and that reactivation is linked in part to sexual maturity. Studies on avian influenza (AI) by NY using the highly pathogenic H5N1 (VN1203/04) isolate showed that thrombocytes play an important role in the pathogenesis in chickens but not in ducks. ON conducted extensive study on gene-expression profiles during infection with different serotypes of Marek’s disease viruses in a variety of tissues known to play a key role in infection, latency and transmission of these MDV. DE studied the effects of innate immune stimulants on the replication of MDV vaccine strains and overall vaccine efficacy. Inclusion of some select innate inducers (e.g. PAMPs) did not interfere with vaccine virus replication, despite potent induction of innate responses but did not have appreciable effects on cell-mediated immune function and MD protection. Gene expression profiling by USDA-ADOL between rMd5 and rMd5Dmeq infected chickens revealed that Meq functions as an immunosuppressive oncogene that results in down-regulation of many immunerelated genes and may be controlling the expression levels of p53 involved in regulating the cell cycle and tumor development. NC worked to characterize numerous circulating strains of the type- 2 turkey astrovirus isolated from commercial turkeys across the U.S. to identify potential virulence markers. In the infected host, astroviruses induced a reduction in the apical expression of sodium/hydrogen exchanger-3 which contributes to mal-absorption and diarrhea. The infected epithelial cells responded to infection by expressing inducible nitric oxide synthase (iNOS), which likely plays a key role in eliminating the virus in the immunologically immature host. VA assessed the differential genetic resistance to clostridial toxins in select chicken lines and conducted serum protein profiling and identification of potential blood markers in Eimeria-infected chickens from commercial genetic stocks. Influence of environmental factors on mucosal and systemic immunity has been a focus of research by AR, NC, NL, ON, and VA. AR and collaborators observed different vasoactive and cytokine responses to local pulmonary inflammatory activities induced by PAMPs or vascular occlusion in ascites-susceptible or -resistant broiler lines. ON developed a probiotic formulation that possesses immune stimulatory activities, which they plan to test in commercial settings in the near future. VA is evaluating the effects of antibiotic alternatives in commercial chickens (e.g. betaglucans, probiotics) using various delivery routes and disease models. Immunomodulatory effects of concurrent administration of model-antigens and PAMPs typically present in the air of poultry houses were observed by NL particularly in young broiler and layer chickens. Adaptive systemic immune responses were also shown to be affected by the absence of microflora in the gut following antibiotic treatment as well as by administration of probiotics. Through these studies, the period of 3-6 weeks of age was identified as a critical time in the development of mucosal immunity. Investigations into the role of nutrition in the immune responses of poultry by UCD established that nutrients that have primarily regulatory functions (vitamins A and D, and essential fatty acids) had greater effects on development of the immune system than nutrients that serve as anabolic precursors (amino acids, energy, minerals). Vitamin A deficiency during development diminished B lymphocyte maturation and the breadth of the antibody repertoire. The entire cost of the adaptive immune response (specific antibodies and lymphocytes) was easily fueled by the decay of the acute phase proteins produced during the innate response, suggesting that adaptive immunity has no net nutritional cost. NC demonstrated that changes in the intestinal microflora of poultry alter the amount of energy consumed by the immune response. PEI characterized local and systemic innate immunity in poultry during nutritional intervention using yeast derivate carbohydrates (YDC). In several comparative studies using broiler chickens fed conventional diets including an anticoccidial (Monensin), a growth promoter (BMD), and an anticoccidial plus YDC they found that the inclusion of YDC (23% mannans) affected several immunological parameters, including regulation of intestinal microflora, intestinal architecture, cytokine expression and enhanced neutrophil activity. DK conducted studies to characterize and examine the function of mannose-binding-lectin (MBL, innate immunity) in susceptibility of poultry to different pathogens. Using chickens selectively bred for a high or a low serum concentration of MBL, low circulating levels of MBL were associated with reduced ability of poultry to respond to pathogens such as IBV, E. coli, Pasteurella muliocida, and Ascaridia galli. These results confirm that MBL, as shown in mammals, plays a major role in the outcome of various infections in chickens and may emerge as a biomarker for disease susceptibility. Similarly, investigations into natural antibodies (NAb) and health in poultry by NL showed that high levels of NAb, especially of the IgM isotype, correlate with lower mortality during a lay period. NAb levels were found to be very heritable (0.4), related to SNP in immune response-, behavioral-, and unknown-gene regions. Whether NAb originate from introduction of the intestinal microbiota or reflect homeostatic auto-antibodies is subject of future studies. Their studies also showed that NAb binding to protein extracts from chicken organs may provide a fingerprint for measuring the health status of individuals.
Objective 3 "To Develop and Employ Genetic Stocks, Methods, Reagents and other Tools to Assess Basic Immune Function, Characterize Immune Evolutionary Processes, Guide Genetic Selection, and Increase Resistance to or Protection Against Avian Diseases"
Efforts by the group resulted in development of a variety of tools and basic data for continued research on the genetic bases of resistance and immunity to avian diseases. A number of stations (AR, UCD, DK, IA, NC, NL, ADOL) developed, maintained, characterized, and made available unique genetic resources to the NE-1034 members and the entirety of the poultry research community. These included genetic lines that are highly inbred and contain MHC-congenic sets, are MHC-defined, and/or exhibit defined disease susceptibility/resistance characteristics. DK breeds of chicken were found to have five B21-like haplotypes including B131 (broiler origin) and BW1 (Red Jungle Fowl, Gallus gallus gallus origin). AL generated a better understanding of the mucosal immune system in chickens induced by IBV vaccines or Ad5 vaccine vectors expressing the avian influenza HA gene. AL found the head associated lymphoid system, i.e., conjunctiva-associated lymphoid tissues (CALT) and Harderian glands (HDGL), to generate immunity to avian pathogens after ocular or in ovo immunization. CALT generated more of a cell-mediated immune response and after priming seemed to contain cytotoxic effector memory T cells, while the HDGL generated more of a B cell response. The spleen played a minor role after mucosal IBV priming, but generated the highest IFN-gamma response after boosting, indicating induction of a central memory T cell response. IgA dominated the primary response, while IgG dominated the memory response to IBV. Advances made by the group to facilitate the study of immunity include development of chicken whole genome 44K gene expression array (UCD) that has been widely used in the poultry community; increased availability of genetic information to conduct targeted qPCR expression analyses of cytokine and other genes; and, B-cell spectratyping including IgA, IgG and IgM isotypes. Western blotting was optimized to measure post translational polymorphism of NAb and auto-antibody fingerprints. A minimally invasive procedure to monitor cellular immune responses in vivo using the growing feather as an in vivo test-tube was developed by AR. Based on analyses of antibody responses using a peptide array, AL reported that mutations in the S1 protein of IBV contributed to immune escape. VT identified innate immune markers correlating with disease resistance to coccidiosis. NC developed reagents for use in the study of turkey immunity, including polyclonal antibodies to the turkey iNOS protein. NY developed an antigen-antibody complex vaccine that does not cause damage in chickens lacking maternal antibodies to CIAV and protected against replication of a challenge virus. Over the last four years, DE’s research on the evolving MDV resulted in identification and development of various MDV mutants for research. Included are mutations in the main oncogene (Meq) of MDV, Meq splice variants observed during MDV pathogenesis, and a glycoprotein L mutation common to high virulence MDVs. Studies with these MDV mutations focused on their effects on transactivation, target gene expression, cell shape and mobility, immune evasion and pathogenesis and the immune response. Use of these mutated MDV viruses has already provided insight into the impact of viral genes on tumor composition, mechanisms by which MDV regulates immuneassociated genes, virulence, viral T cell epitopes presented, and effects on the early patterning of immune responses. While transgenic approaches to study the function of genes is not yet readily available for avian species, virus vectors approaches have been employed by members of the group. ON developed a prototype virus-vectored system for down-regulation the expression of IFN-gamma, a system that can be modified for down-regulation the expression of other cytokines in the future. WI has created a generation 3 self-inactivating lentiviral expression vectors for in ovo administration in preparation for expression of the Mx transcript from the highly pathogenic AI virus resistant Blue-winged Teal in chickens under control of an inducible promoter.
Objectives
-
To determine how allelic variation influences the efficacy of innate and acquired immune functions.
-
To identify factors and agents affecting poultry immune development, function, dysfunction, and pathology.
-
To develop and employ genetic stocks, methods, reagents, and other tools to assess basic immune function, characterize immune evolutionary processes, guide genetic selection, and increase resistance to or protection against avian diseases.
Methods
Over the past 30-40 years, the field of immunology and immunogenetics continuously has seen extensive advances, particularly with regard to immune system function and development in mammals. The increased understanding of immune system development and function stems primarily from studies on the mouse model, where manipulation of gene-expression seems to have unlimited potential in dissecting mechanisms of cell development and function. With the availability of the chicken genome sequence since 2004, holistic approaches such as genome-wide sequencing and whole transcriptome and proteome analyses have been carried out providing new insights into unique features of the avian immune system, disease resistance and susceptibility as well as the mechanistic aspects of immune system development and function in chickens. As additional avian resources are becoming available particularly through contributions of member stations, such as the recently published turkey genome and much improved chicken genome, poultry scientists are well positioned for further discoveries pertaining to avian immunity and their inherent responses to disease challenges. Since the complexity of the immune system’s molecular and cellular components, genetic regulation underlying the functional responses of the immune system, and the interplay between genetic, immune system, environmental, physiological and nutritional factors have become apparent, such discoveries will significantly better our knowledge in this arena and further enhance the U.S. global competitiveness.
- To determine how allelic variation influences the efficacy of innate and acquired immune functions.
Variation in DNA sequence can directly impact immune function, can modulate expression of genes that impact immune functions, and can serve as markers for other causative variants with which they are in linkage disequilibrium. This project will apply genetic, genomic and immunological approaches to assess the impact of allelic variation on response to specific pathogens or other immune stimuli. Several stations will focus on the major histocompatibility complex (MHC) region. BRI will investigate the role of MHC-Y haplotypic variation and, when possible, individual loci variation on immune responses in chickens. WVC will keep investigating the underlying genetic mechanisms of differences in innate immune functions, particularly macrophages, of B2 and B19 haplotypes. WVC will further analyze if epigenetic modifications/variations are responsible for gene dysregulation in B19 haplotypes after stimulation of macrophages with IFN g. UA will expand its studies on analysis of the many alleles of the MHC class II region of mallard ducks by moving to high throughput approaches with additional animals. UCD will study the bases of resistance to respiratory diseases, specifically infectious bronchitis virus (IBV), using MHC congenic lines. In addition to in vivo challenges, UCD will perform in vitro challenges to investigate the specifics of the immune response without background noise. UCD will assess and compare cell activity in tracheal cultures and macrophages derived from relatively resistant and susceptible congenic chicken lines. In UCD and IA, genetic variants in diverse chicken populations that differ in immune response to viral infection will be identified using next generation sequencing and high density SNP genotyping, and associations between genetic variants and immune-related parameters such as viral load, antibody response, and others will be analyzed. IA will investigate the genetic basis of response to avian pathogenic E. coli (APEC), including the impact of allelic variation and allele-specific expression, and association of genetic pathways identified by RNA-seq with immune phenotypes. To identify candidate genes and gene regulatory networks for resistance to herpesvirus infection, UMD will analyze transcriptomic data in immune cells from infected and non-infected individuals of two reciprocal crosses. WV will use sequence and SNP analyses to identify and characterize alloantigen system genes whose products are detected by antibodies. NL will focus their efforts on looking into the associations, using keyhole limpet haemocyanin (KLH) of SNP’s with natural antibodies of isotypes IgM and IgG. These associations will be assessed binding KLH and auto-antigens in laying hens.
2. To identify factors and agents affecting poultry immune development, function, dysfunction, and pathology.
There are numerous genetic, environmental, nutritional, physiological, management, and microbial factors that stimulate, regulate, and shape the immune response of poultry. The members of NE-TEMP 1834 have been at the forefront of research to understand basic mechanisms and unique features of the the avian immune response, to develop novel and effective means to promote poultry health and production. Over the past 4 years the members of NE-TEMP1834 have produced over 250 peer reviewed publications, and awarded approximately 15 competitive NIFA grants worth over $6 million. The majority of these efforts are related to our understanding of factors and agents affecting poultry immune development, function, dysfunction, and pathology, and represent some of the most influential studies related to avian immunity over the past 5 years. As this highly successful project continues, the members of NE-TEMP1834 will continue to use a variety of poultry systems, immunomudulatory approaches, and pathogens to expand our understanding of the avian immune response. AR will determine immunopathology, immune system dysregulation, and the role of environmental factors in multifactorial, non-communicable diseases such as fibrosis/scleroderma, vitiligo, thyroiditis and other (auto-) inflammatory diseases. AR will determine basic innate-and adaptive immune system mechanisms in poultry and immunomodulatory effects of nutrients on immune system development and function. Methods will include a range from whole animal studies to histological, cellular and molecular examinations, including gene-expression at the transcriptome and protein level. UCD will investigate the effects of viral respiratory infection, specifically IBV, on the upper respiratory microbiome. UCD will examine the difference in immune function and development in genetically distinct chicken lines using RNA-seq and flow cytometry. Additionally, the molecular mechanism of disease resistance will be further investigated using CRSPR-cas9. DE will continue working with Industrial partners to test innate immune inducers that increase the resistance of poultry to various microbial agents. DE will use transcriptomic analysis of the effects of these inducers on innate signaling and ultimate patterning of acquired immune responses. DE will also employ a kinomic approach to study poultry health and disease from an immunometabolism perspective. This approach broadens our view of health, metabolism, disease pathogenesis and potential intervention strategies, and identify metabolic intermediates or immune modulatory compounds to be used therapeutically. GA will study the development of T-regulatory cells and the contribution of T-regulatory cells to Salmonella persistence, with ultimate goal of developing a nanoparticle based vaccine against Salmonella. UMD will generate deep sequencing libraries from avian immune cells in order to identify epigenetic markers, patterns of alternative splicing, and noncoding RNA such as microRNA and long intergenic noncoding RNA (lincRNA). Most importantly, UMD will ascertain the factors affecting avian immune development such as enhancer, repressor, insulator and transcription binding sites (TFs) and explore their influences on chromatin and the association with immune function, dysfunction and pathology. NC will investigate the interaction between the host’s intestinal microbiome and development/function of the avian immune system. As a part of these investigations, NC will focus on how differences in host genetics affect this process, identifying key members of the microbiome, their metabolites, and ultimately their role in helping the bird resist colonization and infection by avian pathogens and foodborne pathogens that reside in poultry. NL will use homozygous SNP-typed TLR1A variant chickens, challenged with various types of infectious agents to understand its role in immunity and the production of natural (auto-) antibodies. Additionally, NL will investigate transgenerational epigenesis of specific immunity and innate immunity. OH use in ovo inoculation of d18 embryos with various types of bacteria to investigate the impact of different pioneer bacteria on GIT immune system development. As part of this work the microbiome and proteome will be analyzed up to 10 days of age, leading to deeper insights into which types of bacteria promote appropriate immune development and improved sustainability. PEI will focus on the nutritional immunological factors regulating immune responses, animal health, and food safety, with the ultimate goal to advance our understanding of the nutritional, microbiological; and molecular components affect the chicken immune response. VT will focus on the impact of in ovo and in vivo delivered supplements on the gut microbiota and development of the immune system in poultry (chickens and turkeys). VT will assess how these treatments affect gut physiology (tight junction disassembly/restructure), the impact on cellular and body metabolism, feed intake and performance, and gut immune responses. As part of these studies VT will examine these effects under specific challenges such as; necrotic enteritis, coccidiosis, APEC, salmonellosis, in addition to blackhead and cellulitis in turkeys. WU will analyze epigenetic modifications during macrophage development and investigate what influence immune stimulants (liposomal vaccines, adjuvants and others) have on training of macrophages and other immune responses in different B haplotypes.
- To develop and employ genetic stocks, methods, reagents, and other tools to assess basic immune function, characterize immune evolutionary processes, guide genetic selection, and increase resistance to or protection against avian diseases.
When paired with techniques such as next generation sequencing, SNP analysis, and qPCR, highly inbred chicken and turkey lines, sets of MHC-congenic lines, random breed lines and lines with distinct phenotypes allow the detection and selection of functional genetic elements that are related to immune function. To the extent that facilities and research budgets allow, IA will maintain, study and share with collaborators, several unique genetic stocks of chickens for research on the genetic basis of immune response and response to pathogens. The stocks include highly inbred lines, sets of MHC-congenic lines, and advanced intercrosses of lines with distinct phenotypes. UCD Regulatory elements such as enhancer, insulator, promoter in chicken genome will be functionally annotated and functional elements related to immune function will be identified. Genetic variants associated with disease resistance to virus infection will be used to genetically enhance broad immunity and resistance to specific pathogens in poultry. BRI MHC-Y was originally identified through polymorphic restriction fragments revealed in Southern hybridization. Until recently Southern hybridizations were the only means for revealing MHC-Y genotypes. BRI has developed simpler PCR-based methods for distinguishing MHC-Y haplotypes. These simpler methods make it easier to MHC-Y type large numbers of birds. BRI will continue to improve these methods and make them available for those wishing to define the MHC-Y haplotypes within genetic stocks and for use in defining the role of MHC-Y in genetic resistance to disease. NC To further the long-term goal of understanding how the genetic makeup of poultry determine it response to parasitic infections such as Histomonas meleagridis, experiments will be performed to identify SNPs associated with turkeys that can and cannot survive infection with a virulent strain of H. meleagridis. NL Chickens will be selected and bred such that homozygous genotypes will be obtained for a TLR1A variant on chromosome 4 using SNP-typing in combination with high natural antibody (Nab) levels to KLH (CC variant) and low NAb levels to KLH (GG variant). ARK To aid in research related to cellular and humoral immunity, genetic lines that spontaneously develop autoimmune diseases will be maintained and shared with project collaborators and techniques will be developed to monitor responses to antigen in blood and tissues. WV Congenic lines 003.R2 and 003.R4 will be maintained for use by project collaborators. Line 003.R4 differ from 003.R2 by a 225 bp insert in the BG-1 gene3’ UTR resulting in variation in immune responses to various diseases. ADOL Experiments will be performed to develop assays to assess epigenetic modifications in a commercial or inbred chicken line, followed by analyzing the genetic variations associated with differential immune responses in B2 and B19 haplotypes. These assays will include ChiP seq, ATAC seq, PLAC seq and others. The genomic data will be made available to the poultry community at large as a resource for further research. (ADOL, NC, WVC) To study Salmonella colonization in broilers, two immunologically divergent lines of broilers based on selecting for a high and low phenotype of key innate immune markers in both sires and dams will be generated. Additionally, the role of the gut microbiome and intestinal mucosal response (secretory IgA [sIgA]) will be examined in the founder and the selected High and Low lines to determine the interplay between host genetics, the gut microbiome and local immune response, selection pressures, and S. Enteritidis colonization. (UA) To assess immune function in chicken cells, they will make an interferon reporter construct, mCherry-tagged IRF1 and IRF7 constructs, and many cloned genes and will also develop qRT-PCR primers for many chicken immune genes including IFN-beta, Mx, OASL. (VT) To continue improving the turkey transcriptome by further sequencing of additional tissue RNAs, The data will help in refining the global turkey transcriptome during early development, updating tissue-specific and overall annotations of both transcriptome and genome, and providing public tools for comparative analyses in poultry and other avian species. (UMD) By integrating different “OMICS” data, advanced methods on host-virus interaction with small number of chicken immune cells will be developed. In addition, methods on immune response modeling analysis will be established and share with project collaborators.
ACRONYMS
AR = Arkansas
UCD = Univ of California-Davis
DE = Univ of Delaware
GA = Univ of Georgia
IA = Iowa State
BRI = Beckman Research Institute, City of Hope
UMD = Univ of Maryland
NC = North Carolina State Univ
NL = Wageningen Univ
OH = Ohio State Univ
PEI = Prince Edward Island Univ
UA = University of Alberta
ADOL = United States Department of Agriculture, Avian Disease and Oncology Laboratory
VT = Virginia Tech
WU = University of Washington
WV = West Virginia University
WVC = Western University of Health Sciences
Measurement of Progress and Results
Outputs
- Assess the impact of allelic variation on response to specific pathogens or other immune stimuli Comments: This project will apply genetic, genomic and immunological approaches to assess the impact of allelic variation on response to specific pathogens or other immune stimuli.
- Understand basic mechanisms and unique features of the avian immune response, to develop novel and effective means to promote poultry health and production. Comments: The majority of these efforts are related to our understanding of factors and agents affecting poultry immune development, function, dysfunction, and pathology, and represent some of the most influential studies related to avian immunity over the past 5 years. As this highly successful project continues, the members of NE1834 will continue to use a variety of poultry systems, immunomodulatory approaches, and pathogens to expand our understanding of the avian immune response.
- Detection and selection of functional genetic elements that are related to immune function. Comments: When paired with techniques such as next generation sequencing, SNP analysis, and qPCR, highly inbred chicken and turkey lines, sets of MHC-congenic lines, random breed lines and lines with distinct phenotypes allow the detection and selection of functional genetic elements that are related to immune function
- Continue to maintain unique genetic resources including selected lines, inbred, congenic and recombinant congenic lines as well as experimental lines.
- Develop additional genetic material (e.g. line crosses) as needs arise.
- Generate atlases of transcriptional responses under normal and disease conditions including those to viruses (e.g. MDV, IBV, AI, AIV, and IBDV) and bacteria (e.g. Salmonella, Campylobacter, and Clostridium) using RNA-Seq, microarrays, real-time PCR or other methods.
- Identify individual genes or quantitative trait loci (QTL) associated with disease resistance or immune response via next generation sequencing, microarray technology or emerging high throughput genotyping methods.
- Uncover and develop new single nucleotide polymorphisms (SNP) markers, microarrays, peptides, antisera, primer sets, and serum chemistry analyses to categorize immune responses in normal and disease states.
- Use genetic, environmental, dietary and immunostimulation methods to enhance protective immunity.
- Continue to identify new and characterize recently described immune response elements (e.g. cytokines, receptors, MHC molecules) and their involvement in resistance to disease.
- Use refereed publications, symposia, invited lectures and informal discussions at regional, national and international workshops and meetings to disseminate information to stakeholders and public
Outcomes or Projected Impacts
- Assess the impact of allelic variation on the response to specific pathogens By doing that we will be able to better understand immune responses elicited by chickens in response to a diverse range of pathogens and strategize on their prevention and control
- Promote poultry health By knowing and understanding processes and immunity elicited by avian species to diverse challenges (infectious and non infectious)
- Detection of elements that dictate the immune response in poultry Via molecular tool we will be able to detect these elements and maybe manipulate them to get the best of an immune response generating desired outcomes in terms of protection of the poultry population
- More, better and safer poultry products to feed a population in constant increase Our ultimate goal in this project is to producer more, better and safer poultry product in order to feed an increasing human population in the world
- Identify individual causative genes or quantitative trait loci will improve poultry health and animal agriculture in general through marker-assisted selection and breeding or technological applications.
- Development of new technologies to assess or improve the immune response.
- Appropriate immune responses and improved disease resistance through immune modulators will augment production efficiency.
Milestones
(2018):Better understand poultry immune responses to infectious and non infectious agents focusing on innate and adaptive responses using genetic stocks, molecular, serological and other techniques looking for resistance to diseases and conditions(2019):Using complex molecular tools we will be able to better understand immunological pathways stimulated after infectious and non infectious challenges.
(2020):Better understand factors and agents affecting poultry immune development, function, dysfunction, and pathology
(2022):Detect distinct poultry phenotypes and /or functional elements related with immune function
(2023):Advance knowledge regarding basic aspects of innate and adaptive immune system development and function.
(2023):Improve transgenic technology to more effectively elucidate biological functions of known and unknown genes in the immune system.
(2023):By 2023 we should be able to better understand the effects of MHC against certain diseases and use this info to breed better chicken populations in commercial and non commercial settings
Projected Participation
View Appendix E: ParticipationOutreach Plan
Industry stakeholders are invited to and frequently attend the annual project meetings. Their attendance provides an opportunity for information exchange. For example, representatives of breeder organizations can learn of the latest genetic advances in disease resistance from the project scientists. Technical Committee members gain knowledge of emerging field problems that the project can address through experiments. The combined efforts of the NE-1834 stations will generate new scientific data. Refereed publications, online data bases of genetic lines and genome/transcriptome information, symposia, invited lectures and informal discussions are some methods used to disseminate information. Project investigators have made significant scientific contributions to the improvement of poultry immune responses as well as the genetics of disease resistance. Cooperation among project members and with other researchers will remain a hallmark of NE-1834. This cooperative effort will include sharing scientific expertise and genetic resources held at numerous project stations. The addition of several international members has expanded the research scope and global dissemination of research findings.
Variation in DNA sequence can directly impact immune function, can modulate expression of genes that impact immune functions, and can serve as markers for other causative variants with which they are in linkage disequilibrium. This project will apply genetic, genomic and immunological approaches to assess the impact of allelic variation on response to specific pathogens or other immune stimuli. Several stations will focus on the major histocompatibility complex (MHC) region. Continuous communication and collaboration will be performed. Historically this communication is by direct collaboration of participants and / or by presentation and diffusion of the results at scientific meetings, including our annual meeting. This constant contact allows to strategize and plan in order to avoid research topics duplication.
There are numerous genetic, environmental, nutritional, physiological, management, and microbial factors that stimulate, regulate, and shape the immune response of poultry. The members of NE-1334 have been at the forefront of research to understand basic mechanisms and unique features of the avian immune response, to develop novel and effective means to promote poultry health and production. As an example of this fruitful collaboration, over the past 4 years the members of NE-1334 have produced over 250 peer reviewed publications, and awarded approximately 15 competitive NIFA grants worth over $6 million. The majority of these efforts are related to our understanding of factors and agents affecting poultry immune development, function, dysfunction, and pathology, and represent some of the most influential studies related to avian immunity over the past 5 years. As this highly successful project continues, the members of NE1834 will continue to use a variety of poultry systems, immunomodulatory approaches, and pathogens to expand our understanding of the avian immune response.
When paired with techniques such as next generation sequencing, SNP analysis, and qPCR, highly inbred chicken and turkey lines, sets of MHC-congenic lines, random breed lines and lines with distinct phenotypes allow the detection and selection of functional genetic elements that are related to immune function. Whad has made our project so succesful is the level of collaboration and interaction of our group. Even though the members of the group come from diverse poultry fields all their expertises are imprinted in the work we do. This high level of planning an coordination is due to the communication we have via meetings and collaborations.
Organization/Governance
The planning and supervision of the Multistate Research Project shall be the responsibility of the Multistate Technical Committee. The membership of this committee shall consist of an Administrative Advisor, which this time will be an active member of this project, a technical representative of each participating agency or experiment station, and a representative of the USDA National Institute of Food and Agriculture (NIFA). The voting membership shall consist of the Technical Committee Representatives. The Technical Committee shall be responsible for review and acceptance of contributing projects, preparation of reviews, modification of the multistate project proposal, and preparation of an annual report for transmittal by the Administrative Advisor upon approval to NIFA. Annual written reports will be prepared by each technical committee member and distributed at the annual meeting. A limited number of the compiled annual reports will be available upon request from the Administrative Advisor. The Technical Committee will meet yearly and elect a secretary, who will serve the year after election and as the chairperson the following year. An Executive Committee will be formed to conduct all business of the Technical Committee between annual meetings. The Executive Committee shall consist of the current Technical Committee Chairperson, the Secretary, and the two immediate Past Chairpersons. The chairperson may name other subcommittees as needed to perform specific assignments. They may include subcommittees to develop procedures, manuals, and phases of the multistate project, to review work assignments; to develop research methods, to prepare publications, and to write proposals. Other agencies and institutions may participate and vote at the invitation of the Administrative Advisor. Minimum expectations for Technical Committee members are submission of a written annual report every year, and attendance at an annual meeting including presentation of research results at least one year out of two. Collaborators may include emeritus members with an interest in attending annual meetings, scientists who wish to contribute by virtue of having special expertise or interest, and those who engage in research interactions with an individual Technical Committee member. Collaborators should submit a written report every year, and present their progress when attending the annual meeting. Guests who attend an annual meeting through special connection to the Technical Committee (i.e. host institution) are invited to make a brief presentation of their interests and ongoing research.
Literature Cited
237 total publications from NE-1334 Project participants 2013-2017
*= 33 cooperative publications among 2 or more project participants
*Abernathy, J., X. Li, X. Jia, W. Chou, S. J. Lamont, R. Crooijmans, and H. Zhou, H. 2014. Copy number variation in Fayoumi and Leghorn chickens analyzed using array comparative genomic hybridization. Anim. Genet. 45:400–411.
*Banat, G. R., S. Tkalcic, J. A. Dzielawa, M. W. Jackwood, M. D. Saggese, L. Yates, R. Kopulos, W. Briles, and E. W. Collisson. 2013. Association of the chicken MHC B haplotypes with resistance to avian coronavirus. Dev. Comp. Immunol. 39:430–437.
*Bauer, M. M., M. M. Miller, W. E. Briles, and K. M. Reed. 2013. Genetic variation at the MHC in a population of introduced wild turkeys. Animal biotechnology 24:210-228.
*Coble, D. J., E. E. Sandford, T. Ji, J. Abernathy, D. Fleming, H. Zhou, and S. J. Lamont. 2013. Impacts of Salmonella enteritidis infection on liver transcriptome in broilers. Genesis 51:357–364
*Da Silva A. P., Hauck R., H. Zhou, and R. A. Gallardo. 2017. Understanding immune resistance to infectious bronchitis using major histocompatibility complex chicken lines. Avian Dis. 61(3): 358-365.
*Dawes, M. E., L. M. Griggs, E. W. Collisson, W. E. Briles, and Y. Drechsler. 2014. Dramatic differences in the response of macrophages from B2 and B19 MHC-defined haplotypes to interferon gamma and polyinosinic:polycytidylic acid stimulation. Poultry Science 93:830–838.
*Deist M. S., R. A. Gallardo, D. A. Bunn, T. R. Kelly, J. C. M. Dekkers, H. Zhou, and S. J. Lamont. 2017. Novel mechanisms revealed in the trachea transcriptome of resistant and susceptible chicken lines following infection with Newcastle disease virus. Clin. Vacc. Immunol. (In Press) http://cvi.asm.org/content/24/5/e00027-17.short
*Deist M.S., R. A. Gallardo, D. A. Bunn, J. C. M. Dekkers, H. Zhou, and S. J. Lamont. 2017. Resistant and susceptible chicken lines show distinctive responses to Newcastle disease virus infection in the lung transcriptome. BMC Genomics. 18.989:DOI 10.1186/s12864-017-4380-4
*Deist, H., R. Gallardo, D. Bunn, T. Kelly, J. Dekkers, H. Zhou, and S. Lamont. 2017. Novel mechanisms revealed in the trachea transcriptome of resistant and susceptible chicken lines following infection with Newcastle disease virus. Clin Vaccine Immunol 24(5). pii: e00027-17. doi: 10.1128/CVI.00027-17.
*Deist, M. S., R. A. Gallardo, D. A. Bunn, T. R. Kelly, J. C. M. Dekkers, H. Zhou, and S. J. Lamont. Resistant and susceptible chicken lines show distinctive responses to Newcastle disease virus infection in the lung transcriptome. BMC Genomics. in press
*Deist, M. S., R. A. Gallardo, D. A. Bunn, T. R. Kelly, J. C. M. Dekkers, H. Zhou, and S. J. Lamont. 2017. Novel mechanisms revealed in the trachea transcriptome of resistant and susceptible chicken lines following infection with Newcastle disease virus. Clin. Vaccine Immunol. 24:e00027-17. doi:10.1128/CVI.00027-17.
*Drechsler, Y., S. Tkalcic, M. D. Saggese, H. L. Shivaprasad, D. K. Ajithdoss, and E. W. Collisson. 2013. A DNA Vaccine expressing env and gag offers partial protection against reticuloendotheliosis virus in the prairie chicken (Tympanicus cupido). Journal of Zoo and Wildlife Medicine 44:251–261.
*Dunn, J.R., S. M. Reddy, M. Niikura, V. Nair, J.E. Fulton and H.H. Cheng. 2017. Evaluation and identification of Marek’s disease virus BAC clones as standardized reagents for research. Avian Dis. 61:107-114.
*Fulton, J. E., J. Arango, R. A. Ali, E. B. Bohorquez, A. R. Lund, C. M. Ashwell, P. Settar, N. P. O'Sullivan, and M. D. Koci. 2014. Genetic variation within the Mx gene of commercially selected chicken lines reveals multiple haplotypes, recombination and a protein under selection pressure. PLoS One 9:e108054. doi 10.1371/journal.pone.0108054
*Fulton, J.E., A.M. McCarron, A.R. Lund, K.N. Pinegar, A. Wolc, O. Chazara, B. Bed’Hom, M. E. Berres and M.M. Miller, 2016. A high-density SNP panel reveals extensive diversity, frequent recombination and multiple recombination hotspots within the chicken major histocompatibility complex B region between BG2 and CD1A1. Genetics Sel. Evol. 48:1
*Fulton, J.E., M. E. Berres, J. Kantanen and M. Honkatukia. 2017. MHC-B variability within the Finnish Landrace chicken conservation program. Poult. Sci. in press
*Irizarry, K. J. L., E. Downs, R. Bryden, J. Clark, L. Griggs, R. Kopulos, C. M. Boettger, T. J. Carr, C. L. Keeler, E. Collisson, and Y. Drechsler. 2017. RNA sequencing demonstrates large-scale temporal dysregulation of gene expression in stimulated macrophages derived from MHC-defined chicken haplotypes. Plos One 12.
*Irizarry, K. J., A. Chan, D. Kettle, S. Kezian, D. Ma, L. Palacios, Q. Q. Li, C. L. Keeler, and Y. Drechsler. 2017. Bioinformatics analysis of chicken miRNAs associated with monocyte to macrophage differentiation and subsequent IFNγ stimulated activation. MicroRNA 6:53–70.
*Kim, D. K., H. S. Lillehoj, S. I. Jang, S. H. Lee, Y. H. Hong, and S. J. Lamont. 2015. Genetically disparate Fayoumi chicken lines show different response to avian necrotic enteritis. J. Poult. Sci. doi: 10.2141/jpsa.0140203
*Kogut, M. H., C. L. Swaggerty, H. I. Chiang, K. J. Genovese, H. He, H. Zhou, and R. J. Arsenault. 2014. Critical role of glycogen synthase kinase-3beta in regulating the avian heterophil response to Salmonella enterica Serovar Enteritidis. Front. Vet. Sci. 1:10.
*Kogut, M. H., C. L. Swaggerty, J. A. Byrd, R. Selvaraj, and R. J. Arsenault. 2016. Chicken-Specific Kinome Array Reveals that Salmonella enterica Serovar Enteritidis Modulates Host Immune Signaling Pathways in the Cecum to Establish a Persistence Infection. Int. J. Mol. Sci. 17:1207.
*Kogut, M., C. L. Swaggerty, H. I. Chiang, K. J. Genovese, H. He, H. Zhou, and R. Arsenault. 2014. Critical role of glycogen synthase kinase-3β in regulating the avian heterophil response to Salmonella enterica serovar Enteritidis. Front Vet Sci, doi:10.3389/fvets.2014.00010.
*Lee, M. O., E. Yang, M. Morisson, A. Vignal, Y.-Z. Huang, H. H. Cheng, W. M. Muir, S. J. Lamont, H. S. Lillehoj, S. H. Lee, and J. E. Womack. 2014. Mapping and genotypic analysis of the NK-lysin gene in chicken. Genet. Sel. Evol.46:43. doi: 10.1186/1297-9686-46-43
*Lee, S. H., X. Dong, H. S. Lillehoj, S. J. Lamont, X. Suo, D. K. Kim, K.-W. Lee, and Y. H. Hong. 2016. Comparing the immune responses of two genetically B-complex disparate Fayoumi chicken lines to Eimeria tenella. Brit. Poult. Sci. 57:165–171. doi:10.1080/00071668.2016.1141172
*Li, J., R. Li, Y. Wang, X. Hu, Y. Zhao, L. Li, C. Feng, X. Gu, F. Liang, S. J. Lamont, S. Hu, H. Zhou, and N. Li. 2015. Genome-wide DNA methylome variation in two genetically distinct chicken lines using MethylC-seq. BMC Genomics 16:851. doi: 10.1186/s12864-015-2098-8
*Miller, M. M., and R. L. Taylor, Jr. 2016. Brief review of the chicken major histocompatibility complex – the genes, their distribution on chromosome 16 and their contribution to disease resistance. Poult. Sci. 95:375-392 doi:10.3382/ps/pev379 (review)
*Miller, M., C. Robinson, J. Abernathy, R. M. Goto, M. Hamilton, H. Zhou, and M. Delany. 2014. Mapping genes to chicken microchromosome 16 and discovery of olfactory and scavenger receptor genes near the major histocompatibility complex. J Heredity 105(2):203-15. doi: 10.1093/jhered/est091.
*Nguyen-Phuc, H., J.E. Fulton, and M.E. Berres, 2016. Genetic variation of Major Histocompatibility Complex (MHC) in wild Red JungleFowl (Gallus gallus). Poultry Science 95:400-411.
*Schmid, M., J. Smith, D. W. Burt, B. L. Aken, P. B. Antin, A. L. Archibald, C. Ashwell, P. J. Blackshear, C. Boschiero, C. T. Brown, S. C. Burgess, H. H. Cheng, W. Chow, D. J. Coble, A. Cooksey, R. P. M. A. Crooijmans, J. Damas, R. V. N. Davis, D. J. de Koning, M. E. Delany, T. Derrien, T. T. Desta, I. C. Dunn, M. Dunn, H. Ellegren, L. Eöry, I. Erb, M. Farré, M. Fasold, D. Fleming, P. Flicek, K. E. Fowler, L. Frésard, D. P. Froman, V. Garceau, P. P. Gardner, A. A. Gheyas, D. K. Griffin, M. A. M. Groenen, T. Haaf, O. Hanotte, A. Hart, J. Häsler, S. B. Hedges, J. Hertel, K. Howe, A. Hubbard, D. A. Hume, P. Kaiser, D. Kedra, S. J. Kemp, C. Klopp, K. E. Kniel, R. Kuo, S. Lagarrigue, S. J. Lamont, D. M. Larkin, R. A. Lawal, S. M. Markland, F. McCarthy, H. A. McCormack, M. C. McPherson, A. Motegi, S. A. Muljo, A. Münsterberg, R. Nag, I. Nanda, M. Neuberger, A. Nitsche, C. Notredame, H. Noyes, R. O’Connor, E. A. O''Hare, A. J. Oler, S. C. Ommeh, H. Pais, M. Persia, F. Pitel, L. Preeyanon, P. Prieto Barja, E. M. Pritchett, D. D. Rhoads, C. M. Robinson, M. N. Romanov, M. Rothschild, P. F. Roux, C. J. Schmidt, A. S. Schneider, M. Schwartz, S. M. Searle, M. A. Skinner, C. A. Smith, P. F. Stadler, T. E. Steeves, C. Steinlein, L. Sun, M. Takata, I. Ulitsky, Q. Wang, Y. Wang, W. C. Warren, J. M. D. Wood, D. Wragg, and H. Zhou. Third Report on Chicken Genes and Chromosomes 2015. Cytogenet Genome Res. 2015. 145:79–180.
*Shanmugasundaram, R., M. H. Kogut, R. J. Arsenault, C. L. Swaggerty, K. Cole, J. M. Reddish, and R. K. Selvaraj. 2015. Effect of Salmonella infection on cecal tonsil regulatory T cell properties in chickens. Poult. Sci. 94:1828-1835.
*Wang, Y., B. Lupiani, S. Reddy, S. J. Lamont, and H. Zhou. 2014. RNA-seq analysis revealed novel genes and signaling pathway associated with disease resistance to avian influenza virus infection in chickens. Poult Sci 93(2):485-493. doi: 10.3382/ps.2013-03557.
*Wang, Y., P. Saelao, K. Chanthavixay, R. A. Gallardo, D. Bunn, S. Lamont, J. Dekkers, T. Kelly, and H. Zhou. 2017. Physiological responses to heat stress in two genetically distinct chicken inbred lines. Poult. Sci. in press
*Wolc, A., H.H. Zhao, J. Arango, P. Settar, J.E. Fulton, N.P. O’Sullivan, R. Preisinger, C. Stricker, D. Habier, R.L Fernando, S.H. Lamont, J.C.M. Dekkers, 2015. Response and inbreeding from a genomic selection experiment in layer chickens. Genetic Selection Evolution 47: 59
*Zhang, J., M. Kaiser, M. Deist, R. A. Gallardo, D. Bunn, T. Kelly, J. Dekkers, H. Zhou, and S. J. Lamont. 2017. Transcriptome analysis in spleen reveals differential regulation of response to Newcastle disease virus in two chicken lines. Sci. Rep. in press
204 publications from individual project participants
Abernathy, J., C. Corkill, C. Hinojosa, X. Li, and H. Zhou. 2013. Deletions in the pyruvate pathway of Salmonella Typhimurium alter SPI1-mediated gene expression and infectivity. J. Anim. Sci. Biotechnol. 4:5. doi: 10.1186/2049-1891-4-5.
Abraham, M., L. R. McDougald, and R. B. Beckstead. 2014. Blackhead disease: reduced sensitivity of Histomonas meleagridis to nitarsone in vitro and in vivo. Avian Dis. 58:60-63
Adikari, A. M. J. B., J. Xu, S. Casterlow, H. Li, E. R. Gilbert, A. P. McElroy, D. A. Emmerson, R. A. Dalloul, E. A. Wong, and E. J. Smith. 2017. Haplotype structure and DNA sequence variation of the liver expressed antimicrobial peptide-2 (chLEAP-2) gene in chickens challenged with Eimeria maxima. Int. J. Poult. Sci. 16:336-343. DOI: 10.3923/ijps.2017
Ahrens, B. J., L. Li, A. K. Ciminera, J. Chea, E. Poku, J. R. Bading, M. R. Weist, M. M. Miller, D. M. Colcher, and J. E. Shively. 2017. Diagnostic PET Imaging of Mammary Microcalcifications Using 64Cu-DOTA-Alendronate in a Rat Model of Breast Cancer. J. Nuclear Med. 58:1373-1379.
Allali, I., J. W. Arnold, J. Roach, M. B. Cadenas, N. Butz, H. M. Hassan, M. Koci, A. Ballou, M. Mendoza, R. Ali, and M. A. Azcarate-Peril. 2017. A comparison of sequencing platforms and bioinformatics pipelines for compositional analysis of the gut microbiome. BMC Microbiol 17:194. doi 10.1186/s12866-017-1101-8
Anderson, J. L., C. M. Ashwell, S. C. Smith, R. Shine, E. C. Smith, and R. L. Taylor, Jr. 2013. Atherosclerosis-susceptible and atherosclerosis-resistant pigeon aortic cells express different genes in vivo. Poult. Sci. 92:2668-2680 doi: 10.3382/ps.2013-03306
Anderson, J. L., M. C. Keeley, S. C. Smith, E. C. Smith, and R. L. Taylor, Jr. 2014. Rosiglitizone modulates pigeon atherosclerotic lipid accumulation and gene expression in vitro. Poult. Sci. 93:1368-1374 doi: 10.3382/ps.2013-03840
Anderson, J. L., S. C. Smith and R. L. Taylor, Jr. 2013. Atherosclerosis-susceptible and atherosclerosis-resistant pigeon aortic smooth muscle cells express different genes and proteins in vitro. In: Current Trends in Atherogenesis. R. Rezzani, (ed.) InTech, Inc., Rijeka, Croatia (review) pp. 165-186 accessed February 27, 2013 doi: 10.5772/52948 http://www.intechopen.com/articles/show/title/atherosclerosis-susceptible-and-atherosclerosis-resistant-pigeon-aortic-smooth-muscle-cells-express-
Anderson, J. L., S. C. Smith and R. L. Taylor, Jr. 2014. The pigeon (Columba livia) model of spontaneous atherosclerosis. (review) Poult. Sci. 93:2691-2699 doi: 10.3382/ps.2014-0428
Andersson, L., A. L. Archibald, C. D. Bottema, R. Brauning, S. C. Burgess, D. W. Burt, E. Casas, H. H. Cheng, L. Clarke, C. Couldrey, B. P. Dalrymple, C. G. Elsik, S. Foissac, E. Giuffra, M. A. Groenen, B. J. Hayes, L. S. Huang, H. Khatib, J. W. Kijas, H. Kim, J. K. Lunney, F. M. McCarthy, J. C. McEwan, S. Moore, B. Nanduri, C. Notredame, Y. Palti, G. S. Plastow, J. M. Reecy, G. A. Rohrer, E. Sarropoulou, C. J. Schmidt, J. Silverstein, R. L. Tellam, M. Tixier-Boichard, G.Tosser-Klopp, C. K. Tuggle, J. Vilkki, S. N. White, S. Zhao, and H. Zhou. The FAANG Consortium. 2015. Coordinated international action to accelerate genome-to-phenome with FAANG, the Functional Annotation of Animal Genomes project. Genome Biol 16:57. doi: 10.1186/s13059-015-0622-4
Arsenault, R. J., and M. H. Kogut. 2015. Immunometabolism and the kinome peptide array: a new perspective and tool for the study of gut health. Front. Vet. Sci. Oct 13;2:44. doi: 10.3389/fvets.2015.00044
Arsenault, R. J., B. Trost, and M. H. Kogut. 2014. A comparison of the chicken and turkey proteomes and phosphoproteomes in the development of poultry-specific immuno-metabolism kinome peptide arrays. Front. Vet. Sci. Nov 13;1:22. doi: 10.3389/fvets.2014.00022.
Arsenault, R. J., J. T. Lee, R. Latham, B. Carter, and M. H. Kogut. 2017. Changes in immune and metabolic gut response in broilers fed β-mannanase in β-mannan-containing diets. Poult. Sci. Sep 14. doi: 10.3382/ps/pex246.
Arsenault, R. J., K. J. Genovese, H. He, H. Wu, A. S. Neish, and M. H. Kogut. 2016. Wild-type and mutant AvrA−Salmonella induce broadly similar immune pathways in the chicken ceca with key differences in signaling intermediates and inflammation. Poult. Sci. 95:354–363.
Arsenault, R. J., M. H. Kogut, and H. He. 2013. Combined CpG and poly I:C stimulation of monocytes results in unique signaling activation not observed with the individual ligands. Cell. Signal. 25:2246–2254.
Arsenault, R. J., P. Maattanen, J. Daigle, A. Potter, P. Griebel, and S. Napper. 2014a. From mouth to macrophage: mechanisms of innate immune subversion by Mycobacterium avium subsp. paratuberculosis. Vet. Res. 45:54.
Arsenault, R. J., S. Napper, and M. H. Kogut. 2013c. Salmonella enterica typhimurium infection causes metabolic changes in chicken muscle involving AMPK, fatty acid and insulin/mTOR signaling. Vet. Res. 44:35.
Arsenault, R. J., Y. Li, P. Maattanen, E. Scruten, K. Doig, A. Potter, P. Griebel, A. Kusalik, and S. Napper. 2013b. Altered Toll-Like Receptor 9 Signaling in Mycobacterium avium subsp. paratuberculosis-Infected Bovine Monocytes Reveals Potential Therapeutic Targets. Infect. Immun. 81:226–237.
Arsenault, R., and M. H. Kogut. 2013. Chicken-specific peptide arrays for kinome analysis: Flight for the flightless. Research Gate 7:79–89.
Ballou, A. L., R. A. Ali, M. A. Mendoza, J. C. Ellis, H. M. Hassan, W. J. Croom, and M. D. Koci. 2016. Development of the Chick Microbiome: How Early Exposure Influences Future Microbial Diversity. Front Vet Sci 3:2. doi 10.3389/fvets.2016.00002
Barrios, M. A., A. Kenyon, and R. B. Beckstead. 2017. Development of a dry medium for isolation of Histomonas meleagridis in the field. Avian Dis. 61:242-244
Bickhart, D. M., L. Xu, J. L. Hutchison, J. B. Cole, D. J. Null, S. G. Schroeder, J. Song, J. F. Garcia, T. S. Sonstegard, C. P. Van Tassell, R. D. Schnabel, J. F. Taylor, H. A. Lewin, and G. E. Liu. 2016. Diversity and population-genetic properties of copy number variations and multicopy genes in cattle. DNA Res 23:253-262. doi 10.1093/dnares/dsw013
Boschiero, C., A.A. Gheyas, H.K. Ralph, L. Eory, B. Paton, R. Kuo, J. Fulton, R. Preisinger, P. Kaiser, and D.W. Burt, 2015. Detection and characterization of small insertion and deletion genetic variants in modern layer chicken genomes. BMC Genomics 16:562.
Burks, T. A. and R. L. Taylor, Jr. 2014. Genetic control of Rous sarcoma virus-induced tumor growth in chickens: Role of the major histocompatibility (B) complex. Animal Science Image Gallery. http://animalimagegallery.org/search.php #5178
Carrillo, J. A., Y. He, J. Luo, K. R. Menendez, N. L. Tablante, K. Zhao, J. N. Paulson, B. Li, and J. Song. 2015. Methylome Analysis in Chickens Immunized with Infectious Laryngotracheitis Vaccine. PLoS One 10:e0100476. doi 10.1371/journal.pone.0100476
Carrillo, J. A., Y. He, Y. Li, J. Liu, R. A. Erdman, T. S. Sonstegard, and J. Song. 2016. Integrated metabolomic and transcriptome analyses reveal finishing forage affects metabolic pathways related to beef quality and animal welfare. Sci Rep 6:25948. doi 10.1038/srep25948
Chang, S., Q. Xie, J. R. Dunn, C. W. Ernst, J. Song, and H. Zhang. 2014. Host genetic resistance to Marek's disease sustains protective efficacy of herpesvirus of turkey in both experimental and commercial lines of chickens. Vaccine 32:1820-1827. doi 10.1016/j.vaccine.2014.01.092
Chen, H., Q. Zuo, Y. Wang, J. Song, H. Yang, Y. Zhang, and B. Li. 2017. Inducing goat pluripotent stem cells with four transcription factor mRNAs that activate endogenous promoters. BMC Biotechnol 17:11. doi 10.1186/s12896-017-0336-7
Chen, H., Q. Zuo, Y. Wang, M. F. Ahmed, K. Jin, J. Song, Y. Zhang, and B. Li. 2017. Regulation of Hedgehog Signaling in Chicken Embryonic Stem Cells Differentiation Into Male Germ Cells (Gallus). J Cell Biochem 118:1379-1386. doi 10.1002/jcb.25796
Chen, Y., J. Stookey, R. Arsenault, E. Scruten, P. Griebel, and S. Napper. 2016. Investigation of the physiological, behavioral, and biochemical responses of cattle to restraint stress. J. Anim. Sci. 94:3240–3254.
Chen, Y., R. Arsenault, S. Napper, and P. Griebel. 2015. Models and Methods to Investigate Acute Stress Responses in Cattle. Animals 5:1268–1295.
Cheng, H. H., P. Kaiser, and S. J. Lamont. 2013. Integrated genomic approaches to enhance genetic resistance in chickens. Annu. Rev. Anim. Biosci.1:239–260
Chou, W.-K., C.-H. Chen, C. N. Vuong, D. Abi-Ghanem, S. D. Waghela, W. Mwangi, L. R. Bielke, B. M. Hargis, and L. R. Berghman. 2016. Significant mucosal sIgA production after a single oral or parenteral administration using in vivo CD40 targeting in the chicken. Res. Vet. Sci. 108:112–115.
Clarke, L. L, R. B. Beckstead, J. R. Hayes, and D. R. Rissi. 2017. Pathologic and molecular characterization of histomoniasis in peafowl (Pavo cristatus). J. Vet. Diagn. Invest. 29:237-241
Collisson, E., L. Griggs, and Y. Drechsler. 2017. Macrophages from disease resistant B2 haplotype chickens activate T lymphocytes more effectively than macrophages from disease susceptible B19 birds. Developmental & Comparative Immunology 67:249–256.
Cooper, J., Y. Ding, J. Song, and K. Zhao. 2017. Genome-wide mapping of DNase I hypersensitive sites in rare cell populations using single-cell DNase sequencing. Nat Protoc 12:2342-2354. doi 10.1038/nprot.2017.099
Cox, C. M., and R. A. Dalloul. 2015. Immunomodulatory role of probiotics in poultry and potential in ovo application. Benef. Microbes 6:45-52. DOI: 10.3920/BM2014.0062
Daigle, J., B. Van Wyk, B. Trost, E. Scruten, R. Arsenault, A. Kusalik, P. J. Griebel, and S. Napper. 2014. Peptide arrays for kinome analysis of livestock species. Vet. Infect. Dis. 1:4.
Dalloul, R. A., A. V. Zimin, R. E. Settlage, S. Kim, and K. M. Reed. 2014. Next generation sequencing strategies for characterizing the turkey genome. Poult. Sci. 93:479-484. DOI: 10.3382/ps.2013-03560
Delany, M.E. and T.H. O’Hare. 2013. Genetic stocks for immunological research (Appendix I). In Avian Immunology, 2nd Edition (editors: K.A. Schat, B. Kaspers and P. Kaiser). Elsevier: Academic Press, San Diego, CA. ISBN 9780123969651. 456 pp.
Derksen T. J., Lampron R., Hauck R., M. Pitesky, and R. A. Gallardo. 2017. Biosecurity assessment and seroprevalence of respiratory diseases in backyard poultry flocks located close and far from commercial premises. Avian Dis. (In press).
Drobik-Czwaro, W., A. Wolc, J.E. Fulton, J. Arango, T. Jankowski, N.P. O’Sullivan and J.C.M. Dekkers, 2017. Identifying the genetic basis for resistance to avian influenza in commercial egg layer chickens. Animal. doi: 10.1017/S1751731117002889
Du, J., Z. Yuan, Z. Ma, J. Song, X. Xie, and Y. Chen. 2014. KEGG-PATH: Kyoto encyclopedia of genes and genomes-based pathway analysis using a path analysis model. Molecular Biosystems 10:2441-2447. doi 10.1039/c4mb00287c
Erf, G. F., and I. R. Ramachandran. 2016. The growing feather as a dermal test-site: comparison of leukocyte profiles during the response to Mycobacterium butyricum in growing feathers, wattles, and wing webs. Poult. Sci.: 95:2011-2022.
Erf, G. F., D. M. Falcon, K. A. Sullivan, and S.E. Bourdo. 2017. T lymphocytes dominate local leukocyte infiltration in response to intradermal injection of functionalized graphene-based nanomaterial. J. Applied Toxicol. 37:1317-1324.
Erf, G. F., H. R. Kong, K. A. Byrne, D. M. Falcon, and Z. Aguilar. 2017. Novel approach to simultaneously assess and monitor an individual’s humoral and cellular immune responses in the chicken model. PLOS ONE (submitted; PONE-D-17-42058).
Evans, N. P., D. Collins, F. W. Pierson, H. M. Mahsoub, N. Sriranganathan, M. E. Persia, T. P. Karnezos, M. D. Sims, and R. A. Dalloul. 2017. Investigation of medium chain fatty acid feed supplementation for reducing Salmonella Typhimurium colonization in turkey poults. Foodborne Path. Dis. 14:531-536. DOI: 10.1089/fpd.2016.2273
Figueroa A., R. Hauck, J. Saldias-Rodriguez, and R. A. Gallardo. 2017. Combination of quaternary ammonia and glutaraldehyde as a disinfectant against enveloped and non-enveloped viruses. J. Appl. Poult. Res. 26(4): 491-497.
Fulton, J. E., 2014. The value of resequencing data for poultry breeding: A primary layer breeder perspective. Poultry Science 93:494-497.
Fulton, J.E., A.R. Lund, A.M. McCarron, K.N. Pinegar, D.R. Korver, H.L. Classen, S. Aggrey, C. Utterbach, N.B. Anthony and M.E. Berres, 2016. MHC variability in heritage breeds of chickens. Poultry Science 95:393-399.
Fulton, J.E., J. Arango, J.A. Arthur, P.Settar, K.S. Kreager, and N.P. O’Sullivan, 2013. Improving the outcome of a Marek’s disease challenge in multiple lines of egg type chickens. Avian Dis. 57:395-400.
Gadde, U., T. Rathinam, G. F. Erf, and H. D. Chapman. 2013. Acquisition of immunity to the protozoan parasite Eimeria adenoeides in turkey poults and cellular responses to infection. Poult. Sci. 92:3149-3157.
Gallardo, R. A., R. Carrasco-Medanic, H. Zhou, S. Lyu, Y. Wang, P. R. Woodcock, and F. J. Hoerr. 2014. Effects of very virulent infectious bursal disease virus reassortants challenge in commercial chickens. Avian Dis 58:579-586.
Galvan, S. C., A. Garcia Carranca, J. Song, and F. Recillas-Targa. 2015. Epigenetics and animal virus infections. Front Genet 6:48. doi 10.3389/fgene.2015.00048
Genovese, K. J., H. He, C. L. Swaggerty, and M. H. Kogut. 2013. The avian heterophil. Dev. Comp. Immunol. 41:334-340.
Grenier, B., I. Dohnal, R. Shanmugasundaram, S. D. Eicher, R. K. Selvaraj, G. Schatzmayr, and T. J. Applegate. 2016. Susceptibility of Broiler Chickens to Coccidiosis When Fed Subclinical Doses of Deoxynivalenol and Fumonisins-Special Emphasis on the Immunological Response and the Mycotoxin Interaction. Toxins (Basel) 8.
Hamad, S. K., S. Kim, S. W. El-Kadi, E. A. Wong, and R. A. Dalloul. 2017. Comparative expression of host defense peptides in turkey poults. Poult. Sci. 96:2083-2090. DOI: 10.3382/ps/pew500
Han, B., L. Lian, X. Li, C. Zhao, L. Qu, C. Liu, J. Song, and N. Yang. 2016a. Chicken gga-miR-103-3p Targets CCNE1 and TFDP2 and Inhibits MDCC-MSB1 Cell Migration. G3 (Bethesda) 6:1277-1285. doi 10.1534/g3.116.028498
Han, B., L. Lian, X. Li, C. Zhao, L. Qu, C. Liu, J. Song, and N. Yang. 2016b. Chicken gga-miR-130a targets HOXA3 and MDFIC and inhibits Marek's disease lymphoma cell proliferation and migration. Mol Biol Rep 43:667-676. doi 10.1007/s11033-016-4002-2
Han, B., Y. He, L. Zhang, Y. Ding, L. Lian, C. Zhao, J. Song, and N. Yang. 2017. Long intergenic non-coding RNA GALMD3 in chicken Marek's disease. Sci Rep 7:10294. doi 10.1038/s41598-017-10900-2
Hashim, M. M., R. J. Arsenault, J. A. Byrd, M. H. Kogut, M. Al-Ajeeli, and C. A. Bailey. Influence of different yeast cell wall preparations and their components on performance and immune and metabolic pathways in Clostridium perfringens-challenged broiler chicks. Poult. Sci.
Hauck R., B. Crossley, D. Rejmanek, H. Zhou, and R. A. Gallardo. 2017. Persistence of high and low pathogenic avian influenza viruses in footbaths and poultry manure. Avian Dis. 61:64-69.
Hauck R., C. G. Sentíes-Cué, Y. Wang, C. Kern, H. L. Shivaprasad, H. Zhou, and R. A. Gallardo. 2017. Evolution of avian encephalomyelitis virus during embryo adaptation. Vet. Microbiol. 204:1-7.
Hauck, R., C. G. Sentíes-Cué, Y. Wang, C. Kern, H. L. Shivaprasad, H. Zhou, and R. A. Gallardo. 2017. Evolution of avian encephalomyelitis virus during embryo-adaptation. Vet Microbiol 204:1-7. doi: 10.1016/j.vetmic.2017.04.005. Epub 2017 Apr 9.
Hauck, R., D. Crossley, D. Rejmanek, H. Zhou, and R. A. Gallardo. 2017. Persistence of high and low pathogenic avian influenza viruses in footbaths and poultry manure. Avian Dis 61:64-69.
He, H., K. J. Genovese, C. L. Swaggerty, D. J. Nisbet, and M. H. Kogut. 2013. Nitric oxide as a biomarker of intracellular Salmonella viability and identification of the bacteriostatic activity of protein kinase A inhibitor H-89. PLoS One 8:e58873.
He, Y. H., S. Y. Pu, F. H. Xiao, X. Q. Chen, D. J. Yan, Y. W. Liu, R. Lin, X. P. Liao, Q. Yu, L. Q. Yang, X. L. Yang, M. X. Ge, Y. Li, J. J. Jiang, W. W. Cai, and Q. P. Kong. 2016. Improved lipids, diastolic pressure and kidney function are potential contributors to familial longevity: a study on 60 Chinese centenarian families. Sci Rep 6:21962. doi 10.1038/srep21962
He, Y., M. Song, Y. Zhang, X. Li, J. Song, Y. Zhang, and Y. Yu. 2016. Whole-genome regulation analysis of histone H3 lysin 27 trimethylation in subclinical mastitis cows infected by Staphylococcus aureus. BMC Genomics 17:565. doi 10.1186/s12864-016-2947-0
He, Y., Y. Ding, F. Zhan, H. Zhang, B. Han, G. Hu, K. Zhao, N. Yang, Y. Yu, L. Mao, and J. Song. 2016. Corrigendum: The conservation and signatures of lincRNAs in Marek's disease of chicken. Sci Rep 6:19422. doi 10.1038/srep19422
Heidaritabar, M., A. Wolc, J. Arango, J. Zeng, P.Settar, J.E. Fulton, N.P.O’Sullivan, J.W.M, Bastiaansen, R.L. Fernando, D.J. Garrick, JCM. Dekkers, 2016. Impact of fitting dominance and additive effects on accuracy of genomic prediction of breeding values in layers. J. Anim. Breed. Genet. 5:334-346.
Hughes, R. A., R. A. Ali, M. A. Mendoza, H. M. Hassan, and M. D. Koci. 2017. Impact of dietary galacto-oligosaccharide (GOS) on chicken's gut microbiota, mucosal gene expression, and Salmonella colonization. Front Vet Sci 4:192. doi 10.3389/fvets.2017.00192
Jang, H-M, G. F. Erf, K. C. Rowland, and B.-W Kong. 2014. Genome resequencing and bioinformatics analysis of SNP containing candidate genes in the autoimmune vitiligo Smyth line chicken model. BMC Genomics, 15:707.
Jesudhasan, P. R., J. L. McReynolds, A. J. Byrd, H. He, K. J. Genovese, R. Drolesky, C. L. Swaggerty, M. H. Kogut, D. J. Nisbet, C. Praveen, and S. D. Pillai. 2015. Electron-beam-inactivated vaccine against Salmonella Enteritidis colonization in molting hens. Avian Dis. 59:165-170.
Jia, X., Q. Nie, X. Zhang, L. K. Nolan, and S. J. Lamont. 2017. Novel miRNA involved in host response to avian pathogenic Escherichia coli identified by deep sequencing and integration analysis. Infect. Immun. 85:e00688-16. doi.org/10.1128/IAI.00688-16
Jie, H., L. Lian, L. J. Qu, J. X. Zheng, Z. C. Hou, G. Y. Xu, J. Z. Song, and N. Yang. 2013. Differential expression of Toll-like receptor genes in lymphoid tissues between Marek's disease virus-infected and noninfected chickens. Poultry Science 92:645-654. doi 10.3382/ps.2012-02747
Jordan, B. J., S. Vogel, M. R. Stark, and R. B. Beckstead. 2014. Expression of green fluorescent protein in the chicken using in vivo transfection of the piggyBac transposon. J Biotechnol. 10:86-89
Kim, S., C. M. Cox, M. C. Jenkins, R. H. Fetterer, K. B. Miska, and R. A. Dalloul. 2014. Both host and parasite MIF molecules bind to chicken macrophages via CD74 surface receptor. Dev. Comp. Immunol. 47:319-326. DOI: 10.1016/j.dci.2014.07.021
Kim, S., M. Park, A. E. Leon, J. S. Adelman, D. M. Hawley, and R. A. Dalloul. 2017. Development and validation of a house finch interleukin-1 beta (HfIL-1β) ELISA system. BMC Vet. Res. 13:276. DOI: 10.1186/s12917-017-1199-9
Kim, T.H., and H. Zhou. 2015. Functional analysis of chicken IRF7 in response to dsRNA analog Poly(I:C) by integrating overexpression and knockdown: PLoS One 10.1371/journal.pone.0133450
Kindrachuk, J., V. Wahl-Jensen, D. Safronetz, B. Trost, T. Hoenen, R. Arsenault, F. Feldmann, D. Traynor, E. Postnikova, A. Kusalik, S. Napper, J. E. Blaney, H. Feldmann, and P. B. Jahrling. 2014. Ebola virus modulates transforming growth factor β signaling and cellular markers of mesenchyme-like transition in hepatocytes. J. Virol. 88:9877–9892.
Kjaerup, R. M., T. S. Dalgaard, L. R. Norup, R. M. Goto, M. M. Miller, P. Sorensen, and H. R. Juul-Madsen. 2014. Transcription efficiency of different chicken mannose-binding lectin promoter alleles. Immunogenetics 66:737-742.
Kogut, M. H., and R. J. Arsenault. 2015. A Role for the Non-Canonical Wnt-β-Catenin and TGF-β Signaling Pathways in the Induction of Tolerance during the Establishment of a Salmonella enterica Serovar Enteritidis Persistent Cecal Infection in Chickens. Front. Vet. Sci. 2
Kogut, M. H., and R. J. Arsenault. 2017. Immunometabolic Phenotype Alterations Associated with the Induction of Disease Tolerance and Persistent Asymptomatic Infection of Salmonella in the Chicken Intestine. Front. Immunol. 8
Kogut, M. H., K. J. Genovese, H. He, and R. J. Arsenault. 2016a. AMPK and mTOR: sensors and regulators of immunometabolic changes during Salmonella infection in the chicken. Poult. Sci. 95:345–353.
Kogut, M. H., K. J. Genovese, H. He, C. L. Swaggerty, and Y. Jiang. 2013. Modulation of chicken intestinal immune gene expression by small cationic peptides as feed additives during the first week post-hatch. Clin. Vac. Immunol. 20:1440-1448.
Kranis, A., A.M Gheyas, C. Boschiero, F. Turner, L. Yu, S. Smith, R. Talbot, A. Pirani, F. Brew, P. Kaiser, P.M Hocking, M. Fife, N. Salmon, J. Fulton, T. M. Strom, G. Haberer, S. Weigend, R. Preisinger, M. Gholami, S. Qanbari, H. Simianer, K. A. Watson, J. A Wooliams and D.W Burt. 2013. Development of a high density 600K SNP genotyping array for chicken. BMC Genomics, 14:59
Krishnamoorthy, A. S., C. D. Smith, A. A. Al-Rubaye, G. F. Erf, R. F. Wideman, N. B. Anthony, and D. D. Rhoads. 2014. A quantitative trait locus for ascites on chromosome 9 in broiler chicken lines. Poult Sci. 93:307-317.
Kropp, J., J. A. Carrillo, H. Namous, A. Daniels, S. M. Salih, J. Song, and H. Khatib. 2017. Male fertility status is associated with DNA methylation signatures in sperm and transcriptomic profiles of bovine preimplantation embryos. BMC Genomics 18:280. doi 10.1186/s12864-017-3673-y
Kuttappan, V. A., O. T. Faulkner, A. Wolfenden, G. Tellez, B. M. Hargis, and L. R. Bielke. 2013. Novel recombinant food safety vaccines against Salmonella and Campylobacter in broiler chickens. Int. J. Biotechnol. Bioeng. Res. 4:589–596.
Lai, F. N., H. L. Zhai, M. Cheng, J. Y. Ma, S. F. Cheng, W. Ge, G. L. Zhang, J. J. Wang, R. Q. Zhang, X. Wang, L. J. Min, J. Z. Song, and W. Shen. 2016. Whole-genome scanning for the litter size trait associated genes and SNPs under selection in dairy goat (Capra hircus). Sci Rep 6:38096. doi 10.1038/srep38096
Lan, X., Y. Wang, K. Tian, F. Ye, H.-D. Yin, X.-L. Zhao, H.-Y. Xu, Y. Huang, H. Liu, J. Hsieh, S. Lamont, and Q. Zhu. 2017. Integrated host and viral transcriptome analyses reveal pathology and inflammatory response mechanisms to ALV-J injection in SPF chickens. Sci. Reports 7:46156. doi: 10.1038/srep46156
Lee, M. O., H.-J. Jang, D. Rengaraj, S.-Y. Yang, J. Y. Han, S. J. Lamont, and J. E. Womack. 2016. Tissue expression and antibacterial activity of host defense peptides in chicken. BMC Vet Res 12:231. doi: 10.1186/s12917-016-0866-6
Lee, M. O., L. Andersson, S. J. Lamont, J. Chen, and J. E. Womack. 2016. Duplication of defensin7 gene in Fayoumi chickens generated by gene conversion and homologous recombination. PNAS 113:13815–13820. doi: 10.1073/pnas.1616948113
Li, D., Y. Ji, F. Wang, Y. Wang, M. Wang, C. Zhang, W. Zhang, Z. Lu, C. Sun, M. F. Ahmed, N. He, K. Jin, S. Cheng, Y. Wang, Y. He, J. Song, Y. Zhang, and B. Li. 2017. Regulation of crucial lncRNAs in differentiation of chicken embryonic stem cells to spermatogonia stem cells. Anim Genet 48:191-204. doi 10.1111/age.12510
Li, Y., J. A. Carrillo, Y. Ding, Y. He, C. Zhao, L. Zan, and J. Song. 2015. Ruminal Transcriptomic Analysis of Grass-Fed and Grain-Fed Angus Beef Cattle (vol 10, e0116437, 2015). Plos One 10. doi 10.1371/journal.pone.0134067
Lu, T., A. F. Harper, J. Zhao, and R. A. Dalloul. 2014. Effects of a dietary antioxidant blend and vitamin E on growth performance, oxidative status, and meat quality in broiler chickens fed a diet high in oxidants. Poult. Sci. 93:1649-1657. DOI: 10.3382/ps.2013-03826
Lu, T., A. F. Harper, J. Zhao, B. A. Corl, T. LeRoith, and R. A. Dalloul. 2014. Effects of a dietary antioxidant blend and vitamin E on fatty acid profile, liver function and inflammatory response in broiler chickens fed a diet high in oxidants. Poult. Sci. 93:1658-1666. DOI: 10.3382/ps.2013-03827
Lu, Y., F. D. West, B. J. Jordan, E. T. Jordan, R. C. West, P. Yu, Y. He, M. A. Barrios, Z, Zhu, J. N. Petitte, R. B. Beckstead, and S. L. Stice. 2014. Induced pluripotency in chicken embryonic fibroblast results in a germ cell fate. Stem Cells Dev. 23:1755-1764
Luo, J., J. A. Carrillo, K. R. Menendez, N. L. Tablante, and J. Song. 2014. Transcriptome analysis reveals an activation of major histocompatibility complex 1 and 2 pathways in chicken trachea immunized with infectious laryngotracheitis virus vaccine. Poultry Science 93:848-855. doi 10.3382/ps.2013-03624
Luo, J., S. Chang, H. Zhang, B. Li, and J. Song. 2013a. DNA Methylation Down-Regulates EGFR Expression in Chickens. Avian Diseases 57:366-371.
Luo, J., Y. Yu, A. Mitra, S. Chang, H. Zhang, G. Liu, N. Yang, and J. Song. 2013b. Genome-Wide Copy Number Variant Analysis in Inbred Chickens Lines With Different Susceptibility to Marek's Disease. G3-Genes Genomes Genetics 3:217-223. doi 10.1534/g3.112.005132
Luoma, A., A. Markazi, R. Shanmugasundaram, G. R. Murugesan, M. Mohnl, and R. Selvaraj. 2017. Effect of synbiotic supplementation on layer production and cecal Salmonella load during a Salmonella challenge. Poult Sci. doi: 10.3382/ps/pex251.
Ma, M. Z., R. Lin, J. Carrillo, M. Bhutani, A. Pathak, H. Ren, Y. Li, J. Song, and L. Mao. 2015. DNMT3B4-del Contributes to Aberrant DNA Methylation Patterns in Lung Tumorigenesis. EBioMedicine 2:1340-1350. doi 10.1016/j.ebiom.2015.09.002
Markazi, A. D., V. Perez, M. Sifri, R. Shanmugasundaram, and R. K. Selvaraj. 2017. Effect of whole yeast cell product supplementation (CitriStim(R)) on immune responses and cecal microflora species in pullet and layer chickens during an experimental coccidial challenge. Poult. Sci. 96:2049-2056.
Mason, A.S., J.E. Fulton, P.M Hocking and D.W. Burt, 2016. A new look at the LTR retrotansposon content of the chicken genome. BMC Genomics 17:688. doi: 10.1186/s12864-016-3043-1
McPherson, M.C., and M.E. Delany. 2016. Virus and host genomic, molecular and cellular interactions during Marek’s disease pathogenesis and oncogenesis. Poultry Science 95:412-429. http://ps.oxfordjournals.org/content/early/2016/01/14/ps.pev369.full.pdf?papetoc
McPherson, M.C., H.H Cheng, and M.E. Delany. 2016. Marek’s disease herpesvirus vaccines integrate into chicken host chromosomes yet lack a virus-host phenotype associated with oncogenic transformation. Vaccine 34:5554-5561 http://dx.doi.org/10.1016/j.vaccine.2016.09.051 (Highlighted article November 2016: http://www.journals.elsevier.com/vaccine/highlighted-articles/highlighted-article-november-2016
McPherson, M.C., L. Gehlen, C.M. Robinson, and M. E. Delany. 2014. Comparative cytogenomics of poultry: mapping of single genes and repeat loci in the Japanese quail (Coturnix japonica) Chromosome Res. 22:71-83. doi:10.1007/s10577-014-9411-2 http://link.springer.com/article/10.1007/s10577-014-9411-2
Meliopoulos, V. A., S. A. Marvin, P. Freiden, L. A. Moser, P. Nighot, R. Ali, A. Blikslager, M. Reddivari, R. J. Heath, M. D. Koci, and S. Schultz-Cherry. 2016. Oral Administration of Astrovirus Capsid Protein Is Sufficient To Induce Acute Diarrhea In Vivo. MBio 7. doi 10.1128/mBio.01494-16
Menconi, A., L. R. Bielke, B. M. Hargis, and G. Tellez. 2014. Immuno-modulation and anti-inflammatory effects of antibiotic growth promoters versus probiotics in the intestinal tract. J. Microbiol. Res. Rev. 2: 62-67.
Mitra, A., J. Luo, Y. He, Y. Gu, H. Zhang, K. Zhao, K. Cui, and J. Song. 2015. Histone modifications induced by MDV infection at early cytolytic and latency phases. BMC Genomics 16:311. doi 10.1186/s12864-015-1492-6
Morris, A., and R. K. Selvaraj. 2014. In vitro 25-hydroxycholecalciferol treatment of lipopolysaccharide-stimulated chicken macrophages increases nitric oxide production and mRNA of interleukin- 1 beta and 10. Vet Immunol. Immunopathol. 161:265-270.
Morris, A., R. Shanmugasundaram, J. McDonald, and R. K. Selvaraj. 2015. Effect of in vitro and in vivo 25-hydroxyvitamin D treatment on macrophages, T cells, and layer chickens during a coccidia challenge. J. Anim. Sci. 93:2894-2903.
Morris, A., R. Shanmugasundaram, M. S. Lilburn, and R. K. Selvaraj. 2014. 25-Hydroxycholecalciferol supplementation improves growth performance and decreases inflammation during an experimental lipopolysaccharide injection. Poult. Sci. 93:1951-1956.
Napper, S., S. Dadgar, R. J. Arsenault, B. Trost, E. Scruten, A. Kusalik, and P. Shand. 2015. Induction of tissue- and stressor-specific kinomic responses in chickens exposed to hot and cold stresses. Poult. Sci. 94:1333–1345.
Nazmi A., R. Hauck, A. Davis, M. Hildebrand, L.B. Corbeil, and R. A. Gallardo. 2017. Diatoms and diatomaceous earth as novel poultry vaccine adjuvants. Poult. Sci. 92:288-294
Nazmi A., R. Hauck, L. B. Corbeil, and R. A. Gallardo. 2017. The effect of diatomaceous earth in live attenuated infectious bronchitis vaccine, immune responses and protection against challenge. Poult. Sci. 96:8 2623-2629.
Park, M., S. Kim, R. H. Fetterer, and R. A. Dalloul. 2016. Functional characterization of the turkey macrophage migration inhibitory factor. Dev. Comp. Immunol. 61:198-207. DOI: 10.1016/j.dci.2016.12.004
Park, M., S. Kim, A. E. Leon, J. S. Adelman, D. M. Hawley, and R. A. Dalloul. 2017. Identification and functional characterization of the house finch interleukin-1β. Dev. Comp. Immunol. 69:41-50. DOI: 10.1016/j.dci.2016.12.004
Pender, C. M., S. Kim, T. D. Potter, M. M. Ritzi, M. Young, and R. A. Dalloul. 2016. Effects of in ovo supplementation of probiotics on performance and immunocompetence of broiler chicks to an Eimeria challenge. Benef. Microbes 7:699-705. DOI: 10.3920/BM2016.0080.
Pender, C. M., S. Kim, T. D. Potter, M. M. Ritzi, M. Young, and R. A. Dalloul. 2017. In ovo supplementation of probiotics and its effects on performance and immune-related gene expression in broiler chicks. Poult. Sci. 96:1052-1062. DOI: 10.3382/ps/pew381
Pender, C. M., S. Kim, L. H. Sumners, M. M. Ritzi, M. Young, and R. A. Dalloul. 2017. In ovo and dietary supplementation of probiotics affects post-hatch expression of immune-related genes in broiler chicks. J. Immunobiol. 2:3. DOI: 10.4172/2476-1966.1000126
Perez, V., R. Shanmugasundaram, M. Sifri, T. M. Parr, and R. K. Selvaraj. 2017. Effects of hydroxychloride and sulfate form of zinc and manganese supplementation on superoxide dismutase activity and immune responses post lipopolysaccharide challenge in poultry fed marginally lower doses of zinc and manganese. Poult. Sci.. doi: 10.3382/ps/pex244
Placek, K., G. Hu, K. Cui, D. Zhang, Y. Ding, J. E. Lee, Y. Jang, C. Wang, J. E. Konkel, J. Song, C. Liu, K. Ge, W. Chen, and K. Zhao. 2017. MLL4 prepares the enhancer landscape for Foxp3 induction via chromatin looping. Nat Immunol 18:1035-1045. doi 10.1038/ni.3812
Potter, T. D., P. Glover, N. P. Evans, and R. A. Dalloul. 2016. Differential ex vivo responses of primary leukocytes from turkey pedigree lines to Salmonella Heidelberg. Poult. Sci. 95:364-369. DOI: 10.3382/ps/pev351
Rezvani, M., M. Mendoza, M. D. Koci, C. Daron, J. Levy, and H. M. Hassan. 2016a. Draft Genome Sequence of Lactobacillus crispatus C25 Isolated from Chicken Cecum. Genome Announc 4. doi 10.1128/genomeA.01223-16
Rezvani, M., M. Mendoza, M. D. Koci, C. Daron, J. Levy, and H. M. Hassan. 2016b. Draft Genome Sequences of Lactobacillus animalis Strain P38 and Lactobacillus reuteri Strain P43 Isolated from Chicken Cecum. Genome Announc 4. doi 10.1128/genomeA.01229-16
Ritzi, M. M., W. Abdelrahman, M. Mohnl, and R. A. Dalloul. 2014. Effects of probiotics and application methods on performance and response of broiler chickens to an Eimeria challenge. Poult. Sci. 93:2772-2778. DOI: 10.3382/ps.2014-04207
Ritzi, M. M., W. Abdelrahman, K. van-Heerden, M. Mohnl, N. W. Barrett, and R. A. Dalloul. 2016. Combination of probiotics and coccidiosis vaccine enhances protection against an Eimeria challenge. Vet. Res. 47:111. DOI: 10.1186/s13567-016-0397-y
Robinson, C.M., H.H. Cheng, and M.E. Delany. 2014. Marek’s disease virus and chicken host genome interactions. Viral genome integration occurs early post-infection and over a timeframe associated with latency, yet integration alone is not sufficient for cellular transformation. Cytogenet. Genome Res. 144:142-154.
Schaal, T.P., J. Arango, A. Wolc, J.V. Brady, J.E. Fulton, I. Rubinoff, I.J. Ehr, M.E. Persia and N.P. O’Sullivan, 2016. Commercial Hy-Line W-36 pullet and laying hen venous blood gas and chemistry profiles utilizing the portable i-STAT 1 analyzer. Poultry Science 95:466-471.
Schock, E.N., C.-F. Chang, I. Youngworth, M. Davey, M.E. Delany, and S.A. Brugmann. 2016. Utilizing the chicken as an animal model for human craniofacial ciliopathies. Developmental Biology 45(2):326-337. http://dx.doi.org/10.1016/j.ydbio.2015.10.024 (PMID: 26597494)
Schock, E.N., C.-F. Chang, J. N. Struve, Y.T. Chang, J. Chang, M. E. Delany and S.A. Brugmann. 2015. Using the avian mutant talpid2 as a disease model for understanding the oral-facial phenotypes of Oral-facial-digital syndrome. Disease Models and Mechanisms 8:855-866. http://dmm.biologists.org/content/8/8/855.full.pdf+html (Highlighted in F1000; PMID: 26044959)
Selvaraj, R. K. 2013. Avian CD4(+)CD25(+) regulatory T cells: properties and therapeutic applications. Dev. Comp. Immunol. 41:397-402.
Shanmugasundaram, R., and R. K. Selvaraj. 2013. In ovo injection of anti-chicken CD25 monoclonal antibodies depletes CD4+CD25+ T cells in chickens. Poult. Sci. 92:138-142.
Shanmugasundaram, R., M. Sifri, and R. K. Selvaraj. 2013. Effect of yeast cell product (CitriStim) supplementation on broiler performance and intestinal immune cell parameters during an experimental coccidial infection. Poult. Sci. 92:358-363.
Shanmugasundaram, R., M. Sifri, and R. K. Selvaraj. 2013. Effect of yeast cell product supplementation on broiler cecal microflora species and immune responses during an experimental coccidial infection. Poult. Sci. 92:1195-1201.
Shanmugasundaram, R., M. Sifri, R. Jeyabalan, and R. K. Selvaraj. 2014. Effect of yeast cell product (CitriStim) supplementation on turkey performance and intestinal immune cell parameters during an experimental lipopolysaccharide injection. Poult. Sci. 93:2763-2771.
Shi, S., Y. Shen, S. Zhang, Z. Zhao, Z. Hou, H. Zhou, J. Zou, and Y. Guo. 2017. Combinatory evaluation of transcriptome and metabolome profiles of low temperature-induced resistant ascites syndrome in broiler chickens. Sci Rep 7(1):2389. doi: 10.1038/s41598-017-02492-8.
Shi, S., Y. Shen, Z. Zhao, Z. Hou, Y. Yang, H. Zhou, J. Zou, and Y. Guo. 2014. Integrative analysis of transcriptomic and metabolomic profiling of ascites syndrome in broiler chickens induced by low temperature. Mol Biosyst. 10(11):2984-93. doi: 10.1039/c4mb00360h.
Slawinska, A., J. C.-F. Hsieh, C. Schmidt, and S. J. Lamont. 2016. Heat stress and lipopolysaccharide stimulation of chicken HD11 cell line activates expression of distinct sets of genes. PLOS ONE. doi:10.1371/journal.pone.0164575
Smith, J., D. W. Burt, and the Avian RNAseq Consortium. 2015. The Avian RNAseq Consortium: a community effort to annotate the chicken genome. Cytogenet. Genome Res. 145:78–179. doi: 10.1101/012559
Stepicheva, N. A., and J. L. Song. 2016. Function and regulation of microRNA-31 in development and disease. Mol Reprod Dev 83:654-674. doi 10.1002/mrd.22678
Sullivan K. A., and G. F. Erf. 2017. Leukocyte infiltration profiles during the cutaneous phytohemagglutinin response. Poult. Sci. 96:3574-3580.
Sun, H., P. Liu, L. K. Nolan, and S. J. Lamont. 2015. Avian pathogenic Escherichia coli (APEC) infection alters bone marrow transcriptome in chickens. BMC Genomics 16:690. doi: 10.1186/s12864-015-1850-4
Sun, H., P. Liu, L. K. Nolan, and S. J. Lamont. 2015. Novel pathways revealed in bursa of Fabricius transcriptome in response to extraintestinal pathogenic Escherichia coli (ExPEC) infection. PLOS ONE 10(11): e0142570. doi:10.1371/journal.pone.0142570
Sun, H., P. Liu, L. K. Nolan, and S. J. Lamont. 2016. Thymus transcriptome reveals novel pathways in response to avian pathogenic Escherichia coli infection. Poult. Sci. 95:2803–2814. doi: 10.3382/ps/pew202
Sun, H., R. Bi, P. Liu, L. K. Nolan, and S. J. Lamont. 2016. Combined analysis of primary lymphoid tissues' transcriptomic response to extra-intestinal Escherichia coli (ExPEC) infection. Dev Comp Immunol 57: 99–106
Swaggerty, C. L., I. Y. Pevzner, and M. H. Kogut. 2014. Selection for pro-inflammatory mediators yields chickens with increased resistance against Salmonella enterica serovar Enteritidis. Poult. Sci. 93:535-544.
Swaggerty, C. L., I. Y. Pevzner, and M. H. Kogut. 2015. Selection for pro-inflammatory mediators produces chickens more resistant to Eimeria tenella. Poult. Sci. 94:37-42.
Swaggerty, C. L., I. Y. Pevzner, H. He, K. J. Genovese, and M. H. Kogut. 2017. Selection for pro-inflammatory mediators produces chickens more resistant to Campylobacter jejuni. Poult. Sci. 96:1623-1627.
Swaggerty, C. L., J. L. McReynolds, J. A. Byrd, I. Y. Pevzner, S. E. Duke, K. J. Genovese, H. He, and M. H. Kogut. 2016. Selection for pro-inflammatory mediators produces chickens more resistant to Clostridium perfringens-induced necrotic enteritis. Poult. Sci. 95:370-374.
Swaggerty, C. L., Kogut, M. H., He, H., Genovese, K. J., Johnson, C., and Arsenault, R. J. 2017. Differential levels of cecal colonization by Salmonella enteritidis in chickens triggers distinct immune kinome profiles. Front. Vet. Sci. | doi: 10.3389/fvets.2017.00214
Taylor, R. L., Jr. 2015. Letter to the Editor – An incomplete story told by a single number. Poult. Sci. 94:1995-1996 doi:10.3382/ps/pev221
Taylor, R. L., Jr. 2016. Letter to the Editor – A publication experiment. Poult. Sci. 95:227 doi:10.3382/ps/pev451
Taylor, R. L., Jr. 2015. The future of poultry science research: Challenges as opportunities. AMENA, Asociación Mexicana de Especialistas en Nutrición Animal, Puerta Vallarta, Mexico http://www.poultryscience.org/2015_AMENA_Symposium.asp
Taylor, R. L., Jr. 2016. Nunc Dimitis - W. Elwood Briles. Poult. Sci. 95:2477 doi:10.3382/ps/pew176
Taylor, R. L., Jr. 2017. Renew the priority for manuscript review. Poult. Sci. 96:4133 doi 10.3382/ps/pex267
Taylor, R. L., Jr., J. L. Anderson, and S. C. Smith, 2014. Commentary on: Atherosclerosis-susceptible and atherosclerosis-resistant pigeon aortic cells express different genes in vivo. International Atherosclerosis Society http://www.athero.org/commentaries/comm1188.asp
Taylor, R. L., Jr., Z. Medarova, and W. E. Briles. 2016. Immune effects of chicken non-Mhc alloantigens. Poult. Sci. 95:447-457 doi:10.3382/ps/pev331 (review)
Tian, F., F. Zhan, N. D. VanderKraats, J. F. Hiken, J. R. Edwards, H. Zhang, K. Zhao, and J. Song. 2013. DNMT gene expression and methylome in Marek's disease resistant and susceptible chickens prior to and following infection by MDV. Epigenetics 8:431-444. doi 10.4161/epi.24361
Tilley, J. E. N., J. L. Grimes, M. D. Koci, R. A. Ali, C. R. Stark, P. K. Nighot, T. F. Middleton, and A. C. Fahrenholz. 2017. Efficacy of feed additives to reduce the effect of naturally occurring mycotoxins fed to turkey hen poults reared to 6 weeks of age. Poult Sci. doi 10.3382/ps/pex214
Trost, B., R. Arsenault, P. Griebel, S. Napper, and A. Kusalik. 2013. DAPPLE: a pipeline for the homology-based prediction of phosphorylation sites. Bioinformatics 29:1693–1695.
Troxell, B., N. Petri, C. Daron, R. Pereira, M. Mendoza, H. M. Hassan, and M. D. Koci. 2015. Poultry body temperature contributes to invasion control through reduced expression of Salmonella pathogenicity island 1 genes in Salmonella enterica serovars Typhimurium and Enteritidis. Appl Environ Microbiol 81:8192-8201. doi 10.1128/AEM.02622-15
Tuggle, C. K., E. Giuffra, S. N. White, L. Clarke, H. Zhou, P. J. Ross, H. Acloque, J. M. Reecy, A. Archibald, R. R. Bellone, M. Boichard, A. Chamberlain, H. Cheng, R. P. Crooijmans, M. E. Delany, C. J. Finno, M. A. Groenen, B. Hayes, J. K. Lunney, J. L. Petersen, G. S. Plastow, C. J. Schmidt, J. Song, and M. Watson. 2016. GO-FAANG meeting: a Gathering On Functional Annotation of Animal Genomes. Anim Genet 47:528-533. doi 10.1111/age.12466
Tuo, W., L. Li, Y. Lv, J. Carrillo, D. Brown, W. C. Davis, J. Song, D. Zarlenga, and Z. Xiao. 2016. Abomasal mucosal immune responses of cattle with limited or continuous exposure to pasture-borne gastrointestinal nematode parasite infection. Vet Parasitol 229:118-125. doi 10.1016/j.vetpar.2016.10.005
Van Goor, A., A. Slawinska, C. J. Schmidt, and S. J. Lamont. 2016. Distinct functional responses to stressors of bone marrow derived dendritic cells from diverse inbred chicken lines. Dev. Comp. Immunol. 63: 96–110
Van Goor, A., C. M. Ashwell, M. E. Persia, M. F. Rothschild, C. J. Schmidt, and S. J. Lamont. 2017. Unique genetic responses revealed in RNA-seq of the spleen of chickens stimulated with lipopolysaccharide and heat. PLOS ONE 12(2): e0171414. doi:10.1371/journal.pone.0171414
Vuong, C. N., W.-K. Chou, V. A. Kuttappan, B. M. Hargis, L. R. Bielke, and L. R. Berghman. 2017. A Fast and Inexpensive Protocol for Empirical Verification of Neutralizing Epitopes in Microbial Toxins and Enzymes. Front. Vet. Sci. 4 Available at https://www.frontiersin.org/articles/10.3389/fvets.2017.00091/full
Wang, X., J. Liu, G. Zhou, J. Guo, H. Yan, Y. Niu, Y. Li, C. Yuan, R. Geng, X. Lan, X. An, X. Tian, H. Zhou, J. Song, Y. Jiang, and Y. Chen. 2016. Whole-genome sequencing of eight goat populations for the detection of selection signatures underlying production and adaptive traits. Sci Rep 6:38932. doi 10.1038/srep38932
Warren, W.C., L.W. Hillier, C. Tomlinson, P. Minx, M. Kremitzki, T. Graves, C. Markovic, N. Bouk, K. Pruitt, F. Thibaud-Nissen, V. Schneider. T. Mansour, C.T. Brown, A. Zimin, R. Hawken, A.B. Pyrkosz, M. Morisson, V. Fallon, A. Vignal, W. Chow, K. Howe, J.E. Fulton, M.M. Miller, P.I. Lovell, C. Mello, M. Wirthlin, A.S. Mason, R. Kuo, D.W. Burt, J.B Dodgson and H.H. Cheng, 2017. A new chicken genome assembly provides insight into avian genome structure. G3: Genes, Genomes, Genetics, 7 (1) p 109-117. doi:10.1534/g3.116.035923
Weathers, B., S. L. Branton, R. Jacob, R. L. Taylor, Jr., E. D. Peebles, and G. T. Pharr. 2015. Expression of the ephrin receptor B2 in the embryonic chicken bursa of Fabricius. Int. J. Poult. Sci. 14:485-490
Weintraut, M. L., S. Kim, R. A. Dalloul, and E. A. Wong. 2016. Expression of small intestinal nutrient transporters in embryonic and posthatch turkeys. Poult. Sci. 95:90-98. DOI: 10.3382/ps/pev310
Weng, Z., A. Wolc, X. Shen, R.L. Fernando, J.C.M. Dekkers. J. Arango. P. Settar, J.E. Fulton, N.P. O’Sullivan, and D.J. Garrick, 2016. Effects of number of training generations on genomic prediction for various traits in a layer chicken population. Genetics Selection Evolution 48:22, DOI 10.1186/s12711-016-0198-9.
Wideman, R. F., D. D. Rhoads, G. F. Erf, and N. B. Anthony. 2013. Pulmonary Hypertension Syndrome (PHS, Ascites Syndrome) in Broilers: A Review. Poult. Sci. 92:64-83.
Wolc, A, J. Arango, T. Jankowski, P. Settar, J.E. Fulton, N.P. O’Sullivan, R. Fernando, D. J. Garrick and J.C.M. Dekkers, 2013. Genome wide association study for Marek’s Disease mortality in layer chickens. Avian Diseases 57:519-522.
Wolc, A., A. Kranis, J. Arango, P. Settar, J.E. Fulton, N.P. O’Sullivan, A. Avendano, K.A. Watson, J.M. Hickey, G. de los Campos, R.L. Fernando, D.J. Garrick and J.C.M. Dekkers, 2016. Implementation of genomic selection in the poultry industry. Animal Frontiers 6: 23-31.
Wolc, A., J. Arango, P. Settar, J.E. Fulton, N.P. O’Sullivan, J.C.M. Deckers, R. Fernando and D.J. Garrick, 2016. Mixture models detect large effect QTL better than GBLUP and result in more accurate and persistent predictions. J. Anim. Sci. Biotech. doi 10.1186/s40104-016-0066-z
Wolc, A., J. Arango, P. Settar, J.E. Fulton, N.P. O’Sullivan, R. Preisinger, R. Fernando, D.J. Garrick and J.C.M. Dekkers, 2013. Analysis of egg production in layer chickens using a random regression model with genomic relationships. Poultry Science 92: 1486-1491.
Wolc, A., J. Arango, T. Jankowski, I. Dunn, P.Settar, J.E. Fulton, N.P. O’Sullivan, R. Preisinger, R.L. Fernando, D.J. Garrick and J.C.M. Dekkers, 2014. Genome-wide association study for egg production and quality in layer chickens. Journal of Animal Breeding and Genetics. 131 (3): 173-182.
Wolc, A., J. Arango, T. Jankowski, P. Settar, J.E. Fulton, N.P. O’Sullivan, R. Fernando, D.J. Garrick and J.C.M. Dekkers, 2013. Pedigree and genomic analyses of feed consumption and residual feed intake in laying hens. Poultry Science 92:2270-2275.
Wu, G., L. Liu, Y. Qi, Y. Sun, N. Yang, G. Xu, H. Zhou, and X. Li. 2015. Splenic gene expression profiling in White Leghorn layer inoculated with the Salmonella enterica serovar Enteritidis. Anim Genet. doi: 10.1111/age.12341.
Xu, H., X. Zhu, Y. Hu, Z. Li, X. Zhang, Q. Nie, L. K. Nolan, and S. J. Lamont. 2014. DNA methylome in spleen of avian pathogenic Escherichia coli-challenged broilers and integration with mRNA expression. Sci. Rep. 4:4299. doi:10.1038/srep04299
Xu, L., D. M. Bickhart, J. B. Cole, S. G. Schroeder, J. Song, C. P. Van Tassell, T. S. Sonstegard, and G. E. Liu. 2015. Genomic Signatures Reveal New Evidences for Selection of Important Traits in Domestic Cattle. Molecular Biology and Evolution 32:711-725. doi 10.1093/molbev/msu333
Xu, L., F. Zhao, H. Ren, L. Li, J. Lu, J. Liu, S. Zhang, G. E. Liu, J. Song, L. Zhang, C. Wei, and L. Du. 2014. Co-Expression Analysis of Fetal Weight-Related Genes in Ovine Skeletal Muscle during Mid and Late Fetal Development Stages. International Journal of Biological Sciences 10:1039-1050. doi 10.7150/ijbs.9737
Xu, L., J. B. Cole, D. M. Bickhart, Y. Hou, J. Song, P. M. VanRaden, T. S. Sonstegard, C. P. Van Tassell, and G. E. Liu. 2014. Genome wide CNV analysis reveals additional variants associated with milk production traits in Holsteins. Bmc Genomics 15. doi 10.1186/1471-2164-15-683
Xu, L., R. J. Haasl, J. Sun, Y. Zhou, D. M. Bickhart, J. Li, J. Song, T. S. Sonstegard, C. P. Van Tassell, H. A. Lewin, and G. E. Liu. 2017. Systematic Profiling of Short Tandem Repeats in the Cattle Genome. Genome Biol Evol 9:20-31. doi 10.1093/gbe/evw256
Xu, L., Y. He, Y. Ding, G. Sun, J. A. Carrillo, Y. Li, M. M. Ghaly, L. Ma, H. Zhang, G. E. Liu, and J. Song. 2017. Characterization of Copy Number Variation's Potential Role in Marek's Disease. Int J Mol Sci 18. doi 10.3390/ijms18051020
Xu, L., Y. Hon, D. M. Bickhart, J. Song, C. P. Van Tassell, T. S. Sonstegard, and G. E. Liu. 2014. A genome-wide survey reveals a deletion polymorphism associated with resistance to gastrointestinal nematodes in Angus cattle. Functional & Integrative Genomics 14:333-339. doi 10.1007/s10142-014-0371-6
Xu, L., Y. Hou, D. M. Bickhart, Y. Zhou, H. A. Hay el, J. Song, T. S. Sonstegard, C. P. Van Tassell, and G. E. Liu. 2016. Population-genetic properties of differentiated copy number variations in cattle. Sci Rep 6:23161. doi 10.1038/srep23161
Yin, H., L. H. Sumners, R. A. Dalloul, K. B. Miska, R. H. Fetterer, M. C. Jenkins, Q. Zhu, and E.A. Wong. 2015. Expression of an antimicrobial peptide, digestive enzymes and nutrient transporters in the intestine of E. praecox-infected chickens. Poult. Sci. 94:1521-1526. DOI: 10.3382/ps/pev133
Yu, P., Y. Lu, B. J. Jordan, Y. Liu, J. Y. Yang, J. M. Hutcheson, C. L. Ethridge, J. L. Mumaw, H. A. Kinder, R. B. Beckstead, S. L. Stice, and F. D. West. 2014. Nonviral minicircle generation of induced pluripotent stem cells compatible with production of chimeric chickens. Cell Reprogram. 16:366-378
Zar Mon, K. K., P. Saelao, M. M. Halstead, G. Chanthavixay, H.-C. Chang, L. Garas, E. A Maga, and H. Zhou. 2016. Salmonella enterica serovars Enteritidis infection alters the indigenous microbiota diversity in young layer chicks. Front. Vet. Sci. - Veterinary Infectious Diseases. 2:61. doi: 10.3389/fvets.2015.00061.
Zhang, C., M. Wang, N. He, M. F. Ahmed, Y. Wang, R. Zhao, X. Yu, J. Jin, J. Song, Q. Zuo, Y. Zhang, and B. Li. 2018. Hsd3b2 associated in modulating steroid hormone synthesis pathway regulates the differentiation of chicken embryonic stem cells into spermatogonial stem cells. J Cell Biochem 119:1111-1121. doi 10.1002/jcb.26279
Zhao, C., J. A. Carrillo, F. Tian, L. Zan, S. M. Updike, K. Zhao, F. Zhan, and J. Song. 2015. Genome-Wide H3K4me3 Analysis in Angus Cattle with Divergent Tenderness. Plos One 10. doi 10.1371/journal.pone.0115358
Zhao, C., J. A. Carrillo, F. Tian, L. Zan, S. M. Updike, K. Zhao, F. Zhan, and J. Song. 2015. Genome-Wide H3K4me3 Analysis in Angus Cattle with Divergent Tenderness. PLoS One 10:e0115358. doi 10.1371/journal.pone.0115358
Zhao, C., L. Zan, Y. Wang, M. S. Updike, G. Liu, B. J. Bequette, R. L. Baldwin, and J. Song. 2014. Functional proteomic and interactome analysis of proteins associated with beef tenderness in Angus cattle. Livestock Science 161:201-209. doi 10.1016/j.livsci.2013.11.030
Zhao, C., X. Li, B. Han, Z. You, L. Qu, C. Liu, J. Song, L. Lian, and N. Yang. 2017. Gga-miR-219b targeting BCL11B suppresses proliferation, migration and invasion of Marek's disease tumor cell MSB1. Sci Rep 7:4247. doi 10.1038/s41598-017-04434-w
Zhou, H., P. J. Ross, C. Kern, P. Saelao, Y. Wang, J. L. Chitwood, I. Korf, M. Delany, and H. Cheng. 2016. Genome-wide functional annotation of regulatory elements in chickens. Pp:48-52. The Proceedings of XXV World’s Poultry Congress, Beijing, China.
Zhu,Y., W. Wang, T. Yuan, L. Fu, L. Zhou, G. Lin, S. Zhao, H. Zhou, G. Wu, and J. Wang. 2017. MicroRNA-29a mediates the impairment of intestinal epithelial integrity induced by intrauterine growth restriction in pig. Am J Physiol Gastrointest Liver Physiol. 312(5):G434-G442. doi: 10.1152/ajpgi.00020.2017. Epub 2017 Mar 9.
Zuo, Q., K. Jin, Y. Zhang, J. Song, and B. Li. 2017. Dynamic expression and regulatory mechanism of TGF-beta signaling in chicken embryonic stem cells differentiating into spermatogonial stem cells. Biosci Rep 37. doi 10.1042/BSR20170179
Zuo, Q., Y. Wang, S. Cheng, C. Lian, B. Tang, F. Wang, Z. Lu, Y. Ji, R. Zhao, W. Zhang, K. Jin, J. Song, Y. Zhang, and B. Li. 2016. Site-Directed Genome Knockout in Chicken Cell Line and Embryos Can Use CRISPR/Cas Gene Editing Technology. G3 (Bethesda) 6:1787-1792. doi 10.1534/g3.116.028803
12 total book chapters from NE-1334 Project participants 2013-2017 *= cooperative publication among 2 or more project participants
*Lamont, S. J., J. C. M. Dekkers, and H. Zhou. 2014. Immunogenetics and mapping immunological functions. Pages 205–221 in Avian Immunology. K. A. Schat, B. Kaspars, P. Kaiser, ed. Elsevier, London, UK.
11 book chapters from individual project participants (7 not shown sue to character restrictions)
Carrillo, J. A. and J. Song. 2014. Bioinformatics in Animal Genetics accepted by book "Molecular and Quantitative Animal Genetics" Molecular and Quantitative Animal Genetics, First Edition. Edited by Hasan Khatib. P143-154 © 2014 John Wiley & Sons, Inc. Published 2014 by John Wiley & Sons, Inc.
Cheng, H. H., and S. J. Lamont. 2013. Genetics of disease resistance. Pages 70–86 in Diseases of Poultry. 13th ed. D. E. Swayne, J. R. Glisson, L. R. McDougald, V. Nair, L. Nolan, and D. L. Suarez, ed. Wiley-Blackwell, Ames, USA
Dalloul, R. A. 2017. Understanding and Boosting Poultry Immune Systems. Chapter 6 in: Achieving Sustainable Production of Poultry Meat, Volume 3: Health and Welfare, Applegate, T. J., editor. Burleigh Dodds Science Publishing, Sawston, Cambridge, UK. DOI: 10.19103/AS.2016.0011.21
Erf, G. F. 2014. Autoimmune diseases of poultry. Pages 315-332 in: Avian Immunology, 2nd edition, Schat, K. A., Kaspers B. and, P. Kaiser, editors. Elsevier, Academic Press, San Dieg