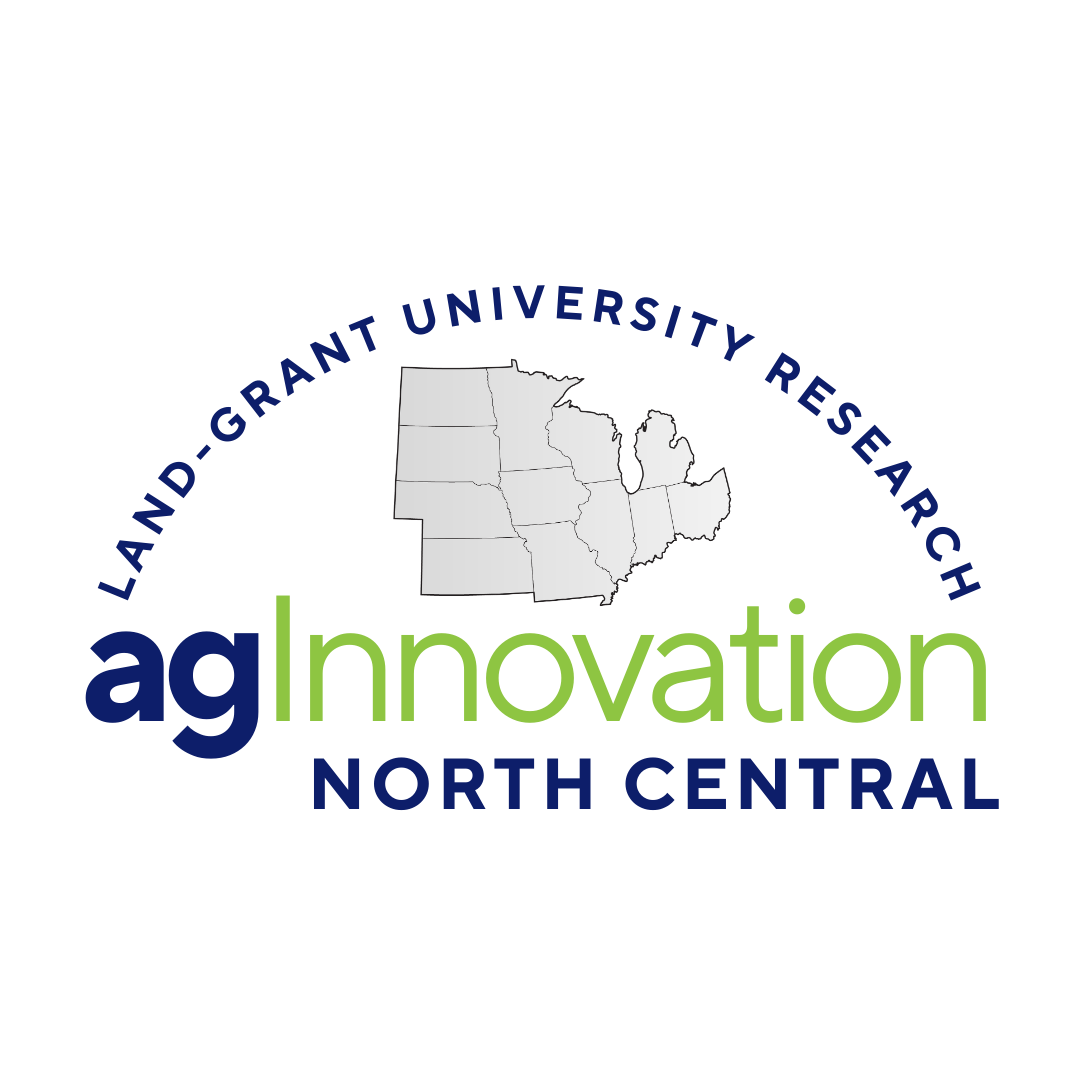
NC_old1184: Molecular Mechanisms Regulating Skeletal Muscle Growth and Differentiation
(Multistate Research Project)
Status: Inactive/Terminating
NC_old1184: Molecular Mechanisms Regulating Skeletal Muscle Growth and Differentiation
Duration: 10/01/2015 to 09/30/2020
Administrative Advisor(s):
NIFA Reps:
Non-Technical Summary
Statement of Issues and Justification
The overall goal of this cooperative, multi-state, multidisciplinary, basic research project is to increase the efficiency of meat production. Based on population projections, world-wide meat production will need to increase 45% by 2050. Moreover, meat animal production contributes some $900 billion to the US economy each year. As such, meat production is not only necessary for global human health and well-being but is also a significant driver of the economy. In order to increase the efficiency of lean muscle accretion, a better understanding of those basic biological mechanisms undergirding skeletal muscle tissue is necessary. Although we have made significant progress towards this goal under the current NC-1184 project, participation in the project has increased nearly 40% in the last few years. This coupled with technological advances has provided new tools and approaches for continued investigation of these critical processes. The goal of this multi-state, multidisciplinary, basic research project is to utilize these tools to elucidate the molecular and cellular processes that control skeletal muscle growth and function; thereby, providing opportunities to increase the efficiency of lean meat production in animals. This renewal describes the collaborative effort of researchers from 25 different Agricultural Experiment Stations that will characterize various aspects of the molecular and cellular mechanisms that regulate skeletal muscle growth and function. Major points that support the continuation of this important, fundamental research project for the next five years are: 1. Meat is a significant source of protein for human consumption. Meeting the needs of a growing population will require significant growth in meat production world-wide. Though some of this growth will be realized by increasing animal units, greater outputs must be realized per animal unit. This will require a more thorough understanding of those mechanisms responsible for the efficiency of protein accretion. 2. In 2012, the meat industry was responsible for nearly 6% of the GDP of the US. Ensuring the viability of this industry in times of elevated energy costs and concerns for the environment will require a better understanding of those inputs, and outputs, required by optimal meat animal production. 3. The project continues to relate directly to the national agricultural research priorities set forth in the NIFA Strategic Plan (2014 – 2018) Goal 1: SCIENCE, subgoal 1.1 “Advance our nation’s ability to fight hunger and ensure global food security”. Performance Measures, as outlined in this same Strategic Plan include: 1.1.3 (conferences); 1.1.4 (peer-reviewed publications and 1.1.5 (research). “Improve understanding of fundamental animal physiological processes” (NIFA knowledge area 305) of this Objective is supported by the efforts of this re-write. Improving the meat animal production efficiency based on a fundamental understanding of mechanisms controlling muscle growth, is directly related to this goal. 4. One of the Actionable Strategies of NIFA Goal 1, subgoal 1.7.5 is "Number of graduate students post-doctorates engaged in NIFA-administered projects and programs with an integrated education component to improve educational opportunities in agriculture”. This is an important component of this project. Training undergraduate and graduate students, and postdoctorates in basic fields will relate to long-term improvements in agricultural productivity. 5. The NC-1184 Committee continues to be highly productive. Many papers emanating from this group of scientists are published in top tier journals often reserved for reporting fundamental knowledge. This reflects well on the aptitude of the membership and the basic nature of their approach in creation of new knowledge. 6. The NC-1184 project is both a multi-state and a multidisciplinary project, involving the effort of investigators at 25 different State Agricultural Experiment Stations. The Principal Investigators represent a variety of basic science disciplines that complement each other and provide the expertise necessary to complete the objectives. 7. The project continues to enjoy a strong collaborative environment. During the current project, there have been exchanges of data, scientists, students, samples, cell lines, reagents, etc. In addition, there has been vast knowledge sharing of techniques and on occasion, use of equipment only available at particular stations. The fruits of these collaborations are best illustrated by the number publications jointly authored among the membership. 8. Members of the NC-1184 committee have been and will continue to be highly successful in obtaining outside support to fund this research. Funding from the USDA NRICGP (AFRI) Program, NIH, NSF, health-related granting agencies, and industry sources are used to maintain a high level of productivity. In summary, this project describes a fundamental research approach to an important agricultural issue, providing the world a high quality source of protein. The current membership has grown substantially and is highly qualified for making immediate and unprecedented progress towards the goals outlined in this revised project proposal.
Related, Current and Previous Work
There is little duplication between this project and other funded multi-state (regional) projects. Searching the CRIS Multi-State Projects database using the keywords "muscle” retrieved only one project - NC-1131, which was the precursor of this committee. As muscle is the precursor of meat, meat was used as a single keyword and yielded 1 active project: NCCC210. This projected is entitled "Regulation of Adipose Tissue Accretion in Meat-Producing Animals”. Given the role of adipose tissue to meat, some overlap is expected. The only other overlap with NCCC210 would be where investigators may be studying the interplay, communication, or transdifferentiation of cell types. This is particularly germane given reports suggesting muscle cells are capable of transdifferentiating into adipocytes. This overlap is quite small, nonetheless. The following is a literature review, though not comprehensive, of major elements of muscle growth, development and metabolism. EXTRACELLULAR MATRIX - Extracellular matrix (ECM) was classically viewed as a structural substance that cells were embedded in and was passive in terms of modulating cell behavior. With the progression of molecular and cellular biology, ECM is now known to play significant roles in tissue structure and cellular function. The extracellular matrix regulates cell behavior by: 1) being a substrate for cell migration; 2) modulating growth factor activity; 3) transmitting instructive and permissive signals; and 4) serving as a tissue structural framework necessary for normal functional integrity. The interaction of the cell with the ECM is largely governed by heterodimeric integrin cell membrane receptors. Integrins are involved in cell proliferation, adhesion, and apoptosis in muscle cells (Liu et al., 2008). The cell membrane specifically functions in the attachment of cytoskeletal and extracellular matrix macromolecules. The extracellular matrix is composed of a diverse group of macromolecules including collagens, non-collagenous glycoproteins, glycosaminoglycans (GAGs), and proteoglycans. A complete understanding of how ECM participates, facilitates or otherwise modulates muscle development and subsequent growth is lacking. MUSCLE FORMATION AND MYOGENESIS - Muscle development results from restriction of pluripotent stem cells to a muscle cell lineage through an event referred to as commitment, or determination. Muscle regulatory factors (MRFs) are basic helix-loop-helix (HLH) transcription factors that participate intimately in this process by regulating the transcription of muscle specific genes (reviewed by Weintraub, 1993). There are four members of this family; MyoD1, myogenin, myf-5 and MRF4/herculin/myf-6. MRFs form heterodimers with ubiquitously expressed E2A, E12 and E47 gene products, and these heterodimers bind to the DNA consensus sequence. Understanding how these factors are controlled or may be manipulated to enhance muscle development is not known. STEM CELLS - Stems cells are defined by morphology, biochemical parameters and expression of gene-specific markers. Using these credentials, three classes of stem cells are universally recognized; embryonic (ES), mesenchymal (MSC) and tissue-restricted. Due to these unique yet malleable properties, ES cells are viewed as valuable tools for both scientific discovery and regenerative medicine. However, the mechanisms that underlie ES potency and plasticity are not universal. Although differences in morphology and niche factor requirements are evident between the specie ES cell lines, a common core genetic signature does exist. iPS cells behave equivalent to ES cells in that they are capable of forming embryoid bodies containing cells of the three germ layers as well as chimeric mice by tetraploid complementation (Boland et al., 2009). The second major class of stem cells contains those that are restricted in their plasticity and give rise typically to 3-4 different somatic cell types. Within the MSC-enriched population, subsets of cells exist underscoring the heterogeneity of the loosely defined population and contributing to the wide-ranging efficiency of differentiation amongst the various sources of MSCs (Lv et al., 2014). Unipotent stem cells include those found in skeletal muscle, mammary gland, intestine and a host of other adult tissues. These cells divide to give rise to a daughter or progenitor cell that further differentiates irreversibly into a terminal cell type. SATELLITE CELLS – Unipotent satellite cells were originally identified and named based upon their position adjacent to the muscle fiber (Mauro, 1961). These cells typically reside in a quiescent state in vivo that is denoted by a high nuclear:cytoplasmic volume and condensed chromatin (Shafiq, et al. 1968). The field of satellite cell biology rapidly expanded with the discovery of Pax7 as a lineage marker (Mansouri et al., 1996). Elimination of the satellite cell pool by genetic ablation of Pax7 causes early neonatal lethality. By contrast, elimination of the Pax7-expressing satellite cell in young mice (? 3 wk age) caused no detriments to skeletal muscle growth and repair. These results point to the dispensable nature of satellite cells to adult muscle growth. However, evidence clearly supports the necessity of a robust satellite cell pool for neonatal and juvenile animal muscle fiber growth (Lepper et al., 2009) as well as post-exercise fiber recovery (Sambasivan et al., 2011). Regarding meat animal production, some of the muscle fiber damage in growth-selected turkey lines and other species may be due to limitations in satellite cell availability or function. GROWTH FACTORS - Once committed to the muscle lineage, myoblasts stop replicating and differentiate, which is demarcated by an up-regulation in muscle-specific proteins. This whole process is either directly or indirectly mediated by a number of growth factors (GF). Members of this and previous regional projects have made significant contributions to our understanding of the roles of insulin-like growth factors (IGFs), fibroblast growth factors, transforming growth factor-beta (TGF-beta) and myostatin, anabolic steroids, hepatocyte growth factor (HGF), leptin, Insulin-like growth factor binding proteins (IGFBP), ERK, E2F, and beta-adrenergic agonists in regulating muscle proliferation and differentiation in meat animals. In bovine satellite cell (BSC) cultures, both IGF-1 and Estradiol-17? (E2) stimulate rates of proliferation and protein synthesis and decrease rate of protein degradation; and IGF-1 stimulates differentiation. Understanding the mechanism by which widely used E2 implants stimulate bovine muscle growth is desperately needed but also will elucidate a novel mechanism by which IGF-1 affects bovine muscle growth. In the future this kind of fundamental information will help in developing safe and effective strategies to increase rate and efficiency of muscle growth in meat animals. MYOSTATIN - Mutations in the myostatin (MSTN) gene are responsible for the well-known double-muscled syndrome in cattle. MSTN is a member of the transforming growth factor-beta superfamily and is a negative regulator of skeletal muscle growth (McPherron and Lee, 1997). Mice and cattle lacking a functional myostatin gene exhibit dramatic increases in skeletal muscle mass (Wegner et al., 2000). The condition in several cattle breeds results in excessive muscle fiber formation (hyperplasia). Skeletal muscles of these cattle contain almost double the number of fibers compared with other cattle breeds, whereas fiber size is nearly equal or slightly larger than normal animals. This tremendous increase in muscle fiber number is associated with increases in muscle mass of > 20% and decreases in fat. The activity of native MSTN is suppressed by the MSTN propeptide (MSTNPro), the N-terminal part of unprocessed MSTN that is cleaved off during post-translational MSTN processing. Creating an available source of MSTNPro is therefore essential to investigating the potential of the MSTN axis to enhance muscle growth in agricultural animal species. MUSCLE CELL SIGNALING PATHWAYS - Growth factors affect muscle through complex intracellular signal transduction pathways that are initiated by binding of growth factors or hormones to specific intracellular or cell surface receptors (Lee et al., 2000). The formation of the ligand receptor complex initiates intracellular signal transduction mechanisms resulting in activation of specific molecules and/or expression of specific genes that regulate muscle differentiation and growth. Signal transduction pathways also interact with each other (cross-talk), or with structural proteins within the cell in a myriad of ways that are not completely understood at present. Although the general intracellular pathways utilized by a number of growth factors have been identified, little is currently known about how these specific pathways function in muscle to control proliferation and differentiation of embryonic myogenic cells and satellite cells or hypertrophy of muscle fibers in postnatal muscle. For example, titin, a giant muscle protein, is the “backbone” of the sarcomere. Titin plays a molecular spring-like role in myocyte contractility and integrates diverse myocyte signaling pathways that may regulate protein turnover, hypertrophic activation, and mechanosensing. STAC - Another novel signaling pathways is modulated through STAC3, a member of the STAC gene family that includes STAC1 and STAC2. These functionally unknown genes are predicted to encode proteins containing Src homology 3 (SH3) and cysteine-rich (C1) domains. The SH3 domain regulates the activity or subcellular location of signaling proteins, the assembly of multiprotein complexes, and cell proliferation. The C1 domain is involved in protein-membrane association. While the STAC1 and STAC2 genes are specifically expressed in neurons, studies in mice indicated that the STAC3 gene is expressed only in skeletal muscle. Skeletal muscle of STAC3 knockout mice is lethal and mice possess muscle that cannot contract and had significant morphological abnormalities, yet conditional knockout develop normally with a minimal postnatal phenotype. Understanding how this pathway alters muscle function may provide insights into how muscle growth may be augmented. O-linked ?-D-N-acetylglucosamine (O-GlcNAc) - The mechanistic basis of these hierarchical “nutrient-sensor” signal transduction pathways and their functional links remains yet to be fully defined. The addition and removal of O-linked ?-D-N-acetylglucosamine (O-GlcNAc), a sugar derivative that integrates carbohydrate, lipid, protein, energy, and nucleotide metabolisms in cells, is a novel signaling pathway that plays critical roles in a number of cell types. However, the functional role of O-GlcNAc in modulating skeletal muscle growth, metabolism and global metabolic diseases remains yet to be investigated. AMP-activated protein kinase (AMPK) – AMPK is a heterotrimeric serine/threonine kinase composed of catalytic ? and regulatory ? and ? subunits; in response to low cellular energy status (high AMP:ATP), AMPK inhibits energy consuming pathways, including protein synthesis. The porcine R200Q mutation in the AMPK?3 subunit result in constitutive AMPK activation, thereby contributing to increased mitochondrial protein content and enhanced oxidative capacity in “glycolytic” skeletal muscles (Garcia-Roves et al., 2008). Understanding how these pigs are capable of depositing protein in the presence of a known inhibitor (activated AMPK) may provide insights into the control of protein turnover and improved growth efficiency. MUSCLE PROTEIN SYNTHESIS - Accumulation of muscle protein mass depends on the balance between rate of muscle protein synthesis and rate of muscle protein degradation. It is now well-established that muscle protein synthesis has an important and often predominant role in rate of skeletal muscle growth. Decreasing the rate of general muscle protein synthesis is directly related to increasing the efficiency with which ingested nutrients are converted to muscle tissue. Insulin and growth factors like IGFs activate mammalian target of rapamycin (mTOR), a serine–threonine kinase, via signaling transduction pathway involving protein kinase B (PKB) (Weigl, 2012) to increase general or global rates of muscle protein synthesis. Less understood are the mechanisms used to alter gene-specific translation of transcription factors and other proteins which can influence muscle capacity for growth. The role of various nutritional and environmental management strategies on gene-specfic translation remains obscure. MUSCLE PROTEIN DEGRADATION - Skeletal muscle proteins become the main source of free amino acids when dietary supplies fall short of meeting the needs of vital organs and/or providing gluconeogenic substrates, protein degradation could also occur in response to growth and tissue remodeling (Ventadour and Attaix, 2006). Several degradation pathways exist in mammalian cells. Ubiquitin-proteasome pathway. Polyubiquitination of protein prior to degradation occurs via three enzymes: ubiquitin activating enzyme (E1), ubiquitin-conjugating enzymes (E2), and ubiquitin-protein ligases (E3) ( Ventadour and Attaix, 2006). Autophagy-lysosomal pathway. Protein degradation by the autophagy-lysosomal system, involves the formation of the autophagosomes and their fusion with lysosomes containing hydrolases (proteases, glycosidases, lipases, nucleases, and phosphatases) (Bechet et al., 2005). Calpain system. The calpain system includes 14 different members plus calpastatin, a protein inhibitor that is specific for inhibition of the two, ubiquitous, well-characterized calpains, µ-calpain and m-calpain. Skeletal muscle contains appreciable quantities of the calpains, and evidence from a variety of sources indicates that the two ubiquitous calpains have an important role in metabolic turnover of myofibrillar proteins. Members of the NC-1184 Project have examined the Calpain system in the major model animal and livestock species including fish (Salem and Killifer, 2004; Saito et al., 2000). Caspase system. Proteases in the caspase family are responsible for degradation of proteins during apoptosis. The caspases are cysteine protease, like µ-and m-calpain are, but they do not require Ca2+ for activity. Of the four protease systems in skeletal muscle, there is some debate as to which are most important, especially given muscle cells contain a very complex filamentous structure consisting of a number of rather large proteins. Specifically, calpains do not degrade polypeptides to amino acids but rapidly degrade those proteins that are responsible for maintaining the myofibrillar assembly. The proteasome, on the other hand, cannot degrade intact myofibrils or even intact myofilaments but can rapidly degrade individual polypeptides to small, 4-10-amino acid peptides. Hence, although the mechanism described in the dissociation /proteasome degradation model has been widely quoted as being the mechanism used to turnover myofibrillar proteins in striated muscle, this model has never been critically examined. MUSCLE MITOCHONDRIA: Mitochondria largely govern the energy generating capacity of muscle cells. Increasing mitochondrial content augments energy production, whereas altering functional parameters, such as proton leak, can impact the efficiency of mitochondrial ATP production. In addition to serving as the powerhouse of the cell, mitochondria play significant roles in calcium homeostasis, reactive oxygen species production, and apoptosis. Thus, perturbing mitochondrial function can contribute to energy deficits, oxidative stress, and even cell death. The influence of various stimuli on mitochondrial function is not well-documented, particularly in livestock. Developing a better understanding of mitochondrial function/dysfunction is of increasing importance as we consider targets for improving efficiency of meat production. MUSCLE WASTING - Muscle experiences atrophy from an imbalance in muscle protein synthesis and degradation, and is commonly associated with aging (sarcopenia) and disease (cachexia). Both sarcopenia and cachexia are associated with a systemic pro-inflammatory environment including increased levels of interleukin (IL)-6 and IL-1? (Alvarez-Rodriguez et al., 2012). Pro-inflammatory cytokines can regulate muscle mass by regulating satellite cell behavior, affecting proliferation and differentiation of myoblasts, and/or by increasing signaling through intracellular signaling pathways that lead to muscle atrophy. Elevated levels of circulating IL-6 result in decreased muscle mass, which was reversed in the presence of an IL-6 receptor antagonist (Tsujinaka et al., 1996). These data indicate that the presence of systemic inflammation, in particular increased levels of pro-inflammatory interleukins, may negatively affect muscle mass by increasing protein degradation. MUSCLE DYSTROPHY - Despite the long-term promise of gene and stem cell therapies these approaches are unlikely to provide substantial clinical benefit to Duchenne muscular dystrophy (DMD) patients in the near future. Consequently, it is imperative to identify interventional strategies that have a high probability for more immediate translational success. Understanding a human muscle-based malady like DMD will undoubtedly lead to novel strategies for improve muscle growth in farm animals. HEAT STRESS - Advances in management strategies (e.g. cooling systems and barn design) and utilizing low heat increment diets have partially alleviated the negative impact of heat stress (HS) in the agricultural industry, but production continues to markedly decrease during the summer (Baumgard and Rhoads, 2012). HS stimulates a decrease in nutrient intake and depresses growth, yet increases carcass lipid accretion and decreases carcass nitrogen content (Rhoads et al., 2013a). However, few experiments accounted for the effect of depressed feed intake during HS vs. direct effects of HS on production parameters and metabolic pathways. INTRAUTERINE GROWTH RETARDATION - Optimum growth of the fetus and neonate is critical to achieving the maximal growth potential of animals. In spite of improvements in management strategies, IUGR remains a significant problem, especially in pigs depriving the entire industry of profits largely because the mechanisms underlying IUGR growth remain unclear (Wu et al., 2006). Most importantly, IUGR animals have retarded skeletal muscle development, which affects their growth through adulthood (Rehfeldt and Kuhn, 2006). A general lack of knowledge regarding those mechanisms responsible for IUGR manifestation across all species has prevented development of an effective approach to enhance growth in these highly valued food producing animals (Wu et al., 2006). THE ADIPOCYTE AND MYOCYTE, THEIR COLLECTIVE METABOLISM AND COMMUNICATION, AND POSSIBLE TRANSDIFFERENTIATION – Besides its primary motor function, skeletal muscle plays important roles in regulating systemic energy metabolism and insulin sensitivity. First, it acts as an energy sink/store due to its ability to utilize glucose to generate force for movements. Second, skeletal muscle interacts with adipose tissues to regulate the plasticity and function of adipocytes. For example, muscle-derived irisin (a peptide hormone) robustly converts energy-storing white adipocytes into energy-dissipating brown adipocytes, thus reducing blood glucose levels and improving body insulin sensitivity (Borstrom et al., 2012). Another muscle-derived factors that may regulate adipocyte plasticity and muscle metabolism is FGF21 (Lin et al., 2013). Although muscle and fat are known to interact, the molecular mechanisms mediating their cross-talk are poorly understood. Given the importance of these two tissues in mediating insulin sensitivity and energy metabolism, understanding mechanisms involved with cellular fate is a critical component of controlling cellular composition of tissues and animals. TENDERNESS AND MARBLING - According to the past surveys of beef producers by National Cattlemen’s Beef Association, marbling and tenderness were consistently identified as the top beef quality problems (Garcia et al., 2008). Beef tenderness is mainly determined by myofibril overlap and connective tissue. Management practices can resolve overlap issues but efforts to reduce connective tissue content and cross-linking are needed. Marbling, on the other hand, results from adipogenesis within muscles. Recent studies show that intramuscular adipocytes and fibroblasts are developed from common progenitor cells (Uezumi et al., 2011). Thus, enhancing adipogenic and inhibiting fibrogenic differentiation from progenitor cells will increase marbling and tenderness of meat. Enhancing knowledge of intramuscular adipogenesis in meat animals will allow us to develop strategies to reduce excessive fat accumulation in other fat depots, elevating the production efficiency of beef cattle. Members of the NC-1184 regional project have made significant contributions to our fundamental understanding of muscle biology and product quality development. Studies proposed herein build on these findings and will further our knowledge of the basic processes regulating muscle growth. This fundamental knowledge will provide the framework necessary to develop management strategies to increase the efficiency of meat-animal growth.
Objectives
-
Characterize the signal transduction pathways that regulate skeletal muscle growth and metabolism including the influence of endogenous growth factors and various production practices.
-
Characterize the cellular and molecular basis of myogenesis.
-
Characterize mechanisms of protein assembly and degradation in skeletal muscle.
Methods
The objectives of the currently active NC-1184 project were broadly defined in order to allow for changes in direction of work based on results of current studies both within and outside of the project. Consequently, the overall objectives of the revised project are similar to those for the current NC-1184 project. However, approaches and methodologies have been changed to reflect advances in knowledge during the five year duration of the currently active project. Because of page limitations general approaches rather than detailed methods are described below. Objective 1. Characterize the mechanisms of skeletal muscle protein turnover. Work will focus on those mechanisms responsible for controlling protein determining the protein synthesis, breakdown and subsequently protein accretion. Four stations, New Jersey, Virginia, Mississippi and Iowa will use diverse approaches to test study different aspects of these processes. The New Jersey station will study how environmental perturbations alter general versus gene-specific translation. Some of these perturbations may disrupt the folding capacity of the endoplasmic reticulum (ER), activating a tripartite mechanism called the Unfolded Protein Response (UPR, also called the ER Stress Response). Phosphorylation of the translation factor called eIF2 constitutes one arm of the UPR which serves to alleviate cell stress through a reprogramming of gene expression driven by increases in the synthesis of the transcription factor ATF4. The station will also explore how gene-specific translation contributes to the regulation of muscle growth in a wide variety of environmental stressors. The Virginia station will use the IUGR model to explore the limitations to postnatal muscle protein deposition. Control piglets and those meeting the definitions of growth retardation will be collected from commercial litters. Piglets will be artificially raised and stable isotopes will be used to determine if amino acids or other nutrients can rescue growth retardation. Piglets will also be sacrificed and the transcriptome of muscle tissues will be described. These studies should provide insight into how muscle is impacted by nutrition prenatally and explore management strategies to overcome these deficiencies. The New Jersey station will also study the interface of dietary protein and exercise as it relates to weight control and optimization of lean body mass. In particular, they will study how the composition, distribution, source and/or timing of dietary protein can influence signaling pathways that regulate tissue growth and development. Aside from the eIF2 signaling pathway, the mTOR complex 1 (mTORC1) signaling pathway is a major transducer of cellular information regulating both protein synthesis and protein degradation processes in response to four major signal inputs: nutrients (i.e., amino acids), growth factors, energy and stress. Information gained in this area will be used to create novel approaches to better prevent and treat diabetes and obesity, and augment animal growth efficiency. The Mississippi station will use the contemporary commercial swine breeds (pure or cross among Yorkshire, Landrace, Duroc, and Hampshire) as research models to study the molecular mechanisms in terms of limiting AA regulation of muscle protein turnover and animal growth performance (such as fat:lean ratio). The metabolic and cell signaling pathways, as well as gene networks that regulate muscle protein synthesis and degradation in vivo will be explored. Various research techniques commonly used in animal nutrition and molecular biology, such as Microarray, RNA-seq, Real-time RT-PCR, proteomics, Western-blotting, etc. will be alternatively utilized in Mississippi research projects. Results of these projects will likely help swine nutritionists and producers to understand why imbalanced diets can limit muscle growth and can increase fat:lean ratio at the molecular and cellular level. In a collaboration with the Iowa station, the Virginia station will determine the extent to which heat stress (HS) alters cellular developments before and following return to thermoneutral (TN) conditions. Specifically, these scientists will determine: 1) the extent to which gestational and postnatal heat stress alters muscle biochemistry to limit protein accretion, 2) determine the extent to which the intracellular environment remains unfavorable for protein accretion in skeletal muscle following a return to thermoneutral conditions, and 3) identify the relationship between skeletal muscle bioenergetics, energy substrate supply, protein synthesis and degradation during heat stress. The Wyoming station will study interaction between titin and main protein quality control systems such as the calpain system and the ubiquitin-proteasome system. Since titin directly connects to ubiquitous calpain-1 at the NH2 terminus of the I-band segment and skeletal muscle-specific calpain-3 at both the N2A domain and the M-band, the Wyoming station will use RBM20 (a regulator of titin isoform switching) knockout rat model to determine whether the alteration of titin isoform affects the binding of calpains to titin, and thus induces sarcomere degradation. The Wyoming station will also determine whether the alteration of titin isoform is associated with meat tenderness. Objective 2. Characterize the cellular and molecular basis of myogenesis and muscle growth. Studies in this objective focus primarily on those factors, biological or otherwise, that alter muscle development and impinge on subsequent postnatal growth. Fourteen stations (AZ, ID, NC, KS, WY, TX, MN, MI, IL, CT, HI, OH, VA, WA, IN) are involved in studies contributing to this objective and several of the proposed studies involve collaborations among participating stations. To address the issue of global climate changes and animal performance, the Michigan station will collaborate with the Minnesota and Ohio stations to define the effects of an acute thermal change on turkey satellite cell growth and development. Both in ovo and post-hatch thermal challenges will be used to isolate those times and tissue events most sensitive to increases in temperature. Finally, these investigators will attempt to develop embryonic thermal manipulation approaches to mitigate the damaging effects of post-hatch thermal challenges on long-term muscle growth and development in turkeys. The Texas station is investigating genetic components that regulate muscle growth, and are important for efficient growth of “tropically adapted” breeds of beef cattle suitable for the warmer climates of the southern U.S. Two stations (Illinois and Hawaii) are studying the mechanism(s) by which myostatin (MSTN) can be exploited to increase muscle growth using separate approaches. The Hawaii station is currently producing recombinant MSTN-suppressing proteins of various animal species. Those proteins include MSTN propeptide and follistatin, and these recombinant proteins will be used in studying the mechanism(s) of action of MSTN through administration to pigs and other meat animals. The Illinois station will make myostatin null pigs. Once created, investigators will strategically mate myostatin null pigs with a mutated IGF2 (g3072A). Results from these studies provide an answer to long-standing questions of why naturally occurring myostatin null pigs do not exist and how growth factors interact with the myostatin cytokine. The Connecticut, Illinois, North Carolina, Missouri, Mississippi, Kansas and Virginia stations will work on various aspects of prenatal determinants of muscle growth using pigs, chickens, sheep, cattle and horses. Results of these studies will likely help us understand why maternal and fetal nutrition can affect pre- and post-natal muscle growth, and contribute to reduced meat production, poor product quality, and increased cost of production in livestock. Along those same lines and using a model of maternal infection with porcine respiratory and reproductive syndrome (PRRS) virus, the Illinois station will determine the effects of a maternal infection during pregnancy on piglet muscle development and postnatal muscle growth, as well as the epigenetic programming of muscle. The Connecticut station will use in vitro models to determine the signaling mechanisms by which inflammatory cytokines regulate satellite cell function in multiple species (sheep/horses/mice). The Illinois station will determine the extent to which acute dietary protein ingestion regulates amino acid transporter expression on satellite cells, and alters the quantity and paracrine factor expression of MSCs in healthy weight, overweight and obese adults. The New Jersey station will also look at the role of limited or minimal protein nutrition in determining muscle mass with age and its relationship to longevity. Using a turkey model, the Ohio station will determine the effects of age and growth selection on muscle development and cellular mechanisms as regulated by syndecan-4 and glypican-1. The group will also establish the effects of nutritional status on growth, differentiation, and syndecan-4 and glypican-1 signal transduction in satellite cells derived from turkeys differing in growth potential and age. Finally, the Ohio station will determine the effect of immediate post-hatch feed restriction on growth, breast muscle structure, satellite cell proliferation and differentiation, cellular signal transduction pathways regulated by syndecan-4 and glypican-1, and adipocyte formation. The Indiana station will study the satellite cell niche and define the mechanisms responsible for the maintaining the cellular heterogeneity found in this locale. This hierarchical composition of readily differentiating progenitors and self-renewable stem cells assures the extraordinary regenerative capacity of skeletal muscles while maintaining a sustainable pool of satellite cells for sustained postnatal growth. Using exercise mouse models and genetic models exhibiting either lean or obese phenotype, the station will also explore what molecules are involved in mediating the muscle-fat cross-talk, and how such molecules can be targeted to achieve a better muscle function and metabolic profile. Finally, the station will use a number of in vitro and in vivo modules to explore the signaling mechanisms that differentially regulate these subpopulations of satellite cells and how such mechanisms are employed in muscle regeneration. In conjunction with the North Carolina station, researchers at the Virginia station will elucidate the role of neonatal nutrition in impacting the developmental programming of muscle and fat growth, provide a comprehensive understanding of the potential genetic regulatory networks responsible for alterations in the differentiation potential of the tissue-specific stem cells responsible for muscle and fat growth, and identify differences in the genetic regulation of cellular activities among related tissue-specific stem cells to provide insight into developmental programs in pigs. The Indiana station will continue to explore mechanisms responsible for cell transdifferentiation. Building on the seminal discovery that brown fat and skeletal muscle share a common progenitor cell origin distinct from white adipose progenitors, investigators will use transgenic mouse models that genetically labels stem cell lineages aim to determine the developmental origin of various depots of white adipose, especially the brown-adipocytes within the white adipose tissues (also called brite or beige adipoyctes). Similarly, Washington station will separate intramuscular progenitor cells from beef muscle using cell surface markers. Expression of key genes regulating myogenesis, adipogenesis and fibrogenesis will be analyzed, and the underlying mechanisms will be explored, focusing on epigenetic modifications of key genes committing progenitor cells to different lineages. Moreover, histone and other DNA modifications will be studied under various nutritional regimes and treatments. The Minnesota Station will examine the mechanism by which IGF-I and E2 stimulate bovine muscle growth by assessing: 1) the impacts of a selective IGF-1 receptor (IGF-1R) tyrosine kinase inhibitor and EGFR tyrosine kinase inhibitors on EGFR and IGF-1R phosphorylation and on rates of proliferation, differentiation, protein synthesis, and protein degradation in IGF-1- or E2-treated BSC cultures; 2) the impact of IGF-1R or EGFR knockdown on the ability of IGF-1 or E2 to affect EGFR and IGF-1R phosphorylation and to affect rates of proliferation, differentiation, protein synthesis, and protein degradation in BSC cultures; 3) the effects of E2 or IGF-1 treatment on MMP2 and MMP9 mRNA and activity levels in BSC cultures and the effects of a specific MMP2 and MMP9 inhibitor and a hbEGF neutralizing antibody on EGFR phosphorylation and on rates of proliferation, differentiation, protein synthesis, and protein degradation in IGF-1- or E2-treated BSC cultures; 4) the effects of IGF-1 or E2 on selected intracellular signaling pathways activated by either IGF-1R or EGFR; 5) the effects of E2 infusion on IGF-1R and EGFR phosphorylation and on MMP2/9 activity in skeletal muscle of calves; 6) the effect of E2 implantation on MMP2/9 mRNA level and activity in muscle of steers implanted with an E2 implant. The North Carolina station will study the impact of ammonia/nitrogen on myostatin regulatory mechanisms in the developing chick embryo as a model system. Moreover, the station will also study the effect of the embryonic environment on ultimate meat yield by transplanting somites from chickens of different growth potentials, and studying the ability of the cells to participate and adapt to a differing cellular environment potential The Virginia station will exploit the long term selection for juvenile body size in chickens that has resulted in two lines of birds that exhibit divergent postnatal growth patterns. The consequences of the single trait selection on embryogenesis are unresolved. Embryos from the high-weight select (HWS) and low-weight select (LWS) lines will be compared at various time points for global growth and developmental differences and possible alterations in myogenesis. Objective 3. Characterize the signaling networks regulating skeletal muscle structure, function and metabolism. Characterization of the signaling pathways that regulate muscle-specific genes is clearly key to understanding muscle growth. Cooperating units responsible for the work under this objective are the Iowa, Florida, Michigan, Missouri, New Jersey, Ohio, Texas, Virginia and Wyoming stations. In order to address molecular signaling mechanisms of titin isoform transition, the Wyoming station will use primary cell cultures and rat models that are lack of RNA binding motif 20 (RBM20), a major regulator of titin isoform transition, and treat these cell and rat models with thyroid hormone (T3). Next, the Wyoming station will identify the translational modification of RBM20 using mass spectrometry, and also try to understand the regulation of RBM20 gene expression via mTOR pathway. The Wyoming station will also try to find other hormones or growth factors regulating titin isoform transition since titin only expresses larger titin isoform with RBM20 in fetuses, and without RBM20 at all ages. The Iowa station found that activation of the peroxisome proliferator-activated receptor gamma coactivator 1-alpha (PGC-1?) pathway simultaneously offset multiple secondary pathologies in muscular dystrophin, increased utrophin protein expression, and decreased injury in dystrophic skeletal muscle. The group will conduct comprehensive studies focused on the disease mitigating properties of quercetin – an orally available PGC-1? activator. The station will partner with DART Pharmaceuticals. The Missouri station will explore the use endocrine profiling as a means to evaluate environmental impacts on animal phenotype, permitting a greater understanding of the physiological mechanisms impacted by those environmental variables and therefore facilitating better livestock management decisions. In addition, the station is currently collaborating with a number of other stations (Virginia, Minnesota, Iowa, Illinois, Florida and Nebraska) to aid in their endocrine measures of animal performance. Working with the Florida station, the Virginia station will define mechanisms responsible for changes in muscle metabolism and protein turnover in growing animals using the Rendement Napole pigs (AMPK?3R200Q) as a model. The Arkansas station has found that specific nutrients can regulate skeletal muscle mitochondrial biogenesis and cellular bioenergetics. They are working with chicken, pig and rat models to define the mechanisms responsible for these changes and to determine if long-term treatment can actually increases muscle mass. The Texas station is evaluating mechanisms of RNA regulation of gene signaling, as well as validating structural gene pathways that affect meat quality and tenderness, especially in Bos indicus-influenced breeds of cattle. The Florida station will use nutritional and genetic models to understand the signaling networks influencing muscle metabolism. Metabolic characteristics change during an animal’s lifetime and are also responsive to various environmental stimuli, such as exercise or macronutrient composition of the diet. This station will focus on understanding the regulation of mitochondrial content and function, as well as how oxidative capacity relates to composition, efficiency of growth, and muscle function.Measurement of Progress and Results
Outputs
- Research supported by this project will result in training of new researchers equipped to use molecular and cell biology methods to study factors regulating proliferation and differentiation of muscle cells in meat-producing animals.
- Publications will be the primary tangible result expected from the project because it focuses on basic research. It is anticipated that utilization of these published results will lead to improved efficiency of lean meat production in domestic livestock and poultry.
- The Committee will sponsor a Symposium on Molecular Mechanisms Regulating Skeletal Muscle Growth and Differentiation at a national meeting of the American Society of Animal Sciences (2015). This symposium will provide a mechanism to convey new research information and will increase the visibility of the project.
Outcomes or Projected Impacts
- Understanding the role of heat stress on pre and postnatal animal growth.
- Elucidation of the prenatal nutrition on subsequent animal performance.
- Increased knowledge of the role of specific genes in regulating proliferation and differentiation of muscle cells in meat animals.
- Increased understanding of the role of the extracellular matrix in regulating proliferation and differentiaton of muscle cells in meat animals.
- Increased understanding of the role of specific proteases in protein degradation in skeletal muscle.
- Knowledge of whether the myostatin axis can be manipulated to increase meat production in pigs. Understand the key regulators of muscle development. Increased knowledge of the genes responsible for making muscle and fat. Increased understanding of the role of growth factors and cell signaling pathways in regulating proliferation and differentiation of muscle cells may lead to molecular- and cellular biology-based strategies for more efficient production of lean meat which will benefit both producers and consumers. For example, it may be possible to alter fiber type distribution in muscle to increase muscle mass and/or meat quality. Additionally, it may be possible to increase or prolong the proliferative activity of muscle satellite cells so that they provide more DNA to support postnatal muscle fiber growth. Elucidation of mechanisms responsible for muscle protein degradation may provide information necessary to utilize molecular- or cell biology-based methods to reduce protein degradation in growing muscle. This will allow more rapid muscle growth without increasing energy requirements. Gene expression studies may lead to identification of genes that may be used to produce transgenic animals that more efficiently produce muscle. Additionally, because this project combines the skills of muscle biologists and molecular biologists, collaboration between committee members should result in elucidation of the function in muscle growth and differentiation of proteins produced by identified genes.
Milestones
(2016): Sponsor a symposium on Molecular Mechanisms Regulating Skeletal Muscle Growth, Development and Metabolism at ASAS National Meeting. This will allow us to convey research findings to scientists from academia, federal laboratories, and the private sector that usually attend these meetings.Projected Participation
View Appendix E: ParticipationOutreach Plan
Dissemination of the results of the research will be done via the timely publication of refereed articles in established journals. Because this is a basic research project, publications will be the primary tangible result expected from the project. It is anticipated that utilization of these published results will lead to improved efficiency of lean meat production in domestic livestock and poultry. Additionally, in 2016 the committee will organize a symposium on Molecular Mechanisms Regulating Skeletal Muscle Growth and Differentiation at the ASAS/ADSA National Meeting that will convey recent research findings to the members of these organizations.
Organization/Governance
The Technical Committee will consist of at least one representative from each participating unit, appointed as described in the Manual for Cooperative Regional Research, with one representative from each unit designated as the voting member. The North Central Regional Association of Directors will appoint one Director to serve as Administrative Advisor and a representative of the USDA/NIFA will also serve as an Advisor; both will be ex officio member of this committee. The Technical Committee will elect a Chair and a Secretary to serve for a period of one year, and these officers will continue to be voting members. In succeeding years, the Secretary will become Chair, and only a new Secretary will be elected. The Chair, the Secretary, the immediate Past Chair and the Administrative Advisor will serve as the Executive Committee, and they will have the authority to act on behalf of the Technical Committee during the periods between meetings. Because this is a proposal for renewal of the NC-1184 project, the current Chair will serve as Past Chair for the first year of the revised project and the current Secretary will become Chair if the renewal is approved. The Chair, with approval of the Administrative Advisor, will call yearly meetings of the Technical Committee and interim meetings of the Executive Committee if needed. A report of research results from each unit will be presented orally and in writing at the Technical Committee meetings. The written report should include a list of publications resulting from research related to the project for the year. Reports will be critically reviewed by the Technical Committee and recommendations will be made for future research and coordination of research between units to maximize attainment of the Objectives. The Secretary will prepare minutes and an Annual Report for distribution to Technical Committee members, Directors and Department Chairs of participating State Agricultural Experiment Stations and USDA Laboratories, and the Regional Research Office, NIFA. Because submission of a yearly progress report is essential to assess progress, ensure participation, and prepare the Annual Report, any unit that does not submit a written progress report for two consecutive years will be considered not to be participating and will be dropped from the project. A station, agency, or institution not currently listed as a participant in the project can petition to join by submitting an addendum to the Methods section of this proposal. This addendum should describe the proposed work and how it contributes to the project. The Administrative Advisor will circulate the addendum to the voting members of the Technical Committee for their consideration and approval. If approved by a majority of the voting members of the Technical Committee, the addendum will be added to the official project outline.
Literature Cited
Alvarez-Rodríguez L, López-Hoyos M, Muñoz-Cacho P, Martínez-Taboada VM. 2012. Aging is associated with circulating cytokine dysregulation. Cell Immunol. 273(2):124-32. Attaix D, Ventadour S, Codran A, Béchet D, Taillandier D, Combaret L. 2005. The ubiquitin-proteasome system and skeletal muscle wasting. Essays Biochem. 41:173-86. Baumgard LH, Rhoads RP. 2012. Ruminant Nutrition Symposium: ruminant production and metabolic responses to heat stress. J Anim Sci. 90(6):1855-65. Boland MJ, Hazen JL, Nazor KL, Rodriguez AR, Gifford W, Martin G, Kupriyanov S, Baldwin KK. 2009. Adult mice generated from induced pluripotent stem cells. Nature. 3:461(7260):91-4. Boström P, Wu J, Jedrychowski MP, Korde A, Ye L, Lo JC, Rasbach KA, Bostrom EA, Choi JH, Long JZ, Kajimura S, Zigaretti MC, Vind BF, Tu H, Cinti S, Hojlund K, Gygi SP Speigelman BM. 2012 A PGC1-?-dependent myokine that drives brown-fat-like development of white fat and thermogenesis Nature 481(7382): 463-468. Fujita J, Tsujinaka T, Yano M, Ebisui C, Saito H, Katsume A, Akamatsu K, Ohsugi Y, Shiozaki H, Monden M. 1996. Anti-interleukin-6 receptor antibody prevents muscle atrophy in colon-26 adenocarcinoma-bearing mice with modulation of lysosomal and ATP-ubiquitin-dependent proteolytic pathways. Int J Cancer. 68(5):637-43. Garcia-Roves PM, Osler ME, Holmström MH, Zierath JR. 2008. Gain-of-function R225Q mutation in AMP-activated protein kinase gamma3 subunit increases mitochondrial biogenesis in glycolytic skeletal muscle. J Biol Chem. 283(51):35724-34. Lepper C, Conway SJ, Fan CM. 2009. Adult satellite cells and embryonic muscle progenitors have distinct genetic requirements. Nature 460:627–631. Lin Z, Tian H, Lam KSL, Lin S, Hoo RCL, Monishi M, Itoh N, Wang Y, Borstein SR, Xu A, Li X. 2013. Adiponectin mediates the metabolic effects of FGF21 on glucose homeostasis and insulin sensitivity in mice. Cell Metab. 17(5):779–789 Liu A, Nishimura T, Takahashi K. 1995. Structural weakening of intramuscular connective tissue during postmortem ageing of chicken semitendinosus muscle. Meat Sci. 39:135–142. Lv W, Guo X, Wang G, Xu Y, Kang J. 2014. Histone deacetylase 1 and 3 regulate the mesodermal lineage commitment of mouse embryonic stem cells. PLoS One. 20;9(11):e113262. Mansouri A, Stoykova A, Torres M, Gruss P. 1996. Dysgenesis of cephalic neural crest derivatives in Pax7?/? mutant mice. Development 122:831–838. Mauro A, 1961. Satellite cell of skeletal muscle fibers. J. Biophys. Biochem. Cytol. 9:493–495. McPherron AC, Lee SJ. 1997. Double muscling in cattle due to mutations in the myostatin gene. Proc. Natl. Acad. Sci. U. S. A 94:12457-12461. Rehfeldt C, Kuhn G. 2006. Consequences of birth weight for postnatal growth performance and carcass quality in pigs as related to myogenesis. J Anim Sci. Suppl:E113-23. Saito M, Li H, Thompson VF, Kunisaki N, Goll, DE. 2007. Purification and characterization of calpain and calpastatin from rainbow trout, Oncorhynchus mykiss. Comp. Biochem. Physiol. 146: 445-455. Salem M, Killefer J. 2004. Cloning of the calpain regulatory subunit cDNA from fish revelas a divergent domain V. Anim. Biotech. 15:1-13. Sambasivan R, Yao R, Kissenpfennig A, Van Wittenberghe L, Paldi A, Gayraud-Morel B, Guenou H, Malissen B, Tajbakhsh S, Galy A. 2011. Pax7-expressing satellite cells are indispensable for adult skeletal muscle regeneration. Development. 138(17):3647-56. Erratum in: Development. 138(19):4333. Uezumi A, Fukada S, Yamamoto N, Takeda S, Tsuchida K. 2010. Mesenchymal progenitors distinct from satellite cells contribute to ectopic fat cell formation in skeletal muscle. Nat Cell Biol. 12(2):143-52. Ventadour S, Attaix D. 2006. Mechanisms of skeletal muscle atrophy. Curr Opin Rheumatol. 18(6):631-5. Wegner J, Albrecht E, Fiedler I, Teuscher F, Papstein HJ, Ender K. 2000. Growth- and breed-related changes of muscle fiber characteristics in cattle. J. Anim Sci. 78:1485-1496. Weigl LG. 2012. Lost in translation: regulation of skeletal muscle protein synthesis. Curr Opin Pharmacol. 12(3):377-82. Weintraub H. 1993. The MyoD family and myogenesis: redundancy, networks, and thresholds. Cell. 31;75(7):1241-4. Wu G, Bazer FW, Wallace JM, Spencer TE. 2006. Board-invited review: intrauterine growth retardation: implications for the animal sciences. J Anim Sci. 84(9):2316-37.