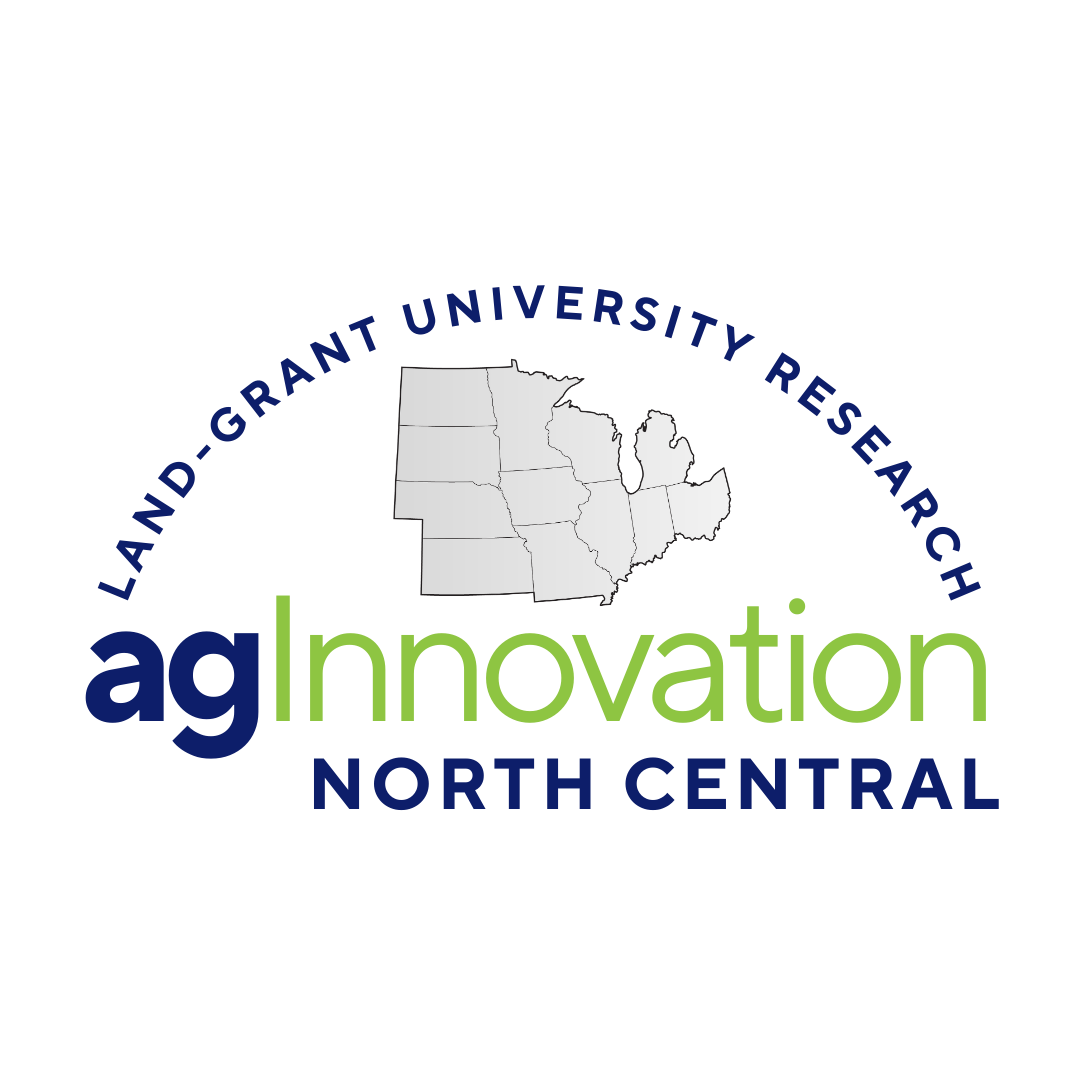
NC_old1194: Nanotechnology and Biosensors
(Multistate Research Project)
Status: Inactive/Terminating
NC_old1194: Nanotechnology and Biosensors
Duration: 10/01/2011 to 09/30/2016
Administrative Advisor(s):
NIFA Reps:
Non-Technical Summary
Statement of Issues and Justification
Development of sensing and processing technologies based on nanoscale phenomena becomes increasingly relevant for our society as we continue to advance our knowledge of biological phenomena related to food, agriculture, environment and energy. As we understand the molecular mechanisms that underlie the emergence and spread of pathogens and their consequent impact on our agricultural and food systems, it becomes evident that the technology to intervene and mitigate their effects on public health need to be correspondingly small, i.e. within the realm of nanotechnology. According to the FY 2010-2015 Strategic Plan published by the USDA, one of the strategic goals for this period is to Ensure that all of America's children have access to safe, nutritious and balanced meals. Within this goal, Specific Objective 3 states that we must Protect public health by ensuring that food is safe. Reduction in the number of foodborne illnesses will require a comprehensive "farm-to-fork" risk assessment, coupled with appropriate technological measures to screen, identify, and eliminate offending food pathogens. In addition to safety concerns in processed foods, plant/animal pathogens also represent major threats to worldwide food security. Rapid responses to crops and livestock epidemics require fast and accurate evaluation of plant/animal pathogens, which also calls for advances in biosensor technology to provide more user-friendly tools and techniques. In the last five years, members of the multistate committee NC-1031 have demonstrated leadership and world-class abilities to develop biosensing and processing technologies based on technology platforms such as micro and nanofluidics, micro and nanoeletromechanicals systems (MEMS / NEMS), optical / electromagnetic nanoparticle technologies (multifunctional, magnetic, electrically active, SERS / fluorescent nanoparticles), and using top-down microfabrication, bottom-up self assembly, bioconjugation techniques and tools from molecular and cellular biology. Our collective expertise provides a strong foundation to address current issues pertinent to food safety and food security including, 1) Developing sensing and analytical devices and systems for detecting pathogens relevant to agricultural and food safety/security, 2) Developing innovative technologies to aid life-sciences researchers in probing biological phenomena relevant to mechanisms of pathogenicity and pathogen inactivation at the nano scale and 3) Inventing advanced packaging and processing techniques that increase safety and prolong shelf life of packaged and / or processed food products. Members of this committee have well-established relations with stakeholder groups and participate in research centers built around university - industry partnerships. They also have access to world-class analytical and fabrication facilities, which are crucial to the success of research programs relevant to this committee. Justification for renewal: Although this committee is highly relevant to the current national priorities of ensuring food safety/security, the subject of nanotechnology and biosensing is critical to a broader spectrum of issues in the areas of food, agriculture, environment and bioenergy. Members of the NC-1031 multistate committee have published research addressing the research and educational objectives outlined in the original research proposal as it relates to the broader context. They have developed and shared graduate and undergraduate course materials in nanotechnology. A number of publications and some intellectual property have been developed over the award period. In addition, committee representatives from the University of Illinois and Purdue University have played a leadership role in bringing together a larger nanotechnology community to establish an industry-university partnership entitled, the Center for Agricultural and Pharmaceutical Nanotechnology. Similarly, the committee representative from Michigan State University has played a key role in the multi-institutional program development and implementation of the missions of the National Center for Food Protection and Defense (a Homeland Security center of excellence) through the use of nanoscale materials and biosensing devices. In addition, committee members have been invited and have presented at each other's respective institutions, thus creating the environment necessary to pursue grant opportunities in nanotechnology and biosensing. In previous years, the annual meeting also was held in conjunction with the annual meeting of the Institute of Biological Engineering to provide greater visibility to the committee and its members and to encourage greater membership. The committee meetings have benefited the members through open discussions and have led to better science. These regular meetings also have ensured that the scientific pursuit utilizes the latest advances in the fields of nanotechnology and reorients priorities to remain relevant to the latest challenges. For example, in the first half of the award period, the bulk of the research conducted by the members was in the area of development of devices and systems for the purpose of detection. However, in the latter half of the project, there has been an increasing trend towards understanding the environmental and toxicological implications of nanotechnology with an increasing focus on the biological aspects of biosensors and nanoscience. This exemplifies a growth trajectory within the group and can be attributed to a sustained communication between the participants. Objectives for the next five years: Through a member survey conducted in the fourth year (2009), it was concluded that the original five objectives continue to be relevant to the research and professional goals of the NC-1031 community. However, there has been an increasing trend towards the biological aspects of the science as mentioned above. In the renewal proposal, we will specifically address this issue. In addition to the scientific objectives, we also will develop a plan to increase the membership of this committee by involving members of the research community interested in understanding the biological and environmental effects of nanotechnology, as well as those interested in using these tools to elucidate the life sciences. Such new members are expected to bring complementary skills to the present group of device and systems engineers that comprise the committee. At this time we have a membership of 26 individuals representing 18 experimental stations. This is an increase of 6 member stations over the previous renewal. In addition, NC-1031 does not duplicate the efforts of any other multistate committee and stands unique in its objectives. The committee also realizes that there is an emerging national need to train highly skilled next-generation scientists in biosensor instrumentation and nanotechnology. In the next five years we also will develop educational and outreach programs on the use and impacts of nanotechnology and bring such training to a broader audience in food, agriculture and biological systems who are traditionally outsiders to the nanotechnology community.
Related, Current and Previous Work
In the past five years (2006-2011), members of the multistate committee NC-1031 have worked on individual and collaborative projects that span multiple objectives. Detailed year-to-year accomplishments are available in the annual reports. A recent annual report is attached to this document. Some of the representative accomplishments and previous work are featured here. Protein nanoarray construction (Arizona, Objectives 1, 2, 3): A protein nanoarray made by size-dependent self-assembly (SDSA) successfully detected Octamer-4 (a differentiation factor from human embryonic stem cells) through utilizing FRET as sensing modality (Tran et al. 2010). This protein nanoarray is currently being implemented in creating a blood vessel mimic (BVM), by patterning a certain combination of receptors/cytokines/growth factors in a desired nanoarray geometry within a synthetic vascular graft. The protein nanoarray system can become an important tool in designing a better blood vessel mimic, so that tissue-engineered vascular grafts can be used also for small-diameter vessels, hopefully resolving many complications in cardiovascular diseases. BSA-loaded PLA to encapsulate bioactive ingredients (Nebraska, Objectives 2,3): The successful preparation of bovine serum albumin (BSA)-loaded polylactic acid (PLA) using a two-phase coaxial jet electrospray technique demonstrated the feasibility of a two-phase coaxial jet electrospray technique to encapsulate bioactive ingredients (Xu and Hanna, 2008), as well as triggered our interest in extending its application to foods. Whey Protein-based Nanocomposites (Wisconsin, Objectives 2,3): Nanocrystalline zinc oxide (ZnO) particles coated with whey protein isolate (WPI) were fabricated in the weak basic aqueous solution condition at near room temperature. The X-ray diffraction and transmission electron microscopy measurements confirmed the nano-scaled composite structure of ZnO-WPI. The average composite granules size was about 300 nm and the embedded ZnO nanoparticles were uniform and monodisperse with an average diameter of 65 nm. In addition, pectin-ZnO nanocomposite was prepared in the aqueous solution condition at room temperature. The Fourier transform infrared, X-ray diffraction, and transmission electron microscope (TEM) measurements confirmed the nano-scaled structure of pectin-ZnO composite (Shi and Gunasekaran 2008). Development of Nanoscale Devices and Analytical Systems (Michigan, Objectives 1 and 3) The Nanoscale Devices and Systems group has been highly successful in developing excellent approaches of combining nanotechnology, biology, chemistry, physics, and engineering into rapid testing devices and systems. As indicated in the first proposal, the group focused on developing prototype devices employing various platform designs, such as protein nano-arrays, micro-TAS (total analysis system), protein-based conductometric biosensors, and protein as well as DNA-based electrochemical biosensors, for pathogen detection in food and water. The project has exceeded the objectives and successfully produced devices, knowledge, and educational materials far greater than the earlier stated deliverables. These devices and systems, particularly biosensors and sensing technologies, have been applied to rapidly detect pathogens and other contaminants of concern to food and water safety, food defense, environmental quality, animal health, food production, and other agricultural-related systems. They are shown to possess increased sensitivity and reduced response time compared to standard methods (Liu et al., 2010a, 2010b; Jin et al., 2010; Yuk et al., 2009; Liu et al., 2008; Liu et al., 2007; Muhammad-Tahir et al., 2007; Zuo et al., 2006; Gore et al., 2006). Proof-of- concept designs and biosensor prototype technologies have been developed for rapid detection of E. coli O157:H7 (Setterington et al., 2010; Yuk et al., 2010; Luo et al., 2010), Salmonella species (Zhang et al., 2010; Zhang et al., 2009; Zhang and Alocilja 2008; Jin et al., 2008), Bacillus anthracis (Anderson et al., 2010; Pal and Alocilja 2010; Pal and Alocilja 2009; Pal et al., 2008), Bacillus cereus (Pal et al., 2008),bovine viral diarrhea virus (Luo et al., 2010), avian influenza virus (Kamikawa et al., 2011; Kamikawa et al., 2010), Mycobacterium avium paratuberculosis (Okafor et al., 2008). In support to the development of biosensing technologies, the members have also developed nanomaterial-based reagents, such as electrically active magnetic nanoparticles (Pal ald alocilja, 2010; Pal and Alocilja 2009) and gold nanoparticles (Anderson et al. 2011; Torres-Chavolla et al., 2010). We will build on these accomplishments as we move towards addressing the next set of challenges on rapid detection of pathogens and contaminants in food and agricultural systems. These challenges include field operability, portability, reproducibility of results, robustness of the device, simplicity and cost of production. Evaluation of the environmental impact of nanotechnology (Illinois, Purdue, Objective 4). Over the last five years, this objective has grown in relative importance. In the last two years specifically, a number of the members have increased their research, education and outreach activities to address issues related to the biological and environmental impact of the nanotechnology-based devices and systems. Ongoing research in various member programs of Prof. Irudayaraj (single cell visualization), Prof. Rickus (biomineralization), Prof. Yoon (systems and devices for measuring airborne viral particles and their effects) and Prof. Bhalerao (methods for understanding nanoparticle uptake in environmental microbes) fall within this objective. The proposed committee will allow interactions between such members to continue and will provide a forum for sharing ideas and future collaborations in this area. Development of educational and outreach materials (Arizona, Iowa, Illinois, Objective 5) Several members of the committee have developed and regularly teach courses in the areas of biosensing and nanotechnology. We have leveraged the Web to share such course materials through ongoing educational projects such as the nanoHUB (www.nanohub.org), an online lecture, multimedia and software repository for educational and outreach information related to nanotechnology. Prior to the development of this renewal, we also surveyed the participants to discuss if the current objective areas continued to be relevant. It became clear from the survey that some objectives were more mature than others, while others, though in their infancy, were becoming more important to the committee. For example, in the first phase, we shared active development of nanotechnology devices and systems, especially in the area of biosensing. However, we expect that the role of evaluating environmental impact will continue to grow into the future. The renewal proposal for the next five years actively focuses on such growth areas while maintaining a stronghold in existing areas.
Objectives
-
Develop new technologies for characterizing fundamental nanoscale processes
-
Construct and characterize self-assembled nanostructures
-
Develop devices and systems incorporating microfabrication and nanotechnology
-
Develop a framework for economic, environmental and health risk assessment for nanotechnologies applied to food, agriculture and biological systems
-
Produce education and outreach materials on nanofabrication, sensing, systems integration and application risk assessment
Methods
OBJECTIVE 1. Develop new technologies for characterizing fundamental nanoscale processes To successfully and safely exploit nanotechnology it is essential first to have quantitative bases for observing, understanding, and predicting nanoscale phenomena. Specific needs include a) characterization of nanofabrication methods; b) characterization of molecular and cellular interactions at the nanoscale; c) characterization of optical and other material properties of nanomaterials; d) new tools to manipulate cellular processes, and; e) study of the transport and fate of nanoparticles in the environment and in living tissues. Methods pertaining to aims (a) through (d) are outlined below, while specifics related to objective (e) are presented under Objective 4. Participants of NC-1031 propose to contribute to this knowledge base in the following ways: a) Characterization of nanofabrication methods. A variety of physical tools for nanofabrication and manipulation will be investigated. Work on this area will focus primarily on micro-patterning technologies (e.g. lithography and ultrasonic embossing) and compatible materials to prepare low cost, integrated functional systems. Systems will be designed to facilitate manipulation and analysis at the molecular scale in a self contained, low-power format. Collaborators in Indiana will investigate methods for miniaturization and integration of multiple systems including microscale power for battery-free operation of microdevices, taking advantage of sophisticated facilities and trans-disciplinary expertise at Purdue University. A primary objective of these collaborators is to develop microsystems capable of sustaining living cells in a nanobioreactor for inexpensive parallel biological analysis and experimentation. These capabilities will enrich our understanding of basic biological processes leading to more effective therapies in medicine and technologies for enhancing the value of agricultural and biological products. Researchers at other stations will develop integrated microdevices primarily for biomolecular manipulation and detection. These include miniaturized bioaffinity based pathogen-detection platforms based on optical interactions (Arizona, Arkansas, New York), as well as impedance and other electrochemical measurements (Arkansas, Michigan). Alternative approaches will develop integrated microsystems to miniaturize and accelerate gene-based detection such as PCR (Arizona). Alternative top down approaches to preparation of nanomaterials will rely on processing technologies such as two phase coaxial jet electrospraying to prepare formulations of nanomaterials with specific biological functionality (Nebraska). For example, nanoscale formulations of encapsulated bioactive compounds will be developed, and the effects of process variables like field strength, pressure, and material composition on the yield, activity, stability, release rates, structure, and surface characteristics of the formulation will be investigated. Approaches include organic and chemical synthesis, molecular self-assembly, and crystallization, and surface chemistry. Novel techniques will be developed and characterized primarily for developing nanomaterials suitable for use in biomolecular manipulation and detection. Examples include the preparation of crystalline nanowires to facilitate the processing of biological samples (Arkansas, Michigan, New York), nanostructured surfaces for self directed sample manipulation (Arizona), and functionalization of nanoparticle labels to enhance signals for optical (Arkansas, Indiana), electrochemical (Michigan), or inertial-based (Arkansas, Wisconsin) detection systems. b) Characterization of molecular and cellular interactions at the nanoscale One of the compelling possibilities of nanotechnology is the ability to manipulate interactions at the molecular level to achieve a desired outcome. This functionality can be harnessed for a variety of applications including molecular isolation, stabilization and manipulation of biological function, molecular targeting for medical therapies and targeted pesticides/ biocides, and highly selective and sensitive molecular recognition. A key area of research for this group will include development and characterization of molecular interactions at the nanoscale. In addition to using traditional molecular recognition elements such as antibodies, research will focus on developing novel processes and materials for molecular manipulation and detection. These include conformationally-gated or alignment-dependent molecules to detect specific binding events through mechanisms such as FRET or change of electron transfer characteristics (Arizona, Hawaii), manipulation of nanoparticle surface chemistries to facilitate analyte capture and isolation (Arizona, Arkansas, New York, Hawaii), adapting rapid combinatorial approaches to develop a new generation of selective molecular-recognition elements such as aptamers (Arkansas, Michigan), adaptation of novel processes of template dependent molecular replication for sensitive single step detection (Hawaii), reaction catalysis and visualization on functionalized nano-surfaces (Iowa), stabilized and controlled release nutraceutical formulations (Nebraska), and novel biocidal films and preparations exploiting microbial carbohydrate receptors (South Carolina). c) Characterization of optical and other material properties of nanomaterials For this task, collaborators will investigate material properties of nanomaterials that can be used to help monitor the transport and fate of nanomaterials in the biological systems they are deployed in as well as their transport in the environment. Primary activities include investigating optical interactions such as enhanced Raman scattering by metal nanoparticles (Indiana, Iowa) or plasmon interactions on a thin biofilm (Surface Plasmon Resonance or SPR) (Arkansas, Iowa), characterization of nanomaterial spectral signatures to allow multiplexed detection and background correction (Arkansas, Indiana, Iowa), characterization of nano-textured superhydrophobic surfaces and other nanomaterials for directed manipulation of samples (e.g., guided droplet manipulation for process sequencing, physical filtration or adhesion on nanowires) (Arizona, New York), characterization of magnetic properties to facilitate separation processes (Arkansas), and evaluation of the electrical characteristics of nanowires and other nanumaterials to facilitate molecular detection (Michigan, Arkansas). d) New tools to manipulate cellular processes Biological organisms and even simpler biological entities such as viruses have developed exquisite cascades of interdependent biochemical reactions and signals for replication/ reproduction, basic metabolic function, and response to biochemical and other stimuli. Whole cells have been used as reporters for environmental contaminants by monitoring cell viability or general metabolic indicators, but given the complexity and multiplicity of biochemical cascades and interactions within the cell this approach generally lacks the ability to specifically classify the nature of the contaminant or environmental stress. Recent advances in our understanding of this complex nano-machinery and our ability to manipulate signal pathways through recombinant DNA have revolutionized our capability to use living organisms to monitor specific biochemical compounds or monitor effects of certain biological interactions or environmental conditions on gene expression. Collaborators in Illinois will focus on developing and characterizing modular tools to engineer functional reporter cells sensitive to specific molecular events. These tools could also be exploited to produce microorganisms with other desirable characteristics such as stress tolerance, or new metabolic pathways to improve remediation of waste or biological conversion processes (e.g. for biofuel production or for use in a biological fuel cell). Engineering of positive and negative feedback cascades can also allow the development of biological equivalents of electrical circuits such as memory, pulse generators, or oscillators- elements that may prove practical for a variety of biological applications. e) Study of the transport and fate of nanoparticles in the environment and in living tissues This is a critical and increasingly important aspect of nanotechnology development, and has been considered under its own section (Objective 4). OBJECTIVE 2. Construct and characterize self-assembled nanostructures Polymeric nanoparticles are matrix systems of dense polymer networks in which bioactive components may be dispersed. Since the nanoparticles are submicron and sub-cellular in size, they provide distinct advantages for delivering biologically active compounds directly to individual cells. For example, protein-based particles are advantageous due to their biocompatibility, degradability, and chemical functionality, whereas polysaccharide-based materials may be more suitable for agricultural applications due to their relative stability in the environment. Controlled release of incorporated bioactive molecules can be controlled by alterations in the formulation conditions, such as loading concentration, pH of the protein solution, and concentration of cross-linking agent. We will investigate the development and characterization of the following classes of nanoparticle technologies: a) Nanoencapsulation. Currently, we are working on encapsulating vitamin C with zein to generate micro/nano capsules using two-phase coaxial jet electrospraying. The effects of electric field, physical properties and flow rates of the two immiscible solutions on capsule size, morphology, and physiochemical properties of the resulting particles will be investigated in the following years. The properties to be measured include apparent characteristics, surface characteristics, core-shell structure, yield, encapsulation efficiency, and controlled release. b) Self-assembled nano-structures. Materials such as natural spider silk, and biological hard tissues such as bones, sea-shells, teeth and spicules are produced in aqueous solution close to neutral pH at atmospheric temperature and pressure and exhibit properties that are better than many synthetics. A significant body of literature exists on crystal deposition and morphology. Most research has been dedicated to elucidating the formation of inorganic nanoparticles in saturated solutions and understanding the mechanisms of crystallization and mutual transformations. Participants from Wisconsin, Purdue and Minnesota will develop methodologies to elucidate such mechanisms by quantifying the kinetics, properties and process variability for such nanoscale self-assembly processes. c) Nanocomposites. Polymer-clay nanocomposites are a class of hybrid materials composed of organic polymer matrices and nanoscale organophilic clay fillers. Of nanoscale clays, montmorillonite (MMT) is of particular interest and has been studied widely. The inclusion of a small percentage, by weight, of clay in a polymer matrix significantly enhances the mechanical and thermal properties, the moisture stability and flame and weathering resistances. Chitosan derived from chitin possesses a unique cationic nature and has also been used in nanocomposites. Chitosan is nontoxic and compatible with living tissue and thus has potential use in healing, artificial skin, food preservation, cosmetics, and wastewater treatment. Developing chitosan layered silicate nanocomposites by inserting chitosan chains into interlayers of silicate can improve its mechanical properties. Nanoclays can be also used as sorbents for anionic, cationic and nonionic dyes. Dye sorption is an important parameter for coloration and wastewater control. Other nanoclay composites may be used as carrier systems for agriculturally relevant compounds (such as biopesticides) to increase their environmental stability. Project participants will continue to work on the sub tasks mentioned above as follows: 1) encapsulation of nanoparticles, (Arizona, Wisconsin) 2) self-assembled nano-composites (Purdue, Illinois), 3) nano-patterned materials (Illinois, Michigan, New York), and 4) PLA-nanoclay composites. (Minnesota, Nebraska) OBJECTIVE 3: Develop biological interfaces and sensing systems incorporating microfabrication and nanotechnology a) Development of nano-transducers and bio-recognition systems. Research activities will revolve around the development of nanoparticles, nanowires, nanobars, and nano-pillars. Specific activities include i) the design and development of electrically active magnetic nanoparticles and gold nanoparticles with uniformly structured size and properties (electrical and magnetic). We will develop novel and green approaches to the synthesis that will be bio-compatible and environmentally friendly. ii) Development of controlled interfacing techniques for biological (such as DNA, proteins and antibodies) and abiological materials (such as carbon nanotubes and nanoparticles). iii) Biofunctionalization of composite nanoparticles and materials with multiple functionalities for the development of DNA and protein sensors. iv) Conversion of existing fluorescence and chem/bioluminescence-based sensing recognition solution schemes into nanopatternable solid state materials. v) Identification of receptor molecules for the fabrication of biosensor. vi) Synthesis of metaloxide nano-patterned substrate for biosensor applications. vii) Use of antibody-DNA, aptamer-DNA and other DNA conjugates for production of a uniform RNA/DNA signal correlated to biological recognition of proteins, whole cells and DNA/RNA through bio-nano-transduction and viii) Development of nano-structured materials to be used as bio-mimetic transducers representing mammalian sensing capabilities (olfactory sensing, taste, touch etc.) b) Device design. Focus is on the design and fabrication of novel biosensor platforms using conventional and state-of-the-art fabrication facilities. Specific activities include, i) Fabrication of multi-array channels for simultaneous detection in one sample application using electrochemical and optical signal measurement. ii) Fabrication of deep devices in glass and plastic for cell transport and recovery as well as micro and nano-optical arrays for non-labeled sensing. iii) Development of nano and micro array patterned materials for fluorescent and luminescent based chemical and biological sensing of trace contaminants, toxins, and biological signaling molecules. iv) Development of a multi-component protein nanoarray (Also described in the previous objective). v) System design and protocol development. vi) Fabrication of devices incorporating field effect transistors for detection of molecular charge. vii) Fabrication of MEMS-based components to be integrated with nano-scale systems/devices and viii) Use of computational simulation and modeling techniques for rapid development and evaluation of MEMS/nano-scale devices. The institutions contributing to this objective are: Auburn University, Clemson University, Cornell University, Michigan State University, North Dakota State University, Purdue University, Rutgers University, University of Arizona, University of Arkansas, and the University of Idaho. OBJECTIVE 4: evelop a framework for economic, environmental and health risk assessment for nanotechnologies applied to food, agriculture and biological systems Engineered nanoparticles are increasingly finding applications in imaging-based diagnostics, intracellular monitoring, therapeutics, cosmetics and even food packaging. While there is great potential economic potential in exploiting the novel structural / electromagnetic / biological properties of engineered nanoparticles in these applications, there is also an increased concern surrounding our lack of understanding of the potential environmental and health impact. In addition to concerns about safe handling and disposal of these materials, there is also very little information available about the impact of an accidental discharge of nanomaterials into the environment. Previous studies about nanoparticle uptake were motivated primarily by the diagnostic and therapeutic applications of nanoparticles to human health. However, the emerging applications of nanotechnology in agricultural and environmental applications will require systematic studies to understand the scientific basis and empirical manifestations of the environmental impact of nanotechnology. One of the primary concerns about the developing nanotechnology industry is related to the uncertainty of the toxicity and fate of nanomaterials in the environment. In many instances, the small physical size of nanoparticles facilitates their uptake by living organisms and interaction with natural metabolites in the living system. However, the effects of these materials on biological organisms and the environment remain largely unknown. For this task, collaborators will use novel biological tools and instrumentation to monitor the transport and fate of nanomaterials in the environment, as well as to determine and understand toxicological and other effects of these materials on natural organisms and ecosystems. While fate and metabolism of natural molecules and organisms are well understood and constitute an essential step in the cycle of life, the fate of newly emerging nanomaterials and molecules are not sufficiently well understood. This is especially true for purely synthetic nanomaterials that do not exist or that exist in limited quantities naturally, but is also true of materials such as genetically modified organisms which may transfer engineered genes through natural recombination events, or other largely organic biomaterials such as proteins with unnatural amino acids or biopolymers labeled with synthetic materials/ chemicals. To better understand the fate of these materials, collaborators will study the mechanisms of dispersal in the environment as well as their interactions with living cells and other materials in the natural environment. Study of dispersion mechanisms will primarily focus on aerosolization of improperly contained particles, transport, leaching, and adhesion through soil, and uptake into microbial cells and animal tissues, and bioaccumulation through the food chain. To investigate the toxicological and other interactions of nanomaterials within cells, the effect of nanoparticles will be investigated on viability, growth rate, metabolism, gene expression, and phenotype of cell cultures ranging from basic unicellular organisms to animal tissues. The attached figure below outlines a schematic by which systematic and comprehensive analytical information obtained regarding various nanoscale technologies may be integrated into a framework for assessing the environmental risk for the benefit of public policy. Briefly, the quantitative risk of a nanotechnology can be assessed along three principal axes: a) the mobility of the nanomaterial through the environment (including movement through the ecosystem), b) the toxicity of the nanomaterial to living organisms and c) The stability of the nanomaterial as it moves through various partitions in living organisms as well as ecologies. Over the course of the next five years, we will structure our research activities around the following tasks: a) Development of technologies for interrogating the impact of nanotechnology on living organisms. The uncertainty behind the environmental impact of nanotechnology arises from the following premise: nanoscale components of a system have the potential to interfere with biological processes that also happen to operate at the nanoscale. Conversely, in order to study the mechanisms of interaction between nanoscale systems with the biological world, we will need to develop advanced nanotechnology-based biosensing and monitoring systems. The present NC-1031 committee includes experts on organic and inorganic nanoparticle synthesis, bioconjugation technologies to functionalize the nanoparticles, spectroscopic characterization techniques and high throughput biomolecular analysis. We propose to continue to develop and share such technologies and apply them to the analysis of the interactions between nanotechnology and living systems. b) Systematic studies of the environmental impact of industrially relevant nanotechnologies. In addition to developing a suite of physico-chemical analytical techniques, we also need to develop an ecologically-relevant understanding the impact of nanotechnology. Hitherto, most of the biological analysis of nanotechnologies (such as nanoparticles, nanomaterials, nanopharmaceuticals etc) has revolved around understanding the toxicity to humans in the course of development of nanodiagnostics and therapeutics. Similar, and equally comprehensive studies need to be performed on the environmental impact of nanotechnologies relevant to accidental and routine discharge of these materials into the environment in the course of their use and disposal. On classes of materials with high industrial relevance (such as quantum dots and carbon-based nanoparticles) we will develop biological assays to study the uptake, biodistribution, transformation and recycle of such nanoparticles in primarily aquatic and terrestrial microorganisms. Such microorganisms include cyanobacteria, which are relevant from an ecological and a bio-geo-chemical processes perspective. c) Dissemination of gained knowledge through various professional societies and publications. The present NC-1031 provides an opportunity for the highly multidisciplinary committee members to discuss advances in technology and in our understanding of nanoparticle systems. In the last five years, we held our committee meeting in conjunction with the Annual Meeting of the Institute for Biological Engineers. This allowed the participants to reach a broader audience for the purpose of collaboration and recruitment of new members. Additionally, members of the committee are active in a number of different professional committees including ASABE, AIChE, ACS, IFT, BMES, ISBBN etc, and journals associated with these societies We propose to continue to leverage these professional synergies, and to provide leadership in the application of biosensing and nanotechnologies to agricultural and environmental applications. Further, in recent meetings, we have considered to explore the feasibility of developing and publishing standards for research methodologies pertaining to nanotechnology, including the environmental impact of nanotechnology. d) Exploration into programs related to measuring the positive and negative impacts of nanotechnology on economic development. Among the tasks mentioned above, a number of the committee members already have active and funded research programs. They also participate actively in developing technologies in conjunction with the agricultural and pharmaceutical industries. An example of such an activity is the recent establishment of the Center for Agricultural and Pharmaceutical Nanotechnology, which is an NSF-funded, Industry/University Research Co-operation Center jointly housed at University of Illinois and Purdue University. Representatives from these states are not only participating, but providing leadership in this activity as well. However, the area of scholarship specific to the economic impact of nanotechnology is relatively underdeveloped compared to the other tasks within this objective. In order to increase the impact of the committee in this area, we will reach out and invite participation from researchers in the field of agricultural / consumer /environmental economics and engage them on issues related to the impact of nanotechnology. We will also reach out to Centers for Public Policy (e.g. The Woodrow Wilson Center for Public Policy in Washington, DC) and provide support on technical issues related to nanotechnology in order to advance the discussion on the economic and public impact of nanotechnology.Measurement of Progress and Results
Outputs
- Research articles in peer reviewed journals and publications, presentations at national and international meetings and workshops and patents.
- Course modules on different aspects of nanotechnology which are accessible to the educational community and public.
- Workshops and seminars on nanotechnology with proceedings of the workshops made available to public.
- Website which serves as a resource clearing house for educational communities and the public.
- Prototype nano-biosensors incorporating nanoparticles, nanowires, nanobars, nano-pillars, and nanopores
- Output 6 Prototype devices tested with bacteria, viruses, and toxins, chemicals, and other contaminants.
Outcomes or Projected Impacts
- Greater understanding of nanotechnology by the public and the agricultural and food industry.
- Increased awareness and application of nanotechnology to agricultural, food and biological systems.
- Increased number of students from land grant universities with training in the basic techniques of nanotechnology.
- Development of tools and products which exploit the novel properties of nanomaterials and nanoscale devices and benefit different aspects of agriculture, food safety and biological engineering research.
- Understanding of the fundamental nanoscale phenomena and processes in food and agricultural products as well as the processes that apply to these products.
- Outcome/Impact 6 Understanding of the potential of certain techniques and devices and what is needed to optimize and improve them within a theoretical context. Outcome/Impact 7 Development of nanoscale devices and systems that will advance the capabilities of currently designed devices for higher performance (sensitivity, speed of detection, and applications for example). Outcome/Impact 8 Development of a prototype bio-nanosensors including specifications for the design and synthesis of corresponding nanomaterials. Outcome/Impact 9 Development of nanoarrays for use in detecting and screening proteins from complicated expression systems (such as production of recombinant therapeutic proteins from mammalian cell culture or even from transgenic plants).
Milestones
Projected Participation
View Appendix E: ParticipationOutreach Plan
The general educational goal of NC1031 committee is to provide a framework for biosensor and bionanotechnology education to a broad audience of agriculture and food science students outside the traditional research community (physical science/engineering graduate students) that is supported by and integrated into the educational and outreach goals of our departments and institutions. We firmly believe that undergraduates will benefit from such an education, even those who will not pursue a scientific career. These students will be the backbone of our future workforce who may be responsible for handling agricultural products and/or food safety issues. Nanotechnology is continuing to have a profound impact over our society, by bringing new technical breakthroughs in material and biological, and by creating new environmental and ethical challenges related to its implementation in many biological applications. To manage these complex issues, we will need future generations to understand the technology in order to make educated decisions concerning its use. Courses on modern biosensors and nanotechnology are not widely available for agricultural and food sciences students in today's college curriculum, the NC1031 committee on biosensors and nanotechnology is uniquely positioned to address these educational needs and serve our students' best interests. Our educational program will target on achieving three goals: a) Pool the expertise of the committee members to work towards generating online classes that will bring nanotechnology and biosensors training to a broad audience of agricultural and food sciences students across campus boundaries. In future committee meetings, we will pool our expertise together to design new courses on nanotechnology and biosensors that are tailored to meet the needs of agricultural and food sciences students. We will create syllabus and teaching materials, and each member will contribute collectively to this effort. We will explore and take advantage of the existing long distance education programs in our institutions, and offer online courses to students across the country. One of the examples of the online education network is the AG*IDEA (www.agidea.org) consortium, to which many of our institutions are members (i.e., ISU, Purdue). It provides an excellent framework to develop and teach online courses in biosensors and bionanotechnology for students in agriculture across our campuses. All the technical support as well as administrative agreements and assistance are in place within the framework of the consortium for students to access online courses and gain credits. Another example is the NanoHUB (nanohub.org), a repository for educational and outreach materials for nanotechnology. Some participants (Illinois, Purdue) already host their courses on this web resource. With the logistic help through the AG*IDEA and similar long distance education services at our institutions, we will plan on developing two courses during the duration of the project to be taught online for students in all agriculture disciplines in our schools, as well as other universities who are interested in participating. We expect to have a major impact in educating our agricultural and food sciences students throughput the country. As of the courses to be developed, our first aim is to develop a biosensors course focusing on the principles and applications of modern biosensors in agriculture, food and biological engineering, for students in all member institutions through internet-based long-distance education. All of us will contribute to developing course materials, teaching and advising students eventually. Another course will be developed to focus on nanotechnology. Part of the bioengineering revolution we are experiencing now is due to the latest progress in nanotechnology. Novel nanomaterials and nano-scaled devices/structures are becoming prominent in agriculture and biotechnology. Therefore, it will be of great value to include nanotechnology training into the curriculum of agricultural and food sciences students. Our second aim is to develop a course in nanotechnology that will provide a broad coverage over the main aspects of bionanotechnology, including nanomaterials, nano-photonics and bioimaging, and nanofabrication. It will tailor-cut it to meet the needs of agricultural and food sciences students. All committee members will contribute to the discussion of the development of course materials and syllabus. Eventually we will create a collective structure to teach these courses online, and assure a high-quality learning experience for our students. b) Outreach to the general public Within the past several years, online video sharing/viewing has become a dominant component of pop and internet culture, particularly among youth, thanks in large part to the services of YouTube. This video sharing website allows ordinary users to share video clips, as well as view clips posted by others from around the world. According to the online marketing research company comScore, ~6.4 billion videos were viewed on YouTube in January 2009 alone, making it the leading online video sharing/viewing source in the U.S. The PIs will harness the incredible popularity and accessibility that YouTube commands to expose youth, particularly high school seniors and undergraduate students, as well as general public, i.e., farmers, food safety and public health personnel, to nano-biosensor education. This will be accomplished through the development of an online, video series called Nanosensor TV. Nanosensor TV segments will consist of webisodes (online video episodes) posted on YouTube. Each member of the committee will contribute at least one webisode. Each webisode will focus on a particular concept or application of a particular type of nano-biosensors and will be 5-10 minutes in duration. The maximum 10-minute length is standard for videos posted on YouTube, and has the benefit of retaining the attention span of a general audience. The webisodes will be primarily targeted to non-engineering/science high school seniors and undergraduate students, as well as people who are interested in learning about sensor technologies. The protagonist(s) in each webisode will be senior undergraduate students and beginning graduate students who participate in research at our labs. The committee members will help the student actors with writing, direction, and scientific content for each webisode, but the students will be free to execute the webisodes in a manner that they feel would best impact their younger peers. In order to facilitate learning, each webisode will have 2 main characters (students), one who serves as the voice of the viewer and asks the basic questions, and the other who answers. Occasionally, both characters will have to go to an expert to find an answer to a question. The PIs will serve as the Mr. Wizard characters for the students to go to. We will recruit a diverse cast to target a multicultural audience by also advertising in local student chapters of the National Society of Black Engineers and the Society of Women Engineers. Venues for these could vary, conceivable examples are student cafeteria, local café, committee members' labs, a pilot plant, or an open farmland. All webisodes will be made available on our institutional sites. Eventually they can be played on PBS public channel, if chosen.
Organization/Governance
NC-1031 is organized according to the guidelines in the USDA Multistate Research Manual, as found at http://www.wisc.edu/ncra/regionalmanual.doc. Membership includes an administrative advisor (Dr. Vincent Bralts), a CSREES representative (Dr. Hongda Chen) and project leaders from 12 cooperating stations. A Steering Committee was created at the 2010 Annual meeting with the specific goals to develop a renewal proposal consisting of representatives from Arizona, Hawaii, Illinois, Iowa and Michigan. Meetings are held annually, typically in October. The secretary for the coming year is elected at the end of each meeting. The previous secretary moves up to vice-chair and the vice-chair to chair. These three officers, the past chair, and the administrative advisor constitute the executive committee. The dates of the annual meeting (typically two or three days) are determined by the host and the chair after consulting the Committee at the preceding meeting. The chair develops an agenda for the upcoming meeting in consultation with the executive committee and the feedback from the membership. The annual meeting includes technical reports from each represented station, discussion of results and future areas of collaboration, and meetings among smaller ad hoc committees. The location of the meeting for the coming year is determined by vote at the end of each meeting. Meetings are typically held at or near a member station and hosted by the official representative of that station. Minutes are prepared by the secretary and sent to members. An annual report is prepared by the chair, submitted to the administrative advisor, and posted at the NC-1031 web site.
Literature Cited
Anderson, M., Zhang, D., and Alocilja, E.C., 2011. Spectral and electrical naniparticle-based molecular detection of Bacillus anthracis using co-polymer mass amplification. IEEE Transaction on Nanotechnology (accepted for publication). Anderson, J. M., Torres-Chavolla, E., Castro, A. B., and Alocilja, C. E., 2011. Novel alkaline synthesis of biocompatible gold nanoparticles using dextrin as reducing and capping agent. Journal of Nanoparticle Research (accepted for publication). Gore, A., Chakrabartty, S., Pal, S., and Alocilja, E.C., 2006. A multi-channel femtoampere-sensitivity potentiostat array for biosensing applications. IEEE Transactions on Circuits and Systems, 53(11), 2357-2363. Jin, J., Alocilja, E.C., and Grooms, D., 2010. Fabrication and electroanalytical characterization of label-free DNA sensor based on direct electropolymerization of pyrrole on p-type porous silicon substrates. Journal of Porous Materials, 17(2), 169-176. (DOI 10.1007/s10934-009-9277-4) Jin, J.H., Zhang, D., Alocilja, E.C., and Grooms, D., 2008. Label-free DNA sensor on nano-porous silicon-polypyrrole chip for monitoring Salmonella species. IEEE Sensors Journal, 8(6), 891-895. Kamikawa, T.L., Mikolajczyk, M.G., Kennedy, M., Zhang, P., Wang, W., Scott, D.E., and Alocilja, E.C., 2010b. Nanoparticle-based biosensor for the detection of emerging pandemic influenza strains. Biosensors and Bioelectronics, available online at http://dx.doi.org/10.1016/j.bios.2010.07.047 Kamikawa, T.L., Mikolajczyk, M.G., Kennedy, M., Zhong, L., Zhang, P., Setterington, E., Scott, D.E., and Alocilja, E.C., 2011. Pandemic influenza detection by electrically active magnetic nanoparticles and surface Plasmon resonance. IEEE Transaction on Nanotechnology (accepted for publication). Liu, Y., Chakrabartty, S., and Alocilja, E.C., 2010. Co-detection: ultra-reliable nanoparticle-based electrical detection of biomolecules in the presence of large background interference. Biosensors and Bioelectronics, 26(3), 10871092. Liu, Y., Chakrabartty, S., and Alocilja, E.C., 2007. Fundamental building blocks for molecular bio-wire based forward-error correcting biosensors, Nanotechnology Journal, 18(42), 424017 (6pp) Liu, Y., Gore, A., Chakrabartty, S., and Alocilja, E.C., 2008. Characterization of sub-systems of a molecular bio-based biosensor device. Microchimica Acta, 163(1-2), 49-56. Liu, Y., Zhang, D., Alocilja, E.C., and Chakrabartty, S., 2010. Biomolecules Detection using a Silver-Enhanced Gold Nanoparticle-Based Biochip. Nanoscale Research Letters, 5(3), 533-538. DOI: 10.1007/s11671-010-9542-0. Luo, Y., Nartker, S., Miller, H., Hochhalter, D., Wiederoder, M., Wiederoder, S., Setterington, E., Drzal, L.T., and Alocilja, E.C., 2010. Surface Functionalization of Electrospun Nanofibers for Detecting E. coli O157:H7 and BVDV cells in a Direct-Charge Transfer Biosensor. Biosensors and Bioelectronics, available online at http://dx.doi.org/10.1016/j.bios.2010.08.028 Muhammad-Tahir, Z. Alocilja, E.C., and Grooms, D.L., 2007. Indium Tin Oxide-Polyaniline Biosensor: Fabrication and Characterization. Sensors, 7, 1123-1140. Okafor, C., Grooms, D., Alocilja, E. Bolin, S., 2008. Fabrication of a Novel Conductometric Biosensor for Detecting Mycobacterium avium subsp. paratuberculosis Antibodies. Sensors, 8(9), 6015-6025 (http://www.mdpi.org/sensors/papers/s8096015.pdf) Pal, S. and Alocilja, E.C., 2010. Electricallyactive magnetic nanoparticles as novel concentrator and electrochemical redox transducer in Bacillus anthracis DNA detection. Biosensors and Bioelectronics, available online at http://dx.doi.org/10.1016/j.bios.2010.08.035 Pal, S. and Alocilja, E.C., 2009. Electrically-Active Polyaniline Coated Magnetic (EAPM) Nanoparticle as Novel Transducer in Biosensor for Detection of Bacillus anthracis Spores in Food Samples. Biosensors and Bioelectronics, 24(5), 1437-1444 (http://dx.doi.org/10.1016/j.bios.2008.08.020) Pal, S., Setterington, E., and Alocilja, E.C., 2008. Electrically-Active Magnetic Nanoparticles for Concentrating and Detecting Bacillus anthracis Spores in a Direct-Charge Transfer Biosensor. IEEE Sensors Journal, 8(6), 647-654. Pal, S., Ying, W., Alocilja,E.C., Downes, F.P., 2008. Sensitivity and Specificity Performance of a Direct-Charge Transfer Biosensor for Detecting Bacillus cereus in Selected Food Matrices. Biosystems Engineering, 99(4), 461-468 Pal, S., Alocilja,E.C., Downes, F.P., 2007. Nanowire Labeled Direct-Charge Transfer Biosensor for Detecting Bacillus species. Biosensors & Bioelectronics, 22(9-10), 23292336. Setterington, E.H. and Alocilja, E.C., 2010. Rapid electrochemical detection of polyaniline-labeled Escherichia coli O157:H7. Biosensors and Bioelectronics, available online at http://dx.doi.org/10.1016/j.bios.2010.09.036. Shi L, S Gunasekaran. 2008. Preparation of pectin-ZnO nanocomposite. Nanoscale Research Letters 3(12): 491-495. Torres-Chavolla, E., Ranasinghe, R.J., and Alocilja, E.C., 2010. Characterization and functionalization of biogenic gold nanoparticles for biosensing enhancement. IEEE Transaction on Nanotechnology, 9(5), 533-538. Tran PL, Gamboa JR, You DJ, Yoon JY. 2010. FRET detection of octamer-4 on a protein nanoarray made by size-dependent self-assembly. Analytical Bioanalytical Chemistry 398(2): 759-768. Xu, Y.X., and Hanna, M.A. 2008. Morphological and structural properties of two-phase coaxial jet electrosprayed BSA-PLA capsules. Journal of Microencapsulation 25(7): 469-477. Yuk, J.S., Jin, J.H., Alocilja, E.C., and Rose, J.B., 2009. Performance enhancement of polyaniline-based polymeric wire biosensor. Biosensors and Bioelectronics, 24(5), 1348-1352. (http://dx.doi.org/10.1016/j.bios.2008.07.079) Yuk, J.S., Rose, J.B., and Alocilja, E.C., 2010. Characterization of polyaniline-coated magnetic nanoparticles for application of a disposable membrane strip biosensor. European Physical Journal of Applied Physics, 50(1), 11401. Zhang, D., Carr, D.J., and Alocilja, E.C., 2009. Fluorescent bio-barcode DNA assay for the detection of Salmonella enterica serovar Enteritidis. Biosensors and Bioelectronics, 24(5), 1377-1381 (http://dx.doi.org/10.1016/j.bios.2008.07.081) Zhang, D. and Alocilja, E.C., 2008. Characterization of nano-porous silicon-based DNA biosensor for the detection of Salmonella Enteritidis. IEEE Sensors Journal, 8(6), 775-780. Zhang, D., Huarng, M.C., and Alocilja, E.C., 2010. A Multiplex Nanoparticle-based Bio-barcoded DNA Sensor for the Simultaneous Detection of Multiple Pathogens. Biosensors and Bioelectronics, available online at http://dx.doi.org/10.1016/j.bios.2010.08.012 Zuo, Y., Chakrabartty, S., Muhammad-Tahir, Z., Pal, S., and Alocilja, E.C., 2006. Spatio-Temporal Processing for Multichannel Biosensors using Support Vector Machines. IEEE Sensors Journal, 6(6), 1644-1651.