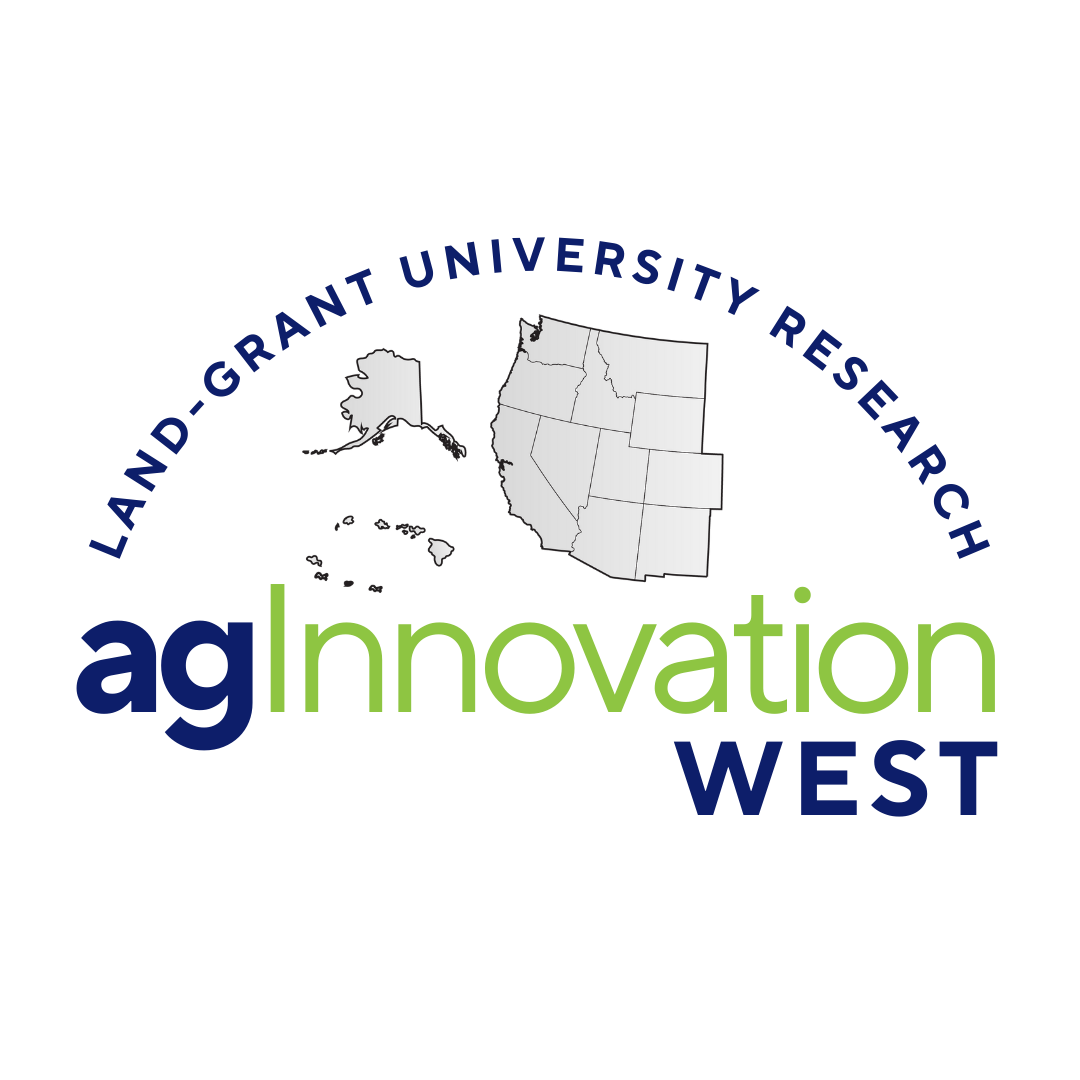
W2150: Breeding Common Bean (Phaseolus vulgaris L.) for Resistance to Abiotic and Biotic Stresses, Sustainable Production, and Enhanced Nutritional
(Multistate Research Project)
Status: Inactive/Terminating
W2150: Breeding Common Bean (Phaseolus vulgaris L.) for Resistance to Abiotic and Biotic Stresses, Sustainable Production, and Enhanced Nutritional
Duration: 10/01/2010 to 09/30/2015
Administrative Advisor(s):
NIFA Reps:
Non-Technical Summary
Statement of Issues and Justification
STATMENT OF THE PROBLEM: Common bean (Phaseolus vulgaris L.), introduced to North America by both the Native Americans and settlers from Mexico, Central America, and South America, has been cultivated in the U.S. for millennia. However, compared to corn, soybean, and wheat, the average national seed yield of dry bean is low (~1700 lbs/acre). This may suggest that some market classes suffer from a narrow genetic base, or show some degree of inbreeding depression. In addition, several abiotic and biotic stresses can severely limit both dry and snap (vegetable form) bean production. Fungal, bacterial, and viral diseases are among the main production constraints (Beaver and Osorno, 2009, Schwartz et al., 2005), whereas drought, heat, soil mineral deficiencies, and short growing seasons, reduce productivity in many production areas. In order to remain competitive with other crops produced in the U.S., these production constraints need to be addressed. In spite of these limitations, beans are one of the most nutritious and healthiest foods, being the only item included into two different food groups in the USDA food pyramid (Britten et al., 2006; Leterme, 2002; Mitchell et al., 2009; Thompson et al., 2009; Tietyen-Clark, 1986). In addition to human health, beans promote soil and environmental health through biological fixation of atmospheric nitrogen which allows beans to be produced with less N-fertilizer than other crops. Several diseases can be present simultaneously and reduce dry and snap bean yield and quality within and across different production regions. Yield losses can range from 10 to 90%, depending on the diseases involved and severity. For example, in the Western U.S., Beet curly top virus (BCTV), Bean common mosaic virus (BCMV), Fusarium root rot (caused by Fusarium solani f.sp. phaseoli) and wilt (caused by Fusarium oxysporum f.sp. phaseoli), and white mold (caused by Sclerotinia sclerotiorum), can simultaneously attack susceptible cultivars. Similarly, in Michigan, Minnesota, North Dakota, and Wisconsin, anthracnose (caused by Colletotrichum lindemuthianum), bacterial brown spot [caused by Pseudomonas syringae pv. syringae (Psp)], BCMV, common bacterial blight [caused by Xanthomonas axonopodis pv. phaseoli (Xcp) and X. axonopodis pv. phaseoli var. fuscans (Xcpf), Syn. with X. campestris], halo blight (caused by Pseudomonas syringae pv. phaseolicola), root rots (caused by a fungal complex in most cases), rust (caused by Uromyces appendiculatus), and white mold can occur together and cause severe yield losses. The root rot pathogens cause serious problems in snap beans across all production regions. In addition, snap beans are vulnerable to regional epidemics of virus diseases including Beet Mild Curly Top Virus (BMCTV) in the western states (e.g., California, Idaho and Washington), and a new virus complex in the Great Lakes states which includes Alfalfa mosaic virus (AMV), Cucumber mosaic virus (CMV), Bean yellow mosaic virus (BYMV), and Clover yellow vein virus (ClYVV), among others. Many of these pathogens are highly variable and new races or strains can appear in different regions. A recent example is the new rust races reported in Michigan and North Dakota (Markell et al., 2009, Wright et al., 2008). Furthermore, the introduction of soybean rust into the southeastern U.S. has serious potential implications for dry and snap bean as they are susceptible to the soybean rust pathogens, Phakopsora pachyrhizi and P. meibomiae. Many of these diseases are seed-borne, are caused by genetically variable pathogens, and cannot be economically controlled with chemicals (e.g., common bacterial blight). Fungicides increase production costs and can result in environmental and human health hazards if improperly used. As shown in several studies, the genetic base of dry and snap bean cultivars within most market classes in the United States is narrow (McClean et al., 1993; Miklas, 2000; Silbernagel and Hannan, 1992; Sonnante et al., 1994), because only a very small number of wild bean ancestors were domesticated (Gepts et al., 1986; Papa and Gepts, 2003; Kwak et al., 2009). Consequently, useful traits such as resistance to bruchids (Zabrotes subfasciatus) are not found in cultivars (Schoonhoven et al., 1983) supporting the evidence that a large reduction in genetic diversity occurred early during domestication (Gepts et al., 1986; Koenig et al., 1990). Resistance to heat, drought, and diseases such as common bacterial blight and white mold are inadequate in most cultivars grown in the U.S, thus new sources of resistance are needed in order to broaden the genetic base of common beans in the U.S. and in order to provide broader resistance to highly variable pathogens. The conversion of germplasm is important in order to make traits available in photoperiod sensitive, non-adapted materials. Stringent requirements in terms of visual seed quality and canning quality for each market class slow genetic improvement (Singh, 1999). This interdisciplinary, multi-state, collaborative W-1150 project proposal comprises several complementary sub-projects (see Appendix Table 1). Key collaboration among participants in these sub-projects is designed to achieve our overall goals and objectives of developing high yielding cultivars with enhanced culinary and nutritional qualities and resistance to major abiotic and biotic stresses. These cultivars will help reduce production costs and pesticide use, increase yield and competitiveness of the U.S. bean growers, and sustain production for domestic consumption and export. Researchers participating in each sub-project have complementary expertise and represent two or more institutions. This research scheme has been very successful as evidenced by the "Excellence in Multistate Research Award" given to the W1150 multistate project by the Western Association of Agricultural Experiment Station Directors (WAAESD) in March of 2009. The inclusive group of researchers jointly prepared the project renewal and is committed to collaborating with each other to achieve the overall objectives. For simplicity, these projects are grouped into the following priorities: (1) biotic stresses, (2) abiotic stresses and production/sustainability, (3) nutritional and health related benefits in the human diet, and (4) characterization/utilization of exotic germplasm. Details of each sub-project listed in the Appendix 1 can be provided upon request. JUSTIFICATION: Why the need for a multi-state collaborative project? Many of the production problems are common to more than one region in the United States. Therefore, collaborative research promotes efficiency (less time and resources in some cases), faster genetic progress, and avoids duplication of work that may lead to the same results. With collaboration, there are mutual benefits for each research program including conservation of economic and physical resources and also expansion of the impact of some outputs. For instance, new cultivars with wider adaptation and broader resistance provide growers with a stable crop, more resistant to pathogen variability and environmental fluctuations that occur year to year. Moreover, dry and snap beans may share production constraints, and a project such as this one encourages communication and collaboration between dry and snap bean breeders. Ultimately, the whole bean industry (both seed and food) benefits from the new science and products developed by this project. Specific examples describing the needs for this multistate collaborative project and the benefits derived are described below. Anthracnose, begomoviruses, curtoviruses, halo blight, rust, and other diseases caused by highly-variable and/or emerging pathogens, require extensive investigation, including the development of screening methods and multi-location field and greenhouse environments. Most private and public cultivars are grown in multiple states and thus require multi-state trials for cultivar development. White mold, for example, requires field and greenhouse trials from multiple locations for the identification of avoidance and physiological resistance with any degree of assurance. It is therefore essential to continue to characterize and monitor genetic variability of bacterial, fungal, and viral pathogens causing major bean diseases in the United States. Also, it is imperative to determine the reaction of useful germplasm to the pathogenic diversity so breeders can identify additional resistance genes and mechanisms for broadening the genetic base and development of improved cultivars. Introgression and pyramiding of favorable alleles and QTL from across races, gene pools, and related wild and cultivated Phaseolus species into cultivars is often achieved only through a stepwise tiered breeding approach that often involves introgression of useful genes from wild or unadapted germplasm into adapted cultivars for the temperate regions of North America (Kelly et al., 1998; Singh, 2001; Singh et al., 2007b; White and Singh, 1991). Most researchers often work within one or two tiers, and depend on other collaborators for the first step of gene introgression (Kelly et al., 1998). Furthermore, the role of genomics and marker-assisted selection as an additional tool for bean breeders is becoming increasingly important (Miklas et al., 2006a) and requires collaborations among scientists across different states or countries (McClean et al., 2008; Gepts et al., 2008). Inter-disciplinary and inter-institutional collaborative research must also continue to find alternative recombination and selection methods and identify and use molecular markers to facilitate efficient introgression and pyramiding of favorable alleles and QTL into improved cultivars for diverse cropping systems. Thus, to develop germplasm and cultivars with multiple-disease resistance and tolerance to abiotic stresses, researchers with limited expertise and facilities share responsibilities and exchange segregating populations and breeding lines to complement screening and selection in contrasting field environments, laboratories, and greenhouses regionally and nationally. The use of winter nurseries in Puerto Rico accelerates the development of breeding lines and expedites the conversion of useful tropical and sub-tropical germplasm that are poorly adapted to the U.S. bean growing environments. Breeding populations can be rapidly developed from crosses between adapted x exotic germplasm, followed by backcrossing in the short-day photoperiods of the tropics (e.g., Mayaguez, PR) or in the greenhouse during the winter months. Furthermore, hybridization in the tropics is often alternated by selection for photoperiod insensitivity on the U.S. mainland during the growing season. Because exotic germplasm is increasingly being used to broaden the genetic base and develop cultivars with higher yield potential, enhanced nutritional quality, and greater resistance to abiotic and biotic stresses, it is essential to evaluate advanced breeding lines and cultivars developed from the conversion process across production regions, in order to select for broad adaptation and stability of performance. Regional and national germplasm development and testing are also important because only one growing season per year is feasible in the continental U.S. In addition, the W-1150 project runs every year several multi-location testing trials such as the Bean Rust Nursery (BRN), national Cooperative Dry Bean Nursery (CDBN), Midwest Regional Performance Nursery (MRPN), White Mold Nursery (WMN), and Western Regional Bean Trial (WRBT). These nurseries are essential to identify high yielding broadly adapted cultivars and breeding lines with durable disease resistance, to estimate genetic progress over time, and to detect pathogen diversity in the shortest time possible. Therefore, these nurseries form an integral part and foundation for strong collaborative efforts within the W-1150 project. No single state or institution can conduct all the research necessary to develop improved bean cultivars for sustainable production, consumption, and export. This is especially true when most programs have inadequate resources and personnel to carry out a relevant and efficient breeding program for their own state. Certain expertise is available in a few states (e.g. nutritionists and pathologists), but there are some bean-producing states (e.g., Arizona, Florida, Montana, New Mexico, and Wyoming) that do not have public dry or snap bean breeding programs. Due to the collaborative nature of the W-1150 project, researchers in these states also will have access to new breeding lines and cultivars of all market classes. Moreover, research and outreach efforts of agronomists, breeders, molecular geneticists, food scientists, human nutritionists, and plant pathologists must be coordinated to improve domestic consumption and export. Thus, additional resources and multi-state regional and national collaboration are essential to ameliorate the effects of major abiotic and biotic constraints, and food quality problems that currently limit the seed yield potential, domestic consumption and export of dry and snap bean. This comprehensive, multidisciplinary, and multi-state collaborative project is essential to maintain, monitor, and exchange pathogens, parental stocks and improved breeding lines and cultivars, to share research data among all related areas, and to allow a more efficient use of exotic germplasm. The accomplishments for this project during the last funding cycles have been well documented in numerous publications and recognized by other scientists (i.e. WAAESD Excellence Award). The collaborative project offers a broad range of selection environments whereby researchers can share and complement findings and advances. Moreover, a coordinated, multidisciplinary effort will allow the efficient shared use of genetic resources, avoid duplication of research, and maximize efforts to increase bean production, consumption, and export. The W-1150 team includes both young and experienced scientists, which provides a good balance between new cutting edge technologies, but also the expertise and results gained through years of scientific work. Long-term collaboration among a multi-disciplinary group of scientists enables the multi-state W-1150 project to conduct core research activities and to possess the ability to rapidly address new challenges identified by stakeholders. Based on this feedback from stakeholders, the W-1150 group proposes to continue to enhance genetic resistance to biotic and abiotic stresses. Exotic bean germplasm needs to be characterized and utilized to broaden the genetic base of the crop. Improved nutritional and quality traits promise to enhance the health benefits and utilization of beans. Improved agronomic/production practices should lead to more efficient and sustainable bean production systems.
Related, Current and Previous Work
The following is a list of the most important accomplishments and current research activities of the W-1150. Refer to Appendix 1 and preceding annual reports for more specific details on previous research activities and results. Biotic Stresses: Common bacterial blight: Characterization of Xanthomonas axonopodis pv. phaseoli (Xap) isolates using PCR-based probes and host response continues. The Xap (non-fuscans) and Xanthomonas axonopodis pv. Phaseoli var. fuscans (Xcpf) strains are separable into genetically distinct bacteria (Mkandawire et al., 2004; Lopez et al., 2006; Duncan et al., 2008a). For the first time, a major gene conditioning resistance to specific strains of Xap is being confirmed (Zapata et al., 2009). The SU91 QTL on LG 8 appears to be the most important QTL with the effect of other QTL dependent upon its presence. A combination of traditional and marker-assisted breeding has been used to transfer the major QTL from exotic sources into the major dry bean market classes (Mutlu et al., 2005a), resulting in the release of many advanced lines and cultivars with improved levels of CBB resistance. Halo blight/Bacterial Brown Spot: The last survey of Psp conducted by Lamppa et al. (2002) revealed Race 6 as the most prevalent race in North Dakota. Duncan et al. (2008b) identified and characterized resistance to Race 6 in the old pinto cultivar US 14, which was conditioned by two independent recessive genes. Miklas et al. (2009) tagged and mapped the Pse-1 gene, which conditions resistance to Psp races 1, 5, 7, and 9, to linkage group 10. Recent severe outbreaks of halo blight in some production areas (e.g. Wyoming), emphasizes the need to renew pathogen variability surveys, and to continue breeding efforts to deploy and enhance resistance to this disease. Bacterial Wilt: The pathogen (Xanthomonas axonopodis pv. phaseoli) is a frequent problem in states such as Colorado and Nebraska. This pathogen is considered an A2 quarantine pest in Europe and is subject to phytosanitary regulations in some countries (EPPO/CABI, 1997). Research during the last 5 years has focused on limited evaluation of commercial cultivars, germplasm and selections from the USDA-GRIN Core Collection against local isolates of the bacterial pathogen from Nebraska (R. Harveson and C. Urrea) and Colorado (H. Schwartz and M. Brick). Results have been variable and may reflect differences in pathogenic isolates, environmental conditions, inoculation methodology, etc. Previously reported resistant great northern Emerson and PI 165078 (Coyne and Schuster, 1976), were consistently resistant in Colorado tests with yellow, orange, and purple isolates of the pathogen (Schwartz et al., 2009b). Inheritance of resistance has been variable (quantitative, recessive) in earlier research (Coyne and Schuster, 1971); but there is no additional information on inheritance in recent cultivars and lines such as Resolute (great northern), Agrinto (pinto) and Early Rose (pink) with resistance to bacterial wilt in Canada (Huang et al., 2007). Geminiviruses: Prominent, whitefly-transmitted Begomoviruses currently threatening bean production (Brown, 1994; Brown et al., 1999; Faria et al., 1994; Garrido-Ramirez et al., 2000; Gilbertson et al., 1993; Idris and Brown, 1998) include Tomato yellow leaf curl virus from Israel, which was recently introduced in the U.S. Sunbelt States and the Caribbean Basin (Bird et al., 2001; Nahkla et al., 1994); Bean golden mosaic virus (BGMV) prominent in S. America; Bean golden yellow mosaic virus (BGYMV) and relatives prevalent in the Florida, the Caribbean Basin and Central America. Resistance to BGYMV has been well characterized by W-1150 researchers who have identified numerous genes, bgm-1, bgm-2, and bgm-3, which reduce leaf chlorosis (Osorno et al., 2007; Velez et al., 1998), and the Bgp-1 gene which prevents pod deformation (Acevedo-Román et al., 2004). Dominant resistance conditioned by Bdm-1 gene, widely distributed within the Middle American gene pool, blocks the long-distance movement of BDMV (Seo et al., 2004). Deployment of resistance using MAS (Beaver and Miklas, 1999; Urrea et al., 1996; Singh et al., 2000) and traditional breeding combined with IPM strategies significantly controlled the threat of BGYMV in the Caribbean Basin and elsewhere. The University of Puerto Rico released numerous cultivars with resistance to BGYMV (Beaver et al., 2008; Blair et al., 2006; Beaver et al., 2005, Rosas et al., 2005). BGYMV is no longer an important bean disease in Puerto Rico after the deployment of resistant cultivars (Osorno et al., 2007). However, BGYMV continues to cause significant yield loss in the Dominican Republic and Haiti where most bean cultivars are susceptible to BGYMV. Potyviruses. The seed-borne Bean common mosaic virus (BCMV) and related Bean common mosaic necrosis virus (BCMNV) are the most economically important potyvirus diseases of bean worldwide. Other potyviruses problematic in bean include Bean yellow mosaic virus (BYMV), Clover yellow vein virus (CLYVV), and Peanut mottle virus (PeMoV). Some of these potyviruses, namely ClYVV, contribute to the virus disease complex along with Cucumber mosaic virus (CMV) and Alfalfa mosaic virus (AMV) which has plagued snap bean production in the Great Lakes region since 2001 (Grau et al., 2002; Larsen et al., 2002). The host-virus interaction is well defined for BCMV and BCMNV. Markers have been generated for the dominant I gene, and strain specific recessive resistance genes bc-12 and bc-3, and then successfully applied toward MAS of improved germplasm (Vandemark and Miklas, 2005; Miklas et al., 2006b). Rust: Two new races of rust have been recently reported in Michigan and North Dakota. The new races have reoccurred in Michigan since 2007 and in North Dakota since 2008. Preliminary results are showing that both races are similar, but not identical (Markell et al., 2009). Resistance to both races is conditioned by the Ur-5, Ur-11, and CNC genes. A new source of resistance was mapped to LG 4 near the Ur-5 and Ur-Dorado108 loci in black bean populations derived from Tacana (Wright et al., 2008, 2009). Several new cultivars with different combinations of rust resistance genes have been released. Salient among these are six unique great northern bean germplasm lines named BelDakMi-RMR-8, to -13. These are the first great northern beans that combine four genes for rust resistance and two genes for resistance to the two bean common mosaic potyviruses. These beans combine two Andean (Ur-4 and Ur-6) and two Middle American (Ur-3 and Ur-11) rust resistance genes (Pastor-Corrales et al., 2007). Other rust resistant cultivars include great northern bean cultivars ABC-Weihing (Ur-3 and Ur-6) (Mutlu et al., 2008), and Coyne (Ur-3 and Ur-6) (Urrea, et al., 2009a), and Pinto CO46348 (Ur-4 and Ur-11) (Brick et al., 2008). In the case of soybean rust, the common bean lines Compuesto Negro Chimaltenengo (CNC) and PI 181996 were among the most resistant to all six isolates. Inheritance of SBR resistance in CNC was studied by crossing Mx309/CNC. Based on severity, the segregation for SBR resistance in the F2 population fit a 9 resistant to 7 susceptible ratio. White mold. There have been many incongruities between field and greenhouse evaluations, and results obtained across locations (Otto-Hanson and Steadman, 2007). The efficacy of three S. sclerotiorum inoculation methods, namely, infected oat seeds (IOS), detached infected flowers (DIL), and cut-stem (CS) was compared in the greenhouse (Terán and Singh, 2009a). The IOS method was least effective in separating among resistant, intermediate, and susceptible dry bean genotypes. While the DIL and CS methods were equally effective, it took twice as long to inoculate each plant with the DIL compared to the CS method. Partial white mold resistance was found in P. coccineus (Gilmore et al., 2002), and in other secondary gene pool species (Singh et al., 2009a) and common bean accessions (Grafton et al., 2002; Steadman et al., 2001). White mold resistance was derived from congruity backcrossing with a P. coccineus accession and from recurrent backcrossing with a P. costaricensis accession (Singh et al., 2009a, b). Griffiths et al. (2004) developed a snap bean breeding line Cornell 501 with partial resistance. Light red kidney, dark red kidney and black bean breeding lines were developed primarily by selection in greenhouse tests using snap bean lines NY 6603 and NY6333 (Griffiths, 2009). A highly resistant dry bean breeding line A 195, identified by Singh et al. (2007a), and the USPT-WM-1 pinto breeding line with ICA Bunsi derived resistance released by Miklas et al. (2006a) have performed well in the national white mold nursery as well. Ender and Kelly (2005) detected QTL located on linkage groups B2, B5, B7, and B8 for white mold resistance in an ICA Bunsi/Raven dry bean population. Miklas et al. (2007) identified two QTL located on linkage groups B2 and B3 conditioning ICA Bunsi-derived resistance. Maxwell et al. (2007) identified five QTL for greenhouse resistance on linkage groups B1, B2, B8, and B9, and one QTL in G 122/CO72548 dry bean population. Marker-assisted selection for select white mold resistance QTL shows promise as demonstrated by Miklas et al., (2007) and Ender et al. (2007). Root rots: Root rot of dry bean is a yield-limiting disease problem for growers in the North-Central region of the U.S. (Bradley and Luecke, 2004). In North Dakota and Minnesota, Fusarium solani was considered to be the most common causal agent of root rot followed by Rhizoctonia solani (Venette and Lamey, 1998). However, recent findings have highlighted the ability of other Fusarium species to cause root rot in dry beans (Bilgi et al., 2007; Gambhir et al., 2008). Little is known about the prevalence and virulence of the four subspecies of Rhizoctonia solani that are found on common bean. Crops grown in rotation with beans, such as sugar beets, are also hosts for R. solani. Cramer et al. (2003) found low genetic diversity among 166 isolates of the Fusarium wilt pathogen from the U.S. Central High Plains using RAPD markers. Resistance to Fusarium wilt in race Durango dry beans CO 33142 and Fisher were controlled by a single dominant gene, whereas polygenic control (h2 ranged from 0.25 to 0.60) was found for resistance in race Mesoamerica cultivars Rio Tibagi and Jamapa (Cross et al., 2000; Velasquez and Schwartz, 2000). In addition, limited research has been conducted on Aphanomyces euteiches f.sp. phaseoli, but this fungus occurs frequently in the sandy soils in the Upper Midwest. Anthracnose. Two new sources of anthracnose resistance within the Andean gene pool were identified in germplasm from Brazil (Gonçalves-Vidigal et al., 2008, 2009; Vidigal et al., 2007). The two independent genes were identified as Co-12 in Jalo Vermelho and Co-13 in Jalo Listras Pretas and represent unique resistance patterns. These are significant findings as the multiallelic Co-1 locus with five alleles was the only resistance sources previously known in Andean germplasm. This is particularly important given the recent breakdown of the Co-12 gene by race 105 in Manitoba. The rapid evolution of this new race underscores the need to monitor the pathogenic variability in different production areas. The availability of new resistance genes of Andean origin offers breeders more choices for pyramiding genes with the more common Middle American resistance sources. Abiotic Stresses: Heat tolerance. High average maximum daytime (> 30°C) and minimum nighttime (> 20°C) temperatures can significantly affect common bean yields (Singh, 1995). Considering rising temperatures due to global warming, heat tolerance needs to play an increasingly important role in common bean breeding programs. Collaborative work within the W1150 has resulted in improved selection techniques focused on tolerance to high temperatures during reproductive development (Porch, 2001; 2006), the identification of improved sources of heat tolerance for breeding (Rainey and Griffiths, 2005a), the elucidation of the genetics of heat tolerance (Porch et al., 2004; Rainey and Griffiths, 2005b), and the generation of improved common bean cultivars (Beaver et al., 2008). Due to the coincidence of high temperature stress with other abiotic and biotic stressors, current breeding work has focused on the pyramiding of multiple stress tolerance in several major market classes. Drought tolerance. Early genetic studies found that drought tolerance, measured as yield, was an additive trait that interacted with the environment (White et al., 1994). A wide range of heritabilities (0.09 to 0.80) were reported depending on environmental conditions and market class of the bean lines evaluated (Schneider et al., 1997; Singh, 1995). A few cultivars, such as Matterhorn (Kelly et al., 1999), have been released as drought tolerant in the U.S., but other lines with tropical and/or temperate adaptation have previously been identified with drought tolerance including: A686, Negro Vizcaya, L88-63, B98311, G21212, SEA 5, SEA 15, SEN 10, SEN 21, SER 16, SER 21, SER 22, Pinto Villa, RAB 651, RAB 655, Viva, G 13637, San Cristobal 83, ICA Palmar, LEF 2RB, and AC 1028. Exotic germplasm evaluation and conversion has played an important role in the incorporation of new traits into U.S. germplasm. The cultivars Beryl and Matterhorn and CIATs line SER 22 showed the highest yield under non-irrigated and irrigated conditions (Urrea et al., 2009b). In another study in 2007 and 2008, 29 drought-tolerant tropical germplasm lines were evaluated under non-stress and drought stress conditions in Puerto Rico, leading to the identification of superior genotypes for breeding and genetic analysis (Porch et al., 2009b). Adapted germplasm from the U.S. and CIAT core germplasm collections, elite U.S. cultivars, and advanced breeding lines were also evaluated in Nebraska and Puerto Rico under drought stress and non-stress conditions, and about 300 genotypes were determined to be photoperiod insensitive and adapted (Urrea and Porch, 2009). Nutritional Value. Type 2 diabetes, and cardiovascular diseases in the U.S. could be prevented or alleviated by increasing bean consumption (Anderson et al., 1984; Tietyen-Clark, 1986). Even more, some studies suggest that the consumption of beans can delay the onset of symptoms of HIV in patients (Wong et al., 2006).Therefore, identification and promotion of the health-benefits of dry beans is extremely important. The health benefits of beans was recently underscored by the funding by USDA-NIFA of $4 million for a Coordinated Agricultural Project (BeanCAP) to support research on the discovery of genes related with nutritional and health traits in beans. Current research in bean nutrition and quality includes the Cornell evaluation of 400 to 500 lines and cultivars each season for yield and processing quality. The relative ability of bean extracts to inhibit lipid peroxidation was ranked by color class, with red beans having the highest value, followed by brown, black, and white (Beninger and Hosfield, 2003). Antioxidant activity was most strongly related to quantity of condensed tannins (proanthocyanidins). Beans devoid of condensed tannins had low antioxidant activity. In addition, Fe and Zn bioavailability was evaluated using in vivo studies with pigs fed a 37% bean diet of either white or red bean cultivars. It was concluded that bean color does not affect Fe or Zn bioavailability in vivo and that beans are a good source of bioavailable Fe and Zn (Tako et al., 2009). One of the major factors contributing to the difficulty digesting beans is the raffinose and stachyose oligosaccharides present in dry bean seeds. Genes involved in the production of these sugars have been identified in pea (Pisum sativum), soybean (Glycine max), and adzuki bean (Vigna angularis) (Peterbauer et al., 2002). There have also been low raffinose and stachyose genotypes developed in pea and soybean via genetic engineering and chemical mutagenesis (Polowick et al., 2009; Kerr and Sebastian, 2000). Results have shown that although bean fed and control mice had similar degrees of obesity, bean fed mice had lower levels of inflammatory markers in their blood. Fermentation of bean fiber accounts for more than 50% of the gas associated with eating beans. However, oligosaccharides may function as prebiotics and many researchers contend that prebiotics help maintain a healthy colon. The addition of cooked, freeze-dried, and milled dry bean powder were fed to laboratory rats used to determine if bean had an effect on post initiation phase of chemically-induced mammary carcinogenesis compared to the standard diet without bean. Dry bean consumption reduced cancer incidence from 95% in the control to 67% in animals fed beans in the diet (P< 0.001) and cancer multiplicity from 3.23 tumors per animal to 1.46 per animal, respectively (Thompson et al., 2009). Dry bean market classes differed for anticancer activity (P = 0.038), but anticancer activity was not associated with ORAC, phenolic or flavonoid content, seed coat color, or nutrient content assessed by a proximate analyses. Dry bean market classes from the Andean center of domestication (COD) had greater anticancer activity than those from the Middle American COD (p=0.007), and dry beans from race Nueva Granada were more protective than those from race Mesoamerican (p=0.0083). Characterization/Utilization of exotic germplasm. The use of exotic germplasm as a source of genetic diversity is of key importance to broaden the genetic base of both dry and snap beans. The traits that distinguish wild and domesticated beans have been described (Gepts and Debouck, 1991). They include growth habit, seed dispersal and dormancy, pod and seed size, pigmentation, and photoperiod sensitivity. Alleles and QTL controlling these traits have been identified and mapped (Koinange et al., 1996). Thus, alleles and QTL that should facilitate exotic germplasm conversion are the St for pod string, a QTL for seed dormancy, fin for growth habit, and ppd and hr for insensitivity to photoperiod. A candidate gene for the fin locus has been identified, which facilitates marker-assisted selection of this recessive trait in backcrossing procedures for conversion (Kwak et al., 2009). Conversion programs can produce genotypes that are more adapted and breeder-friendly, which significantly reduces the amount of linkage drag usually observed when using exotic material, saving a lot of time and resources for breeding programs. In addition, the study of the genetic and molecular basis of the principal domestication traits will speed the introgression and pyramiding of favorable alleles and QTL especially from wild beans. In United States, common bean grows outside of the range of its native pollinators and seed set is generally 95% selfed. However, insect tripping of flowers may increase seed yield in determinate market classes by encouraging fertilization of more embryos within a pod (Ibarra-Perez et al., 1999). If pollinating insects, such as bumble bees, are present, domesticated common bean will outcross from 0-78% in the U.S. (Ibarra-Perez et al., 1997), depending on the population density of pollinators. Seeds in a pod may show multiple-paternity, with up to three male parents detected in the same pod (Ibarra-Perez et al., 1996). Common bean flowers are not attractive to honeybees, but they are to some species of bumble bee (Bombus sp.) in the U.S. and carpenter bee (Xylocopa sp.) in Puerto Rico (Brunner and Beaver, 1989), where extensive outcrossing was used to increase genetic variability in populations prior to selection (Bliss, 1980). Genomics/Marker Assisted Selection. Bean breeders have been among the first to adopt and widely implement marker-assisted selection in breeding programs, in most cases to select for disease resistance. Breeders have sought to pyramid different genes conferring resistance to different strains of the same or different pathogens. To date some 70 markers have been published tagging major genes or QTLs for disease resistance (Kelly et al., 2003; Miklas et al., 2006b; SCAR markers table, 2009: http://www.css.msu.edu/bic/PDF/SCAR_Markers_2009.pdf; P. Gepts, unpubl. results). In most cases, RAPD markers linked to important traits were sequenced and primers were designed in order to convert them to SCAR markers. This is a cumbersome process that could be greatly improved with the availability of a whole-genome sequence. In the mean time, the soybean sequence can be used as a reference sequence against which Phaseolus sequences can be mapped (Repinski and Gepts, 2009). National/Regional Nurseries. As mentioned previously, this multi-state project coordinates four nurseries grown every year: The Bean Rust Nursery (BRN) grown in Beltsville, MD, the national Cooperative Dry Bean Nursery (CDBN) grown at 12 locations across the country, the Midwest Regional Performance Nursery (MRPN) grown in four states, the White Mold Nursery (WMN) grown at seven locations, and the Western Regional Bean Trial (WRBT) grown in four states. These nurseries have facilitated the evaluation of genotypes across multiple environments and consequently, the release of several cultivars and germplasm lines that have been used in more than one production area. Private bean breeding programs are invited to submit genotypes to the CDBN, which allows mutual benefits, communication, and collaboration among the public breeding programs and the private sector. In addition, these nurseries provide long-term databases with genetic and agronomic information that can be used as a tool to estimate genetic gains achieved over time by the bean scientific community.
Objectives
-
Improving bean yield potential by incorporating resistance/tolerance to major biotic and abiotic stresses, broadening the genetic base, developing genomic resources, and coordinating field trial nurseries. Sub-objectives: 1a) Facilitate the genetic elucidation and breeding for resistance to bacterial, fungal, and viral diseases, facilitated by monitoring and characterizing pathogen variation. 1b) Advance in the genetics and breeding for tolerance to heat and drought in response to global climate change. 1c) Broaden the genetic base of major bean market classes by characterizing and using exotic germplasm. 1d) Develop and utilize genomic resources for marker-assisted breeding. 1e) Coordinate and expand national and regional bean trials. (CA, CO, ID, MI, NE, ND, OR, Puerto Rico)
-
Identify and implement sustainable agricultural systems that improve bean seed yield, conserve natural resources, and protect the environment. (AZ, CA, CO, ID, MI, NY, ND, Puerto Rico)
-
Characterize the genetic control and physiology of nutritional and processing quality traits in bean and elucidate the health benefits of bean consumption. (CA, ID, ND, WA).
Methods
The overall strategy is based on collaborative research of constraints shared across different production regions. This collaboration includes germplasm and pathogen exchange, sharing of protocols and techniques (e.g. DNA markers, virus isolates and infectious viral clones, field/greenhouse/lab. screening methodologies, etc.), regional nurseries and trials, and screening of genotypes for the traits of interest. As a result of this exchange of knowledge and material, breeding projects will be able to introgress and pyramid favorable alleles and QTL for enhanced seed yield potential, nutritional value, and resistance to multiple abiotic and biotic stresses using a multi-disciplinary and multi-institutional team approach. To accomplish these objectives, our research activities are divided into various sub-projects (see Appendix 2) in which researchers from two or more participating states and institutions conduct research on each major problem as a team. To identify and set priorities, all W-1150 participating researchers and stakeholders (such as growers and industry), will be periodically consulted about production problems and deficiencies in the available germplasm. A broad genetic base that allows discovery of novel and useful genes and the utilization these genes is the first step to guarantee success. This first step is accomplished in great part, by facilitating collaborative breeding efforts between programs, identifying common goals, and exchanging material. Breeding methods and selection techniques employed vary from project to project depending upon the type of population, genetic distance between parents used, inheritance of the traits under improvement, screening or evaluation methods employed, breeding objectives, and resources available. For diseases caused by variable pathogens, plant samples and/or pathogen isolates are collected and sent to the lead researchers on each area for characterization using conventional as well as cutting-edge PCR-based fingerprinting and other techniques. Promising germplasm and improved breeding lines and cultivars from all breeding projects are systematically tested yearly in the regional and national project nurseries (BRN, CDBN, MRPN, WMN, and WRBT), for agronomic performance, seed yield, nutritional value and cooking qualities, and reaction to major abiotic and biotic stresses. Subsequently, the most promising breeding lines are tested in state and regional trials and in farmers' fields for identification, release, and commercialization of new cultivars. Due to the inter-disciplinary, inter-institutional, multi-state nature of this collaboration, improved cultivars and germplasm is often released jointly among two or more institutions. This collaboration is facilitated by discussions and work plans made during the annual W-1150 meetings. Specific research procedures for the biotic and abiotic stresses currently included in the project can be found in the previous version of the W-1150. The following are mainly the new components added to the project or modified from the previous, based on feedback from scientists and other stakeholders: Objective 1: Improving Yield Potential (CA, CO, ID, MI, NE, NY, ND, OR, Puerto Rico) Subobjectives 1a and 1b - Resistance and pathogen variability for biotic stresses: Bacterial diseases Bacterial Wilt. Subsets (representing a range of seed colors and origins) of the Phaseolus vulgaris core collection Mexican Subgroup accessions and the combined Latin American Subgroup accessions will be screened for reaction separately to yellow, purple, and orange bacterial wilt isolates at multiple locations. In addition, a subset of currently-grown cultivars from various market classes grown in the U. S. will be also evaluated. In addition, there will be efforts to develop insight into the molecular basis of host-pathogen interaction and conduct QTL analysis of new bacterial wilt resistant sources to complement other genomic approaches within the W-1150 project. Common bacterial blight. Xap isolates from across the U.S. will be monitored and characterized for morphological characteristics, brown pigment production, rep-PCR fingerprinting, and pathogenicity on select Andean and Middle American beans. Approximately 100 to 200 RILs developed from each of Othello/VAX 1, Othello/VAX 3, Red Hawk/VAX 3 and other crosses, will be evaluated for CBB reaction in the greenhouse using the multiple-needle inoculation method and in field trials under natural and artificial inoculation. Data will be used to identify new resistance QTL. A set of Xap strains (UPR-Zapata) with differential reactions will be used to characterize and attempt to identify additional genes for resistance. The SAP6-linked QTL marker is associated with dominant resistance to common bacterial blight, but the marker has limited utility for MAS in Middle American background. Inbred populations segregating for this gene will be used to identify a new marker with broader utility for MAS. Fine mapping of major QTL (SU91 and BC420) will continue in collaboration with Canadian researchers. Development of cultivars with improved resistance to CBB using MAS and traditional breeding will continue for the major common bean market classes. Halo blight/Brown Spot. The races represented by Psp isolates sampled across regions will be determined primarily by inoculation of host differentials. Isolates will be assessed for phaseolotoxin production using a bacterial growth inhibition assay and with gene-based PCR primers. Subsequently, they will be characterized by repetitive element PCR to assess whether Psp races have distinct DNA fingerprints when tested by the rep-PCR method. Strains of the brown spot pathogen (Pseudomonas syringae pv. syringae, Pss), will be similarly characterized, as there has been a resurgence of this disease in some states in the Midwest. Thus, there is a need to characterize strains associated with these outbreaks. Approximately 100-150 RILs developed from each of Canadian Wonder (CW)/ZAA 12, Kranskop/HR-Kranskop, and other crosses will be evaluated for halo blight reactions in the greenhouse and under field conditions. These populations will be used to characterize existing and identify novel genes and QTL. Markers with utility for MAS of the genes/QTL conditioning resistance to halo blight will be developed. Fungal diseases Anthracnose. We will continue to monitor the pathogenic variability of anthracnose isolates occurring in the major production areas to ensure that the resistance gene pyramids being incorporated into new cultivars continues to be effective. With the rapid emergence of race 105 in Manitoba which defeats the Andean Co-12 gene in the cultivars Kaboon and Envoy, breeders need to be aware of the changing race structure of the pathogen to ensure that effective resistance genes are being deployed in their breeding programs. Breeding efforts will continue to introgress the Co-42 gene into all commercial market classes commonly grown in the Midwest. Root rots. Fusarium species: Disease surveys will be conducted and root samples collected from ten plants in each grower field. Pathogen isolation and identification will be conducted using standard microbiological methods followed by molecular confirmation using PCR and sequencing. A sand-cornmeal inoculum layer method (Bilgi et al., 2008) will be used for evaluating pathogenicity of the Fusarium isolates under greenhouse conditions. Breeding lines of different market classes from resistant x susceptible crosses will be screened in the greenhouse and field for Fusarium root rot and Fusarium wilt pathogen isolates from the Central High Plains and Michigan. Promising breeding lines will be tested in adaptation nurseries and yield trials across the country. For Rhizoctonia species, a pot test with inoculum consisting of mycelia applied to the seeds of the host plant in a soil similar to the local soil type will test both virulence on differential bean lines and resistance of breeding lines. Rust. New sources of Andean and Middle American beans with resistance to the broadest spectrum of rust pathogens will be identified. They will be crossed with susceptible genotypes to study the genetics of resistance, and to identify and tag new resistance alleles. A new set of host differentials that includes six Andean and six Middle American beans, as well as molecular tools, including the elongation factor 1±, IGS, ITS, and others, will be used to determine pathogen diversity and its co-evolution with the common bean. In addition, we will continue to monitor the virulence diversity of the common bean rust pathogen occurring in the major production areas to ensure that the resistance gene pyramids being incorporated into new cultivars continues to be effective. The recent appearance of two new races of the rust pathogen in Michigan and North Dakota that overcome the Middle American gene Ur-3 and the Andean Ur-6, is a reminder of the need to continue monitoring the virulence and diversity of the rust pathogen in commercial production fields. In the same way, the search for new sources and genes for resistance to SBR will continue, in order to incorporate SBR resistance genes in selected common bean cultivars. White mold. To determine pathogen variation among mycelial compatibility groups of Sclerotinia, virulence, ribosomal DNA polymorphism, and microsatellites will be used. Further QTL discovery and analysis is proposed for the Benton/VA19, AB136/Red Kidney, G122/Taylor Horticulture and other populations. Fine mapping of select QTL will be attempted by leveraging synteny with soybean, and using differential gene expression in near-isogenic lines. A comprehensive map of white mold resistance QTL will be developed. Potential resistant germplasm and breeding lines will be subjected to multi-state greenhouse and field testing for identification of stable white mold resistance. New resistance sources will be used to further improve existing white mold resistance. Previously available interspecific breeding lines will be evaluated for white mold in the greenhouse and field, and new sets of breeding lines will be developed from pinto bean x P. coccineus crosses. Six new breeding lines, from Univ. of Cornell will be tested in future multi-state trials. Viral diseases Geminiviruses. Efforts will be focused in three major areas: 1) biotic and molecular characterization of emerging bean-infecting begomoviruses, 2) application of P. vulgaris genomics, in relation to begomoviral pathogenesis and herbivory of phloem-feeding homopteran insects (Homoptera/Hemiptera), and 3) identification and genetic characterization of geminivirus resistance genes including generating markers for MAS (e.g. markers for Bgp-1). For characterization studies, geminiviruses causing curly top in common bean in the Western U.S., will be monitored by PCR probes. In terms of the application of genomics, efforts will be made to clone the dominant resistance gene for BDMV. For gene identification, putative novel resistance to curly top in G122/T. Hort. RIL population will be characterized, and tagged with DNA markers. Progeny lines from Morales/BAT 93 and PR9771-3-1/BAT 93 populations will be evaluated for reaction to BGYMV in an effort to tag the bgm-2 and Bgp-1 resistance genes. Examination of existing genes conferring resistance to specific geminiviruses will be characterized for broad effect against geminiviruses in general using existing RIL populations. Vigilant monitoring of geminivirus variability and of new species which threaten bean will continue. Potyviruses. W-1150 researchers will continue to work closely with the snap bean industry to monitor the aphid-transmitted virus complex in the Great Lakes region and emergent potyvirus problems in general. Pyramiding resistance genes to control potyviruses in snap bean will continue as an objective. Genetic probes will be developed to monitor strain variability and host resistance will be sought and characterized for emergent or re-emerging potyviruses including Peanut mottle virus, BYMV, and ClYVV. Resistance linked markers will be identified and improved to facilitate marker-assisted selection. Subobjective 1b. Abiotic stresses: Heat. Collaborative breeding for heat tolerance in the dry bean and snap bean market classes will continue under both greenhouse conditions (32°C/28°C; day/night) in NY and under hot summer field conditions (35C/24°C) in Puerto Rico. Thus, both high day and high night temperature conditions will be effectively tested using both environments. Phenotypic selection of advanced lines will be based on yield components. Advanced lines selected for drought tolerance through a shuttle breeding program between PR and NE will be tested for heat tolerance and superior lines selected. In addition, intra-gene pool RIL populations will be developed and QTL analysis of heat tolerance will be completed using parents adapted to tropical and temperate environments. Drought. Putative sources of drought tolerance will be evaluated in a new annual trial, the Dry Bean Drought Nursery (DBDN). The shuttle breeding program between Puerto Rico and Nebraska will continue through the introgression of diverse sources of tropical drought tolerance. Segregating populations will be evaluated in drought stress (DS), non-stress (NS) environments, and under root rot pressure in at least three states. DNA from F4 plants of the corresponding F4:6 individuals will be extracted and used to generate a genetic map of the RIL populations using CAP, microsatellite, and newly developed SNP markers. Putative QTLs will be detected and significant association between drought tolerance and marker genotype will be determined. Finally, an attempt will be made to introgress drought tolerant genes into cultivated beans through the generation of high-yielding, drought-tolerant germplasm and cultivars. Subobjective 1c. Characterization/Utilization of Exotic Germplasm: Conversion of exotic germplasm will continue to be focused on traits of critical importance in the U.S. Selected exotic germplasm for root rot, common bacterial blight, rust, and angular leaf spot disease resistance, for Empoasca insect resistance, and for drought, heat, and low fertility tolerance will be converted. General adaptation and the introgression of photoperiod insensitivity (ppd) will be achieved through the use of recurrent parents representing major market classes in the U.S. Alternating cycles of hybridization and selection for the target traits will be coordinated with W1150 collaborators. Additionally, alleles for growth habit and photoperiod insensitivity will be investigated in order to develop tightly-linked markers to these important traits. F1 heterosis for seed yield in common bean may be considerable, up to 174% above the mid-parent and 146% above the high parent in a cross between growth habit type I and type III (Neinhuis and Singh, 1986). A cytoplasmic male sterility system (CMS) requires an adequate fertility restorer system in the field (Mackenzie and Bassett, 1987; Mackenzie, 1991; Myers and Baggett, 1999). A genetic male sterility system (GMS) requires the ability to separate male-sterile seeds from male-fertile seeds before planting, or male-sterile seedlings from male-fertile seedlings before flowering in the field. Both CMS and GMS systems require an efficient pollinator system in the field in the U.S. This may be achieved using different methods (Ibarra-Perez et al., 1999; Gepts, 1998). The causes of the difference in attractiveness between common bean (predominantly selfing; non-preference by insect pollinators) and runner bean (predominantly outcrossing; preferred by insect pollinators) will be investigated by comparing the chemical composition and quantity of nectar and aromatic volatiles produced by flowers of the two species. Subobjective 1d. Genomics/Marker Assisted Selection: Sequenced DNA allows us to better understand how genes function and interact to form the transcriptome. Several sources of DNA sequences have become available and more will become available in the future. Among existing sources of DNA sequences are some 90,000 BAC-end sequences resulting from the construction of a draft physical map (Schlueter et al., 2008) and an increasing, but still insufficient number of Expressed Sequence Tags (EST) (N~ 80,000; Ramirez et al., 2005; Melotto et al., 2005; Thibivilliers et al., 2009; K. Bett, pers. comm.). Within one to two years, significant additional sources of DNA sequence will become available. First, a whole-genome sequencing project, funded by USDA/NIFA/AFRI/Plant Genome and complemented with funds of the American Recovery Act, will provide an estimated 20x coverage of the entire bean genome. It provides the ultimate genetic map of the common bean genome and allows comparison and transfer of genetic information among organisms through synteny and homology. In addition, a 1x gene sequence of methyl-filtrated genomic DNA of the breeding line BAT 93 has been generated, and was funded by the Kirkhouse Trust. This sequence will complement the whole-genome sequence effort. The genotypes sequenced in the two efforts belong to different gene pools: landrace G19833 of the Andean gene pool vs. breeding line BAT93 of the Mesoamerican gene pool, respectively. In addition, methyl filtration will focus sequencing on the expressed genome sequence, in contrast with the whole-genome sequencing effort, which will cover the entire genome. Bean DNA sequence information will be made available through several resources including the DOE-JGI plant genome sequence web site (www.phytozome.net) and a bean database currently in development at UCDavis (PhaseolusGenes), funded by the Kirkhouse Trust and the USDA-NIFA-AFRI-Plant Genome Bean Coordinated Agricultural Project (BeanCAP). The DNA sequencing and database-building efforts will contribute to the objectives of the W1150 multi-state project in several ways. First, new markers will be developed that are reliable and reveal polymorphisms between trait source genotypes and target cultivars, even when these are closely related. Second, the DNA sequence information will provide information on synteny with other legume species and model species such as Arabidopsis, which will assist in candidate gene discovery. Third, PhaseolusGenes will provide a repository for phenotypic and molecular marker polymorphism data, obtained during this and other projects. Subobjective 1e. National/Regional Nurseries: The winter nurseries in Puerto Rico will continue to allow W-1150 breeding programs to rapidly advance generations in their progenies, and multiply seed of breeding lines during the winter months. Dry bean breeding programs on the mainland U.S. can screen bean breeding lines in the greenhouse for reaction to diseases or can use marker-assisted selection in the laboratory while the winter nursery is growing in Puerto Rico. A new Dry Bean Drought Nursery (DBDN) will be planted at Nebraska every year to evaluate the tolerance of the genotypes to this important abiotic stress. In the same way, a new Winter Cooperative Dry Bean Nursery (WCDBN) will be conducted in Puerto Rico. We propose to compare the performance of bean lines in Puerto Rico with their performance in different bean production regions of the U.S. A multi-location analysis approach will be used to analyze the results from the CDBN and the WCDBN. Results from the analysis should help to determine how well the performance of bean breeding lines in Puerto Rico predicts the performance of bean lines in different bean production regions of the U.S. A similar approach will be used for other nurseries such as the MRPN and the WRBT in an attempt to estimate genetic gains across years and regions. The Bean Rust and White Mold Multi-state nurseries will give breeders a good evaluation of broad resistance to these two diseases. Objective 2. Agronomy/Production systems: This is a new area to be implemented in the project and inclusion was based on inputs from scientists and other stakeholders. To attain sustainable production of beans, research is needed to define more efficient production techniques with the minimum amount of inputs (Cogger, 2009; Parmenter, 2003). Three main areas will be considered: i) Nitrogen use efficiency and Biological Nitrogen Fixation (BNF), ii) Minimum tillage, and iii) Direct harvest systems. Research that identifies genotypes suitable for organic production will also be conducted. Nutrient-use efficiency, such as for nitrogen, might be improved by breeding bean market classes with a larger root system (Lynch, 2007). (AZ, CA, CO, ID, MI, NY, ND, Puerto Rico). Objective 3. Nutritional Value, Health Benefits, and Quality: Nutritional quality traits. Since many members of the W1150 project are also directly involved with the BeanCAP, there will be numerous opportunities for interaction among the two projects. This project will work closely with dry beans of different market classes which will be screened for several nutritional traits. Antioxidant capacity of processed beans will be assessed since antioxidant activity is known to be lost during cooking (Thompson et al., 2009). It is hypothesized that beans possessing the greatest in vivo antioxidant activity, the lowest glycemic index, and the greatest anti-inflammatory response will have cancer inhibitory activity. This hypothesis will be tested in a chemically induced mammary cancer experiment with rats. The potential for navy beans to mitigate the colon cancer enhancing properties of obesity, hyperglycemia, and hyperinsulinemia will be tested in a chemically induced colon cancer experiment in mice genetically predisposed to these conditions. (CA, ID, ND, WA). Processing quality. All advanced breeding lines with potential to be released will be canned to ensure that canning quality is maintained or improved. Extrusion will be used to prepare prototype-snack items made from beans. The snack items will be promoted as healthy snacks with intent to move continued product development and marketing to the commercial sector. Gluten-free flour will be produced for people suffering from celiac disease, for vegetarians, and for health-conscious individuals desiring alternate food choices that will provide protein and fiber. Reduced or oligosaccharide free flour will be produced by enzymatic hydrolysis of the tri- and tetraoligosaccharides to produce products for individuals concerned with flatulence. The variability in indigestible starch and indigestible protein will be determined in the nursery maintained at East Lansing (MI) to estimate inheritance of these traits. Identification of low raffinose and/or low stachyose genotypes would be valuable to improve consumer acceptance and expand market potential of dry bean. Screening populations of chemically mutagenized lines will be the approach employed to identify low raffinose and stachyose lines. An EMS mutagenized population of BAT 93 has been developed (Porch et al., 2009a) and approximately 2000 M4 lines will be screened for oligosaccharide content. Such an approach has been used to identify a low raffinose and stachyose line (75% total reduction) in soybean (Kerr and Sebastian, 2000; Dierking and Bilyeu, 2009). Additional mutagenized bean populations are also being developed in kidney, small red, and pinto market classes and these lines will also be screened for low oligosaccharide levels.Measurement of Progress and Results
Outputs
- New germplasm, improved breeding lines and cultivars of major market classes will be developed and released. The improved breeding lines and cultivars will possess high levels of resistance/tolerance to biotic and abiotic stresses.
- A detailed understanding of the extent and nature of genetic diversity of the pathogens causing economically important diseases in the U.S. will be obtained. Similarly, new alternative methods of detection, inoculation, and/or screening environments for bean diseases will be developed. Together, this will help determine the most appropriate inoculation methods and the number and type of strains/isolates that need to be used for resistance breeding.
- Germplasm exchange, and tropical germplasm characterization and conversion will provide researchers novel genes to broaden the genetic base of U.S. cultivars (e.g., new resistance genes to abiotic and biotic stresses). In addition, markers tightly linked to favorable alleles and QTL involved in domestication will be developed.
- Genomic information generated will help to understand the genetic control of many traits of interest. New genes for resistance/tolerance and nutritional attributes will be discovered. Concurrently, molecular markers for new resistance alleles and QTL for many diseases, abiotic stresses, and nutritional and processing quality traits will be generated from this project.
- Information generated on efficient production systems and agronomic practices that facilitate sustainable bean production will be shared with scientists, growers and other stakeholders.
- New information about the genes related to nutritional quality and health benefits of eating dry beans and snap beans will be produced and disseminated to consumers. Market classes of beans that have comparatively higher bioactivity to reduce the incidence of cancer and diabetes will be identified and this should provide some insight into whether we can breed for greater nutritional value. ;Components of dry beans that impart health benefits will be identified. New value-added, bean-based snacks and convenience foods will be investigated as a way to increase overall bean consumption.
Outcomes or Projected Impacts
- Improved high yielding bean cultivars resistant to multiple abiotic and biotic stresses (especially multiple-diseases) will dominate the regional and national production. Area planted to new cultivars may increase by more than 10% leading to substantial yield increases in the participating states.
- Commercial value of new cultivars may exceed $250 million annually.
- Adoption of multiple-disease resistant cultivars may reduce fungicide use by 25% or more resulting in savings to producers and in cleaner environment.
- Bean productions systems that minimize certain inputs such as fertilizers, that allow direct harvest, and that still obtain competitive yields, will allow for more sustainable production.
- The genes responsible for several of the key nutrients and health-related benefits (including specific components that impart health benefits) of consuming different market classes of dry beans will be known.
- Availability of new value-added, bean-based snacks and convenience foods are expected to increase overall bean consumption in the U.S.
Milestones
(2011): New upright vine cranberry bean cultivar with excellent canning quality. Release of germplasm with drought and heat tolerance in several market classes. Complete evaluation of mapping populations in multiple locations. Development of a marker table listing all markers produced for common bean (i.e., DNA sequences accompanied with PCR conditions, including primers and annealing temperature)(2012): Identification of additional QTL for yield under severe white mold pressure. Begin pyramiding drought tolerance with multiple abiotic and biotic stress tolerance. Generate genetic map for RIL population and evaluate RILs in multiple locations under drought stress. Understand variation of S. sclerotiorum for use of relevant isolates in screening. Annotation and mapping of 1x sequence of methyl-filtrated P. vulgaris sequence; addition of phenotypic data from bean breeders nurseries to PhaseolusGenes database in this and following year.
(2013): New navy and black bean cultivars with combination of anthracnose and common bacterial blight resistance. Perform QTL analysis on drought tolerance in RIL population Molecular tools available for monitoring root rot pathogens. Incorporation of whole-genome sequence of Phaseolus into PhaseolusGenes genome database.
(2014): Broad-based anthracnose resistance in new pinto and great northern cultivars. Test lines with tolerance to multiple stresses, including drought, heat, and diseases. Test newly identified drought QTL markers in breeding lines for use in marker assisted selection (MAS). Snap bean lines and germplasm with MAS white mold resistance released. Generate information on chemical flower attractants of Phaseolus flowers obtained.
(2015): New pink and small red bean cultivars with common bacterial blight resistance. Release advance lines with multiple abiotic and biotic stress tolerance. Test MAS with newly developed QTL in segregating populations.
Projected Participation
View Appendix E: ParticipationOutreach Plan
Research results from each sub-project will be promptly published in refereed and non-refereed journals, extension bulletins, flyers, etc., and posted on the web sites of individual institutions or programs, including the Legume-IPM-PIPE system (http://legume.ipmpipe.org/cgi-bin/sbr/public.cgi) which is coordinated by one of the project members (Schwartz et al., 2009a). Breeding lines and cultivars will be extensively tested statewide, regionally (e.g., the Midwest Regional Performance Nursery and Western Regional Bean Trials), and nationally (e.g., the Cooperative Dry Bean Nursery), including on-farm strip-plantings of the most promising or outstanding genotypes in different cropping systems. Breeder, Foundation, Registered, and Certified seed of the new cultivars will be produced and distributed to bean growers and the seed industry. Field days will be held each year at or near crop maturity. In addition, the most important findings will be shared with all interested parties through workshops, news media, and electronic mail. The released cultivars and germplasm lines will be registered with the Crop Science Society of America, Bean Improvement Cooperative, or American Society of Horticultural Sciences, and seed deposited with the National Center for Genetic Resources Preservation.
Organization/Governance
Present officers of the W-1150 Regional Project are: Chair, Carlos Urrea, University of Nebraska, Lincoln, Scottsbluff, NE. Vice-Chair, Steve Noffsinger, Seneca Foods Corp., Dayton, WA. Secretary, Rubella Goswami, North Dakota State University, Fargo, ND. Administrative Advisor, Donn Thill (assigned Dec. 2009), University of Idaho, Moscow, ID. The directors of the various participating state institutions designate the W-1150 participating researchers, who elect the members or officers of the Technical Committee. The project is considered a Western Regional Research Project, but has always had substantial participation by states from other bean producing regions of the U.S. and USDA-ARS researchers. The Technical Committee officers are a Chairperson, Vice-Chairperson, and Secretary. Unless he/she declines to serve, the Vice-Chairperson will succeed the Chairperson. The Secretary is elected annually and the previous Secretary will succeed the Vice-Chairperson, unless he/she declines to serve. An election will be held if any officer declines to serve in his/her office. The officers will be elected from the officially designated representatives each year at the annual meetings. The Western Association of Agricultural Experiment Station Directors selects the Administrative Advisor who has no voting rights. The Technical Committee will meet annually, unless otherwise planned, at a place and on a date designated by a majority vote of the committee. Minutes will be recorded and an annual progress report will be prepared by the Technical Committee and submitted through proper channels.
Literature Cited
Acevedo-Román, M., A. Molina-Castañeda, J.C. Angel-Sánchez, C.G. Muñoz, and J.S. Beaver. 2004. Inheritance of normal pod development in bean golden yellow mosaic resistant common bean. J. Am. Soc. Hort. Sci. 129:549-552. Anderson, J.W., L. Story, B. Sieling, W.-J.L. Chen, M.S. Petro, and J. Story. 1984. Hypocholesterolemic effects of oat-bran or bean intake for hypercholesterolemic men. Am. J. Clinical Nutri. 40:1146-1155. Awale, H., S. M. Ismail, V.A. Vallejo, and J.D. Kelly. 2008. SQ4 SCAR marker linked to the Co-2 gene on B11 appears to be linked to the Ur-11 gene. Annu. Rep. Bean Improv. Coop 51:174-175. Beaver, J.S., and P.N. Miklas. 1999. Registration of Morales small white bean. Crop Sci. 39:1257. Beaver, J.S., and J.M. Osorno. 2009. Achievements and limitations of contemporary common bean breeding using conventional and molecular approaches. Euphytica 168:145-176. Beaver, J.S., J.M. Osorno, F.H. Ferwerda, C.G. Muñoz-Perea. 2005. Registration of bean golden yellow mosaic virus resistant germplasms PR9771-3-1, PR0247-49 and PR0157-4-1. Crop Sci. 45:2126-2127. Beaver, J.S., T.G. Porch, and M. Zapata. 2008. Registration of Verano White Bean. J. of Plant Reg. 2:187-189. Beninger, C.W., and G.L. Hosfield. 2003. Antioxidant activity of extracts, condensed tannin fractions, and pure flavonoids from Phaseolus vulgaris L. seed coat color genotypes. J. Agric. Fd. Chem. 51:7879-7883. Berrios, J.D., B.G. Swanson, and W.A. Cheong. 1999. Physico chemical characterization of stored black beans (Phaseolus vulgaris L.). Food Res. Int. 32:669-676. Bilgi, V.N., C.A.Bradley, S. Ali, S.D. Khot, and J.B. Rasmussen. 2007. Reaction of dry bean genotypes to root rot caused by Fusarium graminearum. Phytopathology 97:S10. Bilgi, V. N., C.A. Bradley, S.D. Khot, K.F. Grafton, and J.B. Rasmussen. 2008. Response of dry bean genotypes to Fusarium root rot, caused by Fusarium solani f. sp. phaseoli, under field and controlled conditions. Plant Dis. 92:1197-1200. Bird, J., A.M. Idris, D. Rogan, and J.K. Brown. 2001. Introduction of the exotic Tomato yellow leaf curl vrus-Israel in tomato into Puerto Rico. Plant Dis. 85:1028. Blair, M.W, J.S. Beaver, J.C. Nin, E. Prophete, and S.P. Singh. 2006. Registration of PR9745232 and RMC-3 red-mottled dry bean germplasm lines with resistance to bean golden yellow mosaic virus. Crop Sci. 46:1000-1002. Bliss F.A., 1980. Common Bean. In W.R. Fehr and H. Hadley (eds.). Hybridization of Crop Plants. ASA, CSSA, Madison WI, p.273-284. Bobe, G., K.G. Barrett, R.A. Mentor-Marcel, U. Saffiotti, M.R. Young, N.H. Colburn, P.S. Albert, M.R. Bennink, and E. Lanza. 2008. Dietary cooked navy beans and their fractions attenuate colon carcinogenesis in azoxymethane-induced Ob/Ob mice. Nutr. Cancer. 60(3): 373 - 381. Bradley, C.A., and J.L. Luecke. 2004. 2002 dry bean grower survey of pest problems and pesticide use in Minnesota and North Dakota. Ext. Rep. 1265. North Dakota State Univ., Fargo. Brick, M. A., Ogg, J.B., Singh, S.P., Schwartz, H.F., Johnson, J.J., and Pastor-Corrales, M.A. 2008. Registration of drought tolerant, rust resistant, high yielding pinto bean germplasm line CO46348. J. of Plant Reg. 2: 120-124. Britten, P., K. Marcoe, S. Yamini, and C. Davis. 2006. Development of food intake patterns for MyPyramid food guidance system. J. Nutr. Ed. Behav. 38 (Suppl.):S78-S92. Brown, J.K. 1994. The Status of Bemisia tabaci (Genn.) as a pest and vector in world agroecosystems. FAO Plant Prot. Bull. 42: 3-32. Brown, J.K. 2007. The Bemisia tabaci complex: genetic and phenotypic variability drives begomovirus spread and virus diversification. Plant Disease Feature Jan 2007. http://www.apsnet.org/online/feature/btabaci/. Brown, J.K., K.M. Ostrow, A.M. Idris, and D.C. Stenger. 1999. Biotic, molecular and phylogenetic characterization of bean calico mosaic virus, a distinct /geminivirus/ species with affiliation in the squash leaf curl virus cluster. Phytopathology 89: 273-280. Brunner, B.R., and J.S. Beaver.1989. Estimation of outcrossing of the common bean in Puerto Rico. HortScience 24:669-671. Brunt, A.A, and R.H. Kenten. 1973. Cowpea mild mottle, a newly recognized virus infecting cowpeas(Vigna unguiculata) in Ghana. Ann. Appl. Biol. 74:h7-74. Chen, L.F., and R.L. Gilbertson. 2009. Curtovirus-cucurbit interaction: Acquisition host plays a role in leafhopper transmission in a host-dependent manner. Phytopathology 99:101-108. Chen, L.F., K. Brannigan, R. Clark, and R.L. Gilbertson. 2010. Characterization of curtoviruses associated with curly top disease of tomato in California and monitoring for these viruses in leafhoppers. Plant Dis. 94: 99-108. Chung, Y.S., M.E. Sass, and J. Nienhuis. 2008. Validation of RAPD markers for white mold resistance in two snap bean populations based on field and greenhouse evaluations. Crop Sci. 48:2265-2273. Cogger, M.T. 2009. Impact of conservation tillage practices on weed management, soil properties, and sweet corn and dry bean yield and quality. M.S. Thesis, Cornell University. 75 pp. Coyne, D.P., and M.L. Schuster. 1971. Genetic variation in bacterial pathogens. Euphytica 24:143-147. Coyne, D.P., and M.L. Schuster. 1976. Great Northern Star dry bean tolerant to bacterial diseases. HortSci. 11:621. Cramer, R.A., P.F. Byrne, M.A. Brick, L. Panella, E. Wickliffe, and H.F. Schwartz. 2003. Characterization of Fusarium oxysporum isolates from common bean and sugar beet using pathogenicity assays and random-amplified polymorphic DNA markers. J. Phytopathology 151:352-306. Cross, H., M.A. Brick, H.F. Schwartz, L.W. Panella, and P.F. Byrne. 2000. Inheritance of resistance to Fusarium wilt in two common bean races. Crop Sci. 40:954-958. Dierking, E.C., and K.D. Bilyeu. 2009. Raffinose and stachyose metabolism are not required for efficient soybean seed germination. J. Plant Physiol. 166: 1329-1335. Duncan, R.W., S.P. Singh, and R.L. Gilbertson. 2008a. Genetic diversity in Xanthomonas campestris pv. phaseoli and X. campestris pv. phaseoli var. fuscans and interaction with resistant common bean genotypes. Annu. Rept. Bean Improv. Coop. 51: 38-39. Duncan, R.W., S.P. Singh, and R.L.Gilbertson. 2008b. Identification and inheritance of a new source of halo blight resistance in common beans. Annu Rept. Bean Improv. Coop. 51:48-49. Ehdaie B., and J.G. Waines. 2008. Larger root system increases water-nitrogen uptake and grain yield in bread wheat. Proc. 11th. Int. Wheat Genet. Symp. Brisbane, Qld. Australia. 2: 659-661. Ender, M., and J.D. Kelly. 2005. Identification of QTL associated with white mold resistance in common bean. Crop Sci. 45:2482-2490. Ender, M., K. Terpstra, and J.D. Kelly. 2007. Marker-assisted selection for white mold resistance in common bean. Molecular Breeding. 21:149-157. EPPO/CABI. 1997. Curtobacterium flaccumfaciens pv. flaccumfaciens. In I.M. Smith, D.G. McNamara, P.R. Scott, and H. Holderness (eds.) Quaratine Pests for Europe, 2nd Ed. CAB International, Wallingford, UK. p. 991-994. Fall, A.L., P.F. Byrne, G. Jung, D.P. Coyne, M.A. Brick, and H.F. Schwartz. 2001. Detection and mapping of a major locus for Fusarium wilt resistance in common bean. Crop Sci. 41:1494-1498. Faria, J.C., R.L. Gilbertson, S.F. Hanson, F.J. Morales, P. Ahlquist, A.O. Loniello and D.P. Maxwell, 1994. Bean golden mosaic geminivirus type II isolates from Dominican Republic and Guatemala: Nucleotide sequences, infectious pseudorecombinants, and phylogenetic relationships. Phytopathology 84:321-329. Gambhir, A., R.S. Lamppa, J.B. Rasmussen, and R.S. Goswami. 2008. Fusarium and Rhizoctonia species associated with root rots of dry beans in North Dakota and Minnesota. Phytopathology 98:S57. Garrido-Ramirez, E.R., M.R. Sudarshana, and R.L. Gilbertson. 2000. Bean golden yellow mosaic virus from Chiapas, Mexico: characterization, pseudorecombination with other bean-infecting geminiviruses and germ plasm screening. Phytopathology 90:1224-1232. Geffroy, V., D. Sicard, J. de Oliveira, M. Sévignac, S. Cohen, P. Gepts, C. Neema, and M. Dron. 1999. Identification of an ancestral resistance gene cluster involved in the coevolution process between Phaseolus vulgaris and its fungal pathogen Colletotrichum lindemuthianum. Molec Plant-Micr Interactions 12:774-784 Gepts, P. 1998. Origin and evolution of common bean: past events and recent trends. HortSci. 33: 1124-1130. Gepts, P., F.J.L. Aragão, E.D. Barros, M.W. Blair, R. Brondani, W. Broughton, I. Galasso, G. Hernández, J. Kami, P. Lariguet, P. McClean, M. Melotto, P. Miklas, P. Pauls, A. Pedrosa-Harand, T. Porch, F. Sánchez, F. Sparvoli, and K. Yu. 2008. Genomics of Phaseolus beans, a major source of dietary protein and micronutrients in the Tropics. In Moore, P.H. and Ming, R. (eds.) Genomics of Tropical Crop Plants. Springer, Berlin, pp 113-143. Gepts, P., and F.A. Bliss. 1985. F1 hybrid weakness in the common bean: Differential geographic origin suggests two gene pools in cultivated gene germplasm. J. Hered. 76(6):447-450. Gepts, P., and D.G. Debouck. 1991. Origin, domestication, and evolution of the common bean, Phaseolus vulgaris, p. 7-53. In O. Voysest and A. Van Schoonhoven (eds.) Common beans: Research for crop improvement. C.A.B. Intl., Wallingford, UK and CIAT, Cali, Colombia. Gepts, P., T.C. Osborn, K. Rashka, and F.A. Bliss. 1986. Phaseolin protein variability in wild forms and landraces of the common bean (Phaseolus vulgaris): evidence for multiple centers of domestication. Econ. Bot. 40:451-468. Gilbertson, R.L., J.C. Faria, P.G. Ahlquist, and D.P. Maxwell. 1993. Genetic diversity in geminiviruses causing bean golden mosaic disease: the nucleotide sequence of the infectious cloned DNA components of a Brazilian isolate of bean golden mosaic virus. Phytopathology 83: 709-715. Gilmore, B., J.R. Myers, and D. Kean. 2002. Completion of testing of Phaseolus coccineus plant introductions (PIs) for white mold, Sclerotinia sclerotiorum, resistance. Annu. Rpt. Bean Improv. Coop. 45:64-65. Gonçalves-Vidigal, M.C., G.F. Lacanallo, and P.S. Vidigal Filho. 2008. A new gene conferring resistance to anthracnose in Andean common bean (Phaseolus vulgaris L.) cultivar Jalo vermelho. Plant Breeding 127:592-596. Gonçalves-Vidigal, M.C., P.S. Vidigal Filho, A.F. Medeiros, and M.A. Pastor-Corrales. 2009. Common bean landrace Jalo Listras Pretas is the source of a new Andean anthracnose resistance gene. Crop Sci. 49:133-138. Grafton, K.F., J.B. Rasmussen, J.R. Steadman, D.C. Hauf, and C. Donohue. 2002. Potential new sources of resistance to white mold in the Phaseolus core collections. Annu. Rpt. Bean Improv. Coop. 45: 58-59. Grau, C.R., W.R. Stevenson, and A.M. Mondjana. 2002. Viruses causing losses on processing beans in the Mid-west. Proc. 2002 Wis. Fertilizer, Aglime, Pest Manage. Conf. 41: 248256. Griffiths, P.D. 2009. Release of Cornell 601-606: Common bean breeding lines with resistance to white mold. HortSci. 44 (2): 463-465. Griffiths, P.D., M.M. Jahn, and M.H. Dickson. 2004. Cornell 501: A white mold tolerant snap bean breeding line. HortSci. 39(6):1507-1508. Gu, W., J. Zhu, D. Wallace, S. Singh, and N. Weeden. 1998. Analysis of genes controlling photoperiod sensitivity in common bean using DNA markers. Euphytica 102:125-132. Guzmán, P., R.L. Gilbertson, R. Nodari, W.C. Johnson, S.R. Temple, D. Mandala, A.B.C. Mkandawire, and P. Gepts. 1995. Characterization of variability in the fungus Phaeoisariopsis griseola suggests coevolution with the common bean (Phaseolus vulgaris). Phytopathology 85:600-607. Harveson, R.M., and H.F. Schwartz. 2007. Bacterial diseases of dry edible beans in the central high plains. Online. Plant Health Progress doi:10.1094/PHP-2007-0125-01-DG. Harveson, R.M., H.F. Schwartz, A.K. Vidaver, P.A. Lambrecht, and K. Otto. 2006. New outbreaks of bacterial wilt of dry beans in Nebraska observed from field infections. Plant Dis. 90: 681. Harveson, R.M., and A.K. Vidaver. 2007. First report of the natural occurrence of soybean bacterial wilt isolates pathogenic to dry beans in Nebraska. Plant Health Progress doi:10.1094/PHP-2007-0822-01-BR. Harveson, R.M., A.K. Vidaver, and H.F. Schwartz. 2005. Bacterial wilt of dry beans in western Nebraska. NebGuide. University of Nebraska. G05-1562A. Huang, H. C., H.H. Mundel, R.S. Erickson, C.D. Chelle, P.M. Balasubramanian, F. Kiehn, and R.L. Conner. 2007. Resistance of common bean (Phaseolus vulgaris L.) cultivars and germplasm lines to the purple variant of bacterial wilt (Curtobacterium flaccumfaciens pv. flaccumfaciens). Plant Path. Bull. 16:91-95. Ibarra-Perez F.J., D. Barnhart, B. Ehdaie, K.M. Knio, and J.G. Waines. 1999. Effects of insect tripping on seed yield in common bean. Crop Sci. 39:428-433. Ibarra-Perez F.J., B. Ehdaie, and J.G. Waines. 1997. Estimation of outcrossing in common bean. Crop Sci. 37:60-65. Ibarra-Perez F.J., N.C. Ellstrand, and J.G. Waines. 1996. Multiple paternity in common bean (Phaseolus vulgaris L., Fabaceae). Am. J. Bot. 83:749-758. Idris, A. M., and J.K. Brown. 1998. Sinaloa tomato leaf curl geminivirus: biological and molecular evidence for a new subgroup III virus. Phytopathology 88: 648-657. Kelly, J.D., P. Gepts, P.N. Miklas, and D.P. Coyne. 2003. Tagging and mapping genes and QTL and molecular marker-assisted selection for traits of economic importance in bean and cowpea. Field Crops Res. 82:135-154. Kelly, J.D., G.L. Hosfield, G.V. Varner, M.A. Uebersax, and J. Taylor. 1999. Registration of 'Matterhorn' great northern bean. Crop Sci. 39:589-590. Kelly, J. D., J.M. Kolkman, and K. Schneider. 1998. Breeding for yield in dry bean (Phaseolus vulgaris L.). Euphytica 102:343-356. Kerr, P.S., and S.A. Sebastian. 2000. US Patent 6147193. Date Issued: November 14, 2000. King J., A. Gay, R. Sylvester-Bradley, I. Bingham, J. Foulkes, P. Gregory, and D. Robinson. 2003. Modeling cereal root systems for water and nitrogen capture: Towards an economic optimum. Ann. Bot. 91:383-390. Koenig, R., S.P. Singh, and P. Gepts. 1990. Novel phaseolin types in wild and cultivated common bean (Phaseolus vulgaris, Fabaceae). Econ. Bot. 44: 50-60. Koinange, E.M.K., S.P. Singh, and P. Gepts. 1996. Genetic control of the domestication syndrome in common-bean. Crop Sci. 36:1037-1045. Kull, L.S., W.L. Pedersen, D. Palmquist, and G.L. Hartman. 2004. Mycelial compatibility grouping and aggressiveness of Sclerotinia sclerotiorum. Plant Dis. 88: 325-332. Kwak, M., D.M. Velasco, and P. Gepts. 2009. Mapping homologous sequences for determinacy and photoperiod sensitivity in common bean (Phaseolus vulgaris). J. Hered. 99:283-291. Lamppa, R.S., P.L. Gross, and L.E. del Rio. 2002. Identification of races of Pseudomonas syringae pv. phaseolicola present in North Dakota. Phytopatology 92(Suppl.):S139. Larsen, R.C., P.N. Miklas, K.L. Druffel, and S.D. Wyatt. 2005. NL-3 K strain is a stable and naturally occurring interspecific recombinant derived from Bean common mosaic necrosis virus and Bean common mosaic virus. Phytopathology 95: 10371042. Larsen, R.C., P.N. Miklas, K.C. Eastwell, and C.R. Grau. 2008. A strain of Clover yellow vein virus that causes severe pod necrosis disease in snap bean. Plant Dis. 92:1026-1032. Larsen, R.C., P.N. Miklas, K.C. Eastwell, C.R. Grau, and A. Mondjana. 2002. A virus disease complex devastating late season snap bean production in the Midwest. Annu. Rep. Bean Improv. Coop. 45: 3637. Leterme, P. 2002. Recommendations by health organizations for pulse consumption. Brit. J. of Nutr. 88(Suppl.):S239-S242. Liebenberg, M.A., L.A. Madubanya, C.M.S. Mienie and J.D. Kelly. 2009. A closer look at the resistance gene cluster on common bean chromosome 11. Annu. Rep. Bean Improv. Coop. 52:80-81. Lopez, R., C. Asensio, and R.L. Gilbertson. 2006. Phenotypic and genetic diversity in strains of common blight bacteria (Xanthomonas campestris pv. phaseoli and X. campestris pv. phaseoli var. fuscans) in a secondary center of diversity of the common bean host suggests multiple introduction events. Phytopathology 96: 1204-1213. Lynch J.P. 2007. Roots of the second green revolution. Australian J. of Botany 55:493-512. Mackenzie, S.A. 1991. Identification of a sterility-inducing cytoplasm in a fertile accession line of Phaseolus vulgaris L. Genetics 127:411-416. MacKenzie, S.A., and M.J. Bassett. 1987. Genetics of fertility restoration in cytoplasmic male sterile Phaseolus vulgaris L. I. Cytoplasmic alteration by a nuclear restorer gene. Theor. Appl. Genet. 74: 642-645. Madubanya, L.A., M.A. Liebenberg, C.M.S. Mienie and J.D. Kelly. 2009. Mapping rust resistance genes on the distal portion of bean chromosome 11. Annu. Rep. Bean Improv. Coop. 52:78-79. Markell, S.M., M.A. Pastor-Corrales, J.G. Jordahl, R.S. Lampa, F.B. Mathew, J.M. Osorno, and R.S. Goswami. 2009. Virulence of Uromyces appendiculatus to the resistance gene Ur-3 identified in North Dakota in 2008. Ann. Rep. Bean Improv. Coop. 52: 82-83. Maxwell, J., M. Brick, P. Byrne, X. Shan, J. Ogg, and R. Henson. 2007. Quantitative trait loci linked to white mold resistance in common bean. Crop Sci. 47:2285-2294. McClean, P.E., J.R. Myers, and J.J. Hammond. 1993. Coefficient of parentage and cluster analysis of North American dry bean cultivars. Crop Sci. 33:190-97. McClean, P.E., M. Lavin, P. Gepts, S.A. Jackson. 2008. Phaseolus vulgaris: a diploid model for soybean. In Stacey, G. (ed) Genetics and Genomics of Soybean. Springer, New York, pp 55-76. Melotto, M., C.B. Monteiro-Vitorello, A.G. Bruschi, and L.E.A. Camargo. 2005. Comparative bioinformatic analysis of genes expressed in common bean (Phaseolus vulgaris L.) seedlings Genome 48: 562570. Miklas, P.N. 2000. Use of Phaseolus germplasm in breeding pinto, great northern, pink, and red bean for the Pacific Northwest and intermountain region. p. 13-29. In S.P. Singh (ed.) Bean research, production and utilization. Proc. of the Idaho bean workshop. Univ. of Idaho, Moscow, ID. Miklas, P.N., K.F. Grafton, D. Hauf, and J.D. Kelly. 2006a. Registration of partial white mold resistant pinto bean germplasm line USPT-WM-1. Crop Sci. 46:2339. Miklas P.N., J.D. Kelly, S.E. Beebe, and M.W. Blair. 2006b. Common bean breeding for resistance against biotic and abiotic stresses: From classical to MAS breeding. Euphytica 147:105-131. Miklas, P.N., J.R. Smith, and S.P. Singh. 2006c. Registration of common bacterial blight resistant dark red kidney bean germplasm line USDK-CBB-15. Crop Sci. 46:1005-1007. Miklas, P.N., J.R. Smith, and S.P. Singh. 2006d. Registration of common bacterial blight resistant white kidney bean germplasm line USWK-CBB-17. Crop Sci. 46:2338-2339. Miklas, P.N., J.R. Smith, and S.P. Singh. 2006e. Release of common bacterial blight resistant pinto bean germplasm lines USPT-CBB-5 and USPT-CBB-6. Annu. Rep. Bean Improv. Coop. 49:283-284. Miklas, P.N., K.M. Larsen, K.A. Terpstra, D.C. Hauf, K.F. Grafton, and J.D. Kelly. 2007. QTL Analysis of ICA Bunsi-derived resistance to white mold in a pinto x navy bean cross. Crop Sci. 47:174-179. Miklas, P.N., J.R. Smith, S.P. Singh, and J.D. Kelly. 2008. Notice of release of common bacterial blight resistant cranberry germplasm line USCR-CBB-20. USDA-ARS Germplasm Release Notice, 3 p. Miklas, P.N., D. Fourie, J. Wagner, R.C. Larsen, and C.M.S. Mienie. 2009. Tagging and mapping Pse-1 gene for resistance to halo blight in common bean host differential cultivar UI-3. Crop Sci. 49:41-48. Miles, M.R., M.A. Pastor-Corrales, G.L. Hartman, and R.D. Frederick. 2007. Differential Response of common bean cultivars to Phakopsora pachyrhizi. Plant Dis.91:698-704. Mitchell, D.C., F.R. Lawrence, T.J. Hartman, and J.M. Curran. 2009. Consumption of dry beans, peas, and lentils could improve diet quality in the US population. J. Amer. Dietetic Assoc. 109:909-913. Mkandawire, A.B.C., R.B. Mabagala, P. Guzman, P. Gepts, and R.L. Gilbertson. 2004. Genetic diversity and pathogenic variation of common blight bacteria (Xanthomonas campestris pv. phaseoli and X. campestris pv. phaseoli var. fuscans) suggests coevolution with the common bean. Phytopathology 94:593-603. Mutlu, N., P. N. Miklas, J. Reiser, and D. Coyne. 2005a. Backcross breeding for improved resistance to common bacterial blight in pinto bean (Phaseolus vulgaris L.). Plant Breeding 124:282-287. Mutlu, N., P.N. Miklas, J.R. Steadman, A.V. Vidaver, D. Lindgren, J. Reiser, and M.A. Pastor-Corrales. 2005b. Registration of pinto bean germplasm line ABCP-8 with resistance to common bacterial blight. Crop Sci. 45:806-807. Mutlu, N., C.A. Urrea, P.N. Miklas, J.R. Steadman, M.A. Pastor-Corrales, D.T. Lindgren, J. Reiser, A.K. Vidaver, and D.P. Coyne. 2008. Registration of common bacterial blight resistant great northern bean germplasm line ABC-Weihing. J. of Plant Reg. 2: 53-55. Myers J.R., and J.R. Baggett. 1999. Improvement of snap bean. In Singh S.P. (ed.) Common bean improvement in the twenty first century. Kluwer, Dordrecht. p. 289-329. Nakhla, M. K., Maxwell, D. P., Martinez, R. T., Carvalho, M. G., and Gilbertson, R. L. 1994. Widespread occurrence of the Eastern Mediterranean strain of tomato yellow leaf curl geminivirus in the Dominican Republic. Plant Dis. 78:926. OBoyle, P.D., W.W. Kirk, and J.D. Kelly. 2007. Use of marker-assisted selection to breed for resistance to common bacterial blight in common bean. J. Amer. Soc. Hort. Sci. 132:381-386. Osorno, J.M., C.G. Muñoz, J.S. Beaver, F.H. Ferwerda, M.J. Bassett, P.N. Miklas, T. Olczyc, and B. Bussey. 2007. Two genes from Phaseolus coccineus confer resistance to bean golden yellow mosaic virus in common bean. J. Amer. Soc. Hort. Sci. 132:530-533. Otto-Hanson, L.K. and J.R. Steadman. 2007. Identification of partial resistance to Sclerotinia sclerotiorum in common bean at multiple locations in 2006. Ann. Rep. Bean Improv. Coop. 50: 133-134. Otto-Hanson, L.K., J.R. Steadman, and S. McCoy. 2009. Multisite screening for Sclerotinia sclerotiorum: a successful approach to identifying resistance in dry and snap beans. Program Book of 14th International Sclerotinia Workshop 31 May 4 June, 2009. North Carolina. Abst. 13. Papa, R. and P. Gepts. 2003. Asymmetry of gene flow and differential geographical structure of molecular diversity in wild and domesticated common bean (Phaseolus vulgaris L.) from Mesoamerica. Theor. Appl. Gen. 106:239-250. Parmenter, D.M. 2003. Cultural practice study for the improvement of dry bean production in New York State. M.S. Thesis, Cornell University. 166pp. Pastor-Corrales, M.A., A. Sartorato, M.M. Liebenberg, M.J. del Peloso, P.A. Arraes-Pereira, J. Nunes-Junior, and H. Dinis-Campo. 2007. Evaluation of common bean cultivars from the United States for their reaction to soybean rust under field conditions in Brazil and South Africa. Ann. Rep. Bean Improv. Coop. 50: 123-124. Peterbauer, T., J. Mucha, L. Mach, and A. Richer. 2002. Chain Elongation of Raffinose in Pea Seeds. J. Biolog. Chem. 277: 194-200. Polowick, P.L., D.S. Baliski, C. Bock, H. Ray, and F. Georges. 2009. Over-expression of ±-galactosidase in pea seeds to reduce raffinose oligosaccharide content. Botany 87: 526-532. Porch, T.G. 2001. Genetics and applications of heat tolerance in common bean. PhD Diss. Cornell Univ. N.Y. Porch, T.G. 2006. Application of stress indices for heat tolerance screening of common bean. J. Agron. Crop Sc. 192:390-394. Porch, T.G., M.W. Blair, P. Lariguet, C. Galeano, C.E. Pankhurst, and W.J. Broughton. 2009a. Generation of a mutant population for TILLING common bean genotype Bat 93. J. Am. Soc. Hort. Sci. 134: 348-355. Porch, T.G., M.H. Dickson, M.C. Long, D.R. Viands, and M. Jahn. 2004. GCA effects of reproductive heat tolerance in snap bean. J. Agric. Univ. Puerto Rico 88:161-164. Porch, T.G., V.H. Ramirez, D. Santana, and E.W. Harmsen. 2009b. Evaluation of drought tolerance in common bean germplasm in Juana Díaz, Puerto Rico. J. Agron. Crop Sc. 195:1-7. Posa-Macalincag, M.C.T., G.L. Hosfield, K.F. Grafton, M.A. Uebersax, and J.D. Kelly. 2002. Quantitative trait loci (QTL) analysis of canning quality traits in kidney bean (Phaseolus vulgaris L.). J. Am. Soc. Hort. Sci. 127:608-615. Rainey, K.M., and P.D. Griffiths. 2005a. Identification of heat tolerant Phaseolus acutifolius A. Gray plant introductions following exposure to high temperatures in a controlled environment. Gen. Res. Crop. Evol. 52:17-120. Rainey, K.M., and P.D. Griffiths. 2005b. Differential responses of common bean genotypes to high temperatures. J. Am. Soc. Hort. Sci. 130:18-23. Ramirez, M., M.A. Graham, L. Blanco-Lopez, S. Silvente, A. Medrano-Soto, M.W. Blair, G. Hernandez, C.P. Vance, and M. Lara. 2005. Sequencing and analysis of common bean ESTs. Building a foundation for functional genomics. Plant Phys 137:12111227. Repinski, S., and P.Gepts. 2009. The use of the soybean genome sequence for marker mapping and development in common bean. Annu. Rept. Bean Improv. Coop. 52:2-3. Rojas, M.R., C. Hagen, W.J. Lucas and R.L. Gilbertson. 2005. Exploiting chinks in the plant's armor: evolution and emergence of geminiviruses, Ann. Rev. Phytopathol. 43:16.116.39. Rosas, J.C., J.S. Beaver, M. Alameda-Lozada, D. Escoto, J.C. Hernandez, and R. Araya. 2005. Registration of Carrizalito Small Red Bean. Crop Sci. 45:2656-2657. Schlueter, J.A., J. L. Goicoechea, K. Collura, N.Gill, J-Y. Lin, Y. Yu, D. Kudrna, A. Zuccolo, C.E. Vallejos, M. Muñoz-Torres, M.W. Blair, J. Tohme, J. Tomkins, P. McClean, R. A. Wing, and S. A. Jackson. 2008. BAC-end sequence analysis and a draft physical map of the common bean (Phaseolus vulgaris L.). Genome. Tropical Plant Biol. 1:4048. Schneider, K., M. Brothers, and J.D. Kelly. 1997. Marker-assisted selection to improve drought resistance in common bean. Crop Sci. 37: 51-60. Schneider, K.A., K.F. Grafton, and J.D. Kelly. 2001. Genetic and QTL analysis of resistance to Fusarium root rot in bean. Crop Sci. 41:535-542. Schoonhoven, A. van, C. Cardona, and J. Valor. 1983. Resistance to the bean weevil and the Mexican bean weevil (Coleoptera:Bruchidae) in noncultivated common bean accessions. J. Econ. Entomol. 76:1255-1259. Schwartz, H.F., M.A.C. Langham, J. Golod, S.A. Tolin, J. LaForest, and K.F. Cardwell. 2009a. Legume ipmPIPEThe next evolution of web-based interactive tools for disease management and extension outreach. APSnet-http://www.apsnet.org/online/feature/ipmPIPE/ Schwartz, H.F., K. Otto, M.A. Brick, and J.B. Ogg. 2009b. Resistance to bacterial wilt in the Phaseolus core collection. Ann. Rept. Bean Improv. Coop. 52:68-69. Schwartz, H.F., K. Otto, H. Terán, M. Lema, M., and S.P. Singh. 2006. Inheritance of white mold resistance in Phaseolus vulgaris x P. coccineus crosses. Plant Dis. 90:1167-1170. Schwartz H.F., J.R. Steadman, R. Hall, and R.L. Forster. 2005. Compendium of bean diseases. Second Ed. APS press. St. Paul, MN. Seo, Y.-S., P. Gepts, and R.L. Gilbertson. 2004. Genetics of resistance to the geminivirus, Bean dwarf mosaic virus, and the role of the hypersensitive response in common bean. Theor. Appl. Genet. 108:786-793. Seo, Y.-S., J.-S. Jeon, M.R. Rojas, and R.L. Gilbertson. 2007. Characterization of a novel Toll/interleukin-1 receptor (TIR)-TIR gene differentially expressed in common bean (Phaseolus vulgaris cv. Othello) undergoing a defense response to the geminivirus Bean dwarf mosaic virus. Mol. Plant Pathol. 8:151-162. Silbernagel M.J., and R.M. Hannan. 1992. Use of plant introductions to develop U.S. bean cultivars. p 1-8. In H. Shands and L.E. Weisner (eds.) Use of plant introductions in cultivar development. Part 2. CSSA Spec. Publ. 20. CSSA, Madison, WI. Singh, S.P. 1995. Selection for water stress tolerance in interracial populations of common bean. Crop Sci. 35:118-124. Singh S.P. 1999. Production and Utilization. p. 1-24. In Singh S.P. (ed). Common bean improvement in the twenty-first century. Kluwer Academic Publishers, Boston, MA. Singh, S.P. 2001. Broadening the genetic base of common bean cultivars: a review. Crop Sci. 41:1659-1675. Singh, S.P., P. Gepts, and D.G. Debouck. 1991. Races of common bean (Phaseolus vulgaris, Fabaceae). Econ. Bot. 45:379-396. Singh, S.P., F.J. Morales, P.N. Miklas, and H. Terán 2000. Selection for bean golden mosaic resistance in intra- and inter-racial bean populations. Crop Sci. 40:1565-1572. Singh, S.P., C.G. Muñoz, and H. Terán. 2001. Registration of common bacterial blight resistant dry bean germplasm VAX 1, VAX 3, and VAX 4. Crop Sci. 41:275-276. Singh, S., H. Terán, M. Lema, H. Schwartz, P. Miklas. 2007a. Registration of white mold resistant dry bean germplasm A 195. J. Plant Registration 1:62-63. Singh, S.P., H. Terán, M. Lema, D.M. Webster, C.A. Strausbaugh, P.N. Miklas, H.F. Schwartz, and M.A. Brick. 2007b. Seventy-five years of breeding dry bean of the western United States. Crop Sci. 47:981989. Singh, S.P., H. Terán, H.F. Schwartz, K. Otto, and M. Lema. 2009a. Introgressing white mold resistance from Phaseolus species of the secondary gene pool into common bean. Crop Sci. 49: 1629-1637. Singh, S.P., H. Terán, H.F. Schwartz, K. Otto, and M. Lema. 2009b. Development of white mold resistant interspecific common bean germplasm lines VCW 54 and VCW 55. J. Plant Regist. 3:191-197. Sonnante, G., T. Stockton, R.O. Nodari, V.L. Becerra Velásquez, and P. Gepts. 1994. Evolution of genetic diversity during the domestication of common-bean (Phaseolus vulgaris L.). Theor. Appl. Genet. 89:629-635. Soto, M.J. and R.L. Gilbertson. 2003. Distribution and rate of movement of the Curtovirus Beet mild curly top virus (Family Geminiviridae) in the beet leafhopper. Phytopatology 93:478-484. Sousa, L.L., M.C. Gonçalves-Vidigal, P.S. Vidigal Filho, A.S. Cruz , and T.R. Costa. 2009. Allelic relationships of anthracnose resistance in MSU 7-1 line. Annu. Rep. Bean Improv. Coop. 52:48-49. Steadman, J.R., K. Eskridge, J. Costs, K. Grafton, J. Kelly, K. Kriecek, J. Kolkman, J. Myers, and P. Miklas. 2001. Evaluation of sources of resistance to Sclerotinia sclerotiorium in common bean with five methods at multiple locations. Annu. Rpt. Bean Improv. Coop. 44:89-90. Tako, E., R.P. Glahn, J. M. Laparra, R.M. Welch, X. Lei, J.D. Kelly, M.A. Rutzke, and D.D. Miller. 2009. Iron and zinc bioavailabilities to pigs from red and white beans (Phaseolus vulgaris L.) are similar. J. Agric. Food Chem. 57: 31343140. Terán, H., and S.P. Singh. 2009a. Gamete selection for improving physiological resistance to white mold in common bean. Euphytica. 167:271-280. Terán, H., and S.P. Singh. 2009b. Recurrent selection for physiological resistance to white mold in dry bean. Plant Breed. Doi:10.1111/j.1439-0523.2009.01679.x. Terán, H., C. Strausbaugh, I. Eujayl, J. Beaver, P.N. Miklas, and S.P. Singh. 2008. Response of BGMV and BGYMV resistant common bean to beet curly top virus. Ann. Rept. Bean Improv. Coop.51:166-167. Thibivilliers, S., Joshi, T., Campbell, K., Scheffler, B., Borerma, R., Xu, D., Cooper, B., Nguyen, H.T., Stacey, G. 2009. Generation of Phaseolus vulgaris ESTs and investigation of the regulation upon Uromyces appendiculatus infection. BMC Pl. Biol. 9:46. Thompson, M.D, H.J. Thompson, M.A Brick, J.N. McGinley, W. Jiang, Z. Zhu and P. Wolfe. 2008. Mechanisms associated with dose dependent inhibition of rat mammary carcinogenesis by dry bean (Phaseolus vulgaris L.). J. Nutr. 138: 20912097. Thompson, M.D, M.A. Brick, J.N. McGinley, and H.J. Thompson. 2009. Chemical composition and mammary cancer inhibitory activity of dry bean. Crop Sci. 49:179186. Tietyen-Clark, J. 1986. The benefits of bean. p. 1. In J.W. Andersen (ed.) Diabetes and Nutrition News. HCF Diabetes Foundation, Lexington, U.S.A. Urrea,C.A., R.M. Harveson, K. Nielsen, and J. Venegas. 2008. Identification of sources of bacterial wilt resistance in dry beans (Phaseolus vulgaris L.). Annu. Rept. Bean Improv. Coop. 51:56-57. Urrea, C.A., P.N. Miklas, J.S. Beaver, and R.H. Riley. 1996. A codominant randomly amplified polymorphic DNA (RAPD) marker useful for indirect selection of BGMV resistance in common bean. J. Amer. Soc. Hort. Sci. 121:1035-1039. Urrea, C.A., and T. Porch. 2009. Phenotypic evaluation of a subset of the Phaseolus vulgaris core collections and the P. acutifolius. Annu. Rept. Bean Improv. Coop. 52: 104-105. Urrea, C.A., J. R. Steadman, M.A. Pastor-Corrales, D.T. Lindgren, and J.P. Venegas. 2009a. Registration of great northern common bean cultivar Coyne with enhanced disease resistance to Common bacterial blight and bean rust. Journal of Plant Registrations 3: 219-222. Urrea, C.A., C.D. Yonts, D.J. Lyon, and A.E. Koehler. 2009b. Selection for drought resistance in dry bean (Phaseolus vulgaris L.) derived from the Mesoamerican gene pool in western Nebraska. Crop Sci. 49(6):2005-2010. Vallejo, V.A., and J. D. Kelly. 2008. Molecular tagging and genetic characterization of alleles at the Co-1 anthracnose resistance locus in common bean. ICFAI Univ. J. Genetics & Evolution 1:7-20. Vallejo, V., and J. D. Kelly. 2009. New insights into the anthracnose resistance of common bean landrace G 2333. The Open Horticulture J. 2:29-33. Vandemark, G. J., D. Fourie, and P. N. Miklas. 2008. Genotyping with real-time PCR reveals recessive epistasis between independent QTL conferring resistance to common bacterial blight in dry bean. Theor. Appl. Genet. 117:513-522. Vandemark, G. J, D. Fourie, R. C. Larsen, and P. N. Miklas. 2009. Resistance to common bacterial blight in red kidney and pinto bean populations is conditioned by the QTL SU91 in the presence or absence of QTL SAP6. Euphytica 170:371-381. Vandemark, G.J., and P.N. Miklas. 2005. Genotyping Common Bean for the Potyvirus Resistance Alleles I and bc-12 with a Multiplex Real-Time Polymerase Chain Reaction Assay. Phytopathology 95(5): 499-505. Velasquez V.R., and H.F. Schwartz. 2000. Resistance of two bean lines to wilt by Fusarium oxysporum f. sp. phaseoli under different soil temperatures. Agro-Ciencia 16:81-86.[in Spanish] Velez, J., M.J. Bassett, J.S. Beaver, and A. Molina. 1998. Inheritance of resistance to bean golden mosaic virus in common bean. J. Am. Soc. Hort. Sci. 123:628-631. Venette, J. R., and H.A. Lamey. 1998. Dry Edible Bean Diseases. NDSU Extension Ag. Natl. Res. Pub. No. PP576. Vidigal Filho, P.S., M.C. Gonçalves-Vidigal, J.D. Kelly, and W.W. Kirk. 2007. Sources of resistance to anthracnose in traditional common bean cultivars from Paraná, Brazil. J. Phytopathology 155:108-113. Waines J.G., and B. Ehdaie. 2007. Domestication and crop physiology: Roots of green revolution wheat. Ann.Bot. 100:991-998. White, J.W., M. Ochoa, P. Ibarra, and S.P. Singh. 1994. Inheritance of seed yield, maturity and seed weight of common bean (Phaseolus vulgaris) under semi-arid rainfed conditions. J. Agric. Sci. 122:265-273. White, J.W., and S.P. Singh. 1991. Breeding for adaptation to drought. p. 501-560. In A. van Schoonhoven and O. Voysest (eds.) Common beans: Research for crop improvement. C.A.B. Int., Wallingford, U.K. and CIAT, Cali, Colombia. Wong, J.H., X.Q. Zhang, H.X. Wang, and T.B. Ng. 2006. A mitogenic defensin from white cloud beans (Phaseolus vulgaris L.) Peptides 27:2075-2081. Wright, E.W., H.E. Awale, and J.D. Kelly. 2008. Use of TRAP markers to map resistance to a new race of common bean rust in Michigan. Annu. Rep. Bean Improv. Coop 51:210-211. Wright, E.W., H.E. Awale, M.A. Pastor-Corrales, and J.D. Kelly. 2009. Persistence of a new race of the common bean rust in Michigan. Annu. Rep. Bean Improv. Coop 52:84-85. Zapata, M. and J.S.Beaver. 2005. Differential leaf reaction of common bean lines to pathogenic races of Xanthomonas axonopodis pv. phaseoli from Costa Rica, Nicaragua, and Puerto Rico. Annu. Rpt. Bean Improv. Coop. 48:102-103. Zapata, M., J.S Beaver, and T. Porch. 2009. Evidence for a dominant gene on leaves of common bean to the common bacterial blight pathogen, Xanthomonas axonopodis pv. phaseoli. Annu. Rpt. Bean Improv. Coop. 52:72-73. Zhou, Y.-C., E.R. Garrido-Ramirez, M.R. Sudarshana, S. Yendluri, and R.L. Gilbertson. 2007. The N-terminus of the Begomovirus nuclear shuttle protein (BV1) determines virulence or avirulence in Phaseolus vulgaris. Mol. Plant-Microbe Interact. 20:1523-1534.