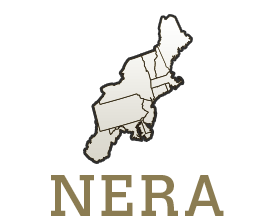
NE1034: Genetic Bases for Resistance and Immunity to Avian Diseases
(Multistate Research Project)
Status: Inactive/Terminating
NE1034: Genetic Bases for Resistance and Immunity to Avian Diseases
Duration: 10/01/2008 to 09/30/2013
Administrative Advisor(s):
NIFA Reps:
Non-Technical Summary
Statement of Issues and Justification
STATEMENT OF THE ISSUE Disease losses represent a significant component in the overall cost of poultry production. These costs not only include the direct losses due to increased mortality and condemnations but also increased production costs caused by suboptimal food conversion, cost of vaccines and vaccination. Several pathogens, e.g., chicken infectious anemia virus (CIAV), may cause subclinical infections that interfere with protective immune responses to other pathogens. In some cases, pathogens are in a continuing 'arms race' with vaccines and genetic resistance in becoming more pathogenic as was eloquently described by Witter (2001) for Marek's disease. In order to battle these pathogens it will be essential to increase our understanding of the genetic bases for disease resistance and immunity, which will lead to more effective prevention and treatment procedures. These new methods will increase production efficiency and lower costs.
JUSTIFICATION
World-wide consumption of poultry products has increased drastically during the last 30 years and is expected to increase over the next decades (Roenigk, 1999). Poultry consumption rose world-wide from 26 million metric tons in 1984 to an estimated 66 million metric tons in 2004, an increase of 154%, while the world population increased with 36% during the same period. The increase in poultry consumption is also reflected in the per capita consumption which increased from 6 kg in 1984 to 12 kg in 2004 based on world-wide data (http://www.north-south.nl/files/Peter/Brazil/Poultry%20Brazil.ppt). The continuing increase in poultry production has important economic benefits for the USA. The United States has one of the worlds most efficient poultry production systems and is currently an exporter of poultry products producing approximately 15,539,000 metric tons in 2004. That number reflects an annual increase of 8.7% since 2000 (http://www.north-south.nl/files/Peter/Brazil/Poultry%20 Brazil.ppt). In India, poultry production almost doubled between 1999 and 2004 and currently represents approximately 8% of the Gross National Product. Further growth in broiler production is expected in the USA and certainly in Asia as well as Latin America. It is anticipated that the USA poultry producers will profit from increased worldwide demand based on the highly efficient production in this country. In addition, poultry feed is also largely dependent on the USA grain and soybean production, providing further benefits to the economy. The impact of diseases is one major impediment for increased productivity. Total losses caused by specific diseases not only include mortality, decreased egg production, and condemnations but also costs of vaccination, chemotherapy, and eradication programs. Although there are no recent published data available on these losses, Biggs (1982) quoted a loss of $1,427 x 106 in 1975 for the US alone. Witter and Schat (2003) estimated that the total losses (mortality, vaccination costs, reduced egg production) caused by Marek's disease (MD) in 1984 were close to one billion dollars worldwide. Recently, Morrow and Fehler (2004) estimated the annual loss for MD to be between US$ 1 and 2 billion, although they admitted that this was a crude estimate. This is in part caused by the continuing evolution of MDV to more pathogenic strains. Currently, the so-called vv+MDV strains are causing MD outbreaks in chickens properly vaccinated with MDV (Witter, 2001) although the increased use of the Rispens vaccine (Rispens et al., 1972) seems to be able to control the disease at this time. However, the concern is that a further increase in pathogenicity will leave the poultry industry susceptible to increased losses. Recent problems with Salmonella enteriditis have caused considerable economic losses and further losses can be expected. For example, if FDA suggestions for S. enteriditis elimination are implemented, the costs of table eggs will increase dramatically (Dr. D. Kradel, personal communication). The losses due to S. enteriditis in the U.S. are based on the human health impact of only a small number of positive hens. In addition to the clearly identifiable problems, a substantial portion of the losses is caused by suboptimal production as a consequence of interactions among management, genetic resistance and disease agents (Biggs, 1982). Reduction of these losses depends on several interrelated factors; the interaction between genetic background of the chicken and the development of the immune responsiveness is especially relevant. Immune responsiveness is at least in part determined by the major histocompatibility complex (MHC) as well as by other genetic traits. The collaborating NE-1016 stations have demonstrated MHC-related resistance to a number of diseases. Information demonstrating that genetic selection related to immunity may reduce economical losses is important, because successful adaptation of appropriate selection procedures by primary breeders may lead to a rapid dissemination of more resistant strains and a subsequent reduction in losses. NE-1016 has invited representatives of breeder organizations to the annual meetings to disseminate new information on an informal basis. These breeder representatives also bring information on field problems and comments on the importance of approaches taken by NE-1016. These aspects will become even more important with the advent of biotechnology applications based on the completion of the chicken genome sequence. The chicken genome has been published although there are still some sequence gaps. An important part of the sequence of the MHC related genes is also available (Kaufman et al, 1999) and additional information on the MHC sequence has been provided. These data will be important toward development of tailored vaccines presenting epitopes that are recognized by specific haplotypes. The ADOL station has already shown that certain genetic strains respond differently to different MD vaccines, while several groups (including NYC and ADOL) have used MDV BAC clones and overlapping phage clones for the identification of MDV genes involved in pathogenicity, immunogenicity and immune evasion. The combined data from these groups indicate that tailored vaccines may become a distinct possibility in the future and research towards this goal is included in this proposal. Similarly, several NE-1016 groups are working on the regulation of immune responses by studying cytokine activation pathways that are important for innate and acquired immune response. In the outlines of the previous two five-year projects, we predicted a rapid progress in the understanding of the interactions between genetic background and disease resistance in the next decade. This prediction was based, in part, on the development of MHC-congenic strains and the development of monoclonal antibodies (Mabs) for lymphocyte (sub)populations by several participating stations of NE-1016. Indeed, the stations participating in NE-60 and subsequently in NE-1016 have made significant contributions to the rapid progress in our understanding of the interactions between genetic resistance to disease and immune responses to several important pathogens. This is very clearly illustrated in the contributions by NE-1016 members to the new book on Avian Immunology (available early 2008) with 7/22 chapters and one of the two appendices contributed by NE-1016 members. The book was co-edited by a NE-1016 member. The impact of NE-1016 is also evidenced by the continuing high productivity of peer reviewed papers with more than 247 papers published or in press both of which include a substantial number of joint publications among NE-1016 stations (Publications list). In addition, three NE-1016 members were recognized during the last 3 years for their contributions with the the Poultry Science Association Merck Award for Achievement in Poultry Science. Further progress in improving genetic resistance to diseases will require the application of advanced techniques in immunology, biochemistry, virology, bacteriology, parasitology, molecular biology and genetics to study pathogens using well-defined strains of chickens in facilities designed to contain infectious agents. NE-1016 members form the ideal team to pursue the proposed studies, because they have: i) a proven collaborative research record, and ii) as a group the needed expertise listed above.
Related, Current and Previous Work
Extensive publication searches indexed in the comprehensive databases (Agricola, Biosis, CAB, CRIS, Health Index, and Medline) for the last five year period revealed substantial scientific contributions that NE-1016 members have made in genetics of disease resistance and immune response in poultry. The truly essential, cooperative, multidisciplinary nature of the project is illustrated by the many joint-authored publications among participating stations. Other groups outside the U.S. conduct research complimentary to that of NE-1016. Institutions in Australia, Czech Republic, Denmark, France, Israel, the Netherlands, and the U.K. conduct research on the chicken MHC. Selection based on non-MHC traits is also performed in the Netherlands, France, and Israel. Marek's disease research programs exist in Japan and the Netherlands. Working relationships, either formal or informal, exist between NE-1016 stations and the international laboratories conducting similar research. This assures coordination of efforts and avoidance of unnecessary research duplication. Addition of international contributors from Canada and the Netherlands to the new proposal demonstrates the stature of the project. The NE-1016 project addresses issues in poultry genetics or disease not investigated by other multistate projects related to poultry. Two projects have no emphasis on immunity or disease. NE-127 "Biophysical models for poultry production systems" studies physiological responses to various environmental factors. Project S-1037 "Integrative functional and physiological genomics of poultry" focuses on genetic variation in performance traits. Another project, NC-1019 "Control of emerging and re-emerging poultry respiratory diseases in the United States" examines respiratory diseases. The NC-1008 project "Advanced technologies for the genetic improvement of poultry" may be the most related to NE-1016. Although certain gene families are being examined in both projects, the two efforts remain quite distinct in their objectives and application of work with these genes. The NC-1008 project identifies and maps poultry genes while developing strategies for incorporating new genes into selection programs. By contrast, the NE-1016 project studies poultry genes to elucidate their role in genetic resistance and immunity. The NRSP-8 National Animal Genome Research Program emphasizes mapping the genomes of various agriculturally important animal species including chickens. Several stations (CA, DE, IA, NC) as well as collaborators participate on both NC-1008 and NE-1016 technical committees. This representation will enhance communication to coordinate complementary efforts, encourage appropriate joint efforts and avoid duplication. Other NE-1016 members also interact with NC-1008. Collaborative efforts between both technical committees are expected to continue. For example, genes important for production traits identified by NC-1008 can be evaluated for effects on immunity and disease resistance. Disease resistance genes identified by NE-1016 can be examined for their production effects by NC-1008. Likewise, lines selected for particular characteristics by NE-1016 may be used for NC-1008 genome mapping efforts, whereas transgenic chickens or new selection methods developed by NC-1008 may be examined for use in immune response and disease resistance studies in NE-1016. Two other U.S. laboratories that are NC-1008 members conduct poultry genetics research relevant to immunity and disease resistance. OH has studied the genetics of disease resistance in turkeys and the turkey MHC. VA has selected lines for antibody response to SRBC and has used these lines to examine the relationships among blood groups, MHC and disease resistance. Various laboratories have made progress in elucidating the molecular structure of MHC genes and antigens as well as their sequences. Continued work is needed to define additional polymorphisms, regulation of gene expression and their relationships to disease resistance. Studies of the MHC association with diseases have augmented identification of beneficial alleles for resistance to Marek's disease virus, Rous sarcoma virus, Salmonella enteriditis, and Eimeria species. These diseases represent only a small fraction of the pathogens that can affect poultry so the repertoire of pathogens under study must be expanded. A more detailed understanding of the pathogen-host relationship is also needed. Most studies have utilized genetic stocks of the egg-laying type but this work is now expanding into meat-type birds. All these components of chicken MHC research will be addressed by the proposed studies in this project renewal. The NE-1016 multistate research project scientists design, create, maintain, and study unique poultry genetic lines. Some members carry out all of these functions and others a subset as an integral part of our research. These efforts have been our contribution as well as our responsibility to achieve the project objectives of understanding the genetic basis for immunity to disease. Special genetic lines, established over the last 75 years, are at risk at many research stations. If lost, these unique avian genetic resources (e.g., congenic, recombinant, and inbred lines) are unlikely to be recreated. Since member scientists share these genetic resources in collaborative research, their elimination will impact the project, collaborators as well as the avian research community at large. The Technical Committee recognizes the imperative to conserve the resources currently available. Several Technical Committee members served on the Avian Genetic Resources Task Force and are now part of the National Animal Germplasm Program, Poultry Committee. The members are committed to the establishment of a national system of networked researchers and a site for orphaned stock conservation to support our objectives of understanding and improving resistance to disease in poultry. Innovative technologies such as candidate gene indentification, applications of recombinant DNA, monoclonal antibodies, DNA probes, and QTL analysis, have been effectively used to identify and characterize many facets of disease resistance or immune function. These techniques expand upon the pioneering work conducted by NE-1016 members throughout the project history. Project results continue to be an important and readily applicable in both research and industry. Commercial poultry breeders lead other animal breeders in terms of improvement of a variety of economic traits, including genetic resistance to disease. Further research on new methods to select for disease resistance in poultry must, and will, continue in the NE-60 project. Recent scientific advances in understanding the immune system, and enhanced knowledge about poultry pathogens, promise imminent, significant improvements in poultry health, production efficiency, food safety and animal well-being through genetic selection.
Objectives
-
Identify and characterize genes and their relationships to disease resistance in poultry with an emphasis on the major histocompatibility complex as well as other genes encoding alloantigens, communication molecules and their receptors and other candidate systems.
-
Identify and characterize environmental, dietary and physiologic factors that modulate immune system development, optimal immune function and immune system related disease resistance and welfare in poultry genetic stocks.
-
Develop, evaluate and characterize methodologies, reagents and genotypes to assess immune function and disease resistance to enhance production efficiency through genetic selection in poultry.
Methods
OBJECTIVE 1. Two stations will examine the structure and functional characteristics of major histocompatibility complex (MHC) genes. Cytogenetic and molecular analyses will investigate the comparative chromosomal organization of GGA 16 in chicken and other poultry and avian species to evaluate locus spatial relationships in regard to centromeres and telomeres to understand the evolution of this chromosome within and among species [CA]. BRI will expand the gene map for the MHC by identifying genes in regions not yet mapped. This work will define boundaries of the B complex and MHC-Y regions relative to adjacent segments of chromosome 16 including the nucleolar organizer region, centromere and telomeres. NIU will differentiate MHC-YF haplotypes in NIU Lines, AR Regressor and Progressor Lines, and commercial lines. MHC-YF seems to recombine at a higher rate with more variability than its B-F counterpart. WAU will further refine characterization of MHC 'haplotypes' covering the presence of single nucleotide polymorphisms (SNP), in genes other than the classical MHC Class I and II. DNA sequencing based polymorphism in the TAP1, TAP2 and tapasin genes has been performed for MHC haplotypes segregating in the WAU SRBC antibody selection lines. The station will analyze other genes located more distal from tapasin such as the BG locus and regions for innate immunity receptor genes [WAU]. BRI will assess MHC variability identifying polymorphic sequences and crossover breakpoints positions that contribute to recombination. The data will be assembled into a useful database that will be made available to the scientific community. CA has shown that certain MHC-Y alleles are associated with an increased Marek's disease (MD) incidence, these alleles were evaluated in a highly resistant B11 background. An inbred Ancona X White Leghorn parent stocks that are either homozygous or heterozygous for B2 or B5, congenic for background genes, and varied for the MHC-Y system alleles will be used by CA to evaluate MHC-Y effects. The MD pathogenesis studies will be conducted through gross and microscopic lesions. Blood will be obtained for serum chemistry analysis. Global transcriptional analysis profiles will be conducted (IA) on tissues or cells from chickens that demonstrate either high or low pathology after E. coli challenge, and both groups will also be contrasted with unchallenged birds. Comparative genomic analysis (chicken vs pig) will be conducted on transcriptional profiles of animals that are challenged with Salmonella to identify a set of avian-mammalian consensus genes that are differentially regulated with exposure of hosts to Salmonella. TX will identify and characterize genes related to bacterial infections, Salmonella and Campylobacter, in chickens using microarray and q-RT-PCR Microarray technology will be employed to conduct a global gene expression profiling in Marek's disease virus (MDV)-infected chickens. Chickens from lines 63 (MD-resistant) and 72 (MD-susceptible) will be inoculated with a pathogenic strain of MDV. RNA samples isolated from the spleen tissues of birds at 5 and 14 dpi (cytolytic and latency phases of MDV infection, respectively) will be used for array analysis of host and viral gene expression (ADOL). If differences occur in ADOL lines 63 and 72 then a series of 19 ADOL Recombinant Congenic Strains can be used to explore the genetic basis. ADOL will investigate the molecular mechanisms of vaccine protection in MD-susceptible line 72 in a microarray experiment. ADOL will identify genes and elucidate specific pathways associated with modulation of key immune components induced by vaccination and MDV transcriptional changes that produce the innate immune suppression and evasion of adaptive immunity in non-vaccinated chickens. This study will use a comparative comprehensive gene expression profiling in vaccinated and naïve chickens challenged with a highly pathogenic and oncogenic strain of MDV. GUELPH will further characterize gene and protein expression associated with genetic resistance/susceptibility to MD using the immune system microarray developed at the station. The studies will focus on Th1/Th2 responses to MDV in B19 and B21 haplotypes. The molecular biology of MDV evolving pathogenesis will be the focus at DE. Mutations in MDV and their effects on virus pathogenicity as well as immune evasion will be evaluated. NH and NC will collaborate to measure gene expression during development in immune tissues (bursa, thymus, and spleen) from high and low antibody selected lines from NC. RNA from these tissues will be examined on immune focused microarrays in prehatch development. Gene expression in the antibody selected lines may be compared with the WAU lines selected against the same antigen. Genes expressed in lines selected for pulmonary hypertension resistance and susceptibility will be analyzed before and after disease induction by NH and NC. Heart, lung and liver RNA will be placed on an immune focused microarrays. CA will study of the telomere-stability and telomerase-regulation pathway controlling cellular senescence, immortalization and transformation of cells with an emphasis on MD. Infected cells and tumors from specific genotypes during the disease course will be investigated using cytogenetic, genetic and genomic approaches. Comparative differential gene expression analysis using real-time RT-PCR in MDV-infected chickens at different time points will be conducted at ADOL. Studies on the spleen and brain samples from MDV-infected chickens, indicate that the expression levels of many soluble components of immune system, including pro-inflammatory cytokines (IFNs, IL-1b, IL-6, IL-8, and IL-18) are up-regulated as early as 3 dpi. High transcriptional activity persists up to two weeks after inoculation. High expression levels of proinflammatory cytokines have been implicated in the neurological disorder associated with MD. CA will complete the evaluation of B11 MD resistance in inbred Ancona X White Leghorn chickens. Progeny chicks from this stock will be compared to White Leghorn chicken lines either homozygous or heterozygous for MD resistant B21 and MD susceptible B19 haplotypes in MD pathogenesis studies that examine gross and microscopic lesions. Blood will be obtained from all birds for serum chemistry analysis. Avian cellular immune memory responses to avian influenza virus (AIV) and infectious bronchitis virus (IBV) will be examined by WUHS using both intramuscular inoculation of DNA plasmids and respiratory inoculation of non-replicating adenovirus vector expressing viral proteins. The nature of chicken memory CD8+ T cells specific for AIV will be determined including cell response kinetics, AIV protein specificity of the T cell response and quantitative and qualitative phenotype characteristics of T cell responses. Research will identify immune molecules that regulate memory T cell responses. Experiments will examine in vitro effects of cytokines and accessory antigens and in vivo potential for cytokines of preference to modulate memory T cells. Factors responsible for differences in clinical illness of distinct MHC haplotypes following IBV infection will be investigated [WUHS]. Differences for both adaptive and innate immunity, in the responses of NK and macrophages/monocytes, B cells and T cells following infection with IBV will be identified. The clinical, histopathological and viral parameters will be compared among the B2, B5, B8, B12, B19 and B21 types following infection with nephropathogenic Gray and respiratory tropic Ark viruses. Research will define the innate responses, B lymphocyte responses, and T lymphocyte responses of lines of chickens with representative patterns of infection. The NCSU laboratory will investigate innate immune gene expression following viral infection. Studies will examine in vitro infections of primary cells from distinct genetic lineages and differences in the innate gene response kinetics and profiles following infection to different viral families. Additional studies will examine the localized expression of innate antiviral genes of the poult intestine following enteric viral infection. Continuing with the innate immune response, WAU will measure differences in Toll-like receptor (TLR) and other innate receptor mRNA and protein expression using the WAU SRBC antibody selection lines. Similarly, line differences in cytokine expression levels and B-cell idiotype characteristics will be measured. AR will continue collaborative studies with the University of Uppsala, Sweden to identify genes of the mutant Smyth line chicken involved in the genetic susceptibility to develop autoimmune vitiligo. NIU, NH and MS will collaborate to identify the precise nature of the non-MHC alloantigen systems in order to determine how they influence disease resistance or susceptibility. These two stations have made progress in identifying the L system antigen and will continue to work on the other alloantigen systems. The L system effect on MD infection will be evaluated in additional trials on the non MHC alloantigens [CA]. OBJECTIVE 2. The AR station will investigate the etiopathology of autoimmune vitiligo in the mutant Smyth Line (SL) chicken with emphasis on 1) innate and adaptive immune system components involved in the development and onset of vitiligo; 2) target cell (pigment cell) alterations; and, 3) environmental factors, such as HVT and other MDV serotypes as well as various inflammatory stimulators. These studies involve primarily the use of B101 MHC-matched lines of chickens including the Light Brown Leghorn line (LBL101 control, vitiligo resistant), the Brown line (BL101 parental control, vitiligo-susceptible; but <2% incidence of vitiligo) and the Smyth line (SL101, vitiligo-susceptible; between 75-95% incidence). NYC will continue studies to determine if immune complex vaccines consisting of antigen and antibody can prevent immunosuppression. The station will also determine the mechanisms of chicken anemia virus (CAV)-induced immunosuppression by examining the effects on cytokine expression. Protection will be examined by using differential quantitative (q)PCR assays. GUELPH will examine host responses to MDV infection in various tissues, especially in the feather. The station will also attempt to devise means to control virus replication and shedding from the feather. Another AR project contribution will focus on the role of innate immunity in pulmonary hypertension. This research endeavor will use pulmonary hypertension resistant and susceptible broiler lines to examine the interplay of vasoconstrictors and vasodilators, as well as, endothelial cells, smooth muscle cells and leukocytes in the susceptibility/resistance to pulmonary hypertension syndrome (ascites). A new initiative will focus on understanding the inflammatory response in poultry and the qualitative differences of innate immune activities initiated by different types of pathogen-associated molecular patterns (PAMP). Studies on the effect of supplementation of homotopes (PAMP), protein antigens and natural antibodies (Nabs) in vivo and in vitro on immune responsiveness of poultry will continue at WAU. Emphasis areas are intratracheal and or intestinal exposure to mimic the effects of environmental exposure to microbiota and antigens on immune competence of chickens. The repeatedly observed refractory responses of chickens to PAMP, both immunologically as well as physiologically suggest that chickens are able to adapt to the environment, which may be initiated at a very young age. The effect of probiotics on the maturation of the neonatal intestinal immune system will be studied. The high levels of NAb to carbohydrate residues in chickens comparable to those in man suggest an important role of NAbs in disease resistance and health maintenance. WAU will explore active or passive innate vaccination using NAb and PAMP of young chickens to adapt to an environment with high microbial pressure. The changing husbandry conditions in Europe demand a chicken which is able to resist infection with low metabolic costs. Dietary immunomodulators will be evaluated for their impact on expression of cytokines and other immune-related genes, under normal husbandry conditions and with immune-activating treatments (LPS injection or differing hygiene levels) [WAU]. The impact of dietary immunoenhancers on cellular response to Salmonella challenge will be assessed in vitro and in vivo [IA]. NC will examine the effect of probiotic supplementation on poultry immune function to understand the physiological mechanisms involved in modulating the expression of immune, kinetics, and activity of the host response to antigenic challenge. The role and the induction of mucosal immunity in chickens will be examined [AL]. Experiments will address the induction of mucosal immunity using various vaccine strategies, including live vectors and the use of mucosal adjuvants. The role of mucosal immunity to protect against infectious diseases in various chicken lines will be addressed. Avian influenza, immunosuppressive viruses and immune mechanisms pertaining to mucosal immune protection are the principal emphasis areas for these studies [AL]. Thrombocyte mRNA sampled from different commercial genetic stocks will be examined for gene expression [SC]. This stations experiments will determine the array of genes for cell surface molecules, signal transduction proteins and cytokines expressed by thrombocytes that are either stimulated in vitro with thrombin, collagen, as well as bacterial and viral reagents or unstimulated controls. OBJECTIVE 3. One of the hallmarks for the NE-1016 stations has been the development of chicken strains genetically-selected that have facilitated evaluation of resistance and/or susceptibility to disease. Special B congenic chicken strains that differ only at the B complex have been produced by repeated backcrossing of certain B haplotypes to an inbred line [CA, IA, NC, ARS-ADOL]. Highly inbred lines and experimental chicken lines selected for unique immune functions are available from several stations [AR, CA, IA, NC, NIU, NYC, ARS-ADOL, WAU]. These populations remain valuable genetic resources for research conducted by collaborating NE-1016 members and other research institutions. The planning, cooperation and sharing of resources or expertise which takes place through participation in this project enhances discovery. Preservation of specialized genetic lines is dependent upon adequate financial resources. Loss of funding eliminated the NH genetic stocks which had been developed for more than 25 years. Some of the NH stocks were transferred to NC and NIU. These include some congenic lines containing MHC recombinants on the Line UCD 003 background. MHC recombinants are valuable to assess specific genetic control of immune responses and disease resistance. The IA Station will maintain genetic lines of chickens for use in immune-related studies. Many of the IA long-term (10 to 100 generations) lines have been characterized for specific genes or features of immune responsiveness. These will include highly inbred lines in MHC-congenic pairs and trios, and advanced intercross lines for fine-mapping. Primers for immune-related genes will be developed and their use for quantitative RT-PCR optimized. Correlations of immune-gene expression with parameters of growth and fitness will be assessed. Chicken genetic resources at CA will be conserved by maintaining genetic stocks of chickens and promoting their usage by other experimental researchers within and external to the project. Research will be conducted to explore molecular genetic variation (SNP comparisons) within and among inbred and MHC-congenic genetic lines of chickens held at CA to investigate mutation rates across the genome and at specific loci. ADOL will maintain eight B-congenic lines and B2B2 recombinant congenic strains (RCS) that reveal non-MHC genes influencing tumor resistance and immune development. NYC plans to maintain the P2a (B19B19C2C2) and N2a (B21B21C2C2) SPF flocks and continue monitoring chicken infectious anemia virus (CIAV) serology. Four additional MHC recombinants designated as R101, R103, R105 and R109 have been described at NIU. BRI and NIU will evaluate and map breakpoints between B-F and B-G regions in these recombinants. NIU will produce antisera used in haplotyping MHC and non-MHC alloantigen systems found in the chicken. This station will also develop the stocks useful for evaluating the function of alloantigen systems. Cross-line hatching eggs and chicks will be supplied to interested collaborators for challenges involving immune response effects of the alloantigen systems. NYC plans to examine if genetic differences exist in early immune responses to MD vaccines that protect against MDV replication. The station will examine the early immune response between 1 and 5 days post infection (dpi). One-day-old P2a and N2a chick will be vaccinated with serotype 1, 2, and 3 vaccines or infected with MDV strains representing virulent, very virulent, and very virulent + pathotypes. Cytokine profiles and MDV expression using qPCR and qRT-PCR will be determined for spleen cells harvested at 1 to 4 days post inoculation. Experiments wil measure cytotoxic T lymphocyte (CTL) activity against a mutant MDV protein (glycoprotein L or gL) from the most very virulent strains of MDV. The gL of less virulent MDV strains is recognized by CTL from genetically resistant but not susceptible chickens. The work will develop target cells expressing the mutant protein in vitro for use as CTL assay target cells. GUELPH will continue work to construct MHC class I and II tetramers to identify peptide-restricted T cells in MDV-infected chickens. The station will develop real-time PCR assays for measuring immune system genes in the chicken, including genes associated with Th1 and Th2 responses as well as those involved in regulation of T cell response. In collaboration with a breeding company, DE will be aiding in the genetic selection of commercial lines for MDV-resistance via MD challenge and mating of surviving families. Turkey-specific assays and reagents which include recombinant protein, antibodies, and molecular reagents for real-time RT-PCR assays to assess cytokine expression and immune function will be developed by NC. Nucleotide analysis of chicken cytokine genes available in the GeneBank database will be used to design degenerate primers to amplify homologous genes from turkey mRNA. Resulting RT-PCR product will be confirmed by sequence analysis. Complete gene sequence will be used to design specific diagnostic tests. RT-PCR and CDR3-VH spectratyping will be further optimized by WAU to characterize both cytokine regulation as well as the T-cell and B-cell receptor repertoire (idiotype) of chickens of various breeds and ages, kept under various husbandry and management conditions. This work will investigate the adaptive capacity of poultry to changing environments. TX will develop a chicken microarray to study gene expression profiling in different environments. WAU will use SNP typing methods on different platforms to evaluate genetic effects on measured phenotypes related to immune function. This will be applied to haplotypes present many genetic and commercial breeding stocks in cooperation with members of the NE 1016 group. AR will develop assay techniques to examine qualitative and quantitative aspects of cellular immune responses. CA will analyze serum enzymes following MD pathogenesis trials. Serum will also be analyzed from challenge trials conducted with the following disease agents: E. coli, S. enteritidis, P. multocida, and E. tenella. Results will be compared to those obtained from age matched uninfected control birds. CA will also analyze serum enzymes associated with other disease conditions obtained through other project collaborators.Measurement of Progress and Results
Outputs
- Maintain unique genetic resources including selected lines, inbred, congenic and recombinant congenic lines as well as experimental lines. Develop additional genetic material as needs arise.
- Profile gene expression in normal and disease conditions including Mareks disease, avian influenza and infectious bronchitis using microarray technology, real-time PCR or other methods.
- Identify individual genes or quantitative trait loci (QTL) associated with disease resistance or immune response via DNA markers, microarray technology or single nucleotide polymorphisms (SNP).
- Develop new single nucleotide polymorphisms (SNP) markers, microarrays, peptides, antisera, primer sets, and serum chemistry analyses to categorize immune responses in normal and disease states. Use genetic, dietary and immunization methods to enhance protective immunity.
- Use referred publications, symposia, invited lectures and informal discussions at national and international meetings to distribute information to stakeholders.
Outcomes or Projected Impacts
- Identified individual genes or quantitative trait loci will improve poultry health and animal agriculture in general through breeding or technological applications.
- Knowledge of basic mechanisms of immunity and disease resistance will increase through project efforts. This knowledge will allow development of new technologies to assess or improve the immune response.
- Greater immune responses and improved disease resistance will augment production efficiency.
Milestones
(2009): Generate line crosses, new breeding stock and other resource populations, to produce experimental progeny for the proposed studies.(2009): Identify new candidate genes or quantitative trait loci (QTL) associated with disease resistance or immune function through DNA markers, microarray technology or single nucleotide polymorphisms (SNP).
(2010): Improve microarrays using newly identified genes to more effectively assess differential gene expression in the immune system.
(2010): Classify specific dietary ingredients that increase or decrease immune function for use as immune modulators. Use new formulated additives (specific ingredients alone or in combination) to assess improved responses against disease.
Projected Participation
View Appendix E: ParticipationOutreach Plan
Industry stakeholders are invited to and frequently attend the annual project meetings. Their attendance provides an opportunity for information exchange. For example, breeder organizations representatives can learn of the latest genetic advances in disease resistance from the project scientists. Technical committee members gain knowledge of emerging field problems that the project can address through experiments. The combined efforts of the NE-1016 stations will generate new scientific data. Referred publications, symposia, invited lectures and informal discussions are some methods used to disseminate information. Project investigators have made significant scientific contributions to the improvement of poultry immune responses as well as the genetics of disease resistance. Cooperation among project members and with other researchers will remain a hallmark of NE-1016. This cooperative effort will include sharing scientific expertise and genetic resources held at numerous project stations. The addition of several international members has expanded the research scope.
Organization/Governance
The planning and supervision of the Multistate Research project shall be the responsibility of the Multistate Technical Committee. The membership of this committee shall consist of an Administrative Advisor, a technical representative of each participating agency or experiment station, and a representative of the USDA Cooperative State Research Education and Extension Service (CSREES). The voting membership shall consist of the Technical Committee Representatives.
The Technical Committee shall be responsible for review and acceptance of contributing projects, preparation of reviews, modification of the multistate project proposal, and preparation of an annual report for transmittal by the Administrative Advisor upon approval to CSREES. Annual written reports will be prepared by each technical committee member and distributed at the annual meeting. A limited number of the compiled annual reports will be available upon request from the Administrative Advisor.
The Technical Committee will meet yearly and elect a secretary, who will serve the year after election and as the chairperson the following year. An Executive Committee will be formed to conduct all business of the Technical Committee between annual meetings. The Executive Committee shall consist of the current Technical Committee Chairperson, the Secretary, and the two immediate Past Chairpersons.
The chairperson may name other subcommittees as needed to perform specific assignments. They may include subcommittees to develop procedures, manuals, and phases of the multistate project, to review work assignments; to develop research methods, to prepare publications, and to write proposals.
Other agencies and institutions may participate and vote at the invitation of the Administrative Advisor. Minimum expectations for Technical Committee members are submission of a written annual report every year, and attendance at an annual meeting including presentation of research results at least one year out of two. Collaborators may include emeritus members with an interest in attending annual meetings, scientists who wish to contribute by virtue of having special expertise or interest, and those who engage in research interactions with an individual Technical Committee member. Collaborators should submit a written annual report every year, and present their progress when attending the annual meeting. Guests who attend an annual meeting through special connection to the Technical Committee (i.e. host institution) are invited to make a brief presentation of their interests and ongoing research.
Literature Cited
The project proposal was developed from information in these references. Abasht, B., J. C. Dekkers, and S. J. Lamont. 2006. Review of quantitative trait loci identified in the chicken. Poult. Sci. 85:2079-2096. Abdul Careem, M.F., B.D. Hunter, A. J. Sarson, A. Mayameei, H. Zhou, and S. Sharif. 2006. Mareks Disease Virus-induced transient paralysis is associated with cytokine gene expression in the nervous system. Viral Immunol. 19:167-176. Abdul-Careem, M. F., B. D. Hunter, É. Nagy, L. R. Read, B. Sanei, J. L. Spencer and S. Sharif. 2006. Development of a real-time PCR assay using SYBR Green chemistry for monitoring Mareks disease virus genome load in feather tips. J. Virol. Methods 133:34-40 Abdul-Careem, M. F., B. D. Hunter, P. Parvizi, H. R. Haghighi, N. Thanthrige-Don and S. Sharif. 2007. Cytokine gene expression patterns associated with immunization against Mareks disease in chickens. Vaccine 25:424-432. Abdul-Careem, M. F., D. B. Hunter, M. D. Lambourne, J. Barta, and S. Sharif. 2007. Ontogeny of cytokine gene expression in the chicken spleen. Poult. Sci. 86:1351-1355. Anobile, J. M., V. Arumugaswami, D. Downs, K. Czymmek, M. Parcells, and C. J. Schmidt. 2006. Nuclear localization and dynamic properties of the Marek's disease virus oncogene products Meq and Meq/vIL8. J. Virol. 80:1160-1166. Asif, M., J. W. Lowenthal, M. E. Ford, K. A. Schat, W. G. Kimpton, and A. G. D. Bean. 2007. IL-6 expression following IBV infection in chickens. Viral Immunol. 20:479-486. Baelmans, R., H. K. Parmentier, H. M. J. Udo, F. Demey, and D. Berkvens. 2004. Different serum haemolytic complement levels in indigenous chickens from Benin, Bolivia, Cameroon, India and Tanzania. Trop. Anim. Health Prod. 36:731-742. Baelmans, R., H. K. Parmentier, M. G. B. Nieuwland, P. Dorny, and F. Demey. 2005. Serological screening for MHC (B)-polymorphism in indigenous chickens. Trop. Anim. Health Prod. 37:93-102. Baelmans, R., H. K. Parmentier, P. Dorny, H. F. J. Savelkoul, F. Demey, and D. Berkvens. 2006. Reciprocal antibody and complement responses of two chicken breeds to vaccine strains of Newcastle disease virus, infectious bursal disease virus and infectious bronchitis virus. Vet. Res. Commun. 30:567-576. Biggs, P. M., 1982. The epidemiology of avian herpesviruses in veterinary medicine. Dev. Biol. Stand. 52:3-11. Blackmore, C. S., K. C. Klasing, and P. S. Wakenell. 2006. Effect of infectious bursal disease virus insult on iron, copper and zinc metabolism in liver, bursa of Fabricius, spleen, pancreas and serum of chickens. Avian Dis. 50:303-305. Bohls, R. L., E. W. Collisson, N. J. Silvy, and D. Phalen. 2006. Pathogenesis of reticuloendo-theliosis virus in the endangered Attwaters prairie chicken. Avian Dis. 50:613-9. Bohls, R. L., J. A. Linares, S. L. Gross, P. J. Ferro, N. J. Silvy and E. W. Collisson, EW. 2006. Phylogenetic analyses indicate little variation among reticuloendotheliosis viruses infecting avian species, including the endangered Attwater's prairie chicken. Virus Res. 119:187-194. Bohls, R., P. J. Ferro, R. Smith, Z. Li, N. Silvy, and E. W. Collisson. 2006. The use of flow cytometry to discriminate avian lymphocytes from contaminating thrombocyte. Dev. Comp. Immunol. 30:843-850. Bowen, O. T., G. F. Erf, M. E. Chapman, and R. F. Wideman, Jr. 2007. Plasma nitric oxide concentration in broilers after intravenous injections of lipopolysaccharide or microparticles. Poult. Sci. 86:2550-2554. Bowen, O. T., G. F. Erf, N. B. Anthony, and R. F. Wideman. 2006. Pulmonary hypertension triggered by lipopolysaccharide in ascites-susceptible and -resistant broilers is not amplified by aminoguanidine, a specific inhibitor of inducible nitric oxide synthase. Poult. Sci. 85:528-536. Bowen, O. T., R. F. Wideman, N. B. Anthony, and G. F. Erf. 2006. Variation in the pulmonary hypertensive responsiveness of broilers to lipopolysaccharide and innate variation in nitric oxide production by mononuclear cells. Poult. Sci. 85:1349-1363. Brentano, L., S. Lazzarin, T. A. P. Klein, and K. A. Schat. 2005. Detection of chicken anemia virus in the gonads and in the progeny of broiler breeder hens with high neutralizing antibody titer. Vet. Microbiol. 105:65-72. Briles, W. E. 2004. Non-Major histocompatibility complex alloantigen genes affecting immunity. Poult. Sci. 83:606-610. Brooks, J. E., A. C. Rainer, R. L. Parr, P. Woolcock, F. Hoerr, and E. W. Collisson. 2004 Comparisons of the membrane gene of various strains of avian infectious bronchitis virus show evidence of recombination. Virus Res. 100:191-198. Brown, D. C., C. V. Maxwell, G. F. Erf, M. E. Davis, S. Singh, and Z. B. Johnson. 2006. The influence of different management systems and age on intestinal morphology, immune cell numbers and mucin production from goblet cells in post-weaning pigs. Vet. Immunol. Immunopathol. 111:187-98. Buitenhuis, A. J., T. B. Rodenburg, M. Siwek, S. J. Cornelissen, M. G. Nieuwland, R. P. Crooijmans, M. A. Groenen, P. Koene, H. Bovenhuis, and J. J. van der Poel. 2003. Identification of quantitative trait loci for receiving pecks in young and adult laying hens. Poult. Sci. 82:1661-1667. Erratum in: Poult. Sci. 2006: 85:1117. Buitenhuis, A. J., T. B. Rodenburg, M. Siwek, S. J. Cornelissen, M. G. Nieuwland, R. P. Crooijmans, M. A. Groenen, P. Koene, H. Bovenhuis, and J. J. van der Poel. 2004. Identification of QTLs involved in open-field behavior in young and adult laying hens. Behav. Genet. 34: 325-333. Erratum in: Behav. Genet. 2007; 37:257-258. Buitenhuis, A. J., T. B. Rodenburg, P. H. Wissink, J. Visscher, P. Koene, H. Bovenhuis, B. J. Ducro and J. J. van der Poel. 2004. Genetic and phenotypic correlations between feather pecking behavior, stress response, immune response, and egg quality traits in laying hens. Poult. Sci. 83:1077-1082. Bull, M. E., T. W. Vahlenkamp, J. L. Dow, E. W. Collisson, B. J. Winslow, A. P. Phadke, M. B. Tompkins, and W. A. Tompkins. 2004. Spontaneous T cell apoptosis in feline immuno-deficiency virus (FIV)-infected cats is inhibited by IL2 and anti-B7.1 antibodies. Vet. Immun. Immunopath. 99:25-37. Burgess, S. C., J. R. Young, B. J. Baaten, L. Hunt, L. N. Ross, M. S. Parcells, P. M. Kumar, C. A. Tregaskes, L. F. Lee, and T. F. Davison. 2004. Marek's disease is a natural model for lymphomas overexpressing Hodgkin's disease antigen (CD30). Proc. Natl. Acad. Sci. USA 101:13879-13884. Chang, H., and M. E. Delany. 2004. Karyotype stability of the DT40 cell B cell line: Macrochromosome variation and cytogenetic mosaicism. Chromosome Res. 12:299-307. Chang, H., and M. E. Delany. 2006. Complicated RNA splicing of chicken telomerase reverse transcriptase revealed by profiling cells both positive and negative for telomerase activity. Gene 379:33-39. Chapman, M. E., R. L. Taylor, Jr., and R. F. Wideman, Jr., 2008. Analysis of plasma serotonin levels and hemodynamic responses following chronic serotonin infusion in broilers challenged with bacterial lipopolysaccharide and microparticles. Poult. Sci. 87:116-124 Cheeseman, J. H., M. G. Kaiser, and S. J. Lamont. 2004. Genetic line effect on peripheral blood leukocyte cell surface marker expression in chickens. Poult. Sci. 83:911-916. Cheeseman, J. H., M. G. Kaiser, C. Ciraci, P. Kaiser, and S. J. Lamont. 2007. Breed effect on early cytokine mRNA expression in spleen and cecum of chickens with and without Salmonella enteritidis infection. Dev. Comp. Immunol. 31:52-60. Collisson, E. W. and R. L. Bohls. 2006. Development of regents for the study of reticuloendotheliosis virus in the endangered Attwaters praire chicken. 55th Western Poul. Dis., Ann. Mtg. March, Sacramento, CA. Collisson, E. W., S. Singh and T. Omran. 2007. Developments in avian influenza virus vaccines. J. Poul. Sci. 44:238-257. Cotter, P. F. J. Ayoub, and H. K. Parmentier. 2005. Directional selection for specific sheep cell antibody responses affects natural rabbit agglutinins of chickens. Poultry Sci. 84:220-225. Cumberbatch, J. A., D. Brewer, I. Vidavsky, and S. Sharif. 2006. Chicken major histocompatibility complex class II molecules of B19 haplotype present self and foreign peptides. Anim. Genet. 37:393-396. Daniels, L. M., and M. E. Delany. 2003. Molecular and cytogenetic organization of the 5S ribosomal DNA in chicken (Gallus gallus). Chromosome Res. 11:305-317. Davis, M. E., C. V. Maxwell, G. F. Erf, D. C. Brown, and T. J. Wistuba. 2004. Dietary supplementation with phosphorylated mannans improves growth responses and modulates immune function of weanling pigs. J. Anim. Sci. 82-1882-1891. Deeb, N., and S. J. Lamont. 2003. Use of a novel outbred by inbred F1 cross to detect genetic markers for growth. Anim. Genet. 34:205-212. Delany, M. E. 2004. Genetic variants for chick biology research: From breeds to mutants. Mech. Dev. 121:1169-1177. Delany, M. E. 2004. Importance of biodiversity preservation for research and industry. Zootec. Int. 4:38-43. Delany, M. E. 2006. Avian genetic stocks: the high and low points from an academia researcher. Poult. Sci. 85:223-226. Delany, M. E., and L. M. Daniels. 2003. The chicken telomerase RNA gene: Conservation of sequence, regulatory elements and synteny among viral, avian and mammalian genomes. Cytogenet. Genome Res. 102:309-317. Delany, M. E., and L. M. Daniels. 2004. The chicken telomerase reverse transcriptase (chTERT): Molecular and cytogenetic characterization with a comparative analysis. Gene 339:61-69. Delany, M. E., L. M. Daniels, S. E. Swanberg, and H. A. Taylor. 2003. Telomeres in the chicken: Chromosome ends and genome stability. Poult. Sci. 82:917-926. Delany, M. E., L. M. Daniels, T. M. Gessaro, and K. L. Rodrigue. 2007. Chromosomal mapping of chicken mega-telomere arrays to GGA9, 16, 28 and W using a cytogenomic approach. Cytogenet. Genome Res. 117:54-63. Dorny, P., R. Baelmans, H. K. Parmentier, M. G. B. Nieuwland, F. Demey, and D. Berkvens. Serum haemolytic complement levels in German Dahlem Red chickens are affected by three major genes (naked neck, dwarf, frizzled) of tropical interest. Trop. Anim. Health Prod. 37:1-9. Erf, G. F. 2004. Avian cell-mediated immunity. Poult. Sci. 83:580-590. Ewald, S. J., and E. J. Livant. 2004. Distinctive polymorphism of chicken B-FI (MHC class I) molecules. Poult. Sci. 83:600-645. Ewald, S. J., X. Ye, S. Avendano, S. McLeod, S. J. Lamont, and J. C. Dekkers. 2007. Associations of BF2 alleles with antibody titres and production traits in commercial pure line broiler chickens. Anim Genet. 38:174-176. Ferdous, F., D. V. Maurice, and T. R. Scott. 2008. Broiler chick thrombocyte response to lipopolysaccharide. Poult. Sci. 87:61-63 Fulton, J. E., and M. E. Delany. 2003. Diminishing poultry genetic research resources: Rescue needed. Science 300:1667-1668. Fulton, J. E., H. Juul-Madsen, C. M. Ashwell, A. M. McCarron, J. A. Arthur, N. OSullivan and R. L. Taylor, Jr. 2006. Molecular genotype identification of the Gallus gallus major histocompatibility complex. Immunogenetics 58:407-421. Goodenbour, J. M., M. G. Kaiser, and S. J. Lamont. 2004. Linkage mapping of inhibitor of apoptosis protein-1 (IAP 1) to chicken chromosome 1. Anim. Genet. 35:158-159. Guo, F. C., R. P. Kwakkel, B. A. Williams, H. K. Parmentier, W. K. Li, and M. A. W. Verstegen. 2004. Effects of mushroom and herb polysaccharides on cellular and humoral immune responses of Eimeria tenella-infected chickens. Poult. Sci. 83:1124-1132. Haeri, M., L. R. Read, B. N. Wilkie, and S. Sharif. 2005. Identification of peptides associated with chicken major histocompatibility complex class II molecules of B21 and B19 haplotypes. Immunogenetics 56:854-859. Haghighi H. R., J. Gong, C. L. Gyles, M. A. Hayes, H. Zhou, B. Sanei, T. Hayes, J. R. Chambers, and S. Sharif. 2006. Probiotics stimulate production of natural antibodies in chickens. Clin. Vaccine Immunol. 13:975-980. Haghighi, H. R., J. Gong, C. L. Gyles, M. A. Hayes, B. Sanei, P. Parvizi, H. Gisavi, J. R. Chambers, and S. Sharif. 2005. Modulation of antibody-mediated immune response by probiotics in chickens. Clin. Diagn. Lab. Immunol. 12:1387-1392. Hangalapura, B. N., M. G. B. Nieuwland, G. de Vries Reilingh, H. van den Brand, B. Kemp, and H. K. Parmentier. 2004. Durations of cold stress modulates overall immunity of chicken lines divergently selected for antibody responses. Poult. Sci. 83: 765-775. Hangalapura, B. N., M. G. B. Nieuwland, G. De Vries Reilingh, J. Buyse, H. van den Brand, B. Kemp, and H. K. Parmentier. 2005. Effects of moderate and severe feed restriction on immune responses in chicken lines selected for antibody responses. Poult. Sci. 84:1520-1529. Hangalapura, B. N., M. G. B. Nieuwland, G. de Vries Reilingh, M. J. W. Heetkamp, H. van den Brand, and H. K. Parmentier. 2003. Effect of cold stress on immune responses and body weight of chicken lines divergently selected for antibody responses to sheep red blood cells. Poult. Sci. 82:692-1700. Hasenstein, J. R., and S. J. Lamont. 2007. Chicken gallinacin gene cluster associated with Salmonella response in advanced intercross line. Avian Dis. 51:561-567. Hasenstein, J. R., G. Zhang, and S. J. Lamont. 2006. Analyses of Five gallinacin genes and the Salmonella enterica serovar Enteritidis response in poultry. Infect. Immun. 74:3375-3380. Herlong, J. L. and T. R. Scott. 2006. Positioning prostanoids of the D and J series in the immunopathogenic scheme. Immunol. Let. 102:121-131. Higgins, S. E., G. F. Erf, J. P. Higgins, S. N. Henderson, A. D. Wolfenden, G. Gaona-Ramirez, and B.M. Hargis. 2007. Effect of probiotic treatment in broiler chicks on intestinal macrophage numbers and phagocytosis of Salmonella enteritidis by abdominal exudate cells. Poult. Sci. 86:2315-2321. Hunt, H. D., R. M. Goto, D. N. Foster, L. D. Bacon, and M. M. Miller. 2006. At least one YMHCI molecule in the chicken is alloimmunogenic and dynamically expressed on spleen cells during development. Immunogenetics 58:297-307. Iglesias, G. M., L. A. Soria, R. M. Goto, A. Jar, M. C. Miquel, O. J. Lopez, and M. M. Miller. 2003. Genotypic variability at the major histocompatibility complex (B and Rfp-Y) in Camperos broiler chickens. Animal Genetics 34:88-95. Jarosinski, K. W., and K. A. Schat. 2007. Multiple alternative splicing to exons II and III of viral interleukin 8 (vIL-8) in the Mareks disease virus genome: the importance of vIL-8 exon I. Virus Genes 34:9-21. Jarosinski, K. W., B. L. Njaa, P. H. OConnell, and K. A. Schat. 2005. Pro-inflammatory responses in chicken spleen and brain tissues after infection with very virulent Mareks disease virus. Viral Immunol. 18:148-161. Jarosinski, K. W., N. Osterrieder, V. K. Nair, and K. A. Schat. 2005. Attenuation of Mareks disease virus (MDV) by deletion of open reading frame RLORF4 but not RLORF5a. J. Virol. 79:11647-11659. Jarosinski, K. W., R. W. Yunis, P. H. O'Connell, C. J. Markowski-Grimsrud, and K. A. Schat. 2002. Influence of genetic resistance of the chicken and virulence of Marek's disease virus (MDV) on nitric oxide responses after MDV infection. Avian Dis. 46:636-649. Jayaram, J., S. Youn, and E. W. Collisson. 2005. The virion infectious bronchitis virus N protein is more phosphorylated than the N protein from infected cell lysates. Virology 339:127-135. Joiner, K. S., F. J. Hoerr, E. van Santen, and S. J. Ewald. 2005. The avian major histocom-patibility complex influences bacterial skeletal disease in broiler breeder chickens. Vet. Pathol. 42:275-281. Joiner, K. S., S. J. Ewald, F. J. Hoerr, V. L. van Santen, and H. Toro. 2005. Oral infection with chicken anemia virus in 4-wk broiler breeders: lack of effect of major histocompatibility B complex genotype. Avian Dis. 49:482-487. Kaiser, M. G., J. H. Cheeseman, P. Kaiser, and S. J. Lamont. 2006. Cytokine expression in chicken peripheral blood mononuclear cells after in vitro exposure to Salmonella enterica serovar Enteritidis. Poult. Sci. 85:1907-1911. Kaufman. J., S. Milne, T. W. Gobel, B. A. Walker, J. P. Jacob, C. Auffray, R. Zoorob, and S. Beck, 1999. The chicken B locus is a minimal essential major histocompatibility complex. Nature 401:923-925 Konjufca, V. K., T. K. Bersi, W. G. Bottje, and G. F. Erf. 2004. Influence of dietary vitamin E on phagocytic functions of macrophages in broilers. Poult. Sci. 83:1530-1534. Kramer, J., M. Malek, and S. J. Lamont. 2003. Association of twelve candidate gene polymorphisms and response to challenge with Salmonella enteritidis in poultry. Anim. Genet. 34:339-348. Kulkarni, R. R., V. R. Parreira, S. Sharif, and J. F Prescott. 2007. Immunization of broiler chickens against Clostridium perfringens-induced necrotic enteritis. Clin. Vaccine Immunol. 14:1070-1077 Lambourne, M., W. Si, P. K. Niemiec, L. R. Read, S. Kariyawasam, and S. Sharif. 2005. Identification of novel polymorphisms in the B-LB locus of Gallus lafayettei. Anim. Genet. 36:445-448. Lammers, A., H. F. J. Savelkoul, and H. K. Parmentier. 2003. Identification and immunomodulatory activity of chicken natural antibodies. Immunol. Letters 87:40. Lammers, A., M. E. V. Klomp, M. G. B. Nieuwland, H. F. J. Savelkoul, and H. K. Parmentier. 2004. Adoptive transfer of natural antibodies to non-immunized chickens affects subsequent antigen-specific humoral and cellular immune responses. Dev. Comp. Immunol. 28:51-60. Lamont, S. J. 2003. Unique population designs used to address molecular genetics questions in poultry. Poult. Sci. 82:882-884. Li, H., N. Deeb, H. Zhou, A. D. Mitchell, C. M. Ashwell, and S. J. Lamont. 2003. Chicken quantitative trait loci for growth and body composition associated with transforming growth factor-beta genes. Poult. Sci. 82:347-356. Li, H., N. Deeb, H. Zhou, C. M. Ashwell, and S. J. Lamont. 2005. Chicken quantitative trait loci for growth and body composition associated with the very low density apolipoprotein-II gene. Poult. Sci. 84:697-703. Li, H., N. Neeb, H. Zhou, A. D. Mitchell, C. M. Ashwell, and S. J. Lamont. 2003. Chicken quantitative trait loci for growth and body composition associated with transforming growth factor-beta family genes. Poult. Sci. 82:347-356. Li, X., K. W. Jarosinski, and K. A. Schat. 2006. Expression of Mareks disease virus phosphorylated polypeptide pp38 produces splice variants and enhances metabolic activity. Vet. Microbiol. 117:154-168. Liu, W., M. G. Kaiser, and S. J. Lamont. 2003. Natural resistance-associated macrophage protein 1 gene polymorphisms and response to vaccine against or challenge with Salmonella enteritidis in young chicks. Poult. Sci. 82:259-266. Livant, E. J., and S. J. Ewald. 2005. High-resolution typing for chicken BF2 (MHC class I) alleles by automated sequencing. Anim. Genet. 36:432-434. Livant, E. J., J. R. Brigati, and S. J. Ewald. 2004. Diversity and locus specificity of chicken MHC class I sequences. Anim. Genet. 35:18-27. Livant, E. J., S. Avendano, S. McLeod, X. Ye, S. J. Lamont, J. C. Dekkers, and S. J. Ewald. 2007. MX1 exon 13 polymorphisms in broiler breeder chickens and associations with commercial traits. Anim Genet. 38:177-179. Maldonado, L. M. E., A. Lammers, M. G. B. Nieuwland, G. De Vries Reilingh, and H. K. Parmentier. 2005. Homotopes affect primary and secondary antibody responses in poultry. Vaccine 23:2731-2739. Malek, M., and S. J. Lamont. 2003. Association of INOS, TRAIL, TGF-beta2, TGF-beta3, and IgL genes with response to Salmonella enteritidis in poultry. Genet. Sel. Evol. 35 Suppl 1:S99-111. Malek, M., J. R. Hasenstein, and S. J. Lamont. 2004. Analysis of chicken TLR4, CD28, MIF, MD-2, and LITAF genes in a Salmonella enteritidis resource population. Poult. Sci. 83:544-549. Mao, A., V. Paharkova-Vatchkova, J. Hardy, M. M. Miller, and S. Kovats. 2005. Estrogen selectively promotes the differentiation of dendritic cells with characteristics of Langerhans cells. J. Immunol. 175:5146-5151. Markowski-Grimsrud, C. J., and K. A. Schat. 2002. Cytotoxic T lymphocyte responses to Marek's disease herpesvirus-encoded glycoproteins. Vet. Immunol. Immunopathol. 90:133-44. Markowski-Grimsrud, C. J., and K. A. Schat. 2003. Infection with chicken anemia virus impairs the generation of pathogen-specific cytotoxic T lymphocytes. Immunology 109:283-294. Markowski-Grimsrud, C. J., M. M. Miller, and K. A. Schat. 2002. Development of strain-specific real-time PCR and RT-PCR assays for quantitation of chicken anemia virus. J. Virol. Meth. 101:135-147. Martin, M. P., P. S. Wakenell, P. Woolcock, and B. O'Connor. 2007. Evaluation and effectiveness of two infectious bronchitis virus vaccine programs for preventing disease caused by a California IBV field isolate. Avian Dis. 51:584-589. McElroy, J. P., J. C. Dekkers, J. E. Fulton, N. P. O'Sullivan, M. Soller, E. Lipkin, W. Zhang, K. J. Koehler, S. J. Lamont, and H. H. Cheng. 2005. Microsatellite markers associated with resistance to Marek's disease in commercial layer chickens. Poult. Sci. 84:1678-1688. McElroy, J. P., W. Zhang, K. J. Koehler, S. J. Lamont, and J. C. Dekkers. 2006. Comparison of methods for analysis of selective genotyping survival data. Genet. Sel. Evol. 38:637-655. Medarova, Z., W. E. Briles and R. L. Taylor, Jr. 2003. Alloantigen system L affects antibody responses. Int. J. Poult. Sci. 2:23-27. Medarova, Z., W. E. Briles and R. L. Taylor, Jr. 2003. Resistance, susceptibility, and immunity to cecal coccidiosis: B complex and alloantigen system L effects. Poult. Sci. 82:1113-1117. Miller, M. M. 2006. Why do we need to conserve what we have? A post-genome sequencing perspective on existing chicken strains. Poult. Sci. 85:243-245 Miller, M. M., C. Wang, E. Parisini, R. D. Coletta, R. M. Goto, S. Y. Lee, D. C. Barral, M. Townes, C. Roura-Mir, H. L. Ford, M. B. Brenner, and C. C. Dascher. 2005. Characterization of two avian MHC-like genes reveals an ancient origin of the CD1 family. Proc. Natl. Acad. Sci. USA 102:8674-8679. Miller, M. M., K. A. Ealey, W. B. Oswald, and K. A. Schat. 2003. Detection of chicken anemia virus DNA in embryonal tissues and eggshell membranes. Avian Dis. 47:662-671. Miller, M. M., K. W. Jarosinski, and K. A. Schat. 2005. Positive and negative regulation of chicken anemia virus transcription. J. Virol. 79:2859-2868. Miller, M. M., L. D. Bacon, K. Hala, H. D. Hunt, S. J. Ewald, J. Kaufman, R. Zoorob, and W. E. Briles. 2004. 2004 nomenclature for the chicken major histocompatibility (B and Y) complex. Immunogenetics 56:261-279. Minozzi, G., H. K. Parmentier, M. G. B. Nieuwland, B. Bedhom, F. Minvielle, D. Gourichon, and M-H. Pinard-van der Laan. 2007. Antibody responses to keyhole limpet hemocyanin, lipopolysaccharide and Newcastle disease virus vaccine in F2 and backcrosses of White Leghorn lines selected for two different immune response traits. Poult. Sci. 86:1316-1322. Minozzi, G., H. K. Parmentier, S. Mignon-Grasteau, M. G .B. Nieuwland, B. Bedhom, F. Minvielle, M-H. Pinard-van der Laan. Correlated effects of selection for immunity in White Leghorn chicken lines on natural antibodies and specific antibody responses to KLH and M. butyricum. BioMed. Central Genetics. In press Morrow, C., and F. Fehler. 2004. Marek's disease: a worldwide problem, p. 49-61. In F. Davison and V. Nair (ed.), Marek's disease. An evolving problem. Elsevier Academic Press, London. Niemiec, P. K., L. R. Read, and S. Sharif. 2006. Synthesis of chicken major histocompatibility complex class II oligomers using a baculovirus expression system. Protein Expr. Purif. 46:390-400. O'Hare, T. H., and M. E. Delany. 2005. Telomerase gene expression in the chicken: telomerase RNA (TR) and reverse transcriptase (TERT) transcript profiles are tissue-specific and correlated with telomerase activity. AGE 27:257-266. Omar, A. R., and K. A. Schat. 1996. Syngeneic Marek's disease virus (MDV)-specific cell-mediated immune responses against immediate early, late, and unique MDV proteins. Virology 222:87-99. Parmentier, H. K., A. Lammers, J. J. Hoekman, G. de Vries Reilingh, I. Zanen, and H. F. J. Savelkoul. 2004. Different levels of natural antibodies in chickens divergently selected for specific antibody responses. Dev. Comp. Immunol. 28:39-49. Parmentier, H. K., L. Star, S. C. Sodoyer, M. G. B. Nieuwland, G. de Vries Reilingh, A. Lammers, and B. Kemp. 2006. Age- and breed-dependent adapted immune responsiveness of poultry to intra-tracheal administered pathogen-associated molecular patterns. Poult. Sci. 85:2156-2168. Parmentier, H. K., R. Baelmans, H. F. J. Savelkoul, P. Dorny, F. Demey, and D. Berkvens. 2004. Serum haemolytic complement activities in 11 different MHC (B) typed chicken lines. Vet. Imm. Immunopath. 100:25-32. Parmentier, H. K., W. J. A. Van den Kieboom, M. G. B. Nieuwland, G. De Vries Reilingh, B. N. Hangalapura, H. F. J. Savelkoul, and A. Lammers. 2004. Differential effects of lipopolysaccharide and lipoteichoic acid on the primary antibody response to keyhole limpet Hemocyanin of chickens selected for high and low antibody responses to sheep red blood cells. Poult. Sci. 83:1133-1139. Pei, J., W. E. Briles, and E. W. Collisson. 2003. Memory T cells protect chicks from acute infectious bronchitis virus infection. Virology 206:376-384. Ploegaert, T. C. W., G. de Vries Reilingh, M. G. B. Nieuwland, A. Lammers, H. F. J. Savelkoul, and H. K. Parmentier. 2007. Intratracheally administered pathogen associated molecular patterns affect antibody responses of poultry. Poult. Sci. 86:1667-1676. Prigge, J. T., V. Majerciak, H. D. Hunt, R. L. Dienglewicz, and M. S. Parcells. 2004. Construction and characterization of Marek's disease viruses having green fluorescent protein expression tied directly or indirectly to phosphoprotein 38 expression. Avian Dis. 48:471-487. Rath, N. C., M. S. Parcells, H. Xie, and E. Santin. 2003. Characterization of a spontaneously transformed chicken mononuclear cell line. Vet. Immunol. Immunopathol. 96:93-104. Read, L. R., J. A. Cumberbatch, M. M. Buhr, A. J. Bendall, and S. Sharif. 2005. Cloning and characterization of chicken stromal cell derived factor-1. Dev. Comp. Immunol. 29:143-152. Rispens, B. H., H. van Vloten, N. Mastenbroek, J. L. Maas, and K. A. Schat. 1972. Control of Marek's disease in the Netherlands. II. Field trials on vaccination with an avirulent strain (CVI 988) of Marek's disease virus. Avian Dis 16:126-38. Rodenburg T. B., A. J. Buitenhuis, B. Ask, K. A. Uitdehaag, P. Koene, J. J. van der Poel, J. A. van Arendonk and H. Bovenhuis. 2004. Genetic and phenotypic correlations between feather pecking and open-field response in laying hens at two different ages. Behav. Genet. 34: 407-415. Rodrigue, K. L., B. P. May, T. R. Famula, and M. E. Delany. 2005. Meiotic instability of chicken ultra-long telomeres and mapping of a 2.8 megabase array to the W-sex chromosome. Chromosome Res. 13:581-591. Romanov, M. N., L. M. Daniels, J. B. Dodgson, and M. E. Delany. 2005. Integration of the cytogenetic and physical maps of chicken chromosome 17. Chromosome Res. 13:215-222. Sarson A. J., L. R. Read, H. R. Haghighi, M. D. Lambourne, J. T. Brisbin, H. Zhou, and S. Sharif. 2006. Construction of a chicken immune system-specific microarray: Profiling gene expression in B cells following lipopolysaccharide stimulation. Can. J. Vet. Res. 71:108-118. Sarson, A. J., L. R. Read, H. R. Haghighi, M. D. Lambourne, H. Zhou and S. Sharif. 2007. Construction of a cDNA microarray for profiling gene expression in the chicken immune system. Can. J. Vet. Res. 71:108-118. Sarson, A. J., M. F. Abdul Careem, H. Zhou and S. Sharif. 2006. Transcriptional analysis of host response to Mareks disease virus infection. Viral. Immunol. 19:747-758. Schat, K. A. 2004. Understanding Mareks disease immunity: a continuing challenge. Int. J. Poult. Sci. 3:89-95. Schat, K. A., and E. Baranowski. 2007. Animal vaccination and the evolution of viral pathogens. Rev. sci. tech. Off. int. Epiz. 26:237-338. Schulten, E. S., L. M. Yates and R. L. Taylor, Jr. 2007. Antibody response against sheep red blood cells in lines congenic for major histocompatibility (B) complex recombinant haplotypes. Int. J. Poult. Sci. 6:732-738. Scott, T. R. 2004. Our current understanding of humoral immunity of poultry. Poult. Sci. 83:574-579. Scott, T. R. and H. S. Lillehoj. 2006. Monoclonal antibodies against chicken interleukin-6. Vet. Immunol. Immunophatol. 114:173-177. Scott, T. R. and M. D. Owens, 2008. Thrombocytes respond to lipopolysaccharide through toll-like receptor-4, and MAP kinase and NF-KB Pathways leading to expression of interleukin-6 and cyclooxygenase-2 with production of prostaglandin E2. Mol. Immunol. 45:1001-1008. Scott, T. R., A. R. Messersmith, W. J. McCrary, J. L. Herlong, and S. C. Burgess. 2005. Hematopoietic prostaglandin D2 synthase in the chicken Harderian gland. Vet. Immunol. Immunpathol. 108:295-306. Shamblin, C. E., N. Greene, V. Arumugaswami, R. L. Dienglewicz, and M. S. Parcells. 2004. Comparative analysis of Marek's disease virus (MDV) glycoprotein-, lytic antigen pp38- and transformation antigen Meq-encoding genes: association of meq mutations with MDVs of high virulence. Vet. Microbiol. 102:147-167. Shiina, T., W. E. Briles, R. M. Goto, K. Hosomichi, K. Yanagiya, S. Shimizu, H. Inoko, and M. M. Miller. 2007. Extended gene map reveals tripartite motif, C-type lectin, and Ig superfamily type genes within a subregion of the chicken MHC-B affecting infectious disease. J. Immunol. 178:7162-7172. Si, W., J. Gong, Y. Han, H. Yu, J. Brennan, H. Zhou, and S. Chen. 2007. Quantification of cell proliferation and ±-toxin gene expression of Clostridium perfringens in the development of necrotic enteritis in broiler chickens. Appl. Environ. Microbiol. 73:7110-7113. Siwek, M., A. J. Buitenhuis, S. J. B. Cornelissen, M. G. B. Nieuwland, E. F. Knoll, H. Bovenhuis, R. P. M. A. Crooijmans, M. A. M. Groenen, H. K. Parmentier, and J. J. van der Poel. 2006. Detection of QTL for innate: non specific antibody levels binding LPS and LTA in two independent populations of laying hens. Dev. Comp. Immunol. 30:659-666. Siwek, M., S. J. B. Cornelissen, A. J. Buitenhuis, M. G. B. Nieuwland, H. Bovenhuis, R. P. M. A. Crooijmans, M. A. M. Groenen, H. K. Parmentier, and J. J. van der Poel. 2004. Quantitative trait loci for body weight in layers differ from quantitative trait loci specific for antibody responses to sheep red blood cells. Poult. Sci. 83:853-859. Erratum: Poult Sci. 2006. 85:1120 Siwek, M., S. J. B. Cornelissen, M. G. B. Nieuwland, A. J. Buitenhuis, H. Bovenhuis, R. P. M. A. Crooijmans, M. A. M. Groenen, G. de Vries-Reilingh, H. K. Parmentier, and J. J. van der Poel. 2003. Detection of QTL for immune response to sheep red blood cells in laying hens. Anim. Genet. 34:422-428. Soller, M., S. Weigend, M. N. Romanov, J. C. Dekkers, and S. J. Lamont. 2006. Strategies to assess structural variation in the chicken genome and its associations with biodiversity and biological performance. Poult. Sci. 85:2061-2078. Star, L., K. Frankena, B. Kemp, M. G. B. Nieuwland, and H. K. Parmentier. 2007. Innate immune competence and survival in twelve pure bred layer lines. Poult. Sci. 86:1090-1099. Star, L., M. G. B. Nieuwland, B. Kemp, and H. K. Parmentier. 2007. Effect of single or combined climatic and hygienic stress on natural and specific humoral immune competence in four layer lines. Poult. Sci. 86:1894-1903. Sun, J. Y., L. Gaidulis, M. M. Miller, R. M. Goto, R. Rodriguez, S. J. Forman, and D. Senitzer. 2004. Development of a multiplex PCR-SSP method for Killer-cell immunoglobulin-like receptor genotyping. Tissue Antigens 64:462-468. Swanberg, S. E., and M. E. Delany. 2003. Dynamics of telomere erosion in transformed and non-transformed avian cells in vitro. Cytogenet. Genome Res. 102:318-325. Swanberg, S. E., and M. E. Delany. 2005. Differential expression of genes associated with telomere length homeostasis and oncogenesis in an avian model. Mech. Aging Dev. 126:1060-1070. Swanberg, S. E., W. Payne, H. H. Hunt, J. B. Dodgson, and M. E. Delany. 2004. Telomerase activity and differential expression of telomerase genes and c-myc in chicken cells in vitro. Dev. Dynamics 231:14-21. Taylor, R. L., Jr. 2004. Major histocompatibility (B) complex control of responses against Rous sarcomas. Poult. Sci. 83:638-649. (Review) Thoraval, P., M. Afanassieff, D. Bouret, G. Luneau, E. Esnault, R. M. Goto, A. M. Chausse, R. Zoorob, D. Soubieux, M. M. Miller, and G. Dambrine. 2003. Role of nonclassical class I genes of the chicken major histocompatibility complex Rfp-Y locus in transplantation immunity. Immunogenetics 55:647-651. Trapp, S., M. S. Parcells, J. P. Kamil, D. Schumacher, B. K. Tischer, P. M. Kumar, V. K. Nair, and N. Osterrieder. 2006. A virus-encoded telomerase RNA promotes malignant T cell lymphomagenesis. J. Exp. Med. 203:1307-1317. Tupick, T. A., S. E. Bloom and R. L. Taylor, Jr. 2005. Major histocompatibility (B) complex gene dose effects on Rous sarcoma virus tumor growth. Int. J. Poult. Sci. 4:286-291. van de Lavoir M.-C., C. Mather-Love, P. A. Leighton, J. H. Diamond, B. S. Heyer, R. Roberts, L. Zhu, P. Winters-Digiacinto, A. Kerchner, T. Gessaro, S. E. Swanberg, M. E. Delany, and R. J. Etches. 2006. High-grade transgenic somatic chimeras from chicken embryonic stem cells. Mech. Dev. 123:31-41. van de Lavoir M.-C., J. H. Diamond, P. A. Leighton, C. Mather-Love, B. S. Heyer, R. Bradshaw, A. Kerchner, L.T. Hooi, T. Gessaro, S. E. Swanberg, M. E. Delany, and R. J. Etches. 2006. Germline transmission of genetically modified primordial germ cells. Nature 441: 766-769. van den Berg, B. H. J., T. Harris, F. M. McCarthy, S. J. Lamont, and S. C. Burgess. 2007. Non-electrophoretic differential detergent fractionation proteomics using frozen whole organs. Rapid Commun. Mass Spectrom 21(23):3905-3909 van den Brand, H., H. K. Parmentier, and B. Kemp. 2004. Effects of housing system (outdoor vs cages) and age of laying hens on egg characteristics. Brit. Poult. Sci. 45:787-792. van den Brand, H., H. K. Parmentier, and B. Kemp. 2004. Selection for antibody responses against sheep red blood cells and layer age affect egg quality. Brit. Poult. Sci. 45:745-752. van Loon, D. P. R., B. N. Hangalapura, G. de Vries Reilingh, M. G. B. Nieuwland, B. Kemp, and H. K. Parmentier. 2004. Effect of three different housing systems on immune responses and body wieght of chicken lines divergently selected for antibody responses to sheep red blood cells. Livestock Prod. Sci. 85:139-150. Wang X., and G. F. Erf. 2004. Apoptosis in feathers of Smyth line chickens with autoimmune vitiligo. J. Autoimmun. 22: 21-30. Wang, X. and G. F. Erf. 2003. Melanocyte-specific cell mediated immune response in vitiliginous Smyth line chickens. J. Autoimmun. 21:149-160. Wang, X., W. Carre, H. Zhou, S. J. Lamont, and L. A. Cogburn 2004. Duplicated Spot14 genes in the chicken: characterization and identification of polymorphisms associated with abdominal fat deposition. Gene 332: 79-88. Wick, G., L. Andersson, K. Hala, M. E. Gershwin, C. F. Selmi, G. F. Erf, S. J. Lamont, and R. Sgonc. 2006. Avian models with spontaneous autoimmune diseases. Adv. Immunol. 92:71-117. Wideman Jr., R. F., M. E. Chapman, C. M. Owens, M. K. Devabhaktuni, L. C. Cavitt, W. Wang and G. F. Erf. 2003. Broiler survivors of intravenous micro-particle injections: Evaluation of growth, livability, meat-quality and arterial blood gas values during a cycle heat challenge. Poult. Sci. 82:484-495. Wideman R. F., M. E. Chapman, K. R. Hamal, O. T. Bowen, G. A. Lorenzoni, G. F. Erf, and N. B. Anthony. 2007. An inadequate pulmonary vascular capacity and susceptibility to pulmonary arterial hypertension in broilers. Poult. Sci. 86:984-98. Wideman, R. F. Jr., G. F. Erf, and M. E. Chapman. 2005. NÉ-Nitro-L-arginine methyl ester (L-NAME) amplifies the pulmonary hypertensive response to micro-particle injections in broilers. Poult. Sci. 84:1077-1091. Wideman, R. F., O. T. Bowen, G. F. Erf, and M. E. Chapman. 2006. Influence of aminoguanidine, an inhibitor of inducible nitric oxide synthase, on the pulmonary hypertensive response to micro-particle injections in broilers. Poult. Sci. 85:511-27. Witter, R. L, and K. A. Schat. 2003. Marek's disease. Pages 407-465 in Diseases of Poultry, 11th edition. Y. M. Saif, H. J. Barnes, A. M. Fadly, J. R. Glisson, L. R. McDougald, and D. E. Swayne, eds. Iowa State Press, Ames. Witter, R. L., B. W. Calnek, C. Buscaglia, I. M. Gimeno, and K. A. Schat. 2005. Review. Classification of Marek's disease viruses according to pathotype - philosophy and methodology. Avian Pathol. 34:75-90. Ye, X., S. Avendano, J. C. Dekkers, and S. J. Lamont. 2006a. Association of twelve immune-related genes with performance of three broiler lines in two different hygiene environments. Poult. Sci. 85:1555-1569. Ye, X., S. McLeod, D. Elfick, J. C. Dekkers, and S. J. Lamont. 2006b. Rapid identification of single nucleotide polymorphisms and estimation of allele frequencies using sequence traces from DNA pools. Poult. Sci. 85:1165-1168. Ye, X., S. R. Brown, K. Nones, L. L. Coutinho, J. C. Dekkers, and S. J. Lamont. 2007. Associations of myostatin gene polymorphisms with performance and mortality traits in broiler chickens. Genet. Sel. Evol. 39:73-89. Youn, S., E. W. Collisson, and C. E. Machamer. 2006. Transcriptional regulation of RNA3 of infectious bronchitis virus. Adv. Exp. Med. Biol. 581:109-12. Youn, S., J. Leibowitz, and E. W. Collisson. 2005. In vitro assembled, recombinant infectious bronchitis viruses demonstrate that the 5a open reading frame is not essential for replication in Vero cells. Virology 332:206-215. Zhou, H. and S.J. Lamont. 2007. Gene expression profiling of Salmonella enteritidis challenge in two F8 advanced intercross chicken lines. Cytogenet. Genome Res. 117:131-138. Zhou, H., A. D. Mitchell, J. P. McMurtry, C. M. Ashwell, and S. J. Lamont. 2005. Insulin-like growth factor 1 gene polymorphism associations with growth, body composition, skeleton integrity, and metabolic traits in chickens. Poult. Sci. 84, 212-219. Zhou, H., and S. J. Lamont. 2003. Association of transforming growth factor beta genes with quantitative trait loci for antibody response kinetics in hens. Anim. Genet. 34:275-282. Zhou, H., and S. J. Lamont. 2003. Chicken MHC class I and class II gene effects on antibody response kinetics in chickens. Immunogenetics 55:133-140. Zhou, H., C. M. Evock-Clover, J. P. McMurtry, C. M. Ashwell, and S. J. Lamont. 2007. Genome-wide linkage analysis to identify chromosome regions affecting phenotypic traits in the chicken. IV. Metabolic traits. Poult. Sci. 86:255-266. Zhou, H., J. Gong, J. T. Brisbin, H. Yu, B. Sanei, P. Sabour, and S. Sharif. 2007. Appropriate chicken sample size for identifying the composition of broiler intestinal microbiota affected by dietary antibiotics using the PCR-DGGE technique. Poult. Sci. 86:2541-2549. Zhou, H., J. Gu, S. J. Lamont, and X. Gu. 2007. Evolutionary analysis for functional divergence of the toll-like receptor gene family and altered functional constraints. J. Mol. Evol. 65:119-123. Zhou, H., N. Deeb, C. M. Evock-Clover, A. D. Mitchell, C.M. C. M. Ashwell, and S. J. Lamont. 2007. Genome-wide linkage analysis to identify chromosome regions affecting phenotypic traits in the chicken. III. Skeletal Integrity. Poult. Sci. 86:267-276. Zhou, H., N. Deeb, C.M. Ashwell, and S. J. Lamont. 2006. Genome-wide linkage analysis to identify chromosome regions affecting phenotypic traits in the chicken. I. Growth and Average daily gain. Poult. Sci. 85:1700-1711. Zhou, H., N. Deeb, C.M. C. M. Ashwell, and S. J. Lamont. 2006. Genome-wide linkage analysis to identify chromosome regions affecting phenotypic traits in the chicken. II. Body composition. Poult. Sci. 85:1712-1721.