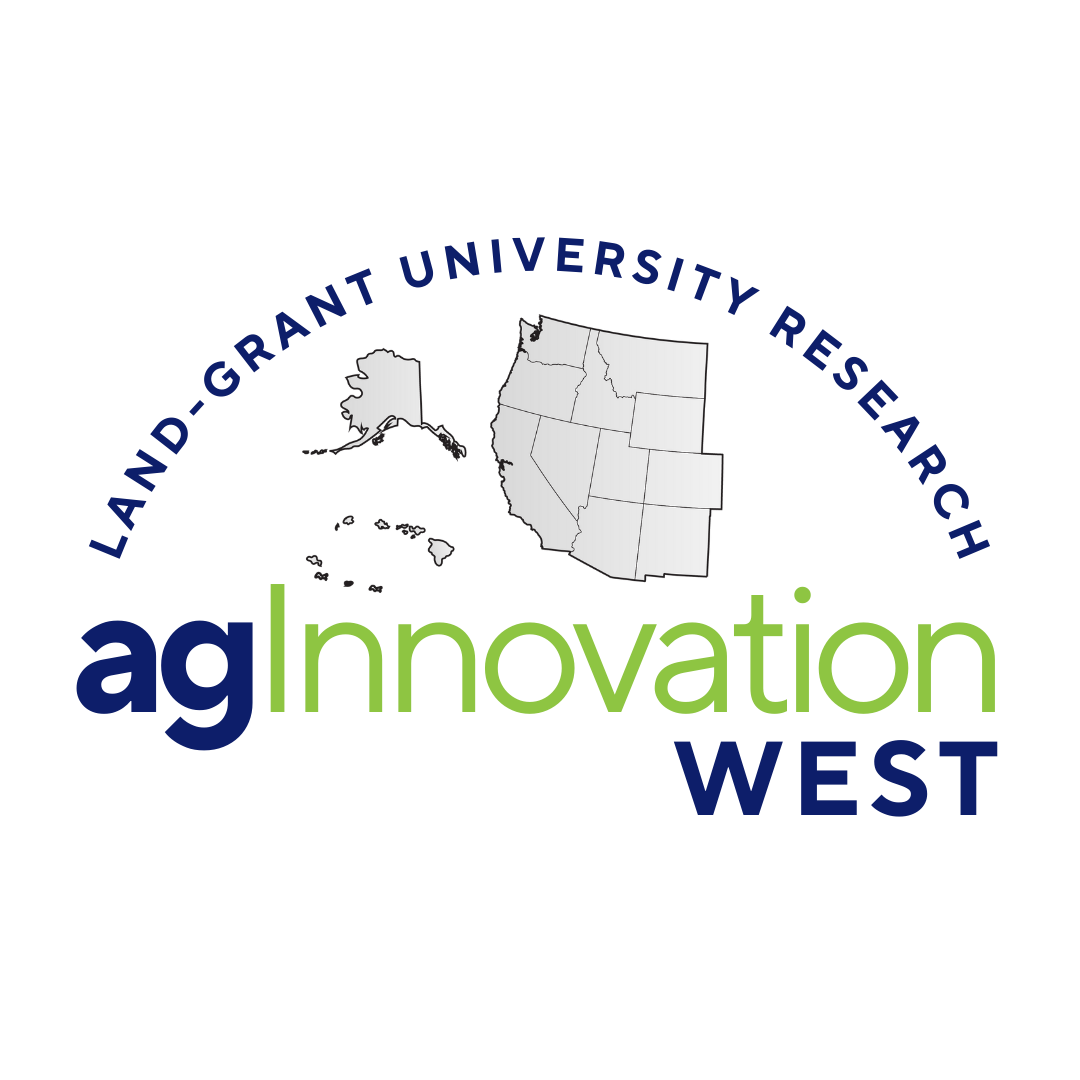
WERA20: Virus and Virus-Like Diseases of Berries, Fruit and Nut Trees, and Grapevines.
(Multistate Research Coordinating Committee and Information Exchange Group)
Status: Inactive/Terminating
Date of Annual Report: 09/18/2017
Report Information
Period the Report Covers: 10/01/2016 - 09/30/2017
Participants
Gratz, Allison (allison.gratz@inspection.gc.ca) - Canadian Food Inspection Agency (CFIA), Government of Canada;Li, Ruhui (Ruhui.Li@ars.usda.gov) - USDA-ARS, Beltsville, MD; Martin, Bob (Bob.Martin@ars.usda.gov) - USDA-ARS, Corvallis, OR;
Poudyal, Dipak (dpoudyal@oda.state.or.us) - Plant Health Program, Oregon Department of Agriculture, Salem, OR; Postman, Joseph (joseph.postman@ars.usda.gov) - National Clonal Germplasm Repository, USDA-ARS, Corvallis, OR; Tzanetakis, Ioannis (itzaneta@uark.edu) - University of Arkansas, Fayetteville, AR;
Hu, John (johnhu@hawaii.edu) - University of Hawaii, Honolulu, HI; Zhang, Shulu (shulu@agdia.com) - Agdia Inc. Elkhart, IN; Rabindran, Shailaja (shailaja.rabindran@aphis.usda.gov) - USDA-APHIS-PPQ-PM-PHP-IRM, Riverdale, MD;
Balci, Yilmaz (yilmaz.balci@aphis.usda.gov) - USDA-APHIS-PPQ-PHP-IRM, Riverdale, MD;
Spaine, Pauline (pauline.c.spaine@aphis.usda.gov) - USDA-APHIS-PPQ-PHP-IRM, Riverdale, MD;
Al Rwahnih, Maher (malrwahnih@ucdavis.edu) - Foundation Plant Services (FPS), University of California, Davis, CA;
Mysore, Sudarshana (mysore.sudarshana@ars.usda.gov) - USDA-ARS, University of California, Davis, CA;
Nikolaeva, Ekaterina (enikolaeva@pa.gov) PA Department of Agriculture, Harrisburg, PA;
Rosenbaum, Robin R (rosenbaumr@michigan.gov) - Michigan Department of Agriculture & Rural Development (MDARD), Lansing, MI;
Rudyj, Erich (Erich.S.Rudyj@aphis.usda.gov) - USDA-APHIS, Raleigh, NC; Prokrym, David (David.R.Prokrym@aphis.usda.gov) - USDA-APHIS, Raleigh, NC;
Kinard, Gary (Gary.Kinard@ars.usda.gov) - USDA-ARS, Maryland, MD;
Mollov, Dimitre (dimitre.mollov@ars.usda.gov) – USDA-ARS, Beltsville, MD;
Nakhla, Mark (mark.k.nakhla@aphis.usda.gov) - CPHST, USDA-APHIS-PPQ, Beltsville, MD;
Mavrodieva, Vessela (vessela.a.mavrodieva@aphis.usda.gov) - CPHST, USDA-APHIS-PPQ, Beltsville, MD;
Hollingsworth, Charla (charla.r.hollingsworth@aphis.usda.gov) - USDA-APHIS-PPQ, Raleigh, NC;
Devorshak, Christina (christina.devorshak@aphis.usda.gov) - USDA-APHIS-PPQ, Raleigh, NC;
Dennis, Geoffrey G (geoffrey.g.dennis@aphis.usda.gov) - USDA-APHIS-PPQ-CPHST-NPPLAP, Raleigh, NC;
Nutter, Forrest (fwn@iastate.edu) - Iowa State University, Ames, IA;
Moyer, James (j.moyer@wsu.edu) Washington State University,
Pullman, WA;
Hoffmann, Mark (mhoffma3@ncsu.edu) - North Carolina State University, Raleigh, NC;
Sit, Tim (tlsit@ncsu.edu) - North Carolina State University, Raleigh, NC;
Sudarshana, Padma (padma.sudarshana@csplabs.com) - CSP Labs, Pleasant Grove, CA;
Almeyda, Christie (cvalmeyd@ncsu.edu) – Micropropagation and Repository Unit (MPRU), North Carolina State University, Raleigh, NC;
Talton, Win (lwtalton@ncsu.edu) – MPRU, North Carolina State University, Raleigh, NC;
McAfee, Jessica (jcmcafee@ncsu.edu) – MPRU, North Carolina State University, Raleigh, NC;
Lindley, Jessica (jrlindle@ncsu.edu) – MPRU, North Carolina State University, Raleigh, NC;
Raitz, Rhiannon (rgminor@ncsu.edu) – MPRU, North Carolina State University, Raleigh, NC;
Gatlin, Connie (cjgatlin@ncsu.edu) – MPRU, North Carolina State University, Raleigh, NC;
Eldridge, Jacqueline (jceldrid@ncsu.edu) – MPRU, North Carolina State University, Raleigh, NC;
Davis, Eric (eric_davis@ncsu.edu) - North Carolina State University, Raleigh, NC;
Lommel, Steve (slommel@ncsu.edu) - North Carolina State University, Raleigh, NC;
Cline, Bill (bcline@ncsu.edu) - North Carolina State University, Raleigh, NC;
Guillermo Chacon (jgchacon@ncsu.edu) - North Carolina State University, Raleigh, NC;
Berger, Philip (philip.h.berger@aphis.usda.gov) - USDA/APHIS – PPQ, CPHST, Raleigh, NC.
Brief Summary of Minutes
WERA – 20 Meeting Minutes
Virus and Virus-like Diseases of Fruit Trees, Small Fruit and Grapevines
North Carolina State University Club
Raleigh, NC
July 17-19, 2017
Monday, July 17
Field Trip
Twenty two people (visitors and locals) attended the field trip. The Horticultural Crops Research Station in Castle Hayne, NC was visited. Bill Cline hosted a tour of the station where muscadine grapes and blueberry research plots were observed. Discussion about muscadine grapes and blueberry viral diseases was led by Bill Cline and Bob Martin while observing some symptoms on plots. After lunch at Wrightsville Beach, the group headed to Rose Hill to tour and wine tasting at Duplin Winery. Muscadine grapes wine production was this winery’s signature. Schedule below was followed.
8:00 Bus leaves Raleigh
10:00 Arrive at Horticultural Crops Research Station in Castle Hayne – Host Bill Cline
Tour of the station: blueberry and muscadine grapes research plots
11:30 Leave the station for Lunch in Wrightsville Beach
12:00 Lunch at Oceanic Restaurant
2:00 Bus heads to Rose Hill
3:00 Tour of production facilities and wine tasting at Duplin Winery
5:00 Bus leaves for Raleigh
7:00 Bus returns to Ramada Hotel, Raleigh
Tuesday, July 18
8:30 Welcome
Forty people attended the first day of the WERA-20 meeting at the North Carolina State University Club.
James Moyer (Washington State University)
Jim opened the meeting with welcome and opening remarks.
Steve Lommel and Eric Davis (North Carolina State University)
Steve and Rick followed up with welcoming remarks from the College of Agriculture and Life Sciences (CALS), NCSU and the Department of Entomology and Plant Pathology (DEPP), NCSU.
Christie Almeyda (Micropropagation and Repository Unit - MPRU, NCSU)
Christie served as the chair of the meeting. She welcomed everyone to the meeting, discussed housekeeping topics and introduced the first speaker.
9:00 Scientific presentations / State and Federal Reports
Allison Gratz (Center for Plant Health, Canadian Food Inspection Agency – CFIA, Government of Canada)
‘CFIA Center for Plant Health - Sidney Laboratory Update 2016-17’
Allison provided an overview of the program, plant material and regulations. Detections by diagnostic unit in Malus spp., Pyrus spp. and Prunus spp. from 2013-2016 in samples collected from certified sources were presented. A test for Cherry Virus A (CVA) was validated and the Centre used heat therapy to eliminate the virus from G1 repository. Currently there is no CVA-infected selections remaining in the virus-certified collection. From Mike Rott’s lab, CFIA has learned that NGS is a valuable and effective tool. Allison talked about the approved project for 2017-2019: ‘Validation and implementation of next generation sequencing technology for routine testing in the Sidney Laboratory Diagnostic Unit’ and its main goals.
Ruhui Li (USDA-ARS, Beltsville, MD)
‘Discovery and Molecular Characterization of Luteoviruses Infecting Fruit Trees’
Ruhui provided an overview of the discovery and molecular characterization of Apple Luteovirus 1 (ALV-1), a new luteovirus detected by HTS and found in rapid/sudden apple decline disease. Samples were collected at different seasons to determine the best time for sampling. The virus titer was different among trees, varieties and seasons. Transmission studies were also carried out.
Bob Martin (USDA-ARS, Corvallis, OR)
‘A Quest for Raspberry Leaf Curl Disease’
Bob provided an update for his investigations on Raspberry Leaf Curl Virus, Grapevine Red Blotch Virus and Blueberry fruit drop-association. Raspberry leaf curl virus is the only virus that requires biological indexing for export of plants from the US. All data has been collected after NGS studies. There is no detection method yet in place for this virus. Studies on Grapevine red blotch field transmission were done to identify when the virus is actually being spread in the field to inform pest management options for controlling the vector(s) of the pathogen. Blueberry fruit drop-associated virus (BFDaV) is a caulimovirus previously described affecting ‘Bluecrop’ only. The virus was not detected in other blueberry varieties planted adjacent to blocks of ‘Bluecrop’ with BFDaV infection. The disease has been observed only in northern Washington and British Columbia. Growers are actively tagging and removing plants while BFDaV has been added for testing of G1 plants.
Joseph Postman (National Clonal Germplasm Repository USDA-ARS, Corvallis, OR)
‘Pathogens Impacting Corvallis USDA Germplasm Collections Management – 2017’
Joseph provided an overview of collections with issues including hazelnut, blueberries, red currants, pear, elderberry and black currants. Apple mosaic virus (ApMV) was eliminated from more than 25 infected hazelnut cultivars using heat therapy and shoot-tip grafting. However, the virus was detected in five infected field trees in 2015. Blueberry shock virus showed a higher incidence in 2016. Tomato ringspot virus (TomRSV) was confirmed by ELISA in red currants. Xyllela fastidiosa was causing significant problems in olive trees in Europe. It was first identified in pear in Oregon. Xyllela is widely distributed in a variety of hosts. Pierce’s disease of grape is caused by a different isolate. Joseph provided an overview of surveys conducted in 2016 and despite multiple positive ELISAs, the pathogen was not confirmed by PCR determining this situation as a ‘fake disease’ that led to resume normal operations in 2017. Black currant reversion virus has a very low risk of spreading.
Dipak Poudyal (Plant Health Program, Oregon Department of Agriculture, Salem, OR)
‘Virus Survey in Fruit and Berry Crops in Oregon’
Dipak presented an overview of fruit tree nurseries in certification programs (latent virus survey in pome fruit nurseries and ilarvirus survey in stone fruit nurseries), virus survey in orchards (plum pox virus survey in stone fruit orchards and grapevine red blotch virus in vineyards) and an update of certification programs (harmonization of grapevine certification programs in the Pacific Northwest - ID, OR and WA - and updating caneberry certification standards in OR).
12:00-1:30 Lunch
Ioannis Tzanetakis (University of Arkansas, Fayetteville, AR)
‘Arkansas Update’
Ioannis provided an overview of his investigations on viruses in Arkansas, including Peach rosette mosaic virus for which a PCR test has been provided using a UTR’3 region; Black currant ideovirus for which a detection test has been developed based on conserved regions of both RNAs; Blackberry leaf mottle virus associated virus and Black currant virus A.
John Hu (University of Hawaii, Honolulu, HI)
‘Virology Research in Hawaii’
John presented an overview of his investigations on Taro vein chlorosis virus (TaVCV) to better understand the diversity of the virus in the region, develop molecular and serological detection assays, identify the vector and develop effective management options for farmers. Low genetic diversity observed in Hawaiian isolates further support the fact that the virus was introduced recently. Improved assays for virus detection were developed, providing better tools for virus surveys, monitoring and surveillance, plant quarantine and germoplasm screening. John presented strong evidence to support that taro planthopper is a vector of the virus.
Shulu Zhang (Agdia Inc. Elkhart, IN)
‘Updates on Rapid Detection of Plant Pathogens by Isothermal AmplifyRP®’
Shulu presented an update on the rapid detection of pathogens using isothermal AmplifyRP. Agdia’s different formats for AmplifyRP can be used for different endpoint applications and for different pathogens. The kits are fast, simple, sensitive and specific.
Maher Al Rwahnih (Foundation Plant Services – FPS, University of California, Davis, CA)
‘Pistachio Metagenomics’
Maher presented an overview of his investigations of an off-type pistachio line showing stunted symptoms and bushy-top syndrome caused by Rhodococcus fascians. A high throughput sequencing (HTS) based discovery method was carried out to identify and characterize viral pathogens in pistachio cultivars showing stunting symptoms. The National Clonal Germoplasm Repository pistachio collection was evaluated to assess the disease status. Pistachio Ampelovirus A (PAVA) and Citrus bark cracking viroid (CBCVd-pistachio) were found in these studies as novel species within the Closteroviridae and Pospoviroidae, respectively. Both viral agents (PAVA and CBCVd-pistachio) are graft transmissible.
2018 Location
Christie discussed the location for next year’s meeting. Bob Martin proposed Oregon for the next meeting location and everyone seconded the motion. Dates for the meeting TBD.
Wednesday, July 19
NGS Session
Maher Al Rwahnih (Foundation Plant Services (FPS), University of California, Davis, CA)
Case Study 1: ‘Limiting Factors in the Detection of Plant Viral Pathogens Using HTS’
Maher presented an overview of the HTS current applications at FPS including study diseases of unknown etiology, metagenomics virome analysis, re-sequencing of known viruses, host-pathogen interaction studies and post-entry quarantine procedures. Technical challenges and establishment of biological significance were indicated as HTS limitations. Maher discussed about the importance of Koch’s postulates while using HTS-based data for virus discovery. Maher stated that we are all in need of standard protocols/minimal requirement for HTS use as well as to establish a framework for the evaluation of risks posed by new viruses discovered by HTS.
Sudarshana Mysore (USDA-ARS, University of California, Davis, CA)
Case Study 2: ‘Dealing with the discovery of new viruses by deep sequencing in perennial crops: a tale of two viruses’
Sudhi presented an overview of grapevine red blotch virus discovery, surveys and impact as well as discovery of a luteovirus in nectarine trees in post-entry quarantine. He emphasized the importance of time of releasing information to stakeholders.
Shailaja Rabindran, Yilmaz Balci and Pauline Spaine (USDA-APHIS, Riverdale, MD)
APHIS presents NGS policy challenges, gaps in scientific knowledge, permits, PRAs, data collection for pests/pathogens
Shaila presented an overview of the actual relationship status between scientists and regulators. HTS is not used as a routine diagnostic tool. There is no requirement to use HTS to export certification. Mark discussed the importance of infrastructure and bioinformatic facilities to develop import policy. Standardization of protocols as well as developing of better diagnostic for imported plants are crucial.
Discussion, Conclusions and Next Steps
Erich gave an overview of the NCPN program, history and crops the program is serving. He pointed out that advocating to increase funding is a work in progress. Main areas for the centers to focus on are laboratory quality controls, economically studies and outreach (growers/stakeholders).
Maher started the discussion about the 2015 WERA-20 meeting purpose: to bring together the scientific and regulatory community to engage in the start of a conversation around NGS and the use of this technology to find pathogens and/or certification purposes. The 2016 WERA-20 meeting served as a platform for the establishment of biological significance of HTS findings. In this year's meeting, the goal is to take action and move forward. Maher and Shaila discussed the harmonization project as a joint effort to standardize minimal steps. Phil stated that the purpose of this initiative is to have standardized protocols. Maher stated that it is imperative to accelerate the process of quarantine. Bob suggested that a proposal be submitted within five weeks (Deadline 08/18/2017) to have a working group for a proficiency test (developing SOPs) and validation. After the procedures are in place, the next step would be to offer a workshop to train people about the SOPs.
Maher brought up other challenges including: naming novel viruses, releasing of sequences, and publishing. He claimed that following the classical way of naming new viruses (using symptoms associated to the disease) might not be a good idea since there is an impact of the name of the new virus on stakeholders. Ioannis and Shaila pointed out that breeders move material all over and there are no restrictions. They discussed the importance of having permits. Regulators can only regulate viruses of which they are knowledgeable. If there is no disease associated with a material, there is no authority to regulate. Regulators have a system of checks and balances that need to be performed for a disease to be regulated. Joseph discussed the issue of formalizing positive controls collections to NCPN center
Accomplishments
<p><strong>CFIA, Center for Plant Health, Government of Canada (Allison Gratz and Anna-Mary Schmidt)</strong></p><br /> <p><strong>Quarantine and Diagnostic Activities</strong></p><br /> <p><strong>Samples from Foreign Certification Programs</strong></p><br /> <p>The Centre for Plant Health continues to test a sampling of accessions taken from grapevine and tree fruit shipments from Canadian approved foreign certification programs in the United States, France and Germany for grapevines, and the United States, France, Belgium, Germany, Netherlands and United Kingdom for tree fruit.</p><br /> <p>In 2016, 20 grapevine accessions were tested (60 vines of each accession) and the only detection was <em>Arabis mosaic virus</em> in a grafted variety from Germany. This virus is not regulated in Canada. Over the past five years, there has been a decrease of virus detections in grapevines coming from approved certified foreign sources. In 2016, 29 tree fruit accessions were tested from approved sources in the United States (Washington, Oregon, and Pennsylvania). No viruses were detected in Malus spp. samples, <em>Apple chlorotic leaf spot virus</em> and <em>Apple stem pitting virus </em>(ASPV) were detected in the single <em>Cydonia</em> sp. sample, ASPV and <em>Pear blister canker viroid</em> were found coinfected in one <em>Pyrus</em> sp. sample, <em>Prune dwarf virus</em> was detected in one <em>Prunus</em> sp. sample, <em>Little cherry virus-1</em> was detected in two samples, and <em>Cherry virus A</em> was detected in 75% of the <em>Prunus</em> samples. None of these viruses are regulated in Canada.</p><br /> <p> </p><br /> <p><strong>Samples from other sources</strong></p><br /> <p>Non-certified material is also accepted and tested, and must be routed directly to the Centre for Plant Health PEQ facility for a full range of testing before release. This material includes imports from non-approved foreign sources or domestic breeding programs. Fifteen new grapevine accessions, two <em>Pyrus</em> spp., 12 <em>Prunus </em>spp., and 21 <em>Malus</em> spp. were received and tested in 2016. The Centre for Plant Health does a limited amount of regulatory testing for virus and virus-like diseases of small fruit (berries). The testing requirements for imports are determined on a case-by-case basis depending on the origin of the material. Since Canada does not have a national small fruit certification program for exports, all testing for export is also done on a case-by-case basis depending on the requirements of the importing country. In 2016, three varieties each of <em>Fragaria</em> and <em>Rubus</em> imported from France were tested, as well as one <em>Fragaria</em> variety from the Netherlands. No viruses were detected. Sixteen varieties of <em>Rubus</em> destined to the USA were tested for <em>Rubus</em> stunt phytoplasma, and there were no detections of this pathogen.</p><br /> <p> </p><br /> <p><strong><em>Cherry virus A</em></strong><strong> (CVA) update</strong></p><br /> <p>Since 2003, when a test for the latent virus CVA was validated, the Centre for Plant Health has worked to eliminate it using heat therapy from our Generation One repository. Currently we can report that no CVA-infected selections remain in the virus-certified collection.</p><br /> <p> </p><br /> <p><strong>Tissue Culture </strong></p><br /> <p>The tissue culture facility at the CFIA Sidney Laboratory supplies virus-free root stock and indicator plants for field and greenhouse woody bioassay testing. Upon request, the facility may produce small numbers of Generation 1 tissue culture initiates, from the CFIA’s tree fruit and grapevine virus-tested repository, for domestic or international distribution. This facility also receives and maintains tissue culture plants from international sources for inclusion in the CFIA Sidney Laboratory’s virus testing program. The tissue culture facility plays an integral part in the virus elimination service of the Sidney Laboratory. Virus elimination, when requested, is conducted on grapevine varieties using meristem culture following in-vivo heat therapy of infected plants, and virus elimination of small fruit and tree fruit varieties occurs with meristem or micro-shoot culture following in-vivo heat therapy.</p><br /> <p> </p><br /> <p><strong>Next Generation Sequencing</strong></p><br /> <p>In early 2017, Anna-Mary Schmidt received funding for a two-year project to transfer NGS technology from research to the Sidney Laboratory Diagnostic Unit. The objectives of the project are to (1) transfer NGS technology from the Sidney Laboratory Research Unit to the Diagnostic Unit for routine testing of viruses and viroids in grapevine, tree fruit, small fruit and other horticultural crops, (2) validate the NGS method in the Diagnostic Unit, (3) develop a diagnostic workflow, and (4) implement NGS technology as an official test method under the Sidney Laboratory’s Quality Assurance System (ISO 17025: General Requirements for the Competence of Testing and Calibration Laboratories). Also, plans for the implementation of a domestic clean plant network for tree fruit and grapevines centred on next generation sequencing (NGS) methods are underway.</p><br /> <p> </p><br /> <p><strong> </strong></p><br /> <p><strong>New Researcher</strong></p><br /> <p>Harvinder Bennypaul joined the Centre for Plant Health as a new research scientist in August 2016. His research interests include development of diagnostic methodologies, molecular characterization and management of plant pathogens.</p><br /> <p> </p><br /> <p><strong>Pome/Prunus Fruit Tree Quarantine Programs, Plant Germplasm Quarantine Program, USDA, APHIS, PPQ, FO (Joseph Foster)</strong></p><br /> <p>In 2017 the Pome quarantine program had the following types of releases: final releases for 25 <em>Malus</em>; 22 <em>Pyrus</em>, 12 quinces, as well as provisional releases for 7 <em>Malus</em> and 1 <em>Pyrus</em>. The <em>Prunus</em> quarantine program had 32 final releases as well as 12 provisional releases. The <em>Prunus</em> seedling program had 229 final releases. The total number of fruit tree releases for 2017 is 340. Last January, Dr. Margarita Bateman accepted a promotion to join the Plants for Planting Policy group in Plant Health Programs at PPQ Headquarters in Riverdale, MD. She made many noteworthy contributions during the 10 years she worked on pome and stone fruit introductions as Team Leader for those crops.</p><br /> <p><strong>Accessions in testing</strong></p><br /> <p><strong>Prunus</strong></p><br /> <p>In 2016, Tom Kim, Horticulturist for <em>Prunus</em>, received and established 36 accessions itemized as follows: 3 <em>P. salicina</em> accessions from South Africa; 4 <em>P. armeniaca</em> from France; 9 <em>P. persica</em> accessions from Valencia, Spain; 10 cherry accessions, including 1 <em>P. campanulata</em>, 1 <em>P. cyclamina</em>, 1 <em>P. pendula</em>, and 1 <em>P. sargentii</em> from The United Kingdom; 6 <em>P. armeniaca</em> from France; 4 <em>P. domestica</em> from Germany. During 2016 we had more than 300 <em>Prunus</em> accessions in quarantine at Bldg 580.</p><br /> <p><strong><strong>Pomes</strong></strong></p><br /> <p>In 2016, Robert Jones, Pomes Crop Specialist, received and established a total of 41 accessions itemized as follows: 21 cider apple accessions and 4 cider pears from The United Kingdom, 1 <em>Malus trilobata</em> from Scotland, UK; 2 accessions (rootstocks) from Brazil, 3 accessions (rootstocks) from South Africa, and 10 apples from France. During 2016, we had more than 400 apples, pears and quinces in quarantine at Bldg 580.</p><br /> <p> </p><br /> <p><strong>Pathogen interceptions </strong></p><br /> <p>Every year we detect pathogens in imported pome and stone fruits. Testing is done using indicators, ELISA serology, and PCR/RT-PCR procedures. Shipments may contain more than 50% infected accessions. Regular detections include the common latent viruses in pomes, cherry virus A in cherries, little cherry virus 2 in flowering cherries, peach latent mosaic viroid in peaches, and ilar viruses (PNRSV & PDV) in all <em>Prunus</em>. Notable recent detections include pear blister canker viroid in pears, hop stunt viroid in 2 peaches, a plum and an apricot, little cherry virus 1 in a plum from the Republic of Georgia, plum bark necrosis stem pitting associated virus in 11 Chinese peaches, nectarine stem pitting associated virus in 4 peaches from France, a phytoplasma in a cherry from UK, and new luteoviruses in pome and stone fruits detected by Dr. Ruhui Li. Trees that test positive are sent to thermotherapy.</p><br /> <p><strong>Therapy</strong></p><br /> <p>Different heat treatment and tip grafting or tissue culture procedures are used to obtain pome and stone fruits free of detected pathogens. Moving all of the infected accessions through the therapy process efficiently has been the greatest challenge for these quarantine programs.</p><br /> <p>Richard Slocum, Tissue Culture Scientist, continues to establish accessions in tissue culture in order to put them through therapy. In the past, he has made excellent progress with apples, pears, peaches, plums and cherries to the extent that these fruit trees are routinely exposed to in vitro thermotherapy. His latest accomplishment has been to establish tissue cultures of almonds and keep them for several growing seasons in tissue culture. At this time he will continue working on the survival of the material post therapy, which has proven to be challenging.</p><br /> <p><strong>Collaborations</strong></p><br /> <p>Dr. Ruhui Li, Dr. Gary Kinard- USDA-ARS: New PCR procedures and NGS</p><br /> <p>Dr. Maher Al-Rwahnih and Dr. Deborah Golino, FPS, Davis, CA: NGS procedures</p><br /> <p>Dr. Scott Harper, NCPN-FT, Prosser, WA: Detection of new Prunus viruses & field testing of pomes</p><br /> <p>Allison Gratz and Dr. Mike Rott, Agriculture Canada: NGS protocols in use in Canada and field testing of pomes</p><br /> <p><strong> </strong></p><br /> <p><strong>New personnel at PGQP </strong></p><br /> <p>Martha Malapi-Wight, Ph. D. is the new Lead Plant Pathologist for the Poaceae Quarantine Programs. Dr. Malapi-Wight joined PGQP in December 2015. She will be working on the quarantine programs for her assigned crops, as well as assisting PGQP with the implementation of NGS for diagnostics for quarantine purposes.</p><br /> <p>Ronald D. French, Ph. D. is the new Lead Plant Pathologist for the potato, sweet potato, and cassava quarantine programs. Dr. French joined PGQP in June. He will manage the quarantine programs for these crops, with the goal of introducing NGS for diagnostics.</p><br /> <p> </p><br /> <p><strong>USDA-ARS, Horticultural Crops Research Unit, Corvallis, OR (Bob Martin)</strong></p><br /> <p>Our laboratory has been working on three projects this past year; 1. Blueberry fruit drop disease, 2. Grapevine red blotch virus and 3, Raspberry leaf curl disease. Some of these are in early stages of development, while others are much further along. A brief description of each follows.</p><br /> <ol><br /> <li><strong>Blueberry fruit drop disease:</strong> Blueberry fruit drop disease (BFDD) results in a nearly 100% fruit drop symptom in ‘Bluecrop’ blueberry, which persists year after year. The plants tend to be much more vigorous than adjacent plants, likely because they are putting very little energy into fruit production. The disease has been observed only in northern Washington and British Columbia to date, and in one cultivar of blueberry at the NCGR in Corvallis, Oregon that originated in Finland. Previously, we characterized a Caulimovirus, named Blueberry fruit drop associated virus (BFDaV), from infected plants that is almost perfectly correlated with the presence of symptoms (>95%). The virus was not detected in ‘Duke’, ‘Liberty’ or ‘Hardiblue’ blueberry plants adjacent to blocks of ‘Bluecrop’ with BFDaV infection. Growers are actively tagging and removing plants and I am optimistic that we can eradicate this virus from blueberry production in North America. We have added testing for BFDaV to testing of G1 plants.</li><br /> </ol><br /> <p> </p><br /> <ol start="2"><br /> <li><strong>Grapevine red blotch virus:</strong> The goal of this project is to determine when Grapevine red blotch virus (GRBV) is spreading in the vineyard. Knowing when the virus is spreading will provide important information on effective management of GRBaV and help focus the efforts to identify additional vectors. This information will also help target control measures to times of the season when the virus is being transmitted in the field. Three vineyards where GRBV has been spreading were used in 2016 and four vineyards are being used in 2017. One vineyard has an adjacent riparian zone, with most virus spread occurring near that edge of the vineyard nearest the riparian zone. In this case the trap plants are placed in a grassy area between the riparian zone and the vineyard. The second vineyard has an adjacent alfalfa field and since the one vector reported to transmit the virus is the Three Cornered Alfalfa Hopper, the plants were placed perpendicular to the alfalfa field, and within vineyard rows. This vineyard was removed after the 2016 season, and another nearby vineyard with GRBV was substituted for the 2017 field trials. The third vineyard has most spread adjacent to a recently disturbed wooded area. In 2017 a fourth vineyard was added to the study, adjacent to a grassy/wooded area, where GRBV movement has been observed. In each vineyard, every plant has a unique number and the location of each plant is being mapped so that where virus spread occurs in each vineyard can be determined. Fifteen plants are placed in each vineyard each month starting April 15 through Sept 15 in 2016; and starting May 2 in 2017 and continuing until October. After one month in the field the plants are returned to Corvallis, treated with a systemic insecticide and maintained in a screenhouse. All 300 plants were tested for GRBV in November 2016 and were negative for GRBV in PCR testing. After overwintering, a set of 90 plants that represented trap plants for the 2016 growing season were tested by PCR in May of 2017. Again, all plants were negative for GRBV. The entire set of 300 plants will be tested in Sept of 2017 and again in Sept. 2018. The plants from the 2017 trial will be tested in 2018 and 2019. This work aims to identify when the virus is actually being spread in the field to inform pest management options for controlling the vector(s) of the pathogen.</li><br /> </ol><br /> <p> </p><br /> <ol start="3"><br /> <li><strong>Raspberry leaf curl virus:</strong> Raspberry leaf curl virus (RLCV) is the only virus of Rubus species that requires a graft indexing result for plant export. This virus (disease) was reported to be widespread in the Great Lakes region in the twentieth century from 1920s to 1970s and aphid transmitted in a persistent manner. We collected wild raspberry from two locations in Wisconsin in 2014 and have identified a several novel in these materials, but this is from a very limited set of samples. We did more collections in Ontario and Quebec Canada, and Maine, Wisconsin and Minnesota in the U.S. in August of 2016. In July of 2017, collections were made in Washington, Montana, Idaho, Wyoming, British Columbia and Alberta. NGS analysis of several samples collected in 2016 revealed three novel viruses, two were cytorhabdoviruses and each was detected at a single location, the third is in the Luteoviridae and was more widespread. The Luteoviridae virus is aphid transmitted. In the collections we focused on wild raspberry since symptom expression in cultivars grown today is unknown. Part of the reason for doing these collections is that no one that we have contacted has an isolate of raspberry leaf curl disease in their collection. This project aims to develop a laboratory based assay for Raspberry leaf curl virus(es) to facilitate international movement of plant material, that is now limited by the requirement for biological indexing for this virus.</li><br /> </ol><br /> <p><strong> </strong></p><br /> <p><strong>University of Arkansas, Fayetteville, AR (Ioannis Tzanetakis)</strong></p><br /> <p>In collaboration with colleagues, several of which participate in WERA-20 we are working on the characterization and population structure of several viruses in blueberry, currant and blackberry. This information is used in the development of detection protocols that have the ability to detect the vast majority of isolates that circulate in the United States.</p><br /> <p> </p><br /> <p><strong>Certification</strong></p><br /> <p>We have open channels of communication with both industry and regulators to optimize the guidelines so as to be implemented in the near future. We are completing a three-year study on the presence of blueberry viruses across major production areas in the United States, information that will be used for the development of a heat map on blueberry viruses and the roadmap for the diagnostic scheme in G2-G4 nurseries.</p><br /> <p> </p><br /> <p><strong> </strong></p><br /> <p><strong> </strong></p><br /> <p><strong> </strong></p><br /> <p><strong>University of Hawaii (Michael Melzer, Wayne Borth, and John Hu)</strong></p><br /> <p><strong>Accomplishments</strong></p><br /> <p><em>Taro vein chlorosis virus</em> (TaVCV; genus Nucleorhabdovirus, family <em>Rhabdoviridae</em>) is a recent discovery in Hawaii that causes veinal chlorosis with a netted appearance, stunting and petiole streaking in taro (<em>Colocasia esculenta</em>). Plant death may occur in severe infections. Nucleotide and amino acid sequence comparisons and phylogenetic analyses revealed low levels of genetic diversity in the partial RNA-dependent RNA polymerase (RdRp) gene of 43 Hawaiian and 3 Palauan TaVCV isolates. This sequence information was used to design six new primer pairs targeting different regions of the RdRp gene. Primer set DCGF5/DCGR5 was identified as the most efficient of the six. Following optimization, highly sensitive and robust reverse transcription-polymerase chain reaction (RT-PCR) and immunocapture-RT-PCR (IC-RT-PCR) assays were developed. Localization of TaVCV in insect body parts essential for propagative, circulative virus transmission suggest that the taro planthopper, <em>Tarophagus proserpina</em>, is a vector of TaVCV.</p><br /> <p> </p><br /> <p><em>Dasheen mosaic virus</em> (DsMV) is one of the major viruses affecting taro production worldwide. We determined the genome sequences of two DsMV strains, Hawaii Strain I (GenBank:KY242358) and Hawaii Strain II (GenBank: KY242359), in taro from Hawaii. They represent the first genomic sequences of DsMV from the United States. Hawaii I and II had 77 and 85% identities respectively with other completely sequenced DsMV isolates. Hawaii I was most closely related to <em>Vanilla mosaic virus</em> (VanMV) (GebBank: KX505964.1), a strain of DsMV infecting vanilla in the southern Pacific Islands. Hawaii II was most closely related to DsMV isolate CTCRI-II-14 (GenBank: KT026108.1) in taro from India. Phylogenetic analysis of all available DsMV isolates based on their coat protein amino acid sequences showed some correlation between host plant and genetic diversity. Recombination analysis of full-length DsMV genome sequences was conducted. Our results showed that the recombination hotspots were mainly in the region of the CP and from C1 to NIa-Pro. To our knowledge, this is the first report of recombination events in DsMV. Both strains of DsMV were found to be widespread throughout the Hawaiian Islands.</p><br /> <p> </p><br /> <p><em>Taro bacilliform CH virus</em> (TaBCHV) is the second <em>badnavirus</em> found infecting taro, after <em>Taro bacilliform virus</em> (TaBV). We determined the complete genome of TaBCHV Hawaii strain (GenBank: KY359389) in taro from Hawaii and examined its occurrence and distribution. This is the first report of the occurrence of this virus in the United States. Alignment analysis showed the TaBCHV Hawaii strain has 93% and 94% sequence identity to China strains TaBCHV-1 and TaBCHV-2 respectively. Phylogenetic analysis based on the whole genome sequence of 21 selected badnaviruses, showed that the TaBCHV Hawaii strain clustered within the same branch as the two China strains. All sequences from available TaBCHV strains are most closely related to Piper yellow mottle virus (PYMoV; GenBank: NC022365) and are most distant from TaBV (GenBank: NC004450). We used RT-PCR to determine the incidence of TaBCHV on taro in Hawaii. Incidences of TaBCHV on the five islands were 72% on Oahu, 53% on Hawaii, 89% on Maui, 73% on Molokai, and 78% on Kauai, with a mean incidence of 73%.</p><br /> <p> </p><br /> <p><strong>Agdia Inc. (Shulu Zhang)</strong></p><br /> <p><strong>An update on rapid detection of viruses and viroids in fruit crops using isothermal AmplifyRP</strong></p><br /> <p>AmplifyRP® is Agdia's isothermal amplification platform for rapid detection of plant pathogens using an advanced isothermal amplification technology – recombinase polymerase amplification. Up to now, Agdia is able to offer test kits in all three AmplifyRP formats – AmplifyRP Acceler8, AmplifyRP XRT and AmplifyRP XRT+. Over the past year or so, Agdia has commercialized another five AmplifyRP test kits in addition to the previously released six AmplifyRP kits. The newly released AmplifyRP kits include AmplifyRP XRT+ for <em>Xylella fastidiosa</em> (XF), AmplifyRP XRT for <em>Clavibacter michiganensis</em> subsp. Michiganensis (CMM), AmplifyRP Acceler8 for <em>Grapevine red blotch virus</em> (GRBV), AmplifyRP Acceler8 for <em>Banana bunchy top virus</em> (BBTV), and AmplifyRP Acceler8 for <em>Tomato chlorotic dwarf viroid</em> (TCDVd). All these AmplifyRP test kits are rapid, simple, as sensitive as PCR, and field-deployable. This rapid diagnostic AmplifyRP technology has been approved to be very useful for rapid diagnostic detection of all sorts of plant pathogens and will continue to help to greatly improve crop production and agriculture.</p><br /> <p> </p><br /> <p><strong>University of California-Davis/Foundation Plant Services, Davis, CA (Maher Al Rwahnih and Deborah Golino)</strong></p><br /> <p><strong>Accomplishments</strong></p><br /> <p>At Foundation Plant Services (FPS), we continue to make advances in developing and refining our methods using high throughput sequencing (HTS) as a superior diagnostic tool. We have used sequence information generated by HTS analysis to design new, specific PCR primers for use in PCR diagnostics. In addition, HTS proves to be an invaluable tool in the discovery of unknown viruses and in establishing a baseline analysis of the virome of a crop.</p><br /> <p> </p><br /> <p>Our research on <em>Grapevine Pinot gris virus</em> (GPGV), first reported in the US in 2015 in a study of the collections at FPS in Davis, California and subsequently in Napa Valley vineyards in 2016, continues to be focused on gaining a better understanding of the virus with a focus on the incidence and distribution in California vineyards, symptomology, optimized detection methods, and horizontal spread. To assess the prevalence of asymptomatic infections, we conducted multiple surveys from different grape growing regions in California. Our field survey was focused primarily in Napa and Sonoma Counties. In Napa County, 275 vines were tested from 14 vineyards growing multiple grape varieties. The percentage of vines sampled that tested positive for GPGV per infected location ranged from 8.7 to 100%. In Sonoma County, no vines tested positive for GPGV of 144 vines from 16 vineyards. In addition, no vines tested positive for GPGV of 236 samples tested from 30 vineyards in multiple grape growing counties in California. While GPGV is known to be asymptomatic, in other cases, it occurs as a mixed infection with other symptomatic viruses. When symptoms are present, they appear as typical GFLV symptoms which include chlorotic mottling, leaf deformation and stunting. A real-time PCR (qPCR/RT-qPCR) analysis of known grapevine viruses on 121 GPGV-positive samples revealed that all symptomatic vines were also infected with GFLV. To characterize viral strains and determine the phylogenetic relationships among symptomatic and asymptomatic isolates, phylogenetic analysis on the genome sequences demonstrated that California isolates share 95-99% homology with asymptomatic reference isolates and 98-100% homology with each other. Our preliminary analysis also demonstrated that the asymptomatic Californian isolates have similar movement protein stop codon polymorphism to Italian isolates. A complete molecular characterization of California isolates enabled us to ascertain if our current detection methods are adequate in capturing all isolates of GPGV. We evaluated multiple sets of primers for their ability to detect GPGV and found that these primers can give false negative results, missing some known positive vines, especially late in the season. To overcome this problem, we developed a new RT-qPCR (real-time) assay for the detection of GPGV using an alignment of all available GPGV isolates. As new isolates of GPGV are sequenced this assay will be consistently updated to ensure that it detects all variant strains of the virus. In addition to developing new assays, we have begun to evaluate the optimal time of year for sampling/testing and the type of tissue which should be used (petioles vs bark scrapings). Thus far, it appears that the virus is a “spring virus” as virus titer is higher in May/June compared to later in the season. In addition, we are monitoring GPGV spread in a subset of 430 vines of Merlot 3 on Teleki 5C rootstock within an 18-acre block immediately adjacent to other GPGV infected blocks in Napa County. In 2016, 47 plants were positive; this data set will be compared with the data from the next three years.</p><br /> <p> </p><br /> <p>Progress has also been made in epidemiological studies of <em>Grapevine leafroll-associated virus 3</em> (GLRaV-3). Five GLRaV-3 epidemics were analyzed utilizing a standardized approach to robustly characterize the temporal and spatial parameters. Published data included in the analysis are from Spain, New Zealand, and Napa Valley, CA together with new data from a historic vineyard in Napa Valley, CA. Linear regression analyses of logit-transformed incidence data indicated a maximum average increase of 11% per year in disease incidence, with considerable variation among locations. Spatial analyses, including distribution fitting, examination of the effective sample size, and evaluation of the parameters of the binary power law fitted to variance data for disease incidence, indicated a high degree of consistency among the data sets. In all cases, except at very low disease incidence, a high degree of spatial aggregation was noted, with evidence that the degree of aggregation varied as a function of mean disease incidence. The polyetic dynamics of disease follow a logistic-like pattern over multiple seasons, consistent with limitation by inoculum availability (infected vines) at low incidence and limitation by non-infected vines at high incidence.</p><br /> <p> </p><br /> <p>New viral discoveries were made in pistachio trees. Pistachio trees from the National Clonal Germplasm Repository (NCGR) and selected orchards in California were surveyed for viruses and virus-like agents by high-throughput sequencing. Analyses of 60 trees, <em>Pistacia vera</em> and clonal UCB-1 hybrid rootstock (<em>P. atlantica</em> × <em>P. integerrima</em>), identified a novel virus found in the NCGR that showed low amino acid sequence homology (~42%) to members of genus Ampelovirus, family Closteroviridae and was provisionally named “Pistachio Ampelovirus A” (PAVA). A putative viroid, also found in the NCGR, showed similarities up to 87% to <em>Citrus bark cracking viroid</em> (CBCVd, genus Cocadviroid, family Pospiviroidae) and was provisionally named “Pistachio Cocadviroid 1” (PisCVd1). Both pathogens were graft transmissible to healthy UCB-1 plants. A field survey of 123 trees from commercial orchards found no incidence of PAVA, but five (4%) samples were infected with PisCVd1. Of the 690 NCGR trees, 16 (2.3%) were positive for PAVA and 172 (24.9%) were positive for PisCVd1. Additionally, several contigs across multiple samples exhibited significant sequence similarity to species in the families Caulimoviridae, Bromoviridae, Virgaviridae, Tombusviridae, Partitiviridae, Potyviridae, Alphaflexiviridae, Betaflexiviridae, Genomoviridae, Ophioviridae, and Phenuiviridae, and the genera Ourmiavirus and Sobemovirus. Results of the study establish the natural occurrence of a broad viral population infecting pistachio trees.</p><br /> <p> </p><br /> <p>While HTS remains a powerful new technology with significant benefits, there are technical challenges associated with the technology that warrants the establishment of guidelines for its use in plant certification and quarantine programs. We have begun efforts in a collaborative project to coordinate the development of minimum basic requirements for the adoption of HTS technologies, including nucleic acid extractions, library preparation, depth of sequencing and bioinformatics, for the detection of viral pathogens.</p><br /> <p> </p><br /> <p><strong>Cornell University (Marc Fuchs)</strong></p><br /> <p><strong>Accomplishments</strong></p><br /> <p>Research efforts focused on <em>grapevine red blotch virus</em> (GRBV), <em>grapevine fanleaf virus</em> (GFLV), <em>grapevine virus E</em> (GVE), <em>Australian grapevine viroid</em> (AGVd), and <em>Agrobacterium vitis</em>.</p><br /> <p> </p><br /> <p>For grapevine red blotch virus (GRBV) from the genus <em>Grablovirus</em> in the family <em>Geminiviridae </em>(Cieniewicz et al., 2017a; Varsani et al., 2017), we monitored GRBV spread over a three-year period (2014–2016) in a 2-hectare <em>Vitis vinifera</em> cv. ‘Cabernet franc’ vineyard based on an initially low disease incidence and an aggregation of symptomatic vines at the edge of the vineyard proximal to a wooded riparian area. The incidence of diseased plants increased by 1-2% annually (Cieniewicz et al., 2017b). Spatial analysis of diseased plants in each year using ordinary runs analysis within rows and Spatial Analysis by Distance IndicEs (SADIE) demonstrated aggregation. Spatiotemporal analysis between consecutive years within the association function of SADIE revealed a strong overall association among all three years (X= 0.874–0.945). Analysis of epidemic spread fitting a stochastic spatiotemporal model using the Monte Carlo Markov Chain method identified strong evidence for localized (within vineyard) spread. A spatial pattern consisting of a combination of strongly aggregated and randomly isolated symptomatic vines within 8-years post-planting suggested unique epidemic attributes compared to those of other grapevine viruses vectored by mealybugs and soft scales or by dagger nematodes for which typical within-row spread and small-scale autocorrelation are well documented. These findings are consistent with the existence of a new type of vector for a grapevine virus (Cieniewicz et al., 2017b). To determine the diversity and distribution of potential vector candidates in an infected vineyard, sticky cards were placed in 2015-2016 in a ‘Cabernet franc’ study site where disease incidence increased by nearly 20% between 2014 and 2016. Insects on sticky card traps were identified to species when possible by morphological characteristics and by sequencing of the mitochondrial cytochrome C oxidase subunit 1 gene. Subsets of insect species/taxa were removed from sticky cards and tested for the presence of GRBV by multiplex polymerase chain reaction. GRBV was consistently detected in <em>Spissistilus festinus</em> (Membracidae), <em>Colladonus reductus</em> (Cicadellidae), <em>Osbornellus borealis</em> (Cicadellidae) and a <em>Melanoliarus </em>species (Cixiidae) (Cieniewicz et al., 2017c). Populations of these four candidate vectors peaked from June to September with viruliferous <em>S. festinus</em> culminating from late June to early July in both years. An assessment of co-occurrence and co-variation between GRBV-infected vines and viruliferous insects using the association function of SADIE identified a significant association between the spatial distribution of infected vines and viruliferous <em>S. festinus</em>. Collectively, the findings revealed the epidemiological significance of <em>S. festinus</em> as a vector of GRBV and the need for testing the transmission capability of <em>C. reductus</em>, <em>O. borealis</em>, and the <em>Melanoliarus </em>species (Cieniewicz et al., 2017c). They also stress the relevance of the greenhouse studies previously carried out by Bahder et al. (2016) on the vectoring ability of <em>S. festinus</em>.</p><br /> <p> </p><br /> <p>We described the presence of GRBV in free-living grapes, including <em>V. californica</em> x <em>V. vinifera</em>, proximal to diseased vineyards (Perry et al., 2016). The full-length GRBV genome sequence was more similar among isolates from free-living grapes and a Merlot isolate than to more proximal Cabernet franc isolates. These results suggested that GRBV can spread by natural means (Perry et al., 2016). Subsequently, Badher et al. (2016) reported on <em>S. festinus</em> as a vector of GRBV. In addition to GRBV in wild grapes, we described a new grablovirus, name wild Vitis virus 1 (WVV1), in free-living grapes in California (Perry et al., 2017). The complete genome of WVV1 ranges from 3,204 to 3,278 nucleotides in size. The genome most closely resembles that of GRBV in both its organization and sequence identity (57-59%) (Perry et al., 2017). No information is available yet on the biology of WVV1 and its host range.</p><br /> <p> </p><br /> <p>In collaboration with Agdia, Inc., we contributed to the development of an AmplifyRP Acceeler8 diagnostic assay for GRBV (Li et al., 2017). This assay is rapid, reliable, specific and sensitive, and adapted to crude sap or nucleic acid extracts. It specifically detects up to 11 copies of GRBaV genomic DNA in a matrix of healthy crude extract. The AmplifyRP Acceeler8 diagnostic assay may assist the timely removal of GRBV-infected vines in vineyards.</p><br /> <p> </p><br /> <p>We also determined the economic cost of GRBV in California, Washington State and New York State. The economic impact was estimated to range from $2,213/ha in eastern Washington, when disease onset occurs at a low initial infection level and there is a low price penalty for poor fruit quality, to $68,548/ha in Napa County, when initial infection rates and quality penalties are both high (Ricketts et al., 2017). These values were further used to develop profit-maximizing disease management options. Roguing symptomatic vines and replanting with clean vines derived from virus-tested stocks minimize losses if GRBV incidence is low to moderate (below 30%), while a full vineyard replacement should be pursued if disease incidence is higher, generally above 30%. These findings should help vineyard managers adopt optimal GRBV management strategies (Ricketts et al., 2017).</p><br /> <p> </p><br /> <p>For <em>grapevine fanleaf virus</em> (GFLV) from the genus <em>Nepovirus</em> in the family <em>Secoviridae</em> (Thompson et al., 2017), we addressed a fundamental question in terms of virus-host interactions for symptom expression. By using infectious clones of GFLV strains F13 and GHu in a reverse genetics approach with wild-type, assortant and chimeric viruses, the molecular determinant of necrotic lesions caused by GFLV-F13 on inoculated leaves of <em>Nicotiana occidentalis</em> was mapped to the RNA2-encoded protein 2AHP, particularly to its 50 C-terminal amino acids (Martin et al., 2017). The necrotic response showed hallmark characteristics of a genuine hypersensitive reaction, such as the accumulation of phytoalexins, reactive oxygen species, pathogenesis-related protein 1c and hypersensitivity-related (hsr) 203J transcripts. Transient expression of the GFLV-F13 protein 2AHP fused to an enhanced green fluorescent protein (EGFP) tag in <em>N. occidentalis</em> by agroinfiltration was sufficient to elicit a hypersensitive reaction. In addition, the GFLV-F13 avirulence factor, when introduced in GFLV-GHu, which causes a compatible reaction on <em>N. occidentalis</em>, elicited necrosis and partially restricted the virus. This is the first identification of a nepovirus avirulence factor that is responsible for a hypersensitive reaction in both the context of virus infection and transient expression (Martin et al., 2017).</p><br /> <p> </p><br /> <p>For <em>grapevine virus E</em> (GVE) from the genus <em>Vitivirus</em> in the family <em>Betaflexiviridae</em>, we analyzed the genetic variability of isolates from California and New York. All GVE isolates were found in mixed infection with closterovirids or vitiviruses (Vargas et al., 2016). Sequence information indicated more than 98% nucleotide identity in the 3’ terminus of the coat protein gene, a short intergenic regions and the 5’ terminus of the putative nucleic acid binding protein gene of several GVE isolates. These results confirmed the presence of GVE in major U.S. grape-growing regions and indicated a very low level of genetic diversity (Vargas et al., 2016).</p><br /> <p> </p><br /> <p>For <em>Australian grapevine viroid</em> (AGVd) from the genus <em>Apscaviroid</em> in the family <em>Pospiviridae</em>, we reported its detection in two vineyards of <em>V. vinifera</em> cv. Syrah in New York. Vines were sampled in 2014, nucleic acid extracts prepared, and Illumina-compatible small RNA libraries constructed, sequenced (Illumina Hiseq 4000; single-end 50 nt reads), and analyzed (Vargas-Ascencio et al., 2017). AGVd was detected in the Syrah vines and its complete genome sequence showed >99% nucleotide identity to that of the original variant described by Rezaian and colleagues. To confirm this initial detection, vines were resampled in 2016 and an RT-PCR amplicon of approximately 270 nucleotides in length was produced; the product was detected in three of five vines and sequenced by the dideoxy DNA sequencing method. This second variant also showed >99% nucleotide sequence identity to the original variant. This study unequivocally confirms the presence of AGVd in North American vineyards (Vargas-Ascencio et al., 2017).</p><br /> <p> </p><br /> <p>For <em>Agrobacterium vitis</em>, we showed that the bacterium can be detected in dormant grape buds as well as on surfaces of leaves collected from commercial vineyards using a highly selective and sensitive method based on magnetic capture hybridization together with real-time PCR (Canik-Orel et al., 2017). Highest percentages of detection occurred in samples collected from vineyards with high incidences of crown gall. The bacterium was also detected in 22% of wild grapevines (<em>Vitis riparia</em>) collected in New York and from 25% of feral grapevines that included <em>V. californica</em> in California. Several of the free-living vines sampled were growing more than 2km from commercial vineyards, demonstrating that wild grapevines can serve as a significant reservoir of inoculum (Canik-Orel et al., 2017). </p><br /> <p> </p><br /> <p><strong>Washington State University (Naidu Rayapati)</strong></p><br /> <p>Virus diseases affecting fruit quality and vine health are one the highest research priorities for the grape and wine industry in Washington State. In response, the grape virology program (http://wine.wsu.edu/virology/ ) is conducting fundamental and applied research to generate science-based knowledge for mitigating negative impacts of virus diseases in vineyards. Among the major viral diseases, grapevine leafroll continues to be a major constraint to vineyard sustainability compared to grapevine red blotch and fanleaf degeneration and decline. Virus indexing of grapevine samples revealed that <em>Grapevine leafroll-associated virus 3</em> is ubiquitous in grower vineyards. Molecular characterization of the genome of <em>Grapevine leafroll-associated virus 1</em> revealed several unique features of the virus compared to other closteroviruses. Studies in commercial vineyards have shown that leafroll, red blotch, and fanleaf degeneration and decline can cause significant reduction in fruit yield and quality. However, these negative impacts were found to be cultivar- and site-specific responses likely influenced by genotype-by-environment interactions. Using baiting assays, the spread of <em>Tobacco ring spot virus</em> (TRSV) was demonstrated from infected grapevines to healthy cucumbers and Cabernet Franc vines. TRSV was detected by RT-PCR only in dagger nematodes isolated from soil samples taken near symptomatic grapevines. Using morphological characteristics and sequence analysis of the 28S large ribosomal D2-D3 expansion segment and the internal transcribed spacer region, the dagger nematode present in the vineyard soil was identified as <em>Xiphinema rivesi</em>. Virus indexing of grapevine samples done so far revealed the absence of <em>Grapevine red blotch virus</em> in certified grapevine mother blocks in Washington State. Participatory approaches were pursued with grape growers to conduct applied research activities in vineyards. The research-based knowledge was shared with grape and wine industry stakeholders via presentations at meetings and workshops and through publications in popular magazines, such as the Good Fruit Grower, for broader dissemination of research outcomes.</p><br /> <p> </p><br /> <p><strong>Clemson University, SC (S.W. Scott)</strong></p><br /> <p>In the summer/fall of 2011 the root suckers of a tree of <em>Prunus serrulata</em> cv Shirofugen grafted onto virus-indexed F12/1 Mazzard growing at the university research farm (Musser Farm) displayed distinct line patterns. Full length genomic sequence for the virus was developed and deposited as accession KX389311 in GenBank. This accession showed 97% identity with accession KF356396 (an isolate of <em>Cherry rusty mottle-associated virus</em> described from Washington State). This virus had not previously been described from South Carolina (SC). It is suggested that the virus originated from ornamental flowering cherry material (‘Yoshino’ cherry [<em>Prunus</em> × <em>yedoensis</em>]) planted on the nearby University Campus and “chip-budded” to the tree of “Shirofugen” as a positive control sample during a bioassay for <em>Prunus necrotic ringspot virus</em>. RT-PCR tests of leaves from both the suckers and the scion detected the virus in the suckers but not in the scion. A survey of 15 other Yoshino cherry trees growing on campus using both PCR, and symptom expression on Mazzard seedlings following graft transmission, detected the presence of the same virus in 4 of the trees sampled.</p><br /> <p> </p><br /> <p>The incidence of 5 viruses frequently found affecting blackberry was examined between 2012- 2014 in 2 “large” plantings in SC by employing sentinel plants. Virus-indexed sentinel plants of Ouachita and Natchez were exposed at a number of different locations in 2 plantings: 35 acres at Cooley’s in Boiling Springs, SC and 29 acres at the Double-J farm in Landrum SC. The exposure was for 30 days in each of the months of May through August. Following exposure the plants were treated with insecticides and then maintained in a screen-house until the following spring when RT-PCR testing of the new growth was completed. The 5 viruses for which tests were completed were <em>Blackberry yellow vein-associated virus</em> (BYVaV), <em>Blackberry chlorotic ringspot virus</em> (BCRV), <em>Blackberry virus Y</em> (BVY), <em>Blackberry virus E</em> (BVE), and <em>Blackberry leaf mottle associated virus</em> (BLMaV). Although the 2 locations were only 30 miles apart, and had blackberry cultivars in common (Natchez), the incidence of viruses detected was different. For example in 2012 a peak of BYVaV (11/100 sentinel plants) was detected in July at Cooley’s whereas in 2013 a peak of BYVaV (19/60 sentinel plants) was detected in May at the Double-J farm and only 4 detections of BYVaV we made for the entire season at Cooley’s. All of the viruses have insect vectors. Although BCRV is thought to be transmitted through the action of thrips feeding on wind-blown infected pollen grains rather than direct acquisition and inoculation of the virus through insect feeding.</p><br /> <p> </p><br /> <p><strong>PA Department of Agriculture, Harrisburg, PA (Katya Nikolaeva)</strong></p><br /> <p>The PA Department of Agriculture continues to operate the Fruit Tree Improvement Program (FTIP), a specialized virus-tested fruit tree certification program. Three nurseries have been participating in the FTIP this year. The purpose of the program is to encourage the use of NCPN-produced or best-available source material in nursery stock production. The program involves working with nurseries to design practices consistent with clean-stock certification regulations; auditing nursery practices; and inspecting and testing nursery source and production materials.</p><br /> <p> </p><br /> <p>In spring 2017, over 2,000 samples were tested for viruses of concern. All stone fruit nursery materials were tested for Prunus necrotic ringspot, prune dwarf, tomato ringspot, and plum pox virus. Sampling in apple and pear was primarily for <em>tomato ringspot virus</em>. All blocks met virus-testing requirements for FTIP certification. No PDV or ToRSV was detected in rootstock blocks or in registered source blocks. PNRSV remains the most commonly found viruses in <em>Prunus </em>in Pennsylvania, although finds in registered blocks and nursery production blocks are rare. All samples tested negative for plum pox virus, a virus declared eradicated from Pennsylvania in 2009.</p><br /> <p> </p><br /> <p>In cooperation with Dr. Kari Peter, Penn State University, a Farm Bill-funded survey for exotic diseases in orchards was conducted targeting <em>plum pox virus</em>, two exotic phytoplasmas, and two exotic brown rot pathogens. No exotic targets were detected, but we did confirm presence of <em>Ca. Phytoplasma pruni</em> (16SrIII-A group, X-disease group) in apple and <em>Ca. Phytoplasma pyri</em> (16SrX, Apple Proliferation group) in pears for a third year. Potentially new phytoplasma species related to ash yellows group was detected in peaches for a second year.</p><br /> <p> </p><br /> <p>Data gathering continued on a Rapid Apple Decline syndrome in 3-4-year-old apple trees on M9 rootstocks. In collaboration with Ruhui Li (USDA ARS) and Kari Peter (Penn State) we discovered a new luteovirus from apple trees in the RAD-affected orchards using high throughput sequencing (HTS) technology. The virus is provisionally named Apple luteovirus 1 (ALV-1). Currently we are conducting a survey of RAD affected orchards in PA for a presence of new luteovirus and known latent viruses to determine their possible contribution to RAD symptoms.</p><br /> <p> </p><br /> <p><strong>Micropropagation and Repository Unit (MPRU), North Carolina State University (Christie Almeyda)</strong></p><br /> <p>The MPRU maintains G1 pathogen-tested (Foundation) in the Repository of Strawberry cultivars and advanced selections (greenhouse and in vitro), blackberry cultivars (greenhouse, screen house and cold storage), raspberry cultivars and advanced selections (greenhouse, screen house and cold storage) and blueberry cultivars and advanced selections (greenhouse, screen house and in vitro). Currently, material that is in various stages of treatment corresponds to 2 blueberry, 2 strawberry, and 7 blackberry advanced selections. P526 permits were obtained and will be used to exchange positive controls with other centers in the NCPN. Virus testing capacity and standardization has improved to follow NCPN standards. Positive controls are being obtained from other centers and commercial sources. Current activities are the ring test with other NCPN centers, yearly testing of strawberry mother plants, clean-up of 2015 and 2016 blackberry selections from Dr. Clark in Arkansas, blueberry selections from Dr. Ballington in North Carolina and raspberry selections from Dr. Fernandez in North Carolina. All this material has to be virus tested and meristem-tip culture or established in tissue culture by tip/nodal cuttings.</p><br /> <p> </p><br /> <p><strong>MPRU leadership changes</strong></p><br /> <p>Dr. Christie Almeyda was appointed as interim director by the Head of the Department of Entomology and Plant Pathology in June 2017.</p>Publications
<p><strong>CFIA, Center for Plant Health, Government of Canada</strong></p><br /> <p>James D. 2017. Perspectives on strategies for controlling the spread of plum pox virus, causal agent of sharka/plum pox disease. Acta Hortic 1163. ISHS 2017 Proceedings of the III International Symposium on Plum Pox Virus; p.129-136.</p><br /> <p>James D, Phelan J. 2017. Complete genome sequence and analysis of blackcurrant leaf chlorosis associated virus, a new member of the genus Idaeovirus. Archives of Virology. 162: 1705-1709.</p><br /> <p>James D, Sanderson D, Dolgikh S, Spiegel S. 2017. Molecular analysis of the complete genomes of apricot and plum isolates of Plum pox virus detected in a Prunus germplasm collection in Almaty’s Pomological Gardens, Kazakhstan. Acta Hortic. 1163. ISHS 2017 Proceedings of the III International Symposium on Plum Pox Virus; p. 101-107.</p><br /> <p>Kesanakurti, P., Belton, M., Saeed, H., Rast, H., Boyes, I., and Rott, M. 2016. Screening for plant viruses by next generation sequencing using a modified double strand RNA extraction protocol with an internal amplification control. Journal of Virological Methods. 236: 35-40</p><br /> <p>Phelan J, James D. 2016. Complete genome sequences of a putative new alphapartitivirus detected in Rosa spp. Archives of Virology. 161: 2623-2626.</p><br /> <p>Poojari S, Boulé J, DeLury N, Lowery D.T., Rott M, Schmidt A-M, Urbez-Torres J.T. 2017. Epidemiology and genetic diversity of grapevine leafroll-associated viruses in British Columbia. Plant Disease.</p><br /> <p>Poojari S, Lowery D.T., Rott M, Schmidt A-M, Urbez-Torres J.T. 2017. Incidence, distribution and genetic diversity of Grapevine red blotch virus in British Columbia. Canadian Journal of Plant Pathology 39:2 201-211.</p><br /> <p>Rott et al. 2017. Application of Next Generation Sequencing for Diagnostic Testing of Tree Fruit Viruses and Viroids. Plant Disease 101:8, 1489-1499.</p><br /> <p>Safarova D, Neoralova V, James D, Navratil M. 2017. Almond (Prunus dulcis L.) - not a natural host of Plum pox virus in the Czech Republic. Acta Hortic 1163. ISHS 2017 Proceedings of the III International Symposium on Plum Pox Virus; 123-127.</p><br /> <p>Sanderson D, Fu J, James D. 2017. Identification of possible evolutionary pathways of Plum pox virus and predicting amino acid residues of importance to host adaptation. Acta Hortic. 1163. ISHS 2017 Proceedings of the III International Symposium on Plum Pox Virus; p. 107-116.</p><br /> <p>James D, Phelan J, Jesperson G. 2017. The Geneva Complex – an interesting disease perspective based on the results of NGS analysis. 24<sup>th</sup> International Conference on Virus and Other Graft Transmissible Diseases of Fruit Crops (ICVF), Thessaloniki, Greece, June 5 – 9, 2017. Book of Abstracts. Pg 43 (Abstr.)</p><br /> <p>James D, Phelan J, Sanderson D. 2017. Evidence of circular RNA associated with blackcurrant leaf chlorosis associated virus. 24<sup>th</sup> International Conference on Virus and Other Graft Transmissible Diseases of Fruit Crops (ICVF), Thessaloniki, Greece, June 5 – 9, 2017. Book of Abstracts. Pg 126 (Abstr.)</p><br /> <p>Jelkmann W, Sanderson D, Berwarth C, James D. 2017. Detection and characterization of the complete genome of the first cherry (C) strain isolate of plum pox virus detected in Germany in sour cherry (<em>Prunus cerasus</em>). 24<sup>th</sup> International Conference on Virus and Other Graft Transmissible Diseases of Fruit Crops (ICVF), Thessaloniki, Greece, June 5 – 9, 2017. Book of Abstracts. Pg 99 (Abstr.)</p><br /> <p>Messmer A, Sanderson D, Braun G, Serra P, Flores R, James D. 2017. Characterization of a new and distinct population of hammerhead viroid-like RNA detected by next-generation sequencing using total RNA from apple (<em>Malus domestica</em>) cv. Pacific Gala. 24<sup>th</sup> International Conference on Virus and Other Graft Transmissible Diseases of Fruit Crops (ICVF), Thessaloniki, Greece, June 5 – 9, 2017. Book of Abstracts. Pg 36 (Abstr.)</p><br /> <p>Milusheva S, Phelan J, Piperkova N, Nikolova V, James D. 2017. Detection and molecular characterization of an unusual virus in cherry. 24<sup>th</sup> International Conference on Virus and Other Graft Transmissible Diseases of Fruit Crops (ICVF), Thessaloniki, Greece, June 5 – 9, 2017. Book of Abstracts. Pg 122 (Abstr.)</p><br /> <p><strong>USDA-ARS, Horticultural Crops Research Unit, Corvallis, OR</strong></p><br /> <p>Diaz-Lara, A. and Martin, R.R. 2016. Blueberry fruit drop associated virus: A new member of the family Caulimoviridae isolated from blueberry exhibiting fruit drop symptoms. Plant Dis. 100:2211-2214.</p><br /> <p> </p><br /> <p>Hassan, M., Di Bello, P.L., Keller, K.E., Martin, R.R., Sabanadzovic, S. and Tzanetakis, I.E. 2017. A new, widespread emaravirus discovered in blackberry. Virus Research 235:1-5.</p><br /> <p> </p><br /> <p>Martin, R.R. and Ellis, P. 2017. In memoriam/A la memoire de, Dr. Richard Stace-Smith, 1924-2017. Canadian Journal Plant Pathology 39:1-4.</p><br /> <p> </p><br /> <p>Tzanetakis, I.E. and Martin, R.R. 2017. A systems-based approach to manage strawberry virus diseases. Canadian Journal Plant Pathology 39:5-10.</p><br /> <p> </p><br /> <p>Diaz-Lara, A., Santamaria, L. and Martin, R.R. 2017. Identification of Tomato mosaic virus (ToMV) and Potato latent virus (PotLV) as mixed infection in Chinese lantern (<em>Physalis alkekengi</em>) in the United States. Plant Dis. 101:1061.</p><br /> <p> </p><br /> <p><strong>University of Arkansas</strong></p><br /> <p>Hassan, M., Di Bello. P.L., Keller, K.E., Martin, R.R., Sabanadzovic S. and Tzanetakis, I.E. 2017. A new, widespread emaravirus discovered in blackberry. Virus Research 235: 1-5.</p><br /> <p> </p><br /> <p>Tzanetakis, I.E. and Martin, R.R. 2017. A systems-based approach to counter strawberry virus diseases. Canadian Journal of Plant Pathology 39: 5-10.</p><br /> <p> </p><br /> <p>Thekke-Veetil, T., Khadgi, A., Johnson, D.T., Burrack, H., Sabanadzovic, S. and Tzanetakis, I.E. 2017. First report of raspberry leaf mottle virus in blackberry in the United States. Plant Disease 101: 265</p><br /> <p> </p><br /> <p>Shahid, M.S., Aboughanem-Sabanadzovic, N., Sabanadzovic, S. and Tzanetakis, I.E. 2017. Genomic characterization and population structure of a badnavirus infecting blackberry. Plant Disease 101: 110-115</p><br /> <p> </p><br /> <p>Martin R.R. and Tzanetakis, I.E. 2017. Introduction to diseases caused by viruses and virus-like agents. Pp. 70-71. In: Martin, R.R., Ellis, M.A., Williamson, B. and Williams, R.N. (Ed) Compendium of Raspberry and Blackberry Diseases and Insects 2nd Edition. APS Press, St. Paul, MN.</p><br /> <p> </p><br /> <p>Tzanetakis, I.E., Susaimuthu, J., Sabanadzovic S., and Martin R.R. 2017. Blackberry Yellow Vein Disease Complex (BYVD). Pp. 71-75. In: Martin, R.R., Ellis, M.A., Williamson, B. and Williams, R.N. (Ed) Compendium of Raspberry and Blackberry Diseases and Insects 2nd Edition. APS Press, St. Paul, MN.</p><br /> <p> </p><br /> <p>MacFarlane, S.A., Tzanetakis, I.E., Halgren, A.B. and Martin, R.R. 2017. Raspberry mosaic disease complex. Pp. 75-78. In: Martin, R.R., Ellis, M.A., Williamson, B. and Williams, R.N. (Ed.) Compendium of Raspberry and Blackberry Diseases and Insects 2nd Edition. APS Press, St. Paul, MN.</p><br /> <p> </p><br /> <p>Tzanetakis, I.E. 2017. Blackberry virus F. Pp. 80. In: Martin, R.R., Ellis, M.A., Williamson, B. and Williams, R.N. (Ed.) Compendium of Raspberry and Blackberry Diseases and Insects 2nd Edition. APS Press, St. Paul, MN.</p><br /> <p> </p><br /> <p>Quito-Avila, D.F., Tzanetakis, I.E. and Martin, R.R. 2017. Raspberry latent virus. Pp. 84-85. In: Martin, R.R., Ellis, M.A., Williamson, B. and Williams, R.N. (Ed) Compendium of Raspberry and Blackberry Diseases and Insects 2nd Edition. APS Press, St. Paul, MN.</p><br /> <p> </p><br /> <p>Tzanetakis, I.E. and Martin, R.R. 2017. Strawberry necrotic shock virus. Pp. 90-91. In: Martin, R.R., Ellis, M.A., Williamson, B. and Williams, R.N. (Ed) Compendium of Raspberry and Blackberry Diseases and Insects 2nd Edition. APS Press, St. Paul, MN.</p><br /> <p> </p><br /> <p>Martin, R.R. and Tzanetakis, I.E. 2017. Other viruses and virus-like agents. In: Pp. 91-93. In: Martin, R.R., Ellis, M.A., Williamson, B. and Williams, R.N. (Ed) Compendium of Raspberry and Blackberry Diseases and Insects 2nd Edition. APS Press, St. Paul, MN.</p><br /> <p> </p><br /> <p>Tzanetakis, I.E. and Martin, R.R. 2016. Blueberry latent spherical virus. Pp. 60, In: Polashock, J.J., Caruso, F.L., Averill, A.L. and Schilder A.C. (Ed) Compendium of Blueberry, Cranberry and Lingonberry Diseases and Pests 2nd Edition, APS Press, St. Paul, MN.</p><br /> <p> </p><br /> <p>Tzanetakis, I.E. and Martin, R.R. 2016. Blueberry latent virus. Pp. 60, In: Polashock, J.J., Caruso, F.L., Averill, A.L. and Schilder A.C. (Ed) Compendium of Blueberry, Cranberry and Lingonberry Diseases and Pests 2nd Edition, APS Press, St. Paul, MN.</p><br /> <p> </p><br /> <p>Tzanetakis, I.E. and Martin, R.R. 2016. Blueberry virus A. Pp. 60-61, In: Polashock, J.J., Caruso, F.L., Averill, A.L. and Schilder A.C. (Ed) Compendium of Blueberry, Cranberry and Lingonberry Diseases and Pests 2nd Edition, APS Press, St. Paul, MN.</p><br /> <p> </p><br /> <p>Martin, R.R. and Tzanetakis, I.E. 2016. Blueberry mosaic virus. Pp. 64, In: Polashock, J.J., Caruso, F.L., Averill, A.L. and Schilder A.C. (Ed) Compendium of Blueberry, Cranberry and Lingonberry Diseases and Pests 2nd Edition, APS Press, St. Paul, MN.</p><br /> <p> </p><br /> <p>Tzanetakis, I.E. and Martin, R.R. 2016. Blueberry Certification. Pp. 154-156, In: Polashock, J.J., Caruso, F.L., Averill, A.L. and Schilder A.C. (Ed) Compendium of Blueberry, Cranberry and Lingonberry Diseases and Pests 2nd Edition, APS Press, St. Paul, MN.</p><br /> <p> </p><br /> <p><strong>University of Hawaii</strong></p><br /> <p>Green, J.C., Borth, W.B., Melzer, M.J., Wang, Y.N., Hamim, I.,and Hu, J.S. 2017. First Report of <em>Bean common mosaic virus</em> infecting <em>Phaseolus lunatus</em> in Hawaii. Plant Disease 101:1557.</p><br /> <p> </p><br /> <p>Green, J.C. and Hu. J.S. 2017. Editing Plants for Virus Resistance Using CRISPR-Cas. Acta Virologica 61: 138 – 142.</p><br /> <p> </p><br /> <p>Li, Y., Wang, Y., Hu, J., Xiao, L., Tan, G., Lan, P., Liu, Y., and Li, F. 2017. Molecular and biological characteristics of <em>Tomato mottle mosaic virus</em> Chinese isolate. Virology Journal 14:15-23.</p><br /> <p> </p><br /> <p>Dey, K., Melzer M., and J. Hu 2017. Virus-Induced Gene Silencing in Plant Biotechnology, Volume 2: Transgenics, Stress Management, and Biosafety Issues. (In press)</p><br /> <p> </p><br /> <p>James C. Green, Wayne Borth, John S. Hu (2016) Engineering Resistance to Viruses. In: Mohandas S, Ravishankar KV (eds) Banana: genomics and transgenic approaches for genetic improvement. Springer, Singapore, pp 237-246.</p><br /> <p> </p><br /> <p>Wang, Y. N., Borth, W. B., Hamim, I., Green, J.I., Melzer, M.J., and Hu, J.S. 2017. First Report of <em>Taro bacilliform CH Virus</em> (TaBCHV) on Taro (<em>Colocasia esculenta</em>) in Hawaii. Plant Disease 101:1334.</p><br /> <p> </p><br /> <p>Wang, Y.N., Melzer, M., Borth, W., Green, J. Hamim, I., and Hu, J.S. 2017. First report of <em>Bean yellow mosaic virus</em> in vanilla in Hawaii. Plant Disease 101:1557.</p><br /> <p> </p><br /> <p>Zhang, J., Borth, W.B., Sether, D., Wang, I., Lin, B, Melzer, M.J., Shen, H., Pu, X, Nelson, S., Hu, J.S. 2017. Characterization of <em>Canna yellow mottle virus</em> in a new host, <em>Alpinia purpurata</em>, in Hawaii. Phytopathology 107:791-799.</p><br /> <p> </p><br /> <p>Watanabe, S., Ruschel, R., Marrero, G., Sether, D., Borth, W., Hu, J., and Melzer, M. 2016. A distinct lineage of <em>Watermelon mosaic virus</em> naturally infects honohono orchid (<em>Dendrobium anosmum</em>) and passionfruit (<em>Passiflora edulis</em>) in Hawaii. New Disease Reports 34:13.</p><br /> <p> </p><br /> <p>Green, K.J., Chikh-Ali, M., Hamasaki, R, Melzer, M.J., and Karasev, A.V. 201X. Potato virus Y (PVY) isolates from <em>Physalis peruviana</em> are unable to systemically infect potato or pepper and form a distinct new lineage within the PVY<sup>C</sup> strain group. Phytopathology. (In press)</p><br /> <p> </p><br /> <p>Dey, K., Melzer, M., Sun, X., and Adkins, S. 201X. <em>Tomato chlorotic spot virus</em> identified in <em>Marsdenia floribunda</em> in Florida. Plant Health Progress. (In press)</p><br /> <p> </p><br /> <p><strong>Agdia Inc.</strong></p><br /> <p>Hammond RW, Zhang S. 2016. Development of a rapid diagnostic assay for the detection of <em>Tomato chlorotic dwarf viroid</em> based on isothermal reverse-transcription-recombinase polymerase amplification. Journal of Virological Methods 236:62-67.</p><br /> <p> </p><br /> <p>Zhang S, Russell P, McOwen N, Davenport B, Li R. 2017. Development of a novel isothermal AmplifyRP® assay for rapid detection of <em>Plum pox virus</em> - a real-time and endpoint assay in a single PCR tube. Acta Hortic. (ISHS) 1163:31-37.</p><br /> <p> </p><br /> <p>Li R, Fuchs MF, Perry KL, Mekuria T, Zhang S. 2017. Development of a fast diagnostic AmplifyRP Acceler8 assay for <em>Grapevine red blotch virus</em>. J. Plant Pathol. (accepted).</p><br /> <p> </p><br /> <p><strong>University of California-Davis/Foundation Plant Services, Davis, CA</strong></p><br /> <p>Al Rwahnih, M., Alabi, O.J., Westrick, N.M., Golino, D. and Rowhani, A. 2017. Description of a novel monopartite geminivirus and its defective subviral sequence in grapevine (<em>Vitis vinifera</em> L.). Phytopathology, 107(2): 240-251.</p><br /> <p> </p><br /> <p>Yahaya, A., Al Rwahnih, M., Dangora, D., Gregg, L., Alegbejo, M., Kumar, P., and Alabi, O. 2017. First report of Maize yellow mosaic virus infecting sugarcane (<em>Saccharum</em> spp.) and itch grass (<em>Rottboellia cochinensis</em>) in Nigeria. Plant Disease/ http://dx.doi.org/10.1094/PDIS-03-17-0315- PDNA.</p><br /> <p> </p><br /> <p>Olufemi J. Alabi, Al Rwahnih. M., Jifon, J. L., Sétamou, M., Brown, J.K., Gregg, L., and J-W. Park. 2017. A Mixed <em>Infection of Lettuce chlorosis virus</em>, <em>Papaya ringspot virus</em>, and <em>Tomato yellow leaf curl virus</em>-IL Detected in a Texas Papaya Orchard Affected by a Virus-Like Disease Outbreak. Plant Disease/ <a href="http://dx.doi.org/10.1094/PDIS-01-17-0118-RE">http://dx.doi.org/10.1094/PDIS-01-17-0118-RE</a>.</p><br /> <p> </p><br /> <p>Voncina, D., Al Rwahnih, M., Rowhani, A., Gouran, M. and Almeida, R. 2017. Viral Diversity in Autochthonous Croatian Grapevine Cultivar. Plant Disease, PDIS-10-16-1543-RE.</p><br /> <p> </p><br /> <p>Al Rwahnih, M., Alabi, O.J., Westrick, N.M., Golino, D. and Rowhani, A. 2017. Near complete genome sequence of grapevine fabavirus, a novel putative member of the genus Fabavirus. Genome Announcements, 4(4): e00703-16.</p><br /> <p> </p><br /> <p>Osman, F., Al Rwahnih, M. and Rowhani, A. 2017. Real-Time RT-qPCR <em>Detection of Cherry Rasp Leaf Virus</em>, <em>Cherry Green Ring Mottle Virus</em>, <em>Cherry Necrotic Rusty Mottle Virus</em>, <em>Cherry Virus A</em> and <em>Apple Chlorotic Leaf Spot Virus</em> in Stone Fruits. Journal of Plant Pathology, 99(1): 279-285.</p><br /> <p> </p><br /> <p>Rasool, S., Naz, S., Rowhani, A., Golino, D.A., Westrick, N.M., Farrar, K.D., and Al Rwahnih, M. 2017. First report of <em>Grapevine Pinot Gris virus</em> infecting grapevine in Pakistan. Plant Disease/https://doi.org/10.1094/PDIS-04-17-0476-PDN.</p><br /> <p> </p><br /> <p>Fagundes Silva, J.M., Al Rwahnih, M., Blawid, R., Nagata, T., and Fajardo, T. 2017. Discovery and molecular characterization of a novel anamovirus, Grapevine enamovirus-1. Virus genes. ** IN PRESS **</p><br /> <p> </p><br /> <p>Arnold, K., Golino, D. A., and N. McRoberts. 2017. A synoptic analysis of the temporal and spatial aspects of grapevine leafroll disease in an historic Napa vineyard and experimental vine blocks. Phytopathology. 107:418 -426.</p><br /> <p><strong> </strong></p><br /> <p>Rowhani, A., Daubert, S.D., Uyemoto, J.K. Al Rwahnih, M. and M. Fuchs. 2017. Grapevine Viruses: Molecular Biology, Diagnostics and Management, Chapter five. American Nepoviruses, 109-126.</p><br /> <p> </p><br /> <p>Al Rwahnih, M., Rowhani, A., and P. Saldarelli. 2017. Grapevine Viruses: Molecular Biology, Diagnostics and Management, Chapter ten. Grapevine leafroll-associated virus-7., 221-228.</p><br /> <p> </p><br /> <p>Rowhani, A., Uyemoto, J.K., Golino, D., Daubert, S.D., and M. Al Rwahnih. 2017. Grapevine Viruses: Molecular Biology, Diagnostics and Management, Chapter thirteen-backspace. Viruses Involved in Graft-Incompatibility and Decline, 289-302.</p><br /> <p> </p><br /> <p>Rowhani, A., Osman, F., Daubert, S.D., Al Rwahnih, M. and P. Saldarelli. 2017. Grapevine Viruses: Molecular Biology, Diagnostics and Management, Chapter twenty. Chain Reaction Methods for the Detection of Grapevine Viruses and Viroids., 431-450.</p><br /> <p> </p><br /> <p>Saldarelli, P., Giampetruzzi, A., Maree, H., and M. Al Rwahnih. 2017. Grapevine Viruses: Molecular Biology, Diagnostics and Management, Chapter thirty. Next generation sequencing: advantages beyond virus identification, 625-642.</p><br /> <p> </p><br /> <p>Golino, D. A., Fuchs, M., Sim, S., Farrar, K., and G. P. Martelli. 2017. Grapevine Viruses: Molecular Biology, Diagnostics, and Management, Chapter twenty-seven. Improvement of grapevine planting stock through sanitary selection a pathogen elimination, 561-580.</p><br /> <p> </p><br /> <p>Golino, D. A., Fuchs, M., Al Rwahnih, M., Farrar, K., Schmidt, A., and G.P. Martelli. 2017. Grapevine Viruses: Molecular Biology, Diagnostics and Management, Chapter twenty-eight. Regulatory aspects of grape viruses and virus diseases: certification, quarantine, and harmonization, 581-598.</p><br /> <p> </p><br /> <p>Al Rwahnih, M., Westrick, N., Golino, D., and Rowhani, A. Survey for <em>grapevine pinot gris virus</em> infecting grapevine in the Foundation Plant Services Vineyards at the University of California, Davis. American Phytopathological Society. Tampa, FL, 2016.</p><br /> <p> </p><br /> <p>Voncina, D., Al Rwahnih, M., Rowhani, A., and Almeida, R. 2016. Survey for viruses of grapevine (<em>Vitis vinifera</em> L.) in coastal vineyards of Croatia. American Phytopathological Society. Tampa, FL.</p><br /> <p> </p><br /> <p>Alabi, O., Al Rwahnih, M., Brown, J., Jifon, J., Park, J., Gregg, L., Setamou, M., and Idris, A. 2016. Association of a mixed infection of <em>Lettuce chlorosis virus</em>, <em>Papaya ringspot virus</em>, and <em>Tomato yellow leaf curl virus</em>-IL in a Texas papaya orchard. American Phytopathological Society. Tampa, FL.</p><br /> <p> </p><br /> <p>Al Rwahnih, M., Golino, D., Westrick, N., Stevens, K., Trouillas, F., Preece, J., Kallsen, C., Farrar, K., and Rowhani, A. Metagenomic analysis of virus and virus-like pathogens infecting pistachio in California. San Antonio. ** SUBMITTED **</p><br /> <p> </p><br /> <p>Al Rwahnih, M., Golino, D., Westrick, N., Diaz Lara, A., Cooper, M., Smith, R., Battany, M., Bettiga, L., Zhuang, S., Arnold, A., Farrar, K., and Rowhani, A. <em>Grapevine pinot gris virus</em>: an emerging virus in Napa Valley vineyards. San Antonio, TX. ** SUBMITTED **</p><br /> <p> </p><br /> <p>Diaz Lara, A., Stevens, K., Westrick, N., Golino, D., and Al Rwahnih, M. Identification of a caulimo-like virus in pistachio via high-throughput sequencing. San Antonio, TX. ** SUBMITTED **</p><br /> <p> </p><br /> <p>Al Rwahnih, M., Klaassen, V., Stevens, K., Arnold, K., Maree, H.J., Westrick, N., and Golino, D. Molecular characterization of divergent <em>Grapevine leafroll-assiciated virus 3</em> isolates in California, USA. San Antonio, TX. ** SUBMITTED **</p><br /> <p> </p><br /> <p>Harvenson, R., Al Rwahnih, M., Tian, T., Karasev, A., and Gulya T. A New Virus Disease of Sunflower in Nebraska. San Antonio, TX. ** SUBMITTED **</p><br /> <p> </p><br /> <p><strong>Cornell University</strong></p><br /> <p>Canik-Orel, D., Reid, C., Fuchs, M. and Burr, T. 2017. Environmental sources of <em>Agrobacterium vitis</em> in vineyards and wild grapevines. American Journal of Enology and Viticulture, 68:213-217.</p><br /> <p> </p><br /> <p>Cieniewicz, E.J., Perry, K.L. and Fuchs, M. 2017a. <em>Grapevine red blotch virus</em>: Molecular biology of the virus and management of the disease. In: Grapevine Viruses: Molecular Biology, Diagnostics and Management. Meng, B.,</p><br /> <p>Martelli, G.P., Golino, D.A. and Fuchs, M.F (eds). Springer Verlag, pp. 303-314.</p><br /> <p> </p><br /> <p>Cieniewicz, E., Pethybridge S., Gorny, A., Madden, L., Perry, K.L., McLane, H. and Fuchs, M. 2017b. Spatiotemporal spread of grapevine red blotch-associated virus in a California vineyard. Virus Research, DOI: 10.1016/J.VIRUSRES.2017.03.020.</p><br /> <p> </p><br /> <p>Cieniewicz, E., Pethybridge S., Loeb, G., Perry, K.L., and Fuchs, M. 2017c. Diversity and spatial distribution of vector candidates of grapevine red blotch virus in a diseased vineyard. Phytopathology, in revision.</p><br /> <p> </p><br /> <p>Fuchs, M. and Lemaire, O. 2017a. Novel approaches for virus disease management. In: Grapevine Viruses: Molecular Biology, Diagnostics and Management. Meng, B., Martelli, G.P., Golino, D.A. and Fuchs, M.F (eds). Springer Verlag, pp. 599-621.</p><br /> <p> </p><br /> <p>Fuchs, M. Schmitt-Keichinger, C. and Sanfaçon, H. 2017b. A renaissance in nepovirus research provides new insights into their molecular interface with hosts and vectors. In: Advances in Virus Research, M. Kielian, K. Maramorosch, T.C Mettenleiter and M.J Roosinck (eds.), pp. 61-105.</p><br /> <p> </p><br /> <p>Golino, D., Fuchs, M., Sim, S., Farrar, K. and Martelli, G. 2017a. Improvement of grapevine planting stock through sanitary selection and pathogen elimination. In: Grapevine Viruses: Molecular Biology, Diagnostics and Management. Meng, B., Martelli, G.P., Golino, D.A. and Fuchs, M.F (eds). Springer Verlag, pp. 561-579.</p><br /> <p> </p><br /> <p>Golino, D., Fuchs, M., Al Rwanih, M., Farrar, K., and Martelli, G.P. 2017b. Regulatory aspects of grape virology: Certification, quarantine and harmonization. In: Grapevine Viruses: Molecular Biology, Diagnostics and Management. Meng, B., Martelli, G.P., Golino, D.A. and Fuchs, M.F (eds). Springer Verlag, pp. 581-598.</p><br /> <p> </p><br /> <p>Li, R., Fuchs, M., Perry, K.L., Mekuria, T., and Zhang, S. 2017. Development of a fast AmplifyRP Acceler8 diagnostic assay for grapevine red blotch-associated virus. Journal of Plant Pathology, in press.</p><br /> <p> </p><br /> <p>Martin, I., Vigne, E., Berthold, F., Komar, V., Lemaire, O., Fuchs, M. and Schmitt-Keichinger, C. 2017. The fifty distal amino acids of the 2AHP homing protein of grapevine fanleaf virus elicit a hypersensitive reaction on Nicotiana occidentalis. Molecular Plant Pathology, DOI: 10.1111/MPP.12558.</p><br /> <p> </p><br /> <p>Ricketts, K.D., Gómez, M.I., Fuchs, M.F., Martinson, T.E., Smith, R.J., Cooper, M.L., Moyer, M. and Wise A. 2017. Mitigating the economic impact of grapevine red blotch: Optimizing disease management strategies in U.S. vineyards. American Journal of Enology and Viticulture, 68:127-135.</p><br /> <p> </p><br /> <p>Rowhani, A., Daubert, S.D., Uyemoto, J.K., Al Rawhnih, M. and Fuchs, M. 2017. American nepoviruses. In: Grapevine Viruses: Molecular Biology, Diagnostics and Management. Meng, B., Martelli, G.P., Golino, D.A. and Fuchs, M.F (eds). Springer Verlag, pp. 109-126.</p><br /> <p> </p><br /> <p>Perry, K.L., McLane, H., Thompson, J.R. and Fuchs, M. 2017. A novel grablovirus from non-cultivated grapevine (<em>Vitis </em>sp.) in North America. Archives of Virology, in press.</p><br /> <p> </p><br /> <p>Perry, K.L., McLane, H., Hyder, M.Z., Dangl, G.S., Thompson, J.R. and Fuchs, M.F. 2016. Grapevine red blotch-associated virus is present in free-living Vitis sp. proximal to cultivated grapevines. Phytopathology, 106:663-670.</p><br /> <p> </p><br /> <p>Thompson, J.R., Dasgupta, I, Fuchs, M., Iwanami, T., Karasev, A.V., Petrzik, K., Sanfaçon, H., Tzanetakis, I., van der Vlugt, R., Wetzel, T. and Yoshikawa, N. 2017. ICTV virus taxonomy profile: Secoviridae. Journal of General Virology, 98:529-531.</p><br /> <p> </p><br /> <p>Vargas-Ascencio, J. Perry, K.L., Wise, A and Fuchs, M. 2017. Detection of <em>Australian grapevine viroid</em> in <em>Vitis vinifera</em> in New York. Plant Disease, http://DX.DOI.ORG/10.1094/PDIS-11-16-1587-PDN.</p><br /> <p> </p><br /> <p>Vargas, J., Al Rwahnih, M., Rowhani, A., Celebi-Toprak, F., Thompson, J.R., Fuchs, M. and Perry, K.L. 2016. Limited genetic variability of American isolates of <em>Grapevine virus E</em> in <em>Vitis</em> sp. Plant Disease, 100:159-163.</p><br /> <p> </p><br /> <p>Varsani, A., Roumagnac, P., Fuchs, M., Navas-Castillo, J., Moriones, E., Idris, I., Briddon, R.W. Rivera-Bustamante, R., Murilo Zerbini, F. and Martin, D.P. 2017. Capulavirus and Grablovirus: Two new genera in the family Geminiviridae. Archives of Virology, DOI 10.1007/s00705-017-3268-6.</p><br /> <p> </p><br /> <p><strong>Washington State University</strong></p><br /> <p>Donda, B. P., Jarugula, S., and Naidu, R. A. 2017. An analysis of the complete genome sequence and subgenomic mRNAs reveals unique features of the ampelovirus, <em>Grapevine leafroll-associated virus 1</em>. Phytopathology 107:1069-1079.</p><br /> <p> </p><br /> <p>Naidu, R.A. 2017. <em>Grapevine leafroll-associated virus 1.</em> In: Meng B., Martelli G., Golino, D., Fuchs M (eds.), Grapevine Viruses: Molecular Biology, Diagnostics and Management. Springer, Cham, Switzerland, pages 127-139.</p><br /> <p> </p><br /> <p>Burger, J., Maree, H.J., Gouveia, P., and Naidu, R.A. 2017. <em>Grapevine leafroll-associated virus 3</em>. In: Meng B., Martelli G., Golino, D., Fuchs M (eds.), Grapevine Viruses: Molecular Biology, Diagnostics and Management. Springer, Cham, Switzerland, pages 167-195.</p><br /> <p> </p><br /> <p>Hoheisel, G., Moyer, M.M., Daniels, C.H., Miller, T.W., Walsh, D., Zasada, I., Naidu, R. A., and Davenport, J.R. 2017. Pest Management Guide for Grapes in Washington. EB0762, 56 pp. (Revised).</p><br /> <p>Naidu, R.A. 2017. Grafting wine grapes. Good Fruit Grower June 2017. Vol. 68, No. 11, pages 38-39.</p><br /> <p><strong> </strong></p><br /> <p><strong>Clemson University</strong></p><br /> <p>A report of Cherry rusty mottle-associated virus in South Carolina. (2017) Poudel, B. & Scott, S.W. Australasian Plant Dis. Notes (2017) 12: 15. <a href="https://doi.org/10.1007/s13314-017-0239-4">https://doi.org/10.1007/s13314-017-0239-4</a></p><br /> <p> </p><br /> <p><strong>PA Department of Agriculture</strong></p><br /> <p>Nikolaeva, E. V., Welliver, R., Rosa, C., Jones, T., Peter, K., Costanzo, S., Davis, R. E. 2017. First Report of Apple (<em>Malus domestica</em>) as a Host of ‘<em>Candidatus Phytoplasma pruni</em>’ in United States. Plant Disease. 101. P. 378.</p><br /> <p> </p><br /> <p>Nikolaeva, E. V., Lesperance J., Peter, K., Jones, T., Costanzo, S., Davis, R. E. 2017. Phytoplasma survey in Pennsylvania. Phytopathology, S xx (Abstract of 2017 APS Meeting. San Antonio, TX).</p><br /> <p> </p><br /> <p>Huawei Liu, Liping Wu, Ekaterina Nikolaeva, Kari Peter, Zongrang Liu, Dimitre Mollov, Mengji Cao, Ruhui Li. Discovery and molecular characterization of a new luteovirus identified by high-throughput sequencing from apple (manuscript in preparation).</p>Impact Statements
- MPRU conducts testing for targeted pathogens and therapy for pathogen elimination; and maintains Rubus, Fragaria and Vaccinium G1 blocks in vitro, in the greenhouses and the screenhouse. Along with Oregon, MPRU is committed to expand current testing and therapy activities to meet the needs of the US berry industries. Tens of millions of strawberry plants are produced in NC, CA and Prince Edward Island nurseries from G1 stocks derived from MPRU and sold to US berry producers, annually. Nurseries have used blackberry, raspberry and blueberry G1 plants to produce, G2, G3 and G4 plants.
Date of Annual Report: 08/30/2018
Report Information
Period the Report Covers: 10/01/2017 - 09/30/2018
Participants
Al Rwahnih, Maher (malrwahnih@ucdavis.edu) University of California-Davis;Almeyda, Christie (cvalmeyd@ncsu.edu) North Carolina State;
Balci, Yilmaz (Yilmaz.Balci@aphis.usda.gov) USDA-APHIS;
Cooper, Cindy (ccooper@agr.wa.gov) Washington State Department of Agriculture;
Di Bello, Patrick (patrick.dibello@oregonstate.edu) Oregon State University;
Gratz, Allison (allison.gratz@inspection.gc.ca) Canadian Food Inpsection Agency – Sidney;
Guerra, Lauri (lguerra@agr.wa.gov) Washington State Department of Agriculture;
Guzman, Melinda (guzmanme@oregonstate.edu) Oregon State University;
Harper, Scott (scott.harper@wsu.edu) Washington State University-Prosser;
Hu, John (johnhu@hawaii.edu) University of Hawaii;
Hurtado-Gonzales, Oscar (Oscar.Hurtado-Gonzales@aphis.usda.gov) USDA-APHIS;
Jones, Robert P (Robert.P.Jones@aphis.usda.gov) USDA-APHIS;
Keller, Karen (karen.keller@ars.usda.gov) USDA-ARS;
Kinard, Gary (Gary.Kinard@ARS.USDA.GOV) USDA-ARS;
Lake, Amanda (amanda.lake@ars.usda.gov) USDA-ARS;
Lavagi, Irene (irenela@ucr.edu) University of California-Riverside;
Li, Ruhui (Ruhui.Li@ARS.USDA.GOV) USDA-ARS;
Lichens Park, Ann (apark@nifa.usda.gov) USDA-NIFA;
Lutes, Lauri Ann (Lauri.Lutes@oregonstate.edu) Oregon State University;
Martin, Bob (Bob.Martin@ars.usda.gov) USDA-ARS;
Mavrodieva, Vessela (Vessela.A.Mavrodieva@aphis.usda.gov) USDA-APHIS;
Nakhla, Mark (Mark.K.Nakhla@aphis.usda.gov) USDA-APHIS;
Olaya, Cristian, Washington State University;
Postman, Joseph (Joseph.Postman@ARS.USDA.GOV) USDA-ARS;
Poudyal, Dipak (dpoudyal@oda.state.or.us) Oregon Department of Agriculture;
Puckett, Josh (jmpuckett@ucdavis.edu) University of California-Davis;
Rabindran, Shailaja (Shailaja.Rabindran@aphis.usda.gov) USDA-APHIS;
Rayapati, Naidu (naidu.rayapati@wsu.edu) Washington State University;
Rosenbaum, Robin (rosenbaumr@michigan.gov) Michigan Department of Agriculture;
Roy, Avijit (avijit.roy@aphis.usda.gov) USDA-APHIS;
Rudyj, Erich (Erich.S.Rudyj@aphis.usda.gov) USDA-APHIS;
Salati, Raquel (raquelsalati@eurofinsUS.com) Eurofins BioDiagnostics;
Shiel, Pat (patrick.j.shiel@aphis.usda.gov) USDA-APHIS-PPQ;
Sudarshana, Mysore (Sudi) (mrsudarshana@ucdavis.edu) USDA-ARS;
Talton, Win (lwtalton@ncsu.edu) North Carolina State University;
Trujillo, Sarah (sarah.g.trujillo@aphis.usda.gov) USDA-APHIS;
Villamor, Dan Edward (dvvillam@uark.edu) University of Arkansas;
Wei, Alan (apwei@agri-analysis.com), Agri-Analysis;
Welliver, Ruth (rwelliver@pa.gov) Pennsylvania Department of Agriculture;
Zhang Shulu (shulu@agdia.com) AGDIA
Brief Summary of Minutes
Accomplishments
<div>Plant Health Program, Oregon Department of Agriculture administrates pathogen-tested certification programs for clonally propagated planting materials such as fruit trees and grapevines, performs statewide survey of state and federal level quarantine or regulated pathogens, and provides diagnostic service to nurseries and growers. In 2017-2018, following major activities related to certification programs were completed.</div><br /> <div><strong><span style="text-decoration: underline;">Major accomplishment:</span></strong></div><br /> <div><strong>Oregon Department of Agriculture Report</strong></div><br /> <div>Twenty-eight nurseries participated in Oregon's virus ornamental and fruit tree certification program in 2017. These nurseries grow registered plants (either G2 or G3 or both) of <em>Malus </em>(apples and crabapples), <em>Prunus </em>(fruiting and ornamental cherries, fruiting and ornamental plums, peaches, apricots, etc.), <em>Pyrus </em>(domestic pears, Asian pears, and flowering pears), and <em>Cydonia </em>(quince). Registered plants are inspected and tested regularly for viruses of economic importance.</div><br /> <div><em>Malus</em>, <em>Pyrus</em>, and <em>Cydonia </em>scions and rootstocks are tested for three latent viruses, Apple chlorotic leaf spot virus (ACLSV), Apple stem grooving virus (ASGV), and Apple stem pitting virus (ASPV). In fall of 2017, a total of 4685 samples from 25 nurseries were tested for these viruses. All the samples tested negative to ACLSV and ASGV but eight samples were found ASPV positive. Delimitation surveys were conducted in these positive sites and infected materials were eradicated.</div><br /> <div>Oregon has been a key stakeholder to implement a multiyear project to create a regional grapevine virus certification program by harmonizing quarantines and programs for grapevine nursery stock certification with neighboring states (Idaho and Washington) in the Pacific Northwest. Grapevine quarantine pests were compared and a common pest list was developed. The Idaho State Department of Agriculture (ISDA), Oregon Department of Agriculture (ODA), Washington State Department of Agriculture (WSDA), and Washington Wine Industry Foundation (WWIF) worked closely with other stakeholders in the grapevine and wine industries to develop harmonized rules for registration and certification of grapevine stock program for the Pacific Northwest.</div><br /> <div>A pilot study was performed based on these registration and certification standards to determine whether Oregon grapevine nurseries currently meet the standards developed for the PNW or not. Three grapevine nurseries participated in the pilot study in the fall of 2017. A detailed questionnaire inquiring about the source of mother plants (G2 and G3) and describing the requirements for registered blocks in fields, greenhouses/screenhouses, or containers, certified nursery stocks (G4), and best management practices was used. In general, the three nurseries met most of the requirements as outlined in the draft standards. Nurseries were also inspected for diseases and samples were collected for testing for indicator pathogens. A total of 59 leaf samples were collected from each nursery to test for <em>Grapevine red blotch virus</em>, <em>Grapevine leaf roll-associated virus 3</em>, and <em>Xylella fastidiosa</em>. All these samples tested negative to these pathogens.</div><br /> <div>Three caneberry nurseries participated in the pilot study to assess similarities and differences between their current practices and the standards based on the State Level Model Regulatory Standard (SLMRS) proposed by the National Clean Plant Network (NCPN). The three nurseries have different nursery stock production systems – Nursery 1 grows stock as plantlets, Nursery 2 grows stock in containers, and Nursery 3 has field-grown stock. Nurseries were assessed for source of mother stocks (G2 or G3), nursery locations for growing mother (G2 or G3) and nursery stocks (G4), and adherence to best management practices.</div><br /> <div>All three nurseries meet the SMLRS standards with a few exceptions. The three nurseries source their mother stocks (G2 or G3) from a Clean Plant Center to varying degrees. Nursery 1 obtains G1 materials from Clean Plant Center. Nursery 2 uses G2 materials and certified materials from other states as mother stocks. Nursery 3 uses plantlets obtained from G2 sources and field grown canes of unknown G level as mother stocks. All the nurseries satisfied requirements for location or sites for producing G2, G3, or G4 materials. Nurseries varied in their adherence to best management practices described by the SMLRS. Nursery 2 and 3 did not meet the requirement for isolation distance from uncertified <em>Rubus</em> spp., and primocane and flowering cultivars. Nursery 1 and 2 grew nursery stock in soil-less media. Nursery 3 produces field-grown stock; nematode testing was not performed prior to planting as required by the standards.</div><br /> <div>A total of 59 samples were collected and tested for five economically important canary viruses, <em>Black raspberry necrosis virus</em> (BRNV), <em>Raspberry bushy dwarf virus</em> (RBDV), <em>Raspberry leaf mottle virus</em> (RLMV), <em>Strawberry necrotic shock virus</em> (SNSV), and <em>Tomato ringspot virus</em> (ToRSV). RBDV, SNSV and ToRSV were tested by ELISA and BRNV and RLMV were tested using virus-specific reverse transcriptase PCR. All of the nursery samples tested negative for BRNV, SNSV, and ToRSV. Samples from Nursery 1 and 2 tested negative for RLMV and RBDV. Of the samples obtained from Nursery 3, 15% and 42% were positive for RLMV and RBDV, respectively. In addition, soil samples were collected from Nurseries 2 and 3 to test for the presence of nematodes. ToRSV<em>,</em> is vectored by <em>Xiphenema americanum. X. americanum </em>nor any other plant parasitic nematodes was found in samples from Nursery 2. In Nursery 3, <em>Xiphinema</em> spp. were not observed but some plant parasitic nematodes were found in low densities.</div><br /> <div><strong>Arkansas report</strong></div><br /> <div>In collaboration with colleagues, several of which participate in WERA-20 we are working on the characterization and population structure of several viruses in blueberry, currant and blackberry. This information is used in the development of detection protocols that have the ability to detect the vast majority of isolates that circulate in the United States</div><br /> <div>We have open channels of communication with both industry and regulators to optimize the guidelines so as to be implemented in the near future. We are holding meetings with state regulators to harmonize certification guidelines among states and commodities.</div><br /> <div>Validation of High Throughput Sequencing as a method for quarantine or certification testing – in collaboration with USDA-ARS Corvallis, OR. We have compared HTS as a detection method with a combination of bioassays, ELISA and PCR for 10 samples each of Strawberry, Blueberry and Rubus. In all cases with one exception, the HTS detected all viruses detected by conventional means. With HTS we also identified several new viruses in each of the crops. Two of the viruses in blueberry have been validated by PCR testing. This project will continue next year.</div><br /> <div><strong>Cornell Report</strong></div><br /> <div><span style="text-decoration: underline;">Grapevine red blotch virus. A</span>n economic study estimated the cost of GRBV to range from $2,210 to $68,550/ha when initial infected rates and quality penalties for suboptimal fruit composition are high. Based on these estimates, roguing symptomatic vines and replanting with clean vines derived from virus-tested stocks minimize losses if virus incidence is low to moderate (below 30%), while a full vineyard replacement should be pursued if disease incidence is higher, generally above 30%.</div><br /> <div>Surveys of GRBV and wild Vitis virus 1 (WVV1) in free-living <em>Vitis</em> species in northern California and New York from 2013 to 2017 showed the presence of these two grabloviruses in 28% (57 of 203) of samples from California but in none (0 of 163) from New York. The incidence of GRBV was significantly higher in free-living vines from California counties with high compared to low grape production, and in samples near (< 5km) to compared to far (> 5km) from vineyards. These results suggested a directional spread of GRBV inoculum predominantly from vineyards to free-living <em>Vitis</em> species. WVV1 incidence was also significantly higher in areas with higher grape production acreage. However, in contrast to GRBV, no differential distribution of WVV1 incidence was observed with regard to distance from vineyards. Two distinct phylogenetic clades were identified for both GRBV and WVV1 isolates from free-living <em>Vitis</em> species, although the nucleotide sequence variability of the genomic diversity fragment was higher for WWV1 than GRBV. Additionally, evidence for intra-specific recombination events was found in WVV1 isolates and confirmed in GRBV isolates. The prevalence of grabloviruses in California free-living vines highlights the need for vigilance regarding potential virus inoculum sources in order to protect new vineyard plantings and foundation stock vineyards in California.</div><br /> <div><strong>For GFLV</strong>, a system of binary plasmids based on the two genomic RNAs of GFLV strains F13 and GHu was designed and optimized parameters to maximize systemic infection frequency in <em>Nicotiana benthamiana</em> via agroinoculation were investigated. The genomic make-up of the inoculum, the identity of the co-infiltrated silencing suppressor, and temperature at which plants were maintained significantly increased systemic infection.</div><br /> <div>Using a reverse genetics approach, the RNA2-encoded protein 2A<sup>HP</sup> of GFLV strain F13, particularly to its 50 C-terminal amino acids, was shown to carry the determinant of necrotic lesions on inoculated leaves of <em>Nicotiana occidentalis</em> (Martin et al., 2018). The necrotic response showed hallmark characteristics of a genuine hypersensitive reaction, such as the accumulation of phytoalexins, reactive oxygen species, pathogenesis-related protein 1c and hypersensitivity-related (hsr) 203J transcripts. Transient expression of the GFLV-F13 protein 2A<sup>HP</sup> fused to an enhanced green fluorescent protein (EGFP) tag in <em>N. occidentalis</em> by agroinfiltration was sufficient to elicit a hypersensitive reaction. In addition, the GFLV-F13 avirulence factor, when introduced in GFLV-GHu, which causes a compatible reaction on <em>N. occidentalis</em>, elicited necrosis and partially restricted the virus.</div><br /> <div><strong> Collaborative research</strong></div><br /> <div>Research at Cornell was collaborative during this reporting period with efforts between (i) Cornell and Washington State University, and extension educators in New York and California, and (ii) Cornell and The Ohio State University, (iii) Cornell and UC-Davis, (iv) Cornell and industry researchers, and (v) Cornell and scientists in France. Research was also interdisciplinary with the involvement of virologists, entomologists, geneticists, viticulturists, quantitative epidemiologists, biologists and agriculture economist.</div><br /> <div><strong> Other accomplishments</strong></div><br /> <div>Efforts to reinstate a grape certification program in New York are under way. It is anticipated that the first NY certified material will be available in 2019 or 2020.</div><br /> <div><strong>APHIS-PPQ Report</strong></div><br /> <div>For us in the regulatory realm, we rely on high quality research to inform our decisions and serve our stakeholders. My contributions to the effort are in alignment of research efforts with regulatory programs to identify, characterize, and mitigate threats to U.S. agriculture and natural resources. Last year, we helped organize and deliver a workshop at the national American Phytopathological Society meeting on assay method validation and use. We have written a review paper submitted for publication in Plant Health Progress.</div><br /> <div>We feel that this paper will contribute to general understanding of method validation by the research community and will inform the current and future development of methods, especially High Throughput Sequencing (HTS). In addition to this, PPQ is also heavily involved in working with the international plant testing community to learn and harmonize assays for smooth and transparent international trade. Over the last year, we have invited researchers like Mahar Al Rwahnih and Mike Rott to update us on the latest technologies. We of course maintain contact with other researchers like you to help provide subject matter expertise on specific pathogens or situations.</div><br /> <ul><br /> <li>Impact: The impact of PPQ on WERA-20 is significant. Many of the new organisms characterized by the WERA-20 researchers are of US regulatory concern. We appreciate the openness and cooperation in working with us to balance the needs of furthering the scientific knowledge of these organisms with our obligations to protect US agriculture and natural resources from the threats of outbreak and possible damage of these organisms</li><br /> <li>Collaboration: PPQ is also heavily involved in working with the international plant testing community to learn and harmonize assays for smooth and transparent international trade. We have invited researchers like Mahar Al Rwahnih and Mike Rott to update us on the latest technologies. We also fund WERA-20 projects that are related to PPQ’s mission and collaborate with researchers on projects that impact our mission.</li><br /> <li>Other accomplishments: We seek to further our interactions with WERA-20 research community in the areas of assay harmonization and inter-laboratory coordination of testing to demonstrate direct comparability and trend tracking of results for overall program improvement. We have developed tools for plant diagnosticians to further these efforts and we look forward to working with the WERA-20 to showcase these tools and explore their applicability to diagnostic testing done by the WERA-20.<strong> <br /></strong></li><br /> </ul><br /> <div> </div><br /> <div><strong>Washington State University Report</strong></div><br /> <div>Virus diseases affecting fruit quality and vine health are one the highest research priorities for the grape and wine industry in Washington State. In response, the grape virology program (<a href="http://wine.wsu.edu/virology/">http://wine.wsu.edu/virology/</a> ) is conducting fundamental and applied research in a holistic manner to generate science-based knowledge for mitigating negative impacts of virus diseases in vineyards. Vineyard surveys have consistently shown that <em>Grapevine leafroll-associated virus 3</em> (GLRaV-3) is more common across the state vineyards than <em>Grapevine red blotch virus</em> (GRBV). Thus, GLRaV-3 is considered as a significant constraint for advancing vineyard health and productivity. Due to similar symptoms produced by leafroll and red blotch diseases in red-fruited wine grape cultivars, molecular diagnostic assays are essential for reliable identification of GLRaV-3 and GRBV in vineyards. Studies in commercial vineyards have shown that both GLRaV-3 and GRBV can cause significant impacts on fruit yield and grape quality in several red-fruited wine grape cultivars. Of the two nepoviruses (<em>Tobacco ring spot virus</em> [TRSV] and <em>Grapevine fanleaf virus</em> [GFLV]) causing fanleaf degeneration and decline symptoms, TRSV was found in one commercial vineyard, whereas GFLV was detected in two commercial vineyards located in distinct geographic locations. Studies have shown that TRSV can be spread by the dagger nematode, <em>Xiphinema rivesi</em>. Conversely, the dagger nematode species currently present in Washington soils (except <em>X. index</em>, the known vector of GFLV) are unlikely to spread GFLV. Thus, a combination of planting virus-tested cuttings and implementing nematode control are necessary for the management of TRSV. In contrast, roguing infected vines and replanting with virus-tested cuttings would help preventing the spread of GFLV in vineyards. Virus indexing of grapevines in Certified Mother blocks in registered nurseries revealed the absence of GRBV and very low incidence of GLRaV-3. Thus, registered nurseries in Washington State appears to be free from red blotch virus. The research-based knowledge was shared with grape and wine industry stakeholders and regulatory agencies via presentations at grower meetings and workshops for broader dissemination of research outcomes.</div><br /> <div>Foundation Plant Services (UC-Davis) report</div><br /> <div>At Foundation Plant Services (FPS), we continue to make advances in developing and refining our methods using high throughput sequencing (HTS) as a superior diagnostic tool. We have used sequence information generated by HTS analysis to design new, specific PCR primers for use in PCR diagnostics. In addition, HTS proves to be an invaluable tool in the discovery of unknown viruses and in establishing a baseline analysis of the virome of a crop.</div><br /> <div>Progress has also been made in improving the efficiency of diagnostic laboratory assays for Grapevine leafroll-associated virus 3 (GLRaV-3). GLRaV-3 has an exceptional number of highly diverse variants. Recent studies based on genome-wide phylogenetic analysis demonstrated that GLRaV-3 variants can be divided into eight distinct groups, six of which have been identified in California. This level of genetic diversity makes it almost impossible to identify a conserved region common to all isolates for design of a single qPCR-based assay. Up to now, FPS has dealt with this problem by designing variant-specific assays, six to date. However, it isn’t feasible to test large numbers of vines using six different assays. FPS embarked on a study to survey and analyze GLRaV-3 genetic variants and work towards improving the RT-qPCR assay design. FPS reconstructed the near complete genomes of 34 GLRaV-3 isolates, including three new divergent variants using HTS and incorporated new genetic data into a more complete characterization of genetic variation across GLRaV-3 variants. A small but highly conserved region was identified that was then used to construct a single new RT-qPCR assay, referred to as FPST, for detecting all GLRaV-3 variants characterized to date. When compared with previous GLRaV-3 assays, FPST was the one RT-qPCR assay that detected ALL variants obtained to date. The assay was further validated with 2452 samples obtained from USDA National Clonal Germplasm Repository in Winters, CA, the Davis Virus Collection at UC-Davis, the FPS pipeline of foreign and domestic introductions, selected vineyards in the main grape-growing areas of California, and samples provided by international collaborators. Further verification of the FPST assay was obtained by testing the above population with a new GLRaV-3 Enzyme-linked immunosorbent assay (ELISA) kit. This new ELISA kit has detected all known GLRaV-3 variants characterized to date and the side-by-side comparison indicated that all samples testing positive by the FPST RT-qPCR assay also tested positive by the new ELISA kit. Finally, the new assay is being shared with stakeholders, thus benefiting growers, researchers, and diagnostic labs involved in the grapevine industry in the US and around the world.</div><br /> <div>New viral discoveries were made in pistachio trees. Pistachio trees from the National Clonal Germplasm Repository (NCGR) and selected orchards in California were surveyed for viruses and virus-like agents by HTS. Analyses of 60 trees, <em>Pistacia vera </em>and clonal UCB-1 hybrid rootstock (<em>P. atlantica </em>× <em>P. integerrima</em>), identified a novel virus found in the NCGR that showed low amino acid sequence homology (~42%) to members of genus Ampelovirus, family Closteroviridae and was provisionally named “Pistachio Ampelovirus A” (PAVA). A putative viroid, provisionally named “Citrus bark cracking viroid-pistachio” (CBCVd-pis), was also found in the NCGR and showed approximately 87% similarity to Citrus bark cracking viroid (CBCVd, genus Cocadviroid, family Pospiviroidae). Both pathogens were graft transmissible to healthy UCB-1 plants. A field survey of 123 trees from commercial orchards found no incidence of PAVA, but five (4%) samples were infected with CBCVd-pis. Of 675 NCGR trees, 16 (2.3%) were positive for PAVA and 172 (25.4%) were positive for CBCVd-pis by reverse-transcription polymerase chain reaction.</div><br /> <div>A novel RNA virus was detected in grapevine cultivar ‘Kizil Sapak’ by HTS and tentatively named “grapevine virus J” (GVJ). The full genome of GVJ is 7,390 nucleotides in length, which comprises five open reading frames (ORFs), including a 20K ORF (ORF 2) between the replicase (ORF 1) and the movement protein (ORF 3) genes. According to the level of sequence homology and phylogenetics, GVJ is proposed as a new member of the genus Vitivirus (subfamily Trivirinae; family Betaflexiviridae), with the closest characterized virus being grapevine virus D (GVD). Further work is needed to identify the mechanisms by which GVJ is transmitted. Field surveys and biological studies are underway to determine the prevalence of GVJ in commercial vineyards and to assess its effect on vine performances. In addition, the incidence of GVJ in other selections introduced to FPS and the USDA National Clonal Germplasm Repository in Winters, CA, will be determined using the newly developed RT-PCR assay.</div><br /> <div>A geminivirus was identified for the first time in Prunus spp. This novel virus, provisionally named Prunus geminivirus A (PrGVA), was identified by Illumina sequencing from an asymptomatic plum tree. PrGVA was subsequently confirmed by rolling cycle amplification, cloning, and Sanger sequencing of its complete genome (3,174 to 3,176 nucleotides) from an additional 18 (9 apricot and 9 plum) field isolates. Apart from the nonanucleotide motif TAATATT↓AC present in its virion strand origin of replication, other conserved motifs of PrGVA support its geminiviral origin. PrGVA shared highest complete genome (73 to 74%), coat protein amino acid (83 to 85%) and rep-associated amino acid (74%) identities with Grapevine red blotch virus (GRBV). PrGVA was graft but not mechanically transmissible. Quantitative polymerase chain reaction screening of Prunus spp. in the National Clonal Germplasm Repository collection using newly designed primers and probes revealed 69.4% (apricot), 55.8% (plum), and 8.3% (cherry) incidences of PrGVA. PrGVA is proposed as a novel member of the genus Grablovirus based on its close genome and phylogenetic relationship with GRBV.</div><br /> <div>Rose rosette disease (RRD) of roses is widely distributed in the eastern U.S. RRD is caused by Rose rosette virus (RRV), which is vectored by the eriophyid mite (<em>Phyllocoptes fructiphilus</em> Keifer). California is one of the main producers of roses in the U.S. with a wholesale value of US$ 17.6 million in 2016. In August 2017, symptoms suggestive of RRD were observed on two roses (cvs. Veterans’ Honor and Brilliant Pink Iceberg) in a commercial nursery in Wasco, CA. A total of 415 samples, representing many rose cultivars were collected from the nursery and tested for RRV by RT-qPCR assay. Only the two symptomatic plants were positive for RRV. Plants were also microscopically examined and the <em>P. fructiphilus</em> mite was observed and recovered from several plants throughout the nursery. In June 2018, a separate incident occurred where three garden roses (unknown cultivars) planted in two neighboring homes in Bakersfield, CA, about 50 km from the 2017 finds, were identified with RRD symptoms. Leaves from all three plants tested positive for RRV by RT-qPCR and were also infested with <em>P. fructiphilus</em> mites. To confirm these results HTS was performed on all five symptomatic plants and analysis revealed that the RRV genomes from the two nursery plants sampled in 2017 were 100% identical to one another. The RRV genomes from the three homeowner plants sampled in 2018 were 100% identical to each other, but not to the nursery samples. These results suggest that the samples from these two locations are likely two separate introductions of RRV. To our knowledge, this is the first detection of RRV causing RRD in CA.</div><br /> <div>Little cherry disease (LCD), associated with Little cherry virus-1 (LChV-1) or -2 (LChV-2), is a common problem of cherries which occurs worldwide, causes unmarketable fruit and often results in tree or orchard removal. Most of the new cherry rootstocks used in cherry production are interspecific Prunus hybrids which introduces an increased risk of an adverse reaction (hypersensitivity) to some viruses. Hypersensitive reactions exhibit graft union gum exudation, premature abscission, and tree death within one or two growing seasons and have been shown to occur in Prunus when infected with Prunus necrotic ringspot virus (PNRSV) and Prune dwarf virus (PDV). FPS has begun evaluating the effects of LChV-1 and LChV-2 on 16 different popular Prunus rootstocks. All rootstocks will be grafted with a scion variety from the same accession. Observations of budtake and tree performance will be recorded and evaluated for two years. Rootstocks will be rated for sensitivity to LChV-1 and LChV-2 and this information will be shared with growers and nurseries to assist in making rootstock selection decisions.</div><br /> <div>An interactive relationship between vitiviruses and grapevine leafroll viruses was characterized in grapevine. Grapevine viruses A and B (GVA and GVB) were found more frequently in the presence of co-infecting Grapevine leafroll associated viruses (GLRaV-1, −2 or −3) than in their absence. The titers of the vitiviruses in co-infection with leafroll viruses were found to be higher than were their titers in the absence of leafroll virus infection. The occurrence of vitivirus-associated stem-pitting symptoms was correlated with leafroll virus co-infection. Specific pairing associations on the species level were found between different viti- and leafroll virus species: GVB was associated preferentially with GLRaV-2; GVA was associated preferentially with GLRaV-1 and GLRaV-3. In contrast to the increase in vitivirus titer seen with leafroll virus co-infection, the incidence and titer of grapevine leafroll virus appeared to be unaltered by vitivirus co-infection. </div><br /> <div>FPS has also worked collaboratively with James Schoelz at the University of Missouri to conduct a state-wide survey of about 400 grapevines samples for common grapevine viruses. Several viruses were detected, including Grapevine red blotch associated virus. The results of this study will help with certification and to establish a clean plant program.</div><br /> <div>While HTS remains a powerful new technology with significant benefits, there are technical challenges associated with the technology that warrants the establishment of guidelines for its use in plant certification and quarantine programs. We have begun efforts in a collaborative project to coordinate the development of minimum basic requirements for the adoption of HTS technologies, including nucleic acid extractions, library preparation, depth of sequencing and bioinformatics, for the detection of viral pathogens.</div><br /> <div><strong>USDA-ARS Corvallis Report</strong></div><br /> <div>Developing reliable testing virus protocols is critical for certification, quarantine and studies on virus epidemiology. We have coordinated a ring test among 6-9 laboratories (depending on crop) for blueberries, strawberries and caneberries. We collected 10-12 samples from each crop, most with known virus infections and several healthy plants for each crop. The tissues were powdered in liquid nitrogen, aliquoted and shipped on dry ice to the various laboratories. Each lab was sent a list of primer pairs to use for the RT-PCR testing along with parameters for running the PCRs, annealing temps, number of cycles, amplicon size. This was completely blind, some of the primers pairs should have been negative in all tests, while others should have given positive results in multiple samples. Some samples had mixed infections, some single infections. Each lab was asked to run the tests, and send back the result. The testing was consistent across most laboratories, but there did appear to be some contamination issues. We are repeating these tests again this year, and will use screw on vials for the samples rather than Eppendorf tubes with snap lids since opening these could cause a release of some of the fine powdered tissue. We also did bioassays on the samples in our lab.</div><br /> <div>We are continuing our work on Raspberry leaf curl disease and now have five viruses from native Rubus species in the northeastern U.S. and eastern Canada where the disease was common in the first half of the 20<sup>th</sup> century. We are propagating a cultivar of red and black raspberry and a blackberry that were widely grown in that region at the time the disease was prevalent. We obtained from the National Clonal Germplasm Repository and in the process of inoculating these plants with various combinations of the viruses detected in native and commercial raspberries in the northeast.</div><br /> <div>We are also working with the harmonization of certification standards for the three major berry crops and grapevines. In addition, we are taking the lead on developing a virus database for the viruses of crops in the National Clean Plant Network. This project is in cooperation with WERA-20 members that are directors of NCPN centers for the various crops (Berries, Citrus, Grapevines, Hops, Roses, Sweetpotatoes and Tree Fruit). The structure of the database is being developed in collaboration with the Center for Applied Software and Systems at Oregon State University.<strong> <br /></strong></div><br /> <div><strong>AGDIA Report</strong></div><br /> <div>Early detection and effective control of plant pathogens is very important to prevent their wide spread resulting in serious economic losses in crops like cherries, citruses, grapes, hops, peaches or plums. Agdia's AmplifyRP<sup>®</sup> utilizes a leading isothermal amplification technology called recombinase polymerase amplification (RPA) and has recently become a versatile diagnostic tool for rapid and accurate detection of nucleic acids in all types of plant pathogens. So far, Agdia has commercialized 13 pathogen-specific AmplifyRP<sup>®</sup> kits in one of the three formats (Acceler8<sup>®</sup>, XRT or XRT<sup>+</sup>) currently available. Among them, 5 AmplifyRP<sup>®</sup> kits including AmplifyRP<sup>®</sup> XRT<sup>+</sup> for <em>Xylella fastidiosa</em> (Xf) are newly released since 2017 WERA-20 meeting. AmplifyRP<sup>®</sup> XRT<sup>+</sup> for <em>Hop stunt viroid</em> (HSVd) has also been developed. In addition to the 13 AmplifyRP<sup>®</sup> kits, Agdia offers two AmplifyRP<sup>®</sup> Discovery kits that can be used for any pathogens. Agdia has recently acquired the intellectual properties right for AmpliFire and started to offer this portable, battery-operable fluorescence reader. Overall, AmplifyRP<sup>®</sup> is simple, as sensitive as PCR or qPCR, and field-deployable. No thermal cycler and DNA/RNA purification are needed as all reactions works well with plant crude extracts at an isothermal temperature 39°C.</div>Publications
<div><strong>WERA-20 Publications 2017/2018</strong></div><br /> <div>Adiputra, J., Kesoju, S.R., Naidu, R.A. 2018. The relative occurrence of <em>Grapevine leafroll</em>-<em>associated virus 3 </em>and <em>Grapevine red blotch virus </em>in Washington State vineyards. Plant Disease (in press).</div><br /> <div>Al Rwahnih, M., Alabi, O. J., Westrick, N. M., and Golino, D. 2018. Prunus geminivirus A: A Novel Grablovirus Infecting Prunus spp. Plant Disease. 102:1246–1253.</div><br /> <div>Al Rwahnih, M., Rowhani, A., Westrick, N., Stevens, K., Diaz-Lara, A., Trouillas, F. P., et al. 2018. Discovery of Viruses and Virus-Like Pathogens in Pistachio using High-Throughput Sequencing. Plant Disease. 102:1419–1425.</div><br /> <div>Cieniewicz, E., Pethybridge S., Gorny, A., Madden, L., Perry, K.L., McLane, H. and Fuchs, M. 2017a. Spatiotemporal spread of grapevine red blotch-associated virus in a California vineyard. Virus Research 230:59-62.</div><br /> <div>Cieniewicz, E., Pethybridge S.J., Loeb, G.M., Perry, K.L. and Fuchs, M. 2018b. Insights into the ecology of grapevine red blotch virus in a diseased vineyard. Phytopathology 108:94-102.</div><br /> <div>Cieniewicz, E., Thompson, J.R., McLane, H., Perry, K.L., Dangl, G.S., Corbett, Q., Martinson, T., Wise, A., Wallis, A., O’Connell, J., Dunst, R., Cox, K. and Fuchs, M. 2018a. Prevalence and diversity of grabloviruses in free-living <em>Vitis</em> spp. Plant Disease, https://apsjournals.apsnet.org/doi/pdf/10.1094/pdis-03-18-0496-re.</div><br /> <div>Coletta-Filho, H.D., Francisco, C.S., Lopes, J.R.S., Muller, C. and Almeida, R.P.P. 2017. Homologous recombination and Xylella fastidiosa host-pathogen associations in South America. Phytopathology 107: 305-312.</div><br /> <div>Cornara, D., Cavalieri, V., Dongiovanni, C., Altamura, G., Palmisano, F., Bosco, D., Porcelli, F., Almeida, R.P.P. and Saponari, M. 2017. Transmission of Xylella fastidiosa by naturally infected Philaenus spumarius (Hemiptera, Aphrophoridae) to different host plants. Journal of Applied Entomology 141: 80-87.</div><br /> <div>Cornara, D., Saponari, M., Zeilinger, A.R., de Stradis, A., Boscia, D., Loconsole, G., Bosco, D., Martelli, G.P., Almeida, R.P.P. and Porcelli, F. 2017. Spittlebugs as vectors of Xylella fastidiosa in olive orchards in Italy. Journal of Pest Science 90: 521-530.</div><br /> <div>Daugherty, M.P., Zeilinger, A.R. and Almeida, R.P.P. 2017. Conflicting effects of climate and vector behavior on the spread of a plant pathogen. Phytobiomes 1: 46-53.</div><br /> <div>Di Bello, P.L., Laney, A.G., Druciarek, T., Ho, T., Gergerich, R.C., Keller, K.E., Martin, R.R. and Tzanetakis, I.E. 2016. A novel <em>Emaravirus</em> is associated with redbud yellow ringspot disease. Virus Res. 222:41-47.</div><br /> <div>Diaz-Lara, A., Golino, D., and Al Rwahnih, M. 2018. Genomic characterization of grapevine virus J, a novel virus identified in grapevine. Archives of Virology. 163:1965–1967.</div><br /> <div>Finn, C.E., Strik, B.C., Yorgey, B.M., Peterson, M.E., Jones, P.A., Lee, J. and Martin, R.R. 2018. ‘Columbia Giant’ thornless trailing blackberry. HortScience 53:251-255.</div><br /> <div>Finn, C.E., Strik, B.C., Yorgey, B.M., Peterson, M.E., Jones, P.A., Lee, J. and Martin, R.R. 2018. ‘Columbia Sunrise’ thornless trailing blackberry. HortScience 53:256-260.</div><br /> <div>Francisco, C.S., Ceresini, P.C., Almeida, R.P.P. and Coletta-Filho, H.D. 2017. Spatial genetic structure of coffee-associated Xylella fastidiosa populations indicates that cross-infection does not occur with sympatric citrus orchards. Phytopathology 107: 395-402.</div><br /> <div>Giampetruzzi, A., Saponari, M., Almeida, R.P.P., Essakhi, S., Boscia, D., Loconsole, G. and Saldarelli, P. 2017. Complete genome sequence of the olive-infecting strain Xylella fastidiosa subsp. pauca De Donno. Genome Announcements 5: e00569-17.</div><br /> <div>Giampetruzzi, A., Saponari, M., Loconsole, G., Boscia, D., Savino, V.N., Almeida, R.P.P., Zicca, S., Landa, B.B., Chacón-Diaz, C. and Saldarelli, P. 2017. Genome-wide analysis provides evidence on the genetic relatedness of the emergent Xylella fastidiosa genotype in Italy to isolates from Central America. Phytopathology 107: 816-827.</div><br /> <div>Hassan, M., Di Bello, P.L., Keller, K.E., Martin, R.R., Sabanadzovic, S. and Tzanetakis, I.E. 2017. A new, widespread emaravirus discovered in blackberry. Virus Research 235:1-5.</div><br /> <div>Herrbach, E., Alliaume, A., Prator, C.A., Daane, K.M., Cooper, M.L. and Almeida, R.P.P. 2017. Vector transmission of grapevine-leafroll associated viruses. In: B. Meng et al. (eds.), Grapevine Viruses: Molecular Biology, Diagnostics and Management. DOI 10.1007/978-3-319-57706-7_24.</div><br /> <div>Ho, T., Harris, A., Katsiani, A., Khadgi, A., Schilder, A. and Tzanetakis, I.E. 2018. Genome sequence and detection of Peach rosette mosaic virus. <em>Journal of Virological Methods</em> 254: 8-12.</div><br /> <div>Jimenez, J., Webster, C.G., Moreno, A., Almeida, R.P.P., Blanc, S., Fereres, A. and Uzest, M. 2017. Fasting alters aphid probing behaviour but does not universally increase the transmission rate of non-circulative viruses. Journal of General Virology 98: 3111-3121.</div><br /> <div>Kandel, P.P., Almeida, R.P.P., Cobine, P.A. and De La Fuente, L. 2017. Natural competence rates are variable among Xylella fastidiosa strains and homologous recombination occurs in vitro between subspecies fastidiosa and multiplex. Molecular Plant-Microbe Interactions 30: 589-600.</div><br /> <div>Koloniuk, I., Thekke-Veetil, T., Reynard, J.S., Mavrič, I.P., Přibylová, J., Brodard, J., Kellenberger, I., Sarkisova, T., Špak, J., Lamovšek, J. and Massart, S., 2018. Molecular Characterization of Divergent Closterovirus Isolates Infecting Ribes Species. <em>Viruses</em> 10: 369</div><br /> <div>Labroussaa, F., Ionescu, M., Zeilinger, A.R., Lindow, S.E. and Almeida, R.P.P. 2017. A chitinase is required for Xylella fastidiosa colonization of its insect and plant hosts. Microbiology 163: 502-509.</div><br /> <div>Li, R., Fuchs, M., Perry, K.L., Mekuria, T. and Zhang, S. 2017. Development of a fast AmplifyRP Acceler8 diagnostic assay for grapevine red blotch-associated virus. Journal of Plant Pathology 99:657-662.</div><br /> <div>Lutes, L. A., and Pscheidt, J. W. 2018. First Report of Cherry leaf roll virus on Sweet Cherry in Oregon. Plant Disease. 102:691–691.</div><br /> <div>Maree, H. J., Fox, A., Al Rwahnih, M., Boonham, N., and Candresse, T. 2018. Application of HTS for routine plant virus diagnostics: State of the art and challenges. Front. Plant Sci. 9 Available at: https://www.frontiersin.org/articles/10.3389/fpls.2018.01082/full [Accessed July 12, 2018].</div><br /> <div>Martin, I., Vigne, E., Berthold, F., Komar, V., Lemaire, O., Fuchs, M. and Schmitt-Keichinger, C. 2018. The fifty distal amino acids of the 2A<sup>HP</sup> homing protein of grapevine fanleaf virus elicit a hypersensitive reaction on <em>Nicotiana occidentalis</em>. Molecular Plant Pathology 19:731-743.</div><br /> <div>Martin, R.R. and Tzanetakis, I.E. 2018. High risk blueberry viruses by region in North America; Implications for certification, nurseries, and fruit production. Viruses 10:342 ; doi:10.3390/v10070342 </div><br /> <div>Osterbaan, L., Schmitt-Keichinger, C. Vigne, E. and Fuchs, M. 2018. Optimal systemic grapevine fanleaf virus infection in <em>Nicotiana benthamiana </em>following agroinoculation. Journal of Virological Methods 257:16-21.</div><br /> <div>Pandey, B., Naidu, R.A. and Grove, G.G. 2018. Detection and analysis of mycovirus‑related RNA viruses from grape powdery mildew fungus <em>Erysiphe necator</em>. Archives of Virology 163: 1019-1030.</div><br /> <div>Perry, K.L., McLane, H., Thompson, J.R. and Fuchs, M. 2018. A novel grablovirus from non-cultivated grapevine (<em>Vitis</em> sp.) in North America. Archives of Virology 163:259-262.</div><br /> <div>Prator, C.A., Kashiwagi, C.M., Voncina, D. and Almeida, R.P.P. 2017. Infection and colonization of Nicotiana benthamiana by Grapevine leafroll-associated virus 3. Virology 510: 60-66.</div><br /> <div>Ricketts, K.D., Gómez, M.I., Fuchs, M.F., Martinson, T.E., Smith, R.J., Cooper, M.L., Moyer, M. and Wise A. 2017. Mitigating the economic impact of grapevine red blotch: Optimizing disease management strategies in U.S. vineyards. American Journal of Enology and Viticulture 68:127-135.</div><br /> <div>Rowhani, A., Daubert, S., Arnold, K., Al Rwahnih, M., Klaassen, V., Golino, D. and Uyemoto, J.K., 2018. Synergy between grapevine vitiviruses and grapevine leafroll viruses. European Journal of Plant Pathology, pp.1-7.</div><br /> <div>Setiono, F.J., Chatterjee, D., Fuchs, M., Perry, K.L. and Thompson, J.R. 2018. The distribution and ability to detect grapevine red blotch virus in its host depends on time of sampling and tissue type. Plant Disease, https://apsjournals.apsnet.org/doi/pdf/10.1094/pdis-03-18-0450-re.</div><br /> <div>Thekke-Veetil, T. and Tzanetakis, I.E. 2017. Development of reliable detection assays for blueberry mosaic- and blackberry vein banding- associated viruses based on their population structures. <em>Journal of Virological Methods </em>248: 191-194.</div><br /> <div>Thekke-Veetil, T., Ho, T., Postman, J.D., and Tzanetakis, I.E. 2017. Characterization and detection of a novel idaeovirus infecting black currant. <em>European Journal of Plant Pathology</em> 149: 751-757.</div><br /> <div>Tzanetakis, I.E. and Martin, R.R. 2017. A systems-based approach to manage strawberry virus diseases. Canadian Journal Plant Pathology 39:5-10.</div><br /> <div>Vargas-Ascencio, J. Perry, K.L., Wise, A. and Fuchs, M. 2017. Detection of <em>Australian grapevine viroid</em> in <em>Vitis vinifera </em>in New York. Plant Disease 101:848.</div><br /> <div>Voncina, D., Rwahnih, M.A., Rowhani, A., Gouran, M. and Almeida, R.P.P. 2017. Viral diversity in autochthonous Croatian grapevine cultivars. Plant Disease 101: 1230-1235.</div><br /> <div>Weiland, J.E., Benedict, C., Zasada, I.A., Scagel, C.R., Beck, B.R., Davis, A., Graham, K., Peetz, A., Martin, R.R., Dung, J.K.S., Gaige, A.R. and Thiessen, L. 201X. Late summer disease symptoms in western Washington red raspberry fields associated with co-occurrence of <em>Phytophthora rubi, Verticillium dahliae</em>, and <em>Pratylenchus penetrans</em>, but not <em>Raspberry bushy dwarf virus</em>. Plant Disease 102:938-947.</div><br /> <div>Wistrom, C.M., Blaisdell, G.K., Wunderlich, L.R., Botton, M., Almeida, R.P.P. and Daane, K.M. 2017. No evidence of transmission of grapevine leafroll-associated viruses by phylloxera (Daktulosphaira vitifoliae). European Journal of Plant Pathology, 147: 937-941.</div><br /> <div>Yepes, L.M. Cieniewicz, E., Krenz, B., McLane, H., Thompson, J.R., Perry, K.L. and Fuchs, M. 2018. Causative role of grapevine red blotch virus in red blotch disease. Phytopathology 108:902-909.</div><br /> <div>Zeilinger, A.R., Rapacciuolo, G., Turek, D., Oboyski, P.T., Almeida, R.P.P. and Roderick, G.K. 2017. Museum specimen data reveal emergence of a plant disease may be linked to increases in vector population. Ecological Applications 27: 1827-1837.</div><br /> <div>Zongoma, A.M., Dangora, D.B., Al Rwahnih, M., Bako, S.P., Alegbejo, M.D. and Alabi, O.J., 2018. First Report of Grapevine yellow speckle viroid 1, Grapevine yellow speckle viroid 2, and Hop stunt viroid Infecting Grapevines (Vitis spp.) in Nigeria. Plant Disease, 102(1), pp.259-259.</div><br /> <div>Zongoma, A.M., Dangora, D.B., Al Rwahnih, M., Bako, S.P., Alegbejo, M.D. and Alabi, O.J., 2018. First Report of Grapevine leafroll-associated virus 1 Infecting Grapevines (Vitis spp.) in Nigeria. Plant Disease, 102(1), pp.258-258.</div><br /> <div><strong>Book Chapters:</strong></div><br /> <div>Burger, J., Maree, H.J., Gouveia, P., and Naidu, R.A. 2017. <em>Grapevine leafroll-associated virus 3</em>. In: Meng B., Martelli G., Golino, D., Fuchs M (eds.), Grapevine Viruses: Molecular Biology, Diagnostics and Management. Springer, Cham, Switzerland, pages 167-195. (Book Chapter)</div><br /> <div>Cieniewicz, E.J., Perry, K.L. and Fuchs, M. 2017b. Grapevine red blotch virus: Molecular biology of the virus and management of the disease. In: Grapevine Viruses: Molecular Biology, Diagnostics and Management. Meng, B., Martelli, G.P., Golino, D.A. and Fuchs, M.F. (eds). Springer Verlag, pp. 303-314.</div><br /> <div>Fuchs, M. and Lemaire, O. 2017. Novel approaches for virus disease management. In: Grapevine Viruses: Molecular Biology, Diagnostics and Management. Meng, B., Martelli, G.P., Golino, D.A. and Fuchs, M.F (eds). Springer Verlag, pp. 599-621.</div><br /> <div>Fuchs, M. Schmitt-Keichinger, C. and Sanfaçon, H. 2017. A renaissance in nepovirus research provides new insights into their molecular interface with hosts and vectors. In: Advances in Virus Research, M. Kielian, K. Maramorosch, T.C Mettenleiter and M.J Roosinck (eds.), pp. 61-105.</div><br /> <div>Golino, D., Fuchs, M., Al Rwanih, M., Farrar, K., and Martelli, G.P. 2017b. Regulatory aspects of grape virology: Certification, quarantine and harmonization. In: Grapevine Viruses: Molecular Biology, Diagnostics and Management. Meng, B., Martelli, G.P., Golino, D.A. and Fuchs, M.F. (eds). Springer Verlag, pp. 581-598.</div><br /> <div>Golino, D., Fuchs, M., Sim, S., Farrar, K. and Martelli, G. 2017a. Improvement of grapevine planting stock through sanitary selection and pathogen elimination. In: grapevine viruses: molecular biology, diagnostics and management. Meng, B., Martelli, G.P., Golino, D.A. and Fuchs, M.F. (eds). Springer Verlag, pp. 561-579.</div><br /> <div>Herrbach, E., Alliaume, A., Prator, C.A., Daane, K.M., Cooper, M.L. and Almeida, R.P.P. 2017. Vector transmission of grapevine-leafroll associated viruses. In: B. Meng et al. (eds.), Grapevine Viruses: Molecular Biology, Diagnostics and Management. DOI 10.1007/978-3-319-57706-7_24.</div><br /> <div>Naidu, R.A. 2017. <em>Grapevine leafroll-associated virus 1</em>. In: Meng B., Martelli G., Golino, D., Fuchs M (eds.), Grapevine Viruses: Molecular Biology, Diagnostics and Management. Springer, Cham, Switzerland, pages 127-139. (Book chapter)</div><br /> <div>Rowhani, A., Daubert, S.D., Uyemoto, J.K., Al Rawhnih, M. and Fuchs, M. 2017. American nepoviruses. In: Grapevine Viruses: Molecular Biology, Diagnostics and Management. Meng, B., Martelli, G.P., Golino, D.A. and Fuchs, M.F (eds). Springer Verlag, pp. 109-126.</div><br /> <div>Schilder, A., Hall, H.K., Tzanetakis, I.E. and Funt, R.C. 2017. Diseases, viruses, insects, and weeds of blackberries and their hybrids. Pp.202-244. In: Funt, R.C. and Hall H.K. (Ed) <em>Blackberries and their hybrids. </em>Wallingford, UK: CAB International</div><br /> <div>Tzanetakis, I.E. and Martin R.R. 2017. Production of high health plants for nuclear stock. Pp. 93-100. In: Funt, R.C. and Hall H.K. (Ed) <em>Blackberries and their hybrids. </em>Wallingford, UK: CAB International</div>Impact Statements
- PPQ seeks to further our interactions with WERA-20 research community in the areas of assay harmonization and inter-laboratory coordination of testing to demonstrate direct comparability and trend tracking of results for overall program improvement. We have developed tools for plant diagnosticians to further these efforts and we look forward to working with the WERA-20 to showcase these tools and explore their applicability to diagnostic testing done by the WERA-20.
Date of Annual Report: 08/02/2019
Report Information
Period the Report Covers: 10/01/2018 - 09/30/2019
Participants
Al Rwahnih, Maher (malrwahnih@ucdavis.edu) - UC Davis;Almeyda, Christie (cvalmeyd@ncsu.edu) - North Carolina State University;
Choi, Jess (jc3398@cornell.edu) – Cornell AgriTech;
Cieniewicz, Elizabeth (ejc238@cornell.edu) - Cornell AgriTech;
Clarke, Alexander (ac2742@cornell.edu) - Cornell AgriTech;\
Cooper, Cindy (ccooper@agr.wa.gov) – Washington State Department of Agriculture;
Flasco, Maddison (mf725@cornell.edu) - Cornell AgriTech;
Fuchs, Marc (mf13@cornell.edu) – Cornell Agritech;
Guerra, Lauri (lguerra@agr.wa.gov) - Washington State Department of Agriculture;
Harper, Scott (scott.harper@wsu.edu) - Washington State University;
Ho, Thien (thienxho@gmail.com) - Driscoll's;
Hoyle, Victoria (vjh9@cornell.edu) - Cornell AgriTech;
Hu, John (johnhu@hawaii.edu) – Unviersity of Hawaii;
Hurtado, Oscar (Oscar.hurtado-gonzales@usda.gov) -USDA-APHIS;
Jones, Tammy (c-tajones@pa.gov) - Pennsylvania Department of Agriculture;
Kelly, Margaret (margaret.kelly@agriculture.ny.gov) - New York State Department of Agriculture & Markets;
Kinard, Gary (gary.kinard@ars.usda.gov) - USDA-ARS;
Li, Ruhui (Ruhui.Li@ars.usda.gov) - USDA-ARS;
Martin, Bob (bob.martin@ars.usda.gov) - USDA-ARS;
Nikolaeva, Ekaterina (enikolaeva@pa.gov) - Pennsylvania Department of Agriculture;
Osterbaan, Larissa (ljo29@cornell.edu) - Cornell AgriTech;
Perry, Keith (klp3@cornell.edu) - Cornell University;
Peter, Kari Anne (kap22@psu.edu) – Penn State University;
Postman, Joseph (joseph.postman@ars.usda.gov) - USDA ARS;
Prokrym, David (David.R.Prokrym@aphis.usda.gov) – USDA-APHIS;
Rayapati, Naidu (naidu.rayapati@wsu.edu) - Washington State University;
Rivera, Yazmin (yazmin.rivera2@usda.gov) - USDA-APHIS-PPQ-S&T;
Roy, Brandon (br005@lvc.edu) - Cornell AgriTech;
Rudyj, Erich (Erich.S.Rudyj@usda.gov) – USDA-APHIS;
Schmidt, Anna-mary (anna-mary.schmidt@canada.ca) - Canadian Food Inspection Agency;
Talton, Win (lwtalton@ncsu.edu) – North Carolina State University;
Trujillo, Sarah (Sarah.G.Trujillo@aphis.usda.gov) – USDA-APHIS;
Tzanetakis, Ioannis (itzaneta@uark.edu) - University of Arkansas;
Zhang, Shulu (shulu@agdia.com) – Agdia, Inc;
Welliver, Ruth (rwelliver@pa.gov) – Pennsylvania Department of Agriculture
Brief Summary of Minutes
Ioannis Tzanetakis, University of Arkansas, was elected as Secretary of the annual WERA-20 2019 meeting. Additionally, it was unanimously agreed that WERA-20 2020 will be hosted by the University of Hawaii. Subsequently, John Hu, University of Hawaii, is proposing the meeting to be held on May 22-26, 2020. Below are the minutes of the meeting taken by the Secretary:
Naidu Rayapati, Washington State University
Surveys were conducted to identify economically important viruses of grapevine and blueberry in Washington State, and determine their distribution. In grape, leafroll-associated viruses, particularly grapevine leafroll-associated virus 3 (GLRaV-3) (87% of the same tested positive for this virus), red blotch, fanleaf, vitiviruses, and nepoviruses are present. The ratio of GLRaV-3 and grapevine red blotch virus (GRBV) is 11:1 in WA vineyards. Symptoms of leafroll and red blotch cannot be distinguished visually. Tobacco ringspot virus (TRSV) starts to emerge as a problem. Nursery material has less than 1% of GLRaV-3 and no GRBV. In blueberry, decline was observed on Draper in association with TRSV. This is the first report of TRSV in blueberry WA and the pacific Northwest. Blueberry mosaic-associated virus and Blueberry latent virus were also detected.
Scott Harper, Washington State University
Surveys of graft-transmissible pathogens in stone fruit trees showed a high incidence of western-X phytoplasma (24%), little cherry virus 2 (14%) and a lower incidence of little cherry virus 1 (less than 1%). Interestingly, little cherry disease is primarily distributed in the north and western X disease in the south of Washington State. Western X is present in peaches and nectarines, pollinator trees and weeds. In apple, declining trees are reported. The phloem tissue of declining trees is dying, and several known but also new viruses are identified in declining trees. In hops, viruses and viroids are detected using in-situ hybridization.
Lauri Guerra, Washington State Department of Agriculture
Registration and certification in Washington State is based on the process rather than the plants. Testing of trees showed that the majority of positive samples are infected by ilarviruses, several positives are infected with cherry leafroll virus (CLRV) but none of the samples has ever tested positive for plum pox virus (PPV). For nepoviruses, there is a need to monitor weeds or use sentinel plants. In stoolbeds, efforts are underway to clean the material from apple chlorotic leaf spot virus (ACLSV) and apple stem grooving virus (ASGV). Challenges are nepoviruses, little cherry disease, western X disease, cherry virus A and new viruses.
Cindy Cooper, Washington State Department of Agriculture
Efforts to revise the grape certification in Washington State are almost complete. Some insect vectors, viruses and pests have been added to the quarantine list.
Kari Peter, Penn State University
Apple decline is a major issue. Several known viruses have been identified in affected trees but none of them is associated with decline. Apple luteovirus 1 (ALV1) was identified by high throughput sequencing in declining trees. Some nurseries have 100% of their stoolbeds infected with ALV1. Rapid apple decline is on the uptick in Pennsylvania because of the multiple rain events.
Katya Nikolaeva, Pennsylvania Department of Agriculture
Surveys of grapes, stone fruits and pome fruits for phytoplasmas identified new species or subspecies. The infection rate was low: 1% for grapes and apples, and 5% for peaches. Phytoplasma americanum was identified in strawberry.
Ruhui Li, USDA-ARS
Two novel viruses were identified in cherry by high throughput sequencing: cherry robigovirus 5 and cherry trichovirus 3. Neither of them is very common.
John Hu, University of Hawaii
Banana bract mosaic virus was identified in ginger showing mosaic symptoms. The virus is similar to the ones found in India and the Philippines. This virus is transmissible from ginger to banana by the banana aphid. Canna yellow mottle virus (CaYMoV) and banana streak virus were also found in plants. Stunted plants had CaYMoV and bean common mosaic virus.
Shulu Zhang, Agdia, Inc
AmplifyRP Acceler8 and AmplifyRP Acceler8 XRT assays based on the end-point rapid DNA amplification technology were developed and released for 13 pathogens and discovery purpose (universal kits).
Larissa Osterbaan, Cornell AgriTech
Grapevine fanleaf virus (GFLV) mutants derived from strains F13 (asymptomatic) and GHu (vein clearing) were produced to identify the molecular determinant of symptom development in Nicotiana benthamiana. Symptom determinants mapped to the 21 nt/7 aa at the C-terminus of the RNA1-coded 1EPol. Symptoms are protein associated, rather than nucleotide-based, and residue 802 determines vein clearing symptomology.
Joseph Postman, USDA-ARS
Among the pathogen affecting the National Clonal Germplasm Repository in Corvallis, ORm Actinidia yellow leaf spot is recognized as a new disease of unknown etiology. Tomato ringspot virus (ToRSV) is spreading in Ribes. BRV is present in the collection but in only four plants. Blueberry shock virus (BlShV) has spread in the Vaccinium collection. There are accessions with potential resistance to BlShV. In apple, knobbed Russet is suspected of being of viral origin. For Xyllela, Oregon and Washington are free zones.
Bob Martin, USDA-ARS
Surveys of Rubus for viruses. At the G1 level, it is important to start clean from all viruses. Nurseries should stay clean using best management practices and testing for ‘canary’ viruses. In production fields, virus identity is not important as long as no disease is identified. A new luteovirus and two new cytorhabdoviruses were identified in raspberry in northern US. Eradication efforts of blueberry fruit drop-associated virus are in progress in Washington State.
Yannis Tzanetakis, University of Arkansas
A new detection protocol for the detection of viruses and taxonomic placement of eriophyiod mites using a single individual was developed and optimized. Using this assay, only viruses in vector species are detected, making this protocol a valuable tool to identify potential eriophyiod vectors of known and new diseases.
Maher Al Rwahnih, UC-Davis
Introduced grape material can receive a provisional release following high throughput sequencing. Are indicators that are used for biological assays of known viruses still useful since known viruses are identified based on laboratory techniques? Efforts within RSMP 35 call for the elimination of agents for which no type isolate or sequence is available.
Margaret Kelly, New York State Department of Agriculture and Markets
Best management practices are important but nurseries need to sell plants. Plants other than those available in foundation blocks can be considered for certification if there is not enough foundation material, they are obtained from reliable sources, and undergo extra testing.
Christie Almeyda, North Carolina State University
The pipeline for the production of clean plants for breeders at MPRU based on testing-clean up (heat therapy)-storage-release was outlined. Meristems from tissue culture plants collected at 39°C show approximately 50% survival. MPRU serves breeding programs in the Southeast and companies in Florida and the West Coast. Berries and grapes were tested for several viruses.
Anna-mary Schmidt, Canadian Food Inspection Agency
CFIA is ISO 17025 certified. Standard operating procedures and method development were advanced to support the implementation on high throughput sequencing in diagnostics. The technology is evaluated for sensitivity, reproducibility and repeatability
Oscar Hurtado, USDA-APHIS
Latent pome viruses are the most commonly intercepted agents at the Plant Germplasm Quarantine Program (PGQP). More than 360 plants, including over 120 pome fruits, have been gone through high throughput sequencing at PGQP.
A virus like citrus concave gum-associated virus was detected in pear from 22 countries. Testing efforts of the Malus germplasm repository in Geneva, NY are under way.
Yazmin, Rivera, USDA-APHIS
The performance of the MinIon technology was tested using ribo-depleted and smallRNA. The MinIon cDNA kit gives better results than RNA. A higher mapping to an RNA virus is obtained using the RNA kit but a lower percentage of complete genomes compared with RNA.
Libby Cieniewicz, Cornell Agritech
Epidemiological studies of grapevine red blotch virus (GRBV) showed an annual increase of virus incidence by 10% in proximity to riparian areas where aggregation of diseased vines occur. Populations of the three-cornered alfalfa hopper (TCAH) pick in summer months in Napa Valley, California. More TCAH are found in grapes close to the riparian zone. No spread of GRBV was found in NY. Wild vitis virus 1 (WWV-1), a new grablovirus, is only present in wild Vitis species in California. GRBV was found 20% of wild vines in California and WVV-1 in only 7 of them. Legume cover crops have not tested positive for GRBV.
Keith Perry, Cornell University
Grapevine DNA viruses consist of two badnaviruses and four grabloviruses. Rolling circle amplification and loop-mediated isothermal amplification are very sensitive and do not require the need of nucleic acid extraction from plant tissue. RNAseq revealed novel transcription patterns of some GRBV genes. GRBV is distributed in many areas around the globe although it appears to be of North American origin.
Accomplishments
<p><strong>Naidu Rayapati, Washington State University</strong></p><br /> <p>In a recent industry-wide survey, vineyard health was identified as one of the top research priorities for sustainable growth of the grape and wine industry in Washington State. Among diseases caused by different pathogens, virus diseases are by far the major constraints affecting vineyard health and longevity. With increased expansion of wine grape (<em>Vitis vinifera</em>) acreage in the state, implementing an integrated approach of planting virus-tested ‘clean’ planting materials and post-planting management of virus diseases has been recognized as an effective strategy for maintaining healthy vineyards. Towards this objective, diagnostic activities were conducted to improve the sanitary status of grapevines in registered mother blocks maintained by certified nurseries. A composite sampling strategy in combination with molecular diagnostic assays were used for high-throughput and reliable testing of grapevine samples from certified nurseries. The results obtained so far showed the absence of grapevine red blotch virus (GRBV) and very low incidence of grapevine leafroll-associated virus 3 (GLRaV-3) in registered mother blocks. Testing grapevine samples received from growers indicated that GLRaV-3 is more common than other GLRaVs and GRBV, independent of cultivars and geographic origin of samples. Although a few additional viruses were documented in commercial vineyards, they were detected largely as co-infections with GLRaV-3 and/or GRBV and found causing asymptomatic infections when present alone. Participatory approaches were pursued in collaboration with growers and nursery personnel to translate science-based knowledge for strengthening grapevine supply chain and to deploy integrated measures for controlling virus diseases in vineyards. For blueberries, Washington State became in recent years the national leader in blueberry production and the crop acreage is increasing rapidly in eastern Washington. Virus-like symptoms were observed in two blueberry fields affecting crop production. Leaf samples showing symptoms were tested by serological and molecular diagnostic assays and analyzed next-generation sequencing to identify viruses present in affected plants. In one field, blueberry mosaic-associated virus and blueberry latent virus were detected in symptomatic plants. In another field, tobacco ringspot virus was detected in symptomatic plants. Together with a previous report of the occurrence of TRSV in wine grapes, the finding of TRSV infecting blueberries highlights the potential significance of TRSV as an emerging virus problem to several specialty fruit crops in eastern Washington.<strong> </strong>Knowledge of viruses and improved understanding of their complex biology helped growers to deploy robust tactics for management of virus diseases in vineyards. </p><br /> <p><strong>Scott Harper, Washington State University</strong></p><br /> <p>Over the past year, research was focused on understanding and developing diagnostic methods and control measures for two major diseases affecting tree fruits in the Pacific Northwest: Little cherry disease and apple decline. We have been active in extension and outreach for affected growers, particularly for little cherry disease which has reached epidemic proportions in Washington State. In the past season, we have tested a total of 1945 cherry samples for the two pathogens that cause this disease, allowing growers to make informed management decisions about whether to remove trees in orchards. We are also actively researching the pathways by which the two unrelated pathogens, little cherry virus-2 and <em>Candidatus</em> Phytoplasma pruni, cause what is basically the same disease, small, bitter or acrid cherries, with the hope of identifying pathogenesis related genes of effectors, so that resistant or tolerant cherry varieties can be bred for commercial production. Work on apple decline has increased engagement with the apple industry, identifying a number of pathogens that may be involved with this disease, and require further study. This work has increased grower awareness of virus-like pathogens and the need for active management. Finally, we have been actively developing and publishing new and improved diagnostic methods for general use in detecting pathogens in both pome and stone fruit and for virus control. </p><br /> <p><strong>Ekatarina Nikolaeva,</strong> <strong>Pennsylvania Department of Agriculture</strong></p><br /> <p>The Pennsylvania Department of Agriculture (PDA) in cooperation with Penn State University conducted 2018 Farm Bill-funded surveys for exotic diseases in orchards and small fruits. Orchard survey targets included plum pox virus, Asian pear blight (<em>Erwinia pyrifoliae</em>), Asiatic brown rot (<em>Monilia polystroma</em>), Apple brown rot (<em>Monilinia fructigena</em>), Apple proliferation (<em>Candidatus</em> Phytoplasma mali), European stone fruit yellow (<em>Ca.</em> Phytoplasma prunorums), and Almond witches’ broom (<em>Ca.</em> Phytoplasma phoenicium). Small fruit survey targeted Asian pear blight (<em>Erwinia pyrifolia</em>), tomato black ring virus, Australian grapevine yellows (<em>Ca</em>. Phytoplasma australiense), Flavescence dorée phytoplasma (<em>Ca.</em> Phytoplasma vitis), and Bois noir phytoplasma (<em>Ca</em>. Phytoplasma solani). No exotic targets were detected, but we did confirm the presence of <em>Ca.</em> Phytoplasma pruni (16SrIII-A group, X-disease group) in peach and grapes, and <em>Ca.</em> Phytoplasma pyri (16SrX, Apple Proliferation group) in pears. In 2018, <em>Ca. </em>phytoplasma americanum has been detected in a strawberry plant (Fragaria × ananassa) with symptoms of stunting and unseasonal reddening and distortion of leaves. To our knowledge, this is a first report to indicate that Fragaria × ananassa can serve as a host of ‘Ca. Phytoplasma americanum’. In addition, data gathering was continued on rapid apple decline. Since August 2018, PDA inspected 27 apple blocks from 14 farms located in seven Pennsylvania counties for symptoms and signs of rapid apple decline, including the presence of declining trees, unseasonal tree discoloration and defoliation, brown cankers around graft union, and presence of root suckers. The main target was the newly described apple luteovirus 1 (ALV-1) which may play a role in RAD. A total of 121 samples from scion leaves, bark, blossoms, fruits, and root sucker leaves were found positive for ALV-1 using one-step reverse transcription (RT)-PCR assay developed by USDA ARS National Germplasm Resources Lab. The virus was detected from a wide range of apple varieties including Crimson Crisp, Fuji, Gala, Honeycrisp, Red Delicious and Golden Delicious. Most of the ALV1 positive trees (67%) were grafted on M9 rootstocks while only few positive trees found on Bud 9, Emla 26, M26, M7A, and G935. Our results suggest the virus does not distribute equally within the tree and the number of positive tree scaffolds increases during the infection process. ALV-1 was detected on some trees in combination with 1-3 apple latent viruses (ApMV, ASGV, ASPV, ACLSV) but there was no consistency in virus combinations. The work on determination of ALV1 pathogenicity was started in August 2018. Two hundred and twelve M9 rootstocks (not grafted) trees from different suppliers were evaluated for the presence of ALV1. Surprisingly, about 50% of the tested trees were found positive. ALV1 positive trees with a single virus infection were used for grafting with ALV1 negative budwood. We plan to revisit grafted trees this summer to evaluate virus transmission to the scion of the grafted trees and possible symptom development. Finally, PDA continues to operate the Fruit Tree Improvement Program (FTIP), a specialized virus-tested fruit tree certification program. Three nurseries have been participating in the FTIP last year. Over 2,000 samples were tested for viruses of concern, including <em>Prunus</em> necrotic ringspot, prune dwarf virus, tomato ringspot virus, and plum pox virus. No PPV was detected in rootstock blocks or in registered source blocks. PNRSV (1.5%) remains the most commonly found virus in <em>Prunus</em> in PA nurseries. PDV (0.6%) and ToRSV (0.2%) finds in registered blocks and nursery production blocks are very rare. All blocks met virus-testing requirements for FTIP certification.</p><br /> <p><strong>Ruhui Li, USDA-ARS</strong></p><br /> <p>Research involved characterization and detection of plant viruses infecting tree fruits and small fruits. The characterization of novel viruses or variants infecting these crops provides a foundation for detection and elimination of these viruses from germplasm and seedlings. Two new viruses have been discovered from currants, and preliminary surveys showed that these viruses were common, and they may be associated with viral diseases. </p><br /> <p><strong>John</strong><strong> Hu, University of Hawaii</strong></p><br /> <p><em>Bean common mosaic virus</em> (BCMV) is a species of the genus <em>Potyvirus</em> in the family <em>Potyviridae</em>. BCMV is transmitted mechanically and in a non-persistent manner by several species of aphids. Flowering ginger (<em>Alpinia purpurata</em>) is an important ornamental crop in Hawaiʻi that has been previously shown to harbor single and mixed infections by the potyvirus banana bract mosaic virus (BBrMV) and the badnavirus canna yellow mottle virus (CaYMV). In March 2019, flowering ginger plants with virus-like symptoms that were distinct from the mosaic and streaking symptoms produced by dual infections of BBrMV and CaYMV were observed on Oʻahu, Hawaiʻi. The symptoms included green mosaic patterns along leaf veins, plant stuntedness, and chlorosis. Symptomatic leaf samples were collected and total nucleic acids were extracted for testing by PCR. Using degenerate primers, the samples were assayed for the presence of potyviral and badnaviral infection. All symptomatic samples were found to be infected by CaYMV, but BBrMV was not detected. Direct Sanger sequencing and analysis of the resultant PCR products generated using the potyviral primers indicated the presence of BCMV. BLASTN search showed that the potyviral sequence (MN073501) shared 93.8% identity to BCMV strain A1 (<a href="https://www.ncbi.nlm.nih.gov/nucleotide/MK282414.1?report=genbank&log$=nucltop&blast_rank=1&RID=GDXUAVF3015">MK282414</a>), a new strain of BCMV characterized from Lima Bean in Hawaiʻi. To confirm BCMV infection, a BCMV-specific primer set (BCMV-CIg-F 5′-AGCTCGCCACATAAACAAGC-3′ and BCMV-CIg-R 5′-CTCAGAATGCGCGGRTTGAGC-3′) was designed to target the cylindrical inclusion (CI) protein gene. The expected 350-bp PCR products were obtained and the resulting sequences (MN043985) displayed a high similarity with the corresponding CI protein gene from a BCMV isolate obtained from a <em>Sesamum indicum</em> plant growing in China (<a href="https://www.ncbi.nlm.nih.gov/nucleotide/MK282414.1?report=genbank&log$=nucltop&blast_rank=1&RID=GDXUAVF3015">MK282414</a>), sharing 96.56% and 95.28% identity at the nucleotide and amino acids levels, respectively. Subsequently, the samples also tested positive using a potyvirus group-specific ELISA and a BCMV-specific ELISA following the manufacturer’s directions (Agdia, Elkhart, IN). In May 2019, four additional samples were collected from the same location. The BCMV primer-specific set was used in RT-PCR to verify infection by BCMV based on the amplification of PCR fragments of the expected size. The results of these assays further confirmed the presence of BCMV in flowering ginger. To our knowledge, this is the first report of BCMV naturally infecting flowering ginger. It is unclear if its presence in ginger is contributing to the current serious die-back disease of flowering ginger in Hawaiʻi. Additional research is needed to determine the potential cause of the threat to the ginger industry in Hawaiʻi. Collaborative work on BCMV is with Dr. Karasev, and on ginger and papaya viruses with Dr. Al Rwahnih.</p><br /> <p><strong>Shulu Zhang, Agdia, Inc</strong></p><br /> <p>Early detection and effective control of plant pathogens is very important to prevent their wide spread resulting in serious economic losses in crops like cherries, citruses, grapes, hops, peaches or plums. Agdia's AmplifyRP<sup>®</sup> utilizes a leading isothermal amplification technology called recombinase polymerase amplification (RPA) and has recently become a versatile diagnostic tool for rapid and accurate detection of nucleic acids in all types of plant pathogens. So far, Agdia has commercialized 13 AmplifyRP<sup>®</sup> kits specific to single species/strains of diverse pathogens and 2 AmplifyRP<sup>®</sup> Discovery kits suitable for any pathogens. Among the pathogen-specific kits, there are 7 kits in AmplifyRP<sup>®</sup> Acceler8<sup>®</sup> format, 3 kits in AmplifyRP<sup>®</sup> XRT format and 3 kits in AmplifyRP<sup>®</sup> XRT<sup>+</sup> format. Two of these kits, AmplifyRP<sup>®</sup> XRT for <em>Clavibacter michiganensis </em>subsp<em>. nebraskensis</em> and AmplifyRP<sup>®</sup> XRT<sup>+</sup> for <em>Dickeya</em> spp. were released since 2018 WERA-20 meeting. There are additional 14 pathogen-specific AmplifyRP<sup>®</sup> kits in development. Agdia has acquired the intellectual properties right for AmpliFire and started to produce and offer this portable, battery-operable fluorescence reader. </p><br /> <p><strong>Bob Martin, USDA-ARS</strong></p><br /> <p>The project on blueberry fruit drop-associated virus is leading to the eradication of this virus from commercial blueberries in the U.S. In a national survey carried out in 2015-2018, this virus was only detected in northwest Washington. For the past three years we have been working with growers in northwest WA to eradicate this virus from their fields. Approximately 2500 samples were tested representing all the major blueberry production areas in the U.S. After the first year of this project we have identified an enamovirus that is only present in the northeast U.S. and eastern Canada thus far. This virus is being studied to determine if it can cause raspberry leaf curl disease in <em>Rubus</em> cultivars that were grown in that region in the 1940s-1970 when the disease was common. We have also identified two novel rhabdoviruses but they are very limited in distribution at this time (one plant each), though one is a strain of raspberry vein chlorosis virus, which is considered a European virus. We are working with entomologists to identify vector(s) of GRBV in Oregon, where the three-cornered alfalfa hopper is very rare but we are seeing significant spread of the virus in commercial vineyards. We are also working with viticulturists and enologists at OSU to evaluate the impact of viticultural practices on fruit quality from plants infected with GRBV. </p><br /> <p><strong>Yannis Tzanetakis, University of Arkansas</strong></p><br /> <p>In collaboration with colleagues, several of which participate in WERA-20, we are working on the characterization and population structure of several viruses in strawberry (rhabdovirus), blueberry (luteo and carlavirus) and blackberry (allexi-, ifla- and reovirus). This information is used in the development of detection protocols that have the ability to detect the vast majority of isolates that circulate in the United States. One of the highlights of the year is the development of a new detection protocol that allows for the detection of viruses and taxonomic placement of eriophyiod mites using a single individual. In our experiments we were only able to detect viruses in vector species, making this protocol a valuable tool when it comes to identifying potential eriophyiod vectors of known and new diseases. To harmonize certification guidelines among states and commodities, we have obtained new funding for two meetings between representatives of several specialty crops, federal and state regulators.</p><br /> <p><strong>Maher</strong><strong> Al Rwahnih, University of California</strong></p><br /> <p>At Foundation Plant Services (FPS), we continue to make advances in developing and refining our methods using high throughput sequencing (HTS) as a superior diagnostic tool. We have used sequence information generated by HTS analysis to design new, specific PCR primers for use in PCR diagnostics. In addition, HTS proves to be an invaluable tool in the discovery of unknown viruses and in establishing a baseline analysis of the virome of a crop. Below are some specific accomplishments:</p><br /> <ul><br /> <li>We have greatly improved the efficiency of diagnostic laboratory assays for grapevine leafroll-associated virus 3 (GLRaV-3). GLRaV-3 has an exceptional number of highly diverse variants. Recent studies based on genome-wide phylogenetic analysis demonstrated that GLRaV-3 variants can be divided into eight distinct groups, six of which have been identified in California. This level of genetic diversity makes it very difficult to identify a conserved region common to all isolates for design of a single qPCR-based assay, however using six different variant specific assays is not desirable. FPS analyzed GLRaV-3 sequence data available in GenBank and those generated in-house to develop a new RT-qPCR assay with the capacity to detect all known GLRaV-3 variants. The new assay, referred to as FPST, was challenged against samples that included plants infected with different GLRaV-3 variants and originating from 46 countries. The FPST assay detected all known GLRaV-3 variants, including the highly divergent variants, by amplifying a small highly conserved region in the 3’ untranslated terminal region (UTR) of the virus genome. The reliability of the new RT-qPCR assay was confirmed by a newly developed enzyme linked immunosorbent assay (ELISA) that can detect all known GLRaV-3 variants characterized to date. The assay was further validated with 2415 samples obtained from the USDA National Clonal Germplasm Repository in Winters, CA, the Davis Virus Collection at UC-Davis, the FPS pipeline of foreign and domestic introductions, selected vineyards in the main grape-growing areas of California, and samples provided by international collaborators. This new assay is being shared with stakeholders, thus benefiting growers, researchers, and diagnostic labs involved in the grapevine industry in the US and around the world.</li><br /> <li>Last year, we reported the discovery of a novel vitivirus, named grapevine virus J (GVJ). Vitiviruses are ssRNA(+) viruses in the family <em>Betaflexiviridae </em>(subfamily <em>Trivirinae</em>). This year we report two novel vitiviruses: grapevine virus L (GVL) and grapevine virus M (GVM). GVL has a genomic sequence that is 7,607 nt long, including a typical genome organization of open reading frames (ORFs) encoding a replicase (RP), a 22 kDa protein, a movement protein, a coat protein (CP) and a nucleic acid binding protein. GVM was discovered by high-throughput sequencing in samples of the American hybrid bunch grape cultivar Blanc du Bois in Texas. The genome length is 7,387 nt, excluding the polyA tail. The genome sequence contains five ORFs that are homologous and phylogenetically related to ORFs of grapevine-infecting vitiviruses. In the last few years at least 5-10 new vitiviruses have been discovered. This high number of discoveries led us to launch a survey in 2018 to determine the incidence of the novel vitiviruses (GVG, GVH, GVI, GVJ and GVL) in California. The survey included 2,327 samples from commercial vineyards (728), FPS’ introduction pipeline (390), and the USDA National Clonal Germplasm Repository (1,209). Results of the survey showed that all the novel vitiviruses were identified in California, 55 of the 2,327 samples were positive, and mixed infections were common.</li><br /> <li>Rose rosette disease (RRD), caused by rose rosette virus (RRV), is a highly destructive disease of roses in the eastern U.S. In 2017, two symptomatic plants from a commercial nursery in Wasco, CA and three garden roses in Bakersfield, CA were positive for RRV. To our knowledge, this was the first report of rose rosette virus associated with rose rosette disease affecting roses in California. Given the high wholesale value of the rose industry at US$ 17.6 million in 2016, a widespread RRV outbreak would be devastating. This year rose plants in Dixon and Sacramento, California with disease symptoms similar to RRD were examined and tested for RRV at FPS. Samples were also sent to the USDA-ARS lab in Beltsville, Maryland to inspect for the vector but no vector was found. FPS is taking all necessary measures to reduce the risk of introducing RRV to the FPS foundation rose collection, the largest public collection of virus-tested roses in the United States and is not accepting introduction of material from sources in which RRD is known to occur.</li><br /> <li>Little cherry disease (LCD), associated with little cherry virus-1 (LChV-1) or -2 (LChV-2), is a common problem of cherries which occurs worldwide, causes unmarketable fruit and often results in tree or orchard removal. Most of the new cherry rootstocks used in cherry production are interspecific <em>Prunus </em>hybrids which introduces an increased risk of an adverse reaction (hypersensitivity) to some viruses. Hypersensitive reactions exhibit graft union gum exudation, premature abscission, and tree death within one or two growing seasons and have been shown to occur in <em>Prunus</em> when infected with prunus necrotic ringspot virus (PNRSV) and prune dwarf virus (PDV). FPS has begun evaluating the effects of LChV-1 and LChV-2 on 16 different popular Prunus rootstocks. All rootstocks will be grafted with a scion variety from the same accession. Observations of bud take and tree performance will be recorded and evaluated for two years. We are currently in the second year of this project; the first trial has been planted in the field. Rootstocks will be rated for sensitivity to LChV-1 and LChV-2 and this information will be shared with growers and nurseries to assist in making rootstock selection decisions.</li><br /> <li>As the fruit tree program at FPS is experiencing significant growth, FPS is working to improve fruit tree virus detection. FPS is running HTS versus the standard biological assay in side-by-side studies to accumulate comparative data. This work will help support our request to revise the Prunus 588 Controlled Import Permit SOP. Currently, the permit requires introduced plants to be held inside greenhouses and screenhouses. FPS would like to have the ability to plant HTS negative plants in the field. In addition, we would like to remove the unnecessary poorly performing woody indicators, Canindex and Kwanzan. We are also harmonizing our efforts with the Plant Inspection Station in Beltsville, Maryland and the Clean Plant Center Northwest, in Prosser, Washington, pursuing similar protocol revisions and establishing a framework for the evaluation of risks posed by new virus discovered by HTS.</li><br /> <li>The development and validation of real time quantitative PCR assays for the detection of fruit tree viruses is a new project. The objectives of the project are to evaluate current published assays and develop new assays, if needed. Representative sets of genome sequences of the targeted viruses were compiled, and current published primers were evaluated. New genetic data were incorporated and used to construct new and improved RT-qPCR assays that have been validated to efficiently and specifically detect 13 different pathogens. Next steps in the project are to design RT-qPCR assays for pear decline and apple stem grooving virus, complete validation of all assays, and large-scale validations of assays with screening samples from commercial orchards.</li><br /> <li>The value of using virus-tested material was documented in two recent papers. Research presented in <em>Economic Benefits from Virus Screening: A Case Study of Grapevine Leafroll in the North Coast of California</em> presents the costs and benefits of a virus screening program for Grapevine Leafroll associated Virus-3 (GLRaV-3) in the North Coast region of California. Grower costs and benefits from using GLRaV-3-free vines were computed and extrapolated to the North Coast wine grape industry as a whole. Economic benefits from the GLRaV-3 testing, therapy and distribution programs were found to be in excess of $20 million per year for the region, and substantially outweighed the costs. The results showed potential benefits from removing and replacing diseased vines rather than leaving them in the vineyard where they can be foci for disease spread. In addition, significant costs were associated with disease entering from infected vines in neighboring properties. In a second paper, research presented in <em>Virus surveys of commercial vineyards show value of planting certified vines</em> demonstrates the value of selecting certified vines for new plantings. Viruses cost the California wine grape industry as much as $91,661 per acre over the life of a vineyard. In the North Coast wine-growing region, mixed infections were predominant in older vineyards, while recently planted certified vines did not have mixed infections. Planting certified material regulated by the California Grapevine Registration and Certification program is a first step toward managing viruses.</li><br /> <li>While HTS remains a powerful new technology with significant benefits, there are technical challenges associated with the technology that warrants the establishment of guidelines for its use in plant certification and quarantine programs. We have begun efforts in a collaborative project to coordinate the development of minimum basic requirements for the adoption of HTS technologies, including nucleic acid extractions, library preparation, depth of sequencing and bioinformatics, for the detection of viral pathogens.</li><br /> </ul><br /> <p><strong>Anna</strong><strong>-mary Schmidt,</strong><strong> Canadian Food Inspection Agency</strong></p><br /> <p>The Centre for Plant Health continues to test a sampling of accessions taken from grapevine and tree fruit shipments from Canadian approved foreign certification programs in the United States, France and Germany for grapevines, and the United States, France, Belgium, Germany, Netherlands and United Kingdom for tree fruit. Non-certified material is also accepted and tested, and must be routed directly to the Centre for Plant Health PEQ facility for a full range of testing before release. This material includes imports from non-approved foreign sources or domestic breeding programs. Additionally, the Centre for Plant Health does a limited amount of regulatory testing for virus and virus-like diseases of berry crops. The testing requirements for imports are determined on a case-by-case basis depending on the origin of the material. Since Canada does not have a national small fruit certification program for exports, all testing for export is also done on a case-by-case basis depending on the requirements of the importing country. Furthermore, the tissue culture facility at the CFIA Sidney Laboratory supplies virus-tested rootstock and indicator plants for field and greenhouse woody bioassay testing. Upon request, the facility may produce small numbers of Generation 1 tissue culture initiates, from the CFIA’s tree fruit and grapevine virus-tested repository, for domestic or international distribution. This facility also receives and maintains tissue culture plants from international sources for inclusion in the CFIA Sidney Laboratory’s virus testing program. The tissue culture facility plays an integral part in the virus elimination service of the Sidney Laboratory. Virus elimination, when requested, is conducted on grapevine varieties using meristem culture following <em>in vivo</em> heat therapy of infected plants, and virus elimination of small fruit and tree fruit varieties occurs with meristem or microshoot culture following <em>in vivo</em> heat therapy. Finally, the Sidney Laboratory staff is involved in various plant-health related groups and committees including:</p><br /> <ul><br /> <li>NAPPO working group: Review of RSPM 35 "Guidelines for the Movement of Stone and Pome Fruit Trees and Grapevines into a NAPPO Member Country";</li><br /> <li>NAPPO panel for the development of Next Generation Sequencing (NGS) standards for plant virus diagnostics;</li><br /> <li>Canadian Grapevine Certification Network (CGCN);</li><br /> <li>BC Plant Protection Advisory council for grapevines;</li><br /> <li>International Council for the Study of Viruses and Virus-like Diseases of Grapevine;</li><br /> <li>Food and Agricultural Organization (FAO) International Plant Protection Convention (IPPC) Technical Panel on Diagnostic Protocols (TPDP);</li><br /> <li>Plant Health Quadrilateral (Canada, USA, New Zealand and Australia) Working Groups: “DNA barcoding”; “Diagnostic Collaboration” and “Managing regulatory issues arising from new diagnostic technologies”;</li><br /> <li>Genomics Research and Development Initiative;</li><br /> <li>Collaborative projects as part of the Euphresco (European Phytosanitary Research Coordination) Network (a network of European organisations funding research projects and coordinating national research in the phytosanitary area);</li><br /> <li>International Organization for Standardization working group for “Horizontal methods for molecular biomarker analysis”</li><br /> </ul><br /> <p><strong>Oscar</strong> <strong>Hurtado, USDA-APHIS, Plant Germplasm Quarantine Program</strong></p><br /> <p>The training received at Foundation Plant Services as well as the use of pipelines and UC Davis cluster HP computing facilities for high throughput sequencing (HTS) data analysis has enabled the Plant Germplasm Quarantine Program to move towards the implementation of HTS as a routine diagnostic tool in the fruit tree program. </p><br /> <p><strong>Keith Perry and Marc Fuchs, Cornell University</strong></p><br /> <p>We documented the role of grapevine red blotch virus (GRBV) in red blotch disease by fulfilling Koch’s postulates using infectious clones of a representative isolate of each of the two phylogenetic clades of virus. Agro-inoculated <em>Vitis vinifera</em> vines that became infected with GRBV manifested typical red blotch disease symptoms. We also developed a quantitative PCR for the detection of GRBV and identified the optimal time of the year and best tissue for optimal diagnosis. Additionally, we developed a rapid diagnostic assay for GRBV based on loop-mediated isothermal amplification (LAMP). This assay should have of high interest for onsite diagnosis. In parallel, a novel grablovirus, wild <em>Vitis</em> virus 1 (WVV1), was identified from non-cultivated <em>Vitis </em>sp. in California. No information is available on the potential role of WVV1 in disease etiology. Furthermore, progress was made on the ecology of grapevine red blotch virus by documenting a 10% annual increase in virus incidence in a vineyard edge proximal to a riparian area in California where disease vines are aggregated. In the remaining of the vineyard, annual disease progress was lower (2-3%). Also, a statistically strong association was found between the distribution of GRBV-infected vines and viruliferous three cornered alfalfa hoppers (TCAH) in a diseased vineyard, suggesting that TCAH is a vector of epidemiological significance. Moreover, a lower TCAH population was found associated with a reduced annual increase of GRBV incidence in California. This suggested that the TCAH population density could play a role in disease dynamics. To the contrary, no spread of GRBV was documented in a diseased vineyard in New York. Finally, GRBV was found widespread in wild grapevines in California, but not in New York. Overall findings on disease ecology informed disease management tactics that were disseminated to growers, vineyard managers, extension educators and service providers.</p>Publications
<p>Al Rwahnih, M., Golino, D. 2018. Prospects and challenges of high throughput sequencing for viral pathogen diagnosis and expedited release of quarantined propagative plant material. American Phytopathological Society. Boston, MA, USA.</p><br /> <p>Al Rwahnih, M., Golino, D., Westrick, N., Diaz-Lara, A., Cooper, M., Smith, R., Battany, M., Bettiga, L., Zhuang, S., Arnold, K., Farrar, K., Rowhani, A. 2018. Field survey and molecular characterization of Californian isolates of Grapevine Pinot gris virus. Proceedings of the 19th Congress of ICVG, Santiago, Chile, 130-131.</p><br /> <p>Al Rwahnih, M. 2018. High Throughput Sequencing as a tool for viral pathogen diagnosis and expedited release of quarantined propagative plant material current prospect and challenges. Proceedings of the 19th Congress of ICVG, Santiago, Chile, 58.</p><br /> <p>Alabi, O.J., McBride, S., Appel, D.N., Al Rwahnih, M. and Pontasch, F.M., 2019. Grapevine virus M, a novel vitivirus discovered in the American hybrid bunch grape cultivar Blanc du Bois in Texas. Archives of virology, pp.1-3.</p><br /> <p>Alzubi, H., Yepes, L.M. and Fuchs, M. 2019. <em>In vitro</em> storage at low temperature of micropropagated grapevine rootstocks.<em> In Vitro Cellular & Developmental Biology - Plant, 55:334-341</em>.</p><br /> <p>Arnold, K.L., N. McRoberts, M.L. Cooper, R. Smith, D. Golino. 2019Virus Surveys of Commercial Vineyards Show Value of Planting Certified Vines. California Agriculture 73: 90-95</p><br /> <p>Beaver-Kanuya E, Harper SJ. 2019. Detection and quantification of four viruses in Prunus pollen: Implications for biosecurity. Journal of Virological Methods 271:113673</p><br /> <p>Beaver-Kanuya E, Szostek S, Harper SJ. 2019. Development of real-time RT-PCR assays for two viruses infecting pome fruit. Journal of Virological Methods 266:25-29</p><br /> <p>Chingandu, N., Jarugula, S., Movva, A. and Naidu, R.A. 2019. The occurrence of economically detrimental viruses in certified nurseries and commercial vineyards in Washington State. Washington Winegrowers Convention and Trade Show, February 12-14, 2019, Kennewick, WA, USA.</p><br /> <p>Cieniewicz, E., Flasco, M., Brunelli, M., Onwumelu A., Wise, A. and Fuchs, M.F. 2019. Differential spread of grapevine red blotch virus in California and New York vineyards. Phytobiomes, <a href="https://doi.org/10.1094/PBIOMES-04-19-0020-R">https://doi.org/10.1094/PBIOMES-04-19-0020-R</a></p><br /> <p>Cieniewicz, E., Wise, A., Smith, R., Cooper, M, Martinson, T. and Fuchs, M. 2019. Studies on red blotch ecology inform disease management recommendations. Wine Business Monthly, March issue, pp. 92-102.</p><br /> <p>Cieniewicz, E. J., Pethybridge, S. J., Loeb, G., Perry, K., & Fuchs, M. 2018. Insights into the Ecology of Grapevine Red Blotch Virus in a Diseased Vineyard. Phytopathology, <em>108</em>, 92–102.</p><br /> <p>Cieniewicz, E., Thompson, J. R., McLane, H., Perry, K. L., Dangl, G. S., Martinson, T., Wise, A., Wallis, A. O'Connell, J., Dunst, R., Cox, K., Fuchs, M. F. 2018. Prevalence and Genetic Diversity of Grabloviruses in Free-Living Vitis spp. <em>Plant Disease.</em> 102:1-9.</p><br /> <p>Cieniewicz, E. and Fuchs, M. 2018. Red blotch disease ecology and management. Appellation Cornell, Research Focus, 4:1-7.</p><br /> <p>Cohen, D., Al Rwahnih, M., Chooi, K. M., Blouin, A., and MacDiarmid, R. M. 2018. Serotyping Grapevine leafroll-associated virus 3. Proceedings of the 19<sup>th</sup> Congress of ICVG, Santiago, Chile, 138-139.</p><br /> <p>Davenport B, Li R, and Zhang S. 2019. AmplifyRP® as a direct and rapid molecular confirmation tool for lateral flow ImmunoStrip® results (poster). 2019 NPDN National Meeting, April 15-18, 2019, Indianapolis, Indiana.</p><br /> <p>Davenport B, Groth-Helms D, Li R, and Zhang S. 2019. Development of a real-time duplex isothermal assay for the detection of Tobacco rattle virus and an endogenous internal RNA control (poster 439-P2). August 3-7, 2019, 2019 APS Annual Meeting, Cleveland, Ohio.</p><br /> <p>Debat, H.J., Zavallo, D., Brisbane, R.S., Voncina, D., Almeida, R.P., Blouin, A.G., Al Rwahnih, M., Gomez-Talquenca, S. and Asurmendi, S., 2019. Grapevine virus L: a Novel Vitivirus in Grapevine. European Journal of Plant Pathology, https://doi.org/10.1007/s10658-019-01727-w</p><br /> <p>Dey, Kishore, Milena Leite, John Hu, Jordan, Ramon, and Mike Melzer 2018. Detection of Jasmine virus H and characterization of a second pelarspovirus infecting star jasmine (<em>Jasminum multiflorum</em>) and angelwing jasmine (J. nitidum) plants displaying virus-like symptoms. Archives of Virology https://doi.org/10.1007/s00705-018-3947-y</p><br /> <p>Dey, K., Melzer M., and J. Hu 2018. Virus-Induced Gene Silencing <em>in</em> Plant Biotechnology,<br /> Volume 2: Transgenics, Stress Management, and Biosafety Issues.</p><br /> <p>Dey, K, James C Green, Michael Melzer, Wayne Borth, John Hu, 2018. Mealybug Wilt of Pineapple and Associated Viruses. Horticulturae. 4:52.</p><br /> <p>Diaz-Lara, A., Klaassen, V., Stevens, K., Sudarshana, M.R., Rowhani, A., Maree, H.J., Chooi, K.M., Blouin, A.G., Habili, N., Song, Y. and Aram, K., 2018. Characterization of grapevine leafroll-associated virus 3 genetic variants and application towards RT-qPCR assay design. PloS One, 13:p.e0208862.</p><br /> <p>Diaz Lara, A., Golino, D. Al Rwahnih, M. 2018. Grapevine virus J, a novel vitivirus identified in grapevine via high-throughput sequencing. American Phytopathological Society. Boston, MA, USA.</p><br /> <p>Diaz-Lara, A., Golino, D., and Al Rwahnih, M. 2018. Genomic characterization of Grapevine virus J, a novel virus identified in grapevine. Proceedings of the 19th Congress of ICVG, Santiago, Chile, 168-169.</p><br /> <p>Druciarek, T., Lewandowski, M. and Tzanetakis I.E. 2019. First report of European mountain ash ringspot-associated emaravirus in <em>Sorbus aucuparia</em> in Poland. Plant Disease 103:166.</p><br /> <p>Farrar, K., Al Rwahnih, M., Byrne, D., Clark, C. A., Finch, D., Fuchs, M., Golino, D. A., Martin, R. R., Matthews, P., Nourse, N., Scott, S., Sim, S. T., Tennis, B., Vidalakis, G. 2018. The National Clean Plant Network: Improving status and availability of clean stock. American Phytopathological Society. Boston, MA, USA.</p><br /> <p>Finn, C.E., Strik, B.C., Yorgey, B.M., Peterson, M.E., Jones, P.A., Lee, J. and Martin, R.R. 2018. ‘Columbia Giant’ thornless trailing blackberry. HortScience 53:251-255.</p><br /> <p>Finn, C.E., Strik, B.C., Yorgey, B.M., Peterson, M.E., Jones, P.A., Lee, J. and Martin, R.R. 2018. ‘Columbia Sunrise’ thornless trailing blackberry. HortScience 53:256-260.</p><br /> <p>Finn, C.E., Strik, B., Mackey, T., Jones, P., Bassil, N., and Martin, R.R. 2018. ‘Echo’ ornamental reflowering blueberry. HortScience 54:368-370.</p><br /> <p>Finn, C.E., Strik, B., Yorgey, B.M., Peterson, M.E., Jones, P.A., Lee, J., Bassil, N. and Martin, R.R. 2018. ‘Hall’s Beauty’ Thornless trailing blackberry. HortScience 54:371-376.</p><br /> <p>Fuller, K. B, Alston, J.M. and Golino, D.A. 2019. Economic Benefits from Virus Screening: A Case Study of Grapevine Leafroll in the North Coast of California. American Journal of Enology and Viticulture 70: 139-146.</p><br /> <p>Habili, N., Wu, Q., Al Rwahnih, M., and Pagay, V. 2018. Group II variants of Grapevine virus A are associated with Shiraz Disease in Australia. Proceedings of the 19th Congress of ICVG, Santiago, Chile, 164-165.</p><br /> <p>Hadaway, K.M. Ball, T., Folwell, R., Jarugula, S. and Naidu, R.A. 2019. To test or not to test? Providing affordable diagnostic assays for the benefit of grapevine nurseries and growers. Washington Winegrowers Convention and Trade Show, February 12-14, 2019, Kennewick, WA, USA.</p><br /> <p>Hamim, I., Al Rwahnih, M., Wayne, B.B., Melzer, M.J., Gonsalves, D., Suzuki, J., Wall, M., Hu, J.S. 2018. Deep sequencing of total RNAs in papaya for genome characterization of Papaya ringspot virus Bangladesh strain. American Phytopathological Society, Boston, MA, USA.</p><br /> <p>Hamim, I., Maher Al Rwahnih, Wayne B. Borth, Jon Y. Suzuki, Michael J. Melzer, Marisa M. Wall, James C. Green, and John S. Hu. 2019. Papaya ringspot virus isolates from papaya in Bangladesh: detection, characterization and distribution. Plant Disease, in press</p><br /> <p>Hamim, I. Wayne B. Borth, Michael J. Melzer, Jon Y. Suzuki, Marisa M. Wall,·John S. Hu 2019. Occurrence of tomato leaf curl Bangladesh virus and associated subviral DNA molecules in papaya in Bangladesh: molecular detection and characterization, Archives of Virology https://doi.org/10.1007/s00705-019-04235-8</p><br /> <p>Hamim, Islam, Wayne B. Borth, Josiah Marquez, James C. Green, Michael J. Melzer, John S. Hu 2018 Transgene-mediated resistance to Papaya ringspot virus: challenges and solutions Phytoparasitica https://doi.org/10.1007/s12600-017-0636-4</p><br /> <p>Hamim, I., Wayne Borth, Michael J. Melzer, and John Hu 2018. Ultra-sensitive detection of <em>Papaya ringspot virus</em> using single-tube nested PCR. Acta Virologica 62: 379 – 385.</p><br /> <p>Hassan, M., Shahid, M.S. and Tzanetakis, I.E., 2018. Molecular characterization and detection of a novel vitivirus infecting blackberry. Archives of Virology 163:2889–2893.</p><br /> <p>Hassan, M., and Tzanetakis I.E. 2019. Population structure, evolution and detection of blackberry leaf mottle associated virus, an emerging Emaravirus. Plant Pathology 68: 775-782.</p><br /> <p>Hoang, N.H., Al Rwahnih, M., Preece, J., Hsu, E., Sim, S. and Golino, D. 2018. Development of a Meristem-tip Culture Procedure for Eradication of Cherry Virus-A in Selected Cultivars of Cherry. In IN VITRO CELLULAR & DEVELOPMENTAL BIOLOGY-ANIMAL (Vol. 54, pp. S30-S31). 233 SPRING ST, NEW YORK, NY 10013 USA: SPRINGER.</p><br /> <p>Jarugula, S., Gowda, S., Dawson, W.O. and Naidu, R.A. 2018. Development of infectious cDNA clones of <em>Grapevine leafroll-associated virus 3</em> and analyses of the 5′ non-translated region for replication and virion formation. Virology 523: 89-99.</p><br /> <p>Jarugula, S., Mitra, A., Adegbola, R., Swamy, P. and Naidu, R.A. 2019<sup>. </sup>Impacts of viral diseases in field-grafted vineyards in Washington State. 70<sup>th</sup> American Society for Enology and Viticulture (ASEV) National Conference, June 17-20, 2019, Napa Valley, CA.</p><br /> <p>James, D., Phelan J., Sanderson, D. 2018. Blackcurrant Leaf Chlorosis Associated Virus: Evidence of the Presence of Circular RNA during Infections. Viruses 10, DOI:10.3390/v10050260</p><br /> <p>James D., Phelan, J., Sanderson, D. 2019. Detection by high throughput sequencing and molecular characterization of complexes of fabaviruses infecting Staccato® sweet cherry (<em>Prunus aviam</em>) in Canada. Canadian Journal of Plant Pathology <a href="https://doi.org/10.1080/07060661.2019.1566179">https://doi.org/10.1080/07060661.2019.1566179</a></p><br /> <p>Jelkmann, W., Sanderson, D., Berwarth, C., James, D. 2018. First detection and complete genome characterization of a Cherry (C) strain isolate of plum pox virus from sour cherry (<em>Prunus cerasus</em>) in Germany. Journal of Plant Diseases 125:267-272.</p><br /> <p>Li R, Davenport B, Groth-Helms D, and Zhang S. 2019. Development of AmplifyRP assays for three important grapevine viruses through recombinase polymerase amplification (poster 434-P2). 2019 APS Annual Meeting, August 3-7, 2019, Cleveland, Ohio.</p><br /> <p>Liu H., Wu L., Nikolaeva EV, Peter K., Liu Z., Mollov D., Cao M., Li R. 2018. Characterization of a new apple luteovirus identified by high-throughput sequencing. Virology Journal 15:85</p><br /> <p>Martin, I., Vigne, E., Berthold, F., Komar, V., Lemaire, O., Fuchs, M. and Schmitt-Keichinger, C. 2018. The fifty distal amino acids of the 2A<sup>HP</sup> homing protein of grapevine fanleaf virus elicit a hypersensitive reaction on <em>Nicotiana occidentalis</em>. Molecular Plant Pathology, 19:731-743.</p><br /> <p>Martin, R.R. and Tzanetakis, I.E. 2018. High risk blueberry viruses by region in North America; Implications for certification, nurseries, and fruit production. Viruses 10:342 doi:10.3390/v10070342</p><br /> <p>Milusheva, S., Phelan, J., Piperkova, N., Nikolova, V., Gozmanova, M., James, D. 2019. Molecular analysis of the complete genome of an unusual virus detected in sweet cherry (<em>Prunus avium</em>) in Bulgaria. European Journal of Plant Pathology 153:197–207</p><br /> <p>Moore, P.P., Hoashi-Erhardt, W., Finn, C.E., Martin, R.R. and Dossett, M. 2019. ‘WSU 2166’ Red raspberry. HortScience 54:564-567. https://doi.org/10.21723/HORTSCI13652-18.</p><br /> <p>Mitra, A., Jarugula, S., Donda, B., Jordan, E., and Naidu, R.A. 2019. Elucidating the genetic makeup of <em>Grapevine leafroll-associated virus 3 </em>for managing leafroll disease in Washington State vineyards<strong>. </strong>Washington Winegrowers Convention and Trade Show, February 12-14, 2019, Kennewick, WA, USA.</p><br /> <p>Naidu, R.A. 2019. An update on grapevine viruses in Washington vineyards. G.S. Long Co., Inc. 2014 Grower Meeting, January 16, 2019, Yakima, WA.</p><br /> <p>Naidu, R.A. 2019. Current and on-going research on roguing in vineyards. Washington Winegrowers Convention and Trade Show, February 12-14, 2019, Kennewick, WA, USA.</p><br /> <p>Nikolaeva E.V., Peter K.A., Jones T., Knier R., C. Molnar. 2019. Pennsylvania Orchard survey for Rapid Apple Decline. 2019. Phytopathology, in press</p><br /> <p>Osterbaan, L. and Fuchs, M. 2019. Dynamic interplays between plant virus and their host interactants for symptom development. Journal of Plant Pathology, https://doi.org/10.1007/s42161-019-00323-5</p><br /> <p>Osterbaan, L.J., Choi, J., Kenney, J., Flasco, M., Vigne, E., Schmitt-Keichinger, C., Rebelo, A.R., Heck, M. and Fuchs, M. 2019. The identity of a single residue of the RNA-dependent RNA polymerase of grapevine fanleaf virus modulates vein clearing symptoms in <em>Nicotiana benthamiana</em>. Molecular Plant-Microbe Interactions, 32:790-801.</p><br /> <p>Osterbaan, L., Schmitt-Keichinger, C. Vigne, E. and Fuchs, M. 2018. Optimal systemic grapevine fanleaf virus infection in <em>Nicotiana benthamiana </em>following agroinoculation. Journal of Virological Methods, 257:16-21.</p><br /> <p>Pallás, V., Sánchez-Navarro, J., James, D. 2018. Recent advances on the multiplex molecular detection of plant viruses and viroids. Frontiers in Microbiology <a href="https://doi.org/10.3389/fmicb.2018.02087">https://doi.org/10.3389/fmicb.2018.02087</a></p><br /> <p>Perry, K. L., McLane, H., Thompson, J. R., & Fuchs, M. 2018. A novel grablovirus from non-cultivated grapevine (<em>Vitis</em> sp.) in North America. Archives of Virology, <em>163</em>:259–262.</p><br /> <p>Pinon, A.F. and Martin, R.R. 2018. Frist report of strawberry necrotic shock virus in strawberry in Benquet, Philippines. Plant Disease 102:2385. <a href="https://doi.org/10.1094/PDIS-03-18-0491-PDN">https://doi.org/10.1094/PDIS-03-18-0491-PDN</a></p><br /> <p>Rasool, S., Naz, S., Rowhani, A., Diaz-Lara, A., Golino, D.A., Farrar, K.D. and Al Rwahnih, M., 2019. Survey of grapevine pathogens in Pakistan. Journal of Plant Pathology, https://doi.org/10.1007/s42161-019-00263-0</p><br /> <p>Romero, J. L. R., Carver, G. D., Johnson, P. A., Perry, K. L., and Thompson, J. R. 2019. A rapid, sensitive and inexpensive method for detection of grapevine red blotch virus without tissue extraction using loop-mediated isothermal amplification. Archives of Virology. 164:1453–1457.</p><br /> <p>Rott, M., Kesanakurti, P., Berwarth, C., Rast, H., Boyes, I., Phelan, J., Jelkmann, W. 2018. Discovery of Negative-Sense RNA Viruses in Trees Infected with Apple Rubbery Wood Disease by Next-Generation Sequencing. Plant Disease DOI:10.1094/PDIS-06-17-0851-RE</p><br /> <p>Sanderson, D., James D. 2019. Analysis of the genetic diversity of genome sequences of isolates/variants of <em>apple hammerhead viroid</em>. Canadian Journal of Plant Pathology DOI: 10.1080/07060661.2019.1614672</p><br /> <p>Serra, P., Messmer, A., Sanderson, D., James, D., Flores, R. 2018. Apple hammerhead viroid-like RNA is a bona fide viroid: Autonomous replication and structural features support its inclusion as a new member in the genus <em>Pelamoviroid</em>. Virus Research 249:8-15</p><br /> <p>Setiono, F. J., Chatterjee, D., Fuchs, M., Perry, K. L., & Thompson, J. R. 2018. The distribution and detection of grapevine red blotch virus in its host depends on time of sampling and tissue type. <em>Plant Disease</em>. 102:2187-2193.</p><br /> <p>Sim, S.T., Khuu, N., Shoulders, J.R., Pudlo, W., Hoang, N.H. and Golino, D.A. 2019. Elimination of rose viruses using microshoot tip tissue culture. Acta Hortic. 1232:241-246</p><br /> <p>Sinha, R., Khot, L.R., Rathnayake, A.P., Gao, Z. and Naidu, R.A. 2019. Visible-near infrared spectroradiometry-based detection of grapevine leafroll-associated virus 3 in a red-fruited wine grape cultivar. Computers and Electronics in Agriculture 162: 165-173.</p><br /> <p>Thekke-Veetil, T., Ho, T., Postman, J.D., Martin, R.R. and Tzanetakis, I.E. 2018. A virus in American blackcurrant (Ribes americanum) with distinct genome features reshapes classification in the Tymovirales. Viruses 10:342 </p><br /> <p>Thompson, B.D., Dahan, J., Lee, J., Martin, R.R. and Karasev, A.V. 2019. A novel genetic variant of <em>Grapevine leafroll-associated virus-3</em> (GLRaV-3) from Idaho grapevines. Plant Disease 103:509-518. </p><br /> <p>Tzanetakis, I.E. and Martin R.R. 2019. Improving plant propagation methods for fruit disease control, in Integrated management of insect pests and diseases of tree fruit (Ed. X. Xu and M. Fountain, Burleigh Dodds, pp14, in press</p><br /> <p>Vargas-Asencio, J., Liou, H., Perry, K. L., and Thompson, J. R. 2019. Evidence for the splicing of grablovirus transcripts reveals a putative novel open reading frame. Journal of General Virology. 100:709–720.</p><br /> <p>Villamor, D.E., Ho, T., Al Rwahnih, M., Martin, R.R. and Tzanetakis, I.E., 2019. High Throughput Sequencing in Plant Virus Detection and Discovery. Phytopathology 109: 716-725.</p><br /> <p>Wang, D., I. Hamim, J. C. Green, W. B. Borth, M. J. Melzer, and J. S. Hu, 2018. First Report of <em>Dasheen mosaic</em> <em>virus</em> infecting Taro (<em>Colocasia esculenta</em>) in Bangladesh. Plant Disease <a href="https://doi.org/10.1094/PDIS-03-18-0442-PDN">https://doi.org/10.1094/PDIS-03-18-0442-PDN</a>.</p><br /> <p>Wang, D., Ocenar<sup>, </sup>J., I. Hamim, J. C. Green, W. B. Borth, M. J. Melzer, Suzuki, J., M. M. Wall, M.M., Matsumoto, T., G. F. Sun, and J. S. Hu, 2018. First Report of <em>Bean yellow mosaic virus</em> Infecting Nasturtium (<em>Tropaeolum majus</em>) in Hawaii.<strong> </strong>Plant Disease<em> </em><a href="https://doi.org/10.1094/PDIS-06-18-1082-PDN">https://doi.org/10.1094/PDIS-06-18-1082-PDN</a>.</p><br /> <p>Wang, D., I. Hamim, J. C. Green, W. B. Borth, M. J. Melzer, Suzuki, J., M. M. Wall, M.M., Matsumoto, T., G. F. Sun, and J. S. Hu, 2018. First Report of Apple of Peru (<em>Nicandra physalodes</em>) Infected with <em>Pepper mottle virus</em> in Hawaii. Plant Disease<em> </em><a href="https://doi.org/10.1094/PDIS-06-18-1061-PDN">https://doi.org/10.1094/PDIS-06-18-1061-PDN</a>.</p><br /> <p>Weiland, J.E., Benedict, C., Zasada, I.A., Scagel, C.R., Beck, B.R., Davis, A., Graham, K., Peetz, A., Martin, R.R., Dung, J.K.S., Gaige, A.R. and Thiessen, L. 2018. Late summer disease symptoms in western Washington red raspberry fields associated with co-occurrence of <em>Phytophthora rubi, Verticillium dahliae</em>, and <em>Pratylenchus penetrans</em>, but not <em>Raspberry bushy dwarf virus</em>. Plant Disease 102:938-947.</p><br /> <p>Wright AA, Szostek S, Harper SJ. 2018. Diversity of three bunya-like viruses infecting apple. Archives of Virology 163:3339–3343</p><br /> <p>Wu, L., Liu, H., Postman, J.D., Li, R. 2018. Molecular characterization of a novel nucleorhabdovirus from black currant identified by high-throughput sequencing. Archives of Virology <a href="https://doi.org/10.1007/s00705-018-3709-x">https://doi.org/10.1007/s00705-018-3709-x</a>.</p><br /> <p>Xiao, H., Li, C., Al Rwahnih, M., Dolja, V. and Meng, B., 2019. Metagenomic Analysis of Riesling Grapevine Reveals a Complex Virome Including Two New and Divergent Variants of Grapevine leafroll-associated virus 3. Plant Disease 103:1275-1285.</p><br /> <p>Xue Feng, Gardenia E. Orellana, James C Green, Michael J. Melzer, John S. Hu and Alexander V. Karasev, 2019. A New Strain of Bean Common Mosaic Virus From Lima Bean (<em>Phaseolus lunatus</em>); Biological and Molecular Characterization. Plant Disease <a href="https://doi.org/10.1094/PDIS-08-18-1307-RE">https://doi.org/10.1094/PDIS-08-18-1307-RE</a></p><br /> <p>Yepes, L., Cieniewicz, E. J., Krenz, B., McLane, H. M., Thompson, J. R., Perry, K. L. and Fuchs, M. 2018. Causative role of grapevine red blotch virus in red blotch disease. Phytopathology 108:902-909.</p><br /> <p>Yepes, L.M., Burr, T.J., Reid, C. and Fuchs, M. 2019. Elimination of the crown gall pathogen, <em>Agrobacterium vitis</em>, from systemically infected grapevines by tissue culture. American Journal of Enology and Viticulture, 70:243-248.</p><br /> <p>Zhang, J., Borth, W.B., Sether, D., Lin, B, Melzer, M.J., Shen, H., Pu, X, Sun, D., Nelson, S., Hu, J.S. 2018. Multiplex Detection of Three Banana Viruses by Reverse Transcription Loop-mediated Isothermal Amplification (RT-LAMP). Tropical Plant Pathology 43:543–551.</p><br /> <p>Zhang, J., John Hu, Huifang Shen, Yucheng Zhang, Dayuan Sun, Xiaoming Pu, Qiyun Yang, Qiurong Fan and Birun Lin. 2018. Genomic analysis of the Phalaenopsis pathogen Dickeya sp. PA1, representing the emerging species Dickeya fangzhongdai. BMC <em>Genomics</em> (2018) 19:782 https://doi.org/10.1186/s12864-018-5154-3</p><br /> <p>Zheng, L., Wu, L., Postman, J.D., Liu, H., Li, R. 2018. Molecular characterization of a novel closterovirus identified from blackcurrant by high-throughput sequencing. Archives of Virology. https://doi.org/10.1007/s11262-018-1598-4.</p><br /> <p>Zurn, J.D., Ho, T., Li, R., Bassil, N.V., Tzanetakis, I.E., Martin, R.R. and Postman, J.D. 2019. First report of blackcurrant reversion virus in <em>Ribes nigrum</em> germplasm in the United States. Plant Disease, 103:1051.</p><br /> <p> </p>Impact Statements
- Keith Perry and Marc Fuchs, Cornell University. Disease ecology studies on grapevine red blotch disease were used to develop disease management strategies by considering patterns of GRBV spread, distribution and abundance of insect vectors, effects of GRBV on vine growth and fruit development, preferred hosts of insect vectors, and alternate hosts of GRBV. Efforts to promptly identify and remove virus inoculum sources, i.e., infected production vines and wild vines adjacent to vineyard and nursery plantings, is critical to reduce secondary spread of GRBV. Rogueing is recommended if disease incidence is less than 30%, and entire vineyard removal is advised if disease incidence is generally higher than 30% although regional differences should be considered for the adoption of this cost-minimizing threshold.
Date of Annual Report: 06/30/2020
Report Information
Period the Report Covers: 10/01/2019 - 09/30/2020
Participants
Al Rwahnih, Maher (malrwahnih@ucdavis.edu) - UC Davis;Allison, Gratz (allison.gratz@inspection.gc.ca) - the CFIA Sidney Laboratory, Canada;
Almeyda, Christie (cvalmeyd@ncsu.edu) - North Carolina State University;
Anderson, Carolyn (carolyna@ucr.edu) – University of California Riverside ;
Bateman, Margarita LAPHIS" (Margarita.L.Bateman@aphis.usda.gov) – USDA-APHIS ;
Bily, Devin (dbily@pa.gov) - PA Department of Ag;
Bodaghi, Soharab (sohrab.bodaghi@ucr.edu ) – University of California Riverside ;
Bowen, Walter (wbowen@hawaii.edu) – University of Hawai’i;
Cieniewicz, Elizabeth Jeannette (ejc238@cornell.edu) – Cornell University;
Cooper, Cindy (ccooper@agr.wa.gov) – Washington State Department of Agriculture;
Dang, Tyler (tyler.dang@ucr.edu) – University of California Riverside ;
Dipak, Poudyal (dpoudyal@oda.state.or.us) – Oregon Department of Agriculture;
Erich (Erich.S.Rudyj@usda.gov) – USDA-APHIS;
Fatima M Osman Fatima M.( fmosman@ucdavis.edu) – University of California at Davis
Fuchs, Marc (mf13@cornell.edu) – Cornell University;
Galanti, Russell (rgalanti@hawaii.edu) – University of Hawaii;
Guerra, Lauri (lguerra@agr.wa.gov) - Washington State Department of Agriculture;
Harper, Scott (scott.harper@wsu.edu) - Washington State University;
Ho, Thien (thienxho@gmail.com) - Driscoll's;
Hu, John (johnhu@hawaii.edu) – Univiersity of Hawaii;
Hurtado, Oscar (Oscar.hurtado-gonzales@usda.gov) -USDA-APHIS;
Jimenez, Randi@CDFA" Randi.Jimenez@cdfa.ca.gov - CA Department of Food & Agriculture;
Karasev, Alexander" (akarasev@uidaho.edu)- University of Idaho;
Kelly, Margaret (margaret.kelly@agriculture.ny.gov) - New York State Department of Agriculture & Markets;
Kong, Alexandra (atk412@hawaii.edu) – University of Hawaii;
Lavagi-Craddock, Irene (irenela@ucr.edu) – University of California Riverside ;
Li, Ruhui (Ruhui.Li@ars.usda.gov) - USDA-ARS;
Lutes, Lauri Ann (Lauri.Lutes@oregonstate.edu) – Oregon State University;
Martin, Bob (bob.martin@ars.usda.gov) - USDA-ARS;
Melzer, Michael (melzer@hawaii.edu) – University of Hawai’i;
Miles, Timothy D. (milesti2@msu.edu) – Michigan State University;
Mollov, Dimitre - ARS" (Dimitre.Mollov@ars.usda.gov ) – USDA-ARS;
Nikolaeva, Ekaterina (enikolaeva@pa.gov) - Pennsylvania Department of Agriculture;
Peter, Kari Anne (kap22@psu.edu) – Penn State University;
Postman, Joseph (joseph.postman@ars.usda.gov) - USDA ARS;
Prokrym, David (David.R.Prokrym@aphis.usda.gov) – USDA-APHIS;
Qiu, Wenping" (wenpingqiu@missouristate.edu) – State University of Missouri;
Rayapati, Naidu (naidu.rayapati@wsu.edu) - Washington State University;
Rivera, Yazmin (yazmin.rivera2@usda.gov) - USDA-APHIS-PPQ-S&T;
Sarmiento, Adriana Larrea (aelarrea@hawaii.edu) – University of Hawai’i;
Schmidt, Anna-mary (anna-mary.schmidt@canada.ca) - Canadian Food Inspection Agency;
Spaine, Pauline C - APHIS" pauline.c.spaine@usda.gov – USDA-APHIS,
Stamp, James (jamesastamp@gmail.com) – Professional Viticultural Services;
Suzuki, Jon" (jon.suzuki@usda.gov) – USDA-ARS;
Talton, Win (lwtalton@ncsu.edu) – North Carolina State University;
Tzanetakis, Ioannis (itzaneta@uark.edu) - University of Arkansas;
Velarde, Alejandro Olmedo (aolmedov@hawaii.edu ) - University of Hawai’i;
Vidalakis, Georgios (vidalg@ucr.edu) - University of California at Riverside;
Villamor, Dan Edward Veloso (dvvillam@uark.edu)- University of Arkansas;
Wall, Marisa (marisa.wall@usda.gov)- USDA - ARS;
Wang, Xupeng (xupeng@hawaii.edu) – University of Hawai’i;
Wei, Alan(apwei@agri-analysis.com)- Agri-Analysis LLC;
Zhang, Shulu (shulu@agdia.com) – Agdia, Inc;
Yilmaz.Balci@aphis.usda.gov – USDA-APHIS;
Hammond, John (john.hammond@usda.gov ) - USDA-ARS;
Nakhla, Mark (mark.k.nakhla@usda.gov) - USDA -APHIS;
Shiel, Patrick (patrick.j.shiel@usda.gov ) - USDA-APHIS;
Elizabeth Savory ( esavory@oda.state.or.us) - OR Department of Agriculture;
Elizabeth Dorman (dormane@michigan.gov) - Michigan Department of Ag;
Brief Summary of Minutes
- Naidu Rayapati, Washington State University,
- USDA-NIFA representative comments
- Next year’s location and host - It was unanimously agreed that WERA-20 2021 will be hosted by Prof. Georgios Vidalakis at the University of California Riverside. Prof. Georgios Vidalakis proposed the meeting to be held on May 22-26, 2021, subject to approval by the NIFA.
- Secretary to write minutes and final report with John and Naidu. (Yannis Tzanetakis) - Ioannis Tzanetakis, University of Arkansas, was elected as Secretary of the annual WERA-20 2020 meeting.
- Introduction of participants
- Group photos
9:30 Break
11:40 - 12:00 Adriana Larrea-Sarmiento/John Hu (University of Hawaii) - “Identification and characterization of a new Sadwavirus infecting field and germplasm of pineapple”
- Cindy Cooper, WA Department of Agriculture
- Elizabeth Savory, OR Department of Agriculture
- Elizabeth Dorman, Michigan Department of Ag
- Margaret Kelly, New York AG and Markets
- Randi Jimenez, CA Department of Food & Agriculture
- Devin Bily, PA Department of Ag
- Maher Al Rwahnuh (UC Davis)
- Yazmín Rivera, USDA APHIS PPQ S&T, Center for Plant Health Science & Technology (CPHST) Lab
- Oscar P. Hurtado-Gonzales, USDA APHIS PPQ Field Operations, Plant Germplasm Quarantine Program (PGQP)
- Yannis Tzanetakis (University of Arkansas)
- Maher Al Rwahnuh (UC Davis)
- Individual reports
- Group report
Below are the minutes of the meeting taken by the Secretary:
Topics presented by individual participants:
Accomplishments
<p><strong>Dan Edward Veloso Villamor</strong><strong>/</strong><strong>Yannis </strong><strong>Tzanetakis </strong><strong>(University of Arkansas)</strong></p><br /> <p> In collaboration with colleagues, several of which participate in WERA-20, we are working on the characterization and population structure of several viruses in strawberry (rhabdoviruses), blueberry (luteo, carlavirus and vitivirus) and blackberry (allexi-, ifla- and reovirus). This information is used in the development of detection protocols that have the ability to detect the vast majority of isolates that are circulating in small fruit crops in the United States. One of the highlights of the year is the completion of the comparison of conventional methods to high throughput sequencing (HTS), another collaboration between WERA-20 participants. The comparison was done using berry selections with known virus profiles. The results revealed the following trend:</p><br /> <ol><br /> <li>Virus detection by HTS and PCR were nearly identical with the exception of some viruses missed by HTS and detected by PCR and vice-versa.</li><br /> <li>Known viruses in some samples were not detected by HTS and PCR in all seasons.</li><br /> <li>HTS detected four novel viruses for which no PCR test is available. The presence of these viruses was validated by overlapping PCR to confirm authenticity. These viruses are the following: (1) a luteovirus in <em>Vaccinium</em>, a nucleorhabdovirus in <em>Fragaria</em>, and a potexvirus and coguvirus-like in <em>Rubus</em>).</li><br /> </ol><br /> <p>Overall, these results suggest the use of HTS for virus detection should be done at least two growing seasons to allow detection of low tittered virus(es). Additionally, the failure of HTS to detect some viruses can be addressed by increasing the amount of sequence reads for each sample. This can be done by either decreasing the amount of multiplex samples per sequencing lane or using a different platform with higher sequencing output (i.e. Novaseq).<strong> </strong></p><br /> <p> </p><br /> <p><strong>Maher </strong><strong>Al Rwahnih (UC-Davis: CA state report)</strong></p><br /> <p>At Foundation Plant Services (FPS), we continue to make advances in developing and refining our methods using high throughput sequencing (HTS) as a superior diagnostic tool. We have used sequence information generated by HTS analysis to design new, species-specific PCR primers for use in PCR diagnostics. In addition, HTS proves to be an invaluable tool in the discovery of unknown viruses and in establishing a baseline analysis of the virome of a crop. </p><br /> <p>In recent years, the fruit tree program at FPS has experienced significant growth in response to industry demand. In 2016, FPS acquired a Controlled Import Permit (P588) to facilitate the introduction, quarantine, and release of imported <em>Prunus </em>for the fruit tree industry. In 2020, we were successful in obtaining USDA-APHIS approval to revise the permit. In the past, regulations have required the grafting of candidate selections to four <em>Prunus</em> biological indicators (<em>P. persica</em> ‘GF 305’, <em>P. avium</em> ‘Canindex’, <em>P. avium</em> ‘Bing’, and <em>P. serrulata</em> ‘Kwanzan’) that were observed for symptom development in a greenhouse for at least one full growing season. If no disease symptoms were observed, the selection might be eligible for full quarantine release. This process frequently needed to be repeated if the imported selection underwent virus therapy, sometimes adding years to the quarantine process. We conducted side-by-side studies comparing HTS analysis to biological indexing which revealed that the performance of the biological indicators was inferior. Furthermore, since reliable laboratory tests were available to test for known pathogens detected by Canindex and Kwanzan, the removal of these two indicators that were redundant to the PCR and HTS tests was proposed. A new protocol for fruit tree introduction and testing was developed in coordination with Dr. Scott Harper at the Clean Plant Center Northwest (CPCNW, Prosser, Washington) to adopt the new testing approach and harmonize the standard operating procedures of the two <em>Prunus</em> quarantine programs. USDA APHIS PPQ and the CDFA approved the new protocol with the removal of Canindex and Kwanzan as biological indicators. Under the new protocol, plants are subjected to greenhouse indexing and molecular testing at two different time points by PCR and HTS. Plants may be released if all test results demonstrate plants are free of viruses or virus like agents. The culmination of this work and collaboration is the release of 20 selections, with an anticipated release of 11 additional selections in late summer 2020. The full release of new plant material under the new protocol will only take eight months to one year if it enters the program free of target viruses. Fruit tree nurseries and growers will greatly benefit from the release of material under this protocol as highly anticipated material be available to nurseries sooner, allowing them to begin their propagation and distribution of quality material to growers. </p><br /> <p>The ‘Development and validation of real time quantitative PCR assays for the detection of fruit tree viruses’ study evaluated the broad-range detection capacity of currently available real-time RT-PCR assays for <em>Prunus</em>-infecting viruses and developed new assays when current tests were inadequate or absent. Available assays for 15 different viruses were exhaustively evaluated <em>in silico</em> to determine their capacity to detect virus isolates deposited in GenBank. During this evaluation, several isolates deposited since the assay was designed exhibited nucleotide mismatches in relation to the existing assay’s primer sequences. In cases where updating an existing assay was impractical, we performed a redesign with the dual goals of assay compactness and comprehensive inclusion of genetic diversity. The efficiency of each developed assay was determined by a standard curve. To validate the assay designs, we tested them against a comprehensive set of 87 positive and negative <em>Prunus</em> samples independently analyzed by high throughput sequencing. As a result, the real-time RT-PCR assays described herein successfully detected the different viruses and their corresponding isolates. To further validate the new and updated assays a <em>Prunus</em> germplasm collection was surveyed. The sensitive and reliable detection methods described here will be used for the large-scale pathogen testing required to maintain the highest quality nursery stock. </p><br /> <p>Last year, we reported the discovery of two novel vitiviruses, “grapevine virus L” (GVL) and “grapevine virus M” (GVM). Vitiviruses are ssRNA(+) viruses in the family <em>Betaflexiviridae </em>(subfamily <em>Trivirinae</em>). This year we report the description of a novel virus detected by high-throughput sequencing (HTS) in a sample of grapevine (<em>Vitis vinifera</em>) cv. Kizil Sapak (sample/isolate 127) that originated from Turkmenistan. The complete genome of the virus, tentatively named “grapevine Kizil Sapak virus” (GKSV), is 7,604 nucleotides in length, excluding the poly(A) tail. The genome organization of GKSV, encoded genes, and sequence domains are typical for members of the family <em>Betaflexiviridae</em>, specifically those belonging to the subfamily <em>Trivirinae</em>. Phylogenetic analysis placed GKSV within the subfamily <em>Trivirinae</em>, in the same clade as fig latent virus 1 (FLV-1) but distinct from the clades formed by members of other genera. A comparative analysis of GKSV-127 with the HTS-derived sequences obtained from two additional isolates showed that they are genetic variants of the same virus species. Based on current ICTV species and genus demarcation criteria, and the results of the sequence and phylogenetic analyses, we propose that GKSV and FLV-1 represent a new genus within the subfamily <em>Trivirinae</em>. </p><br /> <p>Relatively few negative sense (ns)RNA viruses have been associated with infection in plants, including agricultural crops. However, in the last few years, the use of high throughput sequencing (HTS) has allowed the identification of new nsRNA viruses in plants. For example, the first nsRNA viruses identified and transmitted in grapevine, Grapevine Muscat rose virus (GMRV) and grapevine Garan dmak virus (GGDV), were only recently discovered because of HTS. The genomes of both viruses were comprised of three segments each containing a unique gene: RNA-dependent RNA polymerase (RdRp), nucleocapsid protein (NP) and movement protein (MP). Based on sequence identity and phylogenetic analysis, GMRV and GGDV represent new members of the family <em>Phenuiviridae</em>; most phenuiviruses are linked to diseases in vertebrates and are vectored by arthropods. Similarly, HTS was used to characterize nsRNA viruses in apple and citrus associated with different diseases. The discovery of these two nsRNA viruses in grapevine and the parallel report of additional viruses with this type of genetic material in other perennial crops suggests a more extensive distribution. Consequently, the characterization of nsRNA viruses in plants via HTS will remain an active area of research for some time. </p><br /> <p>Little cherry disease (LCD), associated with little cherry virus-1 (LChV-1) or -2 (LChV-2), is a common problem of cherries which occurs worldwide, causes unmarketable fruit and often results in tree or orchard removal. Most of the new cherry rootstocks used in cherry production are interspecific <em>Prunus </em>hybrids which introduces an increased risk of an adverse reaction (hypersensitivity) to some viruses. Hypersensitive reactions exhibit graft union gum exudation, premature abscission, and tree death within one or two growing seasons and have been shown to occur in <em>Prunus</em> when infected with prunus necrotic ringspot virus (PNRSV) and prune dwarf virus (PDV). FPS has been evaluating the effects of LChV-1 and LChV-2 on 15 different popular <em>Prunus</em> rootstocks. Rootstocks were t-bud grafted with a scion variety from the same accession in June 2018. In 2019, bud take was recorded in the spring and rootstock vegetation above the scion buds was tested by RT-qPCR to confirm successful virus transmission in the fall. Two years of budtake and tree performance observations will be recorded and evaluated. Rootstocks will be then be rated for sensitivity to LChV-1 and LChV-2, and this information will be shared with growers and nurseries to assist in making rootstock selection decisions. </p><br /> <p>While HTS remains a powerful new technology with significant benefits, there are technical challenges associated with the technology that warrants the establishment of guidelines for its use in plant certification and quarantine programs. We have begun efforts in a collaborative project with the APHIS Plant Germplasm Quarantine Program (PGQP) and Center for Plant Health Science & Technology (CPHST) in Beltsville Maryland to coordinate the development of minimum basic requirements for the adoption of HTS technologies, including nucleic acid extractions, library preparation, depth of sequencing and bioinformatics, for the detection of viral pathogens. Both labs have optimized several HTS pipelines and data analysis protocols, but an inter-laboratory validation of these protocols is still needed. The goal of this project is to validate an HTS protocol (TruSeq Stranded Total RNA, Ribo-depleted) using the NextSeq Illumina platform via an inter-laboratory comparison among three laboratories (FPS, CPHST, and PGQP). The project will culminate in the preparation of a validation report for each sample panel evaluated. The validation report would be used as a guideline for the preparation of SOPs or another ISO-type document that will include minimum acceptable criteria for quality control (QC). In addition, these results will be used as an example for preparing a workflow (guidelines) for the validation of HTS protocols on other crops. Successful completion of this project will, 1) set up the stage for further HTS protocols verification/validation for other specialty crops; 2) provide a mechanism for the evaluation of validation results for faster HTS protocol acceptance; 3) increase stakeholders confidence in HTS used for regulated pathogen detection in the certification programs by minimizing the risk of false negatives; 4) increase regulators confidence on the reliability and potentials of HTS for detection of regulatory pathogens and ultimately; 5) expedite the release of foreign quarantined and domestic propagative plant material to stakeholders. Clean plant material for clean nursery stock will ultimately facilitate international and domestic movement of nursery material and will safeguard the industry from exotic plant pathogens. </p><br /> <p> </p><br /> <p><strong>Allison Gratz (</strong><strong>the CFIA Sidney Laboratory, Canada) </strong></p><br /> <p><strong><em>Quarantine and Diagnostic Activities - </em></strong>The CPH continues to test non-certified tree fruit and grapevine material (from both non-approved foreign sources routed directly to CPH and domestic programs). Likewise, the CPH carries out some regulatory testing for virus and virus-like diseases of small fruit (berries). The testing requirements for imports and exports are determined on a case-by-case basis depending on the origin of the material and requirements of the importing country. The CPH also tests samples taken from grapevine and tree fruit shipments imported from Canadian approved foreign certification programs from various sources, including the United States, France, Germany, and the Netherlands. </p><br /> <p><strong><em>Tree Fruit Program Overview & Update - </em></strong>From 2014 to 2019, the diagnostics program tested 254 new <em>Malus, Pyrus</em>, and <em>Prunus</em> <em>spp.</em> accessions from non-certified sources in Canada (BC, SK, MB, ON, PQ, NS), USA (WA, CA, OH), Czech Republic, France, Germany, Hungary, Italy, Spain, and Switzerland. Of these, 17% were infected with a virus or viroid that required elimination prior to placement in the G1 repository (ACLSV, ASGV, ASPV, CGRMV, CLRV, CNRMV, CVA, LCV-1, PBNSPaV, PDV, PLMVd, PNRSV, unknown (bioassay positive)). </p><br /> <p>The average length of time to complete testing of non-certified tree fruit samples submitted since 2008 was 3.8 years (<em>Prunus spp</em>.), 4.3 years (<em>Malus spp</em>.) or 4.7 years (<em>Pyrus spp</em>.), with an extra two years required if virus elimination was needed. The annual number of samples received during this period varied from 14 to 44 (average of 29) with the number of shipments ranging from 6 to 14 (average of 10). </p><br /> <p>The purpose of the CPHs Generation 1 (nuclear level) repository is to supply Canadian industry with virus tested propagative material, and also to support Canadian industry by maintaining selections from domestic breeding programs at a level to meet import regulations of major trading partners. Currently it houses 484 Malus, Pyrus, Cydonia, and Prunus accessions. Permission must be obtained from sponsors in order to access the material: 90% are sponsored by Canadian breeding programs (primarily Agriculture and Agri-Food Canada (AAFC), University of Guelph and University of Saskatchewan), or Canadian industry; 1% are sponsored by international companies, and the remainder are publicly available for program use. </p><br /> <p>From 2014 to 2020, about 40, 000 buds were distributed from the repository with 63% in volume (50% of orders) distributed to Canadian clients; 25% of buds (34% of orders) to American clients, and the remainder distributed to other international destinations. </p><br /> <p><strong><em>Other accomplishments - </em></strong>The CPH has hosted scientists from various domestic and international facilities. Typically, these visits have been based around collaborative research/diagnostic interests and projects, whereby methods and information has been shared. Some examples include representatives from the Animal and Plant Health Inspection Service of the United States Department of Agriculture, Vineland Research and Innovation Center, PhytoDiagnostics, University of Victoria, Brock University, AAFC, and CFIA scientists as per the lab-exchange initiative. </p><br /> <p>Staff at the CPH are involved in various plant-health related groups and committees including:</p><br /> <ul><br /> <li>North American Plant Protection Organization (NAPPO) working group to review RSPM 35 "Guidelines for the Movement of Stone and Pome Fruit Trees and Grapevines into a NAPPO Member Country";</li><br /> <li>NAPPO panel for the development of Next Generation Sequencing standards for plant virus diagnostics;</li><br /> <li>Canadian Grapevine Certification Network</li><br /> <li>BC Plant Protection Advisory council for grapevines;</li><br /> <li>International Council for the Study of Virus and other Graft Transmissible Diseases of Fruit Crops</li><br /> <li>International Council for the Study of Viruses and Virus-like Diseases of Grapevine</li><br /> <li>Food and Agricultural Organization International Plant Protection Convention Technical Panel on Diagnostic Protocols ;</li><br /> <li>Plant Health Quadrilateral (Canada, USA, New Zealand and Australia) Working Groups: “DNA barcoding”; “Diagnostic Collaboration” and “Managing regulatory issues arising from new diagnostic technologies”</li><br /> <li>Genomics Research and Development Initiative;</li><br /> <li>Collaborative projects as part of the European Phytosanitary Research Coordination Network<strong> </strong></li><br /> </ul><br /> <p> </p><br /> <p><strong>Alejandro Olmedo-Velarde</strong><strong>/ Mike Melzer (University of Hawaii)</strong></p><br /> <p>Flat mites are minute polyphagous arachnids that belong to the Tenuipalpidae family. <em>Brevipalpus</em> and <em>Tenuipalpus</em> are considered the most relevant genera in the family because they contain quarantine pests and the former have members able to transmit plant viruses. <em>Brevipalpus</em>-transmitted viruses (BTVs) cannot infect systemically their hosts and rather are limited to local lesions where their mite vectors fed. BTVs can be further classified to BTV-C and BTV-N according to the where they replicate in the cytoplasm and nucleus, respectively. BTVs infect citrus, coffee, passion fruit, and ornamentals. Both BTV-C and BTV-N contain causal agents of citrus leprosis, a disease of economic importance for the American Citrus production and whose causal agents are quarantine pests for USDA-APHIS. Among the BTV-N causing citrus leprosis, orchid fleck virus (OFV) is a cosmopolitan virus whose orchid and citrus strains have been associated to the disease. In February 2020, citrus trees showing leprosis-like symptoms on leaves and stems were observed in an abandoned orchard on Hawaii Island. RT-PCR assays revealed the presence of a BTV-N using a universal BTV-N primer set and RNA extracted from rough lemon and mandarin trees. Sequencing show the identity of the BTV-N was OFV. The identity of OFV was corroborated by USDA-APHIS-CPHST laboratory and additionally the identity of the OFV was further determined to be the orchid strain 2 of OFV. An ongoing response plan is being implemented to eradicate this outbreak as a collaborative effort among the University of Hawaii, Hawaii Department of Agriculture and USDA-APHIS-PPQ. In 2019, viral-like symptoms resembling by those caused by BTV-C were observed in passion fruit in Honolulu. High throughput sequencing (HTS) on libraries constructed from dsRNA revealed the presence of citrus leprosis virus C2 (CiLV-C2) among other plant viruses found infecting passion fruit. The identity of the <em>Brevipalpus</em> mite transmitting CiLV-C2 was putatively determined to be <em>B. yothersi</em> using bean common as indicator hosts, 28S rDNA barcoding and RT-PCR. In 2018, viral-like symptoms were observed in papaya fruits only from papaya trees on the Hawaii Island. Symptoms differed by those caused by papaya ringspot virus (PRSV) on fruits, and PRSV-like symptoms on leaves were absent. HTS from a dsRNA library revealed the presence of abundant contigs showing low similarity, below 50% protein identity, to <em>Virgaviridae</em>, <em>Kitaviridae</em>, and Negeviruses members. Considering the similarity of contigs to <em>Kitaviridae</em> members, being BTV-C members, and common presence of <em>Brevipalpus</em> mites feeding on papaya fruits in Hawaii, <em>Brevipalpus</em> mites might represent a putative vector.</p><br /> <p> </p><br /> <p><strong>Adriana Larrea-Sarmiento/John Hu (University of Hawaii)</strong></p><br /> <p>The complete genomic sequence of a novel member of the family <em>Secoviridae</em> was determined by high-throughput sequencing (HTS) of a pineapple accession obtained from the National Plant Germplasm Repository (NPGR) in Hilo, Hawaii. The predicted genome of the putative virus was composed of two RNA molecules of 6,128 and 4,161 nucleotides in length, excluding the poly-A tails. Each genome segment contained one large open reading frame (ORF). BLASTx analysis of the two contigs showed that RNA1 shared 35% identity with dioscorea mosaic-associated virus of the proposed subgenus “<em>Cholivirus</em>” and strawberry mottle virus of the proposed subgenus “<em>Stramovirus</em>”, and RNA2 shared 26% identity with dioscorea mosaic associated virus and chocolate lily virus A of the proposed subgenus “Cholivirus”. These related viruses are currently classified as<em> Sadwavirus</em> members within the family Secoviridae. These results support the placement of this new virus as a putative member of the genus <em>Sadwavirus</em>, family <em>Secoviridae</em>. The name “pineapple secovirus A” (PSV-A) is proposed for this putative new virus infecting pineapple. Two sets of primers designed based on the HTS-derived sequences were used in tandem to detect the presence of PSVA in pineapple. The presence of this new virus in pineapple has been confirmed by RT-PCR and Sanger sequencing from six samples collected in Oahu-Hawaii. Additional testing carried out in China, Australia and pineapple accession samples from different countries retrieved from the USDA-Agricultural Research Service (USDA-ARS-NPGR) at the Daniel K. Inouye U.S. Pacific Basin Agricultural Research Center (PBARC) in Hilo suggests a cosmopolitan distribution of the new <em>Sadwavirus</em>. Two isometric virions particles (A1-A2) were reported in Australia back to 2002 infecting pineapple which suggests the presence of more sadwavirues infecting pineapple. These results support the placement of PSV-A as a putative member of the genus Sadwavirus, family Secoviridae. Further research is needed to identify the biological vector of PSVA, investigate whether PSVA is related to the two isometric viruses infecting pineapple in Australia, and determine if this putative new pineapple virus is involved in the etiology of mealybug wilt of pineapple (MWP).</p><br /> <p> </p><br /> <p><strong>Shulu Zhang (Agdia, Inc.)</strong></p><br /> <p>Early detection and effective control of plant pathogens is very important to prevent their widespread resulting in serious economic losses in crops like cherries, citruses, grapes, hops, peaches, or plums. Agdia utilizes a leading isothermal amplification technology called recombinase polymerase amplification (RPA) and has developed AmplifyRP® tests for rapid and accurate detection of nucleic acids from many plant pathogens. So far, Agdia has commercialized 23 AmplifyRP® kits including 21 AmplifyRP® kits specific to single species/strains of diverse pathogens and 2 AmplifyRP® Discovery kits suitable for any pathogen. Among the pathogen-specific kits, there are 7 kits in AmplifyRP® Acceler8® format, 11 kits in AmplifyRP® XRT format and 3 kits in AmplifyRP® XRT+ format. Eight AmplifyRP® XRT kits have been commercialized during the past 12 months and are specific to the following pathogens listed below: </p><br /> <div>Candidatus phytoplasma solani Bois Noir (BN)</div><br /> <div><em>Candidatus </em>phytoplasma vitis Flavescence doree (FD)</div><br /> <div><em>Fusarium oxysporum </em>f. sp. <em>cubense </em>Tropical Race 4 (Foc TR4)</div><br /> <div><em>Grapevine pinot gris virus </em>(GPGV)</div><br /> <div><em>Hop stunt viroid </em>(HSVd)</div><br /> <div><em>Rhodococcus </em>PBTS1 (R. PBTS-C & R. PBTS-P)</div><br /> <div><em>Rhodococcus </em>PBTS2 (R. PBTS-C & R. PBTS-P)</div><br /> <div><em>Tobacco rattle virus </em>(TRV)</div><br /> <div> </div><br /> <div> </div><br /> <div><strong>Marc F. Fuchs (Cornell University</strong><strong>, NY State report</strong>)</div><br /> <p>Grapevine red blotch virus (GRBV) from the genus <em>Grablovirus</em> in the family <em>Geminiviridae</em> is an emerging virus disease of grapevines (Cieniewicz et al. 2020b, Fuchs 2020). Limited information is available on the spread dynamics of GRBV in vineyards. We investigated red blotch disease progress in three vineyards with a disparate initial inoculum prevalence (Cieniewicz et al. 2019a). Secondary spread was documented in two vineyards in California but not in a vineyard in New York. Increase in annual disease incidence was unrelated to the estimated initial source of inoculum at planting but populations of <em>Spissistilus festinus</em> were absent in the New York vineyard, low in one of the two California vineyards and moderate in the second California vineyard. These results illustrated a differential disease progress in distinct vineyard ecosystems and suggested that GRBV spread dynamics in vineyards could be related to vector abundance (Cieniewicz et al. 2019a). Furthermore, middle-row vineyard cover crop samples collected from GRBV-infected California vineyards, particularly legume species which are preferred hosts of <em>S. festinus</em>, tested negative for GRBV, suggesting a minimal role, if any, as inoculum reservoirs for GRBV spread (Cieniewicz et al. 2019a). </p><br /> <p>No information is available on the genetic relatedness of <em>S. festinus</em> from vineyards in California and other crops in other regions of the United States. To studied the diversity of <em>S. festinus</em> populations, we collected specimens from various crops and geographic locations in the United States, and characterized fragments of the mitochondrial cytochrome C oxidase 1 (mt-COI) gene and the nuclear internal transcribed spacer 2 (ITS2) region by polymerase chain reaction and sequencing (Cieniewicz et al. 2020a). Maximum-likelihood and Bayesian analyses of the mt-COI and ITS2 sequences yielded similar phylogenetic tree topologies, revealing two distinct genetic <em>S. festinus</em> lineages with all of the specimens from California comprising one phylogenetic clade, alongside a single GenBank entry from Arizona, and all of specimens from the Southeastern United States comprising a statistically-supported distinct clade, regardless of host and year of collection. These results suggest the existence of two genotypes within <em>S. festinus</em> in the United States (Cieniewicz et al. 2020a). The only distinct morphological trait between the two genotypes was a less elevated pronotum in the representative specimens from California, compared to the representative specimens from the Southeastern United States. Since this phenotypic feature is inconspicuous, a diagnostic polymerase chain reaction targeting a variable region of the mt-COI fragment was developed to reliably distinguish between the specimens of the two genotypes of <em>S. festinus</em> and to facilitate their specific identification (Cieniewicz et al. 2020a. </p><br /> <p> </p><br /> <p><strong>Christie Almeyda (North Carolina State University)</strong></p><br /> <p>The Micropropagation and Repository Unit (MPRU) at North Carolina State University (NCSU) is currently one of the National Clean Plant Network (NCPN) centers that produces, maintains and distributes pathogen-tested G1 material of berry crops (strawberry, blackberry, raspberry and blueberry) and muscadine grapes to industry and researchers in the U.S. The MPRU presently conducts testing for targeted pathogens and therapy for pathogen elimination (heat treatment and meristem-tip culture) and maintains Fragaria, Rubus and Vaccinium G1 (foundation) blocks in vitro, in the greenhouse and the screenhouse. The same methods are applied for muscadine grapes. </p><br /> <p>In recent years, this facility has cleaned and tested mainly domestic materials from most of the berry breeding programs in the U.S. and muscadine grapes breeding programs from the Southeast. While cleaning up berry crops, the following viruses were detected on blueberries: Blueberry latent virus (BBLV) and Blueberry red ring spot virus (BRRV). Blackberry yellow vein-associated virus (BYVaV), Blackberry virus E (BlVE) and Citrus concave gum-associated virus (CCGaV-like) were detected on blackberries. Not long ago, the MPRU has established a partnership with the NC Plant Disease and Insect Clinic (PDIC). Now NC growers can submit berry and grape samples to be tested for designated pathogens at the MPRU as the unit has expanded its diagnostic services. </p><br /> <p>In partnership with Dr. Hoffmann (NCSU strawberry and grape extension specialist), the MPRU was able to collaborate with its diagnostics capacity for virus surveys on grapes. Various NC grower fields were tested to validate the establishment of molecular testing at the MPRU using protocols previously developed by Foundation Plant Services (FPS), UC-Davis in collaboration with Dr. Maher Al Rwahnih. The MPRU now has the capacity of testing for 10 pathogens affecting grapes using quantitative RT-PCR. Targeted pathogens were selected based on importance and prevalence in the region. The pathogens currently being tested are Grapevine leaf roll viruses (GLRaV-2, GLRaV-3, GLRaV-4, GLRaV-7), Grapevine red blotch virus (GRBV), Grapevine rupestris stem pitting associated virus (GRSPaV), vitiviruses (GVA, GVB), Tobacco Ring Spot Virus (TRSV), and Xyllela fastidiosa. Eighty samples were tested from 8 vineyards in NC (7 Vitis vinifera vineyards and 1 muscadine vineyard) in order to know the incidence of viral pathogens in this area. GLRaV-3, GRBV and Xyllela fastidiosa were detected during the second year of this survey. GLRaV-3 and GRBV were the most predominant (20/80 each). Only 6 samples were positive for Xyllela fastidiosa. The third and last year of the survey will define if there is a clear pattern of these pathogens occurring in NC. As we continue to collaborate with Dr. Hoffman in this survey, we are also working into cleaning and virus testing the material we currently have at the MPRU (10 muscadine cultivars) and new material (5 genotypes) we recently obtained from the AR breeding program.</p><br /> <p> </p><br /> <p><strong>Ruhui Li, USDA-ARS</strong></p><br /> <p>Blackcuurant revision virus was found in the Ribes germplasm of USDA-ARS. An improved method was developed for the detection for a citrus virus. Three different new viruses were identified from epiphyllum cactus. Collaborative Research projects include the following examples: Joseph Foster (USDA-APHIS-PGQP): Viruses infecting stone fruits and small fruits<strong>; </strong>Ekaterina Nikolaeva (Pennsylvania Department of Agriculture) and Kari Peter (Pennsylvania State University): Etiology of Rapid Apple Decline<strong>; </strong>Benjamin Gutierrez (USDA-ARS) and Margarita Bateman (USDA-APHIS): Viruses infecting fruit trees<strong>; </strong>Mengji Cao (Southwest University of China): Citrus and camellias<strong>; </strong>Liping Wu (Nachang University of China): Camellias<strong>; </strong>Luping Zheng (Fujian Agricultural and Forestry University) and Joseph Postman (USDA-ARS): <em>Ribes</em> spp. </p><br /> <p> </p><br /> <p><strong>Oscar Hurtado-Gonzales: (USDA-APHIS)</strong></p><br /> <div><strong><em>Pathogen Detection - </em></strong>Every year we detect pathogens in imported pomes. Pathogen detection is done using indicators plants, RT-PCRs, and the newly implemented high-throughput sequencing procedure. Up to 60% of the imported germplasm is detected to contain one or more plant virus, viroid, and/or phytoplasmas. RT-PCR-based detection now includes five recently identified plant pathogens in the past few years. These new pathogens include Apple Hammerhead Viroid (AHVd) found in apples, Citrus Virus A (CiVA) found in Pears and Quinces, Citrus Concave Gum-associated Virus (CCGaV) found in apples, Apple Luteovirus 1 (ALV1) found in apples, and Apple Rubbery Wood-associated Virus (ARWaV-1 and ARWaV-2) found in apples and pears. Altogether, RT-PCR pathogen detection includes <span style="text-decoration: underline;">six viroids</span>, <span style="text-decoration: underline;">nine viruses</span>, and <span style="text-decoration: underline;">one phytoplasmas test</span>. During 2019 we conducted about 960 bioassays corresponding to 47 unique accessions, 924 nucleic extractions corresponding to 274 unique accessions, and started the 3-4 year field indexing of 31 unique accessions (11 accessions in the Beltsville field and 20 in the Prosser field). During 2020 we expect to conduct all field indexing activities in Beltsville since the field operations have expanded to over 1000 field indicators.</div><br /> <div> </div><br /> <div><strong><em>Implementing HTS Technologies at PGQP - </em></strong>We have enhanced our diagnostic capabilities at PGQP by implementing the use of NGS technologies as a tool for routine plant pathogen detection. This technology is aiding in the detection of known and in some cases unknown “plant pathogens”. 2019 marks the period in which the PGQP Pomes program is releasing germplasm supported by HTS data.</div><br /> <div>Since the establishment of HTS in PGQP, the Pomes program has sequenced 76 unique imported accessions (54 apples, 70 pears, and 5 quinces) and over 40 different Malus, including rootstocks from various companies, weeps from nurseries, accessions maintained in the Malus repository, etc. These activities have supported studies such the phylogenetics of ALV-1, the first report of Citrus virus A in pears and quinces, the first report of Prunus Virus T in pears, among others. We will continue expanding our HTS activities this year by sequencing every accession prior to field indexing and on new importations.</div><br /> <div> </div><br /> <div><strong><em>Therapy - </em></strong>Heat treatments and tip grafting or tissue culture procedures are used to obtain pome and stone fruits free of pathogens. Moving all of the infected accessions through the therapy process efficiently has been the greatest challenge for these quarantine programs. Richard Slocum, Tissue Culture Scientist, continues to establish accessions in tissue culture in order to put them through therapy. In the past, he has made excellent progress with apples and pears. Richard also received training on cryotherapy during 2019 in Fort Collins, ARS. Cryotherapy has the potential to significantly reduce the therapy time (from several weeks to a few hours). Initial attempts to establish this technique has not been successful although additional attempts are in the pipeline. On the brighter side, the current thermotherapies and heat therapies appear to hold steady to free plants off the prevalent Apple Hammerhead Viroid. More tests are needed to confirm this with high confident. We currently have identified approximately over 40 different Malus accessions carrying AHVd alone or in combination with other viruses.<strong> </strong></div><br /> <p> </p><br /> <p><strong>Alexander Karasev (University of Idaho)</strong></p><br /> <p>Wine grape production in Idaho occurs on approximately 1,300 acres, predominately in Canyon County in the Southwest, and Nez Perce County in the Northwest. In the course of the GLRaV-3 testing of wine grapes in southern Idaho, plants of two grapevine cultivars were found to harbor a novel genetic variant of GLRaV-3, named ID45, which exhibited ≤80% nucleotide sequence identity level to the known GLRaV-3 isolates in its most conserved HSP70h gene. The ID45 variant caused no foliar symptoms in ‘Cabernet Sauvignon’ in the fall, and was demonstrated to have poor reactivity to commercial virus-specific antibodies. The entire 18,478-nt genome sequence of the GLRaV-3-ID45 was determined using a combination of high-throughput and conventional Sanger sequencing, and demonstrated to have typical organization for the genus <em>Ampelovirus</em> (family <em>Closteroviridae</em>), with only 70 to 77% identity level to the GLRaV-3 genomes from other established phylogroups. We concluded that ID45 represented a new phylogenetic group IX of GLRaV-3. Database search using ID45 nucleotide sequence as a query suggested that this novel ID45 variant is present in at least one other grape-growing state in the U.S., in California, and in Brazil. An RT-PCR based test was developed to distinguish ID45 from the predominant, GLRaV-3 phylogroup I found in Idaho in single and mixed infections. In September of 2014-2015, a survey of wine grapes was conducted in Canyon and Nez Perce counties of Idaho for the presence of GRBV. Three grapevines were found positive by PCR producing the DNA fragment of expected size, 720-bp; all three positive samples came from a single vineyard in Canyon county, from the same wine grape cultivar, Syrah. To expand the GRBV survey, 434 random grapevine samples collected in 2009-2011 in 14 vineyards in Canyon, Elmore, Ada, and Nez Perce counties were re-analyzed for the presence of GRBV; six additional GRBV-positive samples coming from two additional vineyards (Canyon county) and two additional grapevine cultivars, Merlot and Petite Sirah, were identified by PCR. The five whole genomes for these GRBV isolates were sequenced in DNA plasmids by Sanger methodology, and subjected to phylogenetic analysis. All whole genomes sequenced were assigned to clade 2 of GRBV, most closely related to a group of GRBV isolates from Washington state. This phylogeny of the Idaho GRBV isolates suggests the introduction of GRBV to Idaho from the same infected source, probably through infected planting material. </p><br /> <p> </p><br /> <p><strong>Ekaterina Nikolaeva (Pennsylvania </strong><strong>D</strong><strong>e</strong><strong>p</strong><strong>a</strong><strong>rtment of Agriculture)</strong></p><br /> <p>Pennsylvania Department of Agriculture in cooperation with Penn State University conducted 2019 PPA 7721 funded surveys for exotic diseases in orchards and small fruits. Orchard survey targets included Potyvirus Plum pox virus, Asian Pear Blight (<em>Erwinia pyrifoliae</em>), Asiatic brown rot (<em>Monilia polystroma</em>), Apple brown rot (<em>Monilinia fructigena</em>), Apple Proliferation (<em>Candidatus</em> Phytoplasma mali), European stone fruit yellow (<em>Ca.</em> Phytoplasma prunorums), and Almond witches’ broom (<em>Ca.</em> Phytoplasma phoenicium). Small fruit survey targeted Asian pear blight (<em>Erwinia pyrifolia</em>), Nepovirus Tomato black ring virus, Australian Grapevine Yellows (<em>Ca</em>. Phytoplasma australiense), Flavescence Doreé Phytoplasma (<em>Ca.</em> Phytoplasma vitis), and Bois noir Phytoplasma (<em>Ca</em>. Phytoplasma solani). No exotic targets were detected, but we did confirm presence of <em>Ca.</em> Phytoplasma pyri (16SrX, Apple Proliferation group) in plum tree.</p><br /> <p>Data gathering were continued a Rapid Apple Decline syndrome. In 2019, PDA and PSU team visited 34 apple orchard blocks in 17 PA farms located in Adams, Bedford, Berks, Columbia, Franklin, Luzerne, and Northumberland counties. The orchards were inspected for the symptoms and signs of Rapid Apple Decline (RAD). The main characteristics included presence of dead and declining young dwarf trees, wilting, unseasonal tree discoloration and defoliation, dark brown cankers around graft union, and presence of green suckers. A total of 993 samples was tested in PDA lab for presence of Apple luteovirus 1 (ALV1) via conventional RT PCR. More than 200 samples within the same group were also tested for the presence of other viruses known to infect apple trees, including apple chlorotic leaf spot virus (ACLSV), apple stem grooving virus (ASGV) apple stem pitting virus (ASPV), tomato ringspot virus (ToRSV), and apple mosaic virus (ApMV). In result, 30% tested trees were found positive for ALV-1, 19.6% for ToRSV, 8.9% for ApMV, 14.1% for ACLSV, 54.3% for ASGV and 25.2% for ASPV. Similar to previous year results, ALV1 was detected on a wide range of apple varieties, including Aztec Fuji, Buckeye Gala, Crimson Crisp, Fuji, Gala, Golden Delicious, Honeycrisp, and Jonagold. Most of the ALV1 positive trees (97%) were grafted on M9 rootstocks while only few positive trees were found on Bud 9 (1.5%), Bud 10 (1.5%) and G11 (1.1%). A total of 57 samples (total RNAs) was subjected to Illuminia RNA sequencing by USDA ARS lab. RNA reads of the samples were <em>de novo </em>assembled and resulted contigs were blasted against three local databases retrieved from NCBI GenBank. Four known apple viruses, including ACLSV, ALV1, ASGV and ASPV were detected in the samples in different combinations. A new virus (<em>Bunyaviridae</em>), Citrus concave gum-associated virus (CCGaV), was also identified in the majority of the samples. The genetic diversities were found very low among ALV1 and CCGaV isolates, while high genetic variations occurred among three latent viruses, especially ASPV. The work on determination of ALV1 pathogenicity was started in August 2018 and continued in 2019. Three hundred M9 rootstocks (not grafted) trees from different suppliers were evaluated for the presence of ALV1. ALV1 positive rootstocks were used for grafting with ALV1 negative budwoods. In July 2019, we revisited grafted trees to evaluate virus transmission to the scion of the grafted trees and possible symptom development. In result, ALV1 was detected in 52.8% scions of grafted trees. That confirmed USDA ARS data that ALV1 can be transmitted from rootstocks to the budwood through graft union.</p><br /> <p>PDA continues to operate the Fruit Tree Improvement Program (FTIP), a specialized virus-tested fruit tree certification program. Three nurseries have been participating in the FTIP last year. Over 3,500 samples were tested for viruses of concern, including Prunus Necrotic Ringspot Virus (PNRSV), Prune Dwarf Virus (PDV), Tomato Ringspot Virus (ToRSV), and Plum Pox Virus (PPV). No PPV was detected in rootstock blocks or in registered source blocks. PNRSV (2.4%) remains the most commonly found virus in Prunus in PA nurseries. The occurrence of PDV (0.6%) and ToRSV (0.2%) in registered blocks and nursery production blocks remain low. All blocks met virus-testing requirements for FTIP certification.</p><br /> <p> </p><br /> <p><strong>Scott J. Harper (Washington State University)</strong></p><br /> <p>The Harper lab is one of the major participants in the Washington & Oregon Little cherry disease task force, providing the pathology component in collaboration with entomology, horticulture and extension researchers to understand the pathology and etiology of this disease. The Clean Plant Center Northwest (CPCNW) has been continuing the previous year’s collaboration with Foundation Plant Services (FPS) at UC Davis, on the identification of pathogens present in diseases of unknown etiology. The CPCNW has been active in protecting US agriculture from harmful pests and diseases, and during the reporting period processed and released a total of 59 pome and stone fruit, 3 grapevine, and 7 hop virus-tested cultivars to industry.</p><br /> <p> </p><br /> <p><strong>Lauri Guerra</strong><strong> (WA Department of Agriculture)</strong></p><br /> <p><strong><em>Regular Testing of Registered Prunus trees</em></strong> - Normally we test every 3 years all plants by Elisa for ILAR, CLRV and PPV and on the other 2 years we test half in the lab for Ilar and CLRV by Elisa and the other half in the field by Shirofugen indexing.</p><br /> <p><strong><em>Testing scheme for G2 trees when sourcing G3 trees</em></strong> - One round of greenhouse bio-indexing (dormant material) and one round of lab testing (actively growing material), using PCR or qPCR when available - positives confirmed by sequencing. All testing would be completed before trees are ready to transplant to Registered blocks. If a positive is found, nurserymen can propagate from another source, missing one year only, instead of 3 years, as before.Before we would run twice in the greenhouse, skipping a year. Indicators used for Greenhouse indexing. Malus and Pyrus - Russian, Radiant, Spy 227, Micromalus, Virginia Crab, Nouveau Poiteau, Lord Lambourne (experimenting also Geneva 16 to verify its usefullnes as an indicator), Prunus - Bing, Sam, Kwanzan, Canindex, GF-305, Tilton, Shiro, Tomentosa.</p><br /> <p><em><strong>Nepovirus vectors survey</strong></em> - Finished testing all registered blocks for the presence of nepovirus vectors (2293 soil samples – 20 cores per samples, totaling ~45,000 cores). The only vector nematode identified in some blocks was Meloidogyne rivesi. A proposal was presented and approved for Farm Bill funding, to identify on these sites if there is the presence of ToRSV, TRSV and CRLV.</p><br /> <p> </p><br /> <p><strong>Arunabha Mitra/</strong><strong>Naidu A. Rayapati (Washington State University)</strong></p><br /> <p>Viral diseases are one of the significant concerns to sustainability of the grape and wine industry in Washington State that contributes an estimated $6 billion to the State’s economy. Grapevine leafroll disease (GLD) continues to be the most insidious and widely distributed in Washington vineyards compared to other viral diseases. Vineyard surveys have indicated the occurrence of Grapevine leafroll-associated virus 1 (GLRaV-1), GLRaV-2, GLRaV-3 and GLRaV-4 in Washington vineyards, with GLRaV-3 being the most predominant and economically important than other GLRaVs. During the past few years, we have been studying the genetic diversity of GLRaVs for a better understanding of their role in GLD epidemiology and to implement robust strategies for management of the disease in vineyards. In previous studies, we have reported the presence of molecularly divergent isolates of GLRaV-1 and GLRaV-2 in vineyards (Phytopathology 100 [2010]: 698-707 and Phytopathology 101 [2011]:1446-1456). In recent years, we have extended these studies to examine the molecular variability of natural populations of GLRaV-3 and GLRaV-4. Total RNA preparations were made from representative samples collected from wine grape cultivars that tested positive for GLRaV-3 and subjected to high-throughput sequencing (HTS) to generate near-complete viral genome sequences. A global phylogenetic analysis of these sequences revealed the presence of distinct variants of GLRaV-3 in Washington vineyards that aligned with six genetic variant groups, designated as I, II, III, V, VI, and IX, previously reported from other grapevine-growing regions. Among them, GLRaV-3 isolates belonging to variant group I were found to be predominant in Washington vineyards. Using a combination of HTS and Sanger sequencing, the complete genome of three strains of GLRaV-4 (strain 4, strain 5 and strain 9) was determined to be 13,824 nucleotides (nt), 13,820 nt and 13,850 nt, respectively. An analysis of their genome sequences in comparison with GLRaV-4 strains (strain 4, strain 5, strain 6, strain 9, strain Pr, strain Car, and strain Ob) reported from different grapevine-growing regions revealed intraspecies recombination among a few strains of the virus. Overall, these results expanded our current understanding of the genetic diversity of GLRaVs and provided a foundation to gain insights into the epidemiology of GLD for implementing sustainable disease management strategies in vineyards</p><br /> <p> </p><br /> <p><strong>Cindy Cooper (WA Department of Agriculture)</strong></p><br /> <p><strong><em> “Harmonizing State Certification and Quarantine Programs, presented by members of the National Planting Stock Certification Standards Working Group”</em></strong></p><br /> <p>The National Planting Stock Certification Standards Working Group was created in 2017, with a grant from APHIS, to create an ongoing opportunity to communicate with USDA on issues effecting state level certification programs and plant movement. The goals of the group are:</p><br /> <ol><br /> <li>Communication between state regulators, researchers, and federal counterparts, to improve and promote state level pathogen-tested planting stock certification programs;</li><br /> <li>To work toward understanding and harmonizing program standards between states;</li><br /> <li>Collaborate on rewriting and standardizing the national model certification standards developed for NCPN crops by the tier 2 committees;</li><br /> <li>Mentor emerging state certification programs through sharing combined group knowledge.</li><br /> </ol><br /> <p>We hope to become a permanent committee within the National Plant Board, or another established parent group, and will seek on-going funding to facilitation and face-to-face meetings.</p><br /> <p>Regulators from Washington, Oregon, California, Michigan, Pennsylvania and New York are currently participating, and we invite regulators from other states to join us.</p><br /> <p>The group has identified the common elements included in the national model standards for certification, such as definitions of the limited generation scheme, acceptable G1 sources, site selection criteria, isolation distances, frequency and timing of inspections, sampling and testing, and a list of diseases for which planting stock is monitored under certification. We are conducting a gap analysis comparing state program standards to the national model standard and identifying missing or disparate content, with the goal of harmonizing wherever possible.</p><br /> <p>Each participating state gave an overview of established certification programs they maintain, crop programs that have become inactive, and new crops for which rules are being established. WA, OR, CA, PA and NY have active fruit tree certification programs. NY, WA, OR, CA and MI have active grapevine programs. Several states are developing rules for blueberry and hop certification. Funding for certification programs and lab testing varies from state to state, with some having established assessments on sales of planting stock to draw from, though most state programs are fee-for-service, paid for by the participating nurseries. Likewise, lab capacity is varied, with some states utilizing university labs to conduct molecular testing. All six states utilize molecular testing, but none currently have High Throughput Sequencing capacity. Only Washington and California continue to conduct field or greenhouse bio indexing as part of their testing regime. State regulators feel HTS it is an important tool for diagnostics and support its development for use by clean plant centers.</p><br /> <p>State Departments of Agriculture utilize the clean plant centers as a designated source of G1 material for certification programs. Clean plant centers also provide testing services and will clean up material that nurseries want to bring into a certification program.</p><br /> <p>The National Clean Plant Network (NCPN) is a program consisting of plant pathogen ‘clean plant’ services by clean plant centers to 7 crop taxa; including fruit trees (stone and pome fruits), grapes, hops, berries (primarily <em>Fragaria, Rubus,</em> and <em>Vaccinium</em>) citrus, roses, and sweet potato. The stated purpose of the program indicates that NCPN is “… a ‘Network’ of clean plant centers and allied programs; located at universities, U.S. gov’t agencies, and non-profit entities; with the mission of diagnosing plant pathogens in ‘mother’ (nuclear stock) plants; and in applying therapeutics; to ‘clean’ these plants and maintain them in foundations; in preparation for their acquisition and increase and use by industry; and to engage in program governance and special initiatives.</p><br /> <p>The program is administratively nested in USDA. It was established by the Farm Bill of 2008 as an experimental initiative. Under the Farm Bill of 2014, NCPN was reauthorized, made permanent, given a baseline funding of not less than $5.0 million annually, and given a reaffirmation of the programs purposes.</p><br /> <p>NCPNs Special Initiatives represent an exciting venue under which program participants engage across tradition specialty crop lines to engage with each other in advancing the Network and their own centers organizationally and administratively. 5 special initiatives were highlighted this year at WERA-20 including:</p><br /> <div>NCPN Strategic Planning:</div><br /> <ul><br /> <li><br /> <div>Updating an NCPN Plan for 2020-2024.</div><br /> </li><br /> <li><br /> <div>The new plans intention is to update, validate, and circumscribe the Network.</div><br /> </li><br /> <li><br /> <div>The plan has 3 goals; program operations, governance, and special initiatives.</div><br /> </li><br /> <li><br /> <div>Program values are also highlighted, including quality, service, connectivity, empowerment, and sustainability.</div><br /> </li><br /> <li><br /> <div>The plan also highlighted significant other special initiatives that could be pursued by NCPN such as international collaboration, succession management, and focus on critical issues.</div><br /> </li><br /> <li><br /> <div>The draft is in final form ready for broader review by members.</div><br /> </li><br /> </ul><br /> <p>NCPN Education/Outreach/Communications:</p><br /> <ul><br /> <li>A team of about 60 persons representing NCPNs first special initiative.</li><br /> <li>The team produces NCPN outreach tools such as brochures, websites, newsletters, and various fact sheets and has been vital to advancing the visibility and stature of the program.</li><br /> <li>NCPN currently has a series of new Communications Plans developed by its members in a series of meetings in Portland, OR and Sacramento, CA. The plans are being reviewed by NCPN to ascertain how best to implement them. Core to the plans is a recommendation for NCPN to establish a formal communications Directorate within the program.</li><br /> </ul><br /> <p>NCPN Economics Initiative:</p><br /> <ul><br /> <li>A newly formed team of about 40 members led for NCPN by Cornell Univ. economists and meeting at that location last year to formally launch the program.</li><br /> <li>The team and its associated leadership are assessing existing clean plant program economic studies, analyzing gaps in knowledge, and planning a roadmap forward.</li><br /> <li>The NCPN Governing Board has provided the team with funding to support added economic studies as identified by the members.</li><br /> </ul><br /> <p> NCPN Quality Initiative:</p><br /> <ul><br /> <li>A newly formed team of about 40 members led for NCPN by the University of Riverside in collaboration with select leaders from the National Plant Diagnostic Network and USDA.</li><br /> <li>Meetings to establish program direction were recently held at Riverside, CA with an added training of NCPN technicians in select principles of quality being conducted at the USDA/APHIS/PPQ laboratory in Beltsville, MD.</li><br /> <li>The team is looking at quality in all aspects of NCPN, including scientific laboratories, governance, recordkeeping, program foundations, and communications.</li><br /> <li>Select progress includes the establishment of an NCPN Quality Steering Committee, a team to initiate a draft of laboratory Quality Standards, proposals for added clean plant center staff training, and NCPN support for Quality Managers at clean plant centers.</li><br /> </ul><br /> <p> NCPN Scientific Information Sharing and Hight Throughput Sequencing (HTS):</p><br /> <ul><br /> <li>NCPN continues to support the gathering and work of scientists interested in sharing information about diagnostics and new technologies, such as HTS.</li><br /> <li>Of particular interest to NCPN is the establishment of linkages between NCPN clean plant centers and Federal/State regulators gathering to discuss efficiencies that might allow for the smoother and more rapid movement of clean plant material.</li><br /> </ul><br /> <p>The Network also reported-out on critical and emerging issues that it’s facing in FY 2020 including the impacts of COVID-19 on NCPN (such as the need to extend or adjust existing clean plant center agreements for an added year to compensate for activity changes or slowdowns; program exploration into new governance, networking, and organizational paradigms; continue efforts to accurately circumscribe the programmatic boundaries of NCPN; NCPN foundations and how they might change over time under pressure from vectors of pathogens; and new crops / new centers and criteria for their entry into the Network.</p>Publications
<p>Adiputra, J., Jarugula, S. and Naidu, R.A. 2019. Intra-species recombination among strains of the ampelovirus Grapevine leafroll-associated virus 4. Virology Journal 16: 139.</p><br /> <p>Al Rwahnih, M., Alabi, O.J., Hwang, M.S., Stevens, K. and Golino, D., 2019. Identification and genomic characterization of grapevine Kizil Sapak virus, a novel grapevine-infecting member of the family Betaflexiviridae. Archives of Virology, 164(12), pp.3145-3149.</p><br /> <p>Alabi, O.J., Gaytan, B.C., Al Rwahnih, M., Villegas, C., 2020. A description of the possible etiology of the cilantro yellow blotch disease. Plant Disease. <a href="https://doi.org/10.1094/PDIS-09-19-1958-SC">https://doi.org/10.1094/PDIS-09-19-1958-SC</a></p><br /> <p>Appel, D.N., Alabi, O., McBride, S.A., Al Rwahnih, M. and Pontasch, F., 2019. The Incidence of Grapevine Viruses in Four Texas Blanc du Bois Vineyards. Plant Health 2019.</p><br /> <p>Beaver-Kanuya, E., & Harper, S. J. (2019). Detection and quantification of four viruses in Prunus pollen: Implications for biosecurity. Journal of virological methods, 271, 113673.</p><br /> <p>Beaver-Kanuya, E., & Harper, S. J. (2020). Development of RT-qPCR assays for the detection of three latent viruses of pome. Journal of Virological Methods, 278, 113836.</p><br /> <p>Bennypaul, H., I. Abdullahi, M. W. Harding, and C. Neeser (2019). First Detection of Wheat streak mosaic virus in Two Perennial Weed Species, Agropyron cristatumand Hordeum jubatum subsp. intermedium, in Canada. Plant Disease 2019 103:6, 1441-1441</p><br /> <p>Bennypaul, H., I. Abdullahi, M. W. Harding, and R. Aboukhaddour (2019). First Detection of European Isolates of Wheat streak mosaic virus in Canada. Plant Disease 2019 103:6, 1442-1442</p><br /> <p>Brewer, E., Cao, M., Gutierrez, B.L., Bateman, M., Li, R. 2020. Discovery and molecular characterization of a novel trichovirus infecting sweet cherry. Virus Genes. <a href="https://doi.org/10.1007/s11262-020-01743-7">https://doi.org/10.1007/s11262-020-01743-7</a>.</p><br /> <p>Britt, K., Gebben, S., Levy, A., Al Rwahnih, M., Batuman, O., 2020. The Detection and Surveillance of Asian Citrus Psyllid (Diaphorina citri)-Associated Viruses in Florida Citrus Groves. Frontiers in Plant Science, 10: 1687. <a href="https://doi.org/10.3389/fpls.2019.01687">https://doi.org/10.3389/fpls.2019.01687</a></p><br /> <p>Chingandu, N., Jarugula, S., Movva, A. and Naidu, R.A. 2020. “The absence of grapevine red blotch virus in Washington’s certified grapevine nurseries” at the Washington Winegrowers Association Annual Meeting, Convention & Trade Show, March 2-5, 2020, Kennewick, WA.</p><br /> <p>Cieniewicz, E., Flasco, M., Brunelli, M., Onwumelu A., Wise, A. and Fuchs, M.F. 2019a. Differential spread of grapevine red blotch virus in California and New York vineyards. Phytobiomes Journal, 3:203-211.</p><br /> <p>Cieniewicz, E., Poplaski, V., Brunelli, M., Dombroswkie, J. and Fuchs, M. 2020a. Two distinct Spissistilus festinus genotypes in the United States revealed by phylogenetic and morphological analyses. Insects, 11:80; DOI:10.3390/INSECTS11020080.</p><br /> <p>Cieniewicz, E., Wise, A., Smith, R., Cooper, M, Martinson, T. and Fuchs, M. 2019b. Studies on red blotch ecology inform disease management recommendations. Wine Business Monthly, March issue, pp. 92-102.</p><br /> <p>Cieniewicz, E.J., Qiu, W., Saldarelli, P., Fuchs, M. 2020b. Seeing is believing: Lessons from emerging viruses in grapevine. Journal of Plant Pathology, <a href="https://doi.org/10.1007/s42161-019-00484-3">https://doi.org/10.1007/s42161-019-00484-3</a>.</p><br /> <p>Davenport B., Groth-Helms D., Li R., Zhang S. (2019): Development of a real-time duplex isothermal assay for the detection of Tobacco rattle virus and an endogenous internal RNA control in ornamental hosts. IX International Symposium on New Ornamental Crops. September 30 - October 3, 2019, Guadalajara, Mexico.</p><br /> <p>Davenport B., Li R., Zhang S. (2019): Isothermal detection for Dickeya and Clavibacter michigenesis subsp. sepedonicus, two prominent potato tuber pathogens. Australasian Plant Pathological Society Meeting, November 25–28, 2019, Melbourne, Victoria, Australia.</p><br /> <p>Diaz-Lara, A., Brisbane, R.S., Aram, K., Golino, D. and Al Rwahnih, M., 2019. Detection of new vitiviruses infecting grapevine in California. Archives of virology, 164(10), pp.2573-2580.</p><br /> <p>Diaz-Lara, A., Golino, D., Preece, J.E. and Al Rwahnih, M., 2020. Development of RT-PCR degenerate primers to overcome the high genetic diversity of grapevine virus T. Journal of Virological Methods, p.113883.</p><br /> <p>Diaz-Lara, A., Klaassen, V., Rowhani, A., Stevens, K., Hwang, M., Golino, D.A. and Al Rwahnih, M., 2019. Comparison of newly developed ELISA and RT-PCR assays for the detection of all known genetically diverse variants of GLRaV-3. Plant Health 2019.</p><br /> <p>Diaz-Lara, A., Klaassen, V., Stevens, K., Hwang, M., Golino, D.A. and Al Rwahnih, M., 2019. Improved detection of fruit tree viruses and viroids by real-time quantitative PCR. Plant Health 2019.</p><br /> <p>Diaz-Lara, A., Martin, R.R., Al Rwahnih, M., Vargas, O.L. and Rebollar-Alviter, Á., 2020. First evidence of viruses infecting berries in Mexico. Journal of Plant Pathology, 102(1), pp.183-189.</p><br /> <p>Diaz-Lara, A., Navarro, B., Di Serio, F., Stevens, K., Hwang, M.S., Kohl, J., Vu, S.T., Falk, B.W., Golino, D. and Al Rwahnih, M., 2019. Two Novel Negative-Sense RNA Viruses Infecting Grapevine Are Members of a Newly Proposed Genus within the Family Phenuiviridae. Viruses, 11(8), p.685.</p><br /> <p>Diaz-Lara, A., Stevens, K., Klaassen, V., Golino, D. and Al Rwahnih, M., 2020. Comprehensive Real-Time RT-PCR Assays for the Detection of Fifteen Viruses Infecting Prunus spp. Plants, 9(2), p.273.</p><br /> <p>Druciarek, T., Lewandowski, M. and Tzanetakis I.E. 2019. A new, sensitive and efficient method for taxonomic placement in the Eriophyoidea and virus detection in individual eriophyoids. Experimental and Applied Acarology 78: 247-261.</p><br /> <p>Fuchs, M. 2020. Grapevine red blotch virus. In: Invasive Species Compendium and Crop Protection Compendium, CABI International, Wallingford, Oxfordshire, United Kingdom, in press.</p><br /> <p>Fuchs, M. 2020. Grapevine viruses: A multitude of diverse species with simple but poorly adopted management solutions in the vineyard. Journal of Plant Pathology, in press.</p><br /> <p>Green, J.C., Rwahnih, M.A., Olmedo-Velarde, A., Melzer, M.J., Hamim, I., Borth, W.B., Brower, T.M., Wall, M. and Hu, J.S., 2020. Further genomic characterization of pineapple mealybug wilt-associated viruses using high-throughput sequencing. Tropical Plant Pathology 45:64-72.</p><br /> <p>Hadaway, K., Kogan, C., Jarugula, S. and Naidu, R.A. 2019. “Elucidating differences in red leaf symptoms produced by biotic and abiotic stresses in grapevines” at the IEEE Women in Engineering Leadership Summit, July 30, 2019, Richland, WA (Received best poster award)</p><br /> <p>Hamim, I. Wayne B. Borth · Michael J. Melzer · Jon Y. Suzuki · Marisa M. Wall,· John S. Hu 2019. Occurrence of tomato leaf curl Bangladesh virus and associated subviral DNA molecules in papaya in Bangladesh: molecular detection and characterization. Archives of Virology 164:1661-1665</p><br /> <p>Hamim, I., Al Rwahnih, M., Borth, W.B., Suzuki, J.Y., Melzer, M.J., Wall, M.M., Green, J.C., Hu, J.S., 2019. Papaya Ringspot Virus Isolates from Papaya in Bangladesh: Detection, Characterization, and Distribution. Plant Disease, 103(11): 2920-2924.</p><br /> <p>Hamim, I., Maher Al Rwahnih, Wayne B. Borth, Jon Y. Suzuki, Michael J. Melzer, Marisa M. Wall, James C. Green, and John S. Hu 2019 Papaya ringspot virus isolates from papaya in Bangladesh: detection, characterization and distribution. Plant Disease 103:2920-2924.</p><br /> <p>Hoffmann M, Talton W, Nita M, Jones T, Al Rwahnih M, Sudarshana MR, and Almeyda C. 2020. First Report of Grapevine red blotch virus, the Causal Agent of Grapevine Red Blotch Disease, in Vitis vinifera in North Carolina. PDIS-07-19-1539-PDN.</p><br /> <p>Hoffmann, M., Talton, W., Nita, M., Jones, T.J., Al Rwahnih, M., Sudarshana, M.R. and Almeyda, C.V., 2019. First Report of Grapevine red blotch virus, the causal agent of Grapevine Red Blotch Disease in Vitis vinifera in North Carolina. Plant Disease, (ja).</p><br /> <p>James D., Phelan, J., Sanderson, D. (2019). Detection by high throughput sequencing and molecular characterization of complexes of fabviruses infecting Staccato® sweet cherry (Prunus aviam) in Canada. Canadian Journal of Plant Pathology.</p><br /> <p>Jarugula, S., Adegbola, R., Mitra, A., Chingandu, N., Sekhar, T., Swamy, P., Bagewadi, B. and Naidu, R.A. 2020. “Rogueing symptomatic vines for controlling viral diseases in vineyards” at the Washington Winegrowers Association Annual Meeting, Convention & Trade Show, March 2-5, 2020, Kennewick, WA. (Poster presentation received First place under the People Choice and 3rd place under Professional Category).</p><br /> <p>Katsiani, A., Stainton, D., Lamour, K. and Tzanetakis, I.E. 2020. The population structure of Rose rosette virus in the United States. Journal of General Virology 101, in press</p><br /> <p>Lan, P., Tian, T., Pu, L., Rao, W., Li, F., Li, R. 2019. Characterization and detection of a new badnavirus infecting Epiphyllum spp. Archives of Virology. <a href="https://doi.org/10.1007/s00705-019-04237-6">https://doi.org/10.1007/s00705-019-04237-6</a>.</p><br /> <p>Larrea-Sarmiento, A., Alejandro Olmedo-Velarde, James C. Green, Maher Al Rwahnih, Xupeng Wang, Yun‑He Li, Weihuai Wu, Jingxin Zhang, Tracie Matsumoto Brower, Marisa Wall and John S. Hu 2020, Identification and complete genomic sequence of a novel sadwavirus discovered in pineapple (Ananas comosus) Archives of Virology <a href="https://doi.org/10.1007/s00705-020-04592-9">https://doi.org/10.1007/s00705-020-04592-9</a></p><br /> <p>Larrea-Sarmiento, A., X. Wang, W. B. Borth, R. P. Barone, A. Olmedo-Velarde, M. J. Melzer, J. S. K. Sugano, R. Galanti, J. Y. Suzuki, M. M. Wall, and J. S. Hu 2019 First report of bean common mosaic virus infecting flowering ginger (Alpinia purpurata) in Hawaiʻi. Plant Disease <a href="https://doi.org/10.1094/PDIS-06-19-1264-PDN">https://doi.org/10.1094/PDIS-06-19-1264-PDN</a></p><br /> <p>Li R., Davenport B., Zhang S., Schuetz K., Bai T.T., Fu A.G., Zheng S.J. (2020): Development of a simple, rapid, and sensitive AmplifyRP isothermal assay for detection of Fusarium oxysporum f. sp. cubense Tropical Race 4. Plant Health 2020, Denver, Colorado, USA.</p><br /> <p>Li R., Davenport B., Zhang S., Schuetz K., Ling K.S. (2020): Development of a triplex AmplifyRP molecular assay for a reliable detection of Tomato brown rugose fruit virus. Plant Health 2020, Denver, Colorado, USA.</p><br /> <p>Liu, H., Wu, L., Zheng, L., Cao, M., Li, R. 2019. Characterization of three new viruses of the family Betaflexiviridae associated with camellia ringspot disease. Virus Research. https://doi.org/10.1016/j.virusres.2019.197668.</p><br /> <p>Liu, Q., Xuan, Z., Wu, Y., Li, M., Zhang, S., Wu, D., Li, R., Cao, M. 2019. Loquat is a new natural host of apple stem grooving virus and apple chlorotic leaf spot virus. Plant Disease. https://doi.org/10.1094/PDIS-04-19-0721-PDN.</p><br /> <p>Maree, H.J., Blouin, A.G., Diaz-Lara, A., Mostert, I., Al Rwahnih, M. and Candresse, T., 2020. Status of the current vitivirus taxonomy. Archives of Virology, 165(2), pp.451-458.</p><br /> <p>Martínez-Lüscher, J., Plank, C.M., Brillante, L., Cooper, M.L., Smith, R.J., Al-Rwahnih, M., Yu, R., Oberholster, A., Girardello, R. and Kurtural, S.K., 2019. Grapevine red blotch virus may reduce carbon translocation leading to impaired grape berry ripening. Journal of agricultural and food chemistry, 67(9), pp.2437-2448.</p><br /> <p>Mitra, A., Jarugula, S., Donda, B., Jordan, E. and Naidu, R.A. 2019. “Elucidating the genetic diversity of Grapevine leafroll-associated virus 3 for managing grapevine leafroll disease in vineyards” at the Northwest Center for Small Fruits Research 2019. December 2-4, 2019, Ferndale, WA.</p><br /> <p>Mitra, A., Jarugula, S., Donda, B., Jordan, E. and Naidu, R.A. 2019. “Genetic diversity of Grapevine leafroll-associated virus 3 in Washington State vineyards” at the 2019 American Phytopathological Society Annual Meeting, August 3-7, 2019, Cleveland, OH. (Received “Phytobiomes Student Poster Award” from the scientific journal ‘Phytobiomes’ at the American Phytopathological Society).</p><br /> <p>Naidu, R.A. “Status of Grape Viruses in Washington: Update on Research Progress” and “Virus Testing: How to sample, benefits of testing, interpreting results, economics of testing” at the Washington Advancements in Viticulture and Enology annual research seminar. February 19, 2020, Prosser, WA.</p><br /> <p>Nikolaeva E.V., Knier R., Molnar C., Peter K., Jones T., and Costanzo S. 2019. First Report of Strawberry (Fragaria × ananassa) as a Host of a ‘Candidatus Phytoplasma americanum’-Related Strain in the United States. Plant Disease V. 104, N 2. P.560.</p><br /> <p>Olmedo-Velarde Alejandro, Adam C. Park, Jari Sugano, Janice Y. Uchida, Michael Kawate, Wayne B. Borth, John S. Hu, and Michael J. Melzer 2019. Characterization of Ti ringspot-associated virus, a novel emaravirus associated with an emerging ringspot disease of Cordyline fruticosa (L.) Plant Disease <a href="https://doi.org/10.1094/PDIS-09-18-1513-RE">https://doi.org/10.1094/PDIS-09-18-1513-RE</a></p><br /> <p>Olmedo-Velarde, A., Roy, A., Belanger, C.A., Watanabe, S., Hamasaki, R.T., Mavrodieva, V.A., Nakhla, M.K., Melzer, M.J. (2019). First Report of Tomato Chlorotic Dwarf Viroid Infecting Greenhouse Tomato in Hawaii. Plant Disease 103(5): 1049.</p><br /> <p>Pechinger, K., Chooi, K. M., MacDiarmid, R. M., Harper, S. J., & Ziebell, H. (2019). A new era for mild strain cross-protection. Viruses, 11(7), 670.</p><br /> <p>Peng, L., Wu, L., Grinstead, S.C., Kinard, G.R., Li, R. 2019. Molecular characterization and detection of two novel carlaviruses infecting cactus. Archives of Virology. <a href="https://doi.org/10.1007/s00705-019-04279-w">https://doi.org/10.1007/s00705-019-04279-w</a>.</p><br /> <p>Sanderson, D., & James, D. (2019). Analysis of the genetic diversity of genome sequences of variants of apple hammerhead viroid. Canadian Journal of Plant Pathology.</p><br /> <p>Thekke-Veetil, T., Ho, T., Postman, J. D., and Tzanetakis, I. E. 2020. Comparative analysis of a new blackcurrant waikavirus with other members of the genus. Eur. J. Plant Pathol., in press</p><br /> <p>Thompson, B.D., Dahan, J., Lee, J., Martin, R.R., and Karasev, A.V. 2019. A novel genetic variant of Grapevine leafroll-associated virus-3 (GLRaV-3) from Idaho grapevines. Plant Disease 103: 509-518 (http://dx.doi.org/10.1094/PDIS-08-18-1303-RE).</p><br /> <p>Thompson, B.D., Eid, S., **Vander Pol, D., Lee, J., and Karasev, A.V. 2019. First report of grapevine red blotch virus in Idaho grapevines. Plant Disease 103: 2704 (http://dx.doi.org/10.1094/PDIS-04-19-0780-PDN).</p><br /> <p>Wang, Y., Wang, Q., Yang, Z., Li, R., Liu, Y., Li, J., Li, Z., Zhou, Y. 2020. Development of a sensitive and reliable reverse transcription-droplet digital polymerase chain reaction (RT-ddPCR) assay for the detection of Citrus tristeza virus. European Journal of Plant Pathology. <a href="https://doi.org/10.1007/s10658-019-01920-x">https://doi.org/10.1007/s10658-019-01920-x</a>.</p><br /> <p>Wright, A. A., Cross, A. R., & Harper, S. J. (2020). A bushel of viruses: Identification of seventeen novel putative viruses by RNA-seq in six apple trees. Plos One, 15(1), e0227669.</p><br /> <p>Wright, A. A., Cross, A. R., & Harper, S. J. (2020). A bushel of viruses: Identification of seventeen novel putative viruses by RNA-seq in six apple trees. Plos One, 15(1), e0227669.</p><br /> <p>Wu, L., Du, T., Liu, H., Peng, L., Li, R. 2019. Complete genomic sequence of tea-oil camellia associated deltapartitivirus, a novel virus from Camellia oleifera. Archives of Virology. <a href="https://doi.org/10.1007/s00705-019-04429-0">https://doi.org/10.1007/s00705-019-04429-0</a>.</p><br /> <p>Wu, L., Liu, H., Bateman, M., Komorowaka, B., Li, R. 2019. First identification and molecular characterization of apricot symptomless virus. Archives of Virology. <a href="https://doi.org/10.1007/s00705-019-04401-y">https://doi.org/10.1007/s00705-019-04401-y</a>.</p><br /> <p>Zhang S., Davenport B., Li R., Matousek J., Groth-Helms D., Schuetz K. (2020): Development of an isothermal AmplifyRP XRT assay for rapid real-time detection of Hop stunt viroid through recombinase polymerase amplification. Plant Health 2020, Denver, Colorado, USA.</p><br /> <p>Zhang, S., Yang, L., Ma, L., Tian, X., Li, R., Zhou, C., Cao, M. 2020. Virome of Camellia japonica: discovery and molecular characterization of new viruses of different taxa in camellias. Frontiers in Microbiology. <a href="https://doi.org/10.3389/fmicb.2020.00945">https://doi.org/10.3389/fmicb.2020.00945</a>.</p><br /> <p>Zheng, L., Chen, M., Li, R. 2020. Camellia ringspot associated virus 4, a proposed new foveavirus from Camellia japonica. Archives of Virology. https://doi.org/10.1007/s00705-020-04655-x.</p><br /> <p>Zurn, J.D., Ho, T., Li, R., Bassil, N.V., Tzanetakis, I., Martin, R.R., Postman, J.D. 2019. First report of Blackcurrant reversion virus in Ribes nigrum germplasm in the United States. Plant Disease. 103:1051. https://doi.org/10.1094/PDIS-03-18-0526-PDN.</p>Impact Statements
- Erich Rudyj (USDA- NCPN) Since its inception and over the last 15 years, NCPN has evolved a strong governance structure including a national Governing Board and associated coordination and administrative support; governing bodies with chairs, vice-chairs, administrative coordinators and members for each of its specialty crop focus areas, teams in support of program networking such as Strategic Planning, and working groups supporting NCPN program special initiatives such as Education/Outreach/Communications, Economics, Quality Management, and Scientific Information sharing. NCPN currently supports 47 programs in 34 centers in 20 States or U.S. Territories. Core to NCPN administratively is its annual Cooperative Agreements and Grants program, and initiative administered by USDA, APHIS, PPQ, Science and Technology Division. In FY 2020, USDA increased funding to NCPN, expanding its financial base to $7.5 million. As a result of expanded support, NCPN ‘let’ 31 agreements with its cooperators for a total of $7.15 million with 92% of funding going to support diagnostics, therapy, and foundations at clean plant centers and an added 8% being used to fund NCPN special initiatives. NCPN anticipates opening its FY 2021 Request for Proposals in July 2020 with the RFP remaining open for proposal submissions for 12 weeks.
Date of Annual Report: 07/07/2021
Report Information
Period the Report Covers: 10/01/2020 - 09/30/2021
Participants
Abrahamian, Peter (peter.abrahamian@usda.gov)- USDA-APHIS-PPQ-S&T;Al Rwahnih, Maher (malrwahnih@ucdavis.edu)- UC Davis/Foundation Plant Services;
Almeyda, Christie (cvalmeyd@ncsu.edu) - North Carolina State University;
Anderson, Carolyn (carolyn.anderson@ucr.edu)- UC Riverside;
Balci, Yilmaz (yilmaz.balci@usda.gov)- USDA-APHIS;
Barros, Jaime (jaimeba@ucr.edu)- UCR;
Belanger, Charles (charles.a.belanger@usda.gov)- USDA;
Bodaghi, Sohrab (sohrab.bodaghi@ucr.edu)- UC Riverside;
Broome, Jenny (jenny.broome@driscolls.com)- Driscoll's Inc.;
Buhler, Jason (jason.buhler@driscolls.com)- Driscoll’s Inc.;
Chingandu, Noma (noma.chingandu@wsu.edu)- WA State University-IAREC;
Comstock, Stacey (scoms002@ucr.edu)- UCR;
Conner, Cassie (connekn@auburn.edu)- Auburn University;
Cooper, Cindy (ccooper@agr.wa.gov)- WA State Department of Agriculture/NCPN;
Dang, Tyler (tyler.dang@ucr.edu)- UCR;
Alfredo-Diaz, Lara (adiazlara@ucdavis.edu)- Tecnologico de Monterrey University in Mexico-UC Davis;
Dorman, Elizabeth (dormane@michigan.gov)- Michigan Dept. of Agriculture and Rural Development;
Espindola, Andres (andres.espindola@okstate.edu)- Oklahoma State University;
Fayad, Amer (amer.fayad@usda.gov)- NIFA-USDA;
Fuchs, Marc (mf13@cornell.edu)- Cornell University;
Gadhave, Kiran (kirang@ucr.edu)- UCR;
Gratz, Allison (allison.gratz@canada.ca)- Canadian Food Inspection Agency;
Guerra, Lauri (lguerra@agr.wa.gov)- WA State Department of Agriculture;
Hajeri, Subhas (shajeri@cctea.org)- Citrus Pest Detection Program;
Harper, Scott (scott.harper@wsu.edu) - WA State University;
Hess, Bret W. (bhess@unr.edu)- UNR/ ED of Western Association of Agricultural Experiment Station Directors;
Ho, Thien (thienxho@gmail.com) - Driscoll’s Inc.;
Hooper, Dustin (dustin@sridge.net)- Sunridge Nurseries;
Hu, John (johnhu@hawaii.edu)- University of Hawaii;
Hurtado-Gonzales, Oscar (oscar.hurtado-gonzales@usda.gov)- USDA-APHIS Plant Germplasm Quarantine Program;
Jarugula, Sridhar (sjarugula@wsu.edu)- WA State University;
Jimenez, Randi (randi.Jimenez@cdfa.ca.gov)- CA Department of Food & Agriculture;
Jones, Robert (robert.p.jones@aphis.usda.gov)- USDA-APHIS;
Karasev, Alexander (akarasev@uidaho.edu)- University of Idaho;
Kelly, Margaret (margaret.kelly@agriculture.ny.gov)- NY State Department of Agriculture & Markets;
Kong, Alexandra (atk412@hawaii.edu)- University of Hawaii;
Koundal, Vikas (koundal@wsu.edu)- WA State University;
Kruger, Robert (robert.krueger@ars.usda.gov)- USDA-ARS;
Larrea-Sarmiento, Adriana (aelarrea@hawaii.edu)- University of Hawaii;
Lavagi-Craddock, Irene (irenela@ucr.edu)- UCR;
Leo, Ashton (ashton.leo@usda.gov)- USDA-APHIS;
Mendoza, Joshua (joshua.mendoza@usda.gov)- USDA-APHIS-PGQP;
Martin, Robert (robert.martin@oregonstate.edu)- Oregon State University/USDA-ARS;
Melzer, Michael (melzer@hawaii.edu)- University of Hawaii;
Miller, Nathan (nathan.miller@usda.gov)- USDA-APHIS;
Mitra, Arunabha (arunabha.mitra@wsu.edu)- WA State University-IAREC;
Mollov, Dimitre (dimitre.mollov@usda.gov)- USDA ARS;
Moreland, Brittany (brittany.moreland@ars.usda.gov)- USDA-ARS;
Nikolaeva, Ekaterina (enikolaeva@pa.gov)- PA Dep of Agriculture;
O’Donovan, Wendy (wendy.odonovan@driscolls.com)- Driscoll’s Inc;
Olmedo-Velarde, Alejandro (aolmedov@hawaii.edu)- University of Hawaii at Manoa;
Osman, Fatima (fmosman@ucdavis.edu)- UC Davis;
Padmanabhan, Chellappan (chellappan.padmanabhan@usda.gov)- USDA-APHIS Beltsville Laboratory;
Pagliaccia, Deborah (deborahp@ucr.edu)- California Citrus Nursery Society-UCR;
Peter, Kari (kap22@psu.edu)- Penn State University;
Pokharel, Ramesh (ramesh.pokharel@usda.gov)- APHIS;
Postman, Joseph (joseph@casco.net)- USDA-ARS [retired];
Poudyal, Dipak (dpoudyal@oda.state.or.us)- Oregon Dept. of Agriculture;
Prokrym, David (david.r.prokrym@usda.gov)- USDA/APHIS/PPQ/S&T-NCPN;
Puri, Krishna (krishna.puri@mda.mo.gov)- Missouri Department of Agriculture;
Ramachandran, Vanitharani (vanitharani.ramachandran@usda.gov)- USDA;
Rayapati, Naidu (naidu.rayapati@wsu.edu)- WA State University;
Reinhold, Lauri (lauri.reinhold@usda.gov)- USDA-ARS HCRU;
Rivera, Yazmin (yazmin.rivera@usda.gov)- USDA APHIS;
Rudyj, Erich (erich.s.rudyj@usda.gov)- USDA/APHIS/PPQ/S&T-NCPN;
Savory, Elizabeth (esavory@oda.state.or.us)- Oregon Department of Agriculture;
Schmidt, Anna-Mary (anna-mary.schmidt@canada.ca)- Canadian Food Inspection Agency;
Shiel, Patrick (patrick.j.shiel@usda.gov)- USDA APHIS;
Spaine, Pauline (pauline.c.spaine@usda.gov)- USDA-APHIS;
Stack, James (jstack@ksu.edu)- Kansas State University;
Stamp, James (jstamp@sbcglobal.net)- Stamp Associates Viticulture, INC;
Straub, Katlyn (katlyn@washingtonwinefoundation.org)- WA State Wine Foundation;
Suzuki, Jon (jon.suzuki@usda.gov)- USDA ARS DKI U.S. PBARC;
Tan, Nina (stan016@ucr.edu)- UCR;
Thiessen, Lindsey (lindsey.thiessen@usda.gov)- USDA APHIS PPQ;
Thompson, Sage (sage.thompson@usda.gov)-USDA-APHIS;
Tian, Peng (tianp@missouri.edu)- The University of Missouri;
Tian, Tongan (tongyan@cdfa.ca.gov)- CDFA Plant Pest Diagnostics Branch;
Trujillo, Sarah (sarah.g.trujillo@usda.gov)- USDA/PPQ;
Tzanetakis, Ioannis (itzaneta@uark.edu)- University of Arkansas;
Vidalakis, Georgios (vidalg@ucr.edu)- UC Riverside;
Villamor, Dan (dvvillam@uark.edu)- University of Arkansas;
Wang, Xupeng (xupeng@hawaii.edu)- University of Hawaii;
Wei, Gang (gang.wei@usda.gov) USDA-APHIS-PPQ-S&T Beltsville Lab;
Wei, Alan (apwei@agri-analysis.com) Agri-Analysis LLC;
Weber, Kristina (kristina.weber@cdfa.ca.gov)- CDFA, Nursery, Seed, Cotton and Hemp Program;
Yasuhara-Bell, Jarred (jarred.yasuhara-bell@usda.gov)- USDA-APHIS S&T;
Yokomi, Ray (ray.yokomi@usda.gov)- USDA-ARS;
Zhang, Shulu (shulu@agdia.com)- Agdia Inc.;
Brief Summary of Minutes
For the meeitng agenda and a photo of the participants on the Zoom call, see the attachment at: https://www.nimss.org/projects/attachment/18276
The multi-state WERA-20 virtual annual meeting held during May 12 - 14, 2021, was hosted by Dr. Georgios Vidalakis, Professor & UC Extension Specialist in Plant Pathology and Director, Citrus Clonal Protection Program (CCPP), at the Department of Microbiology & Plant Pathology, University of California, Riverside, CA 92521. Dr. Timothy Paine, Divisional Dean Agricultural & Natural Resources, University of California, Riverside, welcomed the attendees followed by a brief overview of the CCPP and the role of research and extension and partnerships between institutions in agricultural sustainability. Dr. Amer Fayad, USDA-NIFA National Program Leader provided an overview of NIFA/Plant Systems Protection Programs and made a presentation on NIFA Competitive Funding Grant Programs, including opportunities for early career professionals, and Joint NSF/NIFA Program – Plant Biotic Interactions, to pursue collaborative research and extension in different areas of agriculture. Dr. Naidu Rayapati, Administrative Advisor, provided a brief account of the WERA-20 project that will be officially ending by end of September 2021. Subsequently, discussions were held about status of the new five year proposal WERA_TEMP_20 (Management of Diseases Caused by Systemic Pathogens in Temperate and Sub-Tropical Fruit Crops and Woody Ornamentals) submitted to Western Association of Agricultural Experiment Station Directors in December 2020. The Western Region’s Multistate Review Committee (MRC) provided feedback on the proposal and, in view of the importance of the project, recommended for resubmission with appropriate revisions suggested by the MRC and two peer-reviewers. Consequently, the proposal is designated as “Western Development Committee” to reflect that the proposal is “Under Review” until MRC approves the project. Based on MRC’s comments, the group discussed critical elements needed for revising the proposal. The writing committee will revise the proposal addressing MRC’s comments and proposal evaluator’s concerns and submit by January 15, 2022, for consideration by the MRC. It was agreed that Dr. Vidalakis will lead the writing committee to submit the revised proposal. Future venue for the annual meeting in 2022 will be decided when the revised proposal is approved by the MRC. As a special invitee, Dr. Bret Hess, Executive Director, Western Association of Agricultural Experiment Station Directors, explained the process for becoming an official participant of the multi-state project using the NIMSS system (http://www.nimss.org) and encouraged participants to contact him to assist throughout the process. In addition to research and extension faculty from Land-grant universities, scientists from federal programs (USDA-ARS, USDA-APHIS, etc.), personnel from State Departments of Agriculture and Private sector and industry stakeholders can also become official participants of the multi-state projects through NIMSS system. Attendees were invited to become an official participant of the new WERA_TEMP_20 multi-state project by submitting the APPENDIX E through proper channels into the NIMSS system.
An outline of topics presented by participants:
- State and National Reports:
Ioannis Tzanetakis (University of Arkansas)
- “What's new in the berry world”
- Introduction and elaboration on progress made on Strawberry and Blueberry viruses over the past year.
Maher Al Rwahnih (University of California, Davis)
- Overview of FPS Olive Program, Olive Virus Diseases and Certification.
- Continual research in Olive tree viruses, OQDS, virus management and sanitation.
- New control import permit is in place to allow importation of infected material from Italy.
- Olive oil production in California is increasing. Other states are producing as well.
- Due to disease, alternative Markets are needed due to demand.
Kiran Gadhave & Georgios Vidalakis (University of California, Riverside)
- Citrus yellow vein associated virus novel RNA- A 70 year old California tale
- A brief account of the problem and current understanding of the virus
Thein Ho (Driscoll’s Inc.)
- Characterization and detection of Rubus yellow net virus (RYNV) in raspberries
- RYNV strain #1 - Canada and RYNV strain #2 - UK are found.
Alexander Karasev (University of Idaho)
- Viruses of solanaceous fruit crops
Shulu Zhang (Agdia Inc.)
- “Recent developments of AmplifyRP assays for various crops”
Ramesh Pokharel (USDA-APHIS)
- Plant viruses in small berries in Maryland
- Survey, testing and documentation of multiple viruses in small fruits
Marc Fuchs (Cornell University)
- Updates on grapevine red blotch virus transmission by the three-cornered alfalfa hopper.
- Review of ongoing work and research data on this topic.
Christie Almeyda (North Carolina State University)
- Berry and grape viruses detected in North Carolina
- Review of testing capabilities for berries and new findings of viruses in 2021.
- Discussed about field survey of vineyards and testing results from 2018 to 2020
Lauri Guerra (Washington State Dept. of Agriculture)
- Washington State Fruit Trees Certification Updates
Scott Harper (Washington State University)
- Cherry Viruses, update on work over the last year.
- Impacts of little cherry disease and understanding the epidemiology of causal agents associated with the disease.
- New creation APP will be launched for growers to use as a reference in helping them identify virus symptoms in the field.
Arunabha Mitra, Sridhar Jarugula, & Naidu Rayapati (Washington State University)
- Molecular biology of Grapevine leafroll-associated viruses (GLRaVs)
- Recent efforts in building infectious cDNA clones for Grapevine leafroll-associated viruses, and current work on the molecular biology of GLRaV-1.
Adriana Larrea-Smiento & John Hu (University of Hawaii)
- Detection and characterization of plant viruses in Hawaiian pineapples.
Robert Martin (USDA-ARS)
- Presented an in-progress creation of a user-friendly Virus database for NCPN crops for end users.
- Demonstrated the NCPN database search capabilities, content for users. (multi-crop information)
Oscar Hurtado-Gonzales (USDA-APHIS)
- Status report of Fruit Trees held under Quarantine in PGOP
- Transitioning towards the use of field indicators generated in-house via tissue culture (CFIA approach).
- Review of diagnostic workflow through to Quarantine release.
- Data review of PGQP Pomes field survey in East Coast states.
Yilmaz Balci (USDA-APHIS)
- USA: Overview and updates to import requirements of pome & stone fruits
- The Controlled Import Permit (CIP) team introduced
- Review of how plants enter the United States, generally advisable plants, restricted or NAPPRA (Not approved pest plant risk assessment) plants.
- ePermits will no longer be available after the end of Fiscal year 2022.
- New system, APHIS eFile, is put in place to apply and manage your applications, registrations, permits & licenses.
- 2021 WERA-20 Special Topics Updates:
Katlyn Staub (Washington Wine Industry Foundation)
- Update on the status of harmonization of State Certificate & Quarantine programs
- National Nursery Certification - Working Group Liaison w/ USDA APHIS on Certifications.
- Certification standards, State certification programs - funded by APHIS USDA.
- By Jan 2023, all planting materials of fruit crops exported to the EU to originate from approved certification programs.
- Start drafting documents to present for the EU.
- New focus: outreach & education; onboarding new programs
- State regulators participating: Washington, Oregon, California, Michigan, Pennsylvania. New York
Maher Al Rwahnih (UC Davis) and Ioannis Tzanetakis (University of Arkansas)
- Update on the standardization of high-throughput sequencing (HTS), diagnostics & regulatory issues
- HTS vs conventional methods for virus diagnostics in strawberry G1 plant.
- HTS vs PCR.
- Drawbacks of conventional methods for biological testing.
- Study results and ongoing work using HTS data to improve PCR test results.
Bret Hess (Executive Director, Western Association of Agricultural Experiment Station Directors)
- National Information Management & Support System (NIMSS) and Appendix E: the official process to join WERA 20
- Review of NIMSS website, and how to become a user in National Information Management & Support System (how to create new user profile)
- Creating a new Appendix E with a detailed walkthrough in the website.
- Noting Land grant University must use 25% of HATCH funds on multi-state projects.
James Stack (Kansas State University)
- A resource-conscious, near comprehensive approach to quality diagnostics for the National Plant Diagnostic Network (NPDN)
- Quality standards need to be achievable.
- Design system that is appropriate to the labs and resources.
- Quality standards should not be a burden to productivity.
- Review of Quality Proficiency, Accreditation, Core Accreditation Standards.
Patrick Sheil (USDA-APHIS)
- NPDN Project is currenting creating and implementing Professional Development courses that promote Quality Diagnostic Network (NPDN), including training materials. Funded by NPDN & supplemented by PPQ & Farm Bill
Erich Rudyj (USDA-APHIS, National Clean Plant Network)
- Understanding its quality from the perspective of National Clean Plant Network (NCPN) and it’s Strategic Plan.
- Permanent funding secured through the Farm Bill.
- Emphasis on Quality for all goals and objectives and why quality is important to NCPN on ‘National’ POV.
Irene Lavagi-Craddock and Fatima Osman (University of California, Riverside and Davis)
- Lavagi presented “Overview of the NCPN-Quality initiative”
- Restate the NCPN Creation of Quality Steering Committee and the Citrus Clonal Protection Program tasks of Therapy, diagnostics and distribution.
- Osman presented slides on Phase 3 “Building a roadmap for developing a system wide Quality plan.”
- Review of Milestones & Timeline - objectives (FY 2020 - FY 2023)
- Survey will be released soon to collect feedback from all NCPN centers.
Panel Discussion: Summary perspective of Quality- Erich Rudy (Facilitator)
- Introduction of Sarah Trujillo, USDA PDQ Office, NCPN Strategic Planner
- Emphasis on increase of question to better help the understanding of Quality across the board and on the importance of networking with other programs.
- SOP for Diagnostics is already part of the NCPN Strategic plan and surveys will assist in gathering data on how broad training should be.
- James Stack will look into allowing access to Core Quality Standards and the 360 Training Platform.
- Reminders for the importance of coordination of networks and incorporating Therapy Protocols using virus genome, multiple isolates, multiple tests.
Accomplishments
<p><strong>Ioannis Tzanetakis (University of Arkansas)</strong></p><br /> <p>In strawberry, we have fully characterized a new cytorhabdovirus and developed multiplex RT-PCR diagnostics protocols. We also completed transmission with the strawberry and small bramble aphid, but neither proved to be vectors of the virus. We also completed the characterization of another rhabdovirus in the crop. The new virus is the type member of a new genus in the family and transmission trials with the strawberry aphid were unsuccessful. As in the case with the novel cytorhabdovirus a multiplex RT-PCR test targeting two virus genes and an internal control have been developed.</p><br /> <p>In blueberry, the characterization of a novel luteovirus has been completed and revealed its close association with nectarine stem pitting associated virus. A survey of over 600 samples from the Pacific Northwest, Michigan, New Jersey and Pennsylvania indicated that the virus is very widespread as more than half of the samples tested positive. For understanding population structure of the virus, sequence analysis of the partial genome of ~ 300 isolates indicated ~ 18% diversity at the nucleotide level. The new carlavirus discovered in blueberries is most closely related to blueberry scorch virus (BlScV) and was present in ~ 2% of the aforementioned samples. The virus cross-reacts very poorly with antibodies against scorch, whereas its host range and symptomology on alternative hosts is different to that of BlScV. An infectious clone has been developed and will be used to test the reaction of major blueberry cultivars to the virus. This information is used in the development of accurate and reliable protocols for the detection of two blueberry viruses. These tests will be able to detect the vast majority of isolates of the two viruses circulating in the United States.</p><br /> <p><strong>Ekaterina Nikolaeva (Pennsylvania Department of Agriculture)</strong></p><br /> <p>Pennsylvania Department of Agriculture (PDA) in cooperation with Penn State University (PSU) conducted 2020 PPA 7721 funded surveys for exotic diseases in orchards and small fruits. Orchard survey targets included Plum pox virus, Asian Pear Blight (<em>Erwinia pyrifoliae</em>), Asiatic brown rot (<em>Monilia polystroma</em>), Apple brown rot (<em>Monilinia fructigena</em>), Apple Proliferation (<em>Candidatus</em> Phytoplasma mali), European stone fruit yellow (<em>Ca.</em> Phytoplasma prunorum), and Almond witches’ broom (<em>Ca.</em> Phytoplasma phoenicium). Small fruit survey targeted Asian pear blight (<em>Erwinia pyrifolia</em>), Nepovirus Tomato black ring virus, Australian Grapevine Yellows (<em>Ca</em>. Phytoplasma australiense), Flavescence Doreé Phytoplasma (<em>Ca.</em> Phytoplasma vitis), and Bois noir Phytoplasma (<em>Ca</em>. Phytoplasma solani). No exotic targets were detected.</p><br /> <p>In 2020, PDA/PSU team in collaboration with USDA-APHIS Plant Germplasm Quarantine Program (PGQP) evaluated distribution of known pome viruses and viroids on apple trees affected by Rapid Apple Decline (RAD) in PA. The samples collected from 18 orchards and 6 production sites were analyzed in PGQP via HTS and RT-PCR techniques. In result, ASGV, CCGaV, ALV-1, ASPV, TRSV, ToRSV, and GaIV were the most common viruses detected on RAD affected trees. ACLSV, ARWaV1, ARWaV2, AHVd, AGCaV were also detected in PA apple orchards. PDA continues to operate with the Fruit Tree Improvement Program (FTIP), a specialized virus-tested fruit tree certification program. Three nurseries participated in the FTIP last year. Over 1,600 samples were tested for viruses of concern, including Prunus Necrotic Ringspot Virus (PNRSV), Prune Dwarf Virus (PDV), Tomato Ringspot Virus (ToRSV), and Plum Pox Virus (PPV). No PPV was detected in rootstock blocks or in registered source blocks. PNRSV remains most commonly found virus in Prunus in PA nurseries. The occurrence of PDV and ToRSV in registered blocks and nursery production blocks remain low. All blocks met virus-testing requirements for FTIP certification.</p><br /> <p><strong>Maher Al Rwahnih (University of California, Davis)</strong></p><br /> <p>Foundation Plant Services (FPS) continues to make advances in developing and refining methods using high throughput sequencing (HTS) as superior tools for the detection of existing and emerging viruses. We have used sequence information generated by HTS analysis to design new, species-specific PCR primers for use in molecular diagnostics. In addition, HTS proved to be an invaluable tool in the discovery of unknown viruses and in establishing a baseline analysis of the virome of a crop.</p><br /> <p>In 2016, FPS acquired a Controlled Import Permit (P588) to facilitate the introduction, quarantine, and release of imported <em>Prunus </em>for the tree fruit industry. In 2020, we were successful in obtaining USDA-APHIS and CDFA approval to revise our tree fruit protocols, eliminating four ineffective <em>Prunus</em> biological indicators. We conducted side-by-side studies by comparing HTS analysis to biological indexing, which revealed that the performance of the biological indicators was inferior to PCR and HTS testing. Under the new protocol, plants are subjected to greenhouse indexing and molecular testing at two different time points by PCR and HTS. Plants may be released if all test results demonstrate plants are free of viruses and virus-like agents.</p><br /> <p>FPS has now conducted three years of side-by-side studies comparing the efficacy of woody and herbaceous indexing to PCR and HTS testing in <em>Prunus</em> diagnostics. The results of these studies on 82 <em>Prunus</em> selections with 17 infected trees, indicate that GF 305 and Bing Cherry provided false negative results in every case. On the same selections, herbaceous host indexing was ineffective as well, with false negative results ranging from 33% to 100%. We have also compared biological indexing to HTS and PCR testing for detecting viruses on grapevine and roses. In 104 grapevine selections where 60 were infected with at least one virus, woody indicators (Cabernet Franc, St. George, and LN33) gave false negative results 4-6% of the time and herbaceous host indexing gave false negatives 25-100% of the time. In comparative studies on 65 rose selections with 22 infected plants, the woody indicators <em>Rosa multiflora</em> ‘Burr’ and Shirofugen cherry provided false negative results in 23% and 77% of the cases, respectively. The ‘Development and validation of real time quantitative PCR assays for the detection of viruses’ study evaluated the broad-range detection capacity of currently available real-time RT-PCR assays for viruses and developed new assays when current tests were inadequate or absent. assays for 15 different viruses were exhaustively evaluated <em>in silico</em> to determine their capacity to detect virus isolates deposited in GenBank. During this evaluation, several isolates deposited since the assay was designed exhibited nucleotide mismatches in relation to the existing assay’s primer sequences. In cases where updating an existing assay was impractical, we performed a redesign with the dual goals of assay compactness and comprehensive inclusion of genetic diversity. The efficiency of each developed assay was determined by a standard curve. To validate the assay designs, we tested them against a comprehensive set of 87 positive and negative <em>Prunus</em> samples independently analyzed by high throughput sequencing. As a result, the real-time RT-PCR assays described herein successfully detected the different viruses and their corresponding isolates. To further validate the new and updated assays a <em>Prunus</em> germplasm collection was surveyed. The sensitive and reliable detection methods described here will be used for the large-scale pathogen testing required to maintain the highest quality nursery stock. We previously reported 15 new assays for viruses infecting Prunus were developed.</p><br /> <p>Our in-house validation studies have focused on the sensitivity, specificity, repeatability, and reproducibility of HTS as a routine diagnostic tool. We have developed the best sampling strategies by comparing two different types of tissue and we have optimized bioinformatics algorithms to efficiently separate pathogen and host sequences. In addition, we have participated in an inter-laboratory validation and standardization of HTS project with the USDA Center for Plant Health Science & Technology (CPHST) and Plant Germplasm Quarantine Program (PGQP) laboratories Maryland to coordinate the development of minimum basic requirements for the adoption of HTS technologies, including nucleic acid extractions, library preparation, depth of sequencing and bioinformatics, for the detection of viral pathogens. This cooperative study validated the HTS protocol and pipeline used by the participating laboratories for the identification of grapevine and apple viruses. All three laboratories detected 100% of the same viruses indicating the reliability of HTS as a detection method. This is an on-going project that will include the validation of the HTS pipeline for <em>Prunus</em> virus detection in 2021-2022. These advances in protocol development for HTS technologies in plant virus detection are in-line with the International Plant Protection Convention <em>Recommendation on Preparing to use high-throughput sequencing (HTS) technologies as a diagnostic tool for phytosanitary purposes</em>. Our research and collaborative efforts have satisfied all the recommendations outlined in the document, allowing us to collaborate internationally and operate within the European framework.</p><br /> <p><strong>Kiran Gadhave & Georgios Vidalakis (University of California, Riverside)</strong></p><br /> <p>Our team at UC Riverside and the University of Maryland published a paper reporting the discovery of novel virus like RNA (provisionally named citrus yellow-vein associated virus or CYVaV) associated with the citrus yellow-vein disease (CYVD) which was first reported in California in 1957. The CYVaV RNA genome has 2,692 nucleotides and codes for two discernable open reading frames (ORFs). ORF1 encodes a protein of 190 amino acids (aa) whereas ORF2 is presumably generated by a −1 ribosomal frameshifting event just upstream of the ORF1 termination signal. The frameshift product (717 aa) encodes the RNA-dependent RNA polymerase (RdRp). Phylogenetic analyses suggest that CYVaV is closely related to unclassified virus-like RNAs in the family Tombusviridae. Bio-indexing and RNA-seq experiments indicate that CYVaV can induce yellow vein symptoms independently of known citrus viruses or viroids.</p><br /> <p>In an effort to develop CYVaV as a virus induced gene silencing (VIGS) vector, the first efforts involve regeneration of citrus plants from protoplasts of embryonic suspension cultures of citrus transfected with yellow vein RNA at the plant transformation facility at UCR. Over the past few months, we tested a few wild-type and recombinant CYVaV constructs in Daisy and Tango cell lines. We report the successful replication of CYVaV (via T7 RNA transcripts generated from linearized pET17b-CYVaV construct) in both ‘Daisy’ and ‘Tango’ cell lines of citrus. Transfected protoplasts appeared to regenerate cell walls, undergo cell division, and remain viable for up to 2 months post-transfection. The plant regeneration efforts via protoplast and other CYVaV delivery efforts will be continued at UC Riverside. To study the CYVaV distribution in the US, five samples from Texas A&M University and 33 samples from Puerto Rico have been analyzed using qRT-PCR. None of the 38 samples in total have been tested positive for the presence of CYVaV. Multiple researchers in Florida that have access to old citrus orchards have been contacted. Currently, efforts are being undertaken to collect samples. We studied CYVaV mobility and symptomatology (the onset of characteristic yellow vein disease symptoms) at the first time point (6 months after graft-inoculation) in all CYVaV graft-inoculated trees planted in a replicated field trial at Agricultural Operations fields at UC, Riverside. CYVaV mobility was tested via RT-qPCR analyses of root and shoot samples from all 6 graft-inoculated and 2 healthy (negative control) trees per combination (12 rootstock scion combinations in total). Of the 72 graft-inoculated trees, 32% of trees (from different rootstock scion combinations) tested positive, whereas 68% trees tested negative for CYVaV. None of the 16 older trees (various ages) with assorted rootstock scion combinations were positive for CYVaV. Only 5 of 88 trees (including additional combinations) showed characteristic yellow vein disease symptoms. The survival of grafts was checked 6 months after original graft inoculation (all original grafts had survived for at least 2 weeks) and trees with both dead grafts were re-grafted in June 2021.</p><br /> <p><strong>Thein Ho (Driscoll’s Inc.)</strong></p><br /> <p>Rubus yellow net virus (RYNV) belongs to genus <em>Badnavirus</em>. Badnaviruses are found in plants as endogenous (inactive) sequences, and/or in episomal (infectious and active) forms. To study the state of RYNV infections, we sequenced the genomes of 25 raspberry cultivars and mined eight published genome datasets. Sequence analysis revealed the presence of a diverse array of endogenous RYNV (endoRYNV) sequences that differ significantly in their structure; some lineages have nearly complete, yet non-functional genomes whereas others have rudimentary, small sequence fragments. We developed SYBR Green PCR assays to genotype the main endoRYNV lineages as well as the only known episomal lineage in commercial <em>Rubus</em>. This study reveals the widespread presence of endoRYNVs in commercial raspberries, likely because breeding programs have been using a limited pool of germplasm that originally harbored endoRYNVs.</p><br /> <p><strong>Alexander Karasev (University of Idaho)</strong></p><br /> <p>In 2018, leaf and petiole samples from five declining Chardonnay vines were collected from a single vineyard in Canyon County of Idaho. Ribodepleted total RNA prepared from these samples was subjected to a high-throughput sequencing (HTS) analysis on a MiSeq platform, yielding between 3,623,716 and 4,467,149 300-bp paired-end reads. Raw reads were adapted and quality cleaned and mapped against the <em>Vitis vinifera </em>L., reference genome. Unmapped paired reads were assembled, producing between 829 and 1,996 contigs over 1,000-nt in length. All five samples were found to contain GLRaV-3 and the two common viroids, hop stunt viroid and grapevine yellow speckle viroid, while four contigs ranging in size from 1,361 to 6,736 and exhibiting homology with the GRVFV were found in three out of the five Chardonnay samples analyzed. A nearly complete genome of GRVFV-ID was assembled from the HTS data of one sample, and the 3’-terminus of the genome was acquired using the RACE methodology; the 6,736-nt sequence has been deposited in the GenBank database under the accession number MZ027155. In the fall of 2020, six commercially operating vineyards in Canyon and Nez Perce Counties of Idaho, including the original one, were sampled for the total of 26 sampled plants of white and red wine grape cultivars, based on visual symptoms of leaf reddening, leaf rolling, and chlorosis, and tested by reverse transcription (RT)-PCR using newly designed GRVFV-specific primers. Four plants were found positive for GRVFV by RT-PCR; these positive samples came from three vineyards in Canyon County, from the same wine grape cultivar, Chardonnay. Amplified RT-PCR products were directly sequenced using conventional Sanger methodology and confirmed to represent 662-nt segments of the GRVFV genome exhibiting 98.6-99.1% pairwise identity to the HTS-derived full-length genome of GRVFV-ID (MZ027155). This close identity between the GRVFV sequences from three different Idaho vineyards, coming from the same cultivar Chardonnay, may suggest a common origin of the original GRVFV infection, possibly the same supplier of the original Chardonnay planting material. Presence of GRVFV might have contributed to the decline of the original Chardonnay vines, although the exact role of GRVFV in a mixed infection with GLRaV-3 is not clear at the moment.</p><br /> <p>Grapevine red blotch virus (GRBV) infection was recently identified in an Idaho commercial vineyard. Fruit quality from healthy vines (no viruses detected), and GRBV positive (infected) vines was determined for ‘Syrah’ grapes from this vineyard. GRBV infected vines produce grapes with significantly lower total sugars and lower total anthocyanins. They were also lower in a single free amino acid, yet higher in malic acid compared to grapes from healthy vines. No significant differences were seen in total organic acids, yeast assimilable nitrogen content, total free amino acids, total phenolics, or total tannins between the grapes of healthy vines versus those of GRBV infected vines. Overall, GRBV negatively influenced grape quality by reducing total sugars and total anthocyanins adversely affecting wine quality.</p><br /> <p><strong>Shulu Zhang (Agdia Inc.)</strong></p><br /> <p>Agdia Inc., a leading plant diagnostics company, has been utilizing advanced diagnostic technologies to help crop growers and researchers to effectively detect the presence of plant pathogens in their crops and prevent a potential crop loss from infection by pathogens. One such technology is recombinase polymerase amplification, a leading isothermal amplification technology, based on which Agdia Inc. has developed its own isothermal amplification platform – AmplifyRP<sup>®</sup>. In recent years, Agdia has developed and commercialized 28 AmplifyRP<sup>®</sup> kits. Among them, 26 kits are capable of specifically detecting 25 different pathogens infecting a wide range of crops and 2 kits can be used for any pathogen. Over the past year, 5 AmplifyRP<sup>®</sup> kits were commercialized for <em>Grapevine leafroll-associated virus</em> 3, <em>Potato virus</em> Y, <em>Potato mop-top virus</em>, <em>Tomato brown rugose fruit virus</em>, and <em>Hop latent viroid</em>. These five kits specific to a single species of viruses or viroids can produce real-time, quantitative results and are deployable both in laboratories and in the field. They are simple to use, and no thermal cycler and DNA/RNA purification are needed as all reactions works well with plant crude extracts at a constant temperature 39-42°C. The whole assay from sample to result can be completed within 30 minutes and yet it is as sensitive as qPCR or PCR. This isothermal amplification technology AmplifyRP<sup>®</sup> has provided a versatile detection tool for rapid detection of many important plant pathogens and helped growers to effectively manage crops and prevent significant economic losses from damages by pathogens.</p><br /> <p><strong>Marc Fuchs (Cornell University)</strong></p><br /> <p>Research efforts at Cornell have primarily focused on grapevine red blotch virus (GRBV) (Cieniewicz et al.,</p><br /> <p>2020; Flasco et al., 2021) and grapevine fanleaf virus (GFLV) (Osterbaan et al., 2021; Hily et al., 2021). For GRBV, emphasis was placed on virus transmission by the three-cornered alfalfa hopper (Flasco et al., 2021) and on vector ecology (Cieniewicz et al., 2020). For GFLV, virus-host interactions (Osterbaan et al., 2021) and virus evolution (Hily et al., 2021) were investigated. Efforts have also focused on exploring RNA interference as a potential means to control the grape mealybug vector of grapevine leafroll viruses (Arora et al., 2020).</p><br /> <p><strong>Christie Almeyda (North Carolina State University)</strong></p><br /> <p>The Micropropagation and Repository Unit (MPRU) at North Carolina State University (NCSU) produces, maintains and distributes pathogen-tested G1 material of berry crops (strawberry, blackberry, raspberry and blueberry) and muscadine grapes to industry and researchers in the U.S. The MPRU presently conducts testing for targeted pathogens and therapy for pathogen elimination (heat treatment and meristem-tip culture) and maintains Fragaria, Rubus and Vaccinium G1 (foundation) blocks in vitro, in the greenhouse and the screenhouse. The same methods are applied for muscadine grapes.</p><br /> <p>In recent years, this facility has cleaned and tested mainly domestic materials from most of the berry breeding programs in the U.S. and muscadine grapes breeding programs from the Southeast. While cleaning up berry crops, the following viruses were detected on blueberries: Blueberry latent virus (BBLV) and Blueberry red ringspot virus (BRRV). Blackberry yellow vein-associated virus (BYVaV), Blackberry virus E (BlVE) and Citrus concave gum-associated virus (CCGaV-like) were detected on blackberries. Officially in 2021, the MPRU has established a partnership with the NC Plant Disease and Insect Clinic (PDIC) to test berry and grape samples from NC growers. The MPRU has a controlled imported permit (CIP) under which the unit is currently cleaning blueberries from Chile and Peru and strawberries from Korean and Japan.</p><br /> <p>In partnership with Dr. Hoffmann (NCSU strawberry and grape extension specialist), samples from NC grower vineyards were tested using protocols previously developed by Foundation Plant Services (FPS), UC-Davis in collaboration with Dr. Maher Al Rwahnih. The pathogens currently being tested are Grapevine leafroll viruses (GLRaV-2, GLRaV-3, GLRaV-4, GLRaV7), Grapevine red blotch virus (GRBV), Grapevine rupestris stem pitting associated virus (GRSPaV), vitiviruses (GVA, GVB), Tobacco RingSpot Virus (TRSV), and Xyllela fastidiosa. Eighty samples were tested from 8 vineyards in NC (7 Vitis vinifera vineyards and 1 muscadine vineyard) in order to know the incidence of viral pathogens in this area during 2018, 2019 and 2020. GLRaV-3, GRBV and Xyllela fastidiosa were detected during the second year of this survey. GLRaV-3 and GRBV were the most predominant (20/80 each). Only 6 samples were positive for Xyllela fastidiosa. GLRaV-2, GLRaV-2 and GRBV were found in the third year. We are also working into cleaning and virus testing the material we currently have at the MPRU (10 NC muscadine cultivars) and new material (5 genotypes) we obtained from the AR breeding program in 2019.</p><br /> <p><strong>Scott Harper (Washington State University)</strong></p><br /> <p>The Harper lab is one of the major participants in the Washington & Oregon Little Cherry Disease task force, providing the pathology component in collaboration with entomology, horticulture and extension researchers to understand the pathology and etiology of this disease. <strong> </strong>We are studying to track the movement of X-disease phytoplasma strains between hosts in the orchard and extra-orchard environment. Preliminary results suggest that annual weeds play an incidental role in long term spread, while perennial hosts are the main reservoir for the pathogen. Cumulatively, our efforts have changed grower practices: 89% of respondents at the 2020 NW Hort Show said they had changed management practices over the last two years as a result of information from WSU researchers (N=160), 86% had scouted and sampled symptomatic trees (N=215), 77% had removed infected trees (N=197), and 70% had applied post-harvest sprays for leafhoppers (N=188).</p><br /> <p><strong>Adriana Larrea-Smiento, Alejandro Olmedo-Velarde & John Hu (University of Hawaii)</strong></p><br /> <p><strong><em>Flat-mite transmitted plant viruses in Hawaii: update on a multi-crop study.</em></strong></p><br /> <p><em>Hibiscus green spot virus 2</em> is a member of the <em>Kitaviridae</em> family and genus <em>Higrevirus</em>. In Hawaii, hibiscus green spot virus 2 (HGSV2) infection causes leprosis-like symptoms in citrus, and green spot symptoms in <em>Hibiscus</em> spp., including <em>H. arnottianus</em>, a species native to Hawaii. A reverse genetics system for kitavirids has yet to be developed. Such a system would help us better understand basic biological mechanisms of virus-host and virus-vector interactions. HGSV2 is potentially transmitted by <em>Brevipalpus</em> mites (Acari: Tenuipalpidae), although adequate transmission studies are lacking. Therefore, a robust reverse genetics system of HGSV2 for agrobacterium-mediated delivery into <em>Phaseolus vulgaris</em> (common bean) is in development. So far, two separate biological experiments have demonstrated the infectiousness of the HGSV2 reverse genetics system. Additionally, preliminary results of transmission assays of HGSV2, using <em>Brevipalpus</em> mites collected from citrus, suggest that <em>Brevipalpus</em> mites can not only acquire HGSV2, but also potentially transmit HGSV2 from citrus to citrus.</p><br /> <p><strong><em>Re-examination of mealybug wilt of pineapple</em></strong></p><br /> <p>Mealybug wilt of pineapple (MWP) is the most important viral disease affecting pineapple. The disease is caused by members within the genus <em>Ampelovirus</em>, family <em>Closteroviridae</em>. The viruses are named pineapple mealybug wilt-associated virus 1 (PMWaV-1), PMWaV-2, and PMWaV-3. The identification and molecular characterization of pineapple secovirus A (PSV-A), a member within the subgenera <em>Cholivirus</em>, genus <em>Sadwavirus</em>; and the recent report of a putative new PMWaV member, tentatively named pineapple mealybug wilt-associated virus 6 (PMWaV-6) were accomplished utilizing high throughput sequencing (HTS) technologies. Complete viral genomes were obtained including the 5’ and 3’ ends. RNA-dependent RNA polymerase (RdRp), Heat shock protein 70 homolog (HSP70) and the coat protein (CP) encoded by PMWaV-6 share 30-69% identity with homologs of grapevine leafroll-associated virus 3 (GLRaV-3) and PMWaV-2, suggesting the presence of a new member belonging to the subgroup I within the genus <em>Ampelovirus</em>. Robust RT-PCR detection methods were implemented for both PSV-A and PMWaV-6 to assess their presence in both germplasm accessions obtained from the National Plant Germplasm Repository (NPGR) located at Hilo-Hawaii, and from commercial pineapple plants. Most of the MWP-symptomatic samples tested positive for the presence of both PSV-A and PMWaV-6 in surveys performed in Oahu-Hawaii, while healthy looking samples tested negative in RT-PCR assays for these two viruses.</p><br /> <p><strong>Robert Martin (USDA-ARS)</strong></p><br /> <p>The program in Corvallis works closely with the University of Arkansas on characterizing novel viruses of berry crops and developing diagnostics. The material from the blueberry survey was used to study virus diversity of two new blueberry viruses (a luteovirus and a carlavirus), a survey of these two viruses is being carried from the archived samples in the -80C freezer from the blueberry survey done earlier. The samples were sent to University of Arkansas for testing for the novel viruses and studying virus diversity.</p><br /> <p>Other Accomplishments – Stakeholder oriented Virus database for NCPN crops.</p><br /> <p>The Corvallis program has taken the lead on developing a virus database for the NCPN crops. The content development for the database is being done primarily by members of the WERA-20 group, based on the individual’s expertise: Berries (Martin USDA-ARS) and Tzanetakis (University of Arkansas), Citrus (Vidalakis – UC Riverside), Grapes and Roses (Al Rwahnih – UC Davis), Sweetpotato (Clark – Louisiana State University), Tree Fruit and Hops (Harper – Washington State University. The funding for the project is from NCPN. The beta version of the database is at: <a href="http://virusdb-dev.cass.oregonstate.edu/">http://virusdb-dev.cass.oregonstate.edu/</a></p><br /> <p><strong>Arunabha Mitra, Sridhar Jarugula, & Naidu Rayapati (Washington State University)</strong></p><br /> <p>Managing economically detrimental viral diseases in vineyards is a top priority for sustainability of the grape and wine industry in Washington State. Among the viral diseases documented in the state vineyards, grapevine leafroll disease (GLD) is widespread causing significant negative impacts to vine health and productivity and fruit quality. Of the six grapevine leafroll-associated viruses (GLRaVs) reported in grapevines, GLRaV-1, GLRaV-2, GLRaV-3, and GLRaV-4 were documented in Washington State vineyards. Currently, we are conducting fundamental research to better understand the molecular biology of GLRaV-3, the most widely prevalent among the four GLRaVs with a genome size varying between 18,433 and 18,671 nucleotides (nt) and complex genome organization. Recently, we have built full-length genomic cDNA clones for GLRaV-3 and demonstrated that these clones can replicate in <em>Nicotiana benthamiana</em> leaves when introduced via <em>Agrobacterium</em>-mediated infiltration assays (Jarugula et al., 2018, Virology 523: 89-99). This achievement has laid a foundation to establish an <em>in vitro</em> reverse genetics system for elucidating virus-host interactions in a systems biology approach. Recently, we have expanded these studies to GLRaV-1 that has a genome size ranging between 18,731 and 18,946 nt with an unusually long 5ʹ non-translated region (5ʹ-NTR) ranging in length between 857 and 922 nt (Donda et al., 2017, Phytopathology 107:1069-1079). A cDNA copy of the minigenome of GLRaV-1, containing the 5ʹ-NTR, replicase gene module, Green Fluorescence Protein (GFP) gene and the 3ʹ-NTR, was built as a simplified experimental system to study the role of specific viral sequences in RNA replication. After subcloning into a modified pCAM1380 binary vector, the functionality of GLRaV-1 minigenome cDNA clone was confirmed by the expression of GFP in <em>Nicotiana benthamiana</em> leaves agro-coinfiltrated with a heterologous silencing suppressor. Using this minigenome cDNA clone, studies were conducted to examine the role of 5ʹ-non-translated region (5’ NTR) in RNA replication. Minigenome cDNA clones with different portions of the 5ʹ NTR deleted were tested for their functionality using agro-coinfiltration assays. The results showed that the first 32 nucleotides at the 5ʹ-terminus of the non-coding region are sufficient for replication and GFP expression in <em>N. benthamiana</em> leaves. The minigenome clone retained its functionality in agro-coinfiltration assays when the 5ʹ NTR was replaced with corresponding sequences from two genetic variants of GLRaV-1. These results suggest that 5’NTRs with distinct size and nucleotide sequence are exchangeable between genetic variants of GLRaV-1.</p><br /> <p> </p>Publications
<p>Al Rwahnih, M., Diaz-Lara, A., Arnold, K., Cooper, M.L., Smith, R.J., Zhuang, G., Battany, M.C., Bettiga, L.J., Rowhani, A. and Golino, D., 2020. Incidence and Genetic Diversity of Grapevine Pinot gris Virus in California. American Journal of Enology and Viticulture. DOI: 10.5344/ajev.2020.20044*</p><br /> <p>Al Rwahnih, M., Soltani, N., Soltero Brisbane, R., Tian, T. and Golino, D.A., 2021. First Report of Apricot vein clearing-associated virus Infecting flowering apricot (Prunus mume) in the United States. Plant Disease, (ja). DOI: 10.1094/pdis-10-20-2267-pdn*</p><br /> <p>Alabi, O.J., Appel, D. N., McBride, S., Al Rwahnih, M., and Pontasch, F. M. 2020. Complete genome sequence analysis of a genetic variant of grapevine virus L from the grapevine cultivar Blanc du Bois. Archives of Virology. 165:1905–1909. DOI: 10.1007/s00705-019-04252-7</p><br /> <p>Alejandro Olmedo-Velarde Beatriz Navarro John S. Hu Michael J. Melzer and Francesco Di Serio 2020 Novel Fig-Associated Viroid-Like RNAs Containing Hammerhead Ribozymes in Both Polarity Strands Identified by High-Throughput Sequencing Frontiers in Microbiology doi: 10.3389/fmicb.2020.01903</p><br /> <p>Arora, A.K., Clark, N., Wentworth, K.S., Hesler, S., Fuchs, M., Loeb, G. and Douglas A.E. 2020. Evaluation of RNA interference for control of the grape mealybug Pseudococcus maritimus (Hemiptera: Pseudococcidae) Insects 11:739; doi:10.3390/insects11110739</p><br /> <p>Beaver-Kanuya E, Wright AA, Szostek SA, Khuu N, Harper SJ (2021) Development of RT-qPCR assays for the detection and quantification of three Carlaviruses infecting hop. Journal of Virological Methods 292: 114124.</p><br /> <p>Bolus, S., Rwahnih, M. A., Grinstead, S. C., and Mollov, D. 2021. Rose virus R, a cytorhabdovirus infecting rose. Archives of Virology. DOI: 10.1007/s00705-020-04927-6</p><br /> <p>Chingandu, N., Jarugula, S., Adiputra, J., Bagewadi, B., Adegbola, R., Thammina, C. and Naidu, R.A. 2021. First report of grapevine rupestris vein feathering virus in grapevines from Washington State. Plant Disease 105:717.</p><br /> <p>Cieniewicz, E., Poplaski, V., Brunelli, M., Dombroswkie, J. and Fuchs, M. 2020. Two distinct Spissistilus festinus genotypes in the United States revealed by phylogenetic and morphological analyses. Insects11:80; doi:10.3390/insects11020080.</p><br /> <p>Dahan, J., Thompson, B.D., Lee, J., and Karasev, A.V. 2021. First report of grapevine rupestris vein feathering virus in wine grapes in Idaho. Plant Disease 105: published on-line May 2, 2021 (https://doi.org/10.1094/PDIS-04-21-0728-PDN).</p><br /> <p>Davis TJ, Gomez MI, Harper SJ, Twomey M. (2020) Potential economic benefits of using certified clean hop plants vs. hop stunt viroid disease. Cornell University, December 2020.</p><br /> <p>Delic, D., Radulovic, M., Vakic, M., Sunulahpašić, A., Villamor, D.E.V. and Tzanetakis, I.E. 2020. First Report of black currant reversion virus and gooseberry vein banding associated virus in currants in Bosnia and Herzegovina. Plant Disease 104:2036</p><br /> <p>Delić, D., Radulović, M., Vakić, M., Sunulahpašić, A., Villamor, D.E.V. and Tzanetakis, I.E. 2020. Raspberry leaf blotch emaravirus in Bosnia and Herzegovina: population structure and systemic movement. Molecular Biology Reports 47: 4891–4896</p><br /> <p>Diaz-Lara, A., Dangl, G., Yang, J., Golino, D. A., and Rwahnih, M. A. 2021. Identification of grapevine Pinot gris virus in free-living Vitis spp. located in riparian areas adjacent to commercial vineyards. Plant Disease. DOI: 10.1094/pdis-10-20-2121-sc*</p><br /> <p>Diaz-Lara, A., Erickson, M.T., Golino, D., and Al Rwahnih, M. Design and validation of a universal reverse transcription PCR (RT-PCR) assay for vitiviruses infecting grapevine. American-Phytopathological-Society (APS) Plant Health Annual Meeting (Plant Health) Virtual meeting Jul 31-Aug 4, 2020.*</p><br /> <p>Diaz-Lara, A., Mollov, D., Golino, D. and Al Rwahnih, M., 2020. Detection and characterization of a second carlavirus in Rosa sp. Archives of Virology 11:1-3. DOI: 10.1007/s00705-020-04864-4*</p><br /> <p>Diaz-Lara, A., Mollov, D., Golino, D., and Al Rwahnih, M. 2020. Complete genome sequence of rose virus A, the first carlavirus identified in rose. Archives of Virology. 165:241–244. DOI: 10.1007/s00705-019-04460-1*</p><br /> <p>Diaz-Lara, A., Mosier, N.J., Stevens, K., Keller, K.E. and Martin, R.R. 2020. Evidence of Rubus yellow net virus integration into the red raspberry genome. Cytogenetic and Genomic Research 160:329-334.</p><br /> <p>Diaz-Lara, A., Stevens, K., Hwang, M., Golino, D., and Al Rwahnih, M. Discovery of negative sense RNA viruses in grapevine via high throughput sequencing. American- Phytopathological-Society (APS) Plant Health Annual Meeting (Plant Health) Virtual meeting Jul 31-Aug 4 2020.*</p><br /> <p>DuPont ST, Harper SJ. (2020) Better disease detection: Scouting and sampling for X phytoplasma and little cherry virus in 2020. Goodfruit Grower.</p><br /> <p>Finn, C.E., Strik, B.C., Yorgey, B.M., Peterson, M.E., Jones, P.A., Buller, G., Lee, J., Bassil, N.V. and Martin, R.R. 2020. ‘Galaxy’ thornless semierect blackberry. Hortscience https://doi.org/10.21273/HORTSCI14985-20</p><br /> <p>Finn, C.E., Strik, B.C., Yorgey, B.M., Peterson, M.E., Jones, P.A., Buller, G., Serce, S., Lee, J., Bassil, N.V. and Martin, R.R. 2020. ‘Eclipse’ thornless semi-erect blackberry. Hortscience 55:749-754. https://doi.org/10.21273/HORTSCI14891-20</p><br /> <p>Finn, C.E., Strik, B.C., Yorgey, B.M., Peterson, M.E., Jones, P.A., Lee, J., Bassil, N.V. and Martin, R.R. 2020. ‘Twilight’ thornless semi-erect blackberry. https://doi.org/10.21273/HORTSCI14992-20</p><br /> <p>Flasco, M., Hoyle, V., Cieniewicz, E.J., Roy, B.G., McLane, H.L., Perry, K.L., Loeb, G., Nault, B., Heck M. and Fuchs, M. 2021. Grapevine red blotch virus is transmitted by the three-cornered alfalfa hopper in a circulative, nonpropagative transmission mode with unique attributes. Phytopathology <a href="https://doi/org/10/1094/phyto-02-21-0061-r">https://doi/org/10/1094/phyto-02-21-0061-r</a></p><br /> <p>Fuchs, M., Almeyda, C. V., Rwahnih, M. A., Atallah, S. S., Cieniewicz, E. J., Farrar, K., Foote, W., Golino, D.A., Gómez, M., Harper, S. and Kelly, M. 2021. Economic Studies Reinforce Efforts to Safeguard Specialty Crops in the United States. Plant Disease. 105:14–26. DOI: 10.1094/pdis-05-20-1061-fe*</p><br /> <p>Gao, Z., Khot, L.R., Naidu, R.A. and Zhang, Q. 2020. Early detection of grapevine leafroll disease in a red-berried wine grape cultivar using hyperspectral imaging. Computers and Electronics in Agriculture 179:105807.</p><br /> <p>Green, J.C., Rwahnih, M.A., Olmedo-Velarde, A., Melzer, M.J., Hamim, I., Borth, W.B., Brower, T.M., Wall, M. and Hu, J.S., 2020. Further genomic characterization of pineapple mealybug wilt-associated viruses using high-throughput sequencing. Tropical Plant Pathology 45:64-72.</p><br /> <p>Hamilton A, Harper SJ & Critzer F. (2020) Optimization of a Method for the Concentration of Genetic Material in Bacterial and Fungal Communities on Fresh Apple Peel Surfaces. Microorganisms 8(10): 1480.</p><br /> <p>Hamim, I., Borth, W.B., Suzuki, J.Y. et al. Molecular characterization of tomato leaf curl Joydebpur virus and tomato leaf curl New Delhi virus associated with severe leaf curl symptoms of papaya in Bangladesh. Eur J Plant Pathol 158, 457–472 (2020). https://doi.org/10.1007/s10658-020-02086-7</p><br /> <p>Hernandez, R.N., Isakeit, T., Al Rwahnih, M., Hernandez, R. and Alabi, O.J., 2021. First report of Cucurbit chlorotic yellows virus infecting cantaloupe (Cucumis melo L.) in Texas. Plant Disease, (ja). DOI: 10.1094/pdis-02-21-0249-pdn</p><br /> <p>Hernandez, R.N., Isakeit, T., Al Rwahnih, M., Hernandez, R. and Alabi, O.J., 2021. First report of squash vein yellowing virus naturally infecting butternut squash (Cucurbita moschata) in Texas. Plant Disease, (ja). DOI: 10.1094/pdis-02-21-0378-pdn</p><br /> <p>Hily, J.M., Poulicard, N., Kubina, J., Reynard, J.S., Garcia, S., Spilmont, A.S., Fuchs, M., Lemaire, O. and Vigne, E. 2021. Metagenomic analysis of nepoviruses: diversity, evolution and identification of a hitherto undescribed putative amino acid motif for host range in subgroup A species. Archives of Virology, in press.</p><br /> <p>Ho, T., Broome, J. C., Buhler, J.P., O’Donovan, W., Tian, T., Diaz-Lara, A., Martin, R.R., Tzanetakis, I.E., 2021. Characterization of endogenous Rubus yellow net virus in Raspberries. bioRxiv 2021.06.17.448838; doi: https://doi.org/10.1101/2021.06.17.448838</p><br /> <p>Hoffmann M, Talton W, Nita M, Jones T, Al Rwahnih M, Sudarshana MR and Almeyda CV. 2020. First Report of Grapevine leafroll-associated virus 3 (GLRaV-3) in Vitis vinifera in North Carolina. https://doi.org/10.1007/s42161-020-00710-3</p><br /> <p>Hoffmann M, Talton W, Nita M, Jones T, Al Rwahnih M, Sudarshana MR and Almeyda CV. 2020. First Report of Grapevine red blotch virus, the Causal Agent of Grapevine Red Blotch Disease, in Vitis vinifera in North Carolina. https://doi.org/10.1094/PDIS-07-19-1539-PDN</p><br /> <p>Jarugula, S., Chingandu, N., Adiputra, J., Bagewadi, B., Adegbola, R., Thammina, C. and Naidu, R.A. 2021. First Report of grapevine red globe virus in grapevines in Washington State. Plant Disease 105:717.</p><br /> <p>Kwon, S.J., Bodaghi, S., Dang, T., Gadhave, K.R., Ho, T., Osman, F., Al Rwahnih, M., Tzanetakis, I.E., Simon, A.E. and Vidalakis, G., 2021. Complete nucleotide sequence, genome organization and comparative genomic analyses of citrus yellow-vein associated virus (CYVaV). Frontiers in Microbiology, 12: 1371.</p><br /> <p>Larrea-Sarmiento A, Olmedo-Velarde A, Wang X, Borth W, Matsumoto TK, Suzuki JY, Wall MM, Melzer MJ, Hu JS. A novel ampelovirus associated with mealybug wilt of pineapple (Ananas comosus var. comosus). Virus genes. 2021. Accepted for publication.</p><br /> <p>Larrea-Sarmiento, A., Olmedo-Velarde, A., Green, J. C., Al Rwahnih, M., Wang, X., Li, Y.-H., et al. 2020. Identification and complete genomic sequence of a novel sadwavirus discovered in pineapple (Ananas comosus). Archives of Virology. 165:1245–1248. DOI: 10.1007/s00705-020-04592-9</p><br /> <p>Lee, J., Rennaker, C.D., Thompson, B.D., and Karasev, A.V. 2021. Influence of Grapevine red blotch virus (GRBV) on Idaho ‘Syrah’ grape composition. Scientia Horticulturae 282: 110055 (https://doi.org/10.1016/j.scienta.2021.110055).</p><br /> <p>Mulenga, R. M., Miano, D. W., Kaimoyo, E., Akello, J., Nzuve, F. M., Rwahnih, M. A., et al. 2020. First Report of Southern Bean Mosaic Virus Infecting Common Bean in Zambia. Plant Disease. 104:1880–1880. DOI: 10.1094/pdis-11-19-2390-pdn</p><br /> <p>Naidu, R.A. 2020. Viruses of Grapevines. In: 2020 Pest Management Guide for Grapes in Washington. (pp. 53-56). EB0762 (Extension).</p><br /> <p>Olmedo-Velarde, A., Hu, J., and Melzer, M.J. 2021. A virus infecting Hibiscus rosa-sinensis represents an evolutionary link between cileviruses and higreviruses. Frontiers in Microbiology DOI: 10.3389/fmicb.2021.660237</p><br /> <p>Olmedo-Velarde, A., Waisen, P., Kong, A.T., Wang, K.-H., Hu, J.S., and Melzer, M.J. 2021. Characterization of taro reovirus and is status in taro (Colocasia esculenta) germplasm from the Pacific. Archives of Virology (in press)</p><br /> <p>Osterbaan, L.J., Hoyle V., Curtis, M., DeBlasio, S., Heck, M., Rivera, K. and Fuchs, M. 2021. Identification of protein interactions of grapevine fanleaf virus RNA-dependent RNA polymerase during infection of Nicotiana benthamiana by affinity purification and tandem mass spectrometry. Journal of General Virology, in press.</p><br /> <p>Schoelz, J., Volenberg, D., Adhab, M., Fang, Z., Klassen, V., Spinka, C., et al. 2020. A Survey of Viruses Found in Grapevine Cultivars Grown in Missouri. American Journal of Enology and Viticulture. 72:73–84. DOI: 10.5344/ajev.2020.20043</p><br /> <p>Silva, J. M. F., Fajardo, T. V. M., Rwahnih, M. A., and Nagata, T. 2020. First Report of Grapevine Associated Jivivirus 1 Infecting Grapevines in Brazil. Plant Disease. DOI: 10.1094/pdis-08-20-1689-pdn</p><br /> <p>Soltani, N., Golino, D. A., and Al Rwahnih, M., 2021. First report of Rose leaf rosette-associated virus infecting rose (Rosa spp.) in California, USA. Plant Disease. DOI: 10.1094/pdis-10-20-2268-pdn*</p><br /> <p>Spak. J., Koloniouk, I., and Tzanetakis I.E. 2021. Graft-transmissible diseases of Ribes – pathogens, impact and control. Plant Disease 105: 242-250</p><br /> <p>Tamisier L., Haegeman A., Foucart Y., Fouillien N., Al Rwahnih M., Buzkan N., …., Massart S., (2021, March 23). Semi-artificial datasets as a resource for validation of bioinformatics pipelines for plant virus detection. Zenodo. DOI: 10.5281/zenodo.4584718</p><br /> <p>Tan Sh, Osman F, Bodaghi S, Dang T, Greer G, et al. (2019) Full genome characterization of 12 citrus tatter leaf virus isolates for the development of a detection assay. PLOS ONE 14(10): e0223958. <a href="https://doi.org/10.1371/journal.pone.0223958">https://doi.org/10.1371/journal.pone.0223958</a></p><br /> <p>Tzanetakis, I.E. and Sabanadzovic S. Fig viruses, In ‘The Fig: Botany, Production and Uses’, CABI, in press</p><br /> <p>Tzanetakis, I.E., Sabanadzovic S. and Valverde R. 2020. Amalgaviruses (Amalgaviridae) In Reference Module in Life Sciences 2020. https://doi.org/10.1016/B978-0-12-809633-8.21527-6</p><br /> <p>Villamor, D.E.V., Keller, K.E., Martin, R.R. and Tzanetakis, I.E. 2021. Comparison of high throughput sequencing to standard protocols for virus detection in berry crops. Plant Disease (in Press).</p><br /> <p>Wright AA, Shires M, Beaver C, Bishop Gm DuPont ST, Naranjo R, Harper SJ (2021b) The effect of Candidatus Phytoplasma pruni infection on sweet cherry fruit. Phytopathology, Accepted 5/4/21.</p><br /> <p>Wright AA, Shires M, Harper SJ (2021a) Little cherry virus-2 titer and distribution in Prunus avium. Archives of Virology, 166: 1415–1419.</p><br /> <p>Wu, Q., Habili, N., Constable, F., Al Rwahnih, M., Goszczynski, D. E., Wang, Y., et al. 2020. Virus pathogens in Australian vineyards with an emphasis on Shiraz disease. Viruses. 128: 8. DOI: 10.3390/v12080818</p><br /> <p>Zhang S. and Vrient A. (2020): Chapter 8 - Rapid Detection of Plant Viruses and Viroids in Awasthi LP (ed) Applied Plant Virology - Advances, Detection, and Antiviral Strategies, pp. 101-109, Academic Press.</p><br /> <p> </p>Impact Statements
- Members of WERA-20 group have published peer-reviewed articles in high-impact scientific journals for dissemination of research-based knowledge benefiting research, extension communities and shared research-based knowledge with growers and stakeholders via extension publications, outreach presentations at grower field days, workshops and commodity-specific annual meetings (see list of publications).