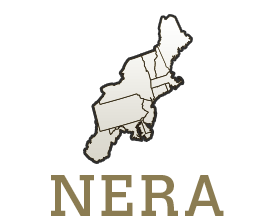
NE1030: Characterization and Mechanisms of Plant Responses to Ozone in the U.S.
(Multistate Research Project)
Status: Inactive/Terminating
NE1030: Characterization and Mechanisms of Plant Responses to Ozone in the U.S.
Duration: 10/01/2007 to 09/30/2012
Administrative Advisor(s):
NIFA Reps:
Non-Technical Summary
Statement of Issues and Justification
(1) The need: Surface level (tropospheric) ozone (O3) is all pervasive and is considered to be the most important phytotoxic air pollutant across many parts of the US and rest of the world (Krupa et al. 2001; US EPA, 2006). Despite the national air quality regulations aimed at controlling ground level O3 pollution, it continues to be of major concern for crop production and forest health in the northeastern US and elsewhere (US EPA, 2006). As of 2005, there were 455 counties in the US, mainly along the eastern seaboard, the Midwest and the south central regions and California that were in violation of the current National Air Quality standard (US EPA, 2006). More importantly, every region of the US except for parts of the Pacific Northwest and Great Plains experiences ambient phytotoxic O3 levels periodically during the growing season. There are 12 major crop species and 8 important tree species within the northeast and elsewhere that are O3-sensitive (Krupa et al. 1998). These crops include alfalfa, barley, bean, clover, cotton, grape, oat, peanut, potato, soybean, tomato, and wheat. Ozone-sensitive tree species include aspen, birch, cottonwood, locust, pine, sycamore, and tulip poplar. In addition to causing visible foliar injury on sensitive plant species, chronic exposures to O3 can result in reductions in crop yield and quality and in forest growth and productivity (Krupa et al. 2001). Current estimates are that O3 alone causes $3-5 billion in crop loss annually (US EPA, 2006; Fiscus et al., 2005). Similar estimates are not available at the present time for forests and native vegetation, although the adverse effects of O3 on our national parks and forests have been documented (Chappelka et al. 1997, 1999; Kohut et al. 1997; Percy et al. 2003; EPA, 2006). There continues to be a critical need to obtain quantitative data on O3 exposure-plant response relationships under ambient conditions and in a changing climate. According to the US EPA's Clean Air Science Advisory Committee (CASAC), the quantitative evidence linking specific ozone concentrations to specific vegetation effects - especially at the complex ecosystem level - must continue to be characterized as having high uncertainties due to the lack of data for verification of those relationships (CASAC, 2006, http://www.epa.gov/sab/pdf/casac-07-001.pdf). Similarly, studies are needed to explain the mechanisms of when and why plants are susceptible or tolerant to O3 stress. These efforts can assist in better defining the geographic extent, magnitude and economic consequences of the adverse effects of tropospheric O3 on crops, forests and natural resources. (2) Importance of work and consequences if not done: There is an ongoing need for research and assessment of O3 effects on crops and forests to determine severities of impact, estimate economic costs, project future effects, and possibly improve plant tolerance to ozone damage. This is especially relevant to current agricultural practices where new crop cultivars, many of which are genetically-modified, are being placed into production without specific consideration of their sensitivity to ambient O3. Crop breeding programs will need to incorporate selection of traits for improved plant tolerance to ambient O3 to maintain and increase crop yields and nutritive quality. In addition, recent evidence suggests that ambient O3 may be damaging forests ecosystems by injuring under-story vegetation, potentially altering plant-herbivore relationships, and worsening soil moisture conditions and stream flow during drought periods. Lastly, there has also been very little consideration of how rising atmospheric CO2 levels could alter plant responses to O3 and vice-versa. This issue is important to air quality regulators, crop producers, ecosystem managers, and climate modelers. As has been shown recently this is a major uncertainty in projecting future national and global food supply (Long et al. 2006). (3) Technical feasibility of the work: The NE-1013 Multi-state Project represents the single largest cooperative work on the effects of O3 on plants among experiment stations, other academic institutions and governmental agencies in the US. NE-1013 scientists bring together a unique diversity of expertise and interests that vary from molecular biology to plant physiology to field studies to numerical modeling. They have a demonstrated record in O3 research and a strong sense of collaboration and scientific integration. During the first four years of the current project (2002-2006), NE-1013 members have published 102 refereed research papers and book chapters (also see publications in: http://www.ncsu.edu/project/usda-ne-1013/) Technical feasibility of the proposed work is supported by the accomplishments of the current NE-1013 project: (a) assessment of physiological and growth responses of mature trees and under-story species to a range of ambient O3 exposures in forested and natural ecosystems in the Southern US, including those designated as Class I air quality (pristine) areas under the Clean Air Act; (b) development, testing and establishment of a nationwide project involving O3-sensitive and resistant snap bean cultivars to evaluate ambient O3 impacts on plants; (c) elucidation of molecular and biochemical mechanisms of O3 phytotoxicity; (d) demonstration that current ambient O3 concentrations can cause substantial yield losses (-15%) to the Midwest and southeastern soybean crop; (e) demonstration that O3 lowers the nutritive quality of herbaceous vegetation (forage) for ruminant animals; (f) experimental evidence that elevated CO2 ameliorates the deleterious effects of O3 on growth and yield of many crops, but its effectiveness declines with increasing O3 concentrations; (g) research showing that long-lasting elevated levels of O3 and increased N deposition increase susceptibility of trees to drought and bark beetle attacks, promote build-up of dead biomass at forest floor, and predispose forests to catastrophic fires; and, (h) establishment of the project's webpage: http://www.ncsu.edu/project/usda-ne-1013/index.htm. (4) Advantages of a multi-state effort: Interactions among scientists from across the U.S who share the common goal of understanding and predicting plant responses to O3 will be highly advantageous for several reasons: i) no single institution can fulfill the stated objectives in isolation because many of the components from molecular to ecosystem responses are interrelated and are very large in scale; ii) plant responses to ambient O3 are highly influenced by environmental and genetic factors that need to be studied in a wide range of environments; and, iii) the breadth of knowledge and experience of scientists participating in NE-1013 will lead to unique approaches and solutions to issues that are beyond the scope of a single researcher or even a single institution. (5) Impacts of work: Research by NE-1013 members has direct relevance to air quality regulatory policies and protection of the food supply and natural resources. Stakeholders include the US EPA, USDA (particularly, the Agricultural Air Quality Task Force), US National Park Service, US Forest Service, crop growers, packers and shippers, the horticulture and forest production/management sectors, urban planners and industries contributing to the air emissions of O3 precursors. At the local level, clientele include home and Master Gardeners, landscape and nursery managers, arborists and managers of public parks and recreational lands, individual crop producers, and state and local air quality regulators.
Related, Current and Previous Work
Current Objective 1: Spatial - temporal variability of adverse O3 effects. Until now, most exposure experiments have focused on the use of controlled environment, greenhouse or field chambers (US EPA 2006). It is difficult to directly extrapolate results from such studies to ambient conditions (Jetten 1992). In experiments utilizing ambient air exposures in New York, North Carolina, and California, O3 concentrations were sufficient to cause respectively a 25%, 39% and >50% biomass reduction in sensitive versus tolerant clones of white clover (Trifolium repens) (McGrath 2000; Heagle et al. 1995). Similarly, in North Carolina, a 50% yield reduction was observed in sensitive versus tolerant cultivars of snap bean (Phaseolus vulgaris) (Burkey et al., 2005). Regarding tree species, the effects of ambient O3 exposures on the growth of loblolly pine (Pinus taeda) were examined under chamber-less conditions, using an O3 protective chemical, ethylene diurea (EDU). Application of EDU increased all growth parameters measured, showing the negative impacts of ambient O3 (Manning et al., 2003). Free-air experiments with O3 in progress with soybean (Morgan et al. 2006) and northern hardwoods (Karnosky et al. 2003) have also found biomass and yield reductions that can be attributed to O3. However, considerable uncertainties continue to exist regarding the influences of O3 exposure dynamics, concurrent environmental conditions, and relative species/cultivar sensitivity on plant responses to ambient O3. Current Objective 2: Effects of O3 on structure, function and diversity of plant communities. In regard to the competitive relationships between native plants, the rate of blackberry (Rubus cuneifolus): broomsedge (Andropogon virginicus) litter decomposition was reduced with increasing O3 concentrations, with implications in altering nutrient cycling and thus, biological diversity (Kim et al. 1998). Decomposition of soybean leaf residues was also found to be slower following growth at elevated O3 (Booker et al., 2005). Chappelka (2002) found initial acceleration in flowering in blackberry with elevated O3. More flowers were initiated and peak production occurring sooner than all other treatments. Total numbers of fruits were not significantly different among treatments, however, numbers of large and ripe fruits were greatest in charcoal-filtered air and nonfiltered air and least in the added O3 treatments. These effects may have serious implications regarding biodiversity of plant communities. Similarly, decreased yield and quality of O3-exposed bahiagrass (Paspalum notatum) (Muntifering et al. 2000) and sericea lespedeza (Lespedeza cuneata) (Powell et al. 1999) were of sufficient magnitude to have nutritional implications in their utilization by mammalian herbivores (Krupa et al. 2004). Lin et al. (2007) reported that proportion of forbs increased from 23.4 to 36.2%, grasses decreased from 67.6 to 60.5%, and legumes decreased from 8.9 to 3.3% of biomass harvested from an extensively managed, semi-natural pasture that had been fumigated for five consecutive growing seasons with 1.5 × ambient O3 concentration. Except for year and seasonal differences, there was no effect of elevated O3 on forage N concentration. However, there were differences between control and O3-enriched plots in forage concentrations of cell-wall constituents and in nutritive quality. In contrast to other reports of negative effects of elevated O3 on nutritive quality resulting from altered leaf chemistry in individual plant species, this is the first report of altered nutritive quality associated with O3-driven shifts in proportions of plant functional groups in a complex, species-rich pasture. Current Objective 3: Joint effects of O3 with other growth regulating factors on crop and tree growth and productivity. There has been relatively little consideration of how rising atmospheric CO2 levels could alter plant responses to O3 and vice-versa. This issue is important to air quality regulators, ecosystem managers, and climate modelers. Field studies using open-top chambers showed that CO2 ameliorated the deleterious effects of O3 on soybean, wheat, peanut and cotton growth and yield (Booker et al., 2006; Booker et al., 2005; Fiscus et al., 2002). In a northern hardwood community, biomass accumulation of trembling aspen exposed to elevated CO2 (550 ppm) and O3 (60 ppb) for six years was not significantly different from that of the control in a free-air treatment system (King et al. 2005). This response occurs primarily due to CO2-induced reductions in stomatal conductance and hence O3 uptake (Booker and Fiscus, 2005; Booker et al., 2006; Fiscus et al., 1997). Research suggests, however, that CO¬2-induced stimulation of photosynthesis and antioxidant synthesis is also involved in protective effects against O3 injury (Booker and Fiscus, 2005). Elevated CO2 and O3 interactions are dependent on concentration of the gases and sensitivity of the cultivar to O3. Increases in CO2 may not protect O3-sensitive plants such as peanut at expected higher tropospheric O3 concentrations in the future (Booker et al., 2006). Protein and oil content of peanut seeds were not affected by O3 or CO2 treatments, but fatty acid composition was altered. In regard to agro-ecosystem level responses, little information is yet available. Ecosystem level responses to elevated CO2 and O3 are highly dependent on complex interactions between plants, associated soil microbial communities, and soil resource availability. However, growth suppression by O3 appears likely to detract from soil carbon sequestration and soil quality while elevated CO2 could enhance these factors. Stands of northern hardwoods have the potential for substantially greater C input to soil due to greater fine-root production under elevated CO2, although greater fine-root biomass will be accompanied by greater soil C efflux as soil respiration. Ozone tended to decrease the above responses to elevated CO2 (King et al., 2001). At the free-air experiment at the University of Illinois (SoyFACE), soybean was treated in large plots with combinations of CO2 and O3 at ambient and predicted levels for 2040-50. The added O3 treatment of 20% above ambient lowered yield by about 20% (Morgan et al. 2006), while elevated CO2 attenuated the O3 effect (McConnaughay et al. 2006). Sub-plots of 20 different soybean germplasm lines from the USDA collection were included in each plot. Yield losses were seen in all germplasm grown, which included current production cultivars and breeding lines. These results confirm that developing lines resistant to O3 is not only needed to protect yield for the future, but also the present. While all soybean germplasm appears affected, some lines are clearly more affected than others and a priority will now be to use the system to identify more tolerant germplasm and understand the genetic and molecular basis of tolerance. The findings provide the first fully open air confirmation that current O3 concentrations are causing very substantial losses to the Midwest soybean crop; and that this will worsen under projected future elevations in the area. Current Objective 4: Molecular and physiological basis of O3 toxicity and tolerance. Ozone is known to adversely affect a number of critical plant processes such as: photosynthesis, water use efficiency, carbon allocation, dry matter production and yield (US EPA, 2006). An intriguing aspect of O3 is its mechanism of action. Ozone is highly reactive, unstable and does not accumulate in plant tissue. Ozone enters the leaf primarily through the stomata where it reacts with cellular components. The organic radicals and various reactive forms of oxygen that are generated through O3 decomposition damage proteins and membranes leading to impaired physiological function and cell death (US EPA, 2006). An oxidative burst may occur as the initial reaction to O3 exposure. In O3-tolerant genotypes, either the oxidative burst is suppressed (Schraudner et al. 1998) or oxidative damage is highly localized (Koch et al. 2000), thereby restricting the extent of foliar lesions. Ozone impairs growth primarily by inhibiting photosynthesis and perhaps translocation processes, which limit availability of photosynthate needed for biomass production (Fiscus et al., 2005; Long and Naidu, 2002). Root production appears particularly susceptible to O3 exposure (Cooley and Manning, 1987; Grantz et al., 2006). Allocation of carbon and energy resources to detoxification and repair processes in O3-stressed plants likely detracts from growth as well (Amthor, 1988; Heath and Taylor, 1997). Experiments with Arabidopsis thaliana mutants and pairs of O3-sensitive and tolerant plant lines suggest that many detrimental effects of O3 are initiated and mediated by increased reactive oxygen species formation along with changes in plant hormone levels, antioxidant metabolism, cellular ion fluxes, and gene expression (US EPA, 2006). The plant antioxidant system (Burkey 1999; Mittler and Zilinskas, 2004), which scavenges naturally occurring reactive oxygen compounds, could function as a primary mechanism to alleviate the oxidative burden resulting from O3 exposure. The ascorbate (vitamin C)-glutathione cycle has been the most intensively studied and generally there is a positive correlation of O3 tolerance with levels of antioxidants and antioxidant enzyme activities (Conklin and Last 1995). For example, mutants that are deficient in vitamin C are very sensitive to O3 (Conklin et al. 1996), as are plants that have been genetically manipulated to produce less ascorbate peroxidase, an enzyme that uses vitamin C to detoxify hydrogen peroxide (Orvar and Ellis 1997). Research is underway to genetically dissect molecular modes of action involving O3 toxicity with the aim of developing O3 tolerant plants. Current Objective 5: Development of numerical models. During the late 1970s and mid 1980s, two major chamber-based O3-crop loss assessment programs were established: The US EPA's National Crop Loss Assessment Network (NCLAN) (Heck et al. 1988) and the European Open-Top Chambers Programme (EOTCP) (Jäger et al. 1993; Fuhrer et al. 1997). Statistical models were developed to define cause-effect relationships. Further refinement of these models will require incorporation of micrometeorological conditions that mediate both the dynamic variations in hourly O3 concentrations, deposition to vegetation, and the uptake by foliage, and the feedback among plant stress, repair and compensation (Grünhage and Haenel 1997; Krupa and Kickert 1997). In recent years, the research thrust in Europe has been on developing cause-effect relationships through micro-meteorological O3 flux-based modeling. At this time extensive validation with independent sets of data remains to be developed and the utility of this approach must be confirmed. The development, testing and establishment by NE-1013 scientists of a multi-regional study involving O3-sensitive and resistant snap bean cultivars to evaluate ambient O3 impacts on plants is a first step toward establishing cause and effect relationships under chamber-less conditions, with concurrent changes in multiple growth regulating parameters (biotic and abiotic). After 5 years of field studies (2000-2005) with alfalfa, an NE-1013 member (MN) has developed a non-linear, multi-variant model that apportions the contributions of O3 among other environmental growth regulating parameters (T, RH, precipitation etc.) to the variability of crop yield (Krupa, unpublished). Application of this model to the snap bean trials is anticipated in the next phase of this project.
Objectives
-
Describe the spatial - temporal characteristics of the adverse effects of current ambient O3 levels on crop productivity, including the development of numerical models to establish cause-effect relationships that apportion the ozone contribution.
-
Assess the effects of O3 on structure, function and inter-species competition in managed and native plant populations, including alterations in their nutrient quality.
-
Examine the joint effects of O3 with other growth regulating factors (e.g., CO2, temperature) that are expected to vary with ongoing climate change on crop growth and productivity.
-
Examine the physiological and molecular basis of O3 toxicity and tolerance in plants.
-
Develop appropriate educational tools (including handouts, digital media, demonstration sites, distance education) and conduct advanced training for K-12 public school teachers, college level instructors, and outreach educators regarding the effects of ambient O3 pollution on plants.
Methods
A. Proposed Research: Objective #1 (Effects on crop productivity) will be addressed through the use of multiple crop models. Ozone sensitive (S) and tolerant (R) snap bean cultivars are under evaluation in open field conditions to assess the effects of current ambient O3 exposures on crop growth and yield. This multi-year study will utilize common experimental protocols and crop management practices among the multi-institutional collaborators (MA, MD, NC, NJ, NY-LI, PA). In addition to the measurements of plant growth and yield parameters, co-located instrumentation will provide hourly data on the ambient O3 concentrations and climate variables (radiation, temperature etc.). Sensitive and resistant crop cultivar data will be treated separately in establishing relationships with the independent variables. A non-chamber exposure study (IL) on soybean cultivars (SoyFACE), will have the continuing objective of screening, selecting, and engineering O3-tolerant soybean cultivars. A specific focus in the snap bean cultivar studies (MA) will be re-defining the exposure-response relationships by examining crop response to low to moderate O3 concentrations (Measurement of Progress and Results
Outputs
- Spatial and temporal patterns of crop and tree responses (foliar injury and/or changes in growth and productivity) to O3 will be described and mapped across the northeast region and other areas to assess the extent of regional scale adverse impacts.
- Select indicator native plant species fitness (indicative of biodiversity) and changes in community structure due to O3 exposures will be described. Impact of O3 on biodiversity will be quantified and information transferred to natural resource managers and federal and state air quality regulators.
- Crop and tree responses to the interactive effects of O3 with other growth regulating factors will be used to predict production changes over time, as a function of ongoing climate change. Information will be provided to both public and private urban and industry planners.
- Information on the mechanisms of O3 toxicity and tolerance in plants and alterations in their functional physiology to O3 exposures will lead to the identification of genes that can mitigate the effects of O3 and other forms of oxidative stress. Once identified, these can be linked to expression levels in impacted ecosystems. This will lead to developing and/or identifying tolerant cultivars and varieties for the production sector and documenting the role of specific mechanistic adaptations to individual species fitness and ecosystem function.
- New statistical methodologies and derived information will establish relationships between O3 exposures and plant responses that incorporate the spatial and temporal stochasticity of such relationships. Such information will be transferred to regulatory policy makers.
Outcomes or Projected Impacts
- Scientific basis for developing State and National Ambient Air Quality Standards for O3 to protect cultivated (crops) or managed (forests) and native vegetation (bio-diversity).
- Environmental and quality of life benefits through post-regulatory (air quality standards) impact reduction and assessment.
- Improved understanding of regional scale issues in resource management.
- Improved understanding of plant response to oxidative stress in general and concomitant assistance in developing tolerant plants through breeding and other approaches.
- Early detection of ecosystems under O3 stress.
Milestones
(2008): Analyze 2007 growing-season results from the collaborative field project on O3 sensitive and tolerant cultivars of snap bean. Establish biodiversity plots where appropriate and begin to examine plant competition and changes in community structure. Continue on-going greenhouse, open-top chamber and free-air exposure studies. Continue collection and characterization of air quality data. Continue physiological-molecular biology studies.(2009): Continue on-going greenhouse, open-top chamber and ambient O3 exposure studies. Expand studies on plant nutritive quality for ruminants. Broaden physiological (functional aspects) and molecular biology studies on the mechanisms of O3 tolerance and sensitivity. Develop one or more interdisciplinary research proposals for extramural funding based on collaborative results.
(2010): Collect and analyze bio-diversity data. Continue work from 2008 - 2009. Test ambient O3 exposure-alfalfa response model for snap bean.
(2011): Continue field and laboratory studies from 2009-2010. Expand ambient O3 exposure - snap bean and other species response models.
(2012): Continue work from 2008-2011, complete and apply O3 - snap bean/soybean response models. Assess data from: (a) controlled exposure studies, (b) foliar injury surveys, (c) O3 exposures and tree/forest response analyses and (d) bio-diversity studies. Develop a database on spatial and temporal distributions of adverse effects of O3 on vegetation and quantitative definitions of cause-effect relationships. Based on physiological-molecular biology studies, develop tools that can explain mechanisms of plant stress and tolerance to O3.
Projected Participation
View Appendix E: ParticipationOutreach Plan
Traditional aspects of communication include publications of the results in peer-reviewed international journals on a regular basis, presentations at national and international scientific meetings, conferences, symposia and workshops. Results will also be used in graduate level teaching. Results will be highlighted at: http://www.ncsu.edu/project/usda-ne-1013/). Non-traditional education will be continued through linkages with Master Gardeners training, interactive presentations to arborist groups and county extension educators and participation in Continuing Education Programs for the public and in field days. PA and NC have Web pages for diagnosis of O3 injury to plants (e.g., http://www.ces.ncsu.edu/depts/pp/notes/Ozone/ozone.html). CA has a diverse web page, with links to these and other resources: http://airqualityeffects.uckac.edu. Several members have established physical sites at which groups of farmers, farm advisors, school children, policy makers and regulators and members of the public may view demonstration projects involving plants exposed to a range of O3 concentrations. These sites function in various ways with ongoing programs, meetings, and educational functions. Linkage to NASA environmental learning program [NASA- GLOBE (Global Learning and Observations to Benefit the Environment) project (http://viz.globe.gov/viz-bin/home.cgi?l=en&b=g&rg=n)] provides an avenue for national and global dissemination of our results to schools.
Organization/Governance
Plans to Expand Project Membership: Current members represent both AES and non-AES scientists. Virtually all of the leading O3-plant effects researchers in the US are members of the project. Efforts are underway to identify AES-Outreach/Extension and USDA Forest Service personnel involved in O3-vegetation impacts assessment for inclusion in the project. In addition, in establishing a close link with scientists in the European Union, several researchers from the UK, Germany and Switzerland have been attending the Annual Technical Committee Meetings. Governance: The recommended Standard Governance for multi-state research activities includes the election of a Chair, a Chair-elect, and a Secretary. All officers are to be elected for at least two-year terms to provide continuity. Administrative guidance will be provided by an assigned Administrative Advisor and a CSREES Representative. That protocol is in place and will continue in this project.
Literature Cited
Amthor, J.S. (1988). Growth and maintenance respiration in leaves of bean (Phaseolus vulgaris L.) exposed to ozone in open-top chambers in the field. New Phytologist 110:319-325. Barbo, D.N., Chappelka, A.H., Somers, G.L., Miller-Goodman, M.S. & Stolte, K. (1998). Diversity of an early successional plant community as influenced by ozone. New Phytologist 138:653-662. Booker, F.L., Burkey, K.O., Overmyer, K. & Jones, A. 2004. Differential responses of G-protein Arabidopsis thaliana mutants to ozone. New Phytologist 162:633-641. Booker, F.L., Burkey,K.O., Pursley, W.A. & Heagle, A.S. 2007. Elevated carbon dioxide and ozone effects in peanut. I. Gas-exchange, biomass, and leaf chemistry. Crop Science: In press (accepted 1/9/07). Booker, F.L. & Fiscus, E.L. 2005. The role of ozone flux and antioxidants in the suppression of ozone injury by elevated carbon dioxide in soybean. Journal of Experimental Botany 56:2139-2151. Booker, F.L., Prior, S.A., Torbert, H.A., Fiscus, E.L., Pursley, W.A. & Hu, S. (2005). Decomposition of soybean grown under elevated concentrations of CO2 and O3. Global Change Biology 11:685-698. Burkey, K.O. (1999). Effects of ozone on the apoplast/cytoplasm partitioning of ascorbic acid in snap bean. Physiologia Plantarum 107:188-193. Burkey, K.O., Miller, J.E. & Fiscus, E.L. 2005. Assessment of ambient ozone effects on vegetation using snap bean as a bioindicator species. Journal of Environmental Quality 34:1081-1086. Chappelka, A., Skelly, J., Somers, G., Renfro, J. & Hildebrand, E. (1999). Mature black cherry used as a bioindicator of ozone injury. Water, Air & Soil Pollution 116:261-266. Chappelka, A.H. (2002). Reproductive development of blackberry (Rubus cuneifolius), as influenced by ozone. New Phytologist 155: 249-255. Conklin, P.L. & Last, R.L. (1995). Differential accumulation of antioxidant mRNAs in Arabidopsis thaliana exposed to ozone. Plant Physiology 109:203-212. Conklin, P.L., Williams, E.H. & Last, R.L. (1996). Environmental stress sensitivity of an ascorbic acid-deficient Arabidopsis mutant. Proceedings of the National Academy of Sciences U.S.A. 93, 9970-9974. Cooley, D.R., & Manning, W.J. (1987). The impact of ozone on assimilate partitioning in plants: a review. Environmental Pollution 47:95-113. Fiscus, E.L., J.E. Miller, F.L. Booker, A.S. Heagle and C.D. Reid. 2002. The impact of ozone and other limitations on the crop productivity response to CO2. Technology 8:181-192. Fiscus, E.L., Booker, F.L. & Burkey, K.O. (2005). Crop responses to ozone: uptake, modes of action, carbon assimilation and partitioning. Plant, Cell and Environment 28:997-1011. Fuhrer, J., Skarby, L. & Ashmore, M.H. (1997). Critical levels for ozone effects on vegetation in Europe. Environmental Pollution 97:91-106. Grantz, D.A. (2003). Ozone impacts on cotton: Towards an integrated mechanism. Environmental Pollution 126: 331-344. Grantz, D.A. & Shrestha, A. (2006). Tropospheric ozone and interspecific competition between yellow nutsedge and pima cotton. Crop Science 46: 1879-1889. Grantz, D.A., Gunn, S. & Vu, H.-B. 2006. O3 impacts on plant development: a meta-analysis of root/shoot allocation and growth. Plant, Cell and Environment 29:1193-1209. Grantz, D.A., Silva, V., Toyota, M. & Ott, N. (2003). Ozone increases root respiration but decreases leaf CO2 assimilation in cotton and melon. Journal of Experimental Botany 43: 2375-2384. Grünhage, L. & Haenel, H.-D. (1997). PLATIN: I. A model of PLant-ATmosphere INteraction for estimating absorbed doses of gaseous pollutants. Environmental Pollution 98:37-50. Heagle, A.S., Miller, J.E., Chevone, B.I., Dreschel, T.W., Manning, W.J., McCool, P.M., Morrison, C.L., Neely, G.E. & Rebbeck, J. (1995). Response of a white clover indicator system to tropospheric ozone at eight locations in the United States. Water, Air & Soil Pollution 85:1373-1378. Heath, R.L. & G.E. Taylor, G.E.Jr. 1997. Physiological processes and plant responses to ozone exposure, pp. 317-368, In Sandermann, H., Wellburn, A.R. & Heath, R.L.(eds). Forest Decline and Ozone: A Comparison of Controlled Chamber and Field Experiments, Vol. 127. Springer-Verlag, Berlin. Heck, W.W., Taylor, O.C. & Tingey, D.T. (eds.) (1988). Assessment of Crop Loss from Air Pollutants. Elsevier Applied Science, London. Jäger, H.-J., Unsworth, M., De Temmerman, L. & Mathy, P. (eds.) (1993). Effects of Air Pollutants on Agricultural Crops in Europe: Results of the European Open-Top Chambers Project. Report No. 46. Air Pollution Series of the Environmental Research Programme of the Commission of the European Communities, Directorate-General for Science, Research and Development, Brussels, Belgium. Jetten, T.H. (1992). Physical description of transport processes inside an open-top chamber in relation to field conditions. Ph.D. dissertation. Agricultural University, Wageningen, the Netherlands. Karnosky, D.F., Percy, K.E., Simpson, C. & Chappelka, A.H. (eds.). (2003). Air Pollution, Global Change and Forests in the New Millennium. Elsevier Science, Amsterdam, The Netherlands. Kim, J.S., Chappelka, A.H. & Miller-Goodman, M.S. (1998). Decomposition of blackberry and broomsedge bluestem as influenced by ozone. Journal of Environmental Quality 27:953-960. King JS, Pregitzer KS, Zak DR, Sober J, Isebrands JG, Dickson RE, Hendrey GR & Karnosky DF (2001) Fine-root biomass and fluxes of soil carbon in young stands of paper birch and trembling aspen as affected by elevated atmospheric CO2 and tropospheric O3. Oecologia 128: 237-250. King JS, Pregitzer KS, Kubiske ME, Hendrey GR, Giardina CP, McDonald EP, Karnosky DF (2005) Tropospheric O3 compromises net primary production in young stands of trembling aspen, paper birch, and sugar maple in response to elevated atmospheric CO2. New Phytologist 168:623-636. Koch, J.R., Creelman, R.A., Eshita, S.M., Seskar, M., Mullet, J.E. & Davis, K.R. (2000). Ozone sensitivity in hybrid poplar correlates with insensitivity to both salicylic acid and jasmonic acid. The role of programmed cell death in lesion formation. Plant Physiologist 123:487-496. Kohut, R., Laurence, J., King, P. & Raba, R. (1997). Identification of Bioindicator Species for Ozone and Assessment of the Responses to Ozone of Native Vegetation at Acadia National Park. Final Report NPS Acadia D-175. National Park Service, Denver, CO. Krupa, S.V. & Kickert, R.N. (1997). Considerations for establishing relationships between ambient ozone (O3) and adverse crop response. Environmental Reviews 5:55-77. Krupa, S., Muntifering, R. & Chappelka, A. (2004). Effects of ozone on plant nutritive quality characteristics for ruminant animals. The Botanica. 54:1-12. Krupa, S.V., Tonneijck, A.E.G. & Manning, W.J. (1998). Ozone. pp 2-1 - 2-28. In Recognition of Air Pollution Injury to Vegetation: A Pictorial Atlas, R.B. Flagler (ed.) Air & Waste Management Association, Pittsburgh, PA. Krupa, S., McGrath, M.T., Andersen, C.P., Booker, F.L., Burkey, K.O., Chappelka, A.H., Chevone, B.I., Pell, E.J. & Zilinskas, B.A. (2001). Ambient ozone and plant health. Plant Disease 85:4-12. Lewis, J.S., Ditchkoff, S.S., Lin, J.C., Muntifering, R.B. & Chappelka, A.H. (2006). Nutritive quality of big bluestem (Andropogon gerardii) and eastern gamagrass (Tripsacum dactylodies) exposed to tropospheric ozone. Rangeland Ecology & Mangement 59: 267-274. Lin, J.C., K. Nadarajah, M. Volk, R. B. Muntifering & J. Fuhrer. 2007. Nutritive quality of a species-rich, extensively managed pasture exposed to elevated ozone in a free-air fumigation system. Journal of Animal Science 85 (Suppl. 1): In press. Long S.P., Ainsworth E.A., Leakey A.D.B., Nosberger J. & Ort D.R. (2006) Food for thought: Lower-than-expected crop yield stimulation with rising CO2 concentrations. Science 312: 1918-1921. Long, S.P. & Naidu, S.L. (2002). Effects of oxidants at the biochemical, cell and physiological levels, with particular reference to ozone. In Air Pollution and Plant Life, J. N. B. Bell and M. Treshow, (eds.), 2nd ed. John Wiley & Sons, Ltd., Chichester, UK. Pp 69-88. Manning, W.J., Flagler, R.B. & Frenkel, M.A. (2003). Assessing plant response to ambient ozone: growth of ozone-sensitive loblolly pine seedlings treated with ethylenediurea or sodium erythorbate. Environmental Pollution 126: 73-81. McConnaughay, K., E. Brewer, A. Abts, P. Beaver, S. Morris, P. Morgan & S. Long. 2006. Farms of the future:soybean productivity and yield in a high-CO2, high-O3 world. Proceeding of 2006 Annual Crop Science Society of America meeting, Indianapolis, IN, Nov. 12-16 (abstract). McGrath, M.T. (2000). Impact of ambient ozone on clover at Long Island, New York. (Abstr.) Phytopathology 90(6):S50. Mittler, R. & Zilinskas, B.A. (2004). Activated oxygen species in multiple stress situations and protective systems. In Ecological Studies: Molecular Ecotoxicology of Plants, H. Sandermann (ed.). Springer-Verlag, Heidelberg. Pp. 51-73. Morgan, P.B., Mies, T.A., Bollero, G.A., Nelson, R.L. & Long, S.P. (2006). Season-long elevation of ozone concentration to projected 2050 levels under fully open-air conditions substantially decreases the growth and production of soybean. New Phytologist 170: 333-343. Muntifering, R.B., Crosby, D.D., Powell, M.C. & Chappelka, A.H. (2000). Yield and quality characteristics of bahiagrass (Paspalum notatum) exposed to ground-level ozone. Animal Feed Science Technology 84:243-256. Muntifering, R.B., Chappelka, A.H., Lin, J.C., Karnosky, D.F. & Somers, G.L. (2006). Chemical composition and digestibility of Trifolium exposed to elevated ozone and carbon dioxide in a free air (FACE) fumigation system. Functional Ecology 20: 269-275. Orvar, B.L. & Ellis, B.E. (1997). Transgenic tobacco plants expressing antisense RNA for cytosolic ascorbate peroxidase show increased susceptibility to ozone injury. Plant Journal 11:1297-1306. Percy, K.E., Legge, A.H. & Krupa, S.V. (2003). Tropospheric ozone: A continuing threat to global forests? In Air Pollution, Global Change and Forests in the New Millennium. Karnosky, D.F., Percy, K.E., Simpson, C. & Chappelka, A.H. (eds.). Elsevier Science, Amsterdam, The Netherlands. pp 85-118. Powell, M.C., Crosby, D.D., Muntifering, R.B. & Chappelka, A.H. (1999). Quality characteristics and secondary chemistry of sericea lespedeza exposed to tropospheric ozone. Journal of Animal Science 77 (Suppl. 1):206. Schraudner, M., Moeder, W.V., Camp, W., Inzi, D., Langebartels, C. & Sandermann, H. (1998). Ozone-induced oxidative burst in the ozone biomonitor plant tobacco W3. Plant Journal 16:235-245. Shrestha, A. & Grantz, D.A. (2005). Ozone impacts on competition between tomato and yellow nutsedge: Above- and below-ground effects. Crop Science 45: 1587-1595. U.S. EPA. (2006). Air Quality Criteria for Ozone and Related Photochemical Oxidants EPA/600/R-05/004aF-cF. U.S. Environmental Protection Agency, Washington, D.C.