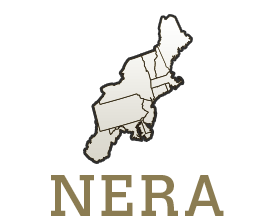
NE132: Environmental and Economic Impacts of Nutrient Management on Dairy Forage Systems
(Multistate Research Project)
Status: Inactive/Terminating
NE132: Environmental and Economic Impacts of Nutrient Management on Dairy Forage Systems
Duration: 10/01/1999 to 09/30/2005
Administrative Advisor(s):
NIFA Reps:
Non-Technical Summary
Statement of Issues and Justification
Dairy farming in the U.S. is under increasing pressure by two major factors. First factor is the decline of milk prices. Milk prices are moving toward a world market price that is causing a continuing trend toward larger dairy farms concentrated in certain geographic locations. The second factor is the relatively recent environmental concern for dairy farm nutrient losses The cost of managing manure nutrients is being assumed by the farm owner. These two factors are colliding on traditional dairy production areas in ways that are having significant negative impacts on rural families and communities.
Integrated research and technology transfer programs are needed discover how to better help dairy farmers manage their farms in a cost effective and environmentally acceptable manner, and in ways that comply with newly imposed farming regulations.
Recently, attention has focused on animal production systems as non-point sources of pollution affecting the quality of streams, estuaries, and ground water resources. In particular, nitrogen and phosphorus may contaminate water after application of manure or chemical fertilizer to soils (Morse, 1995). These nutrients may be lost from farms with runoff water after a rainfall (Baker and Senft, 1993), or nitrogen may leach through soils into ground water (Joshi et al., 1994). Some nitrogen becomes volatilized as ammonia from manure or soils, and eventually contributes to acid rain that endangers forests and water bodies (Luebs et al., 1973). Some nitrogen may be lost from the farm by conversion to atmospheric N2 (denitrification), but the majority is lost to water resources.
Agricultural practices have become more intensive to provide for the nutritional needs of an increasing human population and as a response to economic pressures on individual farms (de Wit, 1992). Higher production levels are possible on farms through the use of chemically fixed fertilizer and feeds imported to farms from other regions. However, such practices also increase the potential for nutrient losses from the farm.
Several technology and incentive programs are aimed at reducing the risk of environmental damage from animal agriculture while maintaining farm productivity. However, in order to better direct these resources, there is a need for routine assessment of which farm management practices are most effective on specific farms. There is a need for the development of farm management decision support tools to help producers and their consultants identify the most appropriate strategies for managing nutrients on a farm by farm basis. In addition, there is a need to collect and analyze data in the field, so as to identify the most appropriate technology transfer programs to address the problems associated with nutrient pollution in a cost effective manner.
Because the environmental impact of a farm management decision depends on farm characteristics (e.g., soil capacity) and other management selections (e.g., crop rotations, tillage, manure storage), the only way to calculate the benefit of a farm practice is to model the changes in nutrient flows through the entire system. Development of integrated mathematical models enables calculation of the environmental benefit from one or more management or infrastructure changes in any management subsystem (crops, soils, feed, animal). The development of such an integrated understanding of the farm nutrient cycle requires participation by scientists in a number of diverse disciplines.
The NE-132 project has brought together a broad range of disciplines and led to the development of quantitative models, the Dairy Forage System Model (DAFOSYM) and the Dairy Nutrient Planner (DNP). These models integrate years of collaborative research and enable an enhanced understanding of nutrient flows in the farm system. However, DAFOSYM is intended for research and teaching purposes rather than extension, and the DNP was targeted for a specific geographic region (Pennsylvania) and still requires further development. These models need to be further evaluated and adapted to address the immediate needs in the field across the nation related to nutrient management on dairy farms. In the new project, models that were previously developed will be used and evaluated under diverse farm conditions to identify improved strategies for management of nutrients on farms. Models will be developed for on-farm use in various regions of the U.S.
To advance whole-farm model evaluation and application, a coordinated effort is needed at several levels. First, field studies of nutrient dynamics and cropping systems are needed to calibrate predictions for different geographic locations and to evaluate model components. Second, model refinements and software development are needed to enable use of the models on individual farms throughout the U.S. Finally, model prediction capacity must be expanded to more accurately partition nutrient losses into those from volatilization, leaching, runoff and denitrification, and consequences of alternative feeding or crop management systems must be expanded.
Related, Current and Previous Work
There is a substantial amount of research and extension activity related to reducing nutrient losses from dairy production systems. Many programs focus on tillage practices or the management of manure and chemical fertilizer. However. management practices related to cropping. feeding. grazing, or herdnutrient utilization also must be considered. The way nutrients flow through the dairy farm is represented in Figure I. Most agricultural research is conducted within specific disciplines (e.g. agronomy animal science. and engineering). Within and among each of these disciplines, models of nutrient utilization are needed. These models must then be integrated to calculate the impact of management at the whole-farm level.
The proposed work will build upon and integrate research in several disciplines to make maximal use of previous research. Major areas include crop growth, crop conservation, animal nutrient utilization. manure handling, pasture and grazing, and systems analysis of dairy farms. Each of these areas is addressed.
Crop Growth
Crop growth modeling now has a substantial history that is well summarized by Hanks and Ritchie (1991), including appraisals of various protocols for modeling pasture production (Humphreys, 1991; Parsons et al., 1988). The incorporation of crop models into DAFOSYM initially was limited to alfalfa and corn (Rotz et al.. 1989a). Alfalfa quality prediction equations based on records of air temperature and herbage maturity were included in DAFOSYM (Fick and Onstad, 1988: Fick et al.. 1988: Kalu. 1982). Similar concepts were developed for grasses as predictors of grass nutritive value (Moore et al.. 1991). Corn production models were necessarily linked to nitrogen management (Bowen et al.. 1993; Jones and Kiniry, 1986). Although the use of cover crops and reduced tillage in corn production has been studied for some time (Mitchell and Teel. 1977), only recently have nitrogen relations of these approaches been modeled (Blevins et al.. 1990: Karlen and Doran, 1991; Shipley et al. 1992). Scheduling of manure handling, tillage and planting operations based on predicted days with suitable weather were added to the DAFOSYM model (Harrigan et al.. 1996). Simulations illustrated that either alfalfa or corn silages provided good forages for dairy farms in the Midwest (Borton et al.. 1997).
Hay crops or pastures based on grasses. are also a component of many dairy farms. and thus these were included in a more comprehensive systems analysis. Work from Pennsylvania helped to formulate. refine and validate pasture models (Buckmaster. 1991; Fales et al.. 1993). In addition. the CANPAS model (Fick. 1980) has been modified for pasture growth simulations in the Great Lakes region (V.R. Kanneganti. 1993. personal communication). A group at Cornell has also developed a general grass growth model for the Northeast ( Schulze. 1992). Numerous grassland models have been developed (Seligman. 1975) along with simulators of nitrogen dynamics in grasslands (Cooper et al.. 1992; McCaskill and Blair, 1990: Seligman and van Keulen, 1981). Models of timothy (Menzi et al.. 1991; Torssell and Kornher, 1983), orchardgrass (Kubota and Agata. 1985), and grass-white clover mixtures (Fick. 1980; Field and Ball. 1985) may serve as useful tools in modeling farm systems.
Successful integration of forage crops into the livestock system can provide a solution for many of the real and perceived environmental problems associated with livestock farming (Cherney and Allen. 1994). High quality forages can reduce the amount of grain needed on dairy farms thus minimizing feed imports. Grasses have advantages over legumes when considering nutrient management. Grasses require N application for optimum growth. while legumes fix N but are not adversely affected by N application. However. production of grass with high nutritive value requires a higher level of management compared to legumes (Cherney et al.. 1993). In addition, farmers generally believe that grasses gill not permit the level of milk production obtainable with alfalfa. so alfalfa is the primary forage used for high producing dairy cows.
Ecological studies of orchardgrass and white clover that were recently completed in New York may contribute to the model (Karsten. 1996). White clover appears to be an important regulator of nitrogen dynamics in pasture ecosystems (Gibson and Cope. 1985; Hay et al.. 1989: Neidhel and Henjum.
1991), but persistence is a serious problem in warm environments (Matches, 1989). In cooler climates. clover dominance is the problem 1 MacAdam et al.. 1998). Harvesting and quality studies in New York are establishing the linkages between grasses and animal production (Cherney et al.. 1993: Chernev and Cherney. 1993 ).
Crop Conservation
McGechan (1990c, I 990d> developed a systems model for studying the economics of grass forage conservation systems for cool, humid. upland climates. The model linked submodels of grass growth. swath drying and rehydration. mechanical field operations. Field and storage losses, and ration formulation for dairy cows. The model was used to compare various hay and silage systems.
Losses during grass conservation are widely documented (Robertson, 1983; Wilkinson, 1981; Rees. l 982). An extensive review was completed by McGechan (1989; 1990a) and Gupta et al. (1990) to obtain loss relationships. This and other previous work provided the basis for models of conserved grass harvest incorporated into DAFOSYM (Rotz, 1995).
Baling hay at moistures between 25 and 350o reduces harvest losses (Rees. 1982), but post harvest drying is necessary for proper storage. Bledsoe et al.. (1985) designed and used a system for drying large hay packages. Experimental data were collected to evaluate the benefits of drying high moisture hay in large round bales (Bledsoe et al., 1996).
Changes in both crop varieties and harvesting technologies may also affect ensiling and subsequent animal utilization. Muck and Hintz (1996) found that newer high-quality alfalfa cultivars had significantly different fermentation characteristics than standard varieties; however, substantial negative effects were not observed. A much broader diversity is found in the corn varieties that are available (Allen et al., 1997), and this diversity may alter the optimum times and conditions for harvest from current recommendations (e.g., Bal et al.. 1997). New harvesting technologies are either being developed (e.g., maceration of forages) or in the process of being adopted by farmers (e.g:,., kernel processing for corn silage). These new technologies may alter currently known interactions of chop length with ensiling and animal performance (Deswysen et al., 1978: Marsh and Hamilton. 1978) and may affect the efficacy of silage additives. Forage maceration is known to speed silage fermentation 1 Muck et al.. 1989), may reduce the efficacy of silage inoculants ( Muck et al.. 19891, increase silo capacity (Skinners et al.. 1988) and improve animal performance (Koegel et al.. 1992). However. the interaction of chop length with maceration is unknown at this time. Kernel processing in corn silage is relatively new in the US. Processing and its accompanying particle size reduction affects silage density, nutrient availability and farm economics (Harrison and Johnson. 1997; Harrison et al.. 1998; Rotz et al.. 1998). More data relative to processing interactions with silage additive efficacy and chop length effects are needed to promote this technology.
The effect of forage management on changes in protein availability in the rumen and digestibility have been reviewed (Kohn. 1998). Yang et al. (1993) found that some heat treatment actually improved forage quality. There is potential for baling wet hay, not only to reduce harvest losses, but to actually improve forage quality as well. Through proper heating. N utilization by the animal can be improved, thus reducing N loss to the environment and reducing N imports in supplemental feeds (Broderick et al.. 1993). ). Ensiling reduces the amount of true protein in forages ( Muck et al.. 1996) and that loss of true protein affects the efficiency of nitrogen utilization by dairy cattle (Nagel and Broderick. 1992). Importantly, losses of true protein during ensiling are reduced in some forages such as red clover (Muck et al.. 1996). suggesting that there may be the potential for reducing losses in more susceptible crops. The effects of corn silage processing and particle size reduction also affects nutrient availability and farm
economics 1 Harrison and Johnson. 1997: Rotz et al 1998). '
Animal Components
Dry matter intake is often predicted by empirical models from animal factors such as milk production, days in milk and body weight (NRC, 1987; NRC. 1989). Several mechanistic models have been developed to predict maximum dry matter intake (Mertens and Ely. 1979: Pienaar et al.. 1980; lllius and Gordon. 1991; Hyer et al.. 1991 ) or maximum fiber intake (Mertens. 1987: Fisher et al., 1987). However, dietary factors such as fiber digestibility, particle size. and environmental factors also affect intake. but these factors are not considered in quantitative models at this time (Illius and Allen, 1994). A series of experiments has shown that high fiber digestibility reduces the filling effect and enables greater intake (Allen. 1996a, Allen. 1996b, Dado and Allen, 1995; Dado and Allen, 1996; Mooney and Allen, 1997). Furthermore, physical till does not always limit intake. Work by Johnson and Combs (1992) and Dado and Allen ( I 993) shows that intake by dairy cattle in early lactation fed high energy diets is not always limited by physical fill.
Models that predict the flow of undigested feed and microbial protein to the small intestine have been reviewed (Kohn et al.. 19q8). Microbial protein production as a result of feedstuff fermentation in the rumen represents over 60% of the protein absorbed by dairy cattle (NRC. 1989). Forage port in is extensively degraded during storage as silage (Knowlton et al., 1992) and in the rumen (NRC, 1985) and must be converted to microbial protein for efficient use. The major determinant of microbial protein production is the availability of ruminally fermentable energy. Insufficient. ruminally fermentable energy reduces microbial protein production and increases excretion of nitrogen as urea. decreasing the efficiency of forage protein utilization and increasing the mobile nitrogen content of the manure. The amount of nitrogen excreted by dairy cattle and the efficiency of nitrogen utilization can be predicted from milk protein and milk urea nitrogen concentrations (Jonker et al.. 1998).
Manure handling can be simplified by applying manure to grass species. However, farmers are unlikely to voluntarily switch to grass production from high quality forage unless they can be shown that doing so is economically, as well as environmentally, advantageous. Forage quality has a large impact on milk production ( Weiss and Shockev. 1991). While laboratory analyses of forage quality are very useful. actual animal feeding trials are still considered essential (Stollenberg and Cherney, 1994). At equal digestibilities, legumes are consumed in greater quantities than grasses. Forage legumes have greater concentrations of protein. cell solubles, and lignin, but lower concentrations of neutral detergent tiber than grasses of similar digestibility. These differences in chemical composition. and resulting higher intakes. have been associated with taster rates of digestion by cattle consuming alfalfa.
Direct comparative studies on milk production and economics between grass and legume forages used in typical dairy diets are lacking. Weiss and Shockey (1991) compared alfalfa and orchardgrass using mid-lactation cows fed concentrations at 20, 40 or 60%; they concluded that properly managed orchardgrass and alfalfa had similar feeding value. Studies have not been done with cows in early lactation. where nutrient demands are higher. Cherney and Chernev (1993) indicated that a good quality grass could compete economically with alfalfa in terms of return per cow over feed costs and return per acre. However. these conclusions were based on model simulation of a hypothetical grass.
Manure and Soil Components
Livestock manure is a major component of the farm nutrient cycle. The concentration of animals on dairy farms with limited land area results in production of more manure than can be distributed on the farm in an environmentally sound manner. Even where total farm area is sufficient for manure application. the concentration of animals around housing and milking facilities often results in a poor distribution of livestock manure or inflated costs for manure handling (Westphal et al.. 1989). Without manure storage, accumulated manure must be spread in the winter. and this greatly increases the risk of polluting surface waters. When manure is stored and spread onto cropland over a relatively short time, heavy rainfall events can again cause washing of manure into surface water supplies.
In the nutrient cycle, livestock manure returned to the soil can contribute to soil fertility reserves. plant production, or be lost to surface and ground water (Russelle. 1992). The nutrient cycle on dairy farms has received much study with recommendations at the national level (Morse et al., 1993) and on a regional basis (Bacon et al.. 1990: Klausner and Bouldin. 1985: Westphal et al.. 1989). Nitrate losses from pastures have been studied in other countries (Ball and Keeney. 1983: Jarvis et al., 1989; Steele et al., 1984), and studies have been initiated in the US (Harris et al.. 1991: Kerensky et al., 1993). An experiment was conducted to measure nitrogen leaching beneath fecal and urine patches on nitrogen fertilized pasture (Stout et al., 1997). Nitrogen recovered in leachate under feces deposited in the summer represented 2% of the total nitrogen in feces. Recovery of nitrogen in leachate under urine patches varied from 18 to 31 %.
In general. nitrate leaching to groundwater is understood well enough to be modeled (Bergstrom and Jarvis. 1991: Hutson and Wagenet. 1991), but data are needed from -,razed systems for model refinement and validation. The NCSWAP model was evaluated for its ability to predict nitrate leaching under orchard grass (Jabro et al.. 1998), and reduced tillage corn production systems that often include winter cover crops (Blevins et al.. 1990: Shipley et al., 1992). Flows to surface waters have also been measured and modeled (Cooper et al.. 1992; Taraba et al., 1983), but they are incompletely described for .
pasture systems in the U.S.
_
Pasture and Grazing
Grazing systems can reduce manure handling costs for at least part of the year. and economics associated with the use of pasture may allow profitable milk production with fewer animals per unit area of land. Thus, pastures promise to alleviate some of the problems associated with nutrient management of intensive dairy farms. However. gazing animals do not distribute their manure uniformly, and they concentrate manure application near water and shade facilities. and therefore. there may be a reduced ability to recycle manure nutrients on pasture-based farms. These aspects of pasture management have not been sufficiently considered when comparing pasture to other dairy feedingy systems.
In addition to the environmental impact, economical pasture utilization by modern. high producing dairy cows requires integrated knowledge of pasture production in terms of yield, intake potential and forage quality. We must also know how these factors affect growth rate (of replacement animals), milk production and animal health, and how all these factors taken together influence composition of animal excreta and the cycling of nutrients. The forage species in the pasture itself must also be analyzed for their role in the nutrient cycle. Pasture growth rates under grazing must be catalogued for different plants, soils. nutrient levels, and weather patterns before pasture models can be developed and validated. Pasture legumes (especially white clover) that can either fix atmospheric N or use recycled N from manure are key buffers in the pasture nutrient cycle that help maintain production rates and forage quality. Because pastures exist on such a wide range of land types, there is also a need to identify grazing management plans that are environmentally and economically appropriate on a variety of resource bases. A mathematical model of the pasture system was developed by Mohtar et al. (1997a). Model predictions were well related to observed data for accumulated biomass. nitrate leaching, and amount of water drained (Mohtar. 1997b).
Research and on-farm demonstrations of intensive grazing on livestock farms have shown a significant increase in farm profitability through a reduction of input costs and improved animal health (Emmick and Toomer. 1991: GoIdberg et al.. 1992: Lucev et al.. 1988: Murphy et al.. 1986: Nichols.
1996; Parker et al.. 1992: Tranel and Frank. 1991) and improvement in sustainabilitv through reductions in environmental risks (Kaffka. 1989: Murphy. 1990). This awareness among livestock producers has created a demand for practical information on intensive grazing. as indicated in a recent producer survey (Heinrichs et al.. 1992), and through extension work in Indiana (Johnson et al.. 1993). DAFOSYM was used to compare grazing and confinement systems for typical Michigan farms. and the farm with a grazing component was predicted to have a lower feeding cost and greater profitability (Rotz. 1996).
Knowledge of pasture growth rates and the resulting potential intake of forage nutrients are essential (Lile and George, 1993). Pasture height is a major determinant of intake potential (Alcock et al., 1985; Wright and Whyte, 1989) as well as regrowth rate (Hodgson, 1985). Rotational grazing with short periods of use (4 hours to 2 days) followed by recovery periods of 2 to 4 weeks is generally recommended to maximize pasture yield and quality (Murphy et al., 1986; Undersander et al., 1991). A two-year intensive grazing study was conducted in Wisconsin by Kanneganti et al., (1998). Available forage was relatively high in May and June, moderate in July and August, and low in September. Under some conditions. carefully controlled continuous grazing can be as productive (Grant et al.. 1983; Parsons et al., 1983) and less expensive because of lower labor and fencing requirements.
Individual pasture species have been evaluated for growth rate (tile and George. 1993); Raybum 1991: Lemaire and Salette. 1982: Kurte et al.. 1984; Carton et al.. 1989a: McFeelv and MacCarthy, 1981). intake potential (Bircham and Sheath. 1986; Stakelum, 1986), and ecological function under grazing (Carton et al.. 1988; Carton et al.. 1989b; Korte et al., 1984). Species adapted to the Great Lakes Region especially need further study under grazing (Clark, 1993). White clover can perhaps be a key species in' = the regulation of the N dynamics of pastures. With forage legumes, soil N is taken up when soil nitrate levels are adequate, yet N fixation maintains forage growth potential during periods of low soil nitrate; this has been demonstrated by Heichel et al. (1984) with alfalfa. The tolerance of white clover to grazing (Gibson and Cope. 1985) allows this species to be used in pastures to maintain adequate N for forage production without flooding the system with excess nitrates that threaten safe groundwater supplies.
Low forage production during hot. dry weather and poor disease resistance have been identified as a limitation of white clover (Karsten. 1996: Karsten, 1997). Reduced forage production of white clover during hot weather and poor persistence due to a combination of diseases, insects, and competition with warm season grasses under continuous grazing are also limitations of white clover in the southeastern US (Brink and Pederson. 1993). Grazing management. more adapted white clover cultivars. and other forages might help improve summer forage production and persistence (Karsten. 1996).
More research is needed to identify grazing management practices that will maintain productive forage species like orchardgrass during hot. dry summer weather. Grazing management recommendations that can maximize animal intake and production on pasture also need to be better defined. Grazing management practices that optimize animal intake and sustain pasture production are not vet well defined in many regions of the U.S.. and need to be researched to improve grazing model accuracy and predictions. Research is also needed to identify pasture species and pasture renovation practices that most economically increase forage production, forage quality, animal intake. and animal production.
Integration and Modeling
The concept of using models to integrate the many components of dairy farms has evolved with the advance of computer technology. Early models developed in the 60's and early 70's were relatively crude by today's standards. Most models were simplistic or empirical in representing component relationships. Advances in computer technology and increased knowledge about various processes have allowed models to become more detailed with more emphasis on describing the mechanisms of system performance. This trend is likely to continue as computer power and our understanding of system mechanisms continue to grow.
One of the first attempts to integrate the dairy forage system in a model was made by Armstrong et al. (1962). Linear programming techniques were used to select the forage-handling practices that would yield maximum family income from a dairy farm. Most of the early models represented only a portion of the total system. Several models were developed and used to evaluate various aspects of forage harvest (Von Bargen. 1966: Tseng and Mears. 1975: Verma et al.. 1976; Kjelgaard. 1979; Pitt, 1982). Other modeline efforts concentrated on alfalfa growth (Fick. 1977) and field drying (Bruck and Van Elderon, 1969). Next. models were developed to describe silo storage (McGillard et al., 1987; Pitt et al., 1985) and animal performance (Williams et al.. 1989; Baldwin et al., 1987a; Baldwin et al.. 1987b). A grass model was developed and included as part of a pasture system model (Thornley, I 998).
The power of modeling grew when models were linked to simulate forage production systems (Miller and Rehkugler, 1972; Parke et al., 1978; McGuckin et al.. 1982). In the next step, storage and animal utilization models were integrated with crop growth and harvest to form models of the whole dairy forage system (Lovering and Mc(saac. 198(; Savoie et al.. 1985: McGechan. 1990b). These comprehensive models were used to evaluate and compare various forage management options available to the dairy farmer. Simulation over many years of historical weather conditions provided an assessment of risk as well as the long-term economic value of alternative technologies and management strategies.
The model developed by Savoie et al. (1985) was named The Dairy Forage System Model (DAFOSYM). Development and validation of this model has continued. More detailed submodels were added to describe field drying (Rotz and Chen, 1985), field losses (Rotz. 1995), hay storage (Buckmaster et al., 1989a). silo storage (Buckmaster, 1989b) and animal utilization (Rotz et al.. 1989b). The emphasis of the model refinement was to improve prediction of forage losses and quality changes throughout the system and their effect on animal performance. DAFOSYM was used to evaluate a variety of technologies and management strategies for forage conservation on dairy farms (Rotz. 1985; Rotz et al.. I 989b: 1990: 1993; Rotz and Savoie. 1991).
Initial development of DAFOSYM concentrated on the prediction of forage yield and quality, changes in quantity and quality during harvest and storage, and consumption by dairy cattle, resulting in milk and meat. To enlarge the model to address environmental concerns. additional elements were added. The nutrient cycle of the dairy farm including the import of nutrients in feed and fertilizer. the export of nutrients in dairy products and feeds. and the loss (and storage of nutrients in the soil and water, were incorporated into the overall model. Because this cycling of nutrients also interacts with crop rotations and land types on dairy farms. additional crop submodels were added to simulate use of grasses, pastures, small grains and soybeans in addition to the corn and alfalfa-based crop rotations previously incorporated. Recent development of DAFOSYM has included the addition of manure production and handling and the comparison of manure systems on dairy farms (Gorton et al.. 1993).
.After 20 years of development and application by researchers of the U.S. Dairy Forage Research Center and other members of the NE-132 Regional Research Project. DAFOSYM has evolved into a powerful whole-farm model. It provides a good basis for the type of analysis necessary to quantify nutrient and financial flows on farms. DAFOSYM simulates crop growth. harvest, storage. feeding. feed use and manure production and handling over many years of weather. N/lajor system inputs and outputs are accounted for to determine production costs and the net return over those costs. The model has been a useful research tool for improving the efficiency and profitability of forage conservation on dairy farms. During the past several years, the model has been widely distributed as a teaching aid. This model will be the basis for further research on identifying cost effective strategies for reducing nutrient losses from dairy farms.
The need for extension-oriented farm management tools resulted in development of the Dairy Nutrient Planner (DNP: Dou et al.. 1996). This computer worksheet enables prediction of the potential nutrient inputs in feeds. fertilizer and by legume fixation based on a farm description and diet formulation. crop selection, and fertilizer application. It can be used to predict the balance of nutrients in manure against fertilizer requirements, and to balance nutrients in feeds and crops against animal teed requirements. The potential for loss or accumulation of nutrients in soil can be calculated. This model was evaluated on dairy farms in Pennsylvania (Dou et a(., 1998). A similar compartmental analysis approach was used to evaluate the critical control points on a typical dairy farm with respect to reducing nutrient losses (Kohn et al.. 1997). Nutrient utilization by the herd and crop selection were considered two essential elements for study when optimizing nutrient management.
A means to balance dairy cow diets with consideration of the potential for use of manure on available crops was introduced (Henry et al., 1995). More manure can be applied to certain crops than others, so the optimal crop selection depends in part on the need for manure utilization as well as the need for animal feeds. (n Europe, models of integrated animal production systems have been used routinely to identify farm management practices that will have the greatest impact on reducing nutrient losses and to quantify those losses on a farm by farm (van Alem and van Scheppingen. 1996) or regional basis (Schroder, 1995). The impact of programs implemented in Denmark in the late 1980's was evaluated and found to have reduced nitrogen inputs to animal production systems primarily through improvements in animal protein utilization (Schroder. 1995).
A grazing simulation model. GRASIM, was developed (Mohtar. Personal Correspondence, October, 1998). The model includes an easy-to-use, WWW-based interface that allows users to input crop, soil. nutrient, initial condition. site location, weather. and pasture management parameters.
Objectives
-
Develop, evaluate and refine cropping, grazing, and feeding strategies to optimize the dairy farm system for nutrient utilization, environmental impact, and profitability.
-
Develop research-based information, recommendations, and educational materials in support of state extension, teaching, and NRCS programs, agricultural consultants, and producers to strengthen the U.S. dairy industry.
-
Methods
Objective 1 Develop, evaluate and refine cropping, grazing, and feeding strategies to optimize the dairy farm system for nutrient utilization, environmental impact, and profitability. 1a. Crop Growth and Conservation Strategies Crop management impacts nutrient flows on dairy farms in several ways. The mix of crops grown and how they are managed affect nutrient removal by the crops and subsequently the levels of nutrients available for loss from the soil system. Crop management (tillage through harvesting and storage) also affects the nutritional value of the crop and the potential efficiency of nutrient utilization of the crop by the dairy cow. Several cropping strategies will be evaluated using the models to identify ways to reduce nutrient excess on farms. These strategies can be placed in general categories such as: 1. Reduce the importation of nutrients on to the farm;2. Fix less nitrogen;
3. Increase the capture of soil nutrients by crops. Conservation strategies most likely will include means to: 1. Reduce nutrient losses;
2. Improve nutrient utilization by cattle. Both field and modeling research are needed prior to evaluation of the selected strategies. This research falls into four general areas: i. Nutrient extraction from the soil by different crop species, crop mixtures and management;
ii. Effects of crop management and environment on the nutritional value of crops; iii. Effects of conservation strategies on losses and nutrient availability;
iv. Improvements in crop models for predicting growth, quality and nutrient content. Planned research in these areas is as follows: i, ii. Nutrient uptake and nutritional value of crops. The nutrients incorporated into plants are affected by species, management and environment. The uptake of nitrogen, phosphorus and potassium by forage crops will be measured at several sites under various management conditions and seasons.Cool season (C3) grasses (Hannaway-UT, MacAdam-UT), legumes (MacAdam-UT), grass-legume mixtures (Karsten-PA, MacAdam-UT) and cover crops (Herbert-MA, Dou-PA) will be studied. Crop management and environmental factors influence the nutritional value (crude protein, fiber and lignin contents; dry matter and fiber digestibilities) of crops. Corn (Allen-MI, Ferguson-PA), alfalfa (Allen-MI, Ferguson-PA), other legumes (MacAdam-UT), cool season grasses (Ferguson-PA, MacAdam-UT) and grass-legume mixtures (Karsten-PA, MacAdam-UT) will be studied in regard to factors affecting their nutritional value. iii. Effects of conservation strategies on losses and nutrient availability. Various nutrient losses and transformations occur in crops during storage, particularly in ensiling where the crop is at a moisture content that permits both plant and microbial activity. Four areas will be investigated as part of the project.First, research will be directed at determining the primary factors affecting N losses during ensiling of legumes, grasses and corn and developing predictive relationships (Muck-WI). Second, studies will be conducted to determine transformations in phosphorus compounds occurring in ensiling and the principal factors influencing those transformations (Muck-WI). Third, polyphenol oxidase and modified atmosphere will be investigated as means of preserving true protein in forage silages (Muck-WI). Fourth, the impact of processing on the ensiling and nutritional value of corn silage will be studied (Harrison-WA). Corn silage will be harvested at multiple stages of maturity, multiple chop lengths, with and without mechanical processing, and with and without inoculation with lactic acid bacteria. Rate of pH decline, production of lactic acid and volatile fatty acids, and dry matter recovery will be determined. These parameters will be used to assess the effect of the above mentioned factors on silage fermentation. In addition, packed density measurements will be determined with corn silage stored in bunker silos and Ag Bags and this will be related to dry matter loss during ensiling.Pertinent results from these four studies will be used to improve the silo model within DAFOSYM (Muck-WI, Rotz-PA, Harrison-WA). iv. Improvements in crop models for predicting growth, quality and nutrient content. The information obtained from the field studies above will be used to improve stand-alone grass (Mohtar-IN) and alfalfa (Fick-NY) models, particularly in the areas of nutrient uptake and nutritional value. Insights gained from these stand-alone models will be incorporated into the crop models within DAFOSYM (Rotz-PA). Once these studies are complete, the cropping strategies selected in the first year of the project will be evaluated using DAFOSYM to determine their overall effects on farm nutrient balances and profitability on representative dairy farms of various sizes and locations across the U.S. (Muck-WI, Mohtar-IN, Fick-NY and others). These analyses will lead to a list of cropping practices by region and by other factors (such as farm size and soil type) that will be most effective at improving farm nutrient management while maintaining or improving farm profit. 1b. Herd Nutrient Utilization Strategies The previous NE-132 project identified that the efficiency of nutrient utilization in the herd profoundly impacts the whole farm nutrient balance and farm profitability (Kohn, et al., 1997). With improved herd management, less manure is produced so fewer manure nutrients are left to runoff, volatilize or leach. In addition, when productivity can be maintained with less feed, there is a lower requirement for crop production and fertilizer use. Both of these changes reduce nutrient inputs and hence losses from the farm. The proposed project will focus on developing cost-effective strategies to improve nutrient utilization in the herd. The current practices used in respective states will be identified and nutrient flows that result from these practices will be quantified (Harrison-WA, Kohn-MD, Murphy-IL). Survey data will be collected and analyzed to determine differences in feeding and management and to determine total feed nutrient inputs (N, P, K, energy). These data will be analyzed to identify feeding and management practices associated with the greatest efficiency of nutrient utilization by the herd (Kohn-MD). The potential impact of introducing new programs aimed at improving common weaknesses on farms will be evaluated. Alternative feeding strategies to improve nutrient utilization will also be evaluated. The ruminal environment affects the fermentation process and alters microbial protein synthesis and feed digestion. Various feeding strategies and feed ingredients affect the ruminal pH and concentrations of volatile fatty acids. These ruminal environment parameters in turn affect microbial growth and ruminal digestion. Studies will be conducted to identify factors that alter ruminal fermentation patterns including pH, methanogenesis, and shifts in volatile fatty acids (VFA) profiles (Allen-MI, Kohn-MD, Murphy-IL, Prigge-WV). The effect of corn silage processing on digestibility by dairy cows will be determined (Harrison-WA). Ruminally and duodenally cannulated and lactating cows will be used in metabolism studies to determine the effects of hybrid, mechanical processing, maturity, and chop length on the nutritive value of corn silage. Digestibility and absorption will be measured for: nitrogen, fiber, and starch. Rumen pH will be monitored for 8 hours after feeding and flow of microbial nitrogen to the duodenum will be determined. Increased milk production per cow dilutes the maintenance requirement and improves the overall nutrient utilization in the herd and reduces manure nutrient losses as a fraction of production. Much previous research was conducted using cows that produced less than 20,000 lbs of milk per year. Commonly today much higher production levels are observed in the field. The animal model within DAFOSYM was developed and evaluated on lower production levels. DAFOSYM predictions will be evaluated for milk production and nutrient flows in high-producing herds (Harrison-WA, Kohn-MD, Rotz-PA). Herds producing more than 28,000 pounds of milk will be studied to characterize the nutrient profile of forages and total diets and determine factors such as body weight and dry matter intake.Information obtained will be used to help refine the animal submodel of DAFOSYM. 1c. Manure Application and Soil and Water Interactions Much of the focus related to nutrient flows from dairy farms is on groundwater contamination by nitrate and surface water deterioration due to N and P enrichment of water running off of manured or fertilized fields. Many states now require that farms develop and implement nutrient management plans. Often, extension programs and NRCS personnel assist farmers with plan development. Although varying from state to state in terms of format and content, all nutrient management plans for dairy farms include one fundamental principle: to balance manure application rates (and fertilizer supplementation, when necessary) with crop needs. Since manure handling and application processes involve unavoidable losses of nutrients, especially N, empirical factors are used to estimate the availability of manure nutrients to facilitate the calculation of application rate. Typically, nutrient availability is affected by weather and soil parameters, nutrient characteristics of the manure, and cropping systems. Thus it is essential to investigate the impact of these factors and their interactions on nutrient availability so that application guidelines may be developed to best utilize manure nutrients for crop production while minimizing detrimental environmental impact. i. Effect of weather and spatial variability on nutrient flows and nutrient availability Large spatial field variability imposes difficulties in predicting chemical movement under intensive dairy production operations and makes managing these systems for high productivity and low pollution very challenging. This large variability makes it impossible to characterize subsurface properties with enough detail to permit the use of deterministic modeling techniques. Recently proposed stochastic design methodologies were developed to overcome some of these difficulties by using Monte Carlo simulation to generate a series of possible movements. The stochastic approach will be evaluated by comparing its predictions to experimental data reported in the literature (R. Mohtar, S. Herber). The stochastic approach for modeling chemical transport in these systems has received increased attention during the past 30 years because of the significant spatial variability of natural subsurface environments. In the stochastic approach, weather input, the soil hydraulic properties, that characterize the subsurface are assumed to be Random Space Functions characterized statistically by their mean, co- and cross-variances and the shape of their distribution. This statistical information is then used to predict the statistics of flow and transport events occurring within the targeted environment using Monte Carlo approach. ii. Characterize manure P for potential runoff loss Phosphorus excretion in manure may be reduced via ration manipulation without decreasing milk production (Morse, et al., 1994; Wu and Satter, 1998). Some fractions of P in manure are more available for plant uptake than others (Barnett, 1994; McAuliffe et al., 1949). Similarly, certain forms of P in manure may be more soluble and thus subject to a higherpotential for runoff loss than others. A laboratory procedure will be developed to characterize manure P under various conditions (Dou-PA, Ferguson-PA, Harrison-WA, Powell-WI). Samples will be obtained of the liquid and solids fractions of manure processed with a slant screen manure separator. Samples will be obtained throughout the year to determine the effects of season and use of fresh water flushing on the nutrient (N, P, K) profile. In addition, samples from feeding trials with different amounts of P or N in the diet will be collected. These samples will be sent to Dou-PA to characterize nutrient fractions. Based on soil P fractionation proceduresand preliminary results of manure P separations, different extracting solutions will be selected, tested, and compared for relative extracting power of manure P. Factors that may affect P dissolution in selected solutions, such as manure/solution ratio, extraction time (hours), and number of repeated extraction, will be investigated. Anticipated outcome is the development of a protocol to set forth laboratory procedures for characterizing manure P regarding potential environmental impact.Further, the protocol may be used to compare management tactics and select the best options that meet specific needs of individual farms.For example, if a farm has relatively high animal density (animal units per unit of cropland) and soil testing results indicate optimum or excessive P level, management consideration should emphasize the reduction of both total P excretion and the more soluble P fractions.On the other hand, where P application is required for economical crop yields, options that increase manure P availability would be more beneficial. (iii) Nutrient efficiencies in different cropping systems Perennial grasses are planted on considerable acreage in many dairy farms. Manure spreading on alfalfa or grasses is a common practice according to survey results (Dou et al., 1997); but manure application guidelines are developed with corn being the primary crop to receive manure. To fill the gap, field experiments will be conducted to determine N and P utilization efficiencies and losses with dairy manure being applied to alfalfa and orchardgrass as compared to corn (Dou-PA, Ferguson-PA, Mohtar-PA, Herber-PA). Orchardgrass and corn will receive N-based manure, P-based manure plus supplemental N fertilizer, and ammonium nitrate, respectively. Alfalfa will receive N- and P-based manure applications only. It is assumed that alfalfa will take up all N needed from soil given sufficient supply, or obtain N through symbiotic N2 fixation if soil N availability is limiting. A control treatment with no N or P addition will be included for each crop. Wick lysimeters installed will facilitate the monitoring of nitrate, and possibly phosphate, leaving the crop root zone.Crop, soil, and leachate samples will be collected to determine: 1. Apparent recovery and removal capacity of N and P as a function of crop species and nutrient source using mass balance procedures;
2. Quality indices of forages (CP, ADF, NDF, etc.) as affected by nutrient source and application rate;
3. Nitrate, and possibly phosphate, losses through leaching;
4. P distribution and movement in the soil profile. 1d. Pasture and Grazing Research and on-farm demonstrations of intensive grazing for dairy production have shown a potential for increased farm profitability through a reduction of input costs (Emmick and Toomer,1991; Lucey et al., 1988; Murphy et al., 1986; Parker et al., 1992). Improvements in sustainability through reductions in environmental risks can also be realized through intensive grazing (Kaffka, 1989; Murphy, 1990). Grazing, however, also requires the producer to make daily management decisionsthe implications of which are not fully understood within the context of a production system. This sub-objective will concentrate on identifying pasture management strategies to improve pasture utilization and ultimately to test these strategies within a production system model. Specifically, study areas under the pasture grazing sub-objective will encompass incorporating legumes into pastures, defining optimal frequencies and intensities of grazing,improving methods of measuring and predicting pasture yield as well as evaluating the significance of errors in estimating pasture yield. i. Legume incorporation The incorporation of legumes into pastures has long been recognized as a method of improving both its quality and yield (Bryan and Prigge, 1990). However, the persistence of the legume component has been identified as a major limitation (Bryan, 1987). Research will be conducted on grass/legume swards (MacAdam-UT, Prigge-WV). The most effective combinations of grasses and legumes determined from simulation studies in the last project will be tested with small-plot studies (MacAdam-UT). Various legumes (alfalfa, white clover, birdsfoot trefoil, cicer milkvetch) will be grown in combination with cool season grasses and detailed growth data will be recorded. Pastures overseeded with mixtures ofred and white clover will be compared to non-overseeded Kentucky bluegrass pastures maintained at two sward heights (4-6 or 8-10 cm) (Prigge-WV). The clover mixture will be initially overseeded prior to grazing, and in subsequent years, one third of the areawill be overseeded to maintain the legume component of the sward. Overseeding periodically with legume seed can be an economical alternative to N fertilization. Stocking rate, forage composition of the pastures, grazing time and animal performance of replacement heifers will be compared over a multi-year study. ii. Defining optimal frequencies and intensities of grazing. A major factor influencing the net energy harvested from a pasture is the frequency and intensity of grazing. Grazing frequency refers to how frequently the pasture is grazed or defoliated and can be described in rotational grazing systems as sward height at or immediately prior to defoliation. It is strongly influenced by the number of days rest that are possible between grazing. Intensity refers to the amount of dry matter removed at each grazing event and it is influenced by the number of animals and the duration of grazing. Although degree of defoliation has been studied andmodels developed (Illius, 1985), these models are based on monoculture swards, and their application to production systems where cattle selectively graze mixed swards is limited. A study was initiated using naturalized pasture comprised predominantly of Kentucky bluegrass at three different sward heights (11.5, 13.5 and 15.5 cm). Within these heights either 50% or 60% of the available forage was removed by grazing. This study will be completed under the new project (Prigge-WV). Similar studies will be initiated in Pennsylvania (Karsten-PA) on predominantly orchardgrass pastures. A high forage availability treatment, in which grazing will be initiated at 25-30 cm and cattle removed at 7-8 cm sward height, will be compared to a low forage availability treatmentin which grazing will be initiated at 20 cm and cattle removed at 4-5 cm. Individual plants will be sampled to quantify differences in stem base storage reserves, tiller density and leaf area index available for regrowth after grazing. Results of the Pennsylvania and West Virginia studies will provide information to establish optimal grazing strategies for both sward types. iii. Methods of measuring and predicting pasture yield. Both the validation of pasture production models and practical pasture management require measurement of pasture yield. Because of variability that occurs within pasture, large numbers of measurements are needed to reach useful accuracy. Consequently indirect measurements have been developed and compared (Murphy et al., 1995; Harmoney et al., 1997). The wide variance associated with these indirect methods however, has hindered the application, appreciation, and integration of the results of many grazing studies between experiment stations. In addition, many commonly used methods are also labor intensive. Studies will be initiated (Prigge-WV, Karsten-PA, Fick-NY) to address these problems. Drs. Fick and Prigge will compare several methods to measure forage availability and develop calibration equations to relate these methods to dry matter yield. The West Virginia pasture plate will be compared to a commercial rising plate meter, the Georgia pasture gauge, and visual estimates (Prigge-WV). In NY, the same commercial rising plate will be compared with the very simple pasture yield stick being distributed by the New York Pasture Association (Fick-NY). DAFOSYM will be used to provide a farm-scale assessment of the production and economic consequences of errors in estimating pasture production by these methods (Sanderson-PA). For grazing cattle, feed intake can be influenced by factors other than the diet. Sward structure, as indicated by forage height or availability, via its influence on grazing time can limit DM intake of cattle on pasture. An inverse relationship exists between forage height and quality of consumed forage, consequently, the grazing time necessary to optimize net energy intake from pasture can be influenced by supplement level (Rook et al., 1994) and environmental temperature (Brumby, 1959). These relationships need to be established to design management programs for efficient utilization of pasture for milk production. Pastures will be maintained at four heights, 4-6, 6-8, 8-10 and 10-12 cm (Prigge-WV). Lactating beef cows will be supplemented with 0,3.5 or 7.0 kg/day of concentrate. Grazing time, voluntary intake, and digestibility of the pastures will be determined during three periods during the grazing season. Strategies will be developed to improve nutrient utilization of cattle on grazing systems. Two different recommended grazing management strategies (that differ in grazing intensity and frequency) will be compared for pasture forage quality and forage availability under rotational management intensive grazing (Karsten-PA, Rotz-PA). A model was developed to predict protein intake from milk composition (Jonker et al., 1998). This model will be evaluated for its ability to predict nitrogen intake on pasture (Kohn-MD). Predictions using this model will be compared to those from markers (e.g. Yb) or direct measurements (Kohn-MD). 1e. System Integration The proposed research on quantifying nutrient flows within each subsystem has been described in the preceding sections. This research will enable modeling of the whole farm system to quantify the impact of management changes within the subsystems on the nutrient and economic flows within the whole farm. The DAFOSYM and DNP models have been and will continue to be used for these calculations. For example, these whole-farm models will be used to evaluate nutrient flows and losses as affected by feeding strategies (Harrison-WA, Kohn-MD, Murphy-IL, Rotz-PA), protein protection in the silo (Muck-WI, Rotz-PA), different cropping strategies (Dou-PA, Fick-NY), and various grazing strategies (Karsten-PA, Rotz-PA). Data representing selected commercial herds in WA will be entered into DAFOSYM to evaluate whole farm nutrient flows (Harrison-WA, Rotz-PA). Simulations will be conducted to evaluate alternative feeding strategies and their impact on nutrient losses. Particular emphasis will be placed on corn silage and grass silage forage systems. The models that are currently available for whole farm prediction need to be evaluated for the different soil, climatic and management conditions that exist around the nation. The evaluation will lead to changes in model equations that will improve prediction accuracy and will identify when application of the models is appropriate. Field-level grazing research will be conducted and the results compared to the predictions using DAFOSYM (Karsten-PA, MacAdam-UT, Rotz-PA). Models such as DAFOSYM and DNP will be used in different states to evaluate utility and prediction accuracy (MacAdam-UT, Kohn-MD). Grazing strategies (e.g. stocking density, frequency) will also be evaluated using whole-farm models (Karsten-PA, Rotz-PA). Data collected from real farms will be used to validate and support simulation results. A specific and comprehensive analysis is planned for a farm in south central Pennsylvania (Rotz-PA). This farm, referred to as the Cove Mountain farm, is a new dairy operation. The farm includes 200 acres of grass pasture and forage for feeding a 100-cow herd. Intensive rotational grazing and a seasonal calving strategy are practiced. The farm will be operated as a commercial farm, but it will be closely monitored. Data collected on pasture production, supplemental feed use, nitrogen leaching, and production costs will provide information for further model development and validation. Long-term simulations will be used to predict the performance and economics of this farming system over many weather years. For comparison, farms using confined feeding and other grazing strategies will also be simulated for the same soil and weather conditions. Simulation results will provide quantitative measures of the benefits and disadvantages of this particular dairy farm system in southern Pennsylvania. After the model is properly validated for the Cove Mountain location, simulations for other soil and weather conditions will document the performance and economics of this and other strategies throughout the Northeast. Objective 2 Develop research-based information, recommendations, and educational materials in support of state extension, teaching, and NRCS programs, agricultural consultants, and producers to strengthen the U.S. dairy industry. The revised Farm Bill mandates integration of research and extension efforts, and it requires the development of multi-state extension programs. There is an immediate demand for information that can be used in the field to reduce nutrient losses from farms.Several states and the nation are moving toward mandated nutrient management planning. The economic and environmental impact of the various types of changes in management is essential to maintain the viability of the dairy industry. There is an immediate need for software tools that can be used in the field. Previous and current research efforts of this regional committee will be developed into field usable information in the form of written information, software, world wide web pages, and methodologies for improved farm management. This information will be research based and directed toward the needs of state extension, teaching and NRCS programs. Establishing a specific objective related to the goal of developing farm management tools is a major new direction for NE-132 even though numerous research efforts of the project were developed into such tools in the past. For example, the mathematical model DAFOSYM, which was developed and evaluated as part of the previous regional project, was used to develop specific recommendations to field consultants and policy personnel on cost-effective strategies to reduce nutrient losses to the environment. A field-usable computer program, the Dairy Nutrient Planner, was developed and introduced to dairy producers to calculate the flow of nutrients though the farm system. The proposed regional project will make this integration of research and extension a documented priority. 2a. Needs Assessment Whereas the collaboration of agricultural researchers with technology transfer personnel is a high priority, representatives of cooperative extension and NRCS will be invited to participate in the project. The location of the next regional meeting (September 15-17, 1999) will be in the District of Columbia to facilitate meeting directly with representatives of NRCS at the national level. A major priority of this meeting will be discussion of strategies to minimize nutrient losses from agriculture, the types of field-usable products needed to assist with implementation of new nutrient management approaches, and the identification of tools that have already been developed and are being developed. Not only will this effort assist in transfer of the research to the field, but the researchers will be able to better direct their own work toward established needs in the field. This discussion will be complemented with systematic research to better clarify the need for research and extension related to nutrient management. A decision grid methodology will be used to characterize the need for extension resources related to all aspects of nutrient management on dairy farms (Hannaway-OR). This method involves the identification of all the information needed to make related decisions in a responsible manner. Once this information need is established, the available resources will be characterized and missing resources that need to be developed will be identified. A survey will be undertaken to characterize existing resources for dairy farm nutrient management including available software and written field-oriented publications for grazing (Rotz-PA). A version of the final decision grid will be made available on the world wide web (Mohtar-IN). 2b. Information Information will be provided that can directly serve dairy producers and their support personnel. This information may be in the form of extension bulletins, world wide web pages, or presentations. Publications will be developed on the strategies for reducing nutrient inputs or losses from farms, and the evaluation of those strategies will be completed. An economic analysis of the fair market value of forages that can be used for partial budgets will be completed (Fick-NY). Publications will be developed related to the economics and environmental impact of grazing and comparison of grazing to confinement feeding (Rotz-PA). Models that can be used to track nutrient flows through the farm system or identify critical control points on individual farms will be made available on the internet (Kohn-MD, Mohtar-IN, Rotz-PA). 2c. Tools Several tools will be developed for field use as a result of the research outlined in objective one. The use of milk composition as a diagnostic tool to evaluate dairy cattle diets will be developed and tested. For example, milk urea nitrogen may be used as an indicator of protein feeding level, and nucleic acid end products (allantoin) can be used to predict microbial growth. Field data will be collected to determine the merits of utilizing milk allantoin (Harrison-WA) or milk urea nitrogen (Kohn-MD) as tools to monitor and adjust diets to maximize rumen microbial protein production (Harrison-WA). Milk composition may also be useful to estimate intake on pasture (Kohn-MD). The grazing stick will be evaluated for use in the field (Karsten-PA, Prigge-WV). Software will be developed that can be used in the field.The model DAFOSYM can be used by agricultural support personnel to evaluate options for farm management. This model is already available and used for this purpose, but it will be improved and evaluated for more farm conditions. In addition, software will be developed that can be used routinely to balance nutrient flows on specific farms and to evaluate areas that can be enhanced for optimal improvement in nutrient utilization efficiency (Dou-PA, Ferguson-PA, Kohn-MD). A software tool will be developed to quantify the appropriate amount of feed supplement needed in different grazing situations (Karsten-PA, Prigge-WV, Rotz-PA). WWW-based interfaces will be developed for the dairy system models such as DAFOSYM, GRASIM and DNP to be utilized in this project (Kohn-MD, Mohtar-IN). The WWW interfaces will allow users to interactively create the model input files, seek help for the models and their inputs, run the models, obtain results, and graphically display the results. The interface will allow users to interactively select the geographic region of interest. Spatial (GIS) databases will provide location specific data required by the models such as soil properties and weather (Mohtar-IN). Choice of the location of interest will result in the selection of weather files, default soil properties and other data from the database.Online supporting educational materials will be developed to provide additional details about the models and to describe the complex systems they simulate. A series of case studies will also be developed (Mohtar-IN) that will serve as exercises for users of the modeling systems and supporting educational materials. The case studies will be based on actual problems encountered in the field. They will solve problems inspired through the contacts the investigators have with producers and farm decision makers. A link (via email) to the principle investigators will be created for any additional information students might require. A major benefit from this process is that users are placed in direct contact with experts in the field of study. An expert system component will be developed (Mohtar-IN) to handle data entry, trouble shooting, and information searches. Specific case studies will serve as a prototype of the many questions students can address with the modeling systems. This set will include but not be limited to: * Evaluate effects of stocking rate on supplementation and amount of harvested feed;
* Estimate the financial and environmental consequences of alternative dairy management strategies including partial mechanical harvest in the context of the year round feed needs of the dairy herd;
* Determine the environmental impact of animal agriculture and the impact of grazing management on pollution;
* Identify on-farm management strategies that yield more efficient utilization of pastures, stocking rate, rotation cycle, beginning/end of grazing period, etc.
Measurement of Progress and Results
Outputs
- Evaluation of several cropping strategies using the models: DAFOSYM, GRASIM, and DNP).
- Information materials on issues related to grazing, manure management and cropping systems that can be used to disseminate information to dairy farmers.
- Software that can be readily used for nutrient management in small and medium sized farms.
- Interactive WWW-based system that is easily accessible and user friendly that can be used to determine the best dairy farm nutrient and waste management strategy.
Outcomes or Projected Impacts
- Farmers that can apply, in their own operations, low cost and effective methods for crop production and manure management.
- Improved nutrient utilization by cattle, and thus, reduced nutrient losses on farms.
- Environmentally sound and profitable dairy farm operations for small and medium scale farmers.