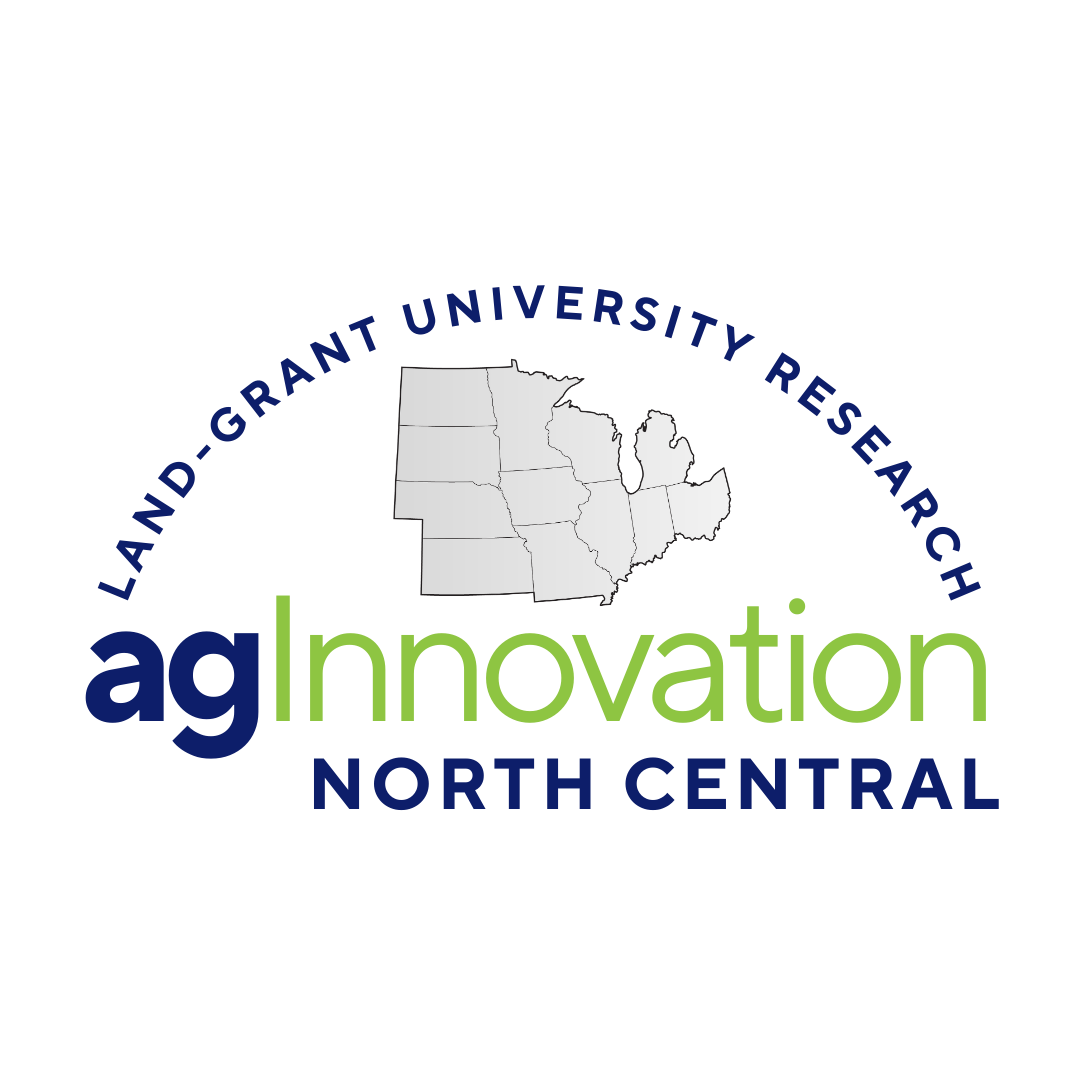
NC1031: Nanotechnology and Biosensors (NCDC201)
(Multistate Research Project)
Status: Inactive/Terminating
NC1031: Nanotechnology and Biosensors (NCDC201)
Duration: 10/01/2006 to 09/30/2011
Administrative Advisor(s):
NIFA Reps:
Non-Technical Summary
Statement of Issues and Justification
Nanoscale science, engineering and technology (nanotechnology) have great potential for application to the food and agricultural system. The novel physical, chemical, and biological properties of systems with structural features in the length scale for nanotechnology (1-100 nanometers) can allow the development of a new understanding of biological and physical phenomena in agricultural and food systems. In addition, nanotechnology allows scientists to measure, control, and manipulate matter at the nanoscale to change those properties and functions to the benefit of these systems.
The National Planning Workshop on nanoscale science and engineering for agriculture and food systems was a major first step in identifying the potential usefulness of nanotechnology in the food and agricultural system. Microfluidics, BioMEMS, nucleic acid bioengineering, smart treatment delivery systems, nanobioprocessing, bioanalytical nanosensors, nanomaterials, and bioselective surfaces were identified as having significant potential for application in the food and agricultural systems. Subsequently, the USDA through the CSREES (http://www.csrees.usda.gov/) has identified the following programmatic areas for potential program emphasis: nanosensors; identity preservation and historical tracking of products; smart treatment of delivery systems; novel tools; nanomaterials; agro-environment; and education.
Nanotechnology will play a major role in agricultural and food biosecurity, food and nutritional quality and safety, plant and animal disease detection and treatment delivery systems, new tools for molecular and cellular biology, new and better materials of agricultural origins, energy production and efficiency, and protection of the environment. Agricultural and food producers should gain a more competitive position through the application of nanotechnology and in the long-term consumers will benefit from the advances in nanotechnology that allow a competitive, innovative domestic agricultural and food system and provide methods for increased safety and nutrition of food products. Nanotechnology will also play a major role in assisting developing countries through enhancements in agricultural productivity and food processing and storage. Despite the strong support for nanotechnology, however, several groups have expressed concern regarding the societal impacts of the application of nanotechnology to the food and agricultural system and have recommend that the potential economic, environmental, safety and health impacts of nanotechnology should also be investigated.
To achieve the knowledge needed for successful application of nanotechnology to food, agricultural and biological systems, multi- and cross-disciplinary approaches must be developed that include not only engineering, biological sciences and chemistry but the social sciences as well. The collaborative structure of NCDS 201 will enable the stations to share knowledge and educational and research facilities and thus achieve these collaborative approaches. NCDC-201 will play an important role in keeping US agricultural and food producers in the forefront on the application of nanotechnology in the world and assure that it is applied to the benefit of the total food and agricultural system as well as society.
A search of the CRIS (USDA) database identified over 66 current or recent projects that are related to nanotechnology and 70 projects that are related to biosensors. This project brings together 12 cooperating stations from across the US and most members of NCDC-201 serve as principal investigators on one of the CRIS projects identified. NCDC-201 does not duplicate any other Multistate Research project and is currently the only such project that integrates several disciplines in the area of nanotechnology.
Related, Current and Previous Work
Fundamental Nanoscale properties and processes
Understanding of the unique and novel physical, chemical, and biological properties of systems at the nanoscale level in agricultural and food systems is essential before these nanoscale systems can be applied effectively to these systems. Several basic sciences must be employed to gain an understanding including quantum theory, genomics and technology (Vo-Dinh, 2005). Fundamental processes and phenomena associated with fabrication of nanomaterials and nanostructures must be better understood. Fabrication methods can take on a top-down approach (micro/nanofabrication based on lithography) or a bottom-up approach (self-assembly, polymerization, crystal growth etc.). Most of the applications related to nanomaterials exploit the small feature size (<100 nm) and high surface/volume ratio. The feature size can be optimized based on the needs associated with particular applications. Understanding of the fundamentals of nanoscale electronic and magnetic and electronic properties of nanoscale materials are especially important for sensing applications. Transport phenomena of liquid solutions at the nanoscale must also be understood. Although it is known that the size of these structures can affect the transport of biomolecules, further understanding of the basic physiological and biological phenomenon at the nanoscale level in biological systems is needed.
Use and development of Nanomaterials
Nanoparticles as Novel Bioactive Carriers. Nanoparticles are matrix systems of a dense polymeric network in which bioactive components may be dispersed. Since the nanoparticles are submicron and sub-cellular in size, they have advantages for delivering bioactive compounds. The choice of matrix depends on the chemical nature of the core material to be loaded, the choice between a biodegradable or inert nondegradable matrix, release characteristics, loading capacity of particles, cost and ease of preparation, and potential cytotoxicities. Proteins, polysaccharides, and synthetic polymers can be used for fabrication although protein-based particles are advantageous due to their biocompatibility, degradability, and chemical functionality. Degradation of the particle matrix allows sustained release of core materials for desired duration (Jones et al., 1989). Rate of release may be controlled by alterations in the preparation conditions, such as loading concentration, pH of the protein solution, and concentration of cross-linking agent (Kumar et al., 1994). Protein-based nanoparticles have been investigated extensively with respect to their preparation methods and release properties. Among these are albumins (Longo et al., 1982; Chen et al., 1987; Gupta and Hung, 1989; Arshady, 1990; Lin et al., 1993; Park et al., 1998), gelatin (Levy and Andry, 1990; Oner and Groves,1993), collagen (Rossler et al., 1995), legumin (Irache et al., 1995), vicilin (Espeleta et al., 1997) and bovine caseins and albumins (Saleh et al., 1989; Willmott et al., 1992; Knepp et al., 1993; Latha et al., 1994; Latha and Jayakrishnan, 1994; Latha et al., 1995; Heelan and Corrigan,1997). Polysaccharide-based carriers can be used but must be chemically modified to increase their hydrophobicity or combined with other hydrophobic polymers (Vandamme et al., 2002).
Self-assembled nano-structures. Biological hard tissues are composites of inorganics and organics and are formed by biomineralization, a process in which organic surfaces regulate the self-assembly of inorganic crystals. The synthesis of these materials involves the formation of hierarchical structures with complex architecture from the nanometer to the millimeter scale and consists primarily of minerals and natural polymers (proteins, polysaccharides, and lipids). Materials such as natural spider silk, and biological hard tissues such as bones, sea-shells, teeth and spicules are produced in aqueous solution close to neutral pH at atmospheric temperature and pressure and exhibit properties that are better than most synthetics. Natural materials display remarkable strength and toughness (Curry, 1977; Jackson 1988; Waite, 1998). Over 40 different naturally occurring biominerals have been reported (Lowenstam and Weiner,1989), the most common being phosphate and carbonate salts of calcium, that are used in conjunction with organic polymers, such as collagen and chitin, to give structural support to bones and shells (Rhee, 2001). A significant body of literature exists on crystal deposition and morphology (Heuer, 1992; Weiner and Addadi 1997; Dabbs and Aksay, 2000; Mann, 2000). Most research has been dedicated to elucidating the formation of inorganic nanoparticles in saturated solutions and understanding the mechanisms of crystallization and mutual transformations between CaCO3 polymorphs- calcite, aragonite and vaterite. Process development leading to data on kinetics, properties, defect detection, and process monitoring will have to become important if self-assembled synthesized biominerals are to realize their potential as practical processing methods (Tomalia, 1999).
Nanocomposites. The formation of polymer nanocomposites has attracted interest since the late 1980's when Toyota researchers found that the mechanical and thermal properties of nylons increased significantly with the addition of nano-sized clays (McGlashan and Halley, 2003). Polymer-clay nanocomposites are a class of hybrid materials composed of organic polymer matrices and nanoscale organophilic clay fillers (Kim et al., 2003). Of nanoscale clays, montmorillonite (MMT) is of particular interest and has been studied widely. MMT is a hydrated alumina-silicate layered clay made up of two silica tetrahedral sheets fused to an edge-shared octahedral sheet of aluminum hydroxide (Uyama et al., 2003; Kim et al., 2003). The inclusion of a small percentage, by weight, of clay in a polymer matrix significantly enhances the mechanical and thermal properties, the moisture stability and flame and weathering resistances. Beyond the conventional approach, where the polymer and clay particles remain immiscible (tactoids), two types of hybrids, intercalation and exfoliation are ideal nano-scale composites. The structure and properties of the resulting nanocomposites can be altered by controlling subtle polymer-clay interactions (Oya and Kurokawa, 2000). Dufresne et al. (1996) and Mathew and Dufresne (2002) studied nanocomposites from starch and amorphous poly(beta-hydroxyoctanoate) and starch and tucinin whiskers, respectively. Uyama et al. (2003) developed nanocomposites from plant oil-clay hybrid materials. Park et al. (2003) prepared thermoplastic starch (TPS)/clay nanocomposites through a melt intercalation method using two clays, Cloisite 30B with ammonium cations located in the silicate gallery and one unmodified Cloisite Na+.
Chitosan derived from chitin possesses a unique cationic nature and ha also been used in nanocomposites. Chitosan is nontoxic and compatible with living tissue and thus has potential use in healing, artificial skin, food preservation, cosmetics, and wastewater treatment (Boddu and Smith, 2002; Juang and Shao, 2002; Klingels et al., 1999; Mao et al., 2003; Risbud et al., 2000; Wu et al., 2002). The hydrophilic character and poor mechanical properties in water limits the use of chitosan in these uses. Developing chitosan layered silicate nanocomposites by inserting chitosan chains into interlayers of silicate can improve its mechanical properties. Such nanocomposites have superior thermal and mechanical properties as compared to the polymer itself (Kumar et al., 2003; Kim et al., 2003). Yamaguchi et al. (2001) prepared a chitosan/hydroxyapatite nanocomposite for orthopedic use.
Nanoclay and modified nanoclay as sorbents for anionic, cationic and nonionic dyes.
Dye sorption is an important parameter for coloration and wastewater control. Cationic treatment of cellulose adds quaternary ammonium onto cellulose to provide strong attraction to anionically charged dyes, such as reactive, acid, and direct dyes (Cardamone and Turner, 2000; Harper and Stone, 1986; Hauser et al., 2003). Anionic treatment of polyester adds a co-monomer with sulfonate onto polyester chain, so that polyester thus produced can attract cationically charged basic dyes (Matsumura et al., 1991; Sasamoto et al., 1986). Sorbent can also be added into the polymer matrix to make a composite and textiles made from that composite have good dye sorption ability (Fan et al., 2003 a, b). Using the sorbent eliminates the need for chemical reactivity and the sorbent may have multifunctions and be more economically feasible. They also may serve as delustrant and improve strength, modulus, UV absorbance, and fire resistance of the textiles. Dye sorption on nanoclay is useful for coloration and wastewater color removal as well as providing understanding of sorption behavior of nanoclays to anionic, cationic, and nonionic chemicals (Fan et al., 2003 a, b; Armagan et al., 2003; Bae et al., 2000; Miyamoto et al., 2000; Vinod and Anirudhan, 2003; Yermiyahu et al., 2003).
Nanoscale Devices and Systems
Combining biology with nanoscale technology could allow development of sensors with increased sensitivity and reduced response time. Such bioanalytical nanosensors could be used for detection of pathogens, contaminants, nutrients, environmental characteristics, heavy metals, particulates, and allergens. Biosensors needed are expected to be small, portable, rapid response and processing (i.e. real-time), sensitive, specific, robust, quantitative, reproducible, accurate, reliable, and stable (Scott et al., 2003). Biosensor systems likely will combine MEMS, wireless communication, chip design, and molecular biology nanotechnology techniques. The development of novel and integrated sensors/sensing mechanisms warrants intensive research. Transducers must also be developed that include smart and efficient algorithms for processing the information developed.
Use of conducting polymers. Electrically conducting polymers provide considerable flexibility in modifying their chemical structure (Muhammad-Tahir et al., 2003, 2005). By chemical modeling and synthesis, it is possible to modulate the required electrical and mechanical properties. Conducting polymers also are also known for their ability to be compatible with biological molecules in neutral aqueous solutions and their efficiency in transferring electrical charges produced by the biochemical reaction into the electronic circuit (Muhammad-Tahir and Alocilja, 2003).
Use of micro-Total Analysis Systems. The development of sensors and complete micro-Total Analysis Systems (micro-TAS) based on silicon technology and soft lithography processes for mass production of biocompatible plastic devices integrating nanometer feature sizes and defined localization of molecules in the nanometer and subnanometer range is important. These devices are used for providing small sample size, fast response time, and high-throughput capabilities to the detection of food and environmental composition, contamination, and adulterations. The adaptation of micro-TAS to the complex matrices of food and environmental samples and the need of lowest detection limits in order to reduce the sample size required to be processed by the system require the integration of detection and sample preparation modules. While many nano- and microsensors have been suggested in the literature, information on their integration with a compatible miniaturized sample preparation is limited, as is the description of effectively miniaturized sample preparation steps and miniaturized detectors.
Protein nanoarrays. Current efforts in constructing a protein nanoarray, such as atomic force microscope (AFM) based dip-pen nanolithography (DPN) must overcome the barriers of complexity and price of the equipment required (AFM), cross-contamination during protein patterning, and the limit in the number of protein components. The proposed protein nanoarray system is expected to be less complicated and capable of building multi-component nanoarray in a much easier manner.
Pathogen detection. One of the first applications of biosensors will be in the area of pathogen detection in agricultural and food systems. Current cell culture techniques for the detection of bacteria are often ineffective, insensitive, and time consuming (Jaykus, 2003; Ivnitiski et al., 1999a, b). Biosensors offer an excellent way of overcoming these problems and can provide a rapid, selective, and highly sensitive method for microbiological analysis of foods. Ivnitiski et al. (1999a, b) divide biosensors into four groups based on the method of signal transduction: optical, mass, electrochemical, and thermal. Several papers have been written on the use of surface plasmon resonance (SPR), fluorescence (Ivnitski et al. 1999a,b), and reflectance (Hartley and Baeumner, 2003; Baeumner, et al., 2003). Several optical techniques are based on fluorescence and Jaykus (2003) described two systems which are commercially available and based on fluorescence in combination with PCR and NASBA. Fluorescence and SPR also have been used for the detection of toxins in foods (Naimushin et al., 2002; Kijek et al., 2000). Electrochemical or electronic detection methods include: conductometric, impedimetric, and voltammetric methods (Muhammad-Tahir and Alocilja, 2003, 2004, 2005; Radke and Alocilja, 2004, 2005a, b; Rodriguez and Alocilja, 2005; Mathew and Alocilja, 2005). Electrochemical methods have been used for detection of bacteria (Ivnitski et al., 1999b) applied to microbial-based, antibody-based (Muhammad-Tahir and Alocilja, 2003, 2004, 2005; Radke and Alocilja, 2004, 2005a, b) and DNA-based biosensors (Rodriguez and Alocilja, 2005). Schoning and Poghossian (2002) also describe the use of biologically sensitive field effect transistors (BioFET) as biosensors. These nano transistors can be utilized to directly detect targets such as bacteria, DNA, and virus (Cui et al., 2001; Lieber, 2001).
Economic, environmental, safety and health impacts of Nanotechnology
Agricultural and food producers are expected to gain a more competitive position through the application of nanotechnology. In the long-term, however, consumers also will benefit from the advances in nanotechnology that allow for competitive, innovative domestic agricultural and food systems as well provide methods for increased safety and nutrition of food products. Ross et al. (2004) states that nanotechnology can benefit health and nutrition through development of more efficient delivery systems; improved food packaging through nanomaterials with enhanced properties; improved food safety through biosensors and new equipment surfaces to prevent surface adhesion; biomaterial characterization at the nanoscopic level; and nanodevices for real-time intracellular sensing. Scott (2005) suggested that nanotechnology could be applied to animal health in drug delivery, disease diagnosis and treatment, and breeding and identity preservation. Salmanaca-Buentello et al. (2005) reported on the potential advantages of nanotechnology for developing countries and both agricultural productivity and food processing were rated in the top ten nanotechnology applications.
Despite the strong support for nanotechnology, groups have expressed concern regarding the societal impacts of the application of nanotechnology to the food and agricultural system. ETC Group (2004) published an article, "Down on the Farm", that discusses the potential economic, environmental, safety, and health impacts of nanotechnology and recommends that society become fully engaged on a wide ranging discussion on the role of the converging nanoscale technologies in food and agriculture. Cranor (2003) made similar recommendations recommending cautionary approaches that allow the benefits of new technologies without the risks and costs. Scott (2005) states that we have the ethical responsibility to apply nanotechnology wisely and to recognize there are unforeseen risks that may come with the tremendous positive potential.
Risk of nanomaterials to the environment. At the present time there has been little or no information developed regarding the impact of nanotubes or nanoparticles on the environment. Dreher (2004) has raised the following issues: toxicology of manufactured nanoparticles; environmental and biological fate, transport, persistence, and transformation of manufactured nanoparticles; and recyclability and overall sustainability of manufactured nanomaterials. The same types of characteristics that make nanoparticles useful could lead to concerns about their impact on the environment. It is thus important to know the stability, solubility, degradation, and fate of nanoparticles in the environment especially in soil and in aqueous environments. Particle dissolution, agglomeration, and precipitation in aqueous solutions as well as changes in particle characteristics are important. In addition, transport through soil and toxicity to aquatic invertebrate species and how the microbes in the environment react and interact with the nanomaterials when exposed need additional study.
Preliminary toxicity tests with "inert" titanium dioxide (TiO2) nanoparticles using Daphnia species have found concentrations lethal to 50% of test organisms (LC50 levels) as low as 4 mg/L (Lovern et al., 2004). Toxicity to higher aquatic organisms such as fish and amphibians, chronic toxicity, and toxicity of more reactive and potentially more toxic metal oxide nanoparticles has not been established. Metal oxide nanoparticles are used in chemical manufacture, optics, electronics, hazardous waste remediation, and medicine (Couvreur et al., 1995, Jortner and Rao, 2002, Hernandez-Santos et al. 2002, Mahato et al., 2003; Zaharescu et al., 2000; Hong et al., 2003; Choo and Kang, 2003; Zang, 2003; Illy-Cherrey et al., 2002). Thus, there is potential for wide-scale environmental release of these nanoparticles and recently concerns have been raised about the safety and toxicity of nanoparticles in the environment (Gogotsi 2003; Kleiner and Hogan 2003; Service 2003; Borm and Kreyling 2004; Dreher 2004, Goodman et al. 2004; Oberdörster 2004). The aquatic environment may be of particular concern (Moore 2002). Nanoparticles need not be absorbed or metabolized by aquatic organisms to elicit toxic effects. For example, in mammals, nanoparticles can induce lung injury without being absorbed by the body (Gradon et al. 2000, Borm 2002, Lam et al. 2004, Warheit et al. 2004). An analogous effect in aquatic organisms would be disruption of gill function, a major contributor to metal-induced toxicity (Niyogi and Wood 2003). There is also evidence that, in mammals, nanoparticles may be absorbed by the gastrointestinal mucosa and pass into the lymphatic system (Jani et al. 2003) or may cross blood vessels to enter the bloodstream and transported to other organs (Bennett 2002). Furthermore, it has been found that fluorescent microspheres, which are much larger than nanoparticles, can enter fish skin and gills and can be engulfed by macrophages (Kiryu et al. 2000). Similar routes of entry may exist for even smaller nanoparticles.
Risk of nanoparticles to health. The molecular design and manipulation of raw materials that typically are required to produce well-defined nanoscale structures can often result in increased toxicity levels, which is unacceptable in food applications. Chen et al. (2005) reported that copper nanoparticles (23.5nm) induced toxicological effects and injuries on kidney, liver and spleen of experimental mice, but micro-copper particles did not, on mass basis. Liu et al. (2005) studied the toxicity of nano-hydroxyapatite on rabbits by vein and suggested that nano-hydroxyapatite has no accumulative toxicity to rabbits and is safe when the hydroxyapatite-sol is applied intravenously as a drug carrier in small dosage. However, there is a lack of knowledge about the occurrence of nanoparticles in the food supply and the influence of these particles on the general health of gastro-epithelial cells and the ability of these cells to resist pathogenic infections. If diffusion resistance of mucosal layer equals that of the transported food, particles penetrate this layer with almost the same rate as the food product. However, depending on the Hamaeker constant of the mucos, the suspension stability can be influenced and aggregation of the nanoparticles can occur. Migration of nanoparticles from the food transported through the GI-tract to the epithelial cells occurs through the mucosal layer. This layer can be considered as a medium with transport properties differing from the GI bulk. Concentration distribution of nanoparticles within the mucosal layer and the number of nanoparticles approaching the epithelial cells is determined by the concentration gradient of the particles, the size distribution of nanoparticles, and the stability of this nano-colloidal suspension in a changing and non-uniform environment.
Educational needs on the use and impacts of nanotechnology
The broad application of nanotechnology will have a major impact on society. To achieve the knowledge needed for successful application of nanotechnology to food, agricultural and biological systems, multi- and cross-disciplinary approaches must be developed that include not only engineering, biological sciences, and chemistry but the social sciences as well. Future generations of scientists and engineers must have an understanding of different aspects of nanotechnologies. Our educational system must prepare students to apply, discover, and create new systems and/or solutions for agricultural and biological problems. It is projected that 2 million workers related to nanotechnology will be needed by 2015, with the US alone needing 0.8-0.9 million workers (National Nanotechnology Infrastructure Network, 2005). In addition, the general public must be trained to engage in active and broad ethical discussions on the use of nanotechnology. This will allow future generations to establish the required checks and balances needed in a nanotechnology society.
Objectives
-
To provide an understanding of fundamental nanoscale phenomena and processes.
-
To develop and characterize nanomaterials.
-
To develop nanoscale devices and systems.
-
To provide an understanding of economic, environmental, safety and health impacts of nanotechnology in agricultural, food and biological systems.
-
To develop educational and outreach programs on the use and impacts of nanotechnology in food, agricultural and biological systems.
Methods
Objective 1. To provide an understanding of fundamental nanoscale phenomena and processes The University of Missouri-Columbia, Michigan State University, Auburn University, Rutgers University, Cornell University, Purdue University, Utah State University; University of Idaho, University of Arkansas, and North Dakota State University will contribute to this objective. Task 1. To study fundamentals processes and phenomena associated with fabrication of nanomaterials and nanostructures. Missouri will contribute to this task by developing core/shell structured quantum dots for fluorescence resonant energy transfer studies with protein and DNA molecules. The group also will work on aptamer-based molecular beacon based on QDs. The QDs will be used for sensor array development and imaging applications. Purdue is doing research on the resonance and enhancement effect of nanoparticle networks. Iron and gold coated iron particles have been fabricated and enzymes and proteins have been attached and their kinetics assessed. Utah will study bottom up engineering of artificial recognition elements through template induced surface patterning. In molecular imprinting the target molecule serves as a template, around which a binding pocket is assembled that is complementary in shape and chemistry to the template. Development of global recognition elements through molecular imprinting of self-assembled monolayer films can report the conformation of the target through altered binding affinities and may lead to surfaces that direct proteins to adopt a given (imprinted) conformation. They will restrict processes that occur readily in bulk solution to a surface that may be coupled to a sensitive and low cost transducing element, or serve as a coating for a material. Michigan State University will study the interaction and conjugation of polyaniline nanowires and carbon nanotubes as they form into nanocomposite wire materials. They are working on developing soluble polyaniline-SWCNT nanowires (Muhammad-Tahir et al., 2005) and the molecular imprinting of protein structures as biomimic to antibodies (Kindschy and Alocilja, 2005, 2006). North Dakota State will develop intelligent sensors for detecting metabolites and pathogens associated with food and health with a focus on understanding how different sensors or sensing mechanisms work for different animals and plants. Task 2. To investigate the size effects related to nanomaterials. Most of the applications related to nanomaterials exploit the small feature size (<100 nm) and high surface/volume ratio. The feature size can be optimized based on the needs associated with particular applications. Task 3. To study fundamentals of nanoscale electronic and magnetic properties. Auburn will contribute to this goal by studying fabrication of nano-scale magnetostrictive particles (nanobars and nanostrips) as a sensor platform. Nanobars will be prepared by electrochemical deposition using polymer and ceramics templates. The surface of the nanobars will be coated with a self-assembling monolayer (SAM) of designed chemicals. Then, the antibody or any other bio-molecular recognition probe will be immobilized onto the SAM-coated magnetostrictive particles. Nanostrips will be fabricated from magnetostrictive thin film using micro-electronic processes. The surface of the strips will be coated by SAM and then immobilized with bio-molecular recognition probe. The magnetostrictive property and magnetic behavior of the nanobars will be characterized to determine the sensor performance and to maximize the performance by optimizing the composition of magnetostrictive materials. Task 4. To study transport phenomena of liquid solutions at nanoscale. Cornell will study microfluidic mixers which are an integral component of microscale total analysis systems (TAS). Turbulence, the primary mechanism for macro-scale mixing, is effectively absent under normal conditions. Cornell has designed a passive mixer based on reciprocal flow and enhanced diffusion via continuously changing and increasing the concentration gradients within the solution. This mixer will be used to study DNA/RNA hybridization and antibody/antigen binding events, as well as the enhancement of enzymatic reactions. Purdue will investigate transport of biopolymeric polyelectrolytes such as dsDNA and SDS denatured proteins under an electrical field in fluidic channels with one critical dimension (the depth) at nanoscale (<100 nm). The electrostatic interaction between the electrical double layer and the charged polyelectrolytes and the electrohydrodynamic chromatography effect due to the non-uniform velocity profile may be important at nanoscale. Purdue also will develop a model to fully characterize the transport phenomena in this case. They also will develop ultrasensitive sensors based on nanoporous silicon for detecting biomolecules and vapor of volatile organic compounds (VOCs) in air. Objective 2. To develop and characterize nanomaterials The University of Nebraska, Michigan State University, Purdue University, North Dakota State University, University of Minnesota, and the University of Wisconsin will contribute to this objective. Task 1. Encapsulation of nanoparticles. Wisconsin will concentrate on using whey protein nanoparticles as novel bioactive carriers for dairy and other food and non-food applications with a focus on the preparation of ²-lactoglobulin (BLG) and whey protein isolate (WPI) nanoparticles. Fairly uniform size nanoparticles can be prepared by carefully adjusting processing conditions. In order to prevent agglomeration between the particles, we will modify the particles using carboxymethylation capable of producing hydrophilic residues, and apply surfactants to lower their interfacial tension. Vanillin, will be encapsulated into whey protein nanoparticles. The type of matrix ingredients and the processing conditions needed for optimal degradation and release properties will be determined. Nebraska will contribute to this effort using electro-spraying to prepare encapsulated materials. The analyses will be the same as proposed by Wisconsin. Purdue will use membrane containing nanoparticles functionalized with biological activity as surrogates or "artificial cells" to replace living cells in whole cell biosensors. The lipid bilayer membrane will be used to mimic the cell membrane creating both an "intracellular" lumen for soluble signaling molecules and a support for membrane associated proteins. Biological functionality will be built by assembling different proteins and signaling molecules to mimic a whole cell response to analyte. Membrane properties and lipid composition will be controlled to manipulate sensors performance including response and selectivity. Liposomal particles will be produced and serve as a platform material for the detection of bacterial toxins by mimicking the mammalian cell response. Particle properties will be characterized through dynamic light scattering, electron microscopy, and fluorescence assays of stability, membrane properties, and response to toxins. Liposomal particles will be encapsulated using sol-gel synthesis of inorganic glass such as silica. Both bulk materials and thick films will be produced and characterized using electron microscopy and various fluorescence assays and spectroscopic methods. The performance of the materials in response to both toxins and bacteria will be characterized. Task 2. Self-assembled nano-composites. Minnesota will develop a template for CaCO3 deposition using a polyelectrolyte in a layer-by-layer (LBL) technique. The polyelectrolytes will be deposited on different substrates (quartz, glass, silica wafer). After treatment with "piranha" solution (and treatment with oxygen plasma if needed) a photoresist layer will be spin coated on the substrate and exposed to UV irradiation. The LBL assembly will include absorption of chitosan followed by aqueous poly (acrylic acid). After repetition for n times, a composite multilayer film will be formed with n bilayers on both sides of the substrates. The template of layered composite will be dipped into calcium chloride (Addadi, 1987) and a photoresist layer will be spin coated on the substrate. After UV irradiation, it will be immersed alternately in collagen and chondroitin sulfate. Multilayer stacks containing (collagen/chondroitin sulfate)n/collagen deposited on the substrate will be evaluated for their morphology and mechanical properties. The materials obtained will be soaked in simulated body fluid (SBF) to examine apatite formation on the surface. A quartz crystal microbalance (QCM) will be used to monitor the mass change of material deposited on the surface; a Nanospec film thickness measurement system or an ellipsometer to measure the thickness of materials deposited on the polymeric or silicon substrate and a Zeta potential analyzer to measure the electronic charges on the surfaces of colloids and nanoparticle suspensions. Transmission electron microscopy (TEM) and scanning electron microscopy (SEM) will be used to distinguish between natural and synthetic polymers. A surface profiler will be used to analyze the surface texture. Quantification of crystals adhesion force to the surface will be estimated using AFM. Matrices will be evaluated for tensile strength, flexural strength, and impact properties as a function of the number of layers. Nano-indentation using AFM will be used to determine the quantitative relationship between load and displacement at various locations on the surface. The surface stress will be estimated from the surface curvature before and after thin film deposition using a Wafer Bow sensing system. The modulus will be estimated from the load displacement curves based on the approach of Kracke and Damaschke (2000). Task 3. Nano-patterned materials. Nanoarrays of sensor materials on fabricated chip devices will enable both high density multianalyte detection and high spatial resolution that is currently only achieved with microscopes. Purdue will use sol-gel synthesis methods to produce hybrid patternable materials for sensor applications. These materials will be doped with organic fluorophores to impart chemical sensitivity. Ion selective fluorophores will be used as the first prototype materials as they have a well established use as sensors and ion sensing has numerous applications in environmental monitoring and biological sensing. In addition, photosensitive precursors will be incorporated into the matrix forming materials in order to enable selective polymerization and patterning. These dual functionality materials will be characterized using electron microscopy and fluorescence spectroscopy. They will also be tested for their ability to form patterned arrays using photolithographic methods. The sensitivity of the materials will be tested after patterning using fluorescence spectroscopy and imaging at various analyte concentrations. Task 4. PLA-nanoclay composites with enhanced physical, mechanical, thermal and adsorption properties and controlled biodegradability characteristics. Biodegradable nanocomposites with enhanced physical, mechanical, thermal, and adsorption properties will be developed for applications in packaging, controlled drug delivery, filtration, and adsorption fields. Nebraska will focus on development of biodegradable nanocomposites such as plastics and foams, using starch, PLA and their derivatives and nanoparticles such as nanoclays . They will also develop models for the interactions of different clays and polymers. Objective 3. To develop nanoscale devices and systems Auburn University, Clemson University, Cornell University, Michigan State University, North Dakota State University, Purdue University, Rutgers University, University of Arizona, University of Arkansas, and the University of Idaho will contribute to this objective. Task 1. Development of nano-transducers and bio-recognition system. The main focus will be the development of nano-transducers with corresponding bio-recognition systems. Research activities will revolve around the development of nanowires, nanobars, nano-pillars, and nanopores. Specific activities include:-Design and development of conductive polyaniline nanowires with uniformly structured length and thickness using organic acids and enzyme synthesis. These nanowires will be characterized for their conductive and physical properties, interaction with bio-recognition elements (antibodies and DNA probe), and nano-structure phenomena.
-Nanopattern of IgG to a desired nanoarray location through size-dependent assembly of gold nanoparticles (AuNPs) or quantum dots (QDs) onto the nanometer channels or squares etched by an electron beam. Biorecognition of IgG will be determined through optical detection of fluorescence-tagged AuNPs or QDs with varying emission wavelengths.
-Development of controlled interfacing techniques for biological (such as DNA, proteins and antibodies) and abiological materials (such as carbon nanotubes and nanoparticles).
-Biofunctionalization of composite nanoparticles and materials with multiple functionalities for the development of DNA and protein sensors.
-Conversion of existing fluorescence and chem/bioluminescence-based sensing recognition solution schemes into nanopatternable solid state materials.
-Identification of receptor molecules for the fabrication of biosensor.
-Synthesis of metaloxide nano-patterned substrate for biosensor applications.
-Use of antibody-DNA, aptamer-DNA and other DNA conjugates for production of a uniform RNA signal correlated to biological recognition of proteins, whole cells and DNA/RNA through bionanotransduction.
-Development of nano-structured materials to be used as bio-mimetic transducers representing mammalian sensing capabilities (olfactory sensing, taste, touch etc.) Task 2. Device design. Focus is on the design and fabrication of novel biosensor platforms using conventional and state-of-the-art fabrication facilities. Specific activities include:
-Fabrication of multi-array channels for simultaneous detection in one sample application using electrochemical and optical signal measurement.
-Fabrication of deep devices in glass and plastic for cell transport and recovery as well as micro and nano-optical arrays for non-labeled sensing.
-Development of nano and micro array patterned materials for fluorescent and luminescent based chemical and biological sensing of trace contaminants, toxins, and biological signaling molecules.
-Development of a multi-component protein nanoarray.
-System design and protocol development.
-Fabrication of devices incorporating field effect transistors for detection of molecular charge.
-Fabrication of MEMS-based components to be integrated with nano-scale systems/devices.
-Use of computational simulation and modeling techniques for rapid development and evaluation of MEMS/nano-scale devices. Task 3. System integration and applications. Effective application of biosensors will require system approaches that integrate all steps into an efficient and effective system. Systems need to be flexible and capable of being used to match the most appropriate target organism/ target type to a detection platform. Nano-transducers, bio-receptors, and signal processing protocols will be integrated into devices for detection of biological and chemical contaminants. Effect of sample matrix will also be evaluated. Specific activities include:
-Integration of multi-array device using electrochemical and optical signal measurement for detecting bacteria, viruses, toxins, and proteins.
-Integration of nanopatterned chemo and bioresponsive materials into nano and microfabricated devices that include integrated optical, power, and wireless transmission components.
-Application of protein nanoarray towards screening/identifying recombinant crops/therapeutic proteins.
-Integration of active components with other modules, such as mixers, heaters, magnets and filters and their performance with sample matrices such as food, surface, run-off and ground water, manure and soil.
-Effect of food matrix (pH, soluble and insoluble solids and particulate inclusions) on biodetection and target recognition.
-Development of intelligent algorithm and software for developing intelligent nano-scale sensors/devices. Objective 4. To provide an understanding of the economic, environmental, safety and health impacts of nanotechnology in agricultural, food and biological processing systems Clemson University, Cornell University, and Rutgers University will contribute to this objective. Task 1. Stability, degradation, and fate of nanoparticles. Clemson will study the behavior of nanoparticles in aqueous environments. Particle dissolution, agglomeration, and precipitation in aqueous solutions simulating natural waters will be measured as well as changes in particle characteristics. For selected particle types, transport through soil columns and toxicity to an aquatic invertebrate specie will be performed. Nanoparticles of polystyrene, titanium dioxide (TiO2), iron oxide (hematite (a-Fe2O3), copper oxide (CuO), and zinc oxide (ZnO) will be synthesized using solution techniques from the literature (Qu et al., 2005, Lee and Liu 2002; Mulvaney et al., 1988; Viano et al., 2003; Bahnemann et al. 1987). The particles sizes and crystal structures will be characterized using electron microscopy techniques (e.g., TEM, EM and FESEM) and x-ray diffraction (XRD). Electron microscopes with energy dispersive x-ray (EDX) and x-ray photoelectron spectroscopy (XPS) capabilities will be used to check elemental composition and valence. Particle surface area will be determined with BET isotherm methods. Suspensions of nanosize particles can be characterized by optical spectroscopic methods such as UV-vis absorption since semiconductor particles (i.e., TiO2, Fe2O3, and ZnO) often show bandgap absorption in the near-UV range. Therefore, UV-vis absorption spectra will be obtained as a function of time for particles suspended in water and in solutions simulating natural waters. Loss of absorption intensity or decrease in bandgap energy will provide an indication of particle growth, coagulation, and precipitation while spectral changes matching dissolved species (e.g., loss of bandgap absorption) will indicate particle dissolution. Such tests will be used to determine the stability of nanoparticle suspensions over time. Particle characteristics will be reexamined by electron microscopy. For particle suspensions that are stable for several days, transport and toxicity tests will be performed. Transport tests using soil columns will be performed following procedures outlined by Schrick (2004). Toxicity of nanoparticle suspensions to an aquatic invertebrate (water flea), will be performed as an indication of bioavailability of toxic substances (Huggett 1999; Suedel 1996). Task 2. Nanoparticle penetration through biological barriers. Rutgers will study the risk of entrapment nanoparticles in the GIT due to interactions of nanoparticles with the epithelial cells and enhancement of the pathogens virulence in the presence of nanoparticles. Nanoparticles trapped inside the cell directly influence cell cytoskeleton and can influence cell metabolism and resistance. It is difficult to predict the overall result of pathogen-epithelium interaction and infection development in the presence of the nanoscale particles. The system response is complex and requires both theoretical and experimental studies including:
-Transport mechanisms of nanoparticles from the model food system to the epithelial cells, including nanoparticles transport through the mucosal layer;
-Survival of the bacterial and epithelial cells in the presence of nanoparticles in the environment; and
-Virulence of the pathogenic bacteria in the presence of nanoparticles. Evaluation of the risk factors related to the presence of nanoparticles on the infection development in model GI-tract (Caco-2, T2336, and other cell lines).
Task 3. Economic estimates of benefits to agriculture and food processing. Numerous studies have been completed in recent years on the economic impact of new technologies. Studies are planned to assess the specific economic and impact of nanotechnology on agricultural and food systems. Task 4. Communication of risk and benefits of nanotechnology. Results from other tasks within this objective as well as the other objectives will be collected and analyzed to assess potential risks and benefits associated with the use of nanomaterials and nanotechnology. Additional reports of the behavior and safety of such materials also will be sought from the literature. Together, researchers supporting this committee will prepare a "Nanotechnology and Biosensors Fact Sheet" to be evaluated by USDA officials for communicating basic information, including potential benefits and risks, to the public. Objective 5. To develop educational and outreach programs on the use and impacts of nanotechnology in food, agricultural and biological systems Cornell, Clemson, Michigan State University, Purdue University, North Dakota State University, University of Idaho, University of Minnesota, University of Nebraska, and the University of Wisconsin will contribute to this objective. Task 1. To develop undergraduate/graduate courses at the university level to train future professionals. Course modules will be developed for: micro/nano fabrication; instrumentation/characterization of micro/nano system; nano bio science; biology (molecular/cellular) for nonbiologist; nano/micro scale modeling techniques and tools; sensors/smart devices; microfluidics; quantum mechanics; and application of nanotechnologies/systems. Committee members will form subcommittees to develop the course modules. These materials will be made available on a shared website so that educators/individuals can use the materials for developing courses or other educational materials. Task 2. To conduct short courses/seminars (team taught). Training will be provided via short courses and at various conferences related to agricultural, food, and biological applications. Specialty conferences will be developed on nanotechnologies and sensors and conducted in conjunction with regional, national, and international conferences held by different professional organizations. Task 3. To develop a website that can be dedicated to provide information on nanotechnologies use for agriculture, food and biological application. A website will be developed having links to other links related to nanotechnology research and education. Task 4. To develop university based outreach extension activities (extension conferences, publications) to disseminate research-based information and create basic awareness about nanotechnologies and its potential/benefit in food, agriculture and biological systems.
Measurement of Progress and Results
Outputs
- Research articles in peer reviewed journals and publications, presentations at national and international meetings and workshops and patents.
- Course modules on different aspects of nanotechnology which are accessible to the educational community and public.
- Workshops and seminars on nanotechnology with proceedings of the workshops made available to public.
- Website which serves as a resource clearing house for educational communities and the public.
- Prototype nano-biosensors incorporating Pani nanowires, nanoparticles, nanobars, nano-pillars, and nanopores.
- Output 6 Prototype devices tested with bacteria, viruses, and toxins, chemicals, and other contaminants. Output 7 Input parameters to the water distribution simulation models such as EPANET so that pathogen distribution can be either interpolated (in between sensors) or extrapolated (away from the sensor networks). Output 8 New and reliable data of the fundamental nanoscale phenomena and processes in food and agricultural products as well as the processes that apply to these products. Output 9 New information on the properties of nanoclays and biodegradable polymers with development of a new class of biodegradable materials that will meet the current and future demands of industry including controlled functional and biodegradability characteristics. Output 10 New processes for the production of new plastics and foams with enhanced properties including methods for fabrication of nanocomposites into foams, sheets and other articles.
Outcomes or Projected Impacts
- Greater understanding of nanotechnology by the public and the agricultural and food industry.
- Increased awareness and application of nanotechnology to agricultural, food and biological systems.
- Increased number of students from land grant universities with training in the basic techniques of nanotechnology.
- Development of tools and products which exploit the novel properties of nanomaterials and nanoscale devices and benefit different aspects of agriculture, food safety and biological engineering research.
- Understanding of the fundamental nanoscale phenomena and processes in food and agricultural products as well as the processes that apply to these products.
- Outcome/Impact 6 Understanding of the potential of certain techniques and devices and what is needed to optimize and improve them within a theoretical context. Outcome/Impact 7 Development of nanoscale devices and systems that will advance the capabilities of currently designed devices for higher performance (sensitivity, speed of detection, and applications for example). o Development of a prototype bio-nanosensors including specifications for the design and synthesis of Pani NW, bio-nanowires and microchannels. o Development of nanoarrays for use in detecting and screening proteins from complicated expression systems (such as production of recombinant therapeutic proteins from mammalian cell culture or even from transgenic plants). o Multi-component protein nanoarray where each grid contains a single protein molecule. o A lab-on-a-chip type high-throughput biosensing system which can assess water quality parameters on-site within a short time period.
Milestones
(2006): Single molecule IgG patterning; Single channel lab-on-a-chip sensor;(2007): Course modules on different aspects of nanotechnology;Workshops and seminars on nanotechnology with proceedings of the workshops made available to public;Development of sensors for use in detection of pathogens in food products.
(2008): Website which serves as a resource clearing house for educational communities and the public.
(2009): System for multi-component detection of BACs
(2010): Development of multi-component nanoarray construction;Development of a database of fundamental nanoscale phenomena and processes in food and agricultural products established;demonstration in a water distribution detection system.
Projected Participation
View Appendix E: ParticipationOutreach Plan
The immediate beneficiaries of this research will be industry, government agencies, and other university scientists who will have access to findings through peer-reviewed journal articles, abstracts, presentations at scientific meetings and workshops, books, and book chapters. A number of participating stations have extension centers that will facilitate transfer of findings to industry. Agricultural and food producers are expected to gain a more competitive position through the application of nanotechnology but in the long-term, consumers also will benefit from the advances in nanotechnology that allow a competitive, innovative domestic agricultural and food system as well provide methods for increased safety and nutrition of food products.
Organization/Governance
NCDC-201 is organized according to the guidelines in the USDA Multistate Research Manual, as found at http://www.wisc.edu/ncra/regionalmanual.doc. Membership includes an administrative advisor (Dr. Vincent Bralts), a CSREES representative (Dr. Hongda Chen) and project leaders from 12 cooperating stations. A Steering Committee was created at the 2004 Annual meeting with the specific goals to develop a proposal. Meetings are held annually, typically in October. The secretary for the coming year is elected at the end of each meeting. The previous secretary moves up to vice-chair and the vice-chair to chair. These three officers, the past chair, and the administrative advisor constitute the executive committee. The dates of the annual meeting (typically two or three days) are determined by the host and the chair after consulting the Committee at the preceding meeting. The chair develops an agenda for the upcoming meeting in consultation with the executive committee and the feedback from the membership. The annual meeting includes technical reports from each represented station, discussion of results and future areas of collaboration, and meetings among smaller ad hoc committees. The location of the meeting for the coming year is determined by vote at the end of each meeting. Meetings are typically held at or near a member station and hosted by the official representative of that station. Minutes are prepared by the secretary and sent to members. An annual report is prepared by the chair, submitted to the administrative advisor, and posted at the NCDC-201 web site.
Literature Cited
Armagan, B.; Oezdemir, O.; Turan, M.; Celik, M. S. 2003. The removal of reactive azo dyes by natural and modified zeolites, Adsorption of anionic dye and surfactant from water onto organomontmorillonite, J. Chem. Tech. Biotech. 78, 725-735.
Arshady, R. 1990. Albumin microspheres and microcapsules: methodology of manufacturing techniques. J. Control. Release 14:111-131.
Bae, J-H; Song, D-I; Jeon, Y-W. 2000. Adsorption of anionic dye and surfactant from water onto organomontmorillonite, Separation Sci. Tech., 35, 353-365.
Baeumner AJ, Cohen RN, Miksic V and Min J. 2003. RNA Biosensor for the rapid detection of viable Escherichia coli in drinking water. Biosensors and Bioelectronics. 18:405-413.
Bahnemann, DW, Kormann C, and Hoffmann MR. 1987. Preparation and characterization of Quantum Size Zinc-Oxide - a Detailed Spectroscopic Study. Journal of Physical Chemistry 91:3789-3798.
Bennett WD. 2002. Rapid translocation of nanoparticles from the lung to the bloodstream? American journal of respiratory and critical care medicine 165(12):1671-1672.
Boddu, V.M.; Smith, E. D. 2002. US Patent Appl Publ., 11.
Borm, PJA. 2002. Particle toxicology: From coal mining to nanotechnology. Inhalation Toxicology 14: 311-324.
Borm, PJA, Kreyling, W. 2004. Toxicological hazards of inhaled nanoparticles - Potential implications for drug delivery. J. Nanosci. Nanotechnol. 4 : 521-531.
Cardamone, J. M.; Turner, J. D. 2000. Cationic applications for union dyeing wool/cotton blends, Text. Chem. & Col. and American. Dyestuff Reporter, 32(6), 49-53.
Chen, Y., et al. 1987. Comparison of albumin and casein microspheres as a carrier for doxorubicin. Journal of Pharmacy and Pharmacology. 39(12):978-985.
Chen, Z., et al. 2005. Acute toxicological effects of copper nanoparticles in vivo. Toxicological Letters xx:xxx (Article in Press)
Choo, KH, Kang, SK. 2003. Removal of residual organic matter from secondary effluent by iron oxides adsorption. Desalination, 154: 139-146.
Couvreur P, Dubernet C, Puisieux F. 1995. Controlled drug-delivery with nanoparticles - current possibilities and future-trends. European Journal of Pharmaceutics and Opharmaceutics 41: 2-13.
Cranor, C.F. 2003. How should society approach the real and potential risks posed by new technologies? Plant Physiology 133:3-9.
Cui Y, Wei Q, Park H and Lieber C. 2001. Nanowire Nanosensors for Highly Sensitive and Selective Detection of Biological and Chemical Species. Science 293, 1289-1293.
Currey JD. 1977. Mechanical properties of mother of pearl in tension. Proc R. Soc. London. B 196:443-463.
Dabbs, DM and Aksay, IA. 2000. Self-assembled ceramics produced by comples-fluid templation. Annual. Rev. Phys. Chem. 51:601-622.
Dreher, K.L. 2004. Health and environmental impact of nanotechnology: Toxicological assessment of manufactured nanoparticles. Toxicol. Sci. 77:3-5.
Dufresne, A., Cavaille, J-Y. and Helbert, W. 1996. New nanocomposite materials:
microcrystalline starch reinforced thermoplastic. Macromol. 29(23):7624-7626.
ETC Group. 2004. Down on the farm. The impact of nano-scale technologies on food and agriculture. November, 2004, ETC Group, Ottawa, Canada.
Espeleta, I., et al. 1997. Properties of glutaraldehyde cross-linked vicilin nano- and microparticles. J. Microencapsul. 14:557-565.
Fan, Q., Ugbolue, S. C., Wilson, A. R., Dar, Y. S.; Yang, Y. 2003. Nanoclay-modified polypropylene dyeable with acid and disperse dyes, AATCC Review, 3(6), 25-28.
Fan, Q.; Yang, Y.; Ugbolue, S. C.; Wilson, A. R. 2003. Methods of enhancing dyeability of polymers, US Patent 6,646,026.
Gogotsi, Y. 2003. How safe are nanotubes and other nanofilaments? Mater. Res. Innov. 7: 192-194.
Goodman, CM, McCusker, CD, Yilmaz, T, Rotello, VM. 2004. Toxicity of gold nanoparticles functionalized with cationic and anionic side chains. Bioconjugate Chemistry 15: 897-900.
Gradon L, Orlicki D, Podgorski A. 2000. Deposition and retention of ultrafine aerosol particles in the human respiratory system. Normal and pathological cases. JOSE 6189-207.
Gupta, P.K. and C.T. Hung. 1989. Albumin microspheres 1: Physicochemical characteristics. J. Microencap. 6(4):427-462.
Gupta, P.K. and C.T. Hung. 1989. Albumin microspheres .2. Applications in drug delivery. J. Microencapsul. 6(4):463-472.
Hartley H A and Baeumner AJ. 2003. Biosensor for the specific detection of a specific detection of a single viable B. anthracis spore. Anal. Bioanal. Chem 376, 319-327.
Harper Jr., R. J.; Stone, R. L. 1986. Cationic cotton plus easy care, Textile Chemist and Colorist, Text. Chem. & Col., 18(11), 33-35.
Hauser, P. J., Draper, S. L., Beck, K. R., Smith, C. B. 2003. Characterization of the dyeing behavior of cationic cotton with acid dyes, AATCC Review, 3(8), 51-53.
Hay, J.N.; Shaw, S.J. Available at http://www.azom.com/details.asp?article ID=936 2000 (Accessed September 30, 2004).
Heelan, B.A. and O.I. Corrigan. 1997. In vitro analysis of the release of incorporated agents from sodium caseinate microspheres. Journal of Microencapsulation. 14(1):63-78.
Hernandez-Santos, D, Gonzalez-Garcia, MB, Garcia, AC. 2002. Metal-nanoparticles based electroanalysis. Electroanalysis 14: 1225-1235.
Heuer AH, Fink DJ, Laraia VJ, Arias JL, Calvert PD, Kendall K, Messing GL, Blackwell J, Rieke PC, Thompson DH, Wheeler AP, Veis A and Caplan AI. 1992. Innovative materials processing startegies: A biomimetic approach. Science. 255: 1098-1105.
Hong, SS, Lee, MS, Hwang, HS, Lim, KT, Park, SS, Ju, CS, Lee, GD. 2003. Preparation of titanium dioxides in the W/C microemulsions and their photocatalytic activity. Solar Energy Materials And Solar Cells 80: 273-282.
Huggett DB, Deaver E and Rodgers JH. 1999. Copper Bioavailability in Steilacoom Lake Sediments. Archives of Environmental Contamination and Toxicology 36: 120-123.
Illy-Cherrey, S., Tillement, O, Dubois, JM, Massicot, F, Fort, Y, Ghanbaja, J, Begin-Colin, S. 2002. Synthesis and characterization of nano-sized nickel(II), copper(I) and zinc(II) oxide nanoparticles. Mater. Sci. Eng. A 338:70-74.
Irache, J.M., et al. 1995. Optimization and in vitro stability of legumin nanoparticles obtained by a coacervation method. International Journal of Pharmaceutics. 126(1-2):103-109.
Ivnitski D, Abdel-Hamid I, Atanasov P, and Wilkins E. 1999a. Biosensors for detection of pathogenic bacteria. Biosensors & Bioelectronics, 14, 599-624.
Ivnitski D, Abdel-Hamid I, Atanasov P, Wilkins E and Stricker S. 1999b. Application of electrochemical biosensors for detection of food pathogenic bacteria. Electroanalysis, 12(5), 317-325.
Jani, P, Halbert, GW, Langridge, J, Florence, AT. 1990. Nanoparticle uptake by the rat gastrointestinal mucosa: Quantitation and particle size dependency. J. Pharm. Pharmacol. 42: 821-826.
Jaykus L. 2003. Challenges to developing real-time methods to detect pathogens in food. ASM News, 7: 341-347.
Jones, C., M.A. Burton, and B.N. Gray. 1989. Albumin microspheres as vehicles for the sustained and controlled release of doxorubicin. J. Pharm. Pharmacol. 41:813-816.
Jortner J and Rao CNR. 2002. Nanostructured Advanced Materials. Perspectives and Directions. Pure Appl Chem 74(9), 1491-1506.
Juang, R.S.; Shao, H.J. 2002. Water Res., 36, 2999.
Kijek TM, Rossi CA, Moss D, Parker RW, Henchal EA. 2000. Rapid and sensitive immunomagnetic-electrochemiluminescent detection of staphylococcal enterotoxin. B. J. Immunol. Methods, 236, 9-17.
Kim, J-T., Lee, D-Y., Oh, T-S. and Lee, D-H. 2003. Characteristics of nitrile-butadiene rubber layered silicate nanocomposites with silane coupling agent. J. Appl. Polym. Sci. 89(10):2633-2640.
Kindschy, L. and Alocilja, E.C. 2005. A Molecularly Imprinted Polymer on Indium Tin Oxide and Silicon. Biosensors and Bioelectronics, 20(10):2163-2167.
Kindschy, L. and Alocilja, E.C. 2006. Development of a molecularly imprinted biomimetic sensor. Biotechnology Progress (in review)
Kiryu, I, Ototake, M, Nakanishi, T, Wakabayashi, H. 2000. The uptake of fluorescent microspheres into the skin, fins and gills of rainbow trout during immersion. Fish Pathology, 35: 41-48.
Kleiner, K, Hogan, J. 2003. How safe is nanotech? New Sci. 177: 14-20.
Klingels, M.; Griesbach, U.; Panzer, C.; Wachter, R. 1999. Henkel -Referate, 35, 78.
Knepp, W.A., et al. 1993. Synthesis, Properties, and Intratumoral Evaluation of Mitoxantrone-Loaded Casein Microspheres in Lewis Lung-Carcinoma. Journal of Pharmacy and Pharmacology. 45(10):887-891.
Kracke B. and Damaschke B. 2000. Measurement of nanohardness and nanoelasticity of thin gold films with scanning force microscopy. App. Phys. Lett. 77:361-363.
Kumar, M.V., S.B. Jayaswal, and V.K. Gupta. 1994. Preparation and in vitro evaluation of streptomycin-loaded albumin microspheres for lung targeting. Pharmazie. 49:691-692.
Kumar, S., Jog, J.P., and Natarajan, U. 2003. Preparation and characterization of poly(methyl methacrylate)-clay nanocomposites via melt intercalation: the effect of organoclay on the structure and thermal properties. J. Appl. Polym. Sci. 89: 1186-1194.
Lam C-W, James JT, McCluskey R, Hunter RL. 2004. Pulmonary toxicity of single-wall carbon nanotubes in mice 7 and 90 days after intratracheal instillation. Toxicol. Sci. 77: 126-134.
Latha, M.S., et al. 1994. Casein as a carrier matrix for 5-fluorouracil - drug-release from microspheres, drug-protein conjugates and in-vivo degradation of microspheres in rat muscle. Journal of Pharmacy and Pharmacology. 46(11):858-862.
Latha, M.S. and A. Jayakrishnan. 1994. Glutaraldehyde cross-linked bovine casein microspheres as a matrix for the controlled-release of theophylline - in-vitro studies. Journal of Pharmacy and Pharmacology. 46(1):8-13.
Latha, M.S., et al. 1995. Bioavailability of theophylline from glutaraldehyde cross-linked casein microspheres in rabbits following oral-administration. Journal of Controlled Release. 34(1):1-7.
Lee, D. S., and T. K. Liu. 2002. Preparation of TiO2 sol using TiCl4 as a precursor. Journal of Sol-Gel Science and Technology 25:121-136.
Levy, M.C. and M.C. Andry. 1990. An evaluation of gelatin microcapsules prepared using interfacial cross-linking process. Life Sci. Adv. 19:219-227.
Lieber CM 2001. The Incredible Shrinking Circuit. Scientific American, 285, No. 3, 58-64.
Lin, W., et al. 1993. Preparation of Sub-100nm human serum albumin nanospheres using a pH-coacervation method. J. Drug Target. 1:237-243.
Liu, L.P., Y.B. Xiao, Z.W. Xiao, Z.B. Wang, C. Li, and X. Gong. 2005. Toxicity of hydroxyapatite nanoparticles on rabbits. Wei Sheng Yan Jiu. 34:474-476.
Longo, W.E., et al., 1982. Preparation of hydrophilic albumin microspheres using polymeric dispersing agents. Journal of Pharmaceutical Sciences. 71(12):1323-1328.
Lovern S, Klaper R, Stricker J. 2004. Exposure of Daphnia to a variety of nanoparticles. SETAC Book of Abstracts, Fourth World Congress, Portland, OR. Society of Environmental Toxicology and Chemistry, Pensacola, FL.
Lowenstam HA and Weiner S. 1989. On Biomineralization. Oxford University Press. Oxford UK.
Mahato, RI, Narang, AS, Thoma, L, Miller, DD. 2003. Emerging trends in oral delivery of peptide and protein drugs. Critical Reviews in Therapeutic Drug Carrier Systems 20: 153-214.
Mann S. 2001. Biomineralization: Principles and Concepts in Biorganic Materials Chemistry. Oxford University Press. Oxford, UK.
Mann S. 2000. The chemistry of form. Agnew. Chem. Int. Ed. 39:3392-3406.
Mao, J.S.; Zhao, L.G.; Yao, K.D.; Shang, Q, X.; Yang, G.H.; Cao, Y.L. 2003. J Biom Mater Res., 64 A(2), 301.
Mathew, F. and Alocilja, E.C. 2005. Porous Silicon-Based Biosensor for Pathogen Detection. Biosensors and Bioelectronics Journal, 20(8):1656-1661.
Mathew, A.P. and Dufresne, A. 2002. Morphological investigation of nanocomposites from sorbitol plasticized starch and tunicin whiskers. Biomacromol. 3(3):609-617.
Matsumura, M.; Umeda, A.; Matsubara, N.; Ogawa, T.; Kamata, K. 1991. Manufacture of acid-modified polyesters dyeable by basic dyes, Jpn. Patent 03227318, Kokai Tokkyo Koho.
McGlashan, S.A., Halley, P. 2003. Preparation and characterization of biodegradable starch based nanocomposite materials. Polym.Int. 52:1767-1773.
Miyamoto, N.; Kawai, R.; Kuroda, K.; Ogawa, M. 2000. Adsorption and aggregation of a cationic cyanine dye on layered clay minerals, Appl. Clay Sci., 16, 161-170.
Moore, MN. 2002. Biocomplexity: the post-genome challenge in Ecotoxicology. Aquat. Toxicol. 59: 1-15.
Muhammad-Tahir, Z. and Alocilja, E.C. 2003. Fabrication of a disposable biosensor for Escherichia coli O157:H7 detection. IEEE Sensors Journal, 3(4):345-351.
Muhammad-Tahir, Z. and Alocilja, E.C. 2004. A Disposable Biosensor for Pathogen Detection in Fresh Produce Samples. Biosystems Engineering Journal, 88(2):145-151.
Muhammad-Tahir, Z. Alocilja, E.C., and Grooms, D. 2005. Polyaniline synthesis and its application in biosensor. Biosensors and Bioelectronics Journal, 20(8):1690-1695.
Mulvaney P, Cooper R, Grieser F and Meisel D. 1988. Charge Trapping in the Reductive Dissolution of Colloidal Suspensions of Iron(III) Oxides. Langmuir 4:1206-1211
Naimushin A N, Soelberg SD, Nguyen DK, Dunlap L, Bartholomew D, Elkind J, Melendez J, Furlong CE. 2002. Detection of Staphylococcus aureus entertoxin B at femtomolar level with a miniature integrated two-channel surface plasmon (SPR) sensor. Biosensors and Bioelectronics, 17, 573-584.
National Nanotechnology Infrastructure Network. 2005. Nanotechnology careers. http://www.nnin.org/nnin_careers.html Accessed Nov. 22, 2005.
Niyogi S, Wood CM. 2003. Effects of chronic waterborne and dietary metal exposures on gill metal-binding: Implications for the Biotic Ligand Model. Hum Ecol Risk Assess 9: 813-846.
Oberdorster, E. 2004. Manufactured nanomaterials (Fullerenes, C-60) induce oxidative stress in the brain of juvenile largemouth bass. Environ. Health Perspect. 112: 1058-1062.
Oner, L. and M.J. Groves. 1993. Optimization of conditions for preparing 2-micron-range to 5-micron-range gelatin microparticles by using chilled dehydration agents. Pharmaceutical Research. 10(4):621-626.
Oya, A. and Kurokawa, Y. 2000. Factors controlling mechanical properties of clay
mineral/polypropylene nanocomposites. J. Mater. Sci. 35:1045-1050.
Park, H-M., Lee, W-K., Park, C-Y., Cho, W-J., and Ha, C-S. 2003. Environmentally friendly polymer hybrids: part I mechanical, thermal, and barrier properties of the thermoplastic starch/clay nanocomposites. J. Mater. Sci. 38: 909-915.
Park, H.Y., et al. 1998. Effect of pH on drug release from polysaccharide tablets. Drug Delivery. 5(1):13-18.
Qu LW, Luo PG, Taylor S, Lin Y, Huang WJ, Anyadike N, Tzeng TRJ, Stutzenberger F, Latour RA, Sun YP. 2005. Visualizing adhesion-induced agglutination of Escherichia coli with mannosylated nanoparticles. Journal of Nanoscience and Nanotechnology 5:319-322.
Radke, S. and Alocilja, E.C. 2004. Design and Fabrication of a Micro-Impedance Biosensor for Bacterial Detection. IEEE Sensors Journal, 4(4):434-440.
Radke, S. and Alocilja, E.C. 2005a. A Microfabricated Biosensor for Detecting Foodborne Bioterrorism Agents. IEEE Sensors Journal, 5(4): 744-750.
Radke, S. and Alocilja, E.C. 2005b. A High Density Microelectrode Array Biosensor for Detection of E. coli O157:H7. Biosensors and Bioelectronics Journal, 20(8):1662-166
Rhee SH, Suetsugu Y and Tanaka J. 2001. Biomimetic configurational arrays of hydroxyapatite nanocrystals on bio-inorganics. Biomaterials. 22:2843-2847.
Rho JY, Ashman, RB. And Turner, CH. 1993. Youngs modulus of trabecular and cortical bone material: Ultrasonic and microtensile measurements. J. Biomech. 26: 111-119.
Rodriguez-Lopez, M. and Alocilja, E.C. 2005. Embedded DNA-polypyrrole biosensor for rapid detection of Escherichia coli. IEEE Sensors Journal, 5(4):733-736.
Risbud, M.; Hardikar, A.; Bhonde, R. 2000. J. Biosci., 25(1), 25.
Ross, S.A., P.R. Srinivas, A.J. Crawford, S.C. Lee, M.A. Philbert and R.L. Hettich. 2004. New technologies for nutrition research. J. Nutr. 134: 681-685.
Rossler, B., J. Kreuter, and D. Scherer. 1995. Collagen microparticles - preparation and properties. J. Microencapsul. 12(1):49-57.
Sahin, S., et al. 2002. Preparation, characterization and in vivo distribution of terbutaline sulfate loaded albumin microspheres. J. Control. Release. 82:345-358.
Salamanca-Buentello, F., D. L. Persad, E. B. Court, D.K. Martin, A.S. Daar, and P.A. Singer. 2005. Nanotechnology and the developing world. PLoS Med 2(5):e97
Saleh, A.M., et al. 1989. Effect of some drugs and additives on the cross-linking of bovine serum-albumin by glutaraldehyde. International Journal of Pharmaceutics. 57(3):205-210.
Sarikaya M. 1994. An introduction to biomimetics A structural viewpoint. Microsc. Res. Technique. 27:360-375.
Sasamoto, F.; Oka, K.; Mineo, M. 1986. Manufacture of polyester fibers dyeable with basic dyes , Jpn. Patent 61225314, Kokai Tokkyo Koho.
Scott, N., H. Chen, and C. Johnson. 2002. Nanoscale science and engineering for agriculture and food systems. National planning workshop, November 18-19, Washington D.C. Website: www.nseafs.cornell.edu (Accessed on September 7, 2005).
Scott, N. 2005. Nanotechnology and animal health. Rev.sci.tech.Off.int.Epiz. 24(1):425-432.
Schoning MJ and Poghossian A. 2002. Recent advances in biologically sensitive field-effect transistors (BioFETS). Analyst, 127, 1137-1151.
Schrick B, Hydutsky BW, Blough JL, Mallouk TE. 2004. Delivery vehicles for zerovalent metal nanoparticles in soil and groundwater. Chemistry of Materials 16: 2187-2193.
Serizawa T, Yamaguchi M and Akashi M. 2002. Enzymatic hydrolysis of layer-by-layer assembly prepared from chitosan and dextran sulphate. Macromolecules. 35:8656-8658.
Service, RF. 2003. Nanomaterials show signs of toxicity. Science 300(5617):24.
Suedel BC, Deaver E and Rodgers JH. 1996. Experimental Factors that May Affect Toxicity of Aqueous and Sediment-Based Copper to Freshwater Organisms. Archives of Environmental Contamination and Toxicology 30: 40-46.
Tomalia DA, Wang ZG and Tirrell MV. 1999. Experimental self-assembly: the many facets of self-assembly. Curr Opin Colloid & Interface Sci. 4: 3-5.
Uyama, H., Kuwabara M., Tsujimoto, T., Nakano, M, Usuki, A. and Kobayashi, S. 2003. Green nanocomposite from renewable resources: plant oil-clay hybrid materials. Chem. Mater. 15:2492-2494.
Vandamme, T.F., et al. 2002. The use of polysaccharides to target drugs to the colon. Carbohydrate Polymers. 48(3):219-231.
Vinod, V. P.; Anirudhan, T. S. 2003. Adsorption Behavior of Basic Dyes on the Humic Acid Immobilized Pillared Clay, Water, Air, and Soil Pollution, 150, 193-217.
Viano A., Mishra S.R., Lloyd R., Losby J., and Gheyi T. 2003. Thermal Effects on ESR Signal Evolution in Nano and Bulk CuO Powder. Journal of Non-Crystalline Solids 325: 16-21.
Vo-Dinh, T. 2005. The new paradigm shift at the convergence of nanotechnology, molecular biology and biomedical sciences. NanoBiotechnology 1:1-3.
Waite JH, Qin XX and Coyne KJ. 1998. The peculiar collagens of mussel byssus. Matrix Biology. 17:93-106.
Warheit, DB, Laurence, BR, Reed, KL, Roach, DH, Reynolds, GAM, Webb, TR. 2004. Comparative pulmonary toxicity assessment of single-wall carbon nanotubes in rats Toxicol. Sci. 77: 117-125.
Weiner S. and Addadi L. 1997. Design strategies in mineralized biological materials. J. Mater. Chem. 7: 689-702.
Willmott, N., et al. 1992. Doxorubicin-loaded casein microspheres - protean nature of drug incorporation. Journal of Pharmacy and Pharmacology. 44(6):472-475.
Wu, J.J.; Zhu, T.Y.; Hu, L.; He. P.; Li, W. W. 2002. J China Burn, 18(1), 19.
Yermiyahu, Z.; Lapides, I.; Yariv, S. 2003. Visible absorption spectroscopy study of the adsorption of Congo Red by montmorillonite, Clay Minerals, 38, 483-500.
Yamaguchi, I.; Tokuchi, K.; Fukuzaki, H.; Koyama, Y.; Takafuda, K.; Monma, H.; Tanaka, J. 2001. J Biomed Mater Res., 55, 20.
Zaharescu, M, Crisan, M, Szatvanyi, A, Gartner, M. 2000. TiO2-based nanostructured sol-gel coatings for water treatment J. Optoelectron. Advanced Mat. 2: 618-622
Zang, W.X. 2003. Nanoscale iron particles for environmental remediation: An overview. J. Nanoparticle Res., 5: 323-332