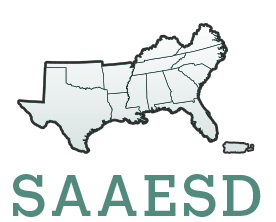
S1033: Control of Food-Borne Pathogens in Pre- and Post-Harvest Environments
(Multistate Research Project)
Status: Inactive/Terminating
S1033: Control of Food-Borne Pathogens in Pre- and Post-Harvest Environments
Duration: 10/01/2007 to 09/30/2012
Administrative Advisor(s):
NIFA Reps:
Non-Technical Summary
Statement of Issues and Justification
The Centers for Disease Control and Prevention (CDC, 1999) reported new, more accurate estimates of foodborne illnesses that occur annually. An estimated 76 million cases of foodborne illness, 325,000 hospitalizations, and 5,000 deaths occur each year from food-borne microorganisms (Mead et al., 1999). The food safety surveillance system, FoodNet, indicates that more cases of food-borne illness occurred, but fewer deaths were caused by foodborne disease agents than previously reported. Campylobacter spp. was responsible for the most cases of foodborne illness. Salmonella (nontyphoidal) caused the most deaths; Listeria monocytogenes also causing a significant number of deaths. In summary, the report indicates that foodborne pathogens have a significant impact on human health and the food industry in the United States. In addition to human suffering, foodborne illnesses also have a substantial economic impact in the United States. The annual cost of foodborne illness in the U.S. is estimated at $5-$6 billion for loss of productivity and medical expenses (Marks and Roberts, 1993). The most costly food-borne illnesses are caused by Toxoplasma gondii, Salmonella spp., Campylobacter spp., and enterohemorrhagic Escherichia coli. New methods to prevent, reduce or eliminate foodborne disease agents at all points of the food chain, from farm to fork, are needed to improve the safety of the food supply to prevent illnesses and deaths and to prevent economic losses to the food industry. Produce: An increasing number of gastrointestinal disease outbreaks have been linked to the consumption of fresh fruits and vegetables. The increase in the number of outbreaks caused by produce appears to be related to the increased demand for fresh fruits and vegetables. Between 1990 and 2001, contaminated fresh produce caused 148 outbreaks that account for approximately 9% of all food-borne outbreaks (Smith-DeWaal et al, 2002). Two of the most virulent foodborne pathogens, Salmonella and pathogenic Escherichia coli, have been responsible for many outbreaks associated with fresh fruits and vegetables. Fresh fruits and vegetables are considered high-risk foods because they are minimally processed and are susceptible to contamination by manure or soil at the farm. The FDA in collaboration with the USDA and the Centers for Disease Control CDC issued a series of guidelines referred as Good Agricultural Practices (GAPs) to reduce the risk of foodborne diseases from fresh fruits and vegetables (FDA/USDA/CDC, 2003). Even with implementation and strict adherence to the GAPs guidelines, a potential still exists for contamination of fresh fruits, vegetables and nutmeats. To further reduce or prevent the potential for finished produce and nutmeat contamination, decontamination methods must be employed. Traditional decontamination treatments (thermal processing, chlorination and irradiation) adversely affect the desirable characteristics of the majority of fresh fruits, vegetables and nutmeats. Therefore, alternative methods or different parameters for existing methods must be developed for the decontamination of produce and nutmeats. Meat and Poultry: Foodborne salmonellosis in the U.S. causes approximately 1.3 million illnesses, 15,600 hospitalizations and 550 deaths annually and is most often associated with meat and egg products. Undercooked and/or improperly chilled meat and eggs have been identified as one of the most frequent causes of foodborne illness in the United States. Heating and chilling of meat, eggs and meat products are critical processes for controlling growth of foodborne pathogens. Chilling of meat animal carcasses, fresh eggs and freshly-cooked, ready-to-eat meat products using air chilling is a complex process that is widely used in industry. Specification and evaluation of rate of temperature decline are essential in controlling the microbiological hazards such as Escherichia coli O157:H7, Clostridium perfringens and Salmonella spp. to insure food safety. Requirements for processing of meat and poultry products are published in Title 9 of the Code of Federal Regulations and include performance standards to control pathogens. The lethality performance standard is based on destruction of the pathogenic microorganism Salmonella (USDA-FSIS, 2002). The lethality standard states that manufacturers must use a combination of thermal and non-thermal processes sufficient to achieve a 6.5-log10 reduction in Salmonella in ready-to-eat, cooked beef, roasted beef, cooked corned beef (9 CFR 318.17) and a 7-log10 reduction in ready-to-eat poultry products (9 CFR 381.150). Alternatively, processors may follow USDA compliance or safe harbor guidelines. Safe harbor guidelines listing specific processing times and temperatures for beef patties are in effect (9 CFR 318.23). If a processor elects to use their own process, they must provide evidence that the process meets the lethality performance standard. More research is needed to understand and predict microbial lethality during thermal processing. The stabilization performance standard is designed to prevent growth of pathogens in cooked product during chilling and cold storage. The stabilization performance standards require no multiplication of toxigenic microorganisms, such as Clostridium botulinum, and no more than a 1 log10 multiplication of C. perfringens within the product after cooking for poultry (9 CFR 381.150), roast beef (9 CFR 318.17) and beef patties (9 CFR 318.23). Specification and evaluation of rate of temperature decline within a product are essential in controlling microbiological hazards due to E. coli O157:H7, C. perfringens and Salmonella spp. to insure food safety. Bovine pre-harvest food safety: Over the past decade, Escherichia coli O157:H7 has emerged as a significant public health concern. Infection with this pathogen in humans is associated with a range of clinical syndromes, including mild to severe bloody diarrhea and hemolytic uremic syndrome. E. coli O157:H7 is considered a transient member of the normal flora of cattle and is only rarely associated with clinical disease in cattle. However, cattle are important sources of E. coli O157:H7, with beef and beef products being implicated in many food borne disease outbreaks of E. coli O157:H7 in humans. E. coli O157:H7-contaminated meat linked to human outbreaks of disease can have devastating effects on the industry because of product recalls and reduced consumer confidence. Thus, from both a humanitarian and an economic perspective, E. coli O157:H7 removal from the food supply is both a state and a national priority. Control of E. coli O157:H7, to be effective, will require the implementation of intervention strategies throughout the food chain continuum, from farm to table. Sanitation efforts after slaughter have been demonstrated to reduce contamination of carcasses with E. coli O157:H7. However, pre-harvest intervention strategies may reduce E. coli O157:H7 levels prior to entry in the food processing chain. At the farm level, intervention measures that have been investigated or are currently under investigation include competitive exclusion, general sanitation strategies, dietary component modifications, and pre-slaughter feeding strategies. An area of on-farm E. coli O157:H7 control that has not been investigated is the role of an immunosuppressive pathogen such as bovine viral diarrhea virus (BVDV) on fecal E. coli O157:H7 shedding. BVDV is widely distributed among cattle, and identifying a potential on-farm risk factor for E. coli O157:H7 will assist the beef and dairy industry by identifying critical control strategies to minimize the threat of human food borne illnesses associated with E. coli O157:H7, as well as ensuring consumer confidence through the provision of wholesome food. Seafood: A major food industry concern is the ability of Listeria monocytogenes to grow at refrigerator temperatures, resist various environmental conditions and thus survive longer under adverse conditions. L. monocytogenes has been found in the environments of crawfish and smoked fish processing plants. These environments include drains, floors, condensate lines, crates, door handles, and conveyor belts (Destro et al., 1996; Thimothe et al., 2002) and can serve as a source for L. monocytogenes contamination of raw seafood and smoked fish and post-processed seafood. Rinse treatments and other measures that reduce L. monocytogenes on smoked fish and seafood would be valuable. The CDC estimates that noroviruses alone cause 9.2 million cases of acute foodborne gastroenteritis a year, and at least 50% of all foodborne gastroenteritis outbreaks can be attributed to noroviruses (Shabin, 2004; CDC, 2003; Schaub and Oshiro. 2000). Generally, foods that are minimally processed or encounter a high degree of handling are susceptible to virus contamination. With a concurrent increase in the use and consumer interest in innovative nonthermal process technologies (such as high hydrostatic pressure processing or HPP) to improve the sensory quality of foods, there is a need to understand and predict the sensitivity of infective viruses in foods to these newly developed technologies to ensure product safety (Calci et al., 2005; Hoover et al., 1989. Antimicrobial drug resistance: Antibiotics and other growth-promoting antimicrobials have been used as feed additives for over 50 years. The economic benefits to the animal producer include improved growth rate and feed conversion, reduced mortality, improved health, and greater resistance to disease challenge (Jukes, 1972; Guest, 1976; Hays, 1978). A recent review of the literature indicated that in 12,153 feeding studies, dietary antimicrobial growth promotants successfully increased growth performance 72% of the time (Rosen, 1996). Today, more than 50% of all antibiotics produced are used in animal feeds. Despite the success of this practice, the inclusion of antibiotics in animal feeds to enhance growth is banned in many countries. The use of antibiotics for growth promotion requires long-term subtherapeutic addition of the antibiotic in the feed. Unfortunately, these practices may increase the prevalence of antibiotic-resistant bacteria in the animal (Fuller et. al. 1960; Langlois et. al., 1978 a, b; Morehead and Dawson, 1992). Some have proposed that this increase in antibiotic-resistant bacteria in the animal may result in an increase in antibiotic-resistant bacteria in the food supply, which could ultimately result in human illnesses (Guest, 1976; Novick, 1981). Antibiotic-resistant bacteria pose a significant human health risk if they are pathogens, or if they transfer the resistance to pathogens (Corpet, 1988). Many consumer groups and researchers in the United States support the elimination of subtherapeutic doses of antibiotics in animal feeds in an attempt to reduce or eliminate antibiotic resistant bacterial populations in livestock.
Related, Current and Previous Work
The participants of this project have a very productive history of collaboration and successful research. For the most part these individuals and their colleagues at their 19 respective Universities also participated in S295 Enhancing Food Safety through Control of Foodborne Disease Agents. The collaborators of this multi state project, which was active from October 2000 to September 30, 2006 was responsible for more than 110 peer reviewed publications in the worlds leading food safety journals. In addition, these researchers had over 160 oral presentations at scientific meetings and sponsored almost 100 graduate students. The results of this research also provided the opportunity for the investigators to average over 3 million dollars a year in outside funding during the duration of the S295 project. One of the most underrated benefits of participating in a project with so much collaboration is the opportunity to work with the finest researchers across the country. As a result, many analytical goals where achieved that may not have been practical or possible working alone. Often these relationships include obtaining samples or microbial cultures, performing analytical services, and the sharing of technical guidance and advice just to name a few. This collaboration is not always obvious through reports and publications but that is what helped to make S295 so successful. The objectives of the new project SDC-313 are the natural extension of the work completed on S295 in addition to addressing the current food safety concerns in our nation today. Since pathogenic organisms are continuing to rapidly, evolve and survive in both the pre- and post harvest environments and foodborne outbreaks are occurring in previously thought to be safe products, research in the control of these organisms is essential. The members of the SDC-313 project will collaborate on a variety of foods including meat, poultry, seafood, fruits, vegetables and nutmeats. In addition to treatment methods, a better understanding of pathogen survival using mathematic models in foods will provide insight into better processing methods and food treatments. Finally, the investigators of SDC-313 will work to better understand and eliminate the transfer of antimicrobial resistance from the farm to the foods. Included in this work will be identifying the factors associated with persistence in the animal production environment and the use of practical interventions steps to reduce the risk to the consumer. Related Multi-state Projects: A CRIS search was conducted and the only other regional projects related to SDC313 were S1027 and NC1007. The multi-state project S1027 is investigating how to improve the quality of eggs and poultry products using cryogenic cooling while our project will focus on the development of mathematical models to describe the chilling and heating rates needed to control foodborne pathogens in eggs and meat products. Multi-state project NC1007 mainly focuses on the isolation of bovine caliciviruses, bovine torovirus and bovine coronavirs from cattle fecal and nasal samples and determine their ability to cross protect against one other in cattle while our project will look at the effect of cattle infected with bovine viral diarrhea virus on the survival and levels of E. coli O157:H7 in cattle. Fruits and Vegetables: Foodborne illness due to fresh fruits and vegetables continues to result in multi-state outbreaks (CDC, 2002, 2002b, 2003, 2004; Harris et al., 2001; Kretsinger et al., 2003; Sivapalasingam et al., 2003). Extensive research has been performed in an attempt to provide effective decontamination methods for fruits and vegetables (Beuchat, 1998; Jaquette et al., 1996; Zhang and Farber, 1996; Sapers et al., 2000, 2001; Annous et al., 2001). However, due to the inherent nature of produce, traditional decontamination methods such as chlorination have shown limited success with only 1 to 2 log reduction being achieved. (Sapers et al., 2000) Traditional processing methods, such as thermal processing, negatively impact the organoleptic qualities of most fresh fruits and vegetables but has been shown to be an effective surface decontamination treatment for oranges, apples and sprout seeds (Pao and Davis, 1999; Hu et al., 2004; Gorny, 2005). Alternative decontamination methods such as High Pressure Processing (HPP), gamma irradiation, electron beam have been investigated for some produce items with varying levels of success based on the type of fresh produce tested (Grove et al., 2006; Kingsley et al., 2002; Mintier and Foley, 2006). In addition, a plethora of alternative sanitizers such as peracetic acid, hydrogen peroxide, and ozone have been evaluated for their effectiveness to decontaminate produce (Beuchat, 1998; Jaquette et al., 1996; Sapers et al., 2000; Sapers et al., 2001; Das et al., 2006). Sanitizers are primarily used as a surface decontamination treatment and researchers have found that pathogens associated with produce can be internalized in stomata of plant cells that act as a protective barrier against surface decontamination treatments (Solomon and Matthews, 2002; Ibarra-Sanchez et al., 2004). Despite a broad range of treatment methods and conditions, limited effectiveness has been achieved against bacterial, parasites and viral pathogens on fresh and fresh cut produce. Future produce safety research will likely be directed towards optimizing existing decontamination methods as well as developing and evaluating new treatments such as germicidal gases, bioprotective cultures, and preprocessing decontamination methods. The primary goal of this research is to identify effective treatments that the fresh fruit and vegetable industry can implement to enhance the safety for all consumers. Meat and Poultry: An integrated model of heat transfer and dynamic growth of Clostridium perfringens in cured ham was developed and validated for three cooling scenarios at the University of Nebraska. Heat transfer was modeled as a two-dimensional axisymmetric heat conduction problem and the model was solved using finite element analysis. The dynamic growth model of Baranyi was used to predict C. perfringens growth under time-varying temperature conditions, and it was solved using a fourth-order Runge-Kutta method. Both numerical schemes were combined and implemented in a computer program. The program was used to assess C. perfringens growth during a cooling process in compliance with the FSIS stabilization performance standards for cured products, as well as two other processes where deviations from the cooling guidelines recommended by these standards occurred. Results showed good agreement between predicted and experimental data, with a maximum deviation of 0.72 log10 CFU/g that occurred for only one validation test of a "worst-case" cooling scenario. For the remaining validation tests, all deviations were within 0.37 log10 CFU/g. The model slightly overestimated observed growth, resulting in prediction bias towards the "fail-safe" zone. Mathematical models that take into account the potential for non-linear survival curves were evaluated at the University of Idaho. The Weibull distribution was used to predict and compare the inactivation of Salmonella spp., Escherichia coli O157:H7 and Listeria monocytogenes in ground beef, turkey and pork under nonisothermal cooking conditions. Isothermal survival curves for each pathogen were described by the Weibull distribution, Log S (t) = -b (T) tn(T), and its simplified linear form (n=1), log S=-bt. In ground beef, the isothermal experimental data for all pathogens exhibited linear trends with slight non-linearity at lower temperatures. Non-linearity was observed in experimental isothermal survival curves of Salmonella spp. in the turkey and pork. The nonisothermal survival curves were obtained by solving the differential form of the Weibull equation with a program written in Mathematica. With a few exceptions, there was good agreement between experimental and predicted non-isothermal survival curves for all three pathogens. Mathematical models that take into account the potential for non-linear survival curves may more accurately predict microbial inactivation and prevent undercooking. Seafood: Listeria monocytogenes is currently one of the major pathogens of concern for the seafood industry (Farber et al., 1991; Thimothe et al., 2002). Seafood products that have contributed to human listeriosis outbreaks include cold-smoked trout, shrimp, and smoked mussels (Brett et al., 1998; Ericsson et al., 1997; Miettinen et al., 1999; Tham et al., 2000;). Other seafood products contaminated with L. monocytogenes include cold-smoked salmon and crawfish (Dalgaard et al., 1998; Thimothe et al., 2002). A major concern of the smoked fish processing industry is the contamination of these products with L. monocytogenes and Salmonella species. The source of contamination of these foodborne pathogens on the surface of smoked fish products after processing has been attributed to the raw products and the processing environment. Contamination of smoked fish products with L. monocytogenes ranges from 17.9% to 22.3% with the highest incidence occurring during the cold-smoked process rather then the hot-smoked process (Hoffmon et al., 2003). The incidence of Salmonella species on smoke fish products ranges from 3.2%. A possible solution for controlling these foodborne pathogens on smoked fish and seafood is edible films containing antimicrobial agents such as nisin, lysozyme and other antimicrobial agents. In addition, chemical sanitizers such as cetylpyridium chloride, acidified sodium chloride could be used to control or eliminate L. monocytogenes and Salmonella species on smoked fish and seafood. The bioaccumulation of harmful agents in oysters poses a significant health risk since these shellfish are sometimes consumed raw or lightly cooked. Several recent outbreaks associated with hepatitis A virus, Vibrio parahaemolyticus, and Vibrio vulnificus have heightened the concern with the safety of shellfish. High pressure processing is currently used commercially on the US Gulf and West Coasts for oyster shucking. Studies have shown that the processing parameters used in this process do not achieve target levels for hepatitis A virus and V. parahaemolyticus inactivation Data obtained by the principle investigator and other researchers have demonstrated that temperature significantly affect pressure inactivation of viruses and bacteria. A clear need is to develop processing techniques (combinations of pressures and optimum temperatures) for oyster processing to inactivate oyster-associated pathogens while maintaining maximum sensory qualities. Bovine Pre-Harvest Food Safety: Enterohemorrhagic Escherichia coli serotype O157:H7 has emerged as a significant public health concern, causing illness and death in humans. Human infections have been reported with increasing frequency over the past 15 years in many countries including the United States, with illness including severe enterocolitis and systemic sequelae, such as hemolytic uremic syndrome (Slutsker et al, 1997; Paton and Paton, 1998). Estimates by the Centers for Disease Control and Prevention indicate that E. coli O157:H7 is responsible for approximately 62,500 cases of food borne infection annually in the United States (Mead et al., 1999). Numerous disease outbreaks have implicated cattle or bovine products as an important source of E. coli O157:H7. As a result of its zoonotic potential, E. coli O157:H7 contamination of beef and dairy products has resulted in costly recalls of product, erosion in consumer confidence in the safety of beef and dairy products, and thus, a reduction in consumer demand for beef and dairy products. Farm animals are widely regarded to be the principal reservoir of E. coli O157:H7. Studies utilizing sensitive detection methods report rates of fecal E. coli O157:H7 shedding from cattle to be as high as 28% (Dargatz, Wells, and Thomas, 1997; Elder et al., 2000; Hancock et al., 1997). Herds with animals shedding E. coli O157:H7 appears to be relatively high in prevalence, ranging from 63-97% of feedlots surveyed having at least one positive animal (Dargatz, Wells, and Thomas, 1997; Sargeant et al., 2003). At the farm level, intervention measures that have been investigated or are currently under investigation include competitive exclusion bacteria, general sanitation strategies, dietary component modifications, and feeding strategies immediately prior to slaughter (Stevens et al, 2002). At present, testing and removal of infected animals would appear to be impractical since the majority of herds are infected at some time. Considerable effort is being made to identify herd management practices and environmental factors that inhibit or facilitate infection of animals with E. coli O157:H7 (Hancock, D.D., 1998; Loneragan and Brashears, 2005). Bovine viral diarrhea virus (BVDV) infection is an economically important viral infection of cattle that can result in a wide spectrum of clinical manifestations, ranging from subclinical infections to severe fatal disease (Baker, 1995). The causative organism is an RNA virus and a member of the genus Pestivirus within the family Flaviviridae (Fauquet and Mayo, 2001). Infection of cattle with BVDV occurs worldwide. Although the majority of BVDV infections in immunocompetent cattle are transient and self-limiting, it is apparent that when infection occurs in the presence of other micro-organisms, BVDV can contribute to a disease that becomes clinically evident (Baker, 1995). Numerous studies, under both natural and experimental conditions, have demonstrated the relationship between BVDV and other infectious agents, suggesting that BVDV has the ability to induce immunosuppression during primary infection in immunocompetent cattle. BVDV affects the immune system in a number of different ways leading to an increased susceptibility to other infectious agents. Impairment of lymphocyte and neutrophil function and decreases in the number of circulating and tissue immune cells have been identified in BVDV-infected cattle (Potgieter, 1995). These abnormalities, combined with environmental and/or management stressors, contribute to immunosuppression and concurrent infection with other pathogens. In an experimental BVDV infection model using the BVDV-2 isolate 890, infected calves develop a severe leukopenia that begins three days after inoculation (Walz et al., 1999). The mechanism of leukopenia is unknown; however, BVDV has a propensity to replicate in immune system cells. A result of this replicative cycle is the destruction of some of these cells and impairment of surviving cells (Roth, Kaeberle, and Griffith, 1981; Welsh, Adair, and Foster, 1995). The consequence of BVDV infection of these critical immune system cells may result in the inability to clear bacterial infections of the gastrointestinal tract, such as E. coli O157:H7 or Salmonella typhimurium. Antimicrobial drug resistance: In May 1972, the University of Kentucky discontinued the application of all antimicrobial agents for therapeutic, prophylactic and sub-therapeutic use in one of their swine herds. This was done in an attempt to evaluate the many factors associated with the development of antimicrobial resistant bacterial populations in pigs. Extensive studies have been conducted on resistance patterns in this herd and in another herd at the University of Kentucky in which antibiotics were fed continuously. Numerous papers were published by Cromwell and his colleagues (references needed) during the first 15-20 years after antibiotics were discontinued in this herd. A paper by Langlois et al. (1986) gives an overall summary of the results of those research studies. During the first few years following antibiotic withdrawal, antibiotic resistance (as measured by the percentage of fecal coliforms that were resistant to tetracycline) decreased from over 90% (initially) to about 50%. Since that time, 30 to 70% of the fecal coliforms have been resistant to tetracycline, even though the pigs have received no antibiotics in their feed or for treatment purposes for over 28 years. The population of antibiotic-resistant bacteria established in this herd during routine antibiotic use has survived from generation to generation over 26 years even in the absence of antibiotic exposure (Langlois et al., 1986). This finding leaves little hope for the possibility that antibiotic resistance will simply disappear if the selective pressure of antibiotic treatment is removed. The use of antibiotics in the animal industry poses the growing concern that the gastrointestinal tract (GIT) of livestock may be a potential reservoir of antibiotic resistance genes, which might contribute to an increased risk of consumer exposure to resistance. Tetracyclines have been used for over 50 years and, therefore, provide an excellent model with which to study antibiotic resistance. There has been extensive documentation of tetracycline resistance genes carried by many bacteria, with most of the research focusing on clinical isolates or pathogens (Whittle et al., 2002). Limited information is available about the extent and distribution of tetracycline resistance genes in the commensal bacteria residing in the GIT. This population is important because resistance genes can be spread between unrelated bacteria by means of horizontal transfer of mobile genetic elements (Shoemaker et al., 1992). Within the commensal population, Lactobacilli are considered to be beneficial and are often used as probiotics but very little research has been conducted evaluating the presence of tetracycline resistance determinants in this population of the GIT. Previously, Lactobacillus spp. have been found to carry three different ribosomal protection type of tetracycline resistance genes from clinical (tetO and tetQ) and food (tetM) isolates (Rice, 1998; Danielson, 2002). The tetracycline resistance genes specific for ribosomal protection are commonly located on conjugative plasmids (classes O and S) or are associated with conjugative chromosomal elements (classes M and Q) such as conjugative transposons. Conjugative transposons are prevalent in bacteria known to colonize the GIT (Rice, 1998) and recent research has supported their extensive role in antibiotic resistance spread throughout the GIT (Scott, 2002). Furthermore, in gram-positive bacteria, such as lactobacilli, tetracycline resistance genes are most commonly found on conjugative transposons (Roberts, 1996). The tetracycline resistance gene, tetQ, is associated with at least five conjugative transposons, most often found in Bacteroides spp. (Salyers et al., 1995). Bacteroides spp. isolated from the GIT have been indicated as major players in the spread of antibiotic resistance genes through the movement of these conjugative transposons (Whittle et al., 2002). The tetQ gene has been identified in Lactobacillus spp. but the presence of a related conjugative transposon has not been investigated. Furthermore, the tetracycline resistance gene, tetM, is found on conjugative transposons from the Tn916 family, which is most often identified in streptococci and enterococci (Rice, 1998). Lactobacilli are within the host range of both Tn916 and Tn1545 (Tn916-like) conjugative transposons (Rice, 1998) but the presence of tetM carrying conjugative transposons in lactobacilli have not yet been identified. But recent research suggests that the tetM carried by some Lactobacillus spp. could potentially be located on a conjugative transposon. Specifically, they observed the conjugal transfer of tetM from both plasmid and chromosomal locations (Gevers et al., 2003). This research is important because dietary treatments considered as potential alternatives in the event of a ban on antibiotic use in the animal industry either directly employ the use of Lactobacillus spp. (i.e., probiotics) or indirectly seek to enhance this population in the GIT (i.e., feeding of prebiotics). It has not yet been demonstrated if lactobacilli shown to carry both tetM and tetQ, house these genes on their characteristic conjugative transposons. In addition, these conjugative transposons often carry additional genes responsible for erythromycin resistance and they are capable of broad-host-range transfer (Rice, 1998). Therefore, with the inherent mobility of conjugative transposons, the potential exists for the transfer of both tetracycline and erythromycin resistance from non-pathogenic to potentially pathogenic bacteria in the GIT.
Objectives
-
Develop or improve methods for control or elimination of pathogens in pre-and post harvest environments including meat, poultry, seafood, fruits and vegetables and nutmeats.
-
Develop and validate mathematical modeling to gain understanding of pathogen behavior in macro and micro-environments.
-
Investigate factors leading to the emergence, persistence and elimination of antimicrobial resistance in food processing and animal production environments
Methods
SEE ATTACHMENT 1 FOR METHODSMeasurement of Progress and Results
Outputs
- Validated decontamination methods that can be used by the fruit, vegetable, seafood, meat and poultry industry to enhance the safety of their finished product
- Outreach/extension education and training materials for regulatory personnel, producers, processors, consumers, extension agents
- Overall enhanced food safety for consumers
Outcomes or Projected Impacts
- Enhanced safety of fruit, vegetable, seafood, meat, and poultry products
- Increased understanding of food safety measures by regulatory personnel, producers, processors, consumers, extension agents
- Overall enhanced food safety for consumers
Milestones
(2007): Pre-harvest food safety: Initiate work on antimicrobial films, high pressure processing of viruses. Modeling: Develop and validate wind tunnel to validate heat transfer models. Antimicrobial drug resistance: Tetracycline resistance genes in the environment. Total bacterial population genomic DNA extracted from fecal samples and analyzed for presence of all tetracycline resistance (Tc-R) genes.(2008): Pre-harvest food safety: Initiate efforts on sanitizers, high pressure processing of vibrios. Modeling: Collect growth data of Salmonella in chicken and beef at isothermal conditions. Develop neural network model and compare its performance to statistical models. Antimicrobial drug resistance: Environmental sample collection from antibiotic free and antibiotic receiving farms and molecular analysis. Analyze tetracycline resistant isolates for (expected) tetracycline resistance genes with PCR.
(2009): Pre-harvest food safety: Investigate optimization of high pressure processing in RTE seafoods. Modeling: Collect growth data of E. coli in ground beef for different fat content at isothermal conditions. Antimicrobial drug resistance: Data analysis and manuscript preparation
(2010): Pre-harvest food safety: Initiate outreach activities. Modeling: Current FSIS risk assessment model for E. coli in ground beef is based on models developed using broth.
(2011): Pre-harvest food safety: continue outreach activities and publish research results. Modeling: Develop heat transfer to obtain temperature profile in shell eggs during cooling.
Projected Participation
View Appendix E: ParticipationOutreach Plan
Dissemination of research findings to stakeholders through peer reviewed publications, presentations at scientific meetings, extension publications and materials, workshops, trade magazines and popular press. A website accessible through NIMSS will be maintained on the SAAESD website to disseminate downloadable documents and podcasts for stakeholders.
Organization/Governance
The membership of the Regional Technical Committee includes: a. The regional administrative advisor (non-voting); b. A technical representative of each cooperative experiment station, appointed by the respective station director. c. A technical representative of each cooperative USDA research division or other Federal agency named by the director of the division or Head of the agency; An executive committee consisting of Chairman, Vice Chairman, and Secretary, elected by the Technical Committee members, is designated to conduct business of the Technical Committee between meetings and to perform other duties assigned by the Technical Committee. The term of office for each Executive Committee member is two years. The progression is Secretary, Vice chairman to Chairman, for a total of six years. The duties of the Technical Committee will be to coordinate planning and work of the project and make such recommendations as are necessary through the Administrative Advisor to the Southern Association of Agricultural Experiment Station Directors. The functions of the chair will be to preside over meeting and edit the annual report. The recording secretary shall take minutes at the annual meeting and distribute these to members of the committee within a month of the meeting. The Technical Committee will meet annually to review progress, develop research plans and coordinate research efforts in order to maintain the Committees focus on the objectives identified in the project. During the annual meeting, the research coordinators from each of the lead states (Table 1) will summarize for the entire Regional Technical Committee the plan of work for the next year in the specified research areas in meeting the goals of each objective. In addition to the annual meeting, the coordinators are responsible for maintaining active communication with their cooperating stations principal investigator to maintain a current knowledge base of research accomplishment within the specified problem area. This linkage will enhance the research effectiveness and productivity, reduce duplication and unnecessary work, and strengthen the regionalism among cooperating stations. Copies of the Annual Progress Report, including major accomplishments of contributing project and minutes of the annual meeting will be distributed each year and available at the NIMSS website.
Literature Cited
Annous, B.A., G.M. Sapers, A.M. Mattrazzo, and D.C.R. Riordan. 2001. efficacy of washing with a commercial flat-bed brush washer, using conventional and experimental washing agents in reducing populations of Escherichia coli on artificially inoculated apples. J. Food Prot., 64(2), 159-163. Baker, J.C. 1995. The clinical manifestations of bovine viral diarrhea infection. Vet. Clin. North Am. Food Anim. Pract. 11:425-445. Brett, M.S.Y., short, P., McLauchlin, J. 1998. A small outbreak of listeriosis associated with smoked mussels. Int. J Food Microbiol 43(3):223-229. Calci, K.R., Meade, G.K., Tezloff, R.C. and Kingsley, D.H. (2005) High-pressure inactivation of hepatitis A virus within oysters. Appl Environ Microbiol. 71, pp. 339-343. Centers for Disease Control and Prevention (CDC). 2002b. Outbreak of Salmonella serotype Kottbus infections with eating alfalfa sprouts Arizona, California, Colorado, and New Mexico, February April, 2001. MMWR, 51 (10), 7-9. Centers for Disease Control and Prevention (CDC). (2003) Hepatitis A outbreak associated with green onions at a restaurant--Monaca, Pennsylvania. MMWR Morb Mortal Wkly Rep. 52, pp. 1155-1157. Centers for Disease Control and Prevention (CDC). 2004. Outbreak of cyclosporiasis associated with snow peas - Pennsylvania. MMWR, 53 (37), 876-878. Dalgaard, P. and Jorgensen, L.V. 1998. Predicted and observed growth of Listeria monocytogenes in seafood challenge tests and in naturally contaminated cold-smoked salmon. Int. J Food Microbiol 40:105-115. Dargatz, D.A., S.J. Wells, and L. Thomas. 1997. Factors associated with the presence of Escherichia coli O157:H7 in feces of feedlot cattle. J. Food Prot. 60:466-470. Das E., G.C. Gurakan, and A. Bayindirli. 2006. Effect of controlled atmosphere storage, modified atmosphere packaging and gaseous ozone treatment on the survival of Salmonella Enteritidis on cherry tomatoes. Food Microbiol. 23(5):430-8. Dean-Nystrom, E.A. 2003. Bovine Escherichia coli O157:H7 infection model. Methods Mol. Med. 73:329-238. Dean-Nystrom, E.A., B.T. Bosworth, W.C. Cray Jr., et al. 1997. Pathogenicity of Escherichia coli O157:H7 in the intestines of neonatal calves. Infect. Immun. 65:1842-1848. Dean-Nystrom, EA, B.T. Bosworth, H.W. Moon. 1999. Pathogenesis of Escherichia coli O157:H7 in weaned calves. Adv. Exp. Med. Biol. 473:173-177. Deng M.Q. and D.O. Cliver. 2001. Inactivation of Cryptosporidium parvum oocysts in cider by flash pasteurization. J Food Prot. 64(4):523-7. Destro, M.T., Leitao, M.F.F., and Farber, J.M. 1996. Use of molecular typing methods to trace the dissemination of Listeria monocytogenes in a shrimp processing plant. Appl. and Environ. Microbiol. 62(2):705-711. Elder, R.O., J.E. Keen, G.R. Siragusa et al. 2000. Correlation of enterohemorrhagic Escherichia coli O157 prevalence in feces, hides, and carcasses of beef cattle during processing. Proc Natl Acad Sci U S A 97:2999-3003. Ericsson, H. Eklow, A., Danielsson-Tham, M.L., Loncarevic, S., Mentzing, L.O., Persson, I., Unnerstad, H., Tham, W. 1997. An outbreak of listeriosis suspected to have been caused by rainbow-trout. J. Clin. Microbiol. 35(11):2904-2907. Farber, J.M., and Peterkin, P.I. 1991. Listeria monocytogenes, a food-borne pathogen. Microbiol. Rev. 55(3):476-511. Fauquet, CM., and M.A. Mayo. 2001. The 7th ICTV report. Arch Virol 146:189-194. FDA/USDA/CDC 2003 1998, posting date. Guide to minimize microbial food safety hazards for fresh fruits and vegetables. CFSAN. [Online.] Givens, M.D., P.K. Galik, K.P. Riddell, et al. 1999. Uterine tubal cells remain uninfected after culture with in vitro-produced embryos exposed to bovine viral diarrhea virus. Vet. Microbiol 70:7-20. Grony, J.G. 2005. A fresh look at produce safety. Food Safety Mag., 11(1), 70-76. Grove SF, Lee A, Lewis T, Stewart CM, Chen H, Hoover DG. 2006. Inactivation of foodborne viruses of significance by high pressure and other processes. J Food Prot. 69(4):957-68. Review. Hancock, D.D. 1998. Ecology of Escherichia coli O157:H7 in cattle and impact of management practices, p. 85-91.. In J. B. Kaper and A.D. OBrien (ed.), Escherichia coli O157:H7 and other Shiga Toxin-producing Escherichia coli, 1st ed., ASM Press, Washington, D.C. Hancock, D.D., T.E. Besser, D.H. Rice et al. 1997. A longitudinal study of Escherichia coli O157 in fourteen cattle herds. Epidemiol Infect. 118:193-195. Harris, L., J.N. Farber, L.R. Beuchat, M.E. Parish, T.V. Suslow, E.H. Garrett, and F.F. Busta. 2001. Outbreaks associated with fresh produce: incidence, growth, and survival of pathogens in fresh and fresh-cut produce, in Analysis and Evaluation of Preventive Control Measures for the Control and Reduction/Elimination of Microbial Hazards on Fresh and Fresh-Cut Produce. Comprehensive Reviews in Food Science and Food Safety. 2, 78S-141S. Hoffman, A.D., Gall, K.L., Norton, D.M., and Wiedmann, M. 2003. Listeria monocytogenes contamination patterns for the smoked fish processing environments and for raw fish. J. Food Prot. 66(1):52-60. Hoover, D.G., Metrick, C., Papineau, A.M., Farkas, D.F. and Knorr, D. (1989) Biological effects of high hydrostatic pressure on food microorganisms. Food Technol. 43, pp. 99-107. Hu H., Churey J.J., and R.W. Worobo. 2004. Heat treatments to enhance the safety of mung bean seeds. J Food Prot. 67(6):1257-60. Ibarra-Sanchez L.S., Alvarado-Casillas S., Rodriguez-Garcia M.O., Martinez-Gonzales N.E., Castillo A. 2004. Internalization of bacterial pathogens in tomatoes and their control by selected chemicals. J Food Prot. 67(7):1353-8. Jaquette, C.B., L.R. Beuchat, and B.E. Mahon. 1996. Efficacy of chlorine and heat treatment in killing Salmonella Stanley inoculated onto alfalfa seeds and growth and survival of the pathogen during sprouting and storage. Appl. Environ. Microbiol., 62, 2212-2215. Kingsley DH, Hoover DG, Papafragkou E, Richards GP. 2002. Inactivation of hepatitis A virus and a calicivirus by high hydrostatic pressure. J Food Prot. 65(10):1605-9. Kretsinger, K., S. Noviello, M. Moll, et al., Tomatoes sicken hundreds: multistate outbreak of Salmonella Newport. Presented at the 52nd Annual Epidemic Intelligence Service Conference, Atlanta, GA. April, 2003. Loneragan, G.H., and M.M. Brashears. 2005. Effects of using retention-pond water for dust abatement on performance of feedlot steers and carriage of Escherichia coli O157 and Salmonella spp. JAVMA 226: 1378-1383. Mead, P.S., L. Slutsker, V. Dietz, et al. 1999. Food-related illness and death in the United States. Emerg Infect Dis. 5:607-625. Miettinen, M.K., Siitonen, A., Heiskanen, P., Haajanen, H., Bjorkroth, K.J., Korkeala, H.J. 1999. Molecular epidemiology of an outbreak of febrile gastroenteritis caused by Listeria monocytogenes in cold-smoked rainbow trout. J. Clin. Microbiol. 37(7):2358-2360. Mintier, A.M. and D.M. Foley. 2006. Electron beam and gamma irradiation effectively reduce Listeria monocytogenes populations on chopped romaine lettuce. J Food Prot. 69(3):570-4. Natrajan, N. and B. W. Sheldon. 2000a. Efficacy of nisin-coated polymer films to inactivate Salmonella typhimurium on fresh broiler skin. J. Food Prot. 63:1189-1196. Natrajan, N. and B. W. Sheldon. 2000b. Inhibition of Salmonella on poultry skin using protein- and polysaccharide-based films containing a nisin formulation. J. Food Prot. 63:1268-1272. Padgett, T., I. Y. Han and P. L. Dawson. 2000. Effect of lauric acid addition on the antimicrobial efficacy and water permeability of corn zein films containing nisin. J. Food Proc. Pres. 24:423-432. Pao S,, and C.L. Davis. 1999. Enhancing microbiological safety of fresh orange juice by fruit immersion in hot water and chemical sanitizers. J Food Prot. 62(7):756-60. Paton, J.C., and A.W. Paton. 1998. Pathogenesis and diagnosis of Shiga toxin-producing Escherichia coli infections. Clin Microbiol Rev. 11:450-479. Potgieter, L.N. 1995. Immunology of bovine viral diarrhea virus. Vet Clin North Am Food Anim Pract 11:501-520. Reed, L.J., and H. Muench. 1938. A simple method of estimating fifty percent endpoints. Am. J. Hyg. 27:492-497. Roth, J.A., M.L. Kaeberle, and R.W. Griffith. 1981. Effects of bovine viral diarrhea virus infection on bovine polymorphonuclear leukocyte function. Am J Vet Res 42:244-250. Sanderson, M.W., T.E. Besser, I.M. Gay, et al. 1999. Fecal Escherichia coli O157:H7 shedding patterns of orally inoculated calves. Vet. Microbiol. 69:199-205. Sapers, G.M., R.L. Miller, M. Jantschke,, and A.M. Mattrazzo. 2000. Factors limiting the efficacy of hydrogen peroxide washes for decontamination of apples containing Escherichia coli. J. Food Sci., 65 (3), 529-532. Sapers, G.M., R.L. Miller, V. Pilizota, and A.M. Mattrazzo. 2001. Anti-microbial treatments for minimally processed cantaloupe melon. J. Food Sci., 66 (2), 345-349. Sargeant, J.M., M.W. Sanderson, R.A. Smith et al. 2003. Escherichia coli O157:H7 in feedlot cattle feces and water in four major feeder cattle states in the USA. Prev. Vet. Med. 61:127-135. Schaub, S.A. and R.K. Oshiro. 2000. Public health concerns about caliciviruses as waterborne contaminants. J. Infect. Dis. 181(Suppl. 2):S374-S380. Schmidt H.M., M.P. Palekar, J.E. Maxim, A. Castillo. 2006. Improving the microbiological quality and safety of fresh-cut tomatoes by low-dose electron beam irradiation. J Food Prot. 69(3):575-81. Shabin, S.N. 2004. Cruise Ships, Oysters, and edible vaccines: revising the non-cultivatable Norwalk-like virus responsible for outbreaks of gastroenteritis. Clinical Micobiology Newsletter. 26(1):1-4. Sivapalasingam, S., E. Barrett, A. Kimura, S. Van Duyne, W. De Witt, M. Ying, A. Frisch, Q. Phan, E. Gould, P. Shillam, V. Reddy, T. Cooper, M. Hoekstra, C. Higgins, J.P. Sanders, R.V. Tauxe, and L. Slutsker. 2003. Multistate outbreak of Salmonella enterica serotype Newport infection linked to mango consumption: impact of water-dip disinfestations technology. Clin. Infect. Dis., 37 (12), 1585-1590. Slutsker, L, A.A. Ries, K.D. Greene, et al. 1997. Escherichia coli O157:H7 diarrhea in the United States: clinical and epidemiologic features. Ann Intern Med. 126:505-513. Smith DeWaal, C., K. Barlow, L. Alderton, and M. F. Jacobson. 2002. Outbreak Alert! Center for Science in the Public Interest. Solomon EB, Yaron S, Matthews KR. 2002. Transmission of Escherichia coli O157:H7 from contaminated manure and irrigation water to lettuce plant tissue and its subsequent internalization. Appl Environ Microbiol. 68(1):397-400. Stevens, M.P., P.M. van Diemen, F. Dziva et al. 2002. Options for the control of enterohaemorrhagic Escherichia coli in ruminants. Microbiology 148:3767-3778. Tham, W., Ericsson, H., Loncarevic, S., Unnerstad, H., Danielsson-Tham, M.L. 2000. Lessons from an outbreak of listeriosis related to vacuum-packed gravid and cold-smoked fish. Int. J. Food Microbiol. 63(3):173-175. Thimothe, J., Walker, J., Suvanich, V., Gall, K.L., Moody, M.W., and Wiedmann, M. 2002. Detection of Listeria in crawfish processing plants and in raw, whole crawfish and processed crawfish (Procambarus spp.). J. Food Prot. 65(11):1735-1739. Walz, P.H., T.G. Bell, B.A. Steficek, et al. 1999. Experimental model of type II bovine viral diarrhea virus-induced thrombocytopenia in neonatal calves. J. Vet.Diag. Invest. 11:505-514. Welsh, M.D., B.M. Adair, and J.C. Foster. 1995. Effect of BVD virus infection on alveolar macrophage functions. Vet Immunol Immunopathol 46:195-210. Woodward, M.J., D. Gavier-Widen, I.M. McLaren, et al. 1999. Infection of gnotobiotic calves with Escherichia coli O157:H7 strain A84. Vet. Rec. 144:466-470. Zhang, S. and J. M. Farber. 1996. The effects of various disinfectants against Listeria monocytogenes on fresh-cut vegetables. Food Microbiol., 13, 311-321.